- 1Department of Emergency and Organ Transplantation (D.E.T.O.), Hematology and Stem Cell Transplantation Unit, University of Bari “Aldo Moro”, Bari, Italy
- 2School of Medicine, University of Bari “Aldo Moro”, Bari, Italy
Since its introduction in clinical practice, eltrombopag (ELT) has demonstrated efficacy in heterogeneous clinical contexts, encompassing both benign and malignant diseases, thus leading researchers to make a more in-depth study of its mechanism of action. As a result, a growing body of evidence demonstrates that ELT displays many effects ranging from native thrombopoietin agonism to immunomodulation, anti-inflammatory, and metabolic properties. These features collectively explain ELT effectiveness in a broad spectrum of indications; moreover, they suggest that ELT could be effective in different, challenging clinical scenarios. We reviewed the extended ELT mechanism of action in various diseases, with the aim of further exploring its full potential and hypothesize new, fascinating indications.
Introduction
Eltrombopag (ELT) is a thrombopoietin receptor agonist (TPO-RA), currently indicated for the treatment of immune thrombocytopenia (ITP), hepatitis C-associated thrombocytopenia and severe aplastic anemia (SAA) (Gilreath et al., 2021). Evidence shows that a subset of ITP patients achieving a complete response can safely suspend ELT without experiencing a relapse (González-López et al., 2015). Similarly, a trilineage response has been maintained in SAA patients after ELT discontinuation (Desmond et al., 2014).
Moreover, ELT has proven efficacy in myelodysplastic syndrome (MDS) and the therapy of post-transplant poor graft function (PGF) (Tang et al., 2018; Vicente et al., 2020), while data in the context of acute myeloid leukemia (AML) are conflicting (Buckstein, 2015; Mittelman et al., 2018; Frey et al., 2019; Shi et al., 2019). ELT can decrease cellular iron and enhance its mobilization in combination with available chelators, thus reducing reactive oxygen species (ROS) levels and improving cellular fitness in various clinical contexts (Roth et al., 2012; Vlachodimitropoulou et al., 2017; Argenziano et al., 2021).
These observations indicate that ELT exerts a complex mechanism of action beyond the stimulation of megakaryocytes proliferation, encompassing stem-cell stimulation, immunomodulation, and anti-inflammatory properties. The broad spectrum of indications and the increasing heterogeneity of reported effects suggest that ELT is able to set off a chain of events whose potential has yet to be fully exploited. We reviewed the main ELT related off-target effects in various clinical contexts to explore its potential: is there room for more?
Mechanism of Action and Pharmacological Properties
ELT is an oral synthetic nonpeptide low molecular weight TPO-RA. After administration, global bioavailability peaks at 2–6 h (Erickson-Miller et al., 2009; Gilreath et al., 2021). It circulates bound to plasma proteins, is metabolized in the liver through cytochrome P450 isoenzymes CYP1A, CYP2C8, and uridine diphosphate glucuronosyltransferase, and its half-life ranges from 21 to 32 h (Deng et al., 2011; Wire et al., 2012). Unlike endogenous TPO, ELT selectively binds to the transmembrane domain of the TPO receptor, stimulating survival, proliferation, and differentiation of megakaryocytes through the JAK/STAT and MAPK pathways (Figure 1) (Erickson-Miller et al., 2009). ELT activates STAT3/5, AKT, and ERK at a higher level than TPO, thus enabling the upregulation of megakaryopoiesis (di Buduo et al., 2016). As a result, ELT has an additive rather than a competitive effect on TPO, making it effective even in patients with high endogenous TPO levels. Evidence demonstrates that ELT displays a more extended spectrum of effects. Given its proven efficacy in treating heterogeneous clinical contexts, a growing body of evidence is unveiling ELT properties concerning the hematopoietic milieu, the immune system (IS), and the inflammation process.
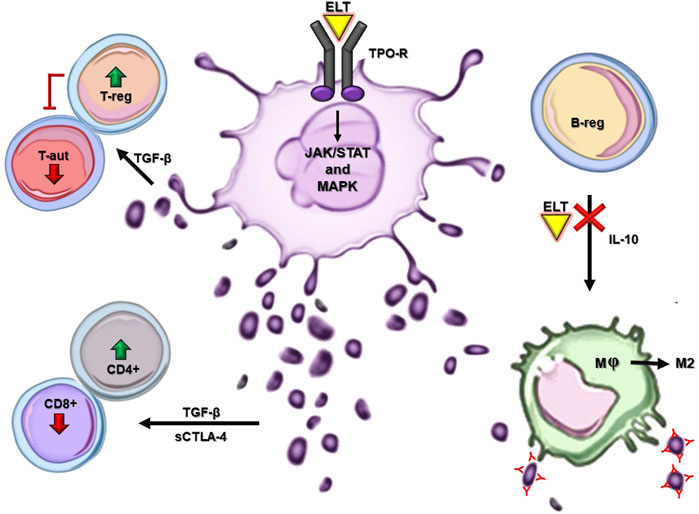
FIGURE 1. ELT mechanism of action and main off-target effects in ITP. Major cellular and molecular pathways exert the immunomodulatory and anti-inflammatory effects of ELT in ITP. In-silico models are not reported in this figure. ELT: eltrombopag, TPO-R: thrombopoietin receptor, T-reg: T regulatory lymphocyte, T-aut: T autoreactive lymphocyte, B-reg: B regulatory lymphocyte, CD4+: T helper lymphocyte, Cd8+: T cytotoxic lymphocyte, M: macrophage, M2: anti-inflammatory macrophage.
ITP: Drawing the Perfect Picture
ELT is indicated for ITP patients > 1-year-old refractory to first-line therapy or splenectomy (Gómez-Almaguer, 2018); its efficacy ranges between 75 and 95% (Gómez-Almaguer, 2018). Robust evidence supports the safety and success of dose tapering, re-treatment, and discontinuation of therapy; after this latter up to approximately 50% of patients maintain a response (Mahévas et al., 2014; González-López et al., 2015; Wong et al., 2017; Al-Samkari and Kuter, 2018; Ghanima et al., 2019). This unique profile has fueled interest in making a more in-depth study of the mechanism of action of ELT beyond TPO-R agonism. Interestingly, while binding only human and primate TPO-R, ELT has proven efficacy in murine models, thus reinforcing the idea of beneficial off-target effects (Erickson-Miller et al., 2009; Raslova et al., 2016).
Based on the physiopathological mechanisms of ITP, the prime suspect was the IS. Firstly, ELT is thought to exert an indirect immunomodulatory effect: by increasing the number of platelets, it should, on one hand, increase the number of CD4+ T helper cells and reduce the number of CD8+ T effector cells (Figure 1) (Bao et al., 2010; Liu et al., 2013). Since platelets are the main reservoir of TGF-β, this effect should partly be mediated by an increased activity of this anti-inflammatory cytokine (Abbonante et al., 2016); its expression has been demonstrated to be positively correlated with soluble CTLA-4 levels, which, in turn, can modulate the immune response (Fujita et al., 2012). TGF-β is also involved in the downregulation of autoreactive T cells and expansion of Tregs (Liu et al., 2018). Additionally, TGF-β is highly expressed in ectosomes: platelet-derived microparticles interacting with immune cells to restore IS homeostasis and dampen inflammation (Figure 1) (Sadallah et al., 2011, 2014). On the other hand, ELT should restore immune tolerance to platelet autoantigens, thus altering the ITP immunopathogenic process (Nishimoto et al., 2014). Moreover, upon ELT treatment in ITP, the TGF-β increased secretion and further fibrogenic cytokines are thought to be responsible for augmented reticulin bone marrow (BM) fibrosis. This process is reversible and tends to worsen in a time-dependent fashion. Furthermore, ELT administration seems to induce myeloproliferative neoplasm-like changes in the ITP BM, such as trilineage hypercellularity, and megakaryocytic pleomorphic proliferation together with the tendence to form clusters (Boiocchi et al., 2012; Ghanima et al., 2014; Brynes et al., 2017).
Moreover, evidence demonstrates that ELT treatment in ITP results in both quantitative and qualitative immunomodulatory effects, namely increased regulatory B-cell numbers and a reduced phagocytic capacity of monocyte-derived macrophages on opsonized platelets (Li et al., 2012; Liu et al., 2016). Furthermore, ELT may influence macrophages polarization towards the M2 anti-inflammatory subtype (Figure 1) (di Paola et al., 2021). Bearing in mind that a skewed proinflammatory T cell profile characterizes ITP, ELT has been shown to exert an antiproliferative effect on T cells and to affect their functionality, reducing the intracellular production of TNF-α, INF-γ and granzyme B (Sayed et al., 2019).
The consistency of all these data is even more accentuated considering that RNA sequencing of blood cells subsets has revealed that TPO is expressed in immune cell subpopulations: T-regs, B lymphocytes, CD4+ and CD8+ T cells, monocytes, neutrophils, and NK cells (Schmiedel et al., 2018; Monaco et al., 2019). Recently, an “in silico” approach has shown different pathways (upregulation of FOXP3 and PPARγ, attenuation of IFN-γ signaling) by which ELT may restore immune tolerance and reduce inflammation in the context of ITP (Lozano et al., 2021). Interestingly, this model hypothesizes a potential pro-apoptotic BCL-2 mediated effect upon long term treatment with ELT, affecting the survival of platelets (Lozano et al., 2021). On the other hand, experimental data show that ELT could directly inhibit the pro-apoptotic factor Bax, thus contributing to the fine regulation of cell survival pathways (Spitz et al., 2021). It remains to be elucidated whether the same effects may occur at the lymphocyte level, ultimately affecting immunomodulation. In this scenario, the body of evidence arising from ITP suggests that the effectiveness of ELT in various clinical contexts on multiple routes should be explored.
SAA: Unlock the Stemness
The rationale for using ELT in SAA lies in the beneficial effect of TPO on the expansion of hematopoietic stem cells (HSCs) (Scheinberg, 2018; Drexler and Passweg, 2021). There is evidence that HSCs and immature progenitors express TPO-R on their cell surface (Ninos et al., 2006; de Graaf and Metcalf, 2011); ELT-mediated stimulation of TPO-R downstream pathways can, therefore, effectively “unlock” bi- or tri-lineage hemopoiesis (Olnes et al., 2012). Interestingly, a sustained hematologic response has been observed in SAA patients after ELT discontinuation, thus raising questions about possible beneficial off-target effects leading to efficient long-term maintenance of HSCs fitness and proliferation (Desmond et al., 2014).
The IS dysregulation is a cornerstone of the SAA pathogenesis, characterized by a clonal expansion of CD8+ T cytotoxic cells and a reduced number of dysfunctional T-regs (Schoettler and Nathan, 2018; Young, 2018). Moreover, T-helper 1, 2 and 17 subsets are also increased, further supporting the effector response and a proinflammatory state sustained by IL-2, TNF-α and IFN-γ (Peffault De Latour et al., 2010; Medinger et al., 2018). It is plausible that the effects mentioned above observed in ITP could also contribute to ELT effectiveness in SAA.
Furthermore, it has been shown that ELT can overcome the inhibitory action exerted by IFN-γ on the TPO-TPO-R axis (Alvarado et al., 2019). IFN-γ, in fact, prevents the full binding of TPO explicitly to TPO-R through steric occlusion of the binding site, thus affecting downstream signaling pathways, resulting in a decreased survival of HSCs. Thanks to its allosteric binding site, ELT bypasses this inhibition, explaining its clinical activity even in the presence of high endogenous TPO levels, such as in SAA and BM failure (Alvarado et al., 2019).
Notably, robust evidence supports ELT iron chelation properties that could ameliorate the fitness of HSCs and hematopoiesis, especially considering that SAA patients are frequently transfusion-dependent (Vlachodimitropoulou et al., 2017; Zhao et al., 2018). Moreover, the ELT-mediated mobilization of intracellular iron and the decrease of serum ferritin levels observed in various clinical contexts (Roth et al., 2012; Vlachodimitropoulou et al., 2017; Punzo et al., 2018; Zhao et al., 2018; Argenziano et al., 2021) could help to prevent long-term multi-organ complications and improve BM function.
Based on this knowledge, the efficacy of ELT in the treatment of SAA can be considered multifactorial (Table 1). The safety of treatment discontinuation seems to suggest the presence of other mechanisms accounting for the ELT success, which have yet to be fully elucidated.
MDS and AML: A Dangerous Path
In the wake of the encouraging results in ITP and SAA, various studies tested ELT efficacy in MDS and AML (Buckstein, 2015; Platzbecker et al., 2015; Dickinson et al., 2018; Tang et al., 2018; Shi et al., 2019; Vicente et al., 2020). Beyond the need for improvement of megakaryopoiesis in both diseases, these attempts were based on founding concepts such as the IS dysregulation shared by SAA and the subset of hypoplastic MDS (Calado, 2011), and the ELT capacity to influence HSCs fitness and the BM microenvironment, and to restore “compromised” hematopoiesis. On the other hand, the main concern was the risk of clonal evolution upon ELT treatment, as previously observed in SAA patients (Table 1) (Olnes et al., 2012; Babushok, 2018). In detail, approximately 15–20% of SAA patients have been demonstrated to develop clonal cytogenetic BM abnormalities (including chromosome 7 aberrations) early upon initiation of treatment with ELT (Winkler et al., 2019). On the other hand, various studies demonstrated that in SAA the presence of mutations in myeloid candidate genes is not sufficient for malignant transformation and weakly predictive of clonal evolution (Winkler et al., 2019). Therefore, it should be noted that there is no consensus as to the exact mechanism of ELT-mediated clonal evolution in SAA. Moreover, preliminary studies aiming to evaluate ELT efficacy and safety in the context of MDS and AML did not show increased blast proliferation or clonogenic properties, while confirming the improvement in platelets counts (Will et al., 2009; Mavroudi et al., 2011). Furthermore, ELT showed antileukemic effects related to its iron chelation properties, the capacity to activate pro-apoptotic signaling through reactive oxygen species (ROS) modulation and induce cellular differentiation (Roth et al., 2012; Kalota et al., 2015; Argenziano et al., 2021). Against this background, results from MDS and AML trials confirm ELT effectiveness in treating hypoplastic and low to intermediate-1 risk MDS, while data from higher-risk MDS and AML are conflicting and affected by the rates of clonal evolution and disease progression (Buckstein, 2015; Mittelman et al., 2018; Frey et al., 2019; Vicente et al., 2020). These findings are of great interest, prompting a reflection on the possible role of ELT off-target effects in hematological malignancies. Considering that preclinical evidence supports no direct ELT detrimental effects on blasts proliferation and clonal evolution in both MDS and AML, data from clinical trials challenge this concept (Platzbecker et al., 2015; Dickinson et al., 2018). We may hypothesize that the above-mentioned ELT effects on the IS could affect the antitumoral immune surveillance and the cytotoxic response, making the leukemic cell’s life much easier; moreover, the concept that dampening the IS could favor the emergence of new clones, contributing to the disease activity, should be further investigated. On the other hand, considering that a proinflammatory state favors the proliferation of mutated HSCs despite normal ones (Lusis, 2021), the anti-inflammatory environmental changes induced by ELT could somehow compensate for the IS attenuation and lack of immune surveillance. This intricate scenario should take into account the interplay between ELT and apoptotic signaling (demonstrated, on one hand, by its interaction with Bax and, on the other hand, by the ROS modulation affecting leukemic cells metabolism) and the ELT-mediated reduction of intracellular iron levels inducing cellular differentiation and the inhibition of blast proliferation.
PGF: Keeping the Balance
PGF occurs in up to 20% of patients; it is defined by the presence of persistent cytopenias (mainly thrombocytopenia) after completion of allo-HSCT procedures. The physiopathology of PGF is not fully understood; factors such as the graft composition, HLA mismatches, conditioning regimen and immunosuppression, graft versus host disease (GvHD), post-transplant infections and viral reactivations are implicated in the PGF pathogenesis (Chen et al., 2020).
Given the lack of effective treatments and considering ELT-induced sustained multilineage response in SAA, various studies evaluated ELT efficacy in PGF, showing encouraging results (Table 1) (Dyba et al., 2016; Marotta et al., 2019; Yuan et al., 2019; Halahleh et al., 2021). Again, beyond TPO agonism, direct HSCs stimulation, immunomodulation, and anti-inflammatory properties are considered the main mechanisms behind the ELT capacity to rescue impaired hematopoiesis in allo-HSCT patients and revert PGF (Marotta et al., 2019; Yuan et al., 2019). Notably, ELT efficacy seems to be irrespective of the putative PGF cause (Marotta et al., 2019). It is noteworthy that ELT is capable of inhibiting human cytomegalovirus (CMV) replication through its iron chelation properties (Vogel et al., 2019); this is of great importance, considering that CMV reactivation is one of the most typical causes of PGF (Chen et al., 2020).
Taken together, these data do not only confirm that ELT can be safely used to treat PGF by different routes (Table 1) but also prompt a reflection on its possible use in the intricate context of GvHD, where immune dysregulation and a generalized proinflammatory state are of pivotal importance (Cooke et al., 2017; Hess et al., 2021). On one hand, we should consider that ELT anti-inflammatory properties, the modulation of cytotoxic response and restoration of iron overload could be helpful in the attenuation of GvHD; on the other, robust evidence underlines the importance of TGF-β signaling in the dynamics of GvHD (Carli et al., 2012). In particular, this master regulator of the IS has been shown to inhibit the development of acute-GvHD and, conversely, to contribute to end-stage organ fibrosis in chronic-GVHD (Carli et al., 2012). Furthermore, the inhibition of TGF-β signaling is thought to positively influence the graft versus leukemia effect (GvL) (Carli et al., 2012). Hence, we should consider the impact of ELT on TGF-β homeostasis; we have previously discussed the interplay between ELT and TGF-β as described in the context of ITP, bearing in mind the physiological role of platelets as the TGF-β reservoir.
Moreover, considering that allo-HSCT patients frequently exhibit high serum ferritin levels and that iron overload affects their prognosis, ELT iron chelation properties could be helpful in the recovery of BM microenvironment fitness, ameliorating their clinical course (Penack et al., 2020). Considering the ELT-related risk of clonal evolution and leukemic cells proliferation, studies are needed to investigate whether the ELT range of beneficial effects in PGF could extend to treating acute GvHD without affecting GvL.
Discussion
Since its introduction in clinical practice, ELT has proven to be efficacious in heterogeneous contexts. Unexpected results in both immune-mediated, benign, and malignant diseases prompted more in-depth study of its mechanisms of action, such that nowadays, TPO agonism, immunomodulation anti-inflammatory and metabolic properties are considered the cornerstones of ELT efficacy. This broad spectrum of effects has led researchers to test ELT in various clinical contexts, with encouraging results. Nevertheless, the increasing knowledge of the IS importance and microenvironmental factors in both the onset and the course of hematological diseases suggests that ELT use should be explore in ever more scenarios to fully exploit its potential: there could be room for more.
Data Availability Statement
The original contributions presented in the study are included in the article/Supplementary material, further inquiries can be directed to the corresponding author.
Author Contributions
Conceptualization: FT, CC, and FA; Writing–original draft preparation: FT, CC, and FA; Writing–review and editing: FT, CC, LA, AZ, MC, IR, GS, PM, and FA; Supervision: FA. All authors have read and agreed to the final version of the manuscript.
Funding
This work was supported by “Associazione Italiana contro le Leucemie (AIL)-BARI”.
Conflict of Interest
The authors declare that the research was conducted in the absence of any commercial or financial relationships that could be construed as a potential conflict of interest.
Publisher’s Note
All claims expressed in this article are solely those of the authors and do not necessarily represent those of their affiliated organizations, or those of the publisher, the editors and the reviewers. Any product that may be evaluated in this article, or claim that may be made by its manufacturer, is not guaranteed or endorsed by the publisher.
References
Abbonante, V., di Buduo, C. A., Gruppi, C., Malara, A., Gianelli, U., Celesti, G., et al. (2016). Thrombopoietin/TGF-β1 Loop Regulates Megakaryocyte Extracellular Matrix Component Synthesis. Stem Cells 34, 1123–1133. doi:10.1002/stem.2285
Al-Samkari, H., and Kuter, D. J. (2018). An Alternative Intermittent Eltrombopag Dosing Protocol for the Treatment of Chronic Immune Thrombocytopenia. Br. J. Clin. Pharmacol. 84, 2673–2677. doi:10.1111/BCP.13717
Alvarado, L. J., Huntsman, H. D., Cheng, H., Townsley, D. M., Winkler, T., Feng, X., et al. (2019). Eltrombopag Maintains Human Hematopoietic Stem and Progenitor Cells under Inflammatory Conditions Mediated by IFN-γ. Blood. 133, 2043–2055. doi:10.1182/blood-2018-11-884486
Argenziano, M., Tortora, C., Di Paola, A., Pota, E., Di Martino, M., Di Pinto, D., et al. (2021). Eltrombopag and its Iron Chelating Properties in Pediatric Acute Myeloid Leukemia. Oncotarget. 12, 1377–1387. doi:10.18632/oncotarget.28000
Babushok, D. V. (2018). A Brief, but Comprehensive, Guide to Clonal Evolution in Aplastic Anemia. Hematol. Am. Soc. Hematol. Educ. Program 2018, 457–466. doi:10.1182/ASHEDUCATION-2018.1.457
Bao, W., Bussel, J. B., Heck, S., He, W., Karpoff, M., Boulad, N., et al. (2010). Improved Regulatory T-Cell Activity in Patients with Chronic Immune Thrombocytopenia Treated with Thrombopoietic Agents. Blood. 116, 4639–4645. doi:10.1182/blood-2010-04-281717
Boiocchi, L., Orazi, A., Ghanima, W., Arabadjief, M., Bussel, J. B., and Geyer, J. T. (2012). Thrombopoietin Receptor Agonist Therapy in Primary Immune Thrombocytopenia Is Associated with Bone Marrow Hypercellularity and Mild Reticulin Fibrosis but Not Other Stromal Abnormalities. Mod. Pathol. 25, 65–74. doi:10.1038/modpathol.2011.128
Brynes, R. K., Wong, R. S., Thein, M. M., Bakshi, K. K., Burgess, P., Theodore, D., et al. (2017). A 2-Year, Longitudinal, Prospective Study of the Effects of Eltrombopag on Bone Marrow in Patients with Chronic Immune Thrombocytopenia. Acta Haematol. 137, 66–72. doi:10.1159/000452992
Buckstein, R. (2015). Eltrombopag in Patients with High-Risk Myelodysplastic Syndrome or Acute Myeloid Leukaemia: Cautious Optimism. Lancet Haematol. 2, e396–7. doi:10.1016/S2352-3026(15)00200-8
Calado, R. T. (2011). Immunologic Aspects of Hypoplastic Myelodysplastic Syndrome. Semin. Oncol. 38, 667–672. doi:10.1053/J.SEMINONCOL.2011.04.006
Carli, C., Giroux, M., and Delisle, J. S. (2012). Roles of Transforming Growth Factor-β in Graft-Versus-Host and Graft-Versus-Tumor Effects. Biol. Blood Marrow Transpl. 18, 1329–1340. doi:10.1016/j.bbmt.2012.01.020
Chen, J., Wang, H., Zhou, J., and Feng, S. (2020). Advances in the Understanding of Poor Graft Function Following Allogeneic Hematopoietic Stem-Cell Transplantation. Ther. Adv. Hematol. 11, 2040620720948743. doi:10.1177/2040620720948743
Cooke, K. R., Luznik, L., Sarantopoulos, S., Hakim, F. T., Jagasia, M., Fowler, D. H., et al. (2017). The Biology of Chronic Graft-Versus-Host Disease: A Task Force Report from the National Institutes of Health Consensus Development Project on Criteria for Clinical Trials in Chronic Graft-Versus-Host Disease. Biol. Blood Marrow Transpl. 23, 211–234. doi:10.1016/j.bbmt.2016.09.023
de Graaf, C. A., and Metcalf, D. (2011). Thrombopoietin and Hematopoietic Stem Cells. Cell Cycle 10, 1582–1589. doi:10.4161/CC.10.10.15619
de Latour, R. P., Visconte, V., Takaku, T., Wu, C., Erie, A. J., Sarcon, A. K., et al. (2010). Th17 Immune Responses Contribute to the Pathophysiology of Aplastic Anemia, Blood. 116, 4175, 4184. doi:10.1182/blood-2010-01-266098
Deng, Y., Madatian, A., Wire, M. B., Bowen, C., Park, J. W., Williams, D., et al. (2011). Metabolism and Disposition of Eltrombopag, an Oral, Nonpeptide Thrombopoietin Receptor Agonist, in Healthy Human Subjects. Drug Metab. Dispos. 39, 1734–1746. doi:10.1124/DMD.111.040170
Desmond, R., Townsley, D. M., Dumitriu, B., Olnes, M. J., Scheinberg, P., Bevans, M., et al. (2014). Eltrombopag Restores Trilineage Hematopoiesis in Refractory Severe Aplastic Anemia that Can Be Sustained on Discontinuation of Drug. Blood. 123, 1818–1825. doi:10.1182/blood-2013-10-534743
di Buduo, C. A., Currao, M., Pecci, A., Kaplan, D. L., Balduini, C. L., and Balduini, A. (2016). Revealing Eltrombopag's Promotion of Human Megakaryopoiesis through AKT/ERK-dependent Pathway Activation. Haematologica 101, 1479–1488. doi:10.3324/haematol.2016.146746
di Paola, A., Palumbo, G., Merli, P., Argenziano, M., Tortora, C., Strocchio, L., et al. (2021). Effects of Eltrombopag on In Vitro Macrophage Polarization in Pediatric Immune Thrombocytopenia. Ijms 22, 97–12. doi:10.3390/ijms22010097
Dickinson, M., Cherif, H., Fenaux, P., Mittelman, M., Verma, A., Portella, M. S. O., et al. (2018). Azacitidine with or without Eltrombopag for First-Line Treatment of Intermediate- or High-Risk MDS with Thrombocytopenia. Blood. 132, 2629–2638. doi:10.1182/blood-2018-06-855221
Drexler, B., and Passweg, J. (2021). Current Evidence and the Emerging Role of Eltrombopag in Severe Aplastic Anemia. Ther. Adv. Hematol. 12, 2040620721998126. doi:10.1177/2040620721998126
Dyba, J., Tinmouth, A., Bredeson, C., Matthews, J., and Allan, D. S. (2016). Eltrombopag after Allogeneic Haematopoietic Cell Transplantation in a Case of Poor Graft Function and Systematic Review of the Literature. Transfus. Med. 26, 202–207. doi:10.1111/tme.12300
Erickson-Miller, C. L., Delorme, E., Tian, S. S., Hopson, C. B., Landis, A. J., Valoret, E. I., et al. (2009). Preclinical Activity of Eltrombopag (SB-497115), an Oral, Nonpeptide Thrombopoietin Receptor Agonist. Stem Cells 27, 424–430. doi:10.1634/STEMCELLS.2008-0366
Frey, N., Jang, J. H., Szer, J., Illés, Á., Kim, H. J., Ram, R., et al. (2019). Eltrombopag Treatment during Induction Chemotherapy for Acute Myeloid Leukaemia: a Randomised, Double-Blind, Phase 2 Study. Lancet Haematol. 6, e122–e131. doi:10.1016/S2352-3026(18)30231-X
Fujita, S., Nakanishi, T., Yoshimura, H., Hotta, M., Nakamichi, N., Tamaki, T., et al. (2012). TGFβ(1) and sCTLA-4 Levels Are Increased in Eltrombopag-Exposed Patients with ITP. Thromb. Res. 130, 415–419. doi:10.1016/j.thromres.2012.06.002
Ghanima, W., Cooper, N., Rodeghiero, F., Godeau, B., and Bussel, J. B. (2019). Thrombopoietin Receptor Agonists: Ten Years Later. Haematologica 104, 1112–1123. doi:10.3324/HAEMATOL.2018.212845
Ghanima, W., Geyer, J. T., Lee, C. S., Boiocchi, L., Imahiyerobo, A. A., Orazi, A., et al. (2014). Bone Marrow Fibrosis in 66 Patients with Immune Thrombocytopenia Treated with Thrombopoietin-Receptor Agonists: a Single-Center, Long-Term Follow-Up. Haematologica 99, 937–944. doi:10.3324/HAEMATOL.2013.098921
Gilreath, J., Lo, M., and Bubalo, J. (2021). Thrombopoietin Receptor Agonists (TPO-RAs): Drug Class Considerations for Pharmacists. Drugs 81, 1285–1305. doi:10.1007/s40265-021-01553-7
Gómez-Almaguer, D. (2018). Eltrombopag-based Combination Treatment for Immune Thrombocytopenia. Ther. Adv. Hematol. 9, 309–317. doi:10.1177/2040620718798798
González-López, T. J., Pascual, C., Álvarez-Román, M. T., Fernández-Fuertes, F., Sánchez-González, B., Caparrós, I., et al. (2015). Successful Discontinuation of Eltrombopag after Complete Remission in Patients with Primary Immune Thrombocytopenia. Am. J. Hematol. 90, E40–E43. doi:10.1002/ajh.23900
Halahleh, K., Gale, R. P., Da'na, W., Ma'koseh, M., Saadeh, S., Alan, W., et al. (2021). Therapy of Posttransplant Poor Graft Function with Eltrombopag. Bone Marrow Transpl. 56, 4–6. doi:10.1038/s41409-020-0975-5
Hess, N. J., Brown, M. E., and Capitini, C. M. (2021). GVHD Pathogenesis, Prevention and Treatment: Lessons From Humanized Mouse Transplant Models. Front. Immunol. 12, 723544. doi:10.3389/fimmu.2021.723544
Kalota, A., Selak, M. A., Garcia-Cid, L. A., and Carroll, M. (2015). Eltrombopag Modulates Reactive Oxygen Species and Decreases Acute Myeloid Leukemia Cell Survival. PLoS ONE 10, e0126691. doi:10.1371/journal.pone.0126691
Li, X., Zhong, H., Bao, W., Boulad, N., Evangelista, J., Haider, M. A., et al. (2012). Defective Regulatory B-Cell Compartment in Patients with Immune Thrombocytopenia. Blood 120, 3318–3325. doi:10.1182/blood-2012-05-432575
Liu, H., Ouyang, X., Li, Y., Zeng, H., Wang, X., Xie, S., et al. (2013). Involvement of Levels of Toll like Receptor-4 in Monocytes, CD4+ T-Lymphocyte Subsets, and Cytokines in Patients with Immune Thrombocytopenic Purpura. Thromb. Res. 132, 196–201. doi:10.1016/j.thromres.2013.04.025
Liu, M., Li, S., and Li, M. O. (2018). TGF-β Control of Adaptive Immune Tolerance: A Break From Treg Cells. Bioessays. 40, e1800063. doi:10.1002/bies.201800063
Liu, X. G., Liu, S., Feng, Q., Liu, X. N., Li, G. S., Sheng, Z., et al. (2016). Thrombopoietin Receptor Agonists Shift the Balance of Fcγ Receptors toward Inhibitory Receptor IIb on Monocytes in ITP. Blood 128, 852–861. doi:10.1182/blood-2016-01-690727
Lozano, M. L., Segú-Vergés, C., Coma, M., Álvarez-Roman, M. T., González-Porras, J. R., Gutiérrez, L., et al. (2021). Elucidating the Mechanism of Action of the Attributed Immunomodulatory Role of Eltrombopag in Primary Immune Thrombocytopenia: An In Silico Approach. Int. J. Mol. Sci. 22, 6907. doi:10.3390/ijms22136907
Lusis, A. J. (2021). A Vicious Cycle in Atherosclerosis. Cell 184, 1139–1141. doi:10.1016/J.CELL.2021.02.005
Mahévas, M., Fain, O., Ebbo, M., Roudot-Thoraval, F., Limal, N., Khellaf, M., et al. (2014). The Temporary Use of Thrombopoietin-Receptor Agonists May Induce a Prolonged Remission in Adult Chronic Immune Thrombocytopenia. Results of a French Observational Study. Br. J. Haematol. 165, 865–869. doi:10.1111/BJH.12888
Marotta, S., Marano, L., Ricci, P., Cacace, F., Frieri, C., Simeone, L., et al. (2019). Eltrombopag for Post-transplant Cytopenias Due to Poor Graft Function. Bone Marrow Transpl. 54, 1346–1353. doi:10.1038/s41409-019-0442-3
Mavroudi, I., Pyrovolaki, K., Pavlaki, K., Kozana, A., Psyllaki, M., Kalpadakis, C., et al. (2011). Effect of the Nonpeptide Thrombopoietin Receptor Agonist Eltrombopag on Megakaryopoiesis of Patients with Lower Risk Myelodysplastic Syndrome. Leuk. Res. 35, 323–328. doi:10.1016/j.leukres.2010.06.029
Medinger, M., Drexler, B., Lengerke, C., and Passweg, J. (2018). Pathogenesis of Acquired Aplastic Anemia and the Role of the Bone Marrow Microenvironment. Front. Oncol. 8, 587. doi:10.3389/FONC.2018.00587/BIBTEX
Mittelman, M., Platzbecker, U., Afanasyev, B., Grosicki, S., Wong, R. S. M., Anagnostopoulos, A., et al. (2018). Eltrombopag for Advanced Myelodysplastic Syndromes or Acute Myeloid Leukaemia and Severe Thrombocytopenia (ASPIRE): a Randomised, Placebo-Controlled, Phase 2 Trial. Lancet Haematol. 5, e34–e43. doi:10.1016/S2352-3026(17)30228-4
Monaco, G., Lee, B., Xu, W., Mustafah, S., Hwang, Y. Y., Carré, C., et al. (2019). RNA-Seq Signatures Normalized by mRNA Abundance Allow Absolute Deconvolution of Human Immune Cell Types. Cell Rep. 26, 1627–e7. doi:10.1016/j.celrep.2019.01.041
Ninos, J. M., Jefferies, L. C., Cogle, C. R., and Kerr, W. G. (2006). The Thrombopoietin Receptor, C-Mpl, Is a Selective Surface Marker for Human Hematopoietic Stem Cells. J. Transl. Med. 4, 9–18. doi:10.1186/1479-5876-4-9/TABLES/5
Nishimoto, T., Numajiri, M., Nakazaki, H., Okazaki, Y., and Kuwana, M. (2014). Induction of Immune Tolerance to Platelet Antigen by Short-Term Thrombopoietin Treatment in a Mouse Model of Immune Thrombocytopenia. Int. J. Hematol. 100, 341–344. doi:10.1007/s12185-014-1661-4
Olnes, M. J., Scheinberg, P., Calvo, K. R., Desmond, R., Tang, Y., Dumitriu, B., et al. (2012). Eltrombopag and Improved Hematopoiesis in Refractory Aplastic Anemia. N. Engl. J. Med. 367, 11–19. doi:10.1056/nejmoa1200931
Penack, O., Peczynski, C., van der Werf, S., Finke, J., Ganser, A., Schoemans, H., et al. (2020). Association of Serum Ferritin Levels Before Start of Conditioning With Mortality After alloSCT - A Prospective, Non-interventional Study of the EBMT Transplant Complications Working Party. Front. Immunol. 11, 586. doi:10.3389/fimmu.2020.00586
Platzbecker, U., Wong, R. S., Verma, A., Abboud, C., Araujo, S., Chiou, T. J., et al. (2015). Safety and Tolerability of Eltrombopag versus Placebo for Treatment of Thrombocytopenia in Patients with Advanced Myelodysplastic Syndromes or Acute Myeloid Leukaemia: A Multicentre, Randomised, Placebo-Controlled, Double-Blind, Phase 1/2 Trial. Lancet Haematol. 2, e417–26. doi:10.1016/S2352-3026(15)00149-0
Punzo, F., Tortora, C., Argenziano, M., Casale, M., Perrotta, S., and Rossi, F. (2018). Iron Chelating Properties of Eltrombopag: Investigating its Role in Thalassemia-Induced Osteoporosis. PLoS ONE 13, e0208102. doi:10.1371/journal.pone.0208102
Raslova, H., Vainchenker, W., and Plo, I. (2016). Eltrombopag, a Potent Stimulator of Megakaryopoiesis. Haematologica 101, 1443–1445. doi:10.3324/HAEMATOL.2016.153668
Roth, M., Will, B., Simkin, G., Narayanagari, S., Barreyro, L., Bartholdy, B., et al. (2012). Eltrombopag Inhibits the Proliferation of Leukemia Cells via Reduction of Intracellular Iron and Induction of Differentiation. Blood 120, 386–394. doi:10.1182/BLOOD-2011-12-399667
Sadallah, S., Amicarella, F., Eken, C., Iezzi, G., and Schifferli, J. A. (2014). Ectosomes Released by Platelets Induce Differentiation of CD4+T Cells into T Regulatory Cells. Thromb. Haemost. 112, 1219–1229. doi:10.1160/TH14-03-0281/ID/JR0281-6
Sadallah, S., Eken, C., and Schifferli, J. A. (2011). Ectosomes as Immunomodulators. Semin. Immunopathol. 33, 487–495. doi:10.1007/S00281-010-0232-X/FIGURES/4
Sayed, A. A., Malik, A., Ayoola, G., Lucchini, E., Candrianita, S., Tan, M. H., et al. (2019). Eltrombopag: More Than Just a Thrombopoietin Receptor Agonist (TPO-RA) in Immune Thrombocytopenia (ITP), Haematologica. 134, 2364. doi:10.1182/blood-2019-122848
Scheinberg, P. (2018). Activity of Eltrombopag in Severe Aplastic Anemia. Hematol. Am. Soc. Hematol. Educ. Program 2018, 450–456. doi:10.1182/bloodadvances.2018020248
Schmiedel, B. J., Singh, D., Madrigal, A., Valdovino-Gonzalez, A. G., White, B. M., Zapardiel-Gonzalo, J., et al. (2018). Impact of Genetic Polymorphisms on Human Immune Cell Gene Expression. Cell 175, 1701–e16. doi:10.1016/j.cell.2018.10.022
Schoettler, M. L., and Nathan, D. G. (2018). The Pathophysiology of Acquired Aplastic Anemia: Current Concepts Revisited. Hematol. Oncol. Clin. North Am. 32, 581–594. doi:10.1016/J.HOC.2018.03.001
Shi, M., Xu, F., Yang, X., Bai, Y., Niu, J., Drokow, E. K., et al. (2019). The Synergistic Antileukemic Effects of Eltrombopag and Decitabine in Myeloid Leukemia Cells. Cancer Manag. Res. 11, 8229–8238. doi:10.2147/CMAR.S213931
Spitz, A. Z., Zacharioudakis, E., Reyna, D. E., Garner, T. P., and Gavathiotis, E. (2021). Eltrombopag Directly Inhibits BAX and Prevents Cell Death. Nat. Commun. 12, 1134. doi:10.1038/s41467-021-21224-1
Tang, C., Chen, F., Kong, D., Ma, Q., Dai, H., Yin, J., et al. (2018). Successful Treatment of Secondary Poor Graft Function Post Allogeneic Hematopoietic Stem Cell Transplantation with Eltrombopag. J. Hematol. Oncol. 11, 103. doi:10.1186/s13045-018-0649-6
Vicente, A., Patel, B. A., Gutierrez-Rodrigues, F., Groarke, E., Giudice, V., Lotter, J., et al. (2020). Eltrombopag Monotherapy Can Improve Hematopoiesis in Patients with Low to Intermediate Risk-1 Myelodysplastic Syndrome. Haematologica 105, 2785–2794. doi:10.3324/haematol.2020.249995
Vlachodimitropoulou, E., Chen, Y. L., Garbowski, M., Koonyosying, P., Psaila, B., Sola-Visner, M., et al. (2017). Eltrombopag: a Powerful Chelator of Cellular or Extracellular Iron(III) Alone or Combined with a Second Chelator. Blood. 130, 1923–1933. doi:10.1182/blood-2016-10-740241
Vogel, J. U., Schmidt, S., Schmidt, D., Rothweiler, F., Koch, B., Baer, P., et al. (2019). The Thrombopoietin Receptor Agonist Eltrombopag Inhibits Human Cytomegalovirus Replication Via Iron Chelation. Cells. 9, 31. doi:10.3390/cells9010031
Will, B., Kawahara, M., Luciano, J. P., Bruns, I., Parekh, S., Erickson-Miller, C. L., et al. (2009). Effect of the Nonpeptide Thrombopoietin Receptor Agonist Eltrombopag on Bone Marrow Cells from Patients with Acute Myeloid Leukemia and Myelodysplastic Syndrome. Blood 114, 3899–3908. doi:10.1182/blood-2009-04-219493
Winkler, T., Fan, X., Cooper, J., Desmond, R., Young, D. J., Townsley, D. M., et al. (2019). Treatment Optimization and Genomic Outcomes in Refractory Severe Aplastic Anemia Treated with Eltrombopag. Blood 133, 2575–2585. doi:10.1182/BLOOD.2019000478
Wire, M. B., Bruce, J., Gauvin, J., Pendry, C. J., McGuire, S., Qian, Y., et al. (2012). A Randomized, Open-Label, 5-period, Balanced Crossover Study to Evaluate the Relative Bioavailability of Eltrombopag Powder for Oral Suspension (PfOS) and Tablet Formulations and the Effect of a High-Calcium Meal on Eltrombopag Pharmacokinetics when Administered with or 2 hours before or after PfOS. Clin. Ther. 34, 699–709. doi:10.1016/J.CLINTHERA.2012.01.011
Wong, R. S. M., Saleh, M. N., Khelif, A., Salama, A., Portella, M. S. O., Burgess, P., et al. (2017). Safety and Efficacy of Long-Term Treatment of Chronic/persistent ITP with Eltrombopag: Final Results of the EXTEND Study. Blood 130, 2527–2536. doi:10.1182/BLOOD-2017-04-748707
Yuan, C., Boyd, A. M., Nelson, J., Patel, R. D., Varela, J. C., Goldstein, S. C., et al. (2019). Eltrombopag for Treating Thrombocytopenia after Allogeneic Stem Cell Transplantation. Biol. Blood Marrow Transpl. 25, 1320–1324. doi:10.1016/j.bbmt.2019.01.027
Keywords: eltrombopag, immune thrombocytopenia, severe aplastic anemia, myelodysplastic syndrome, acute myeloid leukemia, poor graft function
Citation: Tarantini F, Cumbo C, Anelli L, Zagaria A, Conserva MR, Redavid I, Specchia G, Musto P and Albano F (2022) Exploring the Potential of Eltrombopag: Room for More?. Front. Pharmacol. 13:906036. doi: 10.3389/fphar.2022.906036
Received: 28 March 2022; Accepted: 06 May 2022;
Published: 23 May 2022.
Edited by:
Fabrizio Martelli, National Center for Drug Research and Evaluation (NIH) (ISS), ItalyReviewed by:
Naoshi Obara, University of Tsukuba, JapanCopyright © 2022 Tarantini, Cumbo, Anelli, Zagaria, Conserva, Redavid, Specchia, Musto and Albano. This is an open-access article distributed under the terms of the Creative Commons Attribution License (CC BY). The use, distribution or reproduction in other forums is permitted, provided the original author(s) and the copyright owner(s) are credited and that the original publication in this journal is cited, in accordance with accepted academic practice. No use, distribution or reproduction is permitted which does not comply with these terms.
*Correspondence: Francesco Albano, francesco.albano@uniba.it
†These authors have contributed equally to this work