- 1Cancer Biology and Epigenetics Group, Research Center of IPO Porto (CI-IPOP)/RISE@CI-IPOP (He-alth Research Network), Portuguese Oncology Institute of Porto (IPO Porto)/Porto Comprehensive Cancer Centre (Porto.CCC), Porto, Portugal
- 2Department of Pathology, Portuguese Oncology Institute of Porto (IPO Porto), Porto, Portugal
- 3Department of Pathology and Molecular Immunology, School of Medicine and Biomedical Sciences of the University of Porto (ICBAS-UP), Porto, Portugal
Prostate cancer (PCa) is the second most common malignancy among men worldwide. Although early-stage disease is curable, advanced stage PCa is mostly incurable and eventually becomes resistant to standard therapeutic options. Different genetic and epigenetic alterations are associated with the development of therapy resistant PCa, with specific players being particularly involved in this process. Therefore, identification and targeting of these molecules with selective inhibitors might result in anti-tumoral effects. Herein, we describe the mechanisms underlying therapy resistance in PCa, focusing on the most relevant molecules, aiming to enlighten the current state of targeted therapies in PCa. We suggest that selective drug targeting, either alone or in combination with standard treatment options, might improve therapeutic sensitivity of resistant PCa. Moreover, an individualized analysis of tumor biology in each PCa patient might improve treatment selection and therapeutic response, enabling better disease management.
Introduction
Currently, prostate cancer (PCa) constitutes the second most common malignancy and the fifth leading cause of cancer-related death in men, worldwide (1). PCa is a highly heterogeneous disease (2), characterized by several genetic and epigenetic alterations (2, 3), some of which can be used to assist treatment decision-making (3). Localized disease arises from luminal cells’ proliferation (2), being characterized by a slow growth and hormone-responsiveness, more common in elderly men (3). At the time of diagnosis, 80% of all the tumors are confined to the prostate gland (2) and roughly 50% harbor the well-known gene fusion TMPRSS2:ERG (3–5), implicated in PI3K signaling pathway aberrant activation (3, 6), AR overexpression, PTEN loss (6) and deregulation of epigenetic players’ encoding genes (3). Genetic alterations might also occur, specifically in SPOP, TP53, ATM, MED12 and FOXA1 genes (3). Furthermore, epigenetics also plays a role in prostate carcinogenesis, with DNA hypermethylation as one of the first alterations observed at low stages (7). Herein, one of the most well-known promotor’s hypermethylated gene is the GSTP1, which occurs in 90% of the tumors (8). Interestingly, this alteration is also observed in 50% of the PCa precursor lesions, suggesting this as an early event in prostate carcinogenesis (8). Additionally, histone deacetylases (HDACs) overexpression frequently detected in high-grade disease, particularly HDAC1 and HDAC2, has been associated with increased cell proliferation (9).
In locally advanced PCa, tumor cells invade the extra-prostatic tissue and/or metastasize to regional lymph nodes, paving the way to metastatic dissemination at distant organs, most commonly to the bones, liver, and lungs (2). Several genome-wide copy-number alterations have been observed, particularly MYC overexpression and PTEN and SMAD4 deletion, which drives genomic instability and tumor progression (3). Specific epigenetic alterations similarly drive PCa progression, including EZH2 overexpression (2), RASSF1A promoter methylation (10) and overall hypomethylation (11).
Eventually, in due course of disease, PCa becomes resistant to androgen-deprivation therapy (ADT) – castration resistant PCa (CRPC) – disclosing raising serum PSA levels and/or clinical/imagiological tumor progression despite testosterone castrate levels (12). Interestingly, alterations in AR, TP53, PTEN, RB1, ETS2, DNA repair and chromatin and histone modifying genes are commonly found in CRPC (13–15). Moreover, it is observed amplification of the AR co-activator NCOA2 and deletion of the AR co-repressor LATS2 (13, 15). Furthermore, high DNA methylation levels (15), and overexpression of HDAC1, HDAC2, HDCA3, EZH2 (16), G9a (17) and LSD1 (18) have also been associated with CRPC.
Approximately 17% of tumors from CRPC patients eventually become AR indifferent (19, 20), progressing to a neuroendocrine PCa (NEPC) state, that does not respond to hormone therapy (19). NEPC harbors several genetic alterations, including TMPRSS2:ERG fusion, MYC and AKT overexpression, PTEN and RB1 loss, and TP53 mutations (2, 12, 21, 22). Moreover, epigenetic alterations, such as DNA hypermethylation as well as EZH2 and bromodomain and extra-terminal motif (BET) proteins overexpression have been found in NEPC (12).
Standard of Care in Prostate Cancer Treatment
Clinical parameters and tumor stage are crucial for therapy decision making in PCa, with therapeutic recommendations varying for each stage (Figure 1) (23, 24). For localized disease, several possibilities exist, including active surveillance and curative-intent strategies (radical prostatectomy (RP), external beam radiotherapy (EBRT) and brachytherapy) (24, 25). Additionally, for the subset of high-risk localized PCa, neoadjuvant and concurrent ADT may be considered (25). Nevertheless, in approximately 30% of cases that undergo curative-intent treatment, disease progression develops, accompanied with lymph node invasion and/or metastatic dissemination. For these patients, ADT with luteinizing hormone-releasing hormone (LHRH) agonists, anti-androgens, or surgical castration is recommended (26–29). Initially, ADT typically leads to 90-95% decrease in circulating androgen levels, being complemented by a 50% decrease in intraprostatic dihydrotestosterone (DHT) and AR inhibition (30), impairing tumor cells’ survival (26, 28). However, within 18-30 months, cancer cells eventually become resistant to the different castration strategies (31). For CRPC, although no curative options are available, docetaxel is recommended for disease management (25). Moreover, it was reported that patients might also benefit from bicalutamide and low dose corticosteroids, which were found to control PSA levels and improve symptoms, although no increase in overall survival was depicted (32). In the beginning of 2022, the Food and Drug Administration (FDA) approved the use of the novel Novartis Pluvicto™ - Lu177 vipivotide tetraxetan – for the treatment of progressive, PSMA-positive metastatic CRPC (33). This novel approach, in combination with the standard of care, decreased the death risk, improved overall survival and progression-free survival of these subset of patients (33). Neuroendocrine differentiation of tumor cells is observed in 17% of CRPC patients and only palliative options are proposed for this disease state (34).
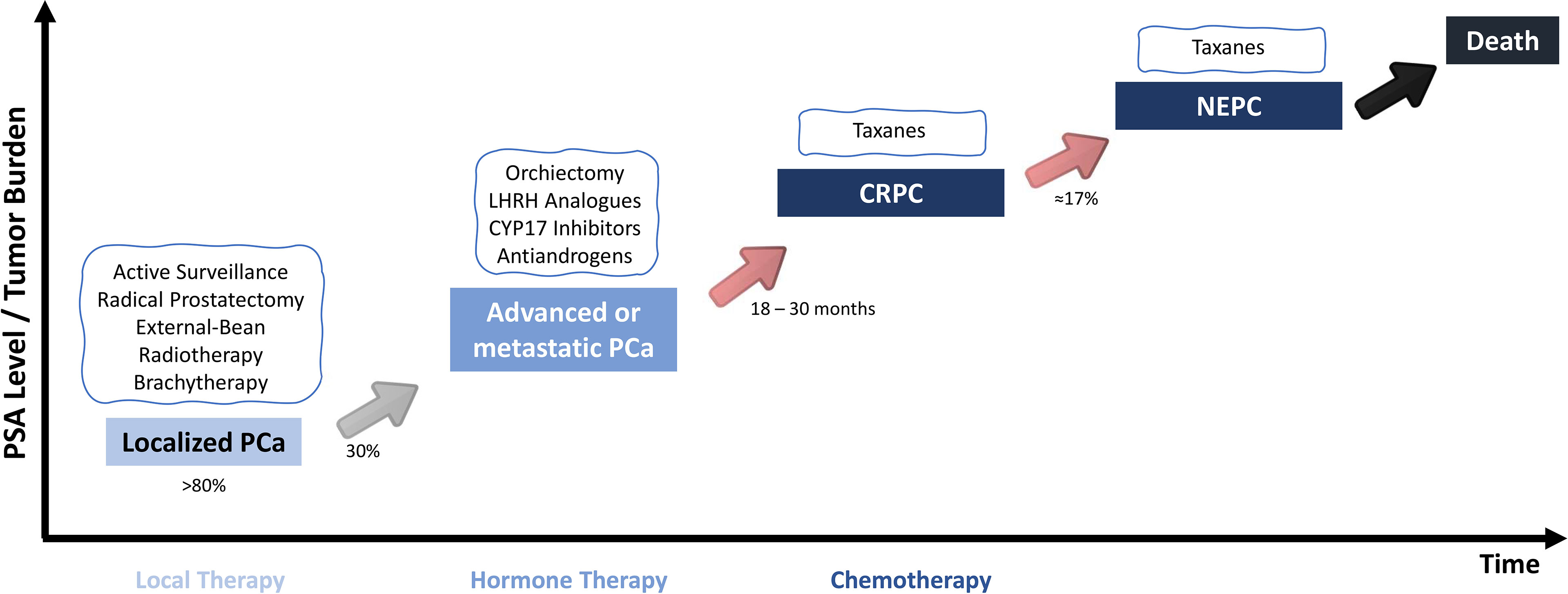
Figure 1 Standard of care for the different PCa stages. For Localized PCa, RP is the interventional standard of care. On the other hand, for advanced and metastatic disease, ADT is the recommended treatment. However, nearly all patients stop responding ADT and progress to a CRPC, for which there are no effective treatment options. Moreover, about 17% of the patients with a castration-resistant form of the disease will develop neuroendocrine differentiation, which is independent of AR signaling pathway. PSA, prostate-specific antigen; PCa, prostate cancer; CRPC, castration-resistant prostate cancer; NEPC, neuroendocrine prostate cancer.
Considering PCa disease progression, herein we intent to describe the mechanisms involved in therapy resistance in PCa, highlighting new potential drug targets.
Resistance Mechanisms
During treatment of advanced and metastatic PCa, most patients develop resistance to ADT (31, 35) and although this process is not fully understood, several mechanisms were reported to be involved in the acquisition of the castration-resistant state (Figure 2). Regardless of castrate levels of testosterone, tumor cells can proliferate due to clonal selection of cells with AR amplification (36). Thus, an enhanced number of receptors may bind to the vestigial androgens in circulation, maintaining AR signaling (36). Moreover, gain-of-function and point mutations in AR results in increased activation and decreased specificity, respectively, both resulting in tumor cell survival (37–39). Decreased AR specificity allows for growth factor-induced activation (39), through insulin-like growth-factor-1 (IGF-1), keratinocyte growth factor (KGF), epidermal growth factor (EGF) (37), and fibroblast growth factor (FGF) (40). Similarly, these growth factors also bind receptor tyrosine kinase (RTK), which can regulate AR activity (38, 40). RTK and their intracellular signaling pathways play an important role in CRPC cells’ proliferation and, among these, the ERBB family (41), PI3K (5), ERK1/2 (42), Src (43), ROR-γ (44) and the glucocorticoid receptor (GR) (45) were found hyperactivated in CRPC (41, 46). Cytokines such as TNFα, IL-6 and IL-23 have been additionally suggested to modulate AR. TNFα was shown to bind to its receptor and activate NF-kB signaling pathway (47), whereas IL-6 was involved in MAPK cascades activation (48), both triggering AR signaling. Calcinotto et al. further reported that IL-23, secreted by myeloid-derived suppressor cells (MDSCs), activates the STAT3-RORγ pathway, by binding to IL-23R on tumor cell surface, culminating in AR activation (49). Subsequently, AR binds to androgen-responsive elements (ARE) on DNA, and in association with different co-regulators, promotes gene expression (50). Importantly, when binding occurs in DNA repair genes’ regulatory regions, especially of PARP1, Ku-70, Ku-80 (51) and TOP2B (52), genomic rearrangements and DNA double stranded breaks may occur (53). The well-known TMPRSS2:ERG fusion can interact with the DNA repair protein and AR co-regulator PARP1, mediating transcription, invasion, and metastization (54). In AR-positive cells, GATA2, under the NOTCH family regulation, acts as an AR co-activator, maintaining AR signaling (55). Furthermore, different AR variants derived from alternative splicing have been shown to be involved in the acquisition of androgen-independent state (56). In CRPC, the most well described is the constitutively active AR-V7, which lacks the ligand-binding domain (LBD) and has an effective role in activating transcription (57). Epigenetic aberrations also contribute to post-ADT progression. In 30% of CRPC cases, AR expression might be completely lost and hypermethylation and histone post-translational modifications seem to be implicated in this process (2, 58, 59).
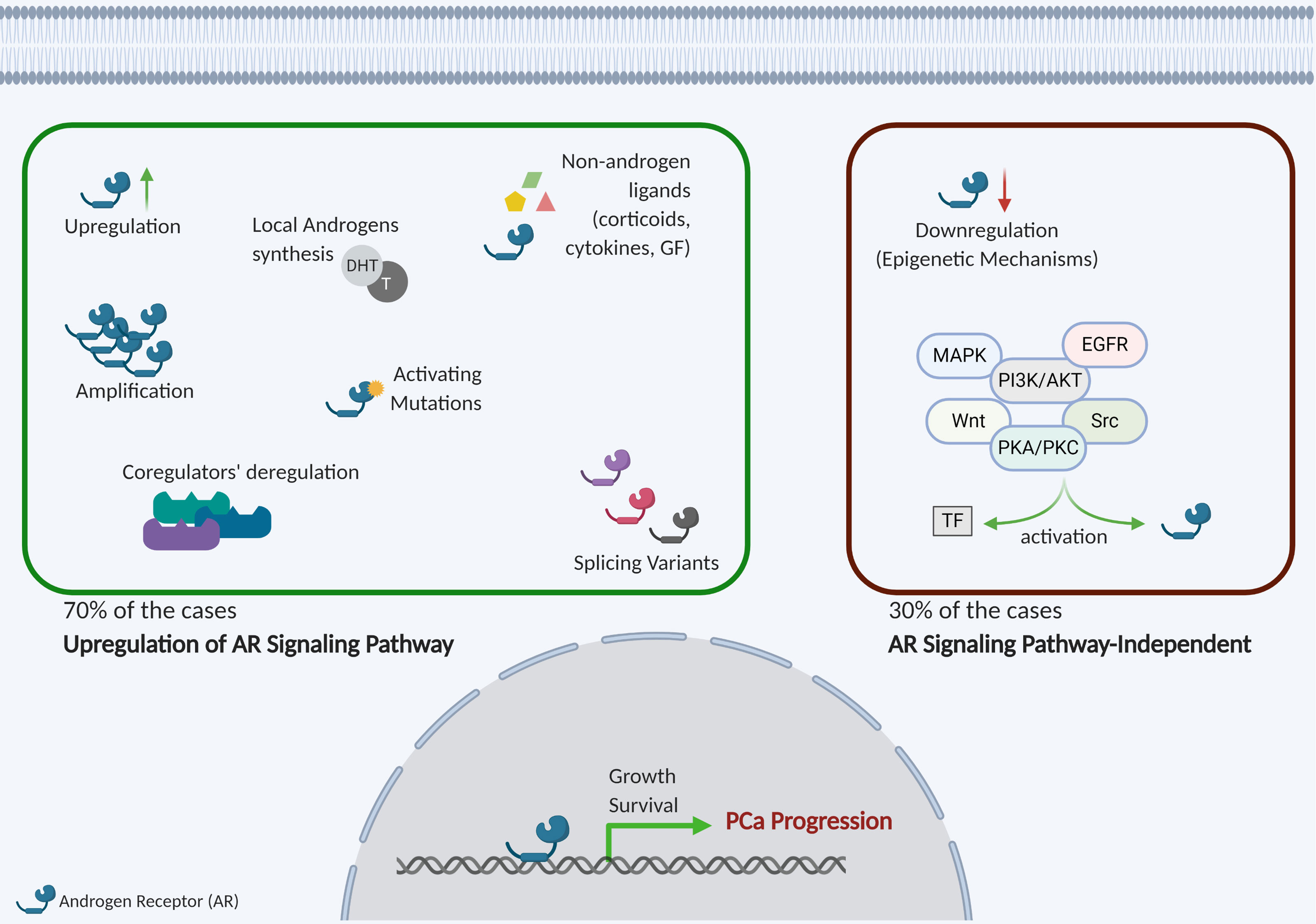
Figure 2 Mechanisms of resistance to androgen deprivation therapy. The process underlying ADT resistance and CRPC development involve, in 70% of the cases, upregulation of AR signaling pathway. In this case, upregulation of AR expression, amplification or activating mutations, AR splice variants, promiscuous AR activation by non-androgen ligands and deregulation of AR coactivators and co-repressors is observed. On the other hand, in 30% of the cases there is AR expression downregulation and activation of other signaling pathways involved in cell survival and growth activation. AR, androgen receptor; T, testosterone; DHT, dihydrotestosterone; GF, growth factors: TF, transcript factors. Created with BioRender.com.
After resistance to first-line ADT, second generation anti-androgens (e.g., enzalutamide, abiraterone acetate) were found to improve survival of CRPC patients. Nonetheless, tumor cells eventually become resistant due to AR signaling reactivation (60). A specific kinase, AURKA, which is involved in chromosome instability, was found overexpressed in AR-positive CRPC cells (60). Kivinummi and colleagues showed that AURKA expression was directly targeted by androgens, with the AR specifically binding to the gene regulatory regions, resulting in reduced progression-free survival (60).
For patients harboring CRPC, taxane-based chemotherapy is the only therapeutic option which increases survival. However, patients eventually become resistant to docetaxel treatment (61). Cancer cells expressing Mdr1 might be selected after therapy pressure, leading to decreased docetaxel intracellular intake (62). Moreover, alterations in microtubule-associated proteins’ expression result in decreased docetaxel efficacy (63). Indeed, tubulin isoform βIII overexpression correlated with docetaxel resistance in CRPC (63, 64).
Although recently approved (33), approximately 1/3 of the PSMA-positive CRPC patients do not benefit from the Lu177 vipivotide tetraxetan PSMA-based targeting (65, 66). Several studies have already pinpointed the PSMA heterogenic expression, defect on DNA repair genes, clonal expansion of PSMA-negative cells and tumor heterogeneity as possible mechanism of resistance (66). A particular work reported, in a mouse model, that TP53-negative tumors were less responsive to treatment, compared to TP53 wild-type tumor-bearing mice, highlighting a potential resistance mechanism (65) and a need for assessing resistance in further studies.
Furthermore, tumor microenvironment (TME) has been shown to be an important driver of resistance to ADT and taxane-based chemotherapy. The stromal component might promote CRPC progression through vascularization, apoptosis inhibition and epithelial mesenchymal transition (EMT) promotion (67). Specifically, cancer-associated fibroblasts (CAFs) are known to stimulate mesenchymal phenotype through αSMA (68), and besides promoting cancer progression through EMT-related mechanisms, TGFβ-dependent activation leads to growth factor secretion and sustainment of cancer cells survival (69).
Methods
A PubMed search was carried out, using the query (AR mutations OR AR variants OR γ-secretase inhibitor OR HERB inhibitor OR PI3K inhibitor OR AKT inhibitor OR mTOR inhibitor OR glucocorticoid receptor inhibitor OR ROR inhibitor OR IGFR inhibitor OR MAPK inhibitor OR AUKRA inhibitor OR Scr inhibitor OR MET inhibitor OR STAT3 inhibitor OR IL-23 antibody OR TOP2 inhibitor OR BET inhibitor OR HAT inhibitor OR HDAC inhibitor OR HMT inhibitor OR HDM inhibitor OR DNMT inhibitor) AND (prostate cancer), with the time interval from 2010 to 2022. Additionally, 23 research articles prior to 2010 covering relevant data were included. Only original research articles, written in English, and those including in vitro and/or in vivo pre-clinical studies reporting drug screening assays in prostate cancer were considered. The records were imported to the reference manager EndNote. Subsequently, all abstracts were critically evaluated and only those providing relevant information for the present topic were selected. Our aim was to address the recently reported targeted therapies and potential combinations that may improve disease management and care in PCa patients.
A summary of the methodology is provided in Figure 3.
Targeted Therapies
Having in mind that the aforementioned molecular alterations may account for PCa therapy resistance, we focused on the development and pre-clinical screening of new and effective targeted therapies enabling Precision Medicine. Hence, we aimed to emphasize the current state of targeted therapies’ screening in PCa, unveiling their potential clinical use.
Potential Targets for PCa Management
Because AR-dependent mechanisms are associated with 70% of ADT-resistant PCa cases (2), targeting the AR itself, its splicing variants or the associated co-regulators might have substantial therapeutic impact in CRPC. In the past few years, drug targeting of AR mutants, variants, and co-regulators has been shown to have anti-tumoral effects in AR-positive CRPC cells (Table 1). Galeterone, a CYP17A1 inhibitor, causes AR T878A mutant degradation and blocks transcription of AR target genes (70), whereas niclosamide induces AR-V7 protein degradation (75). This new AR target approach is under evaluation in clinical trials enrolling PCa patients (Supplementary Table 1). Nevertheless, for most of the described drugs, the anti-neoplastic effect was based on AR N-terminal blocking or AR splicing inhibition, ultimately impairing AR-driven PCa cell proliferation (Table 1 and Supplementary Table 1). Additionally, indirect AR inhibition might be achieved by diminishing the activity of the positive co-regulators of the receptor transcription activity, such as GATA2 and ONECUT2 (Table 1), whose inhibition was reported to not only reduce cell proliferation (Supplementary Table 1), but also synergize with ADT agents (80, 81, 83) and docetaxel (82), displaying enhanced efficacy.
Conversely, 30% of the advanced and metastatic PCa cases progress due to AR bypass mechanisms, which allow tumor cells to survive in an AR-independent manner (2). As previously described, a significant proportion of the bypass is based on RTK intracellular signaling activation, that constitutes a putative therapy target in resistant PCa (2). Many of the existent pre-clinical studies target the HerbB family, PI3K, mTOR, Akt, GR, RORγ, IGFR, MAPK, Src and STAT3 (Table 1 and Supplementary Table 1). Generally, drug treatment inhibited the specific target activity, reduced tumor cell proliferation and viability, and promoted apoptosis (Supplementary Table 1), displaying anti-tumoral effects both in vitro and in vivo.
Nonetheless, as reported for the drugs that target AR co-regulators, the most promising results were achieved when combining a targeted therapy with the standard therapy strategies. For example, the Akt inhibitor ipatasertib, under test in clinical studies in PCa (Supplementary Table 1), re-sensitized CRPC cells to antiandrogens, when combined with enzalutamide, inducing apoptosis, and leading to remarkable tumor cell growth inhibition, both in vitro and in vivo (114). Gefitinib (89), BEZ235 (110), RAD001 (124) or RU486 (131) were also reported to re-sensitize resistant cells to standard chemotherapy agents (Supplementary Table 1). Interestingly, CUDC-907 (101), CB-03-10 (130) and MP470 (135), in addition to selective RTK inhibition, were found to inhibit HDAC6, AR and EGFR, respectively. These drugs caused cytotoxic effects in resistant cells (Supplementary Table 1), indicating a possible benefit of targeting multiple pathways for management of resistant PCa.
Although most of the drugs listed in Table 1 have demonstrated good therapeutic potential, in some cases a possible resistant mechanism was also identified. The PI3K inhibitor CUDC-907 resulted in increased phospo-ERK levels (101), whereas PD325901, by inhibiting the ERK pathway, induced hyperactivation of the pro-proliferative PI3K and hedgehog pathways (139). In both studies, compensatory signaling mechanisms were suggested as the cause of resistance, thus, reinforcing the benefit of combinatory strategies to enhance anti-tumoral effects.
Furthermore, the combination of standard radiation therapy and PARP inhibitors was shown to have a significant effect on tumor cells viability (Table 1 and Supplementary Table 1). Specifically, veliparib (163) and rucaparib (167), two drugs under clinical investigation in PCa (Supplementary Table 1), were shown to re-sensitize CRPC cells to radiotherapy, impairing tumor cell growth. Moreover, this class of inhibitors similarly synergized with ADT agents (159, 160), AUKRA inhibitors (161) and epi-drugs (164, 165), with improved anti-neoplastic effects.
Targeting Epigenetics for PCa Treatment
Epigenetic alterations have been recognized as a hallmark of cancer (169), and since it comprises reversible modifications (59), there is a potential for drug targeting. FDA has approved two drugs that target epigenetic players, 5-azacytidine and 5-aza-2′-deoxycytidine, for myelodysplastic syndrome treatment (170). These two drugs, as DNA methyltransferases (DNMTs) inhibitors, are incorporated into DNA, inhibiting DNMT activity and decreasing global methylation levels (171, 172). Nevertheless, there is a potential for targeting the entire epigenetic machinery in cancer treatment, as we have previously reported (173). In therapy resistant PCa, histone acetyltransferases (HATs), HDACs, histone demethylases (HDMs), histone methyltransferases (HMTs), BET, and DNMTs inhibitors are currently under pre-clinical and clinical studies (Table 2 and Supplementary Table 2), displaying anti-tumoral effects, mostly due to enzyme inhibition and gene expression reprograming (Supplementary Table 2). Interestingly, the most promising results were found when epi-drugs were combined with standard ADT compounds (188, 209, 224, 228, 229, 241, 243), docetaxel (175, 197, 210, 217, 234), radiation therapy (203) or other epi-drugs (196, 204, 235), suggesting an interplay between epigenetic and non-epigenetic targeting in PCa management.
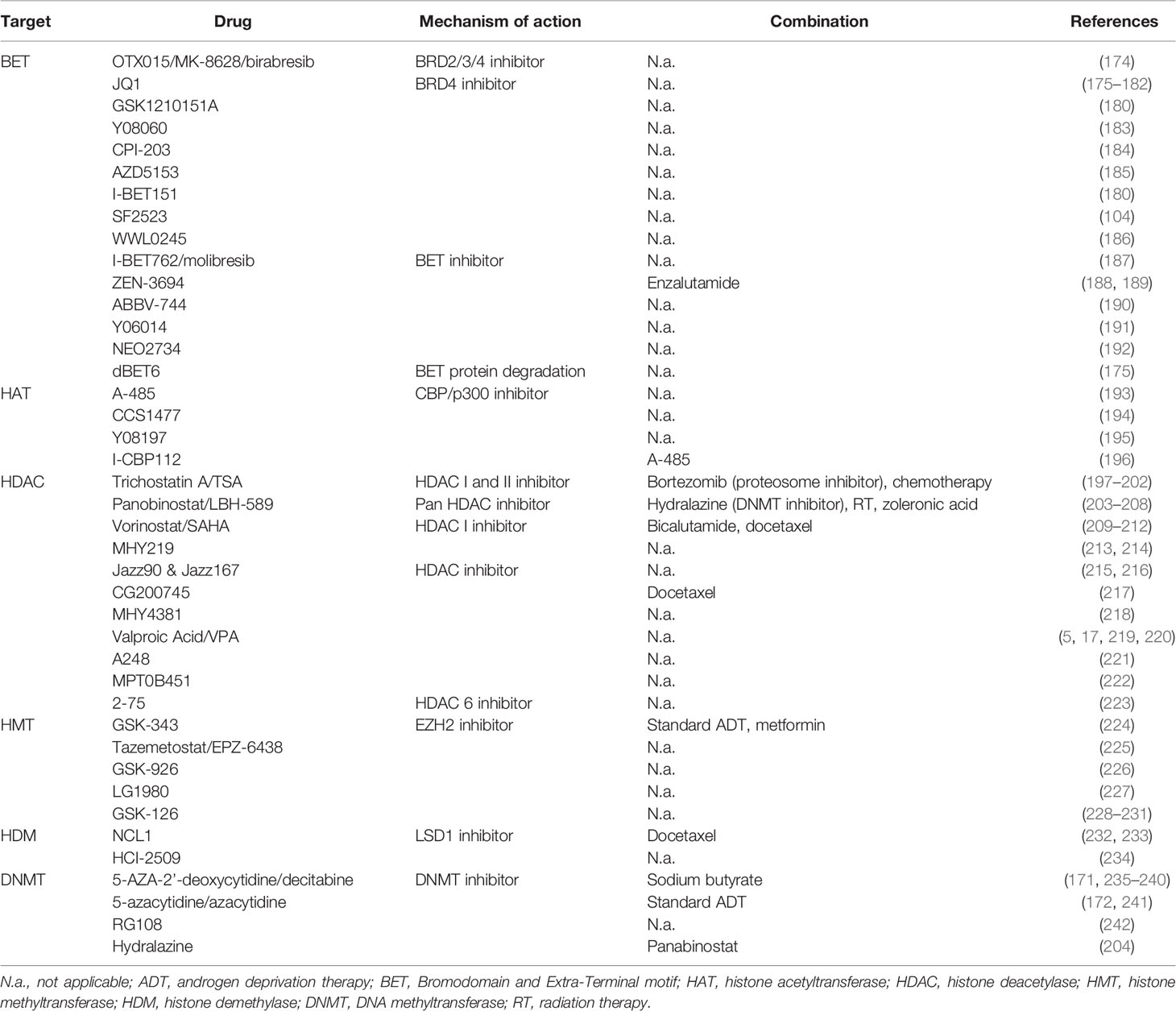
Table 2 Potential epigenetic targets and epi-drugs for the management of therapy resistant prostate cancer.
Remarkably, BET inhibitors such as JQ1, GSK1210151A and I-BET151 were found to decrease AR-fl and AR-V7 expression and activity (Supplementary Table 2), demonstrating a potential to be used with both an epigenetic- and AR-targeting purpose. However, disadvantageous off-target effects were observed after JQ1 treatment. JQ1 was found to promote PCa cell invasion and metastatic potential due to FOXA1 inactivation in a BET-independent manner (176). Therefore, high FOXA1 expression tumors are not suitable for JQ1 treatment (176), highlighting the importance of personalized strategies, based on tumor cell biology, for PCa management.
Notwithstanding all the work that has been accomplished in the epi-drug field, the role of different epigenetic enzymes in cancer, particularly PCa, and its potential targeting for a reprograming purpose remains largely unknow. Therefore, an investment in this field of research might contribute to improve the management of, not only therapy resistant PCa, but also other cancers displaying therapeutically relevant epigenetic modifications.
Immunotherapy-Based Therapies for PCa Management
PCa has long been described as a “cold” tumor, characterized by an immune-suppressive environment (244). However, in the last decade, several efforts have been made to overcome this feature. This includes the use of different immune therapies, alone or in combination with the standard of care (Table 3 and Supplementary Table 3). Published data includes reports from clinical trials targeting PD-L1, PD-1, CTLA-4, and the approved cellular immunotherapy Sipuleucel-T (Table 3). Overall, immunotherapy did not significantly improve the survival of PCa patients, but the effect on PSA was promising (Supplementary Table 3). Interestingly, the most encouraging results were obtained by the combination of pembrolizumab (anti-PD-1 drug), with the ADT enzalutamide (246). These results highlight the need for pre-clinical in vitro studies aiming at understanding the molecular mechanisms behind the “cold” PCa microenvironment, paving the way to further studies of novel immune-based therapies.
Conclusion
Herein, we described the mechanisms underlying the acquisition of therapy resistance in PCa, and potential targetable molecules, listing druggable targets in resistant disease and addressing pre-clinical studies describing the anti-tumoral effects of several drugs. We provided insight on innovative PCa treatments, to be exploited in pre-clinical studies and, if successful, in clinical trials, allowing for improved treatment of CRPC patients. Although several targeted therapies are already under clinical trials in PCa, there is a need for a more personalized analysis of tumor cell biology, enabling the selection of the most suitable therapeutic strategy, improving the management of resistant disease.
Author Contributions
Conceptualization, FM-S and CJ. Systematic review of literature, FM-S. Writing—original draft preparation, FM-S. Writing—review and editing, RH and CJ. Image editing, FM-S. Supervision, CJ. All authors have read and agreed to the published version of the manuscript.
Funding
CJ research is supported by the Research Center of Portuguese Oncology Institute of Porto (CI-IPOP-27-2016). FM-S contract was funded by Porto Comprehensive Cancer Center (Porto.CCC, Contract RNCCCP.CCC-CI-IPOP-LAB3-NORTE-01-0145-FEDER-072678).
Conflict of Interest
The authors declare that the research was conducted in the absence of any commercial or financial relationships that could be construed as a potential conflict of interest.
Publisher’s Note
All claims expressed in this article are solely those of the authors and do not necessarily represent those of their affiliated organizations, or those of the publisher, the editors and the reviewers. Any product that may be evaluated in this article, or claim that may be made by its manufacturer, is not guaranteed or endorsed by the publisher.
Supplementary Material
The Supplementary Material for this article can be found online at: https://www.frontiersin.org/articles/10.3389/fonc.2022.877379/full#supplementary-material
Abbreviations
AR, androgen receptor; AR-fl, androgen receptor full length; AR-V, androgen receptor variant; ADT, androgen-deprivation therapy; ARE, androgen-responsive elements; BET, bromodomain and extra-terminal motif; CAFs, cancer-associated fibroblasts; CRPC, castration resistant prostate cancer; DHT, dihydrotestosterone; DNMTs, DNA methyltransferases; EGF, epidermal growth factor; EMT, epithelial mesenchymal transition; EBRT, external beam radiotherapy; FGF, fibroblast growth factor; FDA, Food and Drug Administration; GR, glucocorticoid receptor; GF, growth factors; HATs, histone acetyltransferases; HDACs, histone deacetylases; HDMs, histone demethylases; HMTs, histone methyltransferases; IGF-1, insulin-like growth-factor-1; KGF, keratinocyte growth factor; LBD, ligand-binding domain; LHRH, luteinizing hormone-releasing hormone; MDSCs, myeloid-derived suppressor cells; NEPC, neuroendocrine prostate cancer; N.a., not applicable; PCa, Prostate cancer; PSA, prostate-specific antigen; RT, radiation therapy; RP, radical prostatectomy; RTK, receptor tyrosine kinase; T, testosterone; TF, transcript factors; TME, tumor microenvironment.
References
1. Sung H, Ferlay J, Siegel RL, Laversanne M, Soerjomataram I, Jemal A, et al. Global Cancer Statistics 2020: GLOBOCAN Estimates of Incidence and Mortality Worldwide for 36 Cancers in 185 Countries. CA Cancer J Clin (2021) 71(3):209–49. doi: 10.3322/caac.21660
2. Wang G, Zhao D, Spring DJ, DePinho RA. Genetics and Biology of Prostate Cancer. Genes Dev (2018) 32(17-18):1105–40. doi: 10.1101/gad.315739.118
3. Cancer Genome Atlas Research N. The Molecular Taxonomy of Primary Prostate Cancer. Cell (2015) 163(4):1011–25. doi: 10.1016/j.cell.2015.10.025
4. Mitchell T, Neal DE. The Genomic Evolution of Human Prostate Cancer. Br J Cancer (2015) 113(2):193–8. doi: 10.1038/bjc.2015.234
5. Taylor BS, Schultz N, Hieronymus H, Gopalan A, Xiao Y, Carver BS, et al. Integrative Genomic Profiling of Human Prostate Cancer. Cancer Cell (2010) 18(1):11–22. doi: 10.1016/j.ccr.2010.05.026
6. Carver BS, Tran J, Gopalan A, Chen Z, Shaikh S, Carracedo A, et al. Aberrant ERG Expression Cooperates With Loss of PTEN to Promote Cancer Progression in the Prostate. Nat Genet (2009) 41(5):619–24. doi: 10.1038/ng.370
7. Graca I, Pereira-Silva E, Henrique R, Packham G, Crabb SJ, Jeronimo C, et al. Epigenetic Modulators as Therapeutic Targets in Prostate Cancer. Clin Epigenet (2016) 8(1):98. doi: 10.1186/s13148-016-0264-8
8. Jerónimo C, Usadel H, Henrique R, Oliveira J, Lopes C, Nelson WG, et al. Quantitation of GSTP1 Methylation in non-Neoplastic Prostatic Tissue and Organ-Confined Prostate Adenocarcinoma. J Natl Cancer Institute (2001) 93(22):1747–52. doi: 10.1093/jnci/93.22.1747
9. Weichert W, Roske A, Gekeler V, Beckers T, Stephan C, Jung K, et al. Histone Deacetylases 1, 2 and 3 are Highly Expressed in Prostate Cancer and HDAC2 Expression is Associated With Shorter PSA Relapse Time After Radical Prostatectomy. Br J Cancer (2008) 98(3):604–10. doi: 10.1038/sj.bjc.6604199
10. Jerónimo C, Henrique R, Hoque MO, Mambo E, Ribeiro FR, Varzim G, et al. A Quantitative Promoter Methylation Profile of Prostate Cancer. Clin Cancer Res: Off J Am Assoc Cancer Res (2004) 10(24):8472–8. doi: 10.1158/1078-0432.CCR-04-0894
11. Yegnasubramanian S, Haffner MC, Zhang Y, Gurel B, Cornish TC, Wu Z, et al. DNA Hypomethylation Arises Later in Prostate Cancer Progression Than CpG Island Hypermethylation and Contributes to Metastatic Tumor Heterogeneity. Cancer Res (2008) 68(21):8954–67. doi: 10.1158/0008-5472.CAN-07-6088
12. Davies A, Conteduca V, Zoubeidi A, Beltran H. Biological Evolution of Castration-Resistant Prostate Cancer. Eur Urol Focus (2019) 5(2):147–54. doi: 10.1016/j.euf.2019.01.016
13. Grasso CS, Wu Y-M, Robinson DR, Cao X, Dhanasekaran SM, Khan AP, et al. The Mutational Landscape of Lethal Castration-Resistant Prostate Cancer. Nature (2012) 487(7406):239–43. doi: 10.1038/nature11125
14. Chan JSK, Sng MK, Teo ZQ, Chong HC, Twang JS, Tan NS. Targeting Nuclear Receptors in Cancer-Associated Fibroblasts as Concurrent Therapy to Inhibit Development of Chemoresistant Tumors. Oncogene (2018) 37(2):160–73. doi: 10.1038/onc.2017.319
15. Friedlander TW, Roy R, Tomlins SA, Ngo VT, Kobayashi Y, Azameera A, et al. Common Structural and Epigenetic Changes in the Genome of Castration-Resistant Prostate Cancer. Cancer Res (2012) 72(3):616–25. doi: 10.1158/0008-5472.CAN-11-2079
16. Varambally S, Dhanasekaran SM, Zhou M, Barrette TR, Kumar-Sinha C, Sanda MG, et al. The Polycomb Group Protein EZH2 is Involved in Progression of Prostate Cancer. Nature (2002) 419(6907):624–9. doi: 10.1038/nature01075
17. Casciello F, Windloch K, Gannon F, Lee JS. Functional Role of G9a Histone Methyltransferase in Cancer. Front Immunol (2015) 6(487). doi: 10.3389/fimmu.2015.00487
18. Metzger E, Wissmann M, Yin N, Muller JM, Schneider R, Peters AH, et al. LSD1 Demethylates Repressive Histone Marks to Promote Androgen-Receptor-Dependent Transcription. Nature (2005) 437(7057):436–9. doi: 10.1038/nature04020
19. Conteduca V, Oromendia C, Eng KW, Bareja R, Sigouros M, Molina A, et al. Clinical Features of Neuroendocrine Prostate Cancer. Eur J Cancer (2019) 121:7–18. doi: 10.1016/j.ejca.2019.08.011
20. Beltran H, Prandi D, Mosquera JM, Benelli M, Puca L, Cyrta J, et al. Divergent Clonal Evolution of Castration-Resistant Neuroendocrine Prostate Cancer. Nat Med (2016) 22(3):298–305. doi: 10.1038/nm.4045
21. Vlachostergios PJ, Puca L, Beltran H. Emerging Variants of Castration-Resistant Prostate Cancer. Curr Oncol Rep (2017) 19(5):32–2. doi: 10.1007/s11912-017-0593-6
22. Nadal R, Schweizer M, Kryvenko ON, Epstein JI, Eisenberger MA. Small Cell Carcinoma of the Prostate. Nat Rev Urol (2014) 11(4):213–9. doi: 10.1038/nrurol.2014.21
23. Gillessen S, Attard G, Beer TM, Beltran H, Bossi A, Bristow R, et al. Management of Patients With Advanced Prostate Cancer: The Report of the Advanced Prostate Cancer Consensus Conference APCCC 2017. Eur Urol (2018) 73(2):178–211. doi: 10.1016/j.eururo.2017.06.002
24. Heidenreich A, Bastian PJ, Bellmunt J, Bolla M, Joniau S, van derKwast T, et al. EAU Guidelines on Prostate Cancer. Part II: Treatment of Advanced, Relapsing, and Castration-Resistant Prostate Cancer. Eur Urol (2014) 65(2):467–79. doi: 10.1016/j.eururo.2013.11.002
25. Parker C, Castro E, Fizazi K, Heidenreich A, Ost P, Procopio G, et al. Prostate Cancer: ESMO Clinical Practice Guidelines for Diagnosis, Treatment and Follow-Up. Ann Oncol (2020) 31(9):1119–34. doi: 10.1016/j.annonc.2020.06.011
26. Cornford P, Bellmunt J, Bolla M, Briers E, De Santis M, Gross T, et al. EAU-ESTRO-SIOG Guidelines on Prostate Cancer. Part II: Treatment of Relapsing, Metastatic, and Castration-Resistant Prostate Cancer. Eur Urol (2017) 71(4):630–42. doi: 10.1016/j.eururo.2016.08.002
27. Ceder Y, Bjartell A, Culig Z, Rubin MA, Tomlins S, Visakorpi T. The Molecular Evolution of Castration-Resistant Prostate Cancer. Eur Urol Focus (2016) 2(5):506–13. doi: 10.1016/j.euf.2016.11.012
28. Parker C, Gillessen S, Heidenreich A, Horwich A. Cancer of the Prostate: ESMO Clinical Practice Guidelines for Diagnosis, Treatment and Follow-Up. Ann Oncol (2015) 26 Suppl 5:v69–77. doi: 10.1093/annonc/mdv222
29. Shore ND, Chowdhury S, Villers A, Klotz L, Siemens DR, Phung D, et al. Efficacy and Safety of Enzalutamide Versus Bicalutamide for Patients With Metastatic Prostate Cancer (TERRAIN): A Randomised, Double-Blind, Phase 2 Study. Lancet Oncol (2016) 17(2):153–63. doi: 10.1016/S1470-2045(15)00518-5
30. Obinata D, Takayama K, Takahashi S, Inoue S. Crosstalk of the Androgen Receptor With Transcriptional Collaborators: Potential Therapeutic Targets for Castration-Resistant Prostate Cancer. Cancers (2017) 9(3):22. doi: 10.3390/cancers9030022
31. Vellky JE, Ricke WA. Development and Prevalence of Castration-Resistant Prostate Cancer Subtypes. Neoplasia (2020) 22(11):566–75. doi: 10.1016/j.neo.2020.09.002
32. Venkitaraman R, Lorente D, Murthy V, Thomas K, Parker L, Ahiabor R, et al. A Randomised Phase 2 Trial of Dexamethasone Versus Prednisolone in Castration-Resistant Prostate Cancer. Eur Urol (2015) 67(4):673–9. doi: 10.1016/j.eururo.2014.10.004
33. Sartor O, de Bono J, Chi KN, Fizazi K, Herrmann K, Rahbar K, et al. Lutetium-177–PSMA-617 for Metastatic Castration-Resistant Prostate Cancer. New Engl J Med (2021) 385(12):1091–103. doi: 10.1056/NEJMoa2107322
34. Lowrance WT, Murad MH, Oh WK, Jarrard DF, Resnick MJ, Cookson MS. Castration-Resistant Prostate Cancer: AUA Guideline Amendment 2018. J Urol (2018) 200(6):1264–72. doi: 10.1016/j.juro.2018.07.090
35. Graca I, Sousa EJ, Costa-Pinheiro P, Vieira FQ, Torres-Ferreira J, Martins MG, et al. Anti-Neoplastic Properties of Hydralazine in Prostate Cancer. Oncotarget (2014) 5(15):5950–64. doi: 10.18632/oncotarget.1909
36. Visakorpi T, Hyytinen E, Koivisto P, Tanner M, Keinänen R, Palmberg C, et al. In Vivo Amplification of the Androgen Receptor Gene and Progression of Human Prostate Cancer. Nat Genet (1995) 9(4):401–6. doi: 10.1038/ng0495-401
37. Culig Z, Hobisch A, Cronauer MV, Radmayr C, Trapman J, Hittmair A, et al. Androgen Receptor Activation in Prostatic Tumor Cell Lines by Insulin-Like Growth Factor-I, Keratinocyte Growth Factor, and Epidermal Growth Factor. Cancer Res (1994) 54(20):5474–8. doi: 10.1159/000475232
38. Kaarbø M, Klokk TI, Saatcioglu F. Androgen Signaling and its Interactions With Other Signaling Pathways in Prostate Cancer. BioEssays (2007) 29(12):1227–38. doi: 10.1002/bies.20676
39. Marcelli M, Ittmann M, Mariani S, Sutherland R, Nigam R, Murthy L, et al. Androgen Receptor Mutations in Prostate Cancer. Cancer Res (2000) 60(4):944–9.
40. Bluemn EG, Coleman IM, Lucas JM, Coleman RT, Hernandez-Lopez S, Tharakan R, et al. Androgen Receptor Pathway-Independent Prostate Cancer Is Sustained Through FGF Signaling. Cancer Cell (2017) 32(4):474–89.e6. doi: 10.1016/j.ccell.2017.09.003
41. Craft N, Shostak Y, Carey M, Sawyers CL. A Mechanism for Hormone-Independent Prostate Cancer Through Modulation of Androgen Receptor Signaling by the HER-2/Neu Tyrosine Kinase. Nat Med (1999) 5(3):280–5. doi: 10.1038/6495
42. Wang J, Kobayashi T, Floc'h N, Kinkade CW, Aytes A, Dankort D, et al. B-Raf Activation Cooperates With PTEN Loss to Drive C-Myc Expression in Advanced Prostate Cancer. Cancer Res (2012) 72(18):4765–76. doi: 10.1158/0008-5472.CAN-12-0820
43. Guo Z, Dai B, Jiang T, Xu K, Xie Y, Kim O, et al. Regulation of Androgen Receptor Activity by Tyrosine Phosphorylation. Cancer Cell (2007) 11(1):97. doi: 10.1016/j.ccr.2006.12.010
44. Wang J, Zou JX, Xue X, Cai D, Zhang Y, Duan Z, et al. ROR-γ Drives Androgen Receptor Expression and Represents a Therapeutic Target in Castration-Resistant Prostate Cancer. Nat Med (2016) 22(5):488–96. doi: 10.1038/nm.4070
45. Isikbay M, Otto K, Kregel S, Kach J, Cai Y, Vander Griend DJ, et al. Glucocorticoid Receptor Activity Contributes to Resistance to Androgen-Targeted Therapy in Prostate Cancer. Horm Cancer (2014) 5(2):72–89. doi: 10.1007/s12672-014-0173-2
46. Yeh S, Lin HK, Kang HY, Thin TH, Lin MF, Chang C. From HER2/Neu Signal Cascade to Androgen Receptor and its Coactivators: A Novel Pathway by Induction of Androgen Target Genes Through MAP Kinase in Prostate Cancer Cells. Proc Natl Acad Sci U S A (1999) 96(10):5458–63. doi: 10.1073/pnas.96.10.5458
47. Harada S, Keller ET, Fujimoto N, Koshida K, Namiki M, Matsumoto T, et al. Long-Term Exposure of Tumor Necrosis Factor α Causes Hypersensitivity to Androgen and Anti-Androgen Withdrawal Phenomenon in LNCaP Prostate Cancer Cells. Prostate (2001) 46(4):319–26. doi: 10.1002/1097-0045(20010301)46:4<319::AID-PROS1039>3.0.CO;2-C
48. Lee SO, Lou W, Hou M, de Miguel F, Gerber L, Gao AC. Interleukin-6 Promotes Androgen-Independent Growth in LNCaP Human Prostate Cancer Cells. Clin Cancer Res Off J Am Assoc Cancer Res (2003) 9(1):370–6. doi: 10.1016/j.mce.2011.05.033
49. Calcinotto A, Spataro C, Zagato E, Di Mitri D, Gil V, Crespo M, et al. IL-23 Secreted by Myeloid Cells Drives Castration-Resistant Prostate Cancer. Nature (2018) 559(7714):363–9. doi: 10.1038/s41586-018-0266-0
50. Feldman BJ, Feldman D. The Development of Androgen-Independent Prostate Cancer. Nat Rev Cancer (2001) 1(1):34–45. doi: 10.1038/35094009
51. Mayeur GL, Kung WJ, Martinez A, Izumiya C, Chen DJ, Kung HJ. Ku is a Novel Transcriptional Recycling Coactivator of the Androgen Receptor in Prostate Cancer Cells. J Biol Chem (2005) 280(11):10827–33. doi: 10.1074/jbc.M413336200
52. Haffner MC, Aryee MJ, Toubaji A, Esopi DM, Albadine R, Gurel B, et al. Androgen-Induced TOP2B-Mediated Double-Strand Breaks and Prostate Cancer Gene Rearrangements. Nat Genet (2010) 42(8):668–75. doi: 10.1038/ng.613
53. Lin C, Yang L, Tanasa B, Hutt K, Ju BG, Ohgi K, et al. Nuclear Receptor-Induced Chromosomal Proximity and DNA Breaks Underlie Specific Translocations in Cancer. Cell (2009) 139(6):1069–83. doi: 10.1016/j.cell.2009.11.030
54. Brenner JC, Ateeq B, Li Y, Yocum AK, Cao Q, Asangani IA, et al. Mechanistic Rationale for Inhibition of Poly(ADP-Ribose) Polymerase in ETS Gene Fusion-Positive Prostate Cancer. Cancer Cell (2011) 19(5):664–78. doi: 10.1016/j.ccr.2011.04.010
55. Wu D, Sunkel B, Chen Z, Liu X, Ye Z, Li Q, et al. Three-Tiered Role of the Pioneer Factor GATA2 in Promoting Androgen-Dependent Gene Expression in Prostate Cancer. Nucleic Acids Res (2014) 42(6):3607–22. doi: 10.1093/nar/gkt1382
56. Tepper CG, Boucher DL, Ryan PE, Ma A-H, Xia L, Lee L-F, et al. Characterization of a Novel Androgen Receptor Mutation in a Relapsed CWR22 Prostate Cancer Xenograft and Cell Line. Cancer Res (2002) 62(22):6606–14.
57. Hu R, Lu C, Mostaghel EA, Yegnasubramanian S, Gurel M, Tannahill C, et al. Distinct Transcriptional Programs Mediated by the Ligand-Dependent Full-Length Androgen Receptor and its Splice Variants in Castration-Resistant Prostate Cancer. Cancer Res (2012) 72(14):3457–62. doi: 10.1158/0008-5472.CAN-11-3892
58. Jarrard DF, Kinoshita H, Shi Y, Sandefur C, Hoff D, Meisner LF, et al. Methylation of the Androgen Receptor Promoter CpG Island is Associated With Loss of Androgen Receptor Expression in Prostate Cancer Cells. Cancer Res (1998) 58(23):5310–4.
59. Jeronimo C, Bastian PJ, Bjartell A, Carbone GM, Catto JW, Clark SJ, et al. Epigenetics in Prostate Cancer: Biologic and Clinical Relevance. Eur Urol (2011) 60(4):753–66. doi: 10.1016/j.eururo.2011.06.035
60. Kivinummi K, Urbanucci A, Leinonen K, Tammela TLJ, Annala M, Isaacs WB, et al. The Expression of AURKA is Androgen Regulated in Castration-Resistant Prostate Cancer. Sci Rep (2017) 7(1):17978. doi: 10.1038/s41598-017-18210-3
61. Madan RA, Pal SK, Sartor O, Dahut WL. Overcoming Chemotherapy Resistance in Prostate Cancer. Clin Cancer Res Off J Am Assoc Cancer Res (2011) 17(12):3892–902. doi: 10.1158/1078-0432.CCR-10-2654
62. Kartner N, Riordan JR, Ling V. Cell Surface P-Glycoprotein Associated With Multidrug Resistance in Mammalian Cell Lines. Science (1983) 221(4617):1285–8. doi: 10.1126/science.6137059
63. Terry S, Ploussard G, Allory Y, Nicolaiew N, Boissière-Michot F, Maillé P, et al. Increased Expression of Class III Beta-Tubulin in Castration-Resistant Human Prostate Cancer. Br J Cancer (2009) 101(6):951–6. doi: 10.1038/sj.bjc.6605245
64. Ploussard G, Terry S, Maillé P, Allory Y, Sirab N, Kheuang L, et al. Class III Beta-Tubulin Expression Predicts Prostate Tumor Aggressiveness and Patient Response to Docetaxel-Based Chemotherapy. Cancer Res (2010) 70(22):9253–64. doi: 10.1158/0008-5472.CAN-10-1447
65. Stuparu AD, Capri JR, Meyer CAL, Le TM, Evans-Axelsson SL, Current K, et al. Mechanisms of Resistance to Prostate-Specific Membrane Antigen-Targeted Radioligand Therapy in a Mouse Model of Prostate Cancer. J Nucl Med (2021) 62(7):989–95. doi: 10.2967/jnumed.120.256263
66. Cao J, Chen Y, Hu M, Zhang W. (177)Lu-PSMA-RLT of Metastatic Castration-Resistant Prostate Cancer: Limitations and Improvements. Ann Nucl Med (2021) 35(8):861–70. doi: 10.1007/s12149-021-01649-w
67. Toivanen R, Mohan A, Shen MM. Basal Progenitors Contribute to Repair of the Prostate Epithelium Following Induced Luminal Anoikis. Stem Cell Rep (2016) 6(5):660–7. doi: 10.1016/j.stemcr.2016.03.007
68. Zhu ML, Kyprianou N. Role of Androgens and the Androgen Receptor in Epithelial-Mesenchymal Transition and Invasion of Prostate Cancer Cells. FASEB J (2010) 24(3):769–77. doi: 10.1096/fj.09-136994
69. Ting HJ, Deep G, Jain AK, Cimic A, Sirintrapun J, Romero LM, et al. Silibinin Prevents Prostate Cancer Cell-Mediated Differentiation of Naïve Fibroblasts Into Cancer-Associated Fibroblast Phenotype by Targeting TGF β2. Mol Carcinog (2015) 54(9):730–41. doi: 10.1002/mc.22135
70. Yu Z, Cai C, Gao S, Simon NI, Shen HC, Balk SP. Galeterone Prevents Androgen Receptor Binding to Chromatin and Enhances Degradation of Mutant Androgen Receptor. Clin Cancer Res Off J Am Assoc Cancer Res (2014) 20(15):4075–85. doi: 10.1158/1078-0432.CCR-14-0292
71. Yang YC, Banuelos CA, Mawji NR, Wang J, Kato M, Haile S, et al. Targeting Androgen Receptor Activation Function-1 With EPI to Overcome Resistance Mechanisms in Castration-Resistant Prostate Cancer. Clin Cancer Res (2016) 22(17):4466–77. doi: 10.1158/1078-0432.CCR-15-2901
72. Kato M, Banuelos CA, Imamura Y, Leung JK, Caley DP, Wang J, et al. Cotargeting Androgen Receptor Splice Variants and mTOR Signaling Pathway for the Treatment of Castration-Resistant Prostate Cancer. Clin Cancer Res Off J Am Assoc Cancer Res (2016) 22(11):2744–54. doi: 10.1158/1078-0432.CCR-15-2119
73. Myung J-K, Banuelos CA, Fernandez JG, Mawji NR, Wang J, Tien AH, et al. An Androgen Receptor N-Terminal Domain Antagonist for Treating Prostate Cancer. J Clin Invest (2013) 123(7):2948–60. doi: 10.1172/JCI66398
74. Andersen RJ, Mawji NR, Wang J, Wang G, Haile S, Myung JK, et al. Regression of Castrate-Recurrent Prostate Cancer by a Small-Molecule Inhibitor of the Amino-Terminus Domain of the Androgen Receptor. Cancer Cell (2010) 17(6):535–46. doi: 10.1016/j.ccr.2010.04.027
75. Liu X, Biswas S, Berg MG, Antapli CM, Xie F, Wang Q, et al. Niclosamide Inhibits Androgen Receptor Variants Expression and Overcomes Enzalutamide Resistance in Castration-Resistant Prostate Cancer. Clin Cancer Res Off J Am Assoc Cancer Res (2014) 20(12):3198–210. doi: 10.1158/1078-0432.CCR-13-3296
76. Liu X, Biswas S, Berg MG, Antapli CM, Xie F, Wang Q, et al. Genomics-Guided Discovery of Thailanstatins A, B, and C As pre-mRNA Splicing Inhibitors and Antiproliferative Agents From Burkholderia Thailandensis MSMB43. J Natural Prod (2013) 76(4):685–93. doi: 10.1021/np300913h
77. Wang B, Lo UG, Wu K, Kapur P, Liu X, Huang J, et al. Developing New Targeting Strategy for Androgen Receptor Variants in Castration Resistant Prostate Cancer. Int J Cancer (2017) 141(10):2121–30. doi: 10.1002/ijc.30893
78. Ravindranathan P, Lee TK, Yang L, Centenera MM, Butler L, Tilley WD, et al. Peptidomimetic Targeting of Critical Androgen Receptor-Coregulator Interactions in Prostate Cancer. Nat Commun (2013) 4:1923. doi: 10.1038/ncomms2912
79. Lev A, Lulla AR, Ross BC, Ralff MD, Makhov PB, Dicker DT, et al. ONC201 Targets AR and AR-V7 Signaling, Reduces PSA, and Synergizes With Everolimus in Prostate Cancer. Mol Cancer Res (2018) 16(5):754–66. doi: 10.1158/1541-7786.MCR-17-0614
80. Du Z, Li L, Sun W, Wang X, Zhang Y, Chen Z, et al. Systematic Evaluation for the Influences of the SOX17/Notch Receptor Family Members on Reversing Enzalutamide Resistance in Castration-Resistant Prostate Cancer Cells. Front Oncol (2021) 11:607291. doi: 10.3389/fonc.2021.607291
81. Du Z, Li L, Sun W, Zhu P, Cheng S, Yang X, et al. HepaCAM Inhibits the Malignant Behavior of Castration-Resistant Prostate Cancer Cells by Downregulating Notch Signaling and PF-3084014 (a γ-Secretase Inhibitor) Partly Reverses the Resistance of Refractory Prostate Cancer to Docetaxel and Enzalutamide In Vitro. Int J Oncol (2018) 53(1):99–112. doi: 10.3892/ijo.2018.4370
82. Cui D, Dai J, Keller JM, Mizokami A, Xia S, Keller ET, et al. Notch Pathway Inhibition Using PF-03084014, a γ-Secretase Inhibitor (GSI), Enhances the Antitumor Effect of Docetaxel in Prostate Cancer. Clin Cancer Res (2015) 21(20):4619. doi: 10.1158/1078-0432.CCR-15-0242
83. Rice MA, Hsu EC, Aslan M, Ghoochani A, Su A, Stoyanova T, et al. Loss of Notch1 Activity Inhibits Prostate Cancer Growth and Metastasis and Sensitizes Prostate Cancer Cells to Antiandrogen Therapies. Mol Cancer Ther (2019) 18(7):1230–42. doi: 10.1158/1535-7163.MCT-18-0804
84. Cui J, Wang Y, Dong B, Qin L, Wang C, Zhou P, et al. Pharmacological Inhibition of the Notch Pathway Enhances the Efficacy of Androgen Deprivation Therapy for Prostate Cancer. Int J Cancer (2018) 143(3):645–56. doi: 10.1002/ijc.31346
85. Rotinen M, You S, Yang J, Coetzee SG, Reis-Sobreiro M, Huang WC, et al. ONECUT2 is a Targetable Master Regulator of Lethal Prostate Cancer That Suppresses the Androgen Axis. Nat Med (2018) 24(12):1887–98. doi: 10.1038/s41591-018-0241-1
86. Mellinghoff IK, Tran C, Sawyers CL. Growth Inhibitory Effects of the Dual ErbB1/ErbB2 Tyrosine Kinase Inhibitor PKI-166 on Human Prostate Cancer Xenografts. Cancer Res (2002) 62(18):5254–9.
87. Kim SJ, Uehara H, Yazici S, Langley RR, He J, Tsan R, et al. Simultaneous Blockade of Platelet-Derived Growth Factor-Receptor and Epidermal Growth Factor-Receptor Signaling and Systemic Administration of Paclitaxel as Therapy for Human Prostate Cancer Metastasis in Bone of Nude Mice. Cancer Res (2004) 64(12):4201–8. doi: 10.1158/0008-5472.CAN-03-3763
88. Kim SJ, Uehara H, Karashima T, Shepherd DL, Killion JJ, Fidler IJ, et al. Blockade of Epidermal Growth Factor Receptor Signaling in Tumor Cells and Tumor-Associated Endothelial Cells for Therapy of Androgen-Independent Human Prostate Cancer Growing in the Bone of Nude Mice. Clin Cancer Res (2003) 9(3):1200–10. doi: 10.1016/S0002-9440(10)64253-8
89. Sirotnak FM, She Y, Lee F, Chen J, Scher HI. Studies With CWR22 Xenografts in Nude Mice Suggest That ZD1839 may Have a Role in the Treatment of Both Androgen-Dependent and Androgen-Independent Human Prostate Cancer. Clin Cancer Res (2002) 8(12):3870–6.
90. Moasser MM, Basso A, Averbuch SD, Rosen N. The Tyrosine Kinase Inhibitor ZD1839 ("Iressa") Inhibits HER2-Driven Signaling and Suppresses the Growth of HER2-Overexpressing Tumor Cells. Cancer Res (2001) 61(19):7184–8.
91. Formento P, Hannoun-Levi JM, Fischel JL, Magné N, Etienne-Grimaldi MC, Milano G. Dual HER 1-2 Targeting of Hormone-Refractory Prostate Cancer by ZD1839 and Trastuzumab. Eur J Cancer (2004) 40(18):2837–44. doi: 10.1016/j.ejca.2004.07.033
92. Sgambato A, Camerini A, Faraglia B, Ardito R, Bianchino G, Spada D, et al. Targeted Inhibition of the Epidermal Growth Factor Receptor-Tyrosine Kinase by ZD1839 ('Iressa') Induces Cell-Cycle Arrest and Inhibits Proliferation in Prostate Cancer Cells. J Cell Physiol (2004) 201(1):97–105. doi: 10.1002/jcp.20045
93. Festuccia C, Muzi P, Millimaggi D, Biordi L, Gravina GL, Speca S, et al. Molecular Aspects of Gefitinib Antiproliferative and Pro-Apoptotic Effects in PTEN-Positive and PTEN-Negative Prostate Cancer Cell Lines. Endocr Relat Cancer (2005) 12(4):983–98. doi: 10.1677/erc.1.00986
94. Bonaccorsi L, Marchiani S, Muratori M, Forti G, Baldi E. Gefitinib ('Iressa', ZD1839) Inhibits EGF-Induced Invasion in Prostate Cancer Cells by Suppressing PI3 K/AKT Activation. J Cancer Res Clin Oncol (2004) 130(10):604–14. doi: 10.1007/s00432-004-0581-8
95. Vicentini C, Festuccia C, Gravina GL, Angelucci A, Marronaro A, Bologna M. Prostate Cancer Cell Proliferation is Strongly Reduced by the Epidermal Growth Factor Receptor Tyrosine Kinase Inhibitor ZD1839 In Vitro on Human Cell Lines and Primary Cultures. J Cancer Res Clin Oncol (2003) 129(3):165–74. doi: 10.1007/s00432-003-0420-3
96. Angelucci A, Gravina GL, Rucci N, Millimaggi D, Festuccia C, Muzi P, et al. Suppression of EGF-R Signaling Reduces the Incidence of Prostate Cancer Metastasis in Nude Mice. Endocr Relat Cancer (2006) 13(1):197–210. doi: 10.1677/erc.1.01100
97. Goehringer N, Biersack B, Peng Y, Schobert R, Herling M, Ma A, et al. Anticancer Activity and Mechanisms of Action of New Chimeric EGFR/HDAC-Inhibitors. Int J Mol Sci (2021) 22(16) 22(16): 8432. doi: 10.3390/ijms22168432
98. Liao Y, Guo Z, Xia X, Liu Y, Huang C, Jiang L, et al. Inhibition of EGFR Signaling With Spautin-1 Represents a Novel Therapeutics for Prostate Cancer. J Exp Clin Cancer Res (2019) 38(1):157. doi: 10.1186/s13046-019-1165-4
99. Zhi Y, Wu X, Shen W, Wang Y, Zhou X, He P, et al. Synthesis and Pharmacological Evaluation of Novel Epidermal Growth Factor Receptor Inhibitors Against Prostate Tumor Cells. Oncol Lett (2018) 16(5):6522–30. doi: 10.3892/ol.2018.9438
100. Pu YS, Hsieh MW, Wang CW, Liu GY, Huang CY, Lin CC, et al. Epidermal Growth Factor Receptor Inhibitor (PD168393) Potentiates Cytotoxic Effects of Paclitaxel Against Androgen-Independent Prostate Cancer Cells. Biochem Pharmacol (2006) 71(6):751–60. doi: 10.1016/j.bcp.2005.12.009
101. Hu C, Xia H, Bai S, Zhao J, Edwards H, Li X, et al. CUDC-907, a Novel Dual PI3K and HDAC Inhibitor, in Prostate Cancer: Antitumour Activity and Molecular Mechanism of Action. J Cell Mol Med (2020) 24(13):7239–53. doi: 10.1111/jcmm.15281
102. Zou Y, Qi Z, Guo W, Zhang L, Ruscetti M, Shenoy T, et al. Cotargeting the Cell-Intrinsic and Microenvironment Pathways of Prostate Cancer by PI3Kα/β/δ Inhibitor Bay1082439. Mol Cancer Ther (2018) 17(10):2091–9. doi: 10.1158/1535-7163.MCT-18-0038
103. Qi Z, Xu Z, Zhang L, Zou Y, Li J, Yan W, et al. Overcoming Resistance to Immune Checkpoint Therapy in PTEN-Null Prostate Cancer by Intermittent Anti-PI3Kα/β/δ Treatment. Nat Commun (2022) 13(1):182. doi: 10.1038/s41467-021-27833-0
104. Shen G, Jiang M, Pu J. Dual Inhibition of BRD4 and PI3K by SF2523 Suppresses Human Prostate Cancer Cell Growth In Vitro and In Vivo. Biochem Biophys Res Commun (2018) 495(1):567–73. doi: 10.1016/j.bbrc.2017.11.062
105. Guerra FS, Rodrigues DA, Fraga CAM, Fernandes PD. Novel Single Inhibitor of HDAC6/8 and Dual Inhibitor of PI3K/HDAC6 as Potential Alternative Treatments for Prostate Cancer. Pharmaceut (Basel) (2021) 14(5):387. doi: 10.3390/ph14050387
106. Zhao W, Guo W, Zhou Q, Ma SN, Wang R, Qiu Y, et al. In Vitro Antimetastatic Effect of Phosphatidylinositol 3-Kinase Inhibitor ZSTK474 on Prostate Cancer PC3 Cells. Int J Mol Sci (2013) 14(7):13577–91. doi: 10.3390/ijms140713577
107. Liu J, Tan X, Zhao W, Liu J, Xing X, Fan G, et al. In Vitro and In Vivo Antimetastatic Effects of ZSTK474 on Prostate Cancer DU145 Cells. Curr Cancer Drug Targets (2019) 19(4):321–9. doi: 10.2174/1568009618666180911101310
108. Cai F, Zhang Y, Li J, Huang S, Gao R. Isorhamnetin Inhibited the Proliferation and Metastasis of Androgen-Independent Prostate Cancer Cells by Targeting the Mitochondrion-Dependent Intrinsic Apoptotic and PI3K/Akt/mTOR Pathway. Biosci Rep (2020) 40(3):BSR20192826. doi: 10.1042/BSR20192826
109. Huang TF, Wang SW, Lai YW, Liu SC, Chen YJ, Hsueh TY, et al. 4-Acetylantroquinonol B Suppresses Prostate Cancer Growth and Angiogenesis via a VEGF/PI3K/ERK/mTOR-Dependent Signaling Pathway in Subcutaneous Xenograft and In Vivo Angiogenesis Models. Int J Mol Sci (2022) 23(3):1446. doi: 10.3390/ijms23031446
110. Yasumizu Y, Miyajima A, Kosaka T, Miyazaki Y, Kikuchi E, Oya M. Dual PI3K/mTOR Inhibitor NVP-BEZ235 Sensitizes Docetaxel in Castration Resistant Prostate Cancer. J Urol (2014) 191(1):227–34. doi: 10.1016/j.juro.2013.07.101
111. Potiron VA, Abderrahmani R, Giang E, Chiavassa S, Di Tomaso E, Maira SM, et al. Radiosensitization of Prostate Cancer Cells by the Dual PI3K/mTOR Inhibitor BEZ235 Under Normoxic and Hypoxic Conditions. Radiother Oncol (2013) 106(1):138–46. doi: 10.1016/j.radonc.2012.11.014
112. Hong SW, Shin JS, Moon JH, Kim YS, Lee J, Choi EK, et al. NVP-BEZ235, a Dual PI3K/mTOR Inhibitor, Induces Cell Death Through Alternate Routes in Prostate Cancer Cells Depending on the PTEN Genotype. Apoptosis (2014) 19(5):895–904. doi: 10.1007/s10495-014-0973-4
113. Carver BS, Chapinski C, Wongvipat J, Hieronymus H, Chen Y, Chandarlapaty S, et al. Reciprocal Feedback Regulation of PI3K and Androgen Receptor Signaling in PTEN-Deficient Prostate Cancer. Cancer Cell (2011) 19(5):575–86. doi: 10.1016/j.ccr.2011.04.008
114. Adelaiye-Ogala R, Gryder BE, Nguyen YTM, Alilin AN, Grayson AR, Bajwa W, et al. Targeting the PI3K/AKT Pathway Overcomes Enzalutamide Resistance by Inhibiting Induction of the Glucocorticoid Receptor. Mol Cancer Ther (2020) 19(7):1436–47. doi: 10.1158/1535-7163.MCT-19-0936
115. Sun L, Huang Y, Liu Y, Zhao Y, He X, Zhang L, et al. Ipatasertib, a Novel Akt Inhibitor, Induces Transcription Factor FoxO3a and NF-κb Directly Regulates PUMA-Dependent Apoptosis. Cell Death Dis (2018) 9(9):911. doi: 10.1038/s41419-018-0943-9
116. Lin J, Sampath D, Nannini MA, Lee BB, Degtyarev M, Oeh J, et al. Targeting Activated Akt With GDC-0068, a Novel Selective Akt Inhibitor That is Efficacious in Multiple Tumor Models. Clin Cancer Res (2013) 19(7):1760–72. doi: 10.1158/1078-0432.CCR-12-3072
117. Tee SS, Suster I, Truong S, Jeong S, Eskandari R, DiGialleonardo V, et al. Targeted AKT Inhibition in Prostate Cancer Cells and Spheroids Reduces Aerobic Glycolysis and Generation of Hyperpolarized [1-(13)C] Lactate. Mol Cancer Res (2018) 16(3):453–60. doi: 10.1158/1541-7786.MCR-17-0458
118. Al-Saffar NMS, Troy H, Wong TeFong A-C, Paravati R, Jackson LE, Gowan S, et al. Metabolic Biomarkers of Response to the AKT Inhibitor MK-2206 in Pre-Clinical Models of Human Colorectal and Prostate Carcinoma. Br J Cancer (2018) 119(9):1118–28. doi: 10.1038/s41416-018-0242-3
119. De Velasco MA, Kura Y, Yoshikawa K, Nishio K, Davies BR, Uemura H, et al. Efficacy of Targeted AKT Inhibition in Genetically Engineered Mouse Models of PTEN-Deficient Prostate Cancer. Oncotarget (2016) 7(13):15959–76. doi: 10.18632/oncotarget.7557
120. Lamoureux F, Thomas C, Crafter C, Kumano M, Zhang F, Davies BR, et al. Blocked Autophagy Using Lysosomotropic Agents Sensitizes Resistant Prostate Tumor Cells to the Novel Akt Inhibitor AZD5363. Clin Cancer Res (2013) 19(4):833–44. doi: 10.1158/1078-0432.CCR-12-3114
121. Marques RB, Aghai A, de Ridder CMA, Stuurman D, Hoeben S, Boer A, et al. High Efficacy of Combination Therapy Using PI3K/AKT Inhibitors With Androgen Deprivation in Prostate Cancer Preclinical Models. Eur Urol (2015) 67(6):1177–85. doi: 10.1016/j.eururo.2014.08.053
122. Thomas C, Lamoureux F, Crafter C, Davies BR, Beraldi E, Fazli L, et al. Synergistic Targeting of PI3K/AKT Pathway and Androgen Receptor Axis Significantly Delays Castration-Resistant Prostate Cancer Progression In Vivo. Mol Cancer Ther (2013) 12(11):2342–55. doi: 10.1158/1535-7163.MCT-13-0032
123. Jin L, Zhang W, Yao MY, Tian Y, Xue BX, Tao W. GNE-493 Inhibits Prostate Cancer Cell Growth via Akt-mTOR-Dependent and -Independent Mechanisms. Cell Death Discovery (2022) 8(1):120. doi: 10.1038/s41420-022-00911-y
124. Morgan TM, Pitts TE, Gross TS, Poliachik SL, Vessella RL, Corey E. RAD001 (Everolimus) Inhibits Growth of Prostate Cancer in the Bone and the Inhibitory Effects are Increased by Combination With Docetaxel and Zoledronic Acid. Prostate (2008) 68(8):861–71. doi: 10.1002/pros.20752
125. Alshaker H, Wang Q, Kawano Y, Arafat T, Böhler T, Winkler M, et al. Everolimus (RAD001) Sensitizes Prostate Cancer Cells to Docetaxel by Down-Regulation of HIF-1α and Sphingosine Kinase 1. Oncotarget (2016) 7(49):80943–56. doi: 10.18632/oncotarget.13115
126. Schayowitz A, Sabnis G, Goloubeva O, Njar VC, Brodie AM. Prolonging Hormone Sensitivity in Prostate Cancer Xenografts Through Dual Inhibition of AR and mTOR. Br J Cancer (2010) 103(7):1001–7. doi: 10.1038/sj.bjc.6605882
127. Alshaker H, Wang Q, Böhler T, Mills R, Winkler M, Arafat T, et al. Combination of RAD001 (Everolimus) and Docetaxel Reduces Prostate and Breast Cancer Cell VEGF Production and Tumour Vascularisation Independently of Sphingosine-Kinase-1. Sci Rep (2017) 7(1):3493. doi: 10.1038/s41598-017-03728-3
128. Zhang W, Haines BB, Efferson C, Zhu J, Ware C, Kunii K, et al. Evidence of mTOR Activation by an AKT-Independent Mechanism Provides Support for the Combined Treatment of PTEN-Deficient Prostate Tumors With mTOR and AKT Inhibitors. Transl Oncol (2012) 5(6):422–9. doi: 10.1593/tlo.12241
129. Floc'h N, Kinkade CW, Kobayashi T, Aytes A, Lefebvre C, Mitrofanova A, et al. Dual Targeting of the Akt/mTOR Signaling Pathway Inhibits Castration-Resistant Prostate Cancer in a Genetically Engineered Mouse Model. Cancer Res (2012) 72(17):4483–93. doi: 10.1158/0008-5472.CAN-12-0283
130. Rosette C, Agan FJ, Rosette N, Mazzetti A, Moro L, Gerloni M. The Dual Androgen Receptor and Glucocorticoid Receptor Antagonist CB-03-10 as Potential Treatment for Tumors That Have Acquired GR-Mediated Resistance to AR Blockade. Mol Cancer Ther (2020) 19(11):2256–66. doi: 10.1158/1535-7163.MCT-19-1137
131. Kroon J, Puhr M, Buijs JT, van derHorst G, Hemmer DM, Marijt KA, et al. Glucocorticoid Receptor Antagonism Reverts Docetaxel Resistance in Human Prostate Cancer. Endocrine-Related Cancer (2016) 23(1):35–45. doi: 10.1530/ERC-15-0343
132. Yan TZ, Jin FS, Xie LP, Li LC. Relationship Between Glucocorticoid Receptor Signal Pathway and Androgen-Independent Prostate Cancer. Urol Int (2008) 81(2):228–33. doi: 10.1159/000144067
133. El Etreby MF, Liang Y, Johnson MH, Lewis RW. Antitumor Activity of Mifepristone in the Human LNCaP, LNCaP-C4, and LNCaP-C4-2 Prostate Cancer Models in Nude Mice. Prostate (2000) 42(2):99–106. doi: 10.1002/(SICI)1097-0045(20000201)42:2<99::AID-PROS3>3.0.CO;2-I
134. Gao M, Guo L, Wang H, Huang J, Han F, Xiang S, et al. Orphan Nuclear Receptor Rorγ Confers Doxorubicin Resistance in Prostate Cancer. Cell Biol Int (2020) 44(10):2170–6. doi: 10.1002/cbin.11411
135. Qi W, Cooke LS, Stejskal A, Riley C, Croce KD, Saldanha JW, et al. MP470, a Novel Receptor Tyrosine Kinase Inhibitor, in Combination With Erlotinib Inhibits the HER Family/PI3K/Akt Pathway and Tumor Growth in Prostate Cancer. BMC Cancer (2009) 9:142. doi: 10.1186/1471-2407-9-142
136. Zhou F, Chen X, Fan S, Tai S, Jiang C, Zhang Y, et al. GSK1838705A, an Insulin-Like Growth Factor-1 Receptor/Insulin Receptor Inhibitor, Induces Apoptosis and Reduces Viability of Docetaxel-Resistant Prostate Cancer Cells Both In Vitro and In Vivo. Oncol Targets Ther (2015) 8:753–60. doi: 10.2147/OTT.S79105
137. Isebaert SF, Swinnen JV, McBride WH, Haustermans KM. Insulin-Like Growth Factor-Type 1 Receptor Inhibitor NVP-AEW541 Enhances Radiosensitivity of PTEN Wild-Type But Not PTEN-Deficient Human Prostate Cancer Cells. Int J Radiat Oncol Biol Phys (2011) 81(1):239–47. doi: 10.1016/j.ijrobp.2011.03.030
138. Chitnis MM, Lodhia KA, Aleksic T, Gao S, Protheroe AS, Macaulay VM. IGF-1R Inhibition Enhances Radiosensitivity and Delays Double-Strand Break Repair by Both non-Homologous End-Joining and Homologous Recombination. Oncogene (2014) 33(45):5262–73. doi: 10.1038/onc.2013.460
139. Gioeli D, Wunderlich W, Sebolt-Leopold J, Bekiranov S, Wulfkuhle JD, Petricoin EF 3rd, et al. Compensatory Pathways Induced by MEK Inhibition are Effective Drug Targets for Combination Therapy Against Castration-Resistant Prostate Cancer. Mol Cancer Ther (2011) 10(9):1581–90. doi: 10.1158/1535-7163.MCT-10-1033
140. Ciccarelli C, Di Rocco A, Gravina GL, Mauro A, Festuccia C, Del Fattore A, et al. Disruption of MEK/ERK/c-Myc Signaling Radiosensitizes Prostate Cancer Cells In Vitro and In Vivo. J Cancer Res Clin Oncol (2018) 144(9):1685–99. doi: 10.1007/s00432-018-2696-3
141. Dayyani F, Parikh NU, Varkaris AS, Song JH, Moorthy S, Chatterji T, et al. Combined Inhibition of IGF-1r/IR and Src Family Kinases Enhances Antitumor Effects in Prostate Cancer by Decreasing Activated Survival Pathways. PLos One (2012) 7(12):e51189. doi: 10.1371/journal.pone.0051189
142. Park SI, Zhang J, Phillips KA, Araujo JC, Najjar AM, Volgin AY, et al. Targeting SRC Family Kinases Inhibits Growth and Lymph Node Metastases of Prostate Cancer in an Orthotopic Nude Mouse Model. Cancer Res (2008) 68(9):3323–33. doi: 10.1158/0008-5472.CAN-07-2997
143. Nam S, Kim D, Cheng JQ, Zhang S, Lee JH, Buettner R, et al. Action of the Src Family Kinase Inhibitor, Dasatinib (BMS-354825), on Human Prostate Cancer Cells. Cancer Res (2005) 65(20):9185–9. doi: 10.1158/0008-5472.CAN-05-1731
144. Rice L, Lepler S, Pampo C, Siemann DW. Impact of the SRC Inhibitor Dasatinib on the Metastatic Phenotype of Human Prostate Cancer Cells. Clin Exp Metastasis (2012) 29(2):133–42. doi: 10.1007/s10585-011-9436-2
145. Liu Y, Karaca M, Zhang Z, Gioeli D, Earp HS, Whang YE, et al. Dasatinib Inhibits Site-Specific Tyrosine Phosphorylation of Androgen Receptor by Ack1 and Src Kinases. Oncogene (2010) 29(22):3208–16. doi: 10.1038/onc.2010.103
146. Chakraborty G, Patail NK, Hirani R, Nandakumar S, Mazzu YZ, Yoshikawa Y, et al. Attenuation of SRC Kinase Activity Augments PARP Inhibitor-Mediated Synthetic Lethality in BRCA2-Altered Prostate Tumors. Clin Cancer Res (2021) 27(6):1792–806. doi: 10.1158/1078-0432.CCR-20-2483
147. Araujo JC, Poblenz A, Corn P, Parikh NU, Starbuck MW, Thompson JT, et al. Dasatinib Inhibits Both Osteoclast Activation and Prostate Cancer PC-3-Cell-Induced Osteoclast Formation. Cancer Biol Ther (2009) 8(22):2153–9. doi: 10.4161/cbt.8.22.9770
148. Chang YM, Bai L, Liu S, Yang JC, Kung HJ, Evans CP. Src Family Kinase Oncogenic Potential and Pathways in Prostate Cancer as Revealed by AZD0530. Oncogene (2008) 27(49):6365–75. doi: 10.1038/onc.2008.250
149. Yang JC, Bai L, Yap S, Gao AC, Kung HJ, Evans CP, et al. Effect of the Specific Src Family Kinase Inhibitor Saracatinib on Osteolytic Lesions Using the PC-3 Bone Model. Mol Cancer Ther (2010) 9(6):1629–37. doi: 10.1158/1535-7163.MCT-09-1058
150. Rabbani SA, Valentino ML, Arakelian A, Ali S, Boschelli F. SKI-606 (Bosutinib) Blocks Prostate Cancer Invasion, Growth, and Metastasis In Vitro and In Vivo Through Regulation of Genes Involved in Cancer Growth and Skeletal Metastasis. Mol Cancer Ther (2010) 9(5):1147–57. doi: 10.1158/1535-7163.MCT-09-0962
151. Dai Y, Siemann DW. BMS-777607, a Small-Molecule Met Kinase Inhibitor, Suppresses Hepatocyte Growth Factor-Stimulated Prostate Cancer Metastatic Phenotype In Vitro. Mol Cancer Ther (2010) 9(6):1554–61. doi: 10.1158/1535-7163.MCT-10-0359
152. Dai Y, Siemann DW. Constitutively Active C-Met Kinase in PC-3 Cells is Autocrine-Independent and can be Blocked by the Met Kinase Inhibitor BMS-777607. BMC Cancer (2012) 12:198. doi: 10.1186/1471-2407-12-198
153. Witt K, Evans-Axelsson S, Lundqvist A, Johansson M, Bjartell A, Hellsten R. Inhibition of STAT3 Augments Antitumor Efficacy of Anti-CTLA-4 Treatment Against Prostate Cancer. Cancer Immunol Immunother (2021) 70(11):3155–66. doi: 10.1007/s00262-021-02915-6
154. Yun S, Lee YJ, Choi J, Kim ND, Han DC, Kwon BM. Acacetin Inhibits the Growth of STAT3-Activated DU145 Prostate Cancer Cells by Directly Binding to Signal Transducer and Activator of Transcription 3 (Stat3). Molecules (2021) 26(20):6204. doi: 10.3390/molecules26206204
155. Canesin G, Maggio V, Palominos M, Stiehm A, Contreras HR, Castellón EA, et al. STAT3 Inhibition With Galiellalactone Effectively Targets the Prostate Cancer Stem-Like Cell Population. Sci Rep (2020) 10(1):13958. doi: 10.1038/s41598-020-70948-5
156. Canesin G, Evans-Axelsson S, Hellsten R, Sterner O, Krzyzanowska A, Andersson T, et al. The STAT3 Inhibitor Galiellalactone Effectively Reduces Tumor Growth and Metastatic Spread in an Orthotopic Xenograft Mouse Model of Prostate Cancer. Eur Urol (2016) 69(3):400–4. doi: 10.1016/j.eururo.2015.06.016
157. Thaper D, Vahid S, Kaur R, Kumar S, Nouruzi S, Bishop JL, et al. Galiellalactone Inhibits the STAT3/AR Signaling Axis and Suppresses Enzalutamide-Resistant Prostate Cancer. Sci Rep (2018) 8(1):17307. doi: 10.1038/s41598-018-35612-z
158. Civenni G, Longoni N, Costales P, Dallavalle C, García Inclán C, Albino D, et al. EC-70124, a Novel Glycosylated Indolocarbazole Multikinase Inhibitor, Reverts Tumorigenic and Stem Cell Properties in Prostate Cancer by Inhibiting STAT3 and NF-κb. Mol Cancer Ther (2016) 15(5):806–18. doi: 10.1158/1535-7163.MCT-15-0791
159. Feiersinger GE, Trattnig K, Leitner PD, Guggenberger F, Oberhuber A, Peer S, et al. Olaparib is Effective in Combination With, and as Maintenance Therapy After, First-Line Endocrine Therapy in Prostate Cancer Cells. Mol Oncol (2018) 12(4):561–76. doi: 10.1002/1878-0261.12185
160. Li L, Karanika S, Yang G, Wang J, Park S, Broom BM, et al. Androgen Receptor Inhibitor-Induced "BRCAness" and PARP Inhibition are Synthetically Lethal for Castration-Resistant Prostate Cancer. Sci Signaling (2017) 10(480):eaam7479. doi: 10.1126/scisignal.aam7479
161. Zhang W, Liu B, Wu W, Li L, Broom BM, Basourakos SP, et al. Targeting the MYCN-PARP-DNA Damage Response Pathway in Neuroendocrine Prostate Cancer. Clin Cancer Res (2018) 24(3):696–707. doi: 10.1158/1078-0432.CCR-17-1872
162. Gani C, Coackley C, Kumareswaran R, Schütze C, Krause M, Zafarana G, et al. In Vivo Studies of the PARP Inhibitor, AZD-2281, in Combination With Fractionated Radiotherapy: An Exploration of the Therapeutic Ratio. Radiother Oncol (2015) 116(3):486–94. doi: 10.1016/j.radonc.2015.08.003
163. Barreto-Andrade JC, Efimova EV, Mauceri HJ, Beckett MA, Sutton HG, Darga TE, et al. Response of Human Prostate Cancer Cells and Tumors to Combining PARP Inhibition With Ionizing Radiation. Mol Cancer Ther (2011) 10(7):1185–93. doi: 10.1158/1535-7163.MCT-11-0061
164. Yin L, Liu Y, Peng Y, Peng Y, Yu X, Gao Y, et al. PARP Inhibitor Veliparib and HDAC Inhibitor SAHA Synergistically Co-Target the UHRF1/BRCA1 DNA Damage Repair Complex in Prostate Cancer Cells. J Exp Clin Cancer Res (2018) 37(1):153. doi: 10.1186/s13046-018-0810-7
165. Sargazi S, Saravani R, Zavar Reza J, Jaliani HZ, Mirinejad S, Rezaei Z, et al. Induction of Apoptosis and Modulation of Homologous Recombination DNA Repair Pathway in Prostate Cancer Cells by the Combination of AZD2461 and Valproic Acid. Excli J (2019) 18:485–98. doi: 10.17179/excli2019-1098
166. Sargazi S, Saravani R, Zavar Reza J, Zarei Jaliani H, Galavi H, Moudi M, et al. Novel Poly(Adenosine Diphosphate-Ribose) Polymerase (PARP) Inhibitor, AZD2461, Down-Regulates VEGF and Induces Apoptosis in Prostate Cancer Cells. Iran BioMed J (2019) 23(5):312–23. doi: 10.29252/ibj.23.5.2
167. Chatterjee P, Choudhary GS, Sharma A, Singh K, Heston WD, Ciezki J, et al. PARP Inhibition Sensitizes to Low Dose-Rate Radiation TMPRSS2-ERG Fusion Gene-Expressing and PTEN-Deficient Prostate Cancer Cells. PLos One (2013) 8(4):e60408. doi: 10.1371/journal.pone.0060408
168. Cattrini C, Capaia M, Boccardo F, Barboro P. Etoposide and Topoisomerase II Inhibition for Aggressive Prostate Cancer: Data From a Translational Study. Cancer Treat Res Commun (2020) 25:100221. doi: 10.1016/j.ctarc.2020.100221
169. Hanahan D, Robert A. Weinberg, Hallmarks of Cancer: The Next Generation. Cell (2011) 144(5):646–74. doi: 10.1016/j.cell.2011.02.013
170. Naveja JJ, Dueñas-González A, Medina-Franco JL. Chapter 12 - Drug Repurposing for Epigenetic Targets Guided by Computational Methods. In: Medina-Franco JL, editor. Epi-Informatics. Boston: Academic Press (2016). p. 327–57.
171. Cheng H, Tang S, Lian X, Meng H, Gu X, Jiang J, et al. The Differential Antitumor Activity of 5-Aza-2'-Deoxycytidine in Prostate Cancer DU145, 22RV1, and LNCaP Cells. J Cancer (2021) 12(18):5593–604. doi: 10.7150/jca.56709
172. Gravina GL, Marampon F, Sanità P, Mancini A, Colapietro A, Scarsella L, et al. Increased Expression and Activity of P75ntr are Crucial Events in Azacitidine-Induced Cell Death in Prostate Cancer. Oncol Rep (2016) 36(1):125–30. doi: 10.3892/or.2016.4832
173. Moreira-Silva F, Camilo V, Gaspar V, Mano JF, Henrique R, Jeronimo C. Repurposing Old Drugs Into New Epigenetic Inhibitors: Promising Candidates for Cancer Treatment? Pharmaceutics (2020) 12(5):410. doi: 10.3390/pharmaceutics12050410
174. Civenni G, Bosotti R, Timpanaro A, Vàzquez R, Merulla J, Pandit S, et al. Epigenetic Control of Mitochondrial Fission Enables Self-Renewal of Stem-Like Tumor Cells in Human Prostate Cancer. Cell Metab (2019) 30(2):303–18.e6. doi: 10.1016/j.cmet.2019.05.004
175. Bauer K, Berghoff AS, Preusser M, Heller G, Zielinski CC, Valent P, et al. Degradation of BRD4 - a Promising Treatment Approach Not Only for Hematologic But Also for Solid Cancer. Am J Cancer Res (2021) 11(2):530–45.
176. Wang L, Xu M, Kao CY, Tsai SY, Tsai MJ. Small Molecule JQ1 Promotes Prostate Cancer Invasion via BET-Independent Inactivation of FOXA1. J Clin Invest (2020) 130(4):1782–92. doi: 10.1172/JCI126327
177. Chan SC, Selth LA, Li Y, Nyquist MD, Miao L, Bradner JE, et al. Targeting Chromatin Binding Regulation of Constitutively Active AR Variants to Overcome Prostate Cancer Resistance to Endocrine-Based Therapies. Nucleic Acids Res (2015) 43(12):5880–97. doi: 10.1093/nar/gkv262
178. Tan Y, Wang L, Du Y, Liu X, Chen Z, Weng X, et al. Inhibition of BRD4 Suppresses Tumor Growth in Prostate Cancer via the Enhancement of FOXO1 Expression. Int J Oncol (2018) 53(6):2503–17. doi: 10.3892/ijo.2018.4577
179. Gao L, Schwartzman J, Gibbs A, Lisac R, Kleinschmidt R, Wilmot B, et al. Androgen Receptor Promotes Ligand-Independent Prostate Cancer Progression Through C-Myc Upregulation. PLos One (2013) 8(5):e63563. doi: 10.1371/journal.pone.0063563
180. Welti J, Sharp A, Yuan W, Dolling D, Nava Rodrigues D, Figueiredo I, et al. Targeting Bromodomain and Extra-Terminal (BET) Family Proteins in Castration-Resistant Prostate Cancer (CRPC). Clin Cancer Res (2018) 24(13):3149–62. doi: 10.1158/1078-0432.CCR-17-3571
181. Mao W, Ghasemzadeh A, Freeman ZT, Obradovic A, Chaimowitz MG, Nirschl TR, et al. Immunogenicity of Prostate Cancer is Augmented by BET Bromodomain Inhibition. J Immunother Cancer (2019) 7(1):277–7. doi: 10.1186/s40425-019-0758-y
182. Liu K, Zhou Z, Gao H, Yang F, Qian Y, Jin H, et al. JQ1, a BET-Bromodomain Inhibitor, Inhibits Human Cancer Growth and Suppresses PD-L1 Expression. Cell Biol Int (2019) 43(6):642–50. doi: 10.1002/cbin.11139
183. Xiang Q, Zhang Y, Li J, Xue X, Wang C, Song M, et al. Y08060: A Selective BET Inhibitor for Treatment of Prostate Cancer. ACS Med Chem Lett (2018) 9(3):262–7. doi: 10.1021/acsmedchemlett.8b00003
184. Xu X, Zhu X, Liu F, Lu W, Wang Y, Yu J. The Effects of Histone Crotonylation and Bromodomain Protein 4 on Prostate Cancer Cell Lines. Trans Androl Urol (2021) 10(2):900–14. doi: 10.21037/tau-21-53
185. Shen G, Chen J, Zhou Y, Wang Z, Ma Z, Xu C, et al. AZD5153 Inhibits Prostate Cancer Cell Growth in Vitro and in Vivo. Cell Physiol Biochem (2018) 50(2):798–809. doi: 10.1159/000494244
186. Hu R, Wang WL, Yang YY, Hu XT, Wang QW, Zuo WQ, et al. Identification of a Selective BRD4 PROTAC With Potent Antiproliferative Effects in AR-Positive Prostate Cancer Based on a Dual BET/PLK1 Inhibitor. Eur J Med Chem (2022) 227:113922. doi: 10.1016/j.ejmech.2021.113922
187. Attwell S CE, Jahagirdar R, Kharenko O, Norek K, Tsujikawa L, Calosing C, et al. (2015). The Clinical Candidate ZEN-3694, a Novel BET Bromodomain Inhibitor, is Efficacious in the Treatment of a Variety of Solid Tumor and Hematological Malignancies, Alone or in Combination With Several Standard of Care and Targeted Therapies, in: Molecular Targets And Cancer Therapeutics, John B, Hynes veterans memorial Convention center Boston, MA.
188. Kim D-H, Sun D, Storck WK, Welker Leng K, Jenkins C, Coleman DJ, et al. BET Bromodomain Inhibition Blocks an AR-Repressed, E2F1-Activated Treatment-Emergent Neuroendocrine Prostate Cancer Lineage Plasticity Program. Clin Cancer Res (2021) 27(17):4923. doi: 10.1158/1078-0432.CCR-20-4968
189. Faivre EJ, Wilcox D, Lin X, Hessler P, Torrent M, He W, et al. Exploitation of Castration-Resistant Prostate Cancer Transcription Factor Dependencies by the Novel BET Inhibitor ABBV-075. Mol Cancer Res (2017) 15(1):35–44. doi: 10.1158/1541-7786.MCR-16-0221
190. Faivre EJ, McDaniel KF, Albert DH, Mantena SR, Plotnik JP, Wilcox D, et al. Selective Inhibition of the BD2 Bromodomain of BET Proteins in Prostate Cancer. Nature (2020) 578(7794):306–10. doi: 10.1038/s41586-020-1930-8
191. Wu TB, et al. Y06014 is a Selective BET Inhibitor for the Treatment of Prostate Cancer. Acta Pharmacol Sin (2021) 42(12):2120–31. doi: 10.1038/s41401-021-00614-7
192. Yan Y, Ma J, Wang D, Lin D, Pang X, Wang S, et al. The Novel BET-CBP/p300 Dual Inhibitor NEO2734 is Active in SPOP Mutant and Wild-Type Prostate Cancer. EMBO Mol Med (2019) 11(11):e10659–9. doi: 10.15252/emmm.201910659
193. Lasko LM, Jakob CG, Edalji RP, Qiu W, Montgomery D, Digiammarino EL, et al. Discovery of a Selective Catalytic P300/CBP Inhibitor That Targets Lineage-Specific Tumours. Nature (2017) 550(7674):128–32. doi: 10.1038/nature24028
194. Welti J, Sharp A, Brooks N, Yuan W, McNair C, Chand SN, et al. Targeting the P300/CBP Axis in Lethal Prostate Cancer. Cancer Discovery (2021) 11(5):1118–37. doi: 10.1158/2159-8290.CD-20-0751
195. Zou LJ, Xiang QP, Xue XQ, Zhang C, Li CC, Wang C, et al. Y08197 is a Novel and Selective CBP/EP300 Bromodomain Inhibitor for the Treatment of Prostate Cancer. Acta Pharmacol Sin (2019) 40(11):1436–47. doi: 10.1038/s41401-019-0237-5
196. Zucconi BE, Makofske JL, Meyers DJ, Hwang Y, Wu M, Kuroda MI, et al. Combination Targeting of the Bromodomain and Acetyltransferase Active Site of P300/CBP. Biochemistry (2019) 58(16):2133–43. doi: 10.1021/acs.biochem.9b00160
197. Kiliccioglu I, Konac E, Varol N, Gurocak S, Yucel Bilen C. Apoptotic Effects of Proteasome and Histone Deacetylase Inhibitors in Prostate Cancer Cell Lines. Genet Mol Res (2014) 13(2):3721–31. doi: 10.4238/2014.May.9.17
198. Zhang H, Zhao X, Liu H, Jin H, Ji Y. Trichostatin A Inhibits Proliferation of PC3 Prostate Cancer Cells by Disrupting the EGFR Pathway. Oncol Lett (2019) 18(1):687–93. doi: 10.3892/ol.2019.10384
199. Wang X, Xu J, Wang H, Wu L, Yuan W, Du J, et al. Trichostatin A, a Histone Deacetylase Inhibitor, Reverses Epithelial-Mesenchymal Transition in Colorectal Cancer SW480 and Prostate Cancer PC3 Cells. Biochem Biophys Res Commun (2015) 456(1):320–6. doi: 10.1016/j.bbrc.2014.11.079
200. Zhu S, Li Y, Zhao L, Hou P, Shangguan C, Yao R, et al. TSA-Induced JMJD2B Downregulation is Associated With Cyclin B1-Dependent Survivin Degradation and Apoptosis in LNCap Cells. J Cell Biochem (2012) 113(7):2375–82. doi: 10.1002/jcb.24109
201. Pulukuri SM, Gorantla B, Knost JA, Rao JS. Frequent Loss of Cystatin E/M Expression Implicated in the Progression of Prostate Cancer. Oncogene (2009) 28(31):2829–38. doi: 10.1038/onc.2009.134
202. Xu Q, Liu X, Zhu S, Hu X, Niu H, Zhang X, et al. Hyper-Acetylation Contributes to the Sensitivity of Chemo-Resistant Prostate Cancer Cells to Histone Deacetylase Inhibitor Trichostatin A. J Cell Mol Med (2018) 22(3):1909–22. doi: 10.1111/jcmm.13475
203. Xiao W, Graham PH, Hao J, Chang L, Ni J, Power CA, et al. Combination Therapy With the Histone Deacetylase Inhibitor LBH589 and Radiation is an Effective Regimen for Prostate Cancer Cells. PLos One (2013) 8(8):e74253. doi: 10.1371/journal.pone.0074253
204. Pacheco MB, Camilo V, Lopes N, Moreira-Silva F, Correia MP, Henrique R, et al. Hydralazine and Panobinostat Attenuate Malignant Properties of Prostate Cancer Cell Lines. Pharmaceut (Basel) (2021) 14(7):670. doi: 10.3390/ph14070670
205. Chen E, Liu N, Zhao Y, Tang M, Ou L, Wu X, et al. Panobinostat Reverses HepaCAM Gene Expression and Suppresses Proliferation by Increasing Histone Acetylation in Prostate Cancer. Gene (2021) 808:145977. doi: 10.1016/j.gene.2021.145977
206. Pettazzoni P, Pizzimenti S, Toaldo C, Sotomayor P, Tagliavacca L, Liu S, et al. Induction of Cell Cycle Arrest and DNA Damage by the HDAC Inhibitor Panobinostat (LBH589) and the Lipid Peroxidation End Product 4-Hydroxynonenal in Prostate Cancer Cells. Free Radic Biol Med (2011) 50(2):313–22. doi: 10.1016/j.freeradbiomed.2010.11.011
207. Chuang MJ, Wu ST, Tang SH, Lai XM, Lai HC, Hsu KH, et al. The HDAC Inhibitor LBH589 Induces ERK-Dependent Prometaphase Arrest in Prostate Cancer via HDAC6 Inactivation and Down-Regulation. PLos One (2013) 8(9):e73401. doi: 10.1371/journal.pone.0073401
208. Bruzzese F, Pucci B, Milone MR, Ciardiello C, Franco R, Chianese MI, et al. Panobinostat Synergizes With Zoledronic Acid in Prostate Cancer and Multiple Myeloma Models by Increasing ROS and Modulating Mevalonate and P38-MAPK Pathways. Cell Death Dis (2013) 4(10):e878. doi: 10.1038/cddis.2013.406
209. Marrocco DL, Tilley WD, Bianco-Miotto T, Evdokiou A, Scher HI, Rifkind RA, et al. Suberoylanilide Hydroxamic Acid (Vorinostat) Represses Androgen Receptor Expression and Acts Synergistically With an Androgen Receptor Antagonist to Inhibit Prostate Cancer Cell Proliferation. Mol Cancer Ther (2007) 6(1):51–60. doi: 10.1158/1535-7163.MCT-06-0144
210. Park SE, Kim HG, Kim DE, Jung YJ, Kim Y, Jeong SY, et al. Combination Treatment With Docetaxel and Histone Deacetylase Inhibitors Downregulates Androgen Receptor Signaling in Castration-Resistant Prostate Cancer. Invest New Drugs (2018) 36(2):195–205. doi: 10.1007/s10637-017-0529-x
211. Wang L, Zou X, Berger AD, Twiss C, Peng Y, Li Y, et al. Increased Expression of Histone Deacetylaces (HDACs) and Inhibition of Prostate Cancer Growth and Invasion by HDAC Inhibitor SAHA. Am J Transl Res (2009) 1(1):62–71.
212. Shi XY, Ding W, Li TQ, Zhang YX, Zhao SC. Histone Deacetylase (HDAC) Inhibitor, Suberoylanilide Hydroxamic Acid (SAHA), Induces Apoptosis in Prostate Cancer Cell Lines via the Akt/FOXO3a Signaling Pathway. Med Sci Monit (2017) 23:5793–802. doi: 10.12659/MSM.904597
213. Patra N, De U, Kim TH, Lee YJ, Ahn MY, Kim ND, et al. A Novel Histone Deacetylase (HDAC) Inhibitor MHY219 Induces Apoptosis via Up-Regulation of Androgen Receptor Expression in Human Prostate Cancer Cells. BioMed Pharmacother (2013) 67(5):407–15. doi: 10.1016/j.biopha.2013.01.006
214. De U, Kundu S, Patra N, Ahn MY, Ahn JH, Son JY, et al. A New Histone Deacetylase Inhibitor, MHY219, Inhibits the Migration of Human Prostate Cancer Cells via HDAC1. Biomol Ther (Seoul) (2015) 23(5):434–41. doi: 10.4062/biomolther.2015.026
215. Rana Z, Diermeier S, Walsh FP, Hanif M, Hartinger CG, Rosengren RJ, et al. Anti-Proliferative, Anti-Angiogenic and Safety Profiles of Novel HDAC Inhibitors for the Treatment of Metastatic Castration-Resistant Prostate Cancer. Pharmaceut (Basel) (2021) 14(10):1020. doi: 10.3390/ph14101020
216. Rana Z, Tyndall JDA, Hanif M, Hartinger CG, Rosengren RJ. Cytostatic Action of Novel Histone Deacetylase Inhibitors in Androgen Receptor-Null Prostate Cancer Cells. Pharmaceut (Basel) (2021) 14(2):103. doi: 10.3390/ph14020103
217. Hwang JJ, Kim YS, Kim T, Kim MJ, Jeong IG, Lee JH, et al. A Novel Histone Deacetylase Inhibitor, CG200745, Potentiates Anticancer Effect of Docetaxel in Prostate Cancer via Decreasing Mcl-1 and Bcl-XL. Invest New Drugs (2012) 30(4):1434–42. doi: 10.1007/s10637-011-9718-1
218. Richa S, Dey P, Park C, Yang J, Son JY, Park JH, et al. A New Histone Deacetylase Inhibitor, MHY4381, Induces Apoptosis via Generation of Reactive Oxygen Species in Human Prostate Cancer Cells. Biomol Ther (Seoul) (2020) 28(2):184–94. doi: 10.4062/biomolther.2019.074
219. Qi G, Lu G, Yu J, Zhao Y, Wang C, Zhang H, et al. Up-Regulation of TIF1γ by Valproic Acid Inhibits the Epithelial Mesenchymal Transition in Prostate Carcinoma Through TGF-β/Smad Signaling Pathway. Eur J Pharmacol (2019) 860:172551. doi: 10.1016/j.ejphar.2019.172551
220. Makarević J, Rutz J, Juengel E, Maxeiner S, Tsaur I, Chun FK, et al. Influence of the HDAC Inhibitor Valproic Acid on the Growth and Proliferation of Temsirolimus-Resistant Prostate Cancer Cells In Vitro. Cancers (Basel) (2019) 11(4):566. doi: 10.3390/cancers11040566
221. Choi ES, Han G, Park SK, Lee K, Kim HJ, Cho SD, et al. A248, a Novel Synthetic HDAC Inhibitor, Induces Apoptosis Through the Inhibition of Specificity Protein 1 and its Downstream Proteins in Human Prostate Cancer Cells. Mol Med Rep (2013) 8(1):195–200. doi: 10.3892/mmr.2013.1481
222. Wu YW, Hsu KC, Lee HY, Huang TC, Lin TE, Chen YL, et al. A Novel Dual HDAC6 and Tubulin Inhibitor, MPT0B451, Displays Anti-Tumor Ability in Human Cancer Cells in Vitro and in Vivo. Front Pharmacol (2018) 9:205. doi: 10.3389/fphar.2018.00205
223. Hu WY, Xu L, Chen B, Ou S, Muzzarelli KM, Hu DP, et al. Targeting Prostate Cancer Cells With Enzalutamide-HDAC Inhibitor Hybrid Drug 2-75. Prostate (2019) 79(10):1166–79. doi: 10.1002/pros.23832
224. Kim J, Lee Y, Lu X, Song B, Fong KW, Cao Q, et al. Polycomb- and Methylation-Independent Roles of EZH2 as a Transcription Activator. Cell Rep (2018) 25(10):2808–2820.e4. doi: 10.1016/j.celrep.2018.11.035
225. Morel KL, Sheahan AV, Burkhart DL, Baca SC, Boufaied N, Liu Y, et al. EZH2 Inhibition Activates a dsRNA-STING-Interferon Stress Axis That Potentiates Response to PD-1 Checkpoint Blockade in Prostate Cancer. Nat Cancer (2021) 2(4):444–56. doi: 10.1038/s43018-021-00185-w
226. Verma SK, Tian X, LaFrance LV, Duquenne C, Suarez DP, Newlander KA, et al. Identification of Potent, Selective, Cell-Active Inhibitors of the Histone Lysine Methyltransferase Ezh2. ACS Med Chem Lett (2012) 3(12):1091–6. doi: 10.1021/ml3003346
227. Li X, Gera L, Zhang S, Chen Y, Lou L, Wilson LM, et al. Pharmacological Inhibition of Noncanonical EED-EZH2 Signaling Overcomes Chemoresistance in Prostate Cancer. Theranostics (2021) 11(14):6873–90. doi: 10.7150/thno.49235
228. Bai Y, Zhang Z, Cheng L, Wang R, Chen X, Kong Y, et al. Inhibition of Enhancer of Zeste Homolog 2 (EZH2) Overcomes Enzalutamide Resistance in Castration-Resistant Prostate Cancer. J Biol Chem (2019) 294(25):9911–23. doi: 10.1074/jbc.RA119.008152
229. Shankar E, Franco D, Iqbal O, Moreton S, Kanwal R, Gupta S. Dual Targeting of EZH2 and Androgen Receptor as a Novel Therapy for Castration-Resistant Prostate Cancer. Toxicol Appl Pharmacol (2020) 404:115200. doi: 10.1016/j.taap.2020.115200
230. Kong Y, Zhang Y, Mao F, Zhang Z, Li Z, Wang R, et al. Inhibition of EZH2 Enhances the Antitumor Efficacy of Metformin in Prostate Cancer. Mol Cancer Ther (2020) 19(12):2490–501. doi: 10.1158/1535-7163.MCT-19-0874
231. Wu C, Jin X, Yang J, Yang Y, He Y, Ding L, et al. Inhibition of EZH2 by Chemo- and Radiotherapy Agents and Small Molecule Inhibitors Induces Cell Death in Castration-Resistant Prostate Cancer. Oncotarget (2016) 7(3):3440–52. doi: 10.18632/oncotarget.6497
232. Etani T, Naiki T, Naiki-Ito A, Suzuki T, Iida K, Nozaki S, et al. NCL1, A Highly Selective Lysine-Specific Demethylase 1 Inhibitor, Suppresses Castration-Resistant Prostate Cancer Growth via Regulation of Apoptosis and Autophagy. J Clin Med (2019) 8(4):442. doi: 10.3390/jcm8040442
233. Etani T, Suzuki T, Naiki T, Naiki-Ito A, Ando R, Iida K, et al. NCL1, a Highly Selective Lysine-Specific Demethylase 1 Inhibitor, Suppresses Prostate Cancer Without Adverse Effect. Oncotarget (2015) 6(5):2865–78. doi: 10.18632/oncotarget.3067
234. Gupta S, Weston A, Bearrs J, Thode T, Neiss A, Soldi R, et al. Reversible Lysine-Specific Demethylase 1 Antagonist HCI-2509 Inhibits Growth and Decreases C-MYC in Castration- and Docetaxel-Resistant Prostate Cancer Cells. Prostate Cancer Prostatic Dis (2016) 19(4):349–57. doi: 10.1038/pcan.2016.21
235. Fialova B, Luzna P, Gursky J, Langova K, Kolar Z, Trtkova KS. Epigenetic Modulation of AR Gene Expression in Prostate Cancer DU145 Cells With the Combination of Sodium Butyrate and 5'-Aza-2'-Deoxycytidine. Oncol Rep (2016) 36(4):2365–74. doi: 10.3892/or.2016.5000
236. Liu T, Qiu X, Zhao X, Yang R, Lian H, Qu F, et al. Hypermethylation of the SPARC Promoter and its Prognostic Value for Prostate Cancer. Oncol Rep (2018) 39(2):659–66. doi: 10.3892/or.2017.6121
237. Wang X, Gao H, Ren L, Gu J, Zhang Y, Zhang Y. Demethylation of the miR-146a Promoter by 5-Aza-2'-Deoxycytidine Correlates With Delayed Progression of Castration-Resistant Prostate Cancer. BMC Cancer (2014) 14:308. doi: 10.1186/1471-2407-14-308
238. Tian J, Lee SO, Liang L, Luo J, Huang CK, Li L, et al. Targeting the Unique Methylation Pattern of Androgen Receptor (AR) Promoter in Prostate Stem/Progenitor Cells With 5-Aza-2'-Deoxycytidine (5-AZA) Leads to Suppressed Prostate Tumorigenesis. J Biol Chem (2012) 287(47):39954–66. doi: 10.1074/jbc.M112.395574
239. Patra A, Deb M, Dahiya R, Patra SK. 5-Aza-2'-Deoxycytidine Stress Response and Apoptosis in Prostate Cancer. Clin Epigenet (2011) 2(2):339–48. doi: 10.1007/s13148-010-0019-x
240. Chiam K, Centenera MM, Butler LM, Tilley WD, Bianco-Miotto T. GSTP1 DNA Methylation and Expression Status is Indicative of 5-Aza-2'-Deoxycytidine Efficacy in Human Prostate Cancer Cells. PLos One (2011) 6(9):e25634. doi: 10.1371/journal.pone.0025634
241. Gravina GL, Marampon F, Di Staso M, Bonfili P, Vitturini A, Jannini EA, et al. 5-Azacitidine Restores and Amplifies the Bicalutamide Response on Preclinical Models of Androgen Receptor Expressing or Deficient Prostate Tumors. Prostate (2010) 70(11):1166–78. doi: 10.1002/pros.21151
242. Graça I, Sousa EJ, Baptista T, Almeida M, Ramalho-Carvalho J, Palmeira C, et al. Anti-Tumoral Effect of the non-Nucleoside DNMT Inhibitor RG108 in Human Prostate Cancer Cells. Curr Pharm Design (2014) 20(11):1803–11. doi: 10.2174/13816128113199990516
243. Chou YW, Chaturvedi NK, Ouyang S, Lin FF, Kaushik D, Wang J, et al. Histone Deacetylase Inhibitor Valproic Acid Suppresses the Growth and Increases the Androgen Responsiveness of Prostate Cancer Cells. Cancer Lett (2011) 311(2):177–86. doi: 10.1016/j.canlet.2011.07.015
244. Stultz J, Fong L. How to Turn Up the Heat on the Cold Immune Microenvironment of Metastatic Prostate Cancer. Prostate Cancer Prostatic Dis (2021) 24(3):697–717. doi: 10.1038/s41391-021-00340-5
245. Powles T, Yuen KC, Gillessen S, Kadel EE, Rathkopf D, Matsubara N, et al. Atezolizumab With Enzalutamide Versus Enzalutamide Alone in Metastatic Castration-Resistant Prostate Cancer: A Randomized Phase 3 Trial. Nat Med (2022) 28(1):144–53. doi: 10.1038/s41591-021-01600-6
246. Graff JN, Beer TM, Alumkal JJ, Slottke RE, Redmond WL, Thomas GV, et al. A Phase II Single-Arm Study of Pembrolizumab With Enzalutamide in Men With Metastatic Castration-Resistant Prostate Cancer Progressing on Enzalutamide Alone. J ImmunoTher Cancer (2020) 8(2):e000642. doi: 10.1136/jitc-2020-000642
247. Hansen AR, Massard C, Ott PA, Haas NB, Lopez JS, Ejadi S, et al. Pembrolizumab for Advanced Prostate Adenocarcinoma: Findings of the KEYNOTE-028 Study. Ann Oncol (2018) 29(8):1807–13. doi: 10.1093/annonc/mdy232
248. Graff JN, Alumkal JJ, Drake CG, Thomas GV, Redmond WL, Farhad M, et al. Early Evidence of Anti-PD-1 Activity in Enzalutamide-Resistant Prostate Cancer. Oncotarget (2016) 7(33):52810–7. doi: 10.18632/oncotarget.10547
249. Antonarakis ES, Piulats JM, Gross-Goupil M, Goh J, Ojamaa K, Hoimes CJ, et al. Pembrolizumab for Treatment-Refractory Metastatic Castration-Resistant Prostate Cancer: Multicohort, Open-Label Phase II KEYNOTE-199 Study. J Clin Oncol (2020) 38(5):395–405. doi: 10.1200/JCO.19.01638
250. Yu EY, Kolinsky MP, Berry WR, Retz M, Mourey L, Piulats JM, et al. Pembrolizumab Plus Docetaxel and Prednisone in Patients With Metastatic Castration-Resistant Prostate Cancer: Long-Term Results From the Phase 1b/2 KEYNOTE-365 Cohort B Study. Eur Urol (2022) S0302-2838(22)01665–7. doi: 10.1016/j.eururo.2022.02.023
251. Lin H, Liu Q, Zeng X, Yu W, Xu G. Pembrolizumab With or Without Enzalutamide in Selected Populations of Men With Previously Untreated Metastatic Castration-Resistant Prostate Cancer Harbouring Programmed Cell Death Ligand-1 Staining: A Retrospective Study. BMC Cancer (2021) 21(1):399. doi: 10.1186/s12885-021-08156-1
252. Kantoff PW, Higano CS, Shore ND, Berger ER, Small EJ, Penson DF, et al. Sipuleucel-T Immunotherapy for Castration-Resistant Prostate Cancer. N Engl J Med (2010) 363(5):411–22. doi: 10.1056/NEJMoa1001294
253. Kwon ED, Drake CG, Scher HI, Fizazi K, Bossi A, van denEertwegh AJ, et al. Ipilimumab Versus Placebo After Radiotherapy in Patients With Metastatic Castration-Resistant Prostate Cancer That had Progressed After Docetaxel Chemotherapy (CA184-043): A Multicentre, Randomised, Double-Blind, Phase 3 Trial. Lancet Oncol (2014) 15(7):700–12. doi: 10.1016/S1470-2045(14)70189-5
254. Beer TM, Kwon ED, Drake CG, Fizazi K, Logothetis C, Gravis G, et al. Randomized, Double-Blind, Phase III Trial of Ipilimumab Versus Placebo in Asymptomatic or Minimally Symptomatic Patients With Metastatic Chemotherapy-Naive Castration-Resistant Prostate Cancer. J Clin Oncol (2017) 35(1):40–7. doi: 10.1200/JCO.2016.69.1584
255. Tollefson M, Karnes RJ, Thompson RH, Granberg C, Hillman D, Breau R, et al. 668 A RANDOMIZED PHASE II STUDY OF IPILIMUMAB WITH ANDROGEN ABLATION COMPARED WITH ANDROGEN ABLATION ALONE IN PATIENTS WITH ADVANCED PROSTATE CANCER. J Urol (2010) 183(4S):e261–1. doi: 10.1016/j.juro.2010.02.1055
256. Slovin SF, Higano CS, Hamid O, Tejwani S, Harzstark A, Alumkal JJ, et al. Ipilimumab Alone or in Combination With Radiotherapy in Metastatic Castration-Resistant Prostate Cancer: Results From an Open-Label, Multicenter Phase I/II Study. Ann Oncol (2013) 24(7):1813–21. doi: 10.1093/annonc/mdt107
Keywords: prostate cancer, castration-resistant prostate cancer, therapy resistance, targeted therapies, epigenetics
Citation: Moreira-Silva F, Henrique R and Jerónimo C (2022) From Therapy Resistance to Targeted Therapies in Prostate Cancer. Front. Oncol. 12:877379. doi: 10.3389/fonc.2022.877379
Received: 16 February 2022; Accepted: 25 April 2022;
Published: 24 May 2022.
Edited by:
Bianca Nitzsche, Charité Universitätsmedizin Berlin, GermanyReviewed by:
Remi Adelaiye-Ogala, University at Buffalo, United StatesGuru Sonpavde, Dana–Farber Cancer Institute, United States
Copyright © 2022 Moreira-Silva, Henrique and Jerónimo. This is an open-access article distributed under the terms of the Creative Commons Attribution License (CC BY). The use, distribution or reproduction in other forums is permitted, provided the original author(s) and the copyright owner(s) are credited and that the original publication in this journal is cited, in accordance with accepted academic practice. No use, distribution or reproduction is permitted which does not comply with these terms.
*Correspondence: Carmen Jerónimo, carmenjeronimo@ipoporto.min-saude.pt