- 1The Laboratory of Immunogenetics, Department of Medical Research, MacKay Memorial Hospital, New Taipei City, Taiwan
- 2MacKay Junior College of Medicine, Nursing, and Management, New Taipei City, Taiwan
- 3Institute of Biomedical Sciences, MacKay Medical College, New Taipei City, Taiwan
Glycophorin A and glycophorin B are structural membrane glycoproteins bound in the band 3 multiprotein complexes on human red blood cells (RBCs). Band 3 is an erythroid-specific anion exchanger (AE1). AE1-mediated HCO3− transport provides the substrate for the enzyme-catalyzed conversion HCO3−(aq) ⇌ CO2(g), which takes place inside the RBCs. Bicarbonate transport via AE1 supports intravascular acid–base homeostasis and respiratory excretion of CO2. In the past decade, we conducted several comparative physiology studies on Taiwanese people having the glycophorin variant GPMur RBC type (which accompanies greater AE1 expression). We found that increased anion transport across the erythrocyte membrane not only enhances gas exchange and lung functions but also elevates blood pressure (BP) and reduces nitric oxide (NO)-dependent vasodilation and exhaled NO fraction (FeNO) in healthy individuals with GP.Mur. Notably, in people carrying the GPMur blood type, the BP and NO-dependent, flow-mediated vasodilation (FMD) are both more strongly correlated with individual hemoglobin (Hb) levels. As blood NO and nitrite (NO2−) are predominantly scavenged by intraerythrocytic Hb, and NO2− primarily enters RBCs via AE1, could a more monoanion-permeable RBC membrane (i.e., GPMur/increased AE1) enhance NO2−/NO3− permeability and Hb scavenging of NO2− and NO to affect blood pressure? In this perspective, a working model is proposed for the potential role of AE1 in intravascular NO availability, blood pressure, and clinical relevance.
Introduction
Hemoglobin (Hb) is the main scavenger of NO species in the mammalian vasculature (Lancaster, 1994; Patel et al., 2011). Band 3, an anion transporter with ∼ a million protein copies per human RBC, has been postulated to play a critical role in erythrocyte processing of NO species since late 1990s (Vaughn et al., 2000; Huang et al., 2001; Pawloski et al., 2001; Han et al., 2003; Kim-Shapiro et al., 2006; Jana et al., 2018; Premont et al., 2020). We came across this perplexing topic of research when we were conducting human exercise tests that examined the impacts of GP.Mur on respiration and unexpectedly found a significant association between GP.Mur and higher blood pressure. GP.Mur (a glycophorin B-A-B variant exclusively expressed on human RBCs) is a blood type unique to Southeast Asian (SEA) populations, with ∼4.7% prevalence in Taiwan and 2–23% in many areas of SEA, and is rare among non-SEA ethnic populations (e.g., Japanese and other ethnic groups of the Northeastern Asian origins) (Prathiba et al., 2002; Hsu et al., 2013; Wei et al., 2016; Jongruamklang et al., 2020; Yang et al., 2021; Kaito et al., 2022). The main molecular function of GP.Mur is to enhance AE1 protein expression on the RBC membrane (Hsu et al., 2009). Since the cytoplasmic domain of AE1 binds hemoglobin (preferentially deoxyHb) in the submembranous interface and deoxyHb also functions as a nitrite reductase that produces nitric oxide (Walder et al., 1984; Gladwin et al., 2009), we hypothesized that AE1 might be involved in regulation of blood pressure through intraerythrocytic Hb–NOx reactions.
Blood pressure and NO-dependent vasodilation are affected by GP.Mur/increased AE1
From the respiratory/exercise physiology studies conducted on healthy adults and professional athletes, we repeatedly observed a slightly but statistically significant higher systolic blood pressure (SBP) among those carrying the GP.Mur blood type (Hsu et al., 2015; Chen et al., 2022; Hsu et al., 2023). For verification, we collaborated with one of our health check-up centers located in Eastern Taiwan, where the GP.Mur+ population is four times higher than that in other regions of Taiwan (Chen et al., 2022). We selected 989 Eastern Taiwanese residents under 55 years of age (one-fifth of them were GP.Mur+) whose clinical laboratory data from the annual health check-up were within the normal ranges and who were not on any drug treatments, including antihypertensives. Among them, the non-diseased men and women with GP.Mur indeed presented 3–5 mmHg higher systolic blood pressure (SBP) than the negative control people (Chen et al., 2022). Intriguingly, in this study, the incidence of early-onset hypertension (BP > 130/80 mmHg in those ≤45 years old) is significantly higher among GP.Mur+ carriers. Young men and women under 45 years of age are 1.6-fold and 3.4-fold more likely to present hypertension than age-matched controls living in the same area. As early-onset hypertension is largely hereditary (Suvila et al., 2020), this type of early-onset hypertension found among GP.Mur+ carriers is unlikely to be mainly due to unhealthy lifestyles (“GP.Mur-unique hypertension”).
To test our hypothesis that AE1-Hb could contribute to “GP.Mur-unique hypertension” via AE1–Hb–NO reactions in RBCs, we examined the impacts of GP.Mur/more AE1 on NO-dependent vasodilation in male college athletes. We measured their flow-mediated vasodilation (FMD, reflecting NO-mediated vasodilation (Green et al., 2014)) and nitroglycerin-induced, NO-independent vasodilation (NID) in this homogeneous population. Ultrasound was used to track vasodilation or the degree of increase in the brachial arterial diameter of each subject during a period of stimulation and recovery (FMD was stimulated by 5-minute vasoconstriction and NID by sublingual nitroglycerin). While NID in GP.Mur+ and the control athletes was not different, FMD measured in the GP.Mur+ athletes was significantly lower than FMD measured in the control athletes (4.6% ± 2.3% [GP.Mur] versus 6.3% ± 2.4% [control]) (Hsu et al., 2021).
Stronger dependence of FMD and BP on Hb levels in GP.Mur+ people
From a seminal study on Andean high-altitude dwellers with excessive erythrocytosis (EE), a significantly inverse correlation was found between Hb and NO-dependent vasodilation (%FMD) in EE patients (Tremblay et al., 2019). In these GP.Mur+ athletes, we also observed a significantly inverse correlation between Hb and %FMD (Figure 1B: the correlation is shown as the solid line); this correlation was not found in the control athletes (Figure 1B: shown as a dotted line as the correlation was not statistically significant) (Hsu et al., 2021). A closer comparison between Tremblay’s and our data revealed a key difference: Hb in the highlanders with EE was abnormally high (20–27 g/dL), and that for the male college athletes in this study with or without GP.Mur was all within the normal range for men (12.5–17 g/dL). The inverse correlation of Hb-FMD was not found in our GPMur-negative control athletes or in the Andean non-patient highlander subjects whose Hb levels were below 21 g/dL (Tremblay et al., 2019). Hb is a major NO scavenger, and abnormally high Hb concentrations (accompanied with an abnormally higher RBC count) undoubtedly scavenge more NO in the vasculature and result in lower FMD (as in the EE cases). In contrast, the significant Hb–FMD inverse correlation found within the normal range of Hb in the GP.Mur+ subjects indicates that their NO-dependent vasodilation is more sensitive to intravascular Hb concentrations (Figure 1B).
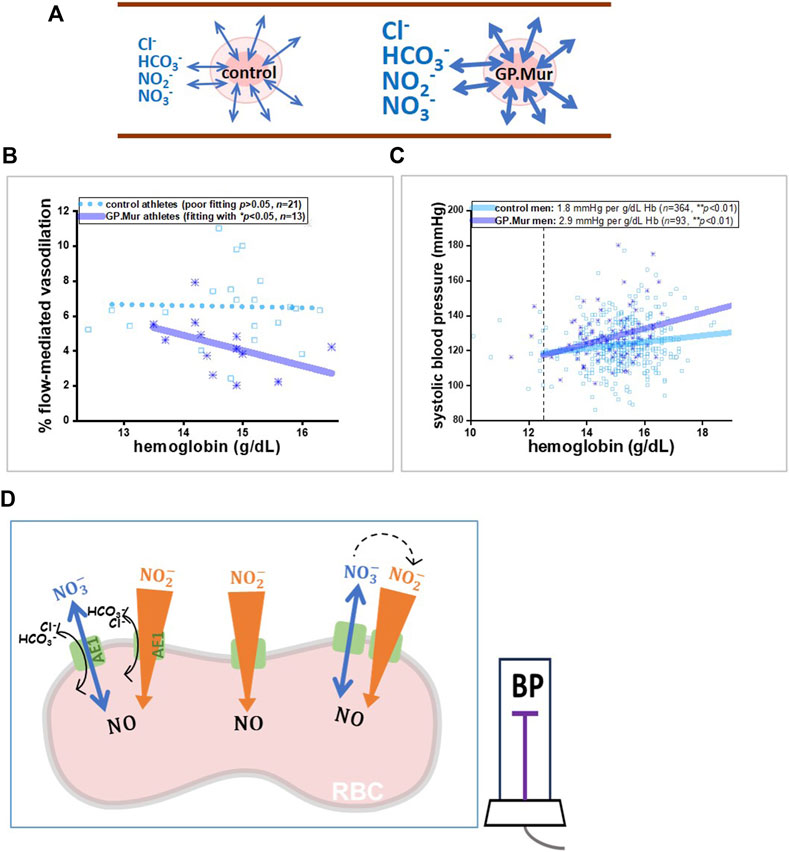
Figure 1. A model illustrating that GP.Mur/higher AE1 expression could increase NOx metabolic cycling and NO scavenging by intraerythrocytic Hb. (A) The bidirectional arrows indicate anion fluxes (mostly HCO3−, Cl−, NO2−, and NO3−) via AE1 (Jennings, 2021). The GP.Mur+ RBC membrane is more permeable to these monoanions (shown as thicker bidirectional arrows). (B, C) Previous studies on healthy people showed that GPMur/increased AE1 imposes higher sensitivities of FMD and SBP to Hb levels. (B) The Hb–FMD correlation for GP.Mur+ male athletes (R ∼ 0.53) was replotted using the published data (Hsu et al., 2021). (C) The Hb–SBP correlations for GP.Mur+ men (R ∼ 0.44) and for control men (R ∼ 0.37) were replotted using the published data collected at the MMH health check-up center in Eastern Taiwan (Chen et al., 2022). These GP.Mur comparative human studies revealed that GP.Mur/higher AE1 expression reduces NO-dependent FMD and increases blood pressure. (D) In the proposed model, AE1 (green gate) transports nitrite (orange-colored arrow) and nitrate (blue arrow) across the red cell membrane. Nitrite influx is followed by rapid and complex redox reactions catalyzed by Hb inside the RBCs, and thus the large concentration gradient of nitrite across the RBC membrane should favor nitrite influx (Chen et al., 2024). Nitrate is generally synthesized from excessive NO by oxyHb and is a stable reservoir of NO that permeates in or out of the RBCs via AE1 following its concentration gradient (bidirectional flux of nitrate). If depletion of plasma nitrite could accelerate the enzymatic conversion of extracellular nitrate to nitrite by nitrate reductase and xanthine oxidoreductase (dotted arrows), this may help explain how GP.Mur/higher AE1 lowers systemic NO and triggers high BP.
Hb and blood pressure are weakly but directly correlated. The direct correlation of Hb and BP was first identified in half a million Dutch blood donors at Sanquin: an increase of 1 g/dL Hb accompanies an increase of ∼0.81 mmHg SBP among Dutch men (Atsma et al., 2012). The degrees of the Hb–BP correlation vary slightly between men and women or among different ethnic populations (Gobel et al., 1991; Kawamoto et al., 2012; Lee et al., 2015; Xuan et al., 2019; Abumohsen et al., 2021).
In our population study conducted at the health check-up center in Eastern Taiwan, an increase of 1 g/dL Hb accompanies a ∼2.9 mmHg increase of SBP in GP.Mur+ men, compared to an ∼1.8 mmHg increase of SBP in the control (GP.Mur-negative) men, after controlled for age and BMI by multivariate regression (Figure 1C) (Chen et al., 2022). Compared to the controls, the blood pressure in the GP.Mur+ men was ∼60% more dependent on individual Hb levels. The positive correlation between Hb and BP could be caused by the following: (1) more circulating RBCs (as shown with an abnormally higher RBC count and Hb concentrations in high-altitude EE) increase blood viscosity and intravascular pressure (Tremblay et al., 2019), and (2) Hb scavenges intravascular NO species more effectively (as shown with a stronger Hb–FMD correlation and stronger Hb–BP correlation found in GP.Mur+ carriers) (Figure 1B, C) (Hsu et al., 2021; Chen et al., 2022). Since Hb is enclosed inside the RBC membrane, the second scenario points to the fact that GP.Mur/increased AE1 activities on the RBC membrane could promote Hb scavenging of NO species during blood circulation.
Toward developing a hypothesis that increased AE1 expression could reduce systemic NO
A higher monoanion permeability of the GP.Mur+ RBC membrane enhances the NO2−/NO3− flux across the RBC membrane and intraerythrocytic processing of NO species (Figure 1A, D). NO2− and NO3− (nitrate) are the major constituents of the intravascular NO reservoir. Excessive NO in the vasculature is mainly converted to nitrate by oxygenated Hb (NO + oxyHb → NO3− + metHb). Compared to nitrite, nitrate is a more stable and much less reactive NO metabolite. Both NO2− and NO3− freely permeate across the RBC membrane through AE1 (Jennings, 2021; Chen et al., 2024). The more reactive nitrite (half-life ∼0.5 h compared to 5–8 h of nitrate) tends to be converted to NO in a less oxygenated environment, e.g., arterioles. This reaction stimulated by hypoxia (NO2− + deoxyHb → NO + metHb) facilitates vasodilation and blood flow (Cosby et al., 2003; Dejam et al., 2005). In the case of GP.Mur, enhanced nitrite flux helps facilitate deoxyHb-catalyzed reduction of nitrite to NO gas. NO, with a half-life of ∼2 msec, dissipates extremely rapidly. Faster erythrocyte processing of NO conceivably may reduce systemic NO (Figure 1D).
However, from the previous study, we did not find blood plasma levels of NO2− and NO3− to be significantly different between GP.Mur+ and GP.Mur-negative athletes (Hsu et al., 2021), although we also did not ask the study participants to have the same diet. Various factors, including individual diet preferences, could dynamically affect their blood NOx levels (NO2− + NO3−). NO bioavailability can be increased in our body with consumption of more leafy green vegetables and beetroot (and even more nitrate-cured meats) in the diet.
On the other hand, fractional exhaled NO (FeNO) was affected by GP.Mur. FeNO was measured using NIOX, a clinical instrument that is intended to measure NO generated from Th2-driven inflammatory iNOS activities for the diagnosis of asthma (Menzies-Gow et al., 2020). We challenged the college athletes with an exhaustive running test protocol and measured their FeNO before the run and at the first and the fourth minute post-run. Their FeNO values decreased substantially from the exhaustive run and then rebounded gradually during the recovery phase, indicating a greater need for NO for vasodilation as intense exercise increased blood flow and gas exchange (Table 1: nearly 10-fold increases in minute ventilation [VE]). Both FeNO and the minute volume of exhaled NO (VNO = VE * FeNO) were generally lower in GP.Mur+ than GP.Mur-negative athletes, and the differences reached statistical significance immediately after exercise (Table 1: 1-min post-run measurements). iNOS-associated type II airway inflammation was not involved, and clinical asthma was not found in these athletes since none of them showed >50 ppb FeNO. Thus, GP.Mur/increased AE1 affected exhaled NO (FeNO), which reflects lower NO in the pulmonary vasculature, especially during exercise-expanded pulmonary circulation (Table 1).
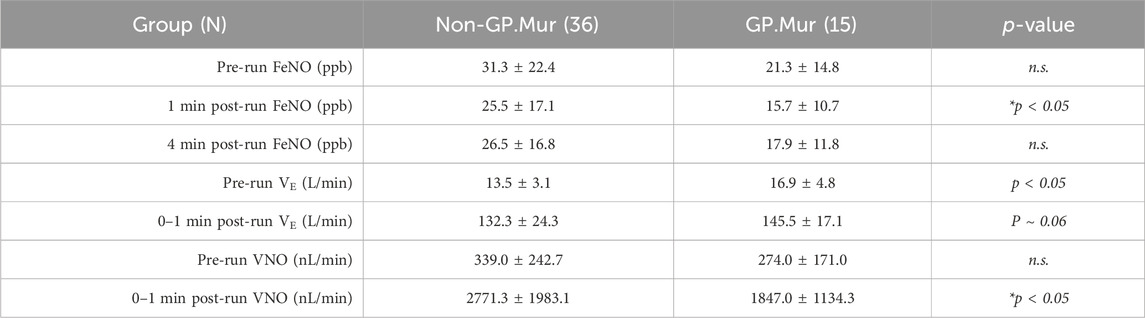
Table 1. FeNO from GP.Mur+ versus non-GP.Mur college athletes before and after an exhaustive running test. Minute ventilation (VE) was measured by cardiopulmonary exercise testing (CPET), which was immediately followed by FeNO measurement within a minute post-run. Statistical testing was performed by unpaired t-test. n.s., not significant. The study was approved by the MMH Institutional Review Board (MMH-IRB registration: 19MMHIS081e).
Although the levels of blood plasma NO metabolites were not different between GP.Mur and non-GP.Mur college athletes (Hsu et al., 2021), in our recently developed GYP.Mur knock-in (GPMur KI) B6J mice, significantly reduced NO bioavailability and early-onset hypertension were observed (unpublished data). Unlike the human study (Hsu et al., 2021), GPMur KI and the wildtype B6J mice are all bred under the same condition and fed with the same chow. The new murine data support the hypothesis that GP.Mur/more AE1 could contribute to reduced NO bioavailability. Perhaps by means of reduction of blood NO bioavailability (e.g., nitrate), the individual blood pressure setting could be elevated. The eNOS knock-out mice also show similar phenotypes—reduced blood NO bioavailability and hypertension (Wood et al., 2013).
AE1 transports NO2−/NO3− for erythrocyte processing of NO
To examine the effects of AE1-mediated NO2−/NO3− transport on intraerythrocytic processing of NO species, we treated human whole blood samples (containing plasma and blood cells) with excessive nitrite (1 mM, compared to the generally submicromolar nitrite in human blood). The excessively added nitrite in the whole blood sample decreased from 1 mM to 10 μM within 20 min, indicating a rapid scavenging and metabolism of NO2− by Hb. Using live-cell image recording with NO-sensitive fluorophore DAF, a surge of DAF fluorescence inside the RBCs appeared visibly within 1–2 min after adding excessive NO2− in the milieu (Chen et al., 2024). If erythroid anion transport was first blocked by AE1-targeting antibodies or was delayed by replacing Cl− (the counter-ion of AE1-mediated transport) with AE1-impermeant gluconate monoanion in the milieu, excessively added nitrite could remain imperishable in the blood plasma for a very long time. These demonstrate that inhibition of erythroid anion transport substantially reduces the rates of NO2−/NO scavenging and metabolism catalyzed by intraerythrocytic Hb (Chen et al., 2024).
Discussion
From our human studies conducted in 2017–2022, we found that GP.Mur/increased AE1 is associated with early-onset hypertension. Non-diseased people with the GP.Mur blood type generally have slightly higher blood pressure, lower NO-dependent vasodilation, and lower fraction of exhaled nitric oxide (Table 1; Figure 1B, C) (Hsu et al., 2021; Chen et al., 2022). It has been a general impression among health workers in Eastern Taiwan that the local populations with higher percentages of GP.Mur are more susceptible to stroke and type II diabetes (T2D), though rigorous epidemiologic surveys have not been reported. Notably, hypertension is the major risk factor for both stroke and T2D.
To understand how the expression of glycophorin B-A-B variant GP.Mur on RBCs leads to higher blood pressure, we began to formulate a working model with the basic science that it is thermodynamically unfavorable for charged ions to permeate through the lipid bilayer (Figure 1A, D). More AE1 embedded in the RBC membrane makes the cells more permeable to monoanions and expedites NO2−/NO3− membrane transport. NO2− influx is particularly affected, as its concentration gradient across the red cell membrane is presumably much bigger due to deoxyHb-mediated NO2− reduction to NO gas, which takes place inside the RBCs. When NO2− enters an erythrocyte, NO2− is also converted to N2O3, GSNO, and other NO species catalyzed by the Hb of different oxidation states (Patel et al., 2011). Thus, higher AE1 expression accelerates intraerythrocytic NO2−/NO metabolism, driving the conversion of NO3− to NO2− by microbiota nitrate reductases and xanthine oxidoreductase (Kapil et al., 2013); this conceivably also lowers plasma nitrate.
Since GP.Mur/increased AE1 is associated with blood NOx reduction, could blockade of AE1 instead help maintain NO bioavailability? Dipyridamole is an old anti-thrombotic and antianginal drug that blocks erythroid AE1 (Allahham et al., 2022). Dipyridamole reduces platelet aggregation and potentiates vasodilation with multiple mechanisms; its most well-studied mechanism for vasodilation is inhibition of adenosine receptors (Gamboa et al., 2005; Allahham et al., 2022). Whether dipyridamole as an AE1 inhibitor could also potentiate vasodilation has not been investigated because the link between AE1 and NO has been unclear for a long time. Dipyridamole, as a blocker of adenosine receptors, reduces the cellular uptake of adenosine and increases extracellular adenosine concentrations, which stimulates the activities of adenosine cyclase to generate more cAMP to potentiate eNOS activities and prostacyclin production (Gamboa et al., 2005; Allahham et al., 2022). Nonetheless, the transcript level of AE1 in human whole blood is 3-fold or higher than that of adenosine receptors (78.9 transcripts per million or TPM [AE1] versus 24.3 TPM [ADORA2A] from the human GTEx consortium) (Consortium et al., 2017). With our recent findings (Hsu et al., 2021; Chen et al., 2022; Chen et al., 2024), it is thus possible that dipyridamole-induced vasodilation could also be due to inhibition of erythroid AE1.
Dipyridamole binds to the AE1 dimer in a 1:1 stoichiometry and with a high affinity (Kd ∼1.2 μM) (Falke and Chan, 1986). As a secondary effect of AE1 inhibition, dipyridamole indirectly reduces K+ efflux from RBCs. Clinically, this function of dipyridamole protects sickle RBCs from excessive cation efflux and consequent dehydration (Joiner et al., 2001). Through the same mechanism, dipyridamole also protects RBC concentrates (i.e., as a transfusion product) from K+ leakage caused by virus-inactivating photosensitizer treatments (vanSteveninck et al., 2000). In the latter example, AE1–dipyridamole binding not only protects RBCs from K+ leak-associated hemolysis but also from peroxidative damage (Nepomuceno et al., 1997; van; Steveninck et al., 2000). Since AE1 is functionally versatile, without a doubt, our current model of AE1 in blood NO processing and vasodilation (Figure 1D) is far from complete.
Similar to the finding that GP.Mur increases erythroid HCO3− permeability and respiratory excretion of CO2 (Hsu et al., 2009; Hsu et al., 2015; Hsu et al., 2023), here, we proposed that GP.Mur also increases erythroid NO3−/NO2− permeability to accelerate erythrocyte processing of NO metabolites, which affects blood pressure (Patel et al., 2011) (Figure 1D). Could the two distinct anion transport modes of AE1 be coupled and AE1 serve as a coordinator for the seemingly separate physiological processes? A recently emerged clue is that GP.Mur+ carriers tend to rely more on aerobic respiration during intense exercise (Hsu et al., 2023). As intense physical activities demand more O2 delivery and blood flow, conceivably intravascular NO is metabolized and dissipated faster. With the new GPMur KI mouse model that recapitulates the hypertensive phenotype of GP.Mur+ people, we hope to uncover these physiologic interplays and health relevance stemming from the AE1-mediated transport of HCO3−/Cl− and NO3−/NO2− in the future.
Data availability statement
The raw data supporting the conclusion of this article will be made available by the authors, without undue reservation.
Ethics statement
The studies involving humans were approved by the MacKay Memorial Hospital Institutional Review Board. The studies were conducted in accordance with the local legislation and institutional requirements. The participants provided their written informed consent to participate in this study.
Author contributions
KH: writing–review and editing and writing–original draft.
Funding
The author(s) declare that financial support was received for the research, authorship, and/or publication of this article. This work was supported by grants from the Taiwan Ministry of Science and Technology (MOST 108-2628-B-195-001; 109-2628-B-195-001; 110-2628-B-195-001) and from MacKay Memorial Hospital to KH(MMH 111-26; 112-63; MMH 111-38; MMH 112-128; MMH 113-67).
Acknowledgments
We thank Hui-Ju Lin (MMH) and the students and faculty members of the University of Taipei for supporting and participating in the FeNO study and Prof. Yenta Lu (MMH Pulmonary medicine) for NIOX.
Conflict of interest
The author declares that the research was conducted in the absence of any commercial or financial relationships that could be construed as a potential conflict of interest.
Publisher’s note
All claims expressed in this article are solely those of the authors and do not necessarily represent those of their affiliated organizations, or those of the publisher, the editors, and the reviewers. Any product that may be evaluated in this article, or claim that may be made by its manufacturer, is not guaranteed or endorsed by the publisher.
References
Abumohsen H., Bustami B., Almusleh A., Yasin O., Farhoud A., Safarini O., et al. (2021). The association between high hemoglobin levels and pregnancy complications, gestational diabetes and hypertension, among Palestinian women. Cureus 13, e18840. doi:10.7759/cureus.18840
Allahham M., Lerman A., Atar D., Birnbaum Y. (2022). Why not dipyridamole: a review of current guidelines and Re-evaluation of utility in the modern era. Cardiovasc Drugs Ther. 36, 525–532. doi:10.1007/s10557-021-07224-9
Atsma F., Veldhuizen I., De Kort W., Van Kraaij M., Pasker-De Jong P., Deinum J. (2012). Hemoglobin level is positively associated with blood pressure in a large cohort of healthy individuals. Hypertension 60, 936–941. doi:10.1161/HYPERTENSIONAHA.112.193565
Chen P. L., Huang K. T., Chen L. Y., Hsu K. (2024). Erythroid anion Exchanger-1 (band 3) transports nitrite for nitric oxide metabolism. Free Radic. Biol. Med. 210, 237–245. doi:10.1016/j.freeradbiomed.2023.11.028
Chen Y. C., Hsu K. N., Lai J. C., Chen L. Y., Kuo M. S., Liao C. C., et al. (2022). Influence of hemoglobin on blood pressure among people with GP.Mur blood type(☆). J. Formos. Med. Assoc. 121, 1721–1727. doi:10.1016/j.jfma.2021.12.014
Consortium G., Laboratory D. A., Enhancing G. G., Fund N. I. H. C., Source Site N., Biospecimen Collection Source Site R., et al. (2017). Genetic effects on gene expression across human tissues. Nature 550, 204–213. doi:10.1038/nature24277
Cosby K., Partovi K. S., Crawford J. H., Patel R. P., Reiter C. D., Martyr S., et al. (2003). Nitrite reduction to nitric oxide by deoxyhemoglobin vasodilates the human circulation. Nat. Med. 9, 1498–1505. doi:10.1038/nm954
Dejam A., Hunter C. J., Pelletier M. M., Hsu L. L., Machado R. F., Shiva S., et al. (2005). Erythrocytes are the major intravascular storage sites of nitrite in human blood. Blood 106, 734–739. doi:10.1182/blood-2005-02-0567
Falke J. J., Chan S. I. (1986). Molecular mechanisms of band 3 inhibitors. 2. Channel blockers. Biochemistry 25, 7895–7898. doi:10.1021/bi00372a016
Gamboa A., Abraham R., Diedrich A., Shibao C., Paranjape S. Y., Farley G., et al. (2005). Role of adenosine and nitric oxide on the mechanisms of action of dipyridamole. Stroke 36, 2170–2175. doi:10.1161/01.STR.0000179044.37760.9d
Gladwin M. T., Grubina R., Doyle M. P. (2009). The new chemical biology of nitrite reactions with hemoglobin: R-state catalysis, oxidative denitrosylation, and nitrite reductase/anhydrase. Acc. Chem. Res. 42, 157–167. doi:10.1021/ar800089j
Gobel B. O., Schulte-Gobel A., Weisser B., Glanzer K., Vetter H., Dusing R. (1991). Arterial blood pressure. Am. J. Hypertens. 4, 14–19. doi:10.1093/ajh/4.1.14
Green D. J., Dawson E. A., Groenewoud H. M., Jones H., Thijssen D. H. (2014). Is flow-mediated dilation nitric oxide mediated? a meta-analysis. Hypertension 63, 376–382. doi:10.1161/HYPERTENSIONAHA.113.02044
Han T. H., Qamirani E., Nelson A. G., Hyduke D. R., Chaudhuri G., Kuo L., et al. (2003). Regulation of nitric oxide consumption by hypoxic red blood cells. Proc. Natl. Acad. Sci. U. S. A. 100, 12504–12509. doi:10.1073/pnas.2133409100
Hsu K., Chi N., Gucek M., Van Eyk J. E., Cole R. N., Lin M., et al. (2009). Miltenberger blood group antigen type III (Mi.III) enhances the expression of band 3. Blood 114, 1919–1928. doi:10.1182/blood-2008-12-195180
Hsu K., Kuo M. S., Yao C. C., Lee T. Y., Chen Y. C., Cheng H. C., et al. (2015). Expedited CO2 respiration in people with Miltenberger erythrocyte phenotype GP.Mur. Sci. Rep. 5, 10327. doi:10.1038/srep10327
Hsu K., Lin Y. C., Chao H. P., Lee T. Y., Lin M., Chan Y. S. (2013). Assessing the frequencies of GP.Mur (mi.III) in several Southeast Asian populations by PCR typing. Transfus. Apher. Sci. 49, 370–371. doi:10.1016/j.transci.2013.05.011
Hsu K., Liu Y., Tseng W., Huang K., Liu C., Chen L., et al. (2021). Comodulation of NO-dependent vasodilation by erythroid band 3 and hemoglobin: a GP.Mur athlete study. Front. Cardiovasc. Med. 8, 740100. doi:10.3389/fcvm.2021.740100
Hsu K., Tseng W. C., Chen L. Y., Chen P. L., Lu Y. X., Chen Y. S., et al. (2023). Effects of greater erythroid Cl(-)/HCO(3)(-) transporter (band 3) expression on ventilation and gas exchange during exhaustive exercise. Am. J. Physiol. Lung Cell Mol. Physiol. 324, L825–L835. doi:10.1152/ajplung.00036.2022
Huang K. T., Han T. H., Hyduke D. R., Vaughn M. W., Van Herle H., Hein T. W., et al. (2001). Modulation of nitric oxide bioavailability by erythrocytes. Proc. Natl. Acad. Sci. U. S. A. 98, 11771–11776. doi:10.1073/pnas.201276698
Jana S., Strader M. B., Meng F., Hicks W., Kassa T., Tarandovskiy I., et al. (2018). Hemoglobin oxidation-dependent reactions promote interactions with band 3 and oxidative changes in sickle cell-derived microparticles. JCI Insight 3, e120451. doi:10.1172/jci.insight.120451
Jennings M. L. (2021). Cell physiology and molecular mechanism of anion transport by erythrocyte band 3/AE1. Am. J. Physiol. Cell Physiol. 321, C1028–C1059. doi:10.1152/ajpcell.00275.2021
Joiner C. H., Jiang M., Claussen W. J., Roszell N. J., Yasin Z., Franco R. S. (2001). Dipyridamole inhibits sickling-induced cation fluxes in sickle red blood cells. Blood 97, 3976–3983. doi:10.1182/blood.v97.12.3976
Jongruamklang P., Grimsley S., Thornton N., Robb J., Olsson M. L., Storry J. R. (2020). Characterization of GYP*Mur and novel GYP*Bun-like hybrids in Thai blood donors reveals a qualitatively altered s antigen. Vox Sang. 115, 472–477. doi:10.1111/vox.12909
Kaito S., Suzuki Y., Masuno A., Isa K., Toyoda C., Onodera T., et al. (2022). Frequencies of glycophorin variants and alloantibodies against Hil and MINY antigens in Japanese. Vox Sang. 117, 94–98. doi:10.1111/vox.13121
Kapil V., Haydar S. M., Pearl V., Lundberg J. O., Weitzberg E., Ahluwalia A. (2013). Physiological role for nitrate-reducing oral bacteria in blood pressure control. Free Radic. Biol. Med. 55, 93–100. doi:10.1016/j.freeradbiomed.2012.11.013
Kawamoto R., Tabara Y., Kohara K., Miki T., Kusunoki T., Katoh T., et al. (2012). A slightly low hemoglobin level is beneficially associated with arterial stiffness in Japanese community-dwelling women. Clin. Exp. Hypertens. 34, 92–98. doi:10.3109/10641963.2011.618202
Kim-Shapiro D. B., Schechter A. N., Gladwin M. T. (2006). Unraveling the reactions of nitric oxide, nitrite, and hemoglobin in physiology and therapeutics. Arterioscler. Thromb. Vasc. Biol. 26, 697–705. doi:10.1161/01.ATV.0000204350.44226.9a
Lancaster J. R. (1994). Simulation of the diffusion and reaction of endogenously produced nitric oxide. Proc. Natl. Acad. Sci. U. S. A. 91, 8137–8141. doi:10.1073/pnas.91.17.8137
Lee S. G., Rim J. H., Kim J. H. (2015). Association of hemoglobin levels with blood pressure and hypertension in a large population-based study: the Korea National Health and Nutrition Examination Surveys 2008-2011. Clin. Chim. Acta 438, 12–18. doi:10.1016/j.cca.2014.07.041
Menzies-Gow A., Mansur A. H., Brightling C. E. (2020). Clinical utility of fractional exhaled nitric oxide in severe asthma management. Eur. Respir. J. 55, 1901633. doi:10.1183/13993003.01633-2019
Nepomuceno M. F., Alonso A., Pereira-Da-Silva L., Tabak M. (1997). Inhibitory effect of dipyridamole and its derivatives on lipid peroxidation in mitochondria. Free Radic. Biol. Med. 23, 1046–1054. doi:10.1016/s0891-5849(97)00135-4
Patel R. P., Hogg N., Kim-Shapiro D. B. (2011). The potential role of the red blood cell in nitrite-dependent regulation of blood flow. Cardiovasc Res. 89, 507–515. doi:10.1093/cvr/cvq323
Pawloski J. R., Hess D. T., Stamler J. S. (2001). Export by red blood cells of nitric oxide bioactivity. Nature 409, 622–626. doi:10.1038/35054560
Prathiba R., Lopez C. G., Usin F. M. (2002). The prevalence of GP.Mur and anti-"Mia" in a tertiary hospital in Peninsula Malaysia. Malays J. Pathol. 24, 95–98.
Premont R. T., Reynolds J. D., Zhang R., Stamler J. S. (2020). Role of nitric oxide carried by hemoglobin in cardiovascular physiology: developments on a three-gas respiratory cycle. Circ. Res. 126, 129–158. doi:10.1161/CIRCRESAHA.119.315626
Steveninck J., Trannoy L. L., Besselink G. A., Dubbelman T. M., Brand A., De Korte D., et al. (2000). Selective protection of RBCs against photodynamic damage by the band 3 ligand dipyridamole. Transfusion 40, 1330–1336. doi:10.1046/j.1537-2995.2000.40111330.x
Suvila K., Langen V., Cheng S., Niiranen T. J. (2020). Age of hypertension onset: overview of research and how to apply in practice. Curr. Hypertens. Rep. 22, 68. doi:10.1007/s11906-020-01071-z
Tremblay J. C., Hoiland R. L., Howe C. A., Coombs G. B., Vizcardo-Galindo G. A., Figueroa-Mujica R. J., et al. (2019). Global REACH 2018: high blood viscosity and hemoglobin concentration contribute to reduced flow-mediated dilation in high-altitude excessive erythrocytosis. Hypertension 73, 1327–1335. doi:10.1161/HYPERTENSIONAHA.119.12780
Vaughn M. W., Huang K. T., Kuo L., Liao J. C. (2000). Erythrocytes possess an intrinsic barrier to nitric oxide consumption. J. Biol. Chem. 275, 2342–2348. doi:10.1074/jbc.275.4.2342
Walder J. A., Chatterjee R., Steck T. L., Low P. S., Musso G. F., Kaiser E. T., et al. (1984). The interaction of hemoglobin with the cytoplasmic domain of band 3 of the human erythrocyte membrane. J. Biol. Chem. 259, 10238–10246. doi:10.1016/s0021-9258(18)90956-7
Wei L., Shan Z. G., Flower R. L., Wang Z., Wen J. Z., Luo G. P., et al. (2016). The distribution of MNS hybrid glycophorins with Mur antigen expression in Chinese donors including identification of a novel GYP.Bun allele. Vox Sang. 111, 308–314. doi:10.1111/vox.12421
Wood K. C., Cortese-Krott M. M., Kovacic J. C., Noguchi A., Liu V. B., Wang X., et al. (2013). Circulating blood endothelial nitric oxide synthase contributes to the regulation of systemic blood pressure and nitrite homeostasis. Arterioscler. Thromb. Vasc. Biol. 33, 1861–1871. doi:10.1161/ATVBAHA.112.301068
Xuan Y., Zuo J., Zheng S., Ji J., Qian Y. (2019). Association of hemoglobin and blood pressure in a Chinese community-dwelling population. Pulse (Basel) 6, 154–160. doi:10.1159/000494735
Yang M. H., Chen J. W., Sayaka K., Uchikawa M., Tsuno N. H., Wei S. T., et al. (2021). Universal detection of mi(a) antigen and frequencies of glycophorin hybrids among blood donors in taiwan by human monoclonal antibodies against mi(a) (MNS7), mur (MNS10), and MUT (MNS35) antigens. Diagn. (Basel) 11, 806. doi:10.3390/diagnostics11050806
Keywords: band 3 (anion exchanger-1, AE1), GP.Mur (Miltenberger subtype III, Mi.III), nitric oxide, blood pressure, vasodilation, dipyridamole, glycophorin, erythrocyte
Citation: Hsu K (2024) Erythroid anion transport, nitric oxide, and blood pressure. Front. Physiol. 15:1363987. doi: 10.3389/fphys.2024.1363987
Received: 31 December 2023; Accepted: 21 March 2024;
Published: 10 April 2024.
Edited by:
Lars Kaestner, Saarland University, GermanyReviewed by:
Vassilis L. Tzounakas, University of Patras, GreeceCopyright © 2024 Hsu. This is an open-access article distributed under the terms of the Creative Commons Attribution License (CC BY). The use, distribution or reproduction in other forums is permitted, provided the original author(s) and the copyright owner(s) are credited and that the original publication in this journal is cited, in accordance with accepted academic practice. No use, distribution or reproduction is permitted which does not comply with these terms.
*Correspondence: Kate Hsu, khsu1@mmh.org.tw
†ORCID: Kate Hsu, orcid.org/0000-0003-1009-0997