- Cancer Center, Union Hospital, Tongji Medical College, Huazhong University of Science and Technology, Wuhan, China
Breast cancer (BC) is the most common cancer in females and BC brain metastasis (BCBM) is considered as the second most frequent brain metastasis. Although the advanced treatment has significantly prolonged the survival in BC patients, the prognosis of BCBM is still poor. The management of BCBM remains challenging. Systemic treatments are important to maintain control of central nervous system disease and improve patients’ survival. BCBM medical treatment is a rapidly advancing area of research. With the emergence of new targeted drugs, more options are provided for the treatment of BM. This review features currently available BCBM treatment strategies and outlines novel drugs and ongoing clinical trials that may be available in the future. These treatment strategies are discovered to be more efficacious and potent, and present a paradigm shift in the management of BCBMs.
1 Introduction
In 2020, the incidence of breast cancer (BC) surpassed lung cancer and was ranked first, accounting for 11.7% of all cancer, with about 2.3 million new cases, worldwide (1). With the BC treatment development, the frequency of central nervous system (CNS) metastases has steadily increased because BC patients survive long enough to be at risk of developing CNS metastasis (2). About 7% of metastatic BC will develop brain metastases (BMs) (3), including parenchymal and leptomeningeal disease, accounting for roughly 17% of all BMs, and the second major cause of BMs after lung cancer (4, 5). Risk of BM is variable across BC subtypes. BM is majorly observed in triple-negative BC (TNBC) and human epidermal growth factor receptor 2 (HER2)-positive BC. In comparison with the hormone receptors (HR) positive subtype, the risk of BM development in HER2 positive and TNBC is 2-5 times higher (6, 7). Although the advanced BC treatment has remarkably prolonged the patient’s survival, the prognosis of BC brain metastasis (BCBM) is still substandard. The median patients’ survival in parenchymal and leptomeningeal disease was 3 to 23 and 3 to 4 months, respectively (8, 9). The survival time for HER2+ patients is reported to be the longest, while that of TNBC patients is considered the least (10). In a large multi-center study, the median overall survival (OS) after the diagnosis of BCBM with HR+/HER2+ was 18.9 months, with HR–/HER2+ was 13.1 months, with HR+/HER2– was 7.1 months and for triple-negative was 4.4 months (11). Due to the substandard prognosis and lack of efficient treatment strategies, BCBM represents a unique and challenging clinical problem.
The brain is the most complex and unique organ in the body, and because of this, there are differences in treatment. The brain microenvironment is composed of two different components, parenchyma and leptomeninges (12). The brain parenchyma comprises cells that are not present anywhere else in the body, which include astrocytes, oligodendrocytes, microglia, and neuronal cells. The leptomeninges is mainly filled with the circulating cerebrospinal fluid (CSF) produced by the choroid plexus (13). The blood-brain barrier (BBB) is the main gatekeeper of the CNS (13). BBB is a unique neurovascular unit consisting of a continuous segment of non-porous blood vessels and interacts with parietal, immune, glial, and nerve cells to carefully modulate the movement of ions, molecules, and cells between the brain and the blood (14, 15), thereby protecting the CNS from pathogens, toxins, injury, inflammation, and diseases, while also providing a barrier for drugs delivery to the brain (16). Another important factor that produces drug resistance during BM treatment is the BBB efflux transporter systems, which reversely transport and prevent drug penetration into CNS. These include P-glycoprotein, BC-resistance protein, multidrug resistance-associated proteins, etc. (17). The formation of metastatic tumors may partially disrupt the BBB, allowing it to become more permeable. However, it is still not sufficiently or homogeneously permeable for effective drug therapy (18).
Currently, the main treatment strategies for BCBM include local (surgical resection and radiotherapy) and systemic treatments (19). In the study by Minniti et al, it has been demonstrated that BM patients who received multifraction (3× 9 Gy) stereotactic radiosurgery after surgery had an improved local control rate of 91% at 12 months (20). Systemic treatments are important for controlling CNS diseases and improving patients’ survival (19). Although heavy literatures on BBB’s effect and anti-cancer drugs’ efficacy on BCBM is deficient, our knowledge about the effect of systemic treatments on BCBM is rapidly increasing. This review focuses on the currently available system treatment drugs for BCBM, such as chemotherapy, target therapy, endocrine therapy, immunotherapy, and novel therapies that may be available in the future (Figure 1).
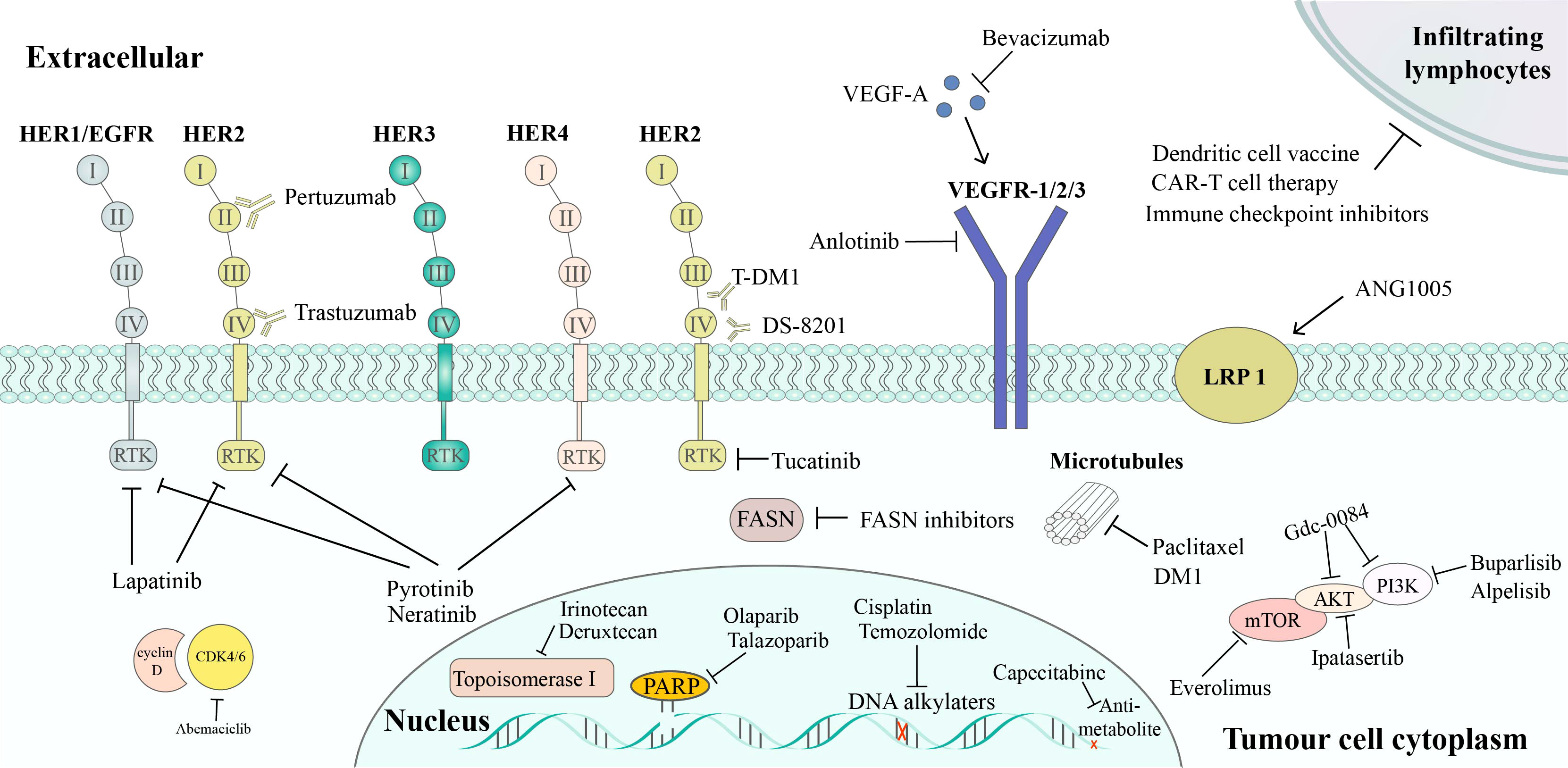
Figure 1 Targeted therapy of BCBM treatment. HER2 inhibitors includes: trastuzumab, which binds to subdomain IV of HER2, leading to the inhibition of HER2 signaling; pertuzumab, which binds to subdomain II of HER2, preventing HER2/HER3 dimerization; T-DM1, an ADC of trastuzumab and the cytotoxic agent DM1; DS-8201, another ADC that combines trastuzumab and deruxtecan, a potent topoisomerase I inhibitor; lapatinib, which is a reversible TKI of HER1 and HER2; tucatinib, a specific HER2 TKI; pyrotinib and neratinib, TKIs that inhibits HER1, HER2, and HER4. Pathway inhibitors include: PI3K/Akt/mTOR pathway inhibitors (buparlisib, alpelisib, Gdc-0084, ipatasertib, and everolimus); CDK4/6 inhibitors (abemaciclib), VEGF pathway inhibitors (anlotinib, bevacizumab). Other therapies include: PARP inhibitors, FASN inhibitors, chemotherapy, and immunotherapy.
2 Treatment for HER2-Positive BCBM
HER2+ BC accounts for approximately 20% of all BC cases (21). HER is a group of reversible tyrosine kinase receptors, comprising four members: epidermal growth factor receptor (EGFR)/HER1, HER2, HER3, and HER4. These have crucial functions in tumorigenesis and help tumor cells escape anti-tumor immunity. Anti-HER2-targeting drugs can inhibit the kinase activity of these HER receptors and prevent further cancer cell survival and proliferation (22). HER2-targeting drugs involve tyrosine kinase inhibitors (TKIs), monoclonal antibodies, and antibody-drug conjugates (ADCs). TKI is a small molecule and comprises lapatinib, neratinib, tukatinib, etc. have a considerably lower molecular weight, allowing them a more efficacious penetration through the BBB (21). Table 1 lists the characteristics of studies reporting on outcomes related to BMs in patients with HER2-positive BC.
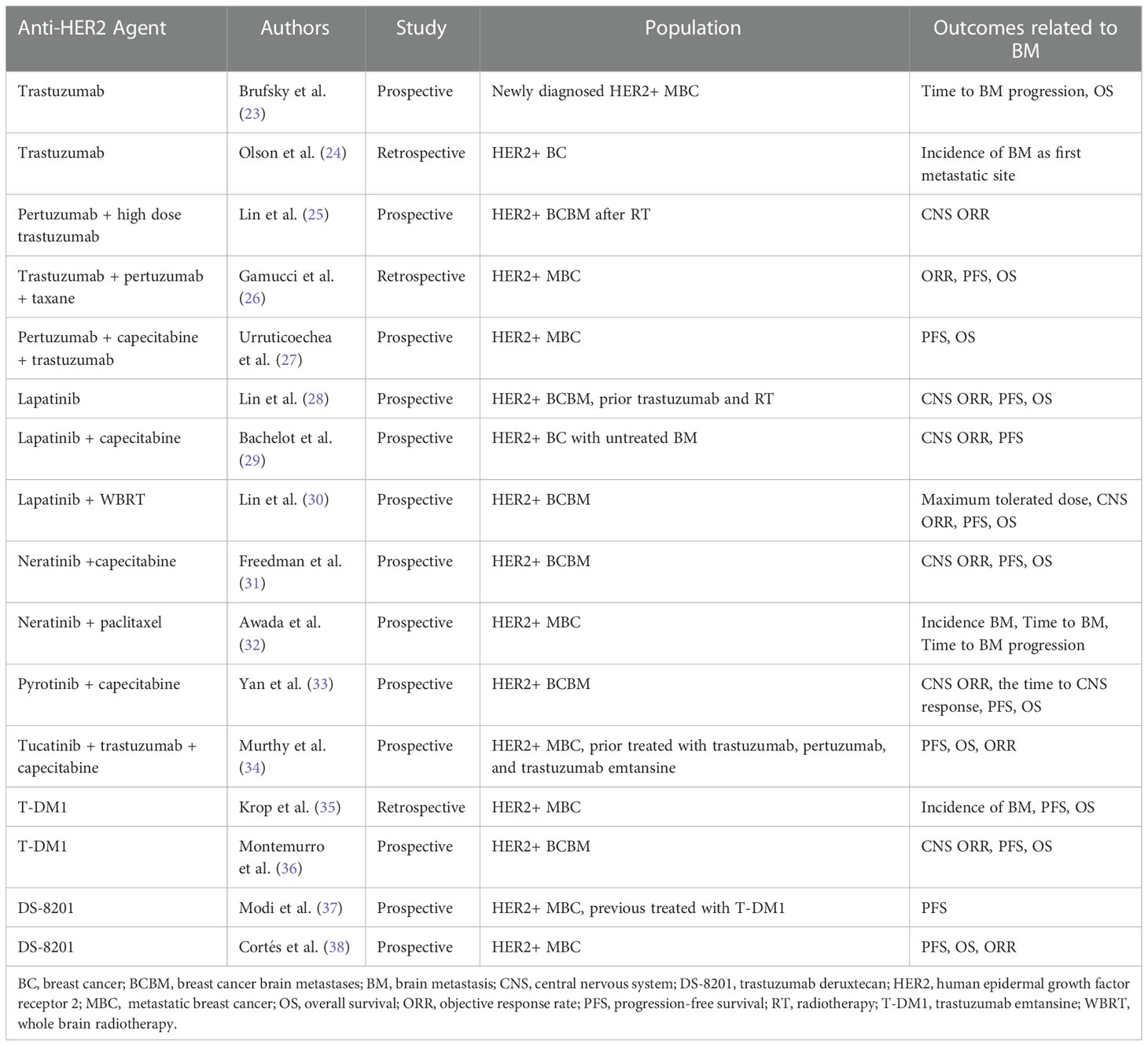
Table 1 Characteristics of studies reporting on outcomes related to BMs in patients with HER2-positive BC.
Monoclonal antibody drug, such as trastuzumab, was the first authorized targeted therapy against HER2+ BC. In the registHER study, trastuzumab treatment after CNS metastatic diagnosis significantly improved OS statistically (treatment vs. no trastuzumab treatment, 17.5 vs. 3.8 months) (23). Trastuzumab also has inconsistent responses in intracranial and extra-cranial tumors, as it cannot entirely cross the BBB to play a preventive role (39). A meta-analysis of HER2+ BC patients (n=9020) showed that CNS metastasis accounted for a greater proportion of the first relapse site in patients receiving trastuzumab treatment (24). Among HER2+ patients receiving trastuzumab adjuvant therapy, the CNS metastasis incidence as the first site of disease recurrence was 2.56% (95% CI 2.07%-3.01%) than in those who did not receive adjuvant trastuzumab 1.94% (95% CI 1.54%-2.38%) (24). Pertuzumab acts on a different HER2 site, and it prevents HER2/HER3 dimerization (40) which can lead to significant PI3K-Akt activation (main signaling pathway in BC cells) (41). When combined with trastuzumab, it can play a complementary role and provides efficient treatment for HER2+ BC patients. However, this dual-target therapy fails to show an advantage in BMs (42). In the phase II PATRICIA investigation, the CNS ORR for pertuzumab plus high-dose trastuzumab was 11% (95% CI 3-25) (25). In early studies, the trastuzumab–pertuzumab dual-target therapy combined with other agents indicated some activity in BCBM (26, 27, 43). A retrospective observational investigation which included 264 HER2+ BCBM patients revealed that dual HER2-blockade and taxanes treatment produced an ORR of 52.4% in baseline BM patients (26). In the phase III PHEREXA trial, after pertuzumab was added to capecitabine + trastuzumab, the PFS markedly elevated in the subgroup of HER2+ baseline BM patients (n = 53; HR 0.29, 95% CI 0.15–0.60) (27). All in all, trastuzumab and partuzumab, as macromolecular monoclonal antibodies, have limited their ability to pass the blood-brain barrier, but their combination with other chemotherapy drugs has increased their intracranial activity to some extent.
Lapatinib inhibits EGFR and HER2 and is authorized to be given with capecitabine as a combined therapy for metastatic BC (44). The CNS ORR of mono lapatinib therapy is approximately only 6% (28), while the CNS ORR of lapatinib + capecitabine in 13 BCBM patients who prior received trastuzumab and radiation therapy is 38% (95% CI, 13.9-68.4) (45). At the same time, a single-arm phase II clinical trial showed that in untreated BCBM patients, the CNS ORR of lapatinib + capecitabine could reach 65.9% (29). Lapatinib + capecitabine is also associated with less incidence of CNS at the first progression. A phase III randomized trial indicated that in comparison with capecitabine monotherapy, the CNS involvement cases at first progression were fewer (2% vs 6%, P = 0.045) in combined therapy (46). Lapatinib combined with fractionated radiotherapy may be useful against HER2+ BCBM in the tumor xenograft model (47). In a Phase I investigation, lapatinib with WBRT had a higher CNS ORR (79%) than that of WBRT alone (36%) in 28 BCBM patients (30).
Neratinib irreversibly inhibits HER2, HER1, and HER4 (48), and may pass through the intact BBB by inhibiting ATP-binding cassette B1 transport function which is one of the dominant efflux transporters in the BBB (49, 50). In a phase II trial of neratinib combined with capecitabine treatment in refractory HER2+ BCBM patients, 33% and 49% of the patients with and without previous lapatinib treatment achieved CNS ORR, respectively (31). In a randomized clinical trial of previously untreated metastatic HER2+ BC, 8.3% and 17% of patients in the neratinib + paclitaxel and trastuzumab + paclitaxel groups experienced symptomatic or progressive CNS recurrence, respectively (32), demonstrating its preventive effect on the CNS metastasis of BC.
Pyrotinib is an irreversible TKI that targets HER1, HER2, and HER4 (51). A phase II clinical trial (PERMEATE trial) showed the intracranial ORR was 74.6% in a radiation-naive population with pirotinib + capecitabine (cohort A) (n=59) and 42.1% in cohort B (n=19) included patients who had progressive disease after radiotherapy (33). The median PFS in cohort A was 11.3 months and 5.6 months in cohort B (33). PERMEATE research adds strong medical evidence for drug treatment of BM, especially for patients with new BM. However, the efficacy of this regimen still needs more randomized controlled trials for further verification.
Tucatinib is a selective HER2-targeting TKI, and has fewer side effects than other TKIs due to its lack of strong EGFR inhibition (10). In 2020, Tucatinib is authorized by the Food and Drug Administration (FDA) for HER2+ BCBM treatment. A randomized clinical trial (HER2CLIMB trail) was conducted where tucatinib was given with trastuzumab+ capecitabine as a combination therapy to patients who were initially treated for HER2+ metastasis BC. In BM patients, the estimated 1-year PFS in the tucatinib and placebo groups was 24.9% (95% CI, 16.5 to 34.3) and 0%, respectively. The median PFS in the tucatinib and placebo groups was 7.6 months (95% CI 6.2-9.5) and 5.4 months (95% CI 4.1-5.7), respectively. The CNS ORR was 40.6% (95% CI 35.3-46.0) in tucatinib group compared with 22.8% (95% CI 16.7-29.8) in placebo group (P<0.001). However, the side effects such as the incidences of diarrhea and hepatic injury (grade 3 or higher) were more frequent than those in the control group (34).
Trastuzumab emtansine (T-DM1), an ADC of trastuzumab and the cytotoxic drug emtansine, a maytansine derivative and microtubule inhibitor, has been aproved for the treatment of HER2+ metastatic BC patients after taxane and trastuzumab therapy, and for the adjuvant therapy of early BC HER2+ patients with the residual invasive disease after neo-adjuvant taxane and trastuzumab therapy (43, 52). In the EMILIA study, the patients with baseline CNS metastases had OS of 26.8 months in the T-DM1 arm, compared with 12.9 months in the capecitabine + lapatinib arm (HR=0.38, P=0.008). PFS in the two treatment arms was similar (5.9 vs. 5.7 months; P=1.0) (35). In a KAMILLA single-arm research, in the 126 measurable BCBM patients, CNS ORR was 21.4% (95% CI 14.6–29.6), whereas, in the 398 baselines BCBM patients, the median PFS and OS were 5.5 months (95% CI 5.3–5.6) and 18.9 (95% CI 17.1–21.3), respectively (36). In short, T-DM1 may be effective in treating HER2+ intracranial lesions.
Another ADC is trastuzumab deruxtecan (DS-8201), comprising a human HER2 antibody, a new enzyme-cleavable linker, and a topoisomerase I inhibitor payload. Compared with T-DM1, the antibody-drug ratio of DS-8201 is higher (8 vs 3–4) (37). The DESTINY-Breast01 trial, evaluating DS-8201 in HER2+ metastatic BC patients with previously treated with T-DM1, revealed a median PFS of 18.1 months (95% CI 6.7–18.1) in the BM subgroup (37). In DESTINY-Breast03, in the 82 BCBM patients, DS-8201 had better efficiency than T-DM1. The median PFS was 15.0 months (95%CI 12.5-22.2) in the DS-8201 group and 3.0 months (95%CI: 2.8-5.8) in the T-DM1 group (HR=0.25; 95%CI 0.31-0.45), and the ORR of DS-8201 group and T-DM1 group were 67.4% and 20.5%, respectively (38, 53).
To summarize, the available data indicate remarkable intracranial efficacy from treatments for HER2-positive BCBM, such as TKIs, monoclonal antibodies, and ADCs. However, some problems need to be solved, for example, treatment-induced diarrhea and hepatic injury may be limiting factors and require appropriate surveillance, and the optimal administration time and sequence of each agent remain uncertain.
3 Treatment for HR-Positive BCBM
The patients suffering from HR+ BC are less likely to develop BMs than patients with other subtypes of BC (6). The effects of hormone therapy specifically on BMs are still unclear (54). Concentrations of tamoxifen and its metabolites in the BM tumor and brain tissue were 46 times higher than those in serum, suggesting that tamoxifen might be clinically beneficial (55). Furthermore, in preclinical models, estrogen promoted BMs by altering polarity and suppressing the phagocytic activity of M2 microglia, whereas tamoxifen blocked its polarization and enhanced its phagocytic ability, thereby inhibiting BMs (56). Other hormone therapies, such as megestrol acetate and letrozole, only a small number of case reports have documented the responses of hormonal therapy in BCBM patients, and the efficacy has not been confirmed in large-sample clinical trials (54, 57–60).
The growth of HR+ BC cells depends on cyclin D1, which activates cyclin-dependent kinases 4 and 6 (CDK4 and CDK6), thereby inducing the G1-S phase transition and entering the cell cycle. CDK4/6 inhibitors are reliable treatment options for HR+ BC patients with extra-cranial diseases (10). In preclinical models, CDK4/6 inhibitor abemaciclib has better central permeability than other existing CDK4/6 inhibitors (61, 62), which can reach the therapeutic level in human BM (62). In the JPBO study, abemaciclib was given to HR+/HER2- BCBM patients, and the results showed that 25% of patients did achieve clinical benefit (CR, PR, or SD>6 months), but definite intracranial ORR was only 5.6% (63). The real effectiveness of CDK4/6 inhibitors in treating BMs is not verified yet.
Everolimus is a kinase that acts selectively to inhibit the mammalian target of rapamycin (mTOR), and can easily penetrate the CNS of a mouse model (64). However, the intracranial response in a phase II study of everolimus, trastuzumab, and vinorelbine for the treatment of progressive HER2+ BCBM was only 4% (65). But in the phase Ib/II trial assessing the effect of everolimus, lapatinib, and capecitabine combined therapy for HER2+ BCBM, the CNS ORR was 28% (66), highlighting the need for exploring better treatment modalities for everolimus.
4 Treatment for Triple-Negative BCBM
4.1 Chemotherapy
Lack of specific therapeutic targets, chemotherapy is the major treatment of TNBC. Chemotherapeutic agents used in BC treatment that can cross the blood brain barrier include capecitabine, platinum compounds, and temozolomide (10). But their efficacy is limited (67) and new chemotherapeutic drugs are still being actively explored.
Capecitabine is an inhibitor of thymidylate synthase (the enzyme required for DNA replication in metastatic cancerous cells) and has been traditionally used as a chemotherapeutic drug (68). Some studies showed that capecitabine or its metabolites may penetrate the BBB, and its efficacy in BCBM patients has been reported in the literature (69–71). Chao and colleagues assessed 873 BCBM patients’ data and reported that those who received chemotherapy survived 2.4 to 12.2 months longer than those who did not. Among patients with recurrent CNS cancer, those who received chemotherapy after local BCBM treatment also had a longer brain metastasizing time. The median OS of capecitabine alone in patients with BCBM was 11.8 months (72).
Cisplatin is a platinum-based drug that alkylates DNA by forming platinum-DNA adducts, which damages DNA, arrest G1/S phase, and promote apoptosis (73). In the in-vitro BBB model, cisplatin at concentrations from 5 µM to 20 µM was shown to reduce the chance of BMs in MDA-MB-231 cells (p<0.05) (74). A phase II investigation comprising 12 BCBM participants revealed that the CNS objective response rate (ORR) of bevacizumab, etoposide, and cisplatin was 75% (95%CI 42.8-94.5) and the median CNS progression-free survival (PFS) was 6.6 months (95% CI 0.8-12.4) (75).
Temozolomide is an oral alkylating agent and has the potential to transport across BBB. It is commonly used for glioma treatment and its efficacy in BC is not clear. However, recent large-scale clinical trials indicated that temozolomide alone or combined with radiotherapy has no clinical advantage (76). In a phase II clinical trial, temozolomide showed no objective responses in 18 patients with observable lesions who previously received extensive treatment (77). In a phase II investigation of whole-brain radiation therapy (WBRT) with or without concurrent temozolomide treatment for BCBM, WBRT combined temozolomide was not better than the WBRT group in ORR, PFS, and OS. At 6 weeks, ORRs for the WBRT arm were 36% and for the WBRT + temozolomide, the ORR was 30%. The median PFS and OS were 7.4 and 11.1 months in the WBRT arm, and 6.9 and 9.4 months in the WBRT + temozolomide arm, respectively (78).
Etirinotecan pegol (NKTR 102) is a four-armed polyethylene glycol polymer, with each arm ending with an irinotecan molecule (79). In its preclinical studies, compared to conventional irinotecan, NKTR 102 treatment can improve the survival rate of the TNBC brain metastatic model (80). In the BEACON trial (a phase III trial), BCBM patients who received NKTR-102 treatment had a markedly lowered death risk than those who received treatment of physician’s choice (TPC) (HR 0.51; P < 0.01), with median OS of 10.0 and 4.8 months, respectively (81). In ATTAIN trial, a phase III study of NKTR 102 versus TPC in metastatic BC patients (82), the median PFS in BM patients of NKTR 102 and TPC was 3.9 and 3.3 months, respectively (HR, 0.59; 95% CI, 0.33-1.05; P =0.07), and the median OS in both the groups was nearly the same (7.8 months for NKTR 102, and 7.5 months for TPC group; HR=0.90; 95% CI, 0.61-1.33; P =0 .60) (83). The ATTAIN randomized clinical trial was not consistent with the positive OS benefit observed in BEACON trial (81, 83).
Sacituzumab govitecan (SG) is an ADC comprising an anti-trophoblast cell-surface antigen 2 (Trop-2) antibody bound with SN-38, an active topoisomerase I inhibitor irinotecan metabolite, capable of crossing BBB (84, 85). Trop-2 is a transmembrane calcium signal transducer greatly expressed in BC (85). In a phase III ASCENT investigation of SG versus TPC for metastatic TNBC, the subgroup assessment of stable BMs patients (n=61) revealed that the median PFS and OS for SG were 2.8 and 6.8 months while these were 1.6 and 7.5 months for TPC, respectively. ORR for SG and TPC was 3% and 0%, respectively (86). Suggesting that about ORR and PFS, SG was better than TPC but not OS.
A new taxane derivative, ANG1005 comprises 3 paclitaxel molecules that are covalently bound with Angiopep-2. It can pass through the BBB and enter the malignant cells by lipoprotein receptor-related protein 1 transport system. ANG1005 has shown a significant CNS efficiency in a phase II clinical trial with an overall intracranial ORR of 15%, intracranial clinical benefit rate (CBR) of 68% in all BCBM patients. The median intracranial PFS and OS were 2.8 months and 7.8 months (87).
In a word, the efficacy of single chemotherapeutic drug was unsatisfactory, and the efficacy of combined chemotherapy was objective (Table 2).
4.2 Vascular Endothelial Growth Factor -A-targeting Monoclonal Antibody
Brain metastatic tumors can utilize the host’s vascular system to make blood vessels abnormal and torturing. Angiogenesis inhibitors can remodel and normalize tumor vascular and can play a crucial part in the treatment of BMs (94). In preclinical mouse models of BCBMs, the vascular endothelial growth factor (VEGF) -A-targeting monoclonal antibody bevacizumab, have been associated with improved OS (94, 95). Two phase II clinical trials have investigated bevacizumab combined with platinum chemotherapy in patients with BCBMs that have progressed after WBRT, with CNS ORR of 63-77% and median OS of 10.5-14.1 months (96, 97). Additionally, bevacizumab also controls intracranial edema. According to a retrospective research, bevacizumab’s edema control rate in the BCBM group was 77.14% (98).
4.3 Poly (ADP-ribose) polymerase inhibitors
Homologous recombination is an error-free mechanism for repairing double-strand DNA breaks, and poly (ADP-ribose) polymerase (PARP) inhibitors promote apoptosis of tumor cells by inhibiting DNA homologous recombination. Mutations in the BC susceptibility gene 1 (BRCA1) or BC susceptibility gene 2 (BRCA2), make tumors sensitive to PARP inhibitor therapy (99, 100). About half of patients with BRCA1 or BRCA2 mutations develop BMs in the late stage of BC (101). Olaparib and talazoparib are the PARP inhibitors that are authorized for the treatment of brca1 and brca2-related metastatic BC (10). In the phase III EMBRACA clinical trial, talazoparib notably improved PFS and ORR than that in the TPC group. In the subgroup of BM patients, the benefit of PFS was even higher than that of patients without BM (HR 0.32 vs. HR 0.58) (102), which suggested its possible effect on BCBM.
5 Potential strategies for the treatment of BCBM
5.1 Immunotherapy
Generally, there are no lymphocytes in the healthy brain parenchyma, which belongs to the immune-privileged site, but human BMs have been confirmed to have obvious T cell infiltration (103). Retrospective research revealed that more than 90% of tumor-infiltrating lymphocytes (TIL) were present in BCBM and its microenvironment (104). The density of TILs infiltrate is related to the size of peritumoral edema and survival prognosis, and CD8+ T cells can delay intracranial progression (103, 105). Taggart et al. demonstrated that in a mouse melanoma BM model, successful immunotherapy is associated with increased CD8+ T cell trafficking (106). Monoclonal antibodies, such as antibodies of programmed death-1 (PD1), programmed death ligand-1(PD-L1), or cytotoxic T-lymphocyte antigen 4 (CTLA4), can block immune checkpoints, and has showed moderate overall response rates in BM patients, especially in melanoma patients (107). The retrospective and prospective clinical trials showed that intracranial response rates were 16–25% after ipilimumab treatment in melanoma patients, however, these studies are very small in patient number (108, 109). More clinical studies are expected to confirm the role of immunotherapy in BCBM in the future.
5.2 Small molecule Vascular Endothelial Growth Factor Receptor tyrosine inhibitor
Anlotinib hydrochloride is a multi-target TKI drug that is administered orally and inhibits tumor angiogenesis and tumor cell growth by suppressing tumor-related kinases, such as TKI receptor, VEGFR 1 to 3, fibroblast growth factor receptor 1 to 4, EGFR, platelet-derived growth factor receptor α, and β, and stem cell factor receptor (110, 111). In the randomized ALTER 0303 clinical trial (a phase III trial) of advanced non-small cell lung cancer patients, in those with baseline BM, the median PFS was 4.17 months for anlotinib treatment than 1.3 months for placebo treatment (HR=0.72; 95% CI 0.15-0.56), and the median OS after anlotinib therapy was 8.57 months than 4.55 months observed after placebo therapy (HR=0.72; 95% CI 0.42–1.12) (112). Apatinib similar to anlotinib, is a small molecule that taken orally and selectively targets VEGFR-2 (113). In a phase II PATHER2 single-arm research on patients with non-small cell lung cancer, in those with baseline BM patients (n=13), the ORR was 53.8% (95% CI, 25.1-80.8%), and the median PFS was 6.7 (95% CI, 4.1-9.7) months (113). Anlotinib and apatinib have shown good efficacy in BMs of lung cancer, and more clinical data are expected to verify these efficacies in BCBM.
5.3 Novel therapy
In addition, there are many novel therapies being explored, including the phosphoinositide 3-kinase (PI3K)/Akt/mTOR pathway inhibitors, fatty acid synthase (FASN) inhibitors and the drugs with new delivery systems.
The PI3K/Akt/mTOR pathway is a key intracellular signaling pathway that activates tyrosine kinase receptors or G protein-coupled receptors through extracellular signals. It promotes many physiological processes such as survival, proliferation, metabolism, and angiogenesis (114). About 43-70% of BCBM patients have mutations in this pathway (115). In a mouse model of extensively metastasized HER2+ BC, buparlisib, an oral pan-PI3K inhibitor, effectively controlled the metastasis in various organs, including the brain (116). Alpelisib is another PI3K inhibition, which is reported to be effective in BCBM in some case reports (117). Ipatasertib, one of the selective ATP-competitive Akt inhibitors, shows an effective response in preclinical BCBM models (115). The mTOR, a downstream effector of the PI3K-Akt pathway, has been indicated to modulate PI3K inhibition resistance in BC, whereas, the combination of PI3K and mTOR inhibitors can overcome this resistance in a HER2+ BCBM model (118). Gdc-0084 is a dual PI3K/mTOR inhibitor with brain permeability, which has been proven to significantly inhibit PIK3CA mutant tumor growth in a patient-derived BM mouse model (119). Relevant clinical trials are underway.
The brain tissues are deficient in several nutrients necessary for cancer cells. Ferraro et al. found up-regulated fatty acid synthesis genes in BMs with a mouse model, whereas no effect was seen in in-vitro BM system, suggesting that the brain microenvironment itself promotes increased tumor fatty acid synthesis. Compared with primary breast tumors or metastasis to other sites, FASN and its encoding mRNA are highly expressed in BCBM. The use of FASN inhibitors can reduce the growth of BCBMs (120).
Since BBB may theoretically hinder the delivery of drugs to the brain, some drug delivery systems are being developed to cross BBB. One such system that is being tested is microbubble-assisted focused ultrasound (FUS), which uses oscillating microbubbles to produce micrometer-scale mechanofluidic effects to increase drug transport. When trastuzumab was combined with FUS, they revealed a potent anticancer activity in the rat brain, and also increased survival significantly (121). Another system being investigated is nanoparticles conjugated anticancer agents (122). Patil et al. demonstrated that the EGFR or HER2 inhibitors carried by nano-conjugates markedly prolong the survival of mice with HER2+ BCBM (122). Hamilton et al. also revealed that tumor-penetrating peptides coated nanoparticles can block tumor progression when tested in a preclinical BCBM model (123).
Furthermore, except the potential strategies mentioned above, there are a lot of novel treatments, such as dendritic cell vaccines, chimeric antigen receptor T-cell therapy, and so on. Table 3 reveals the ongoing clinical trials of BCBM. In order to improve the efficacy, new therapeutic strategies are the combinations of immunotherapy, radiotherapy and targeted therapies, and the corresponding side effects also follow. We look forward to having a treatment scheme that is most beneficial to patients among the controllable side effects.
6 Future prospects
In the future, compared with the systemic treatment of patients with BM, prevention of BM from the primary tumors seems to be a more important clinical goal. How to accurately screen the high-risk population with BM from BC patients requires more clinical research to explore a reliable prediction model. With the rise of liquid biopsy technology represented by circulating tumor cells (CTCs), it provides strong support for the detection of circulating brain-tropic cancer cells before their extravasation (114). The gene signature of CTCs associated with BCBM has revealed the up-regulation of Notch signaling (124). Notch targeted therapy may specifically reduce the incidence of BM in BC.
7 Conclusions
BM is an important clinical source of morbidity and mortality in patients with metastatic BC (43). Local interventions are the pillar of BM management (19). Systemic treatments are often used to complement local strategies to achieve optimal control of CNS diseases (19). At present, with the emergence of various new drugs, some systematic therapies have shown promising clinical results. The best results published to date have been obtained in patients with HER2+ BCBM, especially in new BM, for whom currently used combinations of chemotherapy and anti-HER2 therapy have shown certain efficacy, with particularly impressive results obtained with pirotinib + capecitabine and trastuzumab deruxtecan. However, patients with BM from HR+ BC or TNBC lack effective medical options currently, PI3K inhibitors, FASN inhibitors and immunotherapy are promising therapeutic candidates. In the coming years, the results of ongoing clinical trials with combinations of immunotherapy, radiotherapy and targeted therapies may provide better treatment options for BCBM patients.
Author contributions
Conceptualization, YZ. Writing – original draft, QC and JX. Writing – review and editing. QC, JX, YM and JW. Supervision, CL and YZ. All authors contributed to the article and approved the submitted version.
Funding
This work was supported by National Natural Science Foundation of China (No. 81402197) and Natural Science Foundation of Hubei (No. 2019CFB501).
Conflict of interest
The authors declare that the research was conducted in the absence of any commercial or financial relationships that could be construed as a potential conflict of interest.
Publisher’s note
All claims expressed in this article are solely those of the authors and do not necessarily represent those of their affiliated organizations, or those of the publisher, the editors and the reviewers. Any product that may be evaluated in this article, or claim that may be made by its manufacturer, is not guaranteed or endorsed by the publisher.
References
1. Sung H, Ferlay J, Siegel RL, Laversanne M, Soerjomataram I, Jemal A, et al. Global cancer statistics 2020: Globocan estimates of incidence and mortality worldwide for 36 cancers in 185 countries. CA: Cancer J Clin (2021) 71(3):209–49. doi: 10.3322/caac.21660
2. Kuksis M, Gao Y, Tran W, Hoey C, Kiss A, Komorowski AS, et al. The incidence of brain metastases among patients with metastatic breast cancer: A systematic review and meta-analysis. Neuro-oncology (2021) 23(6):894–904. doi: 10.1093/neuonc/noaa285
3. Vogelbaum MA, Brown PD, Messersmith H, Brastianos PK, Burri S, Cahill D, et al. Treatment for brain metastases: Asco-Sno-Astro guideline. Neuro-Oncology (2021) 40(5):492-516. doi: 10.1093/neuonc/noab262
4. Nieder C, Spanne O, Mehta MP, Grosu AL, Geinitz H. Presentation, patterns of care, and survival in patients with brain metastases: What has changed in the last 20 years? Cancer (2011) 117(11):2505–12. doi: 10.1002/cncr.25707
5. Witzel I, Oliveira-Ferrer L, Pantel K, Müller V, Wikman H. Breast cancer brain metastases: Biology and new clinical perspectives. Breast Cancer Res (2016) 18(1):1–9. doi: 10.1186/s13058-015-0665-1
6. Kennecke H, Yerushalmi R, Woods R, Cheang MCU, Voduc D, Speers CH, et al. Metastatic behavior of breast cancer subtypes. J Clin Oncol (2010) 28(20):3271–7. doi: 10.1200/JCO.2009.25.9820
7. Achrol AS, Rennert RC, Anders C, Soffietti R, Ahluwalia MS, Nayak L, et al. Brain metastases. Nat Rev Dis Primers (2019) 5(1):1–26. doi: 10.1038/s41572-018-0055-y
8. Le Rhun E, Preusser M, van den Bent M, Andratschke N, Weller M. How we treat patients with leptomeningeal metastases. ESMO Open (2019) 4:e000507. doi: 10.1136/esmoopen-2019-000507
9. Lin NU, Amiri-Kordestani L, Palmieri D, Liewehr DJ, Steeg PS. Cns metastases in breast cancer: Old challenge, new frontiers. Clin Cancer Res (2013) 19(23):6404–18. doi: 10.1158/1078-0432.CCR-13-0790
10. Soffietti R, Ahluwalia M, Lin N, Rudà R. Management of brain metastases according to molecular subtypes. Nat Rev Neurol (2020) 16(10):557–74. doi: 10.1038/s41582-020-0391-x
11. Darlix A, Louvel G, Fraisse J, Jacot W, Brain E, Debled M, et al. Impact of breast cancer molecular subtypes on the incidence, kinetics and prognosis of central nervous system metastases in a Large multicentre real-life cohort. Br J Cancer (2019) 121(12):991–1000. doi: 10.1038/s41416-019-0619-y
12. Valiente M, Ahluwalia MS, Boire A, Brastianos PK, Goldberg SB, Lee EQ, et al. The evolving landscape of brain metastasis. Trends Cancer (2018) 4(3):176–96. doi: 10.1016/j.trecan.2018.01.003
13. Boire A, Brastianos PK, Garzia L, Valiente M. Brain metastasis. Nat Rev Cancer (2020) 20(1):4–11. doi: 10.1038/s41568-019-0220-y
14. Zlokovic BV. The blood-brain barrier in health and chronic neurodegenerative disorders. Neuron (2008) 57(2):178–201. doi: 10.1016/j.neuron.2008.01.003
15. Daneman R. The blood–brain barrier in health and disease. Ann Neurol (2012) 72(5):648–72. doi: 10.1002/ana.23648
16. Daneman R, Prat A. The blood–brain barrier. Cold Spring Harbor Perspect Biol (2015) 7(1):a020412. doi: 10.1101/cshperspect.a020412
17. Gomez-Zepeda D, Taghi M, Scherrmann J-M, Decleves X, Menet M-C. Abc transporters at the blood–brain interfaces, their study models, and drug delivery implications in gliomas. Pharmaceutics (2019) 12(1):20. doi: 10.3390/pharmaceutics12010020
18. Do J, Foster D, Renier C, Vogel H, Rosenblum S, Doyle TC, et al. Ex vivo Evans blue assessment of the blood brain barrier in three breast cancer brain metastasis models. Breast Cancer Res Treat (2014) 144(1):93–101. doi: 10.1007/s10549-014-2854-5
19. Le Rhun E, Guckenberger M, Smits M, Dummer R, Bachelot T, Sahm F, et al. Eano–esmo clinical practice guidelines for diagnosis, treatment and follow-up of patients with brain metastasis from solid tumours☆. Ann Oncol (2021) 32(11):1332–47. doi: 10.1016/j.annonc.2021.07.016
20. Minniti G, Scaringi C, Paolini S, Lanzetta G, Romano A, Cicone F, et al. Single-fraction versus multifraction (3× 9 gy) stereotactic radiosurgery for Large (> 2 Cm) brain metastases: A comparative analysis of local control and risk of radiation-induced brain necrosis. Int J Radiat Oncol Biol Phys (2016) 95(4):1142–8. doi: 10.1016/j.ijrobp.2016.03.013
21. Duchnowska R, Loibl S, Jassem J. Tyrosine kinase inhibitors for brain metastases in Her2-positive breast cancer. Cancer Treat Rev (2018) 67:71–7. doi: 10.1016/j.ctrv.2018.05.004
22. Costa R, Carneiro B, Wainwright D, Santa-Maria C, Kumthekar P, Chae Y, et al. Developmental therapeutics for patients with breast cancer and central nervous system metastasis: Current landscape and future perspectives. Ann Oncol (2017) 28(1):44–56. doi: 10.1093/annonc/mdw532
23. Brufsky AM, Mayer M, Rugo HS, Kaufman PA, Tan-Chiu E, Tripathy D, et al. Central nervous system metastases in patients with Her2-positive metastatic breast cancer: Incidence, treatment, and survival in patients from registher. Clin Cancer Res (2011) 17(14):4834–43. doi: 10.1158/1078-0432.CCR-10-2962
24. Olson E, Abdel-Rasoul M, Maly J, Wu C, Lin N, Shapiro C. Incidence and risk of central nervous system metastases as site of first recurrence in patients with Her2-positive breast cancer treated with adjuvant trastuzumab. Ann Oncol (2013) 24(6):1526–33. doi: 10.1093/annonc/mdt036
25. Lin NU, Pegram M, Sahebjam S, Ibrahim N, Fung A, Cheng A, et al. Pertuzumab plus high-dose trastuzumab in patients with progressive brain metastases and Her2-positive metastatic breast cancer: Primary analysis of a phase ii study. J Clin Oncol (2021) 39(24):2667–75. doi: 10.1200/JCO.20.02822
26. Gamucci T, Pizzuti L, Natoli C, Mentuccia L, Sperduti I, Barba M, et al. A multicenter retrospective observational study of first-line treatment with pertuzumab, trastuzumab and taxanes for advanced Her2 positive breast cancer patients. Reper Study Cancer Biol Ther (2019) 20(2):192–200. doi: 10.1080/15384047.2018.1523095
27. Urruticoechea A, Rizwanullah M, Im S-A, Ruiz ACS, Láng I, Tomasello G, et al. Randomized phase iii trial of trastuzumab plus capecitabine with or without pertuzumab in patients with human epidermal growth factor receptor 2–positive metastatic breast cancer who experienced disease progression during or after trastuzumab-based therapy. J Clin Oncol (2017) 35(26):3030–8. doi: 10.1200/JCO.2016.70.6267
28. Lin NU, Diéras V, Paul D, Lossignol D, Christodoulou C, Stemmler H-J, et al. Multicenter phase ii study of lapatinib in patients with brain metastases from Her2-positive breast cancer. Clin Cancer Res (2009) 15(4):1452–9. doi: 10.1158/1078-0432.CCR-08-1080
29. Bachelot T, Romieu G, Campone M, Diéras V, Cropet C, Dalenc F, et al. Lapatinib plus capecitabine in patients with previously untreated brain metastases from Her2-positive metastatic breast cancer (Landscape): A single-group phase 2 study. Lancet Oncol (2013) 14(1):64–71. doi: 10.1016/S1470-2045(12)70432-1
30. Lin NU, Freedman RA, Ramakrishna N, Younger J, Storniolo AM, Bellon JR, et al. A phase I study of lapatinib with whole brain radiotherapy in patients with human epidermal growth factor receptor 2 (Her2)-positive breast cancer brain metastases. Breast Cancer Res Treat (2013) 142(2):405–14. doi: 10.1007/s10549-013-2754-0
31. Stirrups R. Neratinib and capecitabine for breast cancer brain metastases. Lancet Oncol (2019) 20(4):e197. doi: 10.1016/S1470-2045(19)30184-6
32. Awada A, Colomer R, Inoue K, Bondarenko I, Badwe RA, Demetriou G, et al. Neratinib plus paclitaxel vs trastuzumab plus paclitaxel in previously untreated metastatic Erbb2-positive breast cancer: The nefert-T randomized clinical trial. JAMA Oncol (2016) 2(12):1557–64. doi: 10.1001/jamaoncol.2016.0237
33. Yan M, Ouyang Q, Sun T, Niu L, Yang J, Li L, et al. Pyrotinib plus capecitabine for patients with human epidermal growth factor receptor 2-positive breast cancer and brain metastases (Permeate): A multicentre, single-arm, two-cohort, phase 2 trial. Lancet Oncol (2022) 23(3):353–61. doi: 10.1016/S1470-2045(21)00716-6
34. Murthy RK, Loi S, Okines A, Paplomata E, Hamilton E, Hurvitz SA, et al. Tucatinib, trastuzumab, and capecitabine for Her2-positive metastatic breast cancer. New Engl J Med (2020) 382(7):597–609. doi: 10.1056/NEJMoa1914609
35. Krop I, Lin N, Blackwell K, Guardino E, Huober J, Lu M, et al. Trastuzumab emtansine (T-Dm1) versus lapatinib plus capecitabine in patients with Her2-positive metastatic breast cancer and central nervous system metastases: A retrospective, exploratory analysis in Emilia. Ann Oncol (2015) 26(1):113–9. doi: 10.1093/annonc/mdu486
36. Montemurro F, Delaloge S, Barrios C, Wuerstlein R, Anton A, Brain E, et al. Trastuzumab emtansine (T-Dm1) in patients with Her2-positive metastatic breast cancer and brain metastases: Exploratory final analysis of cohort 1 from kamilla, a single-arm phase iiib clinical trial☆. Ann Oncol (2020) 31(10):1350–8. doi: 10.1016/j.annonc.2020.06.020
37. Modi S, Saura C, Yamashita T, Park YH, Kim S-B, Tamura K, et al. Trastuzumab deruxtecan in previously treated Her2-positive breast cancer. New Engl J Med (2020) 382(7):610–21. doi: 10.1056/NEJMoa1914510
38. Cortés J, Kim S-B, Chung W-P, Im S-A, Park YH, Hegg R, et al. Trastuzumab deruxtecan versus trastuzumab emtansine for breast cancer. New Engl J Med (2022) 386(12):1143–54. doi: 10.1056/NEJMoa2115022
39. Dagogo-Jack I, Gill CM, Cahill DP, Santagata S, Brastianos PK. Treatment of brain metastases in the modern genomic era. Pharmacol Ther (2017) 170:64–72. doi: 10.1016/j.pharmthera.2016.10.011
40. Harbeck N, Beckmann MW, Rody A, Schneeweiss A, Müller V, Fehm T, et al. Her2 dimerization inhibitor pertuzumab-mode of action and clinical data in breast cancer. Breast Care (2013) 8(1):49–55. doi: 10.1159/000346837
41. Lee-Hoeflich ST, Crocker L, Yao E, Pham T, Munroe X, Hoeflich KP, et al. A central role for Her3 in Her2-amplified breast cancer: Implications for targeted therapy. Cancer Res (2008) 68(14):5878–87. doi: 10.1158/0008-5472.CAN-08-0380
42. Swain SM, Baselga J, Kim S-B, Ro J, Semiglazov V, Campone M, et al. Pertuzumab, trastuzumab, and docetaxel in Her2-positive metastatic breast cancer. New Engl J Med (2015) 372(8):724–34. doi: 10.1056/NEJMoa1413513
43. Garcia-Alvarez A, Papakonstantinou A, Oliveira M. Brain metastases in Her2-positive breast cancer: Current and novel treatment strategies. Cancers (2021) 13(12):2927. doi: 10.3390/cancers13122927
44. Pedrosa RM, Mustafa DA, Soffietti R, Kros JM. Breast cancer brain metastasis: Molecular mechanisms and directions for treatment. Neuro-oncology (2018) 20(11):1439–49. doi: 10.1093/neuonc/noy044
45. Lin NU, Eierman W, Greil R, Campone M, Kaufman B, Steplewski K, et al. Randomized phase ii study of lapatinib plus capecitabine or lapatinib plus topotecan for patients with Her2-positive breast cancer brain metastases. J neuro-oncol (2011) 105(3):613–20. doi: 10.1007/s11060-011-0629-y
46. Cameron D, Casey M, Press M, Lindquist D, Pienkowski T, Romieu CG, et al. A phase iii randomized comparison of lapatinib plus capecitabine versus capecitabine alone in women with advanced breast cancer that has progressed on trastuzumab: Updated efficacy and biomarker analyses. Breast Cancer Res Treat (2008) 112(3):533–43. doi: 10.1007/s10549-007-9885-0
47. Sambade MJ, Kimple RJ, Camp JT, Peters E, Livasy CA, Sartor CI, et al. Lapatinib in combination with radiation diminishes tumor regrowth in Her2+ and basal-Like/Egfr+ breast tumor xenografts. Int J Radiat Oncol Biol Phys (2010) 77(2):575–81. doi: 10.1016/j.ijrobp.2009.12.063
48. Bose R, Kavuri SM, Searleman AC, Shen W, Shen D, Koboldt DC, et al. Activating Her2 mutations in Her2 gene amplification negative breast cancer. Cancer Discovery (2013) 3(2):224–37. doi: 10.1158/2159-8290.CD-12-0349
49. Zhao X-q, Xie J-d, Chen X-g, Sim HM, Zhang X, Liang Y-j, et al. Neratinib reverses atp-binding cassette B1-mediated chemotherapeutic drug resistance in vitro, in vivo, and ex vivo. Mol Pharmacol (2012) 82(1):47–58. doi: 10.1124/mol.111.076299
50. de Gooijer MC, Buil L, Beijnen JH, van Tellingen O. Atp-binding cassette transporters limit the brain penetration of Wee1 inhibitors. Invest New Drugs (2018) 36(3):380–7. doi: 10.1007/s10637-017-0539-8
51. Zhu Y, Li L, Zhang G, Wan H, Yang C, Diao X, et al. Metabolic characterization of pyrotinib in humans by ultra-performance liquid Chromatography/Quadrupole time-of-Flight mass spectrometry. J Chromatogr B (2016) 1033:117–27. doi: 10.1016/j.jchromb.2016.08.009
52. Von Minckwitz G, Huang C-S, Mano MS, Loibl S, Mamounas EP, Untch M, et al. Trastuzumab emtansine for residual invasive Her2-positive breast cancer. New Engl J Med (2019) 380(7):617–28. doi: 10.1056/NEJMoa1814017
53. Jacobson A. Trastuzumab deruxtecan improves progression-free survival and intracranial response in patients with Her2-positive metastatic breast cancer and brain metastases. Oncol (2022) 27(Supplement_1):S3–4. doi: 10.1093/oncolo/oyac009
54. Liu MC, Cortés J, O’Shaughnessy J. Challenges in the treatment of hormone receptor-positive, Her2-negative metastatic breast cancer with brain metastases. Cancer Metastasis Rev (2016) 35(2):323–32. doi: 10.1007/s10555-016-9619-z
55. Lien EA, Wester K, Lønning P, Solheim E, Ueland P. Distribution of tamoxifen and metabolites into brain tissue and brain metastases in breast cancer patients. Br J Cancer (1991) 63(4):641–5. doi: 10.1038/bjc.1991.147
56. Wu S-Y, Sharma S, Wu K, Tyagi A, Zhao D, Deshpande RP, et al. Tamoxifen suppresses brain metastasis of estrogen receptor-deficient breast cancer by skewing microglia polarization and enhancing their immune functions. Breast Cancer Res (2021) 23(1):1–16. doi: 10.1186/s13058-021-01412-z
57. Madhup R, Kirti S, Bhatt M, Srivastava P, Srivastava M, Kumar S. Letrozole for brain and scalp metastases from breast cancer–a case report. Breast (2006) 15(3):439–41. doi: 10.1016/j.breast.2005.07.006
58. Stewart DJ, Dahrouge S. Response of brain metastases from breast cancer to megestrol acetate: A case report. J neuro-oncol (1995) 24(3):299–301. doi: 10.1007/BF01052847
59. Wang Q, Sun B, Liu C, Shi S, Ding L, Liu J, et al. Brain metastases from breast cancer may respond to endocrine therapy: Report of two cases. OncoTargets Ther (2019) 12:1389. doi: 10.2147/OTT.S188143
60. Salvati M, Cervoni L, Innocenzi G, Bardella L. Prolonged stabilization of multiple and single brain metastases from breast cancer with tamoxifen. Rep Three Cases Tumori J (1993) 79(5):359–62. doi: 10.1177/030089169307900516
61. Raub TJ, Wishart GN, Kulanthaivel P, Staton BA, Ajamie RT, Sawada GA, et al. Brain exposure of two selective dual Cdk4 and Cdk6 inhibitors and the antitumor activity of Cdk4 and Cdk6 inhibition in combination with temozolomide in an intracranial glioblastoma xenograft. Drug Metab Dispos (2015) 43(9):1360–71. doi: 10.1124/dmd.114.062745
62. Sahebjam S, Le Rhun E, Kulanthaivel P, Turner PK, Klise S, Wang HT, et al. Assessment of concentrations of abemaciclib and its major active metabolites in plasma, csf, and brain tumor tissue in patients with brain metastases secondary to hormone receptor positive (Hr+) breast cancer. Am Soc Clin Oncol (2016) 34(15):526. doi: 10.1200/JCO.2016.34.15_suppl.526
63. Anders CK, Le Rhun E, Bachelot TD, Yardley DA, Awada A, Conte PF, et al. A phase ii study of abemaciclib in patients (Pts) with brain metastases (Bm) secondary to hr+, Her2-metastatic breast cancer (Mbc). Am Soc Clin Oncol (2019) 37(15):1017. doi: 10.1200/JCO.2019.37.15_suppl.1017
64. Meikle L, Pollizzi K, Egnor A, Kramvis I, Lane H, Sahin M, et al. Response of a neuronal model of tuberous sclerosis to mammalian target of rapamycin (Mtor) inhibitors: Effects on Mtorc1 and akt signaling lead to improved survival and function. J Neurosci (2008) 28(21):5422–32. doi: 10.1523/JNEUROSCI.0955-08.2008
65. Van Swearingen AE, Siegel MB, Deal AM, Sambade MJ, Hoyle A, Hayes DN, et al. Lccc 1025: A phase ii study of everolimus, trastuzumab, and vinorelbine to treat progressive Her2-positive breast cancer brain metastases. Breast Cancer Res Treat (2018) 171(3):637–48. doi: 10.1007/s10549-018-4852-5
66. Hurvitz S, Singh R, Adams B, Taguchi JA, Chan D, Dichmann RA, et al. Phase Ib/Ii single-arm trial evaluating the combination of everolimus, lapatinib and capecitabine for the treatment of Her2-positive breast cancer with brain metastases (Trio-us b-09). Ther Adv Med Oncol (2018) 10:1758835918807339. doi: 10.1177/1758835918807339
67. Corti C, Antonarelli G, Criscitiello C, Lin NU, Carey LA, Cortés J, et al. Targeting brain metastases in breast cancer. Cancer Treat Rev (2021) 103:102324. doi: 10.1016/j.ctrv.2021.102324
68. Fares J, Kanojia D, Rashidi A, Ulasov I, Lesniak MS. Landscape of combination therapy trials in breast cancer brain metastasis. Int J Cancer (2020) 147(7):1939–52. doi: 10.1002/ijc.32937
69. Mata JF, García-Manteiga JM, Lostao MP, Fernández-Veledo S, Guillén-Gómez E, Larrayoz IM, et al. Role of the human concentrative nucleoside transporter (Hcnt1) in the cytotoxic action of 5 [Prime]-Deoxy-5-Fluorouridine, an active intermediate metabolite of capecitabine, a novel oral anticancer drug. Mol Pharmacol (2001) 59(6):1542–8. doi: 10.1124/mol.59.6.1542
70. Ekenel M, Hormigo AM, Peak S, DeAngelis LM, Abrey LE. Capecitabine therapy of central nervous system metastases from breast cancer. J neuro-oncol (2007) 85(2):223–7. doi: 10.1007/s11060-007-9409-0
71. Wang ML, Yung WA, Royce ME, Schomer DF, Theriault RL. Capecitabine for 5-Fluorouracil-Resistant brain metastases from breast cancer. Am J Clin Oncol (2001) 24(4):421–4. doi: 10.1097/00000421-200108000-00026
72. Gao C, Wang F, Suki D, Strom E, Li J, Sawaya R, et al. Effects of systemic therapy and local therapy on outcomes of 873 breast cancer patients with metastatic breast cancer to brain: Md Anderson cancer center experience. Int J Cancer (2021) 148(4):961–70. doi: 10.1002/ijc.33243
73. Wang H, Guo S, Kim S-J, Shao F, Ho JWK, Wong KU, et al. Cisplatin prevents breast cancer metastasis through blocking early emt and retards cancer growth together with paclitaxel. Theranostics (2021) 11(5):2442. doi: 10.7150/thno.46460
74. Augustine R, Zahid AA, Mraiche F, Alam K, Al Moustafa A-E, Hasan A. Gelatin-methacryloyl hydrogel based in vitro blood–brain barrier model for studying breast cancer-associated brain metastasis. Pharm Dev Technol (2021) 26(4):490–500. doi: 10.1080/10837450.2021.1872624
75. Lu Y-S, Chen W-W, Lin C-H, Tseng L-M, Yeh D-C, Wu P-F, et al. Bevacizumab, etoposide, and cisplatin (Beep) in brain metastases of breast cancer progressing from radiotherapy: Results of the first stage of a multicenter phase ii study. Am Soc Clin Oncol (2012) 30(15):1079. doi: 10.1200/jco.2012.30.15_suppl.1079
76. Tian J, Luo Y, Xiang J, Tang J. Combined treatment for non-small cell lung cancer and breast cancer patients with brain metastases with whole brain radiotherapy and temozolomide: A systematic review and meta-analysis. J Neuro-Oncol (2017) 135(2):217–27. doi: 10.1007/s11060-017-2572-z
77. Trudeau M, Crump M, Charpentier D, Yelle L, Bordeleau L, Matthews S, et al. Temozolomide in metastatic breast cancer (Mbc): A phase ii trial of the national cancer institute of Canada–clinical trials group (Ncic-ctg). Ann Oncol (2006) 17(6):952–6. doi: 10.1093/annonc/mdl056
78. Cao K, Lebas N, Gerber S, Levy C, Le Scodan R, Bourgier C, et al. Phase ii randomized study of whole-brain radiation therapy with or without concurrent temozolomide for brain metastases from breast cancer. Ann Oncol (2015) 26(1):89–94. doi: 10.1093/annonc/mdu488
79. Adkins CE, Nounou MI, Hye T, Mohammad AS, Terrell-Hall T, Mohan NK, et al. Nktr-102 efficacy versus irinotecan in a mouse model of brain metastases of breast cancer. BMC Cancer (2015) 15(1):1–11. doi: 10.1186/s12885-015-1672-4
80. Shah N, Mohammad A, Adkins C, Dolan E, Griffith J, Jagannathan R, et al. Etirinotecan pegol: Survival advantage over standard of care drugs in a model of brain metastases of breast cancer. Cancer Res (2017) 77(4 Supplement):P1-12-05. doi: 10.1158/1538-7445.SABCS16-P1-12-05
81. Cortés J, Rugo HS, Awada A, Twelves C, Perez EA, Im SA, et al. Prolonged survival in patients with breast cancer and a history of brain metastases: Results of a preplanned subgroup analysis from the randomized phase iii beacon trial. Breast Cancer Res Treat (2017) 165(2):329–41. doi: 10.1007/s10549-017-4304-7
82. Tripathy D, Tolaney SM, Seidman AD, Anders CK, Ibrahim N, Rugo HS, et al. Attain: Phase iii study of etirinotecan pegol versus treatment of physician's choice in patients with metastatic breast cancer and brain metastases. Future Oncol (2019) 15(19):2211–25. doi: 10.2217/fon-2019-0180
83. Tripathy D, Tolaney SM, Seidman AD, Anders CK, Ibrahim N, Rugo HS, et al. Treatment with etirinotecan pegol for patients with metastatic breast cancer and brain metastases: Final results from the phase 3 attain randomized clinical trial. JAMA Oncol (2022) 8(7):1047–52. doi: 10.1001/jamaoncol.2022.4346
84. Bardia A, Hurvitz SA, Tolaney SM, Loirat D, Punie K, Oliveira M, et al. Sacituzumab govitecan in metastatic triple-negative breast cancer. New Engl J Med (2021) 384(16):1529–41. doi: 10.1056/NEJMoa2028485
85. Goldenberg DM, Cardillo TM, Govindan SV, Rossi EA, Sharkey RM. Trop-2 is a novel target for solid cancer therapy with sacituzumab govitecan (Immu-132), an antibody-drug conjugate (Adc). Oncotarget (2015) 6(26):22496. doi: 10.18632/oncotarget.4318
86. Diéras V, Weaver R, Tolaney SM, Bardia A, Punie K, Brufsky A, et al. Abstract Pd13-07: Subgroup analysis of patients with brain metastases from the phase 3 ascent study of sacituzumab govitecan versus chemotherapy in metastatic triple-negative breast cancer. Cancer Res (2021) 81(4_Supplement):PD13–07-PD13-07. doi: 10.1158/1538-7445.Sabcs20-pd13-07
87. Kumthekar P, Tang S-C, Brenner AJ, Kesari S, Piccioni DE, Anders C, et al. Ang1005, a brain-penetrating peptide–drug conjugate, shows activity in patients with breast cancer with leptomeningeal carcinomatosis and recurrent brain metastases. Clin Cancer Res (2020) 26(12):2789–99. doi: 10.1158/1078-0432.CCR-19-3258
88. Franciosi V, Cocconi G, Michiara M, Di Costanzo F, Fosser V, Tonato M, et al. Front-line chemotherapy with cisplatin and etoposide for patients with brain metastases from breast carcinoma, nonsmall cell lung carcinoma, or malignant melanoma: A prospective study. Cancer (1999) 85(7):1599–605. doi: 10.1002/(SICI)1097-0142(19990401)85:7<1599::AID-CNCR23>3.0.CO;2-#
89. Christodoulou C, Bafaloukos D, Linardou H, Aravantinos G, Bamias A, Carina M, et al. Temozolomide (Tmz) combined with cisplatin (Cddp) in patients with brain metastases from solid tumors: A Hellenic cooperative oncology group (Hecog) phase ii study. J neuro-oncol (2005) 71(1):61–5. doi: 10.1007/s11060-004-9176-0
90. Erten C, Demir L, Somali I, Alacacioglu A, Kucukzeybek Y, Akyol M, et al. Cisplatin plus gemcitabine for treatment of breast cancer patients with brain metastases: A preferential option for triple negative patients? Asian Pac J Cancer Prev (2013) 14(6):3711–7. doi: 10.7314/APJCP.2013.14.6.3711
91. Cassier PA, Ray-Coquard I, Sunyach MP, Lancry L, Guastalla JP, Ferlay C, et al. A phase 2 trial of whole-brain radiotherapy combined with intravenous chemotherapy in patients with brain metastases from breast cancer. Cancer (2008) 113(9):2532–8. doi: 10.1002/cncr.23858
92. Anders C, Deal AM, Abramson V, Liu MC, Storniolo AM, Carpenter JT, et al. Tbcrc 018: Phase ii study of iniparib in combination with irinotecan to treat progressive triple negative breast cancer brain metastases. Breast Cancer Res Treat (2014) 146(3):557–66. doi: 10.1007/s10549-014-3039-y
93. Mehta MP, Wang D, Wang F, Kleinberg L, Brade A, Robins HI, et al. Veliparib in combination with whole brain radiation therapy in patients with brain metastases: Results of a phase 1 study. J neuro-oncol (2015) 122(2):409–17. doi: 10.1007/s11060-015-1733-1
94. Voutouri C, Kirkpatrick ND, Chung E, Mpekris F, Baish JW, Munn LL, et al. Experimental and computational analyses reveal dynamics of tumor vessel cooption and optimal treatment strategies. Proc Natl Acad Sci (2019) 116(7):2662–71. doi: 10.1073/pnas.1818322116
95. Kodack DP, Chung E, Yamashita H, Incio J, Duyverman AM, Song Y, et al. Combined targeting of Her2 and Vegfr2 for effective treatment of Her2-amplified breast cancer brain metastases. Proc Natl Acad Sci (2012) 109(45):E3119–E27. doi: 10.1073/pnas.1216078109
96. Lu Y-S, Chen TW-W, Lin C-H, Yeh D-C, Tseng L-M, Wu P-F, et al. Bevacizumab preconditioning followed by etoposide and cisplatin is highly effective in treating brain metastases of breast cancer progressing from whole-brain radiotherapy. Clin Cancer Res (2015) 21(8):1851–8. doi: 10.1158/1078-0432.CCR-14-2075
97. Leone JP, Emblem KE, Weitz M, Gelman RS, Schneider BP, Freedman RA, et al. Phase ii trial of carboplatin and bevacizumab in patients with breast cancer brain metastases. Breast Cancer Res (2020) 22(1):1–12. doi: 10.1186/s13058-020-01372-w
98. Bai X, Zhang Y, Ding W, Wang S. Efficacy of bevacizumab in the treatment of refractory brain edema of metastatic tumors from different sources. Neurol Res (2021) 43(12):955–60. doi: 10.1080/01616412.2021.1948740
99. Mirza MR, Monk BJ, Herrstedt J, Oza AM, Mahner S, Redondo A, et al. Niraparib maintenance therapy in platinum-sensitive, recurrent ovarian cancer. New Engl J Med (2016) 375(22):2154–64. doi: 10.1056/NEJMoa1611310
100. Robson M, Im S-A, Senkus E, Xu B, Domchek SM, Masuda N, et al. Olaparib for metastatic breast cancer in patients with a germline brca mutation. New Engl J Med (2017) 377(6):523–33. doi: 10.1056/NEJMoa1706450
101. Song Y, Barry WT, Seah DS, Tung NM, Garber JE, Lin NU. Patterns of recurrence and metastasis in Brca1/Brca2-associated breast cancers. Cancer (2020) 126(2):271–80. doi: 10.1002/cncr.32540
102. Litton JK, Rugo HS, Ettl J, Hurvitz SA, Gonçalves A, Lee K-H, et al. Talazoparib in patients with advanced breast cancer and a germline brca mutation. New Engl J Med (2018) 379(8):753–63. doi: 10.1056/NEJMoa1802905
103. Galea I, Bechmann I, Perry VH. What is immune privilege (Not)? Trends Immunol (2007) 28(1):12–8. doi: 10.1016/j.it.2006.11.004
104. Duchnowska R, Pęksa R, Radecka B, Mandat T, Trojanowski T, Jarosz B, et al. Immune response in breast cancer brain metastases and their microenvironment: The role of the pd-1/Pd-L axis. Breast Cancer Res (2016) 18(1):1–11. doi: 10.1186/s13058-016-0702-8
105. Berghoff AS, Fuchs E, Ricken G, Mlecnik B, Bindea G, Spanberger T, et al. Density of tumor-infiltrating lymphocytes correlates with extent of brain edema and overall survival time in patients with brain metastases. Oncoimmunology (2016) 5(1):e1057388. doi: 10.1080/2162402X.2015.1057388
106. Taggart D, Andreou T, Scott KJ, Williams J, Rippaus N, Brownlie RJ, et al. Anti–Pd-1/Anti–Ctla-4 efficacy in melanoma brain metastases depends on extracranial disease and augmentation of Cd8+ T cell trafficking. Proc Natl Acad Sci (2018) 115(7):E1540–E9. doi: 10.1073/pnas.1714089115
107. Schulz M, Salamero-Boix A, Niesel K, Alekseeva T, Sevenich L. Microenvironmental regulation of tumor progression and therapeutic response in brain metastasis. Front Immunol (2019) 10:1713. doi: 10.3389/fimmu.2019.01713
108. Ajithkumar T, Parkinson C, Fife K, Corrie P, Jefferies S. Evolving treatment options for melanoma brain metastases. Lancet Oncol (2015) 16(13):e486–e97. doi: 10.1016/S1470-2045(15)00141-2
109. Margolin K. Ipilimumab in a phase ii trial of melanoma patients with brain metastases. Oncoimmunology (2012) 1(7):1197–9. doi: 10.4161/onci.20687
110. He Z, Liu J, Ma Y, Jiang H, Cui Z, Wang G, et al. Anlotinib combined with cranial radiotherapy for non-small cell lung cancer patients with brain metastasis: A retrospectively, control study. Cancer Manage Res (2021) 13:6101. doi: 10.2147/CMAR.S319650
111. Han B, Li K, Wang Q, Zhang L, Shi J, Wang Z, et al. Effect of anlotinib as a third-line or further treatment on overall survival of patients with advanced non–small cell lung cancer: The alter 0303 phase 3 randomized clinical trial. JAMA Oncol (2018) 4(11):1569–75. doi: 10.1001/jamaoncol.2018.3039
112. Jiang S, Liang H, Liu Z, Zhao S, Liu J, Xie Z, et al. The impact of anlotinib on brain metastases of non-small cell lung cancer: Post hoc analysis of a phase iii randomized control trial (Alter0303). oncol (2020) 25(5):e870–e4. doi: 10.1634/theoncologist.2019-0838
113. Yang G, Xu H, Yang Y, Zhang S, Xu F, Hao X, et al. Pyrotinib combined with apatinib for targeting metastatic non-small cell lung cancer with Her2 alterations: A prospective, open-label, single-arm phase 2 study (Pather2). BMC Med (2022) 20(1):1–9. doi: 10.1186/s12916-022-02470-6
114. Hosonaga M, Saya H, Arima Y. Molecular and cellular mechanisms underlying brain metastasis of breast cancer. Cancer Metastasis Rev (2020) 39(3):711–20. doi: 10.1007/s10555-020-09881-y
115. Ippen FM, Grosch JK, Subramanian M, Kuter BM, Liederer BM, Plise EG, et al. Targeting the Pi3k/Akt/Mtor pathway with the pan-akt inhibitor gdc-0068 in Pik3ca-mutant breast cancer brain metastases. Neuro-oncology (2019) 21(11):1401–11. doi: 10.1093/neuonc/noz105
116. Nanni P, Nicoletti G, Palladini A, Croci S, Murgo A, Ianzano ML, et al. Multiorgan metastasis of human her-2+ breast cancer in Rag2–/–; Il2rg–/– mice and treatment with Pi3k inhibitor. PloS One (2012) 7(6):e39626. doi: 10.1371/journal.pone.0039626
117. Batalini F, Moulder SL, Winer EP, Rugo HS, Lin NU, Wulf GM. Response of brain metastases from Pik3ca-mutant breast cancer to alpelisib. JCO Precis Oncol (2020) 4:PO.19.00403. doi: 10.1200/PO.19.00403
118. Ni J, Ramkissoon SH, Xie S, Goel S, Stover DG, Guo H, et al. Combination inhibition of Pi3k and Mtorc1 yields durable remissions in mice bearing orthotopic patient-derived xenografts of Her2-positive breast cancer brain metastases. Nat Med (2016) 22(7):723–6. doi: 10.1038/nm.4120
119. Ippen FM, Alvarez-Breckenridge CA, Kuter BM, Fink AL, Bihun IV, Lastrapes M, et al. The dual Pi3k/Mtor pathway inhibitor gdc-0084 achieves antitumor activity in Pik3ca-mutant breast cancer brain metastasesdual Pi3k/Mtor inhibition in breast cancer brain metastases. Clin Cancer Res (2019) 25(11):3374–83. doi: 10.1158/1078-0432.CCR-18-3049
120. Ferraro GB, Ali A, Luengo A, Kodack DP, Deik A, Abbott KL, et al. Fatty acid synthesis is required for breast cancer brain metastasis. Nat Cancer (2021) 2(4):414–28. doi: 10.1038/s43018-021-00183-y
121. Park E-J, Zhang Y-Z, Vykhodtseva N, McDannold N. Ultrasound-mediated blood-Brain/Blood-Tumor barrier disruption improves outcomes with trastuzumab in a breast cancer brain metastasis model. J Controlled Release (2012) 163(3):277–84. doi: 10.1016/j.jconrel.2012.09.007
122. Patil R, Ljubimov AV, Gangalum PR, Ding H, Portilla-Arias J, Wagner S, et al. Mri virtual biopsy and treatment of brain metastatic tumors with targeted nanobioconjugates: Nanoclinic in the brain. ACS nano (2015) 9(5):5594–608. doi: 10.1021/acsnano.5b01872
123. Hamilton AM, Aidoudi-Ahmed S, Sharma S, Kotamraju VR, Foster PJ, Sugahara KN, et al. Nanoparticles coated with the tumor-penetrating peptide irgd reduce experimental breast cancer metastasis in the brain. J Mol Med (2015) 93(9):991–1001. doi: 10.1007/s00109-015-1279-x
Keywords: breast cancer, brain metastasis, systemic treatment, targeted treatment, endocrine treatment
Citation: Chen Q, Xiong J, Ma Y, Wei J, Liu C and Zhao Y (2023) Systemic treatments for breast cancer brain metastasis. Front. Oncol. 12:1086821. doi: 10.3389/fonc.2022.1086821
Received: 01 November 2022; Accepted: 16 December 2022;
Published: 06 January 2023.
Edited by:
Min Yi, University of Texas MD Anderson Cancer Center, United StatesCopyright © 2023 Chen, Xiong, Ma, Wei, Liu and Zhao. This is an open-access article distributed under the terms of the Creative Commons Attribution License (CC BY). The use, distribution or reproduction in other forums is permitted, provided the original author(s) and the copyright owner(s) are credited and that the original publication in this journal is cited, in accordance with accepted academic practice. No use, distribution or reproduction is permitted which does not comply with these terms.
*Correspondence: Cuiwei Liu, cuiweiliu19870620@163.com; Yanxia Zhao, sophia7781@126.com
†These authors have contributed equally to this work and share first authorship