- 1Key Laboratory of Forest Ecology and Environment of National Forestry and Grassland Administration, Ecology and Nature Conservation Institute, Chinese Academy of Forestry, Beijing, China
- 2Miyaluo Research Station of Alpine Forest Ecosystem, Lixian County, Sichuan, China
- 3Co-Innovation Center for Sustainable Forestry in Southern China, Nanjing Forestry University, Nanjing, China
- 4Institute for Sustainable Plant Protection, National Research Council of Italy, Torino, Italy
Interactions between soil fungi and soil environmental factors regulate soil nitrogen (N) mineralization rates on the eastern Qinghai-Tibetan Plateau. Some studies have also illuminated differences in soil N mineralization rate based on different mycorrhizal forests, but the associated effect of soil fungal functional guilds and soil environmental factors underlying this process are not well-understood. Three primary forests respectively dominated by Abies fargesii var. faxoniana (ectomycorrhizal, EcM), Cupressus chengiana (arbuscular mycorrhizal, AM) and Rhododendron phaeochrysum (ericoid mycorrhizal, ErM) trees were selected in this area. Meanwhile, soil net N mineralization rate, soil fungal composition and soil enzyme activity among these three mycorrhizal forests were studied. Our results showed that there were significant differences in the seasonal variation of soil net N mineralization rates among three mycorrhizal forests. Soil net N mineralization rate in the AM forest was faster. EcM fungi and saprotroph are the main functional guilds in these three mycorrhizal forests. Meanwhile, the relative abundances of soil fungal functional guilds, soil temperature and soil peroxidase activity could explain 85.0% in the difference of soil net ammonification rate among three mycorrhizal forests. In addition, soil temperature, soil water-filled pore space and soil ammonium content play a central role in controlling the differing soil net nitrification rate among three mycorrhizal forests. Our results suggest differences in soil net mineralization among different mycorrhizal forest types are driven mainly by soil net ammonification. Soil fungal functional guilds and temperature regulate the rate of soil net ammonification by modulating soil peroxidase activity.
Introduction
Many ecological properties of forests are affected by soil nitrogen (N) uptake and utilization, including plant growth, interspecific competition and soil carbon (C) sequestration (Cole et al., 2008; Levy-Booth et al., 2014). Forest soil N is present in two organic forms: minerals and organic compounds (Adamczyk et al., 2016). However, plants absorb mainly inorganic N (e.g., ammonium , nitrate ) (Zhang et al., 2018), with a minor amount of low molecular weight organic N being absorbed under special environmental conditions (Li et al., 2019). Soil organic N is only absorbed easily by plants after its transformation into inorganic N by soil microbial mineralization (Liu et al., 2017). Therefore, soil N mineralization is a critical process which determines soil N availability and ecosystem primary productivity (Li et al., 2019).
Soil fungi are the primary decomposers of organic matter and driver of nutrient cycling in forest ecosystem (Zeilinger et al., 2016). Soil fungi in forest are broadly classified into two functional groups: free-living saprotrophs and plant root symbiotic fungi (Bödeker et al., 2016). Fungi that live in symbiotic relationships with plant roots may do so through ectomycorrhizal (EcM) or other types of symbiosis, including arbuscular mycorrhizal (AM) and ericoid mycorrhizal (ErM) (Hobbie and Högberg, 2012; Tedersoo et al., 2020; Wang et al., 2022). The differences among these three groups of mycorrhizal fungi are important because of their biogeochemical significance. Specific ecological functions of different mycorrhizal fungi as well as complex interactions between mycorrhizal fungi and other soil microbes might influence the activity and abundance of N cycling functional guilds (Tedersoo et al., 2020; Saifuddin et al., 2021), resulting in changes to N cycling among different mycorrhizal forests (Bahram et al., 2020). Compared to EcM forest or ErM forest, soil N cycling in AM forest is often more ‘rapid’ and ‘open’ which is dominated by inorganic N cycling patterns (Phillips et al., 2013; Tedersoo and Bahram, 2019). Both EcM and ErM fungi could produce a wide range of enzymes (such as oxidases and peroxidases) that enable them to release N from soil organic matter (Orwin et al., 2011; Ward et al., 2021). By contrast, AM fungi are much less capable of producing these enzymes, typically lacking the complement of enzymes that decompose organic matter (Saifuddin et al., 2021). Accordingly, AM plants are primarily responsible for absorbing inorganic N in exchange for carbon derived from plant photosynthesis (Johnson, 2010; Van der Heijden et al., 2015; Han et al., 2020; Babalola et al., 2022), whereas EcM and ErM plants typically obtain more organic N from soil (Wurzburger and Hendrick, 2009). Given that EcM and ErM fungi have broader enzymatic capabilities, they could compete directly with saprotrophs for organic substrates (Adamczyk et al., 2016). This interspecific competition could decrease rates of soil N mineralization in forests (Argiroff et al., 2022). Yet the no-inhibited saprotrophs in AM forest soil could enhance litter decomposition to accelerate soil N mineralization (Midgley and Phillips, 2016; Saifuddin et al., 2021). Previous studies also had shown that eliminating AM fungi from soil could slow soil N mineralization by reducing the substrate supply of saprotrophs (Cheng et al., 2012; Averill et al., 2014). Similarly, Bahram et al. (2020) indicated that the relative abundances of AM fungi, saprotrophs and pathogens were all higher in AM forest than EcM forest. These alterations in microbial composition reflect the rapid nutrient cycling of AM forest soil.
In addition, the soil N mineralization rate features high spatial and temporal heterogeneity among different mycorrhizal forests, it being also affected by soil temperature, soil moisture and soil physicochemical properties (Liu et al., 2017). Recent research has revealed that whereas soil moisture and temperature were the main factors impacting the relative abundance of EcM fungi, soil physicochemical properties are the main factors controlling the relative abundance of saprotrophs (Wang et al., 2019). Soil fungal associations that catalyzed organic matter mineralization responded differently to various soil environmental factors and this might lead to uncertainty in soil N mineralization (Mushinski et al., 2020). Although soil fungi and environmental factors among different mycorrhizal forests arguably have certain effects on soil N mineralization, our knowledge of direct and indirect effects of soil fungi and environmental factors on that process is still quite limited.
The eastern Qinghai-Tibetan Plateau presents a unique natural environment, one that contributes critically to soil and water conservation, climate regulation, and biodiversity protection (Wang et al., 2007). It is an important ecological barrier in the middle and upper reaches of the Yangtze River (Chen, 2019). In addition, this area is abundant in natural resources, among which Abies fargesii var. faxoniana, Cupressus chengiana and Rhododendron phaeochrysum are important forest trees (Feng et al., 2017; Du et al., 2021), which respectively are the typical EcM, AM and ErM trees (Soudzilovskaia et al., 2020). Previous studies in this area have partially explored the changes of soil fungal community structure and soil N mineralization among different forest types (Liu et al., 2021; Chen et al., 2022). Yet the potential mechanism of the difference of soil N mineralization among different mycorrhizal forests remains to be further studied. In order to elucidate the dominant factors responsible for differential soil N mineralization among three mycorrhizal forests. Seasonal variation of soil net N mineralization rate, soil fungal functional guilds composition and soil enzyme activity among these three mycorrhizal forests (EcM, AM and ErM forests) were measured. We hypothesized that (1) the soil net N mineralization rate was the highest in AM forest, (2) the relative abundance of EcM fungi and AM fungi was relatively higher in EcM and AM forests, respectively, (3) synergy between soil fungal functional guilds and soil environmental factors could drive the activity of soil oxidases and peroxidases, and (4) soil oxidases and peroxidases activity have a greater effect on soil net ammonification than soil net nitrification.
Materials and methods
Site description and design
This study was conducted in in the upper reaches of the Minjiang River, western Sichuan Province (31°35′~31°53′ N, 102°2′~102°48′ E), which is located in the outermost part of the fold belt on the eastern Qinghai-Tibetan Plateau (Xu et al., 2021). Its altitude ranges from 2,200 to 5,500 m. The climate with an average annual temperature of 2~4°C, the highest temperature is 23.7°C in summer, and the lowest temperature is -18.1°C in winter. Annual precipitation is 700~1000 mm and concentrated mainly in the growing season (Chen, 2019). The soil in this area is defined as mountain brown soil, mountain brown cinnamon soil and subalpine meadow soil according to the Chinese soil taxonomic classification (Liu, 2010; Feng et al., 2017; Chen, 2019).
Three different primary forests with different mycorrhizal types were selected, including Abies fargesii var. faxoniana primary forest (EcM forest), Cupressus chengiana primary forest (AM forest) and Rhododendron phaeochrysum primary forest (ErM forest) under similar soil and climate conditions. Eight 15 m ×15 m sample plots (≥ 90% the dominant species by basal area in each sample plot) for each forest type were randomly set. In each forest type, the distance between any two sample plots was more than 50 m.
Soil sampling and analysis
The mineral soil (0-10 cm) samples were collected from the four corners and center of each plot with soil drill from May to November 2019. Five mineral soil samples were mixed into a zipper storage bag and transported to laboratory in an icebox within 3 h. Meanwhile, five polyvinyl chloride collar cores (PVC cores, 15 cm in height and 5 cm in diameter) were buried into depth of 10 cm in the vicinity of each soil sampling location (Idol et al., 2003). In order to separate water and allow gas movement, the top of the PVC core was covered with a permeable plastic film and its bottom covered with gauze (Liu et al., 2021). The difference between soil and contents each month was used to quantify the rates of soil net ammonification, net nitrification and net N mineralization (Wang et al., 2017), which was the ideal time to estimate changes in soil N (Becker et al., 2015). For the measurement of soil water-filled pore space (WFPS), we followed the methodology of Wang et al. (2010). The temperature of mineral soil was measured with a soil temperature detector in each plot (Liu et al., 2021). Furthermore, the mixed mineral soil was sifted through a 2-mm sieve and gravel and fine roots were removed (Xu et al., 2021). The fresh mineral soil was separated into two parts: one was dried naturally to measure soil pH, soil organic C (SOC) and soil total N (TN); the other was stored at -20°C to analysis enzyme activity and microbial community structure.
Soil and contents were quantified using an automatic flow injection analyzer (FIAstar 5000 Analyzer, Sweden). Soil pH was determined using the glass electrode meter method, by setting the 1:2.5 (w/v) ratio of soil material to deionized water. The SOC content was measured by applying the wet oxidation method with K2Cr2O7 and H2SO4, and FeSO4 titration. Soil TN content was determined by the Kjeldahl method (Liu et al., 2020). The soil C: N ratio was calculated as the ratio of SOC to soil TN. Finally, the potential activity of peroxidase (PER) and phenol oxidase (POX) which could drive N from soil organic matter (Jian et al., 2016), were determined via microplate fluorescence and photometry (Zheng et al., 2019).
Molecular and bioinformatics analysis
Samples from May, July and November 2019 were selected for soil fungal functional guilds composition analysis. DNA was extracted from 0.25 g of each soil sample by using the PowerSoil® Kit(100) and the concentration of extracted DNA was then determined by a NanoDrop 1000 spectrophotometer. Soil fungi were amplified fungal ITS2region and selected primers pairs fITS7 (5’- GTGARTCATCGAATCTTTG-3’) and ITS4 (5’-AGCCTCCGCTTATTGATATGCTTAART-3’) (Xiong et al., 2021). The 25 μL PCR reaction mixture contained 8.5 μL of sterile deionized water, 0.75 μL of each primer, 12.5 μL of KAPA Polymerase and 2.5 μL of diluted template DNA. The PCR amplification as followed: begin with 3 min of incubation at 95 °C, then 35 cycles of 98 °C for 30 s, 56 °C for 30 s, 72 °C for 30 s, with a final extension at 72 °C for 10 min. Sterile deionized water was used as a template (negative controls) to determine whether there was contamination in our experiment (Yao et al., 2019). Three PCR reactions were pooled for each sample to reduce random of the PCR reaction (Bödeker et al., 2016). In addition, the PCR products from each soil sample were purified by the Product Gel Purification Kit. An equal quality of purified PCR product from each sample was pooled in and adjusted to 10 ng μL-1 (Dong et al., 2021). Sequencing was carried out on an Illumina MiSeq PE platform at the Chengdu Institute of Biology, Chinese Academy of Sciences, China.
The chimeras present in the origin sequencing data were removed using the UNITE database, after which the non-chimeric sequence was quality filtered using Usearch. Remaining sequences were clustered into operational taxonomic units (OTUs) at a 97% sequence similarity. Representative fungal sequences were classified using sintax in the UNITE database with a 0.65 confidence threshold (Edgar, 2016). Finally, the number of sequences per sample was normalized to the smallest sample size using the sub.sample function in MOTHUR (Yao et al., 2019) and then we got 10,952 high-quality reads from 72 soil samples. Soil fungal rarefaction curves (Figures S1) were analyzed by R 4.1 software (using the ‘vegan’ package). Soil fungal functional guilds were assigned using the FUNGuild command in Python 3.7 software (Tedersoo et al., 2014; Nguyen et al., 2016). We excluded OTUs that did not belong to a confidence ranking with “probable” or “highly probable” and that were above the genus level (Pfennigwerth et al., 2018).
Statistical analysis
A two-way ANOVA were used to analyze the effects of forest type, month and their interaction on the changes in soil temperature and soil WFPS. Likewise, we also used this method to analyze differences in the rates of soil net ammonization, soil net nitrification and soil net N mineralization (Liu et al., 2021). For multiple comparisons, Tukey’s HSD method was used. For a given month, differences in soil properties, soil net ammonization rate, soil net nitrification rate, and soil net N mineralization rate and soil enzyme activities among three mycorrhizal forests were performed by a one-way ANOVA.
Pearson correlations were used to analyze the effects of soil enzyme activity, edaphic variables and soil fungal functional guilds classified by FUNGuilds on soil net ammonization rate and soil net nitrification rate among three mycorrhizal forests. Further, the significant influencing factors (P < 0.05) after the correlation analysis, soil net nitrification rate and soil net ammonification rate were selected for a structural equation (SEM) analysis. Model estimation was achieved based on the maximum likelihood method. The adequacy of model fit were determined by non-significant χ2 tests (P > 0.05), comparative fit index (CFI) (values ≥ 0.9) and standardized root mean square residual (SRMR) (values < 0.08) (Ni et al., 2022).
The SEM analysis only selected the data from May, July and November 2019. The ANOVAs and Pearson correlations were carried out in SPSS 26 software. The SEM analysis was performed using R 4.1 software (using the ‘lavaan’ package). Figures were created by Origin 8.0 software.
Results
Edaphic variables
Seasonal variation of soil temperature and soil WFPS among three mycorrhizal forests showed significant differences during the study period (P < 0.01) (Figure 1). Soil temperature of each mycorrhizal forest followed a single peak curve, being highest in July and lowest in November. Soil temperature was the highest in AM forest (6.7-18.0 °C), followed by EcM forest (2.8-11.6 °C) and ErM forest (1.0-11.3 °C) (Figure 1A). Contrary to soil temperature, soil WFPS in AM forest (50.46%-82.56%) was significantly lower than that in EcM forest (62.41%-120.68%) and ErM forest (80.23%-126.30%) (Figure 1B).
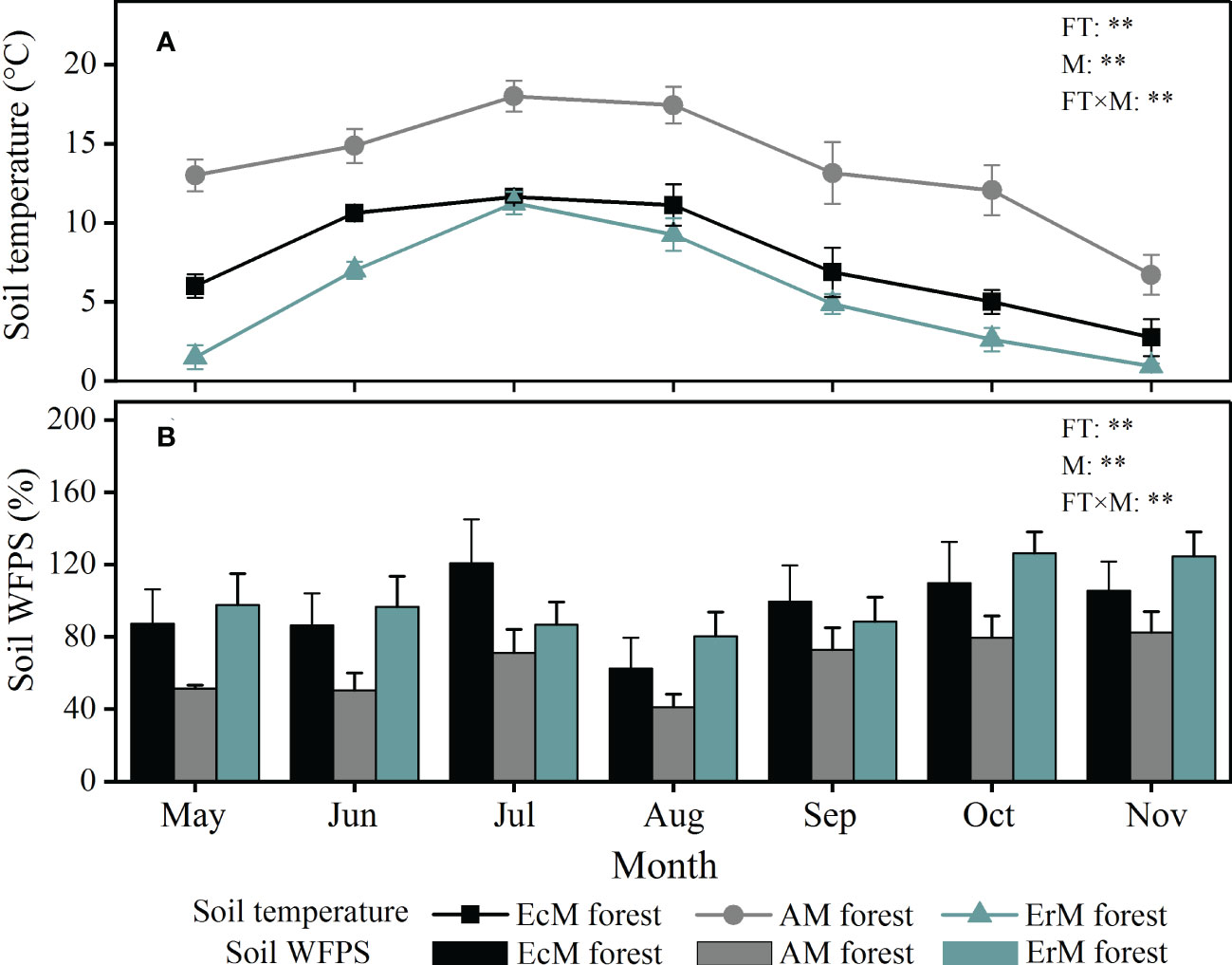
Figure 1 Monthly variations in soil temperature (A) and soil WFPS (B) among three mycorrhizal forests. The vertical bars are the standard error, n=8. EcM forest, Abies fargesii var. faxoniana primary forest; AM forest, Cupressus chengiana primary forest; ErM forest, Rhododendron phaeochrysum primary forest. WFPS, water-filled pore space; FT, forest type; M, month; FT×M, the interaction of forest type and month, **, P < 0.01.
As shown in Table 1, there were significant differences in soil pH and soil TN content, both being highest in AM forest (P < 0.05). SOC content and soil content were significantly lower in EcM forest than those in AM forest and ErM forest (P <0.05). Meanwhile, AM forest had the highest soil content but there was no significant difference between EcM forest and ErM forest. However, soil C: N was significantly lower in AM forest than the other two forests (P < 0.05).
Soil net ammonification, net nitrification and net N mineralization
Forest type, sampling month and their interaction had significant effects on soil net ammonization rate (net Ra), soil net nitrification rate (net Rn) and soil net N mineralization rate (net Nmin) (P < 0.01) (Figure 2). Soil net Ra ranged from -0.16 to 0.31 mg kg-1 d-1 among three mycorrhizal forests, with the highest rates occurring in July (Figure 2A). Soil mean net Ra in ErM forest was negative (-0.09 mg kg-1 d-1) being also significantly lower than that in EcM forest (0.10 mg kg-1 d-1) and AM forest (0.13 mg kg-1 d-1) (P < 0.05) (Figure 2D).
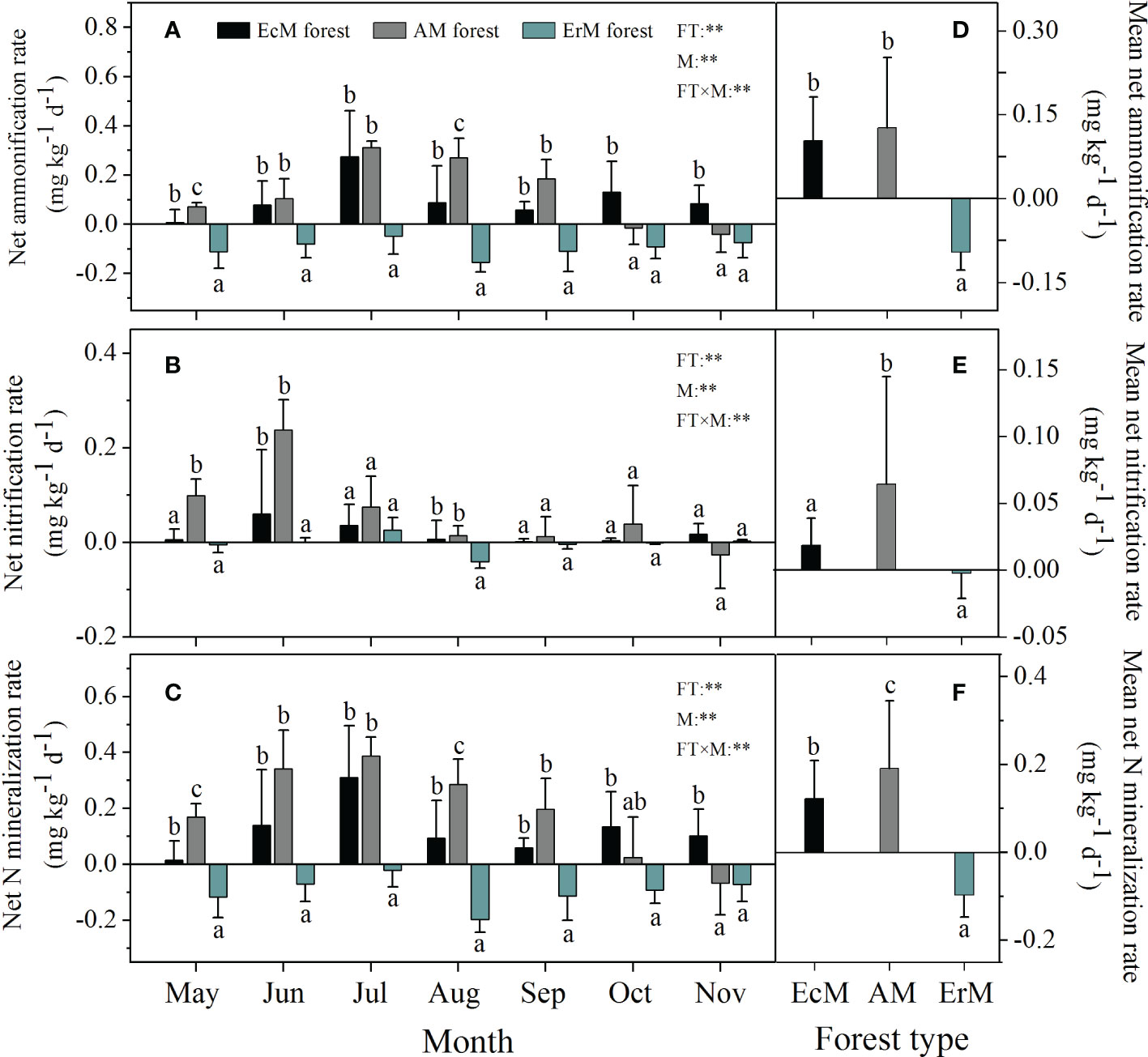
Figure 2 Soil net ammonification rate (A), soil net nitrification rate (B), soil net N mineralization rate (C) varied monthly and their mean rates (D–F) among three mycorrhizal forests. Data and error bars are the means and standard errors respectively, n=8. EcM forest, Abies fargesii var. faxoniana primary forest; AM forest, Cupressus chengiana primary forest; ErM forest, Rhododendron phaeochrysum primary forest. FT, forest type; M, month; FT×M, the interaction of forest type and month. **, P < 0.01. Lowercase letters (a, b and c) indicate significant differences among three mycorrhizal forests (P < 0.05).
The significant effect of mycorrhizal forest types on soil net Rn was detected in May, June and August. Soil net Rn in EcM forest (0.06 mg kg-1 d-1) and AM forest (0.24 mg kg-1 d-1) peaked in June while that in ErM forest (0.03 mg kg-1 d-1) peaked in July (Figure 2B). Overall, AM forest had the highest soil mean net Rn (0.06 mg kg-1 d-1) whereas it did not differ significantly between EcM and ErM forests (Figure 2E).
The trends in monthly variation of soil net Nmin were similar to those of soil net Ra among three mycorrhizal forests (Figure 2C). There were significant differences in soil mean net Nmin among AM forest (0.19 mg kg-1 d-1), EcM forest (0.12 mg kg-1 d-1) and ErM forest (-0.10 mg kg-1 d-1) (P < 0.05) (Figure 2F).
Soil fungal composition and enzyme activity
In general, 53 fungal classes were identified by excluding the unidentified fungal groups from the 72 soil samples. The top five dominant classes of soil fungi were Agaricomycetes (26.98%-59.96%), Leotiomycetes (9.57%-27.47%), Sordariomycetes (3.55%-15.27%), Eurotiomycetes (3.46%-13.51%) and Archaeorhizomycetes (1.53%-16.13%). By contrast, Dothideomycetes (1.08%-5.06%), Mortierellomycetes (2.36%-8.25%) and Umbelopsidomycetes (0.47%-8.44%) presented low relative abundances in the three forests (Figure 3).
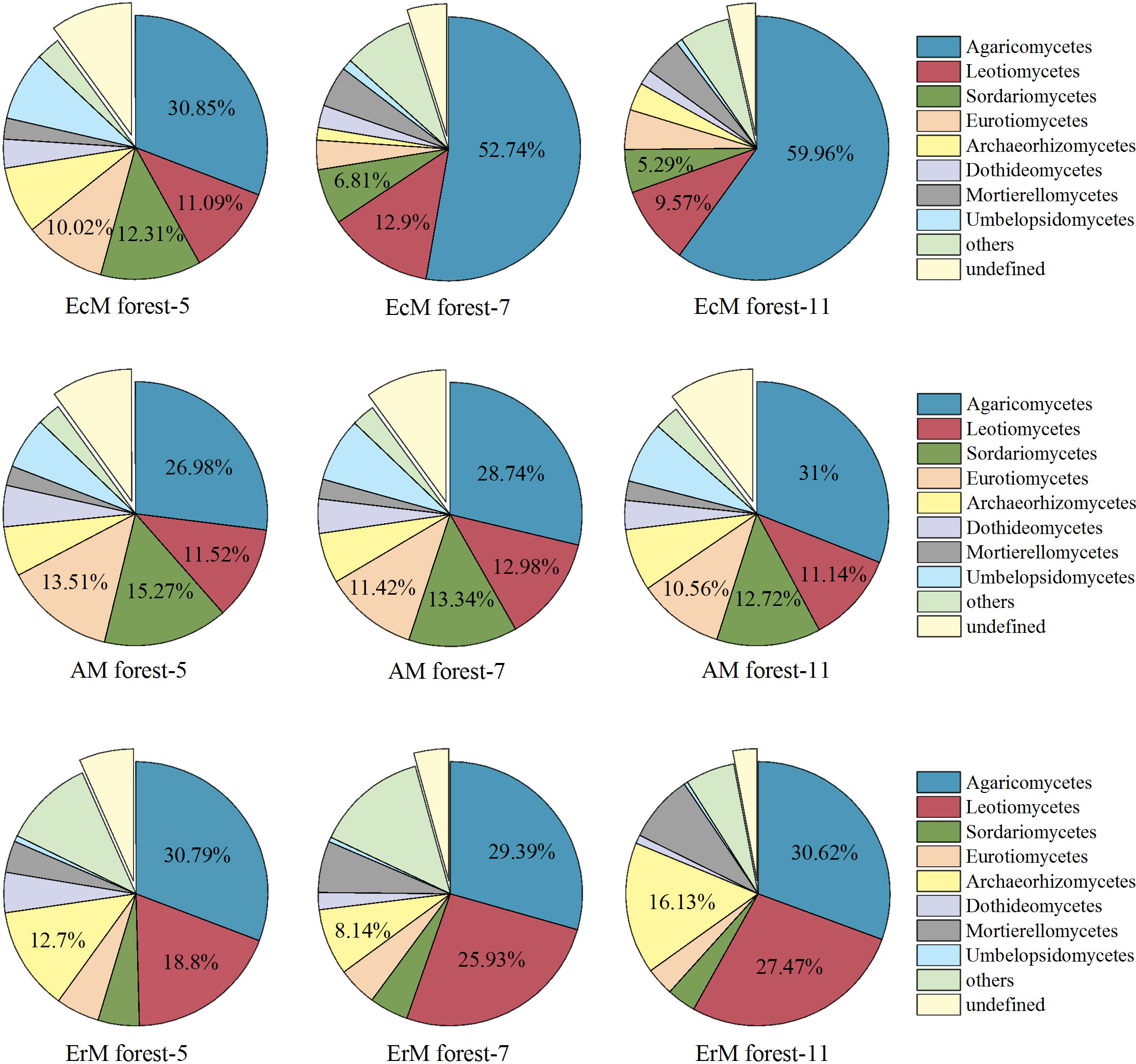
Figure 3 Relative abundances of soil fungal at the class level among three mycorrhizal forests in each different sampling month. ‘Others’ represent the sum of all classes with the relative abundance less than 1%. EcM forest, Abies fargesii var. faxoniana primary forest; AM forest, Cupressuschengiana primary forest; ErM forest, Rhododendron phaeochrysum primary forest; -5, -7 and -11 represented May, July and November respectively.
We also obtained three main trophic modes (saprotroph, symbiotroph and pathotroph) and 10 soil fungal functional guilds from the 10952 OTUs. The relative abundance of the unassigned group (40.49%-77.01%) was dominant in our study. Among the assigned OTUs, the relative abundance of EcM fungi (4.14%-52.80%) was the highest, followed by the undefined saprotroph (SAP) (3.80%-15.21%) and ErM fungi (0.48%-5.18%). The relative abundances of the remaining guilds were lower (Figure 4).
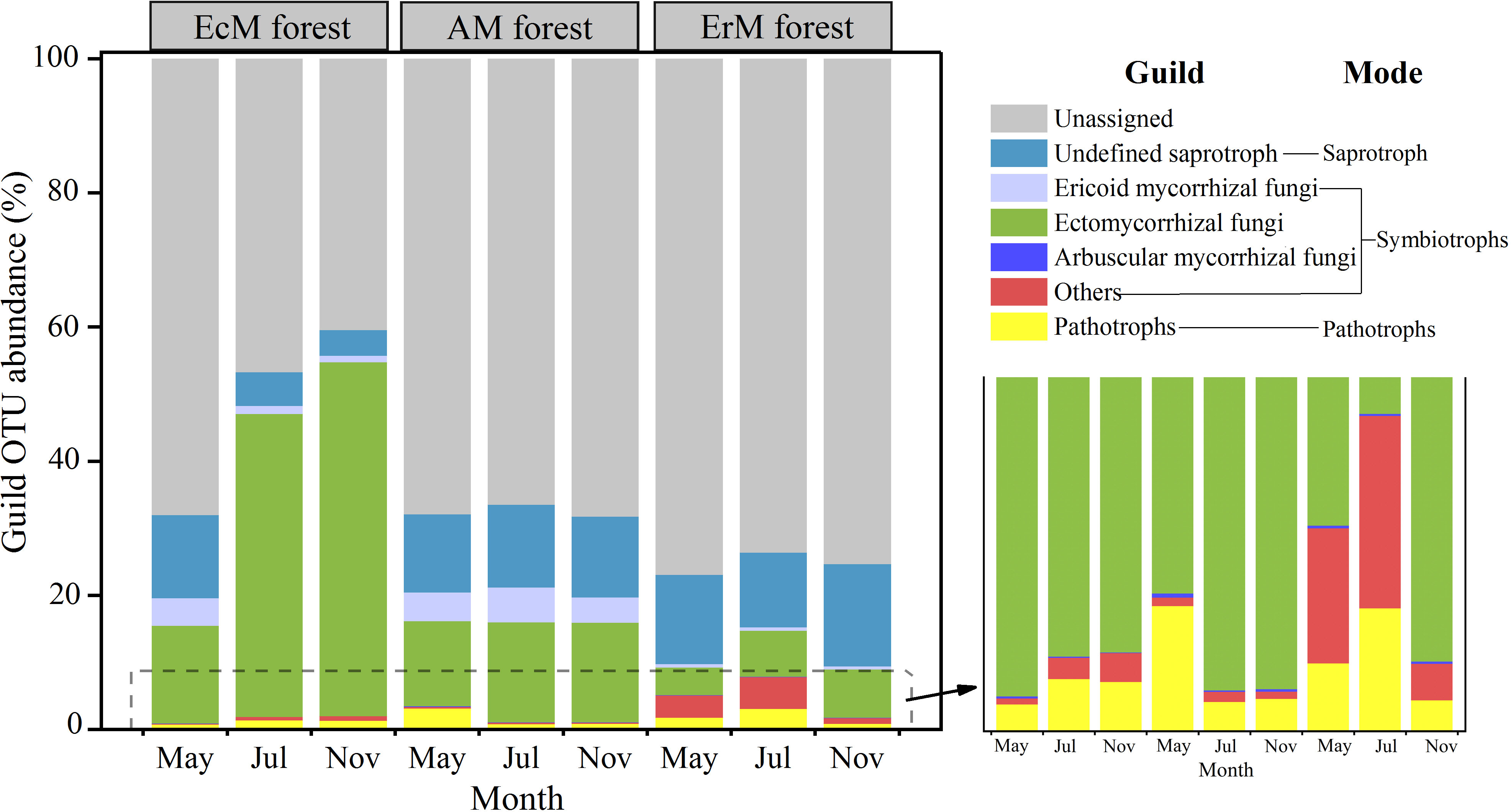
Figure 4 Composition of soil fungal functional guilds among three mycorrhizal forests in each different sampling month. EcM forest, Abies fargesii var. faxoniana primary forest; AM forest, Cupressus chengiana primary forest; ErM forest, Rhododendron phaeochrysum primary forest. ‘Others’ include endophyte and lichenized. Pathotrophs include animal pathogen, plant pathogen and mycoparasites. The lower right corner is a partial enlargement.
Soil PEX and POX activity each differed significantly among three mycorrhizal forests in each month (P < 0.05). Their activity in AM forest and EcM forest peaked in July, while those in ErM forest peaked in November (Figure 5). In general, soil mean PEX activity of EcM forest (41.04 μmol g-1 h-1) was significantly higher than the other two forests (P < 0.05) (Figure 5A). Soil mean POX activity in ErM forest (18.10 μmol g-1 h-1) was significantly lower than in EcM forest (23.13 μmol g-1 h-1) and AM forest (22.46 μmolg-1 h-1) (P < 0.05) (Figure 5B).
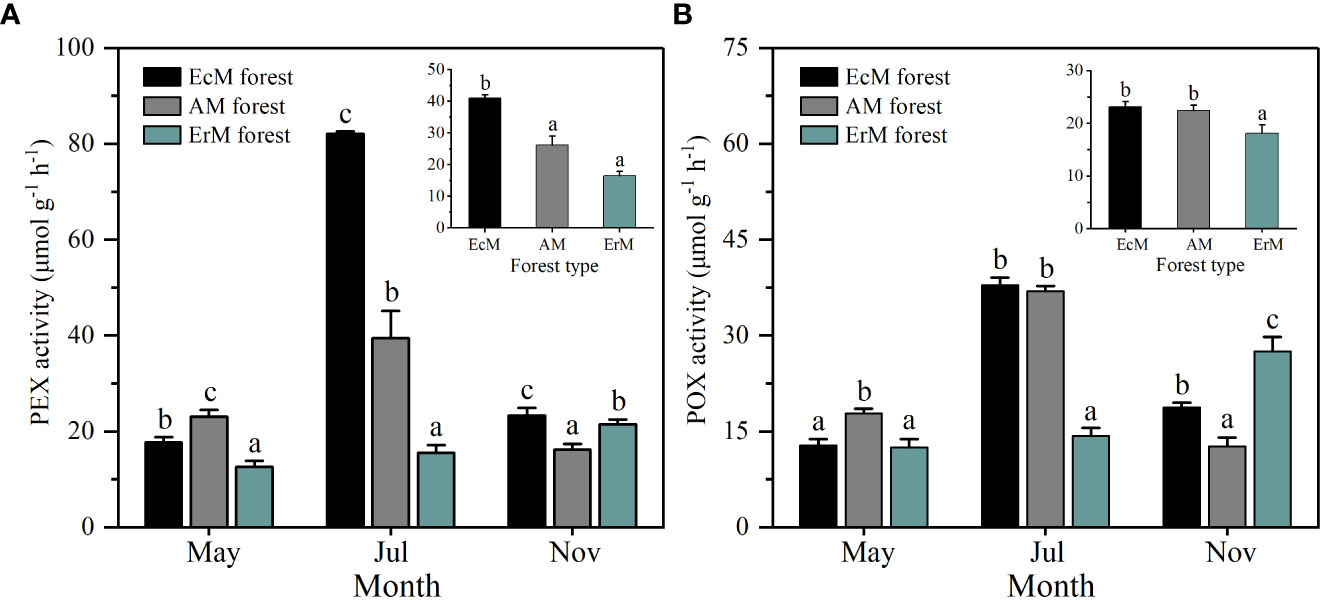
Figure 5 Soil PEX activity (A) and soil POX activity (B) among three mycorrhizal forests in each different sampling month (lower panel) and means (upper panel). Data and error bars are the means and standard errors respectively, n=8. EcM forest, Abies fargesii var. faxoniana primary forest; AM forest, Cupressus chengiana primary forest; ErM forest, Rhododendron phaeochrysum primary forest. PEX, peroxidase; POX, phenol oxidase. Lowercase letters (a, b and c) indicate significant differences among three mycorrhizal forests (P < 0.05).
Main factors affecting on soil net N mineralization
The results showed that soil net Nmin of the three mycorrhizal forests were affected differently by soil temperature and soil WFPS. Soil temperature and soil WFPS had no significant differences on soil net Rn in EcM forest, but soil net Ra in EcM forest was positively correlated with soil WFPS. Both soil net Ra and net Rn in AM forest were positively correlated with soil temperature but negatively correlated with soil WFPS. In contrast, soil net Ra and soil net Rn in ErM forest were positively correlated with soil WFPS (Table 2).
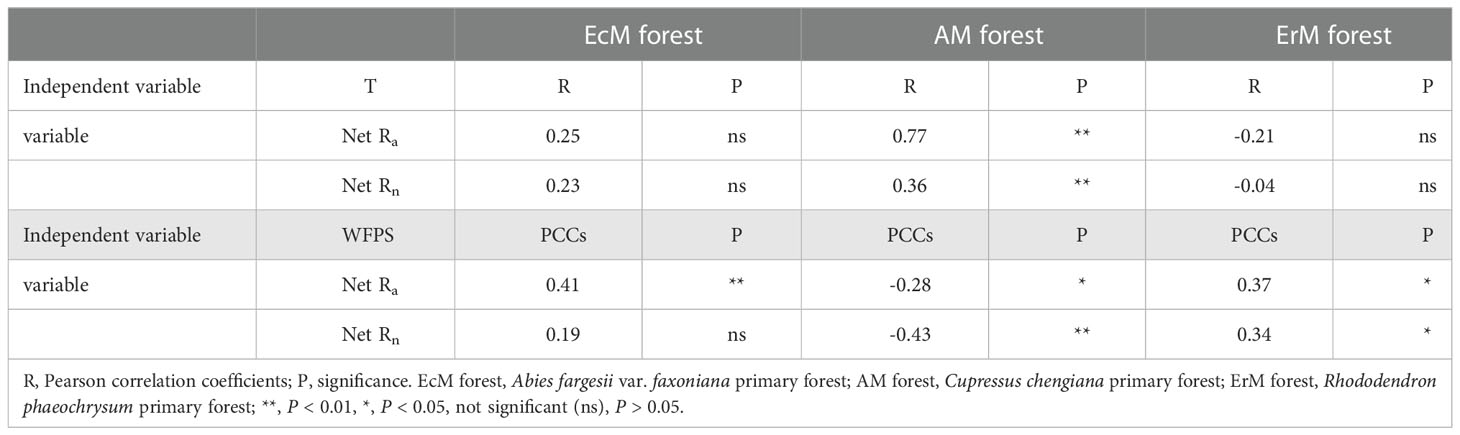
Table 2 Correlations between soil net ammonification rate (net Ra), soil net nitrification rate (net Rn) and microclimate: soil temperature (T) and soil WFPS (water-filled pore space).
Further research revealed that the difference in soil net Ra among three mycorrhizal forests was closely related to their soil environmental factors, soil PEX activity and soil fungal functional guilds (Figures S2, 6, 7). SEM analysis illustrated that soil temperature, the relative abundances of EcM fungi and ErM fungi had not only direct but also indirect effects on differential soil net Ra among three mycorrhizal forests. Similarly, SAP also indirectly affected the difference of soil net Ra among three mycorrhizal forests by regulating soil PEX activity (Figures 6A, 7A).
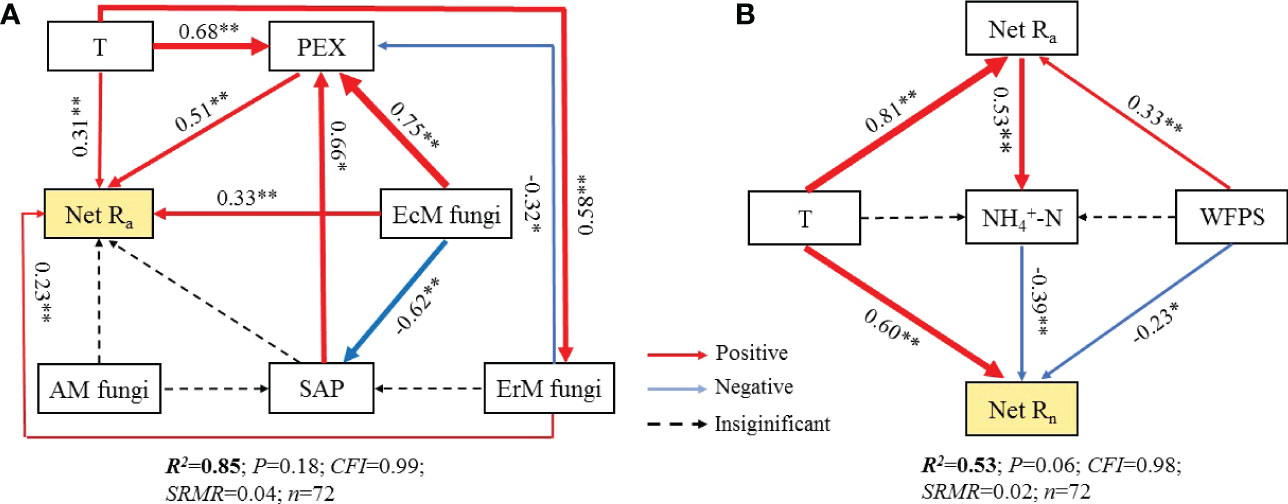
Figure 6 Structural equation models (SEM) analysis depicting the effects of soil PEX (peroxidase) activity, soil fungal functional guilds and soil environment key factors on soil net ammonification rate (Net Ra) (A) and soil net nitrification rate (Net Rn) (B). T, temperature; WFPS, water-filled pore space. EcM fungi, ectomycorrhizal fungi; AM fungi, arbuscular mycorrhizal fungi; ErM fungi, ericoid mycorrhizal fungi; SAP, undefined saprotroph.
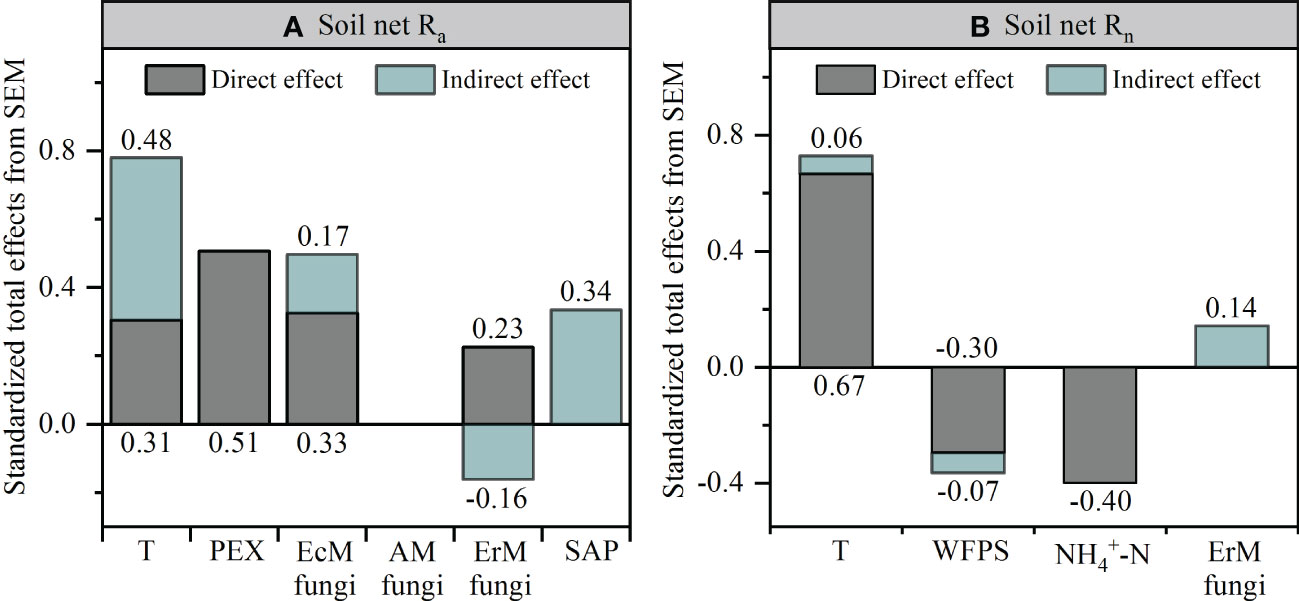
Figure 7 Standardized total effects of each variable on the soil net ammonification rate (Net Ra) (A) and soil net nitrification rate (Net Rn) (B) from the structural equation modeling (SEM) analysis. T, temperature; PEX, peroxidase; WFPS, water-filled pore space; EcM fungi, ectomycorrhizal fungi; AM fungi, arbuscular mycorrhizal fungi; ErM fungi, ericoid mycorrhizal fungi; SAP, undefined saprotroph.
Different from soil Net Ra, soil environmental factors largely drove the difference found in soil Net Rn among three mycorrhizal forests. Soil temperature had a positive effect on the difference in soil net Rn among three mycorrhizal forests, while soil WFPS and soil content had negative effect on it. We also found evidence of an indirect effect of soil net Ra, by changing the soil content, on the difference in soil net Rn among three forests (Figures 6B, 7B).
Discussion
Seasonal patterns of soil net N mineralization
There were significant differences in seasonal variations of soil net Nmin among three mycorrhizal forests in our research (Figures 2A-C). Temporal variation in forest soil net Nmin was also suggested in previous studies (Zhao and Li, 2017; Xiao et al., 2022). Overall, our results indicated that soil mean net Ra and soil mean net Rn were positive in EcM forest and AM forest, while there were negative in ErM forest (Figures 2D-F). This implied the net production of and was dominant in EcM forest and AM forest, while the net consumption of and was dominant in ErM forest. This could be explained by the soil microbial in ErM forest absorbing more and to maintain the growth and its reproduction of the populations (Miller et al., 2009; Liu et al., 2021).
In our study, soil net Ra and soil net Rn in AM forest decreased gradually after July and June respectively (Figures 2A, B). This might be due to the decrease of soil temperature and increase in soil WFPS (Figure 1) which could inhibit soil microbial activity, slowing down soil net Ra and soil net Rn in AM forest (Guntiñas et al., 2012; Hishi et al., 2014). Simultaneously, our findings revealed that the effect of soil temperature on soil net ammonification rate was stronger than that of soil WFPS, while soil WFPS had a stronger effect on soil net nitrification rate than soil temperature in AM forest (Table 2). In contrast to AM forest, soil net Ra and soil net Rn in EcM forest and ErM forest showed trends of increasing in late autumn (Figures 2A, B). This was because soil net Ra and soil net Rn in EcM and ErM forests were not determined significantly by soil temperature. Increased soil WFPS promoted both soil net Ra in EcM forest and soil net Ra and soil net Rn in ErM forest (Table 2) which was consistent with previous study (Kou et al., 2018). This might be related to differing ecological tolerance strategies of soil microorganisms to soil temperature and soil WFPS among three mycorrhizal forests (Castro et al., 2010; Placell et al., 2012).
Spatial (three mycorrhizal forests) effects on soil net N mineralization
Our results showed that ErM forest had the lowest soil net Ra and there was no difference between EcM forest and AM forest (Figure 2D). This might be due to a synergistic effect between soil temperature and different mycorrhizal plants (Tedersoo et al., 2014; Liu et al., 2017; Netherway et al., 2021). In our study, EcM forest had the highest relative abundance of EcM fungi, while the relative abundance of saprophytic fungi was lower (Figure 4). It had been confirmed that the relative abundance of soil EcM fungi increased with the abundance of EcM trees (Cheeke et al., 2017). Previous studies showed that host trees specificity could shape the relative abundances of soil fungi through co-evolution, niche differentiation, and niche conservatism (Tedersoo et al., 2013). EcM fungi have the mutualistic benefits with EcM trees (Velmala et al., 2013), which may be one of the reasons for the relatively high abundance of EcM fungi we found in EcM forest. Moreover, being the main functional groups of soil, EcM and saprophytic fungi would compete for SOC and N, and the strong competitiveness of EcM would limit the relative abundance of saprophytic fungi within the same ecological niche (Chen et al., 2022). Indeed, our results also showed that the relative abundances of soil AM fungi and saprotrophs in AM forests were higher than those in EcM forest and ErM forest (Figure 4), perhaps also due to the fact that the faster nutrient cycling pattern of AM forest (lower C: N, higher inorganic N content) was more suitable for the survival of AM fungi and saprophytic fungi (Bahram et al., 2020).
Our SEM results indicated that soil PEX activity has the strongest direct effect on soil net Ra (Figures 6A, 7A). Previous studies showed that soil EcM fungi and SAP could secret PEX to degrade recalcitrant organic matter which could not be absorbed by plants and promote soil net Ra (Bödeker et al., 2014; Corrales et al., 2016; Ward et al., 2021). However, our results showed that ErM fungi were negatively correlated with soil PEX activity (Figure 6A) which might be due to microbial C limitation when soil C: N was low (Midgley and Phillips, 2014; Xu et al., 2022). As such, ErM fungi would reduce the energy available for synthase soil PEX (Carrara et al., 2018). At the same time, soil EcM fungi was superior to SAP in degrading soil organic N (Bahram et al., 2020). EcM fungi could compete with SAP to inhibit the relative abundance of SAP and thus affected soil net Ra (Bödeker et al., 2016; Argiroff et al., 2022). In addition, EcM fungi and ErM fungi could directly acquire low molecular weight organic matter (Read and Perez-Moreno, 2003). This should promote soil net Ra by reducing the products of decomposition processes in the first step of ammoniation (Levy-Booth et al., 2014). The SEM also revealed that soil temperature not only directly enhanced soil net Ra, but also had a strong indirect effect (Figures 6A, 7A). This principally arose via soil temperature which could affect soil PEX activity and the relative abundance of soil ErM fungi, thus affected soil net Ra (Tedersoo and Bahram, 2019; Fan et al., 2021).
Different from soil net Ra, the SEM suggested that the difference of soil net Rn among three mycorrhizal forests was mainly determined by soil environmental factors (Figures 6B, 7B). On the one hand, soil temperature was positively correlated with soil net Rn, but negatively correlated with soil WFPS, a pattern consistent with other research findings (Borken and Matzner, 2009; Liu et al., 2017). On the other hand, soil temperature and WFPS promoted soil net Ra to produce more which affected soil net Rn. More recent studies also showed that soil as a substrate would accelerate soil net Rn (Elrys et al., 2021). Yet we found that soil content was negatively correlated with soil net Rn (Figure 6B). This discrepancy may be related to the net dynamics of soil being dependent upon substrate content, as well as being affected by microbial absorption, N-fixation, and N-loss (Gao et al., 2015; Xiao et al., 2022).
In this study, SEM indicated that soil environmental factors and soil net Ra explained 53.7% of the difference of soil net Rn among three mycorrhizal forests (Figure 6B). However, soil fungal functional guilds had no effect on soil net Rn (Figure 7B). This might be due to soil net Rn was driven primarily by soil bacteria (Levy-Booth et al., 2014). Our results showed that AM forest soil also characterized by a high pH and a low C: N ratio (Table 1), which implied AM forest had a fast nutrient cycling (Phillips et al., 2013; Lin et al., 2017). It was reported that AM forest soil with less acidity (high pH) supported higher heterotrophic bacterial activity and greater potential for nitrification (Mushinski et al., 2020). Further, the nitrification pathway of bacteria was superior to that of fungi in soil with a low C: N ratio (Deng et al., 2018). In addition, AM forest had a higher ratio of bacteria to fungi than EcM forest and ErM forest, which also suggested that AM forest was more inclined to have soil net Rn driven by bacteria rather than fungi (Bahram et al., 2020).
Conclusions
In this study, differences in soil net Nmin, soil fungal composition and soil enzyme activity among three mycorrhizal forests were highlighted. Notably, there were significant differences in soil net Ra and soil net Rn among three mycorrhizal forests. Further, soil temperature and soil WFPS differed in their effect on soil net Ra and soil net Rn in these three mycorrhizal forests. In response to the forest types, the difference in soil net Ra was determined mainly by soil temperature, soil fungal functional guilds and soil PEX activity. The difference in soil net Rn was related closely to soil temperature, soil WFPS and soil content. Soil net Ra dominated the soil net Nmin among three mycorrhizal forests. Overall, our results provide new insights into the mechanism of soil N dynamics in various mycorrhizal forests.
Data availability statement
The raw data supporting the conclusions of this article will be made available by the authors, without undue reservation.
Author contributions
MZ: investigation, data analysis, writing-original draft preparation, writing-review & editing. SL: methodology, investigation, writing-review & editing. XC: participated in the experiment, data analysis. MC: participated in the experiment, data analysis. JC: participated in the experiment, visualization. GX: Methodology. ZS: conceptualization, methodology, writing-review & editing. All authors contributed to the article and approved the submitted version.
Funding
This work was supported by the Fundamental Research Funds of Chinese Academy of Forestry (CAFYBB2018ZA003, CAFYBB2021ZA002-2) and the National Key Research and Development Program of China (2021YFD2200405).
Conflict of interest
The authors declare that the research was conducted in the absence of any commercial or financial relationships that could be construed as a potential conflict of interest.
Publisher’s note
All claims expressed in this article are solely those of the authors and do not necessarily represent those of their affiliated organizations, or those of the publisher, the editors and the reviewers. Any product that may be evaluated in this article, or claim that may be made by its manufacturer, is not guaranteed or endorsed by the publisher.
Supplementary material
The Supplementary Material for this article can be found online at: https://www.frontiersin.org/articles/10.3389/fpls.2022.1069730/full#supplementary-material
Supplementary Figure 1 | Rarefaction curve showing the sequence depth and observed OTUs. Note: EcM forest, Abies fargesii var. faxoniana primary forest; AM forest, Cupressuschengiana primary forest; ErM forest, Rhododendron phaeochrysum primary forest; -5, -7 and -11 represented May, July and November respectively.
Supplementary Figure 2 | Correlations between the difference in soil net N mineralization rate, biological and abiotic factors. Note: PEX, peroxidase; POX, phenol oxidase; T, temperature; WFPS, water-filled pore space; AM fungi, arbuscular mycorrhizal fungi; EcM fungi, ectomycorrhizal fungi; ErM fungi, ericoid mycorrhizal fungi; SAP, undefined saprotroph; ‘Others’ include endophyte and lichenized. Pathotrophs include animal pathogen, plant pathogen and mycoparasites; Net Ra, net ammonification rate; Net Rn, net nitrification rate; **, P< 0.01; *, P< 0.05; not significant (ns), P > 0.05.
References
Adamczyk, B., Ahvenainen, A., Sietiö, O. M., Kanerva, S., Kieloaho, A. J., Smolander, A., et al. (2016). The contribution of ericoid plants to soil nitrogen chemistry and organic matter decomposition in boreal forest soil. Soil Biol. Biochem. 103, 394–404. doi: 10.1016/j.soilbio.2016.09.016
Argiroff, W. A., Zak, D. R., Pellitier, P. T., Upchurch, R. A., Belke, J. P. (2022). Decay by ectomycorrhizal fungi couples soil organic matter to nitrogen availability. Ecol. Lett. 25, 391–404. doi: 10.1111/ele.13923
Averill, C., Turner, B. L., Finzi, A. C. (2014). Mycorrhiza-mediated competition between plants and decomposers drives soil carbon storage. Nature 505, 543–545. doi: 10.1038/nature12901
Babalola, B. J., Li, J., Willing, C. E., Zheng, Y., Wang, Y. L., Gan, H. Y., et al. (2022). Nitrogen fertilisation disrupts the temporal dynamics of arbuscular mycorrhizal fungal hyphae but not spore density and community composition in a wheat field. New Phytol. 234, 2057–2072. doi: 10.1111/nph.18043
Bahram, M., Netherway, T., Hildebrand, F., Pritsch, K., Drenkhan, R., Loit, K., et al. (2020). Plant nutrient-acquisition strategies drive topsoil microbiome structure and function. New Phytol. 227, 1189–1199. doi: 10.1111/nph.16598
Becker, H., Uri, V., Aosaar, J., Varik, M., Soosaar, K., Hansen, R., et al. (2015). The effects of clear-cut on net nitrogen mineralization and nitrogen losses in a grey alder stand. Ecol. Eng. 85, 237–246. doi: 10.1016/j.ecoleng.2015.10.006
Bödeker, I. T. M., Björn, D. L., Olson, Å., Clemmensen, K. E. (2016). Mycorrhizal and saprotrophic fungal guilds compete for the same organic substrates but affect decomposition differently. Funct. Ecol. 30, 1967–1978. doi: 10.1111/1365-2435.12677
Bödeker, I. T. M., Clemmensen, K. E., Boer, W., Martin, F., Olson, Å., Lindahl, B. D. (2014). Ectomycorrhizal cortinarius species participate in enzymatic oxidation of humus in northern forest ecosystems. New Phytol. 203, 245–256. doi: 10.1111/nph.12791
Borken, W., Matzner, E. (2009). Reappraisal of drying and wetting effects on c and n mineralization and fluxes in soils. Global Change Biol. 15, 808–824. doi: 10.1111/j.1365-2486.2008.01681.x
Carrara, J. E., Walter, C. A., Hawkins, J. S., Peterjohn, W. T., Averill, C., Brzostek, E. R. (2018). Interactions among plants, bacteria, and fungi reduce extracellular enzyme activities under long-term n fertilization. Global Change Biol. 24, 2721–2734. doi: 10.1111/gcb.14081
Castro, H. F., Classen, A. T., Austin, E. E., Norby, R. J., Schadt, C. W. (2010). Soil microbial communityresponses to multiple experimental climate change drivers. Appl. Environ. Microbiol. 76, 999–1007. doi: 10.1128/AEM.02874-09
Cheeke, T. E., Phillips, R. P., Brzostek, E. R., Rosling, A., Bever, J. D., Fransson, P. (2017). Dominant mycorrhizal association of trees alters carbon and nutrient cycling by selecting for microbial groups with distinct enzyme function. New Phytol. 214, 432–442. doi: 10.1111/nph.14343
Chen, H. H. (2019). Forest community diversity, carbon and nitrogen cycling modes and their relationships with mycorrhizal fungi in the subalpine area of western sichuan. dissertation (Chinese Academy of Forestry). doi: 10.27625/d.cnki.gzlky.2019.000062
Cheng, L., Booker, F. L., Tu, C., Burkey, K. O., Zhou, L. S., Shew, H. D., et al. (2012). Arbuscular mycorrhizal fungi increase organic carbon decomposition under elevated CO2. Science 337, 1084–1087. doi: 10.1126/science.1224304
Chen, J., Shi, Z. M., Liu, S., Zhang, M. M., Cao, X. W., Chen, M., et al. (2022). Altitudinal variation influences soil fungal community composition and diversity in alpine-gorge region on the eastern qinghai-Tibetan plateau. J. Fungi. 8, 807. doi: 10.3390/jof8080807
Cole, L., Buckland, S. M., Bardgett, R. D. (2008). Influence of disturbance and nitrogen addition on plant and soil animal diversity in grassland. Soil Biol. Biochem. 40, 505–514. doi: 10.1016/j.soilbio.2007.09.018
Corrales, A., Mangan, S. A., Turner, B. L., Dalling, J. W. (2016). An ectomycorrhizal nitrogen economy facilitates monodominance in a neotropical forest. Ecol. Lett. 19, 383–392. doi: 10.1111/ele.12570
Deng, M. F., Liu, L. L., Jiang, L., Liu, W. X., Wang, X., Li, S. P., et al. (2018). Ecosystem scale trade-off in nitrogen acquisition pathways. Nat. Ecol. Evol. 2, 1724–1734. doi: 10.1038/s41559-018-0677-1
Dong, H. Y., Ge, J. F., Sun, K., Wang, B. Z., Xue, J. M., Wakelin, S. A., et al. (2021). Change in root-associated fungal communities affects soil enzymatic activities during pinus massoniana forest development in subtropical China. For. Ecol. Management. 482, 118817. doi: 10.1016/j.foreco.2020.118817
Du, T., Liu, Y. L., Yang, Y. T., Zhang, Y., You, C. M., Zhang, L., et al. (2021). Effects of forest gaps on cellulose degradation during foliar litter decomposition in a subalpine forest of western sichuan. Chin. J. Appl. Environ. Biol. 27, 617–624. doi: 10.19675/j.cnki.1006-687x.2021.02003
Edgar, R. C. (2016). SINTAX: a simple non-Bayesian taxonomy classifier for 16S and ITS sequences (bioRxiv). doi: 10.1101/074161
Elrys, A. S., Wang, J., Metwally, M. A. S., Cheng, Y., Zhang, J. B., Cai, Z. C., et al. (2021). Global gross nitrification rates are dominantly driven by soil carbon-to-nitrogen stoichiometry and total nitrogen. Global Change Biol. 27, 6512–6524. doi: 10.1111/GCB.15883
Fan, L., Tarin, M. W. K., Zhang, Y. Y., Han, Y. Z., Rong, J. D., Cai, X. H., et al. (2021). Patterns of soil microorganisms and enzymatic activities of various forest types in coastal sandy land. Global Ecol. Conserv. 28, e01625. doi: 10.1016/J.GECCO.2021.E01625
Feng, Q. H., Shi, Z. M., Xu, Z. J. R., Miao, N., Tang, J. C., Liu, X. L., et al. (2017). Phenotypic variations in cones and seeds of natural Cupressus chengiana populations in China. Chin. J. Appl. Ecol. 28, 748–756. doi: 10.13287/j.1001-9332.201703.001
Gao, W. L., Zhao, W., Yang, H., Yang, H. J., Chen, G. Q., Luo, Y. C., et al. (2015). Effects of nitrogen addition on soil inorganic n content and soil n mineralization of a cold-temperate coniferous forest in great xing’an mountains. Acta Ecol. Sin. 35, 130–136. doi: 10.1016/j.chnaes.2015.07.003
Guntiñas, M. E., Leirós, M. C., Trasar-Cepeda, C., Gil-Sotres, F. (2012). Effects of moisture and temperature on net soil nitrogenmineralization: A laboratory study. Eur. J. Soil Biol. 48, 73–80. doi: 10.1016/j.ejsobi.2011.07.015
Han, Y., Feng, J., Han, M., Zhu, B. (2020). Responses of arbuscular mycorrhizal fungi to nitrogen addition: a meta-analysis. Global Change Biol. 26, 7229–7241. doi: 10.1111/gcb.15369
Hishi, T., Urakawa, R., Tashiro, N., Maeda, Y., Shibata, H. (2014). Seasonality of factors controlling n mineralization rates among slope positions and aspects in cool-temperate deciduous natural forests and larch plantations. Biol. Fertil. Soils. 50, 343–356. doi: 10.1007/s00374-013-0863-x
Hobbie, E. A., Högberg, P. (2012). Nitrogen isotopes link mycorrhizal fungi and plants to nitrogen dynamics. New Phytol. 196, 367–382. doi: 10.1111/j.1469-8137.2012.04300.x
Idol, T. W., Pope, P. E., Ponder, F. (2003). N mineralization, nitrification, and n uptake across a 100-year chronosequence of upland hardwood forests. For. Ecol. Management. 176, 509–518. doi: 10.1016/S0378-1127(02)00232-3
Jian, S. Y., Li, J. W., Chen, J., Wang, G. S., Mayes, M. A., Dzantor, K. E., et al. (2016). Soil extracellular enzyme activities, soil carbon and nitrogen storage under nitrogen fertilization: A meta-analysis. Soil Biol. Biochem. 101, 32–43. doi: 10.1016/j.soilbio.2016.07.003
Johnson, N. C. (2010). Resource stoichiometry elucidates the structure and function of arbuscular mycorrhizas across scales. New Phytol. 185, 631–647. doi: 10.1111/j.1469-8137.2009.03110.x
Kou, D., Peng, Y. F., Wang, G. Q., Ding, J. Z., Chen, Y. L., Yang, G. B., et al. (2018). Diverse responses of belowground internal nitrogen cycling to increasing aridity. Soil Biol. Biochem. 116, 189–192. doi: 10.1016/j.soilbio.2017.10.010
Levy-Booth, D. J., Prescott, C. E., Grayston, S. J. (2014). Microbial functional genes involved in nitrogen fixation, nitrification and denitrification in forest ecosystems. Soil Biol. Biochem. 75, 11–25. doi: 10.1016/j.soilbio.2014.03.021
Lin, G. G., Mccormack, M. L., Ma, C., Guo, D. L. (2017). Similar below-ground carbon cycling dynamics but contrasting modes of nitrogen cycling between arbuscular mycorrhizal and ectomycorrhizal forests. New Phytol. 213, 1440–1451. doi: 10.1111/nph.14206
Li, Z. L., Tian, D. S., Wang, B. X., Wang, J. S., Wang, S., Chen, H. Y. H., et al. (2019). Microbes drive global soil nitrogen mineralization and availability. Global Change Biol. 25, 1078–1088. doi: 10.1111/gcb.14557
Liu, Y. (2010). Patterns of above-and belowground biodiversity of alpine timberline ecotone and forest-meadow ecotone in Western sichuan. dissertation (Sichuan Agricultural University).
Liu, S., Luo, D., Cheng, R. M., Wu, J. M., Yang, H. G., Shi, Z. M. (2021). Temporal variability in soil net nitrogen mineralization among forest regeneration patterns in eastern Tibetan plateau. Ecol. Indicators. 128, 107811. doi: 10.1016/j.ecolind.2021.107811
Liu, S., Luo, D., Cheng, R. M., Yang, H. G., Wu, J. M., Shi, Z. M. (2020). Soil-atmosphere exchange of greenhouse gases from typical subalpine forests on the eastern qinghai-Tibetan plateau: effects of forest regeneration patterns. Land. Degrad. Dev. 31, 2019–2032. doi: 10.1002/ldr.3586
Liu, Y., Wang, C. H., He, N. P., Wen, X. F., Gao, Y., Li, S. G., et al. (2017). A global synthesis of the rate and temperature sensitivity of soil nitrogen mineralization: latitudinal patterns and mechanisms. Global Change Biol. 23, 455–464. doi: 10.1111/gcb.13372
Midgley, M. G., Phillips, R. P. (2014). Microbial limitation in a changing world: A stoichiometric approach for predicting microbial resource limitation and fluxes (AGU Fall Meeting Abstracts), B51B–0017.
Midgley, M. G., Phillips, R. P. (2016). Resource stoichiometry and the biogeochemical consequences of nitrogen deposition in a mixed deciduous forest. Ecology 97, 3369–3378. doi: 10.1002/ecy.1595
Miller, A. ,. E., Schimel, J. P., Sickman, J. O., Skeen, K., Meixner, T., Melack, J. M. (2009). Seasonal variation in nitrogen uptake and turnover in two high-elevation soils: mineralization responses are site-dependent. Biogeochemistry 93, 253–270. doi: 10.1007/s10533-009-9301-4
Mushinski, R. M., Payne, Z. C., Raff, J. D., Craig, M. E., Pusede, S. E., Rusch, D. B., et al. (2020). Nitrogen cycling microbiomes are structured by plant mycorrhizal associations with consequences for nitrogen oxide fluxes in forests. Global Change Biol. 27, 1068–1082. doi: 10.1111/GCB.15439
Netherway, T., Bengtsson, J., Krab, E. J., Bahram, M. (2021). Biotic interactions with mycorrhizal systems as extended nutrient acquisition strategies shaping forest soil communities and functions. Basic. Appl. Ecol. 50, 25–42. doi: 10.1016/j.baae.2020.10.002
Nguyen, N. H., Song, Z. W., Bates, S. T., Branco, S., Tedersoo, L., Menke, J., et al. (2016). FUNGuild: An open annotation tool for parsing fungal community datasets by ecological guild. Fungal Ecol. 20, 241–248. doi: 10.1016/j.funeco.2015.06.006
Ni, Y. Y., Jian, Z. J., Zeng, L. X., Liu, J. F., Lei, L., Zhu, J. H., et al. (2022). Climate, soil nutrients, and stand characteristics jointly determine large-scale patterns of biomass growth rates and allocation in Pinus massoniana plantations. For. Ecol. Management. 504, 119839. doi: 10.1016/J.FORECO.2021.119839
Orwin, K. H., Kirschbaum, M. U. F., St, John., M. G., Dickie, L. A. (2011). Organic nutrient uptake by mycorrhizal fungi enhances ecosystem carbon storage: a model-based assessment. Ecol. Lett. 14, 493–502. doi: 10.1111/j.1461-0248.2011.01611.x
Pfennigwerth, A. A., Nuland, M. E. V., Bailey, J. K., Schweitzer, J. A. (2018). Plant-soil feedbacks mediate shrub expansion in declining forests, but only in the right light. J. Ecol. 106, 179–194. doi: 10.1111/1365-2745.12833
Phillips, R. P., Brzostek, E., Midgley, M. G. (2013). The mycorrhizal-associated nutrient economy: a new framework for predicting carbon-nutrient couplings in temperate forests. New Phytol. 199, 41–51. doi: 10.1111/nph.12221
Placell, S. A., Brodie, E. L., Firestone, M. K. (2012). Rainfall-induced carbon dioxide pulses result from sequential resuscitation of phylogenetically clustered microbial groups. Proc. Natl. Acad. Sci. United. States America. 109, 10931–10936. doi: 10.1073/pnas.1204306109
Read, D. J., Perez-Moreno, J. (2003). Mycorrhizas and nutrient cycling in ecosystems-a journey towards relevance? New Phytol. 157, 475–492. doi: 10.1046/j.1469-8137.2003.00704.x
Saifuddin, M., Bhatnagar, J. M., Phillips, R. P., Finzi, A. C. (2021). Ectomycorrhizal fungi are associated with reduced nitrogen cycling rates in temperate forest soils without corresponding trends in bacterial functional groups. Oecologia 196, 863–875. doi: 10.1007/S00442-021-04966-Z
Soudzilovskaia, N. A., Vaessen, S., Barcelo, M., He, J. H., Rahimlou, S., Abarenkov, K., et al. (2020). FungalRoot: global online database of plant mycorrhizal associations. New Phytol. 227, 955–966. doi: 10.1111/nph.16569
Tedersoo, L., Bahram, M. (2019). Mycorrhizal types differ in ecophysiology and alter plant nutrition and soil processes. Biol. Rev. 94, 1857–1880. doi: 10.1111/brv.12538
Tedersoo, L., Bahram, M., Põlme, S., Kõljalg, U., Yorou, N. S., Wijesundera, R., et al. (2014). Global diversity and geography of soil fungi. Science 346, 1078–1078. doi: 10.1126/science.1256688
Tedersoo, L., Bahram, M., Zobel, M. (2020). How mycorrhizal associations drive plant population and community biology. Science 367, eaba1223. doi: 10.1126/science.aba1223
Tedersoo, L., Mett, M., Ishida, T. A., Bahram, M. (2013). Phylogenetic relationships among host plants explain differences in fungal species richness and community composition in ectomycorrhizal symbiosis. New Phytol. 199 (3), 822–831. doi: 10.1111/nph.12328
Van der Heijden, M. G. A., Martin, F. M., Selosse, M. A., Sanders, I. R. (2015). Mycorrhizal ecology and evolution: the past, the present, and the future. New Phytol. 205, 1406–1423. doi: 10.1111/nph.13288
Velmala, S. M., Rajala, T., Haapanen, M., Taylor, A. F. S., Pennanen, T. (2013). Genetic host-tree effects on the ectomycorrhizal community and root characteristics of Norway spruce. Mycorrhiza 23, 2 1–233. doi: 10.1007/s00572-012-0446-y
Wang, P., Chen, Y. J., Sun, Y. T., Tan, S., Zhang, S. Y., Wang, Z. H., et al. (2019). Distinct biogeography of different fungal guilds and their associations with plant species richness in forest ecosystems. Front. Ecol. Evol. 7. doi: 10.3389/fevo.2019.00216
Wang, H., Liu, S. R., Mo, J. M., Zhang, T. (2010). Soil-atmosphere exchange of greenhouse gases in subtropical plantations of indigenous tree species. Plant Soil. 335, 213–227. doi: 10.1007/s11104-010-0408-0
Wang, H. L., Na, D., Wu, D. Y., Hu, S., Kou, M. (2017). Long-term net transformation and quantitative molecular mechanisms of soil nitrogen during natural vegetation recovery of abandoned farmland on the loess plateau of China. Sci. Total. Environ. 607-608, 152–159. doi: 10.1016/j.scitotenv.2017.07.014
Wang, G. X., Wang, T. B., Li, Y. S., Cheng, H. Y. (2007). Influences of alpine ecosystem responses to climatic change on soil properties on the qinghai-Tibet plateau, China. CATENA 70, 506–514. doi: 10.1016/j.catena.2007.01.001
Wang, Y. L., Xu, Y., Maitra, P., Babalola, B. J., Zhao, Y. L. (2022). Temporal variations in root-associated fungal communities of potaninia mongolica, an endangered relict shrub species in the semi-arid desert of Northwest China. Front. Plant Sci. 13. doi: 10.3389/fpls.2022.975369
Ward, E. B., Duguid, M. C., Kuebbing, S. E., Lendemer, J. C., Warren, R. J., Bradford, M. A. (2021). Ericoid mycorrhizal shrubs alter the relationship between tree mycorrhizal dominance and soil carbon and nitrogen. J. Ecol. 109, 3524–3540. doi: 10.1111/1365-2745.13734
Wurzburger, N., Hendrick, R. L. (2009). Plant litter chemistry and mycorrhizal roots promote a nitrogen feedback in a temperate forest. J. Ecol. 97, 528–536. doi: 10.1111/j.1365-2745.2009.01487.x
Xiao, R. H., Man, X. L., Duan, B. X., Cai, T. J., Ge, Z. X., Li, X. F., et al. (2022). Changes in soil bacterial communities and nitrogen mineralization with understory vegetation in boreal larch forests. Soil Biol. Biochem. 166, 108572. doi: 10.1016/J.SOILBIO.2022.108572
Xiong, C., Singh, B. K., He, J. Z., Han, Y. L., Li, P. P., Wan, L. H., et al. (2021). Plant developmental stage drives the differentiation in ecological role of plant bacterial and fungal microbiomes. Microbiome 9, 171. doi: 10.21203/rs.3.rs-241147/v1
Xu, G. X., Chen, H. H., Shi, Z. M., Liu, S., Cao, X. W., Zhang, M. M., et al. (2021). Mycorrhizal and rhizospheric fungal community assembly differs during subalpine forest restoration on the eastern qinghai-Tibetan plateau. Plant Soil. 458, 245–259. doi: 10.1007/s11104-019-04400-7
Xu, H. W., Qu, Q., Li, G. W., Liu, G. B., Geissen, V., Ritsema, C. J., et al. (2022). Impact of nitrogen addition on plant-soil-enzyme c-N-P stoichiometry and microbial nutrient limitation. Soil Biol. Biochem. 170, 108714. doi: 10.1016/J.SOILBIO.2022.108714
Yao, H., Sun, X., He, C., Maitra, P., Li, X. C., Guo, L. D. (2019). Phyllosphere epiphytic and endophytic fungal community and network structures differ in a tropical mangrove ecosystem. Microbiome 7, 57. doi: 10.1186/s40168-019-0671-0
Zeilinger, S., Gupta, V. K., Dahms, T. E. S., Silva, R. N., Singh, H. B., Upadhyay, R. S., et al. (2016). Friends or foes? emerging insights from fungal interactions with plants. FEMS Microbiol. Rev. 40, 182–207. doi: 10.1093/femsre/fuv045
Zhang, Z. L., Li, N., Xiao, J., Zhao, C. Z., Zou, T. T., Li, D. D., et al. (2018). Changes in plant nitrogen acquisition strategies during the restoration of spruce plantations on the eastern Tibetan plateau, China. Soil Biol. Biochem. 119, 50–58. doi: 10.1016/j.soilbio.2018.01.002
Zhao, N. N., Li, X. G. (2017). Effects of aspect-vegetation complex on soil nitrogen mineralization and microbial activity on the Tibetan plateau. Catena 155, 1–9. doi: 10.1016/j.catena.2017.02.025
Keywords: net N mineralization, mycorrhizal associations, soil fungi, soil enzymes, microclimate
Citation: Zhang M, Liu S, Cao X, Chen M, Chen J, Xu G and Shi Z (2023) The effects of ectomycorrhizal and saprotropic fungi on soil nitrogen mineralization differ from those of arbuscular and ericoid mycorrhizal fungi on the eastern Qinghai-Tibetan Plateau. Front. Plant Sci. 13:1069730. doi: 10.3389/fpls.2022.1069730
Received: 14 October 2022; Accepted: 07 December 2022;
Published: 04 January 2023.
Edited by:
Rodica Pena, University of Reading, United KingdomReviewed by:
Haibo Lu, Beijing Normal University, ChinaBusayo Joshua Babalola, University of Wisconsin–Milwaukee, United States
Copyright © 2023 Zhang, Liu, Cao, Chen, Chen, Xu and Shi. This is an open-access article distributed under the terms of the Creative Commons Attribution License (CC BY). The use, distribution or reproduction in other forums is permitted, provided the original author(s) and the copyright owner(s) are credited and that the original publication in this journal is cited, in accordance with accepted academic practice. No use, distribution or reproduction is permitted which does not comply with these terms.
*Correspondence: Zuomin Shi, shizm@caf.ac.cn