Corrigendum: Targeting Endolysosomal Two-Pore Channels to Treat Cardiovascular Disorders in the Novel COronaVIrus Disease 2019
- 1Laboratory of General Physiology, Department of Biology and Biotechnology “L. Spallanzani”, University of Pavia, Pavia, Italy
- 2Department of Medicine and Health Sciences “V. Tiberio”, University of Molise, Campobasso, Italy
- 3Section of Human Anatomy, Department of Mental and Physical Health and Preventive Medicine, University of Campania “Luigi Vanvitelli”, Naples, Italy
- 4Department of Brain and Behavioral Sciences, University of Pavia, Pavia, Italy
- 5School of Medicine, Department of Biomedicine, Benemérita Universidad Autónoma de Puebla, Puebla, Mexico
Emerging evidence hints in favor of a life-threatening link between severe acute respiratory syndrome coronavirus type 2 (SARS-CoV-2) and the cardiovascular system. SARS-CoV-2 may result in dramatic cardiovascular complications, whereas the severity of COronaVIrus Disease 2019 (COVID-19) and the incidence of fatalities tend to increase in patients with pre-existing cardiovascular complications. SARS-CoV-2 is internalized into the host cells by endocytosis and may then escape the endolysosomal system via endosomes. Two-pore channels drive endolysosomal trafficking through the release of endolysosomal Ca2+. Recent evidence suggested that the pharmacological inhibition of TPCs prevents Ebola virus and Middle East Respiratory Syndrome COronaVirus (MERS-CoV) entry into host cells. In this perspective, we briefly summarize the biophysical and pharmacological features of TPCs, illustrate their emerging role in the cardiovascular system, and finally present them as a reliable target to treat cardiovascular complications in COVID-19 patients.
Introduction
The dramatic outbreak of the COronaVIrus Disease 2019 (COVID-19), which is caused by the novel severe acute respiratory syndrome coronavirus type 2 (SARS-CoV-2), is imposing an unmet medical need to the scientific community. SARS-CoV-2 causes viral pneumonia and acute respiratory distress syndrome (ARDS), which may be associated with a multiorgan dysfunction resulting from the cytokine storm produced by viral infection (Huang et al., 2020; Wang et al., 2020; Zhou et al., 2020). Furthermore, SARS-CoV-2 may induce major cardiovascular complications, including myocarditis and myocardial injury, acute myocardial infarction (AMI), arrhythmia, acute coronary syndrome, venous thromboembolism and stroke (Moccia et al., 2020a; Nishiga et al., 2020; Zheng et al., 2020). Moreover, a high prevalence of pre-existing CV comorbidities, such as hypertension and coronary heart disease, has been observed among COVID-19 patients and associated with worse clinical outcome and increased risk of death (Moccia et al., 2020a; Nishiga et al., 2020; Zheng et al., 2020). Coronaviruses, including those responsible for Middle East Respiratory Syndrome (MERS) and SARS-CoV, as well as Ebola virus, exploit the endolysosomal (EL) compartment to infect host cells (Burkard et al., 2014; Falzarano and Feldmann, 2015; Chao et al., 2020). Likewise, SARS-CoV-2 uses the viral spike (S) protein to bind the membrane-bound angiotensin-converting enzyme 2 (ACE2) and then undergoes rapid endocytosis to gain access into the host cell (Coutard et al., 2020; Hoffmann et al., 2020; Walls et al., 2020). ACE2 is a metallopeptidase that plays a crucial role in the cardiovascular system by cleaving angiotensin I to produce angiotensin 1–9; this, in turn, stimulates the angiotensin II type 2 receptor (AT2R) to induce nitric oxide (NO) release, to reduce the vascular tone, and to protect myocardium against ischemia-reperfusion (IR) injury (Mendoza-Torres et al., 2018; Paz Ocaranza et al., 2020). ACE2 is widely expressed in cardiac myocytes, vascular smooth muscle cells (VSMCs), and vascular endothelial cells (Patel et al., 2014; Chen et al., 2020; Evans et al., 2020; Nicin et al., 2020). In agreement with these observations, SARS-CoV-2 infected endothelial cells in several vascular beds (Varga et al., 2020), including cerebral capillaries (Paniz-Mondolfi et al., 2020), and induced endothelial dysfunction associated with apoptosis (Varga et al., 2020; Yin et al., 2020). Conversely, the presence of SARS-CoV-2 in cardiomyocytes is yet to be shown, although COVID-19 may induce myocarditis and AMI. In the present perspective, we discuss the possibility of targeting two-pore channels (TPCs), which are crucial to endocytosis and EL trafficking (Chao et al., 2020; Vassileva et al., 2020), to interfere with SARS-CoV-2 entry into cardiovascular cells.
Basic Insights Into EL Ca2+ Signaling: The Role of TPCs in the Cardiovascular System
The EL Ca2+ store comprises a plethora of organelles enriched with H+ and Ca2+, which are widely distributed across the phylogenetic tree, and includes: lysosomes, lysosome-related organelles, secretory vesicles, vacuoles, acidocalcisomes, and the Golgi apparatus (Patel and Docampo, 2010; Faris et al., 2018). The free Ca2+ concentration in the EL compartment may vary from 2 to 3 μM in early endosomes (EEs) up to 500–600 μM in lysosomes, and therefore emerges as the second largest Ca2+ store in mammalian cells after the endoplasmic reticulum (ER) (Morgan et al., 2011). Lysosomal Ca2+ refilling is supported by the negative (−20 mV/−40 mV, lumen positive) resting lysosomal membrane potential (ΔΨ) and is driven by the H+-gradient, also known as proton-motive force, established across the lysosomal membrane by the vacuolar type H+-ATPase (v-ATPase) (Morgan et al., 2011; Xu and Ren, 2015; Faris et al., 2018). EL Ca2+ uptake may be prevented by blocking the v-ATPase activity with concanamycin or bafilomycin A1 and by increasing intravesicular pH with membrane-permeant bases (i.e., NH4Cl) or protonophores (i.e., monensin and nigericin) (Morgan et al., 2011; Faris et al., 2018, 2019). A lysosomal Ca2+/H+ exchanger (CAX) has been detected in non-placental mammalian cells, but not in higher vertebrates (Melchionda et al., 2016). The molecular mechanism which senses the intraluminal acidic pH to drive EL Ca2+ refilling remains, therefore, to be fully elucidated.
EL Ca2+ release may occur through multiple Ca2+-permeable pathways (Figure 1), including TPCs and Transient Receptor Potential Mucolipin 1 (TRPML1), TRPML2 and TRPML3 (Patel, 2015; Faris et al., 2018). TPCs belong to the super-family of voltage-gated ion channels and represent a molecular intermediate in the transition from a primordial two-domain channel, which underwent two rounds of intragenic duplication to generate, respectively, the tetrameric voltage-gated K+ channels (KV) and the monomeric voltage-gated Na+ (NaV) and Ca2+ (CaV) channels (Rahman et al., 2014). The TPC gene family encompasses three members, TPCN1, TPCN2, and TPCN3, which, respectively, encode for TPC1, TPC2, and TPC3. However, only TPC1 and TPC2 are expressed in primates and rodents (Brailoiu et al., 2010a; Cai and Patel, 2010; Patel, 2015). TPC1 is mainly found in EEs and recycling endosomes, while TPC2 is more abundant in late endosomes (LEs) and lysosomes (Patel, 2015; Grimm et al., 2017a). Nicotinic acid adenine dinucleotide phosphate (NAADP), the most powerful Ca2+-releasing second messenger, has been shown to activate TPCs and mobilize EL Ca2+ in response to extracellular stimulation (Galione, 2015, 2019). In addition, TPCs are activated by phosphatidylinositol 3,5-bisphosphate [PI(3,5)P2], which is the most abundant phosphatidylinositol in LEs and lysosomes, to mediate Na+ and Ca2+-permeable channels (Jha et al., 2014). A recent investigation disclosed that TPC ion-selectivity is finely regulated by the gating stimulus: NAADP and the synthetic compound TPC2-A1-N elicit non-selective Ca2+-permeable currents, which lead to large increase in [Ca2+]i, while PI(3,5)P2 and the synthetic compound TPC2-A1-P activate Na+-selective currents, which result in weaker intracellular Ca2+ signals (Gerndt et al., 2020). The synthetic NAADP analog, NED-19, is widely employed to prevent NAADP binding to TPCs, thereby inhibiting NAADP-induced EL Ca2+ release (Patel, 2015; Pitt et al., 2016). Furthermore, molecular docking studies showed that a number of CaV and NaV antagonists, including dihydropyridines (e.g., nifedipine and nitrendipine), phenylalkylamines (e.g., verapamil), and local anaesthetics (e.g., lidocaine), directly block the TPC pore and inhibit NAADP-induced Ca2+ release (Genazzani et al., 1997; Rahman et al., 2014). Finally, TPCs are sensitive to tetrandrine (Sakurai et al., 2015), a plant-derived bis-benzylisoquinoline alkaloid, which is widely employed in traditional Chinese medicine and may also serve as CaV antagonist (Yao and Jiang, 2002).
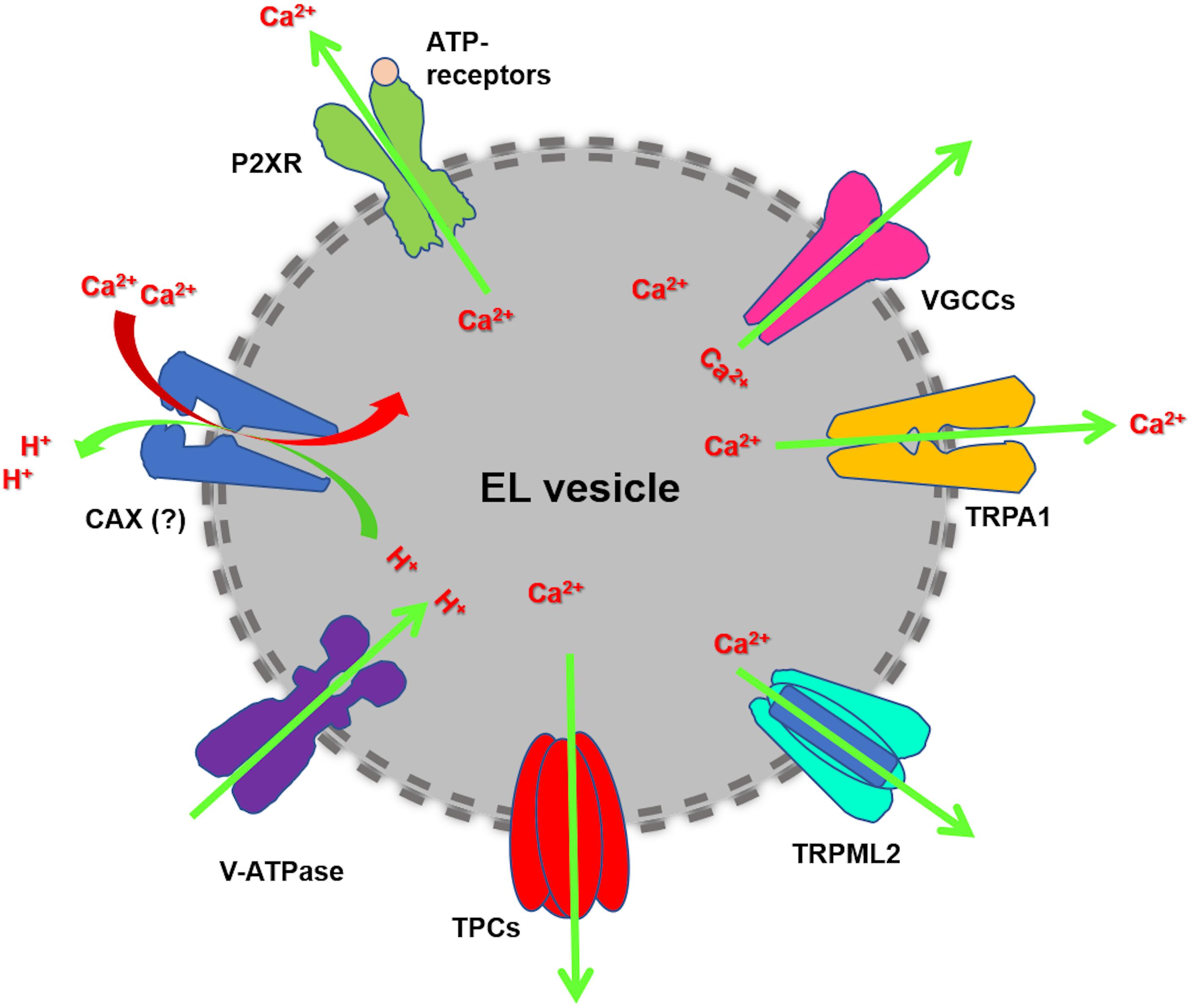
Figure 1. EL Ca2+ handling machinery. Cytosolic Ca2+ is sequestrated into EL vesicles by a pH-sensitive mechanism, which is maintained by the acidic intraluminal pH established through the v-ATPase. The existence of Ca2+/H+ exchanger (CAX) has been postulated in placental mammalian cells. EL Ca2+ mobilization may mainly occur through TPC1-2 and TRPML1. Additional EL Ca2+-permeable pathways can be provided by TRP Melastatin 2 (TRPM2), TRP Ankyrin 1 (TRPA1), voltage-gated Ca2+ channels (VGCCs) and ATP-gated ionotropic P2X receptors.
TPCs may trigger global Ca2+ signals evoked by a growing number of extracellular stimuli (Galione, 2015, 2019). NAADP-induced EL Ca2+ release may be amplified by Ca2+-induced Ca2+ release (CICR) via inositol-1,4,5-trisphosphate (InsP3) receptors (InsP3Rs) and ryanodine receptors (RyRs), possibly at junctions between acidic organelles and the ER (Galione, 2015; Penny et al., 2015; Kilpatrick et al., 2017). Ultrastructural analysis revealed that lysosomes may form close associations with the Sarcoplasmic Reticulum (SR) in both cardiac myocytes (Aston et al., 2017) and VSMCs (Kinnear et al., 2004, 2008; Fameli et al., 2014). It has been shown that β-adrenoreceptor stimulation engages NAADP-induced Ca2+ release through TPC2 to increase the SR Ca2+ load, thereby increasing SR Ca2+ release via RyR2 and increase cardiac contraction (Macgregor et al., 2007; Collins et al., 2011; Lewis et al., 2012). Prolonged β-adrenoreceptor stimulation may, thus, result in ventricular arrhythmia and cardiac hypertrophy in TPC2 wild-type, but not knockout, mice (Capel et al., 2015). On the other hand, TPC1 may contribute to IR injury in cardiac myocytes, by triggering the cytosolic Ca2+ overload and apoptotic cell death (Davidson et al., 2015). Likewise, multiple agonists, such as endothelin 1 and angiotensin II, recruit NAADP-induced EL Ca2+ release through TPC2 to trigger CICR through RyR3 and to promote vasoconstriction (Kinnear et al., 2004; Jiang et al., 2013; Lee et al., 2015; Trufanov et al., 2019). Ultrastructural investigations showed that TPC2 is closely apposed to RyR3 at lysosomal-ER nanojunctions, which tend to cluster in the perinuclear area and provide an ideal signaling platform to amplify the local Ca2+ response to extracellular stimuli (Kinnear et al., 2004, 2008; Fameli et al., 2014). Exaggerated NAADP signaling could be induced by hypoxia in pulmonary artery VSMCs and trigger the complex process of vascular remodeling that leads to pulmonary arterial hypertension (Jiang et al., 2018). Finally, TPCs are emerging as crucial players in endothelial Ca2+ dynamics (Moccia et al., 2019; Zuccolo et al., 2019a). NAADP activates endothelial TPCs to induce the global Ca2+ signals which control NO release and blood pressure (Brailoiu et al., 2010c), secretion of von Willebrand factor (vWF) and platelet aggregation (Esposito et al., 2011), neurovascular coupling (Negri et al., 2019; Zuccolo et al., 2019b; Berra-Romani et al., 2020), angiogenesis (Favia et al., 2014) and vasculogenesis (Zuccolo et al., 2016; Di Nezza et al., 2017). A recent investigation confirmed that, also in the endothelial lineage, NAADP-induced Ca2+ release through TPCs may be amplified into regenerative intracellular Ca2+ oscillations by the Ca2+-dependent recruitment of InsP3Rs (Moccia et al., 2020b).
The Role of TPCs in the Regulation of Lysosomal Functions and Endocytosis
When TPCs are not coupled to juxtaposed RyRs or InsP3Rs, EL Ca2+ signals remain spatially restricted around the EL membrane, thereby regulating lysosomal morphology, transport, and fusion events (Grimm et al., 2017b; Lloyd-Evans and Waller-Evans, 2019; Vassileva et al., 2020). Local EL Ca2+ signals were shown to regulate endocytosis and vesicular trafficking of membrane receptors and protein toxins. For instance, knockout of TPC2 induced epidermal growth factor (EGF) receptor and low-density lipoprotein (LDL) receptor accumulation in LEs (Grimm et al., 2014), and delayed platelet derived growth factor (PDGF) receptor internalization and degradation (Ruas et al., 2014). Similarly, genetic deletion of either TPC1 or TPC2 induced integrin accumulation within EEs (Nguyen et al., 2017). Furthermore, knockout of TPC1 halted the uptake and trafficking through the early endocytic route of the so-called “short trip” bacterial toxins, such as diphteria and anthrax toxins (Castonguay et al., 2017). Three independent interactome screens revealed that TPCs are closely associated with Q- and R-SNARE proteins, which orchestrate intravesicular membrane dynamics (Grimm et al., 2014; Lin-Moshier et al., 2014; Castonguay et al., 2017; Krogsaeter et al., 2019). The Ca2+-sensor that couples EL Ca2+ release to endosomal membrane fusion events is yet to be identified, but could include a member of the Annexin family of Ca2+-binding membrane proteins (ANXA1–7), which are also part of the TPC interactome (Lin-Moshier et al., 2014; Castonguay et al., 2017). Notably, TPC-mediated local Ca2+ signals may also control infection and intracellular trafficking of life-threatening viral pathogens, including EBOV (Sakurai et al., 2015) and MERS-CoV (Gunaratne et al., 2018b).
TPCs Mediate Entry and Trafficking of Viruses in Host Cells
The EL compartment provides a route that can be highjacked by viruses to penetrate into the cytosol of host cells for replication (Burkard et al., 2014; Falzarano and Feldmann, 2015; Chao et al., 2020). EBOV has long been regarded as the main causative pathogen of Ebola hemorrhagic fever or EBOV disease (EVD), which is associated with high-fatality rates in humans and primates (Feldmann and Geisbert, 2011). Following attachment to the surface of host cells, EBOV is internalized via micropinocytosis and then trafficked through EEs and LEs, where the surface viral glycoproteins (GPs) are cleaved by the lysosomal cysteine proteases, cathepsin B and L, and primed for fusion. Subsequently, viral GPs interact with their late endosomal/lysosomal receptor, protein Neimann-Pick C1 (NPC-1), which causes the viral envelope to fuse with the endosomal membrane and release the nucleocapside into the cytosol. Herein, the viral genome is transcribed and replicated, followed by the assembly of viral proteins and virions budding from the cell (White and Schornberg, 2012; Salata et al., 2019). A landmark study by Sakurai et al. (2015) revealed that genetic silencing or knockdown of either TPC1 or TPC2 inhibited EBOV infection in vitro. The same result was obtained by overexpressing a dominant-negative TPC2 mutant in host cells (Brailoiu et al., 2010b). The molecular deletion of TPCs interfered with virus-endosome membrane fusion and prevented nucleocapside release into the cytosol, thereby impeding viral replication (Sakurai et al., 2015). EBOV infection in vitro was also strongly repressed by blocking TPCs with NED-19 and with three structurally distinct inhibitors of L-type voltage-gated Ca2+ channels, i.e., verapamil, diltiazem, and nimodipine (Sakurai et al., 2015). These findings suggested that CaV antagonists, which have been approved by the Food and Drug Administration (FDA) for the treatment of multiple cardiovascular disorders (Godfraind, 2017; Oparil et al., 2018), could represent an alternative strategy to combat Ebola hemorrhagic fever. However, the Chinese medicinal herb, tetrandrine, was the most powerful inhibitor of EBOV infection in vitro and was therapeutically effective also in in vivo mouse models (Sakurai et al., 2015). Notably, tetrandrine inhibited NAADP- and PI(3,5)P2-evoked lysosomal currents and attenuated NAADP-induced intracellular Ca2+ release in living cells (Sakurai et al., 2015). A recent screening campaign confirmed that TPC2 activation regulates EBOV entry in host cells. Measurement of PI(3,5)P2-evoked lysosomal currents, NAADP-induced Ca2+ release and single-channel activity, revealed that FDA-approved dopamine antagonists, such as pimozide and fluphenazine, and selective estrogen receptor modulators, such as clomiphene and raloxifene, were also able to target TPC2 by plugging the channel pore (Penny et al., 2019). Furthermore, these novel inhibitors of TPC2 effectively reduced EBOV infection in vitro (Penny et al., 2019).
Subsequently, TPC2 was found to also regulate MERS-CoV infectivity. MERS-CoV has been isolated for the first time in Saudi Arabia in 2012 and to date has affected > 1,500 individuals worldwide, with a high fatality rate (∼30–50%) (Chan et al., 2015; Memish et al., 2020). The viral S protein binds to the transmembrane receptor, dipeptidyl peptidase 4 (DPP4), and is then primed for fusion with the cell membrane by multiple proteases, including furin, trypsin, and transmembrane protease/serine subfamily member 2 (TMPRSS2) (Chan et al., 2015; V’kovski et al., 2020). In the absence of cell surface proteases, MERS-CoV entry is mediated by clathrin-mediated endocytosis followed by S protein cleavage by the endosomal cathepsins B and L (Shirato et al., 2013; Chan et al., 2015). This, in turn, triggers S protein-dependent fusion of viral and endosomal membranes, which results in the release of viral genome into the cytoplasm (Shirato et al., 2013; Chan et al., 2015). Genetic silencing of endogenous TPC1 or TPC2, but not TRPML1, reduced MERS-CoV infectivity (Gunaratne et al., 2018a). The same inhibitory effect on MERS-CoV infection was achieved by overexpressing TPC1 or TPC2 (Gunaratne et al., 2018a), a maneuver that interferes with EL trafficking. Likewise, MERS-CoV infectivity was reduced by blocking TPCs with CaV antagonists, i.e., nifedipine, nimodipine and nicardipine, NaV antagonists, i.e., benzocaine and procaine, and bisbenzylisoquinoline alkaloids, including fangchinoline, thaligine, and tetrandrine (Gunaratne et al., 2018a). Fangchinoline and thaligine were the most effective drugs at impairing MERS-CoV entry into host cells and Ca2+ imaging confirmed that fangchinoline blocked NAADP-induced intracellular Ca2+ release in living cells (Gunaratne et al., 2018a). Furthermore, MERS-CoV infection was impaired by reducing PI(3,5)P2 levels (Gunaratne et al., 2018a), which suggests that PI(3,5)P2-dependent regulation of TPCs may play a crucial role in controlling MERS-CoV infection. The role of EL Ca2+ release in mediating this process was further suggested by the inhibitory effect on MERS-CoV infectivity of chloroquine and the weak base ammonium chloride (NH4Cl) (Gunaratne et al., 2018a). These drugs inhibit cathepsin activity and, therefore, prevent S protein activation, by neutralizing EL pH (Chan et al., 2015). However, an increase in EL pH also represents a widely employed strategy to deplete the EL Ca2+ store, as intraluminal Ca2+ reloading impinges on the proton-motrive force (Morgan et al., 2011; Faris et al., 2018). Furthermore, bafilomycin A1, which causes an increase in EL pH by inhibiting the v-ATPase activity and discharging the EL Ca2+ pool (Faris et al., 2019; Moccia et al., 2020b), may also reduce MERS-CoV infection in vitro (Gunaratne et al., 2018a). Therefore, TPC-mediated EL Ca2+ mobilization may be regarded as a crucial regulator of MERS-CoV entry in host cells.
TPCs as Putative Targets to Prevent SARS-COV-2 Infection in the Cardiovascular System
SARS-CoV-2 entry in host cells is mediated by pH-dependent endocytosis and requires proteolytic priming of the viral S protein by furin, TMPRSS2 and, to a lesser extent, cathepsins B and L (Coutard et al., 2020; Hoffmann et al., 2020; Walls et al., 2020). Following successful endocytosis, SARS-CoV-2 escapes from the EL compartment and replicates in the cytosol from which it can spread to adjacent cells (Figure 2; Coutard et al., 2020; Hoffmann et al., 2020; Walls et al., 2020). Two pieces of evidence suggest that TPCs may orchestrate SARS-CoV-2 trafficking toward LEs/lysosomes and control the release of viral mRNA into the cytosol (Figure 2). First, SARS-CoV-2 infectivity is reduced by preventing EL Ca2+ refilling with NH4Cl and chloroquine (Hoffmann et al., 2020), whereas bafilomycin A1 blocks SARS-CoV translocation (Yang and Shen, 2020). Second, SARS-CoV-2 endocytosis is inhibited by inhibiting TPCs with tetrandrine and by preventing PI(3,5)P2 production with PIKfyve (Ou et al., 2020). As aforementioned, tetrandrine does not selectively target TPCs and, therefore, additional studies exploiting the genetic manipulation and/or more specific blockers (e.g., NED-19) of TPCs are required to confirm their involvement in SARS-CoV-2 infection. Nevertheless, there is an emerging consensus supporting the permissive role of TPCs in SARS-CoV-2 endocytosis and EL trafficking (Chao et al., 2020; Moccia et al., 2020a; Vassileva et al., 2020; Yang and Shen, 2020). Therefore, we put forward the hypothesis that the pharmacological blockade of TPCs could represent a promising strategy to prevent/attenuate the detrimental consequences of COVID-19 on the cardiovascular system, where ACE2 is widely expressed and which can be directly infected by SARS-CoV-2. This hypothesis would further support the suggested beneficial effect of chloroquine, which may prevent EL Ca2+ refilling, in COVID-19 patients (Inciardi et al., 2020). Unfortunately, this drug is known to induce pro-arrhythmic events at high doses (Mazzanti et al., 2020) and more recent reports failed to validate it as an effective drug against SARS-CoV-2 (Carafoli, 2020). Nevertheless, its efficacy against viral infection endorses the pharmacological inhibition of TPC-mediated EL Ca2+ release as a promising therapeutic option.
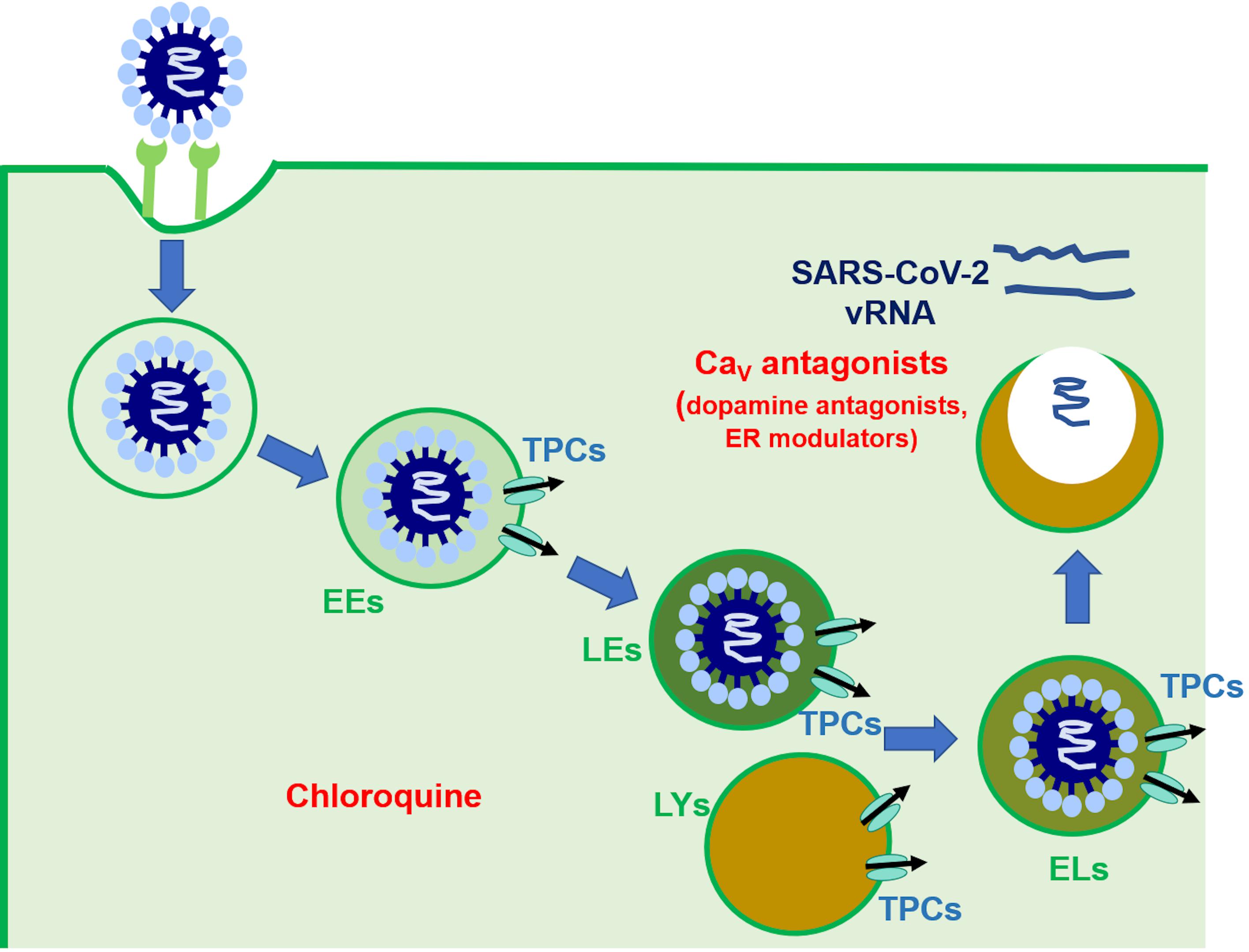
Figure 2. The putative role of TPCs in SARS-CoV-2 endocytosis and EL trafficking. This picture describes the mechanism whereby SARS-CoV-2 has been suggested to penetrate and replicate in host cells. It has been suggested that the endocytosis, onward trafficking, and liberation of SARS-CoV-2 is finely regulated by EL TPCs (see text for further details). The site of action of chloroquine and CaV antagonists [as well as dopamine antagonists and estrogen receptor (ER) modulators] were shown.
Conclusion
Herein, we discussed the hypothesis to target EL TPCs to interfere with the infection of cardiovascular cells, including cardiomyocytes, VSMCs, and vascular endothelial cells, by SARS-CoV-2. The cardiovascular system displays a dangerous sensitivity to SARS-CoV-2-dependent infection, as documented by the cardiovascular complications associated with COVID-19 and to the high incidence of infection among subjects suffering from cardiovascular comorbidities. The pharmacology of TPCs includes established CaV antagonists (Figure 2), that are already in use to treat cardiovascular disorders, including hypertension, acute coronary syndrome, and AMI, and has been recently expanded by novel FDA-approved drugs (Figure 2), such as dopamine antagonists and selective estrogen receptor modulators. We suggest that pre-clinical and clinical studies should investigate the protective effects of these drugs on the cardiovascular system in COVID-19 patients. The role of TPCs in SARS-CoV-2 endocytosis in cardiovascular cells remains, however, to be firmly established.
Data Availability Statement
The original contributions presented in the study are included in the article/supplementary material, further inquiries can be directed to the corresponding author/s.
Author Contributions
FM drafted the manuscript and supervised the work. All authors contributed to the preparation of the manuscript and approved the submitted version.
Funding
The authors gratefully acknowledge financial support from: Italian Ministry of Education, University and Research (MIUR): Dipartimenti di Eccellenza Program (2018–2022)—Department of Biology and Biotechnology “L. Spallanzani,” University of Pavia (FM); Fondo Ricerca Giovani from the University of Pavia (FM); EU Horizon 2020 FETOPEN-2018-2020 Programme “LION-HEARTED,” grant agreement n. 828984 (FM).
Conflict of Interest
The authors declare that the research was conducted in the absence of any commercial or financial relationships that could be construed as a potential conflict of interest.
Acknowledgments
The manuscript is dedicated to the memory of Prof. Laura Botta, a physiologist from the University of Pavia, who passed by on June 18th, 2020.
References
Aston, D., Capel, R. A., Ford, K. L., Christian, H. C., Mirams, G. R., Rog-Zielinska, E. A., et al. (2017). High resolution structural evidence suggests the sarcoplasmic reticulum forms microdomains with Acidic Stores (lysosomes) in the heart. Sci. Rep. 7:40620.
Berra-Romani, R., Faris, P., Pellavio, G., Orgiu, M., Negri, S., Forcaia, G., et al. (2020). Histamine induces intracellular Ca(2+) oscillations and nitric oxide release in endothelial cells from brain microvascular circulation. J. Cell. Physiol. 235, 1515–1530. doi: 10.1002/jcp.29071
Brailoiu, E., Hooper, R., Cai, X., Brailoiu, G. C., Keebler, M. V., Dun, N. J., et al. (2010a). An ancestral deuterostome family of two-pore channels mediates nicotinic acid adenine dinucleotide phosphate-dependent calcium release from acidic organelles. J. Biol. Chem. 285, 2897–2901. doi: 10.1074/jbc.c109.081943
Brailoiu, E., Rahman, T., Churamani, D., Prole, D. L., Brailoiu, G. C., Hooper, R., et al. (2010b). An NAADP-gated two-pore channel targeted to the plasma membrane uncouples triggering from amplifying Ca2+ signals. J. Biol. Chem. 285, 38511–38516. doi: 10.1074/jbc.m110.162073
Brailoiu, G. C., Gurzu, B., Gao, X., Parkesh, R., Aley, P. K., Trifa, D. I., et al. (2010c). Acidic NAADP-sensitive calcium stores in the endothelium: agonist-specific recruitment and role in regulating blood pressure. J. Biol. Chem. 285, 37133–37137. doi: 10.1074/jbc.c110.169763
Burkard, C., Verheije, M. H., Wicht, O., Van Kasteren, S. I., Van Kuppeveld, F. J., Haagmans, B. L., et al. (2014). Coronavirus cell entry occurs through the endo-/lysosomal pathway in a proteolysis-dependent manner. PLoS Pathog. 10:e1004502. doi: 10.1371/journal.ppat.1004502
Cai, X., and Patel, S. (2010). Degeneration of an intracellular ion channel in the primate lineage by relaxation of selective constraints. Mol. Biol. Evol. 27, 2352–2359. doi: 10.1093/molbev/msq122
Capel, R. A., Bolton, E. L., Lin, W. K., Aston, D., Wang, Y., Liu, W., et al. (2015). Two-pore channels (TPC2s) and nicotinic acid adenine dinucleotide phosphate (NAADP) at lysosomal-sarcoplasmic reticular junctions contribute to acute and chronic beta-adrenoceptor signaling in the heart. J. Biol. Chem. 290, 30087–30098. doi: 10.1074/jbc.m115.684076
Carafoli, E. (2020). Chloroquine and hydroxychloroquine in the prophylaxis and therapy of COVID-19 infection. Biochem. Biophys. Res. Commun. [Epub ahead of print]. doi: 10.1016/j.bbrc.2020.09.128
Castonguay, J., Orth, J. H. C., Muller, T., Sleman, F., Grimm, C., Wahl-Schott, C., et al. (2017). The two-pore channel TPC1 is required for efficient protein processing through early and recycling endosomes. Sci. Rep. 7:10038.
Chan, J. F., Lau, S. K., To, K. K., Cheng, V. C., Woo, P. C., and Yuen, K. Y. (2015). Middle east respiratory syndrome coronavirus: another zoonotic betacoronavirus causing SARS-like disease. Clin. Microbiol. Rev. 28, 465–522. doi: 10.1128/cmr.00102-14
Chao, Y. K., Chang, S. Y., and Grimm, C. (2020). Endo-lysosomal cation channels and infectious diseases. Rev. Physiol. Biochem. Pharmacol. [Epub ahead of print]. doi: 10.1007/112_2020_31
Chen, L., Li, X., Chen, M., Feng, Y., and Xiong, C. (2020). The ACE2 expression in human heart indicates new potential mechanism of heart injury among patients infected with SARS-CoV-2. Cardiovasc. Res. 116, 1097–1100. doi: 10.1093/cvr/cvaa078
Collins, T. P., Bayliss, R., Churchill, G. C., Galione, A., and Terrar, D. A. (2011). NAADP influences excitation-contraction coupling by releasing calcium from lysosomes in atrial myocytes. Cell Calcium 50, 449–458. doi: 10.1016/j.ceca.2011.07.007
Coutard, B., Valle, C., De Lamballerie, X., Canard, B., Seidah, N. G., and Decroly, E. (2020). The spike glycoprotein of the new coronavirus 2019-nCoV contains a furin-like cleavage site absent in CoV of the same clade. Antiviral Res. 176:104742. doi: 10.1016/j.antiviral.2020.104742
Davidson, S. M., Foote, K., Kunuthur, S., Gosain, R., Tan, N., Tyser, R., et al. (2015). Inhibition of NAADP signalling on reperfusion protects the heart by preventing lethal calcium oscillations via two-pore channel 1 and opening of the mitochondrial permeability transition pore. Cardiovasc. Res. 108, 357–366. doi: 10.1093/cvr/cvv226
Di Nezza, F., Zuccolo, E., Poletto, V., Rosti, V., De Luca, A., Moccia, F., et al. (2017). Liposomes as a putative tool to investigate NAADP signaling in vasculogenesis. J. Cell. Biochem. 118, 3722–3729. doi: 10.1002/jcb.26019
Esposito, B., Gambara, G., Lewis, A. M., Palombi, F., D’alessio, A., Taylor, L. X., et al. (2011). NAADP links histamine H1 receptors to secretion of von Willebrand factor in human endothelial cells. Blood 117, 4968–4977. doi: 10.1182/blood-2010-02-266338
Evans, P. C., Rainger, G., Mason, J. C., Guzik, T. J., Osto, E., and Stamataki, Z., et al. (eds) (2020). Endothelial dysfunction in COVID-19: a position paper of the ESC working group for atherosclerosis and vascular biology, and the ESC council of basic cardiovascular science. Cardiovasc Res. 116, 2177–2184. doi: 10.1093/cvr/cvaa230
Falzarano, D., and Feldmann, H. (2015). Virology. Delineating ebola entry. Science 347, 947–948. doi: 10.1126/science.aaa8121
Fameli, N., Ogunbayo, O. A., Van Breemen, C., and Evans, A. M. (2014). Cytoplasmic nanojunctions between lysosomes and sarcoplasmic reticulum are required for specific calcium signaling. F1000Reserach 3:93. doi: 10.12688/f1000research.3720.1
Faris, P., Pellavio, G., Ferulli, F., Di Nezza, F., Shekha, M., Lim, D., et al. (2019). Nicotinic acid adenine dinucleotide phosphate (NAADP) induces intracellular Ca(2+) release through the two-pore channel TPC1 in metastatic colorectal cancer cells. Cancers 11:E542.
Faris, P., Shekha, M., Montagna, D., Guerra, G., and Moccia, F. (2018). Endolysosomal Ca(2+) signalling and cancer hallmarks: two-pore channels on the move, TRPML1 lags behind!. Cancers 11:27. doi: 10.3390/cancers11010027
Favia, A., Desideri, M., Gambara, G., D’alessio, A., Ruas, M., Esposito, B., et al. (2014). VEGF-induced neoangiogenesis is mediated by NAADP and two-pore channel-2-dependent Ca2+ signaling. Proc. Natl. Acad. Sci. U.S.A. 111, E4706–E4715.
Feldmann, H., and Geisbert, T. W. (2011). Ebola haemorrhagic fever. Lancet 377, 849–862. doi: 10.1016/s0140-6736(10)60667-8
Galione, A. (2015). A primer of NAADP-mediated Ca(2+) signalling: from sea urchin eggs to mammalian cells. Cell Calcium 58, 27–47. doi: 10.1016/j.ceca.2014.09.010
Galione, A. (2019). NAADP receptors. Cold Spring Harb. Perspect. Biol. 11:a004036. doi: 10.1101/cshperspect.a004036
Genazzani, A. A., Mezna, M., Dickey, D. M., Michelangeli, F., Walseth, T. F., and Galione, A. (1997). Pharmacological properties of the Ca2+-release mechanism sensitive to NAADP in the sea urchin egg. Br. J. Pharmacol. 121, 1489–1495. doi: 10.1038/sj.bjp.0701295
Gerndt, S., Chen, C. C., Chao, Y. K., Yuan, Y., Burgstaller, S., Scotto Rosato, A., et al. (2020). Agonist-mediated switching of ion selectivity in TPC2 differentially promotes lysosomal function. eLife 9:e54712.
Godfraind, T. (2017). Discovery and development of calcium channel blockers. Front. Pharmacol. 8:286. doi: 10.3389/fphar.2017.00286
Grimm, C., Butz, E., Chen, C. C., Wahl-Schott, C., and Biel, M. (2017a). From mucolipidosis type IV to Ebola: TRPML and two-pore channels at the crossroads of endo-lysosomal trafficking and disease. Cell Calcium 67, 148–155. doi: 10.1016/j.ceca.2017.04.003
Grimm, C., Chen, C. C., Wahl-Schott, C., and Biel, M. (2017b). Two-pore channels: catalyzers of endolysosomal transport and function. Front. Pharmacol. 8:45. doi: 10.3389/fphar.2017.00045
Grimm, C., Holdt, L. M., Chen, C. C., Hassan, S., Muller, C., Jors, S., et al. (2014). High susceptibility to fatty liver disease in two-pore channel 2-deficient mice. Nat. Commun. 5:4699.
Gunaratne, G. S., Johns, M. E., Hintz, H. M., Walseth, T. F., and Marchant, J. S. (2018a). A screening campaign in sea urchin egg homogenate as a platform for discovering modulators of NAADP-dependent Ca(2+) signaling in human cells. Cell Calcium 75, 42–52. doi: 10.1016/j.ceca.2018.08.002
Gunaratne, G. S., Yang, Y., Li, F., Walseth, T. F., and Marchant, J. S. (2018b). NAADP-dependent Ca(2+) signaling regulates Middle East respiratory syndrome-coronavirus pseudovirus translocation through the endolysosomal system. Cell Calcium 75, 30–41. doi: 10.1016/j.ceca.2018.08.003
Hoffmann, M., Kleine-Weber, H., Schroeder, S., Kruger, N., Herrler, T., Erichsen, S., et al. (2020). SARS-CoV-2 cell entry depends on ACE2 and TMPRSS2 and is blocked by a clinically proven protease inhibitor. Cell 181, 271.e8–280.e8.
Huang, C., Wang, Y., Li, X., Ren, L., Zhao, J., Hu, Y., et al. (2020). Clinical features of patients infected with 2019 novel coronavirus in Wuhan, China. Lancet 395, 497–506.
Inciardi, R. M., Lupi, L., Zaccone, G., Italia, L., Raffo, M., Tomasoni, D., et al. (2020). Cardiac involvement in a patient with coronavirus disease 2019 (COVID-19). JAMA Cardiol. 5, 819–824.
Jha, A., Ahuja, M., Patel, S., Brailoiu, E., and Muallem, S. (2014). Convergent regulation of the lysosomal two-pore channel-2 by Mg(2)(+), NAADP, PI(3,5)P(2) and multiple protein kinases. EMBO J. 33, 501–511. doi: 10.1002/embj.201387035
Jiang, Y., Zhou, Y., Peng, G., Tian, H., Pan, D., Liu, L., et al. (2018). Two-pore channels mediated receptor-operated Ca(2+) entry in pulmonary artery smooth muscle cells in response to hypoxia. Int. J. Biochem. Cell Biol. 97, 28–35. doi: 10.1016/j.biocel.2018.01.012
Jiang, Y. L., Lin, A. H., Xia, Y., Lee, S., Paudel, O., Sun, H., et al. (2013). Nicotinic acid adenine dinucleotide phosphate (NAADP) activates global and heterogeneous local Ca2+ signals from NAADP- and ryanodine receptor-gated Ca2+ stores in pulmonary arterial myocytes. J. Biol. Chem. 288, 10381–10394. doi: 10.1074/jbc.m112.423053
Kilpatrick, B. S., Eden, E. R., Hockey, L. N., Yates, E., Futter, C. E., and Patel, S. (2017). An endosomal NAADP-sensitive two-pore Ca(2+) channel regulates ER-endosome membrane contact sites to control growth factor signaling. Cell Rep. 18, 1636–1645. doi: 10.1016/j.celrep.2017.01.052
Kinnear, N. P., Boittin, F. X., Thomas, J. M., Galione, A., and Evans, A. M. (2004). Lysosome-sarcoplasmic reticulum junctions. A trigger zone for calcium signaling by nicotinic acid adenine dinucleotide phosphate and endothelin-1. J. Biol. Chem. 279, 54319–54326. doi: 10.1074/jbc.m406132200
Kinnear, N. P., Wyatt, C. N., Clark, J. H., Calcraft, P. J., Fleischer, S., Jeyakumar, L. H., et al. (2008). Lysosomes co-localize with ryanodine receptor subtype 3 to form a trigger zone for calcium signalling by NAADP in rat pulmonary arterial smooth muscle. Cell Calcium 44, 190–201. doi: 10.1016/j.ceca.2007.11.003
Krogsaeter, E. K., Biel, M., Wahl-Schott, C., and Grimm, C. (2019). The protein interaction networks of mucolipins and two-pore channels. Biochim. Biophys. Acta Mol. Cell. Res. 1866, 1111–1123. doi: 10.1016/j.bbamcr.2018.10.020
Lee, S., Paudel, O., Jiang, Y., Yang, X. R., and Sham, J. S. (2015). CD38 mediates angiotensin II-induced intracellular Ca(2+) release in rat pulmonary arterial smooth muscle cells. Am. J. Respir. Cell Mol. Biol. 52, 332–341. doi: 10.1165/rcmb.2014-0141oc
Lewis, A. M., Aley, P. K., Roomi, A., Thomas, J. M., Masgrau, R., Garnham, C., et al. (2012). beta-Adrenergic receptor signaling increases NAADP and cADPR levels in the heart. Biochem. Biophys. Res. Commun. 427, 326–329. doi: 10.1016/j.bbrc.2012.09.054
Lin-Moshier, Y., Keebler, M. V., Hooper, R., Boulware, M. J., Liu, X., Churamani, D., et al. (2014). The Two-pore channel (TPC) interactome unmasks isoform-specific roles for TPCs in endolysosomal morphology and cell pigmentation. Proc. Natl. Acad. Sci. U.S.A. 111, 13087–13092. doi: 10.1073/pnas.1407004111
Lloyd-Evans, E., and Waller-Evans, H. (2019). Lysosomal Ca(2+) homeostasis and signaling in health and disease. Cold Spring Harb Perspect Biol. 12:a035311. doi: 10.1101/cshperspect.a035311
Macgregor, A., Yamasaki, M., Rakovic, S., Sanders, L., Parkesh, R., Churchill, G. C., et al. (2007). NAADP controls cross-talk between distinct Ca2+ stores in the heart. J. Biol. Chem. 282, 15302–15311. doi: 10.1074/jbc.m611167200
Mazzanti, A., Briani, M., Kukavica, D., Bulian, F., Marelli, S., Trancuccio, A., et al. (2020). Association of Hydroxychloroquine with QTc Interval in Patients with COVID-19. Circulation 142, 513–515.
Melchionda, M., Pittman, J. K., Mayor, R., and Patel, S. (2016). Ca2+/H+ exchange by acidic organelles regulates cell migration in vivo. J. Cell Biol. 212, 803–813. doi: 10.1083/jcb.201510019
Memish, Z. A., Perlman, S., Van Kerkhove, M. D., and Zumla, A. (2020). Middle East respiratory syndrome. Lancet 395, 1063–1077.
Mendoza-Torres, E., Riquelme, J. A., Vielma, A., Sagredo, A. R., Gabrielli, L., Bravo-Sagua, R., et al. (2018). Protection of the myocardium against ischemia/reperfusion injury by angiotensin-(1-9) through an AT2R and Akt-dependent mechanism. Pharmacol. Res. 135, 112–121. doi: 10.1016/j.phrs.2018.07.022
Moccia, F., Gerbino, A., Lionetti, V., Miragoli, M., Munaron, L. M., Pagliaro, P., et al. (2020a). COVID-19-associated cardiovascular morbidity in older adults: a position paper from the Italian society of cardiovascular researches. Geroscience 42, 1021–1049. doi: 10.1007/s11357-020-00198-w
Moccia, F., Zuccolo, E., Di Nezza, F., Pellavio, G., Faris, P. S., Negri, S., et al. (2020b). Nicotinic acid adenine dinucleotide phosphate activates two-pore channel TPC1 to mediate lysosomal Ca(2+) release in endothelial colony-forming cells. J. Cell. Physiol. 236, 688–705. doi: 10.1002/jcp.29896
Moccia, F., Negri, S., Shekha, M., Faris, P., and Guerra, G. (2019). Endothelial Ca(2+) signaling, angiogenesis and vasculogenesis: just what it takes to make a blood vessel. Int. J. Mol. Sci. 20:3962. doi: 10.3390/ijms20163962
Morgan, A. J., Platt, F. M., Lloyd-Evans, E., and Galione, A. (2011). Molecular mechanisms of endolysosomal Ca2+ signalling in health and disease. Biochem. J. 439, 349–374. doi: 10.1042/bj20110949
Negri, S., Faris, P., Pellavio, G., Botta, L., Orgiu, M., Forcaia, G., et al. (2019). Group 1 metabotropic glutamate receptors trigger glutamate-induced intracellular Ca(2+) signals and nitric oxide release in human brain microvascular endothelial cells. Cell Mol. Life Sci. 77, 2235–2253. doi: 10.1007/s00018-019-03284-1
Nguyen, O. N., Grimm, C., Schneider, L. S., Chao, Y. K., Atzberger, C., Bartel, K., et al. (2017). Two-Pore Channel Function Is Crucial for the Migration of Invasive Cancer Cells. Cancer Res. 77, 1427–1438. doi: 10.1158/0008-5472.can-16-0852
Nicin, L., Abplanalp, W. T., Mellentin, H., Kattih, B., Tombor, L., John, D., et al. (2020). Cell type-specific expression of the putative SARS-CoV-2 receptor ACE2 in human hearts. Eur. Heart J. 41, 1804–1806. doi: 10.1093/eurheartj/ehaa311
Nishiga, M., Wang, D. W., Han, Y., Lewis, D. B., and Wu, J. C. (2020). COVID-19 and cardiovascular disease: from basic mechanisms to clinical perspectives. Nat. Rev. Cardiol. 17, 543–558. doi: 10.1038/s41569-020-0413-9
Oparil, S., Acelajado, M. C., Bakris, G. L., Berlowitz, D. R., Cifkova, R., Dominiczak, A. F., et al. (2018). Hypertension. Nat. Rev. Dis. Primers 4:18014.
Ou, X., Liu, Y., Lei, X., Li, P., Mi, D., Ren, L., et al. (2020). Characterization of spike glycoprotein of SARS-CoV-2 on virus entry and its immune cross-reactivity with SARS-CoV. Nat. Commun. 11:1620.
Paniz-Mondolfi, A., Bryce, C., Grimes, Z., Gordon, R. E., Reidy, J., Lednicky, J., et al. (2020). Central nervous system involvement by severe acute respiratory syndrome coronavirus-2 (SARS-CoV-2). J. Med. Virol. 92, 699–702.
Patel, S. (2015). Function and dysfunction of two-pore channels. Sci. Signal. 8:re7. doi: 10.1126/scisignal.aab3314
Patel, S., and Docampo, R. (2010). Acidic calcium stores open for business: expanding the potential for intracellular Ca2+ signaling. Trends Cell Biol. 20, 277–286. doi: 10.1016/j.tcb.2010.02.003
Patel, V. B., Zhong, J. C., Fan, D., Basu, R., Morton, J. S., Parajuli, N., et al. (2014). Angiotensin-converting enzyme 2 is a critical determinant of angiotensin II-induced loss of vascular smooth muscle cells and adverse vascular remodeling. Hypertension 64, 157–164. doi: 10.1161/hypertensionaha.114.03388
Paz Ocaranza, M., Riquelme, J. A., Garcia, L., Jalil, J. E., Chiong, M., Santos, R. A. S., et al. (2020). Counter-regulatory renin-angiotensin system in cardiovascular disease. Nat. Rev. Cardiol. 17, 116–129.
Penny, C. J., Kilpatrick, B. S., Eden, E. R., and Patel, S. (2015). Coupling acidic organelles with the ER through Ca(2)(+) microdomains at membrane contact sites. Cell Calcium 58, 387–396. doi: 10.1016/j.ceca.2015.03.006
Penny, C. J., Vassileva, K., Jha, A., Yuan, Y., Chee, X., Yates, E., et al. (2019). Mining of Ebola virus entry inhibitors identifies approved drugs as two-pore channel pore blockers. Biochim. Biophys. Acta Mol. Cell Res. 1866, 1151–1161. doi: 10.1016/j.bbamcr.2018.10.022
Pitt, S. J., Reilly-O’donnell, B., and Sitsapesan, R. (2016). Exploring the biophysical evidence that mammalian two-pore channels are NAADP-activated calcium-permeable channels. J. Physiol. 594, 4171–4179. doi: 10.1113/jp270936
Rahman, T., Cai, X., Brailoiu, G. C., Abood, M. E., Brailoiu, E., and Patel, S. (2014). Two-pore channels provide insight into the evolution of voltage-gated Ca2+ and Na+ channels. Sci. Signal. 7:ra109. doi: 10.1126/scisignal.2005450
Ruas, M., Chuang, K. T., Davis, L. C., Al-Douri, A., Tynan, P. W., Tunn, R., et al. (2014). TPC1 has two variant isoforms, and their removal has different effects on endo-lysosomal functions compared to loss of TPC2. Mol. Cell. Biol. 34, 3981–3992. doi: 10.1128/mcb.00113-14
Sakurai, Y., Kolokoltsov, A. A., Chen, C. C., Tidwell, M. W., Bauta, W. E., Klugbauer, N., et al. (2015). Ebola virus. Two-pore channels control Ebola virus host cell entry and are drug targets for disease treatment. Science 347, 995–998. doi: 10.1126/science.1258758
Salata, C., Calistri, A., Alvisi, G., Celestino, M., Parolin, C., and Palu, G. (2019). Ebola virus entry: from molecular characterization to drug discovery. Viruses 11:274. doi: 10.3390/v11030274
Shirato, K., Kawase, M., and Matsuyama, S. (2013). Middle east respiratory syndrome coronavirus infection mediated by the transmembrane serine protease TMPRSS2. J. Virol. 87, 12552–12561. doi: 10.1128/jvi.01890-13
Trufanov, S. K., Rybakova, E. Y., Avdonin, P. P., Tsitrina, A. A., Zharkikh, I. L., Goncharov, N. V., et al. (2019). The role of two-pore channels in norepinephrine-induced [Ca(2+)]i rise in rat aortic smooth muscle cells and aorta contraction. Cells 8:1144. doi: 10.3390/cells8101144
Varga, Z., Flammer, A. J., Steiger, P., Haberecker, M., Andermatt, R., Zinkernagel, A. S., et al. (2020). Endothelial cell infection and endotheliitis in COVID-19. Lancet 395, 1417–1418. doi: 10.1016/s0140-6736(20)30937-5
Vassileva, K., Marsh, M., and Patel, S. (2020). Two-pore channels as master regulators of membrane trafficking and endocytic well-being. Curr. Opin. Physiol. 17, 163–168. doi: 10.1016/j.cophys.2020.08.002
V’kovski, P., Kratzel, A., Steiner, S., Stalder, H., and Thiel, V. (2020). Coronavirus biology and replication: implications for SARS-CoV-2. Nat. Rev. Microbiol. [Epub ahead of print].
Walls, A. C., Park, Y. J., Tortorici, M. A., Wall, A., Mcguire, A. T., and Veesler, D. (2020). Structure, function, and antigenicity of the SARS-CoV-2 spike glycoprotein. Cell 181:281.e6–292.e6.
Wang, D., Hu, B., Hu, C., Zhu, F., Liu, X., Zhang, J., et al. (2020). Clinical characteristics of 138 hospitalized patients with 2019 novel coronavirus-infected pneumonia in Wuhan, China. JAMA 323, 1061–1069. doi: 10.1001/jama.2020.1585
White, J. M., and Schornberg, K. L. (2012). A new player in the puzzle of filovirus entry. Nat. Rev. Microbiol. 10, 317–322. doi: 10.1038/nrmicro2764
Xu, H., and Ren, D. (2015). Lysosomal physiology. Annu. Rev. Physiol. 77, 57–80. doi: 10.1146/annurev-physiol-021014-071649
Yang, N., and Shen, H. M. (2020). Targeting the endocytic pathway and autophagy process as a novel therapeutic strategy in COVID-19. Int. J. Biol. Sci. 16, 1724–1731. doi: 10.7150/ijbs.45498
Yao, W. X., and Jiang, M. X. (2002). Effects of tetrandrine on cardiovascular electrophysiologic properties. Acta Pharmacol. Sin. 23, 1069–1074.
Yin, X. X., Zheng, X. R., Peng, W., Wu, M. L., and Mao, X. Y. (2020). Vascular endothelial growth factor (VEGF) as a vital target for brain inflammation during the COVID-19 outbreak. ACS Chem. Neurosci. 11, 1704–1705. doi: 10.1021/acschemneuro.0c00294
Zheng, Y. Y., Ma, Y. T., Zhang, J. Y., and Xie, X. (2020). COVID-19 and the cardiovascular system. Nat. Rev. Cardiol. 17, 259–260.
Zhou, P., Yang, X. L., Wang, X. G., Hu, B., Zhang, L., Zhang, W., et al. (2020). A pneumonia outbreak associated with a new coronavirus of probable bat origin. Nature 579, 270–273. doi: 10.1038/s41586-020-2012-7
Zuccolo, E., Dragoni, S., Poletto, V., Catarsi, P., Guido, D., Rappa, A., et al. (2016). Arachidonic acid-evoked Ca2+ signals promote nitric oxide release and proliferation in human endothelial colony forming cells. Vascul. Pharmacol. 87, 159–171. doi: 10.1016/j.vph.2016.09.005
Zuccolo, E., Kheder, D. A., Lim, D., Perna, A., Nezza, F. D., Botta, L., et al. (2019a). Glutamate triggers intracellular Ca(2+) oscillations and nitric oxide release by inducing NAADP- and InsP3 -dependent Ca(2+) release in mouse brain endothelial cells. J. Cell. Physiol. 234, 3538–3554. doi: 10.1002/jcp.26953
Keywords: SARS-CoV-2, COVID-19, cardiovascular system, two-pore channels, PI(3, 5)P2, endolysosomal Ca2+ signaling, NAADP
Citation: Moccia F, Negri S, Faris P, Perna A, De Luca A, Soda T, Berra-Romani R and Guerra G (2021) Targeting Endolysosomal Two-Pore Channels to Treat Cardiovascular Disorders in the Novel COronaVIrus Disease 2019. Front. Physiol. 12:629119. doi: 10.3389/fphys.2021.629119
Received: 13 November 2020; Accepted: 07 January 2021;
Published: 26 January 2021.
Edited by:
Antonio Crisafulli, University of Cagliari, ItalyReviewed by:
Christian Grimm, Ludwig Maximilian University of Munich, GermanyJohn Parrington, University of Oxford, United Kingdom
Copyright © 2021 Moccia, Negri, Faris, Perna, De Luca, Soda, Berra-Romani and Guerra. This is an open-access article distributed under the terms of the Creative Commons Attribution License (CC BY). The use, distribution or reproduction in other forums is permitted, provided the original author(s) and the copyright owner(s) are credited and that the original publication in this journal is cited, in accordance with accepted academic practice. No use, distribution or reproduction is permitted which does not comply with these terms.
*Correspondence: Francesco Moccia, francesco.moccia@unipv.it; Roberto-Berra Romani, rberra001@hotmail.com; Germano Guerra, germano.guerra@unimol.it
†These authors have contributed equally to this work