- 1National Key Laboratory for Conservation and Utilization of Biological Resources in Yunnan, College of Plant Protection, Yunnan Agricultural University, Kunming, China
- 2Yunnan Expo Horticulture Co., Ltd., Kunming, China
Bemisia tabaci is the main pest of agriculture in many regions of the world. The resistance of whitefly to pesticides has increased as a consequence of the continuous irrational use of wide-spectrum pesticides. Thus, pesticides are no longer always effective as a long-term control method. The agricultural landscape can affect the occurrence of an insect population. The objective of this study was to clarify the occurrence of whitefly and its predators in tomato fields in different agricultural landscapes. Different landscapes are classified into urban, flower, water, and mountain landscapes by the principal component analysis method. In 2018–2019, whitefly had the longest main activity period and the lowest density in the flower landscape. The water landscape helped to maintain the highest densities of whitefly during the main activity period. Nine species of predators were sampled, and Nesidiocoris tenuis, Chrysoperla sinica, Menochilus sexmaculata, and Harmonia axyridis were the dominant species throughout the sampling season in both years. During the main activity period, N. tenuis had the highest density in all sampled landscapes. The density of the dominant predators was the highest in the flower landscape, and each natural predator had the largest temporal niche width in the 2-year sampling period. Bemisia tabaci, N. tenuis, and M. sexmaculata were highly synchronized temporally. The flower landscape showed satisfactory results in suppressing whitefly. Increasing the proportion of flowering plants and increasing the diversity of plant crops in the agricultural landscape can effectively reduce the densities of whitefly during an outbreak.
Introduction
The whitefly Bemisia tabaci (Gennadius) (Hemiptera: Aleyrodidae) is the main pest of economic agriculture in several countries and regions of the world (Pan et al., 2021). It is distributed worldwide except in Antarctica and has caused serious economic losses to global agricultural production (Barro et al., 2011). The host plant species of whitefly are very extensive (Barro et al., 2011; Jiang, 2020). In China, plants that are most often damaged include Solanum lycopersicum Miller (Tubiflorae: Solanaceae), Brassica oleracea L. (Brassicales: Brassicaceae), Cucumis sativus L. (Cucurbitales: Cucurbitaceae), Gossypium spp. (Malvales: Malvaceae), and other economic crops (Zhang et al., 2014a). Moreover, whitefly directly sucks plant sap by piercing-sucking mouthparts and secretes honeydew, which induces sooty blotch and seriously affects plant photosynthesis (Zhang et al., 2014b). However, ingestion of plant sap by whiteflies can also indirectly transmit several plant viruses, causing far more economic losses than those from direct feeding (Muniyappa and Veeresh, 1984; Li et al., 2010; Zhang et al., 2020b). Tomato is an important edible vegetable that is widely cultivated in China (Miao, 2017). In 2018, the tomato planting area in Yunnan province was approximately 35,600 hm2, divided mainly into two summer and winter planting seasons. The main method of planting tomatoes in Yunnan province is open field cultivation, and the greenhouse cultivation area accounts for 33.3% of the total planting area (Zhao et al., 2019). The volume of tomato exports in Yunnan province accounts for 37% of the China export volume, and the yield per unit area in Kunming city is the highest (Zhao et al., 2019).
Chemical control methods are widely used in the control of whiteflies because of their advantages of quick action, low cost, and easy operation. However, with the long-term unregulated application of chemical pesticides, the resistance of the whitefly has increased, and several chemical pesticides have been unable to control it (Yang et al., 2014). Biological control has the advantages of economical application and environmental safety (Suzanne, 1982). Due to the increasing resistance of whitefly, the use of biological control methods has become the main trend in the integrated management of whiteflies (Lenteren et al., 2018, 2020).
Natural enemies of whiteflies are abundant. There are 128 species of predators and more than 90 species of parasitoids of whiteflies in the world (Gerling et al., 2001; Li et al., 2011). Currently, 109 species of predators and 59 species of parasitoids of whitefly have been reported in China (Li et al., 2011; Dou et al., 2020a). The biological control of the whitefly has been studied and applied worldwide for more than 40 years (Ren et al., 2001). There are about 13,000 species of insects in Yunnan province, China, accounting for approximately 25.5% of the country (Liu and Xia, 2017), and the natural enemy resources are highly rich (Dou et al., 2020a). At present, only two parasitoids of Aphidius gifuensis Ashmead (Hymenoptera: Aphidiidae) and Scleroderma spp. (Hymenoptera: Bethylidae) in Yunnan province are widely used in agricultural and forest production (Liu and Xia, 2017). However, there are few reports on pest control in the tomato field by adjusting the landscape layout. The efficacy of the control of whitefly by natural enemies is affected by several factors. The landscape pattern of the agroecosystem, especially the composition and configuration of crop and non-crop habitats, can affect the population density of pests and their natural enemies, thus, affecting the efficacy of control by natural enemies (Jonsson et al., 2012; Veres et al., 2013). Agroecosystems are unstable artificial ecosystems. In terms of habitats, it includes crop habitats and surrounding non-farming habitats (You et al., 2004). Crop habitats provide the main breeding ground for pests and their natural enemies. In contrast, non-crop habitats can be used as sites for pests and natural enemies to find alternative hosts and escape adverse environmental conditions (Chaplin-Kramer et al., 2011). At present, the widespread planting pattern is characterized by the increase of intensive production mode, which leads to the single structure of farmland landscape and greatly reduces the biodiversity of farmland landscape (Tscharntke et al., 2002). Recent studies have shown that the pattern of distribution of biodiversity in farmland is affected by the structure of the surrounding landscape (Zhang, 2017). The different agricultural landscape has formed a unique pattern of community planting, and some places have even gradually presented a patchy pattern (Yu et al., 1996). Generally, the non-crop habitat alters the biodiversity of the environment. Non-crop habitats may host several natural enemies, which could have an important role in pest control (Liu et al., 2000). However, with the advent of the intensive production mode, the interspecific relationship of insects has changed, and the control effect of natural enemies has been affected (Bianchi et al., 2006). Some studies analyzed the effects of farmland landscape patterns and pesticides use on ladybugs in cotton fields and demonstrated that Zea mays L. (Poales: Poaceae) and grassland habitats were more conducive to the occurrence of ladybugs in wheat fields (Zhou et al., 2014). The influence of forest cover in different farmland landscapes on ladybug diversity was studied. A large area of woodland in the agricultural landscape was observed to be conducive to ladybug migration to wheat fields (Zhao K. D. et al., 2015). The composition of farmland and its surrounding non-crop habitats plays an important role in the occurrence and migration of insects. By changing the vegetation composition of non-crop habitats in the farmland landscape, the control efficiency of natural enemies can be improved (You et al., 2004). A study of the effects of different agricultural landscapes on the natural enemies of Pyrausta nubilalis (Hubern) (Lepidoptera: Pyralidae) demonstrated that natural enemies of P. nubilalis gathered the most when the proportion of non-cultivated habitats, especially forest land, villages, and grasslands, was 20–30% (Bian et al., 2019). Research on the effects of agricultural landscape structure on the protection of natural enemies and pest control, the composition of non-crop habitats, and the combination of crop and non-crop habitats have emphasized the importance of protecting the diversity of natural enemies (Bianchi et al., 2006). In summary, crop habitat and non-crop habitat compositions and their area ratio in the farmland landscape significantly affect insect richness, and a reasonable landscape layout can promote the control of pests by natural enemy insects.
In this study, the occurrence of whitefly in tomato fields of different agricultural landscapes in Kunming city, Yunnan province, as well as the resultant effects of its predators, were studied. The study also aimed to explore the effects of different agricultural landscapes on the populations of whitefly and their predators. The results could provide a theoretical basis for the ecological regulation of the whitefly and the ecological planning of the farmland landscape.
Materials and methods
Site design
This study was replicated at 12 plot site fields on a landscape diversity gradient in Kunming city, Yunnan province, south China of tomato growing areas during the second half of 2018 and 2019. The climate type belongs to the subtropical monsoon climate. The area of each site is about 800 square meters (20 m × 40 m), located more than 5 km apart from each replicate site. The tomatoes are planted in an open field in all plots. The tomato cultivar is “Zhongyan TV1” (Beijing Zhongyan Yinong Seedling Co., Ltd.). The tomato varieties and the density of the planting are consistent in all tomato planting plots. The sampling started 1 week after tomato planting. The pesticides were not sprayed during the investigation, and we compensated farmers for their losses at all sites. Detailed agronomic parameters are shown in Table 1.
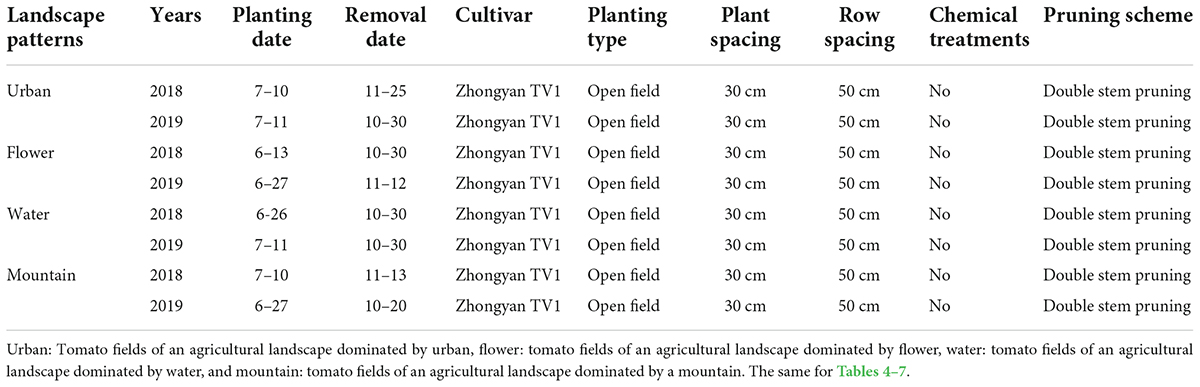
Table 1. Agronomic parameters of summer tomato fields in four different agricultural landscapes both in 2018 and 2019.
Sampling
Summer tomatoes in 2018 were sampled every 10 days from June 20 to the end of the growing season (November 25) in 2018. The summer tomatoes in 2019 started on July 4 and continued every 10 days until the end of the tomato growing season (November 12) in 2019.
Five random positions were selected in each sample plot site, and the five closest tomato plants were randomly selected and sampled from each position (avoiding the plants closest to any edge to minimize edge effects). From each tomato plant, five leaves of similar age were examined in the upper, middle, and lower positions, resulting in a total of 375 leaves being monitored per sample plot (Zhang et al., 2020b). To observe the number of adult B. tabaci and their predators, the unidentified individuals were brought back to the laboratory for identification. The leaves were then removed, placed in a Ziplock bag for marking, and brought back to the laboratory. The number of whitefly nymphs, namely, first, second, third, and fourth instar nymphs, was observed and recorded under the stereomicroscope (OLYMPUS, SZ51). Finally, the leaf area was recorded using a transparent graph paper placed on the leaf, and standardized density data (no. of individuals per 100 cm2 leaf surface) were calculated (Zhang et al., 2020b).
Describing seasonal activity
To reduce the error caused by human factors or crop growth factors in judging the peak or outbreak period of the insect population, we objectively judged the peak period of insect occurrence by the third method. The seasonal activity curves were standardized following the method of Fazekas et al. (1997). The activity period of B. tabaci was divided into four quartiles based on the dates of 25, 50, and 75% of the total recorded individuals of whitefly. The proportion of the number of whiteflies in different sampling periods to the total number of sampling in the whole occurrence period is set as R. The period of the sampling population number of whitefly when R < 25% was defined as the “early activity period,” the period between 25% ≤ R < 75% is defined as the “main activity period,” and the period of 75% ≤ R ≤ 100% is defined as the “late activity period.” We define the date when R = 50% as the population peak during the whole occurrence period (notice that this is not linked to a density peak observed at a given time, it is a product of the cumulative curve, so it can also fall on a date when no census was carried out) (Fazekas et al., 1997; Zhang et al., 2014b).
Landscape analysis
To define our study regions based on the landscape, an area with a radius of 0.5 km centered on the sampling field was demarcated using open-access satellite imagery from Google Earth and, combined with the change of land cover area in the selected area once a month. The principal component analysis method (PCA) (SPSS 20.0) was used to calculate the change factor in land cover with the greatest impact on insect population, determined as landscape type. We ignored features that were smaller than 5 m2 and could not be located during ground verification; the combined area of all unidentified fields was less than 0.1% of each landscape (Liu et al., 2016).
Landscape factors affecting insect populations by a change in land cover are classified into 10 categories: flowers, water, mountains, urban, vegetables, fruit trees, forest timbers, shrubs, grasslands, and wastelands. In all the landscapes sampling, we use the principal component analysis (PCA) method to calculate the eigenvalues and the cumulative proportion of the correlation matrix according to the correlation between variables in the landscape. Based on the results of the PCA, the first three principal components with cumulative contribution rates of > 80% were selected. According to the absolute value of the eigenvalues of different elements in the first principal component, the landscape was classified into four categories with the highest eigenvalue: urban landscape, flower landscape, water landscape, and mountain landscape (Tables 2, 3). Each landscape includes three replicates plots in different regions. The urban included buildings, roads, abandoned land, and other impervious surfaces. The flower included flowering plants such as Rosa chinensis Jacq. (Rosales: Rosaceae), Dianthus caryophyllus L. (Centrospermae: Caryophyllaceae), Myosotis sylvatica F. W. Schmidt (Tubiflorae: Boraginaceae), Eustoma grandiflorum (Raf.) Shinners (Gentianales: Gentianaceae), etc. The water included the river, the water channel, the pond, and the reservoir. The mountain included a forest with an altitude difference of more than 150 m.
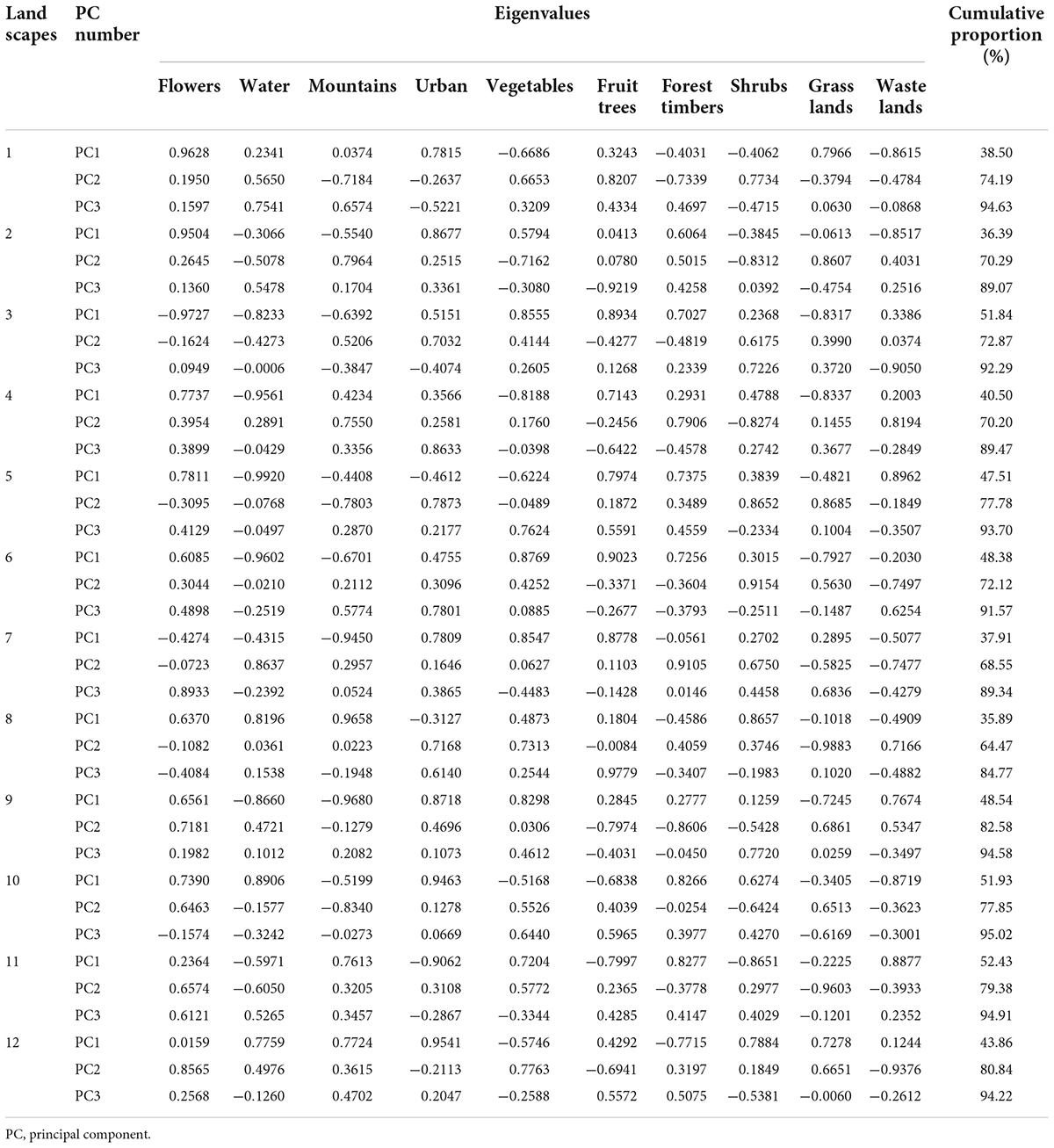
Table 2. Eigenvalues of the covariance matrix and cumulative proportion of principal components at each agriculture landscape in 2018.
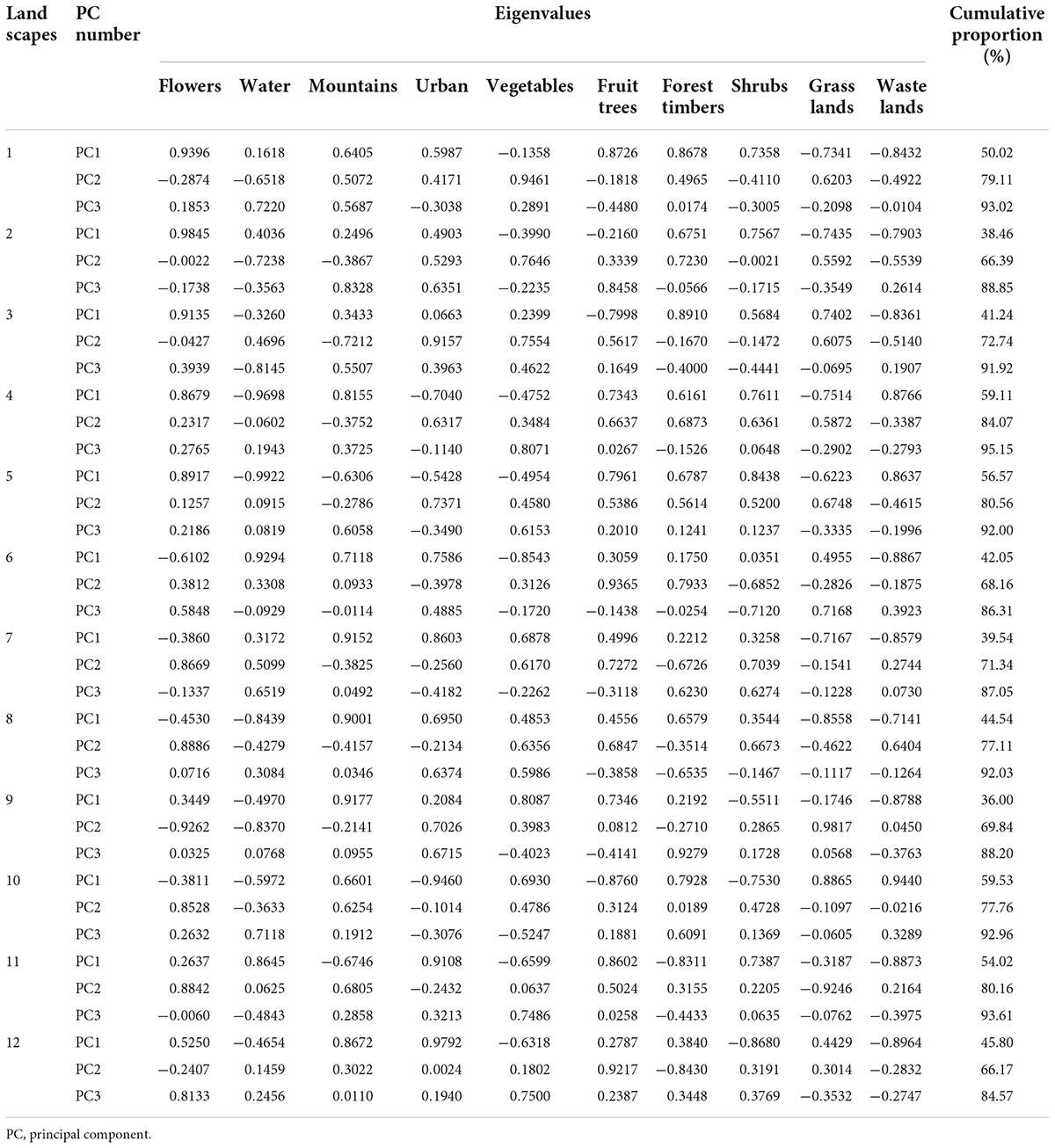
Table 3. Eigenvalues of the covariance matrix and cumulative proportion of principal components at each agriculture landscape in 2019.
Statistical analysis
The number of each species in the community obtained from each leaf survey was used as the basis for the data analysis. This value was used to calculate the dominance of each species and to screen dominant predators in different agricultural landscapes. The dominant species was selected for the temporal niche analysis. Dominance was expressed as relative density, and species with dominance greater than 10% were denoted dominant species (Gao et al., 2014).
The calculation of the temporal niche correlation index was based on Levins and Hurlbert formula (Levins, 1969; Hurlbert, 1978).
Temporal niche width:
where Pi is the proportion of species in unit i in a resource set and S is the total number of units in the resource set.
Temporal niche overlap:
where Pih and Pjh represent the proportions of species i and j in unit h. Bi is the width of the temporal niche of species i.
Data analysis
The census data were initially divided by the quartile method, and the population density of the main activity period was analyzed using one-way ANOVA (repeated measures) after tests of normality (Shapiro–Wilk) and homoscedasticity (Bartlett), while densities during the early activity period and the late activity period were excluded because they were too low to distinguish a difference between the treatments. Differences in B. tabaci and its predator densities were compared between agricultural landscapes on the same dates, as well as between weeks of each agricultural landscape, restricted by season, Tukey’s HSD (P = 0.05). A significance level of P = 0.05 was used for all tests. Data analyzes were performed using SPSS 20.0 (Zhang et al., 2014b). The cumulative seasonal activity curves and population dynamics were made using Origin 2018.
Results
Seasonal activity of Bemisia tabaci
In 2018, the length of the main activity period of B. tabaci nymphs was 21 days in the water landscape, 29 days in the mountain and urban landscape, and 63 days in the flower landscape. Peak activity was recorded between August 27 in the water landscape and September 15 in the urban landscape (Table 4). For adults of B. tabaci, the main activity period lasted 29 days in the urban landscape, 30 days in the water landscape, 32 days in the mountain landscape, and 52 days in the flower landscape. The earliest seasonal activity peak occurred on September 6 in the water landscape and the latest on September 18 in the urban landscape (Table 4 and Figure 1).
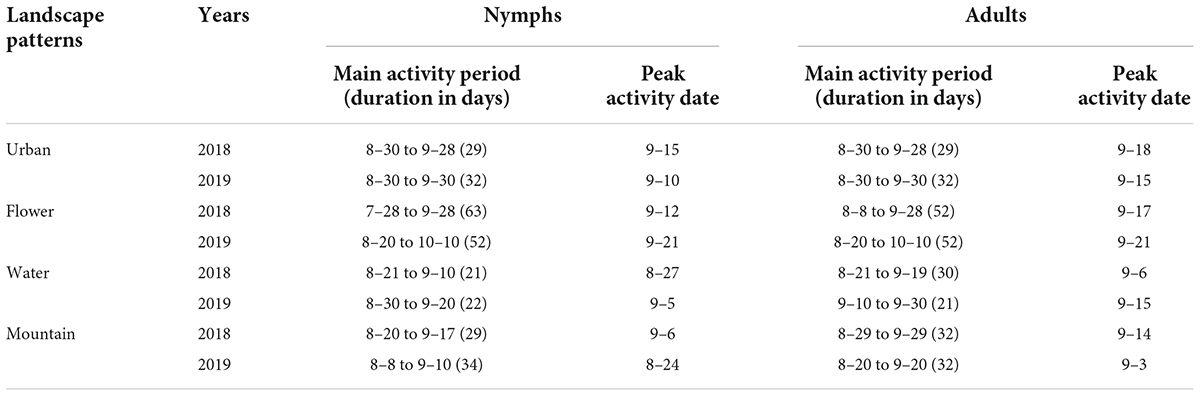
Table 4. The main activity period and dates of peak activity of nymph and adult Bemisia tabaci on summer tomato fields in different agricultural landscapes.
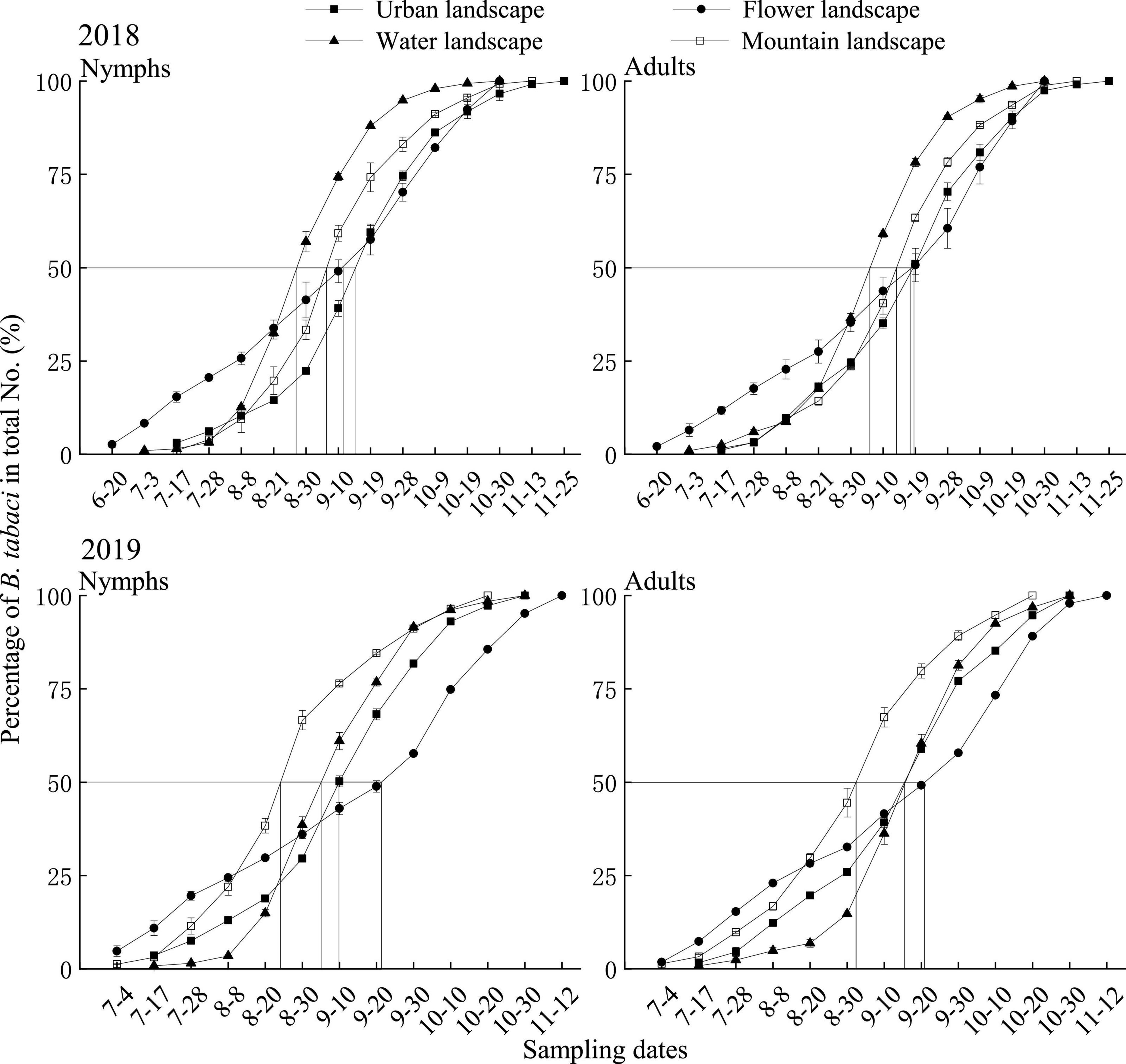
Figure 1. Cumulative seasonal activity curves of Bemisia tabaci on summer tomato fields in different agricultural landscapes in Kunming city, Yunnan province, South China, in 2018 and 2019. Urban landscape: Tomato fields of an agricultural landscape dominated by the urban area, flower landscape: tomato fields of an agricultural landscape dominated by flowers, water landscape: tomato fields of an agricultural landscape dominated by water, and mountain landscape: tomato fields of an agricultural landscape dominated by mountains. The same is applicable to Figures 2, 3.
In 2019, the main activity period of the nymphs ranged from 22 days in water landscapes to 52 days in the flower landscape, and the activity peaked on August 24 in the mountain landscape and September 21 in the flower landscape (Table 4). For adults, the main activity period lasted 21 days in the water landscape, 32 days in the urban and mountain landscapes, and 52 days in the flower landscape (Table 4). The earliest seasonal activity peak was in the mountain landscape on September 3 and the latest in the flower landscape on September 21 (Table 4 and Figure 1).
Seasonal population dynamics of Bemisia tabaci
In 2018, the densities of B. tabaci nymphs in the water landscape exceeded the values observed in the other landscapes throughout the sampling season, with the highest densities (88.41 individuals per 100 cm2 leaves) being recorded on August 30, about 10–20 days earlier than in other landscapes (Figure 2). During the main activity period, the densities of whitefly nymph in the water landscape were the highest (74.36 individuals per 100 cm2 leaves), which was significantly higher than the other three landscapes [F(3, 50) = 79.24; P = 0.0001]. After the water landscape, the urban landscape supported the highest densities of nymphs, and the densities of nymphs have the lowest in the flower landscape (4.85 individuals per 100 cm2 leaves) (Table 5). Bemisia tabaci adult population was first observed on June 20 and continued to increase until the population peaked in September, followed by a gradual decrease until the end of the survey. The densities in the water landscape were significantly [F(3, 50) = 44.52; P = 0.0001] higher than in the other three landscapes during the main activity period (Table 5). The highest density in the water landscape was observed on September 10 as 82.77 adults per 100 cm2 leaves (Figure 2).
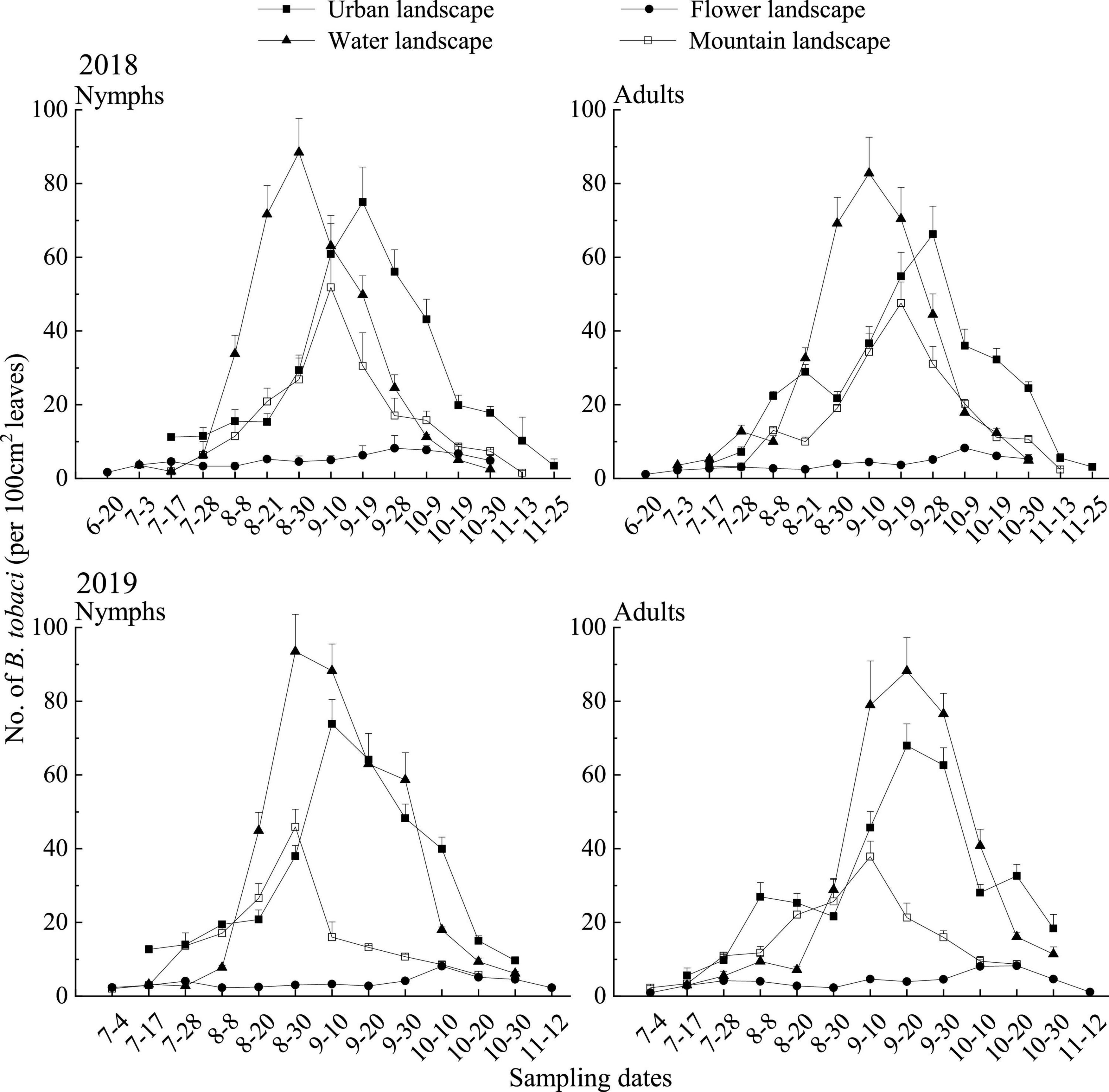
Figure 2. Seasonal dynamics of Bemisia tabaci (mean + SE) on summer tomato fields in different agricultural landscapes at Kunming city, Yunnan province, South China, in 2018 and 2019.
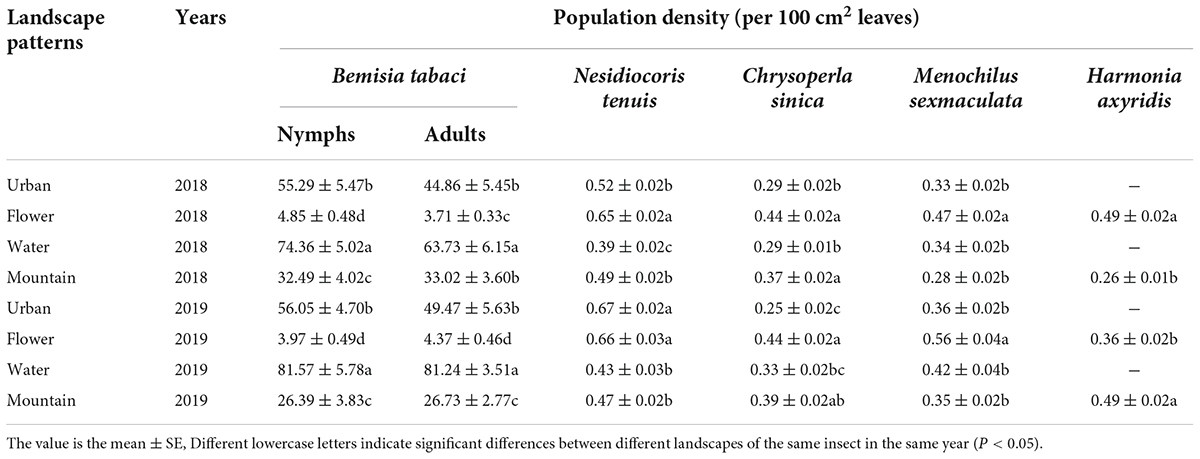
Table 5. Population density of Bemisia tabaci and its dominant predators during the main activity period on summer tomato fields in four different agricultural landscapes.
In 2019, the water landscape had the highest densities of whitefly nymphs during the main activity period (81.57 individuals per 100 cm2 leaves), significantly higher than the other three landscapes [F(3, 50) = 91.99; P = 0.0001], followed by the urban landscape (Table 5). The general trend of the seasonal dynamic curve of adults was similar to that observed in 2018. Adults of whitefly were first observed on July 4, followed by a steady increase until mid-September (Figure 2). During the main activity period, adult densities were the highest in the water landscape (81.24 individuals per 100 cm2 leaves), and significantly higher than in the other three landscapes [F(3, 50) = 100.29; P = 0.0001; Table 5].
Species and dominance of predators of Bemisia tabaci
Nine predator species, belonging to four orders and five families, were sampled in different agricultural landscapes of summer tomato fields. Nesidiocoris tenuis (Reuter) (Hemiptera: Miridae), Chrysoperla sinica Tjeder (Neuroptera: Chrysopidae), and Menochilus sexmaculata (Fabricius) (Coleoptera: Coccinellidae) were the dominant species in urban and water landscape tomato fields, while N. tenuis, C. sinica, M. sexmaculata, and Harmonia axyridis Pallas (Coleoptera: Coccinellidae) were the dominant species in the flower and mountain landscapes, both in 2018 and 2019 (Table 6).
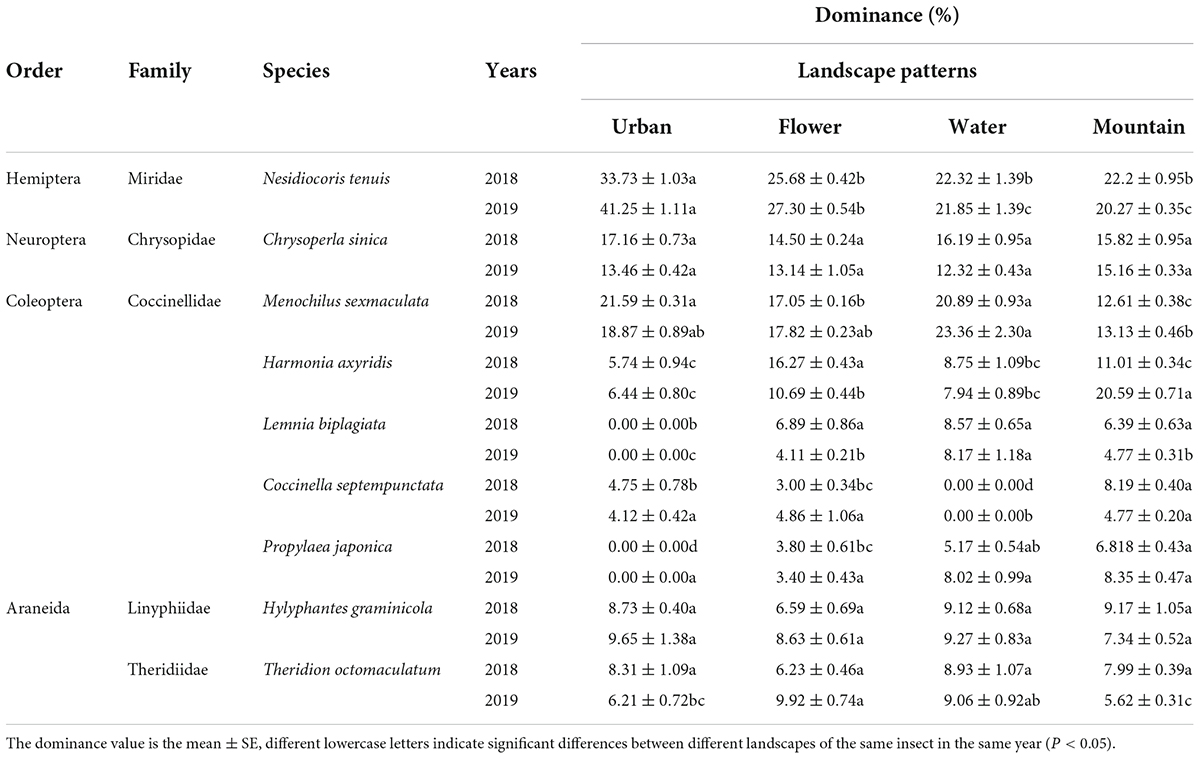
Table 6. Species and dominance of the predators of Bemisia tabaci on summer tomato fields in different agricultural landscapes.
Population dynamics of dominant predators of Bemisia tabaci
In the 2 years of surveys, each reported predatory had a small number of populations at the beginning of the investigation. Thereafter, continued to grow. In 2018, the predator population peaks appeared in urban and mountain landscapes in early September, and predator population peaks appeared in flower and water landscapes in the middle and late August. In 2019, the population peaks of predators appeared in urban and water landscapes in mid-August and the population peaks of predators in flower and mountain landscapes appeared in late August. The population densities of the predators decreased continuously after peaking until the end of the survey during both years of surveys (Figure 3).
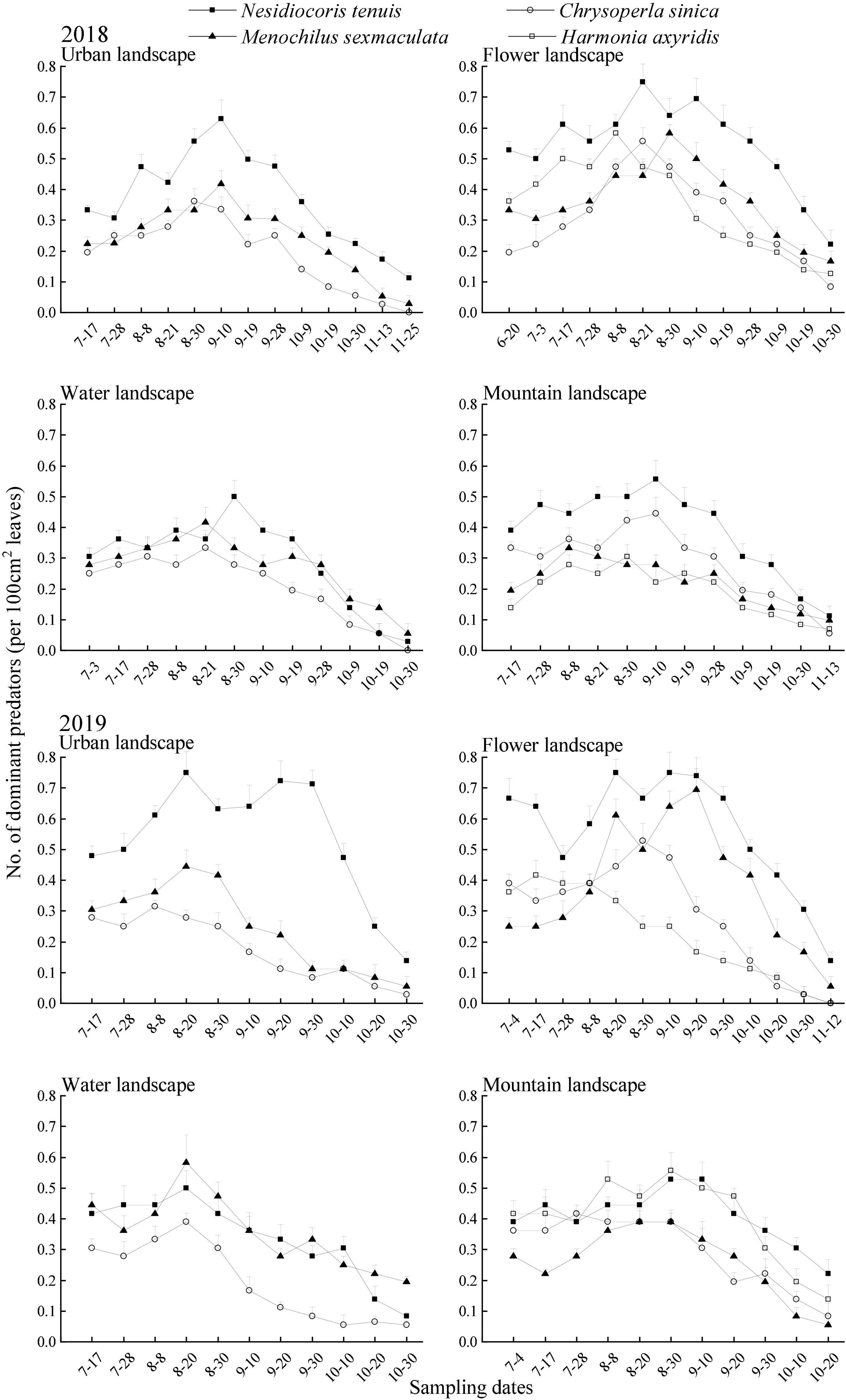
Figure 3. Seasonal dynamics of the dominant predators of Bemisia tabaci (mean + SE) on summer tomato fields in different agricultural landscapes at Kunming city, Yunnan province, South China, in 2018 and 2019.
In 2018, during the main activity period of the predators, the population density of the four dominant predators was the highest in the flower landscape, and the population density of N. tenuis is the highest (0.65 individuals per 100 cm2 leaves; Table 5). In 2019, during the main activity period of the predators, the population density of N. tenuis in the urban landscape was the highest (0.67 individuals per 100 cm2 leaves), and that in the flower landscape was the second (0.67 individuals per 100 cm2 leaves), without significant differences [F(3, 56) = 24.45; P = 0.99]. Both C. sinica and M. sexmaculata showed the highest population density in the flower landscape, while H. axyridis showed the highest population density in the mountain landscape (0.49 individuals per 100 cm2 leaves; Table 5).
Temporal niche analysis of Bemisia tabaci and its dominant predators
Based on the temporal niche widths of B. tabaci and its dominant predators, the two main temporal niche widths in the four different agricultural landscapes were shown by N. tenuis and M. sexmaculata in 2018, while in 2019, the highest temporal niche width was shown by N. tenuis. During the study period of 2 years, each natural enemy had the largest width of the niche in the flower landscape. In the water landscape, the population density of whitefly was the largest, while the temporal niche width was the lowest compared to that in the other three agricultural landscapes (Table 7). Among the four different agricultural landscapes, the temporal niche overlap index of B. tabaci and N. tenuis was highest in 2018, while that of B. tabaci, N. tenuis, and M. sexmaculata was highest in 2019 (Table 7).
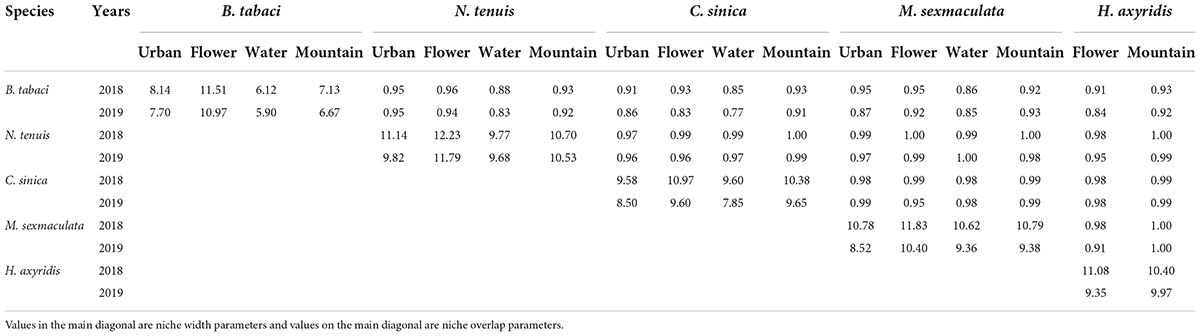
Table 7. Temporal niche parameters of Bemisia tabaci and its dominant predators on summer tomato fields in four different agricultural landscapes.
Discussion
The structure composition of the agricultural landscape is an important factor that affects the occurrence, density, and dynamics of the insect population (Bianchi et al., 2006). The results indicated significant differences in the peak period and population density of B. tabaci and its predators in tomato fields in four different agricultural landscapes. During both years, the water landscape supported the highest density of whiteflies (nymphs and adults). The population density of the nymph and adult of the whitefly was the lowest in the flower landscape. In the study of the population dynamics of whiteflies in different agricultural planting environments, Dou et al. (2020b) also found that the population density of whiteflies in the flower agricultural planting environment was the lowest, which was consistent with the results of our study. We hypothesize that this result is affected by both the composition of the crop and predators in the landscape. The resource concentration hypothesis suggests that phytophagous insects prefer to live in a single host plant habitat (Zhao Z. H. et al., 2015). In this study, the other crops planted around the tomato field in the water landscape were mainly Nelumbo nucifera Gaertn (Proteales: Nelumbonaceae), Vitis vinifera L. (Rhamnales: Vitaceae), and Allium tuberosum L. (Liliflorae: Liliaceae). The diversity of vegetation was low, and they were not the preferred host plant of whiteflies compared to tomatoes (Heng et al., 2017). The proportion of man-made construction land in the urban landscape was also large, and the host plants of the whitefly were relatively single. Therefore, the population density of the whitefly is lower in the early stage of occurrence in the water landscape and urban landscape, and the outbreak is concentrated in tomato fields during the main occurrence period. The number and damage of pests in intercropping of different crops are reduced to varying degrees compared to a single planting (Thies and Tscharntke, 1999). Studies have shown that the intercropping of S. lycopersicum–Apium graveolens L. (Apiales: Apiaceae) or C. sativus–A. graveolens can effectively control the occurrence of Trialeurodes vaporariorum (Westwood) (Hemiptera: Aleyrodidae) (Zhu et al., 2011). Intercropping of main crops with trap plants can be beneficial. Planting Solanum melongena in main Phaseolus vulgaris L. (Rosales: Fabaceae) fields can attract whitefly on P. vulgaris and greatly reduce the population (Smith and Mcsorley, 2000). A study by Russell (1989) demonstrated that the number of pest insects in a single-crop planting system was significantly higher than in a diversified system. Yang et al. (2021) research showed that the population density of whitefly in tomato monoculture fields was higher than in other adjacent cropping patterns during the main occurrence period, which was similar to the findings of our study.
This study shows that the population density of predators was different among the four landscapes, resulting in significant differences in the density of whiteflies. In the flower landscape, the species of dominant predators of whiteflies showed the highest richness and diversity. Studies have shown that landscape factors are the key drivers of predator abundance (Woltz et al., 2012). The natural enemy hypothesis suggests that diverse plant communities can increase natural enemy populations (Zhao Z. H. et al., 2015). Flowering plants can significantly increase the life span of natural enemies and the number of their eggs (Lisa and Steve, 2004). Nectar plants are abundant in the flower landscape. It provides nectar, pollen, the activity place for habitat and reproduction of natural enemy insects (Petanidou et al., 2006; Borghi and Alisdair, 2017), resulting in the highest population density of predators of whitefly in flower landscape tomato fields and inhibiting the outbreak of the whitefly population. This is similar to the research results of Johanowicz and Mitchell (2000), in which the plantation of flowering plants around economic crops had a positive effect on pest control. Moreover, crop diversity can significantly increase the number of natural enemy insects (Dong et al., 2016). The population density of Coccinellidae can be significantly increased by intercropping Saccharum officinarum L. (Poales: Poaceae)-Z. mays (Zhang et al., 2011). The number of parasitoids in a mixed field of Cucurbita moschata (Duch. ex Poiret) (Cucurbitales: Cucurbitaceae)-Z. mays–Pisum sativum L. (Rosales: Fabaceae) is more than two times that in the C. moschata monoculture field (Letourneau, 1987). However, in this study, the diversity of host plant in the urban landscape and the water landscape with low, and the population density of M. sexmaculata is richer than in the mountain landscape, after the flower landscape. This may be due to the relatively large population density of whitefly in urban landscapes and water landscapes, and the strong predation ability of M. sexmaculata, with an obvious following effect (Wu et al., 2010). The population density of N. tenuis is the highest in four different agricultural landscapes, mainly because N. tenuis belongs to omnivorous insects and can suck plant juice when the pest population is low, so it is less affected by the pest population (Zhou et al., 2012). H. axyridis was abundant in the flower landscape in the first year, but in the second year much more was recorded in the mountain landscape. It may be due to the different populations of predators in different landscapes at the beginning of the sampling each year. There is a competitive or predatory relationship between two species with niche overlap (Li et al., 2006). From the perspective of niche, the competitive relationship between several pests and the control of pests by predators can be better analyzed (Dolédec et al., 2000). In this study, B. tabaci, N. tenuis, and M. sexmaculata were temporally synchronized, with high similarity in time resources. This result is consistent with the study by Li et al. (2021) on the population dynamics and temporal niches of whitefly and their dominant predators in cucumber and tomato fields. The values of the temporal niche width parameter of each species and the temporal niche overlap parameter of whitefly and its predators were the highest in flower landscapes. In the flower landscape, the encounter frequency between predators and whitefly at the same time is higher than that in the other landscapes, and it shows that the control effect of predators on whitefly is better (Hurlbert, 1978). Planting flowering plants of Asteraceae in winter wheat fields can significantly enhance the control effect of natural enemies on wheat Chrysomelidae pests (Tschumi et al., 2015, 2016). Planting flowering plants such as Tagetes erecta L. (Asterales: Asteraceae), Callistephus chinensis (L.) (Campanulales: Asteraceae), and Medicago sativa L. (Rosales: Fabaceae) in apple orchards can increase the control effect of natural enemy insects on Grapholita molesta Busck (Lepidoptera: Tortricidae) (Mu et al., 2019). These studies were consistent with our findings.
From the perspective of the vegetation diversity and the landscape complex, flower landscape and mountain landscape have more plant species than other landscapes in our study. Based on the ecological regulation theories of pests such as the landscape complexity hypothesis, using plant diversity for habitat regulation can effectively improve the colonization rate of natural enemies and the ability of sustainable pest control (Cook et al., 2007). Complex plant diversity represents more plant species, which can provide food, wintering, and breeding habitat for natural enemies and help them reduce the possibility of pesticides and farming interference. According to the role and function of natural enemies, it can be divided into banker plant, nectar resource plant, habitat plant, trap plant, indicator plant, and guardian plant (Parolin et al., 2012; Chen et al., 2014). They are conducive to the growth of natural enemy populations in the ecosystem (Chen et al., 2014; Miller et al., 2018). Moreover, complex plant diversity can also increase insect diversity within the ecosystem. Most predatory natural enemies are polyphagous insects. The increase in insect diversity also provides predatory natural enemies with more diverse alternative prey like aphids, thrips, mealybugs, etc., which is also conducive to the reproduction of natural enemy insects (Yongheon et al., 2004; Pineda and Marcos-García, 2008; Rattanapun, 2017).
The natural control ability of the whitefly predators was greatly affected by the change in the planting environment (Zhang et al., 2020a). The flower landscape has obvious advantages among the four agricultural landscapes. Flowering plants can provide nutrients such as pollen and nectar to predators (Zhu et al., 2012). However, herbivorous insects can also use pollen and nectar as food sources to increase their population (Heimpel and Jervis, 2006). Thus, it is necessary to select flowering plants and obtain favorable flower species with care. The principle is to select flowering plants that can maximize the benefits to predators and minimize the interest of pests during crop growth (Evans et al., 2010). In the water landscape, due to the single planting mode and low vegetation diversity, it is easy to cause an outbreak of the pest population, and the occurrence and damage of the tomato field by whitefly was the most serious in that landscape. Therefore, a suitable layout of the agricultural landscape, such as fixing an appropriate proportion of flowering plants and increasing the diversity of planting crops, can effectively reduce the possibility of a whitefly outbreak.
Data availability statement
The raw data supporting the conclusions of this article will be made available by the authors, without undue reservation.
Author contributions
G-HC, X-MZ, and W-JD designed the study. W-JD, M-JL, S-WY, and H-PS performed the experiments. M-JL, S-WY, and X-MZ analyzed the data and wrote the manuscript. All authors read and approved the final manuscript.
Funding
This research was supported by the National Natural Science Foundation of China (31760541), Project of Basic Research in Yunnan Province (202201AT070269), Reserve Talents of Young and Middle-aged Academic and Technological Leaders in Yunnan Province (202105AC160071), the Young Top Talents of “High-level Talents Training Support Program in Yunnan Province” (YNWRQNBJ2020291), Guangdong Natural Science Foundation (2020A1515011098), and the National Fund for Studying Abroad [LJX (2021) No. 15].
Acknowledgments
We thank Ms. Wenjing Duan, Xiaoyun Wang, Jianwen Lyu, and Mr. Fei Wang (Yunnan Agricultural University) for helping in the field sampling. We thank Mr. Jinyao Liu (Xicun Village, Yiliang County, Kunming City), Xingming Pan (Huangpo Village, Fumin County, Kunming City), and Ms. Fengping Duan (Huangpo Village, Fumin County, Kunming City) for helping of to manage our fields during our experiment. We also thank Elsevier Language Editing translation company for editing the English of the manuscript.
Conflict of interest
W-JD was employed by the Yunnan Expo Horticulture Co., Ltd.
The remaining authors declare that the research was conducted in the absence of any commercial or financial relationships that could be construed as a potential conflict of interest.
Publisher’s note
All claims expressed in this article are solely those of the authors and do not necessarily represent those of their affiliated organizations, or those of the publisher, the editors and the reviewers. Any product that may be evaluated in this article, or claim that may be made by its manufacturer, is not guaranteed or endorsed by the publisher.
References
Barro, D. P. J., Liu, S. S., Boykin, L., and Dinsdale, A. (2011). Bemisia tabaci: a statement of species status. Annu. Rev. Entomol. 56, 1–19. doi: 10.1146/annurev-ento-112408-085504
Bian, Z. X., Gong, L. C., Guo, X. Y., and Yu, M. (2019). Effects of agricultural landscape composition on natural enemy population of corn borer. Chin. J. Eco-Agricult. 27, 30–41. doi: 10.13930/j.cnki.cjea.180221
Bianchi, F. J. J. A., Booij, C. J. H., and Tscharntke, T. (2006). Sustainable pest regulation in agricultural landscapes: a review on landscape composition, biodiversity and natural pest control. Proc. Roy. Soc. B-Biol. Sci. 273:1595. doi: 10.1098/rspb.2006.3530
Borghi, M., and Alisdair, R. F. (2017). Floral metabolism of sugars and amino acids: implications for pollinators’ preferences and seed and fruit set. Plant Physiol. 175, 1510–1524. doi: 10.1104/pp.17.01164
Chaplin-Kramer, R., O’Rourke, M. E., Blitzer, E. J., and Kremen, C. (2011). A meta-analysis of crop pest and natural enemy response to landscape complexity. Ecol. Lett. 14, 922–932. doi: 10.1111/j.1461-0248.2011.01642.x
Chen, X. X., Liu, Y. Q., Ren, S. X., Zhang, F., Zhang, W. Q., and Ge, F. (2014). Plant-mediated support system for natural enemies of insect pests. Chin. J. Appl. Entomol. 51, 1–12. doi: 10.7679/j.issn.2095-1353.2014.001
Cook, S. M., Khan, Z. R., and Pickett, J. A. (2007). The use of push-pull strategies in integrated pest management. Annu. Rev. Entomol. 52, 375–400. doi: 10.1146/annurev.ento.52.110405.091407
Dolédec, S., Chessel, D., and Clémentine, G. C. (2000). Niche separation in community analysis: a new method. Ecology 81, 2914–2927. doi: 10.2307/177351
Dong, W. X., Xiao, C., and Li, C. Y. (2016). Effects of diversified on insect pests and natural enemies in agroecosystems. Chin. J. Eco-Agricult. 24, 435–442. doi: 10.13930/j.cnki.cjea.20160106
Dou, W. J., Yang, S. W., Liu, Q., Chen, G. H., and Zhang, X. M. (2020a). Progress in the control of predatory and parasitic natural enemies of Bemisia tabaci in China. J. Environ. Entomol. 42, 342–354. doi: 10.3969/j.issn.1674-0858.2020.02.14
Dou, W. J., Yang, S. W., Liu, Q., Lyu, J. W., Zhang, X. M., and Chen, G. H. (2020b). Population dynamics and spatial distribution of Bemisia tabaci (Gennnadius) in tomato fields under different agricultural growing environments. J. S. Agricult. 51, 2470–2479. doi: 10.3969/j.issn.2095-1191.2020.10.019
Evans, E. W., Anderson, M. R., and Bowling, P. D. (2010). Targeted sugar provision promotes parasitism of the cereal leaf beetle Oulema melanopus. Agr. Forest. Entomol. 12, 41–47. doi: 10.1111/j.1461-9563.2009.00448.x
Fazekas, J., Kádár, F., Sárospataki, M., and Lövei, G. L. (1997). Seasonal activity, age structure and egg production of the ground beetle Anisodactylus signatus (Coloptera: Carabidae) in Hungary. Eur. J. Entomol. 94, 473–484.
Gao, Y. B., Shi, S. S., Sun, W., Zhang, Q., Zhou, J. C., and Li, Q. Y. (2014). Analysis of the temporal niches of dominant species and nutritional relationships within the arthropod community in soybean fields. Chin. J. Appl. Entomol. 51, 392–399. doi: 10.7679/j.issn.2095-1353.2014.046
Gerling, D., Alomar, O., and Arno, J. (2001). Biological control of Bemisia tabaci using predators and parasitoids. Crop. Prot. 20, 779–799. doi: 10.1016/S0261-2194(01)00111-9
Heimpel, G. E., and Jervis, M. A. (2006). Plant-provided food for carnivorous insects: a protective mutualism and its applications. Environ. Entomol. 35, 1137–1137. doi: 10.1603/0046-225X-35.4.1137
Heng, S., Zhou, F. C., Chen, X. H., Su, H. H., Zhao, B., Shao, Y. D., et al. (2017). Repellent effect of several low-preference host vegetables against the tobacco whitefly Bemisia tabaci. J. Biosafety 26, 168–172. doi: 10.3969/j.issn.2095-1787.2017.02.010
Hurlbert, S. H. (1978). The measurement of niche overlap and some relatives. Ecology 59, 67–77. doi: 10.2307/1936632
Jiang, J. (2020). Effects of interactive infesting Chinese kale with Plutella xylostella and Bemisia tabaci on biology of Encarsia formosa and Cotesia plutellae. Yangling: Northwest A&F University, doi: 10.27409/d.cnki.gxbnu.2020.001355
Johanowicz, D. L., and Mitchell, E. R. (2000). A novel method to rear Diadegma insulare (Hymenoptera: Ichneumonidae), a parasitoid of the diamondback moth (Lepidoptera: Plutellidae). Florida Entomol. 83, 41–47. doi: 10.2307/3496363
Jonsson, M., Buckley, H. L., Case, B. S., Wratten, S. D., Hale, R. J., and Didham, R. K. (2012). Agricultural intensification drives landscape-context effects on host-parasitoid interactions in agroecosystems. J. Appl. Ecol. 49, 706–714. doi: 10.1111/j.1365-2664.2012.02130.x
Lenteren, J. C. V., Alomar, O., Ravensberg, W. J., and Urbaneja, A. (2020). “Integrated pest and disease management in greenhouse crops,” in Integrated Pest and Disease Management in Greenhouse Crops, Plant Pathology in the 21st Century 9, eds M. L. Gullino, R. Albajes, and P. C. Nicot (Berlin: Springer International Publishing), 409–439. doi: 10.1007/978-3-030-22304-5
Lenteren, J. C. V., Bolckmans, K., Köhl, J., Ravensberg, W. J., and Urbaneja, A. (2018). Biological control using invertebrates and microorganisms: plenty of new opportunities. Biocontrology 63, 39–59. doi: 10.1007/s10526-017-9801-4
Letourneau, D. K. (1987). The enemies hypothesis: Tritrophic interactions and vegetational diversity in tropical agroecosystems. Ecology 68, 1616–1622. doi: 10.2307/1939853
Levins, R. (1969). Evolution in changing environments: some theoretical explorations. Princeton Univ. Press 19, 659–660. doi: 10.2307/1294958
Li, D. Z., Shi, Q., Zang, R. G., Wang, X. P., Sheng, L. J., Zhu, Z. L., et al. (2006). Models for niche breadth and niche overlap of species or populations. Sci. Silvae Sin. 42, 95–103. doi: 10.11707/j.1001-7488.20060718
Li, G., Wu, Y. Q., Chen, W. H., Du, L. N., and Xu, F. C. (2010). Spatial distribution patterns of tomato leaf curl virus and its vector Bemisia tabaci. Acta Agricult. Zhejiangensis 22, 779–783. doi: 10.3969/j.issn.1004-1524.2010.06.015
Li, S. J., Xue, X., Muhammad, Z. A., Ren, S. X., Du, Y. Z., Wu, J. H., et al. (2011). Host plants and natural enemies of Bemisia tabaci (Hemiptera: Aleyrodidae) in China. Insect Sci. 18, 101–120. doi: 10.1111/j.1744-7917.2010.01395.x
Li, X. X., Yang, S. W., Li, M. J., Jiang, Z. X., Liu, Y. H., Chen, G. H., et al. (2021). Population dynamics and temporal niches of Bemisia tabaci and its dominant natural enemies on cucumber and tomato cultivated under greenhouse planting. J. S. Agricult. 52, 518–526. doi: 10.3969/j.issn.2095-1191.2021.02.029
Lisa, A. B., and Steve, D. W. (2004). Effects of alyssum flower fields on the longevity, fecundity, and sex ratio of the leafroller parasitoid Dolichogenidea tasmanica. Biol. Control 32, 65–69. doi: 10.1016/j.biocontrol.2004.07.014
Liu, B., Yang, L., Yang, Y., and Lu, Y. (2016). Influence of landscape diversity and composition on the parasitism of cotton bollworm eggs in maize. PLoS One 11:e0149476. doi: 10.1371/journal.pone.0149476
Liu, Y. F., Zhang, G. R., and Gu, D. X. (2000). Effect and the acting mechanisms of the habitats and vegetational diversity on arthropod community in agroecosystem. J. Xiangtan Nor. Univ. 21, 74–78.
Liu, Y. J., and Xia, J. F. (2017). Research progress on pest management by natural enemy insects in Yunnan province. Modern Agricult. Sci. Technol. 14, 129–133. doi: 10.3969/j.issn.1007-5739.2017.14.086
Miao, X. W. (2017). Research progress of tomato yellow leaf curl virus disease. China Fruit Veg. 37, 31–35. doi: 10.19590/j.cnki.1008-1038.2017.03.008
Miller, P., Tracey, L., and Rebek, E. J. (2018). Banker plants for aphid biological control in greenhouses. J. Integr. Pest Manage. 9, 1–8. doi: 10.1093/jipm/pmy002
Mu, B. F., Dong, Z. K., Liu, S. F., Li, C., Kou, H. R., and Zhang, Z. Y. (2019). Effects of four species of refuge plants on population dynamics of oriental fruit moth. Grapholita molesta and its major natural enemies. J. Beijing Univ. Agricult. 34, 39–44. doi: 10.13473/j.cnki.issn.1002-3186.2019.0105
Muniyappa, V., and Veeresh, G. K. (1984). Plant virus diseases transmitted by whiteflies in Karnataka. Proc. Anim. Sci. 93, 397–406. doi: 10.1007/BF03186259
Pan, L. L., Du, H., Ye, X. T., and Wang, X. W. (2021). Whitefly adaptation to and manipulation of plant resistance. Sci. China Life Sci. 64, 648–651. doi: 10.1007/s11427-020-1890-9
Parolin, P., Bresch, C., Desneux, N., Brun, R., Bout, A., Boll, R., et al. (2012). Secondary plants used in biological control: a review. Int. J. Pest. Manage. 58, 91–100. doi: 10.1080/09670874.2012.659229
Petanidou, T., Laere, A. V., Ellis, W. N., and Smets, E. (2006). What shapes amino acid and sugar composition in Mediterranean floral nectars? Oikos 115, 155–169. doi: 10.1111/j.2006.0030-1299.14487.x
Pineda, A., and Marcos-García, M. Á (2008). Introducing barley as aphid reservoir in sweet-pepper greenhouses: Effects on native and released hoverflies (Diptera: Syrphidae). Eur. J. Entomol. 105, 531–535. doi: 10.14411/eje.2008.070
Rattanapun, W. (2017). Banker plant system using Hysteroneura setariae (Thomas) (Hemiptera: Aphididae) as a non-pest prey to build up the lady beetle populations. J. Asia-Pac. Entomol. 20, 437–440. doi: 10.1016/j.aspen.2017.02.016
Ren, S. X., Wang, Z. Z., Qiu, B. L., and Xiao, Y. (2001). The pest status of Bemisia tabaci in China and non-chemical control strategies. Entomol. Sin. 3, 279–288. doi: 10.1007/s11769-001-0054-9
Russell, E. P. (1989). Enemies hypothesis: a review of the effect of vegetational diversity on predatory insects and parasitoids. Environ. Entomol. 18, 590–599. doi: 10.1093/ee/18.4.590
Smith, H. A., and Mcsorley, R. (2000). Potential of field corn as a barrier crop and eggplant as a trap crop for management of Bemisia argentifolii (Homoptera: Aleyrodidae) on common bean in north Florida. Florida Entomol. 83, 145–158. doi: 10.2307/3496150
Suzanne, W. T. B. (1982). Biological control in agroecosystems. Science 215, 134–139. doi: 10.1126/science.215.4529.134
Thies, C., and Tscharntke, T. (1999). Landscape structure and biological control in agroecosystems. Science 285, 893–895. doi: 10.1126/science.285.5429.893
Tscharntke, T., Steffan, D. I., Kruess, A., and Thies, C. (2002). Contribution of small habitat fragments to conservation of insect communities of grassland-cropland landscapes. Ecol. Appl. 12, 354–363. doi: 10.2307/3060947
Tschumi, M., Albrecht, M., Collatz, J., Dubsky, V., Entling, M. H., Najar-Rodriguez, A. J., et al. (2016). Tailored flower strips promote natural enemy biodiversity and pest control in potato crops. J. Appl. Ecol. 53, 1169–1176. doi: 10.1111/1365-2664.12653
Tschumi, M., Albrecht, M., Entling, M. H., and Jacot, K. (2015). High effectiveness of tailored flower strips in reducing pests and crop plant damage. Proc. Roy. Soc. B-Biol. Sci. 282:1814. doi: 10.1098/rspb.2015.1369
Veres, A., Petit, S., Conord, C., and Lavigne, C. (2013). Does landscape composition affect pest abundance and their control by natural enemies? A review. Agr. Ecosyst Environ. 166, 110–117. doi: 10.1016/j.agee.2011.05.027
Woltz, J. M., Isaacs, R., and Landis, D. A. (2012). Landscape structure and habitat management differentially influence insect natural enemies in an agricultural landscape. Agr. Ecosyst Environ. 152, 40–49. doi: 10.1016/j.agee.2012.02.008
Wu, H. S., Han, S. C., Zhang, Y. H., Li, Z. G., and Zheng, J. H. (2010). Predation of Lemnia biplagiata and Menochilus sexmaculatus adults to larvae of Aleurodicus dispers. J. Environ. Entomol. 32, 380–383. doi: 10.3969/j.issn.1674-0858.2010.03.015
Yang, S. W., Lyu, J. W., Dou, W. J., Li, M. J., Liu, Y. H., Chen, G. H., et al. (2021). Effects of different adjacent crops on the population dynamics of Bemisia tabaci in tomato fields. Chin. J. Ecol. 40, 163–170. doi: 10.13292/j.1000-4890.202101.008
Yang, X., Xie, W., Wang, S. L., Wu, Q. J., Xu, B. Y., Zhou, X. M., et al. (2014). Resistance and expression of cyp4v2 and cyp6cx1 mRNA in Bemisia tabaci in Beijing, Shandong and Hunan provinces. Plant Protect. 40, 70–75. doi: 10.3969/j.issn.0529-1542.2014.04.013
Yongheon, K., Jeonghwan, K., and Hoddle, M. S. (2004). “Biological control of Aphis gossypii using barley banker plants in greenhouse grown oriental melon,” in Proceedings of the California Conference on Biological Control IV, (Berkeley, CA: University of California), 124–126.
You, M. S., Hou, Y. M., Liu, Y. F., Yang, G., Li, Z. S., and Cai, H. J. (2004). Non-crop habitat manipulation and integrated pest management in agroecosystems. Acta Entomol. Sin. 47, 260–268. doi: 10.3321/j.issn:0454-6296.2004.02.021
Yu, X. P., Hu, C., and Heong, K. L. (1996). The effects of non-crop habitats on crop pests and their natural enemies. Chin. J. Biol. Control 3, 37–40. doi: 10.16409/j.cnki.2095-039x.1996.03.011
Zhang, H. Y., Chen, B., Li, Z. Y., Yang, J. C., Yang, J., Shan, H. H., et al. (2011). Effect of sugarcane plants intercropped with maize on population of Ceratovacuna lanigera Zehntner and Coccinellidae. Southwest China J. Agricult. Sci. 24, 124–127. doi: 10.16213/j.cnki.scjas.2011.01.018
Zhang, X. (2017). The Effects of Agriculture Landscape Pattern on the Diversity of Ground Carabid and Spider. Beijing: China Agricultural University.
Zhang, X. M., Ferrante, M., Wan, F. H., Yang, N. W., and Lövei, G. L. (2020a). The parasitoid Eretmocerus hayati is compatible with barrier cropping to decrease whitefly (Bemisia tabaci MED) densities on cotton in China. Insects 11:57. doi: 10.3390/insects11010057
Zhang, X. M., Lövei, G. L., Ferrante, M., Yang, N. W., and Wan, F. H. (2020b). The potential of trap and barrier cropping to decrease densities of the whitefly Bemisia tabaci MED on cotton in China. Pest. Manag. Sci. 76, 366–374. doi: 10.1002/ps.5524
Zhang, X. M., Yang, N. W., and Wan, F. H. (2014a). Population density of Bemisia tabaci on different plants in the field. Acta Ecol. Sin. 34, 4652–4661. doi: 10.5846/stxb201212191828
Zhang, X. M., Yang, N. W., Wan, F. H., and Lövei, G. L. (2014b). Density and seasonal dynamics of Bemisia tabaci (Gennadius) Mediterranean on common crops and weeds around cotton fields in northern China. J. Integr. Agr. 13, 2211–2220. doi: 10.1016/S2095-3119(13)60613-9
Zhao, J., Dai, J. J., Zhao, H. K., and Zhang, W. L. (2019). Production status and countermeasures of large and medium sized tomato in Yunnan province in 2018. J. Changjiang Veg. 9, 4–5.
Zhao, K. D., Fang, O. Y., Fei, L., and Feng, G. (2015). Shelterbelts in agricultural landscapes enhance ladybeetle abundance in spillover from cropland to adjacent habitats. Bio. Control 60, 351–361. doi: 10.1007/s10526-015-9648-5
Zhao, Z. H., Gao, F., He, D. H., and Ge, F. (2015). Ecologically based pest management at multiple spatial scales. Sci. Sin. Vitae 45, 755–767. doi: 10.1360/n052014-00314
Zhou, K., Huang, J. K., Deng, X. Z., Werf, W. V. D., Zhang, W., Lu, Y. H., et al. (2014). Effects of land use and insecticides on natural enemies of aphids in cotton: first evidence from smallholder agriculture in the North China Plain. Agr. Ecosyst. Environ. 183, 176–184. doi: 10.1016/j.agee.2013.11.008
Zhou, Z., Wang, M. Q., Hu, Y., and Chen, H. Y. (2012). Morphological structure of the antennal sensilla of Nesidiocoris tenuis observed with a scanning electron microscope. Chin. J. Appl. Entomol. 49, 631–635.
Zhu, P. X., Liu, M. C., Qin, Y. C., Xie, J. J., and Liu, Y. H. (2011). Control effects of whitefly by intercropping celery in greenhouse. Chin. J. Appl. Entomol. 48, 375–378.
Keywords: Bemisia tabaci, agriculture landscapes, population dynamics, dominance, temporal niche, predators
Citation: Li M-J, Yang S-W, Chen G-H, Dou W-J, Shang H-P and Zhang X-M (2022) Density and seasonal dynamics of Bemisia tabaci and its predators in different agricultural landscapes in South China. Front. Plant Sci. 13:928634. doi: 10.3389/fpls.2022.928634
Received: 26 April 2022; Accepted: 09 August 2022;
Published: 02 September 2022.
Edited by:
Emanuele Radicetti, University of Ferrara, ItalyReviewed by:
Aqeel Alyousuf, University of Basrah, IraqKonstantina Arvaniti, Agricultural University of Athens, Greece
Copyright © 2022 Li, Yang, Chen, Dou, Shang and Zhang. This is an open-access article distributed under the terms of the Creative Commons Attribution License (CC BY). The use, distribution or reproduction in other forums is permitted, provided the original author(s) and the copyright owner(s) are credited and that the original publication in this journal is cited, in accordance with accepted academic practice. No use, distribution or reproduction is permitted which does not comply with these terms.
*Correspondence: Xiao-Ming Zhang, zxmalex@126.com
†These authors have contributed equally to this work and share first authorship