- 1Department of Surgical Sciences and Integrated Diagnostics, University of Genoa, Genoa, Italy
- 2Anesthesia and Intensive Care, Ospedale Policlinico San Martino Istituto di Ricerca e Cura a Carattere Scientifico (IRCCS) for Oncology and Neurosciences, Genova, Italy
- 3Division of Pulmonary and Pulmonary Critical Care Medicine, Department of Medicine, Ramathibodi Hospital, Mahidol University, Bangkok, Thailand
- 4Department of Neurosciences, Reproductive and Odonthostomatological Sciences, University of Naples Federico II, Naples, Italy
- 5Department of Anaesthesia and Intensive Care, University of Foggia, Foggia, Italy
- 6Mahidol Oxford Tropical Medicine Research Unit (MORU), Mahidol University, Bangkok, Thailand
- 7Department of Intensive Care, Laboratory of Experimental Intensive Care and Anesthesiology (LEICA), Amsterdam University Medical Centers, Location Academic Medical Center (AMC), Amsterdam, Netherlands
- 8Nuffield Department of Medicine, Oxford University, Oxford, United Kingdom
- 9Laboratory of Pulmonary Investigation, Carlos Chagas Filho Institute of Biophysics, Federal University of Rio de Janeiro, Rio de Janeiro, Brazil
- 10Pulmonary Engineering Group, Department of Anaesthesiology and Intensive Care Medicine, University Hospital Carl Gustav Carus, Technische Universität Dresden, Dresden, Germany
Background: Variable pressure support ventilation (vPSV) is an assisted ventilation mode that varies the level of pressure support on a breath-by-breath basis to restore the physiological variability of breathing activity. We aimed to compare the effects of vPSV at different levels of variability and pressure support (ΔPS) in patients with acute respiratory distress syndrome (ARDS).
Methods: This study was a crossover randomized clinical trial. We included patients with mild to moderate ARDS already ventilated in conventional pressure support ventilation (PSV). The study consisted of two blocks of interventions, and variability during vPSV was set as the coefficient of variation of the ΔPS level. In the first block, the effects of three levels of variability were tested at constant ΔPS: 0% (PSV0%, conventional PSV), 15% (vPSV15%), and 30% (vPSV30%). In the second block, two levels of variability (0% and variability set to achieve ±5 cmH2O variability) were tested at two ΔPS levels (baseline ΔPS and ΔPS reduced by 5 cmH2O from baseline). The following four ventilation strategies were tested in the second block: PSV with baseline ΔPS and 0% variability (PSVBL) or ±5 cmH2O variability (vPSVBL), PSV with ΔPS reduced by 5 cmH2O and 0% variability (PSV−5) or ±5 cmH2O variability (vPSV−5). Outcomes included gas exchange, respiratory mechanics, and patient-ventilator asynchronies.
Results: The study enrolled 20 patients. In the first block of interventions, oxygenation and respiratory mechanics parameters did not differ between vPSV15% and vPSV30% compared with PSV0%. The variability of tidal volume (VT) was higher with vPSV15% and vPSV30% compared with PSV0%. The incidence of asynchronies and the variability of transpulmonary pressure (PL) were higher with vPSV30% compared with PSV0%. In the second block of interventions, different levels of pressure support with and without variability did not change oxygenation. The variability of VT and PL was higher with vPSV−5 compared with PSV−5, but not with vPSVBL compared with PSVBL.
Conclusion: In patients with mild-moderate ARDS, the addition of variability did not improve oxygenation at different pressure support levels. Moreover, high variability levels were associated with worse patient-ventilator synchrony.
Clinical Trial Registration: www.clinicaltrials.gov, identifier: NCT01683669.
Introduction
Pressure support ventilation (PSV) is an assisted ventilation mode commonly used in critically ill patients (Esteban et al., 2013). The maintenance of spontaneous respiratory activity in acute respiratory distress syndrome (ARDS) patients improves respiratory function and decreases the need for vasopressor and sedative drugs (Putensen et al., 2001). Assisted ventilation modes have been commonly used in the management of patients with ARDS, in particular those with mild to moderate hypoxemic respiratory failure (Bellani et al., 2016).
In the last years, researchers have proposed to vary the level of pressure support on a breath-by-breath basis to restore the physiological variability of breathing activity (Tobin et al., 1988). Variable pressure support ventilation (vPSV), compared with conventional PSV, improved oxygenation in the experimental models of ARDS (Gama de Abreu et al., 2008) and ventilator-patient synchrony in a small pilot study in critically ill patients with acute respiratory failure (Spieth et al., 2013). These effects could be mediated by an amelioration of the ventilation-perfusion matching (Huhle et al., 2016), as well as a recruitment effect due to the repetitive delivery of breaths with a higher tidal volume, which might also result in a reduction of lung inhomogeneity (Mauri et al., 2017). However, so far, the only clinical study published has used only one variability level at fixed pressure support (ΔPS) (Spieth et al., 2013). Therefore, the effects of different levels of variability and the impact of variability at different ΔPS levels remain unknown. Different levels of variability might modify differently the ventilation perfusion-matching and might affect differently gas exchange and respiratory mechanics.
The aim of this study was to evaluate the effects of vPSV, at different levels of variability and pressure support, on short-term lung function parameters in patients with mild to moderate ARDS. We tested the hypothesis that vPSV would improve gas exchange, respiratory mechanics, and patient-ventilator asynchrony. We also hypothesized that the degree of variability and the level of ΔPS would influence the effects of vPSV.
Methods
Study Design
This was a prospective, crossover, randomized clinical trial conducted in a single university hospital intensive care unit (ICU).
Inclusion and Exclusion Criteria
Patients aged >18 years with mild to moderate ARDS (PaO2/FIO2 ratio between 100 and 300 mmHg with a positive end-expiratory pressure, PEEP ≥5 cmH2O) already receiving PSV per clinical indication were screened for inclusion. Exclusion criteria were pregnancy, chronic obstructive pulmonary disease, presence of pneumothorax or chest tubes, and unavailability of research staff.
Interventions
According to the local clinical practice, conventional PSV was delivered by an Evita Infinity V500 ventilator (Dräger Medical AG, Lübeck, Germany) targeting a VT of 6–8 ml/kg of predicted body weight, respiratory rate ≤ 25 min−1 with PEEP and FIO2 titrated to achieve a peripheral oxygen saturation ≥92%. This ventilator can operate in vPSV mode setting the variability of the ΔPS and delivers breaths with an approximately Gaussian distribution, truncated at 3 SDs from the mean ΔPS. The parameter “variability” of this ventilator refers to the range of ΔPS, e.g., 90% “variability” results in a 30% coefficient of variation (CV). As illustrated in Figure 1, all patients underwent two blocks of interventions, receiving 45-min periods of ventilation with different settings. In the first block, the effects of three levels of variability were tested at constant ΔPS to explore the effect of variability added to a fixed ΔPS level, while in the second block, a variability of ±5 cmH2O was added to ΔPS set at either the baseline level or the baseline level minus 5 cmH2O, to investigate the effects of variability at two ΔPS levels. During the first block, the ΔPS was set at a fixed value corresponding to the level chosen by the treating clinician before enrolment, and three different CV% levels were used: 0% (PSV0%), 15% (vPSV15%), and 30% (vPSV30%). During the second block, four ventilation settings were used: PSV with baseline ΔPS (PSVBL), baseline ΔPS with variability set individually to ±5 cmH2O (vPSVBL), ΔPS reduced by 5 cmH2O compared with the baseline with either no variability (PSV−5) or variability set to ±5 cmH2O (vPSV−5). The two blocks were performed sequentially, within 1 h from each other to allow for nursing assistance if required, and ventilation modes within each intervention block were assigned in random order with a Latin square design (as shown in Figure 1; Supplementary Figures 1, 2). The randomization sequence was generated with an online service, and a sealed envelope was opened at the moment of patient enrolment. Participants were blinded to the treatment assignment as were the operators involved in respiratory mechanics analysis.
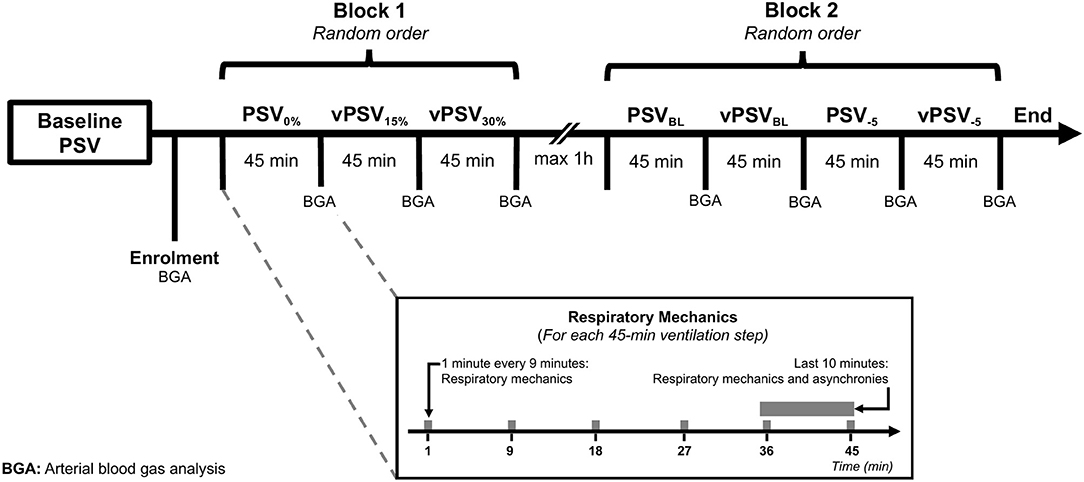
Figure 1. Time course of interventions. Within each intervention block, different ventilation settings were delivered in random order. PSV0%, conventional PSV ventilation with no variability; vPSV15%, variable PSV with variability set to 15% CV; vPSV30%, variable PSV with variability set to 30% CV; PSVBL, PSV with no variability and baseline ΔPS as per clinical indication; vPSVBL, variable pressure support with variability set to achieve ±5 cmH2O and baseline ΔPS as per clinical indication; PSV−5, PSV with no variability and ΔPS reduced by 5 cmH2O from the baseline value; vPSV−5, variable PSV with variability set to achieve ±5 cmH2O and ΔPS reduced by 5 cmH2O from the baseline value; PSV, pressure support ventilation; CV, coefficient of variation.
Patient management procedures not related to mechanical ventilation, including sedation and fluid administration, were at the discretion of the treating clinician. When clinically feasible, we avoided changing FIO2, PEEP, and ΔPS during the study, and in case of desaturation below 92%, FIO2 increase was prioritized over PEEP increase. After completion of the study protocol, ventilation was continued at the discretion of the treating physician.
Measurements
An esophageal balloon catheter (Compliance catheter, Microtek Medical B.V., Zutphen, The Netherlands) was inserted through the nose or mouth, filled with 1.5 ml, and correct positioning was verified with an occlusion maneuver (Akoumianaki et al., 2014). The flow was measured with a heated Fleisch-type pneumotachograph connected to a multi-channel transducer (ICU Lab, KleisTEK Engineering, Bari, Italy), while the tidal volume was measured as the integral of flow over time. Respiratory traces were recorded continuously throughout the study. An arterial blood gas analysis, heart rate, and invasive mean arterial pressure were recorded at baseline and the end of each ventilation step.
Pressure-time and flow-time curves were analyzed offline with a dedicated script written in MATLAB (MathWorks, MA, USA). The following parameters were computed breath by breath: VT, PEEP, ΔPS, mean airway pressure, inspiratory time to total time ratio (Tinsp/Ttot), respiratory rate (RR), esophageal pressure swings (ΔPes), and peak transpulmonary pressure (PL). The respiratory muscle activity was quantified with the esophageal pressure-time product per min (PTPes), calculated as follows (Mauri et al., 2016):
where Pmus is the pressure generated by the respiratory muscles, and Pcw,recoil is the chest wall recoil pressure, calculated assuming a fixed elastance of 5 cmH2O/L. The asynchrony index was computed as the number of asynchronous events divided by the total number of ventilator cycles plus ineffective efforts during expiration multiplied by 100 (Blanch et al., 2015). Asynchronies were classified independently by two experienced operators (LB and MV), and discrepancies were resolved by consensus. The analysis of respiratory mechanics data was performed by three operators blinded to the ventilation settings (ADO, MF, and LM). Also, we measured the evolution of respiratory mechanics at min 1, 9, 18, 27, 36, and 45 from the start of each ventilation step. To allow sufficient time for patient adaptation, main analyses of respiratory mechanics and asynchronies were restricted to the last 10 min of each ventilation step.
Data Analysis and Sample Size Calculation
All variables are reported as medians [25th−75th percentile], if not otherwise specified. Measurements on multiple breaths were aggregated within-patients computing the median and the CV; then, between-patients medians [25th−75th percentile] were computed. Comparisons between continuous variables during the different ventilation steps were sought with Friedman's test and Dunn's post-hoc test. The primary endpoint was the partial pressure of arterial oxygen to FiO2 ratio (PaO2/FiO2). From internal administrative data, we expected a baseline PaO2/FiO2 around 150 ± 50 mmHg. Using a Latin square crossover design, and assuming an intra-subject correlation of the PaO2/FiO2 between treatments with ρ = 0.75, we needed to enroll at least 16 patients to achieve 90% power (1-β) to detect a 20% relative increase in the PaO2/FiO2 ratio (Muller and Barton, 1989; Muller et al., 1992). To account for potential drop-off or missing respiratory mechanics data, we aimed to enroll 20 patients. Repeated measurement analysis of respiratory mechanics parameters at different timepoints within each ventilation block was performed using mixed-effects linear models using patients as random effects and timepoint, ventilation, and their interaction as fixed effects.
In one post-hoc analysis, associations were determined between the respiratory mechanics parameters of each breath and the ΔPS received during the preceding breath in the vPSVBL and vPSV−5 ventilation steps. For this purpose, mixed-effects linear models were used, using patients as random effects and the ΔPS received during the preceding breath as the fixed effect.
All analyses were performed with R 3.2.3 (The R Foundation for Statistical Computing, www.r-project.org). Statistical significance was considered for two-tailed p < 0.05.
Results
Twenty patients were enrolled and completed the study. Baseline characteristics are presented in Table 1. The FiO2 and PEEP were kept constant during the study in all patients; one patient required ΔPS reduction between ventilation block 1 and block 2 according to the treating clinician decision for reasons unrelated to the study procedures. Tables 2, 3 show respiratory mechanics, hemodynamics, and arterial blood gas analysis in ventilation blocks 1 and 2, respectively. The distribution of key respiratory mechanics parameters in ventilation blocks 1 and 2 is illustrated in Figures 2, 3, respectively. Supplementary Figures 3–11 report details the evolution over time of the respiratory mechanics parameters in the different ventilation steps.
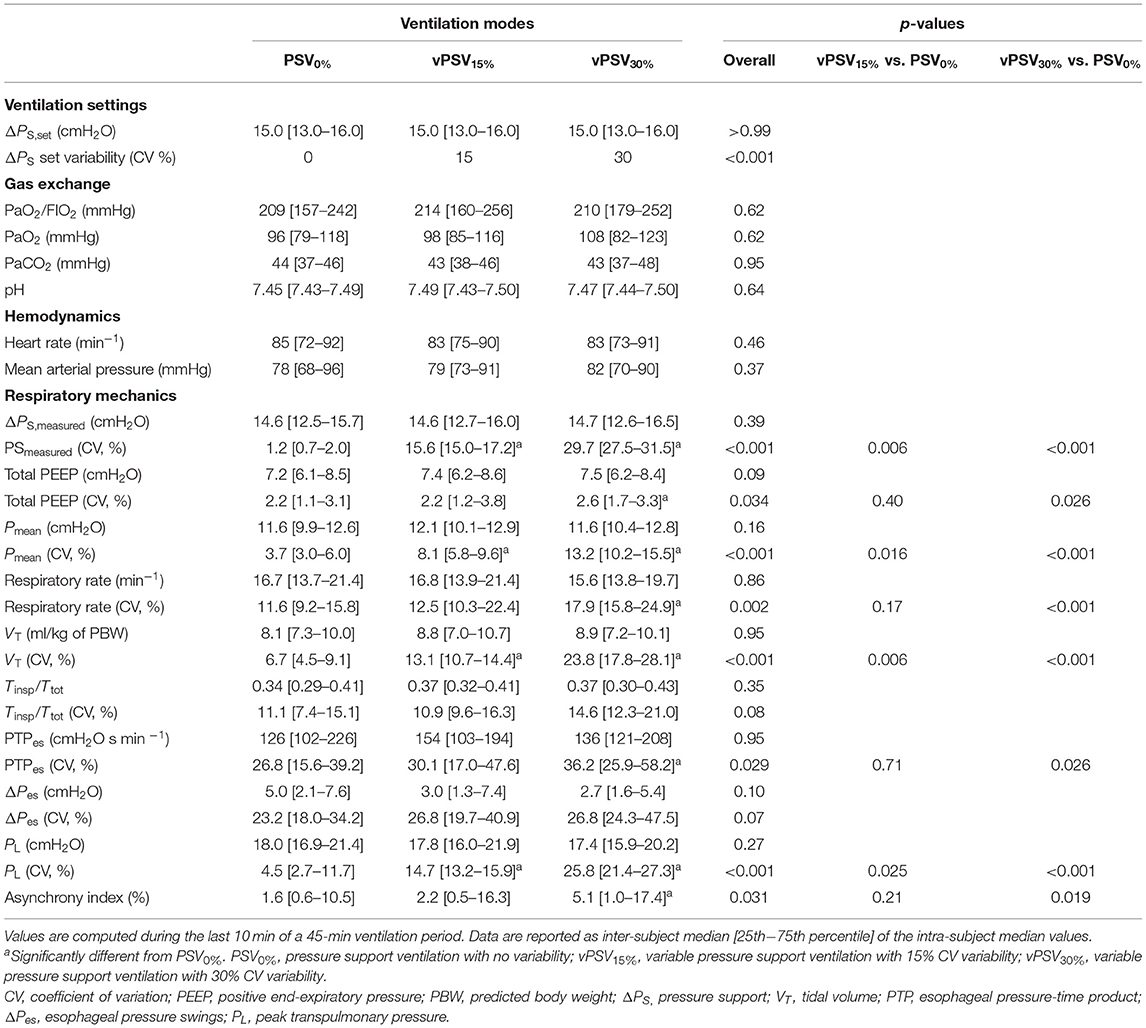
Table 2. Gas exchange, hemodynamics, and respiratory mechanics in patients during pressure support ventilation at different levels of variability (Block 1).
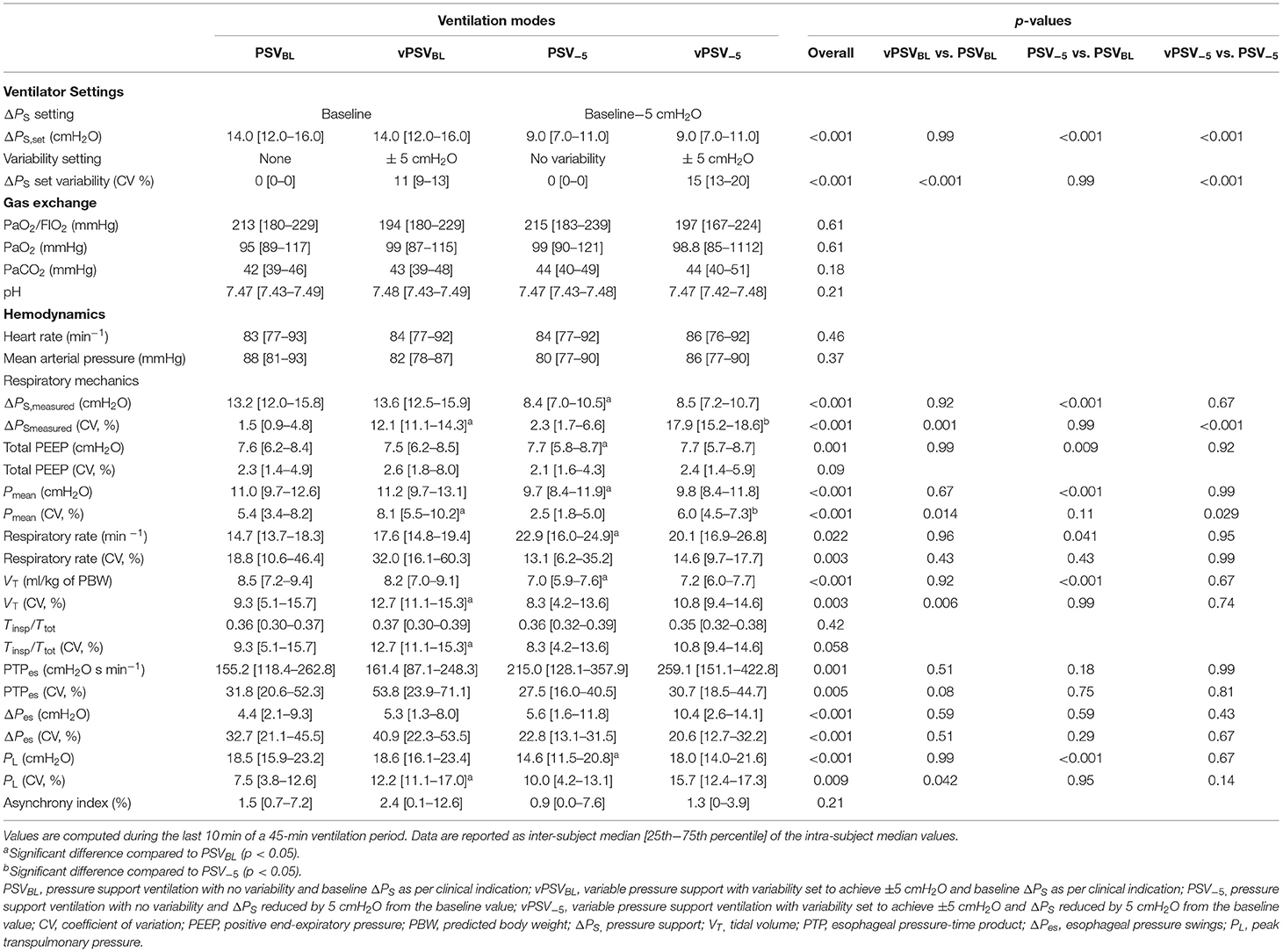
Table 3. Gas exchange, hemodynamics, and respiratory mechanics in patients during pressure support ventilation at different variability and pressure support level (Block 2).
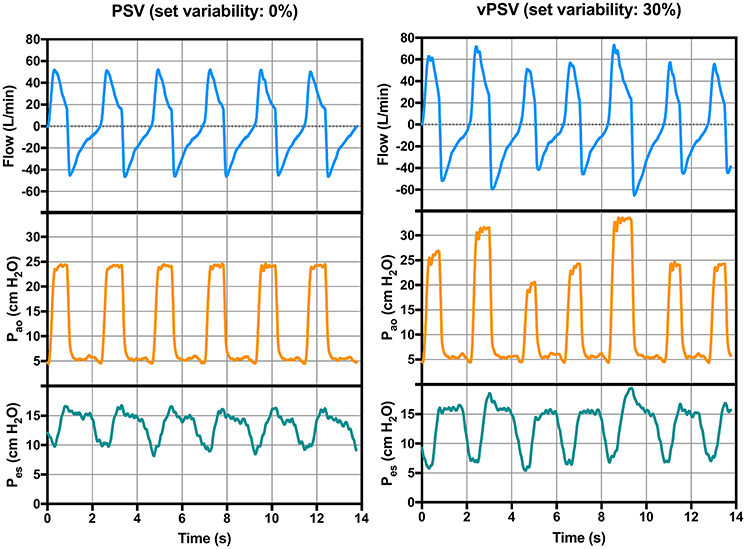
Figure 2. Representative respiratory traces of a patient during conventional (left) and variable (right) pressure support ventilation. Pao, pressure at the airway opening; Pes, esophageal pressure.
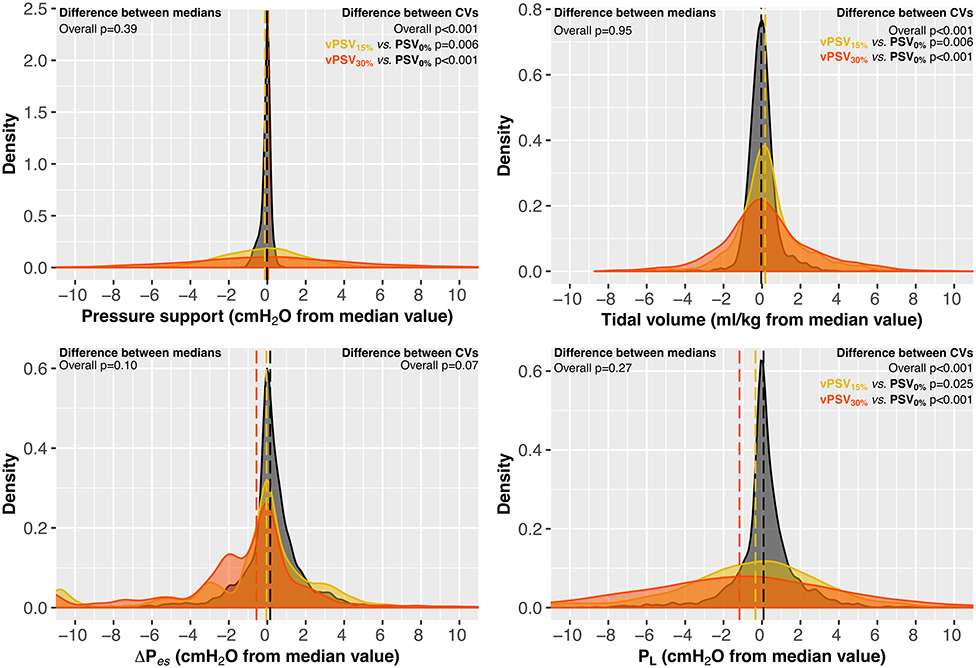
Figure 3. Respiratory mechanics at different levels of variability (block 1). Variables are reported as the difference from the median value achieved during PSV0% to allow between-patients visual comparisons. Dashed lines represent the medians of each ventilation step. PSV0%, conventional PSV ventilation with no variability; vPSV15%, variable PSV with variability set to 15% CV; vPSV30%, variable PSV with variability set to 30% CV; CV, coefficient of variation; ΔPS, pressure support; ΔPes, esophageal pressure swings; PL, peak transpulmonary pressure.
Block 1: Physiological Effects of Different Variability Levels at Constant ΔPS
The PaO2/FiO2 did not differ between ventilation steps in block 1 (p = 0.62, Table 2). Median respiratory mechanics variables, other gas exchange, and hemodynamic parameters did not change between vPSV15% and vPSV30% compared with PSV0% (Table 2). However, the variability of ΔPS, PEEPtot, Pmean, and VT was higher with PSV15% and PSV30% compared with PSV0% (Table 2). The RR and PTPes,min had higher variability only with vPSV30% (Table 2). Moreover, asynchronies were more frequent with vPSV30% compared with PSV0% (p = 0.019, Table 2).
Block 2: Physiological Effects of Variability at Two Levels of ΔPS
The PaO2/FiO2, as well as other gas exchange and hemodynamic parameters, did not differ between ventilation steps in block 2 (Table 3). Ventilation modes with ΔPS reduced by 5 cmH2O (PSV−5 and vPSV−5) had lower Pmean, VT, PL, and higher RR (Table 3). Adding ±5 cmH2O variability (vPSVBL and vPSV−5 steps) increased the variability of ΔPS and Pmean compared to PSV without variability at the corresponding ΔPS level. Adding ±5 cmH2O variability increased the variability of VT and PL only when using the baseline ΔPS, but not when the ΔPS was reduced by 5 cmH2O. The incidence of asynchronies was not different between ventilation steps in block 2 (Table 3).
Tables 2, 3 report extensive details on respiratory mechanics, hemodynamics, and arterial blood gas analysis in ventilation blocks 1 and 2, respectively. The distribution of key respiratory mechanics parameters in ventilation blocks 1 and 2 is illustrated in Figures 3, 4, respectively. Supplemental Figures 3–11 report details the evolution over time of the respiratory mechanics parameters in the different ventilation steps.
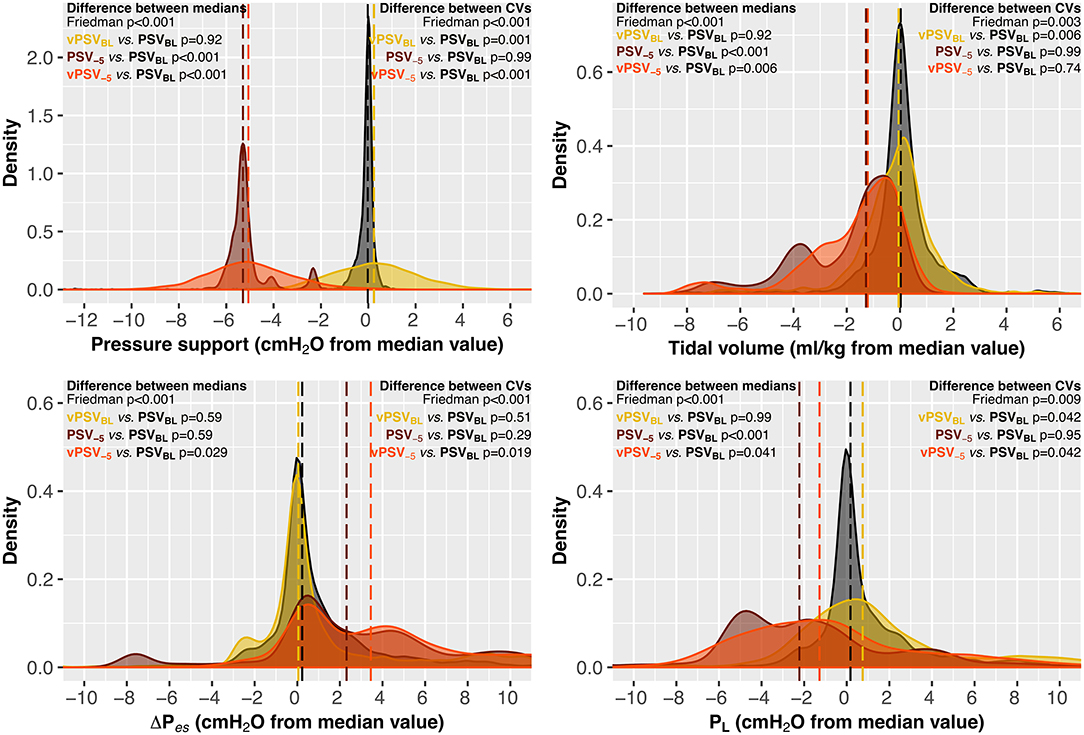
Figure 4. Effects of variability on the distribution of respiratory mechanics parameters at different levels of pressure support (block 2). Variables are reported as the difference from the median value achieved during PSVBL to allow between-patients visual comparisons. Dashed lines represent the medians of each ventilation step. PSVBL, PSV with no variability and baseline ΔPS as per clinical indication; vPSVBL, variable pressure support with variability set to achieve ±5 cmH2O and baseline ΔPS as per clinical indication; PSV−5, PSV with no variability and ΔPS reduced by 5 cmH2O from the baseline value; vPSV−5, variable PSV with variability set to achieve ±5 cmH2O and ΔPS reduced by 5 cmH2O from the baseline value; PSV, pressure support ventilation; CV, coefficient of variation; ΔPS, pressure support; ΔPes, esophageal pressure swings; PL, peak transpulmonary pressure.
Post-hoc Analysis
Associations between respiratory mechanics parameters and the pressure level received in the preceding breath during vPSVBL and vPSV−5 are reported in Figure 5. The ΔPS received in the preceding breath was inversely associated with the magnitude of the inspiratory effort (ΔPes) in the following breath, both during vPSVBL (p = 0.003) and vPSV−5 (p = 0.005).
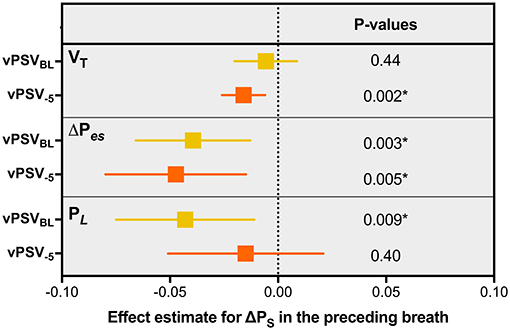
Figure 5. Associations between respiratory mechanics parameters and the pressure level received in the preceding breath during variable PSV. Squares and confidence intervals refer to the effect estimate for ΔPS in a mixed model comprising the ΔPS received during the preceding breath as a fixed effect and the patient as a random effect with random intercept. The units of the estimates are expressed in the untransformed units of the variables, i.e., they represent the absolute change in VT, ΔPes, or PL when the ΔPS received during the preceding breath increases by 1 cmH2O. vPSVBL, variable PSV with variability set to achieve ±5 cmH2O and baseline ΔPS as per clinical indication; vPSV−5, variable PSV ventilation with variability set to achieve ±5 cmH2O and ΔPS reduced by 5 cmH2O from the baseline value; PBW, predicted body weight; PSV, pressure support ventilation; ΔPS, pressure support; VT, tidal volume; ΔPes, esophageal pressure swings; PL, peak transpulmonary pressure. *Significant association (p < 0.05).
Discussion
The main findings of this study are that in our mixed-ICU population of patients with mild to moderate ARDS: (1) vPSV with 15 or 30% variability did not influence gas exchange compared with conventional PSV; (2) at constant ΔPS, vPSV increased the variability of VT and PL; (3) vPSV30% increased the incidence of asynchronies; and (4) when the ΔPS was reduced by 5 cmH2O from the baseline value, adding variability did not increase the variability of VT and PL.
This is the first study comparing the short-term effects of vPSV at different levels of variability and ΔPS in patients with ARDS. In previous studies, vPSV improved oxygenation in the experimental models of ARDS (Gama de Abreu et al., 2008; Spieth et al., 2011, 2012), but not in a cohort of hypoxemic critically ill patients (Spieth et al., 2013). However, that last study included mostly postoperative patients without a confirmed diagnosis of ARDS and investigated a single level of variability and pressure support. Opposite to what was found in preclinical studies in animals, vPSV had no effect on gas exchange, when the ΔPS was set to the baseline value identified by the treating clinician and neither when it was reduced by 5 cmH2O. This could be explained by several mechanisms; most importantly, the time investigated in each ventilation step was relatively short, and the fact that patients had an established diagnosis of ARDS mostly in their recovery phase and received mechanical ventilation for few days prior to the inclusion in this study. Under these conditions, patient lungs could have developed consolidation, namely, the presence of lung regions scarcely responsive to recruitment (Cressoni et al., 2017). In this case, the breaths with higher ΔPS received cyclically during variable pressure support might expose the patient to volutrauma in the aerated regions of the lung (Güldner et al., 2016; Pelosi et al., 2016) due to the reduced size of the lung aerated compartment. Another explanation for the possible lack of effect of variability on oxygenation might be that, different from what happens in PSV with a sigh, vPSV has no control over the time spent at higher pressure during tidal breathing. This might result in random breaths with higher PS and short inspiratory time, both possibly insufficient to achieve recruitment. The tidal volume measured in this cohort was higher than the recommended targets, but this reflects the current clinical practice in patients with ARDS receiving assisted ventilation modes (Bellani et al., 2016; Writing Group for the PReVENT Investigators et al., 2018). During the second block of ventilations, the patients tolerated a ΔPS reduction without worsening the gas exchange in the short term, at the price of a modest increase of the respiratory rate, suggesting that they were slightly over-assisted. This could have influenced patient-ventilator interaction (Kataoka et al., 2018) and the response to variability, as suggested by the finding that, during the second block of interventions, the variability of VT was increased by vPSV compared with PSV only when the baseline ΔPS was used. However, during ventilation steps with baseline ΔPS, patients had a work of breathing estimated with the PTPes of around 150 cmH2O·s·min−1, which is within the recommended range (Mauri et al., 2016). Interestingly, higher PS resulted in a reduction in ΔPES in the following breath at both set ΔPS levels, while the variability of VT and PL was increased by extrinsic variability only at higher ΔPS. This seems to suggest that while a neural response to extrinsic variability is present independent of the level of assistance, its effects on the variability of VT and PL are influenced by the level of ΔPS.
This study is underpowered to demonstrate the effects of vPSV on patient-centered outcomes like duration of ventilation. This is tested in another, yet ongoing clinical trial (Kiss et al., 2013). In the post-hoc analysis, the effects of vPSV on the response of patients in terms of inspiratory effort, transpulmonary pressure, and tidal volume developed in the following breath were studied. An inverse association between the ΔPS received in the preceding breath and the inspiratory effort was observed. Different from other modified PSV modes such as the proportional assist ventilation (PAV) and the neurally adjusted ventilatory assist (NAVA), the variability of ΔPS was random, i.e., is not related to the efforts of patients. This analysis suggests that there might be a complex interaction between the ventilator and a patient, in which the inspiratory effort and the adaptation of the patient to pressure support are influenced by the history of the previous breaths.
Limitations
This study has several limitations. The crossover design allowed the investigation of the effects of different levels of variability and ΔPS in terms of gas exchange and respiratory mechanics in the short term but is intrinsically unable to investigate major clinical outcomes. The sample size is relatively low, no static measurements of respiratory mechanics were performed, and patients received heterogeneous sedation regimens that might have affected differently the respiratory drive. The population included in the study identifies a subgroup of critically ill patients meeting the criteria for mild to moderate ARDS who already received controlled or assisted mechanical ventilation for several days; however, the baseline patient characteristics were similar to those reported in a recent large observational study in patients with ARDS assisted non-invasively (Bellani et al., 2017). These patients with established respiratory failure, thus, possibly consolidated lung areas, might not benefit from the cyclic recruitment effect of vPSV, while patients with early ARDS might respond differently. However, the role of spontaneous breathing in the early management of ARDS is still unclear. This study could neither elucidate the mechanisms of the neural responses of the patients to variability nor the neuromuscular coupling of the respiratory muscles.
Conclusion
In our cohort of patients with mild to moderate ARDS, vPSV did not improve gas exchange at different levels of variability and pressure support. Compared with PSV, vPSV increased the variability of VT, but not when low levels of variability were used in conjunction with lower pressure support. Moreover, vPSV did not exert a clinically relevant effect on the average inspiratory effort and work of breathing.
Data Availability Statement
The raw data supporting the conclusions of this article will be made available by the authors, without undue reservation.
Ethics Statement
The study was approved by the Local Ethical Review Board (Comitato Etico Aziendale Policlinico San Martino protocol no. 1052/12) and prospectively registered on clinicaltrials.gov (study identifier: NCT01683669). According to the local ethical requirements, the next of kin provided written informed assent, followed by delayed written consent from patients in case of recovery of consciousness.
Author Contributions
LB takes responsibility for the integrity of data. LB, PP, MV, and MG designed the study. LB, YS, MF, AD'O, DD'A, PRa, and IB conducted the study. LB, LM, MF, RH, AD'O, and CR analyzed the data. LB, MS, PP, PRo, and MG wrote the manuscript. All authors read and approved the final version of the manuscript.
Funding
This study was performed with institutional funding only.
Conflict of Interest
MG was granted a patent on the variable pressure support ventilation mode of assisted ventilation (noisy PSV), which has been licensed to Dräger Medical AG (Lübeck, Germany).
The remaining authors declare that the research was conducted in the absence of any commercial or financial relationships that could be construed as a potential conflict of interest.
Publisher's Note
All claims expressed in this article are solely those of the authors and do not necessarily represent those of their affiliated organizations, or those of the publisher, the editors and the reviewers. Any product that may be evaluated in this article, or claim that may be made by its manufacturer, is not guaranteed or endorsed by the publisher.
Supplementary Material
The Supplementary Material for this article can be found online at: https://www.frontiersin.org/articles/10.3389/fphys.2021.725738/full#supplementary-material
Abbreviations
PSV, pressure support ventilation; ARDS, acute respiratory distress syndrome; vPSV, variable pressure support ventilation; ΔPS, pressure support level; ICU, intensive care unit; PEEP, positive end-expiratory pressure; PBW, predicted body weight; SAPS, simplified acute physiology score; SOFA, sequential organ failure assessment score; RASS, richmond agitation-sedation scale; PSV0%, pressure support ventilation with no variability; vPSV15%, variable pressure support ventilation with 15% CV variability; vPSV30%, variable pressure support ventilation with 30% CV variability; VT, tidal volume; PTP, esophageal pressure-time product; ΔPes, esophageal pressure swings; PL, peak transpulmonary pressure; PSVBL, pressure support ventilation with no variability and baseline ΔPS as per clinical indication; vPSVBL, variable pressure support with variability set to achieve ±5 cmH2O and baseline ΔPS as per clinical indication; PSV−5, pressure support ventilation with no variability and ΔPS reduced by 5 cmH2O from the baseline value; vPSV−5, variable pressure support ventilation with variability set to achieve ±5 cmH2O and ΔPS reduced by 5 cmH2O from the baseline value.
References
Akoumianaki, E., Maggiore, S. M., Valenza, F., Bellani, G., Jubran, A., Loring, S. H., et al. (2014). The application of esophageal pressure measurement in patients with respiratory failure. Am. J. Respir. Crit. Care Med. 189, 520–531. doi: 10.1164/rccm.201312-2193CI
Bellani, G., Laffey, J. G., Pham, T., Fan, E., Brochard, L., Esteban, A., et al. (2016). Epidemiology, patterns of care, and mortality for patients with acute respiratory distress syndrome in intensive care units in 50 countries. JAMA 315, 788–800. doi: 10.1001/jama.2016.0291
Bellani, G., Laffey, J. G., Pham, T., Madotto, F., Fan, E., Brochard, L., et al. (2017). Noninvasive ventilation of patients with acute respiratory distress syndrome. Insights from the LUNG SAFE study. Am. J. Respir. Crit. Care Med. 195, 67–77. doi: 10.1164/rccm.201606-1306OC
Blanch, L., Villagra, A., Sales, B., Montanya, J., Lucangelo, U., Luján, M., et al. (2015). Asynchronies during mechanical ventilation are associated with mortality. Intensive Care Med. 41, 633–641. doi: 10.1007/s00134-015-3692-6
Cressoni, M., Chiumello, D., Algieri, I., Brioni, M., Chiurazzi, C., Colombo, A., et al. (2017). Opening pressures and atelectrauma in acute respiratory distress syndrome. Intensive Care Med. 43, 603–611. doi: 10.1007/s00134-017-4754-8
Esteban, A., Frutos-Vivar, F., Muriel, A., Ferguson, N. D., Peñuelas, O., Abraira, V., et al. (2013). Evolution of mortality over time in patients receiving mechanical ventilation. Am. J. Respir. Crit. Care Med. 188, 220–230. doi: 10.1164/rccm.201212-2169OC
Gama de Abreu, M., Spieth, P. M., Pelosi, P., Carvalho, A. R., Walter, C., Schreiber-Ferstl, A., et al. (2008). Noisy pressure support ventilation: a pilot study on a new assisted ventilation mode in experimental lung injury. Crit. Care Med. 36, 818–827. doi: 10.1097/01.CCM.0000299736.55039.3A
Güldner, A., Braune, A., Ball, L., Silva, P. L., Samary, C., Insorsi, A., et al. (2016). Comparative effects of volutrauma and atelectrauma on lung inflammation in experimental acute respiratory distress syndrome. Crit. Care Med. doi: 10.1097/CCM.0000000000001721
Huhle, R., Pelosi, P., and de Abreu, M. G. (2016). Variable ventilation from bench to bedside. Crit. Care Lond. Engl. 20:62. doi: 10.1186/s13054-016-1216-6
Kataoka, J., Kuriyama, A., Norisue, Y., and Fujitani, S. (2018). Proportional modes versus pressure support ventilation: a systematic review and meta-analysis. Ann. Intensive Care 8:123. doi: 10.1186/s13613-018-0470-y
Kiss, T., Güldner, A., Bluth, T., Uhlig, C., Spieth, P. M., Markstaller, K., et al. (2013). Rationale and study design of ViPS - variable pressure support for weaning from mechanical ventilation: study protocol for an international multicenter randomized controlled open trial. Trials 14:363. doi: 10.1186/1745-6215-14-363
Mauri, T., Lazzeri, M., Bronco, A., Bellani, G., and Pesenti, A. (2017). Effects of variable pressure support ventilation on regional homogeneity and aeration. Am. J. Respir. Crit. Care Med. 195, e27–e28. doi: 10.1164/rccm.201609-1806IM
Mauri, T., Yoshida, T., Bellani, G., Goligher, E. C., Carteaux, G., Rittayamai, N., et al. (2016). Esophageal and transpulmonary pressure in the clinical setting: meaning, usefulness and perspectives. Intensive Care Med. 42, 1360–1373. doi: 10.1007/s00134-016-4400-x
Muller, K. E., and Barton, C. N. (1989). Approximate power for repeated-measures ANOVA lacking sphericity. J. Am. Stat. Assoc. 84, 549–555. doi: 10.1080/01621459.1989.10478802
Muller, K. E., Lavange, L. M., Ramey, S. L., and Ramey, C. T. (1992). Power calculations for general linear multivariate models including repeated measures applications. J. Am. Stat. Assoc. 87, 1209–1226. doi: 10.1080/01621459.1992.10476281
Pelosi, P., Ball, L., Abreu, M. G., and de Rocco, P. R. M. (2016). General anesthesia closes the lungs: keep them resting. Turk. J. Anesth. Reanim. 44, 163–164. doi: 10.5152/TJAR.2016.002
Putensen, C., Zech, S., Wrigge, H., Zinserling, J., Stüber, F., Von Spiegel, T., et al. (2001). Long-term effects of spontaneous breathing during ventilatory support in patients with acute lung injury. Am. J. Respir. Crit. Care Med. 164, 43–49. doi: 10.1164/ajrccm.164.1.2001078
Spieth, P. M., Carvalho, A. R., Güldner, A., Kasper, M., Schubert, R., Carvalho, N. C., et al. (2011). Pressure support improves oxygenation and lung protection compared to pressure-controlled ventilation and is further improved by random variation of pressure support. Crit. Care Med. 39, 746–755. doi: 10.1097/CCM.0b013e318206bda6
Spieth, P. M., Güldner, A., Beda, A., Carvalho, N., Nowack, T., Krause, A., et al. (2012). Comparative effects of proportional assist and variable pressure support ventilation on lung function and damage in experimental lung injury. Crit. Care Med. 40, 2654–2661. doi: 10.1097/CCM.0b013e3182592021
Spieth, P. M., Güldner, A., Huhle, R., Beda, A., Bluth, T., Schreiter, D., et al. (2013). Short-term effects of noisy pressure support ventilation in patients with acute hypoxemic respiratory failure. Crit. Care Lond. Engl. 17:R261. doi: 10.1186/cc13091
Tobin, M. J., Mador, M. J., Guenther, S. M., Lodato, R. F., and Sackner, M. A. (1988). Variability of resting respiratory drive and timing in healthy subjects. J. Appl. Physiol. (1985) 65, 309–317. doi: 10.1152/jappl.1988.65.1.309
Writing Group for the PReVENT Investigators, Simonis, F. D., Serpa Neto, A., Binnekade, J. M., Braber, A., Bruin, K. C. M., et al. (2018). Effect of a low vs intermediate tidal volume strategy on ventilator-free days in intensive care unit patients without ARDS: a randomized clinical trial. JAMA 320, 1872–1880. doi: 10.1001/jama.2018.14280
Keywords: variable pressure support ventilation, acute respiratory distress (ARDS), asynchronies, respiratory mechanic, assisted ventilation
Citation: Ball L, Sutherasan Y, Fiorito M, Dall'Orto A, Maiello L, Vargas M, Robba C, Brunetti I, D'Antini D, Raimondo P, Huhle R, Schultz MJ, Rocco PRM, Gama de Abreu M and Pelosi P (2021) Effects of Different Levels of Variability and Pressure Support Ventilation on Lung Function in Patients With Mild–Moderate Acute Respiratory Distress Syndrome. Front. Physiol. 12:725738. doi: 10.3389/fphys.2021.725738
Received: 15 June 2021; Accepted: 17 September 2021;
Published: 22 October 2021.
Edited by:
Luigi Camporota, Guy's and St Thomas' NHS Foundation Trust, United KingdomReviewed by:
Elena Spinelli, IRCCS Ca 'Granda Foundation Maggiore Policlinico Hospital, ItalyLise Piquilloud, Centre Hospitalier Universitaire Vaudois (CHUV), Switzerland
Copyright © 2021 Ball, Sutherasan, Fiorito, Dall'Orto, Maiello, Vargas, Robba, Brunetti, D'Antini, Raimondo, Huhle, Schultz, Rocco, Gama de Abreu and Pelosi. This is an open-access article distributed under the terms of the Creative Commons Attribution License (CC BY). The use, distribution or reproduction in other forums is permitted, provided the original author(s) and the copyright owner(s) are credited and that the original publication in this journal is cited, in accordance with accepted academic practice. No use, distribution or reproduction is permitted which does not comply with these terms.
*Correspondence: Lorenzo Ball, lorenzo.ball@unige.it
†These authors share senior authorship