- 1School of Traditional Chinese Medicine, Beijing University of Chinese Medicine, Beijing, China
- 2Key Laboratory of Chinese Internal Medicine of Ministry of Education and Beijing, Dongzhimen Hospital, Affiliated Hospital of Beijing University of Chinese Medicine, Beijing, China
Background: Inflammation-related NLRP3/Caspase-1/GSDMD-mediated pyroptosis is involved in the progression of ulcerative colitis (UC). β-sitosterol (SIT) was reported to have anti-inflammatory effects on experimental colitis, while the regulation of SIT on pyroptosis is unclear. Therefore, the present study aimed to define the protective and healing effects of SIT on dextran sulfate sodium (DSS)-induced experimental UC rats and human epithelial colorectal adenocarcinoma cells (Caco-2) and explore the underlying mechanisms that are responsible for its effects on NLRP3/Caspase-1/GSDMD-mediated pyroptosis in UC.
Methods: UC model rats were established by oral 4% DSS. Following colitis injury, the animals received SIT (doses of 50, 100, and 200 mg/kg) treatment for 2 weeks. For in vitro study, we exposed Caco-2–50 mg/mL DSS with or without SIT (concentrations of 8 and 16 μg/mL). Disease activity index (DAI) and histopathological injury were assessed in vivo. Activation proteins of nuclear factor kappa B (NF-κB) signaling axis, and tight junction-related proteins of zonula occludens-1 (ZO-1) and occludin were detected in colon tissues. TNF-α, IL-1β, and IL-18 in serum and cell supernatant were measured by enzyme-linked immunosorbent assay (ELISA). Changes in NLRP3/Caspase-1/GSDMD-mediated pyroptosis signaling pathway activation were analyzed both in tissues and cells.
Results: Our findings suggested that SIT treatment attenuated the severity of 4% DSS-induced UC by protecting rats from weight and colon length loss, and macroscopic damage. SIT also reduced proinflammatory factors production (TNF-α, IL-1β, and IL-18) in serum and cell supernatant. Mechanistically, SIT downregulated the expression levels of pyroptosis-related proteins including Caspase-1, cleaved-Caspase-1, NLRP3, GSDMD, and GSDMD-N in colon tissues and Caco-2 cells. Further analysis indicated that SIT maintained the colonic barrier integrity by enhancing the protein expression of ZO-1 and occludin.
Conclusion: We confirmed that SIT exerts protective and therapeutic effects on DSS-induced colitis injury by suppressing NLRP3/Caspase-1/GSDMD-mediated pyroptosis and inflammation response. These findings demonstrated that SIT could be a potential medication for UC treatment.
1 Introduction
Ulcerative colitis (UC), a global public health problem, which is a chronic non-specific, non-infectious, and inflammatory intestinal disease. It is a continuous mucosal ulcer with unknown origin and usually begins in the rectum, involves the colonic mucosa and submucosa layer, and then spreads to the cecum for the longest, which is characterized by continuous and diffuse distribution. Clinical manifestations of this illness are chronic and recurrent with abdominal pain, diarrhea, fecal bleeding, weight loss, and intestinal mucosal (Ordás et al., 2012). In recent years, UC has had increasing prevalence and incidence in developing and developed countries worldwide due to the rapid economic development and westernized diet uptake (Ng et al., 2017). Additionally, the incidence among people with UC in China has nearly tripled in the past decades (Ye et al., 2013). Research shows that patients with long-term UC have a higher risk of developing colorectal cancer and colitis-associated cancer is a major cause of death in patients with UC (Rogler, 2014), thereby resulting in a high economic burden on individuals and society health systems. For the above reasons, effective intervention in the course of UC is urgent.
Though the etiology of UC remains undefined, the inflammatory response definitely exists in the pathogenesis of UC (Du and Ha, 2020). Studies have suggested the large aggregation of inflammatory molecules such as TNF-α, IL-1β, and IL-18 could lead to colonic tissue injury and epithelial integrity disruption in the gut while inhibiting cytokine-mediated inflammation could treat or cure UC (Elmaksoud et al., 2021). NF-κB is a key transcriptional regulator in the regulation of proinflammatory mediators and chemokines production and secretion, which leads to an inflammatory cascade (Cui et al., 2019). The abnormal activation of NF-κB signaling was founded in colonic tissues of UC patients and experimental models (Neurath et al., 1996). Pyroptosis is a newly found form of pro-inflammatory programmed cell death unlike traditional necrosis and apoptosis (Shi et al., 2015). Pyroptosis plays an essential role in host defense and inflammatory responses, in which Caspase-1 or Caspase-11/4/5 was activated during this process (Yu P. et al., 2021). Activation of inflammatory caspases can trigger pyroptosis and release pro-inflammatory cytokines IL-1β and IL-18, which are relevant to inflammatory diseases (Yuan et al., 2018). In addition, over-expression of pyroptosis-related proteins, including nucleotide-binding oligomerization segment-like receptor family 3 (NLRP3), apoptosis-associated speck-like protein (ASC), Caspase-1, and gasdermin D-N-terminal (GSDMD-N) in colonic tissues has been observed in experimental colitis (Chao et al., 2020; Wei et al., 2021) and suppression of the NLRP3/Caspase-1/GSDMD-mediated pyroptosis signaling pathway can attenuate the damage of UC (Jie et al., 2021), thus therapy targeting pyroptosis could be promising and merits further investigation.
While immunosuppressants, glucocorticoids, salicylic acid, and biological agents are the frontline therapy for clinical patients with UC (Nakamura et al., 2008; Berends et al., 2019), there are many side effects associated with these pharmaceutical medications (Moreau and Mas, 2015). Therefore, more researchers have paid attention to natural plant substances, which will be safe and beneficial with less deleterious effects (Cao et al., 2019). Phytosterols are a critical class of bioorganic compounds that are abundant in a range of organisms such as plants, animals, and fungi, and exert an important role in the physiological processes of eukaryotes (Zhang et al., 2022). β-sitosterol (SIT) (Figure 1), the major compound of phytosterol, is a plant-derived natural product widely present in many countries. Its pharmacological effects and biological activities were well documented in the literature including anti-inflammation (Loizou et al., 2010; Sun et al., 2020), anti-anxiety (Panayotis et al., 2021), antiviral properties (Chen et al., 2022), anti-oxidant stress, immunomodulation (Cheng et al., 2020), anti-diabetes (Babu et al., 2020), anti-tumor (Bae et al., 2021), anti-microbes (Pierre Luhata and Usuki, 2021), hepatoprotective effects (Kim et al., 2014), cardioprotective effects (Lin et al., 2020), anti-diabetes (Ponnulakshmi et al., 2019), as well as regulation of gut microbiota (Yu Y. et al., 2021). These studies showed that SIT could be a potential measure of various diseases. Furthermore, evidence from experimental studies on SIT indicates its anti-inflammatory ability and can be used as a safe pharmaceutical complement in the treatment of experimental colitis (Lee et al., 2012; Aldini et al., 2014; Bin Sayeed et al., 2016; Feng et al., 2017; Ding et al., 2019). Of note, SIT has been proven experimentally that it does not produce cytotoxic impacts under long-term use (Malini and Vanithakumari, 1990; Paniagua-Pérez et al., 2005; Feng et al., 2020). Despite this, further experimental studies are required to uncover the role of SIT in the recovery of experimental UC and elucidate its possible anti-pyroptosis mechanism behind this.
Thus, in this study, we established the DSS-induced experimental colitis in rats and Caco-2 cells to assess the protective and therapeutic effects of SIT on UC and further explore its underlying mechanism.
2 Materials and methods
2.1 Drug, and reagents
Dextran sulfate sodium and β-sitosterol (SIT, purity >95% and HPLC ≥98%) were purchased from Shanghai Yuanye Bio-Technology Co., Ltd. (Shanghai, China). Carboxymethyl cellulose was purchased from Biotopped Technology Co. Ltd. (Beijing, China). Enzyme-linked immunosorbent assay (ELISA) kits of TNF-α, IL-1β, and IL-18 were obtained from Cloud-Clone Corp (Wuhan, China). Antibodies against NLRP3, occludin, β-Actin, goat-anti-rabbit IgG H&L (HRP), and goat-anti-mouse IgG H&L (HRP) were purchased from Abcam (Cambridge, United Kingdom). Antibodies against Caspase-1, GSDMD, IKB alpha, and ZO-1 were obtained from Proteintech (Wuhan, China). Antibodies against NF-kappaB p65, phospho-NF-kappaB p65, and phospho-IkB alpha were purchased from Invitrogen (Carlsbad, CA). Antibody against cleaved Caspase-1 was purchased from Cell Signalling Technology (Danvers, MA, United States). Antibody against GSDMD-N terminal was purchased from ABclonal (Wuhan, China). The Cell Counting Kit-8 (CCK-8) was purchased from Biorigin (Beijing, China).
The following reagents were obtained from GenePool Biotechnology (Beijing, China): Total RNA Extraction with DNase Ⅰ Kit, mRNA cDNA Synthesis Kit, mRNA qPCR Kit, RNA Loading Buffer, BSA Blocking Buffer, SDS-PAGE Gel Ki, SDS-PAGE Loading Buffer, Tris-Glycine Running Buffer, WB Transfer Buffer, and TBST. The following reagents were purchased from Beyotime Biotechnology (Shanghai, China): Cell lysis buffer for Western and IP, Protein Extraction Kit, BCA protein assay kit, enhanced chemiluminescence (ECL) kit, Citrate-EDTA Antigen Retrieval Solution, and Hematoxylin and Eosin Staining Kit. The following reagents were purchased from Gibco (Carlsbad, CA, United States): fetal bovine serum (FBS), minimum essential medium (MEM), Penicillin-Streptomycin antibiotics, non-essential amino acids (NEAA), and 0.25% trypsin solution with EDTA. All other chemicals were of reagent grade.
2.2 Animal experimental design and treatment
Sixty male Sprague-Dawley rats (6–8 weeks old, weighing 180–200 g) were purchased from Beijing Sibeifu Bioscience, Co., Ltd. (Beijing, China) (License NO. SCXK [Beijing] 2019-0010). All animals were raised under standard specific pathogen-free (SPF) conditions (temperature, 25°C ± 1°C; humidity, 60% ± 5%) with a 12 h light/dark cycle per day and allowed ad libitum eat and drink. Ethical approvals for the animal experiments were obtained from the animal ethics committee of Beijing University of Chinese Medicine (NO. BUCM-4-2022102901-4029).
This experimental design consists of two steps: a DSS-induced colitis stage and a drug treatment stage as described in Figure 2A. 60 rats were randomly assigned to five groups: 1) Control group (CON): received only double-drilled water; 2) DSS group (DSS): received 4% DSS (molecular weight: 40 000 Da; purity >98%) dissolved in double-drilled water in their daily drinking water; 3) Low-dose group (LD): received DSS and then gavaged with 50 mg/kg of SIT (purity >95%) suspended in 0.1% carboxymethyl cellulose; 4) Middle-dose group (MD): received DSS and then gavaged with 100 mg/kg SIT; 5) High-dose group (HD): received DSS and then gavaged with 200 mg/kg SIT. The intervention period of DSS was from day 1 to day 7 and the period of drug treatment was from day 8 to day 21.
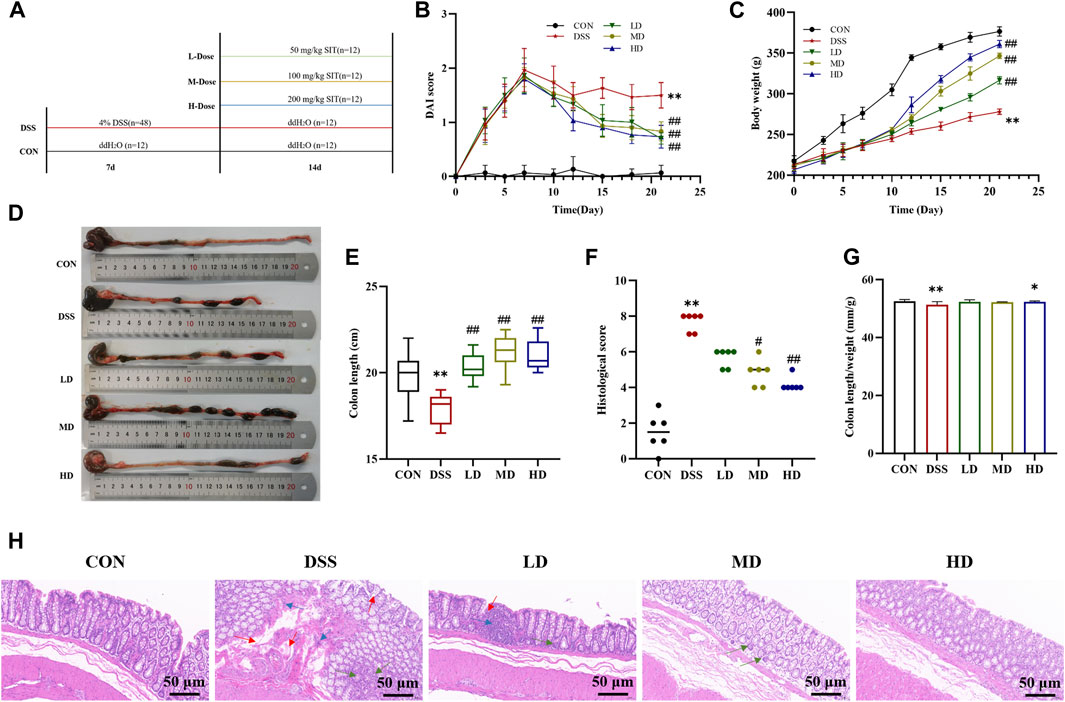
FIGURE 2. SIT protects rats from DSS-induced UC. (A) An experimental design in vivo. (B) Changes of body weight (N = 10 per group). (C) The disease activity index (DAI) scores (N = 10 per group). (D) Representative picture of the colon. (E) The colon lengths (N = 11 per group). (F) The colon length/weight ratio (N = 11 per group). (G) The colonic histopathological scores (N = 6 per group). (H) Representative histopathologic image of colon. H&E staining, 100 ×; scale bar, 50 μm. Red arrow: distortion or loss of crypts; Blue arrow: infiltration of inflammatory cells; Green arrow: depletion of goblet cells. The data are presented as mean ± SD. CON, control group; DSS, dextran sulfate sodium-induced group; SIT, β-sitosterol; LD, low-dose SIT group; MD, middle-dose SIT group; HD, high-dose SIT group; H&E, hematoxylin and eosin; SD, standard deviation. **p < 0.01 compared with CON group; #p < 0.05, ##p < 0.01 compared with DSS group.
Following the 14-day intervention, rats were all deeply anesthetized after 24 h fasting with 1% sodium pentobarbital (40 mg/kg). After recording the colon length and weight, blood samples were collected and centrifuged for serum. Then the colon specimens were immediately removed and then fixed in 4% paraformaldehyde for histopathological studies or stored at −80°C for molecular biology detection.
2.3 Observation of UC symptoms and signs in rats
During this experimental period, body weight, stool status, and rectal bleeding were recorded every day to calculate disease activity index (DAI) scores on a previously established scoring system (Kihara et al., 2003) and listed in Table 1. DAI score is the average of the three categories. All evaluations were performed while unaware of the conditions.
2.4 Histological examination
Hematoxylin and eosin (H&E) detection was conducted with a standard protocol. The colon tissues were fixed in 4% paraformaldehyde and embedded in paraffin. Then, the paraffin-blocked samples were cut into 5-µm sections on slides for staining with hematoxylin and eosin. Slides were scanned using a Panoramic MIDI Scan Whole Slide Scanner (3DHISTECH Ltd., Budapest, Hungary) and viewed with Panoramic Viewer 1.15.4 (3DHISTECH); in addition, histopathological scores for histopathological changes were calculated based on a previously developed scoring system (Dieleman et al., 1998) and listed in Table 2. The individual scoring was blinded to the identity of the slides.
2.5 Cell preparation and viability assay
Human epithelial colorectal adenocarcinoma (Caco-2) cell lines (originally obtained from ATCC) were purchased from IMMOCELL (Xiamen, China). The Caco-2 cells were maintained in minimum essential medium (MEM) containing 10% fetal bovine serum (FBS), 100 U/ml of penicillin, 100 μg/mL of streptomycin, and 1% non-essential amino acids supplements. Cells were maintained at 37°C with 5% CO2 atmosphere.
Caco-2 cell viability was measured by CCK-8 assay followed by a standard protocol. After preparing single-cell suspensions, cells (5 × 104 cells/well) were added to 96-well plates and continued to culture till 80% confluency. Then, 10 μL/well of CCK-8 solution was added and incubated protected from light for 2 h at 37°C. The 450 nm absorbance values were detected with a multifunctional microplate reader (Thermo, Manassas, United States) to measure the cell growth inhibition rate.
2.6 Caco-2 inflammation model establishment and treatment
Cells were cultured overnight with or without SIT (HPLC ≥98%) dissolved in absolute ethanol (concentrations of 8 μg/mL as Low-dose group and 16 μg/mL as High-dose group). Supernatants of cells were discarded and washed with PBS, and 50 mg/mL DSS was added to establish the inflammation model.
2.7 Cytokine assays
The serum of rats and supernatant of cells were collected and then calculated for TNF-α, IL-1β, and IL-18 levels using ELISA kits according to the manufacturer’s scheme.
2.8 Quantitative polymerase chain reaction
Relative levels of NLRP3, Caspase-1, GSDMD, IL-1β, and IL-18 mRNAs in colonic tissues were analyzed by a quantitative real-time (qRT)-PCR. Total RNA of colonic tissues was isolated using the Total RNA Extraction Kit and cDNA was synthesized by using the mRNA cDNA Synthesis Kit in accordance with the manufacturer’s protocols. The specific primers for NLRP3, Caspase-1, GSDMD, IL-1β, and IL-18 were designed and synthesized by GenePool Biotechnology (Beijing, China) and listed in Table 3. The PCR reaction parameters were predetermined: 95°C for 5 min, followed by a 35 cycle-denaturation for 30 s at 95°C, 55°C for 30 s, and extension for 1 min at 72°C. Each sample underwent three biological replications for statistical analysis to determine significant differences. GAPDH was an internal control and relative gene expression levels were calculated using the 2−ΔΔCT method.
2.9 Western blot analysis
The total proteins of tissues and cells were extracted using Western and IP lysate buffer, BCA protein assay kit was applied to determine protein concentration. After homogenization, an equal quantity of proteins was separated with SDS-PAGE and transferred to PVDF membranes (Millipore corp., Massachusetts, United States), then blocked with 5% BSA. Primary antibodies of NLRP3 (dilution 1:1 000), Caspase-1 (dilution 1:2 000), cleaved-Caspase-1 (dilution 1:1 000), GSDMD (dilution 1:2 000), GSDMD-N (dilution 1:1 000), p65 (dilution 1:1 000), p-p65 (dilution 1:1 000), IκBα (dilution 1:1 000), p-IκBα (dilution 1:1 000), ZO-1 (dilution 1:5 000), occludin (dilution 1:1 000), and β-Actin (dilution 1:5 000) were incubated overnight at 4°C. After being washed with TBST three times, membranes were then incubated with secondary antibodies against Goat Anti-Rabbit IgG H&L (HRP) (dilution 1:5 000) or Goat Anti-Mouse IgG H&L (HRP) (dilution 1:5 000) for 1 h at 37°C, and bands were visualized with an ECL kit captured with Tanon 5200 Chemiluminescent Imaging System (Tanon, Shanghai, China). Finally, the relative grey values of the target proteins blots normalized to β-Actin were analyzed by the ImageJ software (National Institutes of Health, United States).
2.10 Statistical analysis
Statistical analysis was performed by SPSS 26.0 system. Quantitative results were expressed as arithmetic mean plus or minus the standard deviation (SD) and analyzed using one-way analysis of variance (ANOVA) followed by least significant difference (LSD)’s multiple-comparison test, while Kruskal-Wallis test was performed for difference analysis of non-parametric data. p-value < 0.05 was considered statistically significant.
3 Results
3.1 SIT alleviates DSS-induced UC
In order to investigate the therapeutic effects of SIT in vivo, UC model rats were induced by 4% DSS for 7 consecutive days. During the experiment period, rats in the control group remained in a normal state, and body weight was rising steadily, while those in the model group represented a poor mental state with disheveled fur, decreased feeding, persistent fecal bleeding, and obvious body weight loss. Compared to the control group DAI score in the DSS-induced model group was significantly increased, which was consistent with the clinical characteristics. Notably, administration of SIT recovered the body weight and colon length gradually (Figures 2C–E) as well as decreased DAI score (Figure 2B) in DSS-induced rats, especially at the dose of 200 mg/kg.
Furthermore, colonic shortening caused by DSS was evidently mitigated after SIT intake. As can be seen from Figure 2F, DSS in the UC group led to a significant decrease in colon length/weight ratio compared with the control group. High dose of SIT agonist partly offset the decrease of colon length/weight ratio. Under microscopy, results by H&E staining showed that there were pathological lesions and superficial inflammation in the DSS-induced colonic tissues characterized by inflammatory cell infiltration, crypt architectural distortion or absence and mucosa defects or damage. Consistent with the remission of clinical signs, the pathological changes were improved to varying degrees following the administration of SIT (Figures 2F,G). Collectively, these results indicated that SIT is protective against DSS-induced colitis and the effects might be dose-dependent.
3.2 Effects of SIT on the viability of Caco-2 cells
Before the formal in vitro experiments, toxicity evaluation of different concentrations of SIT (1, 2, 4, 8, 16, 32, and 64 μg/mL) on Caco-2 was carried out using the CCK-8 assay. After the 24 h-intervention, we observed that there was a significant decline in cell viability of Caco-2 following the higher concentrations of SIT (Figure 3A). Based on this, we chose the relatively safe concentrations for low-dose SIT (LD, 8 μg/mL) and high-dose SIT (HD, 16 μg/mL), respectively. In addition, at the concentration of 46.34 mg/mL DSS, growth of 50% cells was inhibited (Figure 3B). For convenience, we used 50 mg/mL DSS for subsequent experimental Caco-2 modeling, and found that SIT serves protectively against DSS-induced Caco-2 damage, (Figure 3C).
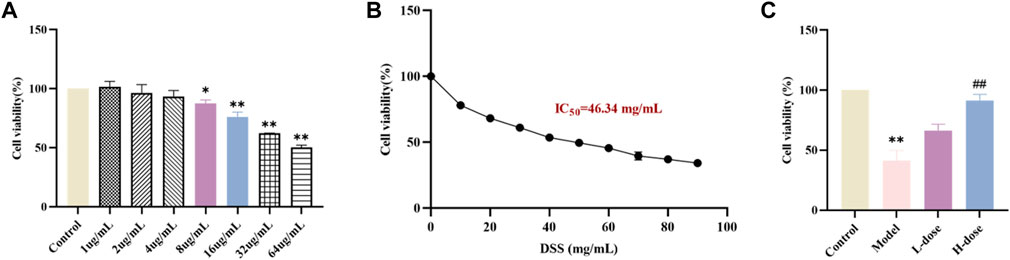
FIGURE 3. SIT protects Caco-2 cells from DSS-induced damage. (A) The viability of Caco-2 cells under the treatment with different concentrations SIT-containing medium for 24 h, and the viability was assayed by CCK-8. (B) Caco-2 cells were cultured with 10, 20, 30, 40, 50, 60, 70, 80, and 90 mg/mL DSS-containing medium for 24 h. (C) The cells were first cultured with SIT-containing medium for 24 h, incubated in 50 mg/mL DSS-containing medium for 6 h, and then replaced with fresh complete medium to culture for 18 h. N = 6 per group. The data are presented as mean ± SD. DSS, dextran sulfate sodium-induced group; SIT, β-sitosterol; SD, standard deviation. *p < 0.05, **p < 0.01 compared with Control group; ##p < 0.01 compared with Model group.
3.3 SIT inhibits proinflammatory mediators
To validate the suppressive effects of SIT on inflammation involved in UC, we detected the levels of inflammatory-related factors. The ELISA results showed that there was a significantly increase of TNF-α, IL-1β, and IL-18 levels after DSS treatment compared with the control group in rats and SIT effectively decreased the expression (Figure 4A). We further noticed the protective effects of SIT on Caco-2 from inflammation after being exposed to DSS (Figure 4B).
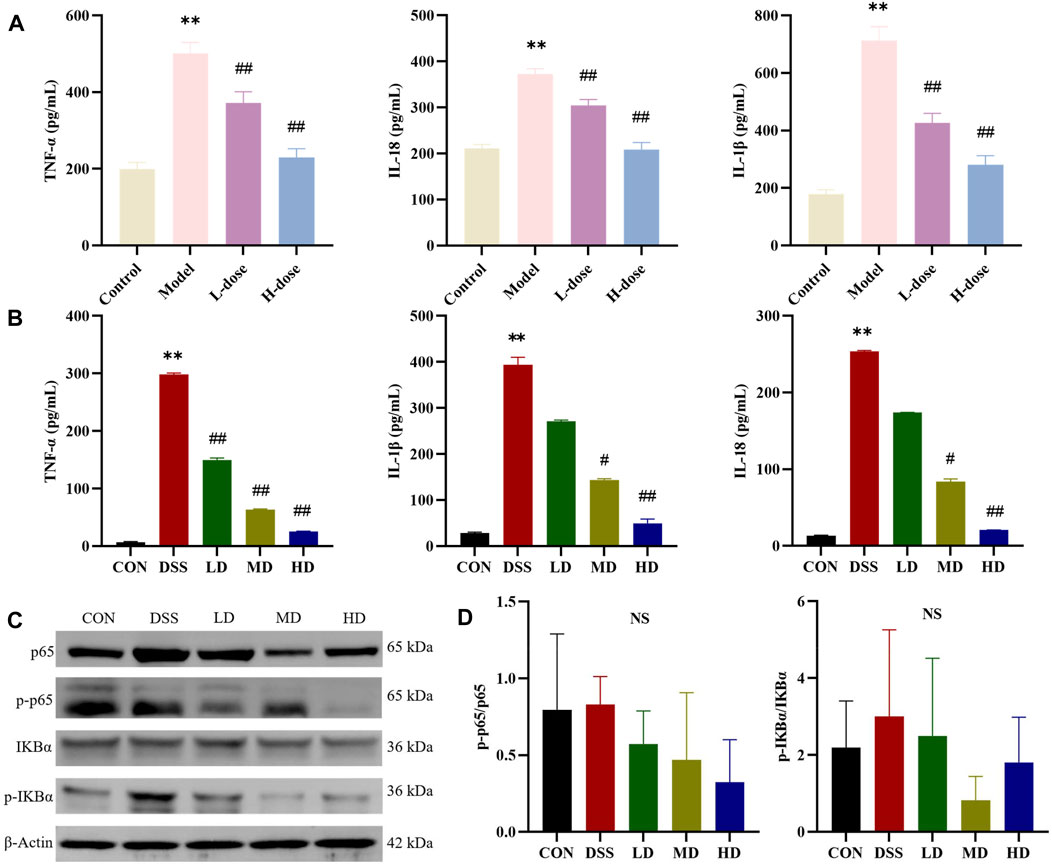
FIGURE 4. SIT regulates the pro-inflammatory cytokines and NF-κB pathway. The expression levels of TNF-α, IL-1β, and IL-18 in serum (A) and Caco-2 cells (B) were analyzed by ELISA (N = 6 per group). (C) Gel electrophoresis images of p-p65, p65, p-IκBα, and IκBα were analyzed by Western blot. (D) Analysis of protein expression levels (N = 3 per group). The data are presented as mean ± SD. NF-κB, nuclear transcription factor-kappa B; CON, control group; DSS, dextran sulfate sodium-induced group; SIT, β-sitosterol; LD, low-dose SIT group; MD, middle-dose SIT group; HD, high-dose SIT group; TNF, tumor necrosis factor; IL, interleukin; ELISA, enzyme-linked immunosorbent assay; SD, standard deviation. **p < 0.01 compared with CON or Control group; #p < 0.05, ##p < 0.01 compared with DSS or Model group; NS, not statistically significant.
3.4 SIT regulates the NF-κB inflammatory pathway
NF-κB signaling participates in the regulation of inflammatory response. We wondered whether the anti-inflammatory role of SIT was associated with the signaling. The expression levels of the major proteins p-p65, p65, p-IκBα, and IκBα in the NF-kB signaling pathways were consequently measured by Western blot. The experimental results showed that the levels of p-p65 and p-IκBα in DSS-induced colonic tissues were abnormally higher compared to the control group. In comparison with the DSS group, the protein expression ratio of p-p65/p65 and p-IκBα/IκBα were attenuated after stimulation with SIT (Figures 4C,D) while none were statistically significant. These results indicate that NF-κB signaling might be involved in the reduction of DSS-induced UC inflammation by SIT administration.
3.5 SIT suppresses critical indicators activity of pyroptosis
Pyroptosis is a type of programmed cell death (PCD) and participates in inflammatory disease processes involved in UC. For this reason, we further measured the expression of critical indicators of NLRP3/Caspase-1/GSDMD-mediated pyroptosis to explicit the anti-inflammatory effects of SIT. RT-qPCR analysis was carried out to assess the gene expression of Caspase-1, NLRP3, GSDMD, IL-1β, and IL-18 in colonic tissues. When compared to the control group, the mRNA expression levels of the above genes were upregulated in the DSS group while significantly downregulated with SIT administration (Figure 5A).
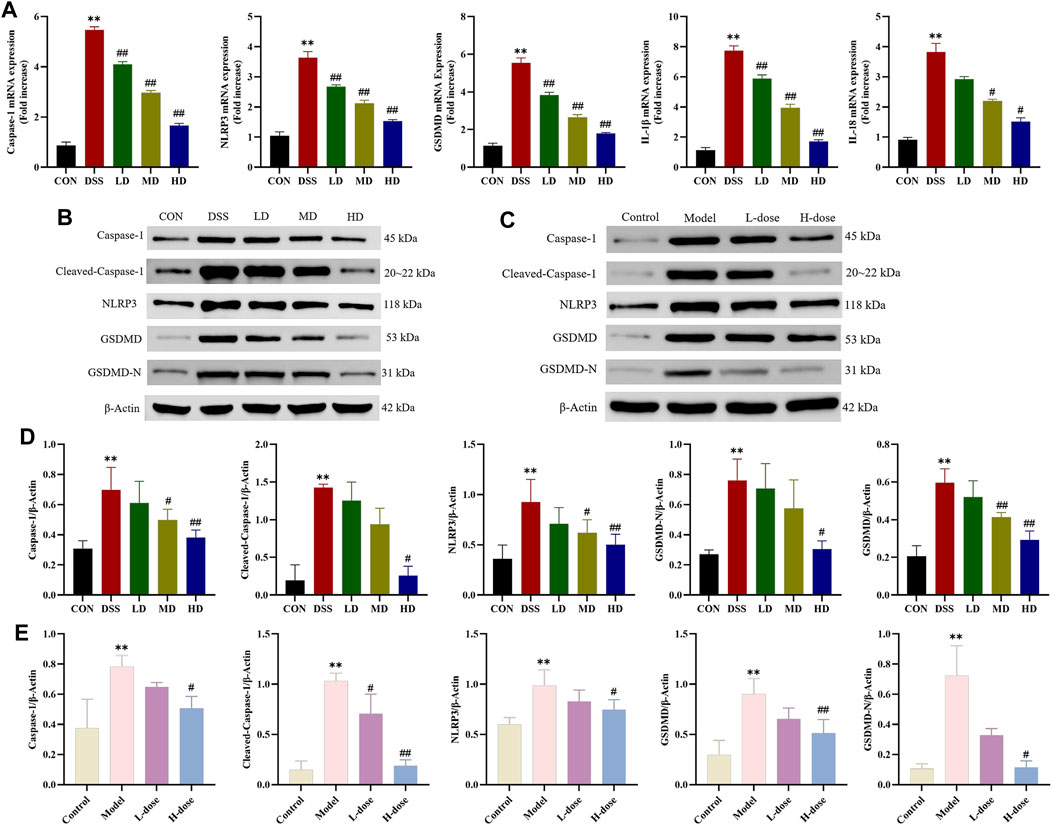
FIGURE 5. SIT inhibits DSS-induced Caspase-1 mediated pyroptosis pathway activation. (A) The mRNA levels of Caspase-1, NLRP3, GSDMD, IL-1β, and IL-18 in colon tissues. Gel electrophoresis images of Caspase-1, Cleaved-Caspase-1, NLRP3, GSDMD, and GSDMD-N in colon tissues (B) and Caco-2 cells (D) were analyzed by Western blot. Analysis of protein expression levels was analyzed and shown in (C) and (E). N = 3 per group. The data are presented as mean ± SD. CON, control group; DSS, dextran sulfate sodium-induced group; SIT, β-sitosterol; LD, low-dose SIT group; MD, middle-dose SIT group; HD, high-dose SIT group; NLRP3, nucleotide-binding oligomerization domain (Nod)-like receptor thermal protein domain associated protein 3; GSDMD, gasdermin D; GSDMD-N, gasdermin D-N terminal; IL, interleukin; SD, standard deviation. **p < 0.01 compared with CON or Control group; #p < 0.05, ##p < 0.01 compared with DSS or Model group.
As expected, Western blot analysis showed that protein expression levels of Caspase-1, Cleaved-Caspase-1, NLRP3, GSDMD, and GSDMD-N distinctly increased after DSS treatment in colonic tissues and Caco-2 cells and significantly counteracted following the intervention with SIT (Figures 5B–E). Meanwhile, ELISA indicated that SIT treatment significantly inhibited DSS-induced increased levels of IL-1β and IL-18 in serum and cell supernatant; it has been shown that secretion of cytokines IL-1β and IL-18 were associated with the NLRP3 inflammasome and induced an inflammatory cell death mode termed as pyroptosis (He et al., 2015). Comprehensively, the above results suggest that SIT plays a possible therapeutic role in UC via regulating Caspase-1-mediated pyroptosis.
3.6 SIT attenuates colonic mucosal barrier
Tight junction proteins are the essential parts for the maintenance of the gut mucosal barrier integrity, which could against the invasion of luminal detrimental substances and lower the risk of microbe-induced inflammation (Parikh et al., 2019). Therefore, we further verified whether the protective mechanism of SIT on the colon is related to the restoration of intestinal barrier function. ZO-1 and occludin, are important two tight junction-associated proteins (Ji et al., 2016), which were measured by Western blot. The expression levels of ZO-1 and occludin were decreased compared with the control group, which were significantly reversed by SIT as provided in Figure 6, suggesting that SIT could alleviate colitis through enhancing mucosal barrier integrity.
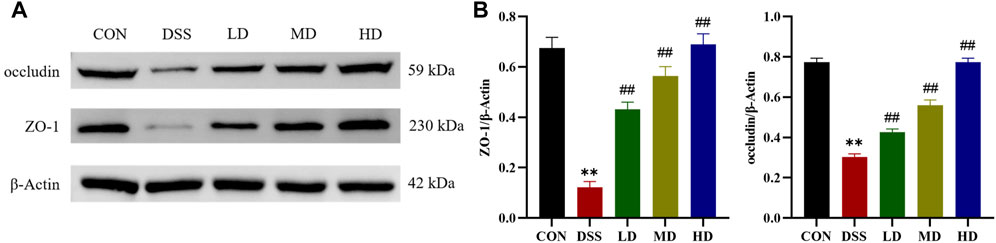
FIGURE 6. SIT alleviates colitis from barrier damage. (A) Gel electrophoresis images of occludin and ZO-1 in colon tissues were analyzed by Western blot. (B) Analysis of protein expression levels (N = 3 per group). The data are presented as mean ± SD. CON, control group; DSS, dextran sulfate sodium-induced group; SIT, β-sitosterol; LD, low-dose SIT group; MD, middle-dose SIT group; HD, high-dose SIT group; ZO, zonula occludens; SD, standard deviation. **p < 0.01 compared with CON group; ##p < 0.01 compared with DSS group.
4 Discussion
UC is a main subtype of chronic relapsing inflammatory bowel disease and is highly prevalent worldwide. The nature of UC is complex and the pathogenesis of UC has not been well elucidated yet. Current therapeutic agents are not entirely desirable in terms of potency with many side effects, erratic efficacy, and recurrence or failure after reduction or termination of administration as well as a heavy financial burden (Bressler et al., 2015; Berends et al., 2019). Hence new alternative therapeutic medication from natural products is of high interest in research. SIT is a well-known bioactive phytosterol naturally plentiful in dietary and non-dietary plant cell membranes, accounting for about 65% of human herbal nutrition forming (Weihrauch and Gardner, 1978). They are not only highly found in lipid-rich plant foods such as nuts, seeds, and legumes but also in vegetables and fruits (Khan et al., 2022). Over the past few decades, research on SIT was at an ever-accelerating pace and has suggested it may exert preventable and therapeutic effects on UC (Lee et al., 2012; Ding et al., 2019) with much less research on its role in pyroptosis.
DSS-induced experimental colitis is a reliable and mature animal model, which resembles clinically pathological symptoms and histological features in chronic UC (Tessner et al., 1998). In our current study, we demonstrated that SIT did significantly relieve the body weight loss in rats with 4% DSS-induced UC, with an increased DAI score, a main indicator in the assessment of the severity of UC. In addition, colonic shortening, another indirect indicator in the evaluation of the severity of UC, was attenuated by SIT treatment, indicating that SIT has therapeutic potential in UC rats. For colon pathological injury, we detected histological analysis and found that administration of SIT could improve cryptal glands and submucosa and reduce inflammatory cells infiltrating. Also, with the increase of SIT dosages, the therapeutic effects improve correspondingly, suggesting that SIT may be a potential drug of choice for UC treatment.
Self-limiting acute inflammation is crucial for the body to eliminate the danger and restore homeostasis, while unresolved inflammation contributes to the pathogenesis of autoimmune diseases including UC (Afonina et al., 2017). An increasing body of evidence has shown that aberrant inflammatory responses are the key contributors to the progression and exacerbation of UC. Pyroptosis, a novel type of programmed cell death, is mainly elicited by either classical Caspase-1-mediated or non-classical Caspase-11/4/5/11-mediated pathways in inflammation (Fang et al., 2020). Research has indicated that both Caspase-1-mediated and Caspase-11-mediated pyroptosis are closely linked to the development of UC (Chao et al., 2020), in which NLRP3 inflammasome plays a key role (Chen et al., 2019; Zhen and Zhang, 2019). NLRP3 first interacts with ASC and combines with Caspase-1 to assemble the inflammasome complex (Lu et al., 2014). Cleaved Caspase-1 then cleaves GSDMD to the N-terminal fragment (GSDMD-N). After that, the activated N-terminal domain of GSDMD translocates and forms cell membrane pores, thus resulting in the occurrence of pyroptosis and triggering the damage of the epithelial cells in the gut, and releasing pro-inflammatory cytokines (Yuan et al., 2018). The secretion of IL-1β and IL-18 can further amplify and perpetuate the inflammatory reaction (He et al., 2015; Shi et al., 2015). Of note, as the co-substrate of multiple inflammasomes and two main pathways of pyroptosis, GSDMD performs the primary function of pyroptosis executioner (Kayagaki et al., 2015; Shi et al., 2015).
Inflammatory cytokines IL-1β and IL-18 are the main markers for pyroptosis and their overproduction was found in various regions of the colon in UC active patients (Thinwa et al., 2014). Recent findings suggested that both Caspase-1 and Caspase-11 activation possess the function to induce the release of IL-1β and IL-18, but only Caspase-1 could directly cleave them (Man and Kanneganti, 2015); in addition, epithelium IL-18 secretion was independent on NLRP3 but dependent on Caspase-1 (Song-Zhao et al., 2014). Numerous experimental studies on animals have demonstrated inhibiting Caspase-1-dependent pyroptosis could protect against DSS-induced colitis (Tian et al., 2020; Cui et al., 2022; Xue et al., 2022). In view of this, we observed the regulation effect of SIT on inflammatory mediators in our present study. The results showed that SIT alleviated DSS-induced inflammation both in UC rats and Caco-2 cells with downregulation of pro-inflammatory cytokines including TNF-α, IL-1β, and IL-18. Furthermore, we explored the canonical Caspase-1 dependent pathway which underlies UC and we found that SIT treatment downregulated the protein levels of Caspase-1, cleaved-Caspase-1, NLRP3, GSDMD, and GSDMD- N in tissues and cells as expected. Taken together, SIT did ameliorate experimental colitis by inhibiting the NLRP3/Caspase-1/GSDMD-dependent pyroptosis pathway.
Furthermore, NF-κB pathway played an important role in the inflammatory responses of UC (Tong et al., 2021). In mammals, NF-κB family can form homo- or heterodimers of which the most common form is a dimer of p50 and p65. Normally, NF-κB remains inactive with the combination of members of IKB family (Lawrence, 2009). Clinical studies also have demonstrated excessive inflammation activated by NF-κB exists in UC (Zhou et al., 2018). Activation of NF-κB signaling cascade response was reported to participate in the regulation of NLRP3 inflammasome transcription and Caspase-1 has been proven as an active activator of NF-κB (Danelishvili et al., 2011; Hu et al., 2019; Zhen and Zhang, 2019). Previous studies have demonstrated that SIT could reduce the secretion of inflammatory mediators such as TNF-α and IL-1β; in addition, SIT has the ability to suppress the initiation of NLRP3 and the activation of Caspase-1 as well as partial inhibition of NF-κB in vitro (Liao et al., 2018). In this study, we also assessed the major proteins of NF-κB pathway. We observed the over-expressed phosphorylation levels of p65 subunit and IκBα, the protein content in colon tissues was suppressed under the SIT treatment. However, it cannot be stated with certainty that SIT-mediated inflammation alleviation is associated with NF-κB pathway in UC in light of our present results.
The mechanical barrier is the most important barrier among the intestinal mucosal barrier, with the structural basis consisting of intact intestinal epithelial cells and tight junction (TJ) proteins between the cells (Odenwald and Turner, 2017). Once the intestinal barrier was disrupted, the bacteria and toxins from the gut would translocate to the mucosa and activate deleterious intestinal inflammation (Liao et al., 2018; Zhang et al., 2018). Previous literature has demonstrated that the dysfunction of intestinal barrier integrity is responsible for the exacerbation of inflammatory bowel disease including UC (Zhao et al., 2020). Therefore, restoring the intestinal barrier integrity can act in preventing or treating UC. To gain further insight into the actions of SIT on the colonic barrier function, we detected the expression levels of the TJ-associated proteins ZO-1 and occludin. Western blot results suggested that the protein expression of ZO-1 and occludin were markedly weakened by DSS treatment while increasing in response to SIT administration. These results suggest that SIT could protect the gut against the occurrence of intestinal inflammation by maintaining mucosal barrier integrity, and therefore halt UC progression.
5 Conclusion
In summary, SIT not only inhibits the production of pro-inflammatory mediators and suppresses pyroptosis via suppression the NLRP3/Caspase-1/GSDMD-dependent pathway, but also enhances the function of the intestinal mucosal barrier through regulation of epithelial tight junction proteins expression (Figure 7). These findings indicate that SIT is an effective drug candidate and may be potential in clinical applications of UC treatment in the future.
As far as we know, it is the first evidence to show that SIT is effective in regulating pyroptosis in experimental UC. Although many studies have shown that SIT has good safety profiles, its poor stability, low water solubility, and short half-life also confine its broader application, and await to be addressed.
Data availability statement
The original contributions presented in the study are included in the article/Supplementary Material, further inquiries can be directed to the corresponding authors.
Ethics statement
Ethical approval was not required for the studies on humans in accordance with the local legislation and institutional requirements because only commercially available established cell lines were used. The animal study was approved by the animal ethics committee of Beijing University of Chinese Medicine. The study was conducted in accordance with the local legislation and institutional requirements.
Author contributions
The contribution of the authors in this work is as follows, D-MZ and MC conceived and designed the research, and provided financial support. DZ performed the experiments, carried out the data processing, and drafted the original manuscript. JJ and FG assisted in the investigation and conducting experiments. Y-JL and F-RZ assisted in the manuscript revision. S-JZ and S-YW provided the test medicine and technical support. D-MZ and MC supervised the research and reviewed the manuscript. All authors contributed to the article and approved the submitted version.
Funding
This research was supported by the Fundamental Research Funds for the Central Universities (Grant No. 2022-JYB-JBZR-017 and Beijing Natural Science Foundation (Grant No. 7232297).
Conflict of interest
The authors declare that the research was conducted in the absence of any commercial or financial relationships that could be construed as a potential conflict of interest.
Publisher’s note
All claims expressed in this article are solely those of the authors and do not necessarily represent those of their affiliated organizations, or those of the publisher, the editors and the reviewers. Any product that may be evaluated in this article, or claim that may be made by its manufacturer, is not guaranteed or endorsed by the publisher.
Supplementary material
The Supplementary Material for this article can be found online at: https://www.frontiersin.org/articles/10.3389/fphar.2023.1218477/full#supplementary-material
Abbreviations
ASC, apoptosis-associated speck-like protein; CON, control; DAI, disease activity index; DSS, dextran sulfate sodium; ELISA, enzyme-linked immunosorbent assay; GAPDH, glyceraldehyde-3-phosphate dehydrogenase; GSDMD, gasdermin D; GSDMD-N, gasdermin D-N-terminal; HD, high-dose; HRP, horse radish peroxidase; IHC, immunohistochemistry; IL, interleukin; IBD, inflammatory bowel disease; IL, interleukin; LD, low-dose; MD, middle-dose; NF-κB, nuclear transcription factor-kappa B; NLRP3, nucleotide-binding oligomerization domain (Nod)-like receptor thermal protein domain associated protein 3; PVDF, polyvinylidene fluoride; TNF, tumor necrosis factor; SD, standard deviation; SDS-PAGE, sodium dodecyl sulfate-polyacrylamide gel; UC, ulcerative colitis; ZO, zonula occludens.
References
Afonina, I. S., Zhong, Z., Karin, M., and Beyaert, R. (2017). Limiting inflammation-the negative regulation of NF-κB and the NLRP3 inflammasome. Nat. Immunol. 18, 861–869. doi:10.1038/ni.3772
Aldini, R., Micucci, M., Cevenini, M., Fato, R., Bergamini, C., Nanni, C., et al. (2014). Antiinflammatory effect of phytosterols in experimental murine colitis model: prevention, induction, remission study. PloS One 9, e108112. doi:10.1371/journal.pone.0108112
Babu, S., Krishnan, M., Rajagopal, P., Periyasamy, V., Veeraraghavan, V., Govindan, R., et al. (2020). Beta-sitosterol attenuates insulin resistance in adipose tissue via IRS-1/Akt mediated insulin signaling in high fat diet and sucrose induced type-2 diabetic rats. Eur. J. Pharmacol. 873, 173004. doi:10.1016/j.ejphar.2020.173004
Bae, H., Park, S., Ham, J., Song, J., Hong, T., Choi, J.-H., et al. (2021). ER-mitochondria calcium flux by β-sitosterol promotes cell death in ovarian cancer. Antioxidants (Basel, Switz. 10, 1583. doi:10.3390/antiox10101583
Berends, S. E., Strik, A. S., Löwenberg, M., D'Haens, G. R., and Mathôt, R. A. A. (2019). Clinical pharmacokinetic and pharmacodynamic considerations in the treatment of ulcerative colitis. Clin. Pharmacokinet. 58, 15–37. doi:10.1007/s40262-018-0676-z
Bin Sayeed, M. S., Karim, S. M. R., Sharmin, T., and Morshed, M. M. (2016). Critical analysis on characterization, systemic effect, and therapeutic potential of beta-sitosterol: a plant-derived orphan phytosterol. Med. (Basel, Switz. 3, 29. doi:10.3390/medicines3040029
Bressler, B., Marshall, J. K., Bernstein, C. N., Bitton, A., Jones, J., Leontiadis, G. I., et al. (2015). Clinical practice guidelines for the medical management of nonhospitalized ulcerative colitis: the Toronto consensus. Gastroenterology 148, 1035–1058.e3. doi:10.1053/j.gastro.2015.03.001
Cao, F., Liu, J., Sha, B.-X., and Pan, H.-F. (2019). Natural products: experimental efficient agents for inflammatory bowel disease therapy. Curr. Pharm. Des. 25, 4893–4913. doi:10.2174/1381612825666191216154224
Chao, L., Li, Z., Zhou, J., Chen, W., Li, Y., Lv, W., et al. (2020). Shen-Ling-Bai-Zhu-San improves dextran sodium sulfate-induced colitis by inhibiting caspase-1/caspase-11-mediated pyroptosis. Front. Pharmacol. 11, 814. doi:10.3389/fphar.2020.00814
Chen, C., Shen, J.-L., Liang, C.-S., Sun, Z.-C., and Jiang, H.-F. (2022). First discovery of beta-sitosterol as a novel antiviral agent against white spot syndrome virus. Int. J. Mol. Sci. 23, 10448. doi:10.3390/ijms231810448
Chen, X., Liu, G., Yuan, Y., Wu, G., Wang, S., and Yuan, L. (2019). NEK7 interacts with NLRP3 to modulate the pyroptosis in inflammatory bowel disease via NF-κB signaling. Cell Death Dis. 10, 906. doi:10.1038/s41419-019-2157-1
Cheng, Y., Chen, Y., Li, J., Qu, H., Zhao, Y., Wen, C., et al. (2020). Dietary β-sitosterol regulates serum lipid level and improves immune function, antioxidant status, and intestinal morphology in broilers. Poult. Sci. 99, 1400–1408. doi:10.1016/j.psj.2019.10.025
Cui, L.-J., Yuan, W., Chen, F.-Y., Wang, Y.-X., Li, Q.-M., Lin, C., et al. (2022). Pectic polysaccharides ameliorate the pathology of ulcerative colitis in mice by reducing pyroptosis. Ann. Transl. Med. 10, 347. doi:10.21037/atm-22-877
Cui, L., Wang, W., Luo, Y., Ning, Q., Xia, Z., Chen, J., et al. (2019). Polysaccharide from Scutellaria baicalensis Georgi ameliorates colitis via suppressing NF-κB signaling and NLRP3 inflammasome activation. Int. J. Biol. Macromol. 132, 393–405. doi:10.1016/j.ijbiomac.2019.03.230
Danelishvili, L., Everman, J. L., McNamara, M. J., and Bermudez, L. E. (2011). Inhibition of the plasma-membrane-associated serine protease cathepsin G by Mycobacterium tuberculosis Rv3364c suppresses caspase-1 and pyroptosis in macrophages. Front. Microbiol. 2, 281. doi:10.3389/fmicb.2011.00281
Dieleman, L. A., Palmen, M. J., Akol, H., Bloemena, E., Peña, A. S., Meuwissen, S. G., et al. (1998). Chronic experimental colitis induced by dextran sulphate sodium (DSS) is characterized by Th1 and Th2 cytokines. Clin. Exp. Immunol. 114, 385–391. doi:10.1046/j.1365-2249.1998.00728.x
Ding, K., Tan, Y.-Y., Ding, Y., Fang, Y., Yang, X., Fang, J., et al. (2019). β-Sitosterol improves experimental colitis in mice with a target against pathogenic bacteria. J. Cell. Biochem. 120, 5687–5694. doi:10.1002/jcb.27853
Du, L., and Ha, C. (2020). Epidemiology and pathogenesis of ulcerative colitis. Gastroenterology Clin. N. Am. 49, 643–654. doi:10.1016/j.gtc.2020.07.005
Elmaksoud, H. A. A., Motawea, M. H., Desoky, A. A., Elharrif, M. G., and Ibrahimi, A. (2021). Hydroxytyrosol alleviate intestinal inflammation, oxidative stress and apoptosis resulted in ulcerative colitis. Biomed. Pharmacother. = Biomedecine Pharmacother. 142, 112073. doi:10.1016/j.biopha.2021.112073
Fang, Y., Tian, S., Pan, Y., Li, W., Wang, Q., Tang, Y., et al. (2020). Pyroptosis: a new frontier in cancer. Biomed. Pharmacother. = Biomedecine Pharmacother. 121, 109595. doi:10.1016/j.biopha.2019.109595
Feng, S., Belwal, T., Li, L., Limwachiranon, J., Liu, X., and Luo, Z. (2020). Phytosterols and their derivatives: potential health-promoting uses against lipid metabolism and associated diseases, mechanism, and safety issues. Compr. Rev. Food Sci. Food Saf. 19, 1243–1267. doi:10.1111/1541-4337.12560
Feng, S., Dai, Z., Liu, A., Wang, H., Chen, J., Luo, Z., et al. (2017). β-Sitosterol and stigmasterol ameliorate dextran sulfate sodium-induced colitis in mice fed a high fat Western-style diet. Food and Funct. 8, 4179–4186. doi:10.1039/c7fo00375g
He, W.-t., Wan, H., Hu, L., Chen, P., Wang, X., Huang, Z., et al. (2015). Gasdermin D is an executor of pyroptosis and required for interleukin-1β secretion. Cell Res. 25, 1285–1298. doi:10.1038/cr.2015.139
Hu, S., Xie, H., Luo, R., Feng, P., Liu, Q., Han, M., et al. (2019). Inhibition of IL-1β by aliskiren improved renal AQP2 expression and urinary concentration defect in ureteral obstruction and release. Front. Physiology 10, 1157. doi:10.3389/fphys.2019.01157
Ji, R., Wang, A., Shang, H., Chen, L., Bao, C., Wu, L., et al. (2016). Herb-partitioned moxibustion upregulated the expression of colonic epithelial tight junction-related proteins in Crohn's disease model rats. Chin. Med. 11, 20. doi:10.1186/s13020-016-0090-0
Jie, F., Xiao, S., Qiao, Y., You, Y., Feng, Y., Long, Y., et al. (2021). Kuijieling decoction suppresses NLRP3-Mediated pyroptosis to alleviate inflammation and experimental colitis in vivo and in vitro. J. Ethnopharmacol. 264, 113243. doi:10.1016/j.jep.2020.113243
Kayagaki, N., Stowe, I. B., Lee, B. L., O'Rourke, K., Anderson, K., Warming, S., et al. (2015). Caspase-11 cleaves gasdermin D for non-canonical inflammasome signalling. Nature 526, 666–671. doi:10.1038/nature15541
Khan, Z., Nath, N., Rauf, A., Emran, T. B., Mitra, S., Islam, F., et al. (2022). Multifunctional roles and pharmacological potential of β-sitosterol: emerging evidence toward clinical applications. Chemico-biological Interact. 365, 110117. doi:10.1016/j.cbi.2022.110117
Kihara, N., de la Fuente, S. G., Fujino, K., Takahashi, T., Pappas, T. N., and Mantyh, C. R. (2003). Vanilloid receptor-1 containing primary sensory neurones mediate dextran sulphate sodium induced colitis in rats. Gut 52, 713–719. doi:10.1136/gut.52.5.713
Kim, K.-S., Yang, H. J., Lee, J.-Y., Na, Y.-C., Kwon, S.-Y., Kim, Y.-C., et al. (2014). Effects of β-sitosterol derived from Artemisia capillaris on the activated human hepatic stellate cells and dimethylnitrosamine-induced mouse liver fibrosis. BMC Complementary Altern. Med. 14, 363. doi:10.1186/1472-6882-14-363
Lawrence, T. (2009). The nuclear factor NF-kappaB pathway in inflammation. Cold Spring Harb. Perspect. Biol. 1, a001651. doi:10.1101/cshperspect.a001651
Lee, I.-A., Kim, E.-J., and Kim, D.-H. (2012). Inhibitory effect of β-sitosterol on TNBS-induced colitis in mice. Planta Medica 78, 896–898. doi:10.1055/s-0031-1298486
Liao, P.-C., Lai, M.-H., Hsu, K.-P., Kuo, Y.-H., Chen, J., Tsai, M.-C., et al. (2018). Identification of β-sitosterol as in vitro anti-inflammatory constituent in moringa oleifera. J. Agric. Food Chem. 66, 10748–10759. doi:10.1021/acs.jafc.8b04555
Lin, F., Xu, L., Huang, M., Deng, B., Zhang, W., Zeng, Z., et al. (2020). β-Sitosterol protects against myocardial ischemia/reperfusion injury via targeting PPARγ/NF-κB signalling. Evidence-based Complementary Altern. Med. 2020, 2679409. doi:10.1155/2020/2679409
Loizou, S., Lekakis, I., Chrousos, G. P., and Moutsatsou, P. (2010). Beta-sitosterol exhibits anti-inflammatory activity in human aortic endothelial cells. Mol. Nutr. Food Res. 54, 551–558. doi:10.1002/mnfr.200900012
Lu, A., Magupalli, V. G., Ruan, J., Yin, Q., Atianand, M. K., Vos, M. R., et al. (2014). Unified polymerization mechanism for the assembly of ASC-dependent inflammasomes. Cell 156, 1193–1206. doi:10.1016/j.cell.2014.02.008
Malini, T., and Vanithakumari, G. (1990). Rat toxicity studies with beta-sitosterol. J. Ethnopharmacol. 28, 221–234. doi:10.1016/0378-8741(90)90032-o
Man, S. M., and Kanneganti, T.-D. (2015). Regulation of inflammasome activation. Immunol. Rev. 265, 6–21. doi:10.1111/imr.12296
Moreau, J., and Mas, E. (2015). Drug resistance in inflammatory bowel diseases. Curr. Opin. Pharmacol. 25, 56–61. doi:10.1016/j.coph.2015.11.003
Nakamura, T., Nagahori, M., Kanai, T., and Watanabe, M. (2008). Current pharmacologic therapies and emerging alternatives in the treatment of ulcerative colitis. Digestion 77 (1), 36–41. doi:10.1159/000111486
Neurath, M. F., Pettersson, S., Meyer zum Büschenfelde, K. H., and Strober, W. (1996). Local administration of antisense phosphorothioate oligonucleotides to the p65 subunit of NF-kappa B abrogates established experimental colitis in mice. Nat. Med. 2, 998–1004. doi:10.1038/nm0996-998
Ng, S. C., Shi, H. Y., Hamidi, N., Underwood, F. E., Tang, W., Benchimol, E. I., et al. (2017). Worldwide incidence and prevalence of inflammatory bowel disease in the 21st century: a systematic review of population-based studies. Lancet (London, Engl. 390, 2769–2778. doi:10.1016/S0140-6736(17)32448-0
Odenwald, M. A., and Turner, J. R. (2017). The intestinal epithelial barrier: a therapeutic target? Nat. Rev. Gastroenterology Hepatology 14, 9–21. doi:10.1038/nrgastro.2016.169
Ordás, I., Eckmann, L., Talamini, M., Baumgart, D. C., and Sandborn, W. J. (2012). Ulcerative colitis. Lancet (London, Engl. 380, 1606–1619. doi:10.1016/S0140-6736(12)60150-0
Panayotis, N., Freund, P. A., Marvaldi, L., Shalit, T., Brandis, A., Mehlman, T., et al. (2021). β-sitosterol reduces anxiety and synergizes with established anxiolytic drugs in mice. Cell Rep. Med. 2, 100281. doi:10.1016/j.xcrm.2021.100281
Paniagua-Pérez, R., Madrigal-Bujaidar, E., Reyes-Cadena, S., Molina-Jasso, D., Gallaga, J. P., Silva-Miranda, A., et al. (2005). Genotoxic and cytotoxic studies of beta-sitosterol and pteropodine in mouse. J. Biomed. Biotechnol. 2005, 242–247. doi:10.1155/JBB.2005.242
Parikh, K., Antanaviciute, A., Fawkner-Corbett, D., Jagielowicz, M., Aulicino, A., Lagerholm, C., et al. (2019). Colonic epithelial cell diversity in health and inflammatory bowel disease. Nature 567, 49–55. doi:10.1038/s41586-019-0992-y
Pierre Luhata, L., and Usuki, T. (2021). Antibacterial activity of β-sitosterol isolated from the leaves of Odontonema strictum (Acanthaceae). Bioorg. Med. Chem. Lett. 48, 128248. doi:10.1016/j.bmcl.2021.128248
Ponnulakshmi, R., Shyamaladevi, B., Vijayalakshmi, P., and Selvaraj, J. (2019). In silico and in vivo analysis to identify the antidiabetic activity of beta sitosterol in adipose tissue of high fat diet and sucrose induced type-2 diabetic experimental rats. Toxicol. Mech. Methods 29, 276–290. doi:10.1080/15376516.2018.1545815
Rogler, G. (2014). Chronic ulcerative colitis and colorectal cancer. Cancer Lett. 345, 235–241. doi:10.1016/j.canlet.2013.07.032
Shi, J., Zhao, Y., Wang, K., Shi, X., Wang, Y., Huang, H., et al. (2015). Cleavage of GSDMD by inflammatory caspases determines pyroptotic cell death. Nature 526, 660–665. doi:10.1038/nature15514
Song-Zhao, G. X., Srinivasan, N., Pott, J., Baban, D., Frankel, G., and Maloy, K. J. (2014). Nlrp3 activation in the intestinal epithelium protects against a mucosal pathogen. Mucosal Immunol. 7, 763–774. doi:10.1038/mi.2013.94
Sun, Y., Gao, L., Hou, W., and Wu, J. (2020). β-Sitosterol alleviates inflammatory response via inhibiting the activation of ERK/p38 and NF-κB pathways in LPS-exposed BV2 cells. BioMed Res. Int. 2020, 7532306. doi:10.1155/2020/7532306
Tessner, T. G., Cohn, S. M., Schloemann, S., and Stenson, W. F. (1998). Prostaglandins prevent decreased epithelial cell proliferation associated with dextran sodium sulfate injury in mice. Gastroenterology 115, 874–882. doi:10.1016/s0016-5085(98)70259-8
Thinwa, J., Segovia, J. A., Bose, S., and Dube, P. H. (2014). Integrin-mediated first signal for inflammasome activation in intestinal epithelial cells. J. Immunol. 193, 1373–1382. doi:10.4049/jimmunol.1400145
Tian, M., Ma, P., Zhang, Y., Mi, Y., and Fan, D. (2020). Ginsenoside Rk3 alleviated DSS-induced ulcerative colitis by protecting colon barrier and inhibiting NLRP3 inflammasome pathway. Int. Immunopharmacol. 85, 106645. doi:10.1016/j.intimp.2020.106645
Tong, L., Hao, H., Zhang, Z., Lv, Y., Liang, X., Liu, Q., et al. (2021). Milk-derived extracellular vesicles alleviate ulcerative colitis by regulating the gut immunity and reshaping the gut microbiota. Theranostics 11, 8570–8586. doi:10.7150/thno.62046
Wei, Y.-Y., Fan, Y.-M., Ga, Y., Zhang, Y.-N., Han, J.-C., and Hao, Z.-H. (2021). Shaoyao decoction attenuates DSS-induced ulcerative colitis, macrophage and NLRP3 inflammasome activation through the MKP1/NF-κB pathway. Phytomedicine 92, 153743. doi:10.1016/j.phymed.2021.153743
Weihrauch, J. L., and Gardner, J. M. (1978). Sterol content of foods of plant origin. J. Am. Dietetic Assoc. 73, 39–47. doi:10.1016/s0002-8223(21)05668-6
Xue, S., Xue, Y., Dou, D., Wu, H., Zhang, P., Gao, Y., et al. (2022). Kui jie tong ameliorates ulcerative colitis by regulating gut microbiota and NLRP3/caspase-1 classical pyroptosis signaling pathway. Dis. Markers 2022, 2782112. doi:10.1155/2022/2782112
Ye, L., Cao, Q., and Cheng, J. (2013). Review of inflammatory bowel disease in China. TheScientificWorldJournal 2013, 296470. doi:10.1155/2013/296470
Yu, P., Zhang, X., Liu, N., Tang, L., Peng, C., and Chen, X. (2021a). Pyroptosis: mechanisms and diseases. Signal Transduct. Target. Ther. 6, 128. doi:10.1038/s41392-021-00507-5
Yu, Y., Cao, Y., Huang, W., Liu, Y., Lu, Y., and Zhao, J. (2021b). β-Sitosterol ameliorates endometrium receptivity in PCOS-like mice: the mediation of gut microbiota. Front. Nutr. 8, 667130. doi:10.3389/fnut.2021.667130
Yuan, Y.-Y., Xie, K.-X., Wang, S.-L., and Yuan, L.-W. (2018). Inflammatory caspase-related pyroptosis: mechanism, regulation and therapeutic potential for inflammatory bowel disease. Gastroenterol. Rep. 6, 167–176. doi:10.1093/gastro/goy011
Zhang, R., Han, Y., McClements, D. J., Xu, D., and Chen, S. (2022). Production, characterization, delivery, and cholesterol-lowering mechanism of phytosterols: a review. J. Agric. Food Chem. 70, 2483–2494. doi:10.1021/acs.jafc.1c07390
Zhang, Y., Zhao, X., Zhu, Y., Ma, J., Ma, H., and Zhang, H. (2018). Probiotic mixture protects dextran sulfate sodium-induced colitis by altering tight junction protein expressions and increasing tregs. Mediat. Inflamm. 2018, 9416391. doi:10.1155/2018/9416391
Zhao, B., Xia, B., Li, X., Zhang, L., Liu, X., Shi, R., et al. (2020). Sesamol supplementation attenuates DSS-induced colitis via mediating gut barrier integrity, inflammatory responses, and reshaping gut microbiome. J. Agric. Food Chem. 68, 10697–10708. doi:10.1021/acs.jafc.0c04370
Zhen, Y., and Zhang, H. (2019). NLRP3 inflammasome and inflammatory bowel disease. Front. Immunol. 10, 276. doi:10.3389/fimmu.2019.00276
Keywords: β-sitosterol, ulcerative colitis, inflammatory factors, Caspase-1, GSDMD, pyroptosis
Citation: Zhang D, Ge F, Ji J, Li Y-J, Zhang F-R, Wang S-Y, Zhang S-J, Zhang D-M and Chen M (2023) β-sitosterol alleviates dextran sulfate sodium-induced experimental colitis via inhibition of NLRP3/Caspase-1/GSDMD-mediated pyroptosis. Front. Pharmacol. 14:1218477. doi: 10.3389/fphar.2023.1218477
Received: 07 May 2023; Accepted: 16 October 2023;
Published: 26 October 2023.
Edited by:
Yan Huang, Anhui Medical University, ChinaReviewed by:
Stefania Tacconelli, University of Studies G. d'Annunzio Chieti and Pescara, ItalySameh Saber, Delta University for Science and Technology, Egypt
Copyright © 2023 Zhang, Ge, Ji, Li, Zhang, Wang, Zhang, Zhang and Chen. This is an open-access article distributed under the terms of the Creative Commons Attribution License (CC BY). The use, distribution or reproduction in other forums is permitted, provided the original author(s) and the copyright owner(s) are credited and that the original publication in this journal is cited, in accordance with accepted academic practice. No use, distribution or reproduction is permitted which does not comply with these terms.
*Correspondence: Shu-Jing Zhang, jingshuzhang@126.com; Meng Chen, chenmeng@bucm.edu.cn; Dong-Mei Zhang, chaweto@126.com
†These authors share first authorship