- 1Head and Neck Oncology Department, Cancer Center, West China Hospital, Sichuan University, Chengdu, China
- 2Department of Radiation Oncology, Cancer Center, West China Hospital, Sichuan University, Chengdu, China
- 3Cancer Center, West China Hospital, Sichuan University, Chengdu, China
- 4West China Clinical Skills Training Center, West China School of Medicine/West China Hospital, Sichuan University, Chengdu, China
- 5Department of Radiation Oncology, Radiation Oncology Key Laboratory of Sichuan Province, Sichuan Clinical Research Center for Cancer, Sichuan Cancer Hospital and Institute, Sichuan Cancer Center, Affiliated Cancer Hospital of University of Electronic Science and Technology of China, Chengdu, China
- 6Radiotherapy Physics and Technology Center, Cancer Center, West China Hospital, Sichuan University, Chengdu, China
Purpose: The study aimed to compare the dosimetric distribution of VMAT plans by increasing the number of half arcs in liver SBRT and investigate the effect by using automatic plan software in plan optimization.
Method: Thirty-one patients with oligo liver tumors were randomly selected. VMAT treatment plans with different numbers of coplanar half arcs were generated.
Result: Adding arcs significantly increased the PTV, D2%, D50%, and CI, but sacrificed the plan homogeneity. It also decreased the maximum dose of normal tissues such as the stomach, duodenum, and spinal cord and reduced Dmean, D500cc, and D700cc for the liver. Nevertheless, the diminishing effect gradually decayed into three arcs. Meanwhile, the addition of arcs substantially extended the beam-on time.
Conclusion: In the context of SBRT for oligo liver tumors, increasing the number of coplanar half arcs will improve PTV conformity and offer better protection for OARs, albeit at the expense of increased treatment duration. Considering the trade-off between plan quality and treatment efficiency, a three-arc plan may be more suitable for clinical implementation.
1 Introduction
Liver tumors are one of the leading causes of cancer-related mortality worldwide (1). Surgical resection with or without interventional therapy, target therapy, or systematic chemotherapy is the primary treatment option for hepatocellular carcinoma (HCC) or oligometastasis, but over 80% of patients present with unresectable tumors (2, 3). For those who are not operable, radiation therapy is another choice. However, radiation-induced liver disease (RILD) limits the use of radiotherapy in the long term.
Recently, the rapid development of radiotherapy techniques, such as intensity-modulated radiation therapy (IMRT) or volumetric-modulated arc therapy (VMAT), has expanded the indications of radiotherapy for liver tumors. Stereotactic body radiotherapy (SBRT) is an alternative option for early-stage HCC patients or oligometastases who are not eligible for surgery or interventional therapy, and it can also be used for locally advanced HCC (4, 5).
SBRT delivers high doses of radiation in a few fractions with better accuracy to the tumor target. Previous studies have shown that the success of SBRT is due to a higher biological effective dose (BED) and sparing of normal tissue (5). Current clinical investigations have unveiled SBRT’s effectiveness, manifesting in promising local control rates (1-year LC 56%–100%) and overall survival rates (1-year OS 32%–94%) (6–8), Additionally, SBRT has exhibited potential in slowing the progression of disease from an oligometastatic state to a polymetastatic one (9).
Different methods can be used for performing SBRT, including 3D-RT, IMRT, DCAT, VMAT, and Cyberknife. Among all these methods of delivery, VMAT is a type of rotational radiotherapy. VMAT is superior to other modalities due to its better dose distribution and treatment efficiency (10–15).
However, high-dose delivery to the target tissue can cause late effects, which can significantly damage nearby normal tissues (16–18). Therefore, it is crucial to reduce the dosage of the organs at risk (OARs) in SBRT treatment plans. It has been shown that the LC rate depends on the size and number of lesions, while the OS rate is strongly associated with liver function before treatment (19).
The quality of radiotherapy planning largely depends on the experience and skills of the medical physicists (20, 21). Manually setting and adjusting parameters is the mainstream in radiotherapy planning and optimization. It has been shown that optimization strategies greatly affect dose outcomes in liver SBRT plans (10). Therefore, we developed an automatic stereotactic body radiation therapy planning (ASP) program to improve the overall plan quality and consistency, prevent bias caused by different physicists while reducing the reliance on personal experience or skills, and accelerate the entire process. The ASP program has been previously evaluated in both the lung and liver with better reproducibility and repeatability (22–24). In this study, we aimed to investigate the efficacy of our ASP program in optimizing the liver SBRT plans by increasing the number of total half arcs in VMAT plans.
2 Materials and methods
2.1 Patient selection
Thirty-one patients with primary or metastatic liver tumors who underwent liver SBRT from 2017 to 2022 were randomly selected for analysis. The Clinical Research Committee of the study institute approved the protocol (Approval number 2022-1902). The need for written informed consent was waived by the Institutional Review Board.
2.2 Treatment plans
Patients were immobilized in a stereotactic body frame in a supine position with arms raised above the head. Portal venous phase contrast-enhanced computed tomography (CT) was obtained, covering the whole abdominal cavity. Diagnostic magnetic resonance imaging (MRI) was fused with multiple registration strategies to guarantee maximal accuracy and delineate the gross tumor volume (GTV). The clinical target volume (CTV) was coincident with GTV, and the planning target volume (PTV) was 5 mm axial and 10 mm cranio-caudal extension from CTV.
The ASP was used to design auxiliary structures, beams, initial objectives, and constraints. Then, the parameters were adjusted for optimization. The details of the ASP program were illustrated in our previous study (23). Objectives and constraints were further adjusted according to the prescription and limitations of OARs as recommended by Dr. Robert Timmerman (25). Parameter adjustment was based on each optimized objective value in the range of 10 to 30 times tolerance (tolerance = 0.0001). The total number of iterations per patient was arbitrarily set to 10. The minimum precision of the automatic adjustment was 2 cGy.
Four different VMAT plans were designed for each patient by adding the number of half arcs (181 degrees to 0 degrees); HA1 to HA4 stood for 1 to 4 half-arc VMAT plans, respectively. According to our previous study, the PTV dose was controlled in the range of 90%–110%, 90%–125%, and 90%–∞% of the prescription dose when utilizing the full arc. Heterogeneous PTV dosage improves lung SBRT planning (24). Considering the target volume located on the right side of the body, we used a half-arc and loosened the homogeneity limitation. The PTV dosage was controlled between 90% and 150%. Then, 48 Gy was delivered in four fractions for PTV; 99% of PTV received at least 90% of the prescription dose; and 95% of PTV was conformally covered by 100% of the prescription isodose curve. The heterogeneity index (HI) was calculated based on the International Commission on Radiation Units and Measurements report 83, and the conformity index (CI) was calculated according to the Paddick index (26). D2cm was the maximum dose (% prescription dose) to any point 2 cm away from the PTV in any direction; R50% was the ratio of 50% prescription isodose volume to PTV. Ten rings (each 5 mm in width) outside the PTV were considered to limit the OAR dose and evaluate the dose fall-off.
2.3 Plan analysis
Data are recorded as median value and their interquartile range (25%, 75%). The percentage differences were calculated as follows: (B − A)/A (A vs. B). The dose-volume parameters and delivery efficiency among the four plans were analyzed by the Friedman test. Values in different groups were compared by the Wilcoxon signed-rank test; p < 0.05 (two-tailed, Friedman) and p < 0.017 (α/3, two-tailed, Wilcoxon) were considered statistically significant.
3 Results
3.1 Clinical characteristics of included patients
The clinical characteristics of the 31 patients are summarized in Table 1. The average age of all the 31 patients was 55, ranging from 28 to 77. Seventeen (54.8%) patients were male, and 14 (45.2%) were female. Nine (29.0%) of them were diagnosed with primary HCC, and 22 (71.0%) were diagnosed with liver metastatic disease. Twenty-five (80.7%) patients were in the Child-Pugh A stage, only 1 (3.2%) patient was in the Child-Pugh B stage, and 5 (6.1%) of these patients remained uncategorized.
3.2 PTV dosage
The major parameters are summarized in Table 2. The average D2% of PTV was 62.23 Gy, 64.23 Gy, 64.63 Gy, and 64.56 Gy for HA1, HA2, HA3, and HA4, respectively. D2% in HA2 was significantly higher than in HA1 by 2.25% (p < 0.05), and there was no significant difference in the following plans. The average D50% of PTV in HA1 to HA4 was 56.64 Gy, 57.19 Gy, 57.13 Gy, and 56.68 Gy, respectively. D50% in HA2 was significantly higher than in HA1 by 0.52% (p = 0.004) (Table 3). In HA1 to HA4 plans, D98% ranged from 46.13 Gy to 46.19 Gy without significant differences (p = 0.357), and D99% ranged from 44.93 Gy to 44.99 Gy; however, there was no statistical difference (p = 0.318). Regarding the CI, HA2 and HA3 showed significantly higher CI, and the mean percentage differences were 0.59% (HA2 vs. HA1, p < 0.001) and 0.14% (HA3 vs. HA2, p = 0.003), respectively. However, the HI index was higher in HA2 than in HA1 (p < 0.001). There was no difference in HA3 or HA4. The average of MUs gradually increased from 2,021.9 to 2,430.4 (Table 2), and the mean percentage differences were 11.35% (HA2 vs. HA1, p < 0.001) and 2.31% (HA3 vs. HA2, p < 0.001). The median beam-on-time was 109, 128, 146, and 177 s for HA1, HA2, HA3, and HA4, respectively. The median beam-on-time significantly increased by 15.45% (HA2 vs. HA1, p < 0.001), 12.77% (HA3 vs. HA2, p < 0.001), and 17.61% (HA4 vs. HA3, p < 0.001), respectively.
3.3 OAR dosage
All the parameters of OARs are summarized in Tables 2, 3.
3.3.1 Spinal cord
When evaluating the spinal cord damage, the D0.35cc and D0.03ccwere gradually decreased from HA1 to HA4. The maximum dosage, including D0.35cc and D0.03cc of HA2, was decreased by 13.03% (CI: 1.99% to 16.99%, p = 0.001) and 9.53% (CI: 1.05% to 18.21%, p = 0.003) compared to HA1, respectively.
3.3.2 Duodenum and bowel
When considering duodenum damage during liver SBRT, D5cc and D0.03cc were the most commonly used parameters. D5cc and D0.03cc were significantly reduced by 3.64% and 4.30% from HA1 to HA2, respectively. However, increasing more arcs did not decrease the D0.03cc. Meanwhile, the Dmean of duodenum was lower in HA2 than in HA1 (p < 0.001). D2cc, D5cc, and D0.03cc were used for the bowel, which includes all segments of the small intestines except the duodenum. From HA1 to HA2, D2cc, D5cc, and D0.03cc significantly decreased by 5.44%, 22.72%, and 5.03%, respectively.
3.3.3 Right kidney
The right kidney is adjacent to the lower segment of the liver; thus, its safety is critical. Compared to HA1, D2cc decreased by 1.98% in HA2 (p = 0.013); however, D0.03cc was not significantly different. Dmean for the right kidney also decreased by 4.46% from HA1 to HA2 (p = 0.003). No significant improvement was observed in HA3 or HA4.
3.3.4 Stomach
For the stomach, from HA1 to HA4, the median D5cc was 5.69 Gy, 4.78 Gy, 4.32 Gy, and 4.35 Gy, and the median D0.03cc was 8.20 Gy, 7.54 Gy, 6.49 Gy, and 6.56 Gy, respectively. From HA1 to HA2, D5cc and D0.03cc reduced 10.73% and 12.86%, respectively.
3.3.5 Normal liver
For normal liver, D500cc, D700cc, Dmean, and V5% were evaluated. D500cc, D700cc, Dmean,and V5% were all lower in HA2 than in HA1 and improved by 1.96%, 3.64%, 0.88%, and 2.10%, respectively (HA2 vs. HA1). Compared with HA2, D500cc, D700cc, Dmean, and V5% improved by 1.36%, 1.46%, 0.37%, and 0.66% in HA3, respectively. Thus, adding arcs may spare the normal liver.
3.4 Dose fall-off
The dose fall-off curves based on Dmean and D0.03ccof PTV are presented in Figures 1A, B, and the differences are shown in Table 4. Dmean dramatically decreased from ring 1 to ring 4, and then moderately decreased from ring 5 to ring 10. Using two arcs decreased the Dmean of each ring compared with using one arc. The value of Dmean got smaller with increasing arcs. However, there was no statistical difference between HA4 and HA3 as shown in Table 4. The D0.03cc in ring 1, ring 2, ring 4, and ring 6 was significantly lower in HA2 than those in HA1.
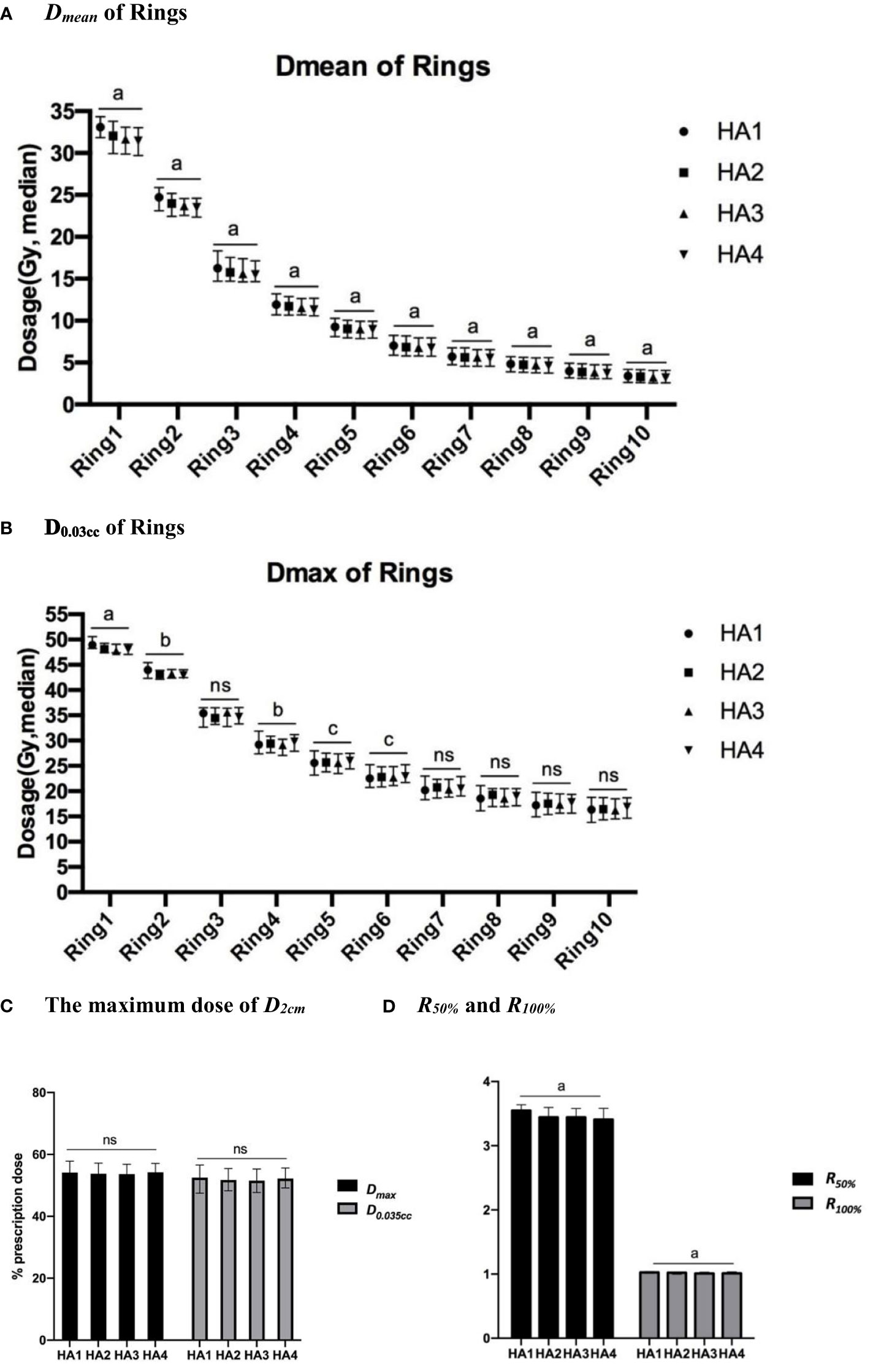
Figure 1 Dose fall-off analysis of different SBRT plans. Dose fall-off analysis among four stereotactic body radiation therapy (SBRT) treatment plans: (A) Dmean (median value) of 10 rings; (B) D0.03cc (median value) of 10 rings; (C) The maximum dose (median) of D2cm, (D) The R50% and R100% of all patients. a, p<0.0001; b, p<0.01; c, p<0.05; ns, p≥0.05.
In this study, we used D2cm, R50%, and R100% to evaluate dose spillage. From HA1 to HA4, the Dmaxand D0.035cc of D2cm (Figure 1C) ranged from 53% to 54% and from 52% to 53%, respectively. Dmax and D0.035cc showed no significant difference after increasing the number of arcs. From HA1 to HA4, the value of R50% was 2.61 to 5.63 in all patients. The mean value of R50% significantly decreased by 1.67% in HA2 compared to HA1. R100% ranged from 0.98 to 1.20 in all the plans. R100% was lower in HA2 than in HA1 and decreased by 0.14% in HA3 compared with HA2 (Figure 1D and Table 4).
4 Discussion
To our knowledge, this is the first study to use the self-coding automatic software program to generate the liver SBRT plan and compare the efficacy by adding the number of half arcs in the VMAT plans. This system was previously validated in lung tumors (22, 24), and this ASP for each plan can be similarly optimized. Thus, we can minimize the impact of the experience or clinical preference of different physicists and reduce the deviations caused by manual adjustments.
In the past few years, IMRT has been the most commonly used method to generate SBRT plans (27). Recently, VMAT became popular due to its more flexible gantry rotation, variable dose rate, and dynamic multileaf collimator movement (28). Thus, VMAT can improve the dose distribution and shorten the treatment duration in both conventional and SBRT radiotherapy plans (29). Recent studies showed that a partial-arc SBRT plan can better protect normal tissues without sacrificing the target dose in lung SBRT (30). Considering that the anatomical location of the liver tumor is mostly unilateral, we set up the half-arc rotating to cover the whole liver instead of using a full arc. Then, we used D2cm, R50%, CI, and HI index to analyze the dosimetric characteristics. D2cm and R50% were normally used to evaluate the low-dose spillage in the SBRT plan, according to RTOG0813 (31). In the standard criteria, the maximum dose of D2cm should be limited to 50%–77% of the prescription dose, and the R50% value should be stipulated to <2.9–5.9. In our plans, the D0.03cc of D2cm was approximately 53%–54% due to the relatively larger size of liver tumors, which was acceptable. Seven of the thirty-one patients did not meet the R50% criteria in HA1. Using multiple arcs helped four of the seven the suggested goal in HA2 to HA4. On the other hand, as the number of arcs increased (from one to three arcs), the CI of PTV improved, indicating that increasing arcs enhanced conformity. However, the four-arc plan did not improve the CI. Thus, more flexible dose delivery in the VMAT plan can limit dose spillage without compromising conformity.
Multiple concentric rings outside the PTV were used for dose fall-off limitations, which have been studied among stereotactic radiosurgery plans (32, 33). In this study, we conducted 10 concentric rings evaluating dose fall-off and measured the dosimetric parameters for each ring. Analyzing the D0.03ccof rings indicated that using two half-arcs enhanced normal tissue safety. However, no further progress was observed in the HA3 or HA4 optimization plans. Moreover, the dose fall-off curve based on the Dmean of rings was steep from ring 1 to 4 and then became mild from ring 4 to 10. Previous studies have demonstrated the advantages of the SBRT plan with heterogeneous plan optimization (24). Thus, we loosened the limitations of HI when establishing the optimization characteristics, providing more conformal radiation plans. In previous studies, multiple arcs increased beam-on-time (34, 35). In this study, the longer beam-on time was observed after using more arcs.
The most dangerous toxicity in liver SBRT is RILD. As a classic parallel organ, the risk of RILD depends on the dose of irradiation and the volume of the irradiated organ (18). Several studies have established different models of dose limitation to minimize the risk of RILD. A group initially used the dose constraint of at least 700 cc of normal liver <15 Gy in three fractions, and no patient experienced grade 3 liver or intestinal dysfunction (36). This clinical trial included patients with liver metastases; however, patients with primary HCC are more susceptible to RILD due to hepatic dysfunction or cirrhosis. Some studies suggested that for patients in the Child-Pugh B stage, the dose to one-third of the uninvolved liver was restricted to ≳18 Gy, and the dose to ≧500 cc of the uninvolved liver was restricted to <12 Gy in five fractions (37, 38).
Meanwhile, the mean dose of a normal liver is also required, according to the QUANTEC report in 2010. The mean dose should be <15 Gy for liver metastases in three fractions and <20 Gy for liver metastases in six fractions (18). In our study, the mean dose of 700 cc of the normal liver and the mean dose of the normal liver were below the constraints. D500cc ranged from 6.71 Gy to 6.29 Gy which met the limitations mentioned above. Dmean ranged from 9.27 Gy to 9.33 Gy. Increasing the number of arcs (one to four arcs) decreased D500cc, D700cc, and Dmean, resulting in a lower probability of RILD.
The right side of the kidney is anatomically adjacent to the liver. Previous studies showed that, as a mixed serial and parallel organ, the kidney obeys a volume–dose-response relationship. Cassady found that a total dose was associated with a 5% and 50% risk of injury after 5 years of 18–23 Gy and 28 Gy radiotherapy, respectively (39). Nevertheless, Cheng et al. showed that a dose of 9.8 Gy was associated with a 5% risk of kidney toxicity (40). In 2010, the AAPM published a recommendation for kidney dose limitation. Based on the threshold dose, 200 cc of the kidney volume should be exposed to less than 16 Gy in three fractions or 17.5 Gy in five fractions (41). Based on RTOG1112, the mean dose of bilateral kidneys should be less than 10 Gy. In this study, the mean dose of the right kidney ranged from 0.83 Gy to 1.15 Gy in HA1 to HA4. Among the 31 patients, only one patient had a Dmean of 4.9 Gy. From HA1 to HA4, D0.03cc ranged from 13.27 Gy to 14.23 Gy, and D2cc ranged from 5.98 Gy to 7.80 Gy. Dmean reduced by 4.46% and 0.43% from HA1 to HA2 and from HA2 to HA3, respectively. Using a multiple-arc plan may help reduce the dose to the kidney (HA1 to HA4) when D0.03cc is significantly decreased.
Among the serial organs, the spinal cord, duodenum, colon, and stomach were the most critical organs. Kopek et al. found that the mean maximum dose of 1 cc of duodenum (D1cc) was significantly higher for patients with grade ≥2 ulceration or stenosis (37.4 Gy vs. 25.3 Gy). However, patients receiving a dosage lower than 25.3 Gy only experienced grade 0 or 1 duodenal toxicity (42). Bae et al. also indicated that D0.03cc can be a valuable predictor of gastroduodenal toxicity, as the D0.03ccof 35 Gy and 38 Gy were respectively associated with a 5% and 10% probability of developing severe gastroduodenal toxicity (43). Thus, we used D0.03cc and D5cc to evaluate the safety of the duodenum, colon, stomach, and small bowel according to RTOG1112 and TG101 recommendations. The D0.03cc or D5cc of each organ met the criteria. Compared with HA1, the D0.03ccand D5cc of the gastrointestinal organs decreased in HA2. Meanwhile, only the D0.03cc and D5cc of the stomach continued to reduce in HA3. These results indicate that gastrointestinal OARs can be reduced by increasing the number of partial arcs, and the effect decreases by using three arcs.
Adding several arcs improved and helped spare the OARs. The stomach, kidneys, and bowels were the most significant ones. Normal liver was also protected by decreasing maximum dose in D700cc and D500cc, respectively.
However, it is important to acknowledge certain limitations in our study. Firstly, this was a retrospective analysis, potentially introducing selection bias. Secondly, our investigation was confined to a single-center setting, relying on a limited patient pool. The relatively large tumor sizes within our cohort may restrict the generalizability of our findings to specific patient groups. Lastly, the optimized plans have yet to be implemented for patient treatment. Assessing the clinical outcomes of patients treated with these optimized plans is essential for verifying their organ-sparing benefits. Moreover, comparing our proposed approach with alternative methods may hold promise for future research endeavors.
5 Conclusion
In the present study, we used an ASP to generate SBRT plans for oligo liver tumors. The process was more objective and had less dependence on physicians’ skills or preferences, which can help rule out manual bias. We comprehensively compared multiple-arc plans by using ASP. Using more arcs improved conformity but sacrificed planning homogeneity. In addition, increasing half-arcs improved dose distribution and dose fall-off setting. A sharper dose of fall-off planning showed prominent benefits in protecting OARs. However, the advantages were mostly found in two or three half-arc plans in the present study. Only the liver, kidney, and bowel were protected in the four-arc plan. All in all, using ASP may improve the consistency of the liver SBRT plan, and using three to four half arcs may improve plan conformity with better protection of surrounding OARs. On the other hand, the beam-on time was prolonged. Considering both treatment quality and efficiency, a three-arc plan is suitable for clinical application.
Data availability statement
The raw data supporting the conclusions of this article will be made available by the authors, without undue reservation.
Ethics statement
The studies involving humans were approved by the Clinical Research Committee of the study institute. The studies were conducted in accordance with local legislation and institutional requirements. The ethics committee/institutional review board waived the requirement of written informed consent for participation from the participants or the participants’ legal guardians/next of kin because this is a retrospective study without any intervention during patients’ previous treatment.
Author contributions
SZ: Conceptualization, Data curation, Formal Analysis, Investigation, Methodology, Validation, Writing – original draft. WZ: Data curation, Formal Analysis, Writing – original draft. NZ: Investigation, Writing – original draft, Data curation, Methodology. JY: Data curation, Formal Analysis, Writing – original draft. MX: Investigation, Writing – review and editing. WL: Funding acquisition, Validation, Writing – review and editing. NC: Supervision, Validation, Writing – review and editing. JX: Conceptualization, Funding acquisition, Supervision, Validation, Writing – review and editing.
Funding
The author(s) declare financial support was received for the research, authorship, and/or publication of this article. This work is supported by the National Nature Science Foundation of China (NSFC 62071314, NSFC 82203197), the Sichuan Science and Technology Program (23ZDYF0638), and the New Technology Program, West China Hospital, Sichuan University (2022-377).
Conflict of interest
The authors declare that the research was conducted in the absence of any commercial or financial relationships that could be construed as a potential conflict of interest.
The author(s) declared that they were an editorial board member of Frontiers at the time of submission. This had no impact on the peer review process or the final decision
Publisher’s note
All claims expressed in this article are solely those of the authors and do not necessarily represent those of their affiliated organizations, or those of the publisher, the editors and the reviewers. Any product that may be evaluated in this article, or claim that may be made by its manufacturer, is not guaranteed or endorsed by the publisher.
Supplementary material
The Supplementary Material for this article can be found online at: https://www.frontiersin.org/articles/10.3389/fonc.2023.1273042/full#supplementary-material
Abbreviations
ASP, Automatic stereotactic body radiation therapy planning; SBRT, Stereotactic body radiation therapy; VMAT, Volumetric-modulated arc therapy; MLD, Mean lung dose; PTV, Planning target volume; Dmax, Maximum dose; OARs, Organs at risk; HCC, Hepatocellular carcinoma; HI, Heterogeneity index; CI, Conformity index; R50% and R100%, Ratio of 50% and 100% prescription isodose volume to the PTV volume; MUs, Monitor units; DV, absorbed dose covering a specified volume V; Dmean, Mean dose; RILD, Radiation-induced liver disease; Dmin, Minimum dose; D2cm, Maximum dose (in % of dose prescribed) 2 cm from PTV in any direction; DN%, N% volume received dose; DNcc, minimum absorbed dose that covers N cc of the volume; VD, the volume that receives at least the absorbed dose of D Gy.
References
1. Llovet JM, Zucman-Rossi J, Pikarsky E, Sangro B, Schwartz M, Sherman M, et al. Hepatocellular carcinoma. Nat Rev Dis Primers (2016) 2:16018. doi: 10.1038/nrdp.2016.18
2. Forner A, Reig M, Bruix J. Hepatocellular carcinoma. Lancet (2018) 391(10127):1301–14. doi: 10.1016/S0140-6736(18)30010-2
3. Chang DT, Swaminath A, Kozak M, Weintraub J, Koong AC, Kim J, et al. Stereotactic body radiotherapy for colorectal liver metastases: a pooled analysis. Cancer (2011) 117(17):4060–9. doi: 10.1002/cncr.25997
4. Mahadevan A, Blanck O, Lanciano R, Peddada A, Sundararaman S, D'Ambrosio D, et al. Stereotactic Body Radiotherapy (SBRT) for liver metastasis - clinical outcomes from the international multi-institutional RSSearch(R) Patient Registry. Radiat Oncol (2018) 13(1):26. doi: 10.1186/s13014-018-0969-2
5. Chen W, Chiang CL, Dawson LA. Efficacy and safety of radiotherapy for primary liver cancer. Chin Clin Oncol (2021) 10(1):9. doi: 10.21037/cco-20-89
6. Lasley FD, Mannina EM, Johnson CS, Perkins SM, Althouse S, Maluccio M, et al. Treatment variables related to liver toxicity in patients with hepatocellular carcinoma, Child-Pugh class A and B enrolled in a phase 1-2 trial of stereotactic body radiation therapy. Pract Radiat Oncol (2015) 5(5):e443–9. doi: 10.1016/j.prro.2015.02.007
7. Bujold A, Massey CA, Kim JJ, Brierley J, Cho C, Wong RK, et al. Sequential phase I and II trials of stereotactic body radiotherapy for locally advanced hepatocellular carcinoma. J Clin Oncol (2013) 31(13):1631–9. doi: 10.1200/JCO.2012.44.1659
8. Moon DH, Wang AZ, Tepper JE. A prospective study of the safety and efficacy of liver stereotactic body radiotherapy in patients with and without prior liver-directed therapy. Radiother Oncol (2018) 126(3):527–33. doi: 10.1016/j.radonc.2018.01.004
9. Nicosia L, Cuccia F, Mazzola R, Figlia V, Giaj-Levra N, Ricchetti F, et al. Stereotactic body radiotherapy (SBRT) can delay polymetastatic conversion in patients affected by liver oligometastases. J Cancer Res Clin Oncol (2020) 146(9):2351–8. doi: 10.1007/s00432-020-03223-9
10. Kuo YC, Chiu YM, Shih WP, Yu HW, Chen CW, Wong PF, et al. Volumetric intensity-modulated Arc (RapidArc) therapy for primary hepatocellular carcinoma: comparison with intensity-modulated radiotherapy and 3-D conformal radiotherapy. Radiat Oncol (2011) 6:76. doi: 10.1186/1748-717X-6-76
11. Park SH, Kim JC, Kang MK. Technical advances in external radiotherapy for hepatocellular carcinoma. World J Gastroenterol (2016) 22(32):7311–21. doi: 10.3748/wjg.v22.i32.7311
12. Chino F, Stephens SJ, Choi SS, Marin D, Kim CY, Morse MA, et al. The role of external beam radiotherapy in the treatment of hepatocellular cancer. Cancer (2018) 124(17):3476–89. doi: 10.1002/cncr.31334
13. Woods K, Nguyen D, Tran A, Yu VY, Cao M, Niu T, et al. Viability of non-coplanar VMAT for liver SBRT as compared to coplanar VMAT and beam orientation optimized 4pi IMRT. Adv Radiat Oncol (2016) 1(1):67–75. doi: 10.1016/j.adro.2015.12.004
14. Sharfo AW, Dirkx ML, Breedveld S, Mendez Romero A, Heijmen BJ. VMAT plus a few computer-optimized non-coplanar IMRT beams (VMAT+) tested for liver SBRT. Radiother Oncol (2017) 123(1):49–56. doi: 10.1016/j.radonc.2017.02.018
15. Stathakis S, Narayanasamy G, Licon AL, Myers P, Li Y, Crownover R, et al. A dosimetric comparison between volumetric-modulated arc therapy and dynamic conformal arc therapy in SBRT. J BUON (2019) 24(2):838–43.
16. Cox JD, Stetz J, Pajak TF. Toxicity criteria of the radiation therapy oncology group (RTOG) and the european organization for research and treatment of cancer (EORTC). Int J Radiat Oncol Biol Phys (1995) 31(5):1341–6. doi: 10.1016/0360-3016(95)00060-C
17. Emami B, Lyman J, Brown A, Coia L, Goitein M, Munzenrider JE, et al. Tolerance of normal tissue to therapeutic irradiation. Int J Radiat Oncol Biol Phys (1991) 21(1):109–22. doi: 10.1016/0360-3016(91)90171-Y
18. Pan CC, Kavanagh BD, Dawson LA, Li XA, Das SK, Miften M, et al. Radiation-associated liver injury. Int J Radiat Oncol Biol Phys (2010) 76(3 Suppl):S94–100. doi: 10.1016/j.ijrobp.2009.06.092
19. Gerum S, Jensen AD, Roeder F. Stereotactic body radiation therapy in patients with hepatocellular carcinoma: A mini-review. World J Gastrointest Oncol (2019) 11(5):367–76. doi: 10.4251/wjgo.v11.i5.367
20. Chung HT, Lee B, Park E, Lu JJ, Xia P. Can all centers plan intensity-modulated radiotherapy (IMRT) effectively? An external audit of dosimetric comparisons between three-dimensional conformal radiotherapy and IMRT for adjuvant chemoradiation for gastric cancer. Int J Radiat Oncol Biol Phys (2008) 71(4):1167–74. doi: 10.1016/j.ijrobp.2007.11.040
21. Bohsung J, Gillis S, Arrans R, Bakai A, De Wagter C, Knoos T, et al. IMRT treatment planning:- a comparative inter-system and inter-centre planning exercise of the ESTRO QUASIMODO group. Radiother Oncol (2005) 76(3):354–61. doi: 10.1016/j.radonc.2005.08.003
22. Wei Z, Peng X, He L, Wang J, Liu Z, Xiao J. Treatment plan comparison of volumetric-modulated arc therapy to intensity-modulated radiotherapy in lung stereotactic body radiotherapy using either 6- or 10-MV photon energies. J Appl Clin Med Phys (2022) 23(8):e13714. doi: 10.1002/acm2.13714
23. He L, Peng X, Chen N, Wei Z, Wang J, Liu Y, et al. Automated treatment planning for liver cancer stereotactic body radiotherapy. Clin Transl Oncol (2023) 25(11):3230–40. doi: 10.1007/s12094-023-03196-4
24. Wei Z, Peng X, Wang Y, Yang L, He L, Liu Z, et al. Influence of target dose heterogeneity on dose sparing of normal tissue in peripheral lung tumor stereotactic body radiation therapy. Radiat Oncol (2021) 16(1):167. doi: 10.1186/s13014-021-01891-6
25. Timmerman R. A story of hypofractionation and the table on the wall. Int J Radiat Oncol Biol Phys (2022) 112(1):4–21. doi: 10.1016/j.ijrobp.2021.09.027
26. Paddick I. A simple scoring ratio to index the conformity of radiosurgical treatment plans. Technical note. J Neurosurg (2000) 93 Suppl 3:219–22. doi: 10.3171/jns.2000.93.supplement_3.0219
27. Rusthoven KE, Kavanagh BD, Cardenes H, Stieber VW, Burri SH, Feigenberg SJ, et al. Multi-institutional phase I/II trial of stereotactic body radiation therapy for liver metastases. J Clin Oncol (2009) 27(10):1572–8. doi: 10.1200/JCO.2008.19.6329
28. Wang P-M, Hsu W-C, Chung N-N, Chang F-L, Jang C-J, Fogliata A, et al. Feasibility of stereotactic body radiation therapy with volumetric modulated arc therapy and high intensity photon beams for hepatocellular carcinoma patients. Radiat Oncol (2014) 9(1):18. doi: 10.1186/1748-717X-9-18
29. Cantaloube M, Castan F, Creoff M, Prunaretty J, Bordeau K, Michalet M, et al. Image-guided liver stereotactic body radiotherapy using VMAT and real-time adaptive tumor gating: evaluation of the efficacy and toxicity for hepatocellular carcinoma. Cancers (Basel) (2021) 13(19):4853. doi: 10.3390/cancers13194853
30. Pokhrel D, Halfman M, Sanford L. FFF-VMAT for SBRT of lung lesions: Improves dose coverage at tumor-lung interface compared to flattened beams. J Appl Clin Med Phys (2020) 21(1):26–35. doi: 10.1002/acm2.12764
31. Bowers J, Bennion NR, Richardson M, Spencer K, Larner J, Kersh R. Stereotactic body radiation therapy (SBRT) for centrally located primary and recurrent non-small cell lung cancer (NSCLC): analysis of toxicity and local control. J Radiat Oncol (2017) 6(3):247–53. doi: 10.1007/s13566-016-0289-9
32. Guckenberger M, Baus WW, Blanck O, Combs SE, Debus J, Engenhart-Cabillic R, et al. Definition and quality requirements for stereotactic radiotherapy: consensus statement from the DEGRO/DGMP Working Group Stereotactic Radiotherapy and Radiosurgery. Strahlenther Onkol (2020) 196(5):417–20. doi: 10.1007/s00066-020-01603-1
33. Fanous AA, Prasad D, Mathieu D, Fabiano AJ. Intracranial stereotactic radiosurgery. J Neurosurg Sci (2019) 63(1):61–82.
34. Richter A, Exner F, Bratengeier K, Polat B, Flentje M, Weick S. Impact of beam configuration on VMAT plan quality for Pinnacle(3)Auto-Planning for head and neck cases. Radiat Oncol (2019) 14(1):12. doi: 10.1186/s13014-019-1211-6
35. Radhakrishnan S, Chandrasekaran A, Sarma Y, Balakrishnan S, Nandigam J. Dosimetric comparison between single and dual arc-volumetric modulated arc radiotherapy and intensity modulated radiotherapy for nasopharyngeal carcinoma using a simultaneous integrated boost technique. Asian Pac J Cancer Prev (2017) 18(5):1395–402. doi: 10.22034/APJCP.2017.18.5.1395
36. Schefter TE, Kavanagh BD, Timmerman RD, Cardenes HR, Baron A, Gaspar LE. A phase I trial of stereotactic body radiation therapy (SBRT) for liver metastases. Int J Radiat Oncol Biol Phys (2005) 62(5):1371–8. doi: 10.1016/j.ijrobp.2005.01.002
37. Cardenes HR, Price TR, Perkins SM, Maluccio M, Kwo P, Breen TE, et al. Phase I feasibility trial of stereotactic body radiation therapy for primary hepatocellular carcinoma. Clin Transl Oncol (2010) 12(3):218–25. doi: 10.1007/s12094-010-0492-x
38. Andolino DL, Johnson CS, Maluccio M, Kwo P, Tector AJ, Zook J, et al. Stereotactic body radiotherapy for primary hepatocellular carcinoma. Int J Radiat Oncol Biol Phys (2011) 81(4):e447–53. doi: 10.1016/j.ijrobp.2011.04.011
39. Cassady JR. Clinical radiation nephropathy. Int J Radiat Oncol Biol Phys (1995) 31(5):1249–56. doi: 10.1016/0360-3016(94)00428-N
40. Cheng JC, Schultheiss TE, Wong JY. Impact of drug therapy, radiation dose, and dose rate on renal toxicity following bone marrow transplantation. Int J Radiat Oncol Biol Phys (2008) 71(5):1436–43. doi: 10.1016/j.ijrobp.2007.12.009
41. Benedict SH, Yenice KM, Followill D, Galvin JM, Hinson W, Kavanagh B, et al. Stereotactic body radiation therapy: the report of AAPM Task Group 101. Med Phys (2010) 37(8):4078–101. doi: 10.1118/1.3438081
42. Kopek N, Holt MI, Hansen AT, Hoyer M. Stereotactic body radiotherapy for unresectable cholangiocarcinoma. Radiother Oncol (2010) 94(1):47–52. doi: 10.1016/j.radonc.2009.11.004
Keywords: liver cancer, dose sparing, stereotactic body radiation therapy, volumetric-modulated arc therapy, auto-planning, number of half arcs
Citation: Zhang S, Zhan W, Zeng N, Yang J, Xiong M, Liao W, Chen N and Xiao J (2023) Dosimetric comparison in sparing normal tissue dosage by using auto-SBRT planning in oligo liver tumors. Front. Oncol. 13:1273042. doi: 10.3389/fonc.2023.1273042
Received: 05 August 2023; Accepted: 18 October 2023;
Published: 09 November 2023.
Edited by:
Christian Graeff, Helmholtz Association of German Research Centres (HZ), GermanyReviewed by:
Francesco Cuccia, ARNAS Ospedali Civico Di Cristina Benfratelli, ItalyRaees Tonse, Baptist Hospital of Miami, United States
Copyright © 2023 Zhang, Zhan, Zeng, Yang, Xiong, Liao, Chen and Xiao. This is an open-access article distributed under the terms of the Creative Commons Attribution License (CC BY). The use, distribution or reproduction in other forums is permitted, provided the original author(s) and the copyright owner(s) are credited and that the original publication in this journal is cited, in accordance with accepted academic practice. No use, distribution or reproduction is permitted which does not comply with these terms.
*Correspondence: Jianghong Xiao, xiaojh@scu.edu.cn