- College of Pharmacy, Beihua University, Jilin, China
Anwulignan is a representative component of Chinese traditional medicine Schisandra sphenanthera, with strong pharmacological activities. However, there are few reports on its pharmacokinetics and metabolites in the body. In this study, a metabolomic method based on UHPLC-Q-Orbitrap-MS was used to study the pharmacokinetics of anwulignan in the blood, organs, urine, and feces samples of mice after the intragastric administration of anwulignan (10 mg/kg). The pharmacokinetic parameters were calculated, and the distribution characteristics and main metabolites of anwulignan in the body of mice were analyzed. The results showed that the retention time of anwulignan in the body of mice was longer (t1/2 = 7.1 h), and anwulignan was widely distributed in the body (Vz/F = 32.81 L/kg), especially in the liver. The order of anwulignan concentration in the tissues of mice from high to low was the liver > heart > brain > kidney > lung > spleen. Anwulignan was mainly excreted through the digestive tract in the form of its prototype and metabolites, indicating that it might experience an enterohepatic circulation. A total of seven metabolites were identified, and the demethylation, hydroxylation, dehydroxylation, and demethoxylation were considered to be the main metabolic ways of anwulignan in the body of mice.
Introduction
Schisandra sphenanthera, the mature and dry fruit of S. sphenanthera Rehd. Et Wils, has been used as a representative tonic drug in China for 2,000 years (Jiang et al., 2019). The active components of S. sphenanthera include lignans, volatile oil, organic acids, and polysaccharides, among which lignans are the main active components, with some pharmacological effects, such as bacteriostasis, sedation and hypnosis, anti-aging, anti-oxidation, and hepatoprotection (Jiang et al., 2016; Zhang et al., 2021). Wuzhi Tablets, an alcohol-extracted preparation of S. sphenanthera, is used for the treatment of hepatitis and liver insufficiency caused by viruses and drugs in clinic (Chen et al., 2019). Anwulignan is a representative lignan component of S. sphenanthera and a characteristic component for the identification of S. sphenanthera, and also one of the main components in Wuzhi Tablets (Lu and Liu, 1992; Yang et al., 2014). However, there are few reports on the efficacy of anwulignan. It was found in our previous study that anwulignan, with a good antioxidant effect, could significantly alleviate the liver injury induced by intestinal ischemia reperfusion in rats (Lin et al., 2020), regulate the immune function of aging mice induced by d-galactose by activating Nrf2/ARE pathway and regulating caspase-3, BCL2, and Bax (Li et al., 2020), and alleviate the heart injury induced by oxidative stress (Liu et al., 2020a), with an anti-fatigue effect (Liu et al., 2020b; Zhang et al., 2020), demonstrating that it is worthy of further development and utilization. The pharmacokinetic study of anwulignan is particularly important to clarify the pharmacological mechanism of anwulignan in vivo, and not only the pharmacological effect of anwulignan on the human body can be observed but also more appropriate administration dosage and method can be found according to the absorption, distribution, and excretion law of anwulignan in various tissues and organs in the study so as to achieve the best curative effect. Therefore, it has a broad prospect for clinical research and social application to explore the action mechanism and potential targets of anwulignan based on pharmacokinetics. However, there are few studies on the pharmacokinetics of anwulignan, especially its biotransformation process in vivo.
The analysis of anwulignan is mainly carried out by HPLC and UV methods, and both of them are used for the study on the determination of its in vitro content. The main purpose of ultra-high-performance liquid chromatography–quadrupole orbitrap mass spectrometry (UHPLC-Q-Orbitrap-MS) analysis is to provide a quantitative method for candidate drugs, so as to obtain accurate and reliable conclusions in the study of pharmacokinetics. In this study, UHPLC-Q-Orbitrap-MS was used to analyze the pharmacokinetic characteristics, the distribution in tissues, and the main metabolites of anwulignan in the body of mice to seek the potentially active metabolites of anwulignan in vivo, which was expected to provide support in data and a reference for the pharmacodynamic material basis of anwulignan as a candidate drug.
Materials and Methods
Chemicals and Reagents
The materials used in this study were as follows: anwulignan and schisantherin A (>98.0%, Chengdu Preferred Biotechnology Limited Company, Chengdu, Sichuan, China); methanol and ethyl acetate (HPLC–grade, TEDIA, Cincinnati, OH, United States); and de-ionized water (prepared by a Milli-Q Water purification system, Millipore, MA, United States).
Preparation of Plasma and Tissue Standards
Male ICR mice (18–22 g, Chang Chun Yisi Experimental Animal Technology Co., Ltd.) were used for experiments after the isolation and adaptation in our laboratory for 1 week. The mice were raised under the standard laboratory conditions for 1 week before experiments, with a free access to water and food. All experimental procedures were approved by Beihua University Laboratory Animal Ethics Committee (No. 20190907) and performed in accordance with the Guide for the Care and Use of Laboratory Animals.
Using methanol as the solvent, the concentration of anwulignan standard and schisandrin A (internal standard, IS) was 100 and 4 μg/ml, respectively. The different volumes of anwulignan standard stock solution and 200 μl schisandrin A were added into 200 μl of blank plasma of mice (or heart, liver, spleen, lung, kidney, and brain tissue homogenate, and a normal saline homogenate at the ratio of 1:9, w/v), which were mixed evenly. The solutions were centrifuged at 3,000 rpm for 10 min, then added with double volumes of methanol and swirled for 30 s, and centrifuged at 13,000 rpm for 10 min for obtaining the supernatants. The supernatants were blown dry until no liquid in them was found. The dried supernatant sentiments were dissolved in methanol up to a volume of 2 ml, respectively, and the solutions were swirled for 30 s and then filtered through a filter with a diameter of 0.45 μm into 1.5-ml sample bottles. Finally, the plasma (tissue) standard solutions at the final concentrations of anwulignan of 0.01, 0.02, 0.05, 0.1, 0.2, 0.5, 1, 2, and 5 μg/ml were prepared, respectively, and the concentration of schisandrin A in each sample was 4 μg/ml. A volume of 10 μl of each sample was analyzed by LC/MS to obtain the calibration curve (repeated three times). The 0.05, 0.5, and 5 μg/ml plasma (tissue) standard solutions were selected as the quality control (QC) samples for the method validation in this study.
Administration of Anwulignan and Collection and Preparation of Plasma and Organ Samples
Carboxymethylcellulose sodium (CMC-Na, 0.5 g) was dissolved in 1,000 ml distilled water, and 10 mg anwulignan was dissolved in 10 ml of the CMC-Na solution prepared above, at the concentration of 1 mg/ml. Sixty mice were fasted for 12 h, and then intragastrically administered with 10 mg/kg anwulignan. At 0.5, 1, 1.5, 2, 3, 4, 6, 8, 12, 24, 36, and 48 h after the administration of anwulignan, five mice were randomly selected at each time point for the preparation of homogenates. The selected mice were placed in a desiccator in which 10 ml ether was placed at the lower part. After the mice were anesthetized (about 0.5 min), the blood samples of mice were collected by removing eyeballs, and then their heart, liver, spleen, lung, kidney, and brain tissues were taken. The blood plasma and tissue homogenates were prepared according to the method in the preparation of plasma and tissue standards, and 200 μl of schisandrin A (4 μg/ml) was added into each of the plasma and tissue homogenates for the preparation of the samples to be tested.
Collection and Preparation of Urine and Feces Samples of Mice After the Intragastric Administration
Five mice were selected and intragastrically administered with anwulignan (10 mg/kg), and then the mice were placed in a mouse metabolism cage and fasted, but with a free access to water. The urine and feces were collected during 0–2 h, 2–4 h, 4–8 h, 8–12 h, 12–24 h, and 24–48 h after the administration of anwulignan, respectively. The urine samples were blown dry, and the concentrated urine was extracted three times with an appropriate amount of ethyl acetate. The five extracts were mixed and blown dry with nitrogen, then the dried extract was added with 1 ml methanol and dissolved by vortexing. The extract-methanol solution was passed through a 0.45 μm microporous membrane and the filtrate was collected, and the volume of filtrate was adjusted to 2 ml with methanol for testing. Each of the collected feces samples was added with 5 ml of water and ground, and then added with 10 ml of ethyl acetate and extracted three times. The three extracts were mixed. The mixed extract was blown dry with nitrogen, and then the dried extract was dissolved in a small amount of methanol. The extract-methanol solution was filtered through a 0.45 μm microporous membrane, and the volume of the collected filtrate was adjusted to 2 ml with methanol for testing.
UHPLC-Q-Orbitrap-MS/MS Conditions
An ultimate 3,000 ultra-high-performance liquid chromatography system (Thermo, San Jose, CA, United States) was used for the separation by liquid chromatography. The separation column of HPLC was C18 column (100 mm × 30 mm, 18 μm), and the column temperature was set at 32°C and the absorbance at 230 nm. Methanol and water were used as mobile phase A and B, respectively. The gradient elution procedures were set as follows: 0–15 min (75%–90% A), 10–15 min (90%–100% A), and 15–18 min (100%–75% A). The flow rate was set at 1 ml/min and the injection volume was 10 μl. The UHPLC system was coupled to a Q-Orbitrap-MS/MS mass spectrometer.
Q-Orbitrap-MS/MS (Thermo, San Jose, CA, United States) was used for the mass spectrometry in positive-ion mode. The capillary voltage was set at +4.0 kV and the capillary temperature at 350°C. The parameters of the ion source were set as follows: the sheath gas flow was 40 Arb, the auxiliary gas flow 10 Arb, and the purge gas flow 1 Arb. A single-ion monitor (SIM) mode was used for the analysis of anwulignan and IS, and the full-scan MS data were used for the analysis of metabolites by setting the m/z as 150–2,000 Da, the resolution as 70,000, automatic gain control (AGC), the target value as 1 × 106, and the maximum injection time (IT) as 100 ms. Full-MS/ddMS2 mode was set to get MS/MS data, in which the resolution was 17,500, the AGC target was 1 × 105, the maximum IT was 50 ms, and the normalized collision energy (NCE) was 25–45.
Multivariate Data Analysis and Metabolite Identification
LC-MS data were extracted, filtered, and normalized using Thermo software Xcalibur (version 4.3) to obtain the molecular weight, retention time, and absorption peak area of the compounds in each sample. SIMCA 14.1 software (Umetrics, Kinnelon, NJ, United States) was used for the analysis of multivariate data, and principal component analysis (PCA) and orthogonal partial least squares discrimination analysis (OPLS-DA) were used to look for the differential compounds among the samples by analyzing the score charts and scatter plots. Xcalibur was used to compare the molecular weight, retention time, and molecular characterization of fragmented ions of the differential compounds by MS/MS mass spectrometry for their identification and structure elucidation.
Specificity
Six different batches of mouse blank plasma (tissues), and the blank plasma and tissue homogenates with anwulignan and IS were compared for the specificity investigation, in which whether the plasma could interfere with the retention time and peak area of anwulignan and IS was observed.
Standard Curve and Limit of Quantitation
The plasma (tissue) standard solution of anwulignan in each concentration gradient was selected, and the standard curve was obtained by plotting the relation curve between the peak area of anwulignan/IS and the concentration of anwulignan. The peak area of anwulignan was recorded, and the signal-to-noise ratio (SNR) 10:1 was used as the LOQ.
Precision and Accuracy
The precision and accuracy were evaluated by the intra-day analysis (three different concentrations of QC samples, six replicates for each concentration, and determined three times) and the inter-day analysis (consecutively for 3 days). The relative error (RE%) calculated based on the peak area should be less than 20%.
Matrix Effect and Stability
Three quality control samples at the different concentrations were detected by HPLC method, in which six parallel samples were set for each concentration, to obtain the peak area set 1. Thirty microliters of the above standard solution at three concentrations were added to 150 μl of the extracted blank mouse plasma and then 100 μl water was added to the standard plasma solution, well mixed by a vortex mixer, in which six parallel samples were set for each concentration, and the solution was detected to obtain the peak area set 2. The matrix effect was calculated according to the value of set 2/set 1. The stability of anwulignan was evaluated by analyzing six duplicate samples from each of the three quality control samples at the different concentrations under the different conditions, including placing at room temperature for 4 h, freezing at 80°C for 30 days, and three freeze–thaw cycles (from −20°C to room temperature), to record the relative peak area of each color spectrum peak for the calculation of its stability.
Recovery
The sample addition recovery method was used for the recovery test. Three quality control samples at the different concentrations (five samples for each concentration) were taken and anwulignan was added to the plasma (tissues), and then their chromatogram peak areas were measured under the UHPLC-Q-Orbitrap-MS/MS conditions. The measured peak areas were compared with those of the blank plasma (tissues) after the treatment with the same amount of anwulignan for the calculation of the recovery rate.
Results
Method Validation
Figure 1 shows the extracted ion chromatograms (EIC) of anwulignan (m/z 329) and schisantherin A (m/z 415). The results showed that their retention time was 11.91 and 7.42 min for them, respectively, and no endogenous interference was found within the retention time of anwulignan and IS.
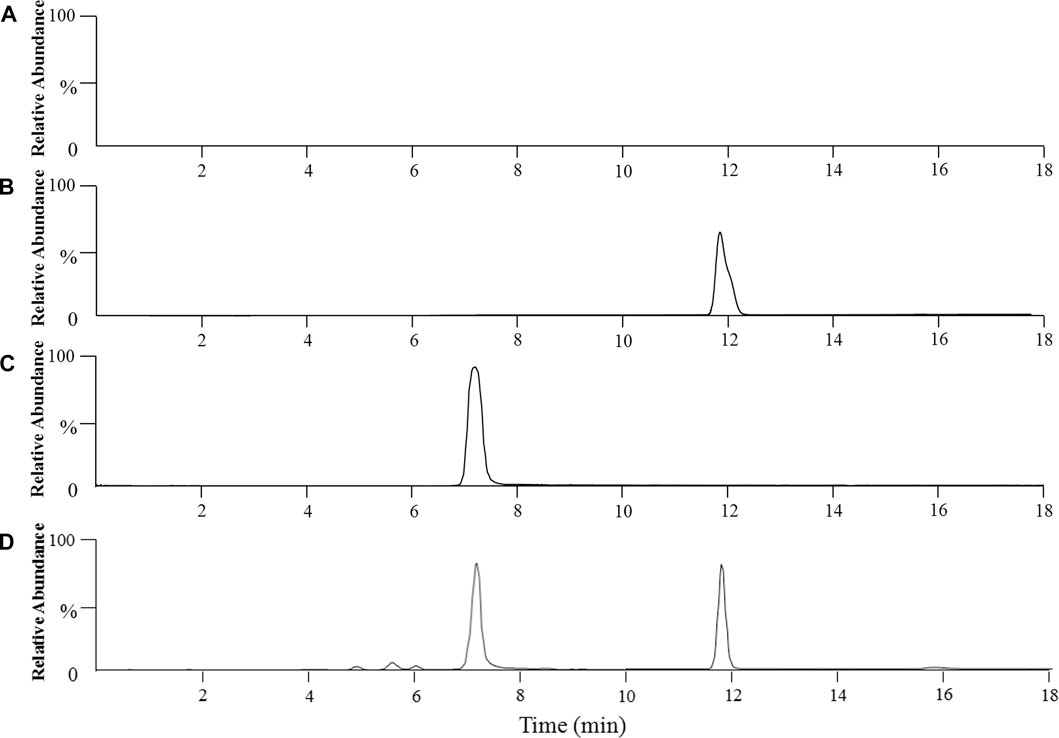
FIGURE 1. Representative chromatograms of anwulignan and schisandrin A in the plasma of mice. Note: (A) blank plasma; (B) blank plasma spiked with anwulignan; (C) blank plasma spiked with schisandrin A; (D) a mice plasma sample collected after administration of a single dosage of anwulignan (3 h).
As shown in Table 1, the linear range of anwulignan in the plasma (n = 3) was 0.01–5 μg/ml, and that of anwulignan in the heart, liver, kidney, spleen, and brain homogenates (n = 3) was 0.02–5.0 μg/ml; under the selected chromatographic conditions, the LOQ was 0.02 μg/ml. The plasma standard curve of anwulignan is shown in Figure 2.
The matrix effect of anwulignan sample was 94.6%–98.7%, and the results of its short-term and long-term stability investigation are shown in Table 2. As shown in Table 3, the RSD value for evaluating the precision of anwulignan in each sample was less than 5.6%, the RSD value for the accuracy was less than 6.2%, the average recovery was between 92.7% and 98.5%, and the stability was less than 3.4%, indicating that the results obtained by the method should be reliable.
Pharmacokinetics and Distribution of Anwulignan In Vivo
After the intragastric administration of anwulignan, the peak area of each time period detected by HPLC was substituted into the standard curve to obtain the corresponding concentrations of anwulignan. The data obtained were analyzed with pharmacokinetics software DAS (version 3.2.8), and the results are shown in Figure 3, the pharmacokinetic parameters are shown in Table 4, and the contents of anwulignan in the different tissues are shown in Figure 4. As shown in Figure 3, the plasma concentration of anwulignan was the highest at 3 h after the intragastric administration of anwulignan, and lasted for a long time. Its Tmax and T1/2 calculated by the judgment method of non-compartment model were 3.00 and 7.10 h, respectively, indicating that the duration of anwulignan is long in the body of mice. The apparent distribution volume of anwulignan was 32.81 ± 4.79 L/kg, indicating that anwulignan has a strong lipophilicity, so it is easy for it to enter cells and be widely distributed in tissues and organs rich in fat. Its AUC0−t and AUC0−∞ were 9.37 ± 2.36 and 12.58 ± 3.51 mg/Lh, respectively, indicating that anwulignan may enter the systemic circulation more and be absorbed more completely. Its CLZ/F was 0.80 ± 0.23 L/h/kg, indicating that the clearance rate of anwulignan in the body is slow. The relative bioavailability of anwulignan was 23.8%, indicating that it may be metabolized through an obvious hepatointestinal circulation. As shown in Figure 4, anwulignan could be detected in the heart, liver, spleen, lung, kidney, and brain tissues of mice, and the order of the total concentration of anwulignan from high to low within 48 h was liver > heart > brain > kidney > lung > spleen (Table 5). At 2 h, the concentration of anwulignan in the liver was the highest, and the content of anwulignan in the liver is higher than that in the other tissues. The content in the liver and heart tissues was the highest within 2 h, and that in the liver, heart, and brain tissues was higher at 3 h.
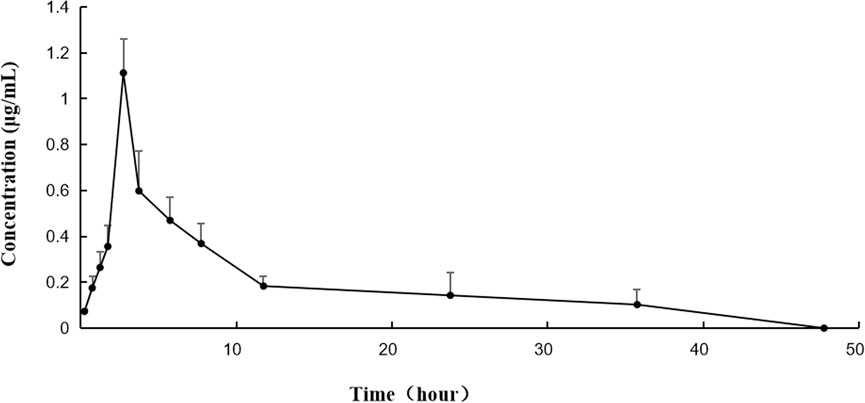
FIGURE 3. Concentration–time curve of anwulignan in mice after the intragastric administration of anwulignan.
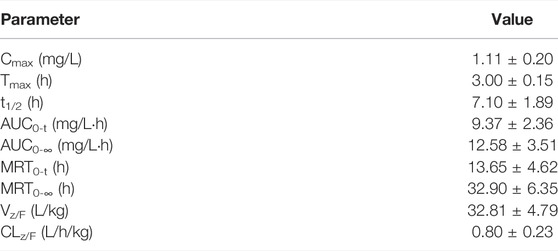
TABLE 4. Primary pharmacokinetic parameters of anwulignan in mice after the intragastric administration of anwulignan.
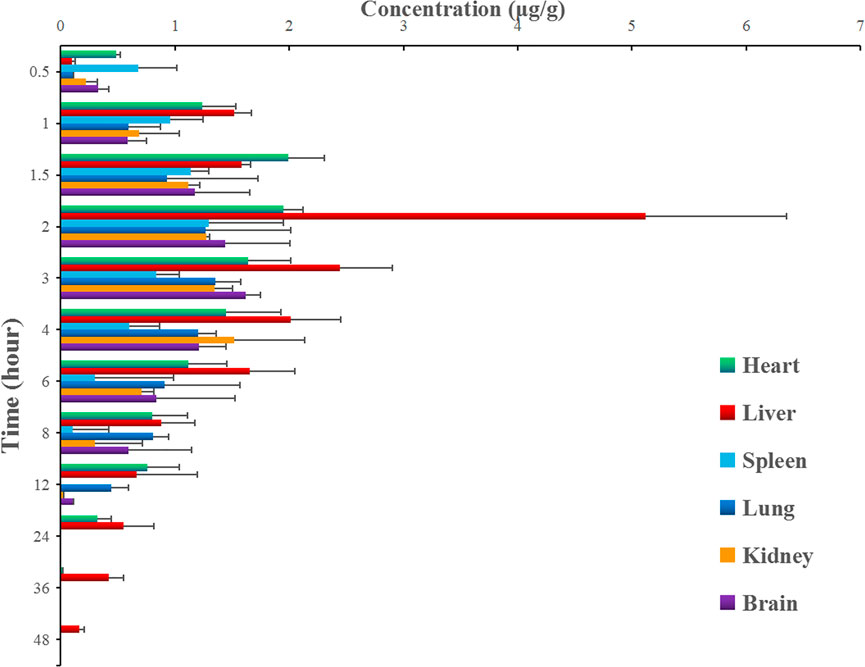
FIGURE 4. Concentrations of anwulignan in the different tissues of mice after the intragastric administration of anwulignan.
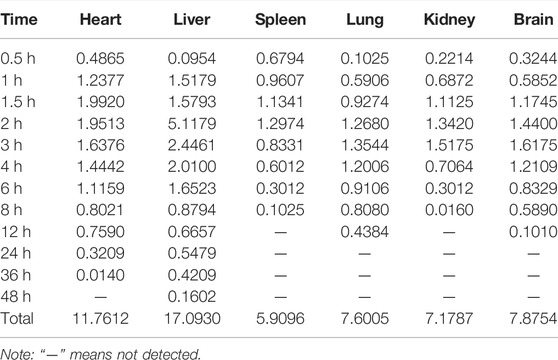
TABLE 5. Concentrations of anwulignan in the different tissues of mice after the intragastric administration of anwulignan.
In Vivo Metabolites of Anwulignan by Metabolomic Method
In order to find the metabolites of anwulignan in mice after the intragastrical administration, a metabolomic method based on UHPLC-Q-Orbitrap-MS/MS was used to analyze the samples, and the multivariate statistical analysis of anwulignan was carried out by metabonomic methods, as shown in Figure 5.
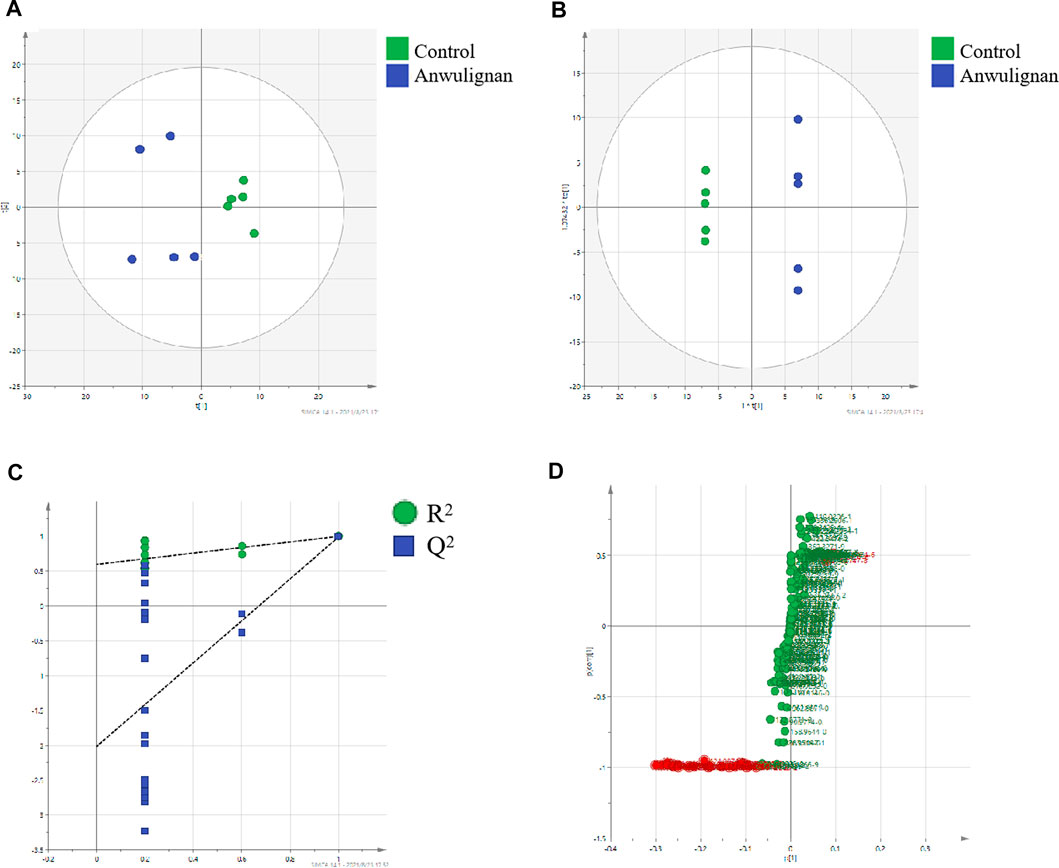
FIGURE 5. Multivariate statistical analysis control and anwulignan group: (A) PCA score plot; (B) OPLS-DA score plot; (C) cross-validation plot of OPLS-DA model; and (D) S-plot of OPLS-DA.
Taking the liver samples as an example, unsupervised PCA method was used for the matrix analysis on the data of liver samples from control mice and those treated with anwulignan at 4 h after the intragastrical administration to analyze the relationship among the data, and it could be understood from the score chart of PCA that the samples were well separated between the two groups (Figure 5A). Because PCA could not be used for the analysis of the unknown grouping samples, an OPLS-DA method was used to analyze the data in the two groups to obtain the score chart of OPLS-DA (Figure 5B), which indicated that there were significant differences between the control group and the anwulignan group, and the validity of the model was evaluated by the external model verification method (arrangement of the experiment). As shown in Figure 5C, both slopes of the two regression lines were larger; R2 and Q2 produced by the left-end alignment were smaller than those by the right-end alignment, and the difference between the two values at the right-most end was smaller, indicating that the model was valid. In order to determine the metabolites of anwulignan in the liver to the greatest extent, S-plot diagram was used for the analysis (Figure 5D). In the S-plot diagram, each point represents an ion, and the farther away from the zero point, the greater the contribution of ions to the fractionation. The ions existing in the liver of mice after the intragastric administration of anwuzhisu, not in the liver of mice in the blank group, were taken as the potential metabolites, and the information on their precise molecular weight and MS/MS fragmentation ions was analyzed.
As shown in Figure 6A, anwulignan presented as [M + H]+ in positive-ion mode and its mass to charge ratio (m/z) was 329, and fragment m/z 311 might be generated from m/z 329 losing H2O (18 Da) at C-4″ position, m/z 205 from m/z 329 losing C7H8O2 (124 Da) at C-4, m/z 176 from m/z 205 losing C2H5 (29 Da) at C-2 and C-3, m/z 135 from m/z 176 losing C3H5 (41 Da) at C-1, m/z 192 from m/z 329 losing C8H9O2 (137 Da) at C-2, m/z 137 from m/z 192 losing C4H7 (55 Da) at C-4, and m/z 123 from m/z 137 losing CH2 (14 Da) at C-1″. The fragmentation mechanism of anwulignan is simulated and shown in Figure 7.
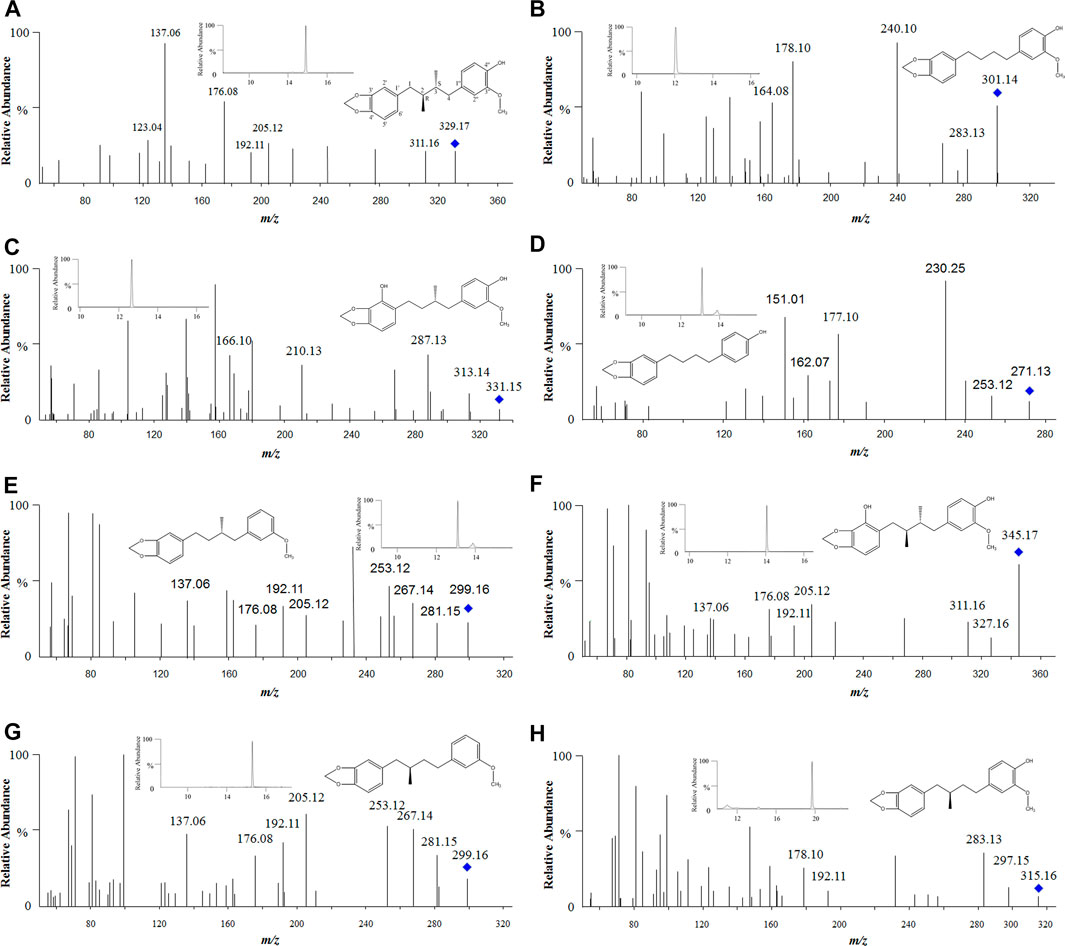
FIGURE 6. Tandem mass spectra of anwulignan and its metabolites. Note: (A) anwulignan at m/z 329; (B) M1 at m/z 301; (C) M2 at m/z 331; (D) M3 at m/z 271; (E) M4 at m/z 299; (F) M5 at m/z 345; (G) M6 at m/z 299; and (H) M7 at m/z 315.
A total of seven metabolites of anwulignan were detected in the blood and liver samples, and the relevant information on these metabolites is shown in Table 6.
The elution time of M1 (m/z 301) was 12.09 min (Figure 6B), suggesting that M1 should be a metabolite of anwulignan of losing two methyl groups, and fragment ion at m/z 283 might be generated from that at m/z 301 of losing H2O (18 Da) at C-4″, m/z 178 from m/z 283 of losing C7H5O (105 Da) at C-4, and m/z 164 from m/z 178 of losing a methyl group (14 Da).
The elution time of M2 (m/z 331) was 12.69 min (Figure 6C), suggesting that M2 should be a demethylation metabolite of anwulignan after hydroxylation, and fragment ion at m/z 313 might be generated from that at m/z 331 of losing H2O (18 Da) at C-4″, m/z 287 from m/z 331 of losing CH2 and OCH2 (44 Da), and m/z 210 from m/z 331 of losing C7H5O2 (121 Da) at C-1.
The elution time of M3 (m/z 271) was 12.96 min (Figure 6D), suggesting that M3 should be the metabolite of anwulignan of losing a methoxyl group at C-3″, and a methyl group at C-2 and C-3, respectively, and fragment ion at m/z 253 might be generated from that at m/z 271 of losing a H2O (18 Da) at C-4″, m/z 177 from m/z 253 of losing C6H4 (76 Da) at C-4, and m/z 162 from m/z 253 of losing C7H7 (91 Da) at C-3.
The elution time of M4 (m/z 299) was 13.07 min (Figure 6E), suggesting that M4 should be a dehydroxylation and demethylation metabolite of anwulignan, and fragment ion at m/z 281 might be generated from that at m/z 299 of losing H2O (18 Da) at C-4″, fragment ions at m/z 267 and 253 might be generated from those at m/z 281 of losing one and two methyl groups, respectively, and the fragmentation characteristics of fragment ions at m/z 205, 192, 176, and 137 might be basically consistent with those of anwulignan. The elution time of M6 (m/z 299) was 15.25 min (Figure 6G), and the fragmentation characteristics of fragment ions at m/z 281, 267, 253, 205, 192, 176, and 137 were consistent with those of M4, but the retention time was different from that of M4, so M6 might be the isomer of M4.
The elution time of M5 (m/z 345) was 14.09 min (Figure 6F), indicating that M5 should be a hydroxylation metabolite of anwulignan, fragment ion at m/z 327 might be generated from that at m/z 345 of losing H2O (18 Da) at C-4″, and the fragmentation characteristics of the other fragment ions at m/z 311, 205, 192, 176, and 137 were consistent with those of anwulignan.
The elution time of M7 (m/z 315) was 19.11 min (Figure 6H), indicating that M7 should be a metabolite of anwulignan of losing a methyl group, and fragment ion at m/z 297 might be generated from that at m/z 315 of losing H2O (18 Da) at C-4″, m/z 283 from m/z 297 of losing a methyl group (14 Da), m/z 192 from m/z 315 (123 Da) of losing C7H7O2 at C-4, and m/z 178 from m/z 192 of losing a methyl group (14 Da).
The excreta of mice within 48 h after the intragastric administration of anwulignan were taken for analysis by HPLC. The results showed that anwulignan prototype and its metabolites were not found in the urine of mice within 48 h, and anwulignan prototypes, M3 and M6, were found in the feces, as shown in Figure 8.
Discussion
The results on the pharmacokinetics of anwulignan in mice indicated that UHPLC-Q-Orbitrap-MS/MS could be well suited for this study. Non-compartment model was used to study the pharmacokinetic characteristics of anwulignan in mice, and it was found that anwulignan was absorbed slowly in mice (Tmax = 3 h), its duration was long (t1/2 = 7.1 h), and the prototype of anwulignan could still be detected in the blood until 24 h, indicating that the elimination rate of anwulignan should be relatively slow and there may be an enterohepatic circulation involved in it. Based on the fact that the content of anwulignan in the liver was higher than that in the other tissues, and its residence time was longer, it could be speculated that there may be a hepatointestinal circulation in the metabolism of anwulignan in the body of mice, so it has a long half-life. Its AUC0−t and AUC0−∞ were 9.37 ± 2.36 and 12. 58 ± 3.51 mg/Lh, respectively, suggesting that more anwulignan can enter the systemic circulation. Apparent distribution volume (Vd) can be used to infer the extent of drug distribution in the body, and the Vd value is close to 0.8–1.0 L/kg if a drug is evenly distributed in the body and the concentration of a drug in tissues is higher than that in blood when the Vd value is greater than 1.0 L/kg, which may indicate that the drug is widely distributed in the body or the tissue protein is highly bound to the drug (Weiss et al., 2021). In this study, the Vd of anwulignan was 32.81 ± 4.79 L/kg, indicating that anwulignan has strong lipophilicity, so it is easy for it to enter cells and be widely distributed in tissues through blood circulation. The relative bioavailability of anwulignan was 23.8%, which may be due to the incomplete absorption in the gastrointestinal tract or the obvious first-pass effect on the liver. This result is also reflected by the distribution data of anwulignan in the main organs, which is consistent with that reported by Song et al. (2020).
After the intragastric administration of anwulignan, the prototype of anwulignan could be detected in all major organs of mice, indicating that anwulignan is widely distributed. The distribution of a drug in tissues is related to the blood circulation, vascular permeability, and drug protein binding (Tang and Cao, 2021), and the differential distribution of compounds in various organs mainly depends on the blood flow rate and the concentration of compounds in the organs and the affinity of compounds to the organs. Liver, kidney, and brain are organs with a rapid blood circulation, so anwulignan could be detected quickly in these tissues after the intragastric administration. The order of the concentration of anwulignan in the organs from high to low was liver > heart > brain > kidney > lung > spleen, and the concentration in liver was the highest, indicating that anwulignan may experience a slow elimination in the liver. This result may explain the reason why anwulignan has a therapeutic effect on liver injuries. The liver may be an accumulation site of anwulignan, so taking a large dosage of anwulignan may induce some adverse reactions related to liver metabolism. The content of anwulignan in the spleen was lower, indicating that the tissue distribution of anwulignan after the intragastric administration is targeted. It is worth noting that the concentration of anwulignan in the brain tissue is higher than that of other Schisandra lignans in the brain tissue (Lu et al., 2013; Liu et al., 2019; Liang et al., 2021), suggesting that anwulignan has a strong ability to penetrate the blood–brain barrier to play a pharmacological role in the central nervous system, which may provide a reference for the future study on the efficacy of anwulignan.
The study on the excreta of mice showed that anwulignan could be not detected in the urine of mice, but could be detected in the feces, which may be the result of the poor water solubility of anwulignan. It was found in this study that the concentration of anwulignan was highest in feces samples during 4–8 h after the intragastric administration, and anwulignan could still be detected during 12–24 h, further confirming that anwulignan may undergo an obvious enterohepatic circulation, combined with the previous results of this study. The total amount of anwulignan excreted from the body in the form of prototype drug was far less than the dosage, indicating that its excretion from the body is mainly through a metabolic elimination.
Seven metabolites of anwulignan were found in this study, of which only M2 was detected in the blood of mice and the other metabolites were found in the liver, suggesting that the main component of anwulignan to play a pharmacological role should be its prototype or M2 (except the liver), and the effect of the other metabolites should mainly act on the liver or act on the intestinal flora through the way of enterohepatic circulation, so as to indirectly exert its effect on other tissues and organs. Combined with the results of in vivo distribution experiment, anwulignan was primarily distributed in the liver after entering the body, and its metabolites were also primarily distributed in the liver, indicating that the liver may be the main organ in which anwulignan can play a role. Some studies have found that anwulignan has an obvious inhibitory effect on carboxylesterases 2 in the liver, and the inhibitory effect is the strongest among many Schisandra lignans (Fu et al., 2019). Anwulignan is mainly metabolized in the way of demethylation, hydroxylation, dehydroxylation, and demethoxylation, and studies on other Schisandra lignans have also shown that demethylation and hydroxylation are the main metabolic ways of lignans (Liu et al., 2014; Su et al., 2016; Liu et al., 2019; Wang et al., 2020). It was the first time that the metabolites of anwulignan in the body of animals were studied, and the main metabolic pathways are shown in Figure 9.
Conclusion
UHPLC-Q-Orbitrap-MS/MS was employed to investigate the pharmacokinetics and tissue distribution of anwulignan in mice. It was found that anwulignan was slowly absorbed in mice and widely distributed in various tissues and organs, especially in the liver. The main metabolites of anwulignan in vivo were analyzed for the first time, and seven metabolites were found. The results indicate that demethylation, hydroxylation, dehydroxylation, and demethoxylation may be the main metabolic ways of anwulignan, and the majority of its prototypes and metabolites may be excreted through feces.
Data Availability Statement
The original contributions presented in the study are included in the article/Supplementary Material; further inquiries can be directed to the corresponding author.
Ethics Statement
The animal study was reviewed and approved by the Experimental Animal Ethics Committee of Beihua University.
Author Contributions
Conceptualization, JS and JC; methodology, CC; software, YF; validation, HNL and HOL; investigation, CC and JS; writing—original draft preparation, YF and JS; writing—review and editing, CW and HEL; and funding acquisition, SJ and JS. All authors have read and agreed to the published version of the manuscript.
Funding
This research was funded by the Natural Science Foundation of Jilin Province, grant number 20200201521JC; Traditional Chinese Medicine Science and Technology project of Jilin Province, grant number 2020119; and Science and Technology Project of Jilin Provincial Education Department grant number JJKH20210065KJ.
Conflict of Interest
The authors declare that the research was conducted in the absence of any commercial or financial relationships that could be construed as a potential conflict of interest.
Publisher’s Note
All claims expressed in this article are solely those of the authors and do not necessarily represent those of their affiliated organizations, or those of the publisher, the editors, and the reviewers. Any product that may be evaluated in this article, or claim that may be made by its manufacturer, is not guaranteed or endorsed by the publisher.
References
Chen, Z., Liu, F., Zheng, N., Guo, M., Bao, L., Zhan, Y., et al. (2019). Wuzhi Capsule (Schisandra Sphenanthera Extract) Attenuates Liver Steatosis and Inflammation during Non-alcoholic Fatty Liver Disease Development. Biomed. Pharmacother. 110, 285–293. doi:10.1016/j.biopha.2018.11.069
Fu, Q., Yang, K., Hu, R. X., Du, Z., Hu, C. M., and Zhang, X. (2019). Evaluation of the Inhibition of Human Carboxylesterases (CESs) by the Active Ingredients from Schisandra Chinensis. Xenobiotica 49, 1260–1268. doi:10.1080/00498254.2018.1548718
Jiang, P., Lu, Y., and Chen, D. (2016). Authentication of Schisandra Chinensis and Schisandra Sphenanthera in Chinese Patent Medicines. J. Pharm. Biomed. Anal. 131, 263–271. doi:10.1016/j.jpba.2016.08.040
Jiang, W., Ren, L., Guo, M., Mantri, N., Zhao, S., and Pang, X. (2019). Detecting Schisandrae Chinensis Fructus and its Chinese Patent Medicines with a Nucleotide Signature. Genes. (Basel) 10, 397. doi:10.3390/genes10050397
Li, X., Gao, J., Yu, Z., Jiang, W., Sun, W., Yu, C., et al. (2020). Regulatory Effect of Anwulignan on the Immune Function through its Antioxidation and Anti-apoptosis in D-Galactose-Induced Aging Mice. Clin. Interv. Aging 15, 97–110. doi:10.2147/CIA.S237601
Liang, D., Wu, Z., Liu, Y., Li, C., Li, X., Yang, B., et al. (2021). HPLC-MS/MS-Mediated Analysis of the Pharmacokinetics, Bioavailability, and Tissue Distribution of Schisandrol B in Rats. Int. J. Anal. Chem. 2021, 8862291. doi:10.1155/2021/8862291
Lin, H. J., Zhang, X., Liu, J., Yuan, L., Liu, J., Wang, C., et al. (2020). Protective Effect of Anwulignan on Liver Injury Caused by Intestinal Ischemia-Reperfusion in Rats. Sci. Technol. Food Industry 42, 330–336. doi:10.13386/j.issn1002-0306.2020050348
Liu, C. N., Wang, Y., Zhang, Y., Yuan, R., Zhang, X., Li, X., et al. (2020a). The Protective Effect and Mechanism of Anwulignan on D-Galactose-Induced Myocardial Injury in Aging Mice. J. Jilin Med. Univ. 41, 11–14. doi:10.13845/j.cnki.issn1673-2995.2020.01.004
Liu, J. W., Zhang, X., Lin, H., Yuan, L., Liu, J., Wang, C., et al. (2020b). Study on the Anti-fatigue Effect of Anwulignan on Aging Mice. Sci. Technol. Food Industry 41, 319–323. doi:10.13386/j.issn1002-0306.2020.18.050
Liu, M., Zhao, S., Wang, Z., Wang, Y., Liu, T., Li, S., et al. (2014). Identification of Metabolites of Deoxyschizandrin in Rats by UPLC-Q-TOF-MS/MS Based on Multiple Mass Defect Filter Data Acquisition and Multiple Data Processing Techniques. J. Chromatogr. B Anal. Technol. Biomed. Life Sci. 949-950, 115–126. doi:10.1016/j.jchromb.2013.12.022
Liu, X., Cong, L., Wang, C., Li, H., Zhang, C., Guan, X., et al. (2019). Pharmacokinetics and Distribution of Schisandrol A and its Major Metabolites in Rats. Xenobiotica 49, 322–331. doi:10.1080/00498254.2017.1418543
Lu, H., and Liu, G. T. (1992). Anti-oxidant Activity of Dibenzocyclooctene Lignans Isolated from Schisandraceae. Planta Med. 58, 311–313. doi:10.1055/s-2006-961473
Lu, S. W., Zhang, A. H., Sun, H., Yan, G. L., Han, Y., Wu, X. H., et al. (2013). Ultra-performance Liquid-Chromatography with Tandem Mass Spectrometry for Rapid Analysis of Pharmacokinetics, Biodistribution and Excretion of Schisandrin after Oral Administration of Shengmaisan. Biomed. Chromatogr. 27, 1657–1663. doi:10.1002/bmc.2976
Song, Y., Zhang, Y., Duan, X. Y., Cui, D. W., Qiu, X., Bian, Y., et al. (2020). Pharmacokinetics and Tissue Distribution of Anwulignan in Rats after Intravenous and Intragastric Administration by Liquid Chromatography-Mass Spectrometry. Molecules 25, 39. doi:10.3390/molecules25010039
Su, L. L., Cheng, X., Ji, J. D., Wang, L. J., Ding, X. Y., Lu, T. L., et al. (2016). Analysis of Lignans and Their Metabolites Derived from Schisandra Chinensis and Vinegar Schisandra Chinensis in Rats’ Plasma, Bile, Urine and Faeces Based on UHPLC-QTOF/MS. Yao Xue Xue Bao 51, 1600–1608. doi:10.16438/j.0513-4870.2016-0315
Tang, Y., and Cao, Y. (2021). Modeling Pharmacokinetics and Pharmacodynamics of Therapeutic Antibodies: Progress, Challenges, and Future Directions. Pharmaceutics 13, 422. doi:10.3390/pharmaceutics13030422
Wang, J., Jiang, B., Shan, Y., Wang, X., Lv, X., Mohamed, J., et al. (2020). Metabolic Mapping of Schisandra Chinensis Lignans and Their Metabolites in Rats Using a Metabolomic Approach Based on HPLC with Quadrupole Time-Of-Flight MS/MS Spectrometry. J. Sep. Sci. 43, 378–388. doi:10.1002/jssc.201900860
Weiss, H. M., Langenickel, T., Cain, M., Kulkarni, S., Shah, B., Vemula, J., et al. (2021). Clinical Investigation of Metabolic and Renal Clearance Pathways Contributing to the Elimination of Fevipiprant Using Probenecid as Perpetrator. Drug Metab. Dispos. 49, 389–394. doi:10.1124/dmd.120.000273
Yang, J., Duan, J. A., Li, G. L., Zhu, Z. H., Zhu, T. L., Qian, D. W., et al. (2014). Determination of Lignans in Schisandrae Sphenantherae Fructus from Different Regions. Zhongguo Zhong Yao Za Zhi 39, 4647–4652. doi:10.4268/cjcmm20142332
Zhang, M. X., Huang, G. Y., Bai, Y. Q., Li, H., and Yang, B. (2021). Research Advances in Chemical Constituents and Hepatoprotective Effect of Schisandrae Sphenantherae Fructus and Schisandrae Chinensis Fructus. Zhongguo Zhong Yao Za Zhi 46, 1017–1025. doi:10.19540/j.cnki.cjcmm.20201127.601
Keywords: Schisandra sphenanthera, anwulignan, pharmacokinetics, metabolites, UHPLC-Q-Orbitrap-MS
Citation: Chen C, Feng Y, Li H, Lin H, Jing S, Li H, Wang C, Chen J and Sun J (2022) Pharmacokinetics and Main Metabolites of Anwulignan in Mice. Front. Pharmacol. 13:929177. doi: 10.3389/fphar.2022.929177
Received: 26 April 2022; Accepted: 20 May 2022;
Published: 05 July 2022.
Edited by:
Guo Ma, Fudan University, ChinaReviewed by:
Lin-Lin Chen, Hubei University of Chinese Medicine, ChinaLing Ye, Southern Medical University, China
Mario Juan Simirgiotis, Austral University of Chile, Chile
Copyright © 2022 Chen, Feng, Li, Lin, Jing, Li, Wang, Chen and Sun. This is an open-access article distributed under the terms of the Creative Commons Attribution License (CC BY). The use, distribution or reproduction in other forums is permitted, provided the original author(s) and the copyright owner(s) are credited and that the original publication in this journal is cited, in accordance with accepted academic practice. No use, distribution or reproduction is permitted which does not comply with these terms.
*Correspondence: Jinghui Sun, sunjinghui2008@126.com