- 1Zhongshan Institute for Drug Discovery, SIMM CAS, Zhongshan, Guangdong, China
- 2Shanghai Institute of Materia Medica, Chinese Academy of Sciences, Shanghai, China
- 3Department of Physiology, University of Toronto, Toronto, ON, Canada
- 4College of Pharmacy, Zunyi Medical University, Zunyi, Guizhou, China
- 5College of Pharmacy, Southern Medical University, Guangzhou, Guangdong, China
- 6University of Chinese Academy of Sciences, Beijing, China
- 7College of Food Science, Shenyang Agricultural University, Shenyang, Liaoning, China
- 8Department of Cardiology, Zhongshan Hospital Affiliated with Guangzhou University of Chinese Medicine (Zhongshan Hospital of Traditional Chinese Medicine), Zhongshan, Guangdong, China
- 9Guangzhou University of Chinese Medicine, Guangzhou, China
- 10College of Life Sciences, China Jiliang University, Hangzhou, Zhejiang, China
Ischemic heart disease (IHD) is a high-risk disease in the middle-aged and elderly population. The ischemic heart may be further damaged after reperfusion therapy with percutaneous coronary intervention (PCI) and other methods, namely, myocardial ischemia–reperfusion injury (MIRI), which further affects revascularization and hinders patient rehabilitation. Therefore, the investigation of new therapies against MIRI has drawn great global attention. Within the long history of the prevention and treatment of MIRI, traditional Chinese medicine (TCM) has increasingly been recognized by the scientific community for its multi-component and multi-target effects. These multi-target effects provide a conspicuous advantage to the anti-MIRI of TCM to overcome the shortcomings of single-component drugs, thereby pointing toward a novel avenue for the treatment of MIRI. However, very few reviews have summarized the currently available anti-MIRI of TCM. Therefore, a systematic data mining of TCM for protecting against MIRI will certainly accelerate the processes of drug discovery and help to identify safe candidates with synergistic formulations. The present review aims to describe TCM-based research in MIRI treatment through electronic retrieval of articles, patents, and ethnopharmacology documents. This review reported the progress of research on the active ingredients, efficacy, and underlying mechanism of anti-MIRI in TCM and TCM formulas, provided scientific support to the clinical use of TCM in the treatment of MIRI, and revealed the corresponding clinical significance and development prospects of TCM in treating MIRI.
1 Introduction
Cardiovascular disease (CVD) is one of the leading causes of death worldwide. As the most common CVD, ischemic heart disease (IHD) has the highest morbidity and mortality rate (Solola et al., 2022). Therefore, effective treatment for IHD is essential to reduce CVD-caused death, while the current existing therapies, such as coronary artery bypass graft, thrombolysis, and percutaneous coronary intervention (PCI), successfully reduce IHD mortality to some extent (Bittl et al., 2022). Post-therapy myocardial ischemia–reperfusion injury (MIRI) potentially causes severe myocardial damage and dysfunction, leading to post-IHD disability (Zhao H. et al., 2021). As a result, MIRI has been considered a major treatment burden for patients with IHD, and reducing myocardial ischemia–reperfusion injury becomes a clinical challenge.
The mechanism of MIRI is linked to multiple factors, and so far, research has mainly focused on oxidative stress (Xiang et al., 2021), inflammation (Yao et al., 2022), calcium overload (Wang F. Y. et al., 2020), energy metabolism disorders (Tian et al., 2019), pyroptosis (Shi H. et al., 2021), and ferroptosis (Fang et al., 2019). Targeting the factors mentioned, a range of drugs act by scavenging free radicals, ischemic preconditioning; inhibiting the exchange between Na+/H+ and Na+/Ca2+; and using adenosine receptor agonists, magnesium, statins, and angiotensin receptor antagonists to attenuate MIRI. However, acting on a single aspect is considered the main cause of unsatisfactory therapeutic outcomes of the aforementioned medicines. In contrast, traditional Chinese medicine (TCM) potentially offers a novel avenue for MIRI treatments based on their advantages of being multi-component and multi-target.
With a long history of medical use, mainly by the Chinese population, TCM has its own unique scientific theory and practical application. Various forms of herbal medicine provide enormous resources for developing a new drug against MIRI. TCM elicits potent cardiovascular protective properties, such as anti-inflammation, antioxidant effect, and immune regulation. In recent years, several studies have reported the prevention and treatment of CVD using TCM, which has achieved considerable success in research and clinical use (Leung and Xu, 2020; Zhang et al., 2020). The well-known concept of “Huo Xue Hua Yu” in Chinese (defined as “activating blood circulation and removing blood stasis” in English) of TCM formulas has been successfully applied to treat diseases by antiplatelet or anti-thrombotic activities (Zhao et al., 2020). Representative herbs include Salvia miltiorrhiza Bge. (or “Danshen”) (Li C. L. et al., 2020) and Panax notoginseng (or “Sanqi”) (Wang D. et al., 2021), which have been widely used in the treatment of CVD for a long time, not only in China but also in other Asian countries and regions. As the pharmacological properties of TCM have been increasingly studied and better understood in medical research using cutting-edge technology, greater interest has been drawn to the mechanism by which TCM contributes to MIRI treatment and the development of novel TCM-based drugs. This review aims to summarize the application of TCM to the treatment of MIRI. Four different research areas will be addressed: 1) pathological mechanisms of myocardial ischemia under the background of modern medicine, 2) mechanisms of MIRI in Chinese medicine theories, 3) single Chinese herbs and their active ingredients, and 4) TCM formulas for MIRI treatment. This review provides scientific support for the utilization and future development of TCM resources and provides a broad prospect for TCM in the treatment of myocardial ischemia through the comprehensive analysis of various kinds of TCM against MIRI.
2 Research progress on the mechanisms of MIRI
2.1 Pathological mechanism of MIRI in modern medicine
Blood reperfusion is an essential process during the treatment of myocardial ischemia, but it may also further aggravate damage or destroy cardiac function, inducing severe arrhythmias or even leading to sudden death (Wang J. et al., 2020). The severity of the reperfusion injury is dependent on many factors, such as the length of ischemia time, the temperature and pressure of the perfusion fluid, and the state of ischemic tissues and organs. From the perspective of cardiac function, the resting tension gradually increases with the prolongation of ischemia time, and the developed tension gradually decreases, while the resting tension increases and the developmental tension becomes lower during reperfusion, indicating that the systolic force of the heart decreases diastolic and systolic dysfunction of the heart (He et al., 2022).
Reperfusion therapy improves myocardial blood supply, but it is accompanied by a complex series of pathophysiological responses, such as oxidative stress, inflammatory response, calcium overload, and mitochondrial dysfunction, of which the key driving factor that causes reperfusion injury is oxidative stress (Zheng et al., 2021).
2.1.1 Oxidative stress
Oxidative stress is a consequence of imbalanced redox systems in the body, which lead to the accumulation of oxygen radicals and a decrease in the activity of antioxidant enzymes, triggering lipid peroxidation and causing cell damage. During myocardial ischemia, there is an insufficient supply of oxygen and ATP cannot be supplied adequately, impairing the ability of the myocardium to clear free radicals. When blood supply is restored by reperfusion, the large accumulation of oxygen radicals leads to lipid peroxidation, producing malondialdehyde (MDA). MDA triggers cytotoxicity and affects the mitochondrial respiratory chain complex and key enzyme activities within the mitochondria (Xiang et al., 2021). In addition, oxygen radicals can destabilize body proteins through oxidation, thereby altering the surface structure of proteases. Oxygen radicals can also induce apoptosis, which is caused by the breakage of DNA/RNA in cells, making nucleic acids non-functional (Granger and Kvietys, 2015).
2.1.2 Calcium overload
Calcium ions (Ca2+) are intracellular secondary messengers involved in maintaining cellular physiological functions. Ca2+ overload is both a result of myocardial damage and a cause of further damage. Upon myocardial ischemia, the myocardial membrane structure is damaged, and the membrane becomes more permeable to Ca2+. Increasing entry of Ca2+ from the extracellular space, following a concentration gradient, leads to myocardial Ca2+ overload. Cells switch from aerobic to anaerobic respiration during myocardial ischemia, which increases lactate production and decreases intracellular pH. Activation of the Na+–H+ exchanger leads to a marked increase in intracellular Na+ concentration. Reperfusion rapidly restores extracellular pH, resulting in a significant difference in intra- and extracellular pH, causing a large inward flow of Na+ through the Na+–H+ exchanger and an excess of intracellular Na+, triggering a reversal of the 2Na+–Ca2+ exchanger, and resulting in intracellular Ca2+ overload (Wang L. et al., 2020; Wang C. et al., 2022).
2.1.3 Mitochondrial dysfunction
Mitochondria act as the body’s energy engine, and most of the body’s energy is produced by mitochondrial oxidative phosphorylation. Mitochondria have been shown to play an important role in the progression of MIRI. Ischemia leads to the interruption of oxidative phosphorylation, which causes a rapid decrease of adenosine triphosphate (ATP) and creatine phosphate (CP) in the myocardium, resulting in an excess of non-phosphorylated purines in cardiomyocytes. The non-phosphorylated purines enter blood vessels and subsequently block the generation of ATP (Bai et al., 2021). After reperfusion, nucleosides are significantly reduced by the reduction of the raw material for the synthesis of high-energy phosphoric acid compounds in the myocardium. This process results in impaired mitochondrial energy metabolism, which affects the recovery of cardiac function. Acidic conditions during ischemia prevent the mitochondrial permeability transition pore (mPTP) from opening (Marzilli et al., 2020). During reperfusion, the electron transport chain is reactivated to produce reactive oxygen species (ROS) (Martins-Marques et al., 2021). ROS act as a neutrophil chemoattractant by inducing mPTP opening and sarcoplasmic reticulum (SR) dysfunction, thereby mediating myocardial reperfusion injury (Andrienko et al., 2017). After reperfusion, the restored physiological pH would relieve the inhibition of mPTP opening and cardiomyocyte contracture (Lin K. et al., 2015). The recovery of mitochondrial membrane potential promotes calcium entry into the mitochondria and induces mPTP opening. Several hours after the onset of myocardial reperfusion, neutrophils accumulate in the infarcted myocardium in response to the release of chemical attractant ROS, cytokines, and activated complements.
2.1.4 Inflammatory response
The inflammatory response is also an important part of MIRI. The membrane structures of myocardial endothelial tissue are damaged during MIRI and attract large numbers of neutrophils into the tissue or adhere to the myocardial vascular endothelium. The accumulation of neutrophils can lead to the release of IL-6, TNF-α, and IL-1β, causing an inflammatory response and inducing microcirculatory disturbances, which can lead to myocardial injury (Sun X. et al., 2021).
2.1.5 Cell death
Cell death is also a consequence of ischemia–reperfusion injury during MIRI. Cell death pathways include autophagy, ferroptosis, pyroptosis, and apoptosis, which are regulated by a variety of signaling pathways that affect the state of the cell (Figure 1).
2.1.5.1 Autophagy in MIRI
Autophagy is a process of phagocytosis and degradation that maintains cell homeostasis. It is a key regulator of ischemia/reperfusion (I/R) injury and is believed to play important roles in the heart during I/R, which can be activated by several stimuli including nutrient starvation, glucose deprivation, caloric restriction, oxidative stress, and brief episodes of ischemia and reperfusion to eliminate damaged mitochondria. Autophagy prevents damaged mitochondria from releasing harmful ROS, decreases apoptosis, and helps maintain mitochondrial homeostasis and cell life activities through the related pathways, mediated by the mammalian rapamycin target protein (mTOR) (Shi et al., 2019). Under the condition of myocardial ischemia, the AMPK–mTOR pathway is activated and initiates autophagy, which would swallow damaged mitochondria and reduce cardiomyocyte damage due to mitochondrial dysfunction and ATP depletion (Gatica et al., 2015). As myocardial energy is depleted, the AMP/ATP ratio increases, which causes GSK-3 activation and downregulation of the expression of the tuberous sclerosis complex 1/2 TSC1/2, thereby inhibiting mTOR signaling (Zhai and Sadoshima, 2011). However, studies have also reported that prolonged ischemia could trigger excessive autophagy and lead to cardiomyocyte death, which further deteriorates cardiac function (Rabinovich-Nikitin et al., 2021).
During myocardial reperfusion, a burst of oxygen-derived free radicals could lead to excessive autophagy that contributes to autophagic cell death and aggravates myocardial injury (Yang H. et al., 2021). Recombinant lysosomal-associated membrane protein 2 (LAMP2) is a critical factor in autophagosome–lysosome fusion, whose decline can lead to an increase in autophagosomes. Thus, upregulating the expression of LAMP2 during reperfusion suppresses autophagy and attenuating myocardial infarction (Ghosh et al., 2022). Furthermore, the effect of autophagy on the protection or aggravation of injury by reperfusion may be related to the expression of Beclin 1, and mTOR also mediates interaction with Beclin 1 through caspase-3, which plays a key role in autophagy (Dong et al., 2019). The Ca2+ overload during reperfusion promotes CaMKII, which phosphorylates Beclin 1 at Ser90, subsequently promoting the ubiquitination of Beclin 1 at the K63 site to cause excessive autophagy and cell death (Chen et al., 2021).
2.1.5.2 Pyroptosis in MIRI
Pyroptosis, a pro-inflammatory cell death process, is mediated by gasdermin D (GSDMD) or gasdermin E (GSDME). ROS could activate the NOD-like receptor protein 3 (NLRP3) inflammasome and then induce the activation of caspase enzymes, which is an important process of MIRI-mediated pyroptosis (Bortolotti et al., 2018). On the one hand, GSDMD is cleaved by activated caspase-1 to form a polypeptide containing the nitrogen-terminated active structural domain of GSDMD (Wang et al., 2020d). On the other hand, apoptosis-associated speck-like protein containing a caspase recruitment domain (ASC) induces pro-caspase-1 inflammasome formation and results in caspase-1 activation. Activated caspase-1 cleaves the precursors of IL-1β and IL-18 to their active forms (Wang et al., 2020e). These processes promote the accumulation of inflammatory cells, which results in pyroptosis.
2.1.5.3 Ferroptosis in MIRI
Ferroptosis is an iron-dependent, non-apoptotic form of cell death that is characterized by iron overload and lipid peroxidation. Ferroptosis is critical for the pathogenesis of MIRI. In the transplant model, ferrostatin-1 (FER-1) has been found to protect the heart from MIRI by reducing the levels of hydrogen peroxide-arachidonic acid-phosphatidylethanolamine, which is an intermediate product that mediates iron death; in this way, FER-1 reduces the death of cardiomyocytes and myocardial fibroblasts. At the same time, iron death specifically promotes the adhesion recruitment of neutrophils to coronary endothelial cells through the TLR4/TRIF/IFN-1 signaling pathway, which aggravates heart damage (Li W. et al., 2019). Meanwhile, in rat models of diabetic MIRI, the DNA methyltransferase-1 (DNMT-1) inhibitor 5-aza-2′-deoxycytidine (5-aza-CdR) has been reported to reduce nuclear receptor coactivator 4 (NCOA4)-mediated ferritin phagocytosis and myocardial damage. Inhibiting DNMT-1 reduces iron death during DM, and NCOA4-mediated phagocytosis of ferritin is potentially involved in this process (Li Q. et al., 2020). Another study showed that the expression of ubiquitin-specific protease 7 (USP7) in rat myocardial tissue with MIRI was significantly upregulated. The expressions of P53 (the human tumor suppressor gene) and transferrin receptor 1 (TfR1) also increased along with the exacerbation of iron death in myocardial tissue (Tang et al., 2021). Further study based on an artificial model of glutathione peroxidase 4 (GPX4) deletion in the proximal tubules indicated that knockout of GPX4 caused cell death in a pathologically relevant form of ferroptosis and spontaneous tubular necrosis, and the inhibition of ferroptosis by liproxstatin-1 was able to mitigate I/R-induced tissue damage (Park et al., 2019).
2.2 Mechanism of MIRI under the background of Chinese medicine theories
“Myocardial ischemia-reperfusion injury” is not defined in the theory of Chinese medicine, but based on its lesion location (heart collateral and heart pulse) and clinical manifestations (chest tightness, chest pain, shortness of breath, and fatigue), MIRI is classified into “chest paralysis,” “heartache,” “palpitation,” and other categories (Xie et al., 2015). Chinese medicine practitioners believe that the core of MIRI pathogenesis is heart vessel blockage caused by various etiologies. Although MIRI originates from heart disease, it also affects the lungs, liver, kidney, spleen, and other organs. The nature of MIRI in Chinese medicine mainly lies in two aspects of root vacuity and tip repletion, which interact with each other and jointly affect the pathological process of MIRI. Among them, root vacuity refers to a deficiency of qi and insufficient blood circulation and Yin and Yang, while tip repletion refers to phlegm turbidity, cold coagulation, qi stagnation, blood stasis, and other phenomena (Li et al., 2021). The TCM pathogenesis of MIRI includes qi deficiency, blood stasis, and phlegm turbidity. Qi deficiency leads to the formation of blood stasis and turbid phlegm, which further aggravates the degree of qi deficiency, forming a vicious circle. The pathogenesis of the disease and the severity of the disease are the trends of MIRI. Based on relative documents and clinical symptoms of patients with MIRI, Chinese medicine has divided MIRI into two main types: turbid phlegm obstruction syndrome and qi and blood stasis obstruction. From a patient’s constitution of different types of symptoms, a multifaceted diagnosis is made to find the optimal treatment program, which has the features and advantages of Chinese medicine treatment of MIRI.
2.2.1 Turbid phlegm obstruction syndrome
Some scholars believe that the etiology and pathogenesis of acute myocardial ischemia are caused by the organ’s dysfunction and qi–blood–body fluid generation, resulting in the turbidity of phlegm. Phlegm turbidity directly attached to the vein wall, resulting in abnormal qi–blood circulation, blood stasis, phlegm–blood stasis, and heart vessel blockage stasis, hinders the operation of meridians and collaterals and thus causes microcirculation disorders (Ma et al., 2013). After subsequent reperfusion, distal blood flow is greatly reduced, causing reperfusion injury. Microthrombus and small atherosclerotic plaques would block distal vessels with blood flow after reperfusion, causing insufficient blood flow in distal vessels and aggravating injuries (Bai and Ren, 2014; Liu et al., 2014). Body fluid is homologous to blood in the body. Phlegm and stasis are the pathological products of blood fluid. Qi–blood stasis and stagnant movement of qi and blood will affect water metabolism. In the initial stage of reperfusion, rapid blood flow is likely to induce water retention, forming “edema” and “phlegm drinking” in cells, which leads to slow blood flow in vessels (Zhu et al., 2015).
2.2.2 Qi and blood stasis syndrome
The blood and oxygen supply are cut off during myocardial ischemia, which leads to myocardial dystrophy and causes varying degrees of insufficiency of the heart qi. The relationship between qi and blood is one of mutual sustenance, interdependence, and mutual use, and blood has the role of moistening and carrying qi.
If there is insufficiency of the heart qi for a long time, blood vessels may show restenosis symptoms due to poor blood flow and venous stasis. Suddenly, large amounts of blood await delivery to distal tissues during reperfusion, instead increasing the burden on the heart, depleting the qi of the heart and leading to reperfusion injury (Wang and Yan, 2016). Qi deficiency inevitably leads to weakened qi transformation function, an inability to complete the mutual transformation of qi, blood, and fluid, and an inability to transform blood into nutrients for tissue use, resulting in a lack of nutrients for cardiac muscle cells and increased cardiac function damage, causing myocardial apoptosis, and thus symptoms of cardiac stasis.
The myocardial tissue will lead to myocardial qi deficiency due to ischemia and hypoxia; however, qi deficiency of the heart results in slow blood flow. Therefore, after reperfusion, the heart is unable to deliver blood quickly to the distal tissues for use, resulting in blood stasis in the distal vessels. In this case, the myocardium becomes deficient in qi and blood, which is called “pulse knotting and palpitations” in Chinese medicine, just like reperfusion arrhythmia in Western medicine (Li and Zhu, 2012).
3 Herb medicines and their active chemical ingredients in TCM formulas for MIRI treatment
TCM plays an important and indispensable role in preventing and treating MIRI. Some Chinese patent drugs have shown significant myocardial protection in MIRI. The chemical components of TCM are structurally diverse and have multiple pharmacological effects. Single Chinese herbs and their chemical constituents, TCM formulas, and TCM-based prescriptions may have therapeutic efficacy against MIRI (Tables 1,2). Classifying and analyzing the active ingredients of TCM reported in previous literature can provide a reference for determining the research trends in this field in the future (Figures 2,3).
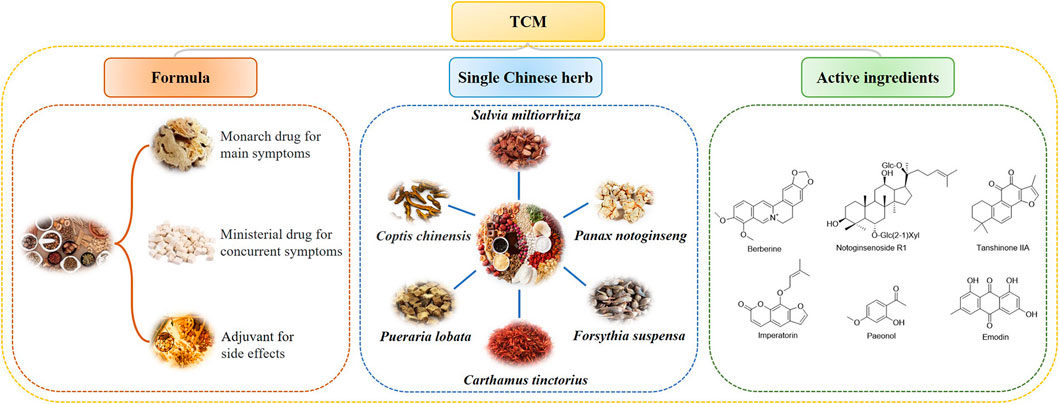
FIGURE 2. TCM formulas, single Chinese herbs, and active compounds that protect the heart from MIRI.
3.1 Single Chinese traditional herbs for treating MIRI
3.1.1 Danshen
Salvia miltiorrhiza, also known as “Danshen” in China, was primarily recorded in “Shen Nong Ben Cao Jing.” The Chinese traditional herb “Danshen” refers to the dry roots and rhizomes of Salvia miltiorrhiza Bge., which is famous for its effects in promoting blood circulation and removing blood stasis; it has been extensively applied for many years to treat various diseases, especially coronary heart diseases and cerebrovascular diseases, either alone or in combination with other Chinese plant-based medicines (Su et al., 2015). Danshen plays a significant role in protection against cardiovascular diseases through the antioxidant effect (Zhou et al., 2020), anti-inflammatory effect (Wang et al., 2020f), and anti-apoptosis effect (Deng et al., 2021), making investigations on how the effective components of Danshen could be applied to MIRI therapies a hot topic.
To date, more than 100 types of components in Danshen have been identified, among which tanshinone IIA (TA) is present in relatively higher concentrations and is one of the most effective components being applied to CVD treatment. Many studies have provided evidence for the remarkable effect of TA on MIRI. For example, TA has been reported to improve cardiac function by inhibiting collagen I, collagen III, tissue inhibitors of metalloproteinases (TIMP-1), and galectin-3 mRNA expression and enhancing matrix metallopeptidase (MMP)-2 mRNA and protein expression to inhibit myocardial fibrosis and improve myocardial remodeling in rats with I/R-induced heart failure (Zhang et al., 2022). Some studies have also demonstrated that the cardioprotective effect of TA is linked to the JAK2/STAT3 signaling pathway to suppress cell apoptosis (Wang H. et al., 2021). Sodium tanshinone IIA sulfonate (STS), one of the derivatives of TA, has been further developed as an injected medication for treating coronary heart disease, angina, and myocardial infarction in China. STS injection not only improves myocardial function but also regulates the immune response by inhibiting cardiomyocyte apoptosis (Li Y. et al., 2019). STS pretreatment applied to the I/R rat model showed that the downregulated expressions of IL-1β and HMGB1, which mediated the expression of the IL-23/IL-17 axis, reduced neutrophil infiltration, reduced myocardial infarct size, and improved MIRI (Ding et al., 2020a; Ding et al., 2020b). Cryptotanshinone (CTS) inhibited I/R-induced cardiomyocyte apoptosis in vivo and in vitro via the MAPK pathway (Wang R. et al., 2021). Moreover, CTS is likely to alleviate MIRI by inhibiting the expressions of caspase-12, CHOP, and GRP78 in the myocardial tissue of the I/R rats via the activation of the JAK1/STAT3 signaling pathway (Wang R. et al., 2022). Dihydrotanshinone I (DT) and protocatechuic aldehyde (PCA) are also two active ingredients of Danshen against cardiovascular ischemic injury. DT has been found to induce transient ROS generation via the reversible inhibition of mitochondrial respiratory complex I and thereby stabilize HIF-1α, which upregulates Nrf2 and activates antioxidant enzymes. PCA can protect cardiomyocytes from reperfusion injury by elevating the levels of reduced glutathione (GSH), which is achieved by providing reducing equivalents to scavenge ROS (Jiang et al., 2019). Furthermore, PCA potently protects cardiomyocytes by suppressing the PERK, IRE1α, and ATF6α signaling pathways, which are involved in regulating endoplasmic reticulum (ER) stress (Wan et al., 2021).
Other components of Danshen have also been found to effectively treat MIRI. Salvianolate is a class of hydrophilic components, among which magnesium lithospermate B is a prominent one and has been shown to improve cardiac function. Salvianolate was reported to significantly inhibit cardiomyocyte apoptosis by regulating apoptosis-related proteins such as Bcl-2, Bax and active caspase-3, indicating the potential therapeutic ability of salvianolate against MIRI (Li M. et al., 2019). Magnesium lithospermate B exhibits the function of resisting myocardial cell programmed necrosis (Wang et al., 2017). Another ingredient called Danshensu (DSS) has been shown to inhibit miR-199a-5p expression. Downregulation of miR-199a-5p mRNA was followed by a rise of phosphorylated Akt (p-Akt) and phosphorylated ERK (p-ERK), implying that miR-199a-5p mRNA plays a role through the PI3K/Akt and ERK1/2 signaling pathways (Shi Y. et al., 2021). A total of 10 μmol/L DSS was able to protect the heart from I/R injury, which was potentially attributed to inhibiting excessive ROS through the Sirt1/FoxO1/Rab7 signaling pathway (Sun et al., 2020). Salvianolic acid A has been shown to protect against MIRI in rats by playing an antiplatelet role and lowering serum levels of TNF-α, IL-1β, and nitric oxide (NO) (Yuan et al., 2017). Sal B attenuates myocardial I/R injury by activating the PI3K/Akt pathway, which was reported to decrease inflammatory responses and inhibit apoptosis in rats (Liu C. et al., 2020).
In addition, the polysaccharides of Danshen have a variety of biological activities such as anti-inflammatory, antioxidant, and immunomodulatory actions (Song et al., 2013). However, reports on the mechanism of action of polysaccharides against MIRI remain rare.
3.1.2 Sanqi
It has been more than 600 years since Panax notoginseng, or Sanqi, was first discovered and recorded in ancient China. Sanqi was conventionally used for stopping bleeding, promoting blood circulation, and pain relief, as recorded in “Ben Cao Gang Mu.” Modern studies have suggested that Sanqi is widely used in the prevention and treatment of cardiovascular and cerebrovascular diseases, owing to its significant effects on cerebrovascular dilation, vessel resistance reduction, and vessel endothelial function improvement.
Panax notoginseng saponins (PNS) are one of the major active components in Sanqi. It has been reported that PNS can effectively improve MIRI by reducing oxidative stress, reducing calcium overload, suppressing neutrophil activation and adherence, improving metabolism, increasing anti-apoptotic activity, and modifying endothelial functions of blood vessels (Wang W. et al., 2021). MIRI impacts the membrane stability of cardiomyocytes, which increases membrane permeability, thus resulting in the leakage of myocardial enzymes into the serum. A study revealed that the activities of both LDH and CK significantly decreased after PNS treatment in MIRI models, suggesting that the cardioprotective role of PNS is linked to stabilizing cardiomyocyte membranes, thus restoring the impaired membrane permeability due to MIRI (Shi et al., 2018). Liu et al. found that PNS pretreatment on I/R rats for 7 days enhanced mitochondrial autophagosomes in myocardial cells, which was linked to an increased ratio of LC3II/LC3I and upregulated expressions of HIF-1a, BNIP3, Atg5, and Beclin 1 in the rat myocardial tissue (Liu et al., 2019). In addition, PNS can protect cardiomyocytes against hypoxia/reoxygenation (H/R) injury by inhibiting apoptosis via FOXO3a/Akt signaling (Liu H. et al., 2021). These cases have shown that PNS could promote autophagy to protect cardiomyocytes from I/R injury, and mitochondrial autophagy has been considered a potential target of PNS in MIRI treatment. Other studies have found that PNS can protect cardiomyocytes by increasing the expression of Mir-30C-5p, which subsequently downregulates p53 expression and inhibits cell apoptosis (Wang et al., 2020g). PNS consists of a variety of monomer components, among which notoginsenoside R1 (NGR1) has been proven to have cardioprotective effects in terms of regulating oxidative stress, endoplasmic reticulum stress, apoptosis, and other relevant signaling pathways (Yu et al., 2016). One study (Zhou and Liu, 2019) demonstrated that NGR1 preconditioning inhibited MIRI by activating the PI3K/Akt signaling pathway, reducing oxidative stress and inflammation, and inhibiting cardiomyocyte apoptosis. NGR1 (20 μM) was likely to alleviate H/R-induced H9c2 cell damage via the upregulation of miR-132 and downregulation of its target protein HBEGF (Jin P. et al., 2021).
As a major ingredient of Radix ginseng, ginsenoside Rg1 (Rg1) has been increasingly recognized to benefit the heart. Rg1 (5 mg/kg/h) could prevent myocardial injury caused by I/R, and the mechanism may be related to inhibiting myocardial apoptosis and regulating energy metabolism via the RhoA signaling pathway (Li et al., 2018). The compatibility of Sanqi compounds with other herbs is also a hot topic for MIRI treatment. Li et al. found that the anti-inflammatory efficacy of PNS combined with safflower total flavonoids (STFs) was stronger than a formulation containing PNS or safflower extract alone (Li W. et al., 2019). More specifically, PNS and STF together inhibit the activation, aggregation, and adherence of neutrophils by suppressing NF-кB activities or suppressing the combination of platelet-activating factor (PAF) and its receptor in an additive manner (Dong et al., 2014). Wang et al. investigated the therapeutic effect of the compatibility of Rhizoma coptidis total alkaloids and PNS on diabetes-induced coronary heart disease using a diabetic MIRI rat model. The combination of components downregulated the expressions of NOX2 and NOX4, which participate in diabetic macroangiopathy and induce ROS synthesis; therefore, the combination treatment inhibited the activation of the NLRP3 inflammasome and other apoptosis-related factors (Wang X. et al., 2021). As most CVD patients are known to develop complications such as diabetes, it is meaningful to explore novel therapies for MIRI patients with different complications.
3.1.3 Huangqi
Astragalus membranaceus is a flowering medicinal plant. “Huangqi,” in China, is specifically referred to as the dried root of Astragalus membranaceus (Fisch.) Bge. Modern studies have confirmed that astragalus has a series of effects attributed to its rich chemical composition, including neuroprotection, anti-fatigue, renal protection, hepatoprotection, anti-cancer, and cardiac protection (Durazzo et al., 2021). Astragaloside-IV (AS-IV), a marker component for the quality evaluation of Huangqi, has been reported to exhibit cardioprotective properties against MIRI through different pathways, whose underlying mechanisms will be discussed as follows. Studies have shown that AS-IV can significantly alleviate the pathological state of MIRI by regulating autophagy (Shao et al., 2020). In a study by Yin et al. (2019), AS-IV was shown to attenuate MIRI in rats via the inhibition of calcium-sensing receptor-mediated apoptotic signaling pathways. Other studies have found that AS-IV could upregulate miR-101a expression, suppress TGFBR1 and TLR2 expressions, and inhibit the MAPK signaling pathway in H/R-injured cardiomyocytes (Wu et al., 2017). Yang et al. (2019) found that AS-IV could regulate the expressions of Nrf2 and Bach1 proteins in the nucleus. AS-IV also promoted the expression of HO-1 (an antioxidant enzyme) to inhibit oxidative stress and inflammation via the PI3K-mediated pathway. Li et al. (2017a) reported that AS-IV might inhibit ER stress and prevent the abnormal opening of mPTP through the regulation of GRP78, GRP94, and IRE1. These studies provided evidence for the ability of AS/IV to ameliorate MIRI via multiple pathways. Astragalus polysaccharide not only has a recognized therapeutic effect on diabetic complications but also has a notable anti-myocardial ischemia–reperfusion injury. Astragalus polysaccharides (ASPs) are another main active ingredient of Astragalus membranaceus. ASP could reduce intracellular ROS and inhibit apoptosis, and these effects were achieved by the downregulation of the expressions of caspase-3 and Bax and the upregulation of the expression of Bcl-2 both in vivo and in vitro (Liu D. et al., 2018). Another study has shown that ASP improves myocardial microvascular endothelial cells in H/R by mediating the PI3K/Akt/eNOS signaling pathway (Zhou et al., 2018). Zhao’s team previously showed that the combined action of AS-IV and Rg1 could not only effectively lower blood lipids but also protect against MIRI. Further studies showed that AS-IV and ginsenoside Rg1 could significantly increase the expressions of eNOS and Bcl-2, while decreasing the expressions of Bax and cytochrome C, suggesting that the formulation protects against MIRI mainly through regulating cell apoptosis, potentially via the upregulation of the eNOS protein (Zhao T. et al., 2021), yet the study did not analyze the optimal dose ratios of AS-IV and Rg1, which needs further study.
3.1.4 Huangqin
The term “Huangqin” refers to the dried root of Scutellaria baicalensis Georgi, which has been involved in medication in China for decades due to its excellent pharmacological properties. Flavonoids are the most abundant components in Scutellaria, mainly including baicalin (BA) and hanbaicalin and their aglycones, which are baicalein and wogonin (WG), respectively (Billah et al., 2019). Studies have confirmed that most flavonoids have a certain protective effect on the myocardium, and the bioactive components of Huangqin elicit a cardiac protective effect through some channels (Sun W. et al., 2021). Li and Yu’s (2021) study has shown that WG could increase Nrf2 expression and regulate the redox system balance in MIRI rats (Li et al., 2021). Additionally, the expression of HO-1 also increased. As an HO-1 inhibitor was found to partly reverse the protective effect, it suggested that WG activated Nrf2, induced the expression of the downstream HO-1 gene, and exhibited antioxidant activity (Song et al., 2019). BA, another component of Huangqin, has shown reverse H/R-induced oxidative stress and apoptosis, which was mainly attributed to the enhancement of the activity and expression of aldehyde dehydrogenase (ALDH) in cardiomyocytes (Jiang et al., 2018). Another study has also reported that BA could promote cell survival under oxidative stress by acting on MARCH5 (a ubiquitin ligase of the mitochondrial outer membrane) in cardiomyocytes (Li W. et al., 2020).
The cardioprotective effect of baicalein (an aglycone of BA) is associated with inflammation-related pathways such as signaling through MAPK, Akt, NF-κB, and JAK-STAT (Ma et al., 2018). However, more research will have to be carried out to specify the underlying mechanism of action.
3.1.5 Dahuang
Rhubarb is a kind of heat-clearing and fire-purging drug from Rheum palmatum L., Rheum tanguticum Maxim. ex Balf., or Rheum offcinale Baill. and has also been shown to have a protective effect on the heart. Emodin (Emo) is an anthraquinone compound extracted from Rheum, the root rhizome of which was primarily used to treat constipation and other gastrointestinal problems. Pharmacological properties of Emo have been demonstrated as anti-inflammation, platelet aggregation inhibition, immune regulation, and anti-tumor (Li et al., 2017b; Gao et al., 2020; Wei et al., 2021). It has been reported that Emo protects against myocardial damage by easing oxidative stress. Other studies have uncovered the effect of Emo as an antioxidant in cardiomyocytes via the Nrf2/ARE/HO-1 signaling pathway, which has been proven to show important protective activity against MIRI (Cui et al., 2020).
Other studies have found that Emo can alleviate myocardial I/R injury by inhibiting pyroptosis due to its powerful anti-inflammatory effect, and the TLR4/MyD88/NF-κB/NLRP3 inflammasome pathway is involved in the process (Ye et al., 2019). Rhein, another anthraquinone compound, upregulated p-Akt and activated the Akt/GSK3β/p38 pathway, thereby protecting H9c2 cells from H/R-induced injury (Liu J. et al., 2018).
3.1.6 Shaoyao
Paeonol (Pae) is a bioactive compound derived from the root bark of the Moutan Cortex. Studies have demonstrated the outstanding antioxidant effect of Pae in multiple pathogenetic processes, implying that Pae is a potential option for treating oxidative stress-induced diseases. A study by Choy et al. (2017) reported that Pae increased the utilization of NO in mice and inhibited the production of ROS in the aorta, thereby improving endothelial function and reducing ER stress-mediated oxidative stress. Another recent study also revealed that Pae alleviated MIRI via the upregulation of sirtuin-1 (SIRT1) (Liu H. et al., 2020). SIRT1 is a protein that shows inhibitory effects on oxidative stress by regulating Nrf2 and the forkhead box O (FoxO) transcription factors. In addition to its antioxidant properties, SIRT1 also contributes to CVD treatment by reducing the inflammatory response (Hori et al., 2013; Wan et al., 2016; Shah et al., 2017). In the model of MIRI, the expression level of SIRT1 markedly decreased, while adding Pae recovered the expression of SIRT1. Knockdown of SIRT1 decreased the cardioprotective effects of Pae, so it is plausible that Pae at least partly mediates the activity of SIRT1 and potentially affects downstream proteins such as Nfr2 and FoxOs.
An intravenous infusion of Pae 15 min before a ligation operation on rats was found to improve the cardiac function in I/R-injured myocardium and reduce cardiac I/R-induced arrhythmias and mortality (Tsai et al., 2021). Pae inhibits apoptosis and autophagy cell death and alleviates I/R injury by controlling the expressions of apoptotic proteins caspase-8, caspase-9, caspase-3, PARP, Beclin-1, P62, LC3-I, and LC3-II. More investigation of the effects of Pae on the downstream pathway is necessary.
3.1.7 Lianqiao
Hyperoside (Hyp) is a kind of flavonoid compound extracted from many plants, such as Hypericum perforatum L. Flavonoid compounds have been proven to contribute to the treatment of CVD (Russo et al., 2019; Parmenter et al., 2020; Gao et al., 2021). Some researchers found that Hyp preconditioning reduced the myocardial infraction area and the apoptotic rate in rats, the mechanism of which was likely to be anti-lipid peroxidation, anti-free radical synthesis, and anti-apoptosis (Xu et al., 2022). Recently, a study revealed the effect of Hyp on H/R-induced cell injury by suppressing Bcl-2-interacting protein 3 (Bnip3) expression (Xiao et al., 2017). Numerous studies have shown that there are large numbers of cytokines associated with the process of H/R, among which Bnip3, as a mitochondrial membrane protein, is a key factor leading to mitochondrial dysfunction and cell damage. Hypericin pretreatment of cardiomyocytes showed that it could inhibit the increase of Bnip3, Bax, and cleaved caspase-3 activity caused by H/R, increase the expression of Bcl-2, and then inhibit cell apoptosis to improve cardiomyocyte injury.
Another study pointed out that Hyp regulated cell proliferation, differentiation, and apoptosis to resist MIRI through PKC/mitoKATP protein kinase C (PKC) (Wang, 2020). Subtypes of PKC include PKCα and PKCε. PKCα has been claimed to be important in the pathological changes during MIRI by reducing Ca2+ overload and inhibiting apoptosis (Trerotola et al., 2017; Xu et al., 2019). Wang suggested that the beneficial effect of Hyp on MIRI is associated with the PKC/mitoKATP signaling pathway. Hyp directly upregulates the activities of both PKCα and PKCε, activating downstream mitoKATPC. In this way, Hyp suppresses the accumulation of excessive Ca2+ in the cytoplasm, maintains mitochondrial functions, reduces cell apoptosis, and exhibits anti-MIRI actions.
3.1.8 Dengzhanxixin
Breviscapine (Bre) is a flavonoid compound extracted from the plant Erigeron breviscapus (vant.) Hand-Mazz. A pharmacological study has shown that Bre not only has beneficial effects on cerebrovascular vessels but also inhibits platelet aggregation and oxygen radicals (Gao et al., 2017; Wen et al., 2021). The study of Wang Y. et al. (2021) emphasized the antioxidant effect of Bre, showing that it promotes the expression of a transcription factor, the cAMP-response element binding protein (CREB), which promotes autophagy and suppresses the expression of toll-like receptor 4 to protect against oxidative damage and apoptosis, thus recusing endothelial cells from MIRI. Additionally, the effect of Bre in rat models of MIRI on diabetes was also studied (Yang N. et al., 2021). mTOR is a vital autophagy-associated factor that is critical for MIRI. The level of mTOR significantly dropped in the sham group, while Bre restored it to normal. Moreover, Bre also increased the levels of phosphorylation of PI3K and Akt and reduced the release of inflammatory factors. Another study reported that the activation of the PI3K/Akt/mTOR signaling pathway exhibited an inhibitory effect on excessive autophagy induced by MIRI (Li et al., 2018), which was consistent with the results of Yang Y. K. et al. (2021). Therefore, through the regulation of the PI3K/Akt/mTOR signaling pathway, Bre suppresses autophagy and the inflammatory response in the myocardium.
3.1.9 Huanglian
Berberine (Ber) is a kind of alkaloid extracted from various kinds of herbs, including Coptis chinensis (“Huanglian”). Ber exhibits anti-inflammatory, antibacterial, and anti-tumor activities (Liu and Zhou, 2019). The cardioprotective effect of Ber has also been widely studied. In a study by Sun et al., after Ber treatment in cardiomyocytes in vivo, mitochondrial swelling and left ventricle damage declined, and the levels of serum CK-MB and cTnI markedly dropped, indicating that Ber relieved mitochondrial injuries and MIRI. The study also demonstrated that the presence of Ber promoted the expressions of PINK1, P62, LC3B, and Parkin and elevated the expression of USP30 back to its normal level. In conclusion, researchers suggested that Ber promoted mitochondrial autophagy through the activation of the PINK1/Parkin/P62/LC3B autophagy pathway and normalized UP30 expression (Kulek et al., 2020; Sun J. et al., 2021).
3.1.10 Shanzha
Vitexin (VT) is a bioactive flavonoid component of dried leaves from Crataegus pinnatifida. According to modern pharmacology, generally, flavonoid compounds extracted from Crataegus pinnatifida leaves have certain benefits such as increasing coronary blood flow, preventing myocardial ischemia, and exhibiting antioxidant properties (Fu J. H. et al., 2013; Li et al., 2023). It has been reported that VT exhibits a wide range of pharmaceutical activities, including blood pressure-lowering, anti-inflammation, anti-tumor, and neuroprotective effects (Lee et al., 2012; Demir Özkay and Can, 2013; Yang et al., 2014). More importantly, VT also shows cardioprotective effects, as it has been found to protect against MIRI in vivo and H/R injury in vitro (Dong et al., 2008; Dong et al., 2011). According to Xue (2020), VT attenuates MIRI through the Epac1–Rap1 pathway. Exchange protein activated by cAMP 1 (Epac1) mainly exists in the heart, kidney, blood vessels, and central nervous system. Rap1 is a downstream GTPase of Epac1. Knockdown of Epac1 was found to prevent MIRI in the laboratory, while agonists of Epac1 worsened myocardial damage and mitochondrial dysfunction. VT preconditioning significantly inhibited the expressions of Epac1 and Rap1, reduced the myocardial infarction area, and eased left ventricle functional failure and mitochondrial failure, indicating that Epac1 signaling plays an important role in the mechanism of VT easing MIRI-induced mitochondrial dysfunction and suppressing the activation of mitochondria-induced apoptosis (Yang H. et al., 2021).
In addition to TCM, the myocardial protective effects of other Chinese herbs have also been confirmed, including antioxidant, anti-inflammatory, immunomodulatory, anti-apoptotic, and other effects to resist MIRI. Examples of these herbs include Rhodiola rosea (Sun et al., 2018; Jin Z. et al., 2021; Tian et al., 2022), flowers of Carthamus tinctorius (Min and Wei, 2017; Lu et al., 2019), Ginkgo biloba (Liu J. et al., 2020), Rhizoma acori graminei (Xiao et al., 2020), and Schisandra chinensis (Zhang et al., 2017).
3.2 Chinese herbal compound prescription for treating MIRI
3.2.1 Yiqi Huayu decoction
Yiqi Huayu decoction is a classical representation of the established Chinese traditional treatment that “activates blood circulation and removes blood stasis.” The decoction is made of five well-known medicinal herbs, namely, Danshen, Sanqi, Wuzhi Maotao (Ficus simplicissima Lour.), Xianhecao (Agrimonia pilosa Ledeb.), and Hongjingtian (Rhodiola crenulata). In addition to Danshen and Sanqi (which were already discussed earlier), the other three herbs also show outstanding cardioprotective effects against MIRI. Psoralen is the major bioactive component of Ficus simplicissima Lour. and contributes to anti-coagulation and immunoregulation. Agrimonia pilosa Ledeb. has been proved to show great anti-inflammatory effect. Rhodiola crenulata is a well-known herb with an antioxidant property (Huang and Liao, 2012; Miao et al., 2013; Feng et al., 2021; Ye et al., 2021). An in vivo study has proven that Yiqi Huayu decoction effectively suppresses the expressions of proteins inducing apoptosis, for instance, Bax, caspase-3, Fas, and P53, and, in contrast, promotes the expression of Bcl-2 (Lin X. et al., 2015). MIRI induces an increase in MDA and a decrease in superoxide dismutase (SOD), which is reversed by Yiqi Huayu decoction (Huang and Liao, 2012; Miao et al., 2013; Lin K. et al., 2015). Additionally, the formulation of the decoction is not fixed. Compared to the formulation described earlier, some people replaced Wuzhi Maotao and Hongjingtian with Tubiechong [the dried body of Eupolyphaga sinensis Walker or Steleophaga plancyi (Boleny)] and Wuzhaolong (the root or branch of Ficus hirta Vahl.), respectively. The new formulation was found to alleviate MIRI in laboratory conditions, which was consistent with clinical observations, and the underlying mechanism was considered to be linked to an increase in SOD activity and a decrease in TNF-α and MMP-9, underpinning the antioxidant and anti-inflammatory effects of Yiqi Huayu decoction (Huang and Liao, 2012).
3.2.2 Angelica sinensis decoction
Liu et al. studied Angelica sinensis decoction, the effective ingredients of which include paeoniflorin, ferulic acid, glycyrrhizic acid, and cinnamic acid. The effects of Angelica sinensis decoction on MIRI-induced oxidative stress in rats and the most optimal concentrations of the components used in an orthogonal test are as follows: the combination that worked best contained ferulic acid, 300 mg/kg; cinnamic acid, 200 mg/kg; and glycyrrhizic acid, 50 mg/kg; which significantly reduced MDA and increased SOD in the myocardium, yet paeoniflorin had a less significant effect on oxidative stress injury (Liu H. H. et al., 2021).
3.2.3 Buyang Huanwu decoction
Song et al. (2020) studied the effects of Buyang Huanwu decoction pretreatment on myocardial enzymes and antioxidant functions in MIRI rats. Compared with the non-treated MIRI model group, serum LDH and CK activities in the Buyang Huanwu decoction group decreased significantly. MDA concentration in the serum of MIRI rats also significantly decreased, while SOD activity and NO concentration increased. The mechanism concluded was: the reduction in lipid peroxidation reactions of cell membranes and the protection of myocardial cells from oxidative stress injury during reperfusion.
3.2.4 Huanglian Jiedu decoction
Liu et al. observed the effect of Huanglian Jiedu decoction on myocardial I/R-induced arrhythmia in rats and explored its mechanism of action. Compared with the I/R group, the morbidity and mortality of ventricular tachycardia (VT) and ventricular fibrillation (VF) in the Huanglian Jiedu decoction group were significantly lower; at the same time, the MDA concentration was significantly decreased, and the SOD activity and NO concentration were significantly increased (Fu X. C. et al., 2013; Liu X. W. et al., 2021).
Furthermore, Shenmai injection (Yu et al., 2019), Si-Miao-Yong-An decoction (Cui et al., 2021; Wang C. et al., 2022), Gualou Xiebai decoction (Yan et al., 2018), Huoxue Huatan decoction (Lin et al., 2020), Si Ni decoction (Li et al., 2014), Dang Gui Si Ni decoction (Qian et al., 2014), Shexiang Baoxin pill (Yu et al., 2022), Cardiotonic pill (Xu et al., 2016), and other Chinese herbal compound prescriptions have been widely used in the clinical treatment of patients with different syndromes, under the guidance of traditional Chinese medicine theory. Due to the large number of components in Chinese herbal compound prescriptions, the mechanisms of their myocardial protective effect are also relatively complex. Current studies have shown that most Chinese herbal compound prescriptions mainly exhibit anti-MIRI effects through anti-inflammatory and anti-oxidation mechanisms and affect other mechanisms in the body.
According to TCM theory, the main pathological mechanisms of MIRI are mainly qi deficiency, blood stasis, and phlegm turbidity. Therefore, herbs and compound prescriptions, with the effects of benefiting qi and nourishing Yin, activating blood stasis, dispelling phlegm, and dispersing nodules, are commonly used in clinical practice to prevent and treat MIRI; good therapeutic effects have been achieved. As research on Chinese herbal medicines and compound drugs continues to deepen, not only will the main active components that exhibit myocardial protective effects be discovered, but also the main mechanisms of myocardial protection, including scavenging of oxygen free radicals, anti-oxidation, inhibition of the inflammatory response, and inhibition of the apoptosis of cardiomyocytes. Whether single herbal medicine, a group formula, or an extracted component of a certain herbal medicine, research on multi-pathway, multi-system, and multi-target effects of Chinese herbal medicines is still insufficient. In addition, there is a gap between animal models and clinical practice, and there are few models of TCM evidence; the application of TCM lacks the guidance of the discriminatory treatment system and cannot reflect the advantages of discriminatory treatment. Therefore, it is important to combine modern medicine and technology with traditional Chinese medicine theories of diagnosis and treatment to explore pathological mechanisms of Chinese medicine in order to prevent MIRI and innovate Chinese medicine prescriptions and medications.
4 Conclusion
As reviewed above, it could be concluded that TCM has made great contributions to the treatment of MIRI. Their benefits in promoting blood circulation and removing stasis, clearing heat, and detoxification are key aspects against MIRI. Salvia miltiorrhiae, Panax notoginseng, and Scutellaria Baicalensis have been highlighted for their efficacy in alleviating myocardial injury through anti-oxidative, anti-inflammatory, anti-apoptotic, and autophagy regulatory effects via the Notch signaling pathway, the JAK2/STAT3 pathway, the PINK/Parkin pathway, and the PI3K/Akt pathway. TCM formulas have been widely used in the clinical treatment of a variety of CVD by adding and subtracting medicine components to achieve disease prevention.
CVD treatment has been a hot topic worldwide that has drawn great interest for decades. Reperfusion injury is a key pathological process in many cardiac diseases and remains a major problem in the medical field. Though a range of Western chemical drugs have been proposed to potently target the causes of CVD, undoubtedly, some chemical drugs can cause severe side effects, and the uses of some of these drugs are also limited by their high costs. In contrast to chemical drugs, TCM provides novel approaches, targeting MIRI due to their characteristics of multi-component, multi-target, and fewer side effects. In particular, the complex chemical composition of TCM formulas allows a single herb or formula to target multiple signaling pathways, highlighting that the components can improve cardiac functions synergistically. The unique advantages of TCM leave it a great space for development. For example, the additive beneficial effects of the combined treatment of TCM and Western medicine are worth further exploration. The beneficial effects are expected to be more remarkable if the dosages of TCM and chemical drugs are well balanced.
Despite the advantages of TCM in treating MIRI, evaluation of the efficacy and safety of herbs and formulas still lacks a systematic method; therefore, advanced technology for TCM assessment is expected to be developed using a variety of research models. Establishing global criteria for evaluating the performances of TCM contributes to popularizing TCM worldwide. Generally speaking, further research on TCM ingredient pharmacokinetics and pharmacodynamics and the most optimal dosages is necessary to provide a clearer blueprint for future TCM development, encouraging the production of more TCM-based treatments targeting MIRI.
Author contributions
NX and X-TL conceived the design, acquired and evaluated the literature, and wrote the manuscript; H-JZ, L-DF, J-YH, and K-XL critically assessed, and supplemented the contents of all the tables; AC, FC, and Y-JX modified orthographic and grammatical errors, facilitated the blueprint, and classified the content of all the figures and tables; CS, KY, and H-BL supervised, revised, and evaluated the manuscript for submission.
Funding
This study was supported by research grants from the National Natural Science Foundation of China (82100391 to H-B. L.), High-level New R&D Institute of Department of Science and Technology of Guangdong Province (2019B090904008 to H-B. L.), and High-level Innovative Research Institute of Department of Science and Technology of Guangdong Province (2021B0909050003 to H-B.L).
Conflict of interest
The authors declare that the research was conducted in the absence of any commercial or financial relationships that could be construed as a potential conflict of interest.
Publisher’s note
All claims expressed in this article are solely those of the authors and do not necessarily represent those of their affiliated organizations, or those of the publisher, the editors, and the reviewers. Any product that may be evaluated in this article, or claim that may be made by its manufacturer, are not guaranteed or endorsed by the publisher.
References
Andrienko, T. N., Pasdois, P., Pereira, G. C., Ovens, M. J., and Halestrap, A. P. (2017). The role of succinate and ROS in reperfusion injury -A critical appraisal. J. Mol. Cell. Cardiol. 110, 1–14. doi:10.1016/j.yjmcc.2017.06.016
Bai, S., Wang, X., Wu, H., Chen, T., Li, X., Zhang, L., et al. (2021). Cardioprotective effect of anisodamine against ischemia/reperfusion injury through the mitochondrial ATP-sensitive potassium channel. Eur. J. Pharmacol. 901, 174095. doi:10.1016/j.ejphar.2021.174095
Bai, X. J., and Ren, J. X. (2014). The treatment of No-reflow occurring during percutaneous coronary intervention on the pathogenesis of insidious phlegm. Chin. J. Exp. Traditional Med. Formulae 20 (16), 237–240. doi:10.13422/j.cnki.syfjx.2014160237
Billah, M. M., Khan, F., and Niaz, K. (2019). Scutellaria baicalensis Georgi. Nonvitamin Nonmineral Nutr. Suppl. 2019, 403–408. doi:10.1016/B978-0-12-812491-8.00054-0
Bittl, J. A., Tamis-Holland, J. E., and Lawton, J. S. (2022). Does bypass surgery or percutaneous coronary intervention improve survival in stable ischemic heart disease? JACC. Cardiovasc. Interv. 15 (12), 1243–1248. doi:10.1016/j.jcin.2022.05.011
Bortolotti, P., Faure, E., and Kipnis, E. (2018). Inflammasomes in tissue damages and immune disorders after trauma. Front. Immunol. 9, 1900. doi:10.3389/fimmu.2018.01900
Chen, X. Y., Xie, Q. F., Zhu, Y., Xu, J. M., Lin, G. S., Liu, S. J., et al. (2021). Cardio-protective effect of tetrahydrocurcumin, the primary hydrogenated metabolite of curcumin in vivo and in vitro: Induction of apoptosis and autophagy via PI3K/AKT/mTOR pathways. Eur. J. Pharmacol. 911, 174495. doi:10.1016/j.ejphar.2021.174495
Choy, K. W., Lau, Y. S., Murugan, D., and Mustafa, M. R. (2017). Chronic treatment with paeonol improves endothelial function in mice through inhibition of endoplasmic reticulum stress-mediated oxidative stress. PLoS One 12 (5), e0178365. doi:10.1371/journal.pone.0178365
Cui, Q. T., Wang, J. H., Liu, X. C., Wang, X. H., Ma, H. Y., and Su, G. B. (2020). Protective effect of emodin on cardiac function in rats with myocardial ischemia reperfusion injury by activating Nrf2/ARE/HO-1 signaling pathway. J. Anhui Univ. Chin. Med. 55, 894–900. doi:10.19405/j.cnki.issn1000-1492.2020.06.014
Cui, W., Xin, S., Zhu, L., Wang, M., Hao, Y., Zhao, Y., et al. (2021). Si-Miao-Yong-An decoction maintains the cardiac function and protects cardiomyocytes from myocardial ischemia and reperfusion injury. Evid. Based. Complement. Altern. Med. 2021, 8968464. doi:10.1155/2021/8968464
Demir Özkay, U., and Can, O. D. (2013). Anti-nociceptive effect of vitexin mediated by the opioid system in mice. Pharmacol. Biochem. Behav. 109, 23–30. doi:10.1016/j.pbb.2013.04.014
Deng, H., Yu, B., and Li, Y. (2021). Tanshinone IIA alleviates acute ethanol-induced myocardial apoptosis mainly through inhibiting the expression of PDCD4 and activating the PI3K/Akt pathway. Phytother. Res. 35 (8), 4309–4323. doi:10.1002/ptr.7102
Ding, H., Wang, Y., and Huang, Z. (2020a). Effects of TanshinoneⅡA on the expressions of hmgb1il-1β and SOD in myocardial ischemia-reperfusion injury in rats. J. Emerg. Tradit. Chin. Med. 029, 776–778. doi:10.3969/j.issn.1004-745X.2020.05.008
Ding, S. H. (2020b). Effects of tanshinone ⅡA on the expression of HMGB1, IL-23/IL-17 Axis and myeloperoxidase in rats with myocardial ischemia reperfusion injury. BaChu Med. J. 3 (3), 19–23. doi:10.3969/j.issn.2096-6113.2020.03.005
Dong, F., Xue, C. J., Wang, Y., and Zang, B. X. (2014). Inhibitory effect of safflor yellow on endothelium inflammatory factors ex-pression elevation induced by platelet activating factor. J. Cardiovasc. Pulm. Dis. 33, 281–285.
Dong, L., Fan, Y., Shao, X., and Chen, Z. (2011). Vitexin protects against myocardial ischemia/reperfusion injury in langendorff-perfused rat hearts by attenuating inflammatory response and apoptosis. Food Chem. Toxicol. 49 (12), 3211–3216. doi:10.1016/j.fct.2011.09.040
Dong, L. Y., Chen, Z. W., Guo, Y., Cheng, X. P., and Shao, X. (2008). Mechanisms of vitexin preconditioning effects on cultured neonatal rat cardiomyocytes with anoxia and reoxygenation. Am. J. Chin. Med. 36 (2), 385–397. doi:10.1142/S0192415X08005849
Dong, Y., Chen, H. W., Gao, J. J., Liu, Y. M., Li, J., and Wang, J. (2019). Molecular machinery and interplay of apoptosis and autophagy in coronary heart disease. J. Mol. Cell. Cardiol. 136, 27–41. doi:10.1016/j.yjmcc.2019.09.001
Durazzo, A., Nazhand, A., Lucarini, M., Silva, A. M., Souto, S. B., Guerra, F., et al. (2021). Astragalus (Astragalus membranaceus bunge): Botanical, geographical, and historical aspects to pharmaceutical components and beneficial role. Rend. Fis. Acc. Lincei. 32, 625–642. doi:10.1007/s12210-021-01003-2
Fang, X., Wang, H., Han, D., Xie, E., Yang, X., Wei, J., et al. (2019). Ferroptosis as a target for protection against cardiomyopathy. Proc. Natl. Acad. Sci. U. S. A. 116 (7), 2672–2680. doi:10.1073/pnas.1821022116
Feng, J. H., Kim, H. Y., Sim, S. M., Zuo, G. L., Jung, J. S., Hwang, S. H., et al. (2021). The anti-inflammatory and the antinociceptive effects of mixed agrimonia pilosa Ledeb. And Salvia miltiorrhiza bunge extract. Plants 10 (6), 1234. doi:10.3390/plants10061234
Fu, J. H., Zheng, Y. Q., Li, P., Li, X. Z., Shang, X. H., and Liu, J. X. (2013a). Hawthorn leaves flavonoids decreases inflammation related to acute myocardial ischemia/reperfusion in anesthetized dogs. Chin. J. Integr. Med. 19 (8), 582–588. doi:10.1007/s11655-012-1250-4
Fu, X. C., Chen, J. J., and Wang, J. P. (2013b). Effects of Huanglian Jiedu decoction on NF-κB signal transduction pathway of myocardial ischemia-reperfusion injury in rats. Mod. J. Integr. Traditional Chin. West. Med. 22 (1), 18–19.
Gao, J., Chen, G., He, H., Liu, C., Xiong, X., Li, J., et al. (2017). Therapeutic effects of breviscapine in cardiovascular diseases: A review. Front. Pharmacol. 8, 289. doi:10.3389/fphar.2017.00289
Gao, Q., Dong, J. Y., Cui, R., Muraki, I., Yamagishi, K., Sawada, N., et al. (2021). Consumption of flavonoid-rich fruits, flavonoids from fruits and stroke risk: A prospective cohort study. Br. J. Nutr. 126 (11), 1717–1724. doi:10.1017/S0007114521000404
Gao, Z., Sui, J., Fan, R., Qu, W., Dong, X., and Sun, D. (2020). Emodin protects against acute pancreatitis-associated lung injury by inhibiting NLPR3 inflammasome activation via Nrf2/HO-1 signaling. Drug Des. devel. Ther. 14, 1971–1982. doi:10.2147/DDDT.S247103
Gatica, D., Chiong, M., Lavandero, S., and Klionsky, D. J. (2015). Molecular mechanisms of autophagy in the cardiovascular system. Circ. Res. 116 (3), 456–467. doi:10.1161/CIRCRESAHA.114.303788
Ghosh, R., Gillaspie, J. J., Campbell, K. S., Symons, J. D., Boudina, S., and Pattison, J. S. (2022). Chaperone mediated autophagy protects cardiomyocytes against hypoxic-cell death. Am. J. Physiol. Cell Physiol. 323, C1555–C1575. doi:10.1152/ajpcell.00369.202110.1152/ajpcell.00369.2021
Granger, D. N., and Kvietys, P. R. (2015). Reperfusion injury and reactive oxygen species: The evolution of a concept. Redox Biol. 6, 524–551. doi:10.1016/j.redox.2015.08.020
He, J., Liu, D., Zhao, L., Zhou, D., Rong, J., Zhang, L., et al. (2022). Myocardial ischemia/reperfusion injury: Mechanisms of injury and implications for management (review). Exp. Ther. Med. 23 (6), 430. doi:10.3892/etm.2022.11357
Hori, Y. S., Kuno, A., Hosoda, R., and Horio, Y. (2013). Regulation of FOXOs and P53 by SIRT1 modulators under oxidative stress. PLoS One 8 (9), e73875. doi:10.1371/journal.pone.0073875
Huang, G. Q., and Liao, C. M. (2012). Protective mechanism of yiqihuayu decoction on acute myocardial ischemia-reperfusion injury in rats. Inf. Chin. Med. 04, 61–62.
Jiang, L., Zeng, H., Ni, L., Qi, L., Xu, Y., Xia, L., et al. (2019). HIF-1α preconditioning potentiates antioxidant activity in ischemic injury: The role of sequential administration of dihydrotanshinone I and protocatechuic aldehyde in cardioprotection. Antioxid. Redox Signal. 31 (3), 227–242. doi:10.1089/ars.2018.7624
Jiang, W. B., Zhao, W., Chen, H., Wu, Y. Y., Wang, Y., Fu, G. S., et al. (2018). Baicalin protects H9c2 cardiomyocytes against hypoxia/reoxygenation-induced apoptosis and oxidative stress through activation of mitochondrial aldehyde dehydrogenase 2. Clin. Exp. Pharmacol. Physiol. 45 (3), 303–311. doi:10.1111/1440-1681.12876
Jin, P., Li, L. H., Shi, Y., and Hu, N. B. (2021a). Salidroside inhibits apoptosis and autophagy of cardiomyocyte by regulation of circular RNA Hsa_Circ_0000064 in cardiac ischemia-reperfusion injury. Gene 767, 145075. doi:10.1016/j.gene.2020.145075
Jin, Z., Gan, C., Luo, G., Hu, G., Yang, X., Qian, Z., et al. (2021b). Notoginsenoside R1 protects hypoxia-reoxygenation deprivation-induced injury by upregulation of mir-132 in H9c2 cells. Hum. Exp. Toxicol. 40 (12), S29–S38. doi:10.1177/09603271211025589
Kulek, A. R., Anzell, A., Wider, J. M., Sanderson, T. H., and Przyklenk, K. (2020). Mitochondrial quality control: Role in cardiac models of lethal ischemia-reperfusion injury. Cells 9 (1), 214. doi:10.3390/cells9010214
Lee, C. Y., Chien, Y. S., Chiu, T. H., Huang, W. W., Lu, C. C., Chiang, J. H., et al. (2012). Apoptosis triggered by vitexin in U937 human leukemia cells via a mitochondrial signaling pathway. Oncol. Rep. 28 (5), 1883–1888. doi:10.3892/or.2012.2000
Leung, E. L., and Xu, S. (2020). Traditional Chinese medicine in cardiovascular drug discovery. Pharmacol. Res. 160, 105168. doi:10.1016/j.phrs.2020.105168
Li, B. (2019). Inhibitory effect of salvianolate on myocardial ischemia-reperfusion injury in rats and its mechanism. J. Integr. Traditional Chin. West. Med. Cardio-Cerebrovascular Dis. 17 (2), 192–195. doi:10.12102/j.issn.1672-1349-2019.02.008
Li, B., and Yu, H. (2021). Cardioprotective effect of wogonoside against myocardial ischemia reperfusion (I/R) injury in rats by modulating Nrf2/HO-1 pathway. Guangdong Chem. Ind. 48, 37–40.
Li, C. L., Liu, B., Wang, Z. Y., Xie, F., Qiao, W., Cheng, J., et al. (2020a). Salvianolic acid B improves myocardial function in diabetic cardiomyopathy by suppressing IGFBP3. J. Mol. Cell. Cardiol. 139, 98–112. doi:10.1016/j.yjmcc.2020.01.009
Li, H., and Zhu, T. M. (2012). Superficial view on TCM pathogenesis of myocardial ischemia reperfusion injury. Shi Zhen Med. Materia Medica Res. 23 (2), 2.
Li, J., Wang, Y., Hu, X., and Deng, X. (2021). Research progress of TCM intervention in myocardial ischemia reperfusion injury. J. Liaoning Univ. Tradit. Chin. Med. 23, 4–7. doi:10.13194/j.issn.1673-842x.2021.02.002
Li, L., Cai, Z. L., He, Y. F., Zhu, Y., Xi, J. K., and He, Y. G. (2017a). Role of endoplasmic reticulum stress in astragaloside?-induced cardioprotection in H9c2 cardiac cells. Chin. Pharmacol. Bull. 33, 854–858. doi:10.3969/j.issn.1001-1978.2017.06.021
Li, L., Pan, C. S., Yan, L., Cui, Y. C., Liu, Y. Y., Mu, H. N., et al. (2018). Ginsenoside Rg1 ameliorates rat myocardial ischemia-reperfusion injury by modulating energy metabolism pathways. Front. Physiol. 9, 78. doi:10.3389/fphys.2018.00078
Li, L., Sheng, X., Zhao, S., Zou, L., Han, X., Gong, Y., et al. (2017b). Nanoparticle-encapsulated emodin decreases diabetic neuropathic pain probably via A mechanism involving P2X3 receptor in the dorsal root ganglia. Purinergic Signal. 13 (4), 559–568. doi:10.1007/s11302-017-9583-2
Li, M., Wang, Z. J., Liu, J. Y., and Qin, L. (2019c). Regulatory effect of sodium tanshinone IIA sulfonate on cardiac function and immune response in rats with myocardial ischemia-reperfusion injury. Chin. J. Hand Surg. 37 (4), 397–402. doi:10.13418/j.issn-165x.2019.008
Li, Q., Yu, Z., Xiao, D., Wang, Y., Zhao, L., An, Y., et al. (2020b). Baicalein inhibits mitochondrial apoptosis induced by oxidative stress in cardiomyocytes by stabilizing MARCH5 expression. J. Cell. Mol. Med. 24 (2), 2040–2051. doi:10.1111/jcmm.14903
Li, R., Luan, F., Zhao, Y., Wu, M., Lu, Y., Tao, C., et al. (2023). Crataegus pinnatifida: A botanical, ethnopharmacological, phytochemical, and pharmacological overview. J. Ethnopharmacol. 301, 115819. doi:10.1016/j.jep.2022.115819
Li, W., Feng, G., Gauthier, J. M., Lokshina, I., Higashikubo, R., Evans, S., et al. (2019a). Ferroptotic cell death and TLR4/Trif signaling initiate neutrophil recruitment after heart transplantation. J. Clin. Invest. 129 (6), 2293–2304. doi:10.1172/JCI126428
Li, W., Li, W., Leng, Y., Xiong, Y., and Xia, Z. (2020c). Ferroptosis is involved in diabetes myocardial ischemia/reperfusion injury through endoplasmic reticulum stress. DNA Cell Biol. 39 (2), 210–225. doi:10.1089/dna.2019.5097
Li, Y., Fu, C. M., Ren, B., Liu, Y., Gao, F., Yang, H., et al. (2014). Study on attenuate and synergistic mechanism between aconiti lateralis praeparata radix and glycyrrhizae radix for toxicity reduction based on metabonomic of MI-RI mouse cardiomyocytes. China J. Chin. materia medica 39 (16), 3166–3171. doi:10.4268/cjcmm20141632
Li, Y., Wei, Y., Cheng, W., Xu, T., and Zhang, S. (2019b). Protective effects of combination of Panax notoginseng total saponins and safflower total favonoids on myocardium ischemia and reperfusion injury. Int. Forum Allergy Rhinol. 21, 1346–1351. doi:10.1002/alr.22424
Lin, F., Tan, Y. Q., He, X. H., Guo, L. L., Wei, B. J., Li, J. P., et al. (2020). Huoxue huatan decoction ameliorates myocardial ischemia/reperfusion injury in hyperlipidemic rats via PGC-1α-pparα and PGC-1α-NRF1-mtTFA pathways. Front. Pharmacol. 11, 546825. doi:10.3389/fphar.2020.546825
Lin, K., An, H., Miao, C., Huang, G., and Diao, J. (2015a). Effect of Yiqi Huayu decoction on the expression of apoptosis related proteins in myocardial cells of rats with ischemia reperfusion injury. J. Tradit. Chin. Med. 24, 1936–1938. doi:10.3969/j.issn.1004-745x.2015.11.017
Lin, X., More, A. S., Kraut, J. A., and Wu, D. (2015b). Interaction of sodium bicarbonate and Na+/H+ exchanger inhibition in the treatment of acute metabolic acidosis in pigs. Crit. Care Med. 43 (6), e160–e169. doi:10.1097/CCM.0000000000000962
Liu, C., Wang, M., Fan, Y., and Shan, W. (2020a). Paeonol alleviates myocardial ischemia reperfusion injury in rats by promoting SIRT1. Hebei Med. 26, 917–921. doi:10.3969/j.issn.1006-6233.2020.06.008
Liu, D., Chen, L., Zhao, J., and Cui, K. (2018a). Cardioprotection activity and mechanism of Astragalus polysaccharide in vivo and in vitro. Int. J. Biol. Macromol. 111, 947–952. doi:10.1016/j.ijbiomac.2018.01.048
Liu, H., Chen, X., Liu, Y., Fang, C., and Chen, S. (2021a). Antithrombotic effects of huanglian Jiedu decoction in a rat model of ischaemia-reperfusion-induced cerebral stroke. Pharm. Biol. 59 (1), 823–827. doi:10.1080/13880209.2021.1942505
Liu, H. H., He, S. H., and Liu, M. L. (2021b). The combination of the effective components of angelica sinensis decoction inhibiting oxidative stress and intervening myocardial reperfusion injury. Chin. J. Gerontology 41 (01), 112–115. doi:10.3969/j.issn.1005-9202.2021.01.034
Liu, H., Liu, W., Qiu, H., Zou, D., Cai, H., Chen, Q., et al. (2020b). Salvianolic acid B protects against myocardial ischaemia-reperfusion injury in rats via inhibiting high mobility group box 1 protein expression through the PI3K/Akt signalling pathway. Naunyn. Schmiedeb. Arch. Pharmacol. 393 (8), 1527–1539. doi:10.1007/s00210-019-01755-7
Liu, H., and Zhou, Z. (2019). Research progress of berberine in lipid regulating. Drug Eval. Res. 42, 1676–1679. doi:10.7501/j.issn.1674-6376.2019.08.034
Liu, J., Li, Y., Tang, Y., Cheng, J., Wang, J., Li, J., et al. (2018b). Rhein protects the myocardiac cells against hypoxia/reoxygention induced injury by suppressing GSK3β activity. Phytomedicine 51, 1–6. doi:10.1016/j.phymed.2018.06.029
Liu, J., Wu, P., Xu, Z., Zhang, J., Liu, J., and Yang, Z. (2020c). Ginkgolide B inhibits hydrogen peroxide-induced apoptosis and attenuates cytotoxicity via activating the PI3K/Akt/Mtor signaling pathway in H9c2 cells. Mol. Med. Rep. 22 (1), 310–316. doi:10.3892/mmr.2020.11099
Liu, X. W., Lu, M. K., Zhong, H. T., Liu, J. J., and Fu, Y. P. (2021c). Panax notoginseng saponins protect H9c2 cells from hypoxia-reoxygenation injury through the forkhead box O3a hypoxia-inducible factor-1 alpha cell signaling pathway. J. Cardiovasc. Pharmacol. 78 (5), e681–e689. doi:10.1097/FJC.0000000000001120
Liu, X. W., Lu, M. K., Zhong, H. T., Wang, L. H., and Fu, Y. P. (2019). Panax notoginseng saponins attenuate myocardial ischemia-reperfusion injury through the HIF-1α/BNIP3 pathway of autophagy. J. Cardiovasc. Pharmacol. 73 (2), 92–99. doi:10.1097/FJC.0000000000000640
Liu, Z. H., Zhang, H. M., Tang, D. L., Yu, Z., and Cao, H. X. (2014). To explore traditional Chinese medicine on myocardial ischemia-reperfusion without reflow from "yang deficiency phlegm stasis. Chin. J. Basic Med. Traditional Chin. Med. 20 (2), 166–167.
Lu, Q. Y., Ma, J. Q., Duan, Y. Y., Sun, Y., Yu, S., Li, B., et al. (2019). Carthamin yellow protects the heart against ischemia/reperfusion injury with reduced reactive oxygen species release and inflammatory response. J. Cardiovasc. Pharmacol. 74 (3), 228–234. doi:10.1097/FJC.0000000000000710
Ma, L., Li, X. P., Ji, H. S., Liu, Y. F., and Li, E. Z. (2018). Baicalein protects rats with diabetic cardiomyopathy against oxidative stress and inflammation injury via phosphatidylinositol 3-kinase (PI3K)/AKT pathway. Med. Sci. Monit. 24, 5368–5375. doi:10.12659/MSM.911455
Ma, Y. X., Guo, R. Z., Zhou, H. C., Wang, Y. L., Wang, S., He, Z. F., et al. (2013). Research progress of traditional Chinese medicine treatment of myocardial ischemia-reperfusion injury. Inf. Tradit. Chin. Med. 30 (5), 116–118. doi:10.19656/j.cnki.1002-2406.2013.05.045
Martins-Marques, T., Rodriguez-Sinovas, A., and Girao, H. (2021). Cellular crosstalk in cardioprotection: Where and when do reactive oxygen species play a role? Free Radic. Biol. Med. 169, 397–409. doi:10.1016/j.freeradbiomed.2021.03.044
Marzilli, M., Crea, F., Morrone, D., Bonow, R. O., Brown, D. L., Camici, P. G., et al. (2020). Myocardial ischemia: From disease to syndrome. Int. J. Cardiol. 314, 32–35. doi:10.1016/j.ijcard.2020.04.074
Miao, C., Hui, A. N., Huang, G., and Diao, J. (2013). Effect of Yiqi Huayu recipe on myocardial creatases and serum superoxide dismutase and malondialdehyde in ischemia-reperfusion rats. Traditional Chin. Drug Res. Clin. Pharmacol. 24, 585–587. doi:10.3969/j.issn.1003-9783.2013.06.015
Min, J., and Wei, C. (2017). Hydroxysafflor yellow A cardioprotection in ischemia-reperfusion (I/R) injury mainly via akt/hexokinase II independent of ERK/GSK-3β pathway. Biomed. Pharmacother. 87, 419–426. doi:10.1016/j.biopha.2016.12.113
Park, T. J., Park, J. H., Lee, G. S., Lee, J. Y., Shin, J. H., Kim, M. W., et al. (2019). Quantitative proteomic analyses reveal that GPX4 downregulation during myocardial infarction contributes to ferroptosis in cardiomyocytes. Cell Death Dis. 10 (11), 835. doi:10.1038/s41419-019-2061-8
Parmenter, B. H., Croft, K. D., Hodgson, J. M., Dalgaard, F., Bondonno, C. P., Lewis, J. R., et al. (2020). An overview and update on the epidemiology of flavonoid intake and cardiovascular disease risk. Food Funct. 11 (8), 6777–6806. doi:10.1039/d0fo01118e
Qian, G. Q., Peng, X., Cai, C., and Zhao, G. P. (2014). Effect on eNOS/NO Pathway in MIRI rats with preconditioning of GFPC from Dang Gui Si Ni decoction. Pharmacogn. Res. 6 (2), 133–137. doi:10.4103/0974-8490.129032
Rabinovich-Nikitin, I., Rasouli, M., Reitz, C. J., Posen, I., Margulets, V., Dhingra, R., et al. (2021). Mitochondrial autophagy and cell survival is regulated by the circadian Clock gene in cardiac myocytes during ischemic stress. Autophagy 17 (11), 3794–3812. doi:10.1080/15548627.2021.1938913
Russo, P., Prinzi, G., Lamonaca, P., Cardaci, V., and Fini, M. (2019). Flavonoids and reduction of cardiovascular disease (CVD) in chronic obstructive pulmonary disease (COPD). Curr. Med. Chem. 26 (39), 7048–7058. doi:10.2174/0929867325666180514100640
Shah, S. A., Khan, M., Jo, M. H., Jo, M. G., Amin, F. U., and Kim, M. O. (2017). Melatonin stimulates the SIRT1/nrf2 signaling pathway counteracting lipopolysaccharide (LPS)-Induced oxidative stress to rescue postnatal rat brain. CNS Neurosci. Ther. 23 (1), 33–44. doi:10.1111/cns.12588
Shao, L. Q., Bai, T. T., Zhao, Q., Wang, C. G., Xuan, L. Y., Wang, Y., et al. (2020). Astragalus injection mediates autophagy and myocardial remodeling in rats with ischemic cardiomyopathy through calumenin. Chin. J. Appl. Physiol. 36 (4), 346–349. doi:10.12047/j.cjap.5922.2020.074
Shi, B. H., Ma, M. Q., Zheng, Y. T., Pan, Y. Y., and Lin, X. H. (2019). mTOR and Beclin1: Two key autophagy-related molecules and their roles in myocardial ischemia/reperfusion injury. J. Cell. Physiol. 234 (8), 12562–12568. doi:10.1002/jcp.28125
Shi, H., Gao, Y., Dong, Z., Yang, J., Gao, R., Li, X., et al. (2021a). GSDMD-mediated cardiomyocyte pyroptosis promotes myocardial I/R injury. Circ. Res. 129 (3), 383–396. doi:10.1161/CIRCRESAHA.120.318629
Shi, X., Gao, Q., and Shan, Y. (2018). Sanchi total saponins pretreatment protects rats with myocardial ischemia/reperfusion injury. Chin. Foreign Med. Res. 16, 175–176. doi:10.14033/j.cnki.cfmr.2018.36.084
Shi, Y., Gu, X., Li, Y., Li, H., and Li, H. M. (2021b). Protection of Danshensu on myocardium against ischemia-reperfusion injury through inhibiting miR-199a-5p. Drugs Clin. 36, 1104–1111. doi:10.7501/j.issn.1674-5515.2021.06.002
Solola, N. S., Henry, S., Yong, C. M., Daugherty, S. L., Mehran, R., and Poppas, A. (2022). Sex-specific considerations in the presentation, diagnosis, and management of ischemic heart disease: JACC focus seminar 2/7. J. Am. Coll. Cardiol. 79 (14), 1398–1406. doi:10.1016/j.jacc.2021.11.065
Song, M., Huang, L., Zhao, G., and Song, Y. (2013). Beneficial effects of a polysaccharide from Salvia miltiorrhiza on myocardial ischemia-reperfusion injury in rats. Carbohydr. Polym. 98 (2), 1631–1636. doi:10.1016/j.carbpol.2013.08.020
Song, Y., Gong, R., Yang, B., Wang, L., and Zhang, L. (2019). Effects of wogonoside on anoxia-reoxygenation induced H9c2 myocardial cells injury and expression of P38 and ERK1/2. West China Med. J. 31, 1820–1825. doi:10.3969/jissn.1672-3511.2019.12.004
Song, Z. Y., Chen, Y. C., Yong, W. X., Zhang, Z. M., Liu, Y. Q., Zhang, L. Y., et al. (2020). Effects of Buyang Huanwu decoction pretreatment on antioxidant function in rats with myocardial ischemia-rreperfusion injury. J. Basic Chin. Med. 26 (11), 1621–1625.
Su, C. Y., Ming, Q. L., Rahman, K., Han, T., and Qin, L. P. (2015). Salvia miltiorrhiza: Traditional medicinal uses, chemistry, and pharmacology. Chin. J. Nat. Med. 13 (3), 163–182. doi:10.1016/S1875-5364(15)30002-9
Sun, D. W., Gao, Q., and Qi, X. (2020). Danshensu ameliorates cardiac ischaemia reperfusion injury through activating sirt1/FoxO1/rab7 signal pathway. Chin. J. Integr. Med. 26 (4), 283–291. doi:10.1007/s11655-019-3165-9
Sun, J., Zhao, Q., Li, P., and Wei, B. (2021c). Effects of berberine on mitochondrial autophagy and PINK1/parkin pathway in rats with myocardial ischemia-reperfusion. Drugs Clin. 36, 637–644. doi:10.7501/j.issn.1674-5515.2021.04.001
Sun, M. Y., Ma, D. S., Zhao, S., Wang, L., Ma, C. Y., and Bai, Y. (2018). Salidroside mitigates hypoxia/reoxygenation injury by alleviating endoplasmic reticulum stress-induced apoptosis in H9c2 cardiomyocytes. Mol. Med. Rep. 18 (4), 3760–3768. doi:10.3892/mmr.2018.9403
Sun, W., Wang, Z., Sun, M., Huang, W., Wang, Y., and Wang, Y. (2021b). Aloin antagonizes stimulated ischemia/reperfusion-induced damage and inflammatory response in cardiomyocytes by activating the Nrf2/HO-1 defense pathway. Cell Tissue Res. 384 (3), 735–744. doi:10.1007/s00441-020-03345-z
Sun, X., Wang, X., He, Q., Zhang, M., Chu, L., Zhao, Y., et al. (2021a). Investigation of the ameliorative effects of baicalin against arsenic trioxide-induced cardiac toxicity in mice. Int. Immunopharmacol. 99, 108024. doi:10.1016/j.intimp.2021.108024
Tang, L. J., Zhou, Y. J., Xiong, X. M., Li, N. S., Zhang, J. J., Luo, X. J., et al. (2021). Ubiquitin-specific protease 7 promotes ferroptosis via activation of the p53/TfR1 pathway in the rat hearts after ischemia/reperfusion. Free Radic. Biol. Med. 162, 339–352. doi:10.1016/j.freeradbiomed.2020.10.307
Tian, L., Cao, W., Yue, R., Yuan, Y., Guo, X., Qin, D., et al. (2019). Pretreatment with tilianin improves mitochondrial energy metabolism and oxidative stress in rats with myocardial ischemia/reperfusion injury via AMPK/SIRT1/PGC-1 alpha signaling pathway. J. Pharmacol. Sci. 139 (4), 352–360. doi:10.1016/j.jphs.2019.02.008
Tian, X., Huang, Y., Zhang, X., Fang, R., Feng, Y., Zhang, W., et al. (2022). Salidroside attenuates myocardial ischemia/reperfusion injury via AMPK-induced suppression of endoplasmic reticulum stress and mitochondrial fission. Toxicol. Appl. Pharmacol. 448, 116093. doi:10.1016/j.taap.2022.116093
Trerotola, M., Relli, V., Tripaldi, R., Sacchetti, A., Alberti, S., Simeone, P., et al. (2017). Trop-2 activates a dormant Na+/K+-ATPase/PKCα/CD9/ezrin signaling Axis to override the basal growth program of cancer cells. Cancer Res. 77, 367. doi:10.1158/1538-7445.am2017-367
Tsai, C. F., Su, H. H., Chen, K. M., Liao, J. M., Yao, Y. T., Chen, Y. H., et al. (2021). Paeonol protects against myocardial ischemia/reperfusion-induced injury by mediating apoptosis and autophagy crosstalk. Front. Pharmacol. 11, 586498. doi:10.3389/fphar.2020.586498
Wan, X., Wen, J. J., Koo, S. J., Liang, L. Y., and Garg, N. J. (2016). SIRT1-PGC1α-NFκB pathway of oxidative and inflammatory stress during trypanosoma cruzi infection: Benefits of SIRT1-targeted therapy in improving heart function in chagas disease. PLoS Pathog. 12 (10), e1005954. doi:10.1371/journal.ppat.1005954
Wan, Y. J., Wang, Y. H., Guo, Q., Jiang, Y., Tu, P. F., and Zeng, K. W. (2021). Protocatechualdehyde protects oxygen-glucose deprivation/reoxygenation -induced myocardial injury via inhibiting PERK/ATF6α/IRE1α pathway. Eur. J. Pharmacol. 891, 173723. doi:10.1016/j.ejphar.2020.173723
Wang, C., Wang, Y., Song, D., Su, J., and Zhang, F. (2022a). Therapeutic effects of modified Si-Miao-Yong-An decoction in the treatment of rat myocardial ischemia/reperfusion injury. Evid. Based. Complement. Altern. Med. 2022, 1442405. doi:10.1155/2022/1442405
Wang, D., Lv, L., Xu, Y., Jiang, K., Chen, F., Qian, J., et al. (2021a). Cardioprotection of Panax notoginseng saponins against acute myocardial infarction and heart failure through inducing autophagy. Biomed. Pharmacother. 136, 111287. doi:10.1016/j.biopha.2021.111287
Wang, F. Y., Gao, Q. P., Yang, J., Wang, C., Cao, J. X., Sun, J. F., et al. (2020a). Artemisinin suppresses myocardial ischemia-reperfusion injury via NLRP3 inflammasome mechanism. Mol. Cell. Biochem. 474 (1-2), 171–180. doi:10.1007/s11010-020-03842-3
Wang, H., Pang, W., Xu, X., You, B., Zhang, C., and Li, D. (2021b). Cryptotanshinone attenuates ischemia/reperfusion-induced apoptosis in myocardium by upregulating MAPK3. J. Cardiovasc. Pharmacol. 77 (3), 370–377. doi:10.1097/FJC.0000000000000971
Wang, J., Toan, S., and Zhou, H. (2020b). New insights into the role of mitochondria in cardiac microvascular ischemia/reperfusion injury. Angiogenesis 23 (3), 299–314. doi:10.1007/s10456-020-09720-2
Wang, L., Chen, X., Wang, Y., Zhao, L., Zhao, X., and Wang, Y. (2020c). MiR-30c-5p mediates the effects of Panax notoginseng saponins in myocardial ischemia reperfusion injury by inhibiting oxidative stress-induced cell damage. Biomed. Pharmacother. 125, 109963. doi:10.1016/j.biopha.2020.109963
Wang, N., Wu, L., Cao, Y., Wang, Y., and Zhang, Y. (2013). The protective activity of imperatorin in cultured neural cells exposed to hypoxia reoxygenation injury via anti-apoptosis. Fitoterapia 90, 38–43. doi:10.1016/j.fitote.2013.07.007
Wang, N., and Yan, D. (2016). Research progress of traditional Chinese medicine against myocardial ischemia-reperfusion injury. J. Shandong Univ. Tradit. Chin. Med. 40 (2), 188–191. doi:10.16294/j.cnki.1007-659x.2016.02.030
Wang, R., Wang, M., He, S., Sun, G., and Sun, X. (2020d). Targeting calcium homeostasis in myocardial ischemia/reperfusion injury: An overview of regulatory mechanisms and therapeutic reagents. Front. Pharmacol. 11, 872. doi:10.3389/fphar.2020.00872
Wang, R., Wang, M., Liu, B., Xu, H., Ye, J., Sun, X., et al. (2022b). Calenduloside E protects against myocardial ischemia-reperfusion injury induced calcium overload by enhancing autophagy and inhibiting L-type Ca2+ channels through BAG3. Biomed. Pharmacother. 145, 112432. doi:10.1016/j.biopha.2021.112432
Wang, R., Wang, M., Zhou, J., Dai, Z., Sun, G., and Sun, X. (2020e). Calenduloside E suppresses calcium overload by promoting the interaction between L-type calcium channels and bcl2-associated athanogene 3 to alleviate myocardial ischemia/reperfusion injury. J. Adv. Res. 34, 173–186. doi:10.1016/j.jare.2020.10.005
Wang, R., Wang, M., Zhou, J., Wu, D., Ye, J., Sun, G., et al. (2021c). Saponins in Chinese herbal medicine exerts protection in myocardial ischemia-reperfusion injury: Possible mechanism and target analysis. Front. Pharmacol. 11, 570867. doi:10.3389/fphar.2020.570867
Wang, S., Sun, L., Zhu, Z., Liu, J., Ge, W., Li, B., et al. (2022c). Cryptotanshinone alleviates myocardial ischemia and reperfusion injury in rats to mitigate ER stress-dependent apoptosis by modulating the JAK1/STAT3 Axis. Am. J. Transl. Res. 14 (7), 5024–5039. PMCID: PMC9360873
Wang, S. (2020). The effect and mechanism of hyperoside on attenuating myocardial ischemia-reperfusion injury via activating PKC/mito KATP signaling pathway in rats. Wuhu, China: Wan Nan Medical College. doi:10.27374/d.cnki.gwnyy.2020.000229
Wang, W., Ye, H., Sun, L., and Bao, T. (2021d). Scutellarin promotes autophagy, inhibits oxidative damage and apoptosis in microvascular endothelial cells during ischemia-reperfusion injury. Chin. Pharmacol. Bull. 37, 946–951. doi:10.3969/j.issn.1001-1978.2021.07.012
Wang, X., Li, H., Li, W., Xie, J., Wang, F., Peng, X., et al. (2020f). The role of Caspase-1/GSDMD-mediated pyroptosis in Taxol-induced cell death and a Taxol-resistant phenotype in nasopharyngeal carcinoma regulated by autophagy. Cell Biol. Toxicol. 36 (5), 437–457. doi:10.1007/s10565-020-09514-8
Wang, X., Liu, B., Zhang, Y., Wang, X., Zhou, H., and Ji, F. (2021e). Effect of total alkaloids of rhizoma coptidis and Panax notoginseng saponins on myocardial ischemia-reperfusion injury in diabetic rats. Cent. South Pharm. 19, 1092–1097. doi:10.7539/j.issn.1672-2981.2021.06.012
Wang, X., Wang, Q., Li, W., Zhang, Q., Jiang, Y., Guo, D., et al. (2020g). TFEB-NF-κb inflammatory signaling Axis: A novel therapeutic pathway of dihydrotanshinone I in doxorubicin-induced cardiotoxicity. J. Exp. Clin. Cancer Res. 39 (1), 93. doi:10.1186/s13046-020-01595-x
Wang, Y., Li, Y., Jiang, Q., and Li, X. (2021f). Non-muscle myosin heavy chain 9 maintains intestinal homeostasis by preventing epithelium necroptosis and colitis adenoma formation. Stem Cell Rep. 19, 1290–1301. doi:10.1016/j.stemcr.2021.03.027
Wang, Y. X., She, L., Luo, X. J., and Peng, J. (2017). The effect of Salvia magnesium lithospermate B on myocardial ischemia-reperfusion induced programmed necrosis in rat and its mechanisms. Chin. J. Arterioscler. 25 (6), 571575.
Wei, W., Tang, J., Hu, L., Feng, Y., Li, H., Yin, C., et al. (2021). Experimental anti-tumor effect of emodin in suspension- in situ hydrogels formed with self-assembling peptide. Drug Deliv. 28 (1), 1810–1821. doi:10.1080/10717544.2021.1971795
Wen, L., He, T., Yu, A., Sun, S., Li, X., Wei, J., et al. (2021). Breviscapine: A review on its phytochemistry, pharmacokinetics and therapeutic effects. Am. J. Chin. Med. 49 (6), 1369–1397. doi:10.1142/S0192415X21500646
Wu, W., Da, J., Wu, T., Yang, L., and Guo, D. (2017). General situations and several thoughts on Japanese pharmacopoeia, Korean pharmacopoeia and taiwan herbal pharmacopoeia. Mod. Traditional Chin. Med. Materia Medica-World Sci. Technol. 19, 1258–1265. doi:10.11842/wst.2017.07.029
Wu, Y., Fan, Z., Chen, Z., Hu, J., Cui, J., Liu, Y., et al. (2020). Astragaloside IV protects human cardiomyocytes from hypoxia/reoxygenation injury by regulating MiR-101a. Mol. Cell. Biochem. 470 (1-2), 41–51. doi:10.1007/s11010-020-03743-5
Xiang, M., Lu, Y., Xin, L., Gao, J., Shang, C., Jiang, Z., et al. (2021). Role of oxidative stress in reperfusion following myocardial ischemia and its treatments. Oxid. Med. Cell. Longev. 2021, 6614009. doi:10.1155/2021/6614009
Xiao, B., Huang, X., Wang, Q., and Wu, Y. (2020). Beta-asarone alleviates myocardial ischemia-reperfusion injury by inhibiting inflammatory response and NLRP3 inflammasome mediated pyroptosis. Biol. Pharm. Bull. 43 (7), 1046–1051. doi:10.1248/bpb.b19-00926
Xiao, R., Xiang, A. L., Pang, H. B., and Liu, K. Q. (2017). Hyperoside protects against hypoxia/reoxygenation induced injury in cardiomyocytes by suppressing the Bnip3 expression. Gene 629, 86–91. doi:10.1016/j.gene.2017.07.063
Xie, L. D., Liu, Y., Zhou, K., and Wu, Y. (2015). Superficial view on TCM pathogenesis of myocardial ischemia reperfusion injury. Chin. J. Tradit. Chin. Med. Pharm. 30 (9), 3139–3141.
Xu, J., Zhang, W., Cui, W., Shi, B., and Wang, H. (2019). PKCα promotes insulin secretion via TRPC1 phosphorylation in INS-1E cells. Biosci. Biotechnol. Biochem. 83 (9), 1676–1682. doi:10.1080/09168451.2019.1617106
Xu, M., Hao, H., Jiang, L., Wei, Y., Zhou, F., Sun, J., et al. (2016). Cardiotonic Pill reduces myocardial ischemia-reperfusion injury via increasing EET concentrations in rats. Drug Metab. Dispos. 44 (7), 878–887. doi:10.1124/dmd.116.069914
Xu, S., Chen, S., Xia, W., Sui, H., and Fu, X. (2022). Hyperoside: A review of its structure, synthesis, pharmacology, pharmacokinetics and toxicity. Molecules 27 (9), 3009. doi:10.3390/molecules27093009
Xue, W. (2020). Vitexin attenuates myocardial ischemia/reperfusion injury in rats by regulating mitochondrial dysfunction via epac1-rap1 signaling pathway. Hefei, China: Anhui Medical University. doi:10.26921/d.cnki.ganyu.2020.000206
Yan, L. L., Zhang, W. Y., Wei, X. H., Yan, L., Pan, C. S., Yu, Y., et al. (2018). Gualou Xiebai decoction, a traditional Chinese medicine, prevents cardiac reperfusion injury of hyperlipidemia rat via energy modulation. Front. Physiol. 9, 296. doi:10.3389/fphys.2018.00296
Yang, H., Xue, W., Ding, C., Wang, C., Xu, B., Chen, S., et al. (2021a). Vitexin mitigates myocardial ischemia/reperfusion injury in rats by regulating mitochondrial dysfunction via epac1-rap1 signaling. Oxid. Med. Cell. Longev. 2021, 9921982. doi:10.1155/2021/9921982
Yang, L., Yang, Z. M., Zhang, N., Tian, Z., Liu, S. B., and Zhao, M. G. (2014). Neuroprotective effects of vitexin by inhibition of NMDA receptors in primary cultures of mouse cerebral cortical neurons. Mol. Cell. Biochem. 386 (1-2), 251–258. doi:10.1007/s11010-013-1862-9
Yang, N., Cui, Z., Zhang, B., Bi, J., Ma, Q., and Wang, G. (2021b). Reduction of breviscapine on myocardial ischemia-reperfusion injury in diabetic rats by activating mitochondrial autophagy. Drugs Clin. 36, 219–225. doi:10.7501/j.issn.1674-5515.2021.02.002
Yang, P., Zhou, Y., Xia, Q., Yao, L., and Chang, X. (2019). Astragaloside IV regulates the PI3K/Akt/HO-1 signaling pathway and inhibits H9c2 cardiomyocyte injury induced by hypoxia-reoxygenation. Biol. Pharm. Bull. 42 (5), 721–727. doi:10.1248/bpb.b18-00854
Yang, Y. K., Li, J., Rao, T. C., Fang, Z. R., and Zhang, J. Y. (2021c). The role and mechanism of hyperoside against myocardial infarction in mice by regulating autophagy via NLRP1 inflammation pathway. J. Ethnopharmacol. 276, 114187. doi:10.1016/j.jep.2021.114187
Yao, Y., Li, F., Zhang, M., Jin, L., Xie, P., Liu, D., et al. (2022). Targeting CaMKII-δ9 ameliorates cardiac ischemia/reperfusion injury by inhibiting myocardial inflammation. Circ. Res. 130 (6), 887–903. doi:10.1161/CIRCRESAHA.121.319478
Ye, B., Chen, X., Dai, S., Han, J., Liang, X., Lin, S., et al. (2019). Emodin alleviates myocardial ischemia/reperfusion injury by inhibiting gasdermin D-mediated pyroptosis in cardiomyocytes. Drug Des. devel. Ther. 13, 975–990. doi:10.2147/DDDT.S195412
Ye, M., Qu, J., Yang, L., Zheng, Y., Liu, B., and Zhang, J. (2021). The analysis of psoralen synthase in Ficus hirta Vahl by rough set theory. Mol. Plant Breed. 19 (22), 1–12. doi:10.13271/j.mpb.019.007398
Yin, B., Hou, X. W., and Lu, M. L. (2019). Astragaloside IV attenuates myocardial ischemia/reperfusion injury in rats via inhibition of calcium-sensing receptor-mediated apoptotic signaling pathways. Acta Pharmacol. Sin. 40 (5), 599–607. doi:10.1038/s41401-018-0082-y
Yu, J., Li, Y., Liu, X., Ma, Z., Michael, S., Orgah, J. O., et al. (2019). Mitochondrial dynamics modulation as a critical contribution for Shenmai injection in attenuating hypoxia/reoxygenation injury. J. Ethnopharmacol. 237, 9–19. doi:10.1016/j.jep.2019.03.033
Yu, Y., Sun, G., Luo, Y., Wang, M., Chen, R., Zhang, J., et al. (2016). Cardioprotective effects of notoginsenoside R1 against ischemia/reperfusion injuries by regulating oxidative stress- and endoplasmic reticulum stress- related signaling pathways. Sci. Rep. 6, 21730. doi:10.1038/srep21730
Yu, Y. W., Liu, S., Zhou, Y. Y., Huang, K. Y., Wu, B. S., Lin, Z. H., et al. (2022). Shexiang Baoxin Pill attenuates myocardial ischemia/reperfusion injury by activating autophagy via modulating the cerna-map3k8 pathway. Phytomedicine. 104, 154336. doi:10.1016/j.phymed.2022.154336
Yuan, X., Xiang, Y., Zhu, N., Zhao, X., Ye, S., Zhong, P., et al. (2017). Salvianolic acid A protects against myocardial ischemia/reperfusion injury by reducing platelet activation and inflammation. Exp. Ther. Med. 14 (2), 961–966. doi:10.3892/etm.2017.4619
Zhai, P. Y., and Sadoshima, J. (2012). Glycogen synthase kinase-3β controls autophagy during myocardial ischemia and reperfusion. Autophagy 8 (1), 138–139. doi:10.4161/auto.8.1.18314
Zhang, M., Cheng, K., Yu, L., Wu, W., Wang, Y., and Chen, Y. (2022). Mechanism of tanshinone II A inhibiting myocardial remodeling by galectin-3. Zhonghua Wei Zhong Bing Ji Jiu Yi Xue 34 (6), 640–645. doi:10.3760/cma.j.cn121430-20220309-00232
Zhang, W., Sun, Z., and Meng, F. (2017). Schisandrin B ameliorates myocardial ischemia/reperfusion injury through attenuation of endoplasmic reticulum stress-induced apoptosis. Inflammation 40 (6), 1903–1911. doi:10.1007/s10753-017-0631-4
Zhang, Y., Coats, A., Zheng, Z., Adamo, M., Ambrosio, G., Anker, S. D., et al. (2020). Management of heart failure patients with COVID-19: A joint position paper of the Chinese heart failure association & national heart failure committee and the heart failure association of the European society of cardiology. Eur. J. Heart Fail. 22 (6), 941–956. doi:10.1002/ejhf.1915
Zhao, H., Zhang, D., Pang, L., and Zhang, H. Yu, R. (2021a). Effects of astragaloside IV and ginsenoside Rg1 on myocardial apoptosis after myocardial ischemia reperfusion injury in hyperlipidemia rats. Liaoning J. Tradit. Chin. Med. 48, 188–191. doi:10.13192/j.issn.1000-1719.2021.02.050
Zhao, T., Wu, W., Sui, L., Huang, Q., Nan, Y., Liu, J., et al. (2021b). Reactive oxygen species-based nanomaterials for the treatment of myocardial ischemia reperfusion injuries. Bioact. Mat. 7, 47–72. doi:10.1016/j.bioactmat.2021.06.006
Zhao, Z., Wang, X., Wang, S., Zhou, R., Su, Q., Liu, Y., et al. (2020). Research based on the core pathogenesis in the treatment according to traditional Chinese medicine syndrome differentiation for heart failure with normal ejection fraction. Medicine 99 (37), e21663. doi:10.1097/MD.0000000000021663
Zheng, J., Chen, P., Zhong, J., Cheng, Y., Chen, H., He, Y., et al. (2021). HIF-1α in myocardial ischemia-reperfusion injury (Review). Mol. Med. Rep. 23 (5), 352. doi:10.3892/mmr.2021.11991
Zhou, J., Zhang, L., Zheng, B., Zhang, L., Qin, Y., Zhang, X., et al. (2020). Salvia miltiorrhiza bunge exerts anti-oxidative effects through inhibiting KLF10 expression in vascular smooth muscle cells exposed to high glucose. J. Ethnopharmacol. 262, 113208. doi:10.1016/j.jep.2020.113208
Zhou, Q., Meng, G., Teng, F., Sun, Q., and Zhang, Y. (2018). Effects of Astragalus polysaccharide on apoptosis of myocardial microvascular endothelial cells in rats undergoing hypoxia/reoxygenation by mediation of the PI3K/Akt/Enos signaling pathway. J. Cell. Biochem. 119 (1), 806–816. doi:10.1002/jcb.26243
Zhou, W., and Liu, Z. (2019). Protective effect of notoginsenoside R1 preconditioning on myocardial ischemia/reperfusion injury in rats and its related mechanism. Chin. J. Clin. Pharmacol. 035, 2589–2592. doi:10.13699/j.cnki.1001-6821.2019.20.016
Keywords: myocardial ischemia–reperfusion injury, pathogenesis, traditional Chinese medicine, cardioprotective effects, molecular mechanisms
Citation: Xing N, Long X-T, Zhang H-J, Fu L-D, Huang J-Y, Chaurembo AI, Chanda F, Xu Y-J, Shu C, Lin K-X, Yang K and Lin H-B (2022) Research progress on effects of traditional Chinese medicine on myocardial ischemia–reperfusion injury: A review. Front. Pharmacol. 13:1055248. doi: 10.3389/fphar.2022.1055248
Received: 27 September 2022; Accepted: 16 November 2022;
Published: 06 December 2022.
Edited by:
Mohammad S. Mubarak, The University of Jordan, JordanReviewed by:
Wen Wang, Xuanwu Hospital, Capital Medical University, ChinaShouzhu Xu, Shaanxi University of Chinese Medicine, China
Copyright © 2022 Xing, Long, Zhang, Fu, Huang, Chaurembo, Chanda, Xu, Shu, Lin, Yang and Lin. This is an open-access article distributed under the terms of the Creative Commons Attribution License (CC BY). The use, distribution or reproduction in other forums is permitted, provided the original author(s) and the copyright owner(s) are credited and that the original publication in this journal is cited, in accordance with accepted academic practice. No use, distribution or reproduction is permitted which does not comply with these terms.
*Correspondence: Ke Yang, yangyongyao168@sina.com; Han-Bin Lin, linhanbin@simm.ac.cn
†These authors have contributed equally to this work and share first authorship