- 1State Key Laboratory of Respiratory Disease, National Clinical Research Center for Respiratory Disease, Guangzhou Institute of Respiratory Health, The First Affiliated Hospital of Guangzhou Medical University, Guangzhou, Guangdong, China
- 2Innovation Centre for Advanced Interdisciplinary Medicine, Key Laboratory of Biological Targeting Diagnosis, Therapy and Rehabilitation of Guangdong Higher Education Institutes, The Fifth Affiliated Hospital of Guangzhou Medical University, Guangzhou, Guangdong, China
- 3Huizhou Municipal Central Hospital, Guangzhou, Guangdong, China
Umbilical cord mesenchymal stem cells (UCMSCs) are a reportedly promising choice in the treatment of irreversible pulmonary fibrosis and lethal interstitial lung disease with limited drug treatment options. In this study, we investigated the therapeutic efficacy of UCMSCs overexpressing hepatocyte growth factor (HGF), which is considered one of the main anti-fibrotic factors secreted by MSCs. Adenovirus vector carrying the HGF gene was transfected into UCMSCs to produce HGF-modified UCMSCs (HGF-UCMSCs). Transfection promoted the proliferation of UCMSCs and did not change the morphology, and differentiation ability, or biomarkers. Rats were injected with HGF-UCMSCs on days 7 and 11 after intratracheal administration of bleomycin (10 mg/kg). We performed an analysis of histopathology and lung function to evaluate the anti-fibrotic effect. The results showed that HGF-UCMSCs decreased the Ashcroft scores in hematoxylin and eosin-stained sections, the percentage positive area in Masson trichrome-stained sections, and the hydroxyproline level in lungs. Forced expiratory volume in the first 300 m/forced vital capacity was also improved by HGF-UCMSCs. To explore the possible therapeutic mechanism of HGF-UCMSCs, we detected inflammatory factors in the lungs and performed mRNA sequencing in UCMSCs and HGF-UCMSCs. The data indicated that inhibition of interleukin-17 in the lung may be related to the anti-fibrosis of HGF-UCMSCs, and overexpressed HGF probably played a primary role in the treatment. Collectively, our study findings suggested that the overexpression of HGF may improve the anti-fibrotic effect of UCMSCs through directly or indirectly interacting with interleukin-17-producing cells in fibrotic lungs.
Introduction
Idiopathic pulmonary fibrosis (IPF) is one of the forms of chronic progressive fibrotic interstitial lung disease (Lederer and Martinez, 2018). Patients with IPF eventually develop and ultimately die of irreversible respiratory failure (Richeldi et al., 2017). The death rate among patients with IPF has gradually increased, with an annually increasing trend (Hutchinson et al., 2014; Raghu et al., 2014; Hutchinson et al., 2015; Dove et al., 2019; Navaratnam and Hubbard, 2019; Tran et al., 2020). At present, the most effective treatment is lung transplantation, but transplant rejection and a lack of donor’s lungs make it impossible for more patients to benefit from transplantation (Raghu et al., 2015; George et al., 2019). Although pirfenidone and nintedanib can improve lung function and exercise tolerance to some extent, it is still difficult to prevent IPF from progressing (King Jr et al., 2014; Richeldi et al., 2014). Thus, new curative methods for patients with IPF are urgently needed.
Mesenchymal stem cells (MSCs) have been widely proven to effectively treat pulmonary fibrosis in animals through secreting anti-fibrotic factors (Huang et al., 2015; Kotani et al., 2017; Chen et al., 2018). Given that MSCs were likely an effective way to cure patients with IPF, clinical studies were subsequently conducted. The results showed that MSC administration was safe and could delay the deterioration of pulmonary function over time (Tzouvelekis et al., 2013; Chambers et al., 2014; Glassberg et al., 2017). Nevertheless, the progression of pulmonary fibrosis still cannot be stopped. Therefore, researchers must find ways of enhancing the anti-fibrotic efficacy of MSCs.
Gene modification of anti-fibrotic factors is a valid means to achieve the abovementioned goal (Madrigal et al., 2014; Ocansey et al., 2020). The hepatocyte growth factor (HGF) is a crucial factor in anti-fibrosis (Dong et al., 2015; Cahill et al., 2016). It has been shown that HGF can repair damaged alveolar epithelium and inhibit the profibrotic ability of fibroblasts and myofibroblasts in the lungs (Mizuno et al., 2005; Lee et al., 2008; Shukla et al., 2009). Umbilical cord MSCs (UCMSCs) are most likely the appropriate choice for modification due to their naturally high secretion of HGF, abundant supply, and no invasive extraction or donor site morbidity (Prasanna et al., 2010; Balasubramanian et al., 2012; Han et al., 2013; El Omar et al., 2014; Marmotti et al., 2017; Kim et al., 2018; Al Naem et al., 2020). HGF-modified UCMSCs (HGF-UCMSCs) have been used in the treatment of bronchiolitis obliterans, wound healing, acute kidney injury, and liver fibrosis, among others (Chen et al., 2011; Li et al., 2015; Cao et al., 2016; Yin et al., 2020). However, the application of HGF-UCMSCs in the treatment of bleomycin-induced pulmonary fibrosis has rarely been reported. A comparison of the therapeutic effects between HGF-UCMSCs and identified anti-fibrotic drugs is also needful.
In the present study, we evaluated the therapeutic outcome of HGF-UCMSCs in rats with pulmonary fibrosis induced by bleomycin. The therapeutic mechanism of HGF-UCMSCs was also explored using lung cytokines detection together with mRNA sequencing.
Materials and methods
Isolation and culture of human UCMSCs
Clinical-grade UCMSCs were obtained from Beijing SH Biotechnology (http://www.bjshbio.com/). The isolation and culture of UCMSCs were based on previously described methods (Chu et al., 2019), as follows. The umbilical cord was minced into small pieces then washed thoroughly with phosphate buffer saline (PBS) (Gibco, United States) and digested using collagenase (Gibco, United States) at 37°C for 60 min. The digestion was stopped using an MSCs growth medium (Beijing SH Technology, China), and the digested mixture was passed through a 70-μm cell strainer (BD, United States) to obtain a single-cell suspension. All primary UCMSCs were seeded in flasks at a density of 8,000/cm2 and cultured at 37°C in a humidified atmosphere containing 5% CO2.
Production of HGF-UCMSCs
The protocol for producing HGF-UCMSCs was referred to in the previous paper (Wang et al., 2013). A replication-defective adenovirus expressing human HGF (Ad-HGF) and a replication-defective adenovirus not carrying exogenous genes (Ad-Null) were constructed with the AdEasy system (Stratagene, United States) and were purified by double cesium chloride density gradient ultracentrifugation. Ad-HGF and Ad-Null dissolved in storage buffer (Hanks’ buffer, 10% glycerol) were stored at −80°C. According to the previous protocol, UCMSCs were infected with 150 multiplicities of infection of Ad-Null or Ad-HGF. The cells were collected 48 h post-infection for further usage in vitro and in vivo experiments. Before treatment, the conditioned medium of MSCs infected with Ad-Null and Ad-HGF was collected for HGF testing according to the instructions in an HGF ELISA kit (ExCell, China). Medium not used for cell culture was utilized as a negative control in the ELISA reaction.
Identification of MSCs
Cultured MSCs were identified for cell morphology and adherence, immune surface markers, and differentiation potential (Nadri et al., 2002; Soleimani and Nadri, 2009). MSCs were photographed for observation using a fluorescence digital microscope BZ-X800 (Keyence, Japan). Immune surface markers (i.e., CD105, CD73, CD90, CD34, CD11b, CD19, CD45, and HLA-DR) were analyzed by flow cytometry using a Human MSC Analysis Kit (BD, United States). For osteogenesis or adipogenesis, MSCs were respectively incubated in an osteogenic or adipogenic medium (Cyagen, China) for 3 weeks, and were then fixed with methanol. A Leica DMI 3000B fluorescence microscope (Leica, Germany) was used for photographs of osteoblasts or adipoblasts stained with alizarin red or Oil Red O, respectively.
Animals and experimental design
Sprague-Dawley rats (6 weeks, 200–220 g) were purchased from Guangdong Medical Laboratory Animal Center (China) and were housed in a specific pathogen-free animal facility. All studies were approved by the Animal Ethics Committee of Guangdong Medical Laboratory Animal Center.
Rats were divided into five groups (six rats per group): a CTRL group, BLM group, UCMSC group, HGF-UCMSC group, and PFD group. A microsprayer aerosolizer (Yuyan Instruments Co., Ltd. China) (Figure 1) was used to deliver sterile PBS or bleomycin into the lungs. On day 0, rats in the CTRL group were instilled intratracheally with sterile PBS; the remaining rats were instilled with 10 mg/kg body weight bleomycin (Hanhui co. LTD., China). From day 7–20, rats in the PFD group were infused orally with 100 mg/kg body weight pirfenidone once a day. The remaining rats were injected with sterile PBS, UCMSCs, or HGF-UCMSCs via tail vein on both days 7 and 11, as follows. 1) CTRL group and BLM group: sterile PBS, 400 μL/day per rat; 2) UCMSC group: UCMSCs, 2 * 10^6/400 μL/day per rat; 3) HGF-UCMSC group: HGF-UCMSCs, 2 * 10^6/400 μL/day per rat.
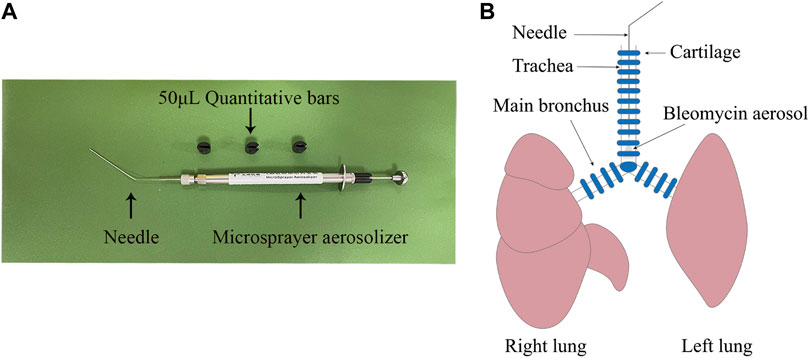
FIGURE 1. Establishment of pulmonary fibrosis model. (A) A microsprayer aerosolizer was used to deliver aerosols of PBS or bleomycin into the lungs of rats (B) The aerosols were presented in the lower tracheas.
For the collected lungs on day 21, left lungs were inflated and immersed with 4% paraformaldehyde (Biosharp, China), and right lungs were frozen at −80°C in a refrigerator as quickly as possible.
Lung function test
Rats were anesthetized with pentobarbital sodium and endotracheal intubation was performed. The intubated catheter was connected to a Buxco pulmonary function testing system (DSI Buxco, United States) to measure lung function as follows: forced expiratory volume in the first 300 m/forced vital capacity (FEV300/FVC), peak expiratory flow (PEF), chord compliance (Cchord), and total lung capacity.
Hydroxyproline evaluation
The right lung lobes of each rat were ground into a powder in liquid nitrogen and used for hydroxyproline (HYP) detection. To assess the total collagen content of lung tissue, we used an HYP assay kit (Nanjing Jiancheng Bioengineering Institute, China). The experiment was performed according to the manufacturer’s instructions.
Cytokine detection
Some lung tissue powder was prepared for cytokine detection, referring to the protocol of the Rat Cytokine/Chemokine Magnetic Bead Panel (Millipore, United States). Interleukin-17 (IL-17), IL-10, vascular endothelial growth factor (VEGF), and granulocyte-macrophage colony-stimulating factor (GM-CSF) were detected in 96-well plates using MILLIPLEX® MAGPIX with MILLIPLEX Analyst. V5.1 software. Median fluorescence intensity data were analyzed using a five-parameter logistic method for calculating analyte concentrations in samples.
Histopathological analysis
Left lungs were processed by Bios Biological Co. Ltd. (China). For paraffin sections, lungs were dehydrated using gradient ethanol and then embedded in paraffin blocks. The blocks were cut into sections of 3–5 μm thickness; sections were placed on polylysine-coated glass slides and stored at room temperature for further use. Hematoxylin and eosin (H&E) and Masson trichrome staining were performed following the standard protocol. The stained sections were captured, and the pictures were sent to two pathologists for evaluation. The severity of the injury was quantified using the Ashcroft scoring system in H&E-stained lung sections (Ashcroft et al., 1988). The percentage of blue-stained area in Masson trichrome-stained lung sections was quantified using ImageJ (National Institutes of Health, United States).
mRNA sequencing
UCMSCs (N = 3) and HGF-UCMSCs (N = 3) were lysed and total RNA was extracted using a Trizol reagent kit (Invitrogen, United States), following the manufacturer’s protocol. For mRNA sequencing, samples were submitted to Gene Denovo Biotechnology Co. (Guangzhou, China), where RNA quality evaluation, mRNA enrichment, and cDNA library preparation were performed. The cDNA libraries were sequenced on the Illumina sequencing platform Novaseq6000 by Gene Denovo Biotechnology Co., Ltd. (Guangzhou, China). RNA differential expression was analyzed using DESeq2 [7] software. Transcripts with a false discovery rate (FDR) < 0.05 and absolute fold change (FC) ≥ 2 were considered s differentially expressed genes/transcripts. The upregulation or downregulation of genes depended on the change in mean value of Fragments Per Kilobase of transcript per Million mapped reads (FPKM) in HGF-UCMSCs in comparison with UCMSCs. A volcanic map was drawn to display the differentially significant genes.
Statistical analysis
Statistical analysis was performed using GraphPad Prism 8.0 (GraphPad Software, Inc. United States). Two groups were compared using an unpaired t test. Comparisons among more than two groups were performed using a one-way analysis of variance, followed by Tukey’s multiple comparison test. All values were expressed as the mean ± standard deviation. P < 0.05 was considered statistically significant.
Results
Cell identification and quality control
In bright-field microscopy, both UCMSCs and HGF-UCMSCs were plastic-adherent and appeared spindle-shaped (Figure 2A). Ad-HGF transfection hardly changed the morphology of UCMSCs. Cultured in a specific differentiation induction medium, UCMSCs and HGF-UCMSCs differentiated into osteoblasts and adipoblasts (Figure 2A) with no obvious difference between the two groups. To identify MSC biomarkers, we performed flow cytometry. Overexpression of HGF did not alter the size and granularity of UCMSCs (Figures 2B, C). CD73, CD90, and CD105 were all positively expressed and CD34, CD11b, CD19, CD45, and HLA-DR were seldom expressed on the surface of the cells (Figures 2D–2G). Similar to the results of cell differentiation, gene modification did not influence the proportion of the markers mentioned above. To verify whether Ad-HGF transfection was successful, we measured the concentration of HGF in CM. The level of HGF was much higher in the CM of HGF-UCMSCs than that of UCMSCs (p < 0.0001) (Figure 2H). Moreover, HGF-UCMSCs proliferated faster than did UCMSCs (p = 0.0378) (Figure 2I). Collectively, the transfection of Ad-HGF promoted HGF secretion and cell proliferation without changing the basic characteristics of UCMSCs.
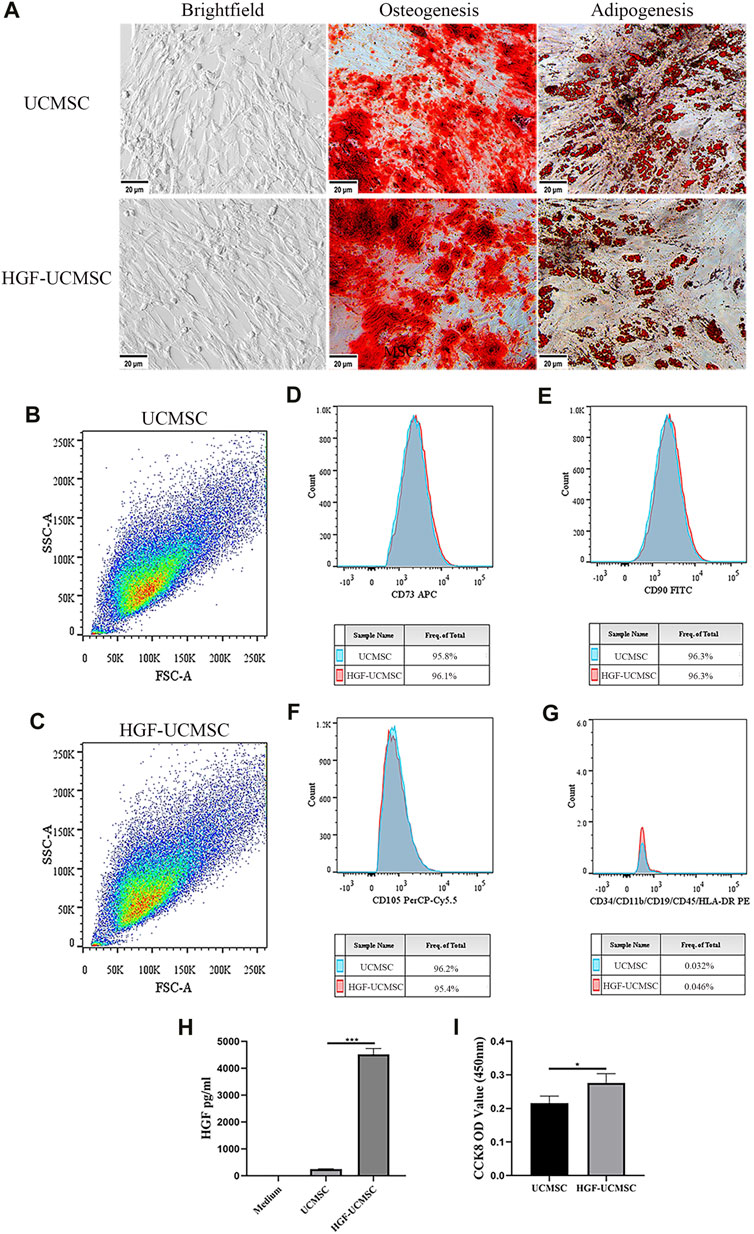
FIGURE 2. Characteristics of UCMSCs and HGF-UCMSCs. (A) Regularly cultured MSCs were induced into osteoblasts and adipoblasts stained with alizarin red and Oil Red O, respectively (magnification: ×100). (B–G) Flow cytometry was utilized to analyze the physical features and cell surface markers of MSCs (H) The concentration of HGF in the conditioned medium was detected using a commercial ELISA kit (I) The proliferation of MSCs was assessed by CCK8 assay. Values are presented as mean ± standard deviation. * p < 0.05; ** p < 0.01; *** p < 0.001.
Efficacy of HGF-UCMSCs in lung function
Before euthanizing the rats, we evaluated the physiological function of the lungs. Compared with the CTRL group, FEV300/FVC, PEF, Cchord and TLC in the BLM group decreased significantly (p < 0.05) (Figure 3). The PFD, UCMSC and HGF-UCMSC groups alleviated the pulmonary function injury, but the statistical difference was only found between the BLM and HGF-UCMSC group (p < 0.05). The therapeutic effect was not present for PEF, Cchord and TLC in the PFD, UCMSC and HGF-UCMSC group (p > 0.05).
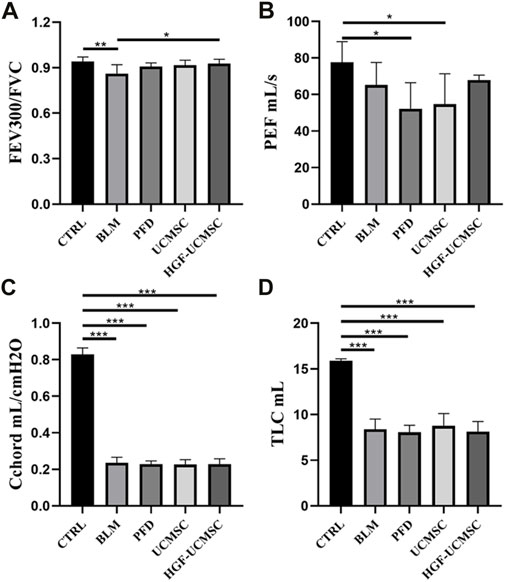
FIGURE 3. Lung function test on day 21 before euthanasia. (A,B) Pulmonary ventilatory function: forced expiratory volume in the first 300 m/forced vital capacity (FEV300/FVC); peak expiratory flow (PEF). (C) Pulmonary compliance: chord compliance (Cchord). (D) Pulmonary volume: total lung capacity (TLC). Values are presented in mean ± standard deviation. * p < 0.05; ** p < 0.01; *** p < 0.001.
Effect of HGF-UCMSCs on the improvement of lung structure
The histopathology was analyzed to estimate the improvement effect on lung structure. In H&E-stained sections, pulmonary fibrosis of differing degrees was induced in the other groups but was not seen in the CTRL group. The alveolar septum was filled with mesenchymal tissue stained with “acidophilic eosin” (Figure 4A). Ashcroft scores in the BLM group were significantly higher than that in the CTRL group (p < 0.05) (Figure 4B). The scores were decreased in all therapeutic groups, in which the PFD group got the lowest scores followed by the HGF-UCMSC group (p < 0.05). In sections stained with Masson trichrome, there was a large amount of collagen abnormally present in the interstitial lung tissue (Figure 4A). Similar to the result of Ashcroft scoring, the CTRL group showed significantly less Masson area% than the BLM group (p < 0.05) (Figure 4C). In comparison to the BLM group, the PFD, UCMSC and HGF-UCMSC groups significantly decreased the Masson area% (p < 0.05). Of note, the HGF-UCMSC group had the lowest p-value among the treatment groups in comparison with the BLM group (p = 0.0005). For the evaluation of collagen content, we detected HYP levels in the lungs. A higher level of HYP was present in the BLM group than in the CTRL group (p < 0.05) (Figure 4D). The treatment groups had various degrees of inhibitory effect on collagen deposition. HYP was significantly decreased in PFD and HGF-MSC group than the BLM group (PFD vs. BLM, p = 0.0378; HGF-UCMSC vs BLM, p = 0.0088); the UCMSC group exhibited less HYP, with no statistical significance (p > 0.05). Though the statistical difference was not present between the UCMSC and HGF-UCMSC groups, it is likely that HGF modification probably promoted the anti-fibrotic effect of UCMSCs in bleomycin-induced pulmonary fibrosis.
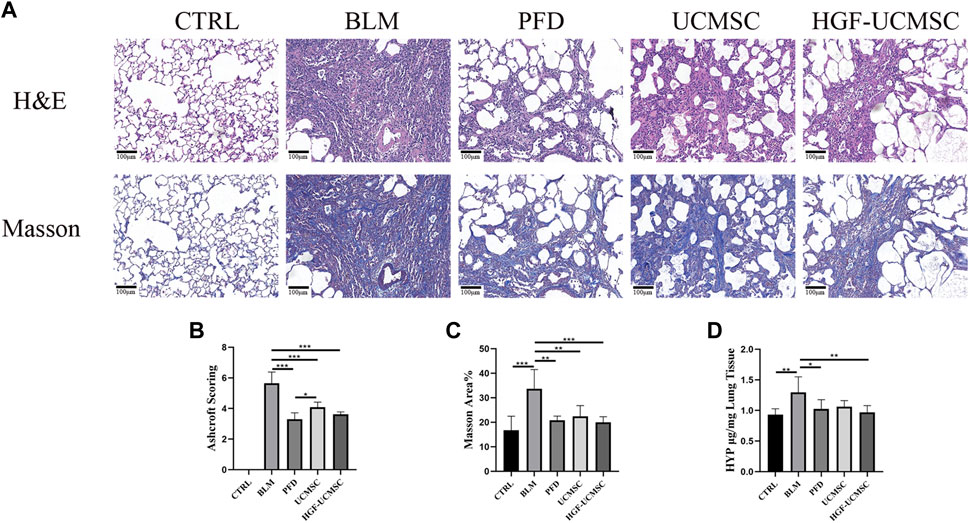
FIGURE 4. Evaluation of pulmonary histopathology. (A) Lung sections were stained with H&E or Masson’s trichrome (magnification: ×100). (B) Ashcroft scoring was performed in H&E-stained sections (C) The percentage positive area was calculated in Masson’s trichrome-stained sections (D) HYP concentrations were detected in the lungs. Values are presented in mean ± standard deviation. * p < 0.05; ** p < 0.01; *** p < 0.001.
Influence of HGF-UCMSC treatment on lung cytokines
To explain why HGF-UCMSCs had better efficacy than UCMSCs in treating pulmonary fibrosis, cytokines that may be involved were detected according to previous studies (François et al., 2015). The concentration of IL-17 in the BLM group was higher than that in the CTRL group (p < 0.05) (Figure 5A). HGF-UCMSCs had significantly decreased levels of IL-17 compared with the BLM, PFD, and UCMSC groups (p < 0.05). There was no significance in the PFD and UCMSC group in comparison with the BLM group (p > 0.05), although these two groups showed lower levels of IL-17. Compared with the CTRL group, all groups administered with bleomycin exhibited much lower levels of IL-10, and no significance was seen among the bleomycin groups (p > 0.05) (Figure 5B). Significantly decreased VEGF and GM-CSF levels were observed in the BLM, PFD, and HGF-UCMSC groups, as compared with the CTRL group (p < 0.05) (Figures 5C, D). The UCMSC group had a numerically higher level of VEGF and GM-CSF than did the PFD and HGF-UCMSC groups (p < 0.05), although there was no significant difference between the UCMSC and BLM groups (p > 0.05). In short, IL-17 may play a role in treatment with respect to HGF-UCMSCs.
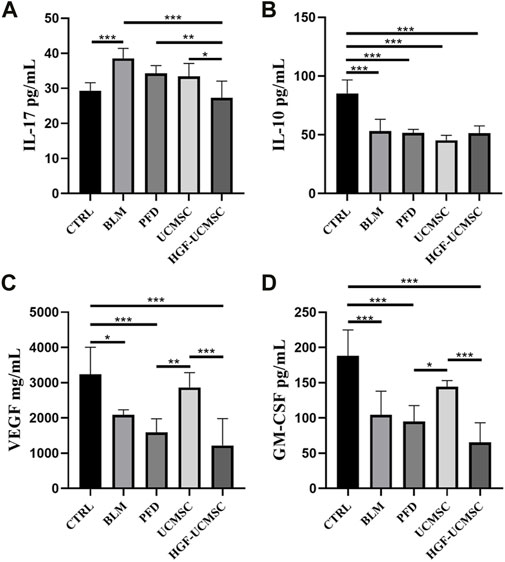
FIGURE 5. Detection of cytokines in the lungs. (A) Interleukin-17 (IL-17). (B) IL-10 (C) Vascular endothelial growth factor (VEGF). (D) Granulocyte-macrophage colony-stimulating factor (GM-CSF). Values are presented as mean ± standard deviation. * p < 0.05; ** p < 0.01; *** p < 0.001.
Transcriptome difference between UCMSCs and HGF-UCMSCs
Owing to few studies that have depicted the influence of HGF modification on UCMSCs, we conducted mRNA sequencing to uncover the transcriptional change of transfecting Ad-HGF into UCMSCs, thereby ascertaining whether HGF alone participates in treating pulmonary fibrosis. In total, 57% of genes were down-regulated and 43% of genes were up-regulated in all detected genes, without considering the statistical significance (Figure 6A). Among them, three genes (AC092718.2, POC1B-GALNT4 and AC007325.4) were significantly down-regulated, and five genes (HGF, DIO2, IGFBP5, SPTBN2 and SCRG1) were significantly up-regulated, with p values <0.05. (Figure 6B). As shown in Table 1, the log2(FC) value was higher and the FDR value was lower in the HGF gene than in the other genes, which suggested that the anti-fibrotic effect may be primarily owing to HGF.
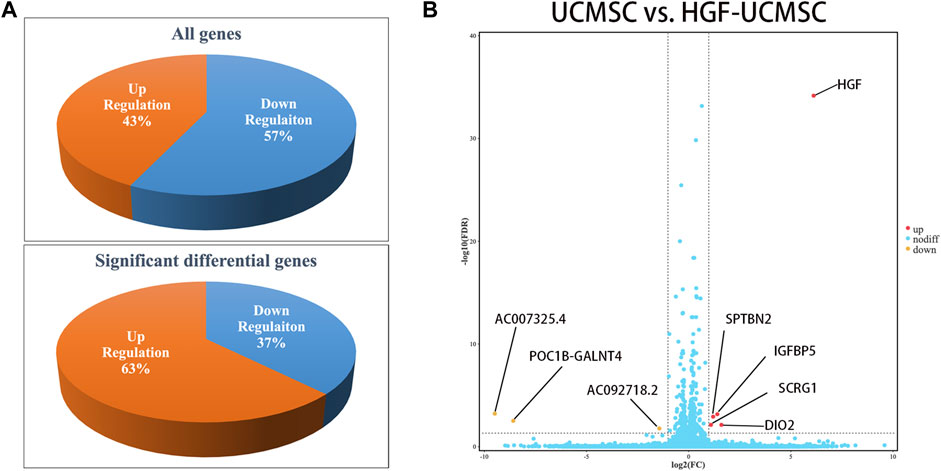
FIGURE 6. Analysis of transcriptional expression of UCMSCs and HGF- UCMSCs. (A) The percentage of up-regulated and down-regulated genes was calculated among all genes (upper panel) or the significant differential genes (lower panel) (B) Significant differential gene symbols are shown in the volcanic map.
Discussion
Gene modification is a promising strategy in the application of MSCs in treating pulmonary fibrosis (Min et al., 2015; Lan et al., 2017). As such, individually designed MSCs may function precisely in the treatment of pulmonary fibrosis. It has been demonstrated that HGF functions as a protective protein in pulmonary fibrosis through binding its receptor, c-met, which is expressed by many types of cells (e.g., epithelial cells, fibroblasts) (Dohi et al., 2000; Watanabe et al., 2005; Gazdhar et al., 2007; Gazdhar et al., 2013; Gazdhar et al., 2018). We therefore performed HGF modification in UCMSCs in order to enhance the anti-fibrotic ability of the cells. Our data showed that the modification improved the anti-fibrotic efficacy of UCMSCs in pulmonary ventilatory function and collagen deposition in the lung.
To explore the possible mechanism of HGF-UCMSCs in treating pulmonary fibrosis, we detected the cytokines that may participate in the process. The results showed that IL-17 may be affected by the treatment of HGF-UCMSCs. Produced by T helper 17 (Th17) cells, IL-17 acts as a driver of pulmonary fibrosis (François et al., 2015; Ting et al., 2017). The deletion of IL-17 in mice ameliorated the severity of pulmonary fibrosis induced by bleomycin (Wilson et al., 2010). In our study, compared with the BLM group, all treatment groups had numerically decreased IL-17 levels but only the HGF-UCMSC group showed a significant inhibitory effect (p < 0.0001, Figure 6A). Thus, HGF-UCMSCs may ameliorate pulmonary fibrosis by inhibiting IL-17 in the lung. Our data also showed that significantly lower IL-17 levels were seen in the HGF-UCMSC group than those in the UCMSC group (p = 0.0285), which means that the inhibitory effect may be associated with HGF modification. To explore whether other anti-fibrotic factors influenced the inhibition of IL-17 apart from HGF, we performed mRNA sequencing in UCMSCs and HGF-UCMSCs. The data showed that the transcriptional level of HGF was relatively higher than that of other significantly different genes, which suggested that HGF may play the main role in inhibiting IL-17. Interestingly, HGF secreted by MSCs is reported to mediate the differentiation from CD4+ cells to regulatory T cells but not Th17 cells, the IL-17-producing cells (Chen et al., 2020). Therefore, we propose the possible therapeutic mechanism that HGF-UCMSCs may directly or indirectly interact with CD4+ cells or Th17 cells through HGF in fibrotic lungs.
Apart from HGF, the transfection of Ad-HGF also change the transcriptional expression of other genes in UCMSCs. DIO2 acts as an activator of the thyroid hormone, which is critical for the maintenance of cellular homeostasis during stress responses (Sagliocchi et al., 2019). Yu et al. (Yu et al., 2018) reported that DIO2-knockout mice exhibited more severe pulmonary fibrosis. The authors also used thyroid hormone to treat pulmonary fibrosis in mice; their results showed increased survival and resolved lung fibrosis. Insulin-like growth factor binding protein 5 (IGFBP5) was reported as a pro-fibrotic factor in pulmonary fibrosis (Yasuoka et al., 2006). The expression of the human IGFBP5 gene in transgenic mice induced the up-regulation of ECM genes in the lungs (Nguyen et al., 2021). The role of crapie responsive gene 1 (SCRG1) in pulmonary fibrosis is unknown, but it was reported that SCRG1 is associated with the stemness of MSCs (Chosa and Ishisaki, 2018). The relationship between other significantly changed genes and pulmonary fibrosis or MSCs remains unclear in the present.
Our study had some limitations. First, HGF-UCMSCs did not show significantly anti-fibrotic effect in the comparison to UCMSCs, albeit the administration of HGF-UCMSCs but not wild-type UCMSCs significantly improved FEV300/FVC and lung HYP level in our study. This might be attributed to the use of bleomycin in over dosage that is higher than the dosage reported in the published papers (Lee et al., 2010; Rathinasabapathy et al., 2016; Chu et al., 2019). Second, we did not investigate the specific mechanism of the interaction between HGF-UCMSCs and IL-17-producing cells. Thus, in vitro and in vivo experiments are needed to confirm whether IL-17-producing cells are the targets of HGF and whether IGFBP5 and SCRG1 participate in the treatment of pulmonary fibrosis. Thrid, we did not explore how HGF-UCMSCs function at different stages after treatment and whether there is an appropriate time window for HGF-UCMSC treatment for better therapeutic efficacy. These may be the keys to reverse fibrosis. Fourth, it remains unclear how the levels of VEGF and GM-CSFin lungs were decreased by HGF-UCMSCs in comparison to UCMSCs. To sample in earlier timing after the administration of HGF-UCMSCs and to determine the main cells secreting VEGF and GM-CSF might be important to reveal the possible mechanism.
Conclusion
In this study, we confirmed that treatment with UCMSCs or HGF-UCMSCs could alleviate pulmonary fibrosis caused by bleomycin in mice. Furthermore, the enhancement of HGF secretion may improve the anti-fibrotic effect of UCMSCs. The improved anti-fibrotic effect may be associated with the inhibition of IL-17 in the lungs.
Data availability statement
The original contributions presented in the study are publicly available. This data can be found here: https://www.ncbi.nlm.nih.gov/sra/PRJNA915313, accession number PRJNA915313.
Ethics statement
The studies involving the isolation of UCMSCs from human participants were reviewed and approved by the Ethics Committee of Beijing 307 Hospital. The participants provided their written informed consent to participate in this study.
Author contributions
HC, YL, SL, and ML contributed to the study design. HC, YL, and YZ performed the experiments and manuscript writing. YY, DC, XS, and ZX participated in the implementation of some experiments and data analysis. All authors contributed to the article and approved the submitted version.
Funding
This research was supported by Innovation team of respiratory diseases and regenerative medicine (2021KCXTD028), Zhongnanshan medical foundation of Guangdong Province (ZNSA-2020013), the Guangzhou Science and Technology Project (202102010358) and Basic Research Foundation of Guangzhou (202102020916).
Acknowledgments
Clinical-grade UCMSCs were shared by Hua Wang, Yuefeng Yang, Chengfeng Sun and Xiaona Zhu from Beijing SH Biotechnology (Beijing, China). MILLIPLEX analysis was performed in Asbio Technology, Inc. (Guangzhou, China). mRNA sequencing and analysis were performed in Gene Denovo Biotechnology Co. (Guangzhou, China). The authors thank all of them for helping.
Conflict of interest
The authors declare that the research was conducted in the absence of any commercial or financial relationships that could be construed as a potential conflict of interest.
Publisher’s note
All claims expressed in this article are solely those of the authors and do not necessarily represent those of their affiliated organizations, or those of the publisher, the editors and the reviewers. Any product that may be evaluated in this article, or claim that may be made by its manufacturer, is not guaranteed or endorsed by the publisher.
References
Al Naem, M., Bourebaba, L., Kucharczyk, K., Röcken, M., and Marycz, K. (2020). Therapeutic mesenchymal stromal stem cells: Isolation, characterization and role in equine regenerative medicine and metabolic disorders. Stem Cell. Rev. Rep. 16 (2), 301–322. doi:10.1007/s12015-019-09932-0
Ashcroft, T., Simpson, J. M., and Timbrell, V. (1988). Simple method of estimating severity of pulmonary fibrosis on a numerical scale. J. Clin. Pathol. 41 (4), 467–470. doi:10.1136/jcp.41.4.467
Balasubramanian, S., Venugopal, P., Sundarraj, S., Zakaria, Z., Majumdar, A. S., and Ta, M. (2012). Comparison of chemokine and receptor gene expression between Wharton's jelly and bone marrow-derived mesenchymal stromal cells. Cytotherapy 14 (1), 26–33. doi:10.3109/14653249.2011.605119
Cahill, E. F., Kennelly, H., Carty, F., Mahon, B. P., and English, K. (2016). Hepatocyte growth factor is required for mesenchymal stromal cell protection against bleomycin-induced pulmonary fibrosis. Stem Cells Transl. Med. 5 (10), 1307–1318. doi:10.5966/sctm.2015-0337
Cao, X.-P., Han, D.-M., Zhao, L., Guo, Z.-K., Xiao, F.-J., Zhang, Y.-K., et al. (2016). Hepatocyte growth factor enhances the inflammation-alleviating effect of umbilical cord–derived mesenchymal stromal cells in a bronchiolitis obliterans model. Cytotherapy 18 (3), 402–412. doi:10.1016/j.jcyt.2015.12.006
Chambers, D. C., Enever, D., Ilic, N., Sparks, L., Whitelaw, K., Ayres, J., et al. (2014). A phase 1b study of placenta-derived mesenchymal stromal cells in patients with idiopathic pulmonary fibrosis. Respirology 19 (7), 1013–1018. doi:10.1111/resp.12343
Chen, Q. H., Wu, F., Liu, L., Chen, H. B., Yu, L. N., Wang, H. L., et al. (2020). Mesenchymal stem cells regulate the Th17/Treg cell balance partly through hepatocyte growth factor in vitro. Stem Cell. Res. Ther. 11 (1), 91. doi:10.1186/s13287-020-01612-y
Chen, S., Cui, G., Peng, C., Lavin, M. F., Sun, X., Zhang, E., et al. (2018). Transplantation of adipose-derived mesenchymal stem cells attenuates pulmonary fibrosis of silicosis via anti-inflammatory and anti-apoptosis effects in rats. Stem Cell. Res. Ther. 9 (1), 110–112. doi:10.1186/s13287-018-0846-9
Chen, Y., Qian, H., Zhu, W., Zhang, X., Yan, Y., Ye, S., et al. (2011). Hepatocyte growth factor modification promotes the amelioration effects of human umbilical cord mesenchymal stem cells on rat acute kidney injury. Stem Cells Dev. 20 (1), 103–113. doi:10.1089/scd.2009.0495
Chosa, N., and Ishisaki, A. (2018). Two novel mechanisms for maintenance of stemness in mesenchymal stem cells: SCRG1/BST1 axis and cell–cell adhesion through N-cadherin. Jpn. Dent. Sci. Rev. 54 (1), 37–44. doi:10.1016/j.jdsr.2017.10.001
Chu, K.-A., Wang, S.-Y., Yeh, C.-C., Fu, T.-W., Fu, Y.-Y., Ko, T.-L., et al. (2019). Reversal of bleomycin-induced rat pulmonary fibrosis by a xenograft of human umbilical mesenchymal stem cells from Wharton's jelly. Theranostics 9 (22), 6646–6664. doi:10.7150/thno.33741
Dohi, M., Hasegawa, T., Yamamoto, K., and Marshall, B. C. (2000). Hepatocyte growth factor attenuates collagen accumulation in a murine model of pulmonary fibrosis. Am. J. Respir. Crit. Care Med. 162 (6), 2302–2307. doi:10.1164/ajrccm.162.6.9908097
Dong, J., Yu, X., Porter, D. W., Battelli, L. A., Kashon, M. L., and Ma, Q. (2016). Common and distinct mechanisms of induced pulmonary fibrosis by particulate and soluble chemical fibrogenic agents. Arch. Toxicol. 90 (2), 385–402. doi:10.1007/s00204-015-1589-3
Dong, L.-H., Jiang, Y.-Y., Liu, Y.-J., Cui, S., Xia, C.-C., Qu, C., et al. (2015). The anti-fibrotic effects of mesenchymal stem cells on irradiated lungs via stimulating endogenous secretion of HGF and PGE2. Sci. Rep. 5 (1), 8713–8810. doi:10.1038/srep08713
Dove, E. P., Olson, A. L., and Glassberg, M. K. (2019). Trends in idiopathic pulmonary fibrosis–related mortality in the United States: 2000–2017. Am. J. Respir. Crit. Care Med. 200 (7), 929–931. doi:10.1164/rccm.201905-0958LE
El Omar, R., Beroud, J., Stoltz, J.-F., Menu, P., Velot, E., and Decot, V. (2014). Umbilical cord mesenchymal stem cells: The new gold standard for mesenchymal stem cell-based therapies?Tissue Eng. Part B Rev. 20 (5), 523–544. doi:10.1089/ten.teb.2013.0664
François, A., Gombault, A., Villeret, B., Alsaleh, G., Fanny, M., Gasse, P., et al. (2015). B cell activating factor is central to bleomycin-and IL-17-mediated experimental pulmonary fibrosis. J. Autoimmun. 56, 1–11. doi:10.1016/j.jaut.2014.08.003
Gazdhar, A., Fachinger, P., van Leer, C., Pierog, J., Gugger, M., Friis, R., et al. (2007). Gene transfer of hepatocyte growth factor by electroporation reduces bleomycin-induced lung fibrosis. Am. J. Physiol. Lung Cell. Mol. Physiol. 292, L529–L536. doi:10.1152/ajplung.00082.2006
Gazdhar, A., Fytianos, K., Knudsen, L., Schliep, R., Mueller, S., and Geiser, T. (2018). Electroporation of Hepatocyte growth factor to the lung induces migration of bone marrow mesenchymal stem cells and reduces lung fibrosis. Eur. Respir. Soc. doi:10.1183/13993003.congress-2018.PA593
Gazdhar, A., Temuri, A., Knudsen, L., Gugger, M., Schmid, R. A., Ochs, M., et al. (2013). Targeted gene transfer of hepatocyte growth factor to alveolar type II epithelial cells reduces lung fibrosis in rats. Hum. Gene Ther. 24 (1), 105–116. doi:10.1089/hum.2012.098
George, P. M., Patterson, C. M., Reed, A. K., and Thillai, M. (2019). Lung transplantation for idiopathic pulmonary fibrosis. Lancet. Respir. Med. 7 (3), 271–282. doi:10.1016/S2213-2600(18)30502-2
Glassberg, M. K., Minkiewicz, J., Toonkel, R. L., Simonet, E. S., Rubio, G. A., DiFede, D., et al. (2017). Allogeneic human mesenchymal stem cells in patients with idiopathic pulmonary fibrosis via intravenous delivery (AETHER): a phase I safety clinical trial. Chest 151 (5), 971–981. doi:10.1016/j.chest.2016.10.061
Habgood, A. N., Tatler, A. L., Porte, J., Wahl, S. M., Laurent, G. J., John, A. E., et al. (2016). Secretory leukocyte protease inhibitor gene deletion alters bleomycin-induced lung injury, but not development of pulmonary fibrosis. Lab. Investig. 96 (6), 623–631. doi:10.1038/labinvest.2016.40
Han, Y.-F., Tao, R., Sun, T.-J., Chai, J.-K., Xu, G., and Liu, J. (2013). Optimization of human umbilical cord mesenchymal stem cell isolation and culture methods. Cytotechnology 65 (5), 819–827. doi:10.1007/s10616-012-9528-0
Huang, K., Kang, X., Wang, X., Wu, S., Xiao, J., Li, Z., et al. (2015). Conversion of bone marrow mesenchymal stem cells into type II alveolar epithelial cells reduces pulmonary fibrosis by decreasing oxidative stress in rats. Mol. Med. Rep. 11 (3), 1685–1692. doi:10.3892/mmr.2014.2981
Hutchinson, J., Fogarty, A., Hubbard, R., and McKeever, T. (2015). Global incidence and mortality of idiopathic pulmonary fibrosis: A systematic review. Eur. Respir. J. 46 (3), 795–806. doi:10.1183/09031936.00185114
Hutchinson, J. P., McKeever, T. M., Fogarty, A. W., Navaratnam, V., and Hubbard, R. B. (2014). Increasing global mortality from idiopathic pulmonary fibrosis in the twenty-first century. Ann. Am. Thorac. Soc. 11 (8), 1176–1185. doi:10.1513/AnnalsATS.201404-145OC
Kim, J.-H., Jo, C. H., Kim, H.-R., and Hwang, Y.-i. (2018). Comparison of immunological characteristics of mesenchymal stem cells from the periodontal ligament, umbilical cord, and adipose tissue. Stem Cells Int. 2018, 8429042. doi:10.1155/2018/8429042
King, T. E., Bradford, W. Z., Castro-Bernardini, S., Fagan, E. A., Glaspole, I., Glassberg, M. K., et al. (2014). A phase 3 trial of pirfenidone in patients with idiopathic pulmonary fibrosis. N. Engl. J. Med. 370 (22), 2083–2092. doi:10.1056/NEJMoa1402582
Kotani, T., Masutani, R., Suzuka, T., Oda, K., Makino, S., and Ii, M. (2017). Anti-inflammatory and anti-fibrotic effects of intravenous adipose-derived stem cell transplantation in a mouse model of bleomycin-induced interstitial pneumonia. Sci. Rep. 7 (1), 14608–14610. doi:10.1038/s41598-017-15022-3
Lan, Y.-W., Theng, S.-M., Huang, T.-T., Choo, K.-B., Chen, C.-M., Kuo, H.-P., et al. (2017). Oncostatin M-preconditioned mesenchymal stem cells alleviate bleomycin-induced pulmonary fibrosis through paracrine effects of the hepatocyte growth factor. Stem Cells Transl. Med. 6 (3), 1006–1017. doi:10.5966/sctm.2016-0054
Lederer, D. J., and Martinez, F. J. (2018). Idiopathic pulmonary fibrosis. N. Engl. J. Med. 378 (19), 1811–1823. doi:10.1056/NEJMra1705751
Lee, S.-H., Jang, A.-S., Kim, Y.-E., Cha, J.-Y., Kim, T.-H., Jung, S., et al. (2010). Modulation of cytokine and nitric oxide by mesenchymal stem cell transfer in lung injury/fibrosis. Respir. Res. 11 (1), 16–14. doi:10.1186/1465-9921-11-16
Lee, Y. H., Suzuki, Y. J., Griffin, A. J., and Day, R. M. (2008). Hepatocyte growth factor regulates cyclooxygenase-2 expression via β-catenin, Akt, and p42/p44 MAPK in human bronchial epithelial cells. Am. J. Physiol. Lung Cell. Mol. Physiol. 294 (4), L778–L786. doi:10.1152/ajplung.00410.2007
Li, J., Zheng, C.-Q., Li, Y., Yang, C., Lin, H., and Duan, H.-G. (2015). Hepatocyte growth factor gene-modified mesenchymal stem cells augment sinonasal wound healing. Stem Cells Dev. 24 (15), 1817–1830. doi:10.1089/scd.2014.0521
Madrigal, M., Rao, K. S., and Riordan, N. H. (2014). A review of therapeutic effects of mesenchymal stem cell secretions and induction of secretory modification by different culture methods. J. Transl. Med. 12 (1), 260–314. doi:10.1186/s12967-014-0260-8
Marmotti, A., Mattia, S., Castoldi, F., Barbero, A., Mangiavini, L., Bonasia, D. E., et al. (2017). Allogeneic umbilical cord-derived mesenchymal stem cells as a potential source for cartilage and bone regeneration: An in vitro study. Stem Cells Int. 2017, 1732094. doi:10.1155/2017/1732094
Min, F., Gao, F., Li, Q., and Liu, Z. (2015). Therapeutic effect of human umbilical cord mesenchymal stem cells modified by angiotensin-converting enzyme 2 gene on bleomycin-induced lung fibrosis injury. Mol. Med. Rep. 11 (4), 2387–2396. doi:10.3892/mmr.2014.3025
Mizuno, S., Matsumoto, K., Li, M.-Y., and Nakamura, T. (2005). HGF reduces advancing lung fibrosis in mice: A potential role for MMP-dependent myofibroblast apoptosis. Faseb J. 19 (6), 580–582. doi:10.1096/fj.04-1535fje
Nadri, S., Soleimani, M., Hosseni, R. H., Massumi, M., Atashi, A., and Izadpanah, R. (2002). An efficient method for isolation of murine bone marrow mesenchymal stem cells. Int. J. Dev. Biol. 51 (8), 723–729. doi:10.1387/ijdb.072352ns
Navaratnam, V., and Hubbard, R. B. (2019). The mortality burden of idiopathic pulmonary fibrosis in the United Kingdom. Am. J. Respir. Crit. Care Med. 200 (2), 256–258. doi:10.1164/rccm.201902-0467LE
Nguyen, X.-X., Renaud, L., and Feghali-Bostwick, C. (2021). Identification of Impacted Pathways and Transcriptomic Markers as Potential Mediators of Pulmonary Fibrosis in Transgenic Mice Expressing Human IGFBP5. Int. J. Moi. Sci. 22 (22), 12609. doi:10.3390/ijms222212609
Ocansey, D. K. W., Pei, B., Yan, Y., Qian, H., Zhang, X., Xu, W., et al. (2020). Improved therapeutics of modified mesenchymal stem cells: An update. J. Transl. Med. 18 (1), 42–14. doi:10.1186/s12967-020-02234-x
Prasanna, S. J., Gopalakrishnan, D., Shankar, S. R., and Vasandan, A. B. (2010). Pro-inflammatory cytokines, IFNgamma and TNFalpha, influence immune properties of human bone marrow and Wharton jelly mesenchymal stem cells differentially. PLoS One 5 (2), e9016. doi:10.1371/journal.pone.0009016
Raghu, G., Chen, S.-Y., Yeh, W.-S., Maroni, B., Li, Q., Lee, Y.-C., et al. (2014). Idiopathic pulmonary fibrosis in US medicare beneficiaries aged 65 years and older: Incidence, prevalence, and survival, 2001–11. Lancet. Respir. Med. 2 (7), 566–572. doi:10.1016/S2213-2600(14)70101-8
Raghu, G., Rochwerg, B., Zhang, Y., Garcia, C. A. C., Azuma, A., Behr, J., et al. (2015). An official ATS/ERS/JRS/ALAT clinical practice guideline: Treatment of idiopathic pulmonary fibrosis. An update of the 2011 clinical practice guideline. Am. J. Respir. Crit. Care Med. 192 (2), e3–e19. doi:10.1164/rccm.201506-1063ST
Rathinasabapathy, A., Bruce, E., Espejo, A., Horowitz, A., Sudhan, D. R., Nair, A., et al. (2016). Therapeutic potential of adipose stem cell-derived conditioned medium against pulmonary hypertension and lung fibrosis. Br. J. Pharmacol. 173 (19), 2859–2879. doi:10.1111/bph.13562
Richeldi, L., Collard, H. R., and Jones, M. G. (2017). Idiopathic pulmonary fibrosis. Lancet 389 (10082), 1941–1952. doi:10.1016/S0140-6736(17)30866-8
Richeldi, L., Du Bois, R. M., Raghu, G., Azuma, A., Brown, K. K., Costabel, U., et al. (2014). Efficacy and safety of nintedanib in idiopathic pulmonary fibrosis. N. Engl. J. Med. 370 (22), 2071–2082. doi:10.1056/NEJMoa1402584
Sagliocchi, S., Cicatiello, A. G., Di Cicco, E., Ambrosio, R., Miro, C., Di Girolamo, D., et al. (2019). The thyroid hormone activating enzyme, type 2 deiodinase, induces myogenic differentiation by regulating mitochondrial metabolism and reducing oxidative stress. Redox Biol. 24, 101228. doi:10.1016/j.redox.2019.101228
Shukla, M. N., Rose, J. L., Ray, R., Lathrop, K. L., Ray, A., and Ray, P. (2009). Hepatocyte growth factor inhibits epithelial to myofibroblast transition in lung cells via Smad7. Am. J. Respir. Cell. Mol. Biol. 40 (6), 643–653. doi:10.1165/rcmb.2008-0217OC
Soleimani, M., and Nadri, S. (2009). A protocol for isolation and culture of mesenchymal stem cells from mouse bone marrow. Nat. Protoc. 4 (1), 102–106. doi:10.1038/nprot.2008.221
Ting, W., Yuan, L., Jing-Feng, Z., Zhen-Shun, C., and Aamir, A. (2017). Interleukin-17 induces human alveolar epithelial to mesenchymal cell transition via the TGF-β1 mediated Smad2/3 and ERK1/2 activation. Plos One 12 (9), e0183972. doi:10.1371/journal.pone.0183972
Tran, T., Šterclová, M., Mogulkoc, N., Lewandowska, K., Müller, V., Hájková, M., et al. (2020). The European MultiPartner IPF registry (EMPIRE): Validating long-term prognostic factors in idiopathic pulmonary fibrosis. Respir. Res. 21 (1), 11–19. doi:10.1186/s12931-019-1271-z
Tzouvelekis, A., Paspaliaris, V., Koliakos, G., Ntolios, P., Bouros, E., Oikonomou, A., et al. (2013). A prospective, non-randomized, no placebo-controlled, phase Ib clinical trial to study the safety of the adipose derived stromal cells-stromal vascular fraction in idiopathic pulmonary fibrosis. J. Transl. Med. 11 (1), 1–13. doi:10.1186/1479-5876-11-171
Wang, H., Yang, Y.-F., Zhao, L., Xiao, F.-J., Zhang, Q.-W., Wen, M.-L., et al. (2013). Hepatocyte growth factor gene-modified mesenchymal stem cells reduce radiation-induced lung injury. Hum. Gene Ther. 24 (3), 343–353. doi:10.1089/hum.2012.177
Watanabe, M., Ebina, M., Orson, F. M., Nakamura, A., Kubota, K., Koinuma, D., et al. (2005). Hepatocyte growth factor gene transfer to alveolar septa for effective suppression of lung fibrosis. Mol. Ther. 12 (1), 58–67. doi:10.1016/j.ymthe.2005.02.019
Wilson, M. S., Madala, S. K., Ramalingam, T. R., Gochuico, B. R., Rosas, I. O., Cheever, A. W., et al. (2010). Bleomycin and IL-1beta-mediated pulmonary fibrosis is IL-17A dependent. J. Exp. Med. 207 (3), 535–552. doi:10.1084/jem.20092121
Yasuoka, H., Zhou, Z., Pilewski, J. M., Oury, T. D., Choi, A. M. K., and Feghali-Bostwick, C. A. (2006). Insulin-like growth factor-binding protein-5 induces pulmonary fibrosis and triggers mononuclear cellular infiltration. Am. J. Pathol. 169 (5), 1633–1642. doi:10.2353/ajpath.2006.060501
Yin, F., Wang, W.-Y., Mao, L.-C., Cai, Q.-Q., and Jiang, W.-H. (2020). Effect of human umbilical cord mesenchymal stem cells transfected with HGF on TGF-β1/Smad signaling pathway in carbon tetrachloride-induced liver fibrosis rats. Stem Cells Dev. 29 (21), 1395–1406. doi:10.1089/scd.2020.0060
Keywords: umbilical cord, mesenchymal stem cells, pulmonary fibrosis, hepatocyte growth factor, interleukin-17
Citation: Chen H, Luo Y, Zhu Y, Ye Y, Chen D, Song X, Xiao Z, Liu M and Li S (2023) Enhanced secretion of hepatocyte growth factor in human umbilical cord mesenchymal stem cells ameliorates pulmonary fibrosis induced by bleomycin in rats. Front. Pharmacol. 13:1070736. doi: 10.3389/fphar.2022.1070736
Received: 15 October 2022; Accepted: 29 November 2022;
Published: 06 January 2023.
Edited by:
Changjun Lv, Binzhou Medical University Hospital, ChinaReviewed by:
Pavel Solopov, Old Dominion University, United StatesYing Meng, Southern Medical University, China
Copyright © 2023 Chen, Luo, Zhu, Ye, Chen, Song, Xiao, Liu and Li. This is an open-access article distributed under the terms of the Creative Commons Attribution License (CC BY). The use, distribution or reproduction in other forums is permitted, provided the original author(s) and the copyright owner(s) are credited and that the original publication in this journal is cited, in accordance with accepted academic practice. No use, distribution or reproduction is permitted which does not comply with these terms.
*Correspondence: Ming Liu, mingliu128@hotmail.com; Shiyue Li, lishiyue@188.com
†These authors have contributed equally to this work and share first authorship