- 1Department of Herbal Medicine Research, Korea Institute of Oriental Medicine, Daejeon, South Korea
- 2Department of Clinical Research, Korea Institute of Oriental Medicine, Daejeon, South Korea
The complexity of pathological mechanisms in Alzheimer’s disease (AD) poses significant challenges to the development of corresponding drugs. Symptom-specific pharmacological interventions and alternative treatments provide promising treatment possibilities. Therefore, we considered a combination of selegiline (SEL) and electroacupuncture (EA). We used an animal model with AD to investigate the effect of a combination of these treatments on cognitive function. 5XFAD mice received a week of SEL treatment and 2 weeks of EA. Novel object recognition and Y-maze tests were subsequently performed to assess their cognitive functions. To determine the molecular action of the combination treatment, Western blots, Aβ1-42 enzyme-linked immunosorbent assays (ELISA), and micro-positron-emission tomography were also performed to assess pathological markers and processes. The results were assessed based on the difference between untreated transgenic, SEL-treated, and SEL- and EA-treated groups of mice. Mice in the combined treatment group demonstrated significantly better cognitive functions, and lesser neuroinflammation than the comparative groups. In addition, mice treated with a combination of SEL and EA did not demonstrate a direct modulation of insoluble Aβ but demonstrated greater glucose metabolism. Our findings demonstrated that SEL combined with EA treatment was associated with better cognitive functioning due to inhibition of neuroinflammation and increased glucose metabolism relative to the comparative groups in a mouse model with AD.
Introduction
Alzheimer’s disease (AD), which is characterized by age-related neurodegenerative processes that lead to loss of cognitive and memory functions, is associated with neurofibrillary tangles formed by hyperphosphorylated tau and β-amyloid (Aβ) deposition, and neuroinflammation of the cerebral cortex and hippocampus (Choi et al., 2018; Oyama et al., 1993). The neuroinflammation and amyloid plaques observed in AD are found to be associated with activated astrocytes and microglial cells. The latter is associated with proinflammatory factors, and tumor necrosis factor-alpha or interleukin 6 cytokines in serum and brain tissue (Fillit et al., 1991; Strauss et al., 1992). Activated microglia are also found to co-localize with Aβ plaques in the brains of patients with AD (Sastre et al., 2006; Calsolaro and Edison, 2016), and their inflammatory functions are found to be triggered by Aβ precursor protein (β-APP) (Barger and Harmon, 1997). In addition, autophagic dysfunction causes memory impairment due to Aβ accumulation in 5XFAD mice (Álvarez-Arellano et al., 2018). The same study demonstrated inflammasome activation caused by Aβ plaque accumulation due to autophagy impairment and microglial phagocytic activity reduction. There has also been some evidence regarding the involvement of APOE protein in neuroinflammation and glucose metabolism during AD pathogenesis (Jagust and Landau, 2012; Liu et al., 2013).
Despite the identification of these major pathological markers, no approved drugs targeting Aβ or hyperphosphorylated tau have been established, due to the complexity of their mechanisms of action. In contrast, symptom-specific pharmacological treatments involving dopaminergic drugs have been better researched and used AD treatment. This is premised on the fact that patients with AD demonstrate dysregulations in the dopaminergic system, including the reduction of dopamine and its receptors (Gibb et al., 1989; Storga et al., 1996; Burns et al., 2005), and the progressive dopaminergic neuron loss contributes to memory impairments and synaptic plasticity (Nobili et al., 2017). To this effect, Selegiline (SEL; L-deprenyl), a monoamine oxidase (MAO) inhibitor used for Parkinson’s disease, has been trialed for the treatment of memory dysfunction in AD. While it has been found to improve synaptic plasticity and learning and memory by inhibiting GABA production from reactive astrocytes in AD mice (Jo et al., 2014), there is no evidence supporting its long-term efficacy for cognitive improvement (Piccinin et al., 1990; Mangoni et al., 1991; Sano et al., 1997).
In addition, research has demonstrated that non-drug therapy is helpful in maintaining and even improving cognition among patients with AD (DeVreese et al., 1996; Kukull and Ganguli, 2000; Dysken et al., 2014). In this regard, complementary and alternative medicine therapies such as electroacupuncture (EA) have provided a promising avenue for the treatment of neuronal diseases (No authors listed 1998; Li et al., 2014), with improvements in cognitive function noted among patients with AD (Jia et al., 2017; Shao et al., 2019) and reductions in neuroinflammation and oxidative processes observed in animal studies (Yang et al., 2016; Cai et al., 2019). Specifically, cognitive improvements following acupuncture were found to be associated with increased synaptic transmission, activation of α7 nicotinic acetylcholine receptors (α7nAChR) and the cholinergic anti-inflammatory pathway, and reduction of oxidative damage in the hippocampus, in AD models (Zhang et al., 2010; Liu et al., 2011; Yang et al., 2011; Han et al., 2018). In addition, memory improvements have been shown to correspond with decreased Aβ plaques in the hippocampus of human amyloid precursor protein and presenilin-1 positive (APP+/PS1+) double transgenic mice that demonstrated memory improvements following EA (Yang et al., 2019).
Despite these advances, the combined effects of EA and psychopharmacology have not been studied. Therefore, we examined whether SEL combined with EA treatment improved cognitive function, in a 5XFAD animal model study. We also evaluated if the combined treatment resulted in lesser neuroinflammatory indicators, increased anti-oxidative effects, and lesser incidence of autophagic markers compared to comparison groups.
Materials and Methods
Procedure
The entire study was approved by the Institutional Animal Care and Use Committee (IACUC) of the Korea Institute of Oriental Medicine, and KIOM animal care guidelines were followed. This study involved 29 of female 5XFAD mice that were randomly allocated to three groups, namely 5XFAD transgenic mice (Tg), 5XFAD transgenic mice treated with SEL (Tg + SEL), and 5XFAD transgenic mice treated with a combination of SEL and EA (Tg + SEL + EA). The treatment period commenced when the mice were 6.5 months old. The experimental timeline is shown in Figure 1. The mice in all groups underwent the novel object recognition (NOR) and Y-maze tests following treatment, to evaluate their effect on cognitive function. The NOR probe trial and the Y-maze test were conducted the next day after the last administration of the EA and SEL treatments, based on the protocol followed in a previous study (Yang et al., 2016). All instruments used during these tests were cleaned with 70% ethanol between trials to reduce artifacts in the experiment after each test. Following cognitive testing, three to four prefrontal cortices and hippocampi were each randomly selected from each group to evaluate the treatment effects on neuroinflammation. Thus, the expression levels of microglia, astrocyte, and neuroinflammatory protein COX2 were examined in these brain regions using Western blots. Next, we investigated the anti-oxidative effect of SEL combined with EA treatment. Finally, microPET imaging was used to investigate glucose metabolic activity by SEL combined with EA treatment across groups.
Animals
5XFAD mice overexpressing APP transgenes with Swedish (K670N, M671L), Florida (I716V), and London (V717I) mutations, and PS1 mutations M146L and L286V were obtained from the Jackson Laboratory (Bar Harbor, ME, United States) maintained in the KIOM. 5XFAD males and B6SJ females were mated and transgenic offspring were selected by genotyping with polymerase chain reaction analysis (Oakley et al., 2006; Jeon et al., 2019). All animals were maintained in a specific pathogen-free environment with a temperature of 21 ± 3°C and humidity of 50 ± 10% with a 12-h light/dark cycle (light on 07:00–19:00 h). Access to water and food was ad libitum.
Selegiline and Electroacupuncture Treatment
The treatment protocol included 10 mg/kg SEL that was administered orally daily for a week and EA stimulation (1 mA, 2 Hz) at the Taegye (KI3) acupoint provided for 15 min, 3 times a week for 2 weeks (Figure 1). The KI3 acupoint is located on the medial aspect of the foot, between the medial malleolus and the Achilles tendon (Lu et al., 2017). Acupuncture needles (diameter 0.18 mm, length 8 mm) were purchased from Dongbang Medical (Boryeong, Korea). The EA treatment was performed according to the procedure described by Cai et al. (2019).
Novel Object Recognition Test
The NOR test was performed as previously described (Yang et al., 2016). NOR test was performed over 3 days, with the habituation stage taking place in the first day. This was followed by the training and probe stages, which were performed sequentially for 1 day each. Habituation of the mice to the arena took place for 10 min/day in a black polyvinyl plastic test box (40 × 40 × 40 cm) without any objects. During the training stage, the mice were put in the same box and exposed to two identical objects located at the edges of the box diagonally, and the mice were permitted to freely explore the objects for 5 min. All goods were cleaned using a paper towels and 70% ethanol to remove odors between each test. In the probe test 24 h after the training, the mice were put in the same apparatus with one original object and the other exchanged for a new one with a different shape but similar texture. The mice were allowed to explore for 5 min. Objects were randomized between trials. The exploration time was recorded using a video camera-based Ethovision system (Noldus, Netherlands), and the percentage of exploration time of the novel object or familiar object was calculated using the following equation:
Y-Maze Test
The Y-maze apparatus was 40 cm long, 3 cm wide, and 15-cm high, with arms positioned at 120° angles from each other. All animals were placed in the maze’s center and were allowed to explore freely. The number of arm entries and triads were recorded for 8 min, and the calculation used to determine the percentage of alternation, as shown below, was based on that reported by Cai et al. (2019). A spontaneous alternation was defined as entry into three different arms consecutively (i.e., ABC, CAB, or BCA, but not ABA, BCB, etc.). The apparatus arms were cleaned using 70% ethanol and dried after each test. The percentage of spontaneous alternation was calculated as
Western Blots
The selected prefrontal cortical and hippocampal samples were homogenized using a radio immunoprecipitation assay buffer (Biosesang, Gyeonggi-do, Korea) containing phosphatase and proteinase inhibitors (Thermo Fisher Scientific, Waltham, MA, United States). The protein concentration was quantified using the Bicinchoninic Acid Assay Kit (Pierce, Rockford, IL, United States) and separated by 4–12% Bis-Tris Plus gels (Thermo Fisher Scientific). The equivalent 20 μg protein was loaded on Bolt 4–12% Bis-Tris Plus gels (Thermo Fisher Scientific). The membranes were blocked with 5% skimmed milk at room temperature and incubated with primary antibodies overnight at 4°C. Primary antibodies included 1:1,000 cluster of differentiation 11B (CD11B), heme oxygenase (HO)-1, and COX2 (Abcam, Cambridge, MA, United States); B cell lymphoma-2-associated X protein (Bax), transferrin (Santa Cruz Biotechnology, Dallas, TX, United States); APP, apolipoprotein E (APOE; Merck Millipore, Burlington, MA, United States); and 1:5,000 glial fibrillary acidic protein (GFAP; Agilent Technologies, Santa Clara, CA, United States). Further, 1:1,000 tubulin (Abcam, Cambridge, MA, United States) was used as a loading control for all immunoblots. The membranes were incubated with HRP-conjugated secondary antibodies (Santa Cruz Biotechnology, Santa Cruz, CA, United States). The protein bands were visualized with enhanced chemiluminescence and imaged using a ChemiDoc Imaging System device (Bio-Rad, Hercules, CA, United States). Quantification of immunoblots were normalized to tubulin and calculated using ImageJ (version 1.46j; National Institutes of Health, Bethesda, MD, United States).
Aβ1-42 Enzyme-Linked Immunosorbent Assays
Insoluble Aβ1-42 was elicited from all mice from each group and measured by a beta-amyloid 1-42 quantitative ELISA kit according to the manufacturer's instructions (Anaspec Inc., Fremont, CA, United States). Prefrontal cortex homogenates were briefly diluted to a 1:10,000 ratio in a sample dilution buffer and evaluated according to the manufacturer’s protocol. The amount of insoluble Aβ1-42 was calculated using Aβ (1-42) protein standards.
MicroPET Scanning
MicroPET imaging was performed at the Osong Medical Innovation Foundation (Osong-eup, Chung Buk, South Korea) according to the protocol used by Cai et al. (2019). An animal positron-emission tomography (PET) scanner (Nano PET/CT, Mediso, Hungary) was used for imaging of mice under inhalation anesthesia (1.5–2.0% of isoflurane in 1.0–1.5 L/min of oxygen). For the quantitative analysis, regions of interest (ROIs) were analyzed in the frontal cortex regions using PMOD 3.8 software (PMOD Technologies LLC., Zurich, Switzerland). Subsequently, the regional uptake was analyzed as mean standardized uptake value (SUV) using the following formula for the quantitative comparison of 18F-FDG uptake.
Statistical Analysis
The elicited data were analyzed using one-way analyses of variance (ANOVA) followed by the Newman-Keuls post hoc test for multiple comparisons or the Kruskal-Wallis test. This was followed by the Dunn’s test, performed using Prism v.5.0 (GraphPad, La Jolla, CA, United States). The statistical significance levels were set at p < 0.05.
Results
Combination Treatment With Selegiline and Electroacupuncture Increases Cognitive Function in 5XFAD Mice
SEL (10 mg/kg) was administered orally once daily for 1 week and EA treatment at KI3 was performed in 6.5-month-old 5XFAD mice three times per week for 2 weeks (Figure 1). To evaluate the effect of SEL combined with EA treatment on cognitive function, NOR and Y-maze tests were performed with 5XFAD mice. In the Y-maze task, the spontaneous alternation of 5XFAD mice and SEL-treated 5XFAD mice was significantly reduced by 1.3-fold compared to that of non-transgenic mice (Figure 2A). However, the combined treatment group demonstrated a 1.2-fold increase of spontaneous alternation compared to those in the transgenic group that did not receive any treatment and SEL-treated 5XFAD mice (Tg + SEL) (Figure 2A). The number of total entry was not significant between groups. In addition, exploration time of novel objects in the NOR test was 1.6-fold less in the 5XFAD mice than in the non-transgenic group (Figure 2B). However, exploration time of the novel object in SEL-treated 5XFAD mice (Tg + SEL) and combined treatment group (Tg + SEL + EA) were 1.4-and 1.5-fold greater than in the no treatment group (Tg) (Figure 2B). Consistent with this result, the exploration time of familiar objects of the 5XFAD mice was increased by 1.8-fold compared to the non-transgenic group (Figure 2B). The SEL-treated 5XFAD mice (Tg + SEL) and combined treatment group (Tg + SEL + EA) were significantly reduced by 1.6-and 1.4-fold compared to the transgenic group (Figure 2B). Total exploration times were not significant between groups.
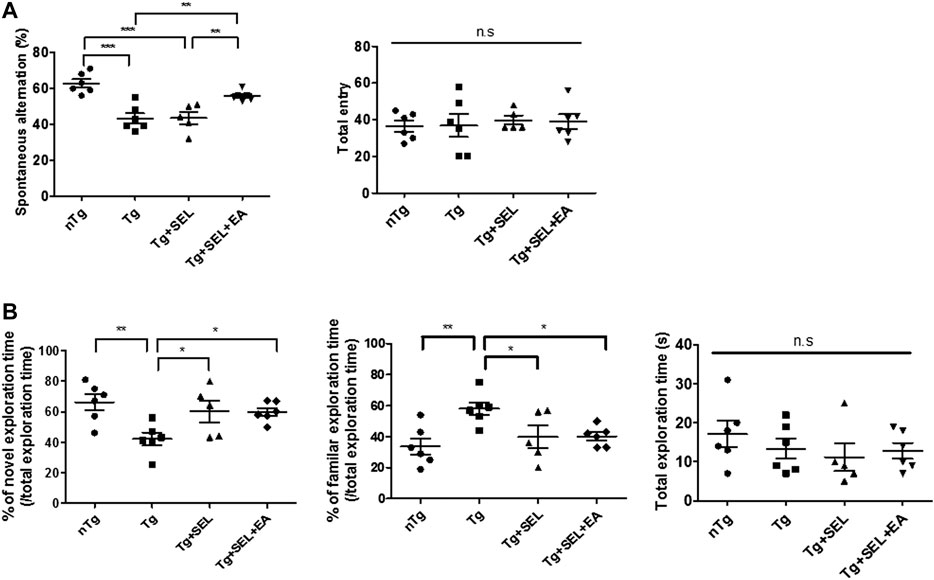
FIGURE 2. Means ± SEM of the results of post-treatment cognitive tests in 5XFAD mice. (A) Spontaneous alternations and total entry during the Y maze test (n = 5–6/group). (B) Percentage of exploration time of the novel or familiar object and total exploration time during the novel object recognition (NOR) test (n = 5–6/group). *p < 0.05; **p < 0.01; SEM, standard error of mean; nTg, non-transgenic mice group; Tg, 5XFAD mice group; Tg + SEL, selegiline-treated 5XFAD mice group; Tg + SEL + EA, 5XFAD mice group treated with selegiline combined with electroacupuncture; n.s, not significant.
Combination Treatment With Selegiline and Electroacupuncture Reduces Neuroinflammation in 5XFAD Mice
In AD, microglia and astrocyte activation are critical factors causing cognitive dysfunction (Fakhoury, 2018). To evaluate the effect of SEL combined with EA treatment on neuroinflammation, we examined the expression level of microglia, astrocyte, and neuroinflammatory protein, COX2, in the hippocampus and prefrontal cortex of 5XFAD mice. As shown in Figure 3A, the expression levels of CD11B (for microglia) and GFAP (for astrocyte) were not significantly reduced by SEL or SEL combined with EA treatment in the hippocampus of 5XFAD mice in comparison with those in their transgenic counterparts. Though COX2 and GFAP expression levels were lower among mice treated with SEL combined with EA than transgenic mice, these differences were not significant (Figures 3A,B). SEL combined with EA treatment decreased the expression levels of CD11B and GFAP in the prefrontal cortices of 5XFAD mice compared to those in Tg mice (Figures 3C,D). Furthermore, SEL combined with EA treatment reduced the expression levels of COX2 by 1.7-fold in the prefrontal cortices of 5XFAD mice in comparison with those in Tg mice (Figures 3C,D).
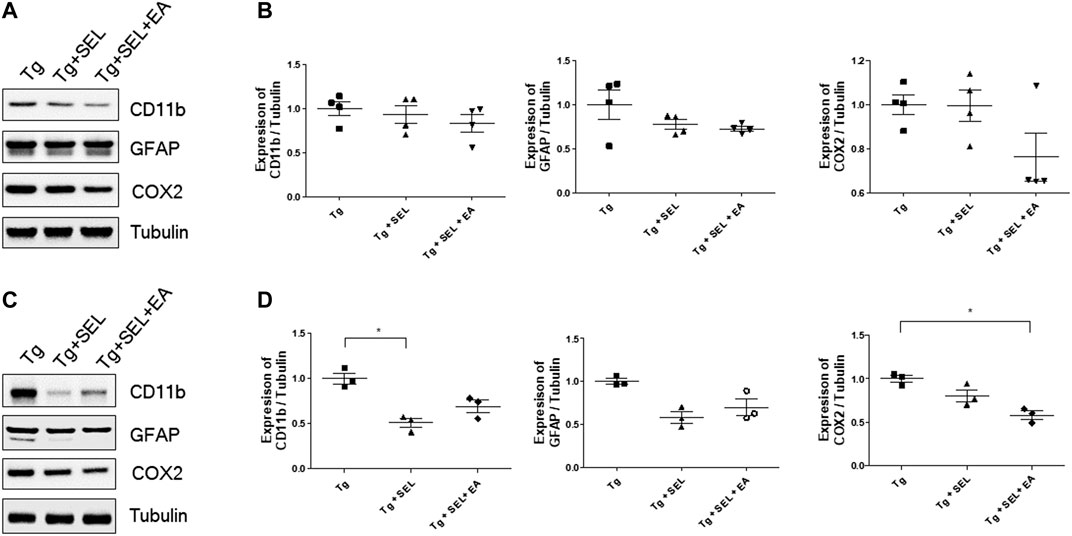
FIGURE 3. Means ± SEM of CD11B, GFAP, and COX2 for Tg, Tg + SEL, and Tg + SEL + EA mice groups. (A) Representative immunoblot images from the hippocampi. (B) Quantification of expression levels in the hippocampi (n = 4/group). (C) Representative immunoblot images from the prefrontal cortex. (D) Quantification of expression levels in the prefrontal cortex (n = 3/group). Statistical analysis of the immuno blots was analyzed using Kruskal–Wallis test and Dunn’s multiple comparison test with p values <0.05 considered statistically significant. SEM, standard error of mean; Tg, 5XFAD mice group; Tg + SEL, selegiline-treated 5XFAD mice group; Tg + SEL + EA, 5XFAD mice group treated with selegiline combined with electroacupuncture.
Combination Treatment With Selegiline and Electroacupuncture Decreases the Expression Level of Oxidative Stress-Related Proteins in 5XFAD Mice
Next, we investigated the effect of SEL combined with EA treatment on anti-oxidative properties, since EA treatment has been shown to enhance anti-oxidative effects (Cai et al., 2019). The expression level of HO1 was decreased by 1.6-fold in the hippocampus of 5XFAD mice that underwent the combined treatment (Figures 4A,B). In addition, the expression levels of HSP70 were also reduced in the combined treatment group but it was not significant (Figures 4A,B).
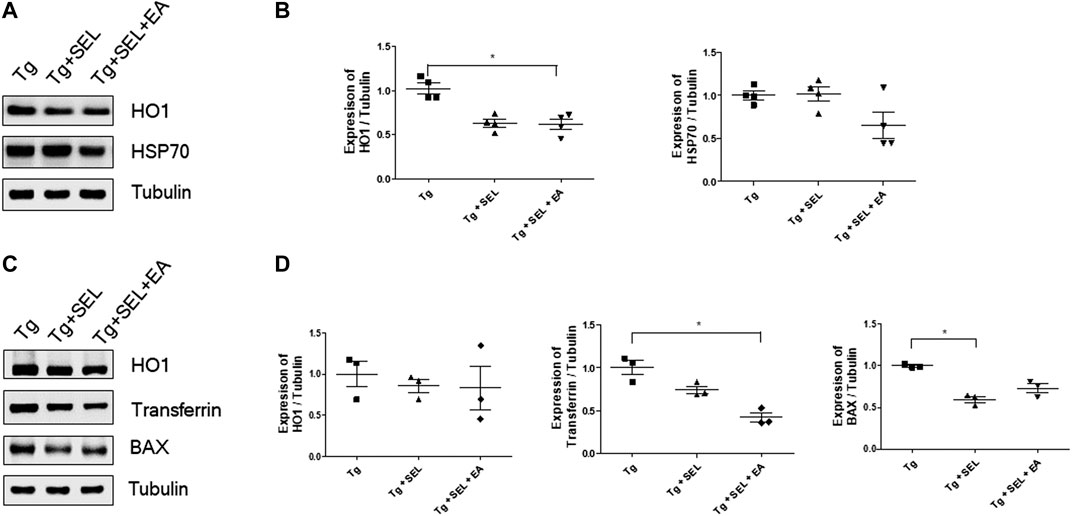
FIGURE 4. Means ± SEM for the expression of HO-1, transferrin, BAX, and HSP70 for Tg, Tg + SEL, and Tg + SEL + EA mice groups. (A) Representative immunoblot images from the hippocampi. (B) Quantification of expression levels in the hippocampi (n = 4/group). (C) Representative the immune blot images also including HO1, transferrin, and BAX from the prefrontal cortex. (D) Quantification of expression levels in the prefrontal cortex (n = 3/group). Statistical analysis of the immune blot was analyzed using Kruskal–Wallis test and Dunn’s multiple comparison test with p values <0.05 considered statistically significant. SEM, standard error of mean; Tg, 5XFAD mice group; Tg + SEL, selegiline-treated 5XFAD mice group; Tg + SEL + EA, 5XFAD mice group treated with selegiline combined with electroacupuncture.
Although the HO1 protein levels were lesser in the combined treatment group than in the transgenic group, these differences were not significant. Nevertheless, transferrin levels in the prefrontal cortex were significantly lesser 2.8-fold in the combined treatment group than in the transgenic group (Figures 4C,D). The expression levels of BAX were also reduced in the combined treatment group, but it was not significant (Figures 4C,D).
Combination Treatment with SEL and EA did not Directly Modulate Aβ generation but Modulated Glucose Metabolism in 5XFAD Mice
To demonstrate whether SEL combined with EA treatment regulated Aβ generation, we investigated the expression level of Aβ generation related protein and the amount of Aβ in 5XFAD mice. As shown in Figure 5A,B, we observed that the levels of APP and APOE were significantly reduced by 2.8- and 1.8-fold in SEL combined with EA treated 5XFAD mice compared to those in Tg mice. The beta-secretase (BACE) level was reduced by SEL combined with EA treatment, but this was not significant. Furthermore, the amount of insoluble Aβ was not changed by SEL combined with EA treatment in 5XFAD mice (Figure 5C). It suggests that improved cognitive function due to SEL combined with EA treatment does not directly involve Aβ generation. To determine the relationship between cognition and SEL combined with EA treatment, we examined the effect of combined treatment on glucose metabolism using micro PET because APOE is involved in neuroinflammation and glucose metabolism in AD pathogenesis (Jagust and Landau, 2012; Liu et al., 2013). As shown in Figures 5D,E, SEL combined with EA treatment caused a 1.2-fold increase in the mean glucose metabolism of the frontal cortex involved in working memory, compared to that in Tg mice.
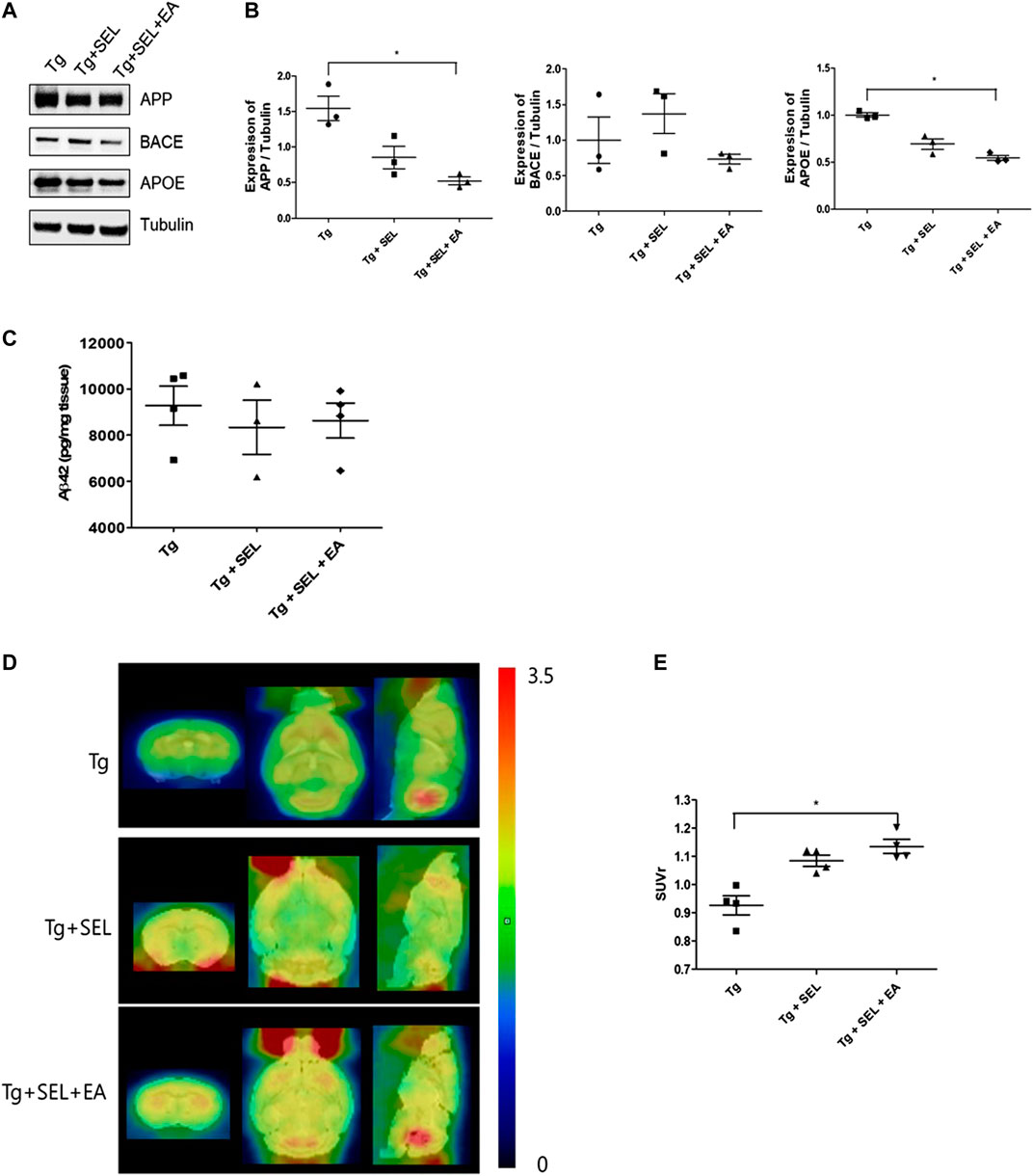
FIGURE 5. Means ± SEM of APP, BACE, APOE, and Aβ in the prefrontal cortex of 5XFAD mice in Tg, Tg + SEL, and Tg + SEL + EA groups. (A) Representative immunoblot images of APP, BACE, and APOE protein expression. (B) Quantification of the expression of APP, BACE, and APOE levels (n = 3/group). (C) Insoluble Aβ1-42 levels demonstrated on ELISA (n = 3–4/group). (D) Representative microPET images. (E) Quantitative analysis of ROI-based 18F-FDG uptake (SUV) in the frontal cortex (n = 4). Statistical analysis of the immuno blots and SUV were analyzed using Kruskal–Wallis test and Dunn's multiple comparison test with p values <0.05 considered statistically significant. SEM, standard error of mean; Aβ, β-amyloid protein; APP, Aβ precursor protein; BACE, β-secretase; APOE, apolipoprotein E; ELISA, Enzyme-Linked Immunosorbent Assays; ROI, region of interest; Tg, 5XFAD mice group; Tg + SEL, selegiline-treated 5XFAD mice group; and Tg + SEL + EA, 5XFAD mice treated with selegiline combined with electroacupuncture.
Discussion
The number of AD patients is on the rise worldwide with aging. However, there is still no effective drug therapy for the treatment of AD. Research has shown that non-drug therapy is helpful for the maintenance or improvement of cognition in AD patients (DeVreese et al., 1996; Kukull and Ganguli, 2000; Dysken et al., 2014); however, that non-drug therapy is more effective than pharmacological therapy is controversial.
This study was designed to demonstrate the effect of a combination of SEL and EA treatment on cognition using an AD animal model. We found that the combined treatment improved cognition through the reduction of neuroinflammation and an increase in the metabolic activity of 5XFAD mice demonstrating AD biomarkers.
Remarkable factors of AD are Aβ plaques and phosphorylated NFT formation caused by neuroinflammation. Specifically, activated microglia induced proinflammatory cytokines, complement components, chemokines, and reactive oxygen species (ROS) lead to broken brain homeostasis (Veerhuis et al., 1999; Szczepanik et al., 2001; McGeer and McGeer, 2010). Further, research has demonstrated that Aβ activated microglia depend on microglial CD14 and toll-like receptors 2 and 4. Proinflammatory cytokines produced by activated microglia regulate Aβ clearance and Aβ degrading enzyme (Panchal et al., 2007; Reed-Geaghan et al., 2009). In addition, Zhang et al. and Quinn et al. suggested that Aβ -induced oxidative stress also lead to neuroinflammation and microglial activation in AD animal model (Quinn et al., 2005; Zhang et al., 2009). Aβ-induced neuroinflammation, including oxidative stress is induced by NF-κB (Akama et al., 1998), receptor for advanced glycation endproducts (RAGE) signaling (Yan et al., 1998), NMDA-receptor, ROS (Milton et al., 2008), nitric oxide synthase (NOS), and nitric oxide (NO) (Yang et al., 2010), as well as activation of NADPH oxidase (Szaingurten-Solodkin et al., 2009).
SEL (L-deprenyl), an irreversible inhibitor of MAO-B, has been used as a drug (an as antioxidant enzyme) for Parkinson’s disease. In addition, SEL inhibits apoptosis induced by oxidative stress and cell death caused by the depletion of glutathione (GSH) (Qin et al., 2003). Furthermore, Knoll reported that SEL delays memory impairment in aging animals (Knoll, 1989). Jo et al. demonstrated that SEL treatment prevents impairment of synaptic plasticity and cognitive function in AD mice (Jo et al., 2014).
EA, one of acupuncture in complementary and alternative medicine (CAM), improves cognitive impairment by alleviating neuroinflammation (Feng et al., 2017) and oxidative stress (Lin et al., 2015) in the hippocampus. Our findings corroborate those of previous studies in that EA treatment was found to attenuate cognitive impairment by the reduction of neuroinflammation in 5XFAD mice (Cai et al., 2019). In addition, a recent study reported that acupuncture improves memory better than donepezil treatment in AD patients (Huang et al., 2019). In this study, we showed that SEL plus EA treatment improved cognitive behavior and reduced neuroinflammation including oxidative stress-related proteins. This suggests that combining classical drug therapy with anon-pharmacological intervention can be more effective than the exclusive use of drugs for the treatment of AD.
In terms of underlying mechanisms, our results suggest greater anti-neuroinflammation and anti-oxidative effects, and lesser autophagic dysfunction in the combined treatment group. These findings are conducive to cognitive improvements, which also align with the results of the cognitive tests performed in this study. Improved cognitive functions were also demonstrated by increased metabolic activity and lesser Aβ accumulation in 18F-FDG scans, similar to previous findings (Jagust and Landau, 2012; Wang et al., 2012; Zhang et al., 2012; Cai et al., 2019; Jiang et al., 2019). Therefore, we suggest that SEL combined with EA treatment improved cognitive function by activation of frontal cortex with an increase in glucose metabolism.
Several studies demonstrated that APOE is related to AD pathology and enhanced neuroinflammation, mitochondrial dysfunction, and impaired synaptic plasticity (Chang et al., 2005; Klein et al., 2010). Interestingly, we found that while the expression of APOE was significantly lesser in the combined treatment group than that in the control group, the amount of insoluble Aβ and expression level of Aβ generation proteins such as APP and BACE did not differ significantly between the groups. Regarding Liu et al., APOE co-localizes with plaque-associated amyloid and microglia for innate immune response, and ApoE4 promotes proinflammatory responses that could exacerbate AD pathogenesis (Liu et al., 2013). In addition, APOE alters the cerebral structure and APOE ε4 is involved in hippocampal CA1 apical neuropil atrophy and damaged episodic memory across the cognitive spectrum (Kerchner et al., 2014). APOE4 decreases glucose metabolism and increases Aβ (Jagust and Landau, 2012). EA regulates glucose metabolism in the frontal cortex of 5XFAD mice (Cai et al., 2019). Jiang et al. demonstrated an increased glucose metabolism rate in acupuncture-treated groups relative to that in the senescence-accelerated mouse prone 8 mice (Jiang et al., 2019). In addition, acupuncture increases the temporal lobe and prefrontal lobe activity associated with memory and cognitive function in functional magnetic resonance imaging (fMRI) studies (Wang et al., 2012; Zhang et al., 2012).
We found that SEL combined with EA improves cognitive function by anti-neuroinflammatory processes and increased in metabolic activity. Altogether, we suggest that the combination of drugs with non-drug treatment could help AD symptom alleviation. Specifically, the data suggest that SEL combined with EA treatment could help improve in working and spatial memory in AD. However, this study has some limitations in using SEL combined with EA treatment for AD patients. Therefore, further research could evaluate whether EA treatment alone would be sufficiently effective in treating AD compared to the evaluated combination treatment, and whether any interactive effects exist between the drug and non-drug treatments. The synergistic molecular mechanism affecting anti-neuroinflammation and glucose metabolism between the two treatments evaluated in this study is unclear. Therefore, future studies could aim to understand the regulatory mechanism related to the signaling pathway of glucose metabolism that underlies a combination of such treatments. Additional research could also evaluate the corresponding effects on mitochondrial function. Furthermore, clinical trials could be performed to confirm the effectiveness of non-drug therapies combined with drug-based interventions for patients with AD.
Data Availability Statement
The data supporting the conclusions of this study will be made available by the authors.
Ethics Statement
The animal study was reviewed and approved by Institutional Animal Care and Use Committee (IACUC) of KIOM.
Author Contributions
MDC performed all experiments. EJY designed this study and analyzed data, and wrote the manuscript.
Funding
This study was supported by the Basic Science Research Program through the National Research Foundation of Korea funded by the Ministry of Science, ICT and Future Planning, South Korea, under Grant NRF-2015R1C1A2A01053248 and the Korea Institute of Oriental Medicine (KIOM) under Grant KSN1621051.
Conflict of Interest
The authors declare that the research was conducted in the absence of any commercial or financial relationships that could be construed as a potential conflict of interest.
References
Akama, K. T., Albanese, C., Pestell, R. G., and Van Eldik, L. J. (1998). Amyloid beta-peptide stimulates nitric oxide production in astrocytes through anNF-κB-dependent mechanism. Proc. Natl. Acad. Sci. U. S. A. 95, 5795–5800. doi:10.1073/pnas.95.10.5795
Álvarez-Arellano, L., Pedraza-Escalona, M., Blanco-Ayala, T., Camacho-Concha, N., Cortés-Mendoza, J., Pérez-Martínez, L., et al. (2018). Autophagy impairment by caspase-1-dependent inflammation mediates memory loss in response to β-Amyloid peptide accumulation. J. Neurosci. Res. 96, 234–246. doi:10.1002/jnr.24130
Barger, S. W.,, and Harmon, A. D. (1997). Microglial activation by Alzheimer amyloid precursor protein and modulation by apolipoprotein E. Nature 388, 878–881. doi:10.1038/42257
Burns, J. M., Galvin, J. E., Roe, C. M., Morri, s. J. C., and McKeel, D. W. (2005). The pathology of the substantia nigra in Alzheimer disease with extrapyramidal signs. Neurology 64, 1397–1403. doi:10.1212/01.WNL.0000158423.05224.7F
Cai, M., Lee, J. H., and Yang, E. J. (2019). Electroacupuncture attenuates cognition impairment via anti-neuroinflammation in an Alzheimer's disease animal model. J. Neuroinflammation 16, 264. doi:10.1186/s12974-019-1665-3
Calsolaro, V.,, and Edison, P. (2016). Neuroinflammation in Alzheimer's disease: current evidence and future directions. Alzheimers Dement. 12, 719–732. doi:10.1016/j.jalz.2016.02.010
Chang, S., ran Ma, T., Miranda, R. D., Balestra, M. E., Mahley, R. W., and Huang, Y. (2005). Lipid- and receptor-binding regions of apolipoprotein E4 fragments act in concert to cause mitochondrial dysfunction and neurotoxicity. Proc. Natl. Acad. Sci. U. S. A. 102, 18694–18699. doi:10.1073/pnas.0508254102
Choi, S. H., Bylykbashi, E., Chatila, Z. K., Lee, S. W., Pulli, B., Clemenson, G. D., et al. (2018). Combined Adult neurogenesis and BDNF mimic exercise effects on cognition in an Alzheimer's mouse model. Science 361, eaan8821. doi:10.1126/science.aan8821
DeVreese, L. P., Neri, M., Boiardi, R., Ferrari, P., Belloi, L., and Salvioli, G. (1996). Memory training and drug therapy act differently on memory and meta-memory functioning: evidence from a pilot study. Arch. Gerontol. Geriatr. 22, 9–22. doi:10.1016/0167-4943(96)86906-8
Dysken, M. W., Sano, M., Asthana, S., Vertrees, J. E., Pallaki, M., Llorente, M., et al. (2014). Effect of vitamin E and memantine on functional decline in Alzheimer disease: the TEAM-AD VA cooperative randomized trial. JAMA 311, 33–44. doi:10.1001/jama.2013.282834
Fakhoury, M. (2018). Microglia and astrocytes in Alzheimer's disease: implications for therapy. Curr. Neuropharmacol. 16, 508–518. doi:10.2174/1570159X15666170720095240
Feng, P. P., Deng, P., Liu, L. H., Ai, Q., Yin, J., Liu, Z., et al. (2017). Electroacupuncture alleviates postoperative cognitive dysfunction in aged rats by inhibiting hippocampal neuroinflammation activated via Microglia/TLRs pathway. Evid. Based Complement Alterna. Med. 2017, 6421260. doi:10.1155/2017/6421260
Fillit, H., Ding, W. H., Buee, L., Kalman, J., Altstiel, L., Lawlor, B., et al. (1991). Elevated circulating tumor necrosis factor levels in Alzheimer’s disease. Neurosci. Lett. 129, 318–320. doi:10.1016/0304-3940(91)90490-k
Gibb, W. R., Mountjoy, C. Q., Mann, D. M., and Lees, A. J. (1989). The substantia nigra and ventral tegmental area in Alzheimer’s disease and Down’s syndrome. J. Neurol. Neurosurg. Psychiatry 52, 193–200. doi:10.1136/jnnp.52.2.193
Han, Y. G., Qin, X., Zhang, T., Lei, M., Sun, F. Y., Sun, J. J., et al. (2018). Electroacupuncture prevents cognitive impairment induced by lipopolysaccharide via inhibition of oxidative stress and neuroinflammation. Neurosci. Lett. 683, 190–195. doi:10.1016/j.neulet.2018.06.003
Huang, Q., Luo, D., Chen, L., Liang, F. X., and Chen, R. (2019). Effectiveness of acupuncture for Alzheimer's disease: an updated systematic review and meta-analysis. Curr. Med. Sci. 39, 500–511. doi:10.1007/s11596-019-2065-8
Jagust, W. J.,, and Landau, S. M.; Alzheimer’s Disease Neuroimaging Inititative. (2012). Apolipoprotein E, not fibrillar beta-amyloid, reduces cerebral glucose metabolism in normal aging. J. Neurosci. 32, 18227–18233. doi:10.1523/JNEUROSCI.3266-12.2012
Jeon, S. G., Cha, M. Y., Kim, J. I., Hwang, T. W., Kim, K. A., Kim, T. H., et al. (2019). Vitamin D-binding protein-loaded PLGA nanoparticles suppress Alzheimer's disease-related pathology in 5XFAD mice. Nanomedicine 17, 297–307. doi:10.1016/j.nano.2019.02.004
Jia, Y., Zhang, X., Yu, J., Han, J., Yu, T., Shi, J., et al. (2017). Acupuncture for patients with mild to moderate Alzheimer's disease: a randomized controlled trial. BMC Complement Altern. Med. 17, 556. doi:10.1186/s12906-017-2064-x
Jiang, J., Liu, G., Shi, S., Li, Y., and Li, Z. (2019). Effects of manual acupuncture combined with donepezil in a mouse model of Alzheimer's disease. Acupunct. Med. 37, 64–71. doi:10.1136/acupmed-2016-011310
Jo, S., Yarishkin, O., Hwang, Y. J., Chun, Y. E., Park, M., Woo, D. H., et al. (2014). GABA from reactive astrocytes impairs memory in mouse models of Alzheimer's disease. Nat. Med. 20, 886–896. doi:10.1038/nm.3639
Kerchner, G. A., Berdnik, D., Shen, J. C., Bernstein, J. D., Fenesy, M. C., Deutsch, G. K., et al. (2014). APOE ε4 worsens hippocampal CA1 apical neuropil atrophy and episodic memory. Neurology 82, 691–697. doi:10.1212/WNL.0000000000000154
Klein, R. C., Mace, B. E., Moore, S. D., and Sullivan, P. M. (2010). Progressive loss of synaptic integrity in human apolipoprotein E4 targeted replacement mice and attenuation by apolipoprotein E2. Neuroscience 171, 1265–1272. doi:10.1016/j.neuroscience.2010.10.027
Knoll, J. (1989). The pharmacology of selegiline ((−)deprenyl). New aspects. Acta Neurol. Scand. Suppl. 126, 83–91. doi:10.1111/j.1600-0404.1989.tb01787.x
Kukull, W. A.,, and Ganguli, M. (2000). Epidemiology of dementia – concepts and overview. Neurol. Clin. 18, 923–950. doi:10.1016/s0733-8619(05)70233-4
Li, X., Fan, G., Zhang, Q., Huo, T., Liu, L., Wei, H., et al. (2014). Electroacupuncture decreases cognitive impairment and promotes neurogenesis in the APP/PS1 transgenic mice. BMC Compl. Altern. Med. 14, 37. doi:10.1186/1472-6882-14-37
Lin, R., Lin, Y., Tao, J., Chen, B., Yu, K., Chen, J., et al. (2015). Electroacupuncture ameliorates learning and memory in rats with cerebral ischemia-reperfusion injury by inhibiting oxidative stress and promoting p-CREB expression in the hippocampus. Mol. Med. Rep. 12, 6807–6814. doi:10.3892/mmr.2015.4321
Liu, C. C., Liu, C. C., Kanekiyo, T., Xu, H., and Bu, G. (2013). Apolipoprotein E and Alzheimer disease: risk, mechanisms and therapy. Nat. Rev. Neurol. 9, 106–118. doi:10.1038/nrneurol.2012.263
Liu, Z. B., Niu, W. M., Yang, X. B., Niu, X. B., and Yuan, W. (2011). Effect of “Xiusanzhen” on expression of hippocampal Bcl-2 and Bax proteins in Alzheimer disease rats. Zhen. Ci. Yan. Jiu. 36, 7–11.
Lu, K. W., Yang, J., Hsieh, C. L., Hsu, Y. C., and Lin, Y. W. (2017). Electroacupuncture restores spatial learning and downregulates phosphorylated N-methyl-D-aspartate receptors in a mouse model of Parkinson's disease. Acupunct. Med. 35, 133–141. doi:10.1136/acupmed-2015-011041
Mangoni, A., Grassi, M. P., Frattola, L., Piolti, R., Bassi, S., Motta, A., et al. (1991). Effects of a MAO-B inhibitor in the treatment of Alzheimer disease. Eur. Neurol. 31, 100–107. doi:10.1159/000116655
McGeer, E. G., and McGeer, P. L. (2010). Neuroinflammation in Alzheimer’s disease and mild cognitive impairment: a field in its infancy. J. Alzheimers Dis. 19, 355–361. doi:10.3233/JAD-2010-1219
Milton, R. H., Abeti, R., Averaimo, S., DeBiasi, S., Vitellaro, L., Jiang Curmi, P. M. G., et al. (2008). CLIC1 function is required for beta-amyloid-induced generation of reactive oxygen species by microglia. J. Neurosci. 28, 11488–11499. doi:10.1523/JNEUROSCI.2431-08.2008
No authors listed, (1998). NIH consensus conference. Acupuncture. J. Am. Med. Assoc. 280, 1518–1524. doi:10.1001/jama.280.17.1518
Nobili, A., Latagliata, E. C., Viscomi, M. T., Cavallucci, V., Cutuli, D., Giacovazzo, G., et al. (2017). Dopamine neuronal loss contributes to memory and reward dysfunction in a model of Alzheimer's disease. Nat. Commun. 8, 14727. doi:10.1038/ncomms14727
Oakley, H., Cole, S. L., Logan, S., Maus, E., Shao, P., Craft, J., et al. (2006). Intraneuronal beta-amyloid aggregates, neurodegeneration, and neuron loss in transgenic mice with five familial Alzheimer's disease mutations: potential factors in amyloid plaque formation. J. Neurosci. 26, 10129–10140. doi:10.1523/JNEUROSCI.1202-06.2006
Oyama, F., Shimada, H., Oyama, R., Titani, K., and Ihara, Y. (1993). Beta-amyloid protein precursor and tau mRNA levels versus beta-amyloid plaque and neurofibrillary tangles in the aged human brain. J. Neurochem. 60, 1658–1664. doi:10.1111/j.1471-4159.1993.tb13388.x
Panchal, M., Lazar, N., Munoz, N., Fahy, C., Clamagirand, C., Brouard, J. P., et al. (2007). Clearance of amyloidbeta peptide by neuronal and non-neuronal cells: proteolytic degradation by secreted and membrane associated proteases. Curr. Neurovasc. Res. 4, 240–251. doi:10.2174/156720207782446315
Piccinin, G. L., Finali, G., and Piccirilli, M. (1990). Neuropsychological effects of l-deprenyl in Alzheimerʼs type dementia. Clin. Neuropharmacol. 13, 147–163. doi:10.1097/00002826-199004000-00004
Qin, F., Shite, J., Mao, W., and Liang, C. S. (2003). Selegiline attenuates cardiac oxidative stress and apoptosis in heart failure: association with improvement of cardiac function. Eur. J. Pharmacol. 461, 149–158. doi:10.1016/s0014-2999(03)01306-2
Quinn, J., Kulhanek, D., Nowlin, J., Jones, R., Praticò, D., Rokach, J., et al. (2005). Chronic melatonin therapy fails to alter amyloid burden or oxidative damage in old Tg2576 mice: implications for clinical trials. Brain Res. 1037, 209–213. doi:10.1016/j.brainres.2005.01.023
Reed-Geaghan, E. G., Savage, J. C., Hise, A. G., and Landreth, G. E. (2009). CD14 and toll-like receptors 2 and 4 are required for fibrillary fibrillary A{beta}-stimulated microglial activation. J. Neurosci. 29, 11982–11992. doi:10.1523/JNEUROSCI.3158-09.2009
Sano, M., Ernesto, C., Thomas, R. G., Klauber, M. R., Schafer, K., Grundman, M., et al. (1997). A controlled trial of selegiline, alpha-tocopherol, or both as treatment for Alzheimer's disease. The Alzheimer's Disease Cooperative Study. N. Engl. J. Med. 336, 1216–1222. doi:10.1056/NEJM199704243361704
Sastre, M., Klockgether, T., and Heneka, M. T. (2006). Contribution of inflammatory processes to Alzheimer’s disease: molecular mechanisms. Int. J. Dev. Neurosci. 24, 167–176. doi:10.1016/j.ijdevneu.2005.11.014
Shao, S., Tang, Y., Guo, Y., Tian, Z., Xiang, D., and Wu, J. (2019). Effects of acupuncture on patients with Alzheimer's disease: protocol for a systematic review and meta-analysis. Medicine (Baltimore) 98, e14242. doi:10.1097/MD.0000000000014242
Storga, D., Vrecko, K., Birkmayer, J. G., and Reibnegger, G. (1996). Monoaminergic neurotransmitters, their precursors and metabolites in brains of Alzheimer patients. Neurosci. Lett. 203, 29–32. doi:10.1016/0304-3940(95)12256-7
Strauss, S., Bauer, J., Ganter, U., Jonas, U., Berger, M., and Volk, B. (1992). Detection of interleukin-6 and alpha 2-macroglobulin immunoreactivity in cortex and hippocampus of Alzheimer’s disease patients. Lab. Invest. 66, 223–230.
Szaingurten-Solodkin, I., Hadad, N., and Levy, R. (2009). Regulatory role of cytosolic phospholipase A2alpha in NADPH oxidase activity and in inducible nitric oxide synthase induction by aggregated Ab1-42 in microglia. Glia 57, 1727–1740. doi:10.1002/glia.20886
Szczepanik, A. M., Funes, S., Petko, W., and Ringheim, G. E. (2001). IL-4, IL-10 and IL-13 modulate A beta(1–42) induced cytokine and chemokine production in primary murine microglia and a human monocyte cell line. J. Neuroimmunol. 113, 49–62. doi:10.1016/s0165-5728(00)00404-5
Veerhuis, R., Janssen, I., De Groot, C. J., Van Muiswinkel, F. L., Hack, C. E., and Eikelenboom, P. (1999). Cytokines associated with amyloid plaques in Alzheimer’s disease brain stimulate human glial and neuronal cell cultures to secrete early complement proteins, but not C1-inhibitor. Exp. Neurol. 160, 289–299. doi:10.1006/exnr.1999.7199
Wang, Z., Liang, P., Jia, X., Jin, G., Song, H., Han, Y., et al. (2012). The baseline and longitudinal changes of PCC connectivity in mild cognitive impairment: a combined structure and restingstate fMRI study. PloS One 7, e36838. doi:10.1371/journal.pone.0036838
Yan, S. D., Stern, D., Kane, M. D., Kuo, Y. M., Lampert, H. C., and Roher, A. E. (1998). RAGE-Aβ interactions in the pathophysiology of Alzheimer’s disease. Restor. Neurol. Neurosci. 12, 167–173.
Yang, E. J., Cai, M., and Lee, J. H. (2016). Neuroprotective effects of electroacupuncture on an animal model of Bilateral common carotid Artery occlusion. Mol. Neurobiol. 53, 7728–7236. doi:10.1007/s12035-015-9610-7
Yang, Q., Zhu, S., Xu, J., Tang, C., Wu, K., and Wu, Y., (2019). Effect of the electro-acupuncture on senile plaques and its formation in APP+/PS1+ double transgenic mice. Genes Dis. 6, 282–289. doi:10.1016/j.gendis.2018.06.002
Yang, S. G., Wang, W. Y., Ling, T. J., Feng, Y., Du, X. T., Zhang, X., et al. (2010). Alpha-tocopherol quinone inhibits beta-amyloid aggregation and cytotoxicity, disaggregates preformed fibrils and decreases the production of reactive oxygen species, NO and inflammatory cytokines. Neurochem. Int. 57, 914–922. doi:10.1016/j.neuint.2010.09.011
Yang, X. H., Liu, Z. B., Niu, W. M., and Niu, X. M. (2011). Effect of “Xiusanzhen” on hippocampal muscarinic cholinergic receptor activity in Alzheimer disease rats. Zhen Ci Yan Jiu 36, 90–94.
Zhang, D. L., Chen, Y. Q., Jiang, X., Ji, T. T., and Mei, B. (2009). Oxidative damage increased in presenilin1/presenilin2 conditional double knockout mice. Neurosci Bull 25, 131–137. doi:10.1007/s12264-009-0114-1
Zhang, G., Yin, H., Zhou, Y. L., Han, H. Y., Wu, Y. H., Xing, W., et al. (2012). Capturing amplitude changes of low-frequency fluctuations in functional magnetic resonance imaging signal: a pilot acupuncture study on NeiGuan (PC6). J. Altern. Complement Med. 18, 387–393. doi:10.1089/acm.2010.0205
Keywords: Alzheimer’s disease, cognitive dysfunction, electroacupuncture, selegiline, 5XFAD
Citation: Cai M and Yang EJ (2020) Effect of Combined Electroacupuncture and Selegiline Treatment in Alzheimer’s Disease: An Animal Model. Front. Pharmacol. 11:606480. doi: 10.3389/fphar.2020.606480
Received: 15 September 2020; Accepted: 10 November 2020;
Published: 10 December 2020.
Edited by:
Chiayu Chiu, University of Valparaíso, ChileReviewed by:
Claudia Duran-Aniotz, Adolfo Ibáñez University, ChileAlvaro O. Ardiles, Universidad de Valparaiso, Chile
Copyright © 2020 Yang and Cai. This is an open-access article distributed under the terms of the Creative Commons Attribution License (CC BY). The use, distribution or reproduction in other forums is permitted, provided the original author(s) and the copyright owner(s) are credited and that the original publication in this journal is cited, in accordance with accepted academic practice. No use, distribution or reproduction is permitted which does not comply with these terms.
*Correspondence: Eun Jin Yang, yej4823@gmail.com