- 1Department of Horticulture and Crop Science, Ohio Agricultural Research and Development Center, The Ohio State University, Wooster, OH, United States
- 2Department of Plant Pathology, Ohio Agricultural Research and Development Center, The Ohio State University, Wooster, OH, United States
Symbiotic fungi in the genus Trichoderma can induce abiotic stress tolerance in crops. The beneficial effects of Trichoderma on water deficit stress are poorly understood and may be isolate-specific. Our objective was to evaluate a collection of Nepalese Trichoderma isolates and their efficacy to improve tomato (Solanum lycopersicum) growth under water deficit. Variable growth in low moisture environments was observed among Trichoderma isolates from Nepal, Ohio, and commercial sources using in vitro assays. The overall performance of the population decreased when cultured under conditions of decreasing matric water potential (0.0, –2.8, –4.8, and –8.5 Ψ). Twelve isolates were selected for evaluation for their potential to elicit drought tolerance in greenhouse-grown ‘Roma Organic’ tomatoes. Plants treated with T. asperelloides-NT33 had higher shoot weight than the non-inoculated control (T0) under water deficit stress conditions. Further, the stress-reducing efficacy of isolates T. asperelloides-NT33, T. asperellum-NT16, T. asperelloides-NT3, and commercial T. harzianum-T22 were tested on tomato genotypes with differing tolerance to drought [‘Roma Organic,’ ‘Jaune Flamme,’ and ‘Punta Banda’]. The water deficit susceptible genotypes ‘Roma Organic’ and ‘Jaune Flamme’ inoculated with isolate NT33 had significantly higher shoot weight (37 and 30% respectively; p < 0.05) compared to the non-inoculated control under water deficit stress conditions. In drought tolerant ‘Punta Banda,’ shoot weight was also significantly greater in NT33 inoculated plants under water deficit stress conditions, but with lower magnitude difference (8%; p < 0.05). Our results demonstrate differences in the ability of Trichoderma isolates to confer tolerance to water deficit in tomato with NT33 potentially relieving stress. Tomato genotypes also play a role in the outcome of interactions with the Trichoderma isolates we tested.
Introduction
By 2050, water consumption for agricultural practices will increase by 60% to support a growing world population (Boretti and Rosa, 2019). Concurrently, drought is becoming a dominant environmental stressor for crop production globally, limiting food security with precarious economic and sociological impacts (Trenberth et al., 2014). Due to climate change, water deficit is spreading to regions where drought was infrequent in the past (Somerville and Briscoe, 2001). Globally, the reduction in yield due to drought is likely to exceed the combined loss of all other possible causes of yield decline (Blum, 1996; Foolad, 2007). Therefore, there is a need to identify solutions to mitigate water deficit stress and its impact on food security.
Tomato (Solanum lycopersicum L.) is the world’s second most important vegetable crop in per capita consumption (Singh et al., 2018). On a global scale, tomatoes are grown on 5 million hectares of land with an annual production of about 180 million tons, and a production value of more than 90 billion USD (FAO, 2022). Currently, drought is a significant environmental stressor challenging tomato productivity in areas of inadequate or erratic rainfall that lack irrigation options (Petrozza et al., 2014; Pal et al., 2016). Drought decreases crop production on 25% of arable land throughout the world. Thus, where tomatoes are produced under rainfed agricultural conditions, there is a need to identify multi-faceted, low-cost, environmentally friendly, and sustainable solutions to water deficit that can be implemented on a short-term basis. Symbiotic associations with stress-mitigating soil microorganisms may serve as one of those solutions.
Naturally occurring beneficial soil microorganisms, including members of the genus Trichoderma (Family – Hypocreaceae), are studied as accessible and sustainable tools for enhancing plant growth and mitigating biotic and abiotic stressors in both research and commercial production settings (Van Wees et al., 2008; Grover et al., 2011; Kim et al., 2012; Meena et al., 2017; Khadka and Miller, 2021). As such, specific commercial Trichoderma isolates are widely used as effective biocontrol agents, biofertilizers, and phyto-stimulators (Harman, 2011; Hermosa et al., 2012; Waghunde et al., 2016; Kashyap et al., 2017; Khan et al., 2017), but evidence of contributions to abiotic stress amelioration in crop species is sparse. Several recent studies suggest that Trichoderma inoculation of water-stressed plants enhanced growth by improving root biomass, enhancing water holding capacity, and mobilizing nutrients (Mastouri et al., 2010; Harman, 2011; Bakhshandeh et al., 2020). Further, Trichoderma inoculated plants exhibit delayed wilting, increased stomatal conductance, enhanced leaf chlorophyll content, and greater net photosynthesis levels under water deficit stress conditions (Bae et al., 2009; Shukla et al., 2012; Alwhibi et al., 2017; Harman et al., 2019).
Plant genotype influences the degree of colonization and crop stress-mitigating potential of Trichoderma even for the established commercial isolates (Harman et al., 2004; Prashar and Vandenberg, 2017). The beneficial effects of Trichoderma, however, are more pronounced when plants are under stress compared to when grown under optimal conditions (Mastouri et al., 2010; Lombardi et al., 2018). As it has been demonstrated for other fungi, there is also potential that changes in climate patterns can influence Trichoderma distribution and abundance, as well as the outcome of the plant–microbial interaction (Compant et al., 2010; Tyagi et al., 2014; Delgado-Baquerizo et al., 2020). However, whether different species or isolates of Trichoderma have adapted as symbionts in stress environments remains unclear.
The overall goal of this research was to evaluate the ability of novel isolates from several species of Trichoderma, primarily native to different agroecological regions in Nepal, to improve growth in different tomato genotypes exposed to water deficit stress. Our study involved a series of experiments focused on: (1) assessing these novel isolates for their desiccation tolerance and their efficacy for alleviating water deficit stress symptoms (e.g., reduced biomass accumulation and diminished physiological function) in tomato genotypes differing in water deficit tolerance; (2) delineating the physiological (e.g., relative water content, electrolyte leakage, and photosynthetic efficiency) and biochemical (e.g., total and individual phenolic, proline and hormonal level) changes in leaves of a Trichoderma-inoculated, susceptible tomato genotype subjected to water stress; and (3) identifying and mapping genes differentially expressed in leaves of the inoculated tomato genotype under water stress and irrigated conditions (Rawal, 2021). Herein, we report our assessment of the novel Trichoderma isolates as plant growth promoters with the potential to alleviate growth-related and physiological stress symptoms across tomato genotypes that differ in water deficit stress tolerance. We hypothesized that (a) Trichoderma isolates will differ in their efficacy to diminish the effects of water deficit stress on tomato plant growth; (b) that isolates collected from the drier regions will tolerate desiccation conditions and have a greater impact on water deficit stress amelioration than those from wetter regions, and (c) that the effect of selected Trichoderma isolates on growth and water deficit stress will depend on tomato genotype. To ascertain the validity of the latter, we pursued a supporting goal of surveying heirloom and land races within The Ohio State University tomato germplasm collection for water deficit susceptibility or tolerance with the aim of including one or more of these genotypes in our Trichoderma trials.
Materials and Methods
Nepalese Trichoderma isolates used in this study were imported under the US Department of Agriculture Animal and Plant Health Inspection Service (APHIS) permit #P526P-19-03121. All experimental procedures involving fungal isolates were approved by The Ohio State University Institutional Biosafety Committee as described in Protocol 2019R00000043. These experiments were performed in BSL-2 rated laboratories and greenhouses. A general overview of the experiments reported in this work is shown in Figure 1.
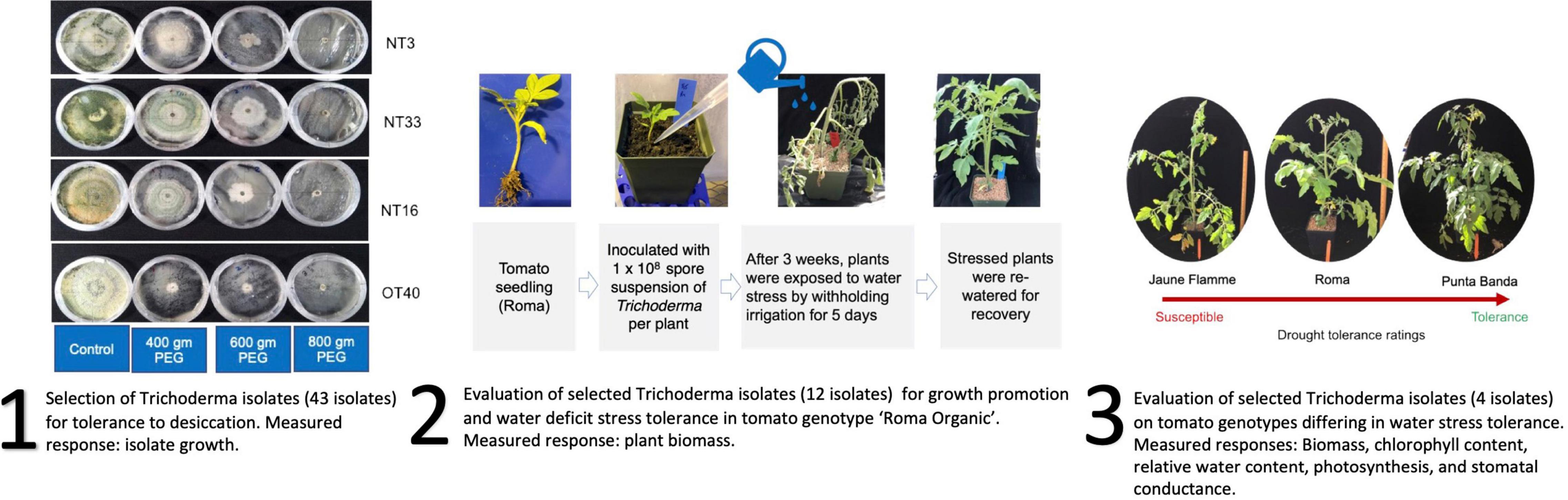
Figure 1. Overview of the replicated experiments reported in this manuscript. First, a collection of isolates from different geographical regions in Nepal were screened for desiccation tolerance. Second, a subset of these isolates was selected to screen for water deficit amelioration in ‘Roma Organic’ tomato. And third, the superior isolates were tested to evaluate Trichoderma × tomato genotype interaction in water deficit stress amelioration. Response variables measured in each set of experiments is included in the figure.
Selection of Trichoderma Isolates for Tolerance to Desiccation
Forty-one different Trichoderma isolates collected from diverse agroecological regions of Nepal (designated as NT1-NT39, NT42, NT43) and two Trichoderma isolates from Ohio (OT41, OT42) were selected for this study. Molecular and morphological characterization of all isolates used in this study is presented in Khadka et al. (2022). Nepalese Trichoderma isolates were recovered from diverse agricultural soils found at environmentally diverse sites (arid, saline, alpine regions), representing potential reservoirs of microorganisms with traits that may benefit plant hosts when grown under differing environmental conditions (Supplementary Figure 1 and Supplementary Table 1). All isolates were evaluated for their capacity to tolerate and grow under severe desiccation conditions. A commercial isolate, T. harzianum Rifai (T22) was used as a positive control. This isolate can induce systemic resistance in plants and is available commercially as a bio-stimulant (Mastouri et al., 2010; Harman, 2011). These Trichoderma isolates were maintained and preserved on silica gel (Samuels, 2015), and isolates were recovered on Potato Dextrose Agar (PDA) plates (Sigma- Aldrich Inc., St. Louis, MO, United States) at 25°C with a 12 h light/dark cycle for seven days.
Desiccation conditions were created by dissolving polyethylene glycol 8000 MW (PEG) in Potato Dextrose Agar (PDA) medium (Sigma- Aldrich Inc., St. Louis, MO, United States) according to the procedure described by Aujla and Paulitz (2017). Different matric water potentials (0.0, –2.8, –4.8, and –8.5 Ψ) were generated by adding 0, 400, 600, and 800 g PEG (8,000 MW, Fisher Scientific Co., Pittsburgh, PA, United States) into 1 l of PDA, respectively. Each medium’s water potential was determined by using a WP4C dew point potentiometer (Decagon Devices, Inc., Pullman, WA, United States). PDA medium without PEG served as a non-desiccation control. Sterilized PDA + PEG mixtures in 10 ml quantities were poured into individual sterile Petri dishes. A 10 cm diameter sterile cellophane disk (Bio-Rad Laboratories Inc., San Francisco, CA, United States) was embedded into each treatment and control Petri dish containing semi-solid PDA + PEG to provide a solid support for the growth of each Trichoderma isolate (Ruano Rosa and López Herrera, 2009). For the matric potential treatments, plates were inoculated with a 5 mm diameter PDA disk of mycelia of an actively growing Trichoderma culture. The Petri dishes were sealed with Parafilm and were then incubated at 28°C for seven days. The growth of the Trichoderma colony was measured (cm) along two diameters perpendicular to each other (D1 and D2) and the average of these two values was calculated (D1 + D2/2). A total of 44 isolates were evaluated for desiccation tolerance. For each isolate, the desiccation treatment was replicated three times, and plates were arranged in a completely randomized design. The isolates were ranked at each osmotic stress condition based on colony diameter. Ranked colony diameters were correlated among treatment groups in individual responses to increased osmotic stress. The experiment was performed twice.
Selection of Water Deficit Susceptible Tomato Genotypes
We tested 70 heirloom and landrace tomato (Solanum lycopersicum L.) varieties from the germplasm collection of The Ohio State University Tomato Genetics and Breeding Program for their susceptibility or tolerance to water deficit stress conditions. Plants with 3-5 expanded leaves were transferred to 3.7-l plastic containers filled with PRO-MIX (Premier Horticulture, Quakerstown, PA, United States), and spaced 30 cm apart on a greenhouse bench. Growing conditions were as follows: 27°C Day/25°C night; 16/8-h day/night photoperiod; 260 μmol average daily light integral (DLI); 51% average relative humidity; and bi-weekly fertigation with 20N-20P2O5-20K2O (J.R. Peters Inc., Allentown, PA, United States) at a concentration of 1,000 mgL–1 twice per week. When the plants reached the growth stage of 6–8 expanded leaves, irrigation was withheld, and plants were evaluated for 6 days. The leaf turgor status of individuals under water deficit stress conditions was assessed daily on a whole plant basis. Pots were weighed at saturation and over the course of the germplasm screen (72 and 144 h after irrigation was halted). Turgor ratings ranged from 1 to 5 (5 = turgid, 4 = soft to the touch, 3 = beginning to wilt, 2 = wilted with complete loss of turgor, and 1 = dead) consistent with previous studies (Waterland et al., 2010). Leaf surface temperatures (°C) of two fully expanded leaves per plant were monitored daily using an infrared thermometer (Zhuhai JiDa Huapu Instrument Hong Kong, SAR China). The experiment was conducted as an augmented design (Federer and Raghavarao, 1975) with two replicates of each variety and 30 replicates of processing tomato variety OH824 (Berry et al., 1991) as an over-replicated, relatively moisture stress-tolerant control.
Evaluation of Selected Trichoderma Isolates for Growth Promotion and Water Deficit Stress Tolerance in Tomato Genotype ‘Roma Organic’
Plant Material
The tomato genotype ‘Roma Organic’ (W. Atlee Burpee & Co, Warminster, PA, United States), reported as drought susceptible and able to support Trichoderma colonization (Vinale et al., 2013; Aghaie et al., 2018), was selected for this experiment. Tomato seeds were surface sterilized by dipping in 10% sodium hypochlorite, followed by 95% ethanol for five minutes. Seeds were then rinsed (5 X) successively in sterile distilled water. The seeds were sown in 98-cell plastic plug trays containing an in-house soil mix. Growing substrate for seed germination, and water deficit stress experiments was prepared using a mix of composted sandy soil and peat (Fafard Sphagnum Peat Moss, Sun Gro Horticulture Products, Agawam, MA, United States) (6:1) plus 1.8 g liter–1 lime and 0.45 g liter–1 fertilizer 20N-20P2O5-20K2O (Jack’s professional All- Purpose Fertilizer, JR Peters INC., Allentown, PA, United States), steamed sterilized for 12 h at 18 psi and 121°C. The growing substrate tested negative for the presence of Trichoderma through dilution plating on Trichoderma selective media. For germination and initial seedling growth, trays were kept in a greenhouse at 25°C with 16/8-h day/night photoperiod and 65% relative humidity for two weeks.
Inoculum Preparation of Fungal Isolates and Treatment Designation
Eleven novel isolates of Trichoderma (NT3, NT14, NT16, NT21, NT22, NT31, NT33, NT37, OT40, OT41, NT43), belonging to T. asperellum, T. asperelloides, T. hamatum, or T. ghanense, (Figure 2) were selected for a greenhouse screening based on differing ability to proliferate under reduced matric water potentials (i.e., low- and high-performing isolates) and to represent environmental diversity in isolate origin. These 11 isolates and the commercial Trichoderma T22 (Positive control) were cultured on Potato Dextrose Agar (PDA) medium (Sigma- Aldrich Inc., St. Louis, MO, United States) at 25°C for 14 days with 12 h light/dark cycle. Conidia were recovered by flooding each plate with sterile distilled water and gently scraping the colony surface with a sterile toothpick. The Trichoderma suspensions were collected on a sterile 15 ml tube and vortexed briefly. The hyphae and mycelia were removed by passing the suspensions through four layers of sterilized cheesecloth (Al-Hazmi and TariqJaveed, 2016). The concentration of Trichoderma conidia was determined using a hemocytometer. The suspension was adjusted to 1 × 108 conidia per ml by adding sterile distilled water.
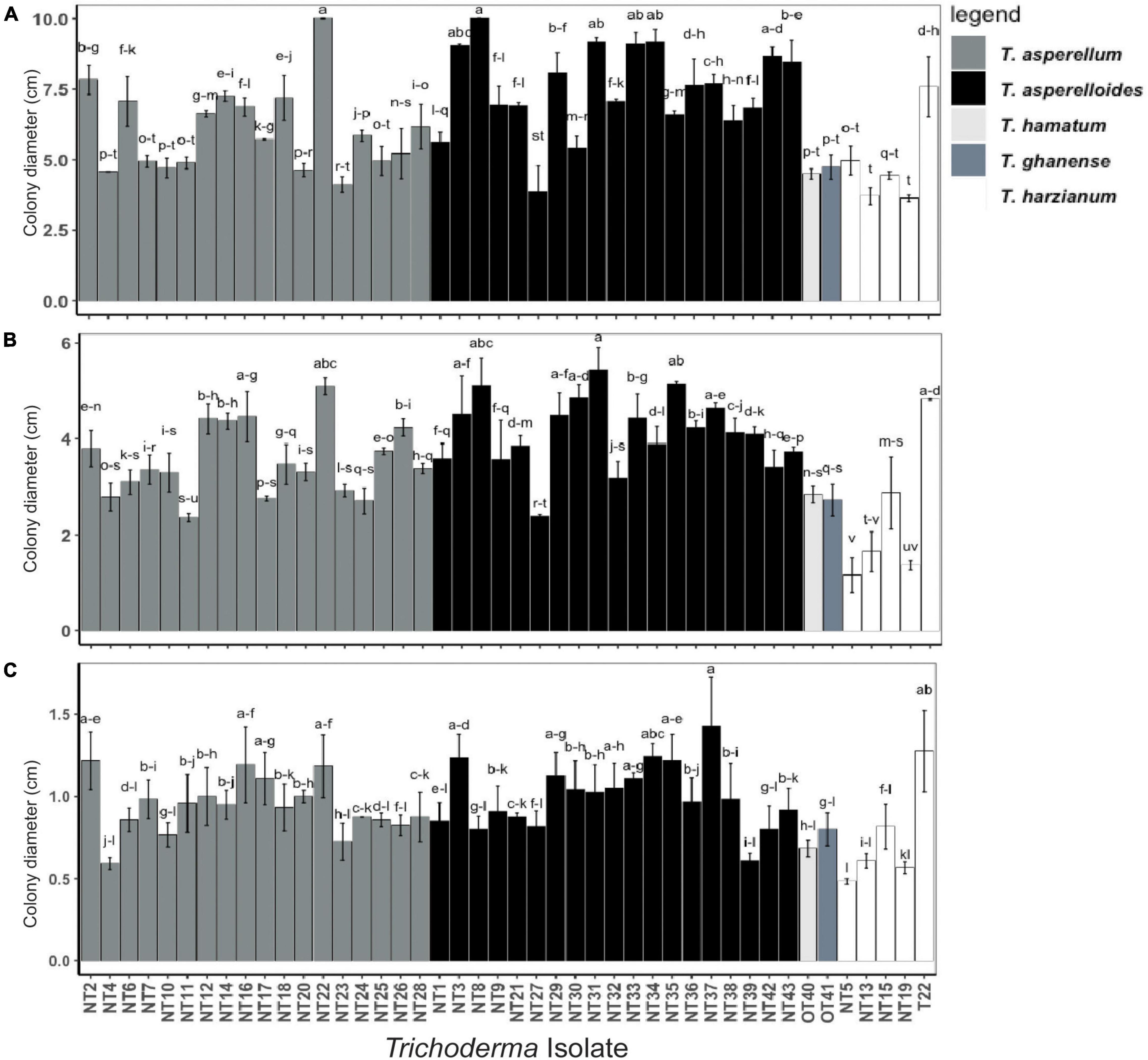
Figure 2. Radial mycelial growth of Trichoderma isolates grown in media at different matric water potentials: (A) –2.8 Ψ, (B) –4.8 Ψ, and (C) –8.5 Ψ; the matric potentials were generated by adding 400, 600, and 800-g PEG into 1-l PDA respectively. Radial growth of the Trichoderma colony (cm) was measured along the plate diameter after 7 days. Values represent the mean growth ± SE of isolates from two trials each performed in triplicate. Isolates with different superscripts are significantly different according to Fisher LSD test (P < 0.05). Bar colors represent the species of tested isolates.
Seedlings were transplanted into 14 cm plastic pots containing steam-sterilized soil substrate. The plants were inoculated with Trichoderma isolates twice during the experiment. First, at seedling transplanting, plants were inoculated by soaking the roots of each 2-week-old seedling in the conidia suspension (1 × 108 conidia per plant) for 1 h prior to transplant, according to Alwhibi et al. (2017). A second Trichoderma inoculation was performed two weeks after transplanting, with a 1 ml suspension of 1 × 108 conidia applied with a pipette to the soil, close to the base of the plant. Non-inoculated control plants (T0) were treated similarly, but with sterile distilled water. The greenhouse conditions were set as follows: 27°C Day/25°C night; 16/8-h day/night photoperiod; 260 μmol average daily light integral (DLI); and 65% average relative humidity. The seedlings were fertilized with 20N-20P2O5- 20K2O (Jack’s professional All- Purpose Fertilizer, JR Peters INC., Allentown, PA, United States) at a concentration of 2 g liter–1 fertilizer once per week.
In the greenhouse the experiment was arranged as a randomized factorial block design with two treatments. The treatment levels were as follows:
Irrigation treatment — continuously irrigated or subjected to water deficit stress.
Trichoderma inoculation — T0, no inoculation, NT3, NT14, NT16, NT21, NT22, NT31, NT33, NT37, OT40, OT41, NT43, T22.
The 26 treatment combinations (i.e., irrigation regimen X Trichoderma inoculation status) were represented by a single plant (and one plant per pot) in each of six blocks for a total of 156 plants. The experiment was repeated two times.
Induction of Water Deficit Stress
All plants were irrigated daily with 500 ml of water per pot prior to inducing water deficit stress. Three weeks after transplanting, replicates representing each inoculation treatment (i.e., inoculated and control plants) were randomly assigned to irrigation treatment groups with six replicates subjected to artificial water deficit stress and six irrigated daily, serving as water deficit stress controls. Water deficit stress was created by withholding irrigation for 5 days. After 5 days, the plants were again irrigated with 500 ml of water in each pot once a day for the next 7 days for recovery.
The soil water potential was measured and recorded through the duration of the experiment (transplanting to sampling) using the Soil Moisture Smart Sensor (S- SMx-M005, Onset Computer Corporation, Bourne, MA, United States) and the HOBO USB Micro Station Data Logger (Onset Computer Corporation, Bourne, MA, United States). Twelve moisture sensors were inserted in the pots with four different treatments (non-inoculated control + water-stressed, commercial Trichoderma-T22 + water-stressed, non-inoculated control + irrigated, commercial Trichoderma-T22 + irrigated) in three replications and the water content in the pots was recorded before water deficit stress, during water deficit stress, and after recovery. After one week of re-watering and recovery, individual plants from both irrigation treatment groups were harvested for shoot and root weight measurements. For this, shoots were cut at the base of growth, and the root balls were excavated from the pots and intact and broken roots were carefully collected. The roots were cleaned thoroughly to remove any attached growing substrate prior to weighing.
Evaluation of Selected Trichoderma Isolates on Tomato Genotypes Differing in Water Stress Tolerance
Plant Material and Treatment Designation
Tomato varieties ‘Punta Banda’ (water deficit tolerant; Native Seeds/SEARCH, Tucson, AZ, United States), ‘Roma Organic’ (water deficit susceptible), and ‘Jaune Flamme’ (water deficit susceptible, OSU Tomato Genetics and Breeding Program) were selected for this experiment. ‘Punta Banda’ and ‘Roma Organic’ were chosen based on tolerance ratings reported by Aghaie et al. (2018) and the water deficit susceptibility of ‘Jaune Flamme’ was determined as part of this research.
The three Nepalese Trichoderma isolates (NT3, NT16, NT33) and the T22 (positive control) were selected based on variability in performance during the greenhouse screening of Trichoderma isolates in ‘Roma Organic’ tomato genotype (see section “Evaluation of Selected Trichoderma Isolates for Growth Promotion and Water Deficit Stress Tolerance in Tomato Genotype ‘Roma Organic”’). This experiment was conducted using a randomized factorial block design with three treatment factors and nine replications per treatment combination.
The treatments and levels were as follows:
Irrigation treatment — continuously irrigated or subjected to water deficit stress.
Trichoderma inoculation — no inoculation (T0), NT3, NT16, NT33, T22.
Tomato genotype — ‘Jaune Flamme,’ ‘Roma Organic,’ ‘Punta Banda.’
The 30 treatment combinations (i.e., irrigation regimen X Trichoderma inoculation status X three genotypes) were represented by a single plant (one plant per pot) in each of the nine blocks for a total of 270 plants. The experiment was repeated two times.
Trichoderma inoculation procedures, assessment of plants to irrigation treatment groups and water deficit regimens followed those described under the experiment 1. After one week of re-watering and recovery, all plants from all treatment groups were harvested for the following growth measurements: total number of leaves per plant, shoot fresh weight, and root fresh weight.
Plant Physiological Analysis
Plant physiological parameters including chlorophyll content, relative water content, photosynthesis, and stomatal conductance were measured in leaves at different stages of the experiment. Chlorophyll content in leaves was estimated with a Soil Plant Analysis Development meter (SPAD 502 Plus, Konica Minolta, Inc., Ramsey, NJ, United States). The SPAD meter records the absorbance at near red light (650 nm) and at infrared (940 nm) regions of the leaves. Based on these two absorbance, the instrument calculates a numerical soil plant analysis development (SPAD) value which is proportional to the chlorophyll content in the leaf (Uddling et al., 2007). SPAD readings were recorded between 9.00 and 11.00 a.m. from the upper three leaves after two days of recovery. The average of the three leaves per plant was used in the analysis.
To measure the leaf relative water content (RWC), a cork borer was used to remove five 5 mm disks from the upper third leaf on the 5th day of water deficit stress. The fresh weight (FW) of the sampled disks was recorded. Afterward, the disks were soaked in distilled water for 4 h at room temperature to achieve maximum turgidity and turgid weight was recorded (TW). Finally, the dry weight (DW) of the disks was recorded after 24 h of oven-drying at 80°C. RWC was calculated by using the following formula: RWC (%) = (FW-DW)/(TW-DW) × 100.
Photosynthesis and stomatal conductance were measured to evaluate the physiological health of water deficit stressed plants and influence of Trichoderma inoculation. The portable photosynthetic system Li- 6400X (LI-COR Inc, Lincoln, NE, United States) was used to measure these physiological parameters. For this, the fully expanded third leaf from the top of a tomato plant was selected and the measurements were recorded between 9.00 am and 1:00 p.m. at the 4th day of water deficit stress. For physiological parameters, the measurements were taken from three replications per treatment combination.
Statistical Analysis
The difference between the treatments were evaluated using the linear mix model function “lmer” using the package lme4 (Bates et al., 2014) in R (R Core Team, 2013; Mendiburu, 2015), where experimental run was treated as a random factor in in vitro experiment. The normality of the data was assessed using the Shapiro–Wilk test followed by the Bartlett test to check the homogeneity of variance using the functions shapiro.test and bartlett.test in R (Kassambara, 2020). Spearman (rank) correlations were used to compare the growth of isolates at different matric water potentials and these relationships were graphed using the ggpubr package (0.4.0) (Kassambara, 2020). To evaluate water deficit tolerance of tomato varieties, best linear unbiased predictors (BLUPs) were extracted from heirloom and landrace tomato raw water deficit stress data using the package lme4 (Bates et al., 2014) to adjust mean values according to greenhouse spatial differences observed in the performance of the replicated control. BLUP-adjusted means were used to create a vector for maximum leaf temperature and maximum wilt phenotypes. A linear mixed model was fitted to the data to determine the effects of Trichoderma isolate and irrigation main effects and their interactions on the response variables in greenhouse screening in tomato genotype ‘Roma Organic.’ Similarly, a linear mixed model was fitted to the data to determine the main effects and their interactions for Trichoderma isolates, tomato genotypes, and irrigation regimens on the response variables in the last greenhouse experiment. Experimental run was considered a random-effect factor and other factors were considered fixed effects. Type III F tests were used for determining significance of effects. Interaction means were partitioned with slices of means to determine the effects of isolate and genotype at each of the irrigation treatments (Schabenberger et al., 2000; Stroup et al., 2018). With slices, an overall F test was performed for each irrigation treatment, and means were compared in a pairwise fashion at each level of irrigation treatment. The GLIMMIX procedure of SAS was used for the analysis.
Results
Desiccation Tolerance of Trichoderma Isolates
In the in vitro screening procedure, all Trichoderma isolates tested on PDA medium without PEG (negative control, 0.0Ψ) were viable and covered the 10 cm Petri plate’s surface within 7 days after inoculation. However, the colony diameter (mycelial growth) of all Trichoderma isolates tested decreased with increasing negative matric water potential as evidenced by the means and standard deviations of the isolate population cultured at –2.8 Ψ (6.47 ± 1.78 cm, Figure 2A), –4.8 Ψ (3.63 ± 1.01 cm, Figure 2B), and –8.5 Ψ (0.93 ± 0.21 cm, Figure 2C). Individuals within the population of isolates exhibited continuous variability in their ability to proliferate at each osmotic stress-imposed level. Ranked isolate colony diameters were significantly correlated among treatment groups [rho (ρ) = 0.72 and 0.60 for treatments –2.8 vs. –4.8 and vs. –8.5 Ψ, respectively and rho (ρ) = 0.64 for treatments –4.8 vs. –8.5 Ψ] indicating a degree of homogeneity in individual responses to increased osmotic stress (Supplementary Figures 2A–C). Isolates NT3, NT16, NT22, NT29, NT31, NT33, NT37, and T22 exhibited colony diameters that ranked within the upper one-third (i.e., rank performance > 15) of the population at each level of osmotic stress. Similarly, Nepalese isolates NT4, NT5, NT10, NT13, NT15, NT19, NT23, and NT27 and the Ohio isolates OT40 and OT41 grew less under all levels of osmotic stress, ranking within the lower one-third (i.e., rank performance ≤ 30) of the population at each level. Different patterns of growth under osmotic stress were observed. For instance, NT8 ranked 2nd and 3rd among the isolates for its ability to proliferate at –2.8 and –4.8 Ψ, respectively. However, its growth appeared to be constrained at the highest level of osmotic stress (i.e., 8.5 Ψ), suggesting limits to its adaptive response. Conversely, NT16 grew modestly at –2.8 Ψ but it successively gained in rank performance compared to other isolates when challenged at –4.8 and –8.5 Ψ. Individual isolate growth (value ± standard error and rank) measurements at each osmotic stress level are displayed in Supplementary Table 2.
Water Deficit Tolerance/Susceptibility Among Tomato Heirloom Varieties and Landraces
Continuous variation in BLUP-adjusted mean leaf temperatures was observed among the 70 genotypes subjected to 6 days of water deficit stress (Supplementary Figure 3A) ranging from –0.9 (water deficit stress-tolerant) to 0.6 (water deficit stress-susceptible). The ability to maintain leaf turgor pressure during water deficit also differed among genotypes with BLUP-adjusted means ranging from –1.0 (water deficit stress-susceptible) to 0.9 (water deficit stress-tolerant) (Supplementary Figure 3B). No significant differences in substrate water loss were detected due to genetic effects (genotype) or environmental effects (row and column). Leaf temperature and turgor maintenance data were complementary [rho (ρ) = –0.73]. OH8245 ranked 9th in ability to maintain leaf temperature and 14th in the ability to maintain leaf turgor confirming its designation as moderately water deficit stress tolerant.
Among the water deficit stress-susceptible genotypes, the heirloom variety ‘Jaune Flamme’ was selected for inclusion in experiments examining differences in tomato cultivar response to Trichoderma inoculation described below. This orange-fruited variety is used regionally by farm market growers, has been studied as a source of β-carotene (provitamin A) in fruit (Orchard et al., 2021) and we were familiar with its horticultural properties. In the water deficit tolerance/susceptibility screen reported here, ‘Jaune Flamme’ ranked 57th in its ability to maintain leaf temperature and 60th in its ability to maintain leaf turgor under water deficit out of 70 genotypes tested (Supplementary Figures 3A,B).
Effect of Trichoderma Isolates on the Water Deficit Stress Response of ‘Roma Organic’ Tomato
The water deficit stress regimen imposed was effective at reducing water availability to the treated plants (Supplementary Figure 4). Irrigation significantly affected the performance of tomato genotype ‘Roma Organic’ (Supplementary Table 3) with the aboveground and belowground biomass of continuously irrigated plants (43.08 ± 1.18; 12.54 ± 3.83, respectively) higher than biomass of those under the water deficit stress regimen (26.85 ± 1.17; 9.62 ± 3.83, respectively).
Shoot and root weights were significantly affected by Trichoderma inoculation (Figures 3A,B and Supplementary Table 3). However, as indicated by parsed analysis of the isolate X irrigation interaction (i.e., the “slices” evaluating isolate differences at each level of irrigation), the significance of Trichoderma inoculation on shoot biomass was evinced primarily among plants under the water deficit stress regimen (p < 0.05; Figure 3A) whereas the effects of inoculation on shoot biomass in continuously irrigated plants were insignificant (p = 0.14; Figure 3B). Under water deficit stress conditions, the plants inoculated with NT3, NT16, NT21, NT31, and NT33 accumulated significantly greater aboveground biomass (31–44% higher shoot weight) as compared to the non-inoculated control (T0) (Figure 3A). The fresh shoot weight of plants inoculated with commercial Trichoderma T22 was not statistically different from that of the non-inoculated control (T0) plants. Plants inoculated with NT14, NT16, and NT33 accumulated significantly greater root biomass (20–25% higher root weight) compared to non-inoculated control (T0) and commercial Trichoderma T22-inoculated plants (Figure 4A). Under irrigation, inoculation with most of the selected Trichoderma isolates did not significantly affect the mean shoot weight and root weight of tomato plants compared with those of the non-inoculated control (Figures 3B, 4B and Supplementary Table 3).
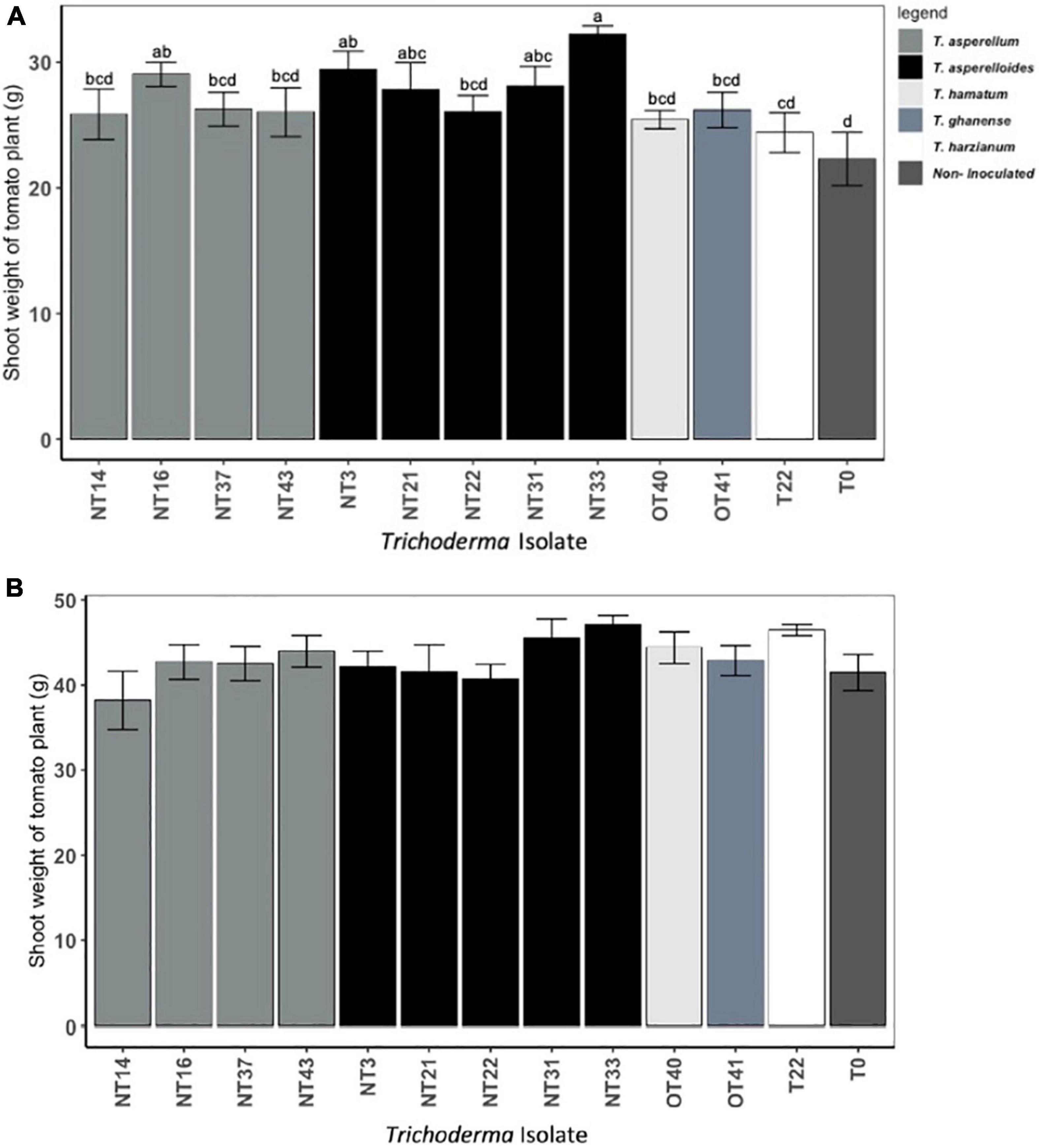
Figure 3. Effect of Trichoderma isolates on shoot weight of Roma tomato subjected to: (A) water deficit stress, (B) continuous irrigated conditions. The water deficit stress was imposed by withholding the irrigation for 5 days. Thereafter, the stressed plants were irrigated again for a 7-day recovery period prior to harvest. The shoot fresh weights per plant were recorded. Values are means ± SE of three trials each with four replicates. Isolates with different superscripts are significantly different according to Fisher LSD test (P < 0.05). Bar colors represent the species of tested isolates.
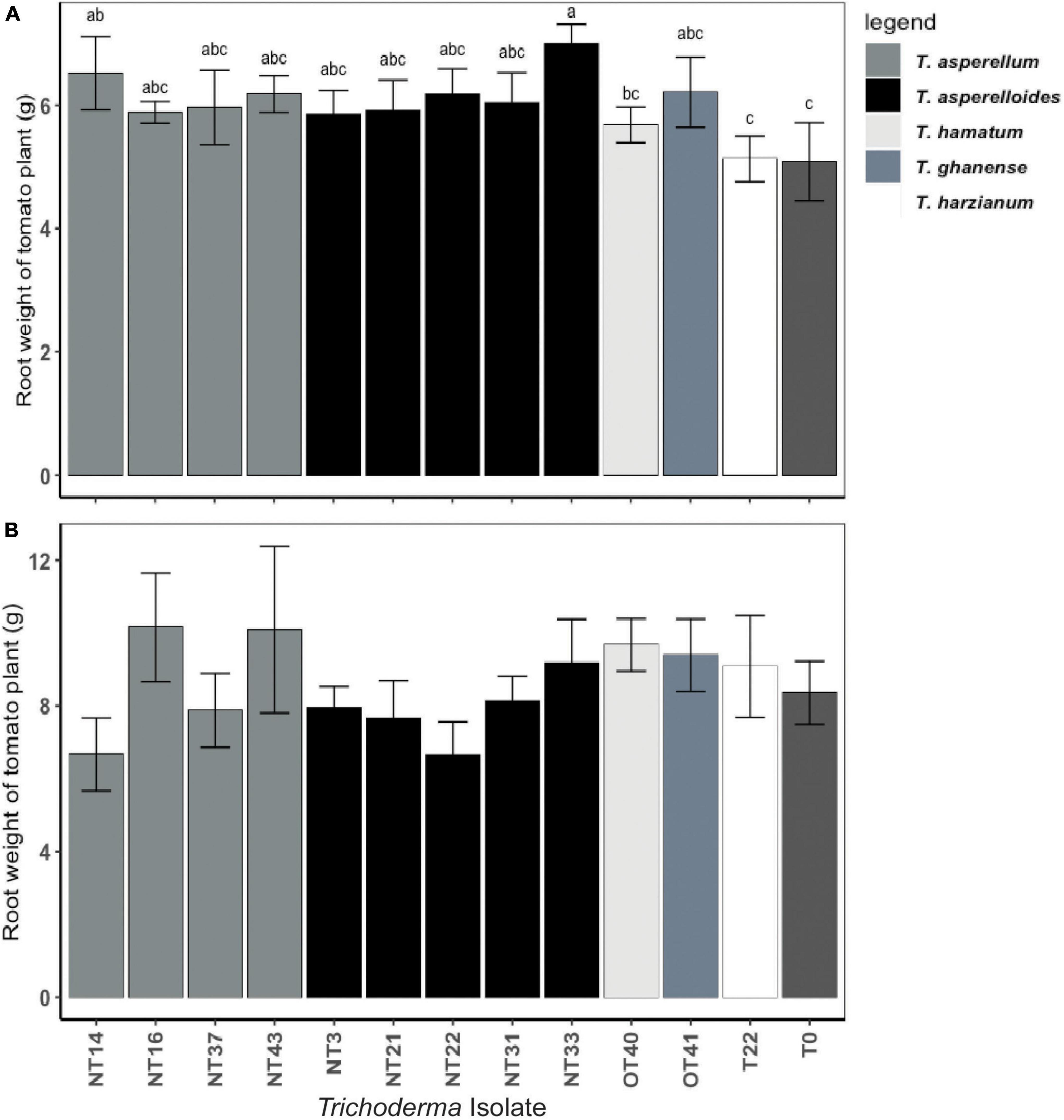
Figure 4. Effect of Trichoderma isolates on root weight of Roma tomato subjected to: (A) water deficit stress, (B) continuous irrigated conditions. Plants were harvested simultaneously with their counterparts subjected to a water deficit stress regimen 32 days after transplanting. The root fresh weights per plant were recorded. Values are means ± SE of three trials each with four replicates. Isolates with different superscripts are significantly different according to Fisher LSD test (P < 0.05). Bar colors represent the species of tested isolates.
Effect of Selected Trichoderma Isolates on the Growth Parameters of Tomato Genotypes Differing in Water Deficit Stress Tolerance
The water deficit regimen curtailed the accumulation of above-ground biomass in water-stressed plants of all three genotypes compared to their continuously irrigated counterparts (Tables 1, 2 and Supplementary Table 4). While evaluating the effects of Trichoderma isolates and genotypes at each level of the irrigation treatments (water deficit stress and irrigation conditions), the significance of Trichoderma inoculation on growth parameters were evinced in three different genotypes of tomato (p < 0.001, Supplementary Table 4).
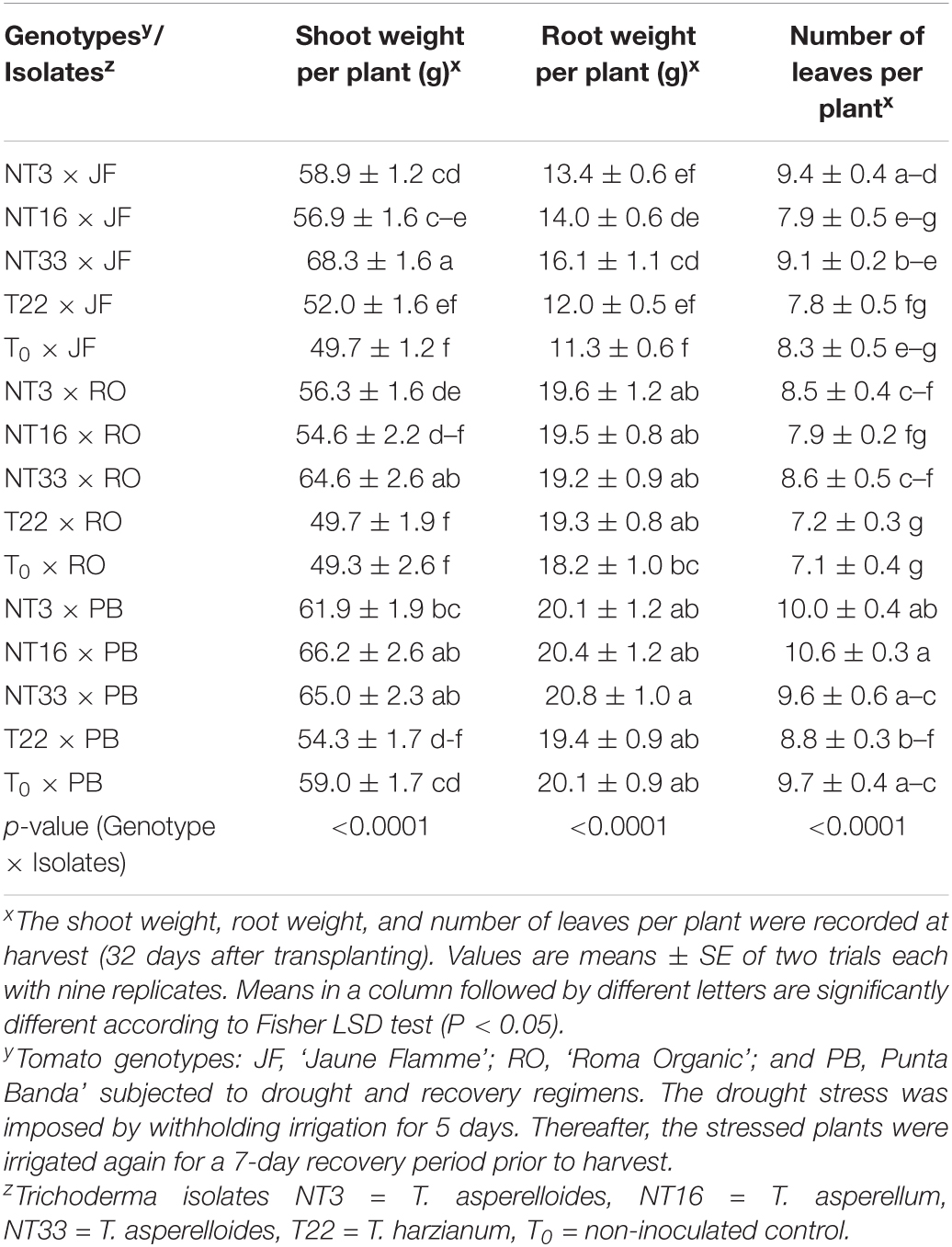
Table 1. Effect of four Trichoderma isolates on fresh shoot weight, fresh root weight, and number of leaves on three tomato genotypes under water deficit stress conditionsx.
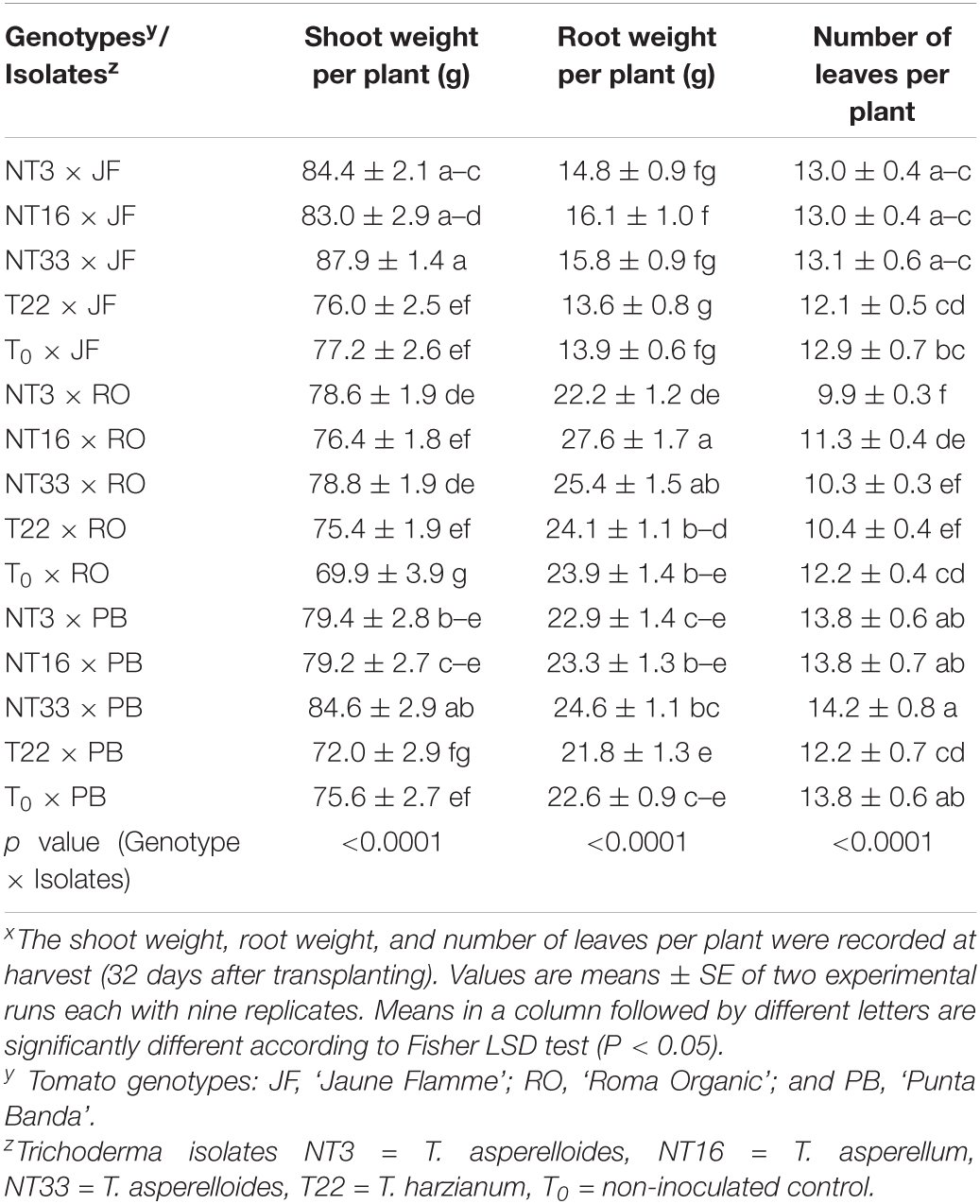
Table 2. Effect of four Trichoderma isolates on fresh shoot weight, fresh root weight, and number of leaves on three tomato genotypes under irrigated conditionsx.
Under water deficit stress conditions, ‘Jaune Flamme’ plants inoculated with NT3 and NT33 had significantly higher shoot weight (about 18 and 37%, respectively) than non-inoculated control (T0 × JF) and commercial Trichoderma inoculated plants (T22 × JF) (Table 1 and Figure 5). ‘Jaune Flamme’ plants inoculated with NT16 had significantly higher shoot weight (14%) compared to non-inoculated control plants (T0 × JF). In ‘Roma Organic,’ plants application of Trichoderma isolates NT3 and NT33 significantly increased the shoot biomass (14 and 30% respectively) as compared to non-inoculated control (T0 × RO) and T22 inoculated tomato plants (T22 × RO) (Table 1). Similarly, inoculation of ‘Punta Banda’ plants with NT16 and NT33 resulted in significantly greater shoot weight (12%) compared to non-inoculated control plants (T0 × PB) (Table 1). Only ‘Jaune Flamme’ plants inoculated with NT33 had significantly higher root weight (34–48%) compared to commercial Trichoderma T22-inoculated (T22 × JF) and non-inoculated control plants (T0 × JF) under water deficit stress conditions (Table 1). ‘Jaune Flamme’ plants inoculated with NT3 maintained significantly higher leaf numbers (13%) than non-inoculated controls (T0 × JF) (Table 1). In ‘Roma Organic,’ plants inoculated with NT3 and NT33 maintained significantly higher leaf numbers (20%) than non-inoculated controls (T0 × RO) or commercial Trichoderma inoculated plants (T22 × RO) (Table 1). However, Trichoderma inoculation had no effect on root weight and number of leaves per plant in ‘Punta Banda’ compared to the non-inoculated control (T0 × PB) plants under water deficit stress conditions (Table 1).
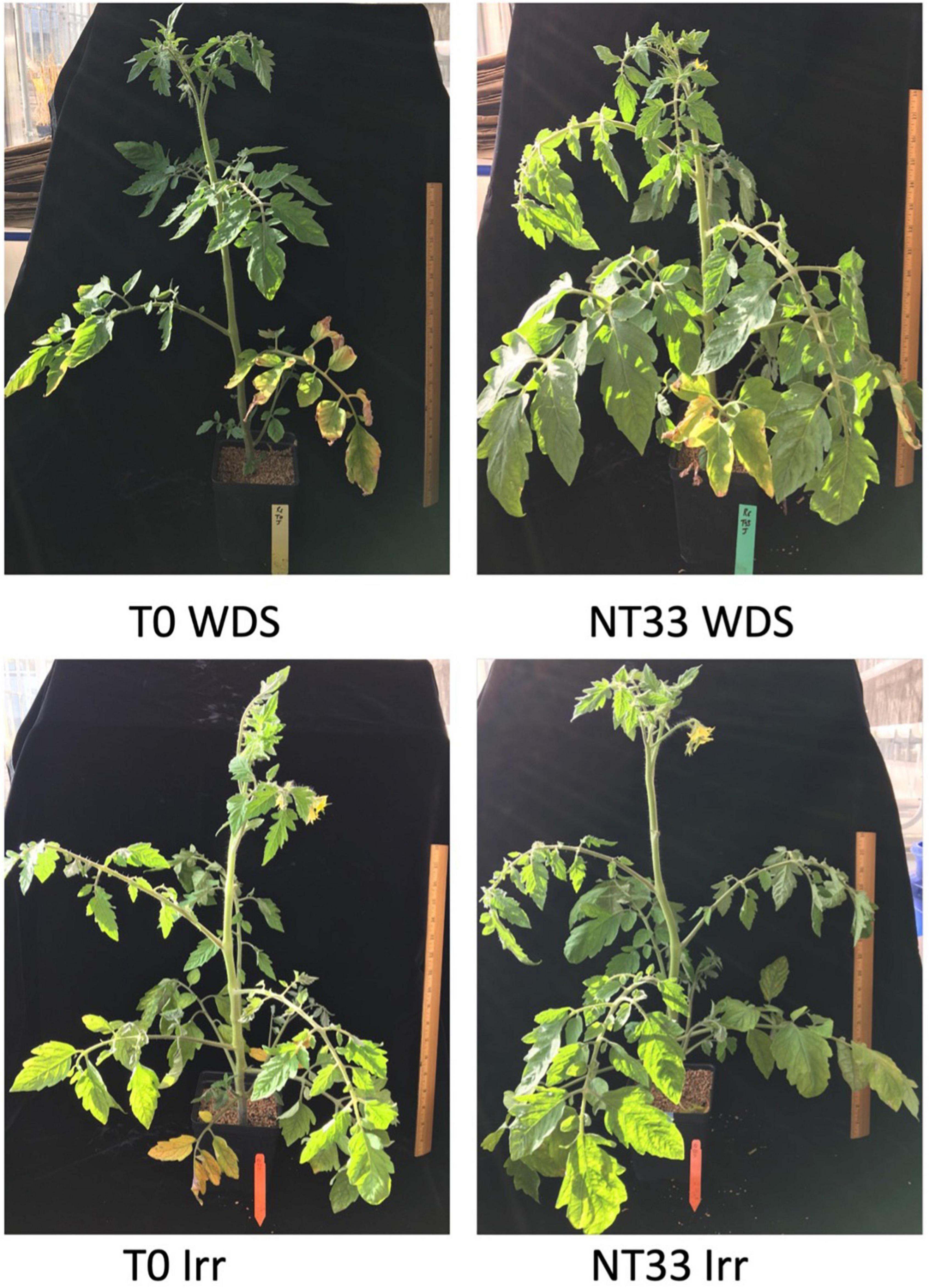
Figure 5. Example ‘Jaune Flamme’ tomato plants grown under different Trichoderma inoculation and water deficit stress treatments. NT33, T. asperelloides isolate. T0WDS, non-inoculated water stressed plants; NT33WDS, NT33 inoculated water stressed plants; T0 Irr, non-inoculated irrigated plants; NT33 Irr, NT33 inoculated irrigated plants. Images were obtained after recovery from water deficit stress.
Under irrigated conditions, inoculation with the Trichoderma isolates NT16, NT3, and NT33 resulted in higher shoot biomass, 9–13% in ‘Jaune Flamme’ and 9 to 12% in ‘Roma Organic’ plants compared to their non-inoculated control plants (T0 × JF and T0 × RO, respectively) (Table 2). Similarly, in ‘Punta Banda,’ Trichoderma isolate NT33 significantly increased the shoot weight by 12% as compared to the non-inoculated control plants (T0 × PB). ‘Roma Organic’ plants inoculated with NT16 had significantly higher root biomass than non-inoculated control plants (T0 × PB) but the root weights of Trichoderma inoculated plants were not significantly different from the non-inoculated control in other tomato genotypes (Table 2). Trichoderma inoculation of ‘Jaune Flamme’ plants had no effect on the number of leaves per plant, but T22-inoculated ‘Punta Banda’ and ‘Roma Organic’ plants inoculated with T22, NT3, or NT33 produced approximately 1-2 fewer leaves in comparison to non-inoculated controls (Table 2 and Supplementary Table 5).
Plant Physiological Responses to Water Deficit Stress and Trichoderma Inoculation
Water deficit stress significantly reduced the relative water content percentage (RWC) in the tomato leaves (p < 0.001; Tables 3, 4 and Supplementary Table 5). Application of NT33 resulted in significantly higher RWC (16%) in the genotype ‘Jaune Flamme’ compared to non-inoculated controls (T0 × JF) and T. harzianum T22 inoculated tomato plants (T22 × JF), whereas in ‘Roma Organic’ plants there was 36% more RWC in NT3 inoculated plants compared to non-inoculated controls (T0 × RO) and T22 inoculated tomato (T22 × RO) plants (Table 3). Similarly, the NT33 application significantly increased the chlorophyll content in ‘Jaune Flamme’ and ‘Roma Organic’ plants by (11 and 12%, respectively) compared to non-inoculated control plants (Table 3). In addition, ‘Jaune Flamme’ plants inoculated with NT3 and T22, and ‘Roma Organic’ plants inoculated with NT3 and NT16 had significantly higher chlorophyll content (4.4 and 4.5%, respectively) compared to non-inoculated control plants (T0 × JF and T0 × RO) (Table 3). However, Trichoderma treatment had no significant effect on RWC content and chlorophyll content in ‘Punta Banda’ genotype compared to non-inoculated control under water deficit stress conditions.
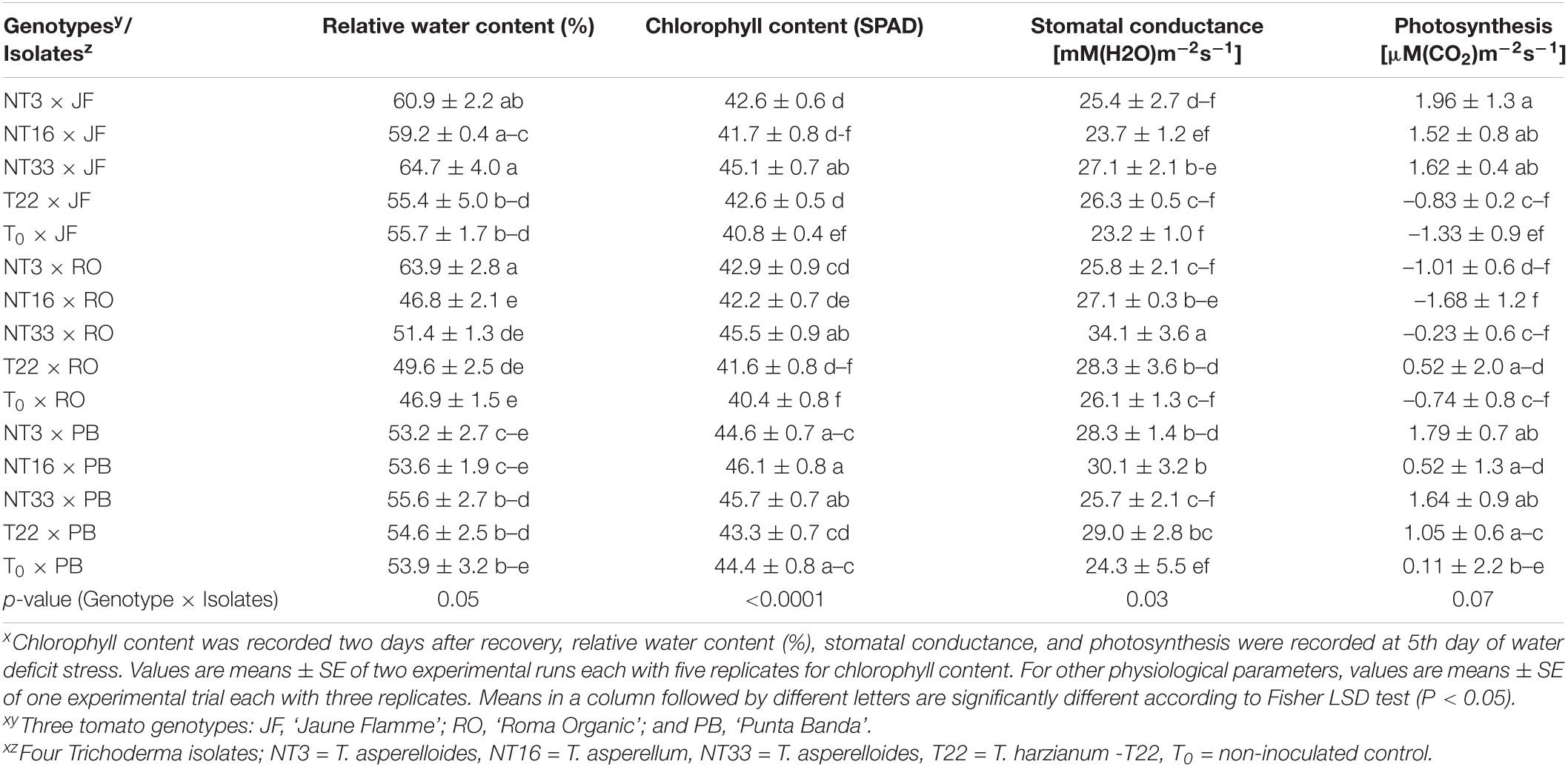
Table 3. Effect of four Trichoderma isolates on chlorophyll content, relative water content, stomatal conductance and net photosynthesis on three tomato genotypes under water deficit stress conditionsx.
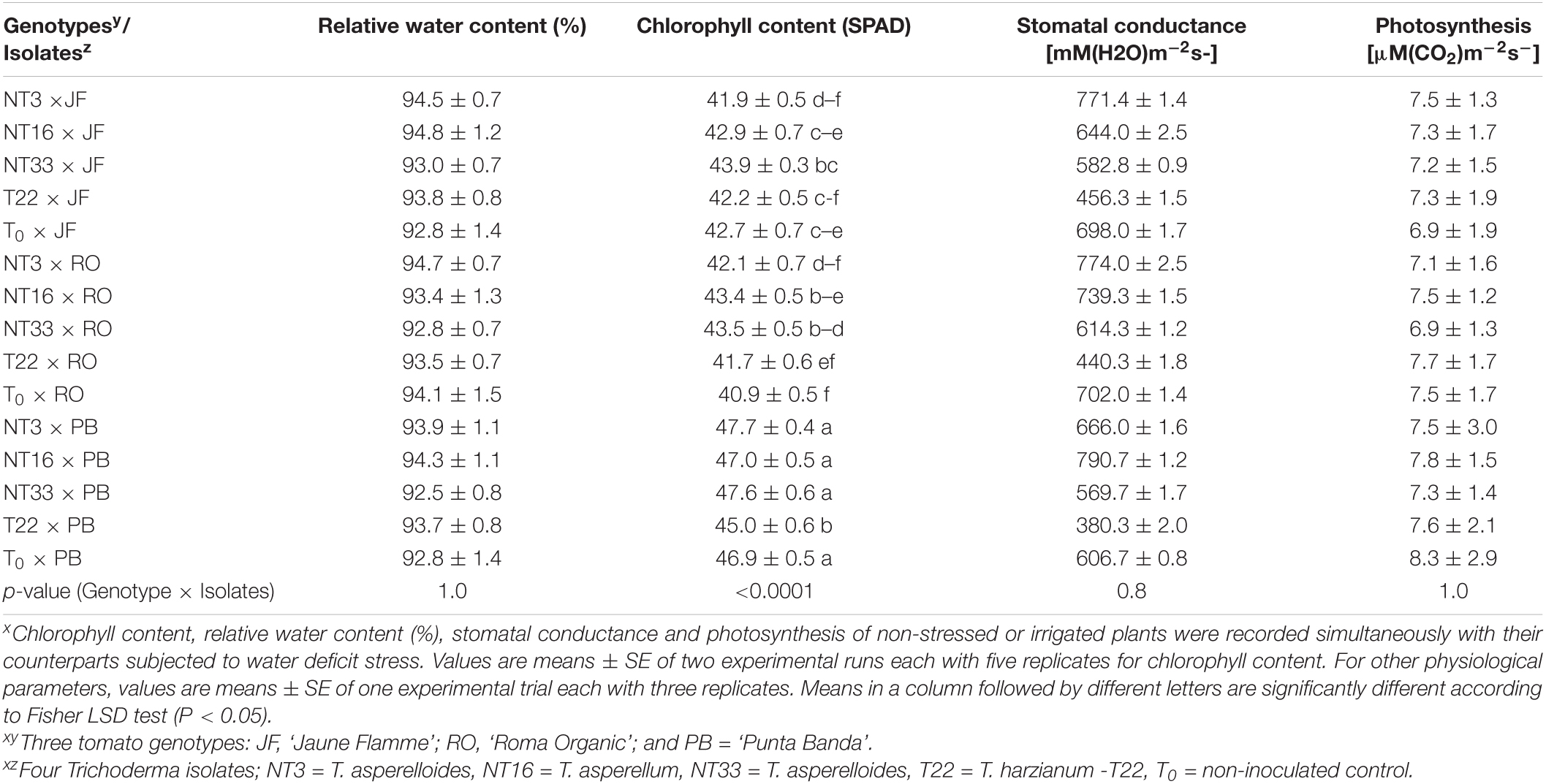
Table 4. Effect of four Trichoderma isolates on chlorophyll content, relative water content, stomatal conductance, and net photosynthesis on three tomato genotypes under irrigated conditionsx.
Physiological parameters such as photosynthetic rate and stomatal conductance were significantly reduced under water deficit stress condition compared to irrigated conditions (p < 0.001; Tables 3, 4 and Supplementary Table 6). Plants inoculated with Trichoderma NT33 had significantly higher stomatal conductance in ‘Jaune Flamme’ and ‘Roma Organic’ plants by 18.8 and 39%, respectively compared to non-inoculated control plants under water deficit stress conditions. ‘Punta Banda’ plants inoculated with NT3 and NT16 had significantly higher (16–23%) stomatal conductance compared to non-inoculated control (T0 × PB). Similarly, Nepalese Trichoderma inoculation resulted in higher photosynthetic rate in ‘Jaune Flamme’ plants than non-inoculated control and T22 inoculated plants (Table 3). However, the effect of Trichoderma application on the photosynthetic health of the other two genotypes were not significantly different from non-inoculated controls under water deficit stress conditions. Under irrigated conditions, the inoculation of ‘Jaune Flamme,’ ‘Roma Organic,’ and ‘Punta Banda’ plants with Trichoderma isolates NT3, NT16, and NT33 did not significantly change the measured physiological parameters when compared to T22 inoculated or the non-inoculated control plants (Table 4 and Supplementary Table 6).
Discussion
The application of biological agents like water deficit-tolerant Trichoderma could be a low-cost, environmentally friendly, and sustainable near-term solution to mitigate drought stress (Shukla et al., 2012; Brotman et al., 2013). However, specific fungal isolate-plant cultivar relationships are complex, and the mechanisms of drought tolerance induced in the plant by Trichoderma colonization are not well-understood. Herein, we report the evaluation of novel Trichoderma isolates collected from different agroecological regions of Nepal for their ability to proliferate under osmotic stress and then to mitigate stress responses in tomato genotypes during and after exposure to water deficit conditions. We had a unique opportunity to test isolates from highly diverse ecological niches ranging in mean annual precipitation from <200 to 5,000 mm, in elevation above sea level from 58 to 8,848 m, and temperature extremes from –12°C to 43.3°C (Karki et al., 2016; Khadka et al., 2022).
Supplementation of growth medium with PEG is designed to measure the effect of water potential on the growth of fungi (Jin et al., 1991; Aujla and Paulitz, 2017). We screened 44 isolates and identified some isolates which exhibited the ability to survive and sporulate under different osmotic stress conditions (Figures 2A–C and Supplementary Table 2). The potential efficacy of these isolates to mitigate water deficit stress in planta was ascertained in greenhouse experiments, in which tomatoes inoculated with NT16, NT3, NT33 and subjected to water deficit stress amassed significantly greater growth parameters than the non-inoculated water deficit stressed control plants in different tomato genotypes (Figure 3A and Table 1). Similarly, other researchers have successfully employed various in vitro culture techniques to identify isolates of Trichoderma (Shukla et al., 2012; Poosapati et al., 2014; Khoshmanzar et al., 2020) or other soil microbiota (Mhadhbi et al., 2011; Subramanian et al., 2016) that conferred tolerance to abiotic stressors, such as osmotic (salt, drought) or temperature (heat, cold) stress.
Microorganisms collected from environmentally diverse sites (arid, saline, alpine regions) are reservoirs of potential traits that may benefit plant hosts when grown under the abiotic stress conditions from where they were isolated (Subramanian et al., 2016; Giauque et al., 2019). Among the six Trichoderma isolates from Nepal that consistently performed in the top third of the collection in the in vitro assay, NT3 was isolated in Jumla, the driest site sampled (766 mm/year precipitation; Supplementary Tables 1, 2 and Supplementary Figure 1). Isolates NT31, NT33, and NT37 were obtained from Banke, another dry site (1175 mm annual precipitation with 85% of the rainfall accumulated during a three-month monsoon season). Conversely, isolates NT10, NT19, NT23, and NT27 ranking in the lower third of the collection for growth under water deficit conditions were collected at sites receiving 1993–2329 mm annual precipitation, from 160 to 200% greater than that received at Jumla. To our knowledge, site-specific Trichoderma adaptation to stress environments, either as free-living organisms or in mutualistic relationships, has yet to be reported. Adaptation to mutualism as a means of survival under harsh environmental conditions may be predicated upon co-evolution involving heritable genetic variation in both plants and microorganisms that promote colonization as well as abiotic stress responses (Hoeksema, 2010). Kubicek et al. (2019) characterized Trichoderma as an “environmental opportunist and generalist” that can rapidly evolve to thrive in new or stressful environments as a free-living organism or a plant symbiont. The fungi can produce the asexual conidiospores and chlamydospores that can survive under severe environmental conditions. Therefore, it is plausible that specific Trichoderma isolates in this study, most notably NT33, are effective at ameliorating water deficit stress in tomatoes because they evolved in drier regions of Nepal. The evolution of these and other effective isolates may also have been influenced by micro-climatic conditions not examined during the collection process.
Water deficit stress significantly inhibits crop productivity and quality. Trichoderma inoculation could ameliorate the negative effect of water deficit stress and facilitate growth promotion in plants (De Palma et al., 2016; Bashyal et al., 2021). Mastouri et al. (2010, 2012) demonstrated the effects of Trichoderma inoculation on plant performance to be apparent only in stressful environments. In their studies, inoculation of tomato seed with T22 improved germination and other growth parameters when grown at water deficit conditions. Other researchers have uncovered similar trends among treatments and controls, suggesting significant Trichoderma effects under different biotic or abiotic stress conditions (Donoso et al., 2008; Lombardi et al., 2018). Our results support these findings in the first greenhouse experiment where shoot weights of ‘Roma Organic’ tomato genotype inoculated with five Trichoderma isolates belonging to two different species T. asperellum and T. asperelloides were higher than those of controls when subjected to the water deficit regimen (Figure 3A). However, in the Trichoderma × Tomato Genotype experiment, there was a significant effect of Trichoderma inoculation on growth parameters of tomato plants under both irrigated and water deficit stress conditions (Tables 1, 2), supporting Trichoderma’s role as growth promoting agent.
Genotypes of the plant and symbiont are essential factors determining the level of the beneficial effect of Trichoderma inoculation, even for established and commercial isolates of Trichoderma like T22 and the T. virens strain G-41 (Harman et al., 2004; Prashar and Vandenberg, 2017). Trichoderma-cultivar specificity has been studied most extensively upon exposure to biotic stresses, but our study documents this relationship under the abiotic stress condition (water deficit stress) as well. We conducted the greenhouse trial with three genotypes of tomato differing in their drought tolerance capacity. Since plant- microbe interaction is host specific, it is important to identify the isolates that are effective across wide range of crops. In our study, NT33 generally outperformed the other Nepalese Trichoderma isolates and the commercial Trichoderma T22 when inoculated in the different tomato genotypes under both water deficit stress and non-stress conditions. We also found that the overall performance of the selected Trichoderma isolates was better in drought susceptible genotypes ‘Jaune Flamme’ and ‘Roma Organic’ as compared to drought tolerant ‘Punta Banda’ (Tables 1–4). Prashar and Vandenberg (2017) exposed 23 wild and cultivated lentil genotypes colonized with either commercial Trichoderma strain T22 or G41 to the root rot pathogen Aphanomyces euteiches. Responses to the pathogen and overall plant performance were strongly governed by the origin and genetic makeup of the lentil genotype. Similarly, using Trichoderma commercial strains P1 (T. atroviride) and T22 and five tomato genotypes, Tucci et al. (2011) demonstrated significant variability in performance (negative, neutral, positive) among inoculated pairs vs. controls. These authors introduced the feasibility of breeding plant genotypes to be specifically responsive to common commercial Trichoderma isolates.
Water deficit stress stimulates different physiological responses such as stomatal closure, repression of photosynthesis, activation of respiration, and reduction of cell growth in the plants. The plants inoculated with Trichoderma isolates can enhance water-deficit tolerance by improving root development, regulation of drought-induced changes in the stomatal opening, photosynthesis, and chlorophyll content in leaf (Contreras-Cornejo et al., 2009; Brotman et al., 2013; Guler et al., 2016). Our study also found that the net photosynthetic rate and stomatal conductance in the tomato leaves were less affected by water deficit stress in the NT33 inoculated tomato plants compared to non-inoculated control (Table 3). In addition, NT33 and NT3 increased the chlorophyll content in the drought susceptible genotypes as compared to non-inoculated controls, which is an important pigment essential for absorbing light during photosynthetic process (Table 3). Bashyal et al. (2021) reported that genes related to the photosynthesis process, such as those coding for photosystem I subunits, photosystem II core complex proteins, osmotin like proteins, and stomatal development were upregulated in the Trichoderma inoculated plants as compared to non-inoculated controls. Water deficit exposed plants inoculated with Trichoderma exhibit improved physiological health (Shukla et al., 2012; Harman et al., 2019; Khoshmanzar et al., 2020), similar to our observation in NT33-colonized tomato plants. It has been reported that the plants inoculated with Trichoderma species increase the accumulation of different metabolites like phenolics, flavonoids, and proline which increase antioxidant activity, protect photosynthetic pigments, and photosynthetic pathways from photooxidative damage (Mona et al., 2017; Scudeletti et al., 2021; Tripathi et al., 2021). The earliest response of plants to drought is considered to be due to an increase in abscisic acid content and regulates stomatal movements and helps to maintain water balance. Martinez-Medina et al. (2013) reported that Trichoderma harzianum colonized plants displayed higher expression levels of the ABA-responsive marker gene involve in different defense-related pathways compared to non-inoculated control plants.
Relative water content is an important indicator of the physiological water status of the leaf. It indicates the appropriate water status of plant in terms of cellular hydration and is used as a selection criterion for drought tolerance in different crops (Teulat et al., 1997). Under water deficit stress conditions, the RWC% in the plants is significantly reduced; however, the Trichoderma colonization augmented the relative water status in the leaves (Bae et al., 2009; Guler et al., 2016). In our study NT33 and NT3 inoculation increased the relative water content in the leaf of Jaune Flamme’ and ‘Roma Organic’ plants and allowed them to defend against the abiotic stress (Table 3). Trichoderma spp. can improve the drought avoidance mechanism in plants through morphological adaptation by increasing root growth and also changing root architecture in many crops, thereby increasing the plant yield under the water deficit stress condition (Contreras-Cornejo et al., 2009; Meng et al., 2019). Some Trichoderma species secrete auxin (indole acetic acid) and other indole derivatives like nitrilase, that induce the lateral root growth and increase the root surface area (Hermosa et al., 2012). In this study we found that the ‘Jaune Flamme’ plants inoculated with NT33 had higher root biomass compared to T0 (Table 1) under water deficit stress condition and also increases the lateral root number (Rawal, 2021) that could have increased the water status in the plant.
The salient results of this study are as follows: (1) We demonstrated the ability of Trichoderma isolates to reduce water deficit stress in tomato, which may provide a grower-friendly strategy for crop improvement and crop protection. (2) We identified superior strains from among a novel collection of 41 Trichoderma isolates collected from diverse ecological niches in Nepal with the ability to thrive under osmotic stress and to improve the horticultural performance of inoculated tomatoes when cultured in the greenhouse under water deficit stress conditions. (3) We ascertained that the best performing isolates were collected in dry to relatively dry regions of the country, suggesting adaptations to mutualism predisposing them to confer water deficit tolerance to the plants they colonized. This potential adaptation response to environmental conditions may have significant implications for the acquisition of Trichoderma strains capable of enhancing crop performance under specific biotic and abiotic stress conditions. (4) We validated plant cultivar-Trichoderma isolate specificity in greenhouse experiments with inoculated tomato plants differing in water stress tolerance. The improvement in horticultural performance was most evident in inoculated, water stress-susceptible tomato genotypes. (5) We distinguished NT33 (T. asperelloides) isolate for its ability to deliver consistent performance advantages to its tomato partners suggesting its potential as an element in multi-faceted environmentally sound approaches to manage crop water deficit stress (Figure 5). It is noteworthy that NT33 also consistently outperformed the commercially available T22 (T. harzianum) strain suggesting that limits to the potential benefits of Trichoderma inoculation under abiotic stress conditions have yet to be reached. Different biochemical and genetic determinants underlying the induction of resistance in the plants are unknown and are currently under investigation. In the future, research should also focus on the mechanisms adopted by these isolates like solubilization and mobilization of nutrients, production of plant growth-promoting hormones, and other secondary metabolites for plant growth promotion and adoption to abiotic stress.
Data Availability Statement
The original contributions presented in the study are included in the article/Supplementary Material, further inquiries can be directed to the corresponding author/s.
Author Contributions
RR, JS, and M-SB conceived the project. RR performed most of the experiments, analyzed the data, and wrote the manuscript. SF and DF conceived the experiments on water deficit susceptible tomato genotypes. SF performed those experiments and wrote the corresponding sections. SM provided Trichoderma isolates. JS, M-SB, SM, and DF edited the manuscript. All the authors contributed to the article and approved the submitted version.
Funding
RR’s Ph.D. Program was financially supported by the Schlumberger Foundation, Faculty for the Future Program. Partial salaries (M-SB and JS) and infrastructural support provided by USDA National Institute of Food and Agriculture (NIFA) Hatch Projects OHO01331 and OHO01456.
Conflict of Interest
The authors declare that the research was conducted in the absence of any commercial or financial relationships that could be construed as a potential conflict of interest.
Publisher’s Note
All claims expressed in this article are solely those of the authors and do not necessarily represent those of their affiliated organizations, or those of the publisher, the editors and the reviewers. Any product that may be evaluated in this article, or claim that may be made by its manufacturer, is not guaranteed or endorsed by the publisher.
Acknowledgments
We would like to acknowledge Ram Khadka for the collection, molecular characterization, and species identification of the selected Trichoderma isolates and Leslie Taylor for technical assistance. We would also like to thank Larry Madden for his help with statistical analysis.
Supplementary Material
The Supplementary Material for this article can be found online at: https://www.frontiersin.org/articles/10.3389/fpls.2022.869090/full#supplementary-material
References
Aghaie, P., Hosseini Tafreshi, S. A., Ebrahimi, M. A., and Haerinasab, M. (2018). Tolerance evaluation and clustering of fourteen tomato cultivars grown under mild and severe drought conditions. Sci. Hortic. 232:1–12.
Al-Hazmi, A. S., and TariqJaveed, M. (2016). Effects of different inoculum densities of Trichoderma harzianum and Trichoderma viride against Meloidogyne javanica on tomato. Saudi J. Biol. Sci. 23:288–292. doi: 10.1016/j.sjbs.2015.04.007
Alwhibi, S., Hashem, A., Abd_Allah, E. F., Alqarawi, A. A., Soliman, D. W. K., Wirth, S., et al. (2017). Increased resistance of drought by Trichoderma harzianum fungal treatment correlates with increased secondary metabolites and proline content. J. Integr. Agric. 16:1751–1757. doi: 10.1016/s2095-3119(17)61695-2
Aujla, I. S., and Paulitz, T. C. (2017). An improved method for establishing accurate water potential levels at different temperatures in growth media. Front. Microbiol. 8:1497. doi: 10.3389/fmicb.2017.01497
Bae, H., Sicher, R. C., Kim, M. S., Kim, S.-H., Strem, M. D., Melnick, R. L., et al. (2009). The beneficial endophyte Trichoderma hamatum isolate DIS 219b promotes growth and delays the onset of the drought response in Theobroma cacao. J. Exp. Bot. 60:3279–3295. doi: 10.1093/jxb/erp165
Bakhshandeh, E., Gholamhosseini, M., Yaghoubian, Y., and Pirdashti, H. (2020). Plant growth promoting microorganisms can improve germination, seedling growth and potassium uptake of soybean under drought and salt stress. Plant Growth Regul. 90:123–136. doi: 10.1007/s10725-019-00556-5
Bashyal, B. M., Parmar, P., Zaidi, N. W., and Aggarwal, R. (2021). Molecular programming of drought-challenged Trichoderma harzianum-bioprimed Rice (Oryza sativa L.). Front. Microbiol. 12:655165. doi: 10.3389/fmicb.2021.655165
Bates, D., Mächler, M., Bolker, B., and Walker, S. (2014). Fitting linear mixed-effects models using lme4. J. Stat. Softw. 67:1–48.
Berry, S.Z., Gould, W.A., and Wiese, K.L. (1991). Ohio ‘8245’ processing tomato. HortScience 26:1093 doi: 10.21273/hortsci.26.8.1093
Blum, A. (1996). Crop responses to drought and the interpretation of adaptation. Plant Growth Regul. 20:135–148. doi: 10.1007/bf00024010
Boretti, A., and Rosa, L. (2019). Reassessing the projections of the World Water Development Report. Npj Clean Water. 2:15.
Brotman, Y., Landau, U., Cuadros-Inostroza, Á., Takayuki, T., Fernie, A. R., Chet, I., et al. (2013). Trichoderma-plant root colonization: escaping early plant defense responses and activation of the antioxidant machinery for saline stress tolerance. PLoS Pathog. 9:e1003221. doi: 10.1371/journal.ppat.1003221
Compant, S., Van Der Heijden, M.G.A., and Sessitsch, A. (2010). Climate change effects on beneficial plant–microorganism interactions. FEMS Microbiol. Ecol. 73: 197–214. doi: 10.1111/j.1574-6941.2010.00900.x
Contreras-Cornejo, H. A., Macías-Rodríguez, L., Cortés-Penagos, C., and López-Bucio, J. (2009). Trichoderma virens, a Plant Beneficial Fungus, Enhances Biomass Production and Promotes Lateral Root Growth through an Auxin-Dependent Mechanism in Arabidopsis. Plant Physiol. 149:1579–1592. doi: 10.1104/pp.108.130369
De Palma, M., D’Agostino, N., Proietti, S., Bertini, L., Lorito, M., Ruocco, M., et al. (2016). Suppression Subtractive Hybridization analysis provides new insights into the tomato (Solanum lycopersicum L.) response to the plant probiotic microorganism Trichoderma longibrachiatum MK1. J. Plant Physiol. 190:79–94. doi: 10.1016/j.jplph.2015.11.005
Delgado-Baquerizo, M., Guerra, C.A., Cano-Díaz, C., et al. (2020). The proportion of soil-borne pathogens increases with warming at the global scale. Nat. Clim. Chang. 10, 550–554. doi: 10.1038/s41558-020-0759-3
Donoso, E., Lobos, G. A., and Rojas, N. (2008). Efecto de Trichoderma harzianum y compost sobre el crecimiento de plántulas de Pinus radiata en vivero. Bosque Valdivia. 29:52–57.
FAO. (2022). FAOSTAT. License: CC BY-NC-SA 3.0 IGO. Available online at: https://fao.org/faostat (accessed March 31, 2022).
Federer, W. T., and Raghavarao, D. (1975). On Augmented Designs. Biometrics 31: 29–35. doi: 10.2307/2529707
Foolad, M. R. (2007). “Current status of breeding tomatoes for salt and drought tolerance”. In Advances in Molecular Breeding Toward Drought and Salt Tolerant Crops, eds Jenks, M.A., Hasegawa, P.M., Jain, S.M, (Dordrecht: Springer Netherlands), 669–700. doi: 10.1007/978-1-4020-5578-2_27
Giauque, H., Connor, E. W., and Hawkes, C. V. (2019). Endophyte traits relevant to stress tolerance, resource use and habitat of origin predict effects on host plants. New Phytol. 221:2239–2249. doi: 10.1111/nph.15504
Grover, M., Ali, S. Z., Sandhya, V., Rasul, A., and Venkateswarlu, B. (2011). Role of microorganisms in adaptation of agriculture crops to abiotic stresses. World J. Microbiol. Biotechnol. 27:1231–1240. doi: 10.1007/s11274-010-0572-7
Guler, N. S., Pehlivan, N., Karaoglu, S. A., Guzel, S., and Bozdeveci, A. (2016). Trichoderma atroviride ID20G inoculation ameliorates drought stress-induced damages by improving antioxidant defence in maize seedlings. Acta Physiol. Plant. 38:132.
Harman, G. E. (2011). Trichoderma—not just for biocontrol anymore. Phytoparasitica 39:103–108. doi: 10.1007/s12600-011-0151-y
Harman, G. E., Doni, F., Khadka, R. B., and Uphoff, N. (2019). Endophytic strains of Trichoderma increase plants’ photosynthetic capability. J. Appl. Microbiol. 130:529–546. doi: 10.1111/jam.14368
Harman, G. E., Petzoldt, R., Comis, A., and Chen, J. (2004). Interactions between Trichoderma harzianum strain T22 and maize inbred line Mo17 and effects of these interactions on diseases caused by Pythium ultimum and Colletotrichum graminicola. Phytopathology 94:147–153. doi: 10.1094/PHYTO.2004.94.2.147
Hermosa, R., Viterbo, A., Chet, I., and Monte, E. (2012). Plant-beneficial effects of Trichoderma and of its genes. Microbiology 158:17–25. doi: 10.1099/mic.0.052274-0
Hoeksema, J. D. (2010). Ongoing coevolution in mycorrhizal interactions: Tansley review. New Phytol. 187:286–300. doi: 10.1111/j.1469-8137.2010.03305.x
Jin, X., Harman, G. E., and Taylor, A. G. (1991). Conidial biomass and desiccation tolerance of Trichoderma harzianum produced at different medium water potentials. Biol. Control. 1:237–243. doi: 10.1016/1049-9644(91)90072-8
Karki, R., Talchabhadel, R., Aalto, J., and Baidya, S. K. (2016). New climatic classification of Nepal. Theor. Appl. Climatol. 125:799–808. doi: 10.1007/s00704-015-1549-0
Kashyap, P. L., Rai, P., Srivastava, A. K., and Kumar, S. (2017). Trichoderma for climate resilient agriculture. World J. Microbiol. Biotechnol. 33:155. doi: 10.1007/s11274-017-2319-1
Kassambara, A. (2020). ggpubr: “ggplot2” Based Publication Ready Plots. R package version 0.6.0. Available online at: https://CRAN.R-project.org/packages=rstatix
Khadka, R. B., and Miller, S. A. (2021). Synergy of anaerobic soil disinfestation and Trichoderma spp. in Rhizoctonia root rot suppression. Front. Sustain. Food Syst. 5:645736. doi: 10.3389/fsufs.2021.645736
Khadka, R., Rotondo, F., and Miller, S. A. (2022). The pursuit in Nepal of native Trichoderma spp. for plant disease biocontrol. PHYTOFR 8:21.
Khan, M. Y., Haque, M. M., Molla, A. H., Rahman, M. M., and Alam, M. Z. (2017). Antioxidant compounds and minerals in tomatoes by Trichoderma-enriched biofertilizer and their relationship with the soil environments. J. Integr. Agric. 16:691–703. doi: 10.1016/s2095-3119(16)61350-3
Khoshmanzar, E., Aliasgharzad, N., Neyshabouri, M. R., Khoshru, B., Arzanlou, M., and Asgari Lajayer, B. (2020). Effects of Trichoderma isolates on tomato growth and inducing its tolerance to water-deficit stress. Int. J. Environ. Sci. Technol. 17:869–878. doi: 10.1007/s13762-019-02405-4
Kim, Y.-C., Glick, B. R., Bashan, Y., and Ryu, C.-M. (2012). “Enhancement of Plant Drought Tolerance by Microbes,” in Plant Responses to Drought Stress: From Morphological to Molecular Features, ed. R. Aroca (Berlin, Heidelberg: Springer Berlin Heidelberg), 383–413. doi: 10.1007/978-3-642-32653-0_15
Kubicek, C. P., Steindorff, A. S., Chenthamara, K., Manganiello, G., Henrissat, B., Zhang, J., et al. (2019). Evolution and comparative genomics of the most common Trichoderma species. BMC Genomics. 20:485. doi: 10.1186/s12864-019-5680-7
Lombardi, N., Vitale, S., Turrà, D., Reverberi, M., Fanelli, C., Vinale, F., et al. (2018). Root exudates of stressed plants stimulate and attract Trichoderma soil fungi. Mol. Plant. Microbe Interact. 31, 982–994. doi: 10.1094/MPMI-12-17-0310-R
Martinez-Medina, A., Fernandez, I., Sánchez-Guzmán, M. J., Jung, S. C., Pascual, J. A., and Pozo, M. J. (2013). Deciphering the hormonal signalling network behind the systemic resistance induced by Trichoderma harzianum in tomato. Front. Plant Sci. 4:206. doi: 10.3389/fpls.2013.00206
Mastouri, F., Björkman, T., and Harman, G. E. (2010). Seed treatment with Trichoderma harzianum alleviates biotic, abiotic, and physiological stresses in germinating seeds and seedlings. Phytopathology 100, 1213–1221. doi: 10.1094/PHYTO-03-10-0091
Mastouri, F., Björkman, T., and Harman, G. E. (2012). Trichoderma harzianum enhances antioxidant defense of tomato seedlings and resistance to water deficit. Mol. Plant. Microbe Interact. 25, 1264–1271. doi: 10.1094/MPMI-09-11-0240
Meena, K. K., Sorty, A. M., Bitla, U. M., Choudhary, K., Gupta, P., Pareek, A., et al. (2017). Abiotic stress responses and microbe-mediated mitigation in plants: The omics strategies. Front. Plant Sci. 8:172. doi: 10.3389/fpls.2017.00172
Mendiburu, F. D. (2015). Package ‘agricolae’. R Package. Version 1.2-3. Available online at: http://CRAN.R-project.org/package=agricolae
Meng, X., Miao, Y., Liu, Q., Ma, L., Guo, K., Liu, D., et al. (2019). TgSWO from Trichoderma guizhouense NJAU4742 promotes growth in cucumber plants by modifying the root morphology and the cell wall architecture. Microb. Cell Factories. 18:148. doi: 10.1186/s12934-019-1196-8
Mhadhbi, H., Chihaoui, S., Mhamdi, R., Mnasri, B., Jebara, M., and Mhamdi, R. (2011). A highly osmotolerant rhizobial strain confers a better tolerance of nitrogen fixation and enhances protective activities to nodules of Phaseolus vulgaris under drought stress. Afr. J. Biotechnol. 10, 4555–4563.
Mona, S. A., Hashem, A. Abd-Allah, E. F., Alqarawi, A. A., Soliman, D. W. K., Wirth, S., et al. (2017). Increased resistance of drought by Trichoderma harzianum fungal treatment correlates with increased secondary metabolites and proline content. J. Integr. Agric. 16, 1751–1757. doi: 10.1016/S2095-3119(17)61695-2
Orchard, C. J., Cooperstone, J. L., Gas-Pascual, E., Andrade, M. C., Abud, G., Schwartz, S. J., et al. (2021). Identification and assessment of alleles in the promoter of the Cyc-B gene that modulate levels of β-carotene in ripe tomato fruit. Plant Genome. 14:e20085. doi: 10.1002/tpg2.20085
Pal, S., Zhao, J., Khan, A., Yadav, N. S., Batushansky, A., Barak, S., et al. (2016). Paclobutrazol induces tolerance in tomato to deficit irrigation through diversified effects on plant morphology, physiology and metabolism. Sci. Rep. 6:39321. doi: 10.1038/srep39321
Petrozza, A., Santaniello, A., Summerer, S., Di Tommaso, G., Di Tommaso, D., Paparelli, E., et al. (2014). Physiological responses to Megafol® treatments in tomato plants under drought stress: A phenomic and molecular approach. Sci. Hortic. 174, 185–192. doi: 10.1016/j.scienta.2014.05.023
Poosapati, S., Ravulapalli, P. D., Tippirishetty, N., Vishwanathaswamy, D. K., and Chunduri, S. (2014). Selection of high temperature and salinity tolerant Trichoderma isolates with antagonistic activity against Sclerotium rolfsii. SpringerPlus. 3:641. doi: 10.1186/2193-1801-3-641
Prashar, P., and Vandenberg, A. (2017). Genotype-specific responses to the effects of commercial Trichoderma formulations in lentil (Lens culinaris ssp. culinaris) in the presence and absence of the oomycete pathogen Aphanomyces euteiches. Biocontrol Sci. Technol. 27, 1123–1144. doi: 10.1080/09583157.2017.1376035
R Core Team (2013). R: A Language and Environment for Statistical Computing. Vienna, Austria: R Foundation for Statistical Computing.
Rawal, R. (2021). Potential of Beneficial Trichoderma Isolates in Alleviating Water Deficit Stress in Tomato. Ph.D.thesis, Columbus, Ohio: The Ohio State University.
Ruano Rosa, D., and López Herrera, C. J. (2009). Evaluation of Trichoderma spp. as biocontrol agents against avocado white root rot. Biol. Control. 51, 66–71. doi: 10.1016/j.biocontrol.2009.05.005
Samuels, G. L. (2015). Trichoderma: Identification and Agricultural Applications. St. Paul, MN: The American Phytopathological Society (APS Press).
Schabenberger, O., Gregoire, T. G., and Kong, F. (2000). Collections of Simple Effects and Their Relationship to Main Effects and Interactions in Factorials. Am. Stat. 54, 210–214. doi: 10.2307/2685592
Scudeletti, D., Crusciol, C. A. C., Bossolani, J. W., Moretti, L. G, Momesso, L. Servaz Tubaña, B., et al. (2021). Trichoderma asperellum inoculation as a tool for attenuating drought stress in sugarcane. Front. Plant Sci. 12:645542. doi: 10.3389/fpls.2021.645542
Shukla, N., Awasthi, R. P., Rawat, L., and Kumar, J. (2012). Biochemical and physiological responses of rice (Oryza sativa L.) as influenced by Trichoderma harzianum under drought stress. Plant Physiol. Biochem. 54, 78–88. doi: 10.1016/j.plaphy.2012.02.001
Singh, V. K., Singh, A. K., Singh, P. P., and Kumar, A. (2018). Interaction of plant growth promoting bacteria with tomato under abiotic stress: A review. Agric. Ecosyst. Environ. 267, 129–140. doi: 10.1016/j.agee.2018.08.020
Somerville, C., and Briscoe, J. (2001). Editorial: Genetic Engineering and Water. Sci. New Ser. 292:2217.
Stroup, W. W., Milliken, G. A., Claassen, E. A., and Wolfinger, R. D. (2018). SAS for Mixed Models: Introduction and Basic Applications. Cary, NC: SAS Inst. Inc.
Subramanian, P., Kim, K., Krishnamoorthy, R., Mageswari, A., Selvakumar, G., and Sa, T. (2016). Cold stress tolerance in psychotolerant soil bacteria and their conferred Chilling resistance in tomato (Solanum lycopersicum Mill.) under low temperatures. PLoS One 11:e0161592. doi: 10.1371/journal.pone.0161592
Teulat, B., Monneveux, P., Wery, J., Borries, C., Souyris, I., Charrier, A., et al. (1997). Relationships between relative water content and growth parameters under water stress in barley: a QTL study. New Phytol. 137, 99–107. doi: 10.1046/j.1469-8137.1997.00815.x
Trenberth, K. E., Dai, A., van der Schrier, G., Jones, P. D., Barichivich, J., Briffa, K. R., et al. (2014). Global warming and changes in drought. Nat. Clim. Change 4, 17–22.
Tripathi, R., Keswani, C., and Tewari, R. (2021). Trichoderma Koningii enhances tolerance against thermal stress by regulating ROS metabolism in tomato (Solanum lycopersicum L.) plants. J. Plant Interact. 16, 116–126. doi: 10.1080/17429145.2021.1908634
Tucci, M., Ruocco, M., Masi, L. D., Palma, M. D., and Lorito, M. (2011). The beneficial effect of Trichoderma spp. on tomato is modulated by the plant genotype. Mol. Plant Pathol. 12, 341–354. doi: 10.1111/j.1364-3703.2010.00674.x
Tyagi, S., Singh, R., and Javeria, S. (2014). Effect of Climate Change on Plant-Microbe Interaction: An Overview. Eur. J. Mol. Biotechnol. 5, 149–156. doi: 10.13187/ejmb.2014.5.149
Uddling, J., Gelang-Alfredsson, J., Piikki, K., and Pleijel, H. (2007). Evaluating the relationship between leaf chlorophyll concentration and SPAD-502 chlorophyll meter readings. Photosynth. Res. 91, 37–46. doi: 10.1007/s11120-006-9077-5
Van Wees, S. C., Van der Ent, S., and Pieterse, C. M. (2008). Plant immune responses triggered by beneficial microbes. Curr. Opin. Plant Biol. 11, 443–448. doi: 10.1016/j.pbi.2008.05.005
Vinale, F., Nigro, M., Sivasithamparam, K., Flematti, G., Ghisalberti, E. L., Ruocco, M., et al. (2013). Harzianic acid: a novel siderophore from Trichoderma harzianum. FEMS Microbiol. Lett. 347, 123–129. doi: 10.1111/1574-6968.12231
Waghunde, R. R., Shelake, R. M., and Sabalpara, A. N. (2016). Trichoderma: A significant fungus for agriculture and environment. Afr. J. Agric. Res. 11, 1952–1965. doi: 10.5897/ajar2015.10584
Keywords: abiotic stress, fungi, plant growth-promoting microbes, PEG, arid region, horticulture
Citation: Rawal R, Scheerens JC, Fenstemaker SM, Francis DM, Miller SA and Benitez M-S (2022) Novel Trichoderma Isolates Alleviate Water Deficit Stress in Susceptible Tomato Genotypes. Front. Plant Sci. 13:869090. doi: 10.3389/fpls.2022.869090
Received: 03 February 2022; Accepted: 01 April 2022;
Published: 02 May 2022.
Edited by:
Walter Chitarra, Council for Agricultural Research and Economics Research (CREA), ItalyReviewed by:
M. Aydin Akbudak, Akdeniz University, TurkeyNandani Shukla, Department of Science and Technology, India
Copyright © 2022 Rawal, Scheerens, Fenstemaker, Francis, Miller and Benitez. This is an open-access article distributed under the terms of the Creative Commons Attribution License (CC BY). The use, distribution or reproduction in other forums is permitted, provided the original author(s) and the copyright owner(s) are credited and that the original publication in this journal is cited, in accordance with accepted academic practice. No use, distribution or reproduction is permitted which does not comply with these terms.
*Correspondence: Maria-Soledad Benitez, benitezponce.1@osu.edu