- 1Department of Clinical Pharmacology, Faculty of Medicine, King Abdulaziz University, Jeddah, Saudi Arabia
- 2Department of Pharmaceutics, Faculty of Pharmacy, King Abdulaziz University, Jeddah, Saudi Arabia
- 3Department of Pharmacognosy and Phytochemistry, Moulana College of Pharmacy, Perinthalmanna, Kerala, India
Ocimum sanctum L. (Tulsi; Family: libiaceae), also known as “The Queen of herbs” or “Holy Basil,” is an omnipresent, multipurpose plant that has been used in folk medicine of many countries as a remedy against several pathological conditions, including anticancer, antidiabetic, cardio-protective, antispasmodic, diaphoretic, and adaptogenic actions. This study aims to assess O. sanctum L.’s hepatoprotective potential against galactosamine-induced toxicity, as well as investigate bioactive compounds in each extract and identify serum metabolites. The extraction of O. sanctum L as per Ayurveda was simultaneously standardized and quantified for biochemical markers: rutin, ellagic acid, kaempferol, caffeic acid, quercetin, and epicatechin by HPTLC. Hepatotoxicity was induced albino adult rats by intra-peritoneal injection of galactosamine (400 mg/kg). The quantified hydroalcoholic and alcoholic extract of O. sanctum L (100 and 200 mg/kg body weight/day) were compared for evaluation of hepatoprotective potential, which were assessed in terms of reduction in histological damage, change in serum enzymes such as AST, ALT, ALP and increase TBARS. Twenty chemical constituents of serum metabolites of O. sanctum were identified and characterized based on matching recorded mass spectra by GC-MS with those obtained from the library-Wiley/NIST. We evaluated the hepatoprotective activity of various fractions of hydroalcoholic extracts based on the polarity and investigated the activity at each phase (hexane, chloroform, and ethyl acetate) in vitro to determine how they affected the toxicity of CCL4 (40 mM) toward Chang liver cells. The ethyl acetate fraction of the selected plants had a higher hepatoprotective activity than the other fractions, so it was used in vacuum liquid chromatography (VLC). The ethyl acetate fraction contains high amounts of rutin (0.34% w/w), ellagic acid (2.32% w/w), kaempferol (0.017% w/w), caffeic acid (0.005% w/w), quercetin (0.038% w/w), and epicatechin (0.057% w/w) which are responsible for hepatoprotection. In comparison to standard silymarin, isolated bioactive molecules displayed the most significant hepatoprotective activity in Chang liver cells treated to CCl4 toxicity. The significant high hepatoprotection provided by standard silymarin ranged from 77.6% at 100 μg/ml to 83.95% at 200 μg/ml, purified ellagic acid ranged from 70% at 100 μg/ml to 81.33% at 200 μg/ml, purified rutin ranged from 63.4% at 100 μg/ml to 76.34% at 200 μg/ml purified quercetin ranged from 54.33% at 100 μg/ml to 60.64% at 200 μg/ml, purified epicatechin ranged from 53.22% at 100 μg/ml to 65.6% at 200 μg/ml, and purified kaempferol ranged from 52.17% at 100 μg/ml to 60.34% at 200 μg/ml. These findings suggest that the bioactive compounds in O. sanctum L. have significant protective effects against galactosamine-induced hepatotoxicity.
1 Introduction
Humans relied on medicinal plants for their healing properties centuries before the introduction of chemical medicines. The WHO estimates that around 80% of the people in less developed countries rely exclusively on herbal medicines for their primary healthcare. Medicinal plants serve as the backbone of traditional medicines, and nearly 3.3 billion people regularly utilize plants for therapeutic purposes in less developed countries (Davidson-Hunt, 2000). Medicinal plants are a substantial source of hepatoprotective medications. One estimate places the number of mono- and poly-herbal formulations used for treating different liver problems at over 700 in decoction, tincture, and tablets (Ilyas et al., 2016). The earliest Indian medical system, Ayurveda, has designated numerous plants for the treatment of hepatotoxicity. Given that plants have been employed as medicines for a variety of ailments and with the arrival of current synthetic medicines and their accessibility to consistent dosage forms, usage ease, and therapeutic efficiency in acute circumstances, the usage of medicinal plants has declined (Pandey et al., 2013). Traditional medications have a limited range of action and require long-term administration to be effective, working mostly on chronic illnesses, as opposed to synthetic drugs, which have a restricted spectrum of action and accompanying adverse effects (Fair and Tor, 2014). Numerous plant-based medications have been discovered to have hepatoprotective properties, including Trigonella foenum graecum belongs to the family Fabaceae (Zargar, 2014), Andrographis paniculata (Family: Acanthaceae) (Nagalekshmi et al., 2011), Phyllanthus niruri L. (Family: Phyllanthaceae), Tephrosia purpurea L. (Fabaceae), Boerhavia diffusa L (Nyctaginaceae) and A. paniculata (Family: Acanthaceae) (Dey et al., 2020), Phyllanthus maderaspatensis L. (Family: Phyllanthaceae) (Ilyas et al., 2015) and Fumaria indica L (Family: Papaveraceae) (Rathi et al., 2008). Jaundice and hepatitis are serious liver diseases with high fatality rates. An infection called hepatitis damages and inflames the liver. Swelling caused by inflammation occurs when bodily tissues are harmed or infected. Hepatitis is often classified as acute or chronic based on how long the liver has been inflamed and damaged (Schaefer and John, 2021). The five fundamental categories of viruses are categories A to E. Due to the weight of illness and mortality, these are of paramount importance. The problem may self-limit (heal on its own) or it may worsen, leading to cirrhosis and fibrosis (Eming et al., 2017). Aside from alcohol, drugs, chlorinated solvents, herbal remedies, chlorinated solvents, peroxidized fatty acids, industrial pollutants, radioactive isotope intoxication, and fungal toxins, other xenobiotics that can cause hepatic problems include parasite and viral infections, autoimmune diseases, and radio-active isotopes. In particular, types A and C induce chronic illness and are the main factors in liver cirrhosis and cancer, respectively (Ilyas et al., 2016). Only a few uncommon allopathic hepatoprotective medications are currently available for the treatment of liver disorders. Plant extracts are therefore frequently used to treat liver problems. India’s semi-tropical and tropical regions are home to Ocimum sanctum Linn. Ayurveda and Siddha traditions have historically used various components of the plant to treat a variety of illnesses, including infections, skin problems, and hepatic abnormalities, and as an antidote for snake and scorpion stings. This plant’s leaves have long been utilized for their anti-inflammatory, gastroprotective, and hepatoprotective effects (Kamyab and Eshraghian, 2013), anti-plasmodial, neuroprotective, and chemo-preventive properties (Bhattacharyya and Bishayee, 2013; Rajendran et al., 2014; Mataram et al., 2021). The plant is also effective against human pancreatic cancer, stress-induced anxiety, stress-induced oxidative and central monoaminergic changes, typhoid fever, and cerebral ischemia/reperfusion (Ahmad et al., 2012a; Ahmad et al., 2012b; Mandal et al., 2012; Shimizu et al., 2013; Singh et al., 2016). In COVID-19, Tulasi has been found to possess SARS-CoV-2 protease inhibition, lipid-lowering and antioxidant, anxiety and depression (Chatterjee et al., 2011; Suanarunsawat et al., 2011; Shree et al., 2020). This plant also contains a high concentration of polyphenolic chemicals, which have a variety of biological activities such as antiviral, antibacterial, vasodilatory, antioxidant, anti-inflammatory, and antiradical properties (Prochazkova et al., 2011), memory improvement (Spencer, 2009), gastroprotection (Mota et al., 2009), antioxidation, anti-inflammation, cardiovascular protection (Garcia-Lafuente et al., 2009), chemopreventive activity (Mohan et al., 2013) and regulate immune responses (Magrone et al., 2008). Previous research found that these plants possessed a high concentration of flavonoids, which are naturally occurring phenolic phytochemicals that have been linked to a variety of biological functions (Hollman and Katan, 1999), flavonoid glycoside is as a multi potent bioflavonoid with great potential for the prevention and treatment of disease, kaempferol glycosides is antinociceptive and anti-inflammatory (De Melo et al., 2009), anti-tyrosinase activity (Rho et al., 2010). Previously, metabolic profiling was used for a better understanding of the chemical diversity of medicinal plants. This information can be used to make comparisons with other studied taxonomically related plants and to infer their bioactivity. Chromatography coupled with mass spectrometry is the most widely used technology for analyzing samples in extremely complex matrices, such as plant extracts (Jorge et al., 2016). Both morphologically and functionally, the galactosamine-induced experimental model system in rats is known to resemble viral hepatitis in people. Because hepatocytes have significant quantities of galactokinase and galactose-1-uridylyltransferase, galactos-amine has a greater liver selectivity than other hazardous groups, such as acetaminophen, paracetamol, and carbon tetrachloride. Hepatotoxicity, substantial portal and parenchymal infiltration, and patchy hepatocyte necrosis are all induced by galactosamine (Ilyas et al., 2015). By boosting the creation of UDP-sugar derivatives, galactosamine also causes the depletion of uridine diphosphate (UDP), which inhibits the synthesis of RNA and proteins and, in turn, deteriorates cell membranes. Chattopadhyay et al. (1992) and Jain (2015) revealed that only crude extracts are responsible for activity but did not isolate active ingredients. We identified, evaluated and isolated the most active bioactive molecules responsible for hepatoprotection in this study. The plan of the study was focused on bioactivity guided fractionation, O. sanctum (ethyl acetate) fractions showed significant hepatoprotective activity (cell induced with carbon tetrachloride 40 mM) as compared with other fractions, which led to the further separation of ethyl acetate fraction by vacuum Liquid chromatography and followed by the quantitative chemo profiling of the potent fraction. The chemical constituents were potent activity responsible for hepatoprotective activity will be identified and validated. A single newly developed solvent system formic acid, ethyl acetate, and toluene (1:4:5) was used for the densitometric quantification of bioactive compounds by HPTLC in aqueous alcoholic extracts with reference to respective marker compounds such as ellagic acid, rutin, kaempferol, quercetin, caffeic acid, and epicatechin in O. sanctum. The purpose of this study was to use GC-MS in order to investigate the metabolic profile of the hexane of the serum metabolites of O. sanctum and to investigate potent bioactive compounds obtained from O. sanctum for their hepatoprotective activity.
2 Material and methods
2.1 Reagents and chemicals
We bought reference standards from Natural Remedies Pvt. Ltd., including rutin, kaempferol, quercetin, epicatechin, caffeic acid, and catechin (Bangalore, India). We bought ALT, AST, and ALP kits from Span Diagnostics Ltd. in Surat, India. The supplier of galactosamine was SRL in Mumbai, India. Analytical-grade chemicals were employed throughout. As mobile phases for a HPTLC analysis, formic acid, ethyl acetate, toluene, and methanol (CDH Labs, Mumbai, India) were utilized. A 0.22 μm syringe-driven filter was utilized to filter all the solutions for the analysis (HIMEDIA, Mumbai, India).
2.2 Extract preparation
Fresh plant material was taken from the Maruthmallai region of Kanyakumari district, Tamilnadu, India. It was identified and authenticated by Dr. V. Chelladurai, Research Officer, Central Council for Research in Ayurveda and Siddha (Govt. of India), Tirunelveli, Tamil Nadu, India. A voucher specimen has been stored in our laboratory for future reference. Plant materials were extracted using 95% alcohol for 6 h at 37°C and an aqueous-alcoholic solvent (50%) for 5 h at 38°C. The extraction process was carried out three times. The mixed extracts were collected and dried in a rotary evaporator at 40°C under decreased pressure.
2.3 Animals experimental design
The Central Animal House facility (Registration No. 173/CPLSEA/837) provided adult Wistar rats weighing 150–200 g. The animals were fed a typical rodent diet and given access to water as needed while being kept in regular laboratory conditions (12 h light/dark cycles at 25°C with humidity levels between 45% and 65%). The animals were divided into eight groups (n = 6) at random. Group I acted as the vehicle control and was given regular saline for a period of 7 days. In addition to receiving normal saline (1 ml/kg, p.o.) for 7 days, Group II acted as the toxic control group. Groups III to VIII received a prophylactic treatment of a hydroalcoholic and alcoholic extract of O. sanctum in carbon methyl cellulose (0.1%) at various concentrations (100 and 200 mg/kg b.w.p.o) for 7 days. Silymarin (40 mg/kg, p.o.) was administered to Group VIII for 7 days. The current study evaluated the dose of O. sanctum extracts based on prior research studies conducted by Chattopadhyay et al. (1992). On the eighth day, 400 mg/kg ip of galactosamine was administered to Groups II to VIII to cause liver damage (Raish et al., 2016).
2.3.1 Evaluation of liver function
After giving galactosamine for 24 h, blood was drawn from the retro-orbital plexus while the groups were lightly sedated with ether (Van Herck et al., 2001). All of the groups were sacrificed immediately following blood collection. Samples of the liver were obtained for histopathological and biochemical analyses. At 37°C, serum was centrifuged to separate it, and it was then used to measure various biochemical characteristics. Using a motor-driven Teflon pestle, 10% (w/v) liver homogenates were produced in an ice-cold 0.15 M KCl solution after the liver samples were rinsed with chilled normal saline and weighed (AlSaid et al., 2015). Additional biochemical parameters, including the determination of aspartate amino transaminase (AST), alanine amino transaminase (ALT), and alkaline phosphatase (ALP), were estimated using the serum (Patlolla et al., 2011). The liver homogenate’s supernatant was used to measure antioxidant enzymes, such as catalase (CAT) and superoxide dismutase (SOD), using a colorimetric technique (Weydert and Cullen, 2010). The DTNB method was used to calculate glutathione (GSDH), and a modified approach was used to calculate TBARS, an index of lipid peroxidation (Liu et al., 2020). The quickly excised liver tissues were stored in neutral buffered formalin. According to Badawi’s instructions, liver slices were created for histological investigations (Badawi, 2019).
2.4 GC-MS analysis of metabolic serum
An analysis was performed on the chemical makeup of the metabolic serum. Gas chromatography (GC-MS) was used to characterize 20 active compounds. The Agilent 7890AGC system was connected to a 5975C inert XL EI/CI MSD mass spectroscopic system, which was outfitted with a 30 m × 250 μm × 0.25 µm HP-5MS capillary column. A CTCCombiPAL injector was used, and the injection volume was 2.0 L. With a helium flow rate of 1.0 ml·min−1, the inlet temperature was maintained at 270°C. Programmatically, the column’s temperature was increased from 60°C to 300°C at a rate of 5°C per minute for a total run period of 40 min in SCAN mode.
2.4.1 Preparation of sample
The serum of the O. sanctum extract (5 ml) was extracted with acetonitrile (3 × 100). The combined acetonitrile layer was evaporated to dryness under reduced pressure at 40°C in a rotary evaporator to obtain a residue. The residue was dissolved in LC-MS-grade methanol, filtered through a 0.22 µm syringe, and injected into GC-MS.
2.5 Densitometric quantification of bioactive compounds in ethyl acetate fraction using HPTLC
2.5.1 Sample preparations
The aqueous ethanolic extract of O. sanctum was dissolved in 10% distilled water and was successively fractionated thrice with hexane (3 × 600 mL), chloroform (3 × 600 mL), ethyl acetate (3 × 500 mL), and water-soluble fractions. The combined fractions of O. sanctum were evaporated to dryness under reduced pressure at 40°C in a rotary evaporator. 100 mg of ethyl acetate fraction were accurately weighed, dissolved in 10 mL of HPLC grade methanol, sonicated for 10 min, and then made up with 10 mL of methanol. After filtering the solution, a 0.22 µm syringe was used to inject it into the HPTLC system.
2.5.2 Preparation of standard solutions
The standard solution was made by dissolving precisely weighed 10.0 mg of rutin, kaempferol, quercetin, ellagic acid, and epicatechin in 10.0 mL of methanol HPLC grade as stock solution and storing it at 4°C. These standards were further diluted to achieve the desired concentration for quantification.
2.5.3 Preparation of the plate
Prior to usage, pre-coated silica gel 60 F254 aluminium HPTLC plates (Merck, Germany) were washed in methanol and dried. The standard solution of bioactive compounds and samples were applied on an HPTLC plate in the form of bands of 4 mm width using a Linomat V applicator (Muttenz, Switzerland) with a 100 µL syringe. The application rate was kept constant at 200 nL·s−1, and the space between the two bands was 9 mm.
2.5.4 Calibration curve of bioactive compounds
Standard concentrations of rutin (10–1,600 ng/band), epicatechin (100–5,000 ng/band), ellagic acid (20–200 ng/band), kaempferol (40–200 ng/band), quercetin (10–160 ng/band) were applied in triplicate on silica-gel 60 F254 plates using a CAMAG Linomat-5 Automatic Sample Spotter. The plates were developed in formic acid, ethyl acetate, and toluene (1:4:5 v/v/v) solvent in a CAMAG glass twin-trough chamber (20 cm × 100 cm) up to a distance of 8 cm. After development, the plates were dried in air and scanned at 366 nm using a CAMAG TLC Scanner 3 and Win CATS 4 software. The peak areas were recorded. Calibration curves for bioactive compounds were created by plotting peak areas versus applied ethyl acetate fractions conaining rutin, epicatechin, ellagic acid, kaempferol, quercetin respectively.
2.6 Separation and purification of bioactive compounds
The ethyl acetate fraction has been shown to have a strong hepatoprotective impact in comparison to other fractions. To separate the various components contained in the fraction, vacuum liquid chromatography (VLC) was used on this fraction. Dry slurry was made by mixing a small amount of Silica gel G (Merck) with 15 g of ethyl acetate, and this slurry was then placed onto a sintered glass funnel with Silica gel G as the stationary phase. The column was eluted with solvents of increasing polarity step-by-step under a vacuum, starting with a blend of pure toluene and ethyl acetate and finishing with pure ethyl acetate. To further clarify, the toluene levels were decreased when the ethyl acetate component was increased after initially eluting with 5% ethyl acetate in toluene. The ethyl acetate component was then increased by 5% increments up to 50% and then by 10% increments up to 100% ethyl acetate. The methanol content was increased in 5% steps after elution with ethyl acetate, elution with 5% methanol in ethyl acetate, and elution with 100% methanol. The solvents were eluted until they ran clear from the funnel. Throughout the experiment, a steady flow rate of the solvent (100 ml/min) was observed. Individual fractions were collected, and their homogeneity was checked using TLC. The same Rf values from similar fractions were merged and crystallized.
2.7 HPTLC study of column eluents of ethyl acetate fraction
The fractions obtained from the VLC were analyzed by using HPTLC, and fractions with a similar profile were combined. Aliquots of these fractions were reconstituted in methanol and subjected to an HPTLC study. Small quantities of dried eluents were dissolved in about 1 ml of HPLC-grade methanol. The sample was sprayed onto a plate at a distance of 0.8 cm from one end of the plate in the form of a narrow band using the spray-on technique with the help of a Linomate-V applicator attached to a CAMAG HPTLC system that was programmed using win-CATS software, which was included with the apparatus. Following the application of the spot, a chromatogram was generated using several solvent systems in a twin-trough chamber (20 cm × 25 cm) that had previously been saturated with a formic acid, ethyl acetate, and toluene combination (1:4:5 v/v/v). The air-dried plate was viewed under the different wavelengths of UV light and visible light. The spots were scanned using Reprostar 3; the TLC scanner 3 was designed for the densitometric evaluation of plates.
2.8 Hepatoprotective assay of fractions, bioactive compounds, and column eluents of ethyl acetate fraction
Cells were plated in 96-well plates at a density of 1 × 10 6 cells/well and allowed to grow there overnight. After 24 h, the medium was removed, the cells were given different quantities of treatment of each sample (100 and 200 μg/ml), and they were then incubated for 2 h in separate wells of a 96-well plate. As a benchmark, silymarin (100 and 200 μg/ml) was used. CCl4 was applied to the cell and incubated with it for 2 h. Following incubation, the cells were washed, and each well received 20 μL of MTT (5 mg/ml of MTT in PBS) for 1 h. After 2 h, a microscope was used to witness the formation of a formazan crystal. An additional hour of incubation was added if the crystal formation was not accurate. Following the removal of the medium, the leftover formazan crystals in each well were dissolved in 200 μL of DMSO. The cell culture plate was shaken for 15 min, while the absorbance was measured at 540 nm using an ELISA reader.
The following formula was used to calculate the percentage of the hepatoprotection of the polyphenols, fractions, column eluents, and silymarin:
2.9 Statistic evaluation
The results are given as mean S.E.M. Dunnett’s post hoc test was used after a one-way analysis of variance (ANOVA) to estimate the total variation present in a data set. p < 0.01 was regarded as statistically significant.
3 Results and discussion
3.1 Effect of different extracts of O. sanctum on biochemical parameters in rats
The present study showed that analyzed biochemical markers, which included AST, ALT, ALP, and TBARS Figures 1A–D respectively, were found to be considerably higher in galactosamine-treated rat compared to the normal control group (p < 0.01) There was also a substantial drop in SOD, CAT, and glutathione Figures 2A–C respectively levels in the tissue (p > 0.05). Hydroalcoholic extract was found to be more effective than ethanolic extracts. Figures 1, 2 shows the effect of alcoholic, hydroalcoholic and silymarin pretreatment on biochemical parameters of galactosamine-intoxicated rats. Previous study, only O. sanctum extract has been reported to have hepatoprotective activity against paracetamol-induced liver damage in Albino rats (Lahon and Das, 2011). The hepatoprotective and antioxidant activities of the crude fractions of the endophytic fungi of O. sanctum in rats were reported by Shukla et al. (2012) revealed that only crude extracts are responsible for activity but did not isolate active ingredients. Present investigation, the hepatoprotection may be attributable to numerous bioactive active moieties discovered in high concentrations in the hydroalcoholic extract of O. sanctum. Such moieties include bioactive compounds were simultaneously quantified using HPTLC. Bioactive active moieties act as potent free radical scavenging ability of hydroalcoholic extract provides evidence for the role of tannins and flavonoids as potential secondary metabolite responsible for hepatoprotection (Li et al., 2018). Their action may be mediated by preventing liver injury by stopping the development of lipid peroxides and inhibiting oxidative mechanisms that contribute to hepatocyte degeneration (Cichoż-Lach and Michalak, 2014).
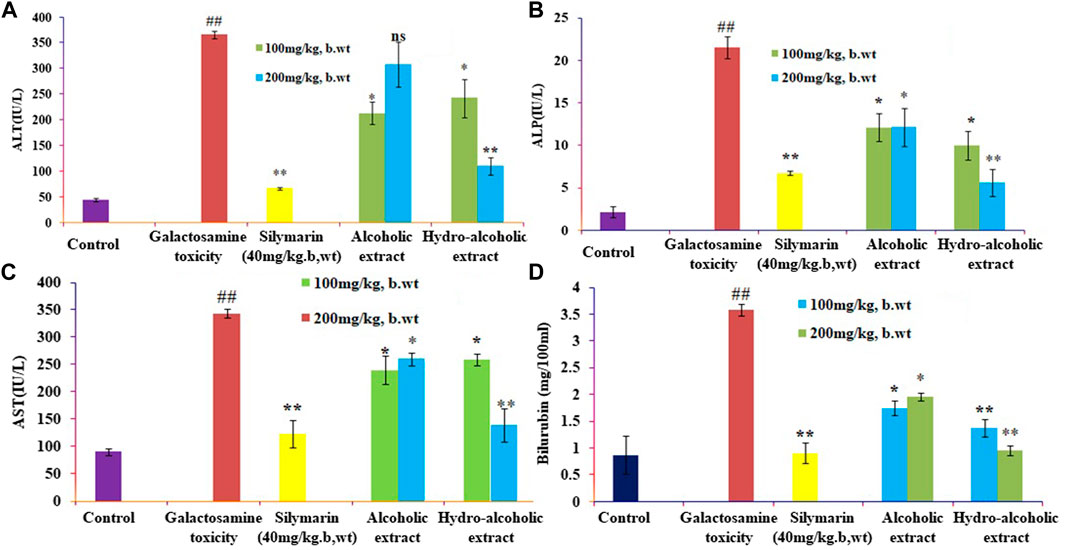
FIGURE 1. Effects of alcoholic and hydroalcoholic extracts (at two different doses of 100 and 200 mg/kg body weight, administered orally for 7 days) and standard silymarin on biochemical parameters such as ALT or alanine aminotransferase (A), ALP or Alkaline phosphatase (B), ASTor aspartate aminotransferase (C), and bilirubin (D) of liver on oxidative stress induced by galactosamine. GalN was administered intraperitoneally on the eighth day as a pretreatment. Each value represents means ± standard error of the mean. ns p > 0.05 (non-significant), *p < 0.05 (significant), **p < 0.01 (more significant).
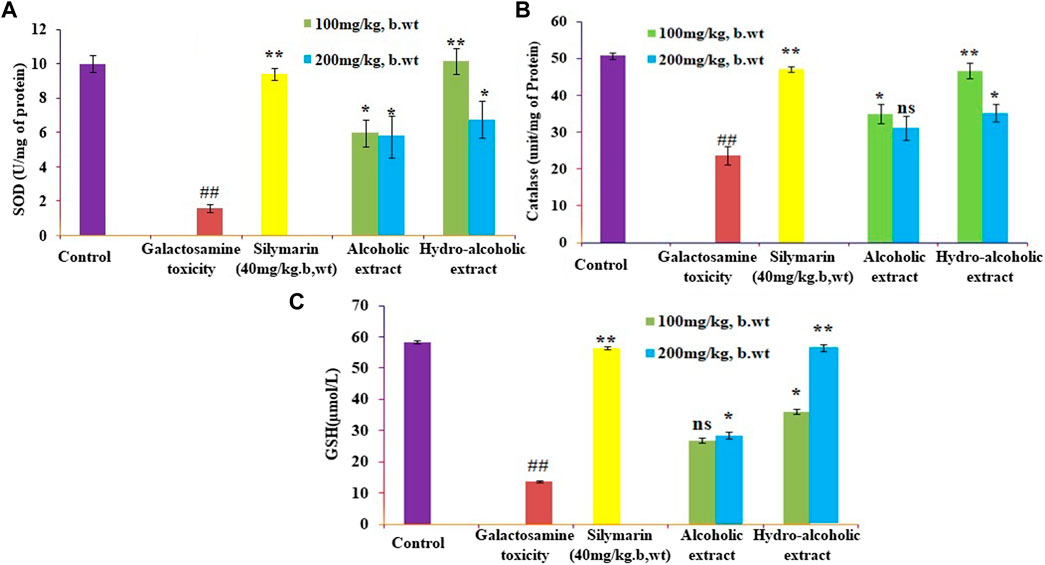
FIGURE 2. Effects of alcoholic and hydroalcoholic extracts (at two different doses of 100 and 200 mg/kg body weight, administered orally for 7 days) and standard silymarin on antioxidant parameters such as SOD or Superoxide dismutase (A), GSH or Glutathione (B), and CAT or catalase (C) of the liver on oxidative stress induced by galactosamine. GalN was administered intraperitoneally on the eighth day as a pretreatment. Each value represents means ± standard error of the mean. ns p > 0.05 (non-significant), *p < 0.05 (significant), **p < 0.01 (more significant).
3.2 Histopathological observation
Following histology, the normal control animals’ livers had a well-defined central vein, a well-preserved cytoplasm, and a conspicuous nucleus and nucleolus. The galactosamine-treated animals’ liver sections revealed severely toxic liver cells that had necrotic liver cells, focal hemorrhages in the periportal region, inflammatory cell collection, and distributed inflammation throughout the liver parenchyma. The hepatic cells with a well-preserved cytoplasm revealed that the O. sanctum hydroalcoholic extracts appeared to be more significant than the other extracts for the avoidance of galactosamine toxicity. Silymarin also defended against the hepatic alterations induced by galactosamine. Further, the hepatotoxic galactosamine-treated rats’ liver sections underwent a histological analysis, which revealed a clear inflammatory cell infiltration around the portal triad. In the periportal region, it was found that there were necrotic liver cells and localized bleeding. However, the absence of necrosis and the development of normal hepatic cells in the liver sections of the rats given silymarin and the alcoholic and hydroalcoholic extracts of O. sanctum in various groups indicated signals of protection at a significant level. Figure 3 shows a histopathologic segment of liver (400X) stained with hematoxylin and eosin to reveal the cell nucleus and cytoplasm with extracellular matrix in purple blue and pink colours, respectively.
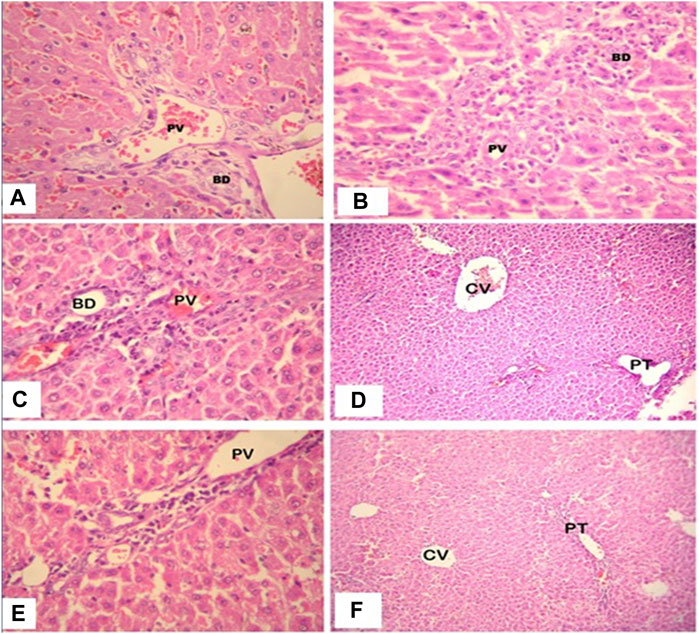
FIGURE 3. High power photomicrograph (400x) of liver section, the control group (A) shows normal hepatic architecture; in the galactosamine-induced necrotic liver cells and focal hemorrhages (B); in the alcoholic extracts plus galactosamine (C,D), there is moderate inflammatory cell infiltration; and in the hydroalcoholic extracts plus galactosamine (E,F), there is only mild inflammatory cell infiltration.
3.3 Metabolic fingerprinting of faded extract serum by GC-MS
The immunomodulatory activity of crude extract in the previous investigation by Bochu et al. (2005) was not significantly affected; however, crude extract metabolites exhibited higher immunomodulatory action than crude extract. In the current investigation, a thorough analysis was done with reference to Bochuet et al. Rat feeding trials were done with various quantities of aqueous-alcoholic (50%) extracts of chosen medicinal plants (100 and 200 mg/kg, b/w). The serum metabolites were initially analyzed using HPTLC. No distinct separation/peak was observed, which indicated that the serum was a mixture of compounds. Subsequently, the serum was analyzed using GC-MS. The serum metabolites from the extract-fed rats were identified using GC-MS, which were found to be fatty and aliphatic compounds. The process of identifying bioactive components was based on comparing the recorded mass spectra to those received from the Wiley/NIST collection. Metabolite serum, which is described in Table 1, characterized 20 different components in total. A literature review revealed that the discovered serum metabolites had no significant impact on hepatoprotective activity, thus we concentrated primarily on the ethyl acetate fraction, which contained the most significant bioactive molecule for hepatoprotection.
3.4 HPTLC quantification of bioactive compounds in ethyl acetate fraction
3.4.1 Development of the perfect mobile phase
For the simultaneous estimation of bioactive compounds, different proportions of toluene, ethyl acetate such as toluene/ethyl acetate (3:0 v/v), toluene/ethyl acetate (5:5 v/v), toluene/ethyl acetate (7:3 v/v), toluene/ethyl acetate/methanol (7:2.8:0.2 v/v/v), toluene/ethyl acetate/formic acid (7:2.5:0.5 v/v/v) and toluene/ethyl acetate/formic acid (5:4:1 v/v/v) were evaluated as the solvent systems for the development of a suitable band. All investigated solvent systems were developed under chamber saturation conditions. From the obtained results, it was observed that the solvent systems toluene/ethyl acetate (3:7 v/v), toluene/ethyl acetate (5:5 v/v), toluene/ethyl acetate (7:3 v/v), toluene/ethyl acetate/methanol (7:2.8:0.2 v/v/v), toluene/ethyl acetate/formic acid (7:2.5:0.5 v/v/v) offered the poor densitometry peaks of bioactive compounds with tailing factor. However, when the toluene/ethyl acetate/formic acid (5:4:1v/v/v) was studied, it was observed that this solvent system offered a well-separated and intact chromatographic peak of rutin at Rf = 0.08, ellagic acid at Rf = 0.55, quercetin at Rf = 0.62, kaempferol at Rf = 0.67 and epicatechin at Rf = 0.57 respectively. The typical band of the bioactive compounds and ethyl acetate fraction of O. sanctum was scanned at 254 nm (Figure 4). Vasilisa et al. (2020) also established the mobile phase mixture of diethyl ether, formic acid, acetic acid, water, acetophenone and heptane (30:3:9:50:30:10) (v/v/v/v/v/v) (Pedan et al., 2020). Ilyas et al. (2015) reported a solvent mixture of toluene: ethyl acetate, formic acid and methanol (3:3:0.8:0.2) (v/v/v/v) respectively, for simultaneous quantification of bioactive compounds (Ilyas et al., 2015). Bioactive compounds are naturally occurring substances with the potential to protect the liver from damage and act as antioxidants (Kamyab and Eshraghian, 2013; Saha et al., 2019). The hepatoprotective and antioxidant potential of bioactive compounds may be due to their capability to normalize impaired membrane function (De Oliveira e Silva et al., 2012).
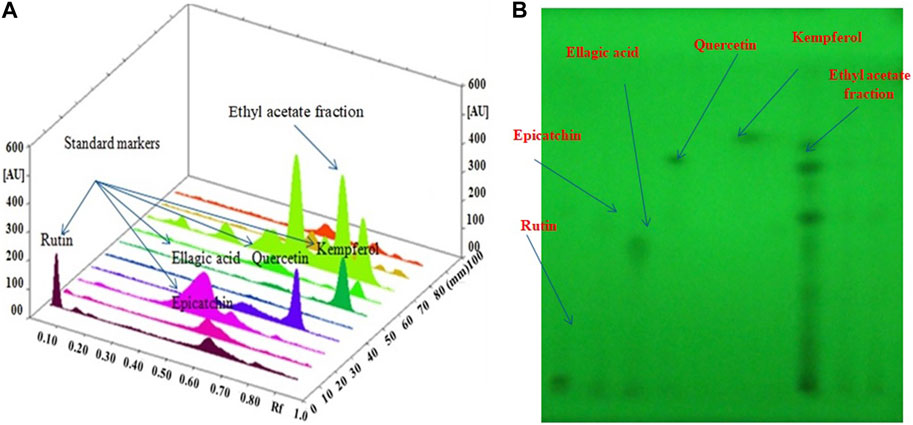
FIGURE 4. (A): 3D image of all tracks at 254 nm demonstrating that the ethyl acetate fraction had bioactive compound; (B): HPTLC Chromatogram of the ethyl acetate fraction contained bioactive compound at 254 nm in the solvent system: a combination mixture of formic acid: ethyl acetate:toluene (1:4:5 v/v/v).
3.5 Hepatoprotective activity of fractionated extracts of O. sanctum
Harsha et al. (2020) investigated cytotoxicity assay of O. sanctum extract on leukemic cell lines: A preliminary in-vitro study. We are looking into the cytotoxicity of extracts against Chang liver cells. Drugs concentrations ranging from 100 to 1000 μg/ml were used to evaluate the percentage growth inhibition of the drugs on cell lines. The drug sample exhibited a CTC50 value greater than 1000 μg/ml (concentration required to inhibit viability by 50%). We assessed the hepatoprotective activity of several O. sanctum fractions based on polarity and studied the activity in each phase (hexane, chloroform, and ethyl acetate) as well as against CCl4-induced hepatotoxicity. The CCL4 treatment of Chang liver cells resulted in a considerable drop in cell viability. When compared to the other fractions, treatment with the ethyl acetate soluble fractions showed more significant dose-dependent protection against cell damage brought on by CCL4 exposure. The results are presented using the formula mean standard error mean (S.E.M.). Figure 5 shows the percentage of protection graphically.
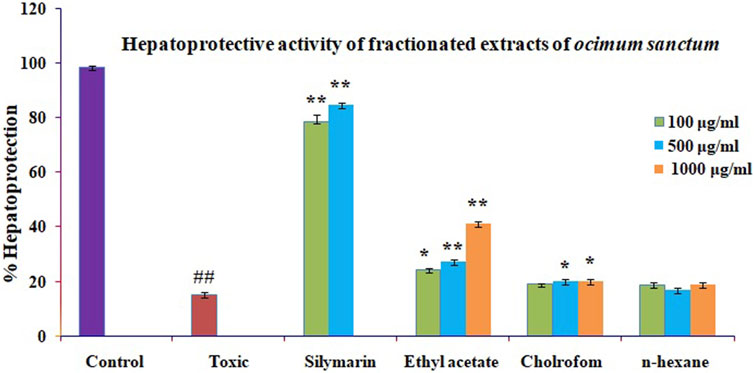
FIGURE 5. Effect of different fractions on toxicity caused by GlaN in Chang liver cells. Before being treated with GalN (40 mM) for 2 h, Chang liver cells were first incubated for 2 h in the presence or absence of extracts and their metabolites. The cells were then prepared for the MTT experiment. The findings are presented as mean S.E.M. Compared to the GalN (40 mM) control, ns p > 0.05, **p < 0.01, and *p < 0.05 are significant.
3.6 Vacuum liquid chromatography of potent ethyl acetate fraction
The ethyl acetate fraction of O. sanctum was used to perform vacuum liquid chromatography, yielding twenty-eight column eluents. HPTLC was used to evaluate these column eluents in order to identify bioactive compound from the ethyl acetate fraction of O. sanctum (Table 2). The different components contained in the eluents were detected by spraying with NP reagents and then comparing the Rf value to the standards (Gallic acid, ellagic acid, Kaemferol, quercetin, rutin, epicatechin, ursolic acid and catechin). These bioactive compounds are responsible for a variety of biological functions, including anticancer (Horcajada-Molteni et al., 2000), anti-oxidant (Afanas’ev et al., 1995), chemopreventive (Araujo et al., 2011), and immunosuppressive (Yu et al., 2011). We selected the most powerful bioactive molecule for hepatoprotection in the current investigation and subsequently isolated active moieties.
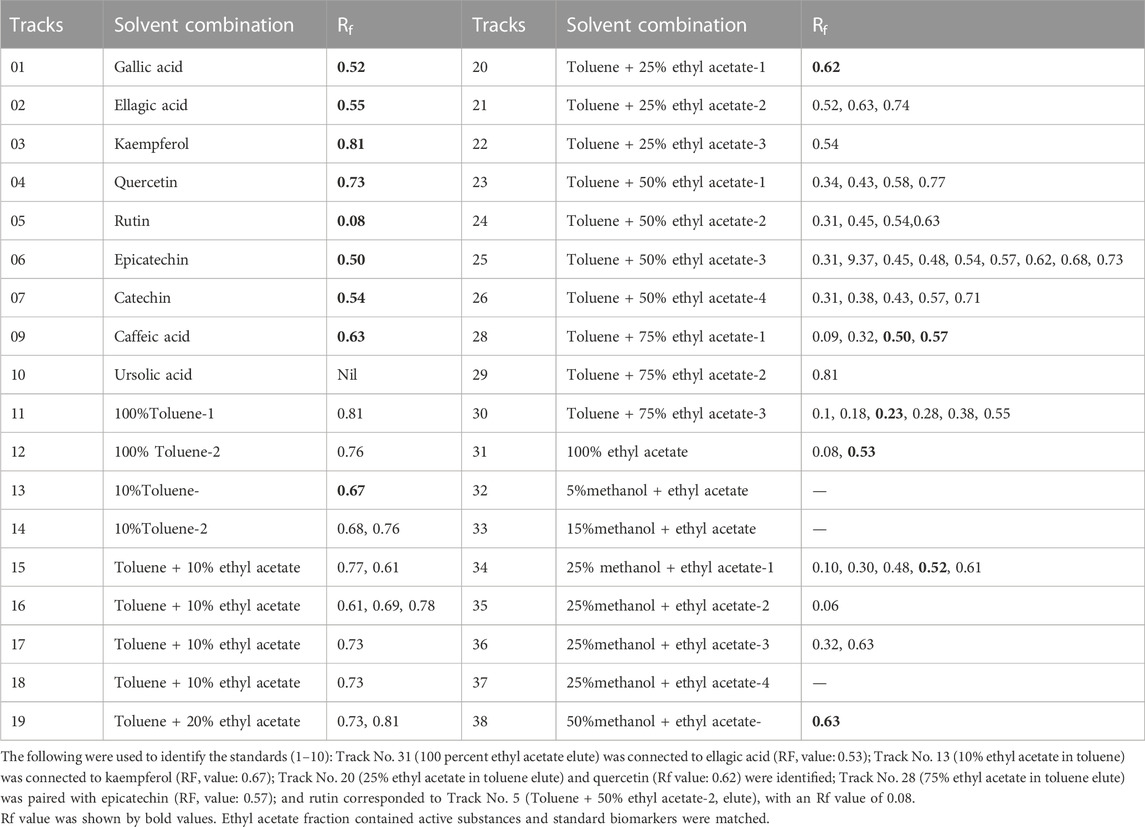
TABLE 2. Solvent combinations and Rf value of column eluents of ethyl acetate fraction of O. sanctum.
3.7 Hepatoprotective activity of column eluents of ethyl acetate fractions
The hepatoprotective activity of the ten ethyl acetate column eluents previously mentioned against CCL4-induced cytotoxicity was examined by pre-incubating the cells with or without the ethyl acetate fractions or silymarin. The Chang liver cells exposed to CCl4 saw a significant reduction in cell viability. The mean standard error means (S.E.M.) formula was used to present the results. The proportion of protection is graphically shown in Figure 6. The elute from the ethyl acetate (100%) column showed that ellagic acid has significant hepatoprotective effects, followed by 75% ethyl acetate elutes (catechin and epicatechin), 50% ethyl acetate (rutin), 25% ethyl acetate (quercetin and kaempferol), and 25% methanol (caffeic acid), when compared with standard silymarin.
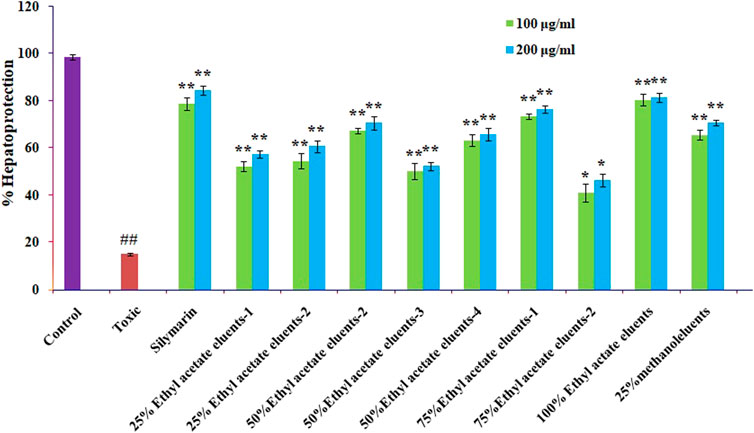
FIGURE 6. Effect of different columns eluting O. sanctum’s ethyl acetate on CCl4-induced toxicity in Chang liver cells. Prior to being treated with CCl4 for 2 h, Chang liver cells were cultured for 2 h in the presence or absence of various columns elutes of O. sanctum’s ethyl acetate. The cells were then prepared for the MTT experiment. The findings are presented as mean S.E.M. Compared to the CCL4 control, ns p > 0.05, **p < 0.01, and *p < 0.05 are significant.
3.8 Hepatoprotective activity of isolated polyphenols
The mentioned bioactive compounds were examined for hepatoprotective effectiveness against CCl4-induced cytotoxicity by pre-incubating the cells with or without the silymarin or extracts. The Chang liver cells’ viability significantly decreased after CCL4 therapy. The results are displayed using the mean standard error mean formula (S.E.M.). The significant high hepatoprotection provided by standard silymarin ranged from 77.6% at 100 μg/ml to 83.95% at 200 μg/ml, purified ellagic acid ranged from 70% at 100 μg/ml to 81.33% at 200 μg/ml, purified rutin ranged from 63.4% at 100 μg/ml to 76.34% at 200 μg/ml purified quercetin ranged from 54.33% at 100 μg/ml to 60.64% at 200 μg/ml, purified catechin ranged from 53.22% at 100 μg/ml to 65.6% at 200 μg/ml, and purified kaempferol ranged from 52.17% at 100 μg/ml to 60.34% at 200 μg/ml, as shown in Figure 7.
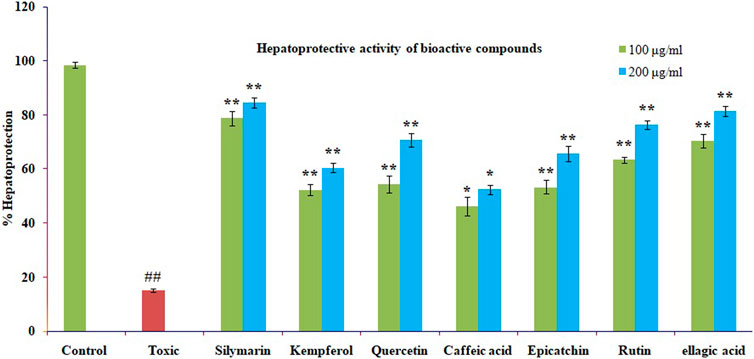
FIGURE 7. The impact of several isolated bioactive compounds on CCl4-induced toxicity in Chang liver cells. Chang liver cells were treated with CCl4 for 2 h after being cultured for 2 h with various substances in either their presence or absence. The cells were then prepared for the MTT experiment. In comparison to the CCl4 control, the data are expressed as mean S.E.M. with ns p > 0.05, **p < 0.01, and *p < 0.05 being significant.
4 Conclusion
The recommended HPTLC method is unique, as it documents the first time that bioactive compounds in O. sanctum (Linn) were identified and quantified using a single solvent system. Feeding experiments were carried out with various concentrations (100 and 200 mg/kg, b w) of alcoholic and aqueous–alcoholic extracts (50%) of selected medicinal plants, and the identification of serum metabolites from the extract-fed rats was carried out using GC-MS. It might be concluded that the metabolites of the serum extract contain a lot of fatty and aliphatic compounds not responsible for hepatoprotective activity. The impact of pretreatment with the alcoholic and hydroalcoholic extracts of O. sanctum and silymarin on the biochemical parameters of the rats’ given galactosamine intoxication was examined. According to the findings, the hydroalcoholic extract had a greater hepatoprotective effect than the alcoholic extracts. The hydroalcoholic extract was fractionated into petroleum ether, ethyl acetate, chloroform, and water based on polarity. O. sanctum’s ethyl acetate fraction had more hepatoprotective effects than the other fractions. Hence, it was concluded that ethyl acetate is the best fraction for vacuum liquid chromatography. Our research reveals that bioactive compounds extracted from O. sanctum provide significant protective benefits against hepatotoxicity caused by galactosamine. This might be a result of the antioxidant and membrane-stabilizing properties of these chemicals. The results show that it is a source of structurally unique bioactive compounds, which may offer possibilities for the creation of novel semi-synthetic molecules for more current indications.
Data availability statement
The original contributions presented in the study are included in the article/Supplementary Material, further inquiries can be directed to the corresponding authors.
Ethics statement
The animal study was approved by the Jamia Hamdard, Hamdard University, New Delhi, India. The study was conducted in accordance with the local legislation and institutional requirements.
Author contributions
FK: Formal analysis, software, investigation, writing—reviewing. ShK: Software, investigation, writing—reviewing and editing; DB and HA: Investigation, reviewing and editing. SaK: Project administration, reviewing, and funding acquisition. UI: Conceptualization, methodology, supervision, writing—original draft preparation, writing—reviewing and editing. All authors contributed to the article and approved the submitted version.
Funding
This research work was funded by the institutional Fund Projects under Grant No. (IFPIP: 738-249-1443). The authors gratefully acknowledge the technical and financial support provided by the Ministry of Education and King Abdulziz University, DSR, Jeddah, Saudi Arabia.
Conflict of interest
The authors declare that the research was conducted in the absence of any commercial or financial relationships that could be construed as a potential conflict of interest.
Publisher’s note
All claims expressed in this article are solely those of the authors and do not necessarily represent those of their affiliated organizations, or those of the publisher, the editors and the reviewers. Any product that may be evaluated in this article, or claim that may be made by its manufacturer, is not guaranteed or endorsed by the publisher.
References
Afanas'ev, I. B., Ostrachovitch, E. A., Abramova, N. E., and Korkina, L. G. (1995). Different antioxidant activities of bioflavonoid rutin in normal and iron-overloading rats. Biochem. Pharmacol. 50, 627–635. doi:10.1016/0006-2952(95)00173-w
Ahmad, A., Rasheed, N., Chand, K., Maurya, R., Banu, N., and Palit, G. (2012a). Restraint stress-induced central monoaminergic & oxidative changes in rats & their prevention by novel Ocimum sanctum compounds. Indian J. Med. Res. 135, 548–554.
Ahmad, A., Khan, M. M., Raza, S. S., Javed, H., Ashafaq, M., Islam, F., et al. (2012b). Ocimum sanctum attenuates oxidative damage and neurological deficits following focal cerebral ischemia/reperfusion injury in rats. Neurol. Sci. 33, 1239–1247. doi:10.1007/s10072-012-0940-1
AlSaid, M., Mothana, R., Raish, M., Al-Sohaibani, M., Al-Yahya, M., Ahmad, A., et al. (2015). Evaluation of the effectiveness of Piper cubeba extract in the amelioration of CCl4-induced liver injuries and oxidative damage in the rodent model. Biomed. Res. Int. 2015, 359358. doi:10.1155/2015/359358
Araujo, J. R., Goncalves, P., and Martel, F. (2011). Chemopreventive effect of dietary polyphenols in colorectal cancer cell lines. Nutr. Res. 31, 77–87. doi:10.1016/j.nutres.2011.01.006
Badawi, M. S. (2019). Histological study of the protective role of ginger on piroxicam-induced liver toxicity in mice. J. Chin. Med. Assoc. 82, 11–18. doi:10.1016/j.jcma.2018.06.006
Bhattacharyya, P., and Bishayee, A. (2013). Ocimum sanctum Linn. (Tulsi): An ethnomedicinal plant for the prevention and treatment of cancer. Anticancer Drugs 7, 659–666. doi:10.1097/CAD.0b013e328361aca1
Bochu, W., Liancai, Z., and Qi, C. (2005). Primary study on the application of Serum Pharmacology in Chinese traditional medicine. Colloids Surf. B Biointerfaces. 43, 194–197. doi:10.1016/j.colsurfb.2005.04.013
Chatterjee, M., Verma, P., Maurya, R., and Palit, G. (2011). Evaluation of ethanol leaf extract of Ocimum sanctum in experimental models of anxiety and depression. Pharm. Biol. 49, 477–483. doi:10.3109/13880209.2010.523832
Chattopadhyay, R. R., Sarkar, S. K., Ganguly, S., Medda, C., and Basu, T. K. (1992). Hepatoprotective activity of Ocimum sanctum leaf extract against paracetamol included hepatic damage in rats. Indian J. Pharmacol. 24, 163–165.
Cichoż-Lach, H., and Michalak, A. (2014). Oxidative stress as a crucial factor in liver diseases. World J. Gastroenterol. 20 (25), 8082–8091. doi:10.3748/wjg.v20.i25.8082
Davidson-Hunt, I. (2000). Ecological ethnobotany: Stumbling toward new practices and paradigms. MASA J. 16, 1–13.
De Melo, G. O., Malvar Ddo, C., Vanderlinde, F. A., Rocha, F. F., Pires, P. A., Costa, E. A., et al. (2009). Antinociceptive and anti-inflammatory kaempferol glycosides from Sedum dendroideum. J. Ethnopharmacol. 124, 228–232. doi:10.1016/j.jep.2009.04.024
De Oliveira e Silva, A. M., Vidal-Novoa, A., Batista-González, A. E., Pinto, J. R., Portari Mancini, D. A., Reina-Urquijo, W., et al. (2012). In vivo and in vitro antioxidant activity and hepatoprotective properties of bioactive compounds from Halimeda opuntia (Linnaeus). Redox Rep. 17, 47–53. doi:10.1179/1351000212Y.0000000003
Dey, D., Chaskar, S., Bhatt, N., and Chitre, D. (2020). Hepatoprotective activity of BV-7310, a proprietary herbal formulation of Phyllanthus niruri,Tephrosia purpurea, boerhaviadiffusa, and Andrographis paniculata, in alcohol-induced HepG2 cells and alcohol plus a haloalkane, CCl4, induced liver damage in rats. BMC Complement. Altern. Med. 2020, 6428906–6428909. doi:10.1155/2020/6428906
Eming, S. A., Wynn, T. A., and Martin, P. (2017). Inflammation and metabolism in tissue repair and regeneration. Science 356, 1026–1030. doi:10.1126/science.aam7928
Fair, R. J., and Tor, Y. (2014). Antibiotics and bacterial resistance in the 21st century. Perspect. Med. Chem. 6, 25–64. doi:10.4137/PMC.S14459
Garcia-Lafuente, A., Guillamon, E., Villares, A., Rostagno, M. A., and Martinez, J. A. (2009). Flavonoids as anti-inflammatory agents: Implications in cancer and cardiovascular disease. Inflamm. Res. official J. Eur. Histamine Res. Soc. 58, 537–552. doi:10.1007/s00011-009-0037-3
Harsha, M., Mohan Kumar, K. P., Kagathur, S., and Amberkar, V. S. (2020). Effect of Ocimum sanctum extract on leukemic cell lines: A preliminary in-vitro study. J. Oral Maxillofac. Pathol. 24 (1), 93–98. doi:10.4103/jomfp.JOMFP_181_19
Hollman, P. C., and Katan, M. B. (1999). Health effects and bioavailability of dietary flavonols. Free Radic. Res. 31, S75–S80. doi:10.1080/10715769900301351
Horcajada-Molteni, M. N., Crespy, V., Coxam, V., Davicco, M. J., Remesy, C., and Barlet, J. P. (2000). Rutin inhibits ovariectomy-induced osteopenia in rats. J. Bone Min. Res. 15, 2251–2258. doi:10.1359/jbmr.2000.15.11.2251
Ilyas, U., Katare, D. P., Aeri, V., and Naseef, P. P. (2016). A review on hepatoprotective and immunomodulatory herbal plants. Pharmacogn. Rev. 10, 66–70. doi:10.4103/0973-7847.176544
Ilyas, U. K., Katare, D. P., and Aeri, V. (2015). Comparative evaluation of standardized alcoholic, hydroalcoholic, and aqueous extracts of Phyllanthus maderaspatensis Linn. against galactosamine-induced hepatopathy in albino rats. Pharmacogn. Mag. 11, 277–282. doi:10.4103/0973-1296.153079
Jain, A. N. U. R. A. G. (2015). To evaluate hepatoprotective activity of leaves of Ocimum sanctum using animal model. Asian J. Pharm. Clin. Res. 8 (4), 255–258.
Jorge, T. F., Mata, A. T., and António, C. (2016). Mass spectrometry as a quantitative tool in plant metabolomics. Philos. Trans. A Math. Phys. Eng. Sci. 28, 20150370. doi:10.1098/rsta.2015.0370
Kamyab, A. A., and Eshraghian, A. (2013b). Anti-inflammatory, gastrointestinal and hepatoprotective effects of Ocimum sanctum Linn: An ancient remedy with new application. Inflamm. Allergy Drug Targets 12, 378–384. doi:10.2174/1871528112666131125110017
Kamyab, A. A., and Eshraghian, A. (2013a). Anti-inflammatory, gastrointestinal and hepatoprotective effects of Ocimum sanctum Linn: An ancient remedy with new application. Inflamm. &allergy drug targets 12, 378–384. doi:10.2174/1871528112666131125110017
Lahon, K., and Das, S. (2011). Hepatoprotective activity of Ocimum sanctum alcoholic leaf extract against paracetamol-induced liver damage in Albino rats. Pharmacogn. Res. 3 (1), 13–18. doi:10.4103/0974-8490.79110
Li, S., Tan, H. Y., Wang, N., Cheung, F., Hong, M., and Feng, Y. (2018). The potential and action mechanism of polyphenols in the treatment of liver diseases. Oxid. Med. Cell Longev. 2018, 8394818. doi:10.1155/2018/8394818
Liu, C., Cai, Y., Wang, J., Liu, X., Ren, H., Yan, L., et al. (2020). Facile preparation of homogeneous copper nanoclusters exhibiting excellent tetraenzyme mimetic activities for colorimetric glutathione sensing and fluorimetric ascorbic acid sensing. ACS Appl. Mat. Interfaces. 23, 42521–42530. doi:10.1021/acsami.0c11983
Magrone, T., Candore, G., Caruso, C., Jirillo, E., and Covelli, V. (2008). Polyphenols from red wine modulate immune responsiveness: Biological and clinical significance. Curr. Pharm. Des. 14, 2733–2748. doi:10.2174/138161208786264098
Mandal, S., Mandal, M. D., and Pal, N. K. (2012). Enhancing chloramphenicol and trimethoprim in vitro activity by Ocimum sanctum Linn. (Lamiaceae) leaf extract against Salmonella enterica serovar Typhi. Asian pac. J. Trop. Med. 5, 220–224. doi:10.1016/S1995-7645(12)60028-5
Mataram, M. B. A., Hening, P., Harjanti, F. N., Karnati, S., Wasityastuti, W., Nugrahaningsih, D. A. A., et al. (2021). The neuroprotective effect of ethanolic extract Ocimum sanctum Linn. in the regulation of neuronal density in hippocampus areas as a central autobiography memory on the rat model of Alzheimer's disease. J. Chem.Neuroanat. 111, 101885–102111. doi:10.1016/j.jchemneu.2020.101885
Mohan, A., Narayanan, S., Sethuraman, S., and Krishnan, U. M. (2013). Combinations of plant polyphenols & anti-cancer molecules: A novel treatment strategy for cancer chemotherapy. Anti-cancer agents Med. Chem. 13, 281–295. doi:10.2174/1871520611313020015
Mota, K. S., Dias, G. E., Pinto, M. E., Luiz-Ferreira, A., Souza-Brito, A. R., Hiruma-Lima, C. A., et al. (2009). Flavonoids with gastroprotective activity. Mol. (Basel, Switz. 14, 979–1012. doi:10.3390/molecules14030979
Nagalekshmi, R., Menon, A., Chandrasekharan, D. K., and Nair, C. K. (2011). Hepatoprotective activity of Andrographis paniculata and Swertia chirayita. Food Chem. Toxicol. 49 (12), 3367–3373. doi:10.1016/j.fct.2011.09.026
Pandey, M. M., Rastogi., S., and Rawat, A. K. S. (2013). Indian traditional Ayurvedic system of medicine and nutritional supplementation. Evid.based Complement. Altern. Med. 12, 1–12. doi:10.1155/2013/376327
Patlolla, A. K., Berry, A., and Tchounwou, P. B. (2011). Study of hepatotoxicity and oxidative stress in male Swiss-Webster mice exposed to functionalized multi-walled carbon nanotubes. Mol. Cell Biochem. 358 (1-2), 189–199. doi:10.1007/s11010-011-0934-y
Pedan, V., Stamm, E., Do, T., Holinger, M., and Reich, E. (2020). HPTLC fingerprint profile analysis of coffee polyphenols during different roast trials. J. Food Compos. 94, 103610. doi:10.1016/j.jfca.2020.103610
Prochazkova, D., Bousova, I., and Wilhelmova, N. (2011). Antioxidant and prooxidant properties of flavonoids. Fitoterapia 82, 513–523. doi:10.1016/j.fitote.2011.01.018
Raish, M., Ahmad, A., Alkharfy, K. M., Ahamad, S. R., Mohsin, K., Al-Jenoobi, F. I., et al. (2016). Hepatoprotective activity of Lepidium sativum seeds against D-galactosamine/lipopolysaccharide induced hepatotoxicity in animal model. BMC Complement. Altern. Med. 16 (1), 501. doi:10.1186/s12906-016-1483-4
Rajendran, C., Begam, M., Kumar, D., Baruah, I., Gogoi, H. K., Srivastava, R. B., et al. (2014). Antiplasmodial activity of certain medicinal plants against chloroquine resistant Plasmodium berghei infected white albino BALB/c mice. J.Parasit. Dis. 38, 148–152. doi:10.1007/s12639-013-0252-2
Rathi, A., Srivastava, A. K., Shirwaikar, A., Singh Rawat, A. K., and Mehrotra, S. (2008). Hepatoprotective potential of Fumaria indica Pugsley whole plant extracts, fractions and an isolated alkaloid protopine. Phytomedicine 15 (6-7), 470–477. doi:10.1016/j.phymed.2007.11.010
Rho, H. S., Ahn, S. M., Lee, B. C., Kim, M. K., Ghimeray, A. K., Jin, C. W., et al. (2010). Changes in flavonoid content and tyrosinase inhibitory activity in kenaf leaf extract after far-infrared treatment. Bioorg. Med. Chem. Lett. 20, 7534–7536. doi:10.1016/j.bmcl.2010.09.082
Saha, P., Talukdar, A. D., Nath, R., Sarker, S. D., Nahar, L., Sahu, J., et al. (2019). Role of natural phenolics in hepatoprotection: A mechanistic review and analysis of regulatory network of associated genes. Front. Pharmacol. 10, 509. doi:10.3389/fphar.2019.00509
Schaefer, T. J., and John, S. (2021). “Acute hepatitis,” in StatPearls treasure island (Petersburg, FL, United States: StatPearls Publishing), 400–410.
Shimizu, T., Torres, M. P., Chakraborty, S., Souchek, J. J., Rachagani, S., Kaur, S., et al. (2013). Holy Basil leaf extract decreases tumorigenicity and metastasis of aggressive human pancreatic cancer cells in vitro and in vivo: Potential role in therapy. Cancer Lett. 336, 270–280. doi:10.1016/j.canlet.2013.03.017
Shree, P., Mishra, P., Selvaraj, C., Singh, S. K., Chaube, R., Garg, N., et al. (2020). Targeting COVID-19 (SARS-CoV-2) main protease through active phytochemicals of ayurvedic medicinal plants -Withaniasomnifera(Ashwagandha),Tinospora cordifolia(Giloy) and Ocimum sanctum(Tulsi) - a molecular docking study. J. Biomol. Struct.Dyn. 27, 190–203. doi:10.1080/07391102.2020.1810778
Shukla, S. T., Kulkarni, V. H., Habbu, P. V., Jagadeesh, K. S., Patil, B. S., and Smita, D. M. (2012). Hepatoprotective and antioxidant activities of crude fractions of endophytic fungi of Ocimum sanctum Linn. in rats. Orient Pharm. Exp. Med. 12 (2), 81–91. doi:10.1007/s13596-012-0061-7
Singh, V., Kahol, A., Singh, I. P., Saraf, I., and Shri, R. (2016). Evaluation of anti-amnesic effect of extracts of selected Ocimum species using in-vitro and in-vivo models. J. Ethnopharmacol. 193, 490–499. doi:10.1016/j.jep.2016.10.026
Spencer, J. P. (2009). The impact of flavonoids on memory: Physiological and molecular considerations. Chem. Soc. Rev. 38, 1152–1161. doi:10.1039/b800422f
Suanarunsawat, T., Ayutthaya, W. D., Songsak, T., Thirawarapan, S., and Poungshompoo, S. (2011). Lipid-lowering and antioxidative activities of aqueous extracts of Ocimum sanctum L. leaves in rats fed with a high-cholesterol diet. Oxid. Med. Cell.longev. 12, 962025–962965. doi:10.1155/2011/962025
Van Herck, H., Baumans, V., Brandt, C. J., Boere, H. A., Hesp, A. P., van Lith, H. A., et al. (2001). Blood sampling from the retro-orbital plexus, the saphenous vein and the tail vein in rats: Comparative effects on selected behavioural and blood variables. Lab. Anim. 35 (2), 131–139. doi:10.1258/0023677011911499
Weydert, C. J., and Cullen, J. J. (2010). Measurement of superoxide dismutase, catalase and glutathione peroxidase in cultured cells and tissue. Nat. Protoc. 5 (1), 51–66. doi:10.1038/nprot.2009.197
Yu, C. P., Wu, P. P., Hou, Y. C., Lin, S. P., Tsai, S. Y., Chen, C. T., et al. (2011). Quercetin and rutin reduced the bioavailability of cyclosporine from Neoral, an immunosuppressant, through activating P-glycoprotein and CYP 3A4. J. Agric. Food Chem. 59, 4644–4648. doi:10.1021/jf104786t
Keywords: Ocimum sanctum Linn, galactosamine-induced rat model, hepatoprotective activity, polyphenols, serum biomarkers
Citation: Kamel FO, Karim S, Bafail DAO, Aldawsari HM, Kotta S and Ilyas UK (2023) Hepatoprotective effects of bioactive compounds from traditional herb Tulsi (Ocimum sanctum Linn) against galactosamine-induced hepatotoxicity in rats. Front. Pharmacol. 14:1213052. doi: 10.3389/fphar.2023.1213052
Received: 27 April 2023; Accepted: 30 August 2023;
Published: 04 October 2023.
Edited by:
Patrícia Mendonça Rijo, Lusofona University, PortugalReviewed by:
Irem I. Tatli, Hacettepe University, TürkiyeMohamed L. Ashour, Ain Shams University, Egypt
Copyright © 2023 Kamel, Karim, Bafail, Aldawsari, Kotta and Ilyas. This is an open-access article distributed under the terms of the Creative Commons Attribution License (CC BY). The use, distribution or reproduction in other forums is permitted, provided the original author(s) and the copyright owner(s) are credited and that the original publication in this journal is cited, in accordance with accepted academic practice. No use, distribution or reproduction is permitted which does not comply with these terms.
*Correspondence: Sabna Kotta, skotta@kau.edu.sa; U. K. Ilyas, dr.ilyasmcp@gmail.com