- Center for Immunity, Inflammation and Regenerative Medicine (CIIR), Division of Nephrology, Department of Medicine, University of Virginia, Charlottesville, VA, United States
Lupus glomerulonephritis (LN) is a complex autoimmune disease characterized by circulating autoantibodies, immune-complex deposition, immune dysregulation and defects in regulatory T cell (Tregs). Treatment options rely on general immunosuppressants and steroids that have serious side effects. Approaches to target immune cells, such as B cells in particular, has had limited success and new approaches are being investigated. Defects in Tregs in the setting of autoimmunity is well known and Treg-replacement strategies are currently being explored. The aim of this minireview is to rekindle interest on Treg-targeting strategies. We discuss the existing evidences for Treg-enhancement strategies using key cytokines interleukin (IL)-2, IL-33 and IL-6 that have shown to provide remission in LN. We also discuss strategies for indirect Treg-modulation for protection from LN.
Introduction
Systemic Lupus Erythematosus (SLE) is a debilitating autoimmune disease characterized by inflammation, increased circulating autoantibodies (autoAb), immune complex (IC) deposition, and multi-organ dysfunction affecting skin, joints, kidneys, brain etc. The cause of SLE is still unclear but genetic, environmental and hormonal factors have been linked to its predisposition. The prevalence of SLE is 366.6/100,000 in USA (2016 estimate) and 97/100,000 in UK (2012 estimate) (Barber et al., 2021). Lupus Foundation of America estimates that at least five million people worldwide may have some form of lupus (https://www.lupus.org/resources/lupus-facts-and-statistics). A majority of the patients affected by lupus progress to lupus nephritis (LN), an end stage renal disease marked by kidney IC deposition and glomerulosclerosis (reviewed in Ward, 2010). There are no approved medications that can cure the disease or provide long term remission with maintenance immunosuppression being the current option. There is thus an urgent need for novel treatment options for LN. Strategies for enhancing regulatory T cells (Treg) have garnered attention recently. While, many studies have employed Tregs for SLE, not enough work has specifically focused on lupus nephritis (LN). Therefore, we also included studies on Treg-based approaches for SLE.
Autoimmunity and Tregs
Tregs constitute an important immune cell subset that prevents abnormal activation of the immune system and provide tolerance in allergy and autoimmunity (reviewed in Sharma and Kinsey, 2018). The role of Treg-deficiency in the onset and progression of autoimmunity has long been appreciated. In humans and mice, Tregs are identified as T-cells expressing high levels of IL-2 receptor alpha (CD25) and the transcription factor forkhead box P3 (Foxp3). Alongside IL-2 and CD25, which facilitate the development, function and stability of Tregs, numerous other cell-surface receptors for Tregs have been identified (reviewed in Sharma and Kinsey, 2018). IL-2 supports Treg development in the thymus (Vang et al., 2008) and is also required for their survival and function in the periphery (Fontenot et al., 2005; Barron et al., 2010; Sharma and Kinsey, 2018). While mechanisms of Treg-intrinsic function are still being recognized, factors including excessive production of pro-inflammatory cytokines and resistance to Treg-mediated suppression are being found to contribute to autoimmunity. A strong association of Treg defect in SLE and other human autoimmune diseases is now well-appreciated. Tregs not only control innate and adaptive immunity, but also regulate cellular damage and promote repair (reviewed in Shevach, 2018; Sharma, 2020). Thus, Treg-enhancement strategies remain an attractive strategy for remission from LN (Figure 1).
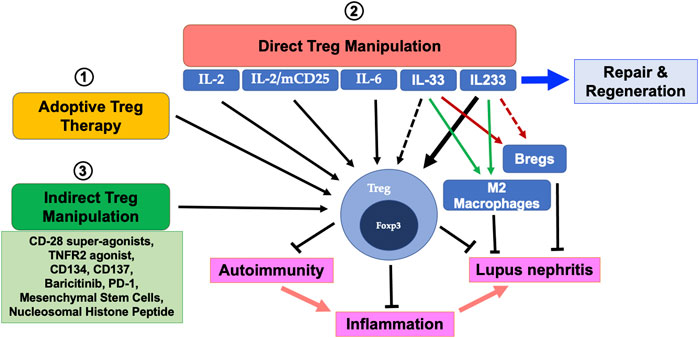
FIGURE 1. Treg supplementation therapies for autoimmunity and lupus nephritis (LN): 1) Adoptive Treg transfer therapy has been successfully employed to attenuate inflammation and render protection in acute injury settings and recently, a similar approach was successfully employed in a SLE patient with beneficial outcomes. 2) Cytokines IL-2, IL-2/mCD25, IL-6 and IL233 have been shown to directly cause robust expansion of Tregs which prevents autoimmunity and LN. Whether IL-33, which is reported to exhibit its protective effects on LN through modulation of M2 macrophages and Bregs have a direct effect on Tregs is yet to be elucidated. Similarly, IL233 has been shown to expand Tregs and induce a M2 phenotype but its effects on Bregs are currently unknown. 3) Non-cytokine-based approaches using agonists, small molecules, mesenchymal stem cells and nucleosomal histone peptides have been shown to support Treg expansion to target LN.
Immune-Dysregulation in Lupus Nephritis
T cell activation is one of the major pathogenic mechanisms in the immune-dysregulation observed in lupus patients (reviewed in Mountz et al., 2019). Lupus patients have an elevated number of T effector cells, indicating a continued pathogenic response triggered either by pathogens that may mimic self-antigen (Zhao et al., 2019a; Múnera et al., 2020; Munroe et al., 2020), uncleared self-antigen from dying cells (reviewed in Muñoz et al., 2010; Prechl et al., 2016), or altered cytokine milieu contributing to effector cell bias (reviewed in Bengtsson and Rönnblom, 2017; Paquissi and Abensur, 2021). The role of complement proteins in LN is quite complex, where tissue damage is induced by complement deposition, and hypocomplementemia of C3 and C4 is pronounced in active disease, suggesting that complement-mediated clearance of autoantigen and IC play a central role in pathogenesis (reviewed in Li et al., 2021). The effector T-cells on one hand can induce cell-mediated organ dysfunction directly, they also activate innate-immune cells including macrophages and dendritic cells (DC) for fueling the immune dysregulation, as well as differentiate in to T-follicular helper (TfH) cells to help auto-reactive B-cells to produce high-affinity autoAb (reviewed in Mountz et al., 2019; Chen and Tsokos, 2021). The role of cytokines in lupus is supported by reports of elevated Tumor necrosis factor (TNF)-α and Interferon (IFN)-γ in SLE patients (Harigai et al., 2008). Other proinflammatory mediators including IL-6 and IL-17 lead to TH17/Treg dysregulation in lupus patients compared to healthy individuals (Tang et al., 2019).
IL-2: A Master Regulator of Tregs in Lupus Nephritis
IL-2 was initially discovered as a growth factor that promoted T-cell proliferation in vitro (Morgan et al., 1976), but was later realized to be more critical for Treg-maintenance (Fontenot et al., 2005) and T-cell regulation (reviewed in Chen and Tsokos, 2021). IL-2 deficiency may contribute to autoimmunity in SLE patients and lupus-prone mice by inducing paucity of Tregs, defective activation-induced cell death (AICD) and increased IL-17 production (Lippe et al., 2012). Two back-to-back reports first showed the induction of IL-17 producing TH17 cells by TGFβ and IL-6 (Bettelli et al., 2006; Mangan et al., 2006). Subsequently, Laurence et al. showed that IL-2 through STAT5 directly inhibits the STAT3-mediated TH17 differentiation, because blocking of IL-2 or deletion of STAT5 in CD4 T-cells enhanced RORγT, a key transcription factor for TH17 differentiation (Yang et al., 2011). The role of IL-2/STAT5 in TH2 responses as well as inhibition of TH1 was first showed by W. E. Paul and colleagues (reviewed in Zhu et al., 2006). Accordingly, in the setting of multi-organ inflammatory disease in the Foxp3-mutant scurfy mice, it was shown that deletion of IL-4 or STAT6 (which is downstream to IL-4/IL-13 signaling) enhanced TH1 responses, thus showing the protective effects of TH2 in autoimmune inflammation in several organs (Sharma et al., 2011; Ju et al., 2012). Recently, Hsiung et al. (2020) showed that inflammation leads to lower levels of IL-2R signaling in Tregs, which further lowers their suppressive function. They concluded that low dose IL-2 therapy could possibly overcome the negative effects of inflammation on Tregs. Several investigators have tried to utilize the ability of IL-2 to expand Tregs for protection in autoimmune diseases (Fontenot et al., 2005; Bayer et al., 2007; Grinberg-Bleyer et al., 2010). However, in a study using the NZB/W mice, long-term treatment with a low dose of 1,500 units/day IL-2 or a high dose of 15,000 units/day did not alter the active lupus-like disease or splenocyte populations (Owen et al., 1989). In an interesting approach, a fusion protein of IL-2 with IL-2Ra (CD25) joined by a linker and termed mIL-2/CD25 inhibited LN in female NZB x NZW and male MRL/lpr mice (Xie et al., 2021). The treatment induced Treg expansion without elevation of NK, CD4+/CD8+ T cells and proinflammatory cytokines.
In clinical studies treatment of SLE patients with low dose IL-2 increased Tregs, decreased AICD and restored CTL responses along with a reduction of serum anti-dsDNA antibodies (von Spee-Mayer et al., 2016; He et al., 2016). Another clinical study in refractory SLE patients reported that the SLEDAI scores (a disease activity index for lupus patients) were significantly lowered with low dose IL-2 and rapamycin treatment and normalization of TH17/Treg ratios were significantly reduced (Zhao et al., 2019b). An interesting study focusing on the safety and efficacy of low dose IL-2 in 11 autoimmune disease in 46 patients including SLE found significant Treg-expansion without effector T-cell activation (Rosenzwajg et al., 2019). While low dose IL-2 has shown promise for SLE patients, several study results are still pending. Patients often receive multiple injections due to the short half-life of IL-2 and there is a concern of side effects triggered by low dose IL-2 mediated activation of cytotoxic lymphocytes and NK cells.
Low Dose IL-2 Therapy for Other Autoimmune Diseases
IL-2 therapy has also been successfully tested in other lesser studied autoimmune diseases. A Phase I/II clinical trial on low dose IL-2 therapy in HCV-induced vasculitis increased Treg levels and reduced vasculitis (Saadoun et al., 2011). In a study on Alopecia areata (Castela et al., 2014), low dose IL-2 achieved local recruitment of Treg and also caused regrowth of scalp hair in the majority of participants. This study emphasized the importance of Treg supplementation in conditions involving skin lesions. In a study focusing on autoimmune hepatitis, low dose IL-2 normalized liver enzyme levels (Lim et al., 2018). The TRANSREG clinical trial that comprised of patients with 11 autoimmune diseases also showed promising findings with low dose IL-2 administration (Rosenzwajg et al., 2019). Other reports in primary Sjogren’s syndrome (Miao et al., 2018), Polymyositis/Dermatomyositis (Zhang et al., 2021), Psoriatic arthritis (Wang et al., 2020) and Amyotrophic lateral sclerosis (Camu et al., 2020) also showed benefits of low dose IL-2 therapy.
IL-33: An Alarmin Cytokine That Regulates Innate Immunity and Inflammation
Interleukin-33 was identified as a nuclear cytokine belonging to the IL-1 family that is known to induce proinflammatory cytokines and expression of integrins on leukocytes and endothelial cells (Dinarello, 2009; Spooner et al., 2013). The pathway for IL-33 and its receptor ST2 is actively being pursued for immune modulation during autoimmune and inflammatory diseases (Monticelli et al., 2011; Byers et al., 2013). Two forms of ST2: transmembrane (mST2) and soluble isoforms (sST2) have been described (Mueller and Jaffe, 2015). The sST2 has been identified as a biomarker for cardiac, pulmonary and graft-versus-host diseases (Bajwa et al., 2015; Bayes-Genis et al., 2015; Xiao and Zheng, 2016). Recent studies have demonstrated a protective role of IL-33/ST2 in several inflammatory settings (Miller et al., 2008; Mato et al., 2009; Milovanovic et al., 2012). In the hTNF.Tg model of inflammation, IL-33-treatment induced alternatively activated macrophages (AAM) and inhibited TNFα-induced bone-loss (Zaiss et al., 2011). Interestingly, IVIG treatment was shown to suppress autoimmunity through IL-33 induced TH2 responses (Anthony et al., 2011), suggesting that IL-33/ST2 pathway may activate anti-inflammatory mechanisms in other settings. Prior studies on IL-33 have established that recombinant IL-33, both in vitro and in vivo, expanded ST2+ Tregs. In vitro, these ST2+ Tregs were more efficient in suppressing IL-12/IL-33 driven CD8+ T cell IFNγ production than their ST2- counterpart (Matta et al., 2014). Early IL-33 administration in lupus prone NZB/W F1 mice was shown to induce regulatory B cells and reduce autoantibody levels. Additionally, an M2 macrophage signature was also induced implying a regulatory role of IL-33 in lupus onset (Mohd Jaya et al., 2020). Contrastingly, in a previously published study, antibody-mediated IL-33 neutralization suppressed disease in lupus prone mice along with an increase in Tregs and reduced IL-17 levels (Li et al., 2014). There is thus a disconnect in the available literature on the effects of IL-33 and its role in LN warrants further investigation.
IL-6: A Paradoxical Target for Treg Expansion
IL-6 was originally identified as a B-cell differentiation factor that affects the autoantibody and cytokine production. IL-6 plays a key role in defense to infections by regulating the proinflammatory and regulatory T cells as well as contribute to autoimmunity. Elevated serum IL-6 levels correlate with disease severity in SLE patients (Chun et al., 2007). Pathological role of IL-6 has been demonstrated in disease development via targeting IL-6 with anti-IL-6 antibodies or by gene knockout approaches by several investigators. Indeed, IL-6 suppression was shown to rescue from rheumatoid arthritis (Narazaki et al., 2017), SLE (Tackey et al., 2004), scleroderma (O’Reilly et al., 2013) and many other diseases. Both TH17 and Tregs require TGFβ for their differentiation through induction of both RORγt and Foxp3 expression, where IL-6 negatively regulates Tregs by promoting TH17 (Zhou et al., 2008). The regulation of TH17/Treg differentiation by IL-6 explains the involvement of this pro-inflammatory cytokine in diseases that exhibit a prominent TH17 signature as a causative factor. While underlying mechanism for the observed efficacy of IL-6 blockade therapies are yet to be uncovered, the paradigm that IL-6 is associated with disease initiation and progression stands challenged by recent observations from Steinmetz and colleagues for a protective role of IL-6 in LN (Hagenstein et al., 2019). In 2016, Tregs dually expressing the transcription factors Foxp3 and RORγt were identified and were given the term “biTregs” for their roles in nephrotoxic nephritis (NTN) model of LN (Kluger et al., 2016). It was found that RORγt+Foxp3+ biTregs expanded with LN in the spleens and kidneys. The expansion of this subset of cells were higher than the expansion of conventional Tregs (cTregs: Foxp3+ Tregs) and IL-17+ Tregs. Further characterization also confirmed that the biTregs did not differentiate into cTregs or Th17 cells and that the IL-17 production by these cells was dependent on RORγt. It was concluded that the biTregs are a novel bifunctional Treg lineage with distinct properties and maybe a novel therapeutic target for LN. The observation that IL-6 did not inhibit Treg development or activation in the disease setting, but rather enhanced T effector activation that caused loss of Treg-mediated suppression led Steinmetz group to focus on the biTregs as an active effector Treg lineage (Nish et al., 2014; Ohnmacht et al., 2015; Sefik et al., 2015; Kluger et al., 2016; Yang et al., 2016). Utilizing IL-6 knockout mice, Hagenstein and colleagues (Hagenstein et al., 2019) further showed that IL-6 is required for the anti-inflammatory function of Tregs, as IL-6 treatment resulted in a significant expansion of RORγt+ biTregs and correlating with protection against LN.
IL233: A Novel Hybrid Cytokine for LN Remission
IL-2 defect parallels with progression of autoimmunity and LN, and also correlates with reduced levels of Tregs. We found that IL-2 also regulates the expression of IL1RL1 (ST2; IL-33 receptor) on CD4 T-cells (Sharma et al., 2011). In addition, it was found that a major subset of Tregs expresses ST2 and that IL-33 expands Tregs in vitro and in vivo. Importantly, a novel cooperation between IL-2 and IL-33 was identified, which protected against inflammatory diseases by expanding, activating and mobilizing Tregs more efficiently than either cytokine alone. For more efficient restriction of this cooperativity to Tregs, a fusion cytokine of IL-2 and IL-33 (termed IL233) was generated (Stremska et al., 2017). IL233 exerted a sustained increase in Tregs, specifically in the renal lymph nodes and protected mice from LN more efficiently than IL-2 and IL-33 injected either alone or together. IL233 treatment not only prevented onset of LN, but also induced lifelong remission in moderate to severely proteinuric NZM2328 mice without any detectable side effects. IL233 treatment also inhibited the progression of LN in proteinuric MRL/lpr mice and protected them from early mortality (Stremska et al., 2019). The mechanisms, in addition to increasing Tregs, suggested restoring of IL-2 production by T-cells and tolerance induction via reduced expression of CD80 and CD86 on macrophages and DC. Interestingly, autoAb production and IC deposition were not significantly inhibited, suggesting a disconnect between autoAb and end-organ failure (Clynes et al., 1998; Ge et al., 2013). Thus, IL233 treatment demonstrated therapeutic potential in IFNα-accelerated and spontaneous LN in NZM2328 as well as MRL/lpr mice, indicating its general applicability.
Alternate Strategies for Treg Expansion
A number of alternate strategies are reported for in vivo Treg activation and expansion. These included the use of Treg-related cell surface proteins, signaling and epigenetic modulation by small molecules, as well as approaches using auto-antigen peptides and microbes. CD28 is a co-stimulatory molecule expressed on T-cells and engaged by CD80/CD86 on antigen presenting cells for activation and cell cycle. In a rat model of anti-Thy1 nephritis, it was observed that CD28 super-agonists (CD28SA) at low levels efficiently expanded Tregs, and decreased proteinuria and serum creatinine levels (Miyasato et al., 2011). The roles of TNFRs (Tumor Necrosis Factor Receptors) are being recognized and TNFR2 is highly expressed in Tregs. TNF-α is a pleiotropic cytokine, which exists in both membrane-bound and soluble forms. The membrane bound TNF-α preferentially interacts with TNFR2 and results in suppressive function due to lack of cytoplasmic death domain. In a model of chronic inflammation, use of TNFR2 specific agonist TNCscTNF80 resulted in Treg expansion and reduced inflammation in mouse model of rheumatoid arthritis (Schmid et al., 2017). Similar to TNRF2, Ox40 (CD134) is expressed on Tregs and effector T cells. Ox-40 expression was elevated in several T-cell subsets in proteinuric NZB/W F1 mice. While, treatment with antagonistic Ox40 mAb accelerated autoAb and LN, administration of an agonistic Ox40:Fc fusion protein in an IFNα-accelerated lupus model significantly delayed the onset of severe proteinuria and improved the survival, suggesting a benefit of targeting this pathway (Sitrin et al., 2017). However, the effect of Ox40:Fc on Tregs was not evaluated, despite earlier observations that Ox40-deficient mice had reduced Tregs and that Ox40L overexpression increased Tregs in the spleen without disease suppression, probably due to evasion of Treg-mediated suppression by Ox40 engagement on effector T-cells (Takeda et al., 2004), Analogous to CD134, CD137 is highly expressed on Tregs and a study by Sun et al., showed that treatment with agonistic monoclonal antibody to CD137 blocked spontaneous autoimmunity in MRL/lpr mice (Sun et al., 2002), likely by promoting Treg expansion (Zhang et al., 2007).
Baricitnib, a selective inhibitor of Janus kinase (JAK1) and JAK2 is approved for use in rheumatoid arthritis. In a recent study, Lee et al. observed that treatment with baricitnib could effectively attenuate lupus-like phenotype in MRL/lpr mice. This was accompanied by reduction of total, CD8+ and abnormal double-negative T-cells along-with a greater proportion of follicular (CXCR5+) and extra-follicular (CXCR5−) Tregs, which could have afforded protection (Lee et al., 2021). Checkpoint inhibitors are currently being tested for treatment of several forms of cancer and their effects on Tregs remains controversial. Interestingly, in NZB/W F1 mice, anti-PD1 antibody treatment reduced CD4+PD-1+ T cells, promoted suppressive capability of Tregs and ameliorated LN (Wong et al., 2013). Mesenchymal stem cells (MSCs) are multipotent progenitor cells exerting immunosuppressive capacity with respect to both innate and adaptive immune response. MSCs are used in clinical settings to treat various lupus like conditions for over a decade (Zhou et al., 2020). Gazdic et al. (2018) demonstrated that indoleamine 2,3-dioxygenase (IDO) secreted from MSCs stimulates expansion and activation of Tregs to produce IL-10. Whether such effects can have therapeutic efficacy in LN setting is yet to be explored.
Several autoantigen peptide-based approaches are being developed to deliver these molecules to autoreactive T cells to promote clonal deletion or to develop immunoregulation. Kang et al. (2005) showed that a very low-dose of nucleosomal histone peptide efficiently controlled lupus in SNF1 lupus prone mice and induced Treg-subsets. The role of microbiome and probiotics in immune-regulation of SLE is also being actively researched. It was found that certain Clostridium strains reduced TH17 cells and induced Tregs. Further, Bifidobacterium bifidum supplementation prevented CD4 T-cell overactivation to restore Treg/Th17/Th1 imbalance in SLE via expanding Foxp3+IL-17+ populations, however, its effect on LN is still pending (López et al., 2016).
Conclusions
As of today, there are 173 clinical trials ongoing for therapy of LN. The field of lupus research, having seen some promising therapeutics is now fraught with termination of ongoing trials due to unwarranted effects. There still is no approved drug that can be safely used long term to treat patients with LN. Tregs can be targeted effectively for LN remission, however, there are limited studies focusing on Treg supplementation and its probable adverse effects, if any. With the identification of key cytokines and alternative strategies to regulate Treg function, expansion and activation, it is imperative to continue research efforts on Treg-based strategies for remission from LN.
Author Contributions
RS and RV conceived the idea. RS, RV, VS and MD wrote the manuscript.
Funding
This study was supported by NIH/NIDDK awards R01DK104963 (RS) and R01DK105833 (RS and Shu Man Fu) and JDRF award 3-SRA-2021-1005-S-B (RS).
Conflict of Interest
RS is an inventor for US patents 6897041 and 9840545 licensed to Slate Bio Inc., holds equity and is a consultant for Slate Bio Inc.
The remaining authors declare that the research was conducted in the absence of any commercial or financial relationships that could be construed as a potential conflict of interest.
Publisher’s Note
All claims expressed in this article are solely those of the authors and do not necessarily represent those of their affiliated organizations, or those of the publisher, the editors and the reviewers. Any product that may be evaluated in this article, or claim that may be made by its manufacturer, is not guaranteed or endorsed by the publisher.
References
Anthony, R. M., Kobayashi, T., Wermeling, F., and Ravetch, J. V. (2011). Intravenous Gammaglobulin Suppresses Inflammation Through a Novel T(H)2 Pathway. Nature 475, 110–113. doi:10.1038/nature10134
Bajwa, E. K., Mebazaa, A., and Januzzi, J. L. (2015). ST2 in Pulmonary Disease. Am. J. Cardiol. 115, 44B–7B. doi:10.1016/j.amjcard.2015.01.040
Barber, M. R. W., Drenkard, C., Falasinnu, T., Hoi, A., Mak, A., Kow, N. Y., et al. (2021). Global Epidemiology of Systemic Lupus Erythematosus. Nat. Rev. Rheumatol. 17, 515–532. doi:10.1038/s41584-021-00668-1
Barron, L., Dooms, H., Hoyer, K. K., Kuswanto, W., Hofmann, J., O’Gorman, W. E., et al. (2010). Cutting Edge: Mechanisms of IL-2-Dependent Maintenance of Functional Regulatory T Cells. J. Immunol. 185, 6426–6430. doi:10.4049/jimmunol.0903940
Bayer, A. L., Yu, A., and Malek, T. R. (2007). Function of the IL-2R for Thymic and Peripheral CD4+CD25+ Foxp3+ T Regulatory Cells. J. Immunol. 178, 4062–4071. doi:10.4049/jimmunol.178.7.4062
Bayes-Genis, A., Zhang, Y., and Ky, B. (2015). ST2 and Patient Prognosis in Chronic Heart Failure. Am. J. Cardiol. 115, 64B–9B. doi:10.1016/j.amjcard.2015.01.043
Bengtsson, A. A., and Rönnblom, L. (2017). Role of Interferons in SLE. Best Pract. Res. Clin. Rheumatol. 31, 415–428. doi:10.1016/j.berh.2017.10.003
Bettelli, E., Carrier, Y., Gao, W., Korn, T., Strom, T. B., Oukka, M., et al. (2006). Reciprocal Developmental Pathways for the Generation of Pathogenic Effector TH17 and Regulatory T Cells. Nature 441, 235–238. doi:10.1038/nature04753
Byers, D. E., Alexander-Brett, J., Patel, A. C., Agapov, E., Dang-Vu, G., Jin, X., et al. (2013). Long-Term IL-33-Producing Epithelial Progenitor Cells in Chronic Obstructive Lung Disease. J. Clin. Invest. 123, 3967–3982. doi:10.1172/JCI65570
Camu, W., Mickunas, M., Veyrune, J. L., Payan, C., Garlanda, C., Locati, M., et al. (2020). Repeated 5-day Cycles of Low Dose Aldesleukin in Amyotrophic Lateral Sclerosis (IMODALS): A Phase 2a Randomised, Double-Blind, Placebo-Controlled Trial. EBioMedicine 59, 102844. doi:10.1016/j.ebiom.2020.102844
Castela, E., Le Duff, F., Butori, C., Ticchioni, M., Hofman, P., Bahadoran, P., et al. (2014). Effects of Low-Dose Recombinant Interleukin 2 to Promote T-Regulatory Cells in Alopecia Areata. JAMA Dermatol. 150, 748–751. doi:10.1001/jamadermatol.2014.504
Chen, P.-M., and Tsokos, G. C. (2021). T Cell Abnormalities in the Pathogenesis of Systemic Lupus Erythematosus: An Update. Curr. Rheumatol. Rep. 23, 12. doi:10.1007/s11926-020-00978-5
Chun, H. Y., Chung, J. W., Kim, H. A., Yun, J. M., Jeon, J. Y., Ye, Y. M., et al. (2007). Cytokine IL-6 and IL-10 as Biomarkers in Systemic Lupus Erythematosus. J. Clin. Immunol. 27, 461–466. doi:10.1007/s10875-007-9104-0
Clynes, R., Dumitru, C., and Ravetch, J. V. (1998). Uncoupling of Immune Complex Formation and Kidney Damage in Autoimmune Glomerulonephritis. Science 279, 1052–1054. doi:10.1126/science.279.5353.1052
Dinarello, C. A. (2009). Immunological and Inflammatory Functions of the Interleukin-1 Family. Annu. Rev. Immunol. 27, 519–550. doi:10.1146/annurev.immunol.021908.132612
Fontenot, J. D., Rasmussen, J. P., Gavin, M. A., and Rudensky, A. Y. (2005). A Function for Interleukin 2 in Foxp3-Expressing Regulatory T Cells. Nat. Immunol. 6, 1142–1151. doi:10.1038/ni1263
Gazdic, M., Markovic, B. S., Arsenijevic, A., Jovicic, N., Acovic, A., Harrell, C. R., et al. (2018). Crosstalk Between Mesenchymal Stem Cells and T Regulatory Cells Is Crucially Important for the Attenuation of Acute Liver Injury. Liver Transpl. 24, 687–702. doi:10.1002/lt.25049
Ge, Y., Jiang, C., Sung, S. S., Bagavant, H., Dai, C., Wang, H., et al. (2013). Cgnz1 Allele Confers Kidney Resistance to Damage Preventing Progression of Immune Complex-Mediated Acute Lupus Glomerulonephritis. J. Exp. Med. 210, 2387–2401. doi:10.1084/jem.20130731
Grinberg-Bleyer, Y., Baeyens, A., You, S., Elhage, R., Fourcade, G., Gregoire, S., et al. (2010). IL-2 Reverses Established Type 1 Diabetes in NOD Mice by a Local Effect on Pancreatic Regulatory T Cells. J. Exp. Med. 207, 1871–1878. doi:10.1084/jem.20100209
Hagenstein, J., Melderis, S., Nosko, A., Warkotsch, M. T., Richter, J. V., Ramcke, T., et al. (2019). A Novel Role for IL-6 Receptor Classic Signaling: Induction of RORγt+Foxp3+ Tregs with Enhanced Suppressive Capacity. J. Am. Soc. Nephrol. 30, 1439–1453. doi:10.1681/ASN.2019020118
Harigai, M., Kawamoto, M., Hara, M., Kubota, T., Kamatani, N., and Miyasaka, N. (2008). Excessive Production of IFN-Gamma in Patients with Systemic Lupus Erythematosus and its Contribution to Induction of B Lymphocyte Stimulator/B Cell-Activating Factor/TNF Ligand superfamily-13B. J. Immunol. 181, 2211–2219. doi:10.4049/jimmunol.181.3.2211
He, J., Zhang, X., Wei, Y., Sun, X., Chen, Y., Deng, J., et al. (2016). Low-Dose Interleukin-2 Treatment Selectively Modulates CD4(+) T Cell Subsets in Patients with Systemic Lupus Erythematosus. Nat. Med. 22, 991–993. doi:10.1038/nm.4148
Hsiung, S., Moro, A., Ban, Y., Chen, X., Savio, A. S., Hernandez, R., et al. (2020). Acute Lipopolysaccharide-Induced Inflammation Lowers IL-2R Signaling and the Proliferative Potential of Regulatory T Cells. Immunohorizons 4, 809–824. doi:10.4049/immunohorizons.2000099
Ju, S. T., Sharma, R., Gaskin, F., and Fu, S. M. (2012). IL-2 Controls Trafficking Receptor Gene Expression and Th2 Response for Skin and Lung Inflammation. Clin. Immunol. 145, 82–88. doi:10.1016/j.clim.2012.07.015
Kang, H. K., Michaels, M. A., Berner, B. R., and Datta, S. K. (2005). Very Low-Dose Tolerance with Nucleosomal Peptides Controls Lupus and Induces Potent Regulatory T Cell Subsets. J. Immunol. 174, 3247–3255. doi:10.4049/jimmunol.174.6.3247
Kluger, M. A., Meyer, M. C., Nosko, A., Goerke, B., Luig, M., Wegscheid, C., et al. (2016). RORγt(+)Foxp3(+) Cells Are an Independent Bifunctional Regulatory T Cell Lineage and Mediate Crescentic GN. J. Am. Soc. Nephrol. 27, 454–465. doi:10.1681/ASN.2014090880
Lee, J., Park, Y., Jang, S. G., Hong, S. M., Song, Y. S., Kim, M. J., et al. (2021). Baricitinib Attenuates Autoimmune Phenotype and Podocyte Injury in a Murine Model of Systemic Lupus Erythematosus. Front. Immunol. 12, 704526. doi:10.3389/fimmu.2021.704526
Li, N. L., Birmingham, D. J., and Rovin, B. H. (2021). Expanding the Role of Complement Therapies: The Case for Lupus Nephritis. J. Clin. Med. 10, 626. doi:10.3390/jcm10040626
Li, P., Lin, W., and Zheng, X. (2014). IL-33 Neutralization Suppresses Lupus Disease in Lupus-Prone Mice. Inflammation 37, 824–832. doi:10.1007/s10753-013-9802-0
Lim, T. Y., Martinez-Llordella, M., Kodela, E., Gray, E., Heneghan, M. A., and Sanchez-Fueyo, A. (2018). Low-Dose Interleukin-2 for Refractory Autoimmune Hepatitis. Hepatology 68, 1649–1652. doi:10.1002/hep.30059
Lippe, R., Ohl, K., Varga, G., Rauen, T., Crispin, J. C., Juang, Y. T., et al. (2012). CREMα Overexpression Decreases IL-2 Production, Induces a T(H)17 Phenotype and Accelerates Autoimmunity. J. Mol. Cell Biol. 4, 121–123. doi:10.1093/jmcb/mjs004
López, P., de Paz, B., Rodríguez-Carrio, J., Hevia, A., Sánchez, B., Margolles, A., et al. (2016). Th17 Responses and Natural IgM Antibodies Are Related to Gut Microbiota Composition in Systemic Lupus Erythematosus Patients. Sci. Rep. 6, 24072. doi:10.1038/srep24072
Mangan, P. R., Harrington, L. E., O'Quinn, D. B., Helms, W. S., Bullard, D. C., Elson, C. O., et al. (2006). Transforming Growth Factor-Beta Induces Development of the T(H)17 Lineage. Nature 441, 231–234. doi:10.1038/nature04754
Mato, N., Fujii, M., Hakamata, Y., Kobayashi, E., Sato, A., Hayakawa, M., et al. (2009). Interleukin-1 Receptor-Related Protein ST2 Suppresses the Initial Stage of Bleomycin-Induced Lung Injury. Eur. Respir. J. 33, 1415–1428. doi:10.1183/09031936.00084307
Matta, B. M., Lott, J. M., Mathews, L. R., Liu, Q., Rosborough, B. R., Blazar, B. R., et al. (2014). IL-33 is an Unconventional Alarmin that Stimulates IL-2 Secretion by Dendritic Cells to Selectively Expand IL-33R/ST2+ Regulatory T Cells. J. Immunol. 193, 4010–4020. doi:10.4049/jimmunol.1400481
Miao, M., Hao, Z., Guo, Y., Zhang, X., Zhang, S., Luo, J., et al. (2018). Short-Term and Low-Dose IL-2 Therapy Restores the Th17/Treg Balance in the Peripheral Blood of Patients with Primary Sjögren's Syndrome. Ann. Rheum. Dis. 77, 1838–1840. doi:10.1136/annrheumdis-2018-213036
Miller, A. M., Xu, D., Asquith, D. L., Denby, L., Li, Y., Sattar, N., et al. (2008). IL-33 Reduces the Development of Atherosclerosis. J. Exp. Med. 205, 339–346. doi:10.1084/jem.20071868
Milovanovic, M., Volarevic, V., Radosavljevic, G., Jovanovic, I., Pejnovic, N., Arsenijevic, N., et al. (2012). IL-33/ST2 Axis in Inflammation and Immunopathology. Immunol. Res. 52, 89–99. doi:10.1007/s12026-012-8283-9
Miyasato, K., Takabatake, Y., Kaimori, J., Kimura, T., Kitamura, H., Kawachi, H., et al. (2011). CD28 Superagonist-Induced Regulatory T Cell Expansion Ameliorates Mesangioproliferative Glomerulonephritis in Rats. Clin. Exp. Nephrol. 15, 50–57. doi:10.1007/s10157-010-0370-4
Mohd Jaya, F. N., Liu, Z., and Chan, G. C. (2020). Early Treatment of Interleukin-33 Can Attenuate Lupus Development in Young NZB/W F1 Mice. Cells 9, 2448. doi:10.3390/cells9112448
Monticelli, L. A., Sonnenberg, G. F., Abt, M. C., Alenghat, T., Ziegler, C. G., Doering, T. A., et al. (2011). Innate Lymphoid Cells Promote Lung-Tissue Homeostasis after Infection with Influenza Virus. Nat. Immunol. 12, 1045–1054. doi:10.1031/ni.2131
Morgan, D. A., Ruscetti, F. W., and Gallo, R. (1976). Selective In Vitro Growth of T Lymphocytes from Normal Human Bone Marrows. Science 193, 1007–1008. doi:10.1126/science.181845
Mountz, J. D., Hsu, H. C., and Ballesteros-Tato, A. (2019). Dysregulation of T Follicular Helper Cells in Lupus. J. Immunol. 202, 1649–1658. doi:10.4049/jimmunol.1801150
Mueller, T., and Jaffe, A. S. (2015). Soluble ST2-Aanalytical Considerations. Am. J. Cardiol. 115, 8B–21B. doi:10.1016/j.amjcard.2015.01.035
Múnera, M., Farak, J., Pérez, M., Rojas, J., Villero, J., Sánchez, A., et al. (2020). Prediction of Molecular Mimicry between Antigens from Leishmania Sp. And Human: Implications for Autoimmune Response in Systemic Lupus Erythematosus. Microb. Pathog. 148, 104444. doi:10.1016/j.micpath.2020.104444
Muñoz, L. E., Lauber, K., Schiller, M., Manfredi, A. A., and Herrmann, M. (2010). The Role of Defective Clearance of Apoptotic Cells in Systemic Autoimmunity. Nat. Rev. Rheumatol. 6, 280–289. doi:10.1038/nrrheum.2010.46
Munroe, M. E., Anderson, J. R., Gross, T. F., Stunz, L. L., Bishop, G. A., and James, J. A. (2020). Epstein-Barr Functional Mimicry: Pathogenicity of Oncogenic Latent Membrane Protein-1 in Systemic Lupus Erythematosus and Autoimmunity. Front. Immunol. 11, 606936. doi:10.3389/fimmu.2020.606936
Narazaki, M., Tanaka, T., and Kishimoto, T. (2017). The Role and Therapeutic Targeting of IL-6 in Rheumatoid Arthritis. Expert Rev. Clin. Immunol. 13, 535–551. doi:10.1080/1744666X.2017.1295850
Nish, S. A., Schenten, D., Wunderlich, F. T., Pope, S. D., Gao, Y., Hoshi, N., et al. (2014). T Cell-Intrinsic Role of IL-6 Signaling in Primary and Memory Responses. Elife 3, e01949. doi:10.7554/eLife.01949
O’Reilly, S., Cant, R., Ciechomska, M., and van Laar, J. M. (2013). Interleukin-6: A New Therapeutic Target in Systemic Sclerosis? Clin. Transl. Immunol. 2, e4. doi:10.1038/cti.2013.2
Ohnmacht, C., Park, J. H., Cording, S., Wing, J. B., Atarashi, K., Obata, Y., et al. (2015). Mucosal IMMUNOLOGY. The Microbiota Regulates Type 2 Immunity Through RORγt⁺ T Cells. Science 349, 989–993. doi:10.1126/science.aac4263
Owen, K. L., Shibata, T., Izui, S., and Walker, S. E. (1989). Recombinant Interleukin-2 Therapy of Systemic Lupus Erythematosus in the New Zealand Black/New Zealand White Mouse. J. Biol. Response Mod. 8, 366–374.
Paquissi, F. C., and Abensur, H. (2021). The Th17/IL-17 Axis and Kidney Diseases, With Focus on Lupus Nephritis. Front. Med. 8, 654912. doi:10.3389/fmed.2021.654912
Prechl, J., Papp, K., Hérincs, Z., Péterfy, H., Lóránd, V., Szittner, Z., et al. (2016). Serological and Genetic Evidence for Altered Complement System Functionality in Systemic Lupus Erythematosus: Findings of the GAPAID Consortium. PLoS One 11, e0150685. doi:10.1371/journal.pone.0150685
Rosenzwajg, M., Lorenzon, R., Cacoub, P., Pham, H. P., Pitoiset, F., El Soufi, K., et al. (2019). Immunological and Clinical Effects of Low-Dose Interleukin-2 across 11 Autoimmune Diseases in a Single, Open Clinical Trial. Ann. Rheum. Dis. 78, 209–217. doi:10.1136/annrheumdis-2018-214229
Saadoun, D., Rosenzwajg, M., Joly, F., Six, A., Carrat, F., Thibault, V., et al. (2011). Regulatory T-Cell Responses to Low-Dose Interleukin-2 in HCV-Induced Vasculitis. N. Engl. J. Med. 365, 2067–2077. doi:10.1056/NEJMoa1105143
Schmid, T., Falter, L., Weber, S., Müller, N., Molitor, K., Zeller, D., et al. (2017). Chronic Inflammation Increases the Sensitivity of Mouse Treg for TNFR2 Costimulation. Front. Immunol. 8, 1471. doi:10.3389/fimmu.2017.01471
Sefik, E., Geva-Zatorsky, N., Oh, S., Konnikova, L., Zemmour, D., McGuire, A. M., et al. (2015). Mucosal Immunology. Individual Intestinal Symbionts Induce a Distinct Population of RORγ⁺ Regulatory T Cells. Science 349, 993–997. doi:10.1126/science.aaa9420
Sharma, R. (2020). Harnessing Endogenous T-Regulatory Cells in Acute Kidney Injury. Nephron 144, 626–628. doi:10.1159/000508503
Sharma, R., and Kinsey, G. R. (2018). Regulatory T Cells in Acute and Chronic Kidney Diseases. Am. J. Physiol. Renal Physiol. 314, F679–F698. doi:10.1152/ajprenal.00236.2017
Sharma, R., Sharma, P. R., Kim, Y. C., Leitinger, N., Lee, J. K., Fu, S. M., et al. (2011). IL-2-Controlled Expression of Multiple T Cell Trafficking Genes and Th2 Cytokines in the Regulatory T Cell-Deficient Scurfy Mice: Implication to Multiorgan Inflammation and Control of Skin and Lung Inflammation. J. Immunol. 186, 1268–1278. doi:10.4049/jimmunol.1002677
Shevach, E. M. (2018). Foxp3+ T Regulatory Cells: Still Many Unanswered Questions-A Perspective After 20 Years of Study. Front. Immunol. 9, 1048. doi:10.3389/fimmu.2018.01048
Sitrin, J., Suto, E., Wuster, A., Eastham-Anderson, J., Kim, J. M., Austin, C. D., et al. (2017). The Ox40/Ox40 Ligand Pathway Promotes Pathogenic Th Cell Responses, Plasmablast Accumulation, and Lupus Nephritis in NZB/W F1 Mice. J. Immunol. 199, 1238–1249. doi:10.4049/jimmunol.1700608
Spooner, C. J., Lesch, J., Yan, D., Khan, A. A., Abbas, A., Ramirez-Carrozzi, V., et al. (2013). Specification of Type 2 Innate Lymphocytes by the Transcriptional Determinant Gfi1. Nat. Immunol. 14, 1229–1236. doi:10.1038/ni.2743
Stremska, M. E., Dai, C., Venkatadri, R., Wang, H., Sabapathy, V., Kumar, G., et al. (2019). IL233, an IL-2-IL-33 Hybrid Cytokine Induces Prolonged Remission of Mouse Lupus Nephritis by Targeting Treg Cells as a Single Therapeutic Agent. J. Autoimmun. 102, 133–141. doi:10.1016/j.jaut.2019.05.005
Stremska, M. E., Jose, S., Sabapathy, V., Huang, L., Bajwa, A., Kinsey, G. R., et al. (2017). IL233, A Novel IL-2 and IL-33 Hybrid Cytokine, Ameliorates Renal Injury. J. Am. Soc. Nephrol. 28, 2681–2693. doi:10.1681/ASN.2016121272
Sun, Y., Chen, H. M., Subudhi, S. K., Chen, J., Koka, R., Chen, L., et al. (2002). Costimulatory Molecule-Targeted Antibody Therapy of a Spontaneous Autoimmune Disease. Nat. Med. 8, 1405–1413. doi:10.1038/nm1202-796
Tackey, E., Lipsky, P. E., and Illei, G. G. (2004). Rationale for Interleukin-6 Blockade in Systemic Lupus Erythematosus. Lupus 13, 339–343. doi:10.1191/0961203304lu1023oa
Takeda, I., Ine, S., Killeen, N., Ndhlovu, L. C., Murata, K., Satomi, S., et al. (2004). Distinct Roles for the OX40-OX40 Ligand Interaction in Regulatory and Nonregulatory T Cells. J. Immunol. 172, 3580–3589. doi:10.4049/jimmunol.172.6.3580
Tang, Y., Tao, H., Gong, Y., Chen, F., Li, C., and Yang, X. (2019). Changes of Serum IL-6, IL-17, and Complements in Systemic Lupus Erythematosus Patients. J. Interferon Cytokine Res. 39, 410–415. doi:10.1089/jir.2018.0169
Vang, K. B., Yang, J., Mahmud, S. A., Burchill, M. A., Vegoe, A. L., and Farrar, M. A. (2008). IL-2, -7, and -15, but Not Thymic Stromal Lymphopoeitin, Redundantly Govern CD4+Foxp3+ Regulatory T Cell Development. J. Immunol. 181, 3285–3290. doi:10.4049/jimmunol.181.5.3285
von Spee-Mayer, C., Siegert, E., Abdirama, D., Rose, A., Klaus, A., Alexander, T., et al. (2016). Low-Dose Interleukin-2 Selectively Corrects Regulatory T Cell Defects in Patients with Systemic Lupus Erythematosus. Ann. Rheum. Dis. 75, 1407–1415. doi:10.1136/annrheumdis-2015-207776
Wang, J., Zhang, S. X., Hao, Y. F., Qiu, M. T., Luo, J., Li, Y. Y., et al. (2020). The Numbers of Peripheral Regulatory T Cells are Reduced in Patients with Psoriatic Arthritis and Are Restored by Low-Dose Interleukin-2. Ther. Adv. Chronic Dis. 11, 2040622320916014. doi:10.1177/2040622320916014
Ward, M. M. (2010). Access to Care and the Incidence of Endstage Renal Disease Due to Systemic Lupus Erythematosus. J. Rheumatol. 37, 1158–1163. doi:10.3899/jrheum.091199
Wong, M., La Cava, A., and Hahn, B. H. (2013). Blockade of Programmed Death-1 in Young (New Zealand Black x New Zealand White)F1 Mice Promotes the Suppressive Capacity of CD4+ Regulatory T Cells Protecting from Lupus-Like Disease. J. Immunol. 190, 5402–5410. doi:10.4049/jimmunol.1202382
Xiao, Y., and Zheng, F. (2016). The Emerging Role of sST2 Blocking in the Therapy of Graft-Versus-Host Disease. Ann. Transl Med. 4, S42. doi:10.21037/atm.2016.10.22
Xie, J. H., Zhang, Y., Loubeau, M., Mangan, P., Heimrich, E., Tovar, C., et al. (2021). Mouse IL-2/CD25 Fusion Protein Induces Regulatory T Cell Expansion and Immune Suppression in Preclinical Models of Systemic Lupus Erythematosus. J. Immunol. 207 (1), 34–43. doi:10.4049/jimmunol.2100078
Yang, B. H., Hagemann, S., Mamareli, P., Lauer, U., Hoffmann, U., Beckstette, M., et al. (2016). Foxp3(+) T Cells Expressing RORγt Represent a Stable Regulatory T-Cell Effector Lineage with Enhanced Suppressive Capacity during Intestinal Inflammation. Mucosal Immunol. 9, 444–457. doi:10.1038/mi.2015.74
Yang, X. P., Ghoreschi, K., Steward-Tharp, S. M., Rodriguez-Canales, J., Zhu, J., Grainger, J. R., et al. (2011). Opposing Regulation of the Locus Encoding IL-17 through Direct, Reciprocal Actions of STAT3 and STAT5. Nat. Immunol. 12, 247–254. doi:10.1038/ni.1995
Zaiss, M. M., Kurowska-Stolarska, M., Böhm, C., Gary, R., Scholtysek, C., Stolarski, B., et al. (2011). IL-33 Shifts the Balance from Osteoclast to Alternatively Activated Macrophage Differentiation and Protects from TNF-Alpha-Mediated Bone Loss. J. Immunol. 186, 6097–6105. doi:10.4049/jimmunol.1003487
Zhang, P., Gao, F., Wang, Q., Wang, X., Zhu, F., Ma, C., et al. (2007). Agonistic Anti-4-1BB Antibody Promotes the Expansion of Natural Regulatory T Cells while Maintaining Foxp3 Expression. Scand. J. Immunol. 66, 435–440. doi:10.1111/j.1365-3083.2007.01994.x
Zhang, S. X., Wang, J., Sun, H. H., Zhang, J. Q., Liu, G. Y., Luo, J., et al. (2021). Circulating Regulatory T Cells Were Absolutely Decreased in Dermatomyositis/Polymyositis Patients and Restored by Low-Dose IL-2. Ann. Rheum. Dis. 80, e130 [Epub ahead of print]. doi:10.1136/annrheumdis-2019-216246
Zhao, C., Chu, Y., Liang, Z., Zhang, B., Wang, X., Jing, X., et al. (2019). Low Dose of IL-2 Combined With Rapamycin Restores and Maintains the Long-Term Balance of Th17/Treg Cells in Refractory SLE Patients. BMC Immunol. 20, 32. doi:10.1186/s12865-019-0305-0
Zhao, Z., Ren, J., Dai, C., Kannapell, C. C., Wang, H., Gaskin, F., et al. (2019). Nature of T Cell Epitopes in Lupus Antigens and HLA-DR Determines Autoantibody Initiation and Diversification. Ann. Rheum. Dis. 78, 380–390. doi:10.1136/annrheumdis-2018-214125
Zhou, L., Lopes, J. E., Chong, M. M., Ivanov, , Min, R., Victora, G. D., et al. (2008). TGF-Beta-Induced Foxp3 Inhibits T(H)17 Cell Differentiation by Antagonizing RORgammat Function. Nature 453, 236–240. doi:10.1038/nature06878
Zhou, T., Li, H. Y., Liao, C., Lin, W., and Lin, S. (2020). Clinical Efficacy and Safety of Mesenchymal Stem Cells for Systemic Lupus Erythematosus. Stem Cells Int. 2020, 6518508. doi:10.1155/2020/6518508
Keywords: IL-2, IL-6, IL-33, Treg cells, SLE, lupus nephritis
Citation: Venkatadri R, Sabapathy V, Dogan M and Sharma R (2022) Targeting Regulatory T Cells for Therapy of Lupus Nephritis. Front. Pharmacol. 12:806612. doi: 10.3389/fphar.2021.806612
Received: 01 November 2021; Accepted: 13 December 2021;
Published: 06 January 2022.
Edited by:
Matthew Griffin, National University of Ireland Galway, IrelandReviewed by:
Niklas Beyersdorf, Julius Maximilian University of Würzburg, GermanyCopyright © 2022 Venkatadri, Sabapathy, Dogan and Sharma. This is an open-access article distributed under the terms of the Creative Commons Attribution License (CC BY). The use, distribution or reproduction in other forums is permitted, provided the original author(s) and the copyright owner(s) are credited and that the original publication in this journal is cited, in accordance with accepted academic practice. No use, distribution or reproduction is permitted which does not comply with these terms.
*Correspondence: Rahul Sharma, rs3wn@virginia.edu