- 1Department of Orthopedics, Guangdong Provincial People’s Hospital, Guangdong Academy of Medical Sciences, Guangzhou, China
- 2Shantou University Medical College, Shantou, China
- 3Research Department of Medical Science, Guangdong Provincial People’s Hospital, Guangdong Academy of Medical Sciences, Guangzhou, China
Traditional Chinese medicine (TCM) has demonstrated superior therapeutic effect for musculoskeletal diseases for thousands of years. Recently, the herbal extracts of TCM have received rapid advances in musculoskeletal tissue engineering (MTE). A literature review collecting both English and Chinese references on bioactive herbal extracts of TCM in biomaterial-based approaches was performed. This review provides an up-to-date overview of application of TCMs in the field of MTE, involving regulation of multiple signaling pathways in osteogenesis, angiogenesis, anti-inflammation, and chondrogenesis. Meanwhile, we highlight the potential advantages of TCM, opening the possibility of its extensive application in MTE. Overall, the superiority of traditional Chinese medicine turns it into an attractive candidate for coupling with advanced additive manufacturing technology.
1 Introduction
Musculoskeletal tissues, composed of bone, cartilage, tendon, ligament, and skeletal muscle, demonstrate poor recovery ability. In fact, the unsatisfactory regeneration of severely damaged tissue often causes pains, joint instability, and even disabilities which remains a tricky problem for surgeons. Although autologous tissue grafts, allografts, or xenografts have been broadly applied in the clinic, they have several limitations, including donor site morbidity and poor plasticity in terms of shape and structure. Furthermore, allografts and xenografts are at risk of transmitting infectious agents or even being rejected (Campana et al., 2014).
Musculoskeletal tissue engineering (MTE) emerged as a promising solution to surmount the limitations of auto- and allografts. Current strategies involve the application of tissues grafts with embedded growth factors to accelerate tissues healing. However, advanced molecules are frequently associated with high production costs or deleterious side-effects, limiting wide applicability, and therapeutic efficacy in clinical practice (Campana et al., 2014). In an effort to discover effective strategies for musculoskeletal tissues regeneration, much focus has been put on the pursuit of natural-based products owing to their availability, cost-effectiveness, and biological activity.
Traditional Chinese medicine (TCM) are becoming increasingly popular in musculoskeletal tissue regeneration. As an indispensable component of complementary and alternative medicine, TCM has evolved over thousands of years and still plays an important role in human health (Campana et al., 2014; Deng et al., 2014; Li et al., 2014). Over the last 3 decades, the application of scientific methodology has partly elucidated the underlying mechanisms of some herbal treatments. The potent bioactivity of some traditional therapies was revealed in recent years, owing to alternative therapies rigor to the study. For example, the 2015 Nobel Prize was awarded for discovering an antimalarial medicine extracted from TCM. With the improved understanding of the underlying mechanism, TCM is becoming increasingly popular in musculoskeletal tissue regeneration (Putnam et al., 2007; Liu et al., 2017; Song et al., 2018b; Zhu et al., 2021).
TCM combining conventional therapies with the MTE is a promising strategy to treat orthopedic diseases with unfavorable outcomes previously. Scientists have successfully isolated, identified, and purified various bioactive components in TCM, such as flavonoids, saponins, terpenes, alkaloids, and others (Lu and Jiang, 2012). Typically, these formulations modulate multiple signaling pathways and exert their effects on different cellular targets, enabling the treatment of orthopedic diseases with multifactorial pathogenesis. This review provides an up-to-date overview of the use of TCMs as signaling molecules and functional materials in the field of MTE, and further highlights their new and promising directions for the future.
2 Traditional Chinese Medicine Theory
TCM originated over 3,500 years ago, and is enjoying a resurgence in the late 20th century. The theoretical medical system of TCM was gradually developed through thousands of years of practice and refinement. It is a sophisticated set of many systematic techniques and methods including acupuncture, herbal medicine, acupressure, “qi gong”, and oriental massage.
Unlike modern medicine, TCM theory not only considers the whole person, but focuses the systematic interrelatedness of the person and nature. It is more focused on the vitalistic and synthetic aspects of humans. The major position of TCM is the balance of “yin” and “yang”. Moreover, it is through the diagnosis of “qi” disturbance that the TCM practitioner restores the “qi” balance, returning the person to a state of health.
According to TCM theories, the occurrence, development, and outcome of diseases are closely related to TCM constitutions. These constitutions form during an individual’s lifetime and are based on natural and acquired endowments (Liang et al., 2020). In addition, constitutions can not only affect disease susceptibility but also the development, outcome, and prognosis of disease. Some aspects of the orthopedic disease in modern mainstream medicine are gradually coinciding with the constitution theory of TCM. For example, the current consensus regarding the etiology and pathogenesis of osteonecrosis states that phlegm and blood-stasis constitutions are the main pathogenic factors (Yu et al., 2016). The blood-stasis constitution has a strong repair ability, but the yang-deficiency constitution has a poor repair ability and tends to collapse, requiring positive treatment. As TCM develops further, intervention measures can be established to improve TCM constitutions.
3 Traditional Chinese Medicine Applications in Orthopedics
Recently, the application of TCM in MTE is booming and show superior therapeutic potential. Some herbs have been proven to be beneficial to bone health, such as Eucommiae Cortex (Du-Zhong) (Qi et al., 2019), Achuranthes (Huai-niu-xi) (Zhang et al., 2018; Song et al., 2018a), Dipsaci Radix (Xu-Duan) (Ke et al., 2016), Testudinis Plastrum (Gui-ban) (Liang et al., 2016; Ren et al., 2017; Shen et al., 2018), Drynariae Rhizoma (Gu sui-bu) (Qiu et al., 2016; Song et al., 2017b), Du Huo Ji Sheng Tang (Wang et al., 2017b), and zuo Gui Wan (Lai et al., 2015). Among these TCMs, the epimedii herb and its multicomponent formulation ‘Xian Ling Gu Bao’ have icariin as their main ingredient and have been used to cure bone diseases such as osteoporosis and bone fracture (Zhang et al., 2014). In parallel, lamiophlomis rotate (Du Yi Wei) pill was used to treatment of pain in clinic, such as bone and muscle pain, joint pain, and dysmenorrhea. It has the effect of promoting blood circulation, relieving pain, stopping bleeding, and removing silt form the perspective of TCM (Li et al., 2010). Moreover, another TCM regent named salvia miltiorrhiza injection, has therapeutic effects in cardiovascular disease with the antioxidant, antibacterial, and anticoagulant properties (Wang et al., 2017b). These herbal medications were approved officially by the Chinese Food and Drug Administration and have been widely used in clinic nowadays. Various studies discussed in the following paragraphs would provide further evidence of the realistic possibility of TCM being used as a therapeutic alternative in the foreseeable future.
4 Multifunctional Traditional Chinese Medicine
4.1 Icariin
Icariin (Figure 1)is a prenyl flavonoid glycoside isolated from Epimedium herb, demonstrating extensive therapeutic capacities such as osteoprotective effect, neuroprotective effect, cardiovascular protective effect, anti-cancer effect, anti-inflammation effect, immunoprotective effect, and reproductive function (Li et al., 2015).
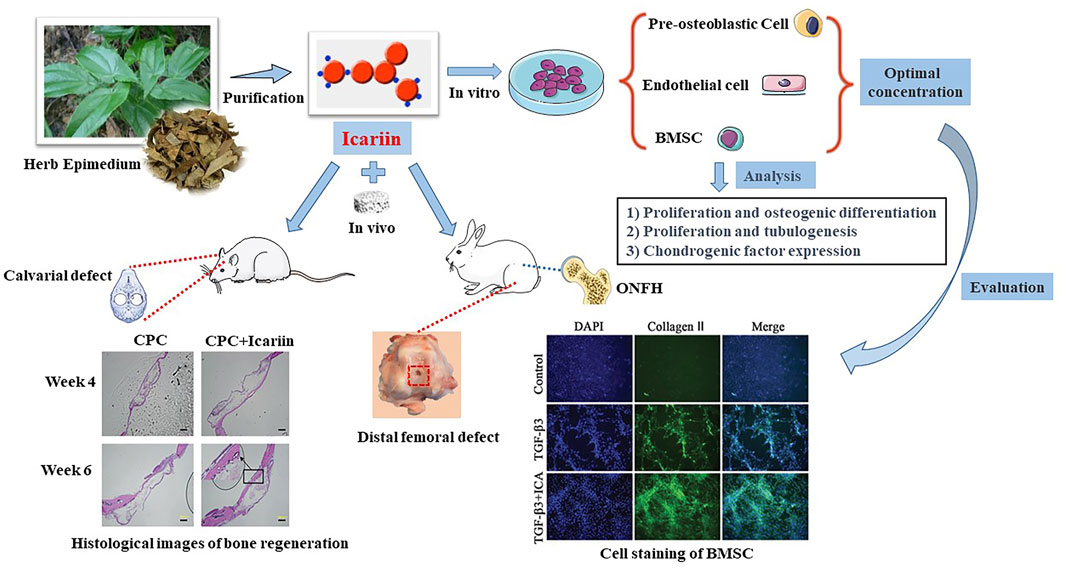
FIGURE 1. Illustration of icariin: the origin, molecular structure, and biomedical researches. Icariin is obtained from the leaves of Epimedium herb. As a widely used TCM, icariin demonstrates extensive therapeutic capacities such as osteogenic differentiation, tubulogenesis, and chondrogenic factor expression. Calvarial defect, distal femoral defect and ONFH mice/rabbits are commonly used animal models.
4.1.1 Osteogenesis
Currently, the significant osteogenic effect of icariin made it a promising drug candidate in bone tissue engineering. As early as 2008, the osteoinductive potential of icariin on pre-osteoblastic cells was elucidated (Zhao et al., 2008). The extremely low cost of icariin and its high abundance makes it appealing for bone regeneration (Fan et al., 2011). Importantly, icariin can be steadily and locally released by using biomaterials, making it an attractive osteoinductive candidate for bone tissue engineering (Zhao et al., 2010; Fan et al., 2012; Zhang et al., 2013).
Previous studies have shown that icariin could induce osteogenic differentiation of preosteoblastic cells. For example, Zhao et al. confirmed the anabolic effect of icariin in vivo employing a mouse calvarial defect model. Calcium phosphate cement loaded with icariin filled in the mouse calvarial bone defect induced significant new bone formation and increased bone thickness. The study utilizing senescence-accelerated mouse models further demonstrated that icariin significantly enhanced bone formation in vivo (Zhao et al., 2010). Moreover, Wu et al. constructed the calcium phosphate cement scaffolds, which loaded with icariin and then implanted into the calvarial defect of the ovariectomized rats. The results demonstrated that icariin could up-regulate the expression of osteogenic and angiogenic genes in bone marrow stem cells (BMSCs) (Wu et al., 2017).
In parallel, icariin could also be loaded into porous β-tricalcium phosphate (β-PTCP) ceramic disks to enhance the ability of calcium phosphate-based biomaterials for bone defect repair. β-TCP has been employed extensively as a substitution material for bone defect repair (Park et al., 2014; Sándor et al., 2014). It was showed that loading icariin in β-TCP (Ica/β-TCP) disks had no effect on the attachment and morphology of Ros17/28 cells. However, the Ica/β-TCP disks expressed support for the proliferation and differentiation of Ros17/28 cells better compared with the β-PTCP disks. After back intramuscular implantation of rats for 3 months, no obvious osteogenic evidence was detected in β-PTCP disks, but new bone formation was observed in Ica/β-TCP disks (Zhang et al., 2011).
In addition to synthetic scaffolds, icariin could be loaded onto the natural scaffold or small intestine submucosa (ICA-SIS) to improve their osteoinductivity (Li et al., 2017). In vitro experiments revealed that expression of osteogenic differentiation markers (Alp, Bsp, and Ocn) was increased after treatment of ICA-SIS scaffold while no significant cytotoxicity was indicated. In an in vivo mouse calvarial defect model, bone regeneration was enhanced by SIS implantation at 8 weeks, compared to the control defect. These results suggest that icariin had the potential to be used for bone defect repair.
4.1.2 Angiogenesis
Vascularization is considered to be a crucial step in bone formation (Wernike et al., 2010). It is reported that icariin stimulated in vitro endothelial cell proliferation, migration, and tubulogenesis, as well as increasing in vivo angiogenesis (Chung et al., 2008). In a recent study, three-dimensional (3D) printing β-TCP scaffold loaded with icariin was implanted into the steroid-induced osteonecrosis of the femoral head rabbit models. Immunohistochemical staining revealed that a higher positive rate of vascular endothelial growth factor in the composite scaffold group. This result suggested that icariin could not only protect the injured vascular endothelial cells, but also stimulate angiogenesis directly (Ji et al., 2005; Wang and Huang, 2005; Xu and Huang, 2007), and it might be the potential drugs in angiogenic therapy.
4.1.3 Chondrogenesis
Hyaline articular cartilage lacks blood vessels, lymphatics, and nerves and exhibits limited self-repair ability after injury. Traditional techniques of articular cartilage repair and regeneration all have certain limitations. The development of tissue engineering technology has brought hope to the regeneration of articular cartilage. Icariin at a low dose of 0.94 G/kg was identified to have significantly promoted the proliferation of chondrocytes and enhance the secretion of glycosaminoglycan (Zhang et al., 2019). Femoral condyle from rabbits treated with icariin conditioned serum and hyaluronic acid was observed to regenerate more native cartilage and subchondral bone regeneration. In vitro study, ICA significantly upregulated the mRNA expression levels and protein synthesis of collagen II, aggrecan, and Sox9, which were chondrogenic differentiation markers (Wang et al., 2014).
In a recent study, BMSCs were cultivated in a self-assembling peptide nanofiber hydrogel scaffold in a chondrogenic medium for 3 weeks (Wang et al., 2018). Icariin was added to the medium throughout the culture period. The results demonstrated that icariin significantly enhanced cartilage extracellular matrix synthesis and gene expression levels of collagen II and Sox9, and additionally promoted more chondrocyte-like rounded morphology in BMSCs. In another study, icariin was added into cell-hydrogel constructs derived from neonatal rabbit chondrocytes and collagen type I (Li et al., 2012). The results showed that icariin-added cell-hydrogel constructs obviously up-regulated the expressions included aggrecan, Sox9, and collagen type II of seeded chondrocytes from 99.7 to 248%. Moreover, the icariin-loaded hydrogel improved the restoration efficiency of supercritical-sized osteochondral defects in adult rabbit model, and enhanced the integration of new-formed cartilage with subchondral bone. These results demonstrated the potential of icariin to promote a reparative response in cartilage defects and the possible application in bioactive material-based cartilage regeneration therapies.
4.2 Ginseng
Ginseng (Figure 2), regards as the king of all herbs, has been used as a traditional medicine for the treatment of diseases for thousands of years in East Asian countries. The principal bioactive components of ginseng are ginsenosides such as Rb1, Rb2, Rc, Rd, Re, and Rg1, showing various anti-inflammatory, antioxidant, antibacterial, antiviral, and antifungal functions. Based on the advantages above, ginseng may provide the basis for the development of novel therapeutic agents.
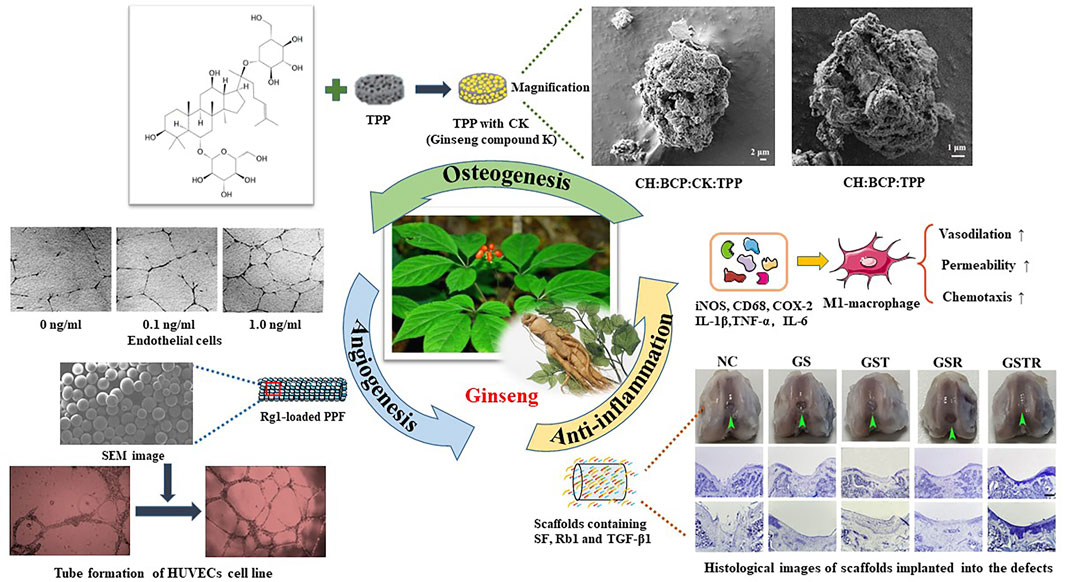
FIGURE 2. Illustration of ginseng: the origin, molecular structure, and biomedical researches. Ginsenoside is the major active ingredient in ginseng, including Rb1, Rb2, Rc, Rd, Re, Rg1, and so on. Thus far, ginseng has been frequently studied form the aspects of cells, biomaterials, and animals. It shows potent angiogenesis, osteogenesis, and anti-inflammation activity by inducing osteogenic cell proliferation, tube formation, and macrophage chemotaxis.
4.2.1 Osteogenesis
Previous studies have demonstrated that Rg1 could improve the anti-aging ability of BMSCs, which was attributed to the anti-oxidant and anti-inflammatory capacities (Hu et al., 2015; Zeng et al., 2018). Moreover, differentiation culture analysis showed that Rg1 could guide human bone marrow-derived mesenchymal stem cells (hBM-MSCs) towards osteogenic lineage while suppressing adipogenic differentiation (Wang et al., 2020c). Additionally, Hong et al. observed that Rg3 increased proliferation and suppressed senescence of hBM-MSCs (Hong et al., 2020). Suitable scaffold materials were prepared using fish scale collagen, hydroxyapatite, chitosan, and beta-tricalcium phosphate. Ginseng compound K was incorporated into the composite scaffold. In vitro analysis showed that the prepared scaffold was biocompatible and supported the growth of MG-63 cells (human osteosarcoma cells), and therefore has potential as an alternative approach for bone regeneration (Muthukumar et al., 2016).
4.2.2 Angiogenesis
On the other hand, Rg1 has been proven to have estrogen-like activity (Chan et al., 2002). It is well known that estrogen could modulate angiogenesis via effects on endothelial cells (Morales et al., 1995). Therefore, it suggests that Rg1 may be used to stimulate angiogenesis.
Based on the above potential, Rg1 was encapsulated into biodegradable poly propylene fumarate (PPF) microspheres to facilitate osteogenesis (Salarian et al., 2016). In the presence of Rg1 within the dose range of 1–32 μg/ml, elongated and robust capillary-like networks were formed in vitro attributed to a greater number of cells compared with the control, indicating the angiogenic activity of Rg1. The previous results suggested that Rg1 could be a novel group of angiogenic agents with superior stability and may be used for the MTE (Liang et al., 2005).
4.2.3 Anti-Inflammation
Inflammatory cytokines induced by traumatic lesions in cartilage and osteoarthritis (OA) are involved in lubricin catabolism and cartilage degeneration, further disrupting the normal homeostasis in articular cartilage to the breakdown of cartilage in the pre-OA conditions (Billinghurst et al., 1997; Elsaid et al., 2007; Goldring and Otero, 2011; Wojdasiewicz et al., 2014). Thus, it is vital to maintain a proper microenvironment for the chondrogenic differentiation of endogenous stem cells at the defected area. Ginseng has been demonstrated to have therapeutic potential in anti-inflammatory, anti-apoptosis, and neuroprotective responses (Radad et al., 2004; Hashimoto et al., 2012).
Porous, stable and biodegradable bone microsphere scaffold loading with ginseng compound was studied, and in vitro results indicated that the composite microspheres expressed higher osteogenic markers in rat bone marrow stem cells seeded (Thangavelu et al., 2020). In another study, Wu et al. designed a novel Rb1/TGF-β1 loaded silk fibroin-gelatin porous scaffold with the advantages of inflammation attenuation and chondrogenesis promotion (Wu et al., 2020). As the results showed, the scaffold promoted the chondrogenic differentiation of BMSCs and suppressed inflammation gene expression. Moreover, it effectively promoted the regeneration of hyaline cartilage of the osteochondral defects in rats. These results prove Rb1 has a great potential to maintain an anti-inflammation microenvironment for cartilage repair.
4.3 Naringin
Gu sui-bu is a commonly used Chinese medical herb for therapeutic treatment of bone-related diseases, and naringin (Figure 3) is the main active component in Gu sui-bu. Recent research has focused on the potential applications as a bone therapeutic or as a mediator of MSC osteogenic lineage differentiation (Chen et al., 2016).
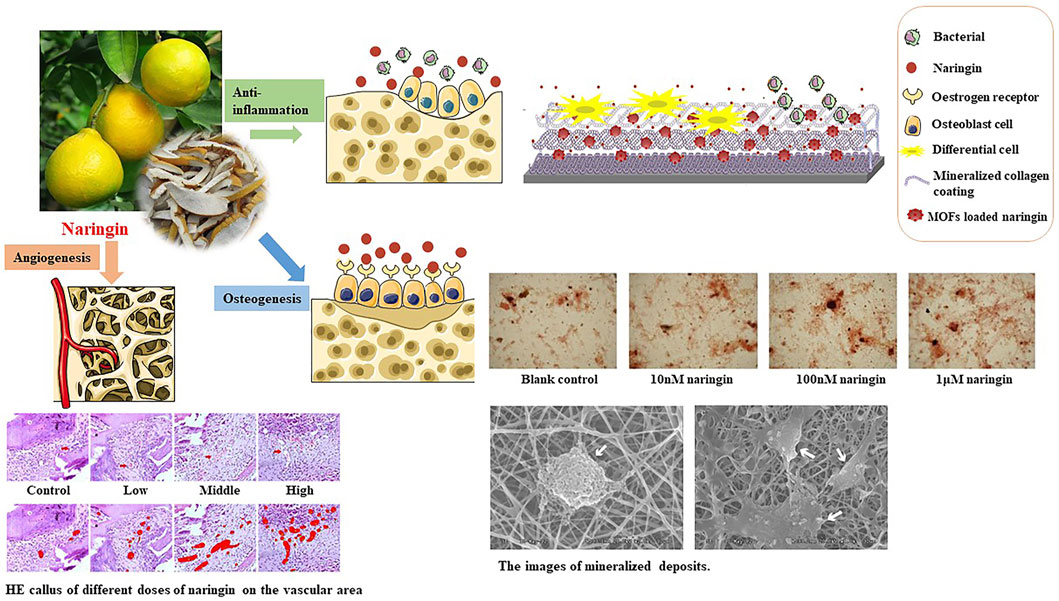
FIGURE 3. Illustration of naringin: the origin and biomedical researches. Naringin is a natural flavonoid present in several fruits of the Citrus genus. As a flavonoid with multiple therapeutic targets in orthopedic tissues, naringin exhibits osteogenic, angiogenic, and anti-inflammation effects in preclinical studies.
4.3.1 Osteogenesis
Pang et al. confirmed naringin significantly prompted osteogenic in osteoblast-like cells via estrogen receptor-dependent pathways (Pang et al., 2010). Importantly, this study demonstrated that naringin exerts tissue-selective estrogenic effects on bone and possibly in adipose tissue, suggesting the potential antiresorptive capacity of naringin. The low bioavailability and extensive metabolism of naringin motivated researchers to explore MTE for immobilizing or protecting it from degradation and for achieving a sustained spatiotemporally controlled release to improve its therapeutic effect.
Initially, human periodontal dental ligament stem cells were seeded in a nanohydroxyapatite scaffold and cultured in a naringin-containing medium for 1 week, following by implanting into healthy mice. The transplant was harvested 8 weeks later and the naringin-treated group exhibited improved trabecular bone maturity surrounding the scaffold (Yin et al., 2015). In a recent study, naringin was incorporated in the electrospun nanoscaffold containing poly (ɛ-caprolactone) (PCL) and poly (ethylene glycol)-block-poly (ɛ-caprolactone) (PEG-b-PCL) (Ji et al., 2014). Osteoblast-nanoscaffold interactions were studied and osteoclast-nanoscaffolds response was evaluated in a mouse calvarial defect organ culture model. The results demonstrated that controlled-release naringin nanoscaffolds supported osteoblast adhesion, proliferation, differentiation, and mineralization more effectively while suppressing osteoclast formation. Alternatively, Chen et al. developed a porous biodegradable composite comprising genipin-crosslinked gelatin and β-Ca3(PO4)2 ceramic microparticles (GGT) mixed with naringin (10 mg/ml) (Chen et al., 2013). The potential of the composites in repairing bone defects was evaluated and compared in vivo by using the biological response of rabbit calvarial bone to these composites. After 8 weeks of implantation, naringin-loaded GGT composites promoted a significant deposition of new bone formation when compared with GGT controls.
4.3.2 Anti-Inflammation
Moreover, previous studies have further demonstrated the antibacterial function of naringin (Tsui et al., 2008). To better exert inherent antimicrobial and pro-osteogenic effects of naringin, Yu et al. designed a multifunctional mineralized collagen coating on titanium with the aid of metal-organic framework nanocrystals to control the release of naringin (Yu et al., 2017). The attachment, proliferation, osteogenic differentiation, and mineralization of mesenchymal stem cells on the coating were significantly enhanced. Meanwhile, antibacterial abilities against Staphylococcus aureus were also promoted.
4.3.3 Angiogenesis
In addition to the properties of osteogenesis and anti-inflammation, naringin could also regulate the function of endothelial cells to promote angiogenesis in bone (Shangguan et al., 2017). Meanwhile, oral administration of naringin could improve the expression of vascular endothelial factor, further augmenting the vascularization of the callus in osteoporotic fractures in ovariectomized rats (Song et al., 2017a). Although these studies have illustrated the angiogenic activity of naringin, the application of naringin to promote vessel ingrowth is still scarce. Given the superior osteogenic, anti-inflammation, and angiogenic properties, naringin is regarded as an excellent candidate for MTE and regenerative medicine application.
5 Monofunctional Traditional Chinese Medicine
5.1 Psoralen
Psoralen is an active component in TCM Buguzhi which means ‘‘material for bone strengthening,” which has been reported to have antibacterial, anti-tumor, coronary artery broadening, and estrogen-like activity (Guo et al., 2003). It has also been reported that the extract of psoralen could promote osteoblastic differentiation as evidence of increased Alp (Xiong et al., 2003).
In order to determine whether psoralen could also increase the amount of new bone formation locally, Wong and Rabie measured the amount of new bone produced by psoralen with collagen matrix carrier grafted into bone defects in rabbits (Wong and Rabie, 2011). As the histological assessment showed, a total of 454% more new bone was present in defects grafted with psoralen in collagen matrix than those grafted with collagen matrix. There was also more amount of bone forming osteoblasts in the psoralen group than the negative control–collagen group. This comparison showed that psoralen was osteogenic when used with the collagen matrix.
5.2 Kaempferol
Kaempferol (Kaem) is a widespread naturally occurring flavonoid in plants and herbs. It is known for its activity in anti-inflammatory, anti-oxidant, anti-cancer, and anti-ulcer properties (Chen and Chen, 2013). In orthopedic aspects, Kaem has been reported to reduce glucocorticoid-induced bone loss and promote osteoblast differentiation (Prouillet et al., 2004; Adhikary et al., 2018). Moreover, Kaem exerts profound anti-osteoclastogenic effects by specifically antagonizing tumor necrosis factor receptor family action on bone cells, by disrupting production of osteoclastogenic cytokines from osteoblasts and attenuating osteoclast precursor cell differentiation (Pang et al., 2006).
A recent study investigating the bioactive glass scaffold loaded with Kaem showed that Kaem could support bioactivity and cell attachment (Ranjbar et al., 2021). In another study, TiO2 implant-immobilized Kaem could be an effective tool for bone regeneration in rats (Tsuchiya et al., 2018). The results showed that BMSCs cultured on alkali-treated TiO2 samples containing Kaem promoted alkaline phosphatase activity, calcium deposition, and osteogenic differentiation. The in vivo histological analysis revealed that Kaem stimulated new bone formation around implants.
5.3 Ursolic Acid
Ursolic acid (UA) is one of many ubiquitous triterpenoids in medicinal herbs. It is found throughout the plant kingdom and constitutes an integral part of the human diet (Xia et al., 2011). Pharmacological effects of UA include anti-cancer (Hsu et al., 1997), pro-differentiation (Lee et al., 1994), anti-viral (Quéré et al., 1996), and anti-invasion activities (Cha et al., 1998).
Interestingly, Lee et al. reported that UA promotes bone formation and induces bone forming activity in vivo (Lee et al., 2008). Furthermore, they showed that the expression of osteoblast-specific genes was enhanced after UA treatment, and that UA could induce osteoblastogenesis and mineralization of osteoblasts in vitro, which was associated with the activation of mitogen-activated protein kinases (MAPKs), activator protein-1 (AP-1), and nuclear factor-kappaB (NF-kB). Studies have also demonstrated that UA activates chondrocytes through the NF-kB/NLRP3 inflammasome pathway, thus preventing cartilage degeneration in osteoarthritis (Wang et al., 2020a). In consideration of the various beneficial effects of UA, it can be considered an effective treatment strategy for OA.
Mesoporous bioglass/chitosan porous scaffolds loaded with UA have been demonstrated to enhance bone regeneration (Ge et al., 2019). The as-released UA drugs from the scaffolds increased the alkaline phosphatase activity, osteogenic differentiation-related gene type I collagen, runt-related transcription factor 2 expression, and osteoblast-associated protein expression remarkably.
5.4 Curcumin
Curcumin, the active constituent for turmeric, is known for its antioxidant, anti-inflammatory, anticancer, and osteogenic activities. Numerous studies published in diverse in vivo models, such as lung inflammation, asthma, sepsis, intestinal inflammation, osteoarthritis, and psoriasis (Daily et al., 2016; Burge et al., 2019; Karimi et al., 2019; Panahi et al., 2019; Shahid et al., 2019; Zahedipour et al., 2020), documenting the anti-inflammatory properties of curcumin. However, its poor bioavailability, rapid metabolism, and rapid systemic elimination led to limiting oral efficacy in various preclinical and clinical studies. To enhance its bioavailability and to provide higher release, several scaffolds loaded with curcumin have been designed.
Cur-loaded microspheres were incorporated into a fish collagen nano-hydroxyapatite scaffold to promote bone repair under diabetic conditions (Li and Zhang, 2018). Curcumin released from the composite scaffolds lasted up to 30 days and remarkably alleviated the negative effects of diabetic serum on the proliferation, migration, and osteogenic differentiation of mesenchymal stem cells. Furthermore, tissue scaffolds containing a low concentration of curcumin could increase gene and protein expression related to osteogenesis (Jain et al., 2016). Interestingly, in another study, liposomal curcumin released from the three-dimensional printed scaffold showed significant cytotoxicity toward in vitro osteosarcoma cells, whereas it promoted osteoblast cell viability, and proliferation (Sarkar and Bose, 2019). Moreover, the composite hydrogel loading with Mg2+ and curcumin could simultaneously exert anti-inflammatory and pro-differentiation effects to accelerate rotator cuff healing (Chen et al., 2021).
Calcium silicate cements have excellent bioactivity and can induce the bone-like apatite formation (Chen et al., 2015; Huang et al., 2015). However, they have degradability and the dissolved calcium silicate can cause the inflammatory response at the early post-implantation stage. Based on these, a study designed the curcumin-loaded mesoporous calcium silicate cement to reduce the inflammatory reaction after implantation (Chen et al., 2017). As the results showed, it could inhibit the expression of TNF-α and IL-1 after inflammatory reaction induced by lipopolysaccharides and had good anti-inflammatory ability. It can provide an excellent strategy to inhibit the inflammatory response for MTE and bone regenerative medicine.
5.5 Thymol
Thymol is a natural product obtained from oregano leaves and is used for various purposes, such as for their antimicrobial, antioxidant, and anti-inflammatory activities (Burt et al., 2005; Liang et al., 2014). It has been shown to reduce the key mediators of inflammatory cytokines (Fachini-Queiroz et al., 2012; Amirghofran et al., 2016). The bioactive effects of thymol combined with MTE is still understudied. A study investigated the effect of thymol on osteogenesis, specifically with osteoblast, and osteoclast cells, from surface-modified Ti6Al4V with plasma sprayed hydroxyapatite coatings (Vu and Bose, 2020). Thymol shows bacterial inhibition of Staphylococcus epidermidis and no cytotoxic effects on osteoblast proliferation in vitro. Despite the scarcity of research, the potential application of thymol in combination with orthopedic biomaterials has been shown.
5.6 Danshen
In order to achieve better bone formation performance, it is necessary to develop some alternative agents with both osteogenesis and angiogenesis, especially for the patients with osteonecrosis of the femoral head. Danshen, or Salvia miltiorrhiza Bunge is a TCM widely used for the treatment of cardiovascular diseases by improving blood circulation and inhibiting inflammatory responses (Zhou et al., 2005). Animal studies have supported the bone protective effect of danshen, and investigation on individual active components of danshen showed a similar effect (Chae et al., 2004; Cui et al., 2011). Salvianolic acid B (SB) is the most abundant molecule isolated from the aqueous fraction of danshen (Yuan et al., 2005), and it has been proven to have the bioactivities of both angiogenesis (Lay et al., 2003; Liu et al., 2007; Tang et al., 2014; Xu et al., 2014; Guo et al., 2014a).
A recent study constructed a SB-loaded chitosan/hydroxyapatite scaffold and loaded it onto the rabbit radius defect model to evaluate the bone repair effect. The angiogenic bioactivities of the SB-loaded chitosan/hydroxyapatite scaffold were proved to be effective by in vivo and in vitro tests (Ji et al., 2019). In another study, SB was bound to the graphene oxide (GO) (Wang et al., 2020b),and silk fibroin (SF) substrates were combined with functionalized GO through the freeze-drying method. After the SF/GO/SB scaffolds were implanted in a rat cranial defect model, the defect area showed more new bone and angiogenesis than that following SF and SF/GO scaffold implantation. Lin et al. developed a bioactive composite scaffold incorporated with SB and evaluated the effects on spinal fusion models (Lin et al., 2019). Results revealed the effect of SB on new bone formation, mineral apposition rate, and vessel density within the scaffold. In summary, these studies suggested that SB could enhance bony fusion through the promotion of angiogenesis.
6 Others
In fact, in addition to the therapeutic potential of TCMs mentioned above, there are other interesting targets. For example, Asperosaponin VI, a natural compound isolated from the well-known traditional Chinese herb Radix Dipsaci, promotes osteoblast formation. The pharmacological study has demonstrated that Asperosaponin VI promoted MC3T3-E1 and primary rat osteoblasts proliferation, and enhanced the formation of bone nodules in osteoblast cells (Niu et al., 2011). In addition, it can promote osteogenic differentiation of adipose-derived stem cells by inducing the expression of bone-related proteins (Ocn and RUNX2, and Smad2/3 phosphorylation) (Ding et al., 2019). Another TCM called cinnamaldehyde, a bioactive cinnamon essential oil from Cinnamomum cassia, has been reported to have multipharmacological activities including anti-inflammation. Recently, researchers have found it can suppress proinflammatory cytokines secretion in rheumatology arthritis synoviocyte cells by Janus kinase/signal transducer and activator of transcription pathway (Luo et al., 2020). As the in vivo results showed, cinnamaldehyde ameliorated collagen-induced arthritis in rats. These findings indicate that cinnamaldehyde is a potential traditional Chinese medicine-derived, disease-modifying, antirheumatic herbal drug.
There have been many in vitro and animal studies provided multidimensional evidence of the efficacy and mechanisms of icariin in treating OA models. Interestingly, a recent study suggests an endocannabinoid-related pathways associated with OA pain and a hypothalamic-related mechanism involving icariin effects (Li et al., 2021). The quantitative proteomics and bioinformatics analyses confirmed that relieving OA pain might be an important mechanism involved in the effects of icariin. These findings contribute to considering icariin as a novel therapy for OA.
MSCs are multipotent stem cells and have the potential to differentiate into several cell linages, including osteoblasts, chondrocytes, adipocytes, cardiomyocytes, and endothelial cells (Ghasroldasht et al., 2014; Oryan et al., 2017). Thus, recruiting MSCs from surrounding tissues or circulation to the fracture callus is very important for bone repair and regeneration (Herrmann et al., 2015; Zhou et al., 2017). In a recent study, Zhu et al. investigated the effect of cyasterone (exact from Radix cyathulae) on MSCs migration and osteogenic differentiation in vitro, as well as its role in the healing of rat fractures (Zhu et al., 2021). As the results showed, cyasterone could promote the migration and osteogenesis capacities of MSCs. The fractured femurs healed faster with the treatment of cyasterone. Meanwhile, cyasterone could significantly increase the level of stromal-derived factor-1α (SDF-1α), which is one of the main chemokines of stem cells, in rats with femur fracture. Even though these findings were not combing with MTE, they enriched the function of TCM as a regenerative medicine and shed new light for the treatment of bone defects in clinical research.
7 Forecast
Musculoskeletal tissues damage, which can be caused by trauma, cancer, and bone disorders, poses formidable public health burdens. Although the strong evidence of the effect of herbal extracts in orthopedics and its potential when combined with biomaterials, there are still some obstacles on the application of herbal extracts in effective clinical use.
7.1 Current Obstacles in TCM Clinical Translation
Despite its promising therapeutic action in several pathologies, particularly in improving degenerative diseases and treating bone defect, most TCM is yet to be widely used in modern medicine. This fact is mainly related to the extensive metabolism of TCM in vivo, a crucial factor that limits their therapeutic efficacy. Nowadays the effects of TCM have been mainly explored via oral absorption; due to the poor solubility and high intestinal liver metabolism of some herbs, it showed limited oral efficacy in various preclinical and clinical studies. Additionally, the intestinal microbiota plays a crucial part in defining the bioavailability of TCM such as naringin (Cassidy and Minihane, 2017). In fact, this microbiome is characterized by substantial heterogeneity between individuals, and defines the clinical efficacy of dietary TCM ultimately.
Adding to this, another problem of studying TCM is to quantify Chinese herbs. Current Chinese herb studies focus on the identification of active components (Guo et al., 2011; Feng et al., 2014; Guo et al., 2014b), which greatly differ from those used in the clinical setting. TCM stresses compatibility when uses medications, which means that active ingredients are combined to produce a therapeutic effect, and the single active ingredient of a single drug cannot fully prove the mechanism of action. Concerning the compatibility of TCM, relevant literature remains scarce and there is a great untapped potential to be exploited for this part. Future exploration should concentrate on enhancing the bioavailability and providing higher release of TCMs.
7.2 Herbal Extracts and Biomaterials
On account of these limitations, there have been many in vitro attempts for improving bioavailability and absorption, by combining biomaterials with herbal extracts, including encapsulation in nanoparticles or microparticles. As recognized from previously highlighted studies with the natural compounds (e.g., quercetin), controlled delivery via nanocarriers can significantly improve their in vivo therapeutic effect (Ahmad et al., 2016). These nanocarriers can provide a sustained release profile for locally improving accumulation at the desired locations, ultimately improving the bioavailability of herbal extracts (Muhamad et al., 2018). Moreover, 3D bio-printing has enabled the fabrication of well-designed 3D constructs for use as transplants with specific shapes and features using various biomaterials and cells (Murphy and Atala, 2014). A new sustained release hydrogel scaffolds composited of mesoporous bioactive glass, sodium alginate, and gelatin were fabricated by the 3D printing technique (Wu et al., 2019). Naringin was used to prepare drug-loaded scaffolds by direct printing or surface absorption. The results showed that MG-63 cells cultured with the extract containing released drugs displayed promoted cell proliferation and the expression of osteogenesis-related genes more effectively compared with the drug-free extract.
In turn, biomaterials used in MTE should have homologous mechanical properties to maintain the morphology and function of tissues. Some herbal extracts can add specific properties to biomaterials, and therefore improves efficacy of the biomaterial. The incorporation of ginseng extract improved the physical characteristics (i.e., hydrophilicity) of PCL nanofibers, as well as the mechanical properties (Pajoumshariati et al., 2016). Although ginseng extract increased the degradation rate of pure PCL nanofibers, the porous structure and morphology of fibers did not change significantly after 42 days. Additionally, a recent study fabricated TCMs incorporated fish collagen film, which has good biocompatibility in mammalian cell growth. In this study, three types of TCMs including genistein, icariin, and naringin were used for film fabrication (Wang et al., 2021). Mechanical properties of collagen films were improved by the addition of TCMs, especially in collagen-naringin films. Furthermore, the solubility and in vitro biodegradation of collagen films were enhanced by the hydrophobicity and chemical interaction of TCMs with collagen. Considering the mutual effects between herb extracts and biomaterials, it can be used to investigate the effect of in vitro stem cell culture.
7.3 Conclusion
In conclusion, TCM have triggered the enthusiasm of many researchers due to their excellent and diverse therapeutic properties. In the past few decades, the demand for safer and more suitable agents has guided researchers to explore the potential of TCM for treating chronic bone diseases. These results reveal the novel approaches toward the fabrication of MTE, which couples the advanced additive manufacturing technology with the wisdom of alternative medicine; the reported potential of TCM makes it a very attractive candidate for coupling with the advanced additive manufacturing technology (Figure 4). Most of these attempts have been effective but remain in a preclinical stage, and more efforts should be paid on in vivo studies. Besides, studies about the regeneration of skeletal muscle tissue are obviously less than those for hard tissues. Thus, further research and development would be necessary to ensure their safe and effective clinical use.
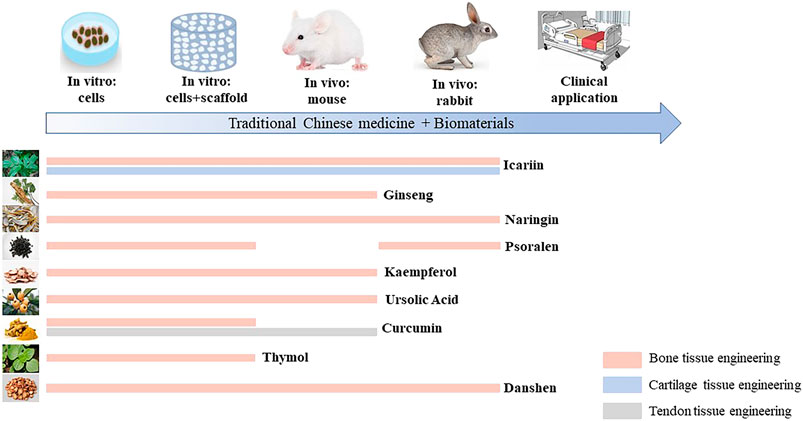
FIGURE 4. Schematic representation of the musculoskeletal application of traditional Chinese medicines.
Author Contributions
HL and RW carried out the literature collection, analysis, and article preparation. HY has contributed to editor the article. QZ and YC have contributed to the funding for supporting this review and supervised the article writing.
Funding
The work was partially supported by National Natural Science Foundation of China (32000958); Guang Dong Basic and Applied Basic Research Foundation (2021A1515011008), and Talent introduction project of Guangdong Provincial People’s Hospital (KJ012020636).
Conflict of Interest
The authors declare that the research was conducted in the absence of any commercial or financial relationships that could be construed as a potential conflict of interest.
Publisher’s Note
All claims expressed in this article are solely those of the authors and do not necessarily represent those of their affiliated organizations, or those of the publisher, the editors and the reviewers. Any product that may be evaluated in this article, or claim that may be made by its manufacturer, is not guaranteed or endorsed by the publisher.
Abbreviations
Alp, alkaline phosphatase; AP-1, activator protein-1; BMSCs, bone marrow stem cells; Bsp, bone sialoprotein; β-TCP, β-tricalcium phosphate; Cur, curcumin; 3D, three-dimensional; GO, graphene oxide; hBM-MSCs, human bone marrow-derived mesenchymal stem cells; ICA, icariin; Kaem, kaempferol; MAPKs, mitogen-activated protein kinases; MTE, musculoskeletal tissue engineering; NF-kB, nuclear factor-kappaB; OA, osteoarthritis; Ocn, osteocalcin; ONFH, osteonecrosis of the femoral head; PEG-b-PCL, poly (ethylene glycol)-block-poly (ɛ-caprolactone); PPF, poly propylene fumarate; SB, salvianolic acid B; SDF-1α, stromal-derived factor-1α; SF, silk fibroin; SIS, small intestine submucosa; TCM, traditional Chinese medicine; UA, ursolic acid.
References
Adhikary, S., Choudhary, D., Ahmad, N., Karvande, A., Kumar, A., Banala, V. T., et al. (2018). Dietary Flavonoid Kaempferol Inhibits Glucocorticoid-Induced Bone Loss by Promoting Osteoblast Survival. Nutrition 53, 64–76. doi:10.1016/j.nut.2017.12.003
Ahmad, N., Banala, V. T., Kushwaha, P., Karvande, A., Sharma, S., Tripathi, A. K., et al. (2016). Quercetin-loaded Solid Lipid Nanoparticles Improve Osteoprotective Activity in an Ovariectomized Rat Model: a Preventive Strategy for post-menopausal Osteoporosis. RSC Adv. 6. doi:10.1039/c6ra17141a
Amirghofran, Z., Ahmadi, H., Karimi, M. H., Kalantar, F., Gholijani, N., and Malek-Hosseini, Z. (2016). In Vitro inhibitory Effects of Thymol and Carvacrol on Dendritic Cell Activation and Function. Pharm. Biol. 54 (7), 1125–1132. doi:10.3109/13880209.2015.1055579
Billinghurst, R. C., Dahlberg, L., Ionescu, M., Reiner, A., Bourne, R., Rorabeck, C., et al. (1997). Enhanced Cleavage of Type II Collagen by Collagenases in Osteoarthritic Articular Cartilage. J. Clin. Invest. 99 (7), 1534–1545. doi:10.1172/JCI119316
Burge, K., Gunasekaran, A., Eckert, J., and Chaaban, H. (2019). Curcumin and Intestinal Inflammatory Diseases: Molecular Mechanisms of Protection. Int. J. Mol. Sci. 20 (8), 1912. doi:10.3390/ijms20081912
Burt, S. A., Vlielander, R., Haagsman, H. P., and Veldhuizen, E. J. (2005). Increase in Activity of Essential Oil Components Carvacrol and Thymol against Escherichia coli O157:H7 by Addition of Food Stabilizers. J. Food Prot. 68 (5), 919–926. doi:10.4315/0362-028x-68.5.919
Campana, V., Milano, G., Pagano, E., Barba, M., Cicione, C., Salonna, G., et al. (2014). Bone Substitutes in Orthopaedic Surgery: from Basic Science to Clinical Practice. J. Mater. Sci. Mater. Med. 25 (10), 2445–2461. doi:10.1007/s10856-014-5240-2
Cassidy, A., and Minihane, A. M. (2017). The Role of Metabolism (And the Microbiome) in Defining the Clinical Efficacy of Dietary Flavonoids. Am. J. Clin. Nutr. 105 (1), 10–22. doi:10.3945/ajcn.116.136051
Cha, H. J., Park, M. T., Chung, H. Y., Kim, N. D., Sato, H., Seiki, M., et al. (1998). Ursolic Acid-Induced Down-Regulation of MMP-9 Gene Is Mediated through the Nuclear Translocation of Glucocorticoid Receptor in HT1080 Human Fibrosarcoma Cells. Oncogene 16 (6), 771–778. doi:10.1038/sj.onc.1201587
Chae, H. J., Chae, S. W., Yun, D. H., Keum, K. S., Yoo, S. K., and Kim, H. R. (2004). Prevention of Bone Loss in Ovariectomized Rats: the Effect of Salvia Miltiorrhiza Extracts. Immunopharmacol. Immunotoxicol. 26 (1), 135–144. doi:10.1081/iph-120029951
Chan, R. Y., Chen, W. F., Dong, A., Guo, D., and Wong, M. S. (2002). Estrogen-like Activity of Ginsenoside Rg1 Derived from Panax Notoginseng. J. Clin. Endocrinol. Metab. 87 (8), 3691–3695. doi:10.1210/jcem.87.8.8717
Chen, A. Y., and Chen, Y. C. (2013). A Review of the Dietary Flavonoid, Kaempferol on Human Health and Cancer Chemoprevention. Food Chem. 138 (4), 2099–2107. doi:10.1016/j.foodchem.2012.11.139
Chen, K. Y., Lin, K. C., Chen, Y. S., and Yao, C. H. (2013). A Novel Porous Gelatin Composite Containing Naringin for Bone Repair. Evid. Based Complement. Alternat. Med. 2013, 283941. doi:10.1155/2013/283941
Chen, Y. W., Yeh, C. H., and Shie, M. Y. (2015). Stimulatory Effects of the Fast Setting and Suitable Degrading Ca-Si-Mg Cement on Both Cementogenesis and Angiogenesis Differentiation of Human Periodontal Ligament Cells. J. Mater. Chem. B 3 (35), 7099–7108. doi:10.1039/c5tb00713e
Chen, R., Qi, Q. L., Wang, M. T., and Li, Q. Y. (2016). Therapeutic Potential of Naringin: an Overview. Pharm. Biol. 54 (12), 3203–3210. doi:10.1080/13880209.2016.1216131
Chen, Y. C., Shie, M. Y., Wu, Y. A., Lee, K. A., Wei, L. J., and Shen, Y. F. (2017). Anti-inflammation Performance of Curcumin-Loaded Mesoporous Calcium Silicate Cement. J. Formos. Med. Assoc. 116 (9), 679–688. doi:10.1016/j.jfma.2017.06.005
Chen, B., Liang, Y., Zhang, J., Bai, L., Xu, M., Han, Q., et al. (2021). Synergistic Enhancement of Tendon-To-Bone Healing via Anti-inflammatory and Pro-differentiation Effects Caused by Sustained Release of Mg2+/curcumin from Injectable Self-Healing Hydrogels. Theranostics 11 (12), 5911–5925. doi:10.7150/thno.56266
Chung, B. H., Kim, J. D., Kim, C. K., Kim, J. H., Kim, J. H., Won, M. H., et al. (2008). Icariin Stimulates Angiogenesis by Activating the MEK/ERK- and PI3K/Akt/eNOS-dependent Signal Pathways in Human Endothelial Cells. Biochem. Biophys. Res. Commun. 376 (2), 404–408. doi:10.1016/j.bbrc.2008.09.001
Cui, Y., Bhandary, B., Marahatta, A., Lee, G. H., Li, B., Kim, D. S., et al. (2011). Characterization of Salvia Miltiorrhiza Ethanol Extract as an Anti-osteoporotic Agent. BMC Complement. Altern. Med. 11, 120. doi:10.1186/1472-6882-11-120
Daily, J. W., Yang, M., and Park, S. (2016). Efficacy of Turmeric Extracts and Curcumin for Alleviating the Symptoms of Joint Arthritis: A Systematic Review and Meta-Analysis of Randomized Clinical Trials. J. Med. Food 19 (8), 717–729. doi:10.1089/jmf.2016.3705
Deng, X., Jiang, M., Zhao, X., and Liang, J. (2014). Efficacy and Safety of Traditional Chinese Medicine for the Treatment of Acquired Immunodeficiency Syndrome: a Systematic Review. J. Tradit. Chin. Med. 34 (1), 1–9. doi:10.1016/s0254-6272(14)60046-7
Ding, X., Li, W., Chen, D., Zhang, C., Wang, L., Zhang, H., et al. (2019). Asperosaponin VI Stimulates Osteogenic Differentiation of Rat Adipose-Derived Stem Cells. Regen. Ther. 11, 17–24. doi:10.1016/j.reth.2019.03.007
Elsaid, K. A., Jay, G. D., and Chichester, C. O. (2007). Reduced Expression and Proteolytic Susceptibility of Lubricin/superficial Zone Protein May Explain Early Elevation in the Coefficient of Friction in the Joints of Rats with Antigen-Induced Arthritis. Arthritis Rheum. 56 (1), 108–116. doi:10.1002/art.22321
Fachini-Queiroz, F. C., Kummer, R., Estevão-Silva, C. F., Carvalho, M. D., Cunha, J. M., Grespan, R., et al. (2012). Effects of Thymol and Carvacrol, Constituents of Thymus Vulgaris L. Essential Oil, on the Inflammatory Response. Evid. Based Complement. Alternat Med. 2012, 657026. doi:10.1155/2012/657026
Fan, J. J., Cao, L. G., Wu, T., Wang, D. X., Jin, D., Jiang, S., et al. (2011). The Dose-Effect of Icariin on the Proliferation and Osteogenic Differentiation of Human Bone Mesenchymal Stem Cells. Molecules 16 (12), 10123–10133. doi:10.3390/molecules161210123
Fan, J., Bi, L., Wu, T., Cao, L., Wang, D., Nan, K., et al. (2012). A Combined Chitosan/nano-Size Hydroxyapatite System for the Controlled Release of Icariin. J. Mater. Sci. Mater. Med. 23 (2), 399–407. doi:10.1007/s10856-011-4491-4
Feng, X., Lyu, Y., Wu, Z., Fang, Y., Xu, H., Zhao, P., et al. (2014). Fructus Ligustri Lucidi Ethanol Extract Improves Bone mineral Density and Properties through Modulating Calcium Absorption-Related Gene Expression in Kidney and Duodenum of Growing Rats. Calcif Tissue Int. 94 (4), 433–441. doi:10.1007/s00223-013-9825-4
Ge, Y. W., Lu, J. W., Sun, Z. Y., Liu, Z. Q., Zhou, J., Ke, Q. F., et al. (2019). Ursolic Acid Loaded-Mesoporous Bioglass/chitosan Porous Scaffolds as Drug Delivery System for Bone Regeneration. Nanomedicine 18, 336–346. doi:10.1016/j.nano.2018.10.010
Ghasroldasht, M. M., Irfan-Maqsood, M., Matin, M. M., Bidkhori, H. R., Naderi-Meshkin, H., Moradi, A., et al. (2014). Mesenchymal Stem Cell Based Therapy for Osteo-Diseases. Cell Biol. Int. 38 (10), 1081–1085. doi:10.1002/cbin.10293
Goldring, M. B., and Otero, M. (2011). Inflammation in Osteoarthritis. Curr. Opin. Rheumatol. 23 (5), 471–478. doi:10.1097/BOR.0b013e328349c2b1
Guo, J., Wu, H., Weng, X., Yan, J., and Bi, K. (2003). Studies on Extraction and Isolation of Active Constituents from Psoralen Corylifolia L. And the Antitumor Effect of the Constituents In Vitro. Zhong Yao Cai 26 (3), 185–187.
Guo, A. J., Choi, R. C., Cheung, A. W., Chen, V. P., Xu, S. L., Dong, T. T., et al. (2011). Baicalin, a Flavone, Induces the Differentiation of Cultured Osteoblasts: an Action via the Wnt/beta-Catenin Signaling Pathway. J. Biol. Chem. 286 (32), 27882–27893. doi:10.1074/jbc.M111.236281
Guo, H. D., Cui, G. H., Tian, J. X., Lu, P. P., Zhu, Q. C., Lv, R., et al. (2014a). Transplantation of Salvianolic Acid B Pretreated Mesenchymal Stem Cells Improves Cardiac Function in Rats with Myocardial Infarction through Angiogenesis and Paracrine Mechanisms. Int. J. Cardiol. 177 (2), 538–542. doi:10.1016/j.ijcard.2014.08.104
Guo, Y., Li, Y., Xue, L., Severino, R. P., Gao, S., Niu, J., et al. (2014b). Salvia Miltiorrhiza: an Ancient Chinese Herbal Medicine as a Source for Anti-osteoporotic Drugs. J. Ethnopharmacol. 155 (3), 1401–1416. doi:10.1016/j.jep.2014.07.058
Hashimoto, R., Yu, J., Koizumi, H., Ouchi, Y., and Okabe, T. (2012). Ginsenoside Rb1 Prevents MPP(+)-Induced Apoptosis in PC12 Cells by Stimulating Estrogen Receptors with Consequent Activation of ERK1/2, Akt and Inhibition of SAPK/JNK, P38 MAPK. Evid. Based Complement. Alternat Med. 2012, 693717. doi:10.1155/2012/693717
Herrmann, M., Verrier, S., and Alini, M. (2015). Strategies to Stimulate Mobilization and Homing of Endogenous Stem and Progenitor Cells for Bone Tissue Repair. Front. Bioeng. Biotechnol. 3, 79. doi:10.3389/fbioe.2015.00079
Hong, T., Kim, M. Y., Da Ly, D., Park, S. J., Eom, Y. W., Park, K. S., et al. (2020). Ca2+-activated Mitochondrial Biogenesis and Functions Improve Stem Cell Fate in Rg3-Treated Human Mesenchymal Stem Cells. Stem Cel Res. Ther. 11 (1), 467. doi:10.1186/s13287-020-01974-3
Hsu, H. Y., Yang, J. J., and Lin, C. C. (1997). Effects of Oleanolic Acid and Ursolic Acid on Inhibiting Tumor Growth and Enhancing the Recovery of Hematopoietic System Postirradiation in Mice. Cancer Lett. 111 (1-2), 7–13. doi:10.1016/s0304-3835(96)04481-3
Hu, W., Jing, P., Wang, L., Zhang, Y., Yong, J., and Wang, Y. (2015). The Positive Effects of Ginsenoside Rg1 upon the Hematopoietic Microenvironment in a D-Galactose-Induced Aged Rat Model. BMC Complement. Altern. Med. 15, 119. doi:10.1186/s12906-015-0642-3
Huang, S. C., Wu, B. C., Kao, C. T., Huang, T. H., Hung, C. J., and Shie, M. Y. (2015). Role of the P38 Pathway in mineral Trioxide Aggregate-Induced Cell Viability and Angiogenesis-Related Proteins of Dental Pulp Cell In Vitro. Int. Endod. J. 48 (3), 236–245. doi:10.1111/iej.12305
Jain, S., Krishna Meka, S. R., and Chatterjee, K. (2016). Curcumin Eluting Nanofibers Augment Osteogenesis toward Phytochemical Based Bone Tissue Engineering. Biomed. Mater. 11 (5), 055007. doi:10.1088/1748-6041/11/5/055007
Ji, R. R., Li, F. Y., and Zhang, X. J. (2005). Effect of Icariin on Hypoxia Induced Vascular Endothelial Cells Injury. Zhongguo Zhong Xi Yi Jie He Za Zhi 25 (6), 525–530.
Ji, Y., Wang, L., Watts, D. C., Qiu, H., You, T., Deng, F., et al. (2014). Controlled-release Naringin Nanoscaffold for Osteoporotic Bone Healing. Dent Mater. 30 (11), 1263–1273. doi:10.1016/j.dental.2014.08.381
Ji, C., Bi, L., Li, J., and Fan, J. (2019). Salvianolic Acid B-Loaded Chitosan/hydroxyapatite Scaffolds Promotes the Repair of Segmental Bone Defect by Angiogenesis and Osteogenesis. Int. J. Nanomedicine 14, 8271–8284. doi:10.2147/ijn.S219105
Karimi, A., Ghodsi, R., Kooshki, F., Karimi, M., Asghariazar, V., and Tarighat-Esfanjani, A. (2019). Therapeutic Effects of Curcumin on Sepsis and Mechanisms of Action: A Systematic Review of Preclinical Studies. Phytother Res. 33 (11), 2798–2820. doi:10.1002/ptr.6467
Ke, K., Li, Q., Yang, X., Xie, Z., Wang, Y., Shi, J., et al. (2016). Asperosaponin VI Promotes Bone Marrow Stromal Cell Osteogenic Differentiation through the PI3K/AKT Signaling Pathway in an Osteoporosis Model. Sci. Rep. 6, 35233. doi:10.1038/srep35233
Lai, N., Zhang, Z., Wang, B., Miao, X., Guo, Y., Yao, C., et al. (2015). Regulatory Effect of Traditional Chinese Medicinal Formula Zuo-Gui-Wan on the Th17/Treg Paradigm in Mice with Bone Loss Induced by Estrogen Deficiency. J. Ethnopharmacol. 166, 228–239. doi:10.1016/j.jep.2015.03.011
Lay, I. S., Chiu, J. H., Shiao, M. S., Lui, W. Y., and Wu, C. W. (2003). Crude Extract of Salvia Miltiorrhiza and Salvianolic Acid B Enhance In Vitro Angiogenesis in Murine SVR Endothelial Cell Line. Planta Med. 69 (1), 26–32. doi:10.1055/s-2003-37034
Lee, H. Y., Chung, H. Y., Kim, K. H., Lee, J. J., and Kim, K. W. (1994). Induction of Differentiation in the Cultured F9 Teratocarcinoma Stem Cells by Triterpene Acids. J. Cancer Res. Clin. Oncol. 120 (9), 513–518. doi:10.1007/bf01221027
Lee, S. U., Park, S. J., Kwak, H. B., Oh, J., Min, Y. K., and Kim, S. H. (2008). Anabolic Activity of Ursolic Acid in Bone: Stimulating Osteoblast Differentiation In Vitro and Inducing New Bone Formation In Vivo. Pharmacol. Res. 58 (5-6), 290–296. doi:10.1016/j.phrs.2008.08.008
Li, Y., and Zhang, Z. Z. (2018). Sustained Curcumin Release from PLGA Microspheres Improves Bone Formation under Diabetic Conditions by Inhibiting the Reactive Oxygen Species Production. Drug Des. Devel. Ther. 12, 1453–1466. doi:10.2147/dddt.S154334
Li, M., Shang, X., Zhang, R., Jia, Z., Fan, P., Ying, Q., et al. (2010). Antinociceptive and Anti-inflammatory Activities of Iridoid Glycosides Extract of Lamiophlomis Rotata (Benth.) Kudo. Fitoterapia 81 (3), 167–172. doi:10.1016/j.fitote.2009.08.018
Li, D., Yuan, T., Zhang, X., Xiao, Y., Wang, R., Fan, Y., et al. (2012). Icariin: a Potential Promoting Compound for Cartilage Tissue Engineering. Osteoarthr. Cartil. 20 (12), 1647–1656. doi:10.1016/j.joca.2012.08.009
Li, H. M., Ye, Z. H., Zhang, J., Gao, X., Chen, Y. M., Yao, X., et al. (2014). Clinical Trial with Traditional Chinese Medicine Intervention ''tonifying the Kidney to Promote Liver Regeneration and Repair by Affecting Stem Cells and Their Microenvironment'' for Chronic Hepatitis B-Associated Liver Failure. World J. Gastroenterol. 20 (48), 18458–18465. doi:10.3748/wjg.v20.i48.18458
Li, C., Li, Q., Mei, Q., and Lu, T. (2015). Pharmacological Effects and Pharmacokinetic Properties of Icariin, the Major Bioactive Component in Herba Epimedii. Life Sci. 126, 57–68. doi:10.1016/j.lfs.2015.01.006
Li, M., Gu, Q., Chen, M., Zhang, C., Chen, S., and Zhao, J. (2017). Controlled Delivery of Icariin on Small Intestine Submucosa for Bone Tissue Engineering. Mater. Sci. Eng. C Mater. Biol. Appl. 71, 260–267. doi:10.1016/j.msec.2016.10.016
Li, X., Xu, Y., Li, H., Jia, L., Wang, J., Liang, S., et al. (2021). Verification of Pain-Related Neuromodulation Mechanisms of Icariin in Knee Osteoarthritis. Biomed. Pharmacother. 144, 112259. doi:10.1016/j.biopha.2021.112259
Liang, H. C., Chen, C. T., Chang, Y., Huang, Y. C., Chen, S. C., and Sung, H. W. (2005). Loading of a Novel Angiogenic Agent, Ginsenoside Rg1 in an Acellular Biological Tissue for Tissue Regeneration. Tissue Eng. 11 (5-6), 835–846. doi:10.1089/ten.2005.11.835
Liang, D., Li, F., Fu, Y., Cao, Y., Song, X., Wang, T., et al. (2014). Thymol Inhibits LPS-Stimulated Inflammatory Response via Down-Regulation of NF-κB and MAPK Signaling Pathways in Mouse Mammary Epithelial Cells. Inflammation 37 (1), 214–222. doi:10.1007/s10753-013-9732-x
Liang, D., Ren, H., Qiu, T., Shen, G., Xie, B., Wei, Q., et al. (2016). Extracts from Plastrum Testudinis Reverse Glucocorticoid-Induced Spinal Osteoporosis of Rats via Targeting Osteoblastic and Osteoclastic Markers. Biomed. Pharmacother. 82, 151–160. doi:10.1016/j.biopha.2016.04.068
Liang, X., Wang, Q., Jiang, Z., Li, Z., Zhang, M., Yang, P., et al. (2020). Clinical Research Linking Traditional Chinese Medicine Constitution Types with Diseases: a Literature Review of 1639 Observational Studies. J. Tradit. Chin. Med. 40 (4), 690–702. doi:10.19852/j.cnki.jtcm.2020.04.019
Lin, S., Cui, L., Chen, G., Huang, J., Yang, Y., Zou, K., et al. (2019). PLGA/β-TCP Composite Scaffold Incorporating Salvianolic Acid B Promotes Bone Fusion by Angiogenesis and Osteogenesis in a Rat Spinal Fusion Model. Biomaterials 196, 109–121. doi:10.1016/j.biomaterials.2018.04.004
Liu, Y. R., Qu, S. X., Maitz, M. F., Tan, R., and Weng, J. (2007). The Effect of the Major Components of Salvia Miltiorrhiza Bunge on Bone Marrow Cells. J. Ethnopharmacol. 111 (3), 573–583. doi:10.1016/j.jep.2007.01.005
Liu, M., Li, Y., and Yang, S. T. (2017). Effects of Naringin on the Proliferation and Osteogenic Differentiation of Human Amniotic Fluid-Derived Stem Cells. J. Tissue Eng. Regen. Med. 11 (1), 276–284. doi:10.1002/term.1911
Lu, Q., and Jiang, J. G. (2012). Chemical Metabolism of Medicinal Compounds from Natural Botanicals. Curr. Med. Chem. 19 (11), 1682–1705. doi:10.2174/092986712799945076
Luo, P., Yu, L., Lin, Q., Wang, C., Yang, D., and Tang, S. (2020). Strontium Modified Calcium Sulfate Hemihydrate Scaffold Incorporating Ginsenoside Rg1/Gelatin Microspheres for Bone Regeneration. Front. Bioeng. Biotechnol. 8, 888. doi:10.3389/fbioe.2020.00888
Morales, D. E., McGowan, K. A., Grant, D. S., Maheshwari, S., Bhartiya, D., Cid, M. C., et al. (1995). Estrogen Promotes Angiogenic Activity in Human Umbilical Vein Endothelial Cells In Vitro and in a Murine Model. Circulation 91 (3), 755–763. doi:10.1161/01.cir.91.3.755
Muhamad, N., Plengsuriyakarn, T., and Na-Bangchang, K. (2018). Application of Active Targeting Nanoparticle Delivery System for Chemotherapeutic Drugs and Traditional/herbal Medicines in Cancer Therapy: a Systematic Review. Int. J. Nanomedicine 13, 3921–3935. doi:10.2147/ijn.S165210
Murphy, S. V., and Atala, A. (2014). 3D Bioprinting of Tissues and Organs. Nat. Biotechnol. 32 (8), 773–785. doi:10.1038/nbt.2958
Muthukumar, T., Aravinthan, A., Sharmila, J., Kim, N. S., and Kim, J. H. (2016). Collagen/chitosan Porous Bone Tissue Engineering Composite Scaffold Incorporated with Ginseng Compound K. Carbohydr. Polym. 152, 566–574. doi:10.1016/j.carbpol.2016.07.003
Niu, Y., Li, Y., Huang, H., Kong, X., Zhang, R., Liu, L., et al. (2011). Asperosaponin VI, a Saponin Component from Dipsacus Asper wall, Induces Osteoblast Differentiation through Bone Morphogenetic Protein-2/p38 and Extracellular Signal-Regulated Kinase 1/2 Pathway. Phytother. Res. 25 (11), 1700–1706. doi:10.1002/ptr.3414
Oryan, A., Kamali, A., Moshiri, A., and Baghaban Eslaminejad, M. (2017). Role of Mesenchymal Stem Cells in Bone Regenerative Medicine: What Is the Evidence? Cells Tissues Organs 204 (2), 59–83. doi:10.1159/000469704
Pajoumshariati, S., Yavari, S. K., and Shokrgozar, M. A. (2016). Physical and Biological Modification of Polycaprolactone Electrospun Nanofiber by Panax Ginseng Extract for Bone Tissue Engineering Application. Ann. Biomed. Eng. 44 (5), 1808–1820. doi:10.1007/s10439-015-1478-1
Panahi, Y., Fazlolahzadeh, O., Atkin, S. L., Majeed, M., Butler, A. E., Johnston, T. P., et al. (2019). Evidence of Curcumin and Curcumin Analogue Effects in Skin Diseases: A Narrative Review. J. Cel Physiol. 234 (2), 1165–1178. doi:10.1002/jcp.27096
Pang, J. L., Ricupero, D. A., Huang, S., Fatma, N., Singh, D. P., Romero, J. R., et al. (2006). Differential Activity of Kaempferol and Quercetin in Attenuating Tumor Necrosis Factor Receptor Family Signaling in Bone Cells. Biochem. Pharmacol. 71 (6), 818–826. doi:10.1016/j.bcp.2005.12.023
Pang, W. Y., Wang, X. L., Mok, S. K., Lai, W. P., Chow, H. K., Leung, P. C., et al. (2010). Naringin Improves Bone Properties in Ovariectomized Mice and Exerts Oestrogen-like Activities in Rat Osteoblast-like (UMR-106) Cells. Br. J. Pharmacol. 159 (8), 1693–1703. doi:10.1111/j.1476-5381.2010.00664.x
Park, J. W., Kim, J. M., Lee, H. J., Jeong, S. H., Suh, J. Y., and Hanawa, T. (2014). Bone Healing with Oxytocin-Loaded Microporous β-TCP Bone Substitute in Ectopic Bone Formation Model and Critical-Sized Osseous Defect of Rat. J. Clin. Periodontol. 41 (2), 181–190. doi:10.1111/jcpe.12198
Prouillet, C., Mazière, J. C., Mazière, C., Wattel, A., Brazier, M., and Kamel, S. (2004). Stimulatory Effect of Naturally Occurring Flavonols Quercetin and Kaempferol on Alkaline Phosphatase Activity in MG-63 Human Osteoblasts through ERK and Estrogen Receptor Pathway. Biochem. Pharmacol. 67 (7), 1307–1313. doi:10.1016/j.bcp.2003.11.009
Putnam, S. E., Scutt, A. M., Bicknell, K., Priestley, C. M., and Williamson, E. M. (2007). Natural Products as Alternative Treatments for Metabolic Bone Disorders and for Maintenance of Bone Health. Phytother Res. 21 (2), 99–112. doi:10.1002/ptr.2030
Qi, S., Zheng, H., Chen, C., and Jiang, H. (2019). Du-Zhong (Eucommia Ulmoides Oliv.) Cortex Extract Alleviates Lead Acetate-Induced Bone Loss in Rats. Biol. Trace Elem. Res. 187 (1), 172–180. doi:10.1007/s12011-018-1362-6
Qiu, Z. C., Dong, X. L., Dai, Y., Xiao, G. K., Wang, X. L., Wong, K. C., et al. (2016). Discovery of a New Class of Cathepsin K Inhibitors in Rhizoma Drynariae as Potential Candidates for the Treatment of Osteoporosis. Int. J. Mol. Sci. 17 (12), 2116. doi:10.3390/ijms17122116
Quéré, L., Wenger, T., and Schramm, H. J. (1996). Triterpenes as Potential Dimerization Inhibitors of HIV-1 Protease. Biochem. Biophys. Res. Commun. 227 (2), 484–488. doi:10.1006/bbrc.1996.1533
Radad, K., Gille, G., Moldzio, R., Saito, H., and Rausch, W. D. (2004). Ginsenosides Rb1 and Rg1 Effects on Mesencephalic Dopaminergic Cells Stressed with Glutamate. Brain Res. 1021 (1), 41–53. doi:10.1016/j.brainres.2004.06.030
Ranjbar, F. E., Foroutan, F., Hajian, M., Ai, J., Farsinejad, A., Ebrahimi-Barough, S., et al. (2021). Preparation and Characterization of 58S Bioactive Glass Based Scaffold with Kaempferol-Containing Zein Coating for Bone Tissue Engineering. J. Biomed. Mater. Res. B Appl. Biomater. 109 (9), 1259–1270. doi:10.1002/jbm.b.34786
Ren, H., Shen, G., Tang, J., Qiu, T., Zhang, Z., Zhao, W., et al. (2017). Promotion Effect of Extracts from Plastrum Testudinis on Alendronate against Glucocorticoid-Induced Osteoporosis in Rat Spine. Sci. Rep. 7 (1), 10617. doi:10.1038/s41598-017-10614-5
Salarian, M., Samimi, R., Xu, W. Z., Wang, Z., Sham, T. K., Lui, E. M. K., et al. (2016). Microfluidic Synthesis and Angiogenic Activity of Ginsenoside Rg1-Loaded PPF Microspheres. ACS Biomater. Sci. Eng. 2 (11), 1872–1882. doi:10.1021/acsbiomaterials.6b00222
Sándor, G. K., Numminen, J., Wolff, J., Thesleff, T., Miettinen, A., Tuovinen, V. J., et al. (2014). Adipose Stem Cells Used to Reconstruct 13 Cases with Cranio-Maxillofacial Hard-Tissue Defects. Stem Cell Transl. Med 3 (4), 530–540. doi:10.5966/sctm.2013-0173
Sarkar, N., and Bose, S. (2019). Liposome-Encapsulated Curcumin-Loaded 3D Printed Scaffold for Bone Tissue Engineering. ACS Appl. Mater. Inter. 11 (19), 17184–17192. doi:10.1021/acsami.9b01218
Shahid, H., Shahzad, M., Shabbir, A., and Saghir, G. (2019). Immunomodulatory and Anti-inflammatory Potential of Curcumin for the Treatment of Allergic Asthma: Effects on Expression Levels of Pro-inflammatory Cytokines and Aquaporins. Inflammation 42 (6), 2037–2047. doi:10.1007/s10753-019-01066-2
Shangguan, W. J., Zhang, Y. H., Li, Z. C., Tang, L. M., Shao, J., and Li, H. (2017). Naringin Inhibits Vascular Endothelial Cell Apoptosis via Endoplasmic Reticulum Stress and Mitochondrial Mediated Pathways and Promotes Intraosseous Angiogenesis in Ovariectomized Rats. Int. J. Mol. Med. 40 (6), 1741–1749. doi:10.3892/ijmm.2017.3160
Shen, G. Y., Ren, H., Huang, J. J., Zhang, Z. D., Zhao, W. H., Yu, X., et al. (2018). Plastrum Testudinis Extracts Promote BMSC Proliferation and Osteogenic Differentiation by Regulating Let-7f-5p and the TNFR2/PI3K/AKT Signaling Pathway. Cell Physiol. Biochem. 47 (6), 2307–2318. doi:10.1159/000491541
Song, N., Zhao, Z., Ma, X., Sun, X., Ma, J., Li, F., et al. (2017a). Naringin Promotes Fracture Healing through Stimulation of Angiogenesis by Regulating the VEGF/VEGFR-2 Signaling Pathway in Osteoporotic Rats. Chem. Biol. Interact. 261, 11–17. doi:10.1016/j.cbi.2016.10.020
Song, S., Gao, Z., Lei, X., Niu, Y., Zhang, Y., Li, C., et al. (2017b). Total Flavonoids of Drynariae Rhizoma Prevent Bone Loss Induced by Hindlimb Unloading in Rats. Molecules 22 (7), 1033. doi:10.3390/molecules22071033
Song, D., Cao, Z., Huang, S., Tickner, J., Li, N., Qiu, H., et al. (2018a). Achyranthes Bidentata Polysaccharide Suppresses Osteoclastogenesis and Bone Resorption via Inhibiting RANKL Signaling. J. Cel Biochem. 119 (6), 4826–4835. doi:10.1002/jcb.26682
Song, J. E., Tripathy, N., Lee, D. H., Park, J. H., and Khang, G. (2018b). Quercetin Inlaid Silk Fibroin/Hydroxyapatite Scaffold Promotes Enhanced Osteogenesis. ACS Appl. Mater. Inter. 10 (39), 32955–32964. doi:10.1021/acsami.8b08119
Tang, Y., Jacobi, A., Vater, C., Zou, X., and Stiehler, M. (2014). Salvianolic Acid B Protects Human Endothelial Progenitor Cells against Oxidative Stress-Mediated Dysfunction by Modulating Akt/mTOR/4EBP1, P38 MAPK/ATF2, and ERK1/2 Signaling Pathways. Biochem. Pharmacol. 90 (1), 34–49. doi:10.1016/j.bcp.2014.04.008
Thangavelu, M., Adithan, A., John Peter, J. S., Hossain, M. A., Kim, N. S., Hwang, K. C., et al. (2020). Ginseng Compound K Incorporated Porous Chitosan/biphasic Calcium Phosphate Composite Microsphere for Bone Regeneration. Int. J. Biol. Macromol. 146, 1024–1029. doi:10.1016/j.ijbiomac.2019.09.228
Tsuchiya, S., Sugimoto, K., Kamio, H., Okabe, K., Kuroda, K., Okido, M., et al. (2018). Kaempferol-immobilized Titanium Dioxide Promotes Formation of New Bone: Effects of Loading Methods on Bone Marrow Stromal Cell Differentiation In Vivo and In Vitro. Int. J. Nanomedicine 13, 1665–1676. doi:10.2147/ijn.S150786
Tsui, V. W., Wong, R. W., and Rabie, A. B. (2008). The Inhibitory Effects of Naringin on the Growth of Periodontal Pathogens In Vitro. Phytother Res. 22 (3), 401–406. doi:10.1002/ptr.2338
Vu, A. A., and Bose, S. (2020). Natural Antibiotic Oregano in Hydroxyapatite-Coated Titanium Reduces Osteoclastic Bone Resorption for Orthopedic and Dental Applications. ACS Appl. Mater. Inter. 12 (47), 52383–52392. doi:10.1021/acsami.0c14993
Wang, Y. K., and Huang, Z. Q. (2005). Protective Effects of Icariin on Human Umbilical Vein Endothelial Cell Injury Induced by H2O2 In Vitro. Pharmacol. Res. 52 (2), 174–182. doi:10.1016/j.phrs.2005.02.023
Wang, Z. C., Sun, H. J., Li, K. H., Fu, C., and Liu, M. Z. (2014). Icariin Promotes Directed Chondrogenic Differentiation of Bone Marrow Mesenchymal Stem Cells but Not Hypertrophy In Vitro. Exp. Ther. Med. 8 (5), 1528–1534. doi:10.3892/etm.2014.1950
Wang, J. Y., Chen, W. M., Wen, C. S., Hung, S. C., Chen, P. W., and Chiu, J. H. (2017a). Du-Huo-Ji-Sheng-Tang and its Active Component Ligusticum Chuanxiong Promote Osteogenic Differentiation and Decrease the Aging Process of Human Mesenchymal Stem Cells. J. Ethnopharmacol. 198, 64–72. doi:10.1016/j.jep.2016.12.011
Wang, L., Ma, R., Liu, C., Liu, H., Zhu, R., Guo, S., et al. (2017b). Salvia Miltiorrhiza: A Potential Red Light to the Development of Cardiovascular Diseases. Curr. Pharm. Des. 23 (7), 1077–1097. doi:10.2174/1381612822666161010105242
Wang, Z., Li, K., Sun, H., Wang, J., Fu, Z., and Liu, M. (2018). Icariin Promotes Stable Chondrogenic Differentiation of Bone Marrow Mesenchymal Stem Cells in Self Assembling Peptide Nanofiber Hydrogel Scaffolds. Mol. Med. Rep. 17 (6), 8237–8243. doi:10.3892/mmr.2018.8913
Wang, C., Gao, Y., Zhang, Z., Chen, C., Chi, Q., Xu, K., et al. (2020a). Ursolic Acid Protects Chondrocytes, Exhibits Anti-inflammatory Properties via Regulation of the NF-κB/NLRP3 Inflammasome Pathway and Ameliorates Osteoarthritis. Biomed. Pharmacother. 130, 110568. doi:10.1016/j.biopha.2020.110568
Wang, W., Liu, Y., Yang, C., Jia, W., Qi, X., Liu, C., et al. (2020b). Delivery of Salvianolic Acid B for Efficient Osteogenesis and Angiogenesis from Silk Fibroin Combined with Graphene Oxide. ACS Biomater. Sci. Eng. 6 (6), 3539–3549. doi:10.1021/acsbiomaterials.0c00558
Wang, Z., Jiang, R., Wang, L., Chen, X., Xiang, Y., Chen, L., et al. (2020c). Ginsenoside Rg1 Improves Differentiation by Inhibiting Senescence of Human Bone Marrow Mesenchymal Stem Cell via GSK-3β and β-Catenin. Stem Cell Int. 2020, 2365814. doi:10.1155/2020/2365814
Wang, R., Bao, B., Wang, S., Elango, J., and Wu, W. (2021). Fabrication of Chinese Traditional Medicines Incorporated Collagen Biomaterials for Human Bone Marrow Mesenchymal Stem Cells. Biomed. Pharmacother. 139, 111659. doi:10.1016/j.biopha.2021.111659
Wernike, E., Montjovent, M. O., Liu, Y., Wismeijer, D., Hunziker, E. B., Siebenrock, K. A., et al. (2010). VEGF Incorporated into Calcium Phosphate Ceramics Promotes Vascularisation and Bone Formation In Vivo. Eur. Cel Mater 19, 30–40. doi:10.22203/ecm.v019a04
Wojdasiewicz, P., Poniatowski, Ł. A., and Szukiewicz, D. (2014). The Role of Inflammatory and Anti-inflammatory Cytokines in the Pathogenesis of Osteoarthritis. Mediators Inflamm. 2014, 561459. doi:10.1155/2014/561459
Wong, R. W., and Rabie, A. B. (2011). Effect of Psoralen on Bone Formation. J. Orthop. Res. 29 (2), 158–164. doi:10.1002/jor.21124
Wu, Y., Cao, L., Xia, L., Wu, Q., Wang, J., Wang, X., et al. (2017). Evaluation of Osteogenesis and Angiogenesis of Icariin in Local Controlled Release and Systemic Delivery for Calvarial Defect in Ovariectomized Rats. Sci. Rep. 7 (1), 5077. doi:10.1038/s41598-017-05392-z
Wu, J., Miao, G., Zheng, Z., Li, Z., Ren, W., Wu, C., et al. (2019). 3D Printing Mesoporous Bioactive Glass/sodium Alginate/gelatin Sustained Release Scaffolds for Bone Repair. J. Biomater. Appl. 33 (6), 755–765. doi:10.1177/0885328218810269
Wu, T., Chen, Y., Liu, W., Tong, K. L., Suen, C. W., Huang, S., et al. (2020). Ginsenoside Rb1/TGF-β1 Loaded Biodegradable Silk Fibroin-Gelatin Porous Scaffolds for Inflammation Inhibition and Cartilage Regeneration. Mater. Sci. Eng. C Mater. Biol. Appl. 111, 110757. doi:10.1016/j.msec.2020.110757
Xia, E. Q., Wang, B. W., Xu, X. R., Zhu, L., Song, Y., and Li, H. B. (2011). Microwave-assisted Extraction of Oleanolic Acid and Ursolic Acid from Ligustrum Lucidum Ait. Int. J. Mol. Sci. 12 (8), 5319–5329. doi:10.3390/ijms12085319
Xiong, Z., Wang, D., Xu, Y., and Li, F. (2003). Osteoblastic Differentiation Bioassay and its Application to Investigating the Activity of Fractions and Compounds from Psoralea Corylifolia L. Pharmazie 58 (12), 925–928.
Xu, H. B., and Huang, Z. Q. (2007). Icariin Enhances Endothelial Nitric-Oxide Synthase Expression on Human Endothelial Cells In Vitro. Vascul. Pharmacol. 47 (1), 18–24. doi:10.1016/j.vph.2007.03.002
Xu, D., Xu, L., Zhou, C., Lee, W. Y., Wu, T., Cui, L., et al. (2014). Salvianolic Acid B Promotes Osteogenesis of Human Mesenchymal Stem Cells through Activating ERK Signaling Pathway. Int. J. Biochem. Cel Biol. 51, 1–9. doi:10.1016/j.biocel.2014.03.005
Yin, L., Cheng, W., Qin, Z., Yu, H., Yu, Z., Zhong, M., et al. (2015). Effects of Naringin on Proliferation and Osteogenic Differentiation of Human Periodontal Ligament Stem Cells In Vitro and In Vivo. Stem Cell Int. 2015, 758706. doi:10.1155/2015/758706
Yu, T., Zhang, Z., Xie, L., Ke, X., and Liu, Y. (2016). The Influence of Traditional Chinese Medicine Constitutions on the Potential Repair Capacity after Osteonecrosis of the Femoral Head. Complement. Ther. Med. 29, 89–93. doi:10.1016/j.ctim.2016.09.010
Yu, M., You, D., Zhuang, J., Lin, S., Dong, L., Weng, S., et al. (2017). Controlled Release of Naringin in Metal-Organic Framework-Loaded Mineralized Collagen Coating to Simultaneously Enhance Osseointegration and Antibacterial Activity. ACS Appl. Mater. Inter. 9 (23), 19698–19705. doi:10.1021/acsami.7b05296
Yuan, D., Pan, Y. N., Fu, W. W., Makino, T., and Kano, Y. (2005). Quantitative Analysis of the Marker Compounds in Salvia Miltiorrihiza Root and its Phytomedicinal Preparations. Chem. Pharm. Bull. (Tokyo) 53 (5), 508–514. doi:10.1248/cpb.53.508
Zahedipour, F., Hosseini, S. A., Sathyapalan, T., Majeed, M., Jamialahmadi, T., Al-Rasadi, K., et al. (2020). Potential Effects of Curcumin in the Treatment of COVID-19 Infection. Phytother Res. 34 (11), 2911–2920. doi:10.1002/ptr.6738
Zeng, Y., Hu, W., Jing, P., Chen, X., Wang, Z., Wang, L., et al. (2018). The Regulation of Ginsenoside Rg1 upon Aging of Bone Marrow Stromal Cell Contribute to Delaying Senescence of Bone Marrow Mononuclear Cells (BMNCs). Life Sci. 209, 63–68. doi:10.1016/j.lfs.2018.07.025
Zhang, X., Guo, Y., Li, D. X., Wang, R., Fan, H. S., Xiao, Y. M., et al. (2011). The Effect of Loading Icariin on Biocompatibility and Bioactivity of Porous β-TCP Ceramic. J. Mater. Sci. Mater. Med. 22 (2), 371–379. doi:10.1007/s10856-010-4198-y
Zhang, X., Xu, M., Song, L., Wei, Y., Lin, Y., Liu, W., et al. (2013). Effects of Compatibility of Deproteinized Antler Cancellous Bone with Various Bioactive Factors on Their Osteogenic Potential. Biomaterials 34 (36), 9103–9114. doi:10.1016/j.biomaterials.2013.08.024
Zhang, X., Liu, T., Huang, Y., Wismeijer, D., and Liu, Y. (2014). Icariin: Does it Have an Osteoinductive Potential for Bone Tissue Engineering? Phytother Res. 28 (4), 498–509. doi:10.1002/ptr.5027
Zhang, S., Zhang, Q., Zhang, D., Wang, C., and Yan, C. (2018). Anti-osteoporosis Activity of a Novel Achyranthes Bidentata Polysaccharide via Stimulating Bone Formation. Carbohydr. Polym. 184, 288–298. doi:10.1016/j.carbpol.2017.12.070
Zhang, J., Zhang, D., Wu, C., Liu, A., Zhang, C., Jiao, J., et al. (2019). Icariin-conditioned Serum Engineered with Hyaluronic Acid Promote Repair of Articular Cartilage Defects in Rabbit Knees. BMC Complement. Altern. Med. 19 (1), 155. doi:10.1186/s12906-019-2570-0
Zhao, J., Ohba, S., Shinkai, M., Chung, U. I., and Nagamune, T. (2008). Icariin Induces Osteogenic Differentiation In Vitro in a BMP- and Runx2-dependent Manner. Biochem. Biophys. Res. Commun. 369 (2), 444–448. doi:10.1016/j.bbrc.2008.02.054
Zhao, J., Ohba, S., Komiyama, Y., Shinkai, M., Chung, U. I., and Nagamune, T. (2010). Icariin: a Potential Osteoinductive Compound for Bone Tissue Engineering. Tissue Eng. Part. A. 16 (1), 233–243. doi:10.1089/ten.TEA.2009.0165
Zhou, L., Zuo, Z., and Chow, M. S. (2005). Danshen: an Overview of its Chemistry, Pharmacology, Pharmacokinetics, and Clinical Use. J. Clin. Pharmacol. 45 (12), 1345–1359. doi:10.1177/0091270005282630
Zhou, Q., Yang, C., and Yang, P. (2017). The Promotional Effect of Mesenchymal Stem Cell Homing on Bone Tissue Regeneration. Curr. Stem Cel Res. Ther. 12 (5), 365–376. doi:10.2174/1574888x10666150211160604
Keywords: traditional Chinese medicine, herbal extracts, orthopedics, musculoskeletal tissue engineering, biomaterials
Citation: Li H, Wu R, Yu H, Zheng Q and Chen Y (2021) Bioactive Herbal Extracts of Traditional Chinese Medicine Applied with the Biomaterials: For the Current Applications and Advances in the Musculoskeletal System. Front. Pharmacol. 12:778041. doi: 10.3389/fphar.2021.778041
Received: 16 September 2021; Accepted: 15 October 2021;
Published: 28 October 2021.
Edited by:
Liangliang Xu, Guangzhou University of Chinese Medicine, ChinaReviewed by:
Meiling Zhu, Sun Yat-sen University, ChinaZhao Wang, Third Affiliated Hospital of Guangzhou Medical University, China
Copyright © 2021 Li, Wu, Yu, Zheng and Chen. This is an open-access article distributed under the terms of the Creative Commons Attribution License (CC BY). The use, distribution or reproduction in other forums is permitted, provided the original author(s) and the copyright owner(s) are credited and that the original publication in this journal is cited, in accordance with accepted academic practice. No use, distribution or reproduction is permitted which does not comply with these terms.
*Correspondence: Qiujian Zheng, zhengqiujian@gdph.org.cn; Yuanfeng Chen, chenyuanfeng@gdph.org.cn
†These authors have contributed equally to this work