- 1Key Laboratory of Natural Medicines of the Changbai Mountain, Ministry of Education, College of Pharmacy, Yanbian University, Yanji, China
- 2Shanghai Engineering Center of Industrial Asymmetric Catalysis for Chiral Drugs, Shanghai, China
- 3College of Medical, Yanbian University, Yanji, China
Lotus (Nelumbo nucifera) leaves are widely used for both edible and medicinal applications. For its further utilization, we studied the vasodilatory activity of lotus leaf extract for the first time. In this study, we obtained the extracts using different ratios of water and ethanol, which was followed by polarity-dependent extraction. We found that the CH2Cl2 layer exhibited better vasodilatory activity (EC50 = 1.21 ± 0.10 μg/ml). HPLC and ESI-HRMS analysis of the CH2Cl2 layer using the standard product as a control revealed that nuciferine (Emax = 97.95 ± 0.76%, EC50 = 0.36 ± 0.02 μM) was the main component in this layer. Further research revealed that nuciferine exerts a multi-target synergistic effect to promote vasodilation, via the NO signaling pathway, K+ channel, Ca2+ channel, intracellular Ca2+ release, α and β receptors, etc. Nuciferine exhibits good vasodilatory activity, and it exhibits the potential to be utilized as a lead compound.
1 Introduction
Hypertension is a common chronic disease encountered in clinical practice. Based on the related complications, the mortality and disability rate are at the forefront of human diseases, which causes great harm to human health (Wang et al., 2014; Navaneethabalakrishnan et al., 2020). At present, most blood pressure-lowering drugs in clinical use are chemically synthesized. They exhibit several limitations, such as dependence, high toxicity, side effects, and large fluctuations in blood pressure during blood pressure reduction. Traditional Chinese medicine is known to exhibit less toxicity and side effects can exert an antihypertensive effect through multiple channels and targets, is rich in resources, and has broad development and application prospects. In recent years, the discovery of new active ingredients or lead compounds from natural products for the development of new drugs has gained attention for resolving global concerns and in research.
Lotus leaves are the dried leaves of Nelumbo nucifera Geartn., a plant belonging to the Nymphaeaceae family (Liu and Fan, 2014). In recent years, more attention has been paid to the research on elucidating the composition and function of lotus leaves. Its main ingredients include alkaloids, volatile oils, organic acids, and flavonoids (Nguyen and Pham, 1998; Ma et al., 2010; Chen and Qi, 2015; Tungmunnithum et al., 2018; Ye et al., 2018). It has various pharmacological activities, such as regulating fat and weight loss (Ma et al., 2015), anti-inflammatory (Wang M. X. et al., 2015), lowering blood pressure (Ye et al., 2014), anti-oxidation (Chen and Zeng, 2001; Chai et al., 2016; Guo et al., 2020), anti-bacterial (Wang et al., 2007; Yuan et al., 2014), anti-spasmodic (Yang et al., 2018), hemostasis (Chen et al., 2020), and hepatoprotective effects (Guo et al., 2013; Qiu et al., 2020; Wang et al., 2020).
Lotus leaves are widely distributed, rich in resources, and inexpensive. They exert the effect of lowering blood pressure, and their alkaloids have been reported to be involved in relaxation (Wang X. et al., 2015; Yang et al., 2018). However, there has been no systematic research on the active ingredients present in lotus leaves. In this study, we aimed to fill in the knowledge gap on lotus leaf and identify the main components of vasodilatory activity, to ensure more effective use of lotus leaves and provide a basis for its future clinical applications.
2 Materials and methods
2.1 Materials
Lotus leaf (Nelumbo nucifera) was purchased from Hunan Kang Biotech Co. Ltd. and identified taxonomically by Prof. Chang-hao Zhang (College of Pharmacy, Yanbian University). Acetylcholine chloride (Ach), Phenylephrine HCl (PhE), Modulators were purchased from Sigma Chemical Co (St. Louis, MO, United States).
2.2 Apparatus
Mass spectrometry (MS) was performed on LTQ Orbitrap XL (Thermo Scientific, United States) in electrospray ionization mode. High-performance liquid chromatography (HPLC) was undertaken on an Ultimate 3,000 (ThermoScientific) system.
2.3 Extraction procedures
The purchased lotus leaves were ground into powder. This 10 g of powder was added to a single-necked round-bottomed flask and then 300 ml of various solvents (H2O; 25% Ethanol; 50% Ethanol; 75% Ethanol; Ethanol) was added to hot melt at 70°C for 6 h. Extracts were passed through a sand-core filter funnel and evaporated under reduced pressure until dry using a rotary evaporator. All dried extracts were weighed and stored at −20°C until use. The yield was calculated as % yield = (weight of dry extract/initial weight of dry sample) × 100.
2.4 Animals and tissues
Animals were strictly handled in accordance with the National Institutes of Health Guidelines for the Care and Use of Laboratory Animals (NIH Publication No. 85–23, revised 1996) and the study was approved by the Institutional Animal Care and Utilization Committee of Yanbian University. Male Sprague-Dawley (SD) rats weighing 250–300 g were used for all experiments, random grouping, 6-8 animals per group. For example, in the intact endothelium experiment, animals were randomly divided into 7 groups with 8 animals in each group (control group, +endo group, Prop group, Atrop group, Indo group, L-NAME group, ODQ group); the experimental results are as follows Figures 6–9. Other animal grouping information was detailed in the Supplementary Information.
SD rats were killed by cervical dislocation. A 3–4 mm long annular segment of the aorta was carefully removed and transferred promptly into ice-cold Krebs solution (NaCl 118.0 mM, KCl 4.7 mm, MgSO4 1.1 mm, KH2PO4 1.2 mm, CaCl2 1.5 mm, NaHCO3 25 mm, and glucose 10.0 mm in water, pH 7.4). In some aortic rings, the endothelium was mechanically removed by gently rubbing the surface of the ring back and forth with a plastic tube. Endothelial integrity or functional removal of the constricted blood vessels was confirmed by the presence of relaxation response (over 80%) or its absence (less than 10%) following the treatment with PhE (1 μM) and Ach (1 μM), respectively.
2.5 Isometric vascular tone record
The aortic rings were continuously suspended in a tissue bath containing Krebs solution (pH 7.4) and bubbling 95% O2–5% CO2 at 37°C by inserting 2 stainless steel wires into the lumen of the tube. After an equilibration period of 60 min, under a basal tension of 1 g, the force-displacement sensor (JH-2, Institute of Space Medical Engineering, Beijing, China), connected to the biological laboratory system (Model BL-420S, Cheng-du TME Technology Co., Ltd., Chengdu, China), was used to record changes in isometric tension.
2.6 Measurement of the activity of extracts
The contraction of aortic rings was achieved by PhE (1 μM). When the contraction reached a plateau, extracts were added cumulatively (100 μg/ml) to rings with intact endothelium. The relaxation effect was calculated as the percentage of the contraction in response to PhE.
2.7 Different solvent extraction and activity detection
The best solvent extract for vasodilation was extracted with PE, CH2Cl2, EA and n-butanol in sequence according to polarity.
2.8 HPLC
TSKgel ODS-100V column (C18, 3 μm particle size, 4.6 mm I.D × 15 cm) was employed to separate components in CH2Cl2 extracts. The mobile phase was 0.1% trifluoroacetic acid in acetonitrile (HPLC grade) (A) and water (B) gradient elution (10:90–95:5). The run time was 35 min, the flow rate was 1.0 ml/min, and the detection wavelength was 273 nm. We diluted extract with acetonitrile to give a concentration of 5 mg/ml (Standard nuciferine, 0.5 mg/ml, 2 μL), which was passed through a 0.45-μm filter. The injection volume was 10 μL.
2.9 Vascular reactivity assessment
2.9.1 The effect of nuciferine on rat thoracic aorta
To observe the direct effect of nuciferine on the isolated rat aorta, the cumulative concentrations of nuciferine were applied when the tension of thoracic aorta strips was stable, and the vehicle was applied in the control group (Hu et al., 2018).
2.9.2 Vasorelaxant effect of nuciferine on the rat thoracic aorta pre-contracted with PhE
To check the vasorelaxation of nuciferine on the aortic strips with intact or denuded endothelium, after the strips were contracted with PhE (1 μM), a cumulative concentration of nuciferine was added successively into the bath and the changes in the tension were recorded. The percentage of tension change (diastolic ratio) after using nuciferine was calculated as (tension by PhE − tension by nuciferine)/tension by PhE × 100%.
2.9.3 Examination of α1-receptor activities
To evaluate whether nuciferine acts as an α1-receptor blockade, endothelium-denuded rings were incubated with the nuciferine (0.3, 0.6,1.2 and 2.4 μM) for 20 min before exposing them to PhE (0.1 nM–10 μM), which was added cumulatively. The obtained results are shown as percentages of contraction, and a comparison was done between the results obtained in the absence (control) and the presence of the nuciferine (Cao et al., 2020).
2.9.4 Examination of β and M receptor activities
To assess whether the vasorelaxation induced by nuciferine is associated with β and M receptors, the aortic rings were pre-treated with 1 μM of propranolol or 1 μM of atropine for 20 min before the addition of 1 μM of PhE. The nuciferine (0.03–2.4 μM) was then added cumulatively.
2.9.5 Effects of PGI2 on nuciferine-induced relaxation
To explore the possible participation of endothelium-derived prostacyclin (PGI2) pathways, the endothelium-intact strips were firstly incubated with 10 μM of Indo, then contracted by PhE (1 μM) and finally a cumulative concentration of nuciferine (0.03–2.4 μM) was added to observe its vasorelaxation (Yang et al., 2020).
2.9.6 Effects of eNOS/sGC/cGMP signaling pathways
To explore the possible participation of endothelium-derived nitric oxide (NO) pathways, the endothelium-intact strips were firstly incubated with L-NAME (100 μM) or ODQ (10 μM) or, then, contracted by PhE (1 μM), and finally a cumulative concentration of nuciferine (0.03–2.4 μM) was added to observe its vasorelaxation PhE (1 μM) was precontracted to endothelium-denuded aortic ring and treated with drugs; SNP was used as a positive control. After the experiment, liquid nitrogen quickly freezes the vascular ring. The experimental operations were performed in the presence of the non-specific phosphodiesterase inhibitor IBMX, 10 μM. The frozen aorta was ground in a stainless steel mortar, and the operation was carried out in the presence of liquid nitrogen. Weigh the tissue and add 0.1 M HCl (w/v: 1:10) to homogenize and centrifuge at 1500 g for 15 min, take the supernatant. cGMP level is determined by enzyme immunoassay using acetylation procedure and direct cyclic GMP ELISA kit ADI-900–014 (Kubacka et al., 2018).
2.9.7 Examination of K+ channel effect
In order to determine whether nuciferine-induced relaxation was related to the activation of K+ channels, we selected four types of K+ channel blockers to inhibit the different K+ channels, BaCl2 (100 μM) for KIR, 4-AP (1 mm) for KV, TEA (1 mm) for KCa, and Gli (100 μM) for KATP.
2.9.8 Observation of intracellular calcium release
Endothelium-denuded rat thoracic aorta strips were incubated in Ca2+ free KBS solution, and then PhE (1 μM) was added to produce the first transient contraction (T1). Subsequently, the strips were rinsed with KBS solution 4 times to supplement the intracellular Ca2+ loss and with Ca2+ free KBS solution for 2 times in succession. Followed that, nuciferine (0.1, 0.3, 0.6 μM) was added and incubated for 10 min and PhE (1 μM) was added again to produce the second transient vasocontraction (T2). T2/T1 × 100% was calculated as contraction (Qu et al., 2015; Hu et al., 2018; Kim et al., 2019).
2.9.9 Observation of extracellular calcium influx
Endothelium-denuded rat thoracic aorta strips were also employed and incubated in Ca2+-free KBS. Then 1 μM PhE or 60 mm KCl was added to produce a basic contraction, and 0.01–10 mM of CaCl2 were added in sequence to obtain a concentration–response curve. In order to examine the relation of nuciferine-induced vasorelaxation to the contractions by PhE or KCl, nuciferine was pre-incubated for 10 min after adding PhE or KCl. The contraction produced by highest concentration of CaCl2 (10 mm) was taken as 100%, and then, based on which, the inhibitory rate of nuciferine could be calculated (Qu et al., 2015; Hu et al., 2018; Kim et al., 2019).
2.10 Cell culture
HUVECs were cultured at 37°C on gelatin-coated plates in the basal nutrient media DMEM supplemented with 10% fetal bovine serum, 100 U/ml penicillin, 100 µg/ml streptomycin.
2.11 Cell viability assay
Cell viability was determined by a standard MTT (3-(4,5-dimethyl-2-thiazolyl)-2,5.
-diphenyl-2-H-tetrazolium bromide) assay. Briefly, HUVECs were seeded in a 96-well plate at 1 cells/well × 104 cells/well (200 μL). 1, 3, 10, 30 or 100 μM nuciferine treatment for 24 h. MTT solution was added to each well. After 4 h incubation, 150 µL of DMSO was added to dissolve the formazan crystals. Cell viability was reflected by absorbance, which was measured at 492 nm using a microplate reader after 10 min of shaking. Cell viability was expressed as a percentage of the control value. Each experiment was performed in quintuplicate using three independent cultures.
2.12 Western blot Analysis
HUVECs were purchased from lonza (Switzerland). HUVECs were pre-incubated with nuciferine, then exposured to TNF-α (10 ng/ml) (Cao et al., 2020). Total cellular proteins were extracted, followed by protein concentration determination using a BCA kit. Followed by protein denaturation, electrophoresis, transferred to a membrane; the membrane was then blocked with a 5% non-fat skim milk solution for 1–2 h. Then incubated with primary and secondary antibodies. Tris-buffered saline and Tween 20 (TBST) was used to wash the membranes for 5 min, which was repeated three times. Densitometry analysis of the protein bands was performed using the ImageJ software.
2.13 Statistical analysis
GraphPad Prism 5 software was used for the statistical analysis. All values were expressed as mean ± SD of three independent experiments. When the data were normally distributed, they were analysed by unpaired two-tailed Student’s t-tests and multiple groups were analysed by one-way analysis of variance (ANOVA). A p value <0.05 was considered significant.
3 Results
3.1 Yield of various solvent extracts
We investigated the yield of lotus leaf extracts in different solvents. The yield of the H2O, 25% ethanol, 50% ethanol, 75% ethanol, and ethanol extracts ranged from 8.94 ± 0.96 to 22.38 ± 1.64% (Table 1). The vasodilatory activity of the H2O, 25% ethanol, 50% ethanol, 75% ethanol, and ethanol extracts ranged from 19.65 ± 0.19 to 90.63 ± 1.82% (Table 1 and Figure 1). Based on the yield and vasodilatory activity, 75% ethanol was selected as the most suitable extraction solution.
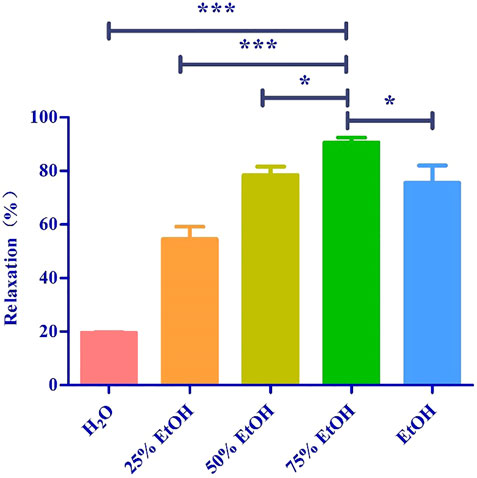
FIGURE 1. Relaxtion of Lotus leaf with different extraction solvents. The data are expressed as the means ± SD of 3 rats (n = 3). (* mean p < 0.05; *** mean p < 0.001 vs. 75% Ethanol group).
3.2 Different solvent extractions
Based on the polarity, the extract obtained using 75% ethanol was further extracted using different solvents (Figure 2). The CH2Cl2 layer exhibited the best vasodilatory activity (98.22 ± 1.01%) (Table 2 and Figure 3). The vasodilatory activity was concentration-dependent (EC50 = 1.21 ± 0.10 µg/ml) (Table 3).
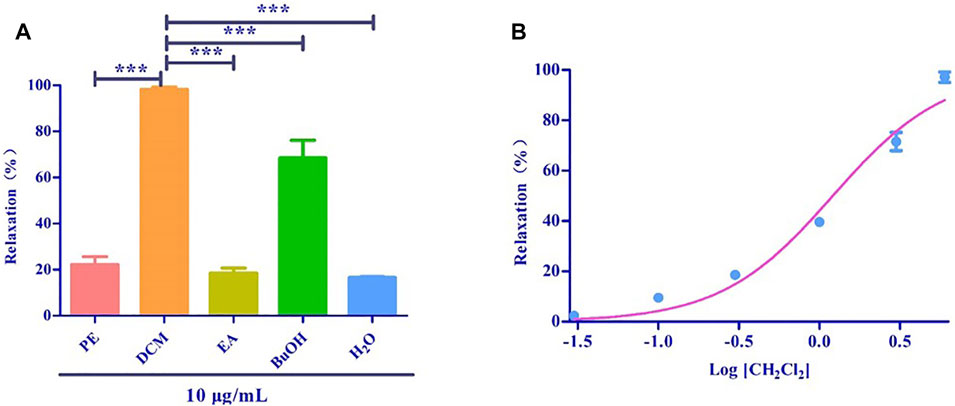
FIGURE 3. (A) Relaxation of different solvent extraction. (B) The cumulative concentration-response curve of the effect of CH2Cl2 (0.03 μg/ml to 6 μg/ml) on isolated rat aortic rings pre-contracted with PhE (1 μM). (*** mean p < 0.001 vs. CH2Cl2 group).
3.3 HPLC and ESI-HRMS analyses
The standard nuciferine product peak appeared at 12.453 min. The component in the CH2Cl2 layer exhibited a peak at 12.480 min (Figure 4A). The peak shape and time were basically the same. Calculated ESI-HRMS value for nuciferine ([M + H] +) was 296.16451, and it was found to be 296.16385. The found ESI-HRMS value of the component in the CH2Cl2 layer ([M + H] +) was 296.16412 (Figure 4B).
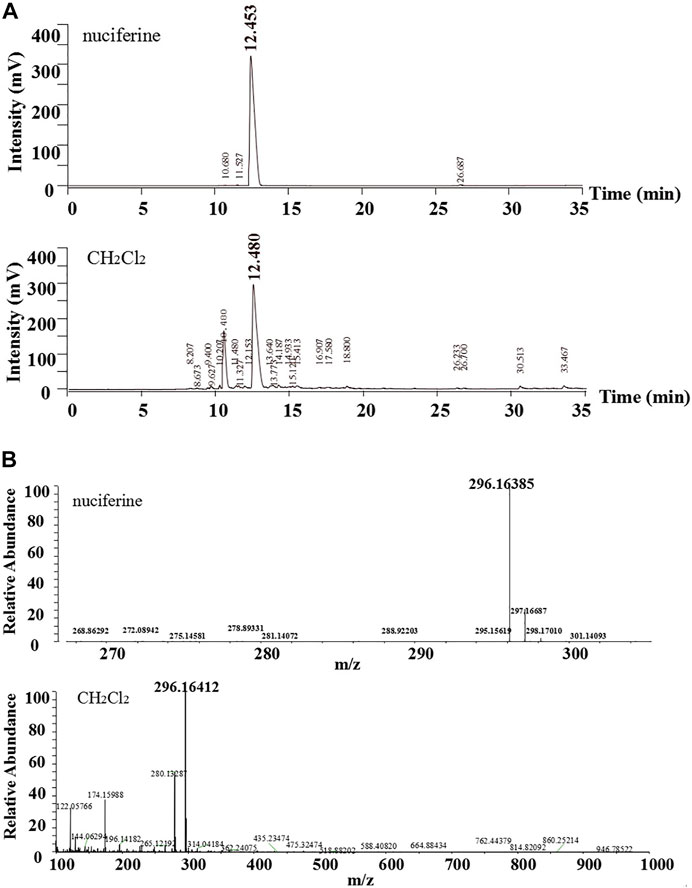
FIGURE 4. Comparative analysis of nuciferine standard substance and CH2Cl2 layer (A)HPLC; (B)ESI-HRMS.
3.4 Vascular reactivity assessment
3.4.1 Effect of nuciferine on rat thoracic aorta
Accumulation of nuciferine (0.03–2.4 μM) had no obvious effects on basal tension in rat thoracic aortic rings when compared to the vehicle control group (Figure 5).
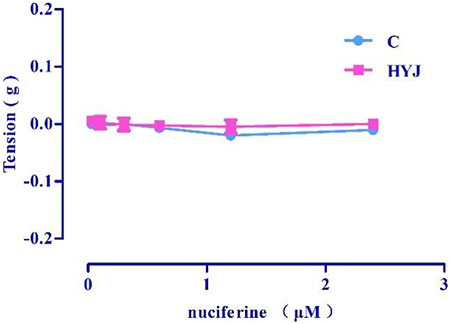
FIGURE 5. The effect of concentration-dependent addition of nuciferine on blood vessels. The data are expressed as the means ± SD of 6–8 rats (n = 6–8).
3.4.2 Nuciferine relaxes the pre-contracted PhE in rat thoracic aorta
Nuciferine exerted concentration-dependent vasorelaxant effect on PhE induced contraction in both endothelium-intact (Emax = 97.95 ± 0.76%, EC50 = 0.36 ± 0.02 μM) (Figure 6A) and endothelium-denuded (Emax = 75.42 ± 1.83%, EC50 = 1.30 ± 0.03 μM) (Figure 6B) arteries. Therefore, the vasodilatory activity of nuciferine is thought to be related to the endothelium (Figure 6C).
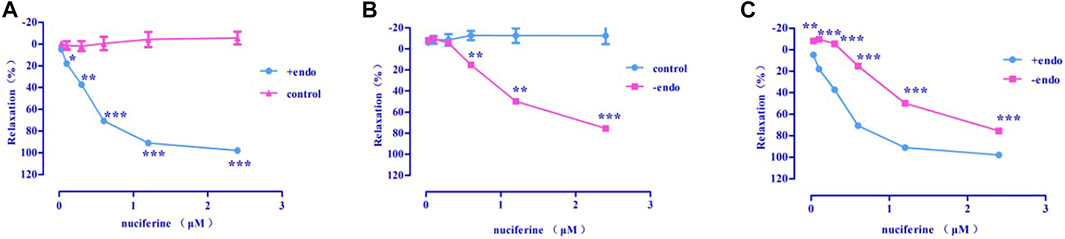
FIGURE 6. The vasodilation effect of nuciferine (A) Intact endothelium (+endo); (B). Endothelium-denuded (-endo); (C) ±endo. The data are expressed as the means ± SD. of 6–8 rats (n = 6–8). (*p < 0.05; **p < 0.01 and ***p < 0.001 vs. control group).
3.4.3 Examination of α-, β-, and M-receptor activities
3.4.3.1 Examination of α-receptor activity
Pre-incubation with various concentrations of nuciferine (0.6, 1.2 μM) significantly inhibited the concentration-response contraction induced by PhE (0.1 nm–10 μM), and suppressed its maximal contraction (Emax) to 90.73 ± 3.26% and 69.39 ± 1.59%, respectively (vs. control group Emax = 100%) (Figure 7A). The vasodilatory activity of nuciferine is thought to be related to the α-receptor activity.
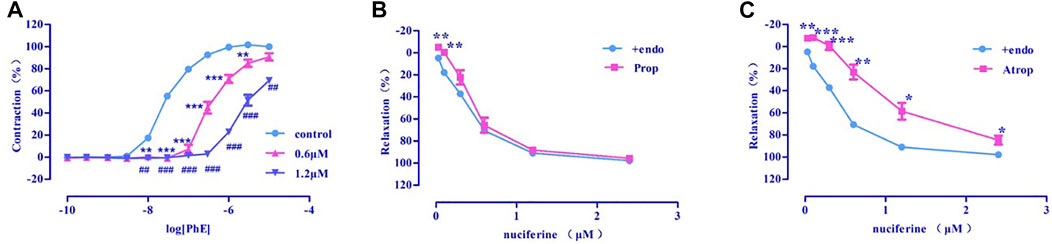
FIGURE 7. (A) α-receptor. (B) β receptor. (C) M receptor. The data are expressed as the means ± SD of 6–8 rats (n = 6–8). (*p < 0.05, **p < 0.01 and ***p < 0.001 vs. control group; #p < 0.05, ##p < 0.01 and ###p < 0.001 vs. control group).
3.4.3.2 Examination of β- and M-receptor activities
There was no significant difference between propranolol pre-incubated vascular ring (Emax = 95.66 ± 1.30%, EC50 = 0.48 ± 0.02 μM) (Figure 7B) and the control group (Emax = 97.95 ± 0.76%, EC50 = 0.36 ± 0.02 μM). The vasorelaxation induced by nuciferine is thought to be associated with M-receptor activity (Emax = 84.67 ± 4.06%, EC50 = 1.05 ± 0.03 μM) (Figure 7C).
3.4.4 Effects of PGI2 on nuciferine-induced relaxation
Indo (Emax = 92.98 ± 0.31%, EC50 = 0.71 ± 0.01 μM) (Figure 8) pretreatment weakened the relaxing effect of nuciferine. Therefore, the vasodilatory effect of nuciferine may be related to PGI2.
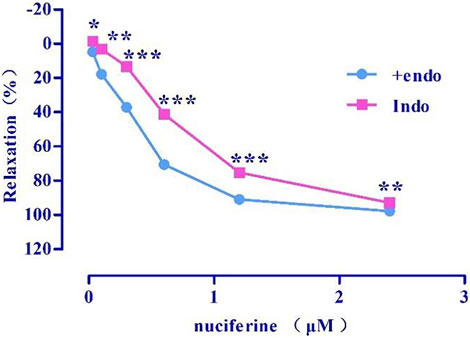
FIGURE 8. Effect of Indo on nuciferine induced vasorelaxation. The data are expressed as the means ± SD of 6–8 rats (n = 6–8). (*p < 0.05, **p < 0.01 and ***p < 0.001 vs. control group).
3.4.5 Effect of eNOS/sGC/cGMP on nuciferine-induced relaxation
After pretreatment with eNOS inhibitor L-NAME (Emax = 72.43 ± 5.00%, EC50 = 1.36 ± 0.04 μM) (Figure 9A) and sGC inhibitor ODQ (Emax = 39.01 ± 5.44%) (Figure 9B), the vasodilatory effect of nuciferine was found to be significantly reduced. The inhibitory effect of ODQ is stronger than that of L-NAME, and it is suspected that nuciferine can directly act on smooth muscle cells. The endothelium was pretreated with lotus leaf alkaline extract to remove the blood vessels, and then, the cGMP content was assessed. The results revealed that there were significant differences between the nuciferine group (5 μM, 2.06 ± 0.24 pmol/ml; 10 μM, 1.88 ± 0.10 pmol/ml) and the control group (1.30 ± 0.05 pmol/ml). The cGMP content of the positive control SNP was 3.23 ± 0.16 pmol/ml (10 μM) (Figure 9C). The vasodilatory effect of nuciferine is thought to be related to the eNOS/sGC/cGMP signaling pathway. The cGMP results also proved that lotus leaf can directly activate sGC in smooth muscle cells to increase the production of cGMP.
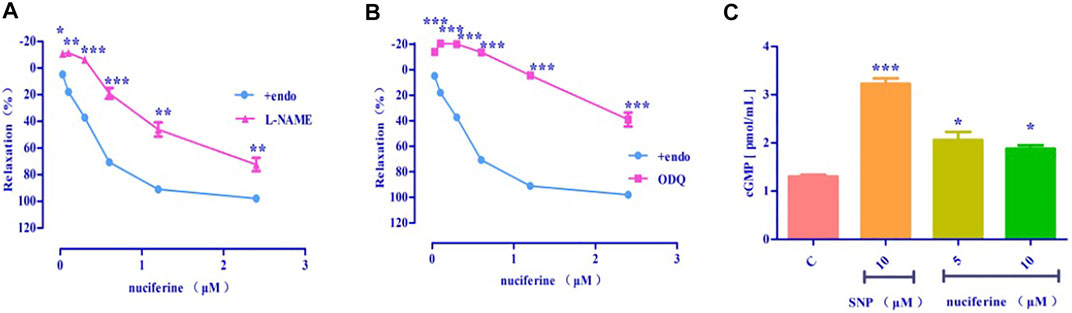
FIGURE 9. Effect of eNOS/sGC/cGMP on nuciferine-induced relaxation (A) L-NAME blocking effect; (B) ODQ blocking effect; (C) Detection of cGMP content. The data are expressed as the means ± SD of 6–8 rats (n = 6–8). (*p < 0.05, **p < 0.01 and ***p < 0.001 vs. control group).
3.4.6 Examination of K+ channel effect
Four types of selective K+ channel blockers, including BaCl2 (Emax = 1.73 ± 2.48%), Gli (Emax = 60.23 ± 2.30%, EC50 = 1.97 ± 0.01 μM), TEA (Emax = 74.93 ± 0.47%, EC50 = 1.63 ± 0.04 μM), and 4-AP (Emax = 75.80 ± 1.60%, EC50 = 1.50 ± 0.02 μM) were added into the incubation solution to obtain endothelium-denuded strips (Emax = 75.42 ± 1.83%, EC50 = 1.30 ± 0.03 μM) separately. The results revealed that all these K+ channel blockers affected the nuciferine-induced relaxation (Figures 10A,B), especially BaCl2.
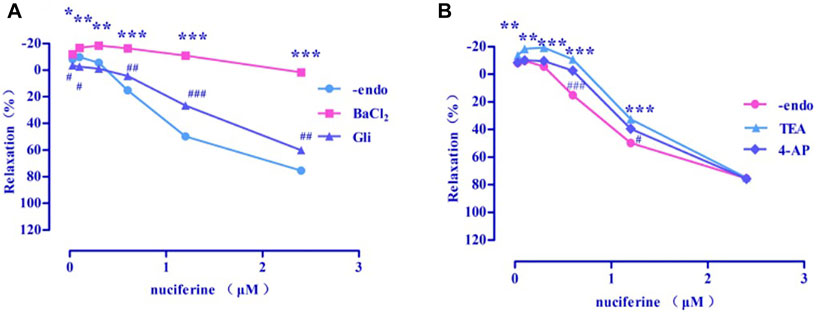
FIGURE 10. Effects of K+ channel blockers on nuciferine induced vasorelaxation. The data are expressed as the means ± SD of 6–8 rats (n = 6–8). (*p < 0.05, **p < 0.01 and ***p < 0.001 vs. control group; #p < 0.05, ##p < 0.01 and ###p < 0.001 vs. control group).
3.4.7 Effect of sarcoplasmic reticulum calcium release
The endothelium-denuded strips were incubated in a Ca2+-free solution and contracted by PhE, which promoted calcium release from the SR. The pre-treatment with nuciferine (0.1, 0.3, and 0.6 μM) significantly affected the PE-mediated vasocontraction (Figure 11). This result suggested that nuciferine interferes with SR Ca2+ release for its vasodilation upon considering the control group as 100%.
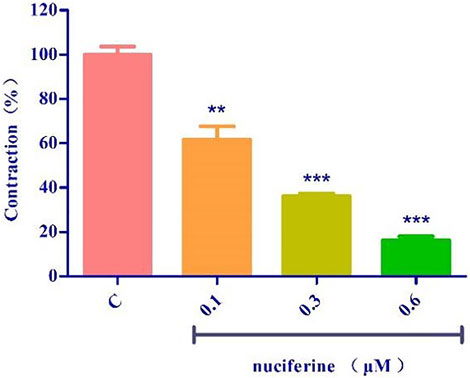
FIGURE 11. Effects of SR calcium release on nuciferine induced vasorelaxation. The data are expressed as the means ± SD of 6–8 rats (n = 6–8). (**p < 0.01 and ***p < 0.001 vs. control group).
3.4.8 Effect of extracellular Ca2+ influx on vasorelaxation by nuciferine
3.4.8.1 Examination of ROCCs effect
PhE promotes Ca2+ influx via ROCCs. The results revealed that nuciferine (0.3 and 0.6 μM) affected the contraction induced by CaCl2 + PhE (Figure 12A), suggesting that the relaxation by nuciferine might be related to the ROCCs.
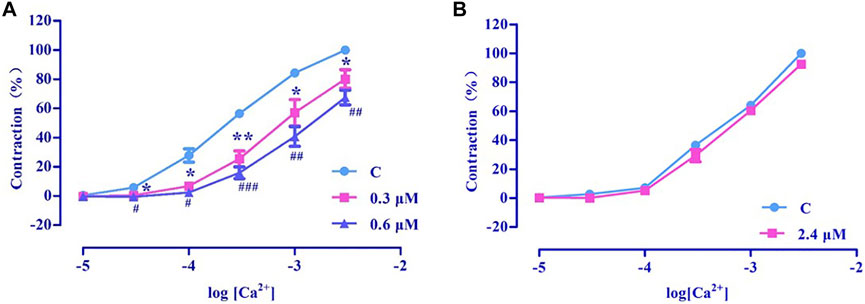
FIGURE 12. Effects of extracellular Ca2+ influx on nuciferine induced vasorelaxation. The data are expressed as the means ± SD of 6–8 rats (n = 6–8). (*p < 0.05 and **p < 0.01 vs. control group; #p < 0.05, ##p < 0.01 and ###p < 0.001 vs. control group).
3.4.8.2 Examination of VDCCs effect
High concentration of KCl promoted Ca2+ influx via VDCCs. The results revealed that 2.4 μM nuciferine did not affect the contraction induced by CaCl2 + KCl (Emax = 91.68 ± 1.01%) (Figure 12B), thereby suggesting that the relaxation by nuciferine might not be related to VDCCs.
3.5 Cell viability assay
Pretreatment with nuciferine (1–100 μM) for 24 h did not result in significant difference between the control group (Figure 13).
3.6 Vascular protection effect of nuciferine
TNF-α treatment increased the expression of cell adhesion molecules, such as ICAM-1 and E-selectin. Interestingly, pretreatment with nuciferine attenuated TNF-α-mediated cell adhesion molecules (Figure 14). Our results revealed that nuciferine may exert a vascular protective effect.
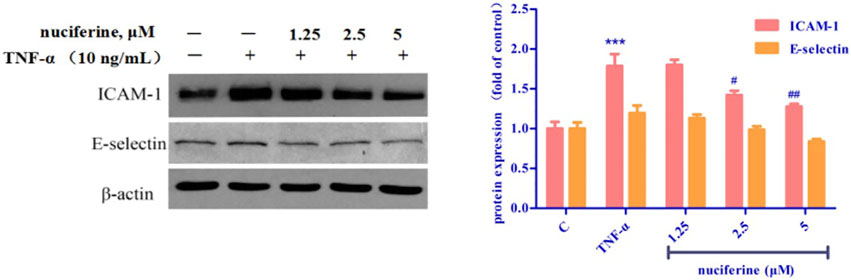
FIGURE 14. Effect of nuciferine on TNF-α-induced expression of adhesion molecules in HUVECs. (*p < 0.01 and ***p < 0.001 vs. control group).
4 Discussion
Lotus leaves contain alkaloids, flavonoids, polysaccharides, and other ingredients (Nguyen and Pham, 1998; Ma et al., 2010; Chen and Qi, 2015; Tungmunnithum et al., 2018; Ye et al., 2018). Different ratios of water and ethanol were used to obtain the extracts, and the 75% ethanol extract was found to exert a strong vasodilator effect. The 75% ethanol extract was further subjected to polarity-dependent extraction, and it was found that the CH2Cl2 layer exhibited the highest vasodilatory activity. Therefore, the component analysis of the CH2Cl2 layer was carried out, and the components that accounted for about half of the entire peak area in the liquid phase analysis were separated by preparative liquid phase. The chromatographic characterization and the comparative analysis of liquid phase/mass spectrometry confirmed that the substance was nuciferine, which was consistent with previous research results that most of the alkaloids were dissolved in the dichloromethane layer (Wang et al., 2008) and nuciferine was the most abundant alkaloid in lotus leaf (Ma et al., 2010). Before this, some scholars have studied the relaxation mechanism of nuciferine on mesenteric arteries (Wang X. et al., 2015) and trachea (Yang et al., 2018). With this, we believe that nuciferine is the main component present in lotus leaves with vasodilator activity. Therefore, the thoracic aorta activity of nuciferine was further studied. In this study, we aimed to provide a basis for future studies on the vasodilatory activity of lotus leaf extracts.
As shown in Figure 6, nuciferine was found to directly act on the rat thoracic aorta without pretreatment, and there was no significant effect. The vasodilatory effect of nuciferine is more related to the endothelium. The vascular endothelium produces vasodilatory factors, such as NO and PGI2 (Yam et al., 2016). When eNOS is activated, L-arginine is converted into NO in vascular endothelial cells. NO penetrates the endothelium and enters the smooth muscle cells, and then, activates the soluble guanylate cyclase, thereby increasing the intracellular levels of cGMP, which is synthesized from guanosine triphosphate (Qu et al., 2015; Kubacka et al., 2018). The thoracic aortic vasorelaxant effect of nuciferine was also blocked in the L-NAME and ODQ experiments, which is consistent with the trend of nuciferine to relax rat mesenteric arteries (Wang X. et al., 2015). But in the thoracic aorta experiment, the blocking effect of ODQ was more obvious. Based on the above phenomenon, nuciferine may directly activate sGCs in smooth muscle cells to promote cGMP production. The nitric oxide donor SNP was selected as a positive control, and nuciferine directly treated smooth muscle cells. The detection of cGMP content in vessels found that nuciferine could directly act on smooth muscle cells, then relate vessels. In conclusion, the vasodilatory effect of nuciferine may be related to the NO signaling pathway.
As another endogenous vasodilator prostacyclin (PGI2) synthesized and released by endothelial cells, both PGI2 and NO act directly on smooth muscle cells. However, the mechanism of action of the two is slightly different. PGI2 binds to smooth muscle cell membrane receptors and promotes the production of cAMP to relax vessels (Moncada et al., 1976; Gomberg-Maitland and Olschewski, 2008). Arachidonic acid (AA) is converted to PGI2 by COX (Yam et al., 2016). In the present study, Indo was found to partially inhibit the relaxation effect of nuciferine. This is different from previous findings that the relaxation of mesenteric arteries by nuciferine is independent of PGI2 (Wang X. et al., 2015). This suggested that the relaxation mechanism of nuciferine in the thoracic aorta and mesenteric arteries was different, and proved that the relaxation of the thoracic aorta by nuciferine may be related to the PGI2 signaling pathway.
Ions play an important role in maintaining blood vessel homeostasis (Qu et al., 2015; Hu et al., 2018). We found that four different types of potassium channels are related to the vasodilatory effect of nuciferine, especially the inwardly rectifying potassium channels. In most cases, the contraction of the vascular smooth muscle is related to an increase in [Ca2+] ions through the influx of extracellular Ca2+ and the release of intracellular Ca2+. The influx of extracellular calcium is mainly through the VDCCs and ROCCs located in the cell membrane. The KCl-induced contraction is mainly caused by the depolarization and opening of the VDCCs membrane, which drives the influx of extracellular Ca2+. PhE leads to the contractions in response to the extracellular Ca2+ influx upon direct activation of ROCCs. We found that the vasodilatory effect of nuciferine is related to the ROCCs pathway of intracellular calcium release and extracellular calcium influx (Kim et al., 2015; Lee et al., 2015; Kim et al., 2019).
Nuciferine relaxes the thoracic aorta through endothelium-dependent and -independent mechanisms. Therefore, nuciferine may act directly on endothelial cells and smooth muscle cells. α- and β-receptors are widely distributed in vascular smooth muscle cells, and muscarinic (M) receptors are distributed in the vascular endothelium. Stimulation of α-receptor results in vasoconstriction, and M- and β-adrenergic receptors are involved in vasodilation (Chiu et al., 2004; Yam et al., 2016). β-adrenergic receptors were not involved in the vasodilatory effect of nuciferine, which was consistent with mesenteric arteries findings (Wang X. et al., 2015). The present study demonstrates that the vasodilatory effects of nuciferine are associated with α- and M-receptors.
A variety of cardiovascular diseases such as hypertension, atherosclerosis and heart failure are often accompanied by endothelial dysfunction (Vanhoutte, 1996). TNF-α is reported to trigger the interaction between monocytes and vascular endothelial cells, enhance the expression of adhesion molecules, such as VCAM-1, ICAM-1, and E-selectin, and finally induce endothelial dysfunction (Yao et al., 2015; Zhou et al., 2017). Nuciferine did not show cytotoxicity at the concentration of 0–100 μM, and the results of western blot experiments showed that nuciferine inhibits the production of vascular adhesion factors in a concentration-dependent manner and protects vessels.
In summary, nuciferine was the main substance with a vasodilatory effect in the lotus leaf extract. The vasodilatory effects of nuciferine were mediated by multiple pathways, multiple-targets and had both endothelium-dependent and -independent mechanisms. Nuciferine mainly mediated the NO/cGMP signaling pathway, activated the KIR channel, and inhibited ROCCs and SR Ca2+ release to relax thoracic aorta. Notably, Nuciferine has low cytotoxicity and endothelial protective effect, which was worth further development and research. This study provides a new research direction for the development and application of lotus leaf extract.
Data availability statement
The original contributions presented in the study are included in the article/Supplementary Material, further inquiries can be directed to the corresponding authors.
Ethics statement
The animal study was reviewed and approved by the Animals were strictly handled in accordance with the National Institutes of Health Guidelines for the Care and Use of Laboratory Animals (NIH Publication No. 85-3, revised 1996) and the study was approved by the Institutional Animal Care and Utilization Committee of Yanbian University.
Author contributions
Z-SQ, HD, and L-HC designed the study. HD, QX, XH, and X-TS performed the experiments. L-LJ, F-EC, and Q-KS analyzed and interpreted the results. DH and XQ wrote the manuscript.
Funding
This work was financially supported by the Higher Education Discipline Innovation Project (111 Project, D18012), National Natural Science Foundation of China (No.81960626, 82060628), Jilin Provincial Education Department of China (JJKH20191156KJ), Doctoral Research Startup Fundation of Yanbian University (No. 602020087) and the Natural Science Foundation of Jilin Province (No.20210101242JC).
Acknowledgments
All authors approved the final manuscript. We would like to thank Editage (www.editage.cn) for English language editing.
Conflict of interest
The authors declare that the research was conducted in the absence of any commercial or financial relationships that could be construed as a potential conflict of interest.
Publisher’s note
All claims expressed in this article are solely those of the authors and do not necessarily represent those of their affiliated organizations, or those of the publisher, the editors and the reviewers. Any product that may be evaluated in this article, or claim that may be made by its manufacturer, is not guaranteed or endorsed by the publisher.
Abbreviations
Ach, acetylcholine chloride; PhE, phenylephrine HCl; MS, mass spectrometry; HPLC, high-performance liquid chromatography; PE, petroleum ether; CH2Cl2, dichloromethane; EA, ethyl acetate; PGI2, prostacyclin; NO, nitric oxide; SNP, sodium nitroprusside; IBMX, 3-isobutyl-1-methylxanthine; cGMP, guanosine 3′,5′-cyclic monophosphate; AA, arachidonic acid; COX, cyclooxygenase; VDCCs, voltage-dependent Ca2+ channels; ROCCs, receptor-operated Ca2+ channels; TNF-α, tumor necrosis factor-α; Modulators (Table 3).
Supplementary material
The Supplementary Material for this article can be found online at: https://www.frontiersin.org/articles/10.3389/fphar.2022.946445/full#supplementary-material
References
Cao, L. H., Lee, H. S., Lee, Y. J., Lee, H. S., Lee, Y. J., Quan, Z. S., et al. (2020). Vascular protective effects of xanthotoxin and its action mechanism in rat aorta and human vascular endothelial cells. J. Vasc. Res. 57 (6), 313–324. doi:10.1159/000509112
Chai, J. Z., Jiang, L., Wei, J. Q., and Yin, G. L. (2016). Research progress of hypolipidemic and antioxidation effect of the lotus leaf. Shipin Yanjiu Yu Kaifa 37 (8), 209–212. doi:10.3969/j.issn.1005-6521.2016.08.049
Chen, H., and Zeng, Q. (2001). Extraction of functional component from lotus leaves and effect on scavenging of oxygen free radicals. Shipin Yu Fajiao Gongye 27, 34–38. doi:10.13995/j.cnki.11-1802/ts.2001.10.008
Chen, X., and Qi, J. (2015). Flavonoids and alkaloids in lotus leaves. Zhongguo Shiyan Fangjixue Zazhi 21 (18), 211–214. doi:10.13422/j.cnki.syfjx.2015180211
Chen, Y., Chen, Q., Wang, X., Sun, F., Fan, Y., Liu, X., et al. (2020). Hemostatic action of lotus leaf charcoal is probably due to transformation of flavonol aglycons from flavonol glycosides in traditional Chinses medicine. J. Ethnopharmacol. 249, 112364. doi:10.1016/j.jep.2019.112364
Chiu, C. C., Wu, J. R., Lee, C. H., Liou, S. F., Dai, Z. K., Chen, I. J., et al. (2004). Anti-hypertension effect of vanylidilol: A phenylaldehyde alpha/beta-adrenoceptor blocker with endothelium-dependent and K+ channels opening-associated vasorelaxant activities. Pharmacology 70 (3), 140–151. doi:10.1159/000074977
Gomberg-Maitland, M., and Olschewski, H. (2008). Prostacyclin therapies for the treatment of pulmonary arterial hypertension. Eur. Respir. J. 31 (4), 891–901. doi:10.1183/09031936.00097107
Guo, C., Zhang, N., Liu, C., Xue, J., Chu, J., and Yao, X. (2020). Qualities and antioxidant activities of lotus leaf affected by different drying methods. Acta Physiol. Plant. 42 (2), 14–18. doi:10.1007/s11738-019-2992-9
Guo, F., Yang, X., Li, X., Feng, R., Guan, C., Wang, Y., et al. (2013). Nuciferine prevents hepatic steatosis and injury induced by a high-fat diet in hamsters. PLoS One 8 (5), e63770. doi:10.1371/journal.pone.0063770
Hu, G. Y., Peng, C., Xie, X. F., Xiong, L., Cao, X. Y., and Zhang, S. Y. (2018). Patchouli alcohol isolated from Pogostemon cablin mediates endothelium-independent vasorelaxation by blockade of Ca2+ channels in rat isolated thoracic aorta. J. Ethnopharmacol. 220, 188–196. doi:10.1016/j.jep.2017.09.036
Kim, B., Lee, K., Chinannai, K. S., Ham, I., Bu, Y., Kim, H., et al. (2015). Endothelium-independent vasorelaxant effect of Ligusticum jeholense root and rhizoma on rat thoracic aorta. Molecules 20 (6), 10721–10733. doi:10.3390/molecules200610721
Kim, B., Ma, S. S., Jo, C., Lee, S., Choi, H., Lee, K., et al. (2019). Vasorelaxant effect of the ethanol extract from Valeriana fauriei briquet root and rhizome on rat thoracic aorta. Pharmacogn. Mag. 14 (60), 59–65. doi:10.4103/pm.pm_152_18
Kubacka, M., Kotanska, M., Kazek, G., Waszkielewicz, A. M., Marona, H., Filipek, B., et al. (2018). Involvement of the NO/sGC/cGMP/K+ channels pathway in vascular relaxation evoked by two non-quinazoline α1-adrenoceptor antagonists. Biomed. Pharmacother. 103, 157–166. doi:10.1016/j.biopha.2018.04.034
Lee, K., Shin, M. S., Ham, I., and Choi, H. Y. (2015). Investigation of the mechanisms of Angelica dahurica root extract-induced vasorelaxation in isolated rat aortic rings. BMC Complement. Altern. Med. 15, 395–398. doi:10.1186/s12906-015-0889-8
Liu, W. J., and Fan, L. P. (2014). Comparison of different extraction technology of lotus leaf tea. Shipin Gongye Keji 35 (14), 305314–306308.
Ma, C., Li, G., He, Y., Xu, B., Mi, X., Wang, H., et al. (2015). Pronuciferine and nuciferine inhibit lipogenesis in 3T3-L1 adipocytes by activating the AMPK signaling pathway. Life Sci. 136, 120–125. doi:10.1016/j.lfs.2015.07.001
Ma, W., Lu, Y., Hu, R., Chen, J., Zhang, Z., and Pan, Y. (2010). Application of ionic liquids based microwave-assisted extraction of three alkaloids N-nornuciferine, O-nornuciferine, and nuciferine from lotus leaf. Talanta 80 (3), 1292–1297. doi:10.1016/j.talanta.2009.09.027
Moncada, S., Gryglewski, R., Bunting, S., and Vane, J. R. (1976). An enzyme isolated from arteries transforms prostaglandin endoperoxides to an unstable substance that inhibits platelet aggregation. Nature 263 (5579), 663–665. doi:10.1038/263663a0
Navaneethabalakrishnan, S., Goodlett, B. L., Lopez, A. H., Rutkowski, J. M., and Mitchell, B. M. (2020). Hypertension and reproductive dysfunction: A possible role of inflammation and inflammation-associated lymphangiogenesis in gonads. Clin. Sci. 134 (24), 3237–3257. doi:10.1042/CS20201023
Nguyen, T., and Pham, T. K. (1998). Study on chemical constituents in leaves of Nelumbo nucifera Gaertn. Pharm. J. 272 (12), 13–18.
Qiu, M., Xiao, F., Wang, T., Zhao, W., Shao, S., Piao, S., et al. (2020). Protective effect of Hedansanqi Tiaozhi Tang against non-alcoholic fatty liver disease in vitro and in vivo through activating Nrf2/HO-1 antioxidant signaling pathway. Phytomedicine 67, 153140. doi:10.1016/j.phymed.2019.153140
Qu, Z., Zhang, J., Huo, L., Chen, H., Li, H., Fan, Y., et al. (2015). Antihypertensive and vasorelaxant effects of Rhizoma corydalis and its active component tetrahydropalmatine via NO/cGMP pathway and calcium channel blockade in isolated rat thoracic aorta. RSC Adv. 5 (114), 94130–94143. doi:10.1039/c5ra17756a
Tungmunnithum, D., Pinthong, D., Hano, C., and Hano, C. (2018). Flavonoids from Nelumbo nucifera gaertn., a medicinal plant: Uses in traditional medicine, phytochemistry and pharmacological activities. Med. (Basel) 5 (4), 127. doi:10.3390/medicines5040127
Vanhoutte, P. M. (1996). Endothelium-dependent responses and inhibition of angiotensin-converting enzyme. Clin. Exp. Pharmacol. Physiol. 23 (8), 23–29. doi:10.1111/j.1440-1681.1996.tb03037.x
Wang, G., Liu, K., Li, Y., Yi, W., Yang, Y., Zhao, D., et al. (2014). Endoplasmic reticulum stress mediates the anti-inflammatory effect of ethyl pyruvate in endothelial cells. PLoS One 9 (12), e113983. doi:10.1371/journal.pone.0113983
Wang, G., Zhang, B., Nie, Q., Li, H., and Zang, C. (2008). Optimal extraction of nuciferine and flavone from lotus leaf based on central composite design and response surface methodology. Zhongguo Zhongyao Zazhi 33 (20), 2332–2335.
Wang, J. T., Kong, Z., Yan, Y. M., Qin, M., Gao, G. L., Yu, X. M., et al. (2007). Study on the antibacterial activity of isoquinoline alkaloids by microcalorimetry. Huaxue Shijie 48, 460–463. doi:10.19500/j.cnki.0367-6358.2007.08.004
Wang, M. X., Liu, Y. L., Yang, Y., Zhang, D. M., and Kong, L. D. (2015). Nuciferine restores potassium oxonate-induced hyperuricemia and kidney inflammation in mice. Eur. J. Pharmacol. 747, 59–70. doi:10.1016/j.ejphar.2014.11.035
Wang, X., Cheang, W. S., Yang, H., Xiao, L., Lai, B., Zhang, M., et al. (2015). Nuciferine relaxes rat mesenteric arteries through endothelium-dependent and -independent mechanisms. Br. J. Pharmacol. 172 (23), 5609–5618. doi:10.1111/bph.13021
Wang, Z., Zhao, P., Zhang, Y., Shi, S., and Chen, X. (2020). The hepatoprotective effect and mechanism of lotus leaf on liver injury induced by Genkwa Flos. J. Pharm. Pharmacol. 72 (12), 1909–1920. doi:10.1111/jphp.13355
Yam, M. F., Tan, C. S., Ahmad, M., and Ruan, S. (2016). Mechanism of vasorelaxation induced by eupatorin in the rats aortic ring. Eur. J. Pharmacol. 789, 27–36. doi:10.1016/j.ejphar.2016.06.047
Yang, S., Xu, Z., Lin, C., Li, H., Sun, J., Chen, J., et al. (2020). Schisantherin A causes endothelium-dependent and -independent vasorelaxation in isolated rat thoracic aorta. Life Sci. 245, 117357. doi:10.1016/j.lfs.2020.117357
Yang, X., Yu, M. F., Lei, J., Peng, Y. B., Zhao, P., Xue, L., et al. (2018). Nuciferine relaxes tracheal rings via the blockade of VDLCC and NSCC channels. Planta Med. 84 (2), 83–90. doi:10.1055/s-0043-118178
Yao, W., Gu, C., Shao, H., Meng, G., Wang, H., Jing, X., et al. (2015). Tetrahydroxystilbene glucoside improves TNF-α-induced endothelial dysfunction: Involvement of tgfβ/smad pathway and inhibition of vimentin expression. Am. J. Chin. Med. 43 (1), 183–198. doi:10.1142/S0192415X15500123
Ye, L. H., He, X. X., Kong, L. T., Liao, Y. H., Pan, R. L., Xiao, B. X., et al. (2014). Identification and characterization of potent CYP2D6 inhibitors in lotus leaves. J. Ethnopharmacol. 153 (1), 190–196. doi:10.1016/j.jep.2014.02.014
Ye, L. H., He, X. X., Tao, X., Wang, L. S., Zhang, M. D., Zhou, Y. F., et al. (2018). Pharmacokinetics of nuciferine and N-nornuciferine, two major alkaloids from Nelumbo nucifera leaves, in rat plasma and the brain. Front. Pharmacol. 9, 902. doi:10.3389/fphar.2018.00902
Yuan, P. L., Chen, L., Liu, X. Y., Yu, B. W., Huang, Q., Chen, K. X., et al. (2014). Alkaloids from lotus leaf and their bioactivities. Zhongchengyao 36 (11), 2330–2333. doi:10.3969/j.issn.1001-1528.2014.11.024
Keywords: lotus leaves, nuciferine, vasodilation, thoracic aorta, HUVECs
Citation: Deng H, Xu Q, Sang X-T, Huang X, Jin L-L, Chen F-E, Shen Q-K, Quan Z-S and Cao L-H (2022) Study on the vasodilatory activity of lotus leaf extract and its representative substance nuciferine on thoracic aorta in rats. Front. Pharmacol. 13:946445. doi: 10.3389/fphar.2022.946445
Received: 17 May 2022; Accepted: 24 August 2022;
Published: 05 October 2022.
Edited by:
Fouad Antoine Zouein, American University of Beirut, LebanonReviewed by:
Immanuel Selvaraj C, VIT University, IndiaDuangjai Tungmunnithum, Mahidol University, Thailand
Copyright © 2022 Deng, Xu, Sang, Huang, Jin, Chen, Shen, Quan and Cao. This is an open-access article distributed under the terms of the Creative Commons Attribution License (CC BY). The use, distribution or reproduction in other forums is permitted, provided the original author(s) and the copyright owner(s) are credited and that the original publication in this journal is cited, in accordance with accepted academic practice. No use, distribution or reproduction is permitted which does not comply with these terms.
*Correspondence: Qing-Kun Shen, qkshen@ybu.edu.cn; Li-Hua Cao, lhcao@ybu.edu.cn
†These authors have contributed equally to this work