- 1Key Laboratory of Plant Resource Conservation and Germplasm Innovation in Mountainous Region (Ministry of Education), Collaborative Innovation Center for Mountain Ecology & Agro-Bioengineering (CICMEAB), College of Life Sciences/Institute of Agro-bioengineering, Guizhou University, Guiyang, China
- 2Guizhou Engineering Center for Innovative Traditional Chinese Medicine and Ethnic Medicine, Guizhou University, Guiyang, China
- 3College of Pharmacy, Guizhou University of Traditional Chinese Medicine, Guiyang, China
Hedychium flavum Roxb., a medicinal, edible, and ornamental plant, is widely cultivated throughout China, India, and Southeast Asia. The rhizome from this plant has been used for food flavoring and in traditional Chinese medicine to treat diverse diseases, but the detailed constituents and bioactivities are still limited known. Therefore, phytochemical analysis by GC-MS and UHPLC-Q-Orbitrap-MS, and antioxidant, antibacterial, cytotoxic, and enzyme inhibitory activities tests have been conducted in the current study. Based on the GC-MS results, the essential oil (EO) of rhizome was mainly composed of coronarin E (20.3%), β-pinene (16.8%), E-nerolidol (11.8%), and linalool (8.5%). Among them, coronarin E was reported in H. flavum EO firstly. Furthermore, the spectrophotometric indicated rhizome had high total phenolic content (TPC, 50.08–57.42 mg GAEs/g extract) and total flavonoid content (TFC, 12.45–21.83 mg REs/g extract), no matter in water extract (WE) or in 70% ethanol extract (EE). UHPLC-Q-Orbitrap-MS was applied to further characterize composition, and 86 compounds were putatively identified from WE and EE, including 13 phenolic components. For the bioactivities, both WE and EE showed remarkable antioxidant activity by DPPH and ABTS tests, being superior to the positive control (butylated hydroxytoluene, BTH). EO revealed significant antibacterial activity against Staphylococcus aureus, Bacillus subtilis, Pseudomonas aeruginosa, and Proteus vulgaris with DIZ (10.34–24.43 mm), MIC (78.13–312.50 μg/mL), and MBC (156.25–625.00 μg/mL). Moreover, EO exhibited a considerable selectivity to human tumor cell K562 (IC50 = 27.16 μg/mL), and its toxicity was more than 3.5-fold different from that of non-cancerous MRC-5 cell (IC50 = 95.96 μg/mL) and L929 cell (IC50 = 129.91 μg/mL). A series of apoptosis analysis demonstrated that EO induced apoptosis against K562 cells in a dose-dependent manner. In enzyme inhibitory effect assays, WE and EE showed strong α-glucosidase inhibition activity, being superior to the positive control (acarbose). Besides, the EO, WE, and EE didn’t show a promising inhibition on tyrosinase (19.30–32.51 mg KAEs/g sample) and exhibited a weak inhibitory effect on cholinesterase. Based on the current results, H. flavum could be considered as a source of bioactive compounds and has high exploitation potential in the cosmetics, food, and pharmaceutical industries.
Introduction
The Hedychium genus (Zingiberaceae), commonly known as ginger lily, includes approximately 87 species, which are mainly distributed in China, India, and Southeast Asia (The Plant List, 2010; Prakash et al., 2016). Hedychium species are extensively cultivated for their various uses in fragrance, paper, ornamental, cosmetics, medicine, and food industries (Sakhanokho and Rajasekaran, 2019). Hedychium plants are rich in essential oil and widely used in traditional medicine for the treatment of flu, gastric diseases, diarrhea, bronchitis, asthma, nausea, snake bites, and leishmaniasis (Tushar et al., 2010; Hartati et al., 2014; Sakhanokho and Rajasekaran, 2019). The extracts and essential oils of Hedychium plants have been demonstrated to have multiple bioactivities, such as antioxidant, antimicrobial, cytotoxicity, anti-acetylcholinesterase, anti-inflammatory, antidiabetic, analgesic, hepatoprotective, and insecticidal activities (Hartati et al., 2014; Ray et al., 2018a; Tavares et al., 2020).
Hedychium flavum Roxb., a perennial plant with aromatic and tuberose characters, is widely cultivated for its aromatic essential oil (EO) and as a medicinal, edible, and ornamental plant in China, India, and Southeast Asia (Wu and Larsen, 2000; Thanh et al., 2014). The young tender shoots of H. flavum are used as vegetable and food flavorings (Devi et al., 2014). The EO from this plant flower has been used as spice and to treat gastric diseases (Pan and Zhang, 2013). The fresh rhizome of H. flavum is sliced or minced and used as food flavoring in Southwestern China. The rhizome has been used in Chinese folk medicine, commonly known as yehansu, to treat cough, cold, rheumatism, headache, abdominal pain, and bruise (CHMC-Chinese Herbal Medicine Company, 1994; Wang, 2010). Additionally, The EO from rhizome has been used in delicate, high-quality perfumes and traditional medicines (Prakash et al., 2016). According to previous studies, the main EO constituents of rhizome were α-pinene (4.3–12.1%), β-pinene (21.8–24.77%), 1,8-cineole (8.90–28.3%), and linalool (6.29–17.5%) (Sakhanokho et al., 2013; Thanh et al., 2014; Ray et al., 2018a). The major compounds of EO in leaf were β-pinene (22.5%), α-humulene (15.7%), and β-caryophyllene (10.4%), as well as the main EO components in stem were α-humulene (18.9%), β-caryophyllene (11.8%), and β-pinene (11.2%) (Thanh et al., 2014). Besides, the predominant EO compounds in aerial parts of H. flavum were β-pinene (49.6%), β-caryophyllene (26.9%), α-pinene (5.9%), sabinene (4.2%), and α-humulene (2.3%) (Mollenbeck et al., 1997). The EO from H. flavum rhizome has been demonstrated to possess antifungal and insecticidal activities (Rajasekaran et al., 2012; Sakhanokho et al., 2013).
H. flavum has been developed as an industrial crop with medicinal, ornamental, and spice values. However, in addition to few reports on the chemical components, antifungal and insecticidal activities of EO, there is no detailed study on the chemical composition and bioactivities of H. flavum, which could impede its exploitation in industry. Therefore, phytochemical analysis by GC-MS and UHPLC-Q-Orbitrap-MS, and antioxidant, antibacterial, cytotoxic, and enzyme inhibitory activities tests of the rhizome from H. flavum were conducted in this work. To our knowledge, the antioxidant, antibacterial, cytotoxic, and enzyme inhibitory activities of EO, as well as the chemical composition and bioactivities of extracts from H. flavum rhizome were reported for the first time.
Materials and Methods
Chemical and Reagents
Gallic acid, rutin, streptomycin, cisplatin, acarbose, kojic acid, galanthamine, resazurin, and Folin-Ciocalteu reagent were obtained from Solarbio Life Sciences (Beijing, China). Ascorbic acid, butylated hydroxytoluene (BHT), 1,1-diphenyl-2-picrylhydrazyl (DPPH), 2,2-azino-bis-3-ethylbenzthiazoline-6-sulphonic acid (ABTS), acridine orange (AO), ethidium bromide (EB), α-glucosidase, tyrosinase, acetylcholinesterase (AChE), butyrylcholinesterase (BChE), p-Nitrophenyl-α-D-glucopyranoside (p-NPG), L-tyrosine, acetylthiocholine iodide (ATCI), and butyrylthiocholine chloride (BTCl) were purchased from Sigma-Aldrich (Germany). Hoechst 33258 and Annexin V-FITC/PI Apoptosis Detection Kit were from Beyotime (Haimen, China). For GC-MS analysis, analytical standard n-alkanes (C8–C22) were from Merck (Darmstadt, Germany). For UPLC-MS analysis, LC/MS-grade acetonitrile, formic acid, and ultrapure water were supplied from Merck (Darmstadt, Germany). All other chemicals and solvents were of analytical grade and purchased from Aladdin (Shanghai, China).
Plant Material
H. flavum rhizome was collected in April 2019 from Guangxi Province, China. The specie was identified by Prof. Guoxiong Hu of Guizhou University. The voucher specimen was deposited at Guizhou Engineering Center for Innovative Traditional Chinese Medicine and Ethnic Medicine, Guizhou University (Voucher No: HF-20190408).
Preparation of EO, WE, and EE
To produce EO, the fresh, finely chopped rhizomes (1.5 kg) were placed into a Clevenger-type apparatus and hydrodistilled (4 h). EO was dried over anhydrous Na2SO4, filtered and stored at 4°C.
WE and EE were prepared by reflux extraction method. The fresh, finely chopped rhizomes (0.5 kg) were placed into a round bottom flask (2 L), mixed with distilled water or 70% ethanol, boiled under reflux for 2 h, and then filtered. The reflux extraction was repeated twice. The combined filtrate was concentrated with a rotary evaporator and dried in a freeze-drier. Then, WE and EE were weighed and stored at 4°C.
GC- FID/MS for Composition Analysis of EO
The EO was analyzed by an Agilent 6890 gas chromatograph equipped with a flame ionization detector (FID). Capillary column: HP-5MS (60 m × 0.25 mm, 0.25 μm film thickness). The flow rate of carrier gas helium was set at 1 mL/min and the split ratio was 1:20. The following GC oven temperature was used with the injection volume of 1 μL: held at 70°C (2 min), 2°C per min to 180°C (55 min), 10°C per min to 310°C (13 min), and kept at 310°C (12 min). The GC-MS analysis was performed using an Agilent 6890 gas chromatograph equipped with an Agilent 5975C mass selective detector (Agilent Technologies Inc., CA, USA). GC column and parameters were the same as in GC-FID. The mass spectra operated in the mass range (m/z 29 to 500) and EI mode (70 eV). The interface temperature and ion source temperature were 280°C and 230°C, respectively. The relative percentage of chemical constituents was determined by the peak area. The retention index (RI) is determined by referring to a series of n-alkanes (C8–C22). The identification of chemical constituents was based on comparison of their retention index and mass spectrum with those in Wiley 275 and NIST 2017 databases.
Total Phenolic and Flavonoid Contents
Total Phenolic Content (TPC)
The TPC was evaluated according to Folin-Ciocalteu method (Slinkard and Singleton, 1977) with marginal modification. The sample solution (0.5 mL) was mixed with Folin-Ciocalteu reagent (2.5 mL) and incubated for 4 min. Subsequently, Na2CO3 solution (4 mL, 7.5%) was added and incubated at room temperature for 60 min. The sample absorbance was read using a UV-5200 spectrophotometer (Shanghai Metash Instruments Co., Ltd, China) at 760 nm. TPC was expressed as equivalents of gallic acid (mg GAEs/g extract).
Total Flavonoid Content (TFC)
The TFC was assayed by NaNO2-Al(NO3)3-NaOH colorimetry (China Pharmacopoeia Commission, 2015) with minor modification. The sample solution (5 mL) was mixed with 5% NaNO2 (0.4 mL) and incubated for 6 min. Subsequently, Al(NO3)3 solution (0.4 mL, 10%) was added and incubated for 6 min. Then, NaOH solution (4 mL, 4%) was added up to a total volume of 10 mL with distilled water. The absorbance was read at 510 nm after 15 min incubation at room temperature. TFC was expressed as rutin equivalents (mg REs/g extract).
UHPLC-Q-Orbitrap-MS Analysis of WE and EE Components
The chemical components of WE and EE were determined using ultra-high-performance liquid chromatography coupled to quadrupole-Orbitrap high-resolution mass spectrometry (UHPLC-Q-Orbitrap-MS). Chromatographic separation was carried out using a Dionex Ultimate 3000 UPLC System (Thermo Fisher Scientific, USA), with a Hypersil GOLD aQ (2.1 mm × 100 mm, i.d. 1.9 μm, Thermo Fisher Scientific) column. Mobile phases A and B were formic acid water (0.1%, v/v) and formic acid acetonitrile (0.1%, v/v), respectively. The flow rate was 0.3 mL/min. The gradient was as follows: 0–2 min, 5% B; 2–42 min, 5–95% B; 42–47 min, 95% B; 47.1 min, 5% B; 47.1–50 min, 5% B. The injection volume was 5 μL and the column temperature was held at 40°C.
The Thermo Scientific Q Exactive Focus hybrid quadrupole-Orbitrap mass spectrometry (Q-Orbitrap-MS) equipped with heated electrospray ionization (HESI-II) source was used for MS data collection. HESI-II source was operated in negative and positive ionization modes with spray voltages of 2.5 and 3.0 kV, respectively. HESI-II source parameters were set as follows: capillary temperature 320°C, vaporizer temperature 350°C, sweep gas pressure 0 arb, auxiliary gas pressure 10 arb, sheath gas pressure 35 arb, RF lens amplitude (S-lens) 60. The mass spectrometer analysis was executed in the mode of full mass/dd-MS2, scanned from m/z 100 to 1,500 with a resolution of 70,000 in full scan MS1, followed by data-dependent MS2 acquisition at 17,500 resolution with stepped normalized collision energy (NCE) (20, 40, and 60 eV). Maximum injection time (IT) for MS1 and MS2 was separately set at 100 and 50 ms and the target values of automatic gain control (AGC) were 1e6 and 2e5, respectively. Loop count was 3, dynamic exclusion time was 5 s, and quadrupole isolation window (isolation width) was 1.5 m/z. The mass spectrum data of the chemical composition was processed with CD3.0 (Thermo Fisher Scientific) and identified by comparing mzVault, mzCloud, and ChemSpider databases. The allowable calculation error was set as 5 ppm.
Antioxidant Activity
DPPH Assay
The 1,1-diphenyl-2-picrylhydrazyl (DPPH) radical scavenging activity was evaluated according to the method with slight modification (Blois, 1958). Two milliliter of sample solution and DPPH solution (2 mL, 0.1 mM) were mixed and incubated for 30 min at room temperature in the dark. The absorbance was recorded at 517 nm. Butylated hydroxytoluene (BHT) and ascorbic acid were used as positive controls. The results were expressed using IC50 values and as equivalents of ascorbic acid (mg AEs/g sample).
ABTS Assay
The 2,2-azino-bis-3-ethylbenzthiazoline-6-sulphonic acid (ABTS) radical scavenging capacity was assayed according to the method with marginal modification (Re et al., 1999). The ABTS•+ solution was generated by reacting 2.45 mM K2S2O8 solution with and 7 mM ABTS solution incubated in the dark at room temperature for 12 h. Before experiments, to obtain an absorbance of 0.70 ± 0.02 at 734 nm, the ABTS•+ solution was diluted with methanol. The diluted ABTS•+ solution (4 mL) and sample solution (0.4 mL) were mixed and the absorbance was read at 734 nm after 10 min incubation in the dark at room temperature. Ascorbic acid and BHT were used as positive controls. The results were expressed using IC50 values and as equivalents of ascorbic acid (mg AEs/g sample).
Antibacterial Activity
Bacterial Strains
The antibacterial capacity of EO, WE, and EE was evaluated performed against the following bacterial strains: Proteus vulgaris (CMCC (B) 49027), Escherichia coli (ATCC 25922), Bacillus subtilis (CMCC (B) 63501), Pseudomonas aeruginosa (CMCC (B) 10104), Enterococcus faecalis (ATCC 29212), and Staphylococcus aureus (ATCC 6538P).
Agar Well Diffusion Assay
The inhibition zone diameter was assayed according to the agar well diffusion method with slight modification (Zhang et al., 2017). Briefly, The WE and EE were dissolved with distilled water (100 mg/mL). Streptomycin distilled water solution (1 mg/mL) was used as the positive control. The bacterial suspension (100 μL, 106 CFU/mL) was evenly spread on the Mueller-Hinton agar medium. Filter paper discs (diameter 6 mm) containing pure essential oil, WE, EE, and streptomycin solution (20 μL) were added and the diameter of inhibition zone (DIZ) was recorded after 24 h incubation at 37°C.
Determination of MIC and MBC
The minimal inhibitory concentration (MIC) and minimal bactericidal concentration (MBC) values were determined by the broth microdilution method with marginal modification (Eloff, 1998). Briefly, the two-fold serially diluted sample solution (100 μL) and the bacterial suspensions (100 μL) were added to each well at a final density of 5 × 105 CFU/mL. The 96-well plates were incubated for 24 h at 37°C. Subsequently, the resazurin aqueous solution (10 μL, 0.01%) was added to each well and incubated for 2 h at 37°C in the dark. The MIC was determined as the minimum sample concentration without color change. For the determination of MBC value, the sample (10 μL) from the wells without color change was subcultured in Mueller Hinton agar plate for 24 h at 37°C. The MBC was defined as the minimum sample concentration showing no bacterial growth (Mzid et al., 2017).
Cytotoxic Activity
The cytotoxic effect was assayed according to our previously published MTT method (Tian et al., 2020) against murine fibroblast cell line (L929) and five human cell lines: fetal lung fibroblasts (MRC-5), lung adenocarcinoma (A549), and non-small cell lung cancer (NCI-H1299), prostatic carcinoma (PC-3), and leukemic (K562) cell lines. EO solution (0.1% DMSO), WE, and EE distilled water solution were two-fold serially diluted with medium. The cell suspensions (80 μL) were added to each well at a density of 5 × 103 cells per well and incubated for 24 h. Subsequently, 20 μL of the diluted sample solution was added and cultured for 72 h. After that, the MTT solution (10 μL, 5 mg/mL in PBS) was added and incubated for 4 h. Then, 150 μL of DMSO was added to each well to dissolve formazan crystal after removing the medium. The absorbance was recorded using an iMark microplate reader (Bio-Rad Laboratories, Inc., Hercules, CA, USA) at 490 nm. The results were expressed using IC50 values.
Apoptosis Analysis
Morphology Assay
The K562 cell suspension was added to a 6-well plate (1×106 cells per well) and incubated for 24 h. Subsequently, the cells were treated with varying concentrations of EO (0, 30, and 60 μg/mL) for 48 h. Morphological changes of the treated cells were recorded using an inverted microscope (Leica DMi8, Leica Microsystems, Germany).
For Acridine orange/ethidium bromide (AO/EB) staining assay, the cells were treated with EO as described above. The treated cell suspensions (1 mL) were collected, centrifuged at 500 g for 10 min, and resuspended in 50 μL PBS, then stained with AO (10 µg/mL) and EB (10 µg/mL) for 10 min. After that, the stained cell suspension (10 μL) was placed onto a glass slide and photographed using an inverted fluorescence microscope (Leica DMi8, Leica Microsystems, Germany).
For Hoechst 33258 staining assay, the cells were treated with EO as described above. The treated cell suspensions (1 mL) were collected, centrifuged, and resuspended in 1 mL PBS. After removing the PBS, the cells were fixed in 4% paraformaldehyde (10 μL) for 10 min. After two PBS washes, the cells were stained with 10 μL of Hoechst 33258 (Beyotime, Haimen, China) for 10 min. After that, the stained cell suspension (10 μL) was placed onto a glass slide and analyzed by an inverted fluorescence microscope.
Annexin V-FITC/PI Assay
Annexin V-FITC and propidium iodide (PI) staining was performed using flow cytometry to quantify EO-induced apoptosis in K562 cell. The K562 cells were seeded in 6-well plates at 2×105/well, and treated with different concentrations of EO (0, 30, and 60 μg/mL) for 48 h. Subsequently, the cells were collected and stained with Annexin V-FITC/PI as described by the manufacturer using Annexin V-FITC/PI Apoptosis Detection Kit (Beyotime, Haimen, China). The labeled cells were analyzed using a BD FACSCalibur flow cytometer (BD Bioscience, Franklin Lakes, NJ, USA).
Enzyme Inhibitory Activities
α-Glucosidase Inhibitory Activity
The α-glucosidase inhibitory activity was evaluated according to the method with slight modification (Silva et al., 2019). Sample solution (30 μL), phosphate buffer (60 μL, pH 6.8), and α-glucosidase solution (10 μL, 0.8 U/mL) were mixed and added to each well. After 15 min incubation at 37°C, p-Nitrophenyl-α-D-glucopyranoside (p-NPG) solution (10 μL, 1 mM) was added and incubated at 37°C for 15 min. Subsequently, the reaction was stopped with the addition of Na2CO3 solution (80 μL, 0.2 M), and the absorbance was recorded at 405 nm. Acarbose was used as positive control. The α-glucosidase inhibitory effect was expressed using IC50 values and as equivalents of acarbose (mmoL ACEs/g sample).
Tyrosinase Inhibitory Activity
The inhibition of tyrosinase was assayed according to the method with L-tyrosine as substrate (Zardi-Bergaoui et al., 2018). Sample solution (70 μL) and tyrosinase solution (10 μL, 100 U/mL) were mixed in a 96-well plate and incubated at 37°C for 5 min. Then, L-tyrosine solution (80 μL, 5.5mM) was added and incubated at 37°C for 30 min. Kojic acid was used as positive reference, and the absorbance was recorded at 492 nm. The tyrosinase inhibitory activity was expressed using IC50 values and as equivalents of kojic acid (mg KAEs/g sample).
Cholinesterase Inhibition Activity
The inhibitory effects of cholinesterase including acetylcholinesterase (AChE) and butyrylcholinesterase (BChE) were evaluated according to Ellman’s method with slight modification (Ellman et al., 1961). Sample solution (50 μL) was mixed AChE or BuChE solution (pH 8.0, 10 μL, 0.5 U/mL) in a 96-well plate and incubated at 4°C for 15 min. Subsequently, acetylthiocholine iodide (ATCI) or butyrylthiocholine chloride (BTCl) solution (20 μL, 2mM) and 5,5′-dithiobis-(2-nitrobenzoic acid) (DTNB) solution (20 μL, pH 8.0, 2 mM) were added and incubated at 37°C for 30 min. After that, the reaction was stopped by adding 20 μL of 0.018 mM physostigmine, and finally the absorbance was read at 405 nm. Galanthamine was used as a positive reference. The cholinesterase inhibitory effects were expressed using IC50 values and as equivalents of galanthamine (mg GALAEs/g sample).
Statistical Analysis
All data were repeated at least three times and expressed as mean ± standard deviation (SD). SPSS software (version 19.0) was used for statistical analysis. The significant difference between the two groups was compared using the two-tailed unpaired t-test (P < 0.05).
Results and Discussion
Chemical Composition of EO
The hydrodistillation of H. flavum fresh rhizomes yielded EO at 0.56% (w/w) on fresh weight basis. Seventy-seven chemical constituents of EO were identified by GC-MS/FID, representing 96.5% of the composition (Table 1). The major constituents of H. flavum EO were coronarin E (20.3%), β-pinene (16.8%), E-nerolidol (11.8%), linalool (8.5%), 1,8-cineole (5.5%), α-pinene (5.4%), α-terpineol (3.8%), α-terpinyl acetate (2.4%), and α-curcumene (2.0%), which were presented in Figure 1. Previous studies have showed that the main compounds of H. flavum rhizome EO were α-pinene (4.3–12.1%), β-pinene (21.8–24.77%), 1,8-cineole (8.90–28.3%), and linalool (6.29–17.5%) (Sakhanokho et al., 2013; Thanh et al., 2014; Ray et al., 2018a). Furthermore, the main EO components in leaf were β-pinene (22.5%), α-humulene (15.7%) and β-caryophyllene (10.4%), as well as the major EO compounds of stem were α-humulene (18.9%), β-caryophyllene (11.8%), and β-pinene (11.2%) (Thanh et al., 2014). The main EO components in aerial parts of H. flavum were β-pinene (49.6%), β-caryophyllene (26.9%), α-pinene (5.9%), sabinene (4.2%), and α-humulene (2.3%) (Mollenbeck et al., 1997). However, in our study, the coronarin E is the most predominant constituent, which has not been detected in previous H. flavum EO studies. The coronarin E has previously been reported in other Hedychium species EO, such as H. coronarium (Rodrigues et al., 2013; Ray et al., 2018b; Ray et al., 2019), H. acumnatum (Weyerstahl et al., 1995) and H. gardnerianum (Weyerstahl et al., 1998). To our knowledge, the coronarin E, as the most predominant constituent, was identified for the first time in H. flavum EO.
Total Phenolic and Flavonoid Contents
The WE presented a higher yield at 2.04% (w/w) on fresh weight basis than the EE (1.37%). The TPC and TFC of H. flavum rhizome WE and EE were shown in Figure 2. The TPC of WE and EE were 50.08 ± 0.71 and 57.42 ± 0.07 gallic acid equivalents (mg GAEs/g) of dry weight of extract, respectively. The TFC in WE and EE were 12.45 ± 1.01 and 21.83 ± 0.33 rutin equivalents (mg REs/g) of dry weight of extract, respectively. Statistical analysis indicated that TPC and TFC of EE were significantly higher (p< 0.01) than that of WE. Plant polyphenols and flavonoids have been widely used in various medicines and food products due to their wide range of pharmacological activities, such as antioxidants, antimicrobial, anticancer, anti-inflammatory, hepatoprotective, antiviral, antiatherosclerotic, antispasmodic, and enzyme inhibitory activities (Fraga, 2010; Agrawal, 2011; Murti and Sharma, 2017). The H. flavum rhizome WE and EE exhibited high TPC and TFC values, which can be used as a rich source of phenolics and flavonoids.
Identification of WE and EE Compounds by UHPLC-Q-Orbitrap-MS
The WE and EE compounds were investigated by UHPLC-Q-Orbitrap-MS using negative and positive ion mode scanning. According to the primary and secondary high-resolution mass spectrometry (MS1/MS2) and comparative analysis using mzCloud, ChemSpider, and mzCloud databases, a total of 86 compounds were putatively identified (Supplementary Material, Table S1). The WE and EE showed differentiation in chemical composition (Supplementary Material, Figure S1). Seventy-one compounds in WE and forty-three components in EE were identified, respectively. Considering the high TPC value of WE and EE, 13 identified phenolic compounds were listed in Figure 3, including L-tyrosine (6), p-coumaric acid (12), cycloolivil (13), ferulaldehyde (16), 1,7-bis(4-hydroxyphenyl)-3,5-heptanediol (17), (-)-5’-desmethylyatein (20), 2-methoxyestradiol (25), 3,5-di-tert-butyl-4-hydroxybenzaldehyde (42), carnosol (44), 5-[(2Z,8Z)-2,8-pentadecadien-1-yl]-1,3-benzenediol (60), ginkgolic acid (C13:0) (62), 2,2’-methylenebis(4-methyl-6-tert-butylphenol) (70), and 2,7-dihydroxycadalene (76). All 86 compounds were identified from H. flavum for the first time.
Antioxidant Activity
The antioxidant activity of EO, WE, and EE was evaluated using DPPH and ABTS methods, and BHT and ascorbic acid were used as positive controls (Table 2). The EO showed low antioxidant activity in both methods. The WE (IC50: 15.36 ± 0.15 μg/mL) and EE (IC50: 16.24 ± 0.61 μg/mL) exhibited significantly stronger DPPH free radical scavenging capacity than BHT (IC50: 109.40 ± 9.68 μg/mL), but lower than ascorbic acid (IC50: 9.39 ± 0.79 μg/mL) (p < 0.05). Base on ABTS analysis, the WE (IC50: 16.83 ± 0.45 μg/mL) and EE (IC50: 12.37 ± 0.13 μg/mL) showed significantly stronger antioxidant ability than both BHT (IC50: 18.86 ± 0.51 μg/mL), and ascorbic acid (IC50: 29.98 ± 1.77μg/mL) (p < 0.05). The free radical scavenging capacity of WE and EE was higher in ABTS assay (1783.16 ± 137.57 and 2424.29 ± 158.31, respectively) than in DPPH assay (611.13 ± 48.36 and 578.62 ± 54.10, respectively), expressed as ascorbic acid equivalents per gram of extract. Previous studies have shown that the DPPH and ABTS free radical scavenging capabilities of plant extracts were positively correlated with their TPC and TFC (Shin et al., 2015; Baloglu et al., 2019). Thus, the antioxidant capacity of WE and EE may be due to the high levels of TPC and TFC. The free radical scavenging agents can be used as a preventive medicine for age-related diseases and cancer (Khansari et al., 2009). The BHT, a synthetic antioxidant, is used as preservative in foods. However, as a food additive, the BHT is rejected by consumers due to its adverse reactions (Imaida et al., 1983; Simon, 2003). Interestingly, the WE and EE showed higher antioxidant activity compared with the BHT. These results indicate that WE and EE can be used as potential substitutes for synthetic antioxidants in the pharmaceutical and food industries. The authors limited the results of the DPPH analysis.
Antibacterial Activity
The antibacterial properties of EO, WE, and EE were qualitatively determined by the diameter of inhibition zones (DIZ) and quantitatively evaluated by MIC and MBC values using streptomycin as positive control (Table 3). The antibacterial property of EO was higher than that of WE and EE. Both WE and EE displayed very low or no inhibition against the tested bacterial strains. The EO revealed significant antibacterial activity against Pseudomonas aeruginosa, Bacillus subtilis, Staphylococcus aureus, and Proteus vulgaris with DIZ values ranged from 10.34 to 24.43 mm, MIC values ranged from 78.13 to 312.50 μg/mL, and MBC values ranged from 156.25 to 625.00 μg/mL. The major constituents of H. flavum EO, such as coronarin E, β-pinene, E-nerolidol, linalool, α-terpineol, α-pinene, and 1,8-cineole, have been demonstrated to have antibacterial activity (Sokovic et al., 2008; Park et al., 2012; Hartati et al., 2015; Silva et al., 2015; Kiryu et al., 2018). Hence, H. flavum EO possessed significant antibacterial ability, probably due to these major constituents, and it can provide natural antibacterial agents for the pharmaceutical industry.
Cytotoxic Activity
The cytotoxic activities of EO, WE, and EE were evaluated against human tumor cell lines (A549, NCI-H1299, PC-3, and K562), human normal cell line (MRC-5), and murine fibroblast cell line (L929) using the MTT method with cisplatin as positive control (Table 4). The EO showed higher inhibitory property on the test cell lines than WE and EE. Both WE and EE displayed very low cytotoxic activity against tumor and non-cancerous cell lines, indicating that WE and EE were less-toxic. The EO exhibited significant cytotoxicity against A549 (IC50 = 72.86 ± 6.39 μg/mL), NCI-H1299 (IC50 = 70.74 ± 9.56 μg/mL), PC-3 (IC50 = 63.16 ± 9.20 μg/mL), and K562 (IC50 = 27.16 ± 2.18 μg/mL) human tumor cell lines. Additionally, the EO displayed a considerable selectivity to human tumor cell K562, and its toxicity was more than 3.5-fold different from that of non-cancerous MRC-5 cell (IC50 = 95.96 ± 4.37μg/mL) and L929 cell (IC50 = 129.91 ± 5.27 μg/mL). According to previous studies, the coronarin E, as the most predominant constituent in H. flavum EO, had higher cytotoxicity to human tumor cell lines (NCI-H187, HL-60, THP-1, and A-549) (IC50: 31.21–53.26 μM) compared to non-cancerous Vero cell (IC50: 150.45 μM), with selective cytotoxicity against tumor cells (Reddy et al., 2009; Kumrit et al., 2010). The cytotoxic properties of other main components in EO, such as 1,8-cineole, β-pinene, α-pinene, E-nerolidol, linalool, and α-curcumene, have been demonstrated in previous reports (Hassan et al., 2010; Shin and Lee, 2013; Dall’Acqua et al., 2017; Salehi et al., 2019). Therefore, the presence of these main components could explain the significant cytotoxic activities of the H. flavum rhizome EO. These results indicate that the H. flavum EO can be used as a new source of natural antitumor active small molecules in the pharmaceutical industry.
EO Induces Apoptosis in K562 Cells
Morphology assay indicated that EO triggered obvious morphological changes with typical characteristics of apoptosis, such as cell shrinkage and fragmentation in K562 cells (Figure 4A). AO/EB staining assay showed that the number of apoptotic cells with red fluorescence increased, while the number of viable cells with bright green fluorescence decreased when the cells were treated with increasing concentrations of EO (Figure 4B). Hoechst 33258 staining revealed that the nucleus of EO-treated cells presented more and brighter blue-fluorescence compared with the control group, which indicated typical apoptotic morphology (Figure 4C). Furthermore, to quantitatively determine the apoptotic effect of the EO, Annexin V-FITC/PI staining assay was performed using a flow-cytometer. As shown in Figure 5, the percentage of apoptotic cells (Q2+Q3) was enhanced to 13.72 ± 0.42% (at 30 μg/mL) and 15.48 ± 0.22% (at 60 μg/mL) in comparison to the control (6.14 ± 0.94%), which suggested that EO triggered apoptosis against K562 cells in a dose-dependent manner.
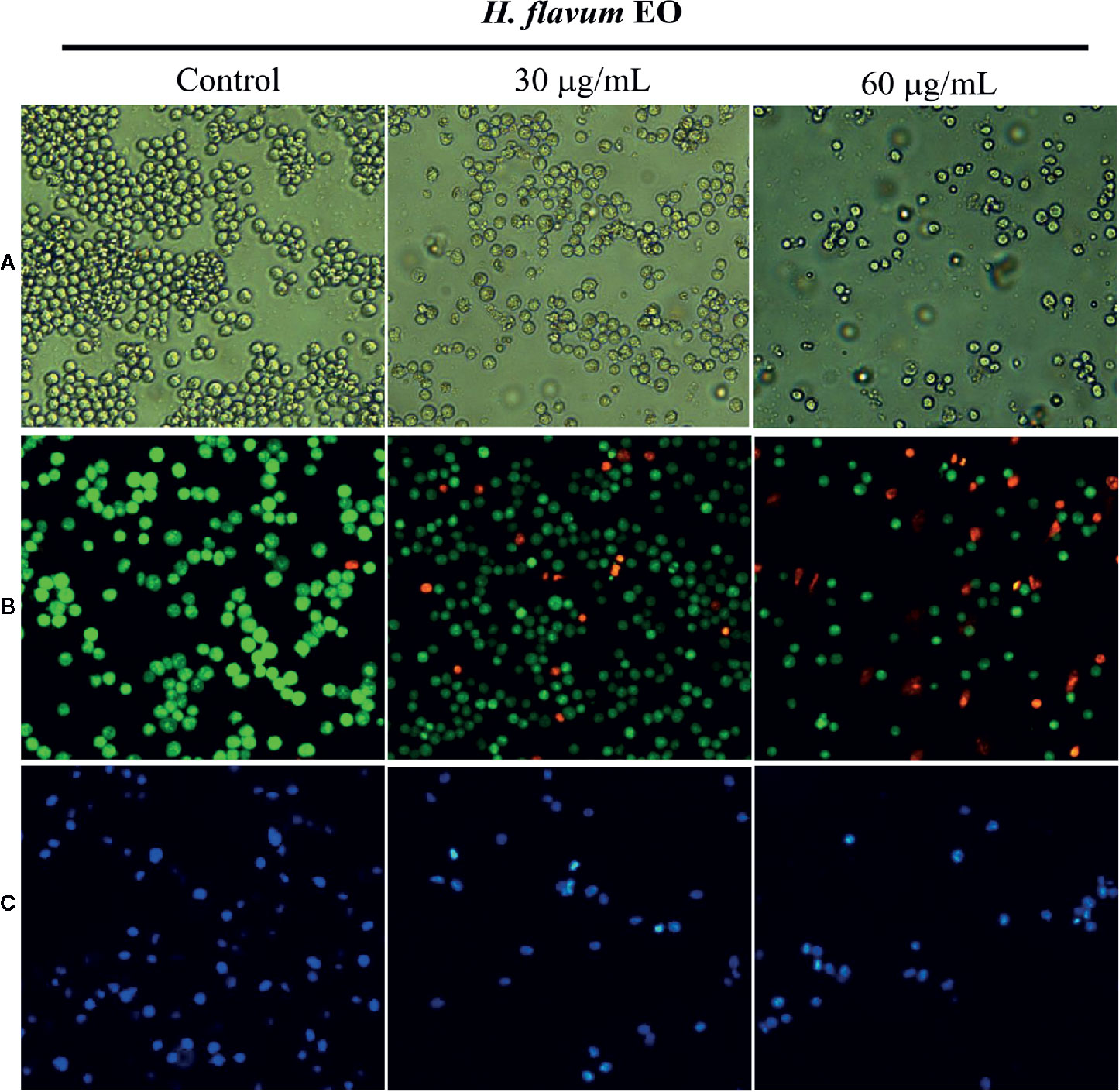
Figure 4 Effect of H. flavum EO on the morphology of K562 cells. (A) The cells were treated with EO (0, 30, 60 μg/mL) and the morphological changes of the cells were observed using an inverted microscope (100×). The cells were treated as described above and then stained with AO/EB (B) and Hoechst 33258 (C). The morphological changes were observed using a fluorescence microscope (100×).
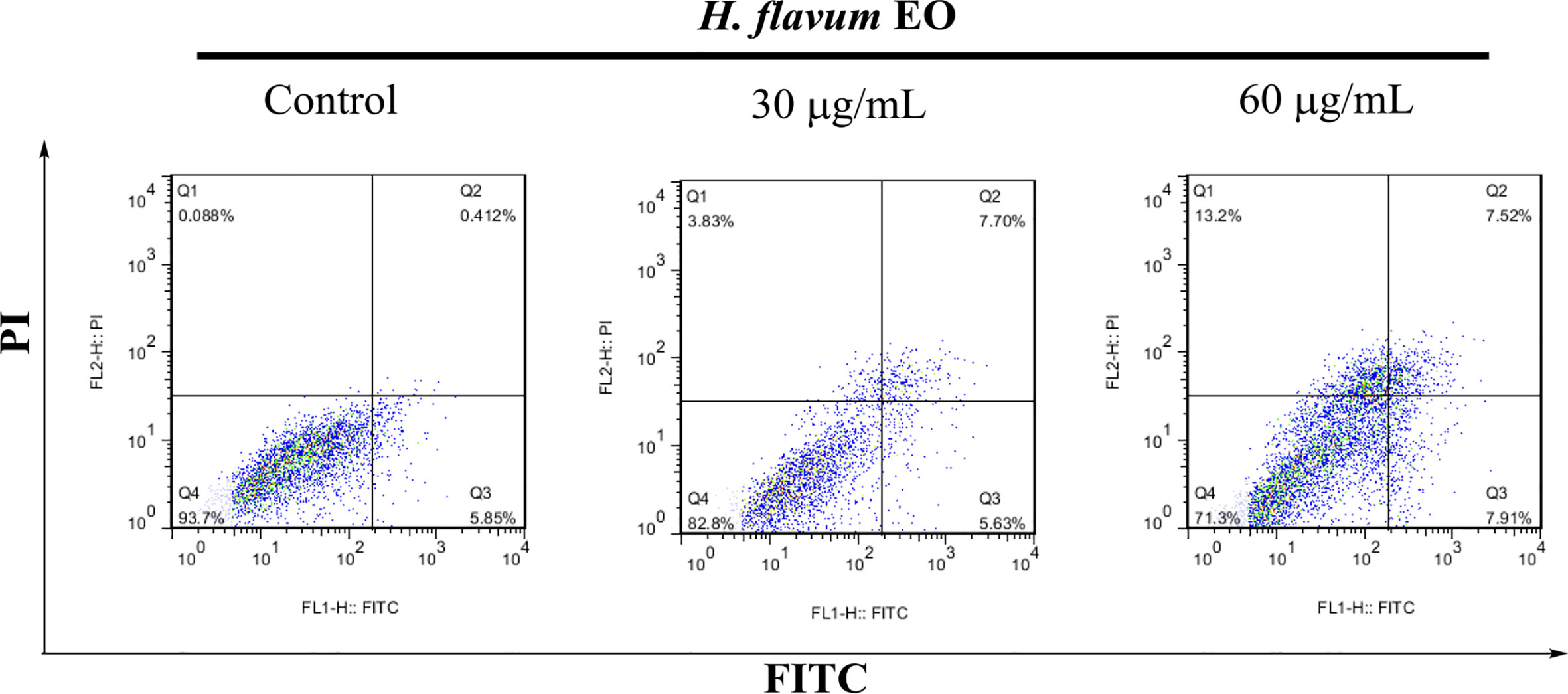
Figure 5 Apoptosis analysis of K562 cells treated with H. flavum EO using a flow-cytometer. Cells in upper left quadrant (Q1-UL: AV–/PI+): necrotic cells; upper right quadrant (Q2-UR: AV+/PI+): late apoptotic cells; lower right quadrant (Q3-LR: AV+/PI–): early apoptotic cells; lower left quadrant (Q4-LL: AV–/PI–): live cells.
Enzyme Inhibitory Activities
The inhibitory effect of H. flavum rhizome EO, WE, and EE on α-glucosidase, tyrosinase, and two cholinesterases (AChE and BChE) were investigated and the results are shown in Table 5.
It is well known that α-glucosidase inhibitors reduce post-meal blood glucose and insulin levels by delaying the absorption of carbohydrates in the small intestine. Therefore, α-glucosidase inhibitors are used to treat type 2 diabetes (van de Laar et al., 2005). In this study, the inhibitory effect of α-glucosidase could be summarized with the following order: WE (IC50 = 0.064 ± 0.004 mg/mL) > EE (IC50 = 0.19 ± 0.01 mg/mL) > acarbose (IC50 = 0.25 ± 0.02 mg/mL) > EO (IC50 = 4.15 ± 0.26 mg/mL) (p < 0.05), which were presented in Table 5 and Figure 6. H. flavum rhizome WE (6.02 ± 0.79 mmoL ACEs/g sample) and EE (2.04 ± 0.20 mmoL ACEs/g sample) showed higher α-glucosidase inhibitory capacity than EO (0.094 ± 0.002 ACEs/g sample), expressed as acarbose equivalents per gram of extract. Interesting findings in this study are that WE and EE had higher α-glucosidase inhibitory activity than acarbose. Hence, H. flavum rhizome WE and EE could be a promising α-glucosidase inhibitor, which could be developed as a therapeutic product for type 2 diabetes.
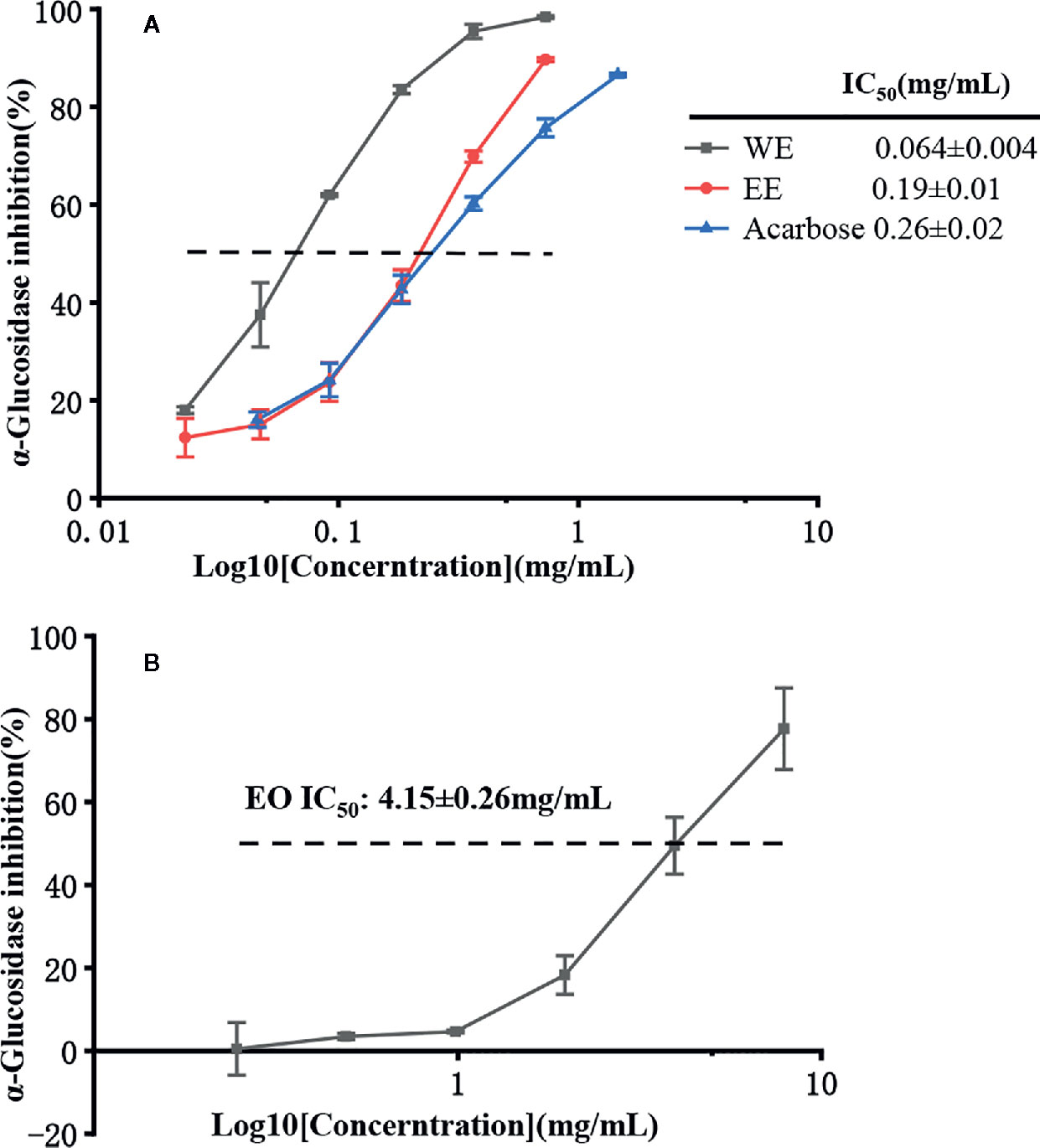
Figure 6 α-Glucosidase inhibitory activity of EO, WE, EE, and acarbose. (A) The inhibitions of WE, EE, and acarbose. (B) The inhibition of EO.
Tyrosinase is a key enzyme involved in the catalysis of mammalian melanogenesis and the enzymatic browning reaction in fruits and vegetables. Therefore, tyrosinase inhibitors are used to control enzymatic browning in foods and for treatment of hyperpigmentation in human skin (Chang, 2009). As shown in Table 5, the best inhibitory effect on tyrosinase was provided by the EO (IC50 = 6.12 ± 0.16 mg/mL, 32.51 ± 0.82 mg KAEs/g sample) followed by the EE (IC50 = 9.62 ± 0.94 mg/mL, 20.85 ± 1.12 mg KAEs/g sample) and WE (IC50 = 10.49 ± 1.48 mg/mL, 19.30 ± 2.72 mg KAEs/g sample). However, compared to positive control kojic acid (IC50 = 0.20 ± 0.01 mg/mL), all of EO, WE, and EE didn’t show a promising inhibition on tyrosinase.
Cholinesterase inhibitors have become the most effective treatment strategy for Alzheimer’s disease by inhibiting the breakdown of acetylcholine to enhance cholinergic neurotransmission (Grutzendler and Morris, 2001). As shown in Table 5, the WE was the most effective AChE inhibitor (IC50 = 2.27 ± 0.13 mg/mL, 0.108 ± 0.011 mg GALAEs/g sample) and BChE (IC50 = 0.65 ± 0.04 mg/mL, 7.24 ± 0.26 mg GALAEs/g sample). Compared with the positive control drug galanthamine, H. flavum rhizome EO, WE, and EE showed weak cholinesterase inhibitory activity.
According to previous studies, the IC50 values of α-glucosidase inhibitory effect of 1,8-cineole and α-pinene were 1.118 and 1.420 μg/mL, respectively (Basak and Candan, 2013). α-terpineol, α-pinene, and 1,8-cineole showed significant tyrosinase inhibitory effect (Chao et al., 2017). The significant inhibited AChE activity of 1,8-cineole, β-pinene, α-pinene, and linalool has been demonstrated in previous studies (Abdelgaleil et al., 2009; Kim et al., 2013; Seo et al., 2015). Additionally, the linalool-rich EO was reported to exhibit strong α-glucosidase, tyrosinase, and cholinesterases (AChE and BChE) inhibitory activity (Sarikurkcu et al., 2015). Therefore, the enzyme inhibitory activities of EO may be due to these main components.
Previous studies have indicated that phenolic compounds play an important role in enzyme inhibitory activity (Gonçalves and Romano, 2017). In this study, the α-glucosidase, and cholinesterases (AChE and BChE) inhibitory activities of WE were significantly higher than that of EE (p<0.05), except for not significant difference in tyrosinase inhibitory effect. However, WE revealed the significantly lower TPC and TFC compared with EE. This finding could be explained by the presence of non-phenolic and non-flavonoid enzyme inhibitors in the extract. Furthermore, UHPLC-Q-Orbitrap-MS analysis showed that many bioactive compounds may contribute to the enzyme inhibitory effect of the extract. For instance, the tyrosinase inhibitory activity of p-Coumaric acid (12) (IC50 = 0.66 μg/mL) was much higher than positive control arbutin (IC50 > 100 μg/mL), and p-Coumaric acid showed a moderate α-glucosidase inhibitory activity (Pei et al., 2016). According to Ma et al. (2020), carnosol (44) displayed a competitive inhibition (Ki =5.57 μg/mL) on α-glucosidase with IC50 value of 12.0 ± 0.8 μg/mL, and showed much stronger inhibitory effect than acarbose (IC50 = 412 ± 26 μg/mL). Ginkgolic acid (C13:0) (62) revealed higher α-glucosidase inhibitory activity (IC50 = 3.5 ± 0.13 μg/mL) than the positive control quercetin (IC50 = 3.8 ± 0.12 μg/mL) (Sukito and Tachibana, 2014).
Conclusions
To our knowledge, the antioxidant, antibacterial, cytotoxic, and enzyme inhibitory activities of essential oil (EO), as well as the chemical composition and bioactivities of water extract (WE) and 70% ethanol extract (EE) from H. flavum rhizome were reported for the first time. The coronarin E was the most main constituent in EO and was first detected in H. flavum. The WE and EE exhibited high TPC and TFC values. Moreover, 86 compounds were putatively identified from WE and EE using UHPLC-Q-Orbitrap-MS, and all the compounds were reported in H. flavum for the first time. In addition to the low antioxidant effect of EO and the weak inhibitory activity of EO and extracts on tyrosinase and cholinesterase, EO demonstrated significant antibacterial activity against P. vulgaris, P. aeruginosa, B. subtilis, and S. aureus, and showed significant α-glucosidase inhibitory effect. Besides, EO exhibited a considerable selectivity to human tumor cell K562, and its toxicity was more than 3.5-fold different from that of non-cancerous MRC-5 and L929 cells. A series of apoptosis analysis suggested that EO triggered apoptosis against K562 cells in a dose-dependent manner. The very low inhibition against the tumor and non-cancerous cell lines suggested that WE and EE were less-toxic. Interesting findings in this study are that WE and EE displayed remarkable antioxidant and α-glucosidase inhibition activities, being superior to their respective positive control drugs BHT and acarbose. Hence, H. flavum could be considered as a source of bioactive compounds and has high exploitation potential in the cosmetics, food, and pharmaceutical industries.
Data Availability Statement
The raw data supporting the conclusions of this article will be made available by the authors, without undue reservation, to any qualified researcher.
Author Contributions
YZ and MT conceived and designed the experiments. MT and XW performed the experiments. TL, XZ, and FW analyzed the data. MT and GD drafted and revised the manuscript. All authors contributed to the article and approved the submitted version.
Conflict of Interest
The authors declare that the research was conducted in the absence of any commercial or financial relationships that could be construed as a potential conflict of interest.
Acknowledgments
The authors are grateful for the financial support grant from the National Key R&D Program of China (2018YFC1708100); Guizhou Science and Technology Program [Qian Ke He Zhi Cheng (2017) 2850, and Qian Ke He Ping Tai Ren Cai (2018) 5781-49; Guizhou Medical Products Administration [DB52/YC12-2013].
Supplementary Material
The Supplementary Material for this article can be found online at: https://www.frontiersin.org/articles/10.3389/fphar.2020.572659/full#supplementary-material
References
Abdelgaleil, S. A. M., Mohamed, M., II, Badawy, M. E., II, El-arami, S. A. A. (2009). Fumigant and contact toxicities of monoterpenes to Sitophilus oryzae (L.) and Tribolium castaneum (Herbst) and their inhibitory effects on acetylcholinesterase activity. J. Chem. Ecol. 35, 518–525. doi: 10.1007/s10886-009-9635-3
Agrawal, A. D. (2011). Pharmacological activities of flavonoids: a review. Int. J. Pharm. Sci. Nanotechnol. 4 (2), 1394–1398. doi: 10.37285/ijpsn.2011.4.2.3
Baloglu, M. C., Llorent-Martínez, E. J., Aumeeruddy, M. Z., Mahomoodally, M. F., Altunoglu, Y. C., Ustaoglu, B., et al. (2019). Multidirectional insights on Chrysophyllum perpulchrum leaves and stem bark extracts: HPLC-ESI-MSn profiles, antioxidant, enzyme inhibitory, antimicrobial and cytotoxic properties. Ind. Crops Prod. 134, 33–42. doi: 10.1016/j.indcrop.2019.03.066
Basak, S. S., Candan, F. (2013). Effect of Laurus nobilis L. essential oil and its main components on α-glucosidase and reactive oxygen species scavenging activity. Iran. J. Pharm. Res. 12 (2), 367–379. doi: 10.22037/IJPR.2013.1315
Blois, M. S. (1958). Antioxidant determinations by the use of a stable free radical. Nature 181, 1199–1200. doi: 10.1038/1811199a0
Chang, T. S. (2009). An updated review of tyrosinase inhibitors. Int. J. Mol. Sci. 10, 2440–2475. doi: 10.3390/ijms10062440
Chao, W. W., Su, C. C., Peng, H. Y., Chou, S. T. (2017). Melaleuca quinquenervia essential oil inhibits α-melanocyte-stimulating hormone-induced melanin production and oxidative stress in B16 melanoma cells. Phytomedicine 34, 191–201. doi: 10.1016/j.phymed.2017.08.024
China Pharmacopoeia Committee (2015). Pharmacopoeia of the People"s Republic of China (Beijing: China Medical Science and Technology Press), 396–397.
CHMC-Chinese Herbal Medicine Company (1994). The Chinese Traditional Medicine Resource Records (Beijing: Science Press), pp.1511.
Dall’Acqua, S., Peron, G., Ferrari, S., Gandin, V., Bramucci, M., Quassinti, L., et al. (2017). Phytochemical investigations and antiproliferative secondary metabolites from Thymus alternans growing in Slovakia. Pharm. Biol. 55, 1162–1170. doi: 10.1080/13880209.2017.1291689
Devi, N. B., Singh, P. K., Das, A. K. (2014). Ethnomedicinal utilization of Zingiberaceae in the valley districts of Manipur. J. Environ. Sci. Toxicol. Food Technol. 8 (2), 21–23. doi: 10.9790/2402-08242123
Ellman, G. L., Courtney, K. D., Andres, V., Featherston, R. M. (1961). A new and rapid colorimetric determination of acetylcholinesterase activity. Biochem. Pharmacol. 7, 88–95. doi: 10.1016/0006-2952(61)90145-9
Eloff, J. N. (1998). A sensitive and quick microplate method to determine the minimal inhibitory concentration of plant extracts for bacteria. Planta Med. 64, 711–713. doi: 10.1055/s-2006-957563
Fraga, C. G. (2010). Plant Phenolics and Human Health: Biochemistry, Nutrition, and Pharmacology (Hoboken, NJ: John Wiley & Sons, Inc., Publication).
Gonçalves, S., Romano, A. (2017). “Inhibitory properties of phenolic compounds against enzymes linked with human diseases,” in Phenolic Compounds-Biological Activity. Ed. Soto-Hernández, M. (London: InTech), 581–770.
Grutzendler, J., Morris, J. C. (2001). Cholinesterase inhibitors for Alzheimer’s disease. Drugs 61 (1), 41–52. doi: 10.2165/00003495-200161010-00005
Hartati, R., Suganda, A. G., Fidrianny, I. (2014). Botanical, phytochemical and pharmacological properties of Hedychium (Zingiberaceae) - a review. Proc. Chem. 13, 150–163. doi: 10.1016/j.proche.2014.12.020
Hartati, R., Suganda, A. G., Fidrianny, I. (2015). Chemical composition and antimicrobial activity of diterpene and essential oils of Hedychium roxburghii Blume rhizome. Asian J. Pharm. Clin. Res. 8 (5), 209–214.
Hassan, S. B., Gali-Muhtasib, H., Göransson, H., Larsson, R. (2010). Alpha terpineol: a potential anticancer agent which acts through suppressing NF-κB signalling. Anticancer Res. 30, 1911–1919.
Imaida, K., Fukushima, S., Shirai, T., Ohtani, M., Nakanishi, K., Ito, N. (1983). Promoting actions of butylated hydroxyanisole and butylated hydroxytoluene on 2-stage urinary bladder carcinogenesis and inhibition of γ-glutamyl transpeptidase-positive foci development in the liver of rats. Carcinogenesis 4, 895–899. doi: 10.1093/carcin/4.7.895
Khansari, N., Shakiba, Y., Mahmoudi, M. (2009). Chronic inflammation and oxidative stress as a major cause of age-related diseases and cancer. Recent Pat. Inflamm. Allergy Drug Discovery 3, 73–80. doi: 10.2174/187221309787158371
Kim, S. W., Kang, J., Park, I. K. (2013). Fumigant toxicity of Apiaceae essential oils and their constituents against Sitophilus oryzae and their acetylcholinesterase inhibitory activity. J. Asia-Pac. Entomol. 16, 443–448. doi: 10.1016/j.aspen.2013.07.002
Kiryu, M., Hamanaka, M., Yoshitomi, K., Mochizuki, S., Akimitsu, K., Gomi, K. (2018). Rice terpene synthase 18 (OsTPS18) encodes a sesquiterpene synthase that produces an antibacterial (E)-nerolidol against a bacterial pathogen of rice. J. Gen. Plant Pathol. 84, 221–229. doi: 10.1007/s10327-018-0774-7
Kumrit, I., Suksamrarn, A., Meepawpan, P., Songsri, S., Nuntawong, N. (2010). Labdane-type diterpenes from Hedychium gardnerianum with potent cytotoxicity against human small cell lung cancer cells. Phytother. Res. 24, 1009–1013. doi: 10.1002/ptr.3057
Ma, Y. Y., Zhao, D. G., Zhang, R. Q., He, X., Li, B. Q., Zhang, X. Z., et al. (2020). Identification of bioactive compounds that contribute to the α-glucosidase inhibitory activity of rosemary. Food Funct. 11, 1692–1701. doi: 10.1039/C9FO02448D
Mollenbeck, S., Konig, T., Schreier, P., Schwab, W., Rajaonarivony, J., Ranarivelo, L. (1997). Chemical composition and analyses of enantiomers of essential oils from Madagascar. Flav. Fragr. J. 12, 63–69. doi: 10.1002/(SICI)1099-1026(199703)12:2<63::AID-FFJ614>3.0.CO;2-Z
Murti, Y., Sharma, S. (2017). Flavonoid: a pharmacologically significant sacffold. World J. Pharm. Pharm. Sci. 6 (5), 488–504. doi: 10.20959/wjpps20175-9143
Mzid, M., Ben Khedir, S., Ben Salem, M., Regaieg, W., Rebai, T. (2017). Antioxidant and antimicrobial activities of ethanol and aqueous extracts from Urtica urens. Pharm. Biol. 55 (1), 775–781. doi: 10.1080/13880209.2016.1275025
Pan, X., Zhang, X. (2013). Rapid Propagation of Hedychium flavum Roxb. J. Trop. Biol. 4 (2), 189–193. doi: 10.15886/j.cnki.rdswxb.2013.02.014
Park, S. N., Lim, Y. K., Freire, M. O., Cho, E., Jin, D., Kook, J. K. (2012). Antimicrobial effect of linalool and α-terpineol against periodontopathic and cariogenic bacteria. Anaerobe 18, 369–372. doi: 10.1016/j.anaerobe.2012.04.001
Pei, K., Ou, J. Y., Huang, J. Q., Ou, S. Y. (2016). p-Coumaric acid and its conjugates: dietary sources, pharmacokinetic properties and biological activities. J. Sci. Food Agr. 96, 2952–2962. doi: 10.1002/jsfa.7578
Prakash, O., Chandra, M., Punetha, H., Pant, A. K., Rawat, D. S. (2016). “Chapter 84-Spiked Ginger Lily (Hedychium spp.) Oils,” in Essential Oils in Food Preservation, Flavor and Safety Preedy, V. (London: Academic Press), 737–750. doi: 10.1016/B978-0-12-416641-7.00084-5
Rajasekaran, K., Sakhanokho, H. F., Tabanca, N. (2012). Antifungal activities of Hedychium essential oils and plant extracts against mycotoxigenic fungi. J. Crop Improv. 26, 389–396. doi: 10.1080/15427528.2011.649395
Ray, A., Jena, S., Kar, B., Sahoo, A., Panda, P. C., Nayak, S., et al. (2018a). Volatile metabolite profiling of ten Hedychium species by gas chromatography mass spectrometry coupled to chemometrics. Ind. Crop Prod. 126, 135–142. doi: 10.1016/j.indcrop.2018.10.012
Ray, A., Jena, S., Dash, B., Kar, B., Halder, T., Chatterjee, T., et al. (2018b). Chemical diversity, antioxidant and antimicrobial activities of the essential oils from Indian populations of Hedychium coronarium Koen. Ind. Crops Prod. 112, 353–362. doi: 10.1016/j.indcrop.2017.12.033
Ray, A., Jena, S., Haldar, T., Sahoo, A., Kar, B., Patnaik, J., et al. (2019). Population genetic structure and diversity analysis in Hedychium coronarium populations using morphological, phytochemical and molecular markers. Ind. Crops Prod. 132, 118–133. doi: 10.1016/j.indcrop.2019.02.015
Re, R., Pellegrini, N., Proteggente, A., Pannala, A., Yang, M., Rice-Evans, C. (1999). Antioxidant activity applying an improved ABTS radical cation decolorization assay. Free Radic. Biol. Med. 26, 1231–1237. doi: 10.1016/S0891-5849(98)00315-3
Reddy, P. P., Rao, R. R., Shashidhar, J., Sastry, B. S., Rao, J. M., Babu, K. S. (2009). Phytochemical investigation of labdane diterpenes from the rhizomes of Hedychium spicatum and their cytotoxic activity. Bioorg. Med. Chem. Lett. 19, 6078–6081. doi: 10.1016/j.bmcl.2009.09.032
Rodrigues, D. F., Arenas Velásquez, A. M., Cavaleiro, C., Salgueiro, L., Martins, G. Z., Magalhães, N. O., et al. (2013). Chemical composition and trypanocidal activity of the essential oils from Hedychium coronarium J. Koenig (Zingiberaceae). ISRN Infect. Dis. 2013, 639275. doi: 10.5402/2013/639275
Sakhanokho, H. F., Rajasekaran, K. (2019). “Hedychium Essential Oils: Composition and Uses,” in Essential Oil Research. Ed. Malik, S. (Cham: Springer), 49–60.
Sakhanokho, H. F., Sampson, B. J., Tabanca, N., Wedge, D. E., Demirci, B., Baser, K. H. C., et al. (2013). Chemical composition, antifungal and insecticidal activities of Hedychium essential oils. Molecules 18, 4308–4327. doi: 10.3390/molecules18044308
Salehi, B., Upadhyay, S., Orhan, E., II, Jugran, K. A., Jayaweera, L. D. S., Dias, A. D., et al. (2019). Therapeutic potential of α- and β-Pinene: a miracle gift of nature. Biomolecules 9, 738. doi: 10.3390/biom9110738
Sarikurkcu, C., Zengin, G., Oskay, M., Uysal, S., Ceylan, R., Aktumsek, A. (2015). Composition, antioxidant, antimicrobial and enzyme inhibition activities of two Origanum vulgare subspecies (subsp. vulgare and subsp. hirtum) essential oils. Ind. Crops Prod. 70, 178–184. doi: 10.1016/j.indcrop.2015.03.030
Seo, S. M., Jung, C. S., Kang, J., Lee, H. R., Kim, S. W., Hyun, J., et al. (2015). Larvicidal and acetylcholinesterase inhibitory activities of Apiaceae plant essential oils and their constituents against Aedes albopictus and formulation development. J. Agr. Food Chem. 63, 9977–9986. doi: 10.1021/acs.jafc.5b03586
Shin, Y., Lee, Y. (2013). Cytotoxic activity from Curcuma zedoaria through mitochondrial activation on ovarian cancer cells. Toxicol. Res. 29, 257–261. doi: 10.5487/TR.2013.29.4.257
Shin, G. R., Lee, S., Lee, S., Do, S. G., Shin, E., Lee, C. H. (2015). Maturity stage-specific metabolite profiling of Cudrania tricuspidata and its correlation with antioxidant activity. Ind. Crops Prod. 70, 322–331. doi: 10.1016/j.indcrop.2015.01.048
Silva, G. N. S. D., Pozzatti, P., Rigatti, F., Hörner, R., Alves, S. H., Mallmann, C. A., et al. (2015). Antimicrobial evaluation of sesquiterpene α-curcumene and its synergism with imipenem. J. Microbiol. Biotechnol. Food Sci. 4 (5), 434–436. doi: 10.15414/jmbfs.2015.4.5.434-436
Silva, T. C., Justino, A. B., Prado, D. G., Koch, G. A., Martins, M. M., Santos, P. S., et al. (2019). Chemical composition, antioxidant activity and inhibitory capacity of α-amylase, α-glucosidase, lipase and non-enzymatic glycation, in vitro, of the leaves of Cassia bakeriana Craib. Ind. Crops Prod. 140, 111641. doi: 10.1016/j.indcrop.2019.111641
Simon, R. A. (2003). Adverse reactions to food additives. Curr. Allergy Asthma. Rep. 3, 62–66. doi: 10.1007/s11882-003-0014-9
Slinkard, K., Singleton, V. L. (1977). Total phenol analysis: automation and comparison with manual methods. Am. J. Enol. Viticult. 28, 49–55.
Sokovic, M., Marin, P. D., Brkic, D., van Griensven, L. J. L. D. (2008). Chemical composition and antibacterial activity of essential oils against human pathogenic bacteria. Food 1 (2), 220–226.
Sukito, A., Tachibana, S. (2014). Potent α-glucosidase inhibitors isolated from Ginkgo biloba leaves. Pak. J. Biol. Sci. 17 (11), 1170–1178. doi: 10.3923/pjbs.2014.1170.1178
Tavares, W. R., Barreto, M. C., Seca, A. M. L. (2020). Uncharted source of medicinal products: the case of the Hedychium genus. Medicines 7, 23. doi: 10.3390/medicines7050023
Thanh, B. V., Dai, D. N., Thang, T. D., Binh, N. Q., Anh, L. D. N., Ogunwande, I. A. (2014). Composition of essential oils of four Hedychium species from Vietnam. Chem. Cent. J. 8, 54. doi: 10.1186/s13065-014-0054-3
The Plant List (2010). Version 1. Published on internet. http://www.theplantlist.org/browse/A/Zingiberaceae/Hedychium/ (Accessed May 30, 2020).
Tian, M. Y., Wu, X. H., Hong, Y., Wang, H. J., Deng, G. D., Zhou, Y. (2020). Comparison of chemical composition and bioactivities of essential oils from fresh and dry rhizomes of Zingiber zerumbet (L.) Smith. BioMed. Res. Int. 2020, 9641284. doi: 10.1155/2020/9641284
Tushar, B. S., Sarma, G. C., Rangan, L. (2010). Ethnomedical uses of Zingiberaceous plants of Northeast India. J. Ethnopharmacol. 132, 286–296. doi: 10.1016/j.jep.2010.08.032
van de Laar, F. A., Lucassen, P. L., Akkermans, R. P., van de Lisdonk, E. H., Rutten, G. E., van Weel, C. (2005). α-Glucosidase inhibitors for patients with type 2 diabetes: results from a Cochrane systematic review and meta-analysis. Diabetes Care 28, 154–163. doi: 10.2337/diacare.28.1.154
Wang, Y. (2010). The Coloured Atlas of Chinese Natural Medicine (the first volume) (Guiyang: Guizhou Publishing Group, Guiyang & Guizhou Science and Technology Publishing House), 200.
Weyerstahl, P., Marschall, H., Schneider, S., Subba, G. C. (1995). Constituents of the essential oil from the rhizomes of Hedychium acumnatum Roscoe. Flavour Frag. J. 10, 179–185. doi: 10.1002/ffj.2730100310
Weyerstahl, P., Marschall, H., Thefeld, K., Subba, G. C. (1998). Constituents of the essential oil from the rhizomes of Hedychium gardnerianum Roscoe. Flavour Fragr. J. 13, 377–388. doi: 10.1002/(SICI)1099-1026(199811/12)13:6<377::AID-FFJ755>3.0.CO;2-F
Wu, T. L., Larsen, K. (2000). “Zingiberaceae,” in Flora of China, vol. 24 . Eds. Wu, Z. Y., Raven, P. H. (St. Louis: Science PressBeijing & Missouri Botanical Garden Press), 322–377.
Zardi-Bergaoui, A., Jelizi, S., Flamini, G., Ascrizzi, R., Jannet, B. H. (2018). Comparative study of the chemical composition and bioactivities of essential oils of fresh and dry seeds from Myoporum insulare R. Br. Ind. Crops Prod. 111, 232–237. doi: 10.1016/j.indcrop.2017.10.019
Keywords: Hedychium flavum Roxb., phytochemical analysis, antioxidant, antibacterial, cytotoxic, enzyme inhibitory
Citation: Tian M, Wu X, Lu T, Zhao X, Wei F, Deng G and Zhou Y (2020) Phytochemical Analysis, Antioxidant, Antibacterial, Cytotoxic, and Enzyme Inhibitory Activities of Hedychium flavum Rhizome. Front. Pharmacol. 11:572659. doi: 10.3389/fphar.2020.572659
Received: 15 June 2020; Accepted: 31 August 2020;
Published: 17 September 2020.
Edited by:
Shuai Ji, Xuzhou Medical University, ChinaReviewed by:
Xionghao Lin, Howard University, United StatesKarthik Loganathan, Salem Microbes Pvt Ltd, India
Copyright © 2020 Tian, Wu, Lu, Zhao, Wei, Deng and Zhou. This is an open-access article distributed under the terms of the Creative Commons Attribution License (CC BY). The use, distribution or reproduction in other forums is permitted, provided the original author(s) and the copyright owner(s) are credited and that the original publication in this journal is cited, in accordance with accepted academic practice. No use, distribution or reproduction is permitted which does not comply with these terms.
*Correspondence: Ying Zhou, yingzhou71@yeah.net