- Key Laboratory of Shaanxi Administration of Traditional Chinese Medicine for TCM Compatibility, State Key Laboratory of Research & Development of Characteristic Qin Medicine Resources (Cultivation), Shaanxi Key Laboratory of Chinese Medicine Fundamentals and New Drugs Research, Shaanxi University of Chinese Medicine, Xi’an, China
Sepsis is described as a dysregulation of the immune response to infection, which leads to life-threatening organ dysfunction. The interaction between intestinal microbiota and sepsis can’t be ignored. Furthermore, the intestinal microbiota may regulate the progress of sepsis and attenuate organ damage. Thus, maintaining or restoring microbiota may be a new way to treat sepsis. Traditional Chinese medicine (TCM) assumes a significant part in the treatment of sepsis through multi-component, multi-pathway, and multi-targeting abilities. Moreover, TCM can prevent the progress of sepsis and improve the prognosis of patients with sepsis by improving the imbalance of intestinal microbiota, improving immunity and reducing the damage to the intestinal barrier. This paper expounds the interaction between intestinal microbiota and sepsis, then reviews the current research on the treatment of sepsis with TCM, to provide a theoretical basis for its clinical application.
1 Introduction
Worldwide, sepsis continues to pose a serious public health concern. According to a third international consensus, sepsis is a systemic inflammatory response caused by infection, which leads to life-threatening organ dysfunction (Singer et al., 2016). Sepsis, which may result in septic shock and multiple organ dysfunction syndrome (MODS), kills over 5.3 million people worldwide per year (Dellinger et al., 2013; Fleischmann et al., 2016). Consequently, sepsis has been designated as one of the global health priorities by the World Health Organization (Fay et al., 2019). Recent studies reported that COVID-19 infection may cause life-threatening sepsis (Liu, et al., 2020b; Wiersinga et al., 2020). Among the 218,184 patients hospitalized for COVID-19, the overall pooled sepsis prevalence was estimated at 51.6% (Karakike et al., 2021). Sepsis, as a major global health issue, needs intensive research, which is also important for improving the clinical care of patients with COVID-19.
The intestinal microbiota is known as the body’s “second genome” and “forgotten organ”, which plays a crucial role relevant to human health and disease (Zhou et al., 2021). The human gut is a complex ecosystem, with the intestinal microbiota of a healthy individual consisting of approximately 400–500 species of bacteria (Zakerska-Banaszak et al., 2021), and they vary across individuals. These intestinal microbiotas mainly have a place with four significant phyla, namely Firmicutes, Bacteroides, Actinobacteria, and Proteobacteria, account for 90% of the intestinal microbiota (Rinninella et al., 2019; Álvarez-Mercado et al., 2019). When an infection occurs, our natural microbiota is our first line of protection. The pathogenesis of sepsis is complex, which is closely linked to a dysregulated inflammatory response and immune dysfunction, and the intestinal microbiota participants in this important process. The intestinal system is protected from colonization by exogenous microbiota and possibly harmful resident microbiota by the intestinal epithelial barrier, immune system, and intestinal microbiota (Kamada et al., 2013). Furthermore, previous examinations have indicated that the intestinal microbiota can influence damage reactions in organs outside of the gastrointestinal tract (Gong et al., 2019). This shows that intestinal microbiota has an important role in the occurrence and development of sepsis, which may represent a new frontier in sepsis treatment.
Sepsis has always been a focus of interest for researchers because of its extremely high incidence and mortality rates and the lack of ideal treatment in clinical medicine. The current western medicine treatment for sepsis is mainly antibiotics and supportive care (Varkouhi et al., 2020). One of the most frequently used medications in the treatment of sepsis in clinical applications is antibiotics. It can alter the intestinal microbiota significantly and negatively impact sepsis outcomes (Dethlefsen et al., 2008; Adelman et al., 2020). For example, one study showed that the use of antibiotics will affect the intestinal microbiota, leading to an imbalance in the intestinal microbiota that can persist for up to 6 months (Faura et al., 2021). According to the clinical manifestations of sepsis, it belongs to the traditional Chinese medicine (TCM) “febrile diseases” category (Lin S. J. et al., 2013). TCM treatment for sepsis mainly includes the methods of clearing heat and detoxifying toxins (Qingrejiedu), promoting blood circulation and removing blood stasis (Huoxuehuayu), and promoting the recovery of physiological function (Fuzhengguben). TCM, as a strong supplement to antibiotic drugs, has been included in the normal treatment of sepsis (Liu L. et al., 2020), such as XueBiJing injection (XBJ), ShenFu injection, ShengMai formula, Xuanbai Chengqi decoction (XBCQ), and Qingwen Baidu decoction (QWBD), etc (Table 1). TCM has been proved to be effective in the treatment of sepsis and has advantages in improving intestinal microbiota disorders and maintaining intestinal homeostasis. In this review, we discussed the intestinal microbiota and sepsis relationship and the TCM in modulating intestinal microbiota for the therapy of sepsis.
2 The relationship between sepsis and intestinal microbiota
2.1 Intestinal microbiota disruption predisposes to sepsis
Two epidemiological studies have provided indirect evidence that disruption of the intestinal microbiota predisposes to sepsis. The first study, which included more than 10,000 Medicare recipients, found that patients with Clostridium difficile infection (CDI) were more likely to be admitted with severe sepsis than without CDI patients (Prescott et al., 2015). The second study, which looked at more than 12 million patients, found that those who were exposed to antibiotics on admission were more likely to be readmitted within 90 days after recovery, compared with those who were not exposed to antibiotics (Baggs et al., 2018). Furthermore, the decreased diversity of the intestinal microbiota also has an impact on sepsis in animal models. Cecal ligation and puncture (CLP) was performed on two groups of mice with the same genetic background to create sepsis models. When the microbiota of the two groups were significantly different, mice with greater alpha diversity had a better chance of survival than mice with less alpha diversity. When the two groups of mice were co-housed, their microbiota was similar, leading to increased mice survival that were formerly more probably to die (Fay et al., 2019). Similar sepsis experiments have demonstrated that a decrease in microbiota diversity can have an impact on animal mortality (Chen et al., 2018b). These findings show that damage to the intestinal microbiota is strongly associated with and subsequent progression of sepsis, and further emphasize the importance of the intestinal microbiota in sepsis. Based on current research, it is unclear how intestinal microbiota disruption prior to sepsis onset affects sepsis outcomes, but at least it can be determined that intestinal microbiota is one of the factors regulating systemic sepsis response; more research is needed to explore this mechanism in the future.
2.2 Sepsis worsens intestinal microbiota disruption
Some studies suggest the intestinal microbiota composition and function in sepsis patients is markedly disrupted compared to healthy individuals by employing multi-omics analysis (Liu et al., 2019; Yang X. et al., 2021). The disturbed intestinal microbiota lost the function of protecting the host, which may further lead to a serious response to sepsis and affect the results of sepsis treatment (Niu and Chen., 2021). When sepsis occurs, specific obligate anaerobes (e.g., Bacteroidetes, Firmicutes), which are usually predominant in healthy individuals, are frequently lost, and taxa with usually low levels of Proteobacteria (e.g., Escherichia coli, Klebsiella pneumoniae) “flowering” (Miller et al., 2021). Compared with healthy volunteers, the total number of anaerobic bacteria (including Bifidobacterium and Lactobacillus) has markedly decreased in systemic inflammatory response syndrome (SIRS) patients (Shimizu et al., 2011). One study found a significant decrease in intestinal microbiota diversity in sepsis patients, including a significant increase in abundance of inflammatory related microbes and pathogenic species (e.g. Enterococcus spp.). It also found a positive correlation between the abundance of Enterococcus species (OTU46) and sepsis mortality (Agudelo-Ochoa et al., 2020). In a study that included 12 intensive care unit (ICU) patients, the results were assessed using the ratio of Bacteroides/Firmicutes (B/F ratio), which found extreme changes in the B/F ratio in five of the six patients who died, suggesting a significant imbalance in the intestinal microbiota was strongly associated with patient prognosis (Ojima et al., 2016). It is evident that sepsis will aggravate the disruption of the intestinal microbiota, which can negatively affect sepsis outcomes.
In addition, the intestinal micro-ecology contains the normal flora of the gut and its environment, but also the metabolites produced by the intestinal microbiota (Chen et al., 2019). The metabolic products of the intestinal microbiota act in multiple ways to influence human health and disease (Connors et al., 2019). These metabolic products, such as short-chain fatty acids (SCFAs), are thought to be beneficial to their hosts (Anand et al., 2016). Compared to healthy individuals, sepsis patients showed a significant decrease in fecal SCFAS, with the change lasting 6 weeks (Yamada et al., 2015). Maintaining SCFAs concentrations also has a non-negligible effect on disease recovery due to its ability to protect intestinal barrier integrity and anti-inflammatory properties.
2.3 Intestinal microbiota modulates sepsis progression
2.3.1 Relationship between intestinal microbiota and intestinal barrier
With trillions of bacteria living in the intestinal tract, the integrity of the intestinal barrier is critical to a healthy status. The intestinal epithelium represents a key protective barrier, keeping pathogens at bay while ensuring the entry of beneficial substances (e.g., nutrients, products of symbiotic bacteria) (Krawczyk et al., 2018; Singh et al., 2019). The composition of tight junction includes occludin, claudins, zonula occludens-1 (ZO-1), junctional adhesion molecules, and many other types of proteins that provide a crucial primary barrier for intestinal space (Yoseph et al., 2016). The onset of sepsis destroys the intestinal barrier, causing an imbalance in the intestinal microbiota and resulting in bacteria migration from the intestinals (Sun et al., 2020). During sepsis, increased inflammatory cytokines can cause impairment of the intestinal barrier. In the clinically relevant models of sepsis, increased levels of cytokines can regulate the protein expression of claudin 2, claudin 5, junctional adhesion molecule-A (JAM-A), occludin, and ZO-1, resulting in increased gut permeability (Yoseph et al., 2016). In addition, myosin light chain kinase (MLCK), one of the signaling proteins involved in regulating intestinal barrier integrity, is positively associated with tumor necrosis factor alpha (TNF-α), and TNF-α promotes phosphorylation of MLCK, leading to disruption of tight junction and inducing loss of the epithelial barrier (Andrews et al., 2018; Zhang et al., 2021). Secondly, changes in the intestinal microbiota can also act on the intestinal barrier, mainly by down regulating the tight junctions between intestinal cells, leading to enhanced gut permeability (Hu et al., 2017; Wei et al., 2020). During sepsis pathogenesis, “beneficial” anaerobic bacteria families, like Lachnospiraceae and Ruminococcaceae, are lost and their absence can further impair the function of intestinal epithelial cells (Haak et al., 2018) (Figure 1).
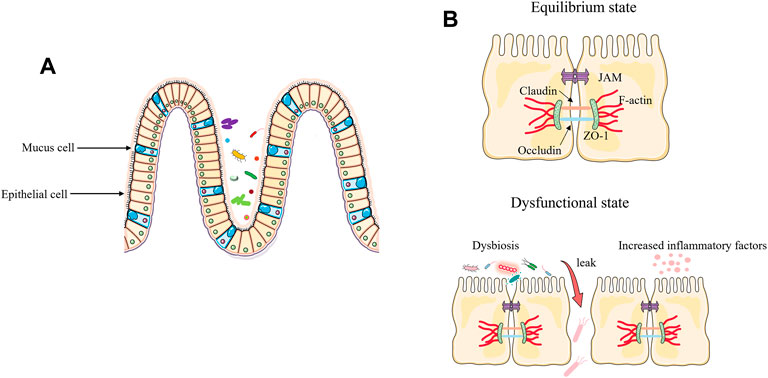
FIGURE 1. Disruption of the intestinal barrier and the function of the microbiota in sepsis. (A) Under healthy conditions, the intestinal epithelium consists of a single layer of intestinal epithelial cells (IECs), which is an important protective physical barrier that maintains intestinal homeostasis. (B) During sepsis, excessive release of inflammatory factors and loss of tight junction of epithelial cells leads to bacterial translocation and exacerbates the progression of sepsis.
2.3.2 Intestinal microbiota influences the immune response during sepsis
From an immunological point of view, sepsis is closely associated with immune response disorder, with key factors including a systemic inflammatory response and disordered immune regulation (Chen and Wei, 2021). Intestinal microbiota are involved in the metabolism of substances and regulate the development and homeostasis of the immune system (Yin et al., 2017), while the immune system can recognize commensal bacteria and respond appropriately to potentially harmful pathogens (Leal-Lopes et al., 2015). Intestinal microbiota plays a significant role in fighting systemic infections by inducing the production of protective immunoglobulin G (IgG) (Zeng et al., 2016), IgA (Macpherson et al., 2015), and antimicrobial protein (Gallo and Hooper, 2012). The intestinal microbiota serves as an important regulator for the host immune system (Shi et al., 2020). The findings of Fay et al. suggested that the microbiome plays an important role in sepsis survival and host immune response (Fay et al., 2019). Microbiota, as a source of peptidoglycan, systematically stimulates the innate immune system and enhances the ability of bone marrow-derived neutrophils to kill pathogens (Clarke et al., 2010). Neutrophils are a critical component of innate immunity, Zhang et al. found that the microbiota gradually leads them to become more functionally active (Zhang et al., 2015). The intestinal microbiota induces local immune responses, and intestinal symbiotic gram-negative bacteria induces IgG responses and confer protection against systemic (Zeng et al., 2016).
The metabolic by-products of intestinal microbiota are not only a vehicle for communication through which the intestinal microbiota communicates with the immune system but are also one of the factors in influencing the immune system (Arpaia et al., 2013). Intestinal microbiota-derived SCFAs, primary vehicles for host-microbiota interactions in the gut, induce metabolic and transcriptional changes in macrophages, thereby enhancing their bactericidal functions (Schulthess et al., 2019). In addition, SCFAs can increase macrophage bacterial clearance by upregulating LAMTOR2 (Wu et al., 2020).
2.3.3 Intestinal microbiota influence organ dysfunction during sepsis
Among sepsis-induced organ dysfunctions, including lung, liver, kidney, and brain injuries, are associated with microbiota disruption. Here, we use acute lung injury (ALI) as an example. The lung is one of the most vulnerable target organs in the pathogenesis of sepsis (Chen and Billiar, 2020). Acute respiratory distress syndrome (ARDS) is the most common organ dysfunction in COVID-19 (Karakike et al., 2021). Also, epidemiological reports indicate an upward trend in the incidence of ALI/ARDS due to sepsis is on the rise, with mortality rates of up to 70% (Zhao et al., 2016b; Chen H. et al., 2019). In a study, a mouse model of ALI caused by lipopolysaccharide (LPS) was established, and it was concluded that the pathological transformation of the ALI microbiota was caused by a group of congenital opportunistic pathogens that thrived in the inflamed lung environment, independent of external infectious factors (Poroyko et al., 2015). New research shows that the lung microbiota is rich in intestinal microbiota, both in animal models of septic ARDS and in patients with septic ARDS (Dickson et al., 2016). The above studies suggest that the intestinal microbiota is closely related to lung injury. Current studies have shown that typical intestinal microbiota (e.g., Bacteroidetes and Enterobacteriaceae) has been detected in the lungs of severe patients, suggesting that bacterial migration and subsequent exacerbation of the inflammatory response may be one of the potential pathogenic mechanisms of ARDS (Siwicka-Gieroba and Czarko-Wicha, 2020). Moreover, the intestinal microbiota can activate pulmonary oxidative stress and mediates lung damage by modulating the TLR4/NF-κB signal pathway (Tang et al., 2021).
In addition, the intestinal microbiota can also have an impact on the lung microbiota. Although traditionally considered sterile, the lung has been shown to contain a complex microbial community known as the lung microbiota, according to recently published investigations (Seixas et al., 2021). Comparing the microbiota in bronchoalveolar lavage (BAL) of healthy volunteers and patients with lung disease, major differences were found in the structure of the bacterial (Dickson et al., 2014). A recent clinical study has suggested that ARDS caused by sepsis is linked to a significant dysbiosis in the patient’s lung microbiota (Schmitt et al., 2020). The intestinal associated Bacteroides OTU, one of the most abundant components of intestinal microbiota, was found in the lungs of ARDS patients, but not in healthy controls (Dickson et al., 2020). On the contrary, changes in the lung microbiota can also influence the intestinal microbiota (He et al., 2017). LPS-induced ALI causes lung bacteria to metastasize into the blood and disrupts the bacteria composition of the cecum, causing a dramatic increase in bacterial numbers (Sze et al., 2014) (Figure 2).
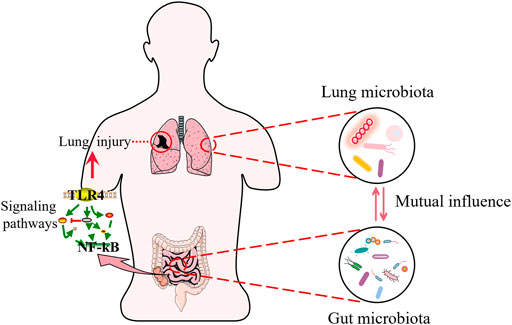
FIGURE 2. Sepsis and lung injury. Intestinal microbiota interact with lung microbiota during sepsis. The gut microbiota regulates signaling pathways and participates in sepsis induced lung injury.
3 Traditional Chinese Medicine and sepsis
3.1 Role of TCM in the treatment of sepsis
The pathogenesis of sepsis is complex and includes multiple aspects such as over-activation of the inflammatory response, immune dysfunction, disorder of blood coagulation and tissue damage. From the perspective of Western medicine, sepsis is a systemic inflammatory response caused by infection (Singer et al., 2016), and antibiotics are one of the most commonly used drugs. In a survey on drug-induced liver injury (DILI) related adverse drug reaction (ADR) (L-ADR) in China, it was found that in all L-ADR reports, TCM accounted for 4.5%, conventional drugs (including chemical and biological drugs) accounted for 95.5%, and antibiotics (including antitubercular agents) accounted for the highest proportion (Wang J. et al., 2022). However, TCM has a unique advantage in this area. A systematic review summarized TCM with potential anti-DILI effects, such as glycyrrhetinic acid, baicalin, and ginsenoside Rg1 (Sun et al., 2022), which also play a certain role in the treatment of sepsis. From the perspective of TCM, it is generally accepted that sepsis belongs to the TCM “febrile diseases” category, mainly based on the main theories such as “shanghanlun” and “wenrelun” (Lin S. J. et al., 2013). Several meta-analyses of clinical studies have shown that herbal medicine has a non-negligible role in the treatment of sepsis (Liang et al., 2015; Wen et al., 2021). Therefore, we summarized the TCM commonly used in sepsis treatment, mainly from the following five types of introduction: 1) Chinese patent medicine, such as Xuebijing injection, Shenfu injection, and Re-Du-Ning injection; 2) Traditional Chinese Medicine compound, such as Bai-Hu-Tang, Dachengqi decoction, and Huanglian Jiedu Decoction; 3) Couplet medicines, such as Coptidis Rhizoma - Euodiae Fructus drug pair; 4) Single herbal medicines, such as borneol, cordyceps sinensis, and Rhizoma Coptidis; 5) Single ingredients, such as Tanshinone IIA, emodin, crocin.
3.1.1 Chinese patent medicine
Xuebijing injection (XBJ) was approved by the CFDA (China Food and Drug Administration) in 2004 for the treatment of sepsis and MODS (Chen et al., 2018a). In April 2020, XBJ was approved by the NMPA (National Medical Products Administration) for use as a treatment for COVID-19. XBJ plays an anti-sepsis protective role by reducing inflammatory factor release and modulating the differentiation of immune cells (Tregs and Th17) (Chen X. et al., 2018). XBJ inhibits the release of pro-inflammatory factors, promotes the production of anti-inflammatory factors, and modulates the GSK-3β pathway to alleviate CLP induced septic liver injury (Cao L. et al., 2021). Furthermore, antibiotic resistance is a pressing issue to be solved in the current treatment of sepsis, such as methicillin-resistant Staphylococcus aureus (MRSA), which is resistant to multiple antibiotics. TCM and its active ingredients have the potential to block antibiotic-resistant bacteria and provide a new treatment idea to reverse antibiotic resistance (Su et al., 2020). In a mouse model of MRSA-induced sepsis, XBJ plays a role in the treatment of drug-resistant bacterial infections by regulating the inflammatory response and inhibiting the Pam3CSK4 signal pathway (Li T. et al., 2020). This provides a preliminary basis for TCM to solve antibiotic resistance. At present, most studies have focused on clarifying the potential material basis and mechanism of XBJ in the treatment of sepsis. The study found that the main components of XBJ in the treatment of sepsis-induced cardiac dysfunction are paeoniflorin and hydroxysafflor yellow A, and its potential target may be related to NF-κB, TNF-α, and CXCL2 (Wang et al., 2021). Three components of Safflower have protective effects on LPS-induced ALI, and the potential mechanism may be related to inhibiting Raf/MEK/ERK pathway and the formation of neutrophil external traps (NET) (Wang Y. P. et al., 2020). In addition, the components of Ligusticum Chuanxiong hort may protect LPS-induced ALI by reducing NET formation (Zha et al., 2021). Similarly, Re-Du-Ning injection (RDN) plays the same role through this pathway and inhibits the MAPK pathway (Yang C. et al., 2021). RDN includes three herbal medicines, namely Artemisia annua, Honeysuckle and Gardenia gardenia (Wang Z. Y. et al., 2022). Shenfu injection may reduce LPS-induced myocardial apoptosis by inhibiting inflammatory response and MEK/ERK pathway activation (Chen et al., 2020b).
3.1.2 Traditional Chinese Medicine compound
Traditional Chinese medicine compound plays an important role in TCM. It attaches importance to the compatibility of drugs and has been proved by most clinical practice in sepsis treatment. Bai-Hu-Tang, composed of Gypsum Fibrosum (Shigao), Anemarrhena asphodeloides Bunge (Zhimu), Glycyrrhiza glabra L. (Gancao), and Nonglutinous rice (Gengmi, polished seed of Oryza sativa L. in Gramineae), plays a certain role in the treatment of sepsis, improves the survival rate and reduces the level of inflammatory factors (Lin C. J. et al., 2013); at the same time, it also has a protective effect on the immune inflammatory damage caused by LPS (Zhang et al., 2013). Dachengqi decoction (DCQD) can relieve sepsis related ALI, regulate capillary permeability, reduce pulmonary edema, and inhibit inflammatory response, which is strongly associated with the TLR4/NF-κB signaling pathway (Hu et al., 2019). DCQD, which is composed of Rheum palmatum L. (Dahuang), Citrus aurantium L. (Zhishi), Magnolia officinalis Rehder and E.H.Wilson (Houpo), and Natrii sulfas (Mangxiao), have been confirmed to regulate gastrointestinal motility in MODS rats, possibly by repairing and protecting the enteric nervous system (Xie et al., 2015). Huanglian Jiedu Decoction (HLJDD) has a protective effect on septic rats. On the one hand, it can relieve excessive inflammatory response, and on the other hand, it can regulate immune response (Wei et al., 2013). Furthermore, HLJDD has a protective effect on sepsis related acute kidney injury (AKI), reduces oxidative stress and improves energy metabolism disorders in septic mice, which may be related to the activation of Akt/HO-1 signaling pathway (Li et al., 2017). Qingwen Baidu Decoction (QBD) has a remarkable anti-inflammatory effect and can be used to treat sepsis (Yu et al., 2014). In addition, QBD can reduce the degree of pulmonary edema in rats and prevent ALI caused by sepsis (Zhang et al., 2017). Dahuang Fuzi Decoction, which is composed of Rheum palmatum L. (Dahuang), Aconitum carmichaeli Debeaux (Fuzi), and Asarum heterotropoides F.Schmidt (Xixin), can improve the survival rate of sepsis rats and reduce the inflammatory response and intestinal barrier injury (Liu F. et al., 2020).
3.1.3 Couplet medicines
Couplet medicines are the most basic and simplest form of compatibility of TCM, which is the bridge between a single herb and traditional Chinese medicine compound (Wang et al., 2012). As the smallest unit of TCM compatibility, the couplet medicine is composed of two relatively fixed herbs. Coptidis Rhizoma (Huanglian, CR) and Euodiae Fructus (Wuzhuyu, EF) is a well-known couplet medicines. Current research shows that, when the compatibility ratio of CR and EF is 6:1, it can inhibit the inflammatory response and reverse the lung injury caused by sepsis, possibly through the JAK1-STAT3 signaling pathway, and 7 components can be detected in rat serum, respectively: berberine, palmatine, jatrorrhizine, coptisine, evodin, chlorogenic acid, evodiamine (Yin et al., 2021).
3.1.4 Single herbal medicines
Cordyceps sinensis has a protective effect on LPS induced intestinal injury, which can promote cell proliferation and restore intestinal barrier function (Gu et al., 2015). Rhizoma Coptidis protects against sepsis related AKI by acting on HO-1, NOS2 and PPAR α and other 17 target proteins (Zheng et al., 2021). Lonicera japonica Thunb. can improve immune dysfunction caused by sepsis through inhibiting immune cell apoptosis (Kim et al., 2015). Borneol is protective against sepsis-related brain injury, reducing neuroinflammation in the brain and protecting neurons and microglia, with potential mechanisms closely related to the NF-κB and MAPK pathways (Wang et al., 2019). Panax notoginseng reduces the inflammatory response of CLP-induced sepsis by inhibiting the NF-κB signaling pathway (Shou et al., 2022). North American ginseng improves LPS-induced cardiac dysfunction in mice with endotoxemia by inhibiting the NOX2-ERK1/2-TNF-α signaling pathway, suggesting its potential for preventing sepsis (Wu et al., 2016). Most of these herbal medicines have anti-inflammatory and immunomodulatory properties, so they are used to study the treatment of sepsis.
3.1.5 Single ingredients
Pristimerin can ameliorate neuronal injury in septic brain injury mice, mainly by regulating PI3K/Akt signaling pathway (Xue et al., 2021). Tanshinone IIA has a neuroprotective effect on sepsis mice, and in addition to its anti-inflammatory effects, its mechanism may be related to inhibiting the activation of astrocytes and microglia (Xiong et al., 2019). Cinnamyl alcohol protected septic mice by inhibiting the NLRP3 inflammatory pathway (Zou et al., 2022). Similarly, emodin attenuates LPS-induced myocardial injury through this pathway (Dai et al., 2021). Glycyrrhizic acid can prevent septic AKI and septic ALI, mainly by inhibiting inflammatory response, reducing oxidative stress damage and reducing cell apoptosis (Zhao et al., 2016a; Zhao et al., 2016b). In addition, glycyrrhizin alleviates ARDS caused by sepsis by inhibiting the HMGB1/TLR9 pathway (Gu et al., 2022; Zhao et al., 2017). Crocin is considered as a potential drug for the treatment of sepsis, because it shows significant anti-inflammatory and anti-apoptotic effects, primarily associated with MAPK/NF-κB and Bax/Bcl-2 signaling pathways (Gao et al., 2022). Studies have shown that ginsenoside Rg3 can alleviate cell and organ damage caused by sepsis, mainly acting on the AMPK signal pathway and alleviating mitochondrial dysfunction caused by sepsis (Xing et al., 2017).
These studies show that TCM has a certain role in the treatment of sepsis. These drugs have many effects in the treatment of sepsis, such as anti-inflammatory, improve gastrointestinal dysfunction, enhance immune function, protect organs and so on (Figure 3).
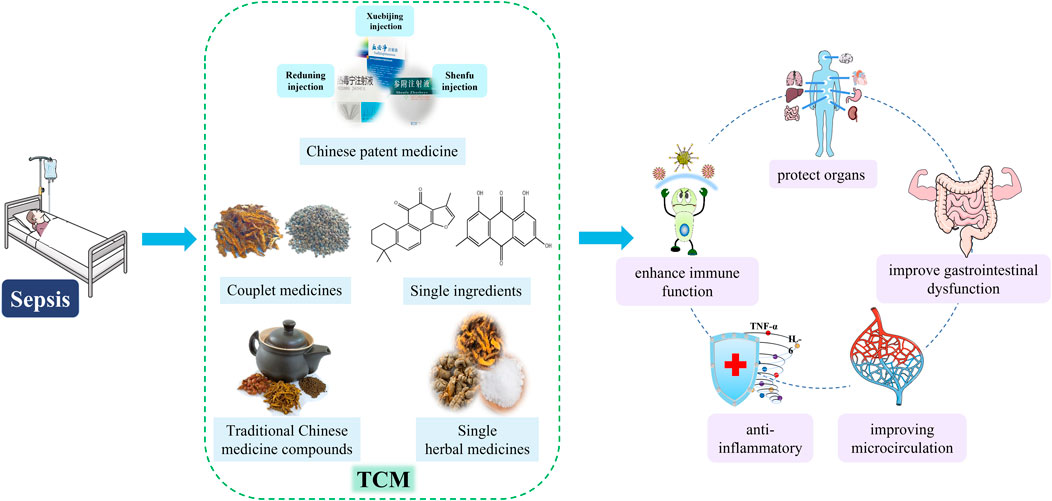
FIGURE 3. Therapeutic effects of TCM on sepsis. TCM, includes Chinese patent medicine, traditional Chinese Medicine compound, couplet medicines, single herbal medicines and single ingredients, treats sepsis in a variety of ways, such as anti-inflammatory, improving gastrointestinal disorders, enhancing immune function, improving microcirculation, and protecting organs.
3.2 Mechanisms of TCM alleviating sepsis via the intestinal microbiota
Antibiotics are essential for the treatment of sepsis (Cao et al., 2019). It is known to fight pathogens, but can also inhibit symbiotic bacteria, disrupt intestinal microbiota and affect host health (Maier et al., 2021). A randomised controlled study showed that antibiotic treatment significantly reduced intestinal microbiota diversity in all subjects (Lankelma et al., 2017). Similar findings were also found in sepsis animal models. The use of antibiotics disrupts the intestinal microbiota, leading to an increase in pathogenic bacteria (Han et al., 2021). In addition, an observational study showed that after antibiotic use in patients with sepsis, the intestinal microbiota was dominated by Klebsiella or Enterococcus, which may further lead to secondary infections (Mu et al., 2022). Taken together, antibiotics affect the intestinal microbiota and may have implications for subsequent treatment, and TCM has unique advantages in this regard. In recent years, researchers have combined intestinal microbiota with TCM, with a view to investigating the mechanisms of TCM in the preventing of sepsis. The interaction between TCM and intestinal microbiota, both direct and indirect, can cause structural changes in the intestinal microbiota, as well as changes in metabolites of the intestinal microbiota, which have an impact on the development and prognosis of sepsis. TCM is generally administered orally, and they can be in direct contact with the intestinal microbiota and therefore affecting the intestinal microbiota composition. These direct effects include promotion, inhibition, and elimination. Flavonoids, polysaccharides and saponins in TCM can act as prebiotics and facilitate growth of certain intestinal microbiota (Feng et al., 2019). In addition, some TCM ingredients could inhibit or kill microorganisms. The indirect impact of TCM on the intestinal microbiota involves adjusting intestinal barrier function and regulation of immune homeostasis (Figure 4). Therefore, we summarize the treatment of sepsis with TCM from the following three aspects.
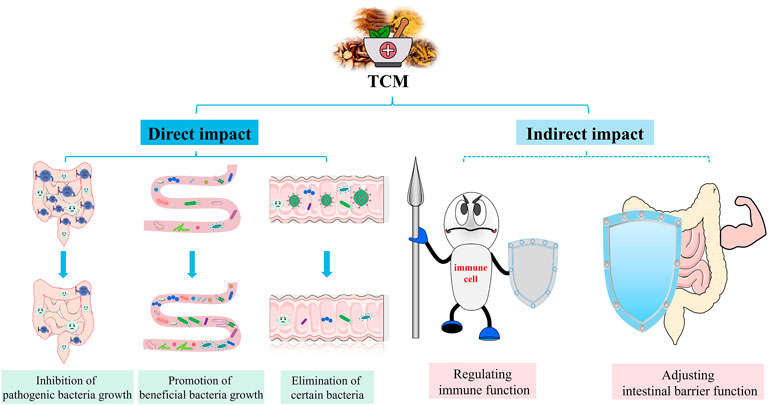
FIGURE 4. Effects of TCM on intestinal microbiota. TCM can directly and indirectly regulate the intestinal microbiota to relieve sepsis. Direct effects include the promotion, suppression and elimination of specific groups of bacteria. Indirect effects include regulation of intestinal barrier function and immune homeostasis.
3.2.1 Regulation of intestinal microbiota structure
TCM can play a role in disease prevention by regulating the dysfunctional intestinal microbiota structure under disease conditions. Sini decoction (SND), consisting of Aconitum carmichaeli Debeaux, Zingiber officinale Roscoe, and Glycyrrhiza glabra L., has been ameliorate sepsis symptoms via modulating the microbiota in the intestinal tract. At the genus level, SND can also promote the consumption of harmful bacteria (Ruminococcaceae, Lachnoclostridium, Faecalibaculum and Oscillibacter) in the faeces of pathological mice induced by CLP (Wang W. et al., 2020). The number of Bacteroidaceae, ClostridiumXI and Parabacteroides in the sepsis group was restored to normal after treatment with Xuanbai Chengqi Decoction (XBCQ) (Mu et al., 2021). A study showed that the intestinal microbiota of septic rats treated with probiotics and QingRe JieDu Decoction (QRD) had the same diversity and composition, suggesting that QRD reduced mortality in septic animals without damaging the characteristics of the intestinal microbiota (Cao H. et al., 2021). Sinomenine, an isoquinoline alkaloid, derived from Sinomenium acutum (Thunb.) Rehder and E.H.Wilson. One study found that sinomenine augmented the number of beneficial bacteria (such as Prevotellaceae UCG-001) and lessened the number of noxious bacteria (such as Escherichia-Shigella) to regulate gut homeostasis (Song et al., 2021). Treatment with emodin can restore the reduced diversity of intestinal microbiota caused by sepsis, as well as inhibit the growth of harmful bacteria that contribute to the development of sepsis, such as facultative anaerobes and Proteobacteria, and restore the number of beneficial bacteria, such as Firmicutes and Bacteroidetes (Zhang et al., 2022).
3.2.2 Regulation of intestinal barrier
The intestinal barrier function plays an important role in maintaining the homeostasis of the body. The intestinal barrier function can effectively prevent the intestinal bacteria and their toxins from migrating to the tissue and organs and protect the body from endogenous microorganisms and their toxins. In addition to regulating the structure of the intestinal microbiota, TCM can also indirectly influence bacterial migration through the protection of the intestinal barrier. Shenfu injection (SFI) had a protective effect on the intestinal mucosa in rats with sepsis (Jin et al., 2019), significantly improving the disruption of tight junctions between intestinal epithelial cells (Xing et al., 2015). Before sepsis, pre-treatment with Rheum palmatum L. can promote the intestinal mucosal capillaries dilatation, protect capillary endothelial cells, and improve intestinal microcirculation in sepsis (Cui et al., 2014), and ameliorates intestinal permeability with septic patients (Fang et al., 2007). Moreover, emodin enhances the expression of ZO-1 and occludin, improves intestinal barrier function, and prevents bacterial translocation again (Zhang et al., 2022). Intervention with Jinzhi reduced the inflammatory factors (i.e., TNF-α, IL-1β, and IL-6) released by LPS-induced intestinal mucosal barrier damage and increased the expression of occludin and claudin-1 (Xu et al., 2020). Berberine can reduce intestinal damage caused by LPS, through increasing the activating of SOD and GSH-Px and inhibiting TLR4 and NF-κB in the ileum (Zhang et al., 2011). Furthermore, berberine can also induce Zrt-Irt-like protein 14 expression to protect the intestinal barrier in sepsis (He et al., 2019), and ameliorates sepsis-induced reduction in intestinal vascular barrier permeability caused by sepsis by modulating the ApoM/S1P pathway (Li Y. et al., 2020). Berberine has also been shown to protect the intestinal mucosal barrier by mediating Toll-like receptors (Li et al., 2015). Sinomenine therapy effectually reduces CLP-induced colonic damage and depletion of tight junction proteins (Song et al., 2021). In conclusion, TCM maintains the intestinal barrier effect to resist pathogenic microbial attack and inhibits the migration of bacteria in sepsis.
3.2.3 Regulating immunity
Sepsis is closely related to disordered immune response. Therefore, during the treatment of sepsis, it is very significant to strengthen the body’s autoimmunity and adjust the immune balance. The immunomodulatory effect of TCM can’t be ignored, which is not only reflected in single herbal medicines or single ingredients, but also in traditional Chinese medicine compound. Numbers of white blood cells, neutrophils, and lymphocytes were decreased significantly in sepsis, which can be improved by HLJDD, indicating that HLJDD can improve bacteria-killing function and enhance immunity (Lv et al., 2017). Sepsis leads to a reduction in T helper (Th) cells, which treatment with Astragalus polysaccharides can improve and further stimulate a balanced Th1/Th2 response to alleviate immune suppression of sepsis (Hou et al., 2015). In LPS-induced macrophages, shionone (SHI) had an opposite effect on the expression of M1 and M2 polarization biomarkers, mainly by inhibiting M1 and promoting M2, possibly via the ECM1/STAT5/NF-κB pathway. Moreover, SHI could promote macrophage function through increasing the levels of GM-CSF (Song et al., 2022).
4 Conclusion and prospect
Sepsis is mainly treated with antibiotics and the following problems may exist in the use. First, antibiotic use may cause DILI, which further exacerbates sepsis-induced organ dysfunction. Second, the use of antibiotics can cause intestinal microbiota disturbance. The intestinal microbiota dysbiosis is a significant hidden risk factor of sepsis. Additionally, the intestinal microbiota is involved in the progress of sepsis and may also lead to organ failure. Therefore, enhancing the gut barrier function and improving the immune system by regulating the intestinal microbiota is expected to be one of the treatment options for sepsis. TCM has been proved to recover microbiota homeostasis, ameliorate intestinal barrier integrity, and modulate immune reactions. At present, from the point of view of intestinal microbiota, some progress has been made in the comprehension and application of TCM. Later, research should keep a watchful eye on the causal relationship among TCM, intestinal microbiota, metabolites and treatment results, and then better apply it to clinical practice. In addition, with the development of high-throughput-omics technologies, metabolomics has been an ideal tool for study of TCM-intestinal microbiota interactions. The integrated analysis of intestinal microbiota and metabolomics allows the identification, quantification and characterization of certain compounds produced by gut microbes, promising further understanding of the possible mechanisms of TCM for the therapy of disease. Finally, antibiotic resistance is an urgent problem to be solved. TCM has a potential role in this aspect and is expected to reverse antibiotic resistance, which also requires more in-depth research. At the same time, TCM also has certain limitations in terms of treatment, such as the lack of basic material research. Thus, a joint multidisciplinary effort will be required to elucidate the material basis of herbal medicine for sepsis. In addition, TCM has certain advantages in the treatment of sepsis and can regulate intestinal microbiota. Therefore, subsequent studies should combine intestinal microbiota with TCM in order to reveal the possible mechanism of TCM in treating diseases.
Author contributions
D-QX and Y-PT conceived and designed the review. X-HW searched the literature and drafted the manuscript. Y-YC and S-JY additions and revisions in manuscript. R-JF and LH edited the manuscript. D-QX and Y-PT made a critical revision of the review. All authors approved the final version of the manuscript.
Funding
This research was funded by National Natural Science Foundation of China (grant number 82204853), the Young Talent fund of University Association for Science and Technology in Shaanxi, China (20210312), Postgraduate Innovation and Practice ability promotion project of Shaanxi University of Chinese Medicine (ZG030), Subject Innovation Team of Shaanxi University of Chinese Medicine (2019-YL10), and the Youth Innovation Team of Shaanxi Universities (21JP036).
Conflict of interest
The authors declare that the research was conducted in the absence of any commercial or financial relationships that could be construed as a potential conflict of interest.
Publisher’s note
All claims expressed in this article are solely those of the authors and do not necessarily represent those of their affiliated organizations, or those of the publisher, the editors and the reviewers. Any product that may be evaluated in this article, or claim that may be made by its manufacturer, is not guaranteed or endorsed by the publisher.
Abbreviations
ADR, adverse drug reaction; ALI, acute lung injury; ARDS, acute respiratory distress syndrome; AKI, acute kidney injury; BAL, bronchoalveolar lavage; CDI, Clostridium difficile infection; CLP, cecal ligation and puncture; CFDA, China food and drug administration; DILI, drug-induced liver injury; GSH-Px, glutathione peroxidase; IgA, immunoglobulin A; IgG, immunoglobulin G; IL-10, interleukin-10; IL-1β, interleukin-1β; IL-6, interleukin-6; JAM-A, junctional adhesion molecule-A; LPS, lipopolysaccharide; MLCK, myosin light chain kinase; MODS, multiple organ dysfunction syndrome; NF-κB, nuclear factor kappa-B; NMPA, national medical products administration; SCFAs, short-chain fatty acids; SIRS, systemic inflammatory response syndrome; SOD, superoxide dismutase; TCM, traditional Chinese Medicine; Th, T helper; TLR4, Toll-like receptors 4; TNF-α, tumor necrosis factor alpha; ZO-1, zonula occludens-1.
References
Adelman, M. W., Woodworth, M. H., Langelier, C., Busch, L. M., Kempker, J. A., Kraft, C. S., et al. (2020). The gut microbiome’s role in the development, maintenance, and outcomes of sepsis. Crit. Care 24 (1), 278. doi:10.1186/s13054-020-02989-1
Agudelo-Ochoa, G. M., Valdés-Duque, B. E., Giraldo-Giraldo, N. A., Jaillier-Ramírez, A. M., Giraldo-Villa, A., Acevedo-Castaño, I., et al. (2020). Gut microbiota profiles in critically ill patients, potential biomarkers and risk variables for sepsis. Gut Microbes 12 (1), 1707610. doi:10.1080/19490976.2019.1707610
Álvarez-Mercado, A. I., Navarro-Oliveros, M., Robles-Sánchez, C., Plaza-Díaz, J., Sáez-Lara, M. J., Muñoz-Quezada, S., et al. (2019). Microbial population changes and their relationship with human health and disease. Microorganisms 7 (3), 68. doi:10.3390/microorganisms7030068
Anand, S., Kaur, H., and Mande, S. S. (2016). Comparative in silico analysis of butyrate production pathways in gut commensals and pathogens. Front. Microbiol. 7, 1945. doi:10.3389/fmicb.2016.01945
Andrews, C., Mclean, M. H., and Durum, S. K. (2018). Cytokine tuning of intestinal epithelial function. Front. Immunol. 9, 1270. doi:10.3389/fimmu.2018.01270
Arpaia, N., Campbell, C., Fan, X., Dikiy, S., van der Veeken, J., Deroos, P., et al. (2013). Metabolites produced by commensal bacteria promote peripheral regulatory T-cell generation. Nature 504 (7480), 451–455. doi:10.1038/nature12726
Baggs, J., Jernigan, J. A., Halpin, A. L., Epstein, L., Hatfield, K. M., and McDonald, L. C. (2018). Risk of subsequent sepsis within 90 Days after a hospital stay by type of antibiotic exposure. Clin. Infect. Dis. 66 (7), 1004–1012. doi:10.1093/cid/cix947
Cao, C., Yu, M., and Chai, Y. (2019). Pathological alteration and therapeutic implications of sepsis-induced immune cell apoptosis. Cell Death Dis. 10 (10), 782. doi:10.1038/s41419-019-2015-1
Cao, H., Zong, C., Dai, W., Gao, Q., Li, D., Wu, X., et al. (2021). The effects of Chinese medicine QRD, antibiotics, and probiotics on therapy and gut microbiota in septic rats. Front. Cell. Infect. Microbiol. 11, 712028. doi:10.3389/fcimb.2021.712028
Cao, L., Li, Z., Ren, Y., Wang, M., Yang, Z., Zhang, W., et al. (2021). Xuebijing protects against septic acute liver injury based on regulation of GSK-3β pathway. Front. Pharmacol. 12, 627716. doi:10.3389/fphar.2021.627716
Chen, C., Yin, Q., Wu, H., Cheng, L., Kwon, J. I., Jin, J., et al. (2019). Different effects of premature infant formula and breast milk on intestinal microecological development in premature infants. Front. Microbiol. 10, 3020. doi:10.3389/fmicb.2019.03020
Chen, G., Gao, Y., Jiang, Y., Yang, F., Li, S., Tan, D., et al. (2018a). Efficacy and safety of xuebijing injection combined with ulinastatin as adjunctive therapy on sepsis: A systematic review and meta-analysis. Front. Pharmacol. 9, 743. doi:10.3389/fphar.2018.00743
Chen, G., Huang, B., Fu, S., Li, B., Ran, X., He, D., et al. (2018b). G protein-coupled receptor 109A and host microbiota modulate intestinal epithelial integrity during sepsis. Front. Immunol. 9, 2079. doi:10.3389/fimmu.2018.02079
Chen, G., Xu, Y., Jing, J., Mackie, B., Zheng, X., Zhang, X., et al. (2017). The anti-sepsis activity of the components of huanglian Jiedu decoction with high lipid A-binding affinity. Int. Immunopharmacol. 46, 87–96. doi:10.1016/j.intimp.2017.02.025
Chen, H., Zhang, Y., Zhang, W., Liu, H., Sun, C., Zhang, B., et al. (2019). Inhibition of myeloid differentiation factor 2 by baicalein protects against acute lung injury. Phytomedicine 63, 152997. doi:10.1016/j.phymed.2019.152997
Chen, J., and Wei, H. (2021). Immune intervention in sepsis. Front. Pharmacol. 12, 718089. doi:10.3389/fphar.2021.718089
Chen, P., and Billiar, T. (2020). Gut microbiota and multiple organ dysfunction syndrome (MODS). Adv. Exp. Med. Biol. 1238, 195–202. doi:10.1007/978-981-15-2385-4_11
Chen, R. J., Rui, Q. L., Wang, Q., Tian, F., Wu, J., and Kong, X. Q. (2020b). Shenfu injection attenuates lipopolysaccharide-induced myocardial inflammation and apoptosis in rats. Chin. J. Nat. Med. 18 (3), 226–233. doi:10.1016/S1875-5364(20)30025-X
Chen, X., Feng, Y., Shen, X., Pan, G., Fan, G., Gao, X., et al. (2018). Anti-sepsis protection of Xuebijing injection is mediated by differential regulation of pro- and anti-inflammatory Th17 and T regulatory cells in a murine model of polymicrobial sepsis. J. Ethnopharmacol. 211, 358–365. doi:10.1016/j.jep.2017.10.001
Clarke, T. B., Davis, K. M., Lysenko, E. S., Zhou, A. Y., Yu, Y., and Weiser, J. N. (2010). Recognition of peptidoglycan from the microbiota by Nod1 enhances systemic innate immunity. Nat. Med. 16 (2), 228–231. doi:10.1038/nm.2087
Connors, J., Dawe, N., and Van Limbergen, J. (2019). The role of succinate in the regulation of intestinal inflammation. Nutrients 11 (1), 25. doi:10.3390/nu11010025
Cui, Y. L., Wang, L., Tian, Z. T., Lin, Z. F., and Chen, D. C. (2014). Effect of rhubarb pre-treatment on intestinal microcirculation in septic rats. Am. J. Chin. Med. 42 (5), 1215–1227. doi:10.1142/S0192415X14500761
Dai, S., Ye, B., Chen, L., Hong, G., Zhao, G., and Lu, Z. (2021). Emodin alleviates LPS-induced myocardial injury through inhibition of NLRP3 inflammasome activation. Phytother. Res. 35 (9), 5203–5213. doi:10.1002/ptr.7191
Dellinger, R. P., Levy, M. M., Rhodes, A., Annane, D., Gerlach, H., Opal, S. M., et al. (2013). Surviving sepsis campaign: International guidelines for management of severe sepsis and septic shock, 2012. Intensive Care Med. 39 (2), 165–228. doi:10.1007/s00134-012-2769-8
Dethlefsen, L., Huse, S., Sogin, M. L., and Relman, D. A. (2008). The pervasive effects of an antibiotic on the human gut microbiota, as revealed by deep 16S rRNA sequencing. PLoS Biol. 6 (11), e280. doi:10.1371/journal.pbio.0060280
Dickson, R. P., Erb-Downward, J. R., and Huffnagle, G. B. (2014). The role of the bacterial microbiome in lung disease. Expert Rev. Respir. Med. 7 (3), 245–257. doi:10.1586/ers.13.24
Dickson, R. P., Schultz, M. J., van der Poll, T., Schouten, L. R., Falkowski, N. R., Luth, J. E., et al. (2020). Lung microbiota predict clinical outcomes in critically ill patients. Am. J. Respir. Crit. Care Med. 201 (5), 555–563. doi:10.1164/rccm.201907-1487OC
Dickson, R. P., Singer, B. H., Newstead, M. W., Falkowski, N. R., Erb-Downward, J. R., Standiford, T. J., et al. (2016). Enrichment of the lung microbiome with gut bacteria in sepsis and the acute respiratory distress syndrome. Nat. Microbiol. 1 (10), 16113. doi:10.1038/nmicrobiol.2016.113
Fang, X. L., Fang, Q., Luo, J. J., and Zheng, X. (2007). Effects of crude rhubarb on intestinal permeability in septic patients. Am. J. Chin. Med. 35 (6), 929–936. doi:10.1142/S0192415X07005399
Faura, J., Bustamante, A., Miró-Mur, F., and Montaner, J. (2021). Stroke-induced immunosuppression: Implications for the prevention and prediction of post-stroke infections. J. Neuroinflammation 18 (1), 127. doi:10.1186/s12974-021-02177-0
Fay, K. T., Klingensmith, N. J., Chen, C. W., Zhang, W., Sun, Y., Morrow, K. N., et al. (2019). The gut microbiome alters immunophenotype and survival from sepsis. FASEB J. 33 (10), 11258–11269. doi:10.1096/fj.201802188R
Feng, W., Ao, H., Peng, C., and Yan, D. (2019). Gut microbiota, a new frontier to understand traditional Chinese medicines. Pharmacol. Res. 142, 176–191. doi:10.1016/j.phrs.2019.02.024
Fleischmann, C., Scherag, A., Adhikari, N. K. J., Hartog, C. S., Tsaganos, T., Schlattmann, P., et al. (2016). Assessment of global incidence and mortality of hospital-treated sepsis. Current estimates and limitations. Am. J. Respir. Crit. Care Med. 193 (3), 259–272. doi:10.1164/rccm.201504-0781OC
Gallo, R. L., and Hooper, L. V. (2012). Epithelial antimicrobial defence of the skin and intestine. Nat. Rev. Immunol. 12 (7), 503–516. doi:10.1038/nri3228
Gao, J., Zhao, F., Yi, S., Li, S., Zhu, A., Tang, Y., et al. (2022). Protective role of crocin against sepsis-induced injury in the liver, kidney and lungs via inhibition of p38 MAPK/NF-κB and Bax/Bcl-2 signalling pathways. Pharm. Biol. 60 (1), 543–552. doi:10.1080/13880209.2022.2042328
Gong, S., Yan, Z., Liu, Z., Niu, M., Fang, H., Li, N., et al. (2019). Intestinal microbiota mediates the susceptibility to polymicrobial sepsis-induced liver injury by granisetron generation in mice. Hepatology 69 (4), 1751–1767. doi:10.1002/hep.30361
Gu, G. S., Ren, J. A., Li, G. W., Yuan, Y. J., Li, N., and Li, J. S. (2015). Cordyceps sinensis preserves intestinal mucosal barrier and may be an adjunct therapy in endotoxin-induced sepsis rat model: A pilot study. Int. J. Clin. Exp. Med. 8 (5), 7333–7341.
Gu, J., Ran, X., Deng, J., Zhang, A., Peng, G., Du, J., et al. (2022). Glycyrrhizin alleviates sepsis-induced acute respiratory distress syndrome via suppressing of HMGB1/TLR9 pathways and neutrophils extracellular traps formation. Int. Immunopharmacol. 108, 108730. doi:10.1016/j.intimp.2022.108730
Haak, B. W., Prescott, H. C., and Wiersinga, W. J. (2018). Therapeutic potential of the gut microbiota in the prevention and treatment of sepsis. Front. Immunol. 9, 2042. doi:10.3389/fimmu.2018.02042
Han, C., Guo, N., Bu, Y., Peng, Y., Li, X., Ma, X., et al. (2021). Intestinal microbiota and antibiotic-associated acute gastrointestinal injury in sepsis mice. Aging (Albany NY) 13 (7), 10099–10111. doi:10.18632/aging.202768
He, Y., Wen, Q., Yao, F., Xu, D., Huang, Y., and Wang, J. (2017). Gut-lung Axis: The microbial contributions and clinical implications. Crit. Rev. Microbiol. 43 (1), 81–95. doi:10.1080/1040841X.2016.1176988
He, Y., Yuan, X., Zuo, H., Li, X., Sun, Y., and Feng, A. (2019). Berberine induces ZIP14 expression and modulates zinc redistribution to protect intestinal mucosal barrier during polymicrobial sepsis. Life Sci. 233, 116697. doi:10.1016/j.lfs.2019.116697
Hou, Y., Wu, J., Wang, M., Wu, M., Chen, K., Yeh, S., et al. (2015). Modulatory effects of Astragalus polysaccharides on T-cell polarization in mice with polymicrobial sepsis. Mediat. Inflamm. 2015, 826319. doi:10.1155/2015/826319
Hu, J., Luo, H., Wang, J., Tang, W., Lu, J., Wu, S., et al. (2017). Enteric dysbiosis-linked gut barrier disruption triggers early renal injury induced by chronic high salt feeding in mice. Exp. Mol. Med. 49 (8), e370. doi:10.1038/emm.2017.122
Hu, X., Liu, S., Zhu, J., and Ni, H. (2019). Dachengqi decoction alleviates acute lung injury and inhibits inflammatory cytokines production through TLR4/NF-κB signaling pathway in vivo and in vitro. J. Cell. Biochem. 120 (6), 8956–8964. doi:10.1002/jcb.27615
Jin, S., Jiang, R., Lei, S., Jin, L., Zhu, C., Feng, W., et al. (2019). Shenfu injection prolongs survival and protects the intestinal mucosa in rats with sepsis by modulating immune response. Turk. J. Gastroenterol. 30 (4), 364–371. doi:10.5152/tjg.2019.18418
Kamada, N., Seo, S., Chen, G. Y., and Núñez, G. (2013). Role of the gut microbiota in immunity and inflammatory disease. Nat. Rev. Immunol. 13 (5), 321–335. doi:10.1038/nri3430
Karakike, E., Giamarellos-Bourboulis, E. J., Kyprianou, M., Fleischmann-Struzek, C., Pletz, M. W., Netea, M. G., et al. (2021). Coronavirus disease 2019 as cause of viral sepsis: A systematic review and meta-analysis. Crit. Care Med. 49 (12), 2042–2057. doi:10.1097/CCM.0000000000005195
Kim, S. J., Kim, J. S., Choi, H. S., Kim, Y. M., Hong, S. W., Yeon, S. H., et al. (2015). HS-23, a Lonicera japonica extract, reverses sepsis-induced immunosuppression by inhibiting lymphocyte apoptosis. J. Ethnopharmacol. 171, 231–239. doi:10.1016/j.jep.2015.05.049
Krawczyk, M., Maciejewska, D., Ryterska, K., Czerwinka-Rogowska, M., Jamiol-Milc, D., Skonieczna-Zydecka, K., et al. (2018). Gut permeability might be improved by dietary fiber in individuals with nonalcoholic fatty liver disease (NAFLD) undergoing weight reduction. Nutrients 10 (11), 1793. doi:10.3390/nu10111793
Lankelma, J. M., Cranendonk, D. R., Belzer, C., de Vos, A. F., de Vos, W. M., van der Poll, T., et al. (2017). Antibiotic-induced gut microbiota disruption during human endotoxemia: A randomised controlled study. Gut 66 (9), 1623–1630. doi:10.1136/gutjnl-2016-312132
Leal-Lopes, C., Velloso, F. J., Campopiano, J. C., Sogayar, M. C., and Correa, R. G. (2015). Roles of commensal microbiota in pancreas homeostasis and pancreatic pathologies. J. Diabetes Res. 2015, 284680. doi:10.1155/2015/284680
Li, A., Li, J., Bao, Y., Yuan, D., and Huang, Z. (2016). Xuebijing injection alleviates cytokine-induced inflammatory liver injury in CLP-induced septic rats through induction of suppressor of cytokine signaling 1. Exp. Ther. Med. 12 (3), 1531–1536. doi:10.3892/etm.2016.3476
Li, G. X., Wang, X. M., Jiang, T., Gong, J. F., Niu, L. Y., and Li, N. (2015). Berberine prevents intestinal mucosal barrier damage during early phase of sepsis in rat through the toll-like receptors signaling pathway. Korean J. Physiol. Pharmacol. 19 (1), 1–7. doi:10.4196/kjpp.2015.19.1.1
Li, P., Liao, S. T., Wang, J. S., Zhang, Q., Xu, D. Q., Lv, Y., et al. (2017). Protection by Huang-Lian-Jie-Du decoction and its constituent herbs of lipopolysaccharide-induced acute kidney injury. FEBS Open Bio 7 (2), 221–236. doi:10.1002/2211-5463.12178
Li, T., Qian, Y., Miao, Z., Zheng, P., Shi, T., Jiang, X., et al. (2020). Xuebijing injection alleviates Pam3CSK4-induced inflammatory response and protects mice from sepsis caused by methicillin-resistant Staphylococcus aureus. Front. Pharmacol. 11, 104. doi:10.3389/fphar.2020.00104
Li, Y., Zhou, J., Qiu, J., Huang, Z., Wang, W., Wu, P., et al. (2020). Berberine reduces gut-vascular barrier permeability via modulation of ApoM/S1P pathway in a model of polymicrobial sepsis. Life Sci. 261, 118460. doi:10.1016/j.lfs.2020.118460
Liang, X., Zhou, M., Ge, X. Y., Li, C. B., Fang, S. P., Tang, L., et al. (2015). Efficacy of traditional Chinese medicine on sepsis: A systematic review and meta-analysis. Int. J. Clin. Exp. Med. 8 (11), 20024–20034.
Lin, C. J., Su, Y. C., Lee, C. H., Li, T. C., Chen, Y. A., and Lin, S. J. (2013). Bai-hu-tang, ancient Chinese medicine formula, may provide a new complementary treatment option for sepsis. Evid. Based. Complement. Altern. Med. 2013, 193084. doi:10.1155/2013/193084
Lin, S. J., Cheng, Y. Y., Chang, C. H., Lee, C. H., Huang, Y. C., and Su, Y. C. (2013). Traditional Chinese medicine diagnosis “yang-xu zheng”: Significant prognostic predictor for patients with severe sepsis and septic shock. Evid. Based Complement. Altern. Med. 2013, 759748. doi:10.1155/2013/759748
Liu, F., Yu, C., Liu, J., Johnson, N., Zhang, Y., Su, Z., et al. (2020). Dahuang Fuzi decoction reduces inflammation levels and alleviates intestinal mucosal barrier damage in septic rats. J. Traditional Chin. Med. Sci. 7 (1), 37–44. doi:10.1016/j.jtcms.2019.12.002
Liu, L., Shi, Y., Li, Z., Zuo, L., Tang, M., Jing, Z., et al. (2020). Metabolomic insights into the synergistic effect of biapenem in combination with xuebijing injection against sepsis. Front. Pharmacol. 11, 502. doi:10.3389/fphar.2020.00502
Liu, T., Guo, Y., Zhao, J., He, S., Bai, Y., Wang, N., et al. (2020b). Systems pharmacology and verification of ShenFuHuang formula in zebrafish model reveal multi-scale treatment strategy for septic syndrome in COVID-19. Front. Pharmacol. 11, 584057. doi:10.3389/fphar.2020.584057
Liu, Z., Li, N., Fang, H., Chen, X., Guo, Y., Gong, S., et al. (2019). Enteric dysbiosis is associated with sepsis in patients. FASEB J. 33 (11), 12299–12310. doi:10.1096/fj.201900398RR
Lv, Y., Wang, J., Xu, D., Liao, S., Li, P., Zhang, Q., et al. (2017). Comparative study of single/combination use of huang-lian-jie-du decoction and berberine on their protection on sepsis induced acute liver injury by NMR metabolic profiling. J. Pharm. Biomed. Anal. 145, 794–804. doi:10.1016/j.jpba.2017.07.062
Macpherson, A. J., Köller, Y., and Mccoy, K. D. (2015). The bilateral responsiveness between intestinal microbes and IgA. Trends Immunol. 36 (8), 460–470. doi:10.1016/j.it.2015.06.006
Maier, L., Goemans, C. V., Wirbel, J., Kuhn, M., Eberl, C., Pruteanu, M., et al. (2021). Unravelling the collateral damage of antibiotics on gut bacteria. Nature 599 (7883), 120–124. doi:10.1038/s41586-021-03986-2
Miller, W. D., Keskey, R., and Alverdy, J. C. (2021). Sepsis and the microbiome: A vicious cycle. J. Infect. Dis. 223, S264–S269. doi:10.1093/infdis/jiaa682
Mu, S., Xiang, H., Wang, Y., Wei, W., Long, X., Han, Y., et al. (2022). The pathogens of secondary infection in septic patients share a similar genotype to those that predominate in the gut. Crit. Care 26 (1), 68. doi:10.1186/s13054-022-03943-z
Mu, S., Zhang, J., Du, S., Zhu, M., Wei, W., Xiang, J., et al. (2021). Gut microbiota modulation and anti-inflammatory properties of Xuanbai Chengqi decoction in septic rats. J. Ethnopharmacol. 267, 113534. doi:10.1016/j.jep.2020.113534
Niu, M., and Chen, P. (2021). Crosstalk between gut microbiota and sepsis. Burns Trauma 9, tkab036. tkab036. doi:10.1093/burnst/tkab036
Ojima, M., Motooka, D., Shimizu, K., Gotoh, K., Shintani, A., Yoshiya, K., et al. (2016). Metagenomic analysis reveals dynamic changes of whole gut microbiota in the acute phase of intensive care unit patients. Dig. Dis. Sci. 61 (6), 1628–1634. doi:10.1007/s10620-015-4011-3
Poroyko, V., Meng, F., Meliton, A., Afonyushkin, T., Ulanov, A., Semenyuk, E., et al. (2015). Alterations of lung microbiota in a mouse model of LPS-induced lung injury. Am. J. Physiol. Lung Cell. Mol. Physiol. 309 (1), L76–L83. doi:10.1152/ajplung.00061.2014
Prescott, H. C., Dickson, R. P., Rogers, M. A. M., Langa, K. M., and Iwashyna, T. J. (2015). Hospitalization type and subsequent severe sepsis. Am. J. Respir. Crit. Care Med. 192 (5), 581–588. doi:10.1164/rccm.201503-0483OC
Rinninella, E., Raoul, P., Cintoni, M., Franceschi, F., Miggiano, G., Gasbarrini, A., et al. (2019). What is the healthy gut microbiota composition? A changing ecosystem Across age, environment, diet, and diseases. Microorganisms 7 (1), 14. doi:10.3390/microorganisms7010014
Schmitt, F. C. F., Lipinski, A., Hofer, S., Uhle, F., Nusshag, C., Hackert, T., et al. (2020). Pulmonary microbiome patterns correlate with the course of disease in patients with sepsis-induced ARDS following major abdominal surgery. J. Hosp. Infect. S0195-6701 (20), 438–446. doi:10.1016/j.jhin.2020.04.028
Schulthess, J., Pandey, S., Capitani, M., Rue-Albrecht, K. C., Arnold, I., Franchini, F., et al. (2019). The short chain fatty acid butyrate imprints an antimicrobial program in macrophages. Immunity 50 (2), 432–445. e7. doi:10.1016/j.immuni.2018.12.018
Seixas, S., Kolbe, A. R., Gomes, S., Sucena, M., Sousa, C., Vaz Rodrigues, L., et al. (2021). Comparative analysis of the bronchoalveolar microbiome in Portuguese patients with different chronic lung disorders. Sci. Rep. 11 (1), 15042. doi:10.1038/s41598-021-94468-y
Shi, H., Yu, Y., Lin, D., Zheng, P., Zhang, P., Hu, M., et al. (2020). β-Glucan attenuates cognitive impairment via the gut-brain Axis in diet-induced obese mice. Microbiome 8 (1), 143. doi:10.1186/s40168-020-00920-y
Shimizu, K., Ogura, H., Hamasaki, T., Goto, M., Tasaki, O., Asahara, T., et al. (2011). Altered gut flora are associated with septic complications and death in critically ill patients with systemic inflammatory response syndrome. Dig. Dis. Sci. 56 (4), 1171–1177. doi:10.1007/s10620-010-1418-8
Shou, D. W., Yu, Z. L., Meng, J. B., Lai, Z. Z., Pang, L. S., Dai, M. H., et al. (2022). Panax notoginseng alleviates sepsis-induced acute kidney injury by reducing inflammation in rats. Evid. Based. Complement. Altern. Med. 2022, 9742169. doi:10.1155/2022/9742169
Singer, M., Deutschman, C. S., Seymour, C. W., Shankar-Hari, M., Annane, D., Bauer, M., et al. (2016). The third international consensus definitions for sepsis and septic shock (Sepsis-3). JAMA 315 (8), 801–810. doi:10.1001/jama.2016.0287
Singh, G., Brass, A., Knight, C. G., and Cruickshank, S. M. (2019). Gut eosinophils and their impact on the mucus-resident microbiota. Immunology 158 (3), 194–205. doi:10.1111/imm.13110
Siwicka-Gieroba, D., and Czarko-Wicha, K. (2020). Lung microbiome - a modern knowledge. Cent. Eur. J. Immunol. 45 (3), 342–345. doi:10.5114/ceji.2020.101266
Song, W., Yang, X., Wang, W., Wang, Z., Wu, J., and Huang, F. (2021). Sinomenine ameliorates septic acute lung injury in mice by modulating gut homeostasis via aryl hydrocarbon receptor/nrf2 pathway. Eur. J. Pharmacol. 912, 174581. doi:10.1016/j.ejphar.2021.174581
Song, Y., Wu, Q., Jiang, H., Hu, A., Xu, L., Tan, C., et al. (2022). The effect of shionone on sepsis-induced acute lung injury by the ECM1/STAT5/NF-κB pathway. Front. Pharmacol. 12, 764247. doi:10.3389/fphar.2021.764247
Su, T., Qiu, Y., Hua, X., Ye, B., Luo, H., Liu, D., et al. (2020). Novel opportunity to reverse antibiotic resistance: To explore traditional Chinese medicine with potential activity against antibiotics-resistance bacteria. Front. Microbiol. 11, 610070. doi:10.3389/fmicb.2020.610070
Sun, J., Ding, X., Liu, S., Duan, X., Liang, H., and Sun, T. (2020). Adipose-derived mesenchymal stem cells attenuate acute lung injury and improve the gut microbiota in septic rats. Stem Cell Res. Ther. 11 (1), 384. doi:10.1186/s13287-020-01902-5
Sun, Y. K., Zhang, Y. F., Xie, L., Rong, F., Zhu, X. Y., Xie, J., et al. (2022). Progress in the treatment of drug-induced liver injury with natural products. Pharmacol. Res. 183, 106361. doi:10.1016/j.phrs.2022.106361
Sze, M. A., Tsuruta, M., Yang, S. W., Oh, Y., Man, S. F., Hogg, J. C., et al. (2014). Changes in the bacterial microbiota in gut, blood, and lungs following acute LPS instillation into mice lungs. PLoS One 9 (10), e111228. doi:10.1371/journal.pone.0111228
Tang, J., Xu, L., Zeng, Y., and Gong, F. (2021). Effect of gut microbiota on LPS-induced acute lung injury by regulating the TLR4/NF-kB signaling pathway. Int. Immunopharmacol. 91, 107272. doi:10.1016/j.intimp.2020.107272
Varkouhi, A. K., Monteiro, A., Tsoporis, J. N., Mei, S., Stewart, D. J., and Dos, S. C. (2020). Genetically modified mesenchymal stromal/stem cells: Application in critical illness. Stem Cell Rev. Rep. 16 (5), 812–827. doi:10.1007/s12015-020-10000-1
Wang, J., Song, H., Ge, F., Xiong, P., Jing, J., He, T., et al. (2022). Landscape of DILI-related adverse drug reaction in China Mainland. Acta Pharm. Sin. B. doi:10.1016/j.apsb.2022.04.019
Wang, L., Liang, Q., Lin, A., Wu, Y., Min, H., Song, S., et al. (2019). Borneol alleviates brain injury in sepsis mice by blocking neuronal effect of endotoxin. Life Sci. 232, 116647. doi:10.1016/j.lfs.2019.116647
Wang, S., Hu, Y., Tan, W., Wu, X., Chen, R., Cao, J., et al. (2012). Compatibility art of traditional Chinese medicine: From the perspective of herb pairs. J. Ethnopharmacol. 143 (2), 412–423. doi:10.1016/j.jep.2012.07.033
Wang, W., Chen, Q., Yang, X., Wu, J., and Huang, F. (2020). Sini decoction ameliorates interrelated lung injury in septic mice by modulating the composition of gut microbiota. Microb. Pathog. 140, 103956. doi:10.1016/j.micpath.2019.103956
Wang, X. T., Peng, Z., An, Y. Y., Shang, T., Xiao, G., He, S., et al. (2021). Paeoniflorin and hydroxysafflor yellow A in xuebijing injection attenuate sepsis-induced cardiac dysfunction and inhibit proinflammatory cytokine production. Front. Pharmacol. 11, 614024. doi:10.3389/fphar.2020.614024
Wang, Y. P., Guo, Y., Wen, P. S., Zhao, Z. Z., Xie, J., Yang, K., et al. (2020). Three ingredients of safflower alleviate acute lung injury and inhibit NET release induced by lipopolysaccharide. Mediat. Inflamm. 2020, 2720369. doi:10.1155/2020/2720369
Wang, Z. Y., Wang, X. S., Guo, Z., Liao, H. Y., Chai, Y., Wang, Z. W., et al. (2022). Reduning attenuates LPS-induced human unmilical vein endothelial cells (HUVECs) apoptosis through PI3K-akt signaling pathway. Front. Pharmacol. 13, 921337. doi:10.3389/fphar.2022.921337
Wei, L., Yue, F., Xing, L., Wu, S., Shi, Y., Li, J., et al. (2020). Constant light exposure alters gut microbiota and promotes the progression of steatohepatitis in high fat diet rats. Front. Microbiol. 11, 1975. doi:10.3389/fmicb.2020.01975
Wei, Y., Shan, L., Qiao, L., Liu, R., Hu, Z., and Zhang, W. (2013). Protective effects of huang-lian-jie-du-tang against polymicrobial sepsis induced by cecal ligation and puncture in rats. Evid. Based. Complement. Altern. Med. 2013, 909624. doi:10.1155/2013/909624
Wen, Y., Feng, C., Chen, W., Chen, C., Kuang, S., Liu, F., et al. (2021). Effect of traditional Chinese medicine on serum inflammation and efficacy in patients with sepsis: A systematic review and meta-analysis. Ann. Palliat. Med. 10 (12), 12456–12466. doi:10.21037/apm-21-3179
Wiersinga, W. J., Rhodes, A., Cheng, A. C., Peacock, S. J., and Prescott, H. C. (2020). Pathophysiology, transmission, diagnosis, and treatment of coronavirus disease 2019 (COVID-19): A review . JAMA 324 (8), 782–793. doi:10.1001/jama.2020.12839
Wu, T., Li, H., Su, C., Xu, F., Yang, G., Sun, K., et al. (2020). Microbiota-derived short-chain fatty acids promote LAMTOR2-mediated immune responses in macrophages. mSystems 5 (6), e00587–20. doi:10.1128/mSystems.00587-20
Wu, Y., Qin, C., Lu, X., Marchiori, J., and Feng, Q. (2016). North American ginseng inhibits myocardial NOX2-ERK1/2 signaling and tumor necrosis factor-α expression in endotoxemia. Pharmacol. Res. 111, 217–225. doi:10.1016/j.phrs.2016.06.010
Xie, M. Z., Qi, Q. H., Zhang, S. L., and Wei, M. M. (2015). Effects of Dachengqi Decoction (大承气汤) on morphological changes in enteric nerve system of rats with multiple organ dysfunction syndrome. Chin. J. Integr. Med. 21 (8), 624–629. doi:10.1007/s11655-015-1978-8
Xing, W., Yang, L., Peng, Y., Wang, Q., Gao, M., Yang, M., et al. (2017). Ginsenoside Rg3 attenuates sepsis-induced injury and mitochondrial dysfunction in liver via AMPK-mediated autophagy flux. Biosci. Rep. 37 (4), BSR20170934. doi:10.1042/BSR20170934
Xing, X., Jiang, R., Wang, L., Lei, S., Zhi, Y., Wu, Y., et al. (2015). Shenfu injection alleviates intestine epithelial damage in septic rats. Am. J. Emerg. Med. 33 (11), 1665–1670. doi:10.1016/j.ajem.2015.08.001
Xiong, C. Q., Zhou, H. C., Wu, J., and Guo, N. Z. (2019). The protective effects and the involved mechanisms of Tanshinone IIA on sepsis-induced brain damage in mice. Inflammation 42 (1), 354–364. doi:10.1007/s10753-018-0899-z
Xu, D., Lv, Y., Wang, J., Yang, M., and Kong, L. (2017). Deciphering the mechanism of huang-lian-jie-du-decoction on the treatment of sepsis by formula decomposition and metabolomics: Enhancement of cholinergic pathways and inhibition of HMGB-1/TLR4/NF-κB signaling. Pharmacol. Res. 121, 94–113. doi:10.1016/j.phrs.2017.04.016
Xu, J., Song, J., Zhang, Y., Wang, Y., Yang, L., Sha, Y., et al. (2020). Jinzhi protects lipopolysaccharide-treated mice against mortality by repairing intestinal mucosal barrier damage and intestinal microecology. Biomed. Pharmacother. 123, 109749. doi:10.1016/j.biopha.2019.109749
Xu, P., Zhang, W. Q., Xie, J., Wen, Y. S., Zhang, G. X., and Lu, S. Q. (2020). Shenfu injection prevents sepsis-induced myocardial injury by inhibiting mitochondrial apoptosis. J. Ethnopharmacol. 261, 113068. doi:10.1016/j.jep.2020.113068
Xue, W., Li, Y., and Zhang, M. (2021). Pristimerin inhibits neuronal inflammation and protects cognitive function in mice with sepsis-induced brain injuries by regulating PI3K/Akt signalling. Pharm. Biol. 59 (1), 1351–1358. doi:10.1080/13880209.2021.1981399
Yamada, T., Shimizu, K., Ogura, H., Asahara, T., Nomoto, K., Yamakawa, K., et al. (2015). Rapid and sustained long-term decrease of fecal short-chain fatty acids in critically ill patients with systemic inflammatory response syndrome. JPEN. J. Parenter. Enter. Nutr. 39 (5), 569–577. doi:10.1177/0148607114529596
Yang, C., Song, C., Liu, Y., Qu, J., Li, H., Xiao, W., et al. (2021). Re-Du-Ning injection ameliorates LPS-induced lung injury through inhibiting neutrophil extracellular traps formation. Phytomedicine. 90, 153635. doi:10.1016/j.phymed.2021.153635
Yang, X., Liu, D., Ren, H., Zhang, X., Zhang, J., and Yang, X. (2021). Effects of sepsis and its treatment measures on intestinal flora structure in critical care patients. World J. Gastroenterol. 27 (19), 2376–2393. doi:10.3748/wjg.v27.i19.2376
Yin, J., Yu, Z., Hou, C., Peng, Y., Xiao, J., and Jiang, J. (2021). Protective effect of zuojin fang on lung injury induced by sepsis through downregulating the JAK1/STAT3 signaling pathway. Biomed. Res. Int. 2021, 1419631. doi:10.1155/2021/1419631
Yin, Y., Fan, B., Liu, W., Ren, R., Chen, H., Bai, S., et al. (2017). Investigation into the stability and culturability of Chinese enterotypes. Sci. Rep. 7 (1), 7947. doi:10.1038/s41598-017-08478-w
Yoseph, B. P., Klingensmith, N. J., Liang, Z., Breed, E. R., Burd, E. M., Mittal, R., et al. (2016). Mechanisms of intestinal barrier dysfunction in sepsis. Shock 46 (1), 52–59. doi:10.1097/SHK.0000000000000565
Yu, Z., Liu, Z., Chen, J., and Zeng, Q. (2014). Anti-inflammatory effect of qingwen Baidu decoction (清瘟败毒饮) in sepsis rats. Chin. J. Integr. Med. 20 (12), 934–943. doi:10.1007/s11655-014-1863-x
Zakerska-Banaszak, O., Tomczak, H., Gabryel, M., Baturo, A., Wolko, L., Michalak, M., et al. (2021). Dysbiosis of gut microbiota in polish patients with ulcerative colitis: A pilot study. Sci. Rep. 11 (1), 2166. doi:10.1038/s41598-021-81628-3
Zeng, M. Y., Cisalpino, D., Varadarajan, S., Hellman, J., Warren, H. S., Cascalho, M., et al. (2016). Gut microbiota-induced immunoglobulin G controls systemic infection by symbiotic bacteria and pathogens. Immunity 44 (3), 647–658. doi:10.1016/j.immuni.2016.02.006
Zha, Y. F., Xie, J., Ding, P., Zhu, C. L., Li, P., Zhao, Z. Z., et al. (2021). Senkyunolide I protect against lung injury via inhibiting formation of neutrophil extracellular trap in a murine model of cecal ligation and puncture. Int. Immunopharmacol. 99, 107922. doi:10.1016/j.intimp.2021.107922
Zhang, D., Chen, G., Manwani, D., Mortha, A., Xu, C., Faith, J. J., et al. (2015). Neutrophil ageing is regulated by the microbiome. Nature 525 (7570), 528–532. doi:10.1038/nature15367
Zhang, M., Lian, B., Zhang, R., Guo, Y., Zhao, J., He, S., et al. (2022). Emodin ameliorates intestinal dysfunction by maintaining intestinal barrier integrity and modulating the microbiota in septic mice. Mediat. Inflamm. 2022, 5026103. doi:10.1155/2022/5026103
Zhang, Q., Lei, H. M., Wang, P. L., Ma, Z. Q., Zhang, Y., Wu, J. J., et al. (2017). Bioactive components from qingwen Baidu decoction against LPS-induced acute lung injury in rats. Molecules 22 (5), 692. doi:10.3390/molecules22050692
Zhang, Q., Piao, X., Piao, X., Lu, T., Wang, D., and Kim, S. W. (2011). Preventive effect of coptis chinensis and berberine on intestinal injury in rats challenged with lipopolysaccharides. Food Chem. Toxicol. 49 (1), 61–69. doi:10.1016/j.fct.2010.09.032
Zhang, S., Wang, D., Wang, X., Li, S., Li, J., Li, H., et al. (2013). Aqueous extract of Bai-Hu-Tang, a classical Chinese herb formula, prevents excessive immune response and liver injury induced by LPS in rabbits. J. Ethnopharmacol. 149 (1), 321–327. doi:10.1016/j.jep.2013.06.041
Zhang, Z., Zhang, L., Zhang, Q., Liu, B., Li, F., Xin, Y., et al. (2021). HO-1/CO maintains intestinal barrier integrity through NF-κB/MLCK pathway in intestinal HO-1-/- mice. Oxid. Med. Cell. Longev. 2021, 6620873. doi:10.1155/2021/6620873
Zhao, F., Fang, Y., Deng, S., Li, X., Zhou, Y., Gong, Y., et al. (2017). Glycyrrhizin protects rats from sepsis by blocking HMGB1 signaling. Biomed. Res. Int. 2017, 9719647. doi:10.1155/2017/9719647
Zhao, H., Liu, Z., Shen, H., Jin, S., and Zhang, S. (2016a). Glycyrrhizic acid pretreatment prevents sepsis-induced acute kidney injury via suppressing inflammation, apoptosis and oxidative stress. Eur. J. Pharmacol. 781, 92–99. doi:10.1016/j.ejphar.2016.04.006
Zhao, H., Zhao, M., Wang, Y., Li, F., and Zhang, Z. (2016b). Glycyrrhizic acid prevents sepsis-induced acute lung injury and mortality in rats. J. Histochem. Cytochem. 64 (2), 125–137. doi:10.1369/0022155415610168
Zheng, Y., Liu, S., Fan, C., Zeng, H., Huang, H., Tian, C., et al. (2020). Holistic quality evaluation of qingwen Baidu decoction and its anti-inflammatory effects. J. Ethnopharmacol. 263, 113145. doi:10.1016/j.jep.2020.113145
Zheng, Y., Shi, X., Hou, J., Gao, S., Chao, Y., Ding, J., et al. (2021). Integrating metabolomics and network pharmacology to explore Rhizoma Coptidis extracts against sepsis-associated acute kidney injury. J. Chromatogr. B Anal. Technol. Biomed. Life Sci. 1164, 122525. doi:10.1016/j.jchromb.2021.122525
Zhou, A., Lei, Y., Tang, L., Hu, S., Yang, M., Wu, L., et al. (2021). Gut microbiota: The emerging link to lung homeostasis and disease. J. Bacteriol. 203 (4), e00454–20. doi:10.1128/JB.00454-20
Keywords: sepsis, intestinal microbiota, traditional Chinese medicines, gut barrier, immune system
Citation: Wang X-H, Xu D-Q, Chen Y-Y, Yue S-J, Fu R-J, Huang L and Tang Y-P (2022) Traditional Chinese Medicine: A promising strategy to regulate inflammation, intestinal disorders and impaired immune function due to sepsis. Front. Pharmacol. 13:952938. doi: 10.3389/fphar.2022.952938
Received: 25 May 2022; Accepted: 29 August 2022;
Published: 16 September 2022.
Edited by:
Alessandra Durazzo, Council for Agricultural Research and Economics, ItalyCopyright © 2022 Wang, Xu, Chen, Yue, Fu, Huang and Tang. This is an open-access article distributed under the terms of the Creative Commons Attribution License (CC BY). The use, distribution or reproduction in other forums is permitted, provided the original author(s) and the copyright owner(s) are credited and that the original publication in this journal is cited, in accordance with accepted academic practice. No use, distribution or reproduction is permitted which does not comply with these terms.
*Correspondence: Ding-Qiao Xu, xudingqiao16@126.com; Yu-Ping Tang, yupingtang@sntcm.edu.cn