- Institute of Clinical Pharmacology, University Medical Center Göttingen, Göttingen, Germany
Psychostimulants are used therapeutically and for illegal recreational purposes. Many of these are inhibitors of the presynaptic noradrenaline, dopamine, and serotonin transporters (NET, DAT, and SERT). According to their physicochemical properties, some might also be substrates of polyspecific organic cation transporters (OCTs) that mediate uptake in liver and kidneys for metabolism and excretion. OCT1 is genetically highly polymorphic, with strong effects on transporter activity and expression. To study potential interindividual differences in their pharmacokinetics, 18 psychostimulants and hallucinogens were assessed in vitro for transport by different OCTs as well as by the high-affinity monoamine transporters NET, DAT, and SERT. The hallucinogenic natural compound mescaline was found to be strongly transported by wild-type OCT1 with a Km of 24.3 µM and a vmax of 642 pmol × mg protein−1 × min−1. Transport was modestly reduced in variants *2 and *7, more strongly reduced in *3 and *4, and lowest in *5 and *6, while *8 showed a moderately increased transport capacity. The other phenylethylamine derivatives methamphetamine, para-methoxymethamphetamine, (-)-ephedrine, and cathine ((+)-norpseudoephedrine), as well as dimethyltryptamine, were substrates of OCT2 with Km values in the range of 7.9–46.0 µM and vmax values between 70.7 and 570 pmol × mg protein−1 × min−1. Affinities were similar or modestly reduced and the transport capacities were reduced down to half in the naturally occurring variant A270S. Cathine was found to be a substrate for NET and DAT, with the Km being 21-fold and the vmax 10-fold higher for DAT but still significantly lower compared to OCT2. This study has shown that several psychostimulants and hallucinogens are substrates for OCTs. Given the extensive cellular uptake of mescaline by the genetically highly polymorphic OCT1, strong interindividual variation in the pharmacokinetics of mescaline might be possible, which could be a reason for highly variable adverse reactions. The involvement of the polymorphic OCT2 in the renal excretion of several psychostimulants could be one reason for individual differences in toxicity.
Introduction
Psychostimulants modulate wakefulness and mental performance. They function as indirect sympathomimetics by raising synaptic concentrations of monoamine neurotransmitters through stimulating their release from presynaptic vesicles and/or inhibiting reuptake. Psychostimulants can also interfere with monoaminergic neurotransmitter metabolism and interact with monoaminergic receptors and other targets (Luethi and Liechti, 2020; Reith and Gnegy, 2020). Amphetamine and other phenylethylamine derivatives (Figure 1 top) form a large group of such indirect sympathomimetics. They are used in the treatment of attention deficit hyperactivity disorder and narcolepsy but are also frequently found in illicit drugs (e.g., “speed”, “ecstasy”, “crystal meth”) (Sharma and Couture, 2014; Luethi and Liechti, 2020). Another indirect sympathomimetic is cocaine (Figure 1 bottom left), a tropa-alkaloid and, historically, the first local anesthetic. Its (widely illegal) use as a psychostimulant nowadays far exceeds its therapeutic application in local anesthesia. Psychostimulants are among the most popular drugs of abuse. A related and partially overlapping class of psychoactive substances are the hallucinogens (psychedelics), which alter perception, cognition, and mood. These include tryptamine derivatives, such as the alkaloid dimethyltryptamine (DMT). It is a main constituent of ayahuasca, the plant brew used traditionally by indigenous inhabitants of the Amazon region for spiritual and religious ceremonies. DMT and its diethyl analogue (Figure 1 bottom right) show structural resemblance to the neurotransmitter serotonin and thereby function as agonists at 5-HT2A and related receptors (Nichols, 2016; Luethi and Liechti, 2020). Another traditional hallucinogen is mescaline, a phenethylamine alkaloid found in cacti (Ogunbodede et al., 2010; Nichols, 2016; Luethi and Liechti, 2020). It is a partial agonist at 5-HT2A and 5-HT2B receptors and a full agonist at the 5-HT2C receptor (Dinis-Oliveira et al., 2019).
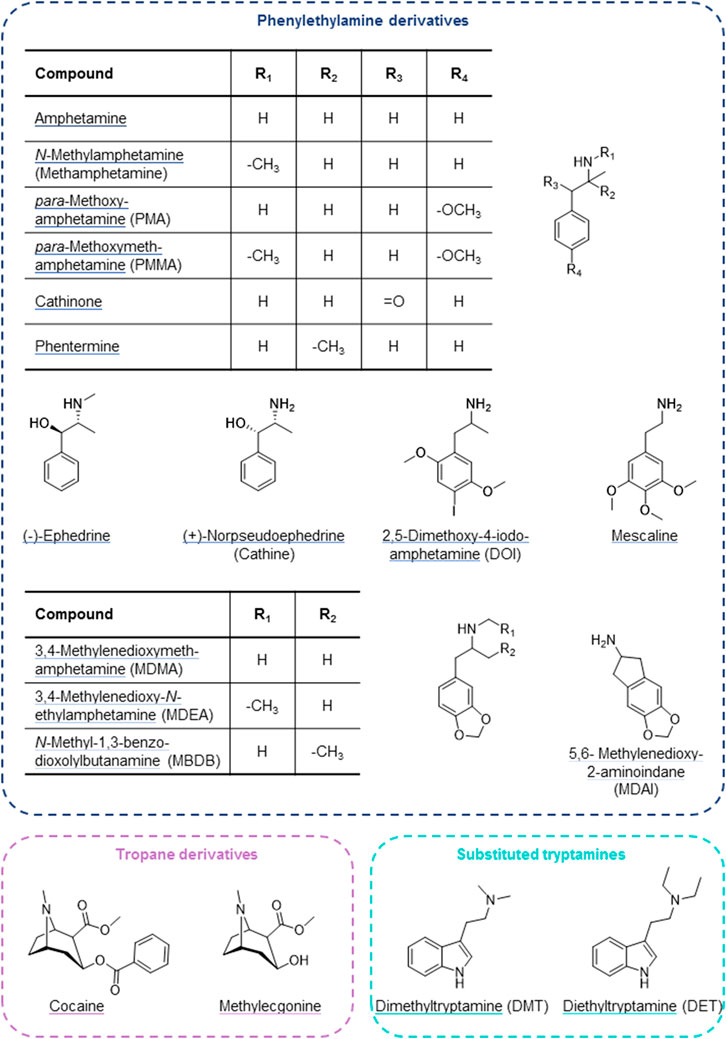
FIGURE 1. Psychostimulant and hallucinogenic drugs assessed for cell uptake by OCTs and high-affinity monoamine transporters.
Many psychoactive substances are substrates or inhibitors of the noradrenaline (norepinephrine) transporter (NET), the dopamine transporter (DAT), and/or the serotonin transporter (SERT) (Luethi and Liechti, 2020). These high-affinity transport proteins are expressed at presynaptic neurons, where they mediate the reuptake of monoamine neurotransmitters from the synaptic cleft to terminate synaptic signal transmission and for recycling (Torres et al., 2003). They are members of the large Solute Carrier (SLC) superfamily and coded for by the genes SLC6A2 (NET), SLC6A3 (DAT), and SLC6A4 (SERT).
Organic cation transporters (OCTs) are also SLCs with a broad, partially overlapping substrate spectrum that is predominantly comprised of hydrophilic, organic cationic substances (including monoamine neurotransmitters as well as many drugs) (Busch et al., 1998; Gründemann et al., 1998; Wu et al., 1998; Koepsell et al., 2007). OCT1 (SLC22A1) and, to a lesser extent, OCT3 (SLC22A3) are expressed on the sinusoidal membrane of hepatocytes, where they mediate cellular uptake for hepatic metabolism (Nishimura and Naito, 2005; Nies et al., 2009). A high degree of genetic variation exists for SLC22A1, and several of these variants strongly impact transporter expression and function (Koepsell et al., 2007; Seitz et al., 2015). This may affect the pharmacokinetics of compounds that are substrates of OCT1, as has been shown, for example, for the opioid analgesics morphine and O-desmethyltramadol (Tzvetkov et al., 2011; Tzvetkov et al., 2013; Venkatasubramanian et al., 2014; Stamer et al., 2016), the antimalarial prodrug proguanil (Matthaei et al., 2019), the anti-asthma drug fenoterol (Tzvetkov et al., 2018), sumatriptan that is used for the treatment of migraine (Matthaei et al., 2016), and, to a minor extent, for the antidiabetic drug metformin (Tzvetkov et al., 2009; Yee et al., 2018). The psychoactive substances studied here (Figure 1) were selected based on physicochemical properties (organic cations with pKa > 8.4 and relatively hydrophilic substances with a logDpH 7.4 < 2; Table 1) that make them potential substrates for OCTs. Consequently, their pharmacokinetics could potentially be affected by OCT polymorphism as well. OCT2 (SLC22A2) is mainly found on the basolateral membrane of kidney epithelial cells (Motohashi et al., 2002; Motohashi et al., 2013). Together with multidrug and toxin extrusion protein 2 kidney-specific (MATE2-K, SLC47A2), an efflux transporter expressed on the brush-border membrane of the proximal tubule, it mediates transport across the epithelium for renal excretion (Motohashi et al., 2013). SLC22A2 variants are less frequent compared to the gene coding for OCT1, and only a few affect OCT2 expression or function. The most frequent of these is Ala270Ser, which causes a moderate decrease in OCT2 activity (Zolk et al., 2009). As many psychoactive substances are structurally related to the neurotransmitters and OCT substrates noradrenaline, dopamine, and serotonin and have physicochemical properties in line with typical OCT substrates, their pharmacokinetics may be determined by OCTs and influenced by OCT1 (and possibly OCT2) polymorphism.
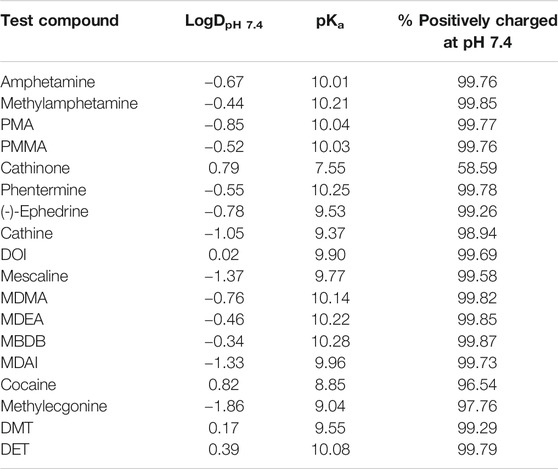
TABLE 1. Physicochemical properties of investigated psychoactive compounds (predicted using MarvinSketch, version 19.8, ChemAxon, Budapest, Hungary).
Although mainly expressed in peripheral tissues, OCT2 and OCT3 are also found on postsynaptic neurons (and OCT3 in astrocytes) predominantly in aminergic regions of the central nervous system. There, they may be involved in reuptake of monoamine neurotransmitters in brain areas lacking the high-affinity transporters, at distance from the aminergic nerve endings, or as an alternative when the high-affinity transporters are saturated or inhibited (Wu et al., 1998; Vialou et al., 2008; Bacq et al., 2012; Couroussé and Gautron, 2015). OCT2 appears to be involved in the uptake of noradrenaline and serotonin in particular, while OCT3 was found to be more strongly responsible for dopamine clearance (Vialou et al., 2008; Bacq et al., 2012). Interestingly, it has also been shown that amphetamines can induce neurotransmitter release through OCT3, which is capable of bi-directional transport (Mayer et al., 2018; Mayer et al., 2019). Thus, OCTs may not only determine the pharmacokinetics of psychoactive drugs but appear to be also involved in their actions.
Given the potential dual role of OCTs with respect to psychoactive drugs and the current lack of understanding of the pharmacokinetics and pharmacogenetics for these compounds, we characterised the transmembrane transport by polyspecific OCTs as well as high-affinity monoamine reuptake transporters. Of particular interest are those psychostimulants that are stereoisomers of one another (ephedrine, norephedrine, their enantiomers and diastereomers), because the impact of stereospecificity on membrane transport is as yet not well understood but previous results suggest partially strong enantiopreferences (Jensen et al., 2020).
Materials and Methods
Test Compounds
The psychoactive compounds studied here were selected based on their physicochemical properties that would make them likely substrates for OCTs. Selection criteria included hydrophilicity (logD at pH 7.4 of less than 2), at least 90% positively charged at physiological pH (pKa > 8.4), and molecular mass not higher than 500 Da. The reasons for these were that lipophilic compounds permeate membranes mostly by diffusion, while membrane transport is mostly relevant for more hydrophilic compounds, as well as the observation that typical OCT1 substrates are usually positively charged and of low to moderate size. Cathinone (pKa of 7.55) did not meet our selection criteria but was nonetheless included due to a low renal elimination (2–7% unchanged in urine) and, consequently, high rate of metabolism which, if taking place in the liver, might depend on hepatic uptake via OCT1 (Kalix and Braenden, 1985; Toennes and Kauert, 2002). Ranitidine-d6 was purchased from Toronto Research Chemicals (Toronto, Canada) and Tulobuterol from Santa Cruz Biotechnology (Darmstadt, Germany); all other test compounds and internal standards were bought from Sigma-Aldrich (Taufkirchen, Germany).
Generation of Transporter-Overexpressing Cell Lines
Transport experiments were done using HEK293 cells stably transfected to overexpress OCT1*1 (wild-type), OCT1*2 (M420del), OCT1*3 (R61C), OCT1*4 (G401S), OCT1*5 (M420del, G465R), OCT1*6 (C88R, M420del), OCT1*7 (S14F), OCT1*8 (R488M), as well as wild-type OCT2, OCT3, NET, DAT, SERT, or MATE2-K. All cell lines were generated using the Flp-In system (Thermo Fisher Scientific, Darmstadt, Germany) as previously described (Saadatmand et al., 2012; Seitz et al., 2015; Chen et al., 2017), except for the OCT3-overexpressing HEK293 cells that were a kind gift from Drs. Koepsell and Gorbulev (University of Würzburg, Germany). The cells were kept in culture for no more than 30 passages.
The high-affinity monoamine transporters were also stably transfected into HEK293 cells by use of the Flp-In system (Thermo Fisher Scientific, Darmstadt, Germany). Coding sequences of SLC6A2 (NET), SLC6A3 (DAT), and SLC6A4 (SERT) were obtained from Source BioScience (Nottingham, United Kingdom; pBluescriptR:SLC6A2) or Addgene (Watertown, MA, United States; pcDNA3.1-hDAT was a gift from Susan Amara, Addgene plasmid # 32810, http://n2t.net/addgene:32810, RRID:Addgene_32810 and hSERT pcDNA3 was a gift from Randy Blakely, Addgene plasmid # 15483, http://n2t.net/addgene:15483, RRID:Addgene_15483 (Ramamoorthy et al., 1993)). After sequence correction and cloning into the pcDNA5 vector, generation and characterization of the cell lines were carried out as described before for the above-mentioned cell lines (Saadatmand et al., 2012; Seitz et al., 2015). Genomic integration was validated for two independent cell clones by three polymerase chain reactions (PCR; Figure 2) to verify proper integration (integration PCR) and exclude multiple integration (multiple integration PCR). The presence of the gene of interest was verified by Sanger sequencing of the product of the third PCR (gene-of-interest PCR) after gel extraction (Figure 2). Overexpression of monoamine transporters was compared between cell clones by TaqMan® gene expression assays (Thermo Fisher Scientific, Darmstadt, Germany; Figure 2). Functional validation of newly generated cell clones was performed using noradrenaline and serotonin as probe drugs and one clone for each transporter was chosen for further transport studies.
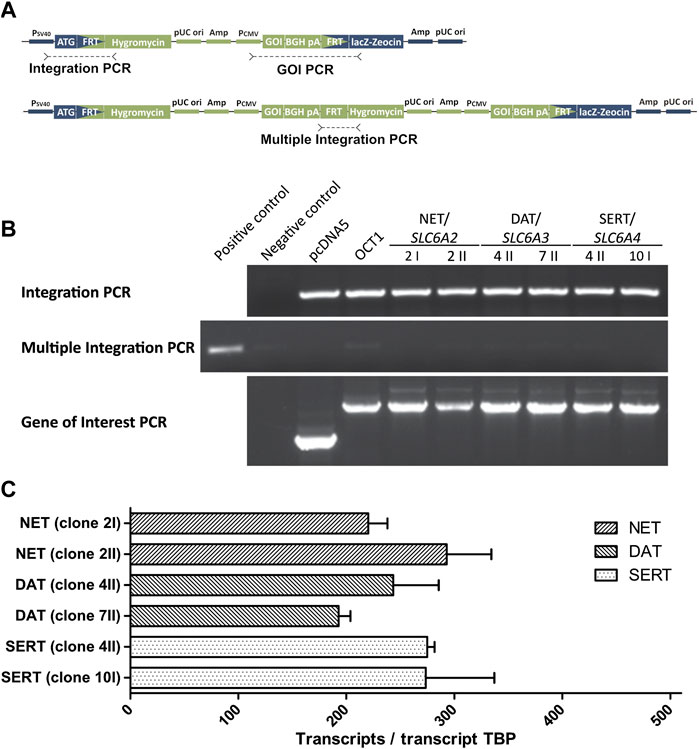
FIGURE 2. Validation of HEK293 cell clones overexpressing monoamine neurotransmitter transporters (A) Schematic representation of the expression plasmid pcDNA5 (green) and the host cell line genome (blue) at the FRT site showing the target positions of the three conducted PCRs (B) Results of the three validation PCRs that show a successful integration (Integration PCR) for all newly created cell clones that overexpress the high-affinity monoamine transporters. The absence of amplicons in the Multiple Integration PCR indicate a single integration of the pcDNA5 plasmid. The correctness of amplified genes in the Gene of Interest (GOI) PCR was validated by Sanger sequencing (C) Quantitative real-time PCR results to confirm comparable overexpression of monoamine transporters, shown as transcripts per transcript of the TATA-binding protein. Only one cell clone was selected per transporter for experiments.
In vitro Cellular Uptake Experiments
The HEK293 cells were cultered in DMEM medium supplemented with 10% (v/v) fetal bovine serum as well as penicillin (100 U/ml) and streptomycin (100 μg/ml) obtained from Thermo Fisher Scientific (Darmstadt, Germany). Cells were seeded on 12-well plates coated with poly-d-lysine (Sigma-Aldrich, Taufkirchen, Germany) 48 h before the transport experiments and incubated at 37°C, 95% relative humidity, and 5% CO2. Cell lines overexpressing MATE2-K were incubated with 30 mM NH4Cl in HBSS+ (10 mM HEPES in HBSS, pH 7.4; Thermo Fisher Scientific, Darmstadt, Germany) for 30 min prior to the assay to invert the direction of transport. All cell lines were washed with 37°C HBSS+ and subsequently incubated with the pre-warmed substrate in HBSS+ at 37°C. The time points for measuring substrate uptake were 1 min for MATE2-K and 2 min for the other SLCs. The uptake rate was experimentally determined to be linear for at least 10 min for OCT1*1. It was assumed to be linear for the other transporters as well, based on previous experience with these expression systems. The reaction was stopped by adding ice-cold HBSS+, and the cells were washed twice with ice-cold HBSS+ before lysis with 80% acetonitrile (LGC Standards, Wesel, Germany) including an internal standard. Subsequently, the intracellular substrate accumulation was determined using LC-MS/MS.
Concentration Analyses
Intracellular accumulation was measured by HPLC-MS/MS using a Shimadzu Nexera HPLC system with a LC-30AD pump, a SIL-30AC autosampler, a CTO-20AC column oven, and a CBM-20A controller (Shimadzu, Kyoto, Japan). Separation was done on a Brownlee SPP RP-Amide column (4.6 × 100 mm inner dimension with 2.7 μm particle size) with a C18 pre-column. The aqueous mobile phase contained 0.1% (v/v) formic acid and either 3% (v/v) organic additive (acetonitrile:methanol 6:1 (v/v)) for methylecgonine, 8% for amphetamine, methylamphetamine, cathinone, cathine, (-)-ephedrine, mescaline, MDAI, and DMT, or 20% for PMA, PMMA, DOI, phentermine, MDMA, MDEA, MBDB, cocaine, and DET. Chromatography was done at a flow rate of 0.3 ml/min. For detection, an API 4000 tandem mass spectrometer (AB SCIEX, Darmstadt, Germany) was used in MRM mode. The analytes, corresponding internal standards, and detection parameters are listed in the Supplementary Table S1. Peak integration and quantification of the analytes was done using the Analyst software (Version 1.6.2, AB SCIEX, Darmstadt, Germany) and determined by simultaneous measurement of standard curves with known concentrations.
Calculations
For the screenings, cellular uptake measured in cell lines overexpressing the respective transporter was divided by the uptake measured in an empty vector control cell line to calculate normalised ratios to enable comparisons between test compounds. For studying transport kinetics, the net transport mediated by the overexpressed transporters was calculated by subtracting the cellular uptake measured in an empty vector control cell line from the uptake in cell lines overexpressing the respective transporter. The parameters Km and vmax were estimated by regression analysis using the Michaelis-Menten equation (GraphPad Prism version 5.01 for Windows, GraphPad Software, La Jolla, CA, United States). Means and standard errors were calculated from individual Km and vmax values of at least three independent experiments. The kinetic parameters vmax and Km were tested for statistical significance over empty vector control cells using Student’s t-test with an alpha value of 0.05.
Results
Screening of Transport Activity at OCTs, Monoamine Transporters, and MATE2-K
Eighteen psychostimulants and hallucinogens were initially screened for their potential to be substrates for different polyspecific OCTs and high-affinity monoamine neurotransmitter transporters (Figure 3), as well as for the efflux transporter MATE2-K (Supplementary Figure S1). The compounds were assessed at a concentration of 1 μM, because it is unlikely that low-affinity transport at higher concentrations may have any medical relevance and the relative contribution of carrier-mediated transport over passive diffusion is significantly greater at lower compared to higher substrate concentrations, as was previously shown for morphine (Tzvetkov et al., 2013). Although the test compounds were selected based on physicochemical properties that are in accordance with those of typical OCT substrates, OCT1 showed high transport activity at this concentration only for mescaline. A cellular uptake in transporter-transfected cells of at least 3-fold higher than in non-overexpressing control cells was selected as the threshold for further studies, as this ratio is suitable to distinguish substrates from non-substrates. Cellular uptake of mescaline was more than 8-fold higher in OCT1-overexpressing cells, which was the highest transport activity that was observed altogether in this study. Interestingly, mescaline was not transported much at 1 µM by any of the other transporters. In contrast to the substrate-specific but very strong transport activity exhibited by OCT1, moderate (4- to 6-fold) cellular uptake by OCT2 was seen for methamphetamine, (-)-ephedrine, and cathine ((+)-norpseudoephedrine) and approximately 3-fold for para-methoxymethamphetamine (PMMA) and DMT. OCT3 and MATE2-K (Supplementary Figure S1) showed little or no transport activity with any of the 18 psychoactive compounds studied here at 1 µM. Our observation, that amphetamine does not appear to be a substrate of OCT3, is in accordance with previous reports (Zhu et al., 2010).
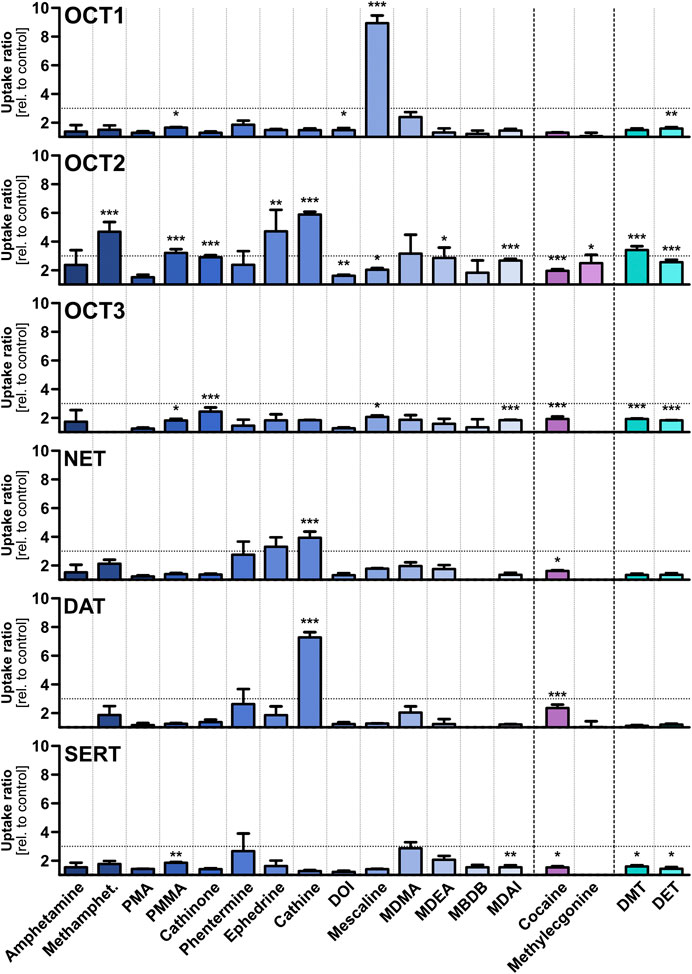
FIGURE 3. Transport of different psychostimulant and hallucinogenic substances at a concentration of 1 µM by OCTs and high-affinity monoamine transporters, shown as the ratios of uptake after 2 min in transporter-transfected cells over empty vector control cells. Shown are the mean values of ≥3 independent experiments +SEM. The horizontal dotted line indicates an uptake ratio of 3, which was set as the minimum threshold for more detailed characterisation. Statistical significance over empty vector control cells was determined using Student’s t-test with *p < 0.05, **p < 0.01, and ***p < 0.001.
The OCTs are known as low-affinity, high-capacity solute carriers with a very broad substrate spectrum that comprises structurally diverse compounds. In contrast, the monoamine neurotransmitter reuptake transporters NET, DAT, and SERT show high affinities to their respective endogenous substrates and a more narrow substrate profile than the OCTs. Cathine was transported modestly (4-fold) by NET and higher (7-fold) by DAT. No notable transport activity was observed for the other compounds, and none by SERT altogether. Cathine and (-)-ephedrine (as well as their stereoisomers) have been described previously as substrates for NET and DAT in vitro experiments with very different setup (Rothman et al., 2003). The slightly higher (albeit still low) uptake of PMA and PMMA by SERT compared to DAT is in line with literature reports that substitution in para-position of the phenyl ring of amphetamine derivatives shifts substrate preference toward SERT (Simmler et al., 2014).
Concentration-dependent Transport of Mescaline by OCT1 Genetic Variants
Mescaline was found in our substrate screenings to be strongly transported by OCT1 and, therefore, it was studied in more detail. Given the high degree of genetic polymorphism and the large differences in transporter activity and expression for some variants, cellular uptake of mescaline was not only characterised for wild-type (OCT1*1) but for OCT1 variants *2 to *8 as well. OCT1*1 transported mescaline with a Km of 24.3 ± 6.3 µM and a vmax of 642 ± 57 pmol × mg protein−1 × min−1 (Figure 4A, Table 2). Time-dependent uptake of 1 µM mescaline showed a faster uptake rate within the first minute of incubation and a constant, linear uptake rate for 2 to at least 10 min (Figure 4B). The apparently more rapid initial uptake rate is likely a result of high-affinity binding to OCT1, but a short-lived more rapid transport might also be possible. The constant transport rate after 2 min of incubation might be the more relevant transport rate for pharmacokinetics because the exposure of the liver and other organs to drugs and other substances usually occurs for several hours. Mescaline uptake could be completely inhibited by the competitive OCT1 inhibitor 1-methyl-4-phenylpyridinium (MPP+; Figure 4C). The Km was slightly higher and the vmax slightly lower for *2, which is analogous to literature data on reduced transport activity for *2 (Seitz et al., 2015; Koepsell, 2020). This was even more pronounced (Km of 93.6 ± 110.8 and 98.2 ± 46.7 µM; vmax of 391 ± 266 and 329 ± 92 pmol × mg protein−1 × min−1) for *3 and *4, which are known to have strongly reduced transport activity (Seitz et al., 2015; Koepsell, 2020). For the variants *5 and *6 that result in impaired translocation to the plasma membrane (Seitz et al., 2015), very low transport activity was observed. Consequently, Km and vmax values could not be reliably calculated. OCT1*7 exhibited a similar Km and a modestly reduced vmax than OCT1*1. OCT1*8, on the other hand, showed a higher vmax than the wild-type, which has been reported previously for a number of substrates as well (Seitz et al., 2015; Koepsell, 2020). To summarise, transport activity of mescaline was slightly lower than wild-type OCT1 in variants *2 and *7, more drastically reduced in *3 and *4, and lowest in *5 and *6, while *8 showed a moderately higher vmax than wild-type OCT1.
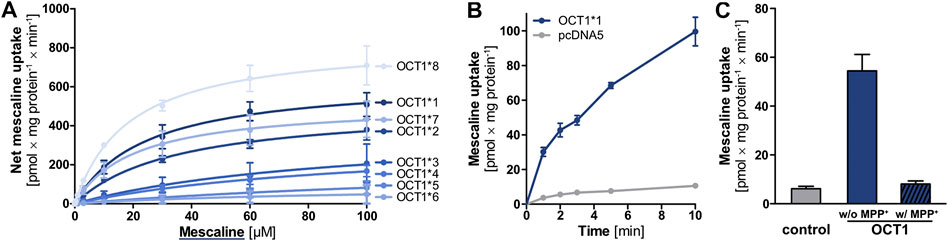
FIGURE 4. (A) Transport of mescaline at different concentrations by OCT1*1 (wild-type) and genetic variants, shown as the cellular uptake in transporter-overexpressing cells with substracted uptake in non-overexpressing control cells. Shown are the mean values of ≥3 independent experiments ±SEM. Km and vmax values are given in Table 2. (B) Time-dependent uptake of 1 µM mescaline in OCT1*1-overexpressing (blue) and non-overexpressing control (pcDNA5, gray) cells, shown as the mean values of 3 independent experiments ±SEM (C) Mescaline transport by OCT1 could be completely inhibited by 1 mM MPP+ to values not significantly different from the unspecific cellular uptake observed in empty vector-transfected cells (control).
Concentration-dependent Transport of Methamphetamine, PMMA, (-)-Ephedrine, Cathine, and DMT by OCT2 Wild-type and A270S Variant
Whereas only mescaline appeared to be a substrate for OCT1, transport via OCT2 was seen for methamphetamine, PMMA, (-)-ephedrine, cathine, and DMT. These compounds were subsequently assessed in greater detail (Figure 5). For methamphetamine, the vmax for wild-type OCT2 was only 70.7 ± 8.3 pmol × mg protein−1 × min−1, whereas it was between 225 and 570 pmol × mg protein−1 × min−1 for the other four compounds. The Km values were around 10 µM except for cathine (46.0 ± 17.3 µM). For the A270S variant, the vmax values were slightly to moderately lower (except for PMMA) and the Km values either similar ((-)-ephedrine and DMT) or up to 4-fold higher (methamphetamine, PMMA, cathine) compared to wild-type OCT2, in agreement with literature reports that the A270S exchange can lead to a moderate decrease in OCT2 activity (Zolk et al., 2009).
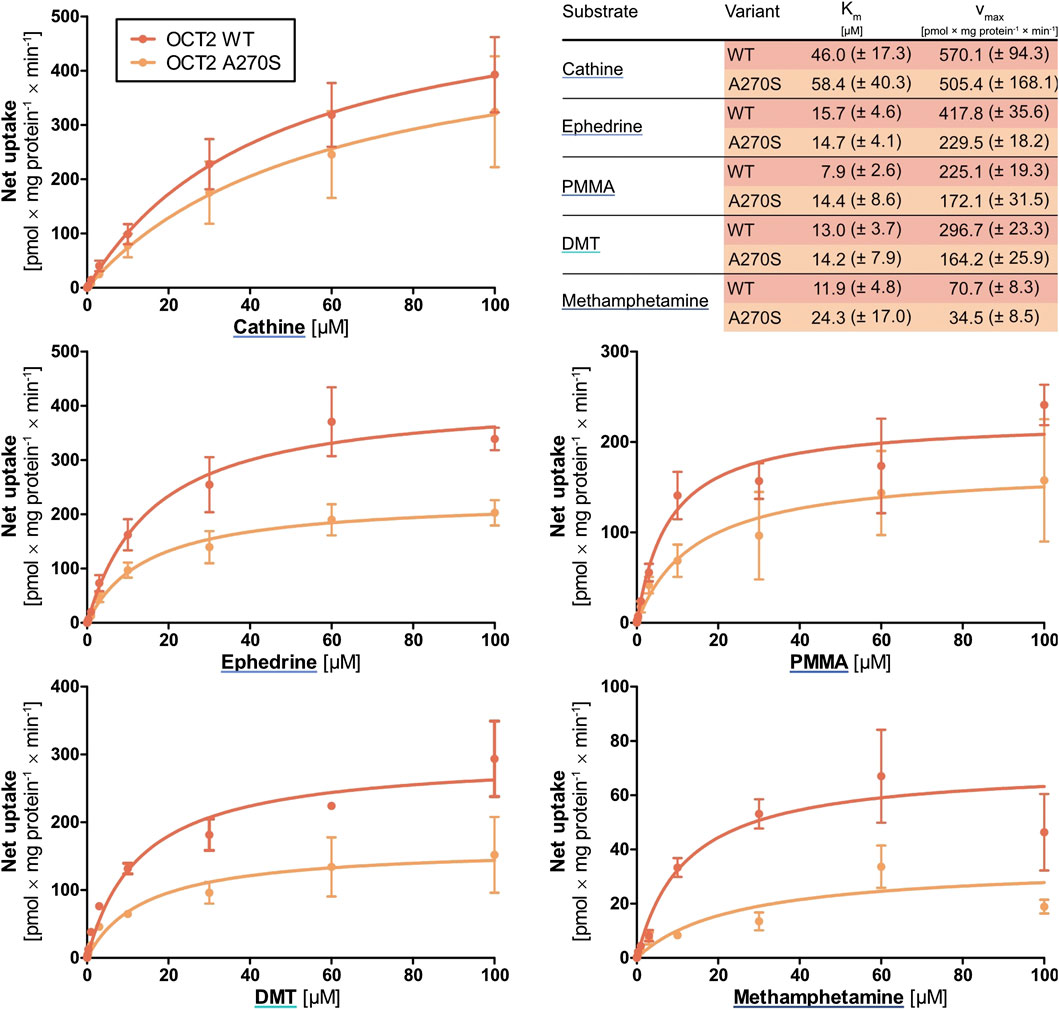
FIGURE 5. Transport of cathine, (-)-ephedrine, PMMA, DMT, and methamphetamine at different concentrations by wild-type OCT2 (red) and the variant A270S (orange), shown as the cellular uptake in transporter-overexpressing cells with substracted uptake in non-overexpressing control cells. Shown are the mean values of ≥3 independent experiments ±SEM.
Concentration-dependent Transport of Cathine by NET and DAT
Cathine was the only compound for which notable cellular uptake was observed by the high-affinity monoamine transporters NET and DAT. Further characterisation and a comparison between NET and DAT revealed that the Km was 21-fold and the vmax 10-fold higher for DAT (Figure 6). Yet, both Km and vmax were still significantly lower compared to OCT2, in line with the general description of NET and DAT as high-affinity and low-capacity transporters.
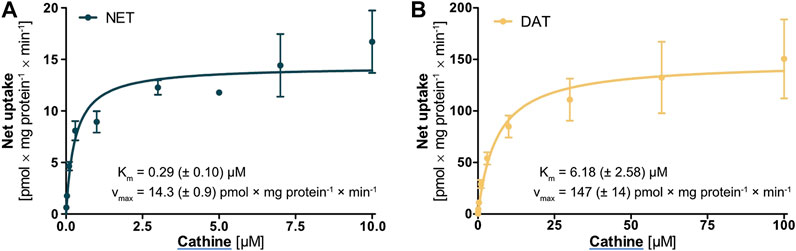
FIGURE 6. Transport of cathine at different concentrations by (A) NET and (B) DAT, shown as the cellular uptake in transporter-overexpressing cells with substracted uptake in non-overexpressing control cells. Shown are the mean values of ≥3 independent experiments ±SEM.
Discussion
In this study, three groups of psychostimulants and hallucinogens (14 phenylethylamine derivatives, the tropanes cocaine and methylecgonine, and the substituted tryptamines dimethyl- and diethyltryptamine) were assessed for their substrate properties for OCTs as well as for high-affinity monoamine transporters. OCTs are known to have a very broad substrate profile that comprises many different structural classes. It is therefore surprising that only relatively few of the 18 psychoactive compounds studied here were moderate or good OCT substrates, especially because these were selected based on physicochemical properties that were in accordance with those of typical OCT substrates. Other transporters, such as OCTN1 and OCTN2, the proposed H+-organic cation antiporter, or ATP-binding cassette efflux transporters might potentially be more relevant for some of the tested psychoactive compounds.
Only mescaline was transported significantly at 1 µM by OCT1, and that this was the highest transport activity observed here altogether. With a pKa of 9.77, a logDpH 7.4 of -1.37, and a molecular mass of 211.3 g/mol, its physicochemical properties are not significantly different from those of the other compounds (Table 1). It is thus reasonable to wonder what properties make mescaline the only substrate at this concentration compared to the 17 other compounds studied here. Possible explanations are not evident from its chemical structure, as it is an amphetamine derivative structurally relatively similar to many of the other phenylethylamines.
Mescaline is an alkaloid biosynthesised from tyrosine in different cacti, where it is found at concentrations of 0.05–4.7% by dry weight (Ogunbodede et al., 2010). Lophophora williamsii (peyote cactus) and several Echinopsis species (e.g., Echinopsis pachanoi and Echinopsis peruvianus, also known as the San Pedro and the Peruvian torch cacti) have a long-standing use in religious ceremonies and traditional medicine of South American indigenous populations. The hallucinogenic effects of these cacti were attributed to their relatively high mescaline contents (Ogunbodede et al., 2010; Dinis-Oliveira et al., 2019; da Silveira Agostini-Costa, 2020). Interestingly, OCT1 deficiency or reduced activity is more frequently found in Central and South American populations than in most other parts of the world and the prevalence of inactive alleles generally increases further south on the American continent (Figure 7) (Seitz et al., 2015). It is likely that OCT1 deficiency was somehow advantageous, e.g., in connection with dietary ingredients that are OCT1 substrates (or perhaps mescaline?), and inactive alleles thus dominated as the first human inhabitants of the continent migrated south.
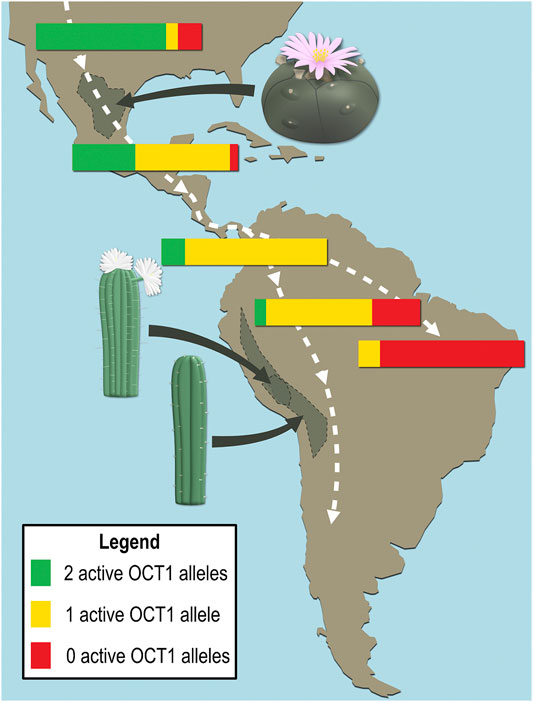
FIGURE 7. Schematic representation of the frequency distributions of active and inactive OCT1 alleles in local populations and natural habitats of the high mescaline-containing cacti Lophophora williamsii (peyote), Echinopsis pachanoi (San Pedro), and Echinopsis peruvianus (Peruvian torch). The white dashed lines broadly illustrate the migration pattern during the first population of the continent by humans.
Typical mescaline dosages are in the range of 170–400 mg, which induce a psychedelic state that may involve visual hallucinations, altered perception, synesthaesia, and euphoria. The lifetime prevalence of mescaline use over the past 3 decades was estimated to be between 3–4% in the United States (Dinis-Oliveira et al., 2019; Johnson et al., 2019). Being a high-affinity partial agonist for the 5-HT2A receptor, potential therapeutic uses for mescaline were proposed for disorders associated with serotonin deficiency, such as addiction, anxiety, and depression (Kyzar et al., 2017; Johnson et al., 2019). Based on the key finding of this study, that mescaline is a strong substrate of the genetically highly polymorphic OCT1, large interindividual variations in mescaline pharmacokinetics might be possible. This could lead to intoxication and other adverse effects due to decreased elimination in carriers of alleles with reduced or absent OCT1 activity (e.g., OCT1 variants *2 to *6, which are particularly common in European populations, or OCT1*7 that is frequently found in Africans and Afro-Americans (Seitz et al., 2015)). However, a substance being identified as OCT1 substrate in vitro may not necessarily be affected by OCT1 genetic polymorphism in vivo, as illustrated by the example of the indirect sympathomimetic compound tyramine (Rafehi et al., 2019). Thus, the effects of OCT1 genotype on mescaline should be studied in vivo and its clinical implications taken into consideration when developing therapeutic interventions involving mescaline.
Another key result of this study was that methamphetamine, PMMA, (-)-ephedrine, cathine, and DMT were substrates of OCT2 and that their transport was moderately reduced in the A270S variant. OCT2 is strongly expressed in the kidneys, where it contributes to transepithelial transport of usually hydrophilic substances and thereby renal elimination. Cathine was excreted unchanged in urine to 46–65% in four healthy volunteers and the renal elimination was reported to be 70% for (-)-ephedrine and 30–54% for methamphetamine (Toennes and Kauert, 2002; www.dosing.de and www.drugbank.ca, both accessed on September 16, 2020). The reduced transport by the A270S variant of OCT2 might thus possibly result in a decreased elimination of these compounds. Besides variation due to inherited polymorphisms, variation in renal elimination of these psychostimulants may additionally arise from drug-drug interactions or conditions associated with increased blood concentrations of endogenous organic cations. DMT, on the other hand, is extensively metabolised and excreted unchanged in urine only to a very low extent (e.g., 0.16% following intramuscular administration) (Sitaram et al., 1987; Barker, 2018). OCT2 polymorphism is thus unlikely to have any significant effects on DMT pharmacokinetics but might still influence local concentrations of DMT as well as of methamphetamine, PMMA, (-)-ephedrine, and cathine in the central nervous system due to OCT2 expression in postsynaptic neurons.
OCT1 and OCT2 polymorphism is not the only form of genetic variation that may affect the above-mentioned compounds. Metabolising enzymes and target receptors may also be polymorphic. A few examples regarding the pharmacogenetics of these compounds are given in Table 3. A good example for discussing the general importance of genetic polymorphism is MDMA, as this psychostimulant has been studied in greater detail. MDMA is widely used as the recreational drug “ecstasy” but therapeutic use for the treatment of posttraumatic stress disorder has also been proposed (Mithoefer et al., 2011; Mithoefer et al., 2013; Amoroso and Workman, 2016; Mithoefer et al., 2016). It is a substrate of the polymorphic enzymes cytochrome P450 (CYP) 2C19, 2B6, and 1A2, which catalyze the conversion to 3,4-methylenedioxyamphetamine. Carriers of genetic variants that result in increased activity of these enzymes showed higher metabolism and CYP2C19 poor metabolisers had greater cardiovascular effects in response to MDMA consumption (Schindler et al., 2014; Vizeli et al., 2017). Poor metabolisers for the highly polymorphic CYP2D6 also showed higher cardiovascular responses, but only to a minor extent due to the inhibition of CYP2D6 (Schmid et al., 2016). Based on in vitro data, the effect of CYP2D6 polymorphism was previously predicted to be higher (La Torre et al., 2012). MDMA has a basic secondary amine group that is protonated to 99.8% at physiological pH (Table 1). It would thus require a transport mechanism for efficient passage across cell membranes and into hepatocytes for metabolism. Our results suggest that OCTs only contribute to a minor extent. Although MDMA is not a good OCT substrate, its metabolites might possibly be (as we had previously shown analogously for different opioids, where their more hydrophilic metabolites were better OCT substrates (Meyer et al., 2019)). For example, the main metabolites 3,4-dihydroxyamphetamine and 3,4-dihydroxymethamphetamine are more hydrophilic than MDMA and might thus potentially be better OCT substrates, as they would likely rely more strongly on transport mechanisms to traverse cell membranes. However, the present study has shown that substrate specificity cannot always be predicted based on physicochemical properties alone. Although a number of contributors to the serotonergic system are polymorphic, significant variation in MDMA effects were not seen in healthy humans (Vizeli et al., 2019). NET polymorphism also showed only minor effects on the cardiovascular response to MDMA in clinical studies (Vizeli et al., 2018). To summarise this, genetic polymorphism significantly determines the pharmacokinetics but not so much the pharmacodynamics of MDMA (and possibly of other psychostimulants as well).
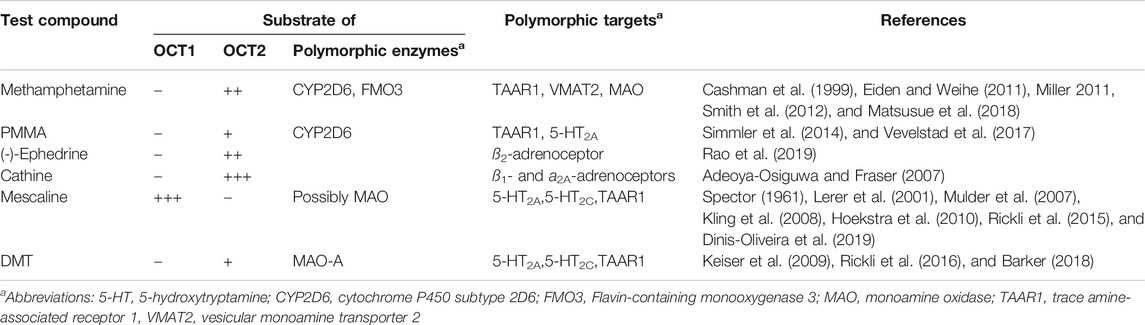
TABLE 3. Pharmacogenetics of methamphetamine, PMMA, (-)-ephedrine, cathine, mescaline, and DMT (this list is not exhaustive).
A concept that has so far not received much attention is stereoselectivity in membrane transport. Recent results from our laboratory have shown that transmembrane transport of adrenergic drugs by OCTs can show strong enantiospecificity (Jensen et al., 2020). The phenylethylamine derivatives cathine (also referred to as (+)-norpseudoephedrine) and (-)-ephedrine that were assessed in this study are chiral compounds and structurally very closely related. If it were not for the methyl substitution at the amino group (Figure 1), both compounds would be stereoisomers of one another. With this in mind, it appears astonishing that cathine was found to be a good substrate of DAT whereas (-)-ephedrine was not, despite their close structural resemblance. Whether this difference in transport was due to the opposite steric orientation of the hydroxyl group or due to the methyl substitution at the amino group cannot be deduced from this study.
To summarise, this study has shown that the classic hallucinogen mescaline is a strong substrate of the genetically highly polymorphic OCT1 (Figure 8) and that genetic variants show altered cell uptake, which may have clinical implications. It was also found that the psychoactive compounds methamphetamine, PMMA, (-)-ephedrine, cathine, and DMT are substrates of OCT2 with partially moderate reductions in cell uptake in the A270S variant. Cathine was also discovered to be a substrate of NET and DAT. As to the question of whether OCT1 is a drug trafficker or not, we would argue that it is one indeed. However, it is a very selective one with a clear preference for the hallucinogenic compound mescaline, which is rather unusual for OCT1 given its generally broad substrate profile.
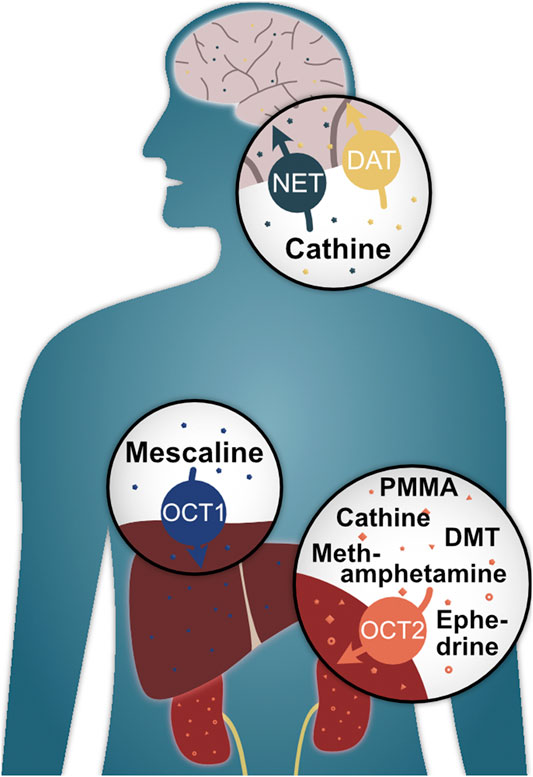
FIGURE 8. Illustrated summary of key findings of this study and their potential biological relevance.
Data Availability Statement
The raw data supporting the conclusions of this article will be made available by the authors, without undue reservation.
Author Contributions
Conceptualisation: OJ, MR, and JB; Funding acquisition: MR and JB; Investigation: OJ and LG; Methodology: OJ and JB; Project administration: OJ, MR, and JB; Supervision: JB; Visualisation: OJ and MR; Writing – original draft: OJ and MR; Writing – review and editing: MR and JB.
Funding
Funded in part by the Deutsche Forschungsgemeinschaft (DFG, German Research Foundation) – Projektnummer 437446827, the research program of the University Medical Center, University of Göttingen, and the Open Access Publication Funds of the University of Göttingen.
Conflict of Interest
The authors declare that the research was conducted in the absence of any commercial or financial relationships that could be construed as a potential conflict of interest.
Acknowledgments
We are grateful to Ellen Bruns for her contributions to the mass spectrometric analyses and to Karoline Jobst for assistance with the transport experiments.
Supplementary Material
The Supplementary Material for this article can be found online at: https://www.frontiersin.org/articles/10.3389/fphar.2020.609811/full#supplementary-material.
References
Adeoya-Osiguwa, S. A., and Fraser, L. R. (2007). Cathine, an amphetamine-related compound, acts on mammalian spermatozoa via beta1- and alpha2A-adrenergic receptors in a capacitation state-dependent manner. Hum. Reprod. 22, 756–765. doi:10.1093/humrep/del454
Amoroso, T., and Workman, M. (2016). Treating posttraumatic stress disorder with MDMA-assisted psychotherapy: a preliminary meta-analysis and comparison to prolonged exposure therapy. J. Psychopharmacol. 30, 595–600. doi:10.1177/0269881116642542
Bacq, A., Balasse, L., Biala, G., Guiard, B., Gardier, A. M., Schinkel, A., et al. (2012). Organic cation transporter 2 controls brain norepinephrine and serotonin clearance and antidepressant response. Mol. Psychiatr. 17, 926–939. doi:10.1038/mp.2011.87
Barker, S. A. (2018). N, N-dimethyltryptamine (DMT), an endogenous hallucinogen: past, present, and future research to determine its role and function. Front. Neurosci. 12, 536. doi:10.3389/fnins.2018.00536
Busch, A. E., Karbach, U., Miska, D., Gorboulev, V., Akhoundova, A., Volk, C., et al. (1998). Human neurons express the polyspecific cation transporter hOCT2, which translocates monoamine neurotransmitters, amantadine, and memantine. Mol. Pharmacol. 54, 342–352. doi:10.1124/mol.54.2.342
Cashman, J. R., Xiong, Y. N., Xu, L., and Janowsky, A. (1999). N-oxygenation of amphetamine and methamphetamine by the human flavin-containing monooxygenase (form 3): role in bioactivation and detoxication. J. Pharmacol. Exp. Therapeut. 288, 1251–1260.
Chen, J., Brockmöller, J., Seitz, T., König, J., Tzvetkov, M. V., and Chen, X. (2017). Erratum to: tropane alkaloids as substrates and inhibitors of human organic cation transporters of the SLC22 (OCT) and the SLC47 (MATE) families. Biol. Chem. 398, 813–249. doi:10.1515/hsz-2016-0236
Couroussé, T., and Gautron, S. (2015). Role of organic cation transporters (OCTs) in the brain. Pharmacol. Therapeut. 146, 94–103. doi:10.1016/j.pharmthera.2014.09.008
da Silveira Agostini-Costa, T. (2020). Bioactive compounds and health benefits of Pereskioideae and Cactoideae: a review. Food Chem. 327, 126961. doi:10.1016/j.foodchem.2020.126961
Dinis-Oliveira, R. J., Pereira, C. L., and da Silva, D. D. (2019). Pharmacokinetic and pharmacodynamic aspects of peyote and mescaline: clinical and forensic repercussions. Curr. Mol. Pharmacol. 12, 184–194. doi:10.2174/1874467211666181010154139
Eiden, L. E., and Weihe, E. (2011). VMAT2: a dynamic regulator of brain monoaminergic neuronal function interacting with drugs of abuse. Ann. N. Y. Acad. Sci. 1216, 86–98. doi:10.1111/j.1749-6632.2010.05906.x
Gründemann, D., Köster, S., Kiefer, N., Breidert, T., Engelhardt, M., Spitzenberger, F., et al. (1998). Transport of monoamine transmitters by the organic cation transporter type 2, OCT2. J. Biol. Chem. 273, 30915–30920. doi:10.1074/jbc.273.47.30915
Hoekstra, P. J., Troost, P. W., Lahuis, B. E., Mulder, H., Mulder, E. J., Franke, B., et al. (2010). Risperidone-induced weight gain in referred children with autism spectrum disorders is associated with a common polymorphism in the 5-hydroxytryptamine 2C receptor gene. J. Child Adolesc. Psychopharmacol. 20, 473–477. doi:10.1089/cap.2009.0071
Jensen, O., Rafehi, M., Tzvetkov, M. V., and Brockmöller, J. (2020). Stereoselective cell uptake of adrenergic agonists and antagonists by organic cation transporters. Biochem. Pharmacol. 171, 113731. doi:10.1016/j.bcp.2019.113731
Johnson, M. W., Hendricks, P. S., Barrett, F. S., and Griffiths, R. R. (2019). Classic psychedelics: an integrative review of epidemiology, therapeutics, mystical experience, and brain network function. Pharmacol. Therapeut. 197, 83–102. doi:10.1016/j.pharmthera.2018.11.010
Kalix, P., and Braenden, O. (1985). Pharmacological aspects of the chewing of khat leaves. Pharmacol. Rev. 37, 149–164.
Keiser, M. J., Setola, V., Irwin, J. J., Laggner, C., Abbas, A. I., Hufeisen, S. J., et al. (2009). Predicting new molecular targets for known drugs. Nature. 462, 175–181. doi:10.1038/nature08506
Kling, A., Seddighzadeh, M., Arlestig, L., Alfredsson, L., Rantapää-Dahlqvist, S., and Padyukov, L. (2008). Genetic variations in the serotonin 5-HT2A receptor gene (HTR2A) are associated with rheumatoid arthritis. Ann. Rheum. Dis. 67, 1111–1115. doi:10.1136/ard.2007.074948
Koepsell, H. (2020). Organic cation transporters in health and disease. Pharmacol. Rev. 72, 253–319. doi:10.1124/pr.118.015578
Koepsell, H., Lips, K., and Volk, C. (2007). Polyspecific organic cation transporters: structure, function, physiological roles, and biopharmaceutical implications. Pharm. Res. 24, 1227–1251. doi:10.1007/s11095-007-9254-z
Kyzar, E. J., Nichols, C. D., Gainetdinov, R. R., Nichols, D. E., and Kalueff, A. V. (2017). Psychedelic drugs in biomedicine. Trends Pharmacol. Sci. 38, 992–1005. doi:10.1016/j.tips.2017.08.003
La Torre, R. de., Yubero-Lahoz, S., Pardo-Lozano, R., and Farré, M. (2012). MDMA, methamphetamine, and CYP2D6 pharmacogenetics: what is clinically relevant? Front. Genet. 3, 235. doi:10.3389/fgene.2012.00235
Lerer, B., Macciardi, F., Segman, R. H., Adolfsson, R., Blackwood, D., Blairy, S., et al. (2001). Variability of 5-HT2C receptor cys23ser polymorphism among European populations and vulnerability to affective disorder. Mol. Psychiatr. 6, 579–585. doi:10.1038/sj.mp.4000883
Luethi, D., and Liechti, M. E. (2020). Designer drugs: mechanism of action and adverse effects. Arch. Toxicol. 94, 1085–1133. doi:10.1007/s00204-020-02693-7
Matsusue, A., Ikeda, T., Tani, N., Waters, B., Hara, K., Kashiwagi, M., et al. (2018). Association between cytochrome P450 2D6 polymorphisms and body fluid methamphetamine concentrations in Japanese forensic autopsy cases. Forensic Sci. Int. 289, 33–39. doi:10.1016/j.forsciint.2018.05.018
Matthaei, J., Kuron, D., Faltraco, F., Knoch, T., Dos Santos Pereira, J. N., Abu Abed, M., et al. (2016). OCT1 mediates hepatic uptake of sumatriptan and loss-of-function OCT1 polymorphisms affect sumatriptan pharmacokinetics. Clin. Pharmacol. Ther. 99, 633–641. doi:10.1002/cpt.317
Matthaei, J., Seitz, T., Jensen, O., Tann, A., Prukop, T., Tadjerpisheh, S., et al. (2019). OCT1 deficiency affects hepatocellular concentrations and pharmacokinetics of cycloguanil, the active metabolite of the antimalarial drug proguanil. Clin. Pharmacol. Ther. 105, 190–200. doi:10.1002/cpt.1128
Mayer, F. P., Schmid, D., Holy, M., Daws, L. C., and Sitte, H. H. (2019). “Polytox” synthetic cathinone abuse: a potential role for organic cation transporter 3 in combined cathinone-induced efflux. Neurochem. Int. 123, 7–12. doi:10.1016/j.neuint.2018.09.008
Mayer, F. P., Schmid, D., Owens, W. A., Gould, G. G., Apuschkin, M., Kudlacek, O., et al. (2018). An unsuspected role for organic cation transporter 3 in the actions of amphetamine, Neuropsychopharmacology. 43, 2408–2417. doi:10.1038/s41386-018-0053-5
Meyer, M. J., Neumann, V. E., Friesacher, H. R., Zdrazil, B., Brockmöller, J., and Tzvetkov, M. V. (2019). Opioids as substrates and inhibitors of the genetically highly variable organic cation transporter OCT1. J. Med. Chem. 62, 9890–9905. doi:10.1021/acs.jmedchem.9b01301
Miller, G. M. (2011). The emerging role of trace amine-associated receptor 1 in the functional regulation of monoamine transporters and dopaminergic activity. J. Neurochem. 116, 164–176. doi:10.1111/j.1471-4159.2010.07109.x
Mithoefer, M. C., Grob, C. S., and Brewerton, T. D. (2016). Novel psychopharmacological therapies for psychiatric disorders: psilocybin and MDMA. Lancet Psychiatry. 3 (5), 481–488. doi:10.1016/S2215-0366(15)00576-3
Mithoefer, M. C., Wagner, M. T., Mithoefer, A. T., Jerome, L., and Doblin, R. (2011). The safety and efficacy of {+/-}3,4-methylenedioxymethamphetamine-assisted psychotherapy in subjects with chronic, treatment-resistant posttraumatic stress disorder: the first randomized controlled pilot study. J. Psychopharmacol. 25, 439–452. doi:10.1177/0269881110378371
Mithoefer, M. C., Wagner, M. T., Mithoefer, A. T., Jerome, L., Martin, S. F., Yazar-Klosinski, B., et al. (2013). Durability of improvement in post-traumatic stress disorder symptoms and absence of harmful effects or drug dependency after 3,4-methylenedioxymethamphetamine-assisted psychotherapy: a prospective long-term follow-up study. J. Psychopharmacol. 27, 28–39. doi:10.1177/0269881112456611
Motohashi, H., Nakao, Y., Masuda, S., Katsura, T., Kamba, T., Ogawa, O., et al. (2013). Precise comparison of protein localization among OCT, OAT, and MATE in human kidney. J. Pharmacol. Sci. 102, 3302–3308. doi:10.1002/jps.23567
Motohashi, H., Sakurai, Y., Saito, H., Masuda, S., Urakami, Y., Goto, M., et al. (2002). Gene expression levels and immunolocalization of organic ion transporters in the human kidney. J. Am. Soc. Nephrol. 13, 866–874.
Mulder, H., Franke, B., van der-Beek van der, A. A., Arends, J., Wilmink, F. W., Scheffer, H., et al. (2007). The association between HTR2C gene polymorphisms and the metabolic syndrome in patients with schizophrenia. J. Clin. Psychopharmacol. 27, 338–343. doi:10.1097/JCP.0b013e3180a76dc0
Nies, A. T., Koepsell, H., Winter, S., Burk, O., Klein, K., Kerb, R., et al. (2009). Expression of organic cation transporters OCT1 (SLC22A1) and OCT3 (SLC22A3) is affected by genetic factors and cholestasis in human liver. Hepatology. 50, 1227–1240. doi:10.1002/hep.23103
Nishimura, M., and Naito, S. (2005). Tissue-specific mRNA expression profiles of human ATP-binding cassette and solute carrier transporter superfamilies. Drug Metabol. Pharmacokinet. 20, 452–477. doi:10.2133/dmpk.20.452
Ogunbodede, O., McCombs, D., Trout, K., Daley, P., and Terry, M. (2010). New mescaline concentrations from 14 taxa/cultivars of Echinopsis spp. (Cactaceae) (“San Pedro”) and their relevance to shamanic practice. J. Ethnopharmacol. 131, 356–362. doi:10.1016/j.jep.2010.07.021
Rafehi, M., Faltraco, F., Matthaei, J., Prukop, T., Jensen, O., Grytzmann, A., et al. (2019). Highly variable pharmacokinetics of tyramine in humans and polymorphisms in OCT1, CYP2D6, and MAO-A. Front. Pharmacol. 10, 1297. doi:10.3389/fphar.2019.01297
Ramamoorthy, S., Bauman, A. L., Moore, K. R., Han, H., Yang-Feng, T., Chang, A. S., et al. (1993). Antidepressant- and cocaine-sensitive human serotonin transporter: molecular cloning, expression, and chromosomal localization. Proc. Natl. Acad. Sci. U.S.A. 90, 2542–2546. doi:10.1073/pnas.90.6.2542
Rao, T., Tan, Z., Peng, J., Guo, Y., Chen, Y., Zhou, H., et al. (2019). The pharmacogenetics of natural products: a pharmacokinetic and pharmacodynamic perspective. Pharmacol. Res. 146, 104283. doi:10.1016/j.phrs.2019.104283
Reith, M. E. A., and Gnegy, M. E. (2020). Molecular mechanisms of amphetamines. Handb. Exp. Pharmacol. 258, 265–297. doi:10.1007/164_2019_251
Rickli, A., Luethi, D., Reinisch, J., Buchy, D., Hoener, M. C., and Liechti, M. E. (2015). Receptor interaction profiles of novel N-2-methoxybenzyl (NBOMe) derivatives of 2,5-dimethoxy-substituted phenethylamines (2C drugs). Neuropharmacology. 99, 546–553. doi:10.1016/j.neuropharm.2015.08.034
Rickli, A., Moning, O. D., Hoener, M. C., and Liechti, M. E. (2016). Receptor interaction profiles of novel psychoactive tryptamines compared with classic hallucinogens. Eur. Neuropsychopharmacol. 26, 1327–1337. doi:10.1016/j.euroneuro.2016.05.001
Rothman, R. B., Vu, N., Partilla, J. S., Roth, B. L., Hufeisen, S. J., Compton-Toth, B. A., et al. (2003). In vitro characterization of ephedrine-related stereoisomers at biogenic amine transporters and the receptorome reveals selective actions as norepinephrine transporter substrates. J. Pharmacol. Exp. Therapeut. 307, 138–145. doi:10.1124/jpet.103.053975
Saadatmand, A. R., Tadjerpisheh, S., Brockmöller, J., and Tzvetkov, M. V. (2012). The prototypic pharmacogenetic drug debrisoquine is a substrate of the genetically polymorphic organic cation transporter OCT1. Biochem. Pharmacol. 83, 1427–1434. doi:10.1016/j.bcp.2012.01.032
Schindler, C. W., Thorndike, E. B., Blough, B. E., Tella, S. R., Goldberg, S. R., and Baumann, M. H. (2014). Effects of 3,4-methylenedioxymethamphetamine (MDMA) and its main metabolites on cardiovascular function in conscious rats. Br. J. Pharmacol. 171, 83–91. doi:10.1111/bph.12423
Schmid, Y., Vizeli, P., Hysek, C. M., Prestin, K., Meyer Zu Schwabedissen, H. E., and Liechti, M. E. (2016). CYP2D6 function moderates the pharmacokinetics and pharmacodynamics of 3,4-methylene-dioxymethamphetamine in a controlled study in healthy individuals. Pharmacogenetics Genom. 26, 397–401. doi:10.1097/FPC.0000000000000231
Seitz, T., Stalmann, R., Dalila, N., Chen, J., Pojar, S., Dos Santos Pereira, J. N., et al. (2015). Global genetic analyses reveal strong inter-ethnic variability in the loss of activity of the organic cation transporter OCT1. Genome Med. 7, 56. doi:10.1186/s13073-015-0172-0
Sharma, A., and Couture, J. (2014). A review of the pathophysiology, etiology, and treatment of attention-deficit hyperactivity disorder (ADHD). Ann. Pharmacother. 48, 209–225. doi:10.1177/1060028013510699
Simmler, L. D., Rickli, A., Hoener, M. C., and Liechti, M. E. (2014). Monoamine transporter and receptor interaction profiles of a new series of designer cathinones. Neuropharmacology. 79, 152–160. doi:10.1016/j.neuropharm.2013.11.008
Sitaram, B. R., Lockett, L., Blackman, G. L., and McLeod, W. R. (1987). Urinary excretion of 5-methoxy-N,N-dimethyltryptamine, N,N-dimethyltryptamine and their N-oxides in the rat. Biochem. Pharmacol. 36, 2235–2237. doi:10.1016/0006-2952(87)90159-6
Smith, S. B., Maixner, D. W., Fillingim, R. B., Slade, G., Gracely, R. H., Ambrose, K., et al. (2012). Large candidate gene association study reveals genetic risk factors and therapeutic targets for fibromyalgia. Arthritis Rheum. 64, 584–593. doi:10.1002/art.33338
Spector, E. (1961). Identification of 3,4,5-trimethoxyphenylacetic acid as the major metabolite of mescaline in the dog. Nature. 189, 751–752. doi:10.1038/189751b0
Stamer, U. M., Musshoff, F., Stüber, F., Brockmöller, J., Steffens, M., and Tzvetkov, M. V. (2016). Loss-of-function polymorphisms in the organic cation transporter OCT1 are associated with reduced postoperative tramadol consumption. Pain. 157, 2467–2475. doi:10.1097/j.pain.0000000000000662
Toennes, S. W., and Kauert, G. F. (2002). Excretion and detection of cathinone, cathine, and phenylpropanolamine in urine after kath chewing. Clin. Chem. 48, 1715–1719. doi:10.1093/clinchem/48.10.1715
Torres, G. E., Gainetdinov, R. R., and Caron, M. G. (2003). Plasma membrane monoamine transporters: structure, regulation and function. Nat. Rev. Neurosci. 4, 13–25. doi:10.1038/nrn1008
Tzvetkov, M. V., Dos Santos Pereira, J. N., Meineke, I., Saadatmand, A. R., Stingl, J. C., and Brockmöller, J. (2013). Morphine is a substrate of the organic cation transporter OCT1 and polymorphisms in OCT1 gene affect morphine pharmacokinetics after codeine administration. Biochem. Pharmacol. 86, 666–678. doi:10.1016/j.bcp.2013.06.019
Tzvetkov, M. V., Matthaei, J., Pojar, S., Faltraco, F., Vogler, S., Prukop, T., et al. (2018). Increased systemic exposure and stronger cardiovascular and metabolic adverse reactions to fenoterol in individuals with heritable OCT1 deficiency. Clin. Pharmacol. Ther. 103, 868. doi:10.1002/cpt.812
Tzvetkov, M. V., Saadatmand, A. R., Lötsch, J., Tegeder, I., Stingl, J. C., and Brockmöller, J. (2011). Genetically polymorphic OCT1: another piece in the puzzle of the variable pharmacokinetics and pharmacodynamics of the opioidergic drug tramadol. Clin. Pharmacol. Ther. 90, 143–150. doi:10.1038/clpt.2011.56
Tzvetkov, M. V., Vormfelde, S. V., Balen, D., Meineke, I., Schmidt, T., Sehrt, D., et al. (2009). The effects of genetic polymorphisms in the organic cation transporters OCT1, OCT2, and OCT3 on the renal clearance of metformin. Clin. Pharmacol. Ther. 86, 299–306. doi:10.1038/clpt.2009.92
Venkatasubramanian, R., Fukuda, T., Niu, J., Mizuno, T., Chidambaran, V., Vinks, A. A., et al. (2014). ABCC3 and OCT1 genotypes influence pharmacokinetics of morphine in children. Pharmacogenomics. 15, 1297–1309. doi:10.2217/pgs.14.99
Vevelstad, M., Øiestad, E. L., Nerem, E., Arnestad, M., and Bogen, I. L. (2017). Studies on para-methoxymethamphetamine (PMMA) metabolite pattern and influence of CYP2D6 genetics in human liver microsomes and authentic samples from fatal PMMA intoxications. Drug Metab. Dispos. 45, 1326–1335. doi:10.1124/dmd.117.077263
Vialou, V., Balasse, L., Callebert, J., Launay, J. M., Giros, B., and Gautron, S. (2008). Altered aminergic neurotransmission in the brain of organic cation transporter 3-deficient mice. J. Neurochem. 106, 1471–1482. doi:10.1111/j.1471-4159.2008.05506.x
Vizeli, P., Meyer Zu Schwabedissen, H. E., and Liechti, M. E. (2018). No major role of norepinephrine transporter gene variations in the cardiostimulant effects of MDMA. Eur. J. Clin. Pharmacol. 74, 275–283. doi:10.1007/s00228-017-2392-2
Vizeli, P., Meyer Zu Schwabedissen, H. E., and Liechti, M. E. (2019). Role of serotonin transporter and receptor gene variations in the acute effects of MDMA in healthy subjects. ACS Chem. Neurosci. 10, 3120–3131. doi:10.1021/acschemneuro.8b00590
Vizeli, P., Schmid, Y., Prestin, K., Meyer Zu Schwabedissen, H. E., and Liechti, M. E. (2017). Pharmacogenetics of ecstasy: CYP1A2, CYP2C19, and CYP2B6 polymorphisms moderate pharmacokinetics of MDMA in healthy subjects. Eur. Neuropsychopharmacol. 27, 232–238. doi:10.1016/j.euroneuro.2017.01.008
Wu, X., Kekuda, R., Huang, W., Fei, Y. J., Leibach, F. H., Chen, J., et al. (1998). Identity of the organic cation transporter OCT3 as the extraneuronal monoamine transporter (uptake2) and evidence for the expression of the transporter in the brain. J. Biol. Chem. 273, 32776–32786. doi:10.1074/jbc.273.49.32776
Yee, S. W., Brackman, D. J., Ennis, E. A., Sugiyama, Y., Kamdem, L. K., Blanchard, R., et al. (2018). Influence of transporter polymorphisms on drug disposition and response: a perspective from the international transporter consortium. Clin. Pharmacol. Ther. 104, 803–817. doi:10.1002/cpt.1098
Zhu, H. J., Appel, D. I., Gründemann, D., and Markowitz, J. S. (2010). Interaction of organic cation transporter 3 (SLC22A3) and amphetamine. J. Neurochem. 114, 142–149. doi:10.1111/j.1471-4159.2010.06738.x
Keywords: membrane transport, monoamine transporter, OCT1, organic cation transporter, psychostimulant, SLC22A1, solute carrier, hallucinogen
Citation: Jensen O, Rafehi M, Gebauer L and Brockmöller J (2021) Cellular Uptake of Psychostimulants – Are High- and Low-Affinity Organic Cation Transporters Drug Traffickers?. Front. Pharmacol. 11:609811. doi: 10.3389/fphar.2020.609811
Received: 28 September 2020; Accepted: 09 December 2020;
Published: 20 January 2021.
Edited by:
Petr Pavek, Charles University, CzechiaReviewed by:
Bruno Hagenbuch, University of Kansas Medical Center, United StatesXiaomin Liang, Gilead, United States
Copyright © 2021 Jensen, Rafehi, Gebauer and Brockmöller. This is an open-access article distributed under the terms of the Creative Commons Attribution License (CC BY). The use, distribution or reproduction in other forums is permitted, provided the original author(s) and the copyright owner(s) are credited and that the original publication in this journal is cited, in accordance with accepted academic practice. No use, distribution or reproduction is permitted which does not comply with these terms.
*Correspondence: Ole Jensen, ole.jensen@med.uni-goettingen.de; Muhammad Rafehi, muhammad.rafehi@med.uni-goettingen.de