Accuracy of lung and diaphragm ultrasound in predicting infant weaning outcomes: a systematic review and meta-analysis
- 1Department of Ultrasound, Shandong Provincial Maternal and Child Health Care Hospital, Jinan, China
- 2Department of Ultrasound, Shandong Provincial Hospital Affiliated to Shandong First Medical University, Jinan, China
Background: Although lung and diaphragm ultrasound are valuable tools for predicting weaning results in adults with MV, their relevance in children is debatable. The goal of this meta-analysis was to determine the predictive value of lung and diaphragm ultrasound in newborn weaning outcomes.
Methods: For eligible studies, the databases MEDLINE, Web of Science, Cochrane Library, PubMed, and Embase were thoroughly searched. The Quality Assessment of Diagnostic Accuracy Studies (QUADAS−2) method was used to evaluate the study's quality. Results were gathered for sensitivity, specificity, diagnostic odds ratio (DOR), and the area under the curve of summary receiver operating characteristic curves (AUSROC). To investigate the causes of heterogeneity, subgroup analyses and meta-regression were conducted.
Results: A total of 11 studies were suitable for inclusion in the meta-analysis, which included 828 patients. The pooled sensitivity and specificity of lung ultrasound (LUS) were 0.88 (95%CI, 0.85–0.90) and 0.81 (95%CI, 0.75–0.87), respectively. The DOR for diaphragmatic excursion (DE) is 13.17 (95%CI, 5.65–30.71). The AUSROC for diaphragm thickening fraction (DTF) is 0.86 (95%CI, 0.82–0.89). The most sensitive and specific method is LUS. The DE and DTF were the key areas where study heterogeneity was evident.
Conclusions: Lung ultrasonography is an extremely accurate method for predicting weaning results in MV infants. DTF outperforms DE in terms of diaphragm ultrasound predictive power.
1. Introduction
Mechanical ventilation (MV) has been widely employed to enhance outcomes in pediatric intensive care unit (PICU) and respiratory care in very preterm newborns (1, 2). However, weaning has long been a cause of concern. Prolonged MV is related with infant ventilator-associated lung injury and neurodevelopmental impairment, whereas premature weaning is associated with extubation failure (EF) and an increase risk of reintubation (3, 4). Moreover, many studies have linked EF and subsequent reintubation to increased mortality and bronchopulmonary dysplasia (BPD) in premature newborns (5–7). Therefore, choosing the best weaning interval has a substantial clinical impact on the prognosis of newborns with MV.
There are currently no standard guidelines to guide clinical weaning of infants with MV (8), and weaning timing is primarily determined by the attending physician based on clinical evaluation, ventilator parameters, and blood gas results (9), resulting in approximately 30% of premature infants failing extubation (10). As a result, new precise indications for predicting successful weaning timing in children with MV are required.
Because of its non-invasive, convenient, and real-time features, ultrasound is appropriate for bedside assessment of MV patients in the ICU (11, 12). Diaphragmatic function and pulmonary ventilation status are two components of ultrasound assessment of whether a patient can be weaned, with the former proven by the diaphragmatic excursion (DE) and diaphragm thickening fraction (DTF) (13), and the latter by the lung ultrasound score (LUS) (14). These three signs have been demonstrated to be accurate predictors of weaning in adult MV patients (15). Short DE distances and high DTF scores indicate poor diaphragm function, whereas high LUS suggests inadequate pulmonary ventilation, implying a significant risk of EF (16). However, because infant physiology and anatomy differ from those of adults, more research is needed to determine whether ultrasound technology can be used to anticipate weaning in infants with MV.
The purpose of this meta-analysis is to establish the accuracy of three diaphragm and lung ultrasound indicators: DE, DTF, and LUS in predicting weaning outcomes in infants with MV.
2. Methods
2.1. Literature search
Two researchers separately and methodically searched data from relevant papers published in MEDLINE, Web of Science, Cochrane Library, PubMed, and Embase from the time the databases were established until April 1, 2,023. Reference lists of relevant literature were also combed for any further relevant literature. The following MESH words were used in our search: [(“lung ultrasound” OR “diaphragm ultrasound” OR “LUS” OR “DE” OR “DTF”) AND (“MV” OR “weaning” OR “extubation” OR “discontinuation of mechanical ventilation” OR “disconnect of mechanical ventilation”) AND (“infants” OR “neonates” OR “children” OR “newborns”)].
2.2. Inclusion and exclusion criteria
The following are the inclusion criteria: (1) Infants receiving MV therapy who are getting ready to wean. (2) Patients who had diaphragm and/or lung ultrasounds prior to weaning. (3) Studies directly or indirectly offer true positive (TP), true negative (TN), false positive (FP), and false negative (FN) data to build 2 × 2 tables. The exclusion criteria are as follows: (1) Studies that are repeated or overlapped. (2) The data in the article is inadequate to create a 2 × 2 table. (3) research that is not original. Disagreements between the two researchers were resolved with the assistance of a third researcher.
2.3. Data extraction and quality assessment
Two researchers separately gathered TP, TN, FP, and FN from source literature data to produce a 2 × 2 form, and any inconsistencies were handled through deliberation. We only built data tables for weaning success due to the dichotomous nature of weaning events. Furthermore, various methodological data linked to research baseline features such as patient age, patient number, measurements and cutoff value, weaning success definition, and weaning failure definition were comprehensively retrieved. The QUADAS-2 tool was used to evaluate the four aspects of patient selection, flow and timing, index testing, and reference standards in all included studies (17). Utilizing Review Manager 5.3, the QUADAS-2 quality evaluation's bias and applicability concerns were rated as having low, high, or uncertain risk.
2.4. Statistical analysis
Pooled TP, TN, FP, and FN were used to compute sensitivity, specificity, positive likelihood ratio (PLR), negative likelihood ratio (NLR), diagnostic odds ratio (DOR), and corresponding 95% confidence intervals (95% CI) for diaphragm and lung ultrasound in MV infants. Furthermore, the summarized receiver operator characteristic (SROC) curve and area under the SROC curve (AUC) are utilized to assess the overall performance of ultrasound parameters (18).
We used I2 statistics and Q test for heterogeneity, and meta-regression to investigate sources of heterogeneity in sensitivity and specificity (19). Zones (6 zones were the most commonly reported zoning scheme); chance of offline success (70% average); cut-off (LUS ≥ 15); and whether or not the operation is blinded were research elements that investigated the accuracy of LUS. Additionally, the Deeks funnel plots were employed to calculate potential publication bias (20). This entire statistical procedure was carried out in Stata 16.0.
3. Results
3.1. Study selection and characteristics
A detailed flow chart of our retrieved literature is shown in Figure 1. After deleting duplicate articles, we had 1,380 records after searching the original database for 1,986 articles. Following a review of the title and abstract, 1,331 records were excluded, and 38 records that did not fulfill the criteria were excluded after full text review. Finally, this meta-analysis contained 11 studies that met the inclusion and exclusion criteria (21c31).
Our study comprised 828 patients from 11 articles, with comprehensive baseline characteristics shown in Table 1. Four of the 11 studies were carried out in Egypt, four in China, two in Canada, and one in Turkey. Except for two unspecified trials (30, 31), the remaining nine were conducted in medical ICU. In addition, seven studies assessed the LUS indicator, four assessed the DE indicator, and four assessed the DTF indicator. Amrousy's study had two LUS cut-off points (24), but Jiang's study had four LUS cut-off points (31). One study looked at left and right DE (25), while the other looked at left and right DTF (29).
Different studies identified different weaning criteria. Weaning success is defined primarily as sustaining spontaneous breathing >48 h without reintubation, whereas weaning failure is defined primarily as failing the Spontaneous Breathing Trial or requiring reintubation within 48 h.
3.2. Assessment of method quality and publication bias
The results of the QUADAS-2 tool assessing risk of bias and applicability concerns of the included studies were shown in Figure 2. The possibility of patient selection bias was mostly related to the fact that four studies included patients who were either eligible for weaning or planned to be weaned rather than patients who were randomly selected (21, 24, 25, 29). Index test poses a risk since they do not clearly reveal whether the ultrasound was performed without knowledge of the clinical outcomes (21, 23, 27, 28, 31). Furthermore, the reference standard poses a danger due to the low success rate of clinical weaning events and the omission to identify whether clinical weaning decisions were taken without knowledge of ultrasound findings (22, 27, 28, 29, 30, 31). The Deeks' test revealed no substantial publication bias in LUS, DE, or DTF, with all P values more than 0.05 (Supplementary Figure S1).
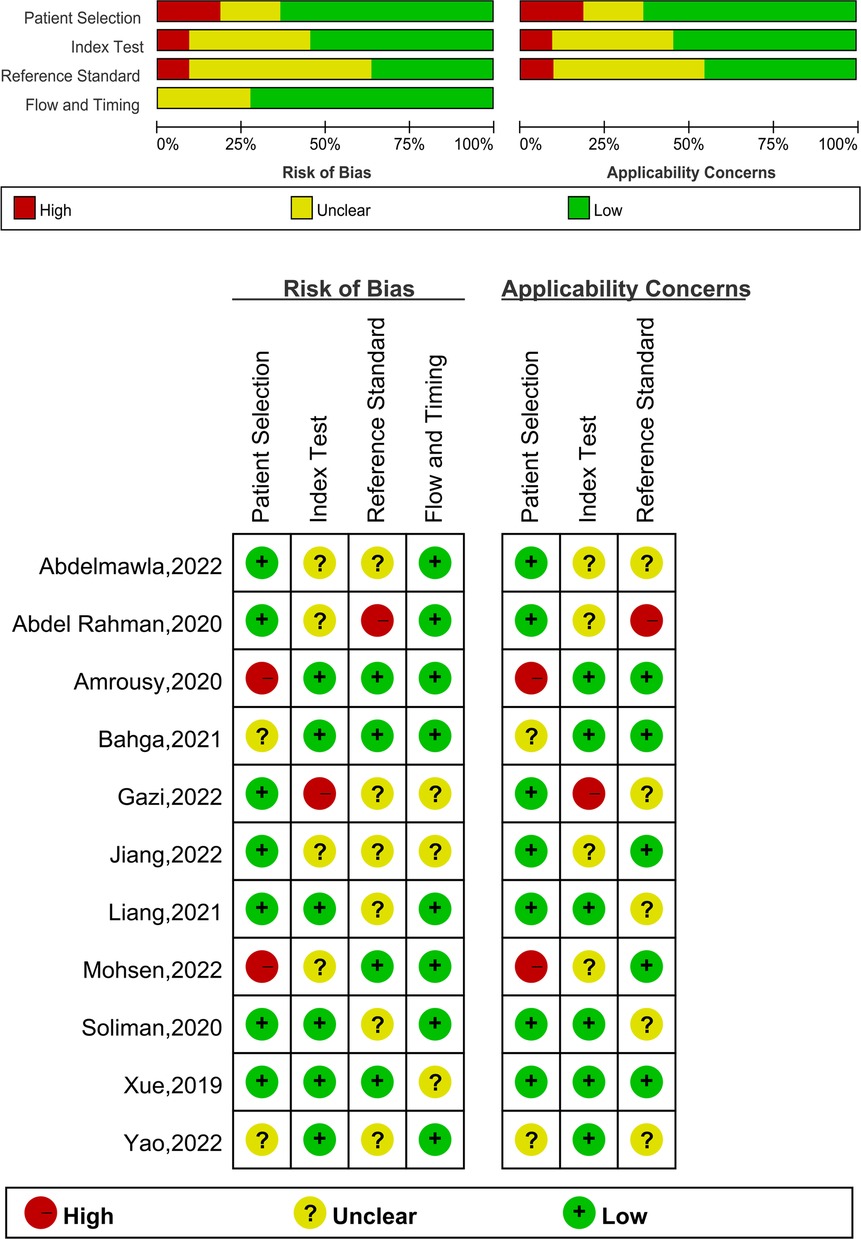
Figure 2. The graphical display of the evaluation of the risk of bias and concerns regarding the applicability of the selected studies.
3.3. Diagnostic accuracy and heterogeneity
We assessed the pooled sensitivity, specificity, PLR, NLR, DOR, and AUC of the ROC for LUS, DE, and DTF to investigate the accuracy of ultrasound in predicting weaning outcomes (Table 2). The pooled sensitivity and specificity of LUS, DE, and DTF were 0.88 (95%CI, 0.85–0.90), 0.81 (95%CI, 0.75–0.87), 0.85 (95%CI, 0.74–0.91), 0.71 (95%CI, 0.60–0.80), 0.89 (95%CI, 0.70–0.96), and 0.79 (95%CI, 0.68–0.86), respectively (Figure 3). The pooled PLR and NLR of LUS, DE, and DTF were 4.68 (95%CI, 3.38–6.47), 0.15 (95%CI, 0.12–0.19), 2.88 (95%CI, 1.99–4.18), 0.22 (95%CI, 0.12–0.39), 4.14 (95%CI, 2.87–5.97) and 0.15 (95%CI, 0.05–0.40), respectively (Figure 4). The LUS, DE and DTF had the ability to accurately predict weaning, with DOR of 30.62 (95%CI, 18.86–49.72), 13.17 (95%CI, 5.65–30.71) and 28.35 (95%CI, 10.19–78.85), respectively. The area under the summary operator receiver characteristic curve (AUSROC) was shown in Figure 5, where LUS had the largest AUSROC of 0.90 (95%CI, 0.87–0.92), while DE and DTF were 0.79 (95%CI, 0.76–0.83) and 0.86 (95%CI, 0.82–0.89), respectively. In addition, heterogeneity was significant in the pooled sensitivity of DE and DTF (I2 > 50%, p < 0.05).
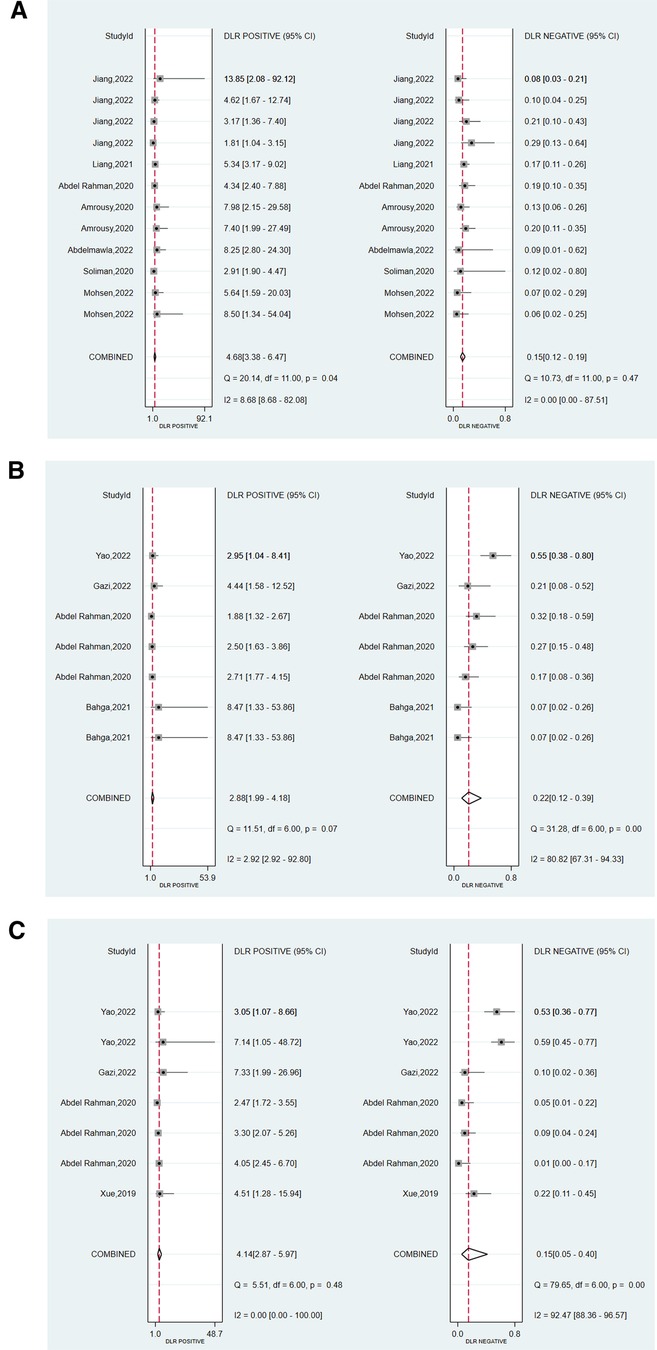
Figure 4. Forest plot of the pooled positive likelihood ratio (PLR) and negative likelihood ratio (NLR) of (A) LUS, (B) DE, and (C) DTF.
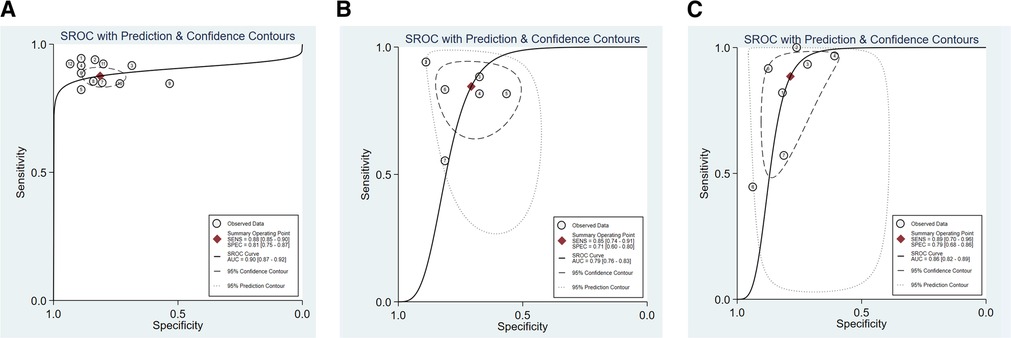
Figure 5. The area under the curve of summary receiver operating characteristic curves (AUSROC) of (A) LUS, (B) DE, and (C) DTF.
3.4. Meta-regression and subgroup analyses
Due to a lack of data for DE and DTF, only sensitivity and specificity meta-regression analysis for LUS was performed, with the results presented in Supplementary Figure S2. Zone, weaning success rates (Pretest), threshold, and blind were all considered as factors influencing lung ultrasonography diagnostic accuracy. The sensitivity of LUS forecasts is affected by six Zone, weaning success rate more than 70%, LUS ≥ 15, and blind. Only six zones and weaning success rates greater than 70% have an effect on the specificity of LUS forecasts. In addition, we conducted subgroup analyses based on the meta-regression results, which were displayed in Table 3. Almost all subgroups demonstrated great high sensitivity, specificity, and DOR, indicating that LUS is a highly accurate technique for predicting infant weaning outcomes.
4. Discussion
Although lung and diaphragmatic ultrasound have been frequently used to predict weaning in adult MV patients (32, 33, 34), their application in newborns has received little attention. In this meta-analysis, we discovered that lung and diaphragm ultrasound were also helpful techniques for predicting newborn weaning outcomes by examining the weaning outcomes of 828 infants who received MV. LUS performed the best overall, with sensitivity and specificity of 0.88 and 0.81, respectively. DTF outperformed DE in the diaphragm ultrasound evaluation index, and AUSROC was 0.86 and 0.79, respectively. It is important to note that the sensitivity of DE and DTF varies greatly. Moreover, some studies were highly susceptible to bias, particularly in patient selection, raising concerns about applicability.
LUS is an important method for evaluating lung ventilation loss, which represents a drop in lung capacity available for gas exchange and can be used to forecast EF (35, 36). Our data also shown a relationship between high LUS and EF, which is consistent with the findings for adults (37, 38). The unique truncation point, however, cannot be identified due to the variety of LUS methods, which include 6-zone, 10-zone, 12-zone, and 14-zone, but LUS has a high AUSROC of 0.90 (95%CI, 0.87–0.92) when assessed using the different cut-off points of each study. Although Jiang et al. (31) believe that prediction accuracy increases with partition size, the results of our subgroup analysis reveal that prediction accuracy for 6-zone and other zones is not significantly different, with AUSROC both above 0.90. We discovered that LUS was more accurate at predicting weaning in newborns than it was at predicting weaning in adults (AUSROC, 0.77) (15). Additionally, similarly to our work, LUS exhibited a good ability to predict weaning in several adult studies, with cut-off levels ranging from 10 to 14 (24, 39). In our subgroup analysis, subgroups with cut-off points higher than 15 had a DOR of 43.54 (95%CI, 21.47–88.28), greater than subgroups with cut-off points lower than 15, which had a DOR of 22.96 (95%CI, 11.67–45.18). The inclusion of groups with high extubation success rates and tight study methods were also sources of variability and variables with increased sensitivity, according to meta-regression.
DE and DTF are two key diaphragm ultrasonography measurements for predicting weaning results in MV patients, with DE being connected to inspiratory volume and DTF being a response measure of diaphragmatic inhalation effort (40, 41). DE and DTF worked well in adult studies, with greatest predictive values of 10–15 mm and 20%–36%, respectively (42). Except for one study, where the DTF cut-off point was chosen at 40.50% because the patients studied were generally healthy (28), the DTF cut-off values in the included studies were similar to those in adults. However, the ideal cut-off for DE in the studies we considered was often less than 10 mm, significantly smaller than the adult figure, with the exception of Gazi et al. (28), who used a cut-off of 12.15 mm. This could be because the diaphragm is a skeletal muscle whose thickness and strength fluctuate with age (43), and the children in Gazi et al. are much older than the children in the other included studies. In addition, infant diaphragms are not the same as adult diaphragms. The neonatal diaphragm, for example, is morphologically flat and implanted into the chest wall at a greater angle than the dome-shaped diaphragm in adults, resulting in a smaller apposition area and a limited range of displacement (44). In contrast to the adult diaphragm, which functions like a piston inside the ribs, the newborn diaphragm moves dorsally, like a bellows (45). Because the newborn diaphragm is structurally made of less anti-fatigue slow twitching (type I) fibers, reserve capacity is minimal and muscle fatigue is likely (46). When the amount of respiratory work rises, the accessory respiratory muscles (scalene and sternocleidomastoid) must be recruited to overcome tiredness (47).
Although the optimal cut-off points chosen by each study differed, our meta-analysis found that DE and DTF had good high sensitivity and moderate specificity for diagnosing infant weaning, with values of 0.85, 0.71, 0.89, and 0.79, respectively. In line with earlier studies, DTF outperformed DE in predicting MV weaning outcomes (48). Unfortunately, we were unable to perform meta-regression or subgroup analysis of DE and DTF results to determine the source of their heterogeneity due to the short number of papers available. We still consider Yao's studies as a significant source of heterogeneity because their sensitivity was 0.45 to 0.57, which was much lower than in other researches. Therefore, we feel that the capabilities of diaphragm ultrasound are underappreciated and that it could play a more prominent role in predicting infant MV weaning outcomes.
Ana M. Llamas-Llvarez et al. (15) found in a systematic analysis of adults that DTF performed better than DE in predicting patient weaning outcomes, and our investigation supported this finding for infants. Even if their findings also explain the power of LUS, they feel that this information should be viewed with caution because they were unable to undertake a thorough investigation of the capabilities of LUS due to limitations in the included literature. We can categorically state that lung ultrasonography is effective at predicting weaning outcomes because we included enough material to enable us to carry out in-depth subgroup analysis. Overall, our study found that both diaphragmatic and lung ultrasound had good accuracy and can be used to forecast the results of newborn weaning.
The lungs and diaphragm are coordinated during breathing motions because the diaphragm acts as a brake at the end of expiration to maintain end-expiratory lung volume (EELV) and prevent lung collapse (49). Patients who receive positive end-expiratory pressure ventilation have their diaphragmatic myofibers remodeled over time with the help of MV, whereas when weaned, sudden changes in EELV when the diaphragm does not have time to respond reduce inspiratory muscle strength and lead to weaning failure (50). Prolonged MV reduces diaphragm thickness, and a study in adults found that a thinner diaphragm is associated with weaning failure (51). A recent agreement on diaphragm ultrasonography in critically ill patients recommends a thickness drop of more than 10% as the cut-off for atrophy (52). Furthermore, excessive diaphragmatic effort can still result in self-inflicted lung injury since spontaneous breathing efforts can cause the lungs to stretch abruptly during inflation, even if tidal volume is not increased (53). Therefore, fresh ideas for lung and diaphragm protective breathing have been put forth (54). Although preliminary clinical trials are still being conducted, we may anticipate further study on lung and diaphragm interactions to increase the likelihood that weaning will be successful in the future.
Our meta-analysis did have certain limitations. First, the infant ages in the research we included varied greatly, thus there could be a consistent effect on the accuracy of the results. Second, differences in diaphragmatic ultrasonography procedures, which might be difficult to identify and harmonize, may have an adverse effect on the outcomes. Moreover, we only made a preliminary conclusion that pulmonary and diaphragm ultrasound are useful instruments for measuring newborn weaning with MV, but there is no consensus on acceptable cut-off values. Finally, because of the small number of studies included, we were unable to investigate the sources of heterogeneity in diaphragm ultrasound measurements.
5. Conclusion
The findings of this meta-analysis demonstrate that high LUS, low DE, and low DTF all indicate EF, implying that lung and diaphragm ultrasound, particularly LUS performance, is an excellent technique for predicting weaning outcomes in infants with MV. Subgroup analysis of diaphragm ultrasonography is also required to establish its predictive accuracy under different definitions and measurement settings.
Data availability statement
The original contributions presented in the study are included in the article/Supplementary Material, further inquiries can be directed to the corresponding author.
Author contributions
YG and Y-HG contributed to the conception and design of the study. M-HW performed the statistical analysis. YG wrote the first draft of the manuscript. HY wrote the method section of the manuscript. Y-HG revised the manuscript. All authors contributed to the article and approved the submitted version.
Funding
The work was supported by the Science and technology innovation project of Shandong Maternal and Child Health Care Association in 2021 (grant number FYXH20210202).
Conflict of interest
The authors declare that the research was conducted in the absence of any commercial or financial relationships that could be construed as a potential conflict of interest.
Publisher's note
All claims expressed in this article are solely those of the authors and do not necessarily represent those of their affiliated organizations, or those of the publisher, the editors and the reviewers. Any product that may be evaluated in this article, or claim that may be made by its manufacturer, is not guaranteed or endorsed by the publisher.
Supplementary material
The Supplementary Material for this article can be found online at: https://www.frontiersin.org/articles/10.3389/fped.2023.1211306/full#supplementary-material
References
1. Newth CJL, Khemani RG, Jouvet PA, Sward KA. Mechanical ventilation and decision support in pediatric intensive care. Pediatr Clin N Am. (2017) 64(5):1057–70. doi: 10.1016/j.pcl.2017.06.006
2. Wang SH, Liou JY, Chen CY, Chou HC, Hsieh WS, Tsao PN. Risk factors for extubation failure in extremely low birth weight infants. Pediatr Neonatol. (2017) 58(2):145–50. doi: 10.1016/j.pedneo.2016.01.006
3. Miller JD, Carlo WA. Pulmonary complications of mechanical ventilation in neonates. Clin Perinatol. (2008) 35(1):273–81. x-xi. doi: 10.1016/j.clp.2007.11.004
4. Walsh MC, Morris BH, Wrage LA, Vohr BR, Poole WK, Tyson JE, et al. Extremely low birthweight neonates with protracted ventilation: mortality and 18-month neurodevelopmental outcomes. J Pediatr. (2005) 146(6):798–804. doi: 10.1016/j.jpeds.2005.01.047
5. Chawla S, Natarajan G, Shankaran S, Carper B, Brion LP, Keszler M, et al. Markers of successful extubation in extremely preterm infants, and morbidity after failed extubation. J Pediatr. (2017) 189:113–9. e2. doi: 10.1016/j.jpeds.2017.04.050
6. Shalish W, Kanbar L, Kovacs L, Chawla S, Keszler M, Rao S, et al. The impact of time interval between extubation and reintubation on death or bronchopulmonary dysplasia in extremely preterm infants. J Pediatr. (2019) 205:70–6. e2. doi: 10.1016/j.jpeds.2018.09.062
7. Shalish W, Keszler M, Kovacs L, Chawla S, Latremouille S, Beltempo M, et al. Age at first extubation attempt and death or respiratory morbidities in extremely preterm infants. J Pediatr. (2023) 252:124–30. e3. doi: 10.1016/j.jpeds.2022.08.025
8. Shalish W, Keszler M, Davis PG, Sant'Anna GM. Decision to extubate extremely preterm infants: art, science or gamble? Arch Dis Child Fetal Neonatal Ed. (2022) 107(1):105–12. doi: 10.1136/archdischild-2020-321282
9. Al-Mandari H, Shalish W, Dempsey E, Keszler M, Davis PG, Sant'Anna G. International survey on periextubation practices in extremely preterm infants. Arch Dis Child Fetal Neonatal Ed. (2015) 100(5):F428–31. doi: 10.1136/archdischild-2015-308549
10. Dimitriou G, Greenough A, Endo A, Cherian S, Rafferty GF. Prediction of extubation failure in preterm infants. Arch Dis Child Fetal Neonatal Ed. (2002) 86(1):F32–5. doi: 10.1136/fn.86.1.F32
11. Mayo P, Arntfield R, Balik M, Kory P, Mathis G, Schmidt G, et al. The ICM research agenda on critical care ultrasonography. Intensive Care Med. (2017) 43(9):1257–69. doi: 10.1007/s00134-017-4734-z
12. Llamas-Álvarez AM, Tenza-Lozano EM, Latour-Pérez J. Accuracy of lung ultrasonography in the diagnosis of pneumonia in adults: systematic review and meta-analysis. Chest. (2017) 151(2):374–82. doi: 10.1016/j.chest.2016.10.039
13. Dres M, Goligher EC, Dubé BP, Morawiec E, Dangers L, Reuter D, et al. Diaphragm function and weaning from mechanical ventilation: an ultrasound and phrenic nerve stimulation clinical study. Ann Intensive Care. (2018) 8(1):53. doi: 10.1186/s13613-018-0401-y
14. Bouhemad B, Liu ZH, Arbelot C, Zhang M, Ferarri F, Le-Guen M, et al. Ultrasound assessment of antibiotic-induced pulmonary reaeration in ventilator-associated pneumonia. Crit Care Med. (2010) 38(1):84–92. doi: 10.1097/CCM.0b013e3181b08cdb
15. Llamas-Álvarez AM, Tenza-Lozano EM, Latour-Pérez J. Diaphragm and lung ultrasound to predict weaning outcome: systematic review and meta-analysis. Chest. (2017) 152(6):1140–50. doi: 10.1016/j.chest.2017.08.028
16. Le Neindre A, Philippart F, Luperto M, Wormser J, Morel-Sapene J, Aho SL, et al. Diagnostic accuracy of diaphragm ultrasound to predict weaning outcome: a systematic review and meta-analysis. Int J Nurs Stud. (2021) 117:103890. doi: 10.1016/j.ijnurstu.2021.103890
17. Schueler S, Schuetz GM, Dewey M. The revised QUADAS-2 tool. Ann Intern Med. (2012) 156(4):323. author reply −4. doi: 10.7326/0003-4819-156-4-201202210-00018
18. Harbord RM, Deeks JJ, Egger M, Whiting P, Sterne JA. A unification of models for meta-analysis of diagnostic accuracy studies. Biostatistics (Oxford, England). (2007) 8(2):239–51. doi: 10.1093/biostatistics/kxl004
19. Dinnes J, Deeks J, Kirby J, Roderick P. A methodological review of how heterogeneity has been examined in systematic reviews of diagnostic test accuracy. Health Technol Assess (Winchester, England). (2005) 9(12):1–113. iii. doi: 10.3310/HTA9120
20. Deeks JJ, Macaskill P, Irwig L. The performance of tests of publication bias and other sample size effects in systematic reviews of diagnostic test accuracy was assessed. J Clin Epidemiol. (2005) 58(9):882–93. doi: 10.1016/j.jclinepi.2005.01.016
21. Mohsen N, Nasef N, Ghanem M, Yeung T, Deekonda V, Ma C, et al. Accuracy of lung and diaphragm ultrasound in predicting successful extubation in extremely preterm infants: a prospective observational study. Pediatr Pulmonol. (2023) 58(2):530–9. doi: 10.1002/ppul.26223
22. Soliman RM, Elsayed Y, Said RN, Abdulbaqi AM, Hashem RH, Aly H. Prediction of extubation readiness using lung ultrasound in preterm infants. Pediatr Pulmonol. (2021) 56(7):2073–80. doi: 10.1002/ppul.25383
23. Abdelmawla M, Seleem W, Farooqui M, Eltayeb A, Elsayed Y. Prediction of weaning readiness off nasal CPAP in preterm infants using point-of-care lung ultrasound. Pediatr Pulmonol. (2022) 57(9):2128–35. doi: 10.1002/ppul.26014
24. El Amrousy D, Elgendy M, Eltomey M, Elmashad AE. Value of lung ultrasonography to predict weaning success in ventilated neonates. Pediatr Pulmonol. (2020) 55(9):2452–6. doi: 10.1002/ppul.24934
25. Bahgat E, El-Halaby H, Abdelrahman A, Nasef N, Abdel-Hady H. Sonographic evaluation of diaphragmatic thickness and excursion as a predictor for successful extubation in mechanically ventilated preterm infants. Eur J Pediatr. (2021) 180(3):899–908. doi: 10.1007/s00431-020-03805-2
26. Xue Y, Zhang Z, Sheng CQ, Li YM, Jia FY. The predictive value of diaphragm ultrasound for weaning outcomes in critically ill children. BMC Pulm Med. (2019) 19(1):270. doi: 10.1186/s12890-019-1034-0
27. Abdel Rahman DA, Saber S, El-Maghraby A. Diaphragm and lung ultrasound indices in prediction of outcome of weaning from mechanical ventilation in pediatric intensive care unit. Indian J Pediatr. (2020) 87(6):413–20. doi: 10.1007/s12098-019-03177-y
28. Arslan G, Besci T, Duman M. Point of care diaphragm ultrasound in mechanically ventilated children: a predictive tool to detect extubation failure. Pediatr Pulmonol. (2022) 57(6):1432–9. doi: 10.1002/ppul.25916
29. Yao Y, He L, Chen W, Zhou H, Lu G, Tao J, et al. Predictive value of diaphragmatic ultrasonography for the weaning outcome in mechanically ventilated children aged 1−3 years. Front Pediatr. (2022) 10:840444. doi: 10.3389/fped.2022.840444
30. Liang Z, Meng Q, You C, Wu B, Li X, Wu Q. Roles of lung ultrasound score in the extubation failure from mechanical ventilation among premature infants with neonatal respiratory distress syndrome. Front Pediatr. (2021) 9:709160. doi: 10.3389/fped.2021.709160
31. Jiang QX, Shi LJ, Shen LY, Li XQ, Huang RS, Chen LJ, et al. Application value of a new lung ultrasound scoring method in neonatal respiratory distress syndrome treatment. Ultrasound Med Biol. (2022) 48(2):275–82. doi: 10.1016/j.ultrasmedbio.2021.10.009
32. Qian Z, Yang M, Li L, Chen Y. Ultrasound assessment of diaphragmatic dysfunction as a predictor of weaning outcome from mechanical ventilation: a systematic review and meta-analysis. BMJ Open. (2018) 8(9):e021189. doi: 10.1136/bmjopen-2017-021189
33. Mojoli F, Bouhemad B, Mongodi S, Lichtenstein D. Lung ultrasound for critically ill patients. Am J Respir Crit Care Med. (2019) 199(6):701–14. doi: 10.1164/rccm.201802-0236CI
34. Abdelwahed WM, Abd Elghafar MS, Amr YM, Alsherif SEI, Eltomey MA. Prospective study: diaphragmatic thickness as a predictor index for weaning from mechanical ventilation. J Crit Care. (2019) 52:10–5. doi: 10.1016/j.jcrc.2019.03.006
35. Caltabeloti FP, Rouby JJ. Lung ultrasound: a useful tool in the weaning process? Rev Bras Ter Intensiva. (2016) 28(1):5–7. doi: 10.5935/0103-507X.20160002
36. Guerin C, Gattinoni L. Assessment of oxygenation response to prone position ventilation in ARDS by lung ultrasonography. Intensive Care Med. (2016) 42(10):1601–3. doi: 10.1007/s00134-016-4440-2
37. Xu SX, Wu CS, Liu SY, Lu X. High-flow nasal cannula oxygen therapy and noninvasive ventilation for preventing extubation failure during weaning from mechanical ventilation assessed by lung ultrasound score: a single-center randomized study. World J Emerg Med. (2021) 12(4):274–80. doi: 10.5847/wjem.j.1920-8642.2021.04.004
38. Wang R, Qi B, Zhang X, Meng L, Wu X. Prophetic values of lung ultrasound score on post-extubation distress in patients with acute respiratory distress syndrome. Eur J Med Res. (2022) 27(1):27. doi: 10.1186/s40001-022-00652-9
39. Soummer A, Perbet S, Brisson H, Arbelot C, Constantin JM, Lu Q, et al. Ultrasound assessment of lung aeration loss during a successful weaning trial predicts postextubation distress. Crit Care Med. (2012) 40(7):2064–72. doi: 10.1097/CCM.0b013e31824e68ae
40. Umbrello M, Formenti P, Longhi D, Galimberti A, Piva I, Pezzi A, et al. Diaphragm ultrasound as indicator of respiratory effort in critically ill patients undergoing assisted mechanical ventilation: a pilot clinical study. Crit Care (London, England). (2015) 19(1):161. doi: 10.1186/s13054-015-0894-9
41. Cohen E, Mier A, Heywood P, Murphy K, Boultbee J, Guz A. Excursion-volume relation of the right hemidiaphragm measured by ultrasonography and respiratory airflow measurements. Thorax. (1994) 49(9):885–9. doi: 10.1136/thx.49.9.885
42. Yoo JW, Lee SJ, Lee JD, Kim HC. Comparison of clinical utility between diaphragm excursion and thickening change using ultrasonography to predict extubation success. Korean J Intern Med. (2019) 34(3):686. doi: 10.3904/kjim.2016.152.e1
43. El-Halaby H, Abdel-Hady H, Alsawah G, Abdelrahman A, El-Tahan H. Sonographic evaluation of diaphragmatic excursion and thickness in healthy infants and children. J Ultrasound Med. (2016) 35(1):167–75. doi: 10.7863/ultra.15.01082
44. Devlieger H, Daniels H, Marchal G, Moerman P, Casaer P, Eggermont E. The diaphragm of the newborn infant: anatomical and ultrasonographic studies. J Dev Physiol. (1991) 16(6):321–9.1824114
45. Dassios T, Vervenioti A, Dimitriou G. Respiratory muscle function in the newborn: a narrative review. Pediatr Res. (2022) 91(4):795–803. doi: 10.1038/s41390-021-01529-z
46. Sieck GC, Fournier M, Blanco CE. Diaphragm muscle fatigue resistance during postnatal development. J Appl Physiol (Bethesda, Md: 1985). (1991) 71(2):458–64. doi: 10.1152/jappl.1991.71.2.458
47. Mortola JP. Dynamics of breathing in newborn mammals. Physiol Rev. (1987) 67(1):187–243. doi: 10.1152/physrev.1987.67.1.187
48. Li C, Li X, Han H, Cui H, Wang G, Wang Z. Diaphragmatic ultrasonography for predicting ventilator weaning: a meta-analysis. Medicine (Baltimore). (2018) 97(22):e10968. doi: 10.1097/MD.0000000000010968
49. Pellegrini M, Hedenstierna G, Roneus A, Segelsjö M, Larsson A, Perchiazzi G. The diaphragm acts as a brake during expiration to prevent lung collapse. Am J Respir Crit Care Med. (2017) 195(12):1608–16. doi: 10.1164/rccm.201605-0992OC
50. Petrof BJ, Sassoon CS. Diaphragm remodeling during application of positive end-expiratory pressure. A case of normal physiologic adaptation gone awry? Am J Respir Crit Care Med. (2018) 198(4):416–8. doi: 10.1164/rccm.201803-0518ED
51. Corradi F, Isirdi A, Malacarne P, Santori G, Barbieri G, Romei C, et al. Low diaphragm muscle mass predicts adverse outcome in patients hospitalized for COVID-19 pneumonia: an exploratory pilot study. Minerva Anestesiol. (2021) 87(4):432–8. doi: 10.23736/S0375-9393.21.15129-6
52. Haaksma ME, Smit JM, Boussuges A, Demoule A, Dres M, Ferrari G, et al. EXpert consensus on diaphragm UltraSonography in the critically ill (EXODUS): a Delphi consensus statement on the measurement of diaphragm ultrasound-derived parameters in a critical care setting. Crit Care (London, England). (2022) 26(1):99. doi: 10.1186/s13054-022-03975-5
53. Yoshida T, Torsani V, Gomes S, De Santis RR, Beraldo MA, Costa EL, et al. Spontaneous effort causes occult pendelluft during mechanical ventilation. Am J Respir Crit Care Med. (2013) 188(12):1420–7. doi: 10.1164/rccm.201303-0539OC
Keywords: lung ultrasound, diaphragm ultrasound, weaning, mechanical ventilation, pediatric critical care, endotracheal extubation
Citation: Gao Y, Yin H, Wang M-H and Gao Y-H (2023) Accuracy of lung and diaphragm ultrasound in predicting infant weaning outcomes: a systematic review and meta-analysis. Front. Pediatr. 11:1211306. doi: 10.3389/fped.2023.1211306
Received: 24 April 2023; Accepted: 28 August 2023;
Published: 6 September 2023.
Edited by:
Stefano Nobile, Agostino Gemelli University Polyclinic (IRCCS), ItalyReviewed by:
Luigi Vetrugno, University of Studies G. d'Annunzio Chieti and Pescara, ItalyCristian Deana, Azienda Sanitaria Universitaria Integrata di Udine, Italy
© 2023 Gao, Yin, Wang and Gao. This is an open-access article distributed under the terms of the Creative Commons Attribution License (CC BY). The use, distribution or reproduction in other forums is permitted, provided the original author(s) and the copyright owner(s) are credited and that the original publication in this journal is cited, in accordance with accepted academic practice. No use, distribution or reproduction is permitted which does not comply with these terms.
*Correspondence: Yue-Hua Gao longshe7@126.com