- 1Department of Immunology, Institute of Basic Medical Sciences, Chinese Academy of Medical Sciences, School of Basic Medicine, Peking Union Medical College, Beijing, China
- 2Collaborative Innovation Center for Biotherapy, Institute of Basic Medical Sciences, Chinese Academy of Medical Sciences, School of Basic Medicine, Peking Union Medical College, Beijing, China
- 3Immunology Research Center, National Health Research Institutes, Zhunan, Taiwan
Increasing evidence reveals that breast cancer stem cells (BCSCs) subtypes with distinct properties are regulated by their abnormal metabolic changes; however, the specific molecular mechanism and its relationship with tumor microenvironment (TME) are not clear. In this study, we explored the mechanism of lactate dehydrogenase A (LDHA), a crucial glycolytic enzyme, in maintaining cancer stemness and BCSCs plasticity, and promoting the interaction of BCSCs with tumor associated macrophages (TAMs). Firstly, the expression of LDHA in breast cancer tissues was much higher than that in adjacent tissues and correlated with the clinical progression and prognosis of breast cancer patients based on The Cancer Genome Atlas (TCGA) data set. Moreover, the orthotopic tumor growth and pulmonary metastasis were remarkable inhibited in mice inoculated with 4T1-shLdha cells. Secondly, the properties of cancer stemness were significantly suppressed in MDA-MB-231-shLDHA or A549-shLDHA cancer cells, including the decrease of ALDH+ cells proportion, the repression of sphere formation and cellular migration, and the reduction of stemness genes (SOX2, OCT4, and NANOG) expression. However, the proportion of ALDH+ cells (epithelial-like BCSCs, E-BCSCs) was increased and the proportion of CD44+ CD24− cells (mesenchyme-like BCSCs, M-BCSCs) was decreased after LDHA silencing, suggesting a regulatory role of LDHA in E-BCSCs/M-BCSCs transformation in mouse breast cancer cells. Thirdly, the expression of epithelial marker E-cadherin, proved to interact with LDHA, was obviously increased in LDHA-silencing cancer cells. The recruitment of TAMs and the secretion of CCL2 were dramatically reduced after LDHA was knocked down in vitro and in vivo. Taken together, LDHA mediates a vicious cycle of mutual promotion between BCSCs plasticity and TAMs infiltration, which may provide an effective treatment strategy by targeting LDHA for breast cancer patients.
Introduction
Breast cancer stem cells (BCSCs), a small population of tumor cells with self-renewal ability and differentiation potential, have been proposed as a driving force in breast cancer initiation and dissemination (1, 2). Substantial evidence indicates that BCSCs are not homogeneous but compose of stem cells with different phenotypes and functions, which is known as BCSCs heterogeneity (3, 4). At present, BCSCs are mainly divided into two subtypes: one is mesenchymal, quiescent type marked by CD44+/CD24− (M-BCSCs); the other is epithelial, proliferative type marked by ALDH+ (E-BCSCs) (5). During tumor progression, BCSCs convert between quiescent mesenchymal-like and proliferative epithelial-like states to acquire specific phenotypes stimulated by their niche cells, ultimately cause tumor relapse and metastasis. Therefore, intensive study on BCSCs heterogeneity may provide a more precise strategy to targeting BCSCs for breast cancer therapy.
Cancer cells exhibit high glycolysis even in the presence of sufficient oxygen, which is known as Warburg effect (6). This abnormal metabolism promotes proliferation and survival of tumor cells with elevated glucose uptake and lactate production, and agents targeting glycolysis may offer a therapeutic opportunity in clinical practice (7–9). Interestingly, recent work has shown that distinct BCSCs states (M- and E-BCSCs) response markedly different to the antiglycolytic treatment (10). Lactate dehydrogenase A (LDHA), a class of 2-hydroxy acid oxidoreductase, mediates the conversion of lactate from pyruvate, NADH from NAD+ in the last step of glycolysis (11). Intensive studies documenting that elevated LDHA has been associated with the progression of aggressive cancers in a variety of tumor types and different responses to LDHA targeted therapy are shown in clinical settings (12–14). Although LDHA is indicated to have an essential role in survival and proliferation of tumor cells, relatively little is known about the mechanisms of it on regulating stemness and BCSCs heterogeneity.
Tumor-associated macrophages (TAMs) account for 30% to 50% of all infiltrating inflammatory cells in the tumor microenvironment of breast cancer, which have significant impact on the tumor occurrence, development, and metastasis (15–18). Stemness-related properties of BCSCs, for example self-renewal, drug-resistance and metastasis initiation are intensively supported by their suitable niche and dynamic interplay between BCSCs and TAMs is one of the most important manner (19). However, the potential molecular link between BCSCs metabolism and tumor immune evasion is not well established in breast cancer. In the present study, using orthotopic syngeneic immunocompetent mouse model, we investigate the fundamental role of LDHA, a metabolic enzyme, in BCSCs maintenance and TAMs recruitment. We demonstrate a fundamental role of LDHA in negative regulation of epithelial marker E-cadherin during E/M-BCSCs transition, and increased tumoral infiltration of CCL2-responding TAMs.
Materials and Methods
Cell Lines and Reagents
MDA-MB-231, 293T, 4T1, and RAW264.7 were obtained from ATCC. All cell lines were cultured according to guidelines from ATCC. The medium was supplemented with 10% FBS (ThermoFisher Scientific, Cat. No. 10099-141) and 100 U/ml penicillin-streptomycin (ThermoFisher Scientific, Cat. No. 15140122). Bone marrow–derived macrophage (BMDM) was extracted from femur of 6- to 8-week-old female Balb/c mice and cultured with RPMI 1640 (Biological Industries, Cat. No. 01-100-1A, containing 30 ng/ml M-CSF (Peprotech, 315-02) for 6 days.
Animal Experiments
The animal study was reviewed and approved by the Ethics Review Committee of Peking Union Medical College. Six- to 8-week-old female Balb/c mice were provided from the Institute of Basic Medical Science of Peking Union Medical College. 105 4T1 cells resuspended in 100-μl PBS were injected into the fourth mammary fat pad of each mouse and treated with oxamate or vehicle after tumor formation. The tumor size was measured and recorded on the 7th day after injection. On day 21 after injection, tumor, lung, and spleen in each mouse were harvested for tested by flow cytometry, hematoxylin, and eosin (H&E) staining and immunohistochemical (IHC) analyses.
Western Blotting
The cells were lysed with protein extraction reagent (ThermoFisher Scientific, USA) and total protein was harvested after centrifuging at 14,000g for 10 min. The concentration of protein was measured by NanoDrop (ThermoFisher Scientific, USA). After being separated in Bis-Tris Gels (180-8008H, Tanon), total protein was transferred to 0.22-μm PVDF membranes (1620177, Bio-Rad), which were then incubated in 5% bovine serum albumin (BSA) for 1 h. Primary antibodies against LDHA (2012, CST), OCT4 (2840, CST) were used to incubate the membranes at 4°C overnight. After washing, the membranes were incubated with secondary antibodies (goat anti-rabbit IgG-HRP; 40295, Bioss) for 1 h and then detected using high-sig ECL Western Blotting Substrate (180-5001, Tanon). The bandings were quantified by ImageJ software.
RNA Experiments
Total RNA was extracted by using Trizol reagent (ThermoFisher Scientific, USA) and 1 μg of total RNA was used for the synthesis of first-strand cDNA with HiScript II Q RT SuperMix for qPCR (+gDNA wiper) reagent (R223-01, Vazyme). Real-time PCR was performed with ChamQ SYBR qPCR Master Mix Reagent (Q311-02, Vazyme) and detected by CFX96 Real-Time PCR Detection System (Bio-Rad).
Generation of Stable Cancer Cell Lines
Mouse shNC and shLdha PLKO.1 plasmid were designed and produced by Beijing Yimeiang Biotechnology Co. Ltd (sequence shLdha 1, 5′-CCGGGUUCCCAGUUAAGUCGUAUAACUCGAGUUAUACGACUUAACUGGGAACUUUUUG-3′; sequence shLdha 2, 5′-CCGGCGUCUCCCUGAAGUCUCUUAACUCGAGUUAAGAGACUUCAGGGAGACGUUUUUG-3′; sequence shLdha 3, 5′-CCGGCGUGAACAUCUUCAAGUUCAUCUCGAGAUGAACUUGAAGAUGUUCACGUUUUUG-3′). The plasmids were amplified by transformed into E. coli and then extracted with TIANprep Mini Plasmid Kit (DP106, TIANGEN). Objective plasmids and packaging plasmids were co-transfected into 293T cells by Lipofectamine™ 2000 Transfection Reagent (ThermoFisher Scientific, USA) and lentiviruses produced by 293T cells were collected. After infection with lentiviruses and 1-week puromycin treatment, stable cancer cell lines were generated successfully.
Cell Migration Assay
2 × 106 cells/ml tumor cells resuspended in 100 μl RPMI1640 (containing 1% FBS) were seeded into 8-μm pore polycarbonate filters, and complete medium (containing 10% FBS) was added into the bottom well. After 16 h culture, the non-migratory cells on the top of the filters were removed by cotton swab, and the migratory cells on the bottom of filters were dyed with 0.1% crystal violet for 10 min. The crystal violet could be eluted by 33% acetic acid, and the absorbance value of eluent was read out by Elisa (Biotec) at the wavelength of 570 nm.
Flow Cytometry
The ALDH+ cells were detected by using ALDEFLUOR assay kit (01700, Stem Cell). 5 × 105 cells were suspended in ALDH assay buffer containing substrate and then incubated at 37°C for 40 min with or without diethylaminobenzaldehyde (DEAB) reagent. The CD44+CD24− CSCs were detected by using CD44-PE and CD24-APC antibodies incubated with tumor cells for 30 min at 4°C from light. Macrophages in tumor tissues were marked by CD45+F4/80+CD11b+ after single cell preparation. Suspensions of single cells were detected by flow cytometer (Millipore, Guava 5HT), and the data were analyzed by FlowJo software.
Tumorsphere Formation Assay
Tumor cells were suspended in Mammocult medium (Stem Cell, Canada) at appropriate concentration and added into ultra-low plates (Corning, USA). The tumorspheres (sphere diameter ≧70 μm) were counted after 5 to 7 days of culture.
ELISA
Culture supernatants of tumor cells was collected after centrifugation, and the concentration of CCL2 was measured by Mouse Uncoated ELISA Kit (88-7391-88, ThermoFisher Scientific, USA) according to providing protocol.
Bioinformatics Analysis
From the GDC of TCGA, the clinical information and LDHA sequencing data of breast cancer patients were downloaded and sorted into a matrix. Patients were divided into tumor and paratumor groups according to source of tissue and compared in pair. Furthermore, patients were divided into three groups according to the expression of LDHA and then classified into four groups characterized by cancer clinical stage. In addition, patients were divided into negative and positive groups according to the distant metastasis information. With the statistical analysis, the correlation between LDHA expression and clinical stage or metastasis were obtained.
Statistical Analysis
All results were presented as mean ± SD. Correlation between LDHA and clinical stage and metastasis of breast cancer in TCGA database was analyzed by one-way ANOVA test. Other results were analyzed with the Student’s t-test (unpaired). The data normality was established before the application of the statistical analysis. P < 0.05 was used as the criterion for statistical significance (*, P < 0.05; **, P < 0.01; ***, P < 0.001).
Results
LDHA Positively Correlated With Clinical Stages and Cancer Metastasis, and Might Be Involved in the Regulation of Tumor Immune Microenvironment
To investigate the role of LDHA in breast cancer progression, we analyzed the correlation of LDHA expression with the tumor clinical stages, metastasis, and survival according to TCGA database. The results showed that LDHA expression was markedly increased in patients at tumor advanced-stage or in metastatic tumors (Figure 1A), and high expression of LDHA was significantly associated with short overall survival of breast cancer patients (Figure 1B, P < 0.0001). In addition, with TCGA data set, we analyzed the relationship between LDHA expression and macrophages/CD8+ T lymphocytes infiltration in tumor microenvironment for the first time. It was meaningful to find that LDHA expression was positively correlated with macrophages infiltration but negatively related with that in CD8+ T lymphocytes in breast cancer (Figure 1C). Abovementioned results suggested that LDHA played an important role in the development of human breast cancer, as well as the immunoregulation of tumor microenvironment.
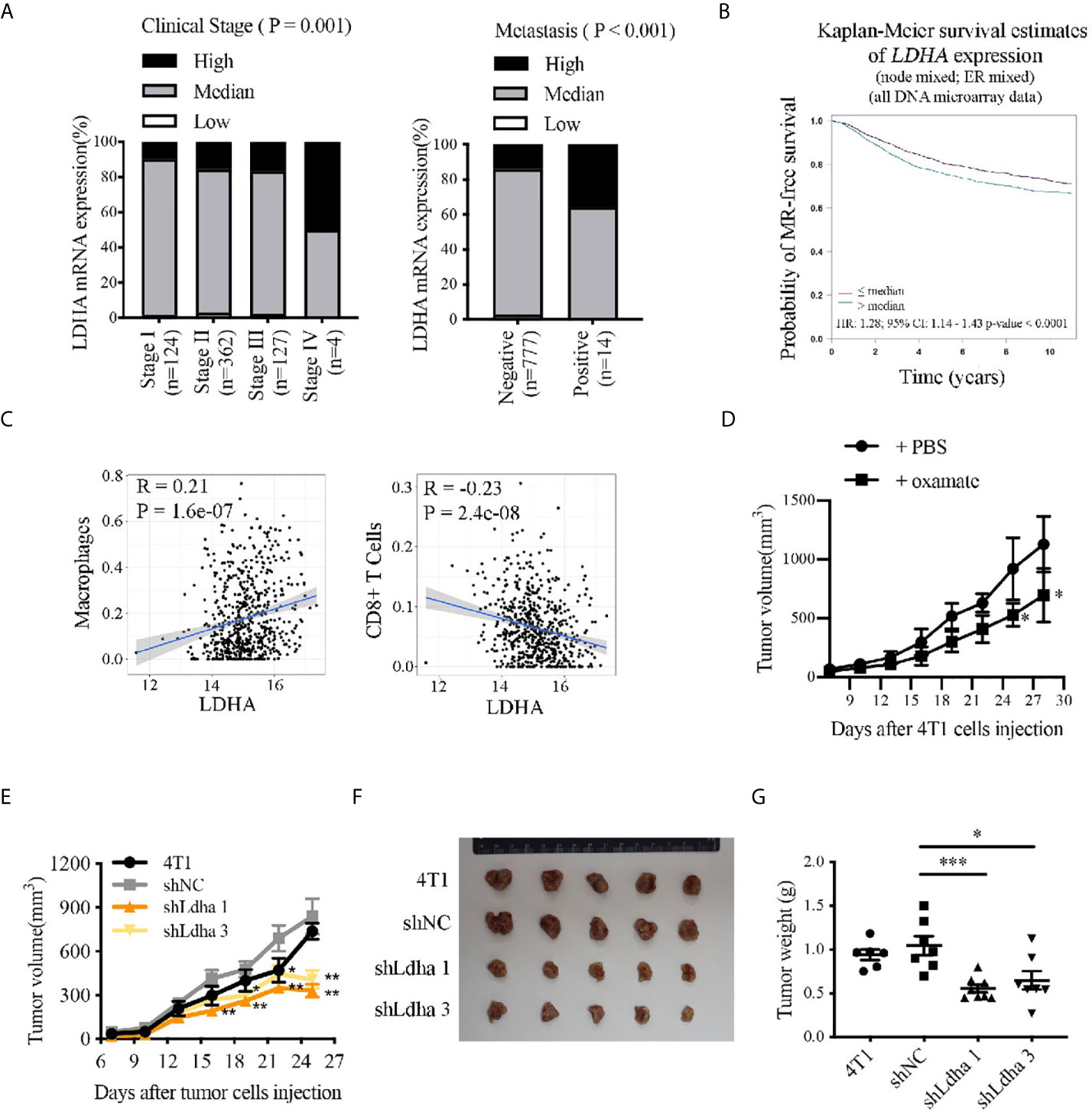
Figure 1 LDHA predicted progression and prognosis of breast cancer patients, correlated with immunocytes infiltration in TME and promoted 4T1 tumor growth in mouse. (A) Proportion of patients depend on the LDHA expression in each clinical stage. Breast cancer samples were classified as high, medium, or low LDHA expression according to log2 (FPKM of LDHA). (B) Kaplan-Meier analysis of the overall survival of breast cancer patients with LDHA high or low expression. The analysis was performed by Breast Cancer Gene-Expression Miner v4.4 in Integrated Center for Oncology. (C) Bioinformatics analysis of the correlation between LDHA expression and infiltration of macrophages or CD8 T cells in breast cancer based on TCGA data set. (D) Tumor growth curve of mice inoculated with 4T1 cells treated with PBS (n = 4) or oxamate (n = 5). (E) Tumor growth curve of mice inoculated with 4T1 wild type, shNC or shLdha cells (n = 8 in each group). 1×105 cells were injected per mice. (F) Tumors were harvested on 25th day after tumor cells inoculation and tumor picture was shown (n = 5). (G) Tumors weight were measured following tumor harvest (n = 8 in each group initially). *P < 0.05; **P < 0.01; ***P < 0.001.
LDHA Was Essential for the Growth of Murine Breast Cancer in an Orthotopic Syngeneic Immunocompetent Mouse Model
Oxamate, a specific LDHA inhibitor, which could suppress LDHA activity (Supplementary Figure 1A) was used to verify the effect of LDHA on tumor generation and progression in mice. For the treatment strategy, oxamate, dissolved in PBS, was intraperitoneally injected into the Balb/c mice xenografted with 4T1 tumor every day for 3 weeks (Supplementary Figure 1B). During the treatment, tumor size was measured every 3 days and as expected, tumor growth was obviously restricted in mice treated with oxamate (Figure 1D). It is important to note that by using two stable 4T1 cell lines with Ldha knock-down (4T1-shLdha1 and 4T1-shLdha3), we found that tumor grew significantly more slowly in both 4T1-shLdha groups compared with the control mice after tumor cells inoculated in the 4th breast fat pad of Balb/c mice, and tumor weight at 25th day was much lower as well (Figures 1E–G). Taken together, growth of 4T1 tumors was significantly suppressed in mice by using LDHA-specific inhibitor or shRNA, which indicated an important role of LDHA in mouse breast cancer progression.
LDHA Maintained Stemness-Related Properties of Breast Cancer Stem Cells
In consideration of elevated glycolysis in BCSCs from our previous study (20), and the important role of LDHA in maintaining glycolysis (Figure 2A), we presumed that LDHA was critical for keeping functionality of BCSCs. To demonstrate the role of LDHA in stemness maintenance, the expression of LDHA was detected in different breast cancer cell lines, and results have shown cells with higher degree of malignancy (HER2+ or basal−like type) have relative higher LDHA level (Supplementary Figures 2A, B). Tumor spheres of MDA-MB-231 cells cultured in vitro was enriched of CSCs population that was determined to have higher LDH activity compared with their adherent cells (Figure 2B). Next, stable cancer cell lines of MDA-MB-231-shLDHA, A549-shLDHA, and 4T1-shLdha were established, and both protein level and enzyme activity of LDHA/Ldha were significantly attenuated in LDHA knockdown cells (Supplementary Figure 3). Thereafter, the tumor spheres’ number, ALDH+ cell proportion, stemness genes (SOX2, OCT4, and NANOG), expression, and migratory cells were all reduced in MDA-MB-231-shLDHA cells (Figures 2C–F). Similarly, the expression of OCT4 also declined in 4T1-shLdha cells (Supplementary Figure 4). Both cellular proliferation and movement were suppressed but cellular apoptosis was rarely changed in 4T1-shLdha cancer cells (Supplementary Figures 5A–C). Taken together, our results highlighted a crucial role of LDHA in cancer stemness maintenance.
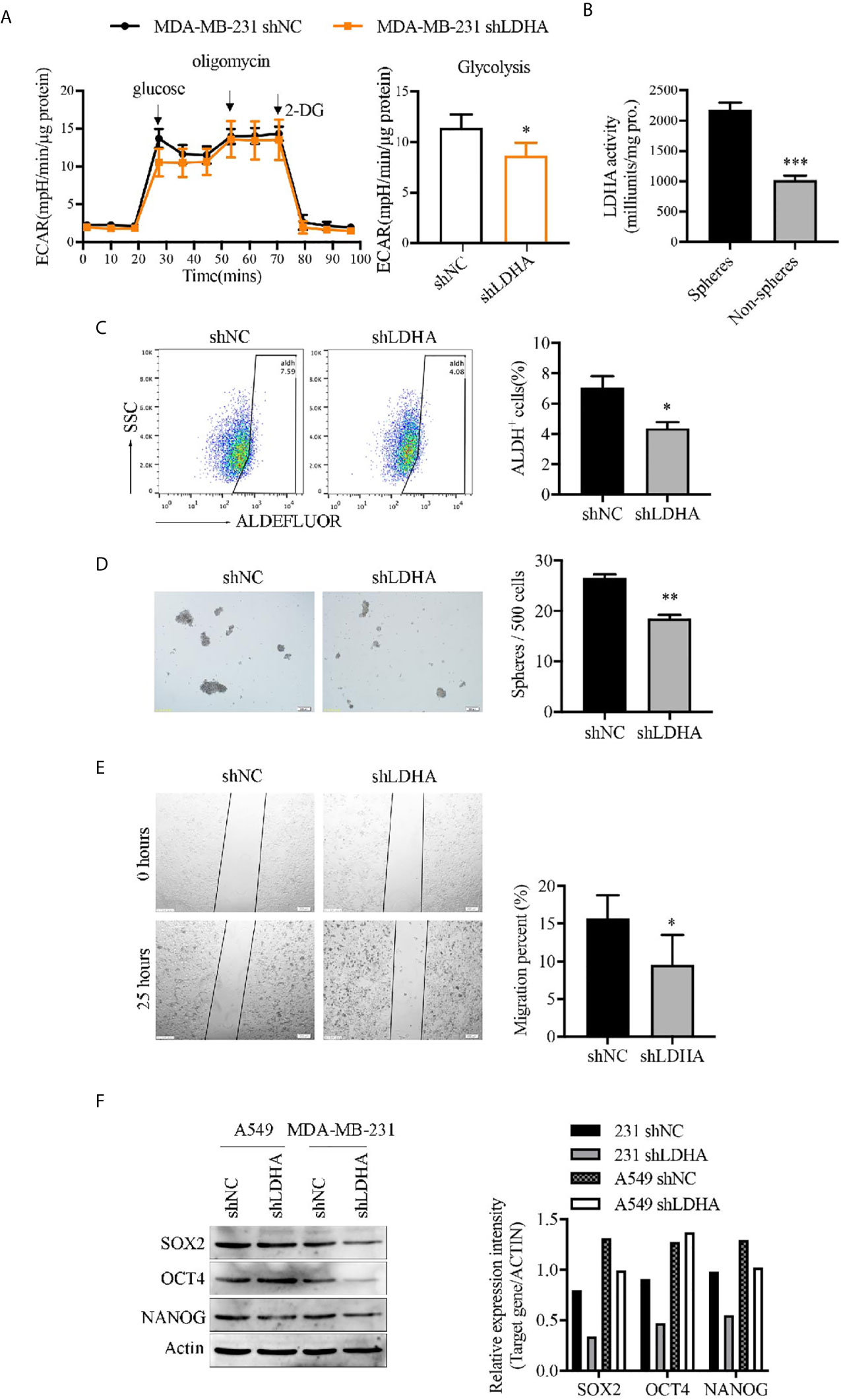
Figure 2 LDHA maintained stemness properties of human breast cancer cells. (A) Extracellular acidification rate (ECAR) of MDA-MB-231-shLDHA or control cells was detected by Seahorse Cellular Bioenergetics Analyzer. (B) LDH activity was detected in spheres or non-spheres cancer cells of MDA-MB-231 by colorimetry. (C) Percentage of ALDH+ cells in MDA-MB-231-shLDHA or control cells was analyzed by flow cytometry. (D) MDA-MB-231-shLDHA or control cells was cultured in sphere formation medium for 5-7 days, and the numbers of tumor-spheres were counted under a microscope. The representative images of spheres were shown on the left. Scale bar, 200 μm (insets). (E) Ability of cellular migration was measured by wound healing assay in MDA-MB-231 shNC and shLDHA cells. (F) Western blot analysis for SOX2, OCT4 and NANOG in A549-shLDHA, MDA-MB-231-shLDHA and their control cells. *P < 0.05; **P < 0.01; ***P < 0.001.
LDHA Promoted the Transformation of M-BCSCs From E-BCSCs in 4T1 Cells
ALDH+ cells represented a population of proliferative epithelial-BCSCs (E-BCSCs) and CD44+CD24− cells enriched the metastatic mesenchymal-BCSCs (M-BCSCs) (5). It is interesting that the proportion of ALDH+ cells appeared to be increased and CD44+CD24− population was reduced while LDHA was downregulated in 4T1 cells (Figures 3A, B), which suggested a regulatory effect of LDHA in the conversion between E-BCSCs and M-BCSCs. Furthermore, the co-expression analysis showed that LDHA was negatively correlated with the expression of ALDH1A1 but positively correlated with the expression of CD44 in human breast cancer samples based on the TCGA data set (Figure 3C). In addition, the formation of tumor spheres (enriched for proliferative BCSCs) was enhanced, but the migratory cells (enriched for metastatic BCSCs) were markedly reduced after silencing of LDHA in 4T1 cells (Figures 3D, E). It is important to note that pulmonary metastasis was also strongly suppressed in mice inoculated with 4T1-shLdha cells (Figure 3F). It was suggested that LDHA could preserve the proportion and properties of M-BCSCs; therefore, it was supposed to be a gatekeeper in BCSCs’ plasticity.
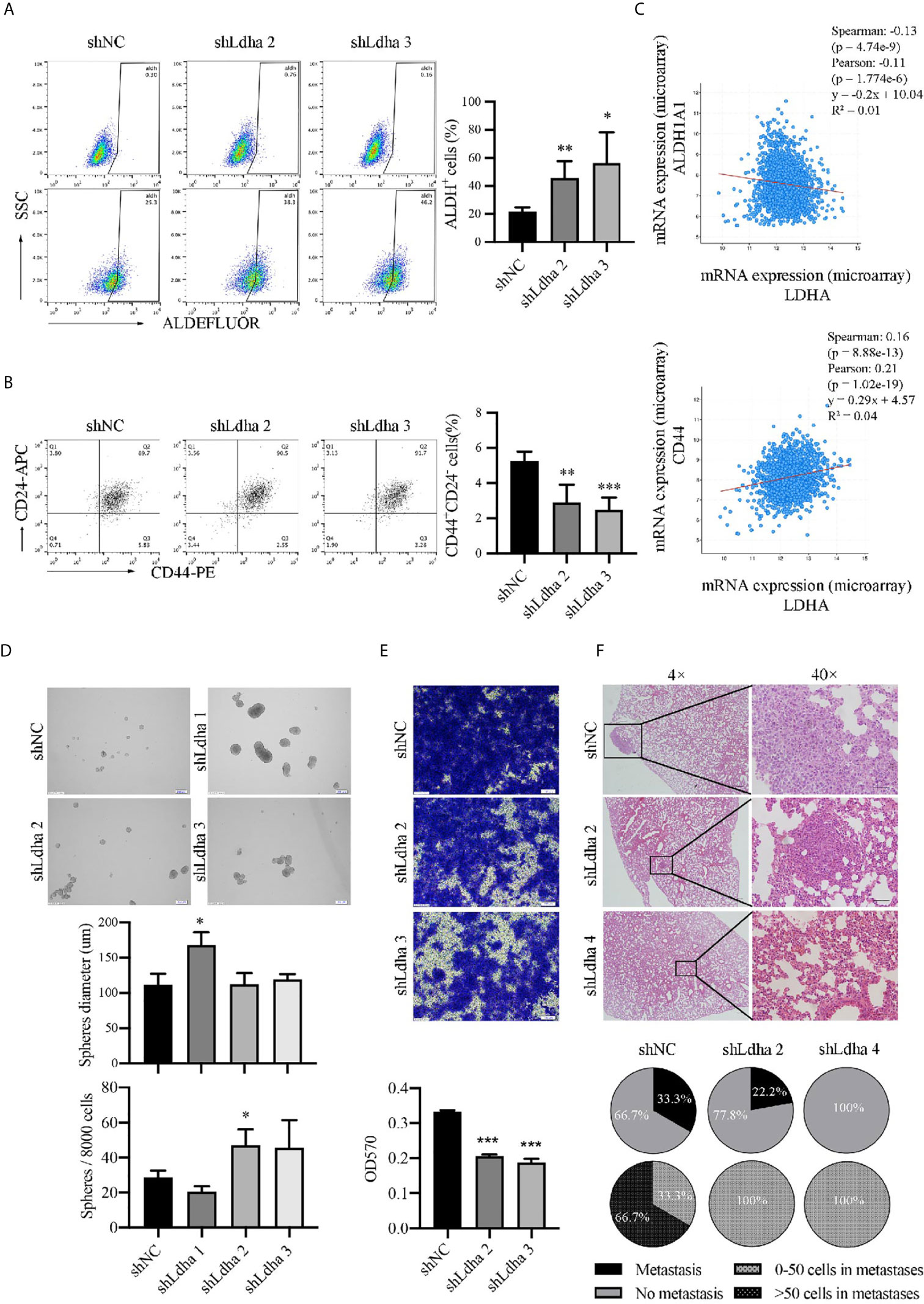
Figure 3 LDHA promoted E to M transition of BCSCs in mouse 4T1 cancer cells. (A) Percentage of ALDH+ cells in 4T1- shNC or 4T1-shLdha cells was analyzed by flow cytometry. (B) Percentage of CD44+CD24− cells in 4T1- shNC or 4T1-shLdha cells was analyzed by flow cytometry. (C) Co-expression analysis of LDHA, ALDH1A1 and CD44 by using cBioportal for cancer genomics. (D) 4T1-shLDHA or control cells was cultured in sphere formation medium for 5-7 days, and the diameters and numbers of tumor-spheres were measured under a microscope. Scale bar, 200 μm (insets). (E) Cellular migration of 4T1-shNC or shLdha cells was measured by Transwell assay. Crystal violet was eluted by 33% acetic acid and determined at 570nm. (F) 4T1 shNC or shLdha cells was injected into 4th breast fat pad of mice and lung was harvested on day 19 after injection. Tumor metastasis was analyzed by hematoxylin-eosin staining. Scale bar, 50 μm (insets). Number of mice with lung metastasis was 3/9(4T1 shNC), 2/9(shLdha 2) and 0/7(shLdha 4). The number of mice with more than 50 tumor cells in metastatic foci was 2/3(4T1 shNC), 0/2(shLdha 2) and 0/0(shLdha 4). *P < 0.05; **P < 0.01; ***P < 0.001.
Epithelial Marker E-Cadherin Was Negatively Regulated by LDHA
To investigate the mechanism of LDHA regulating BCSCs heterogeneity, we performed a bioinformatics analysis by using GSE115302 and GSE59281 data sets. CDH1 encoding E-Cadherin was an epithelial marker, and CDH2/VIM encoding N-Cadherin/vimentin, respectively, represented the mesenchymal markers. As expected, CDH1 was highly expressed in E-BCSCs and CDH2/VIM were highly expressed in M-BCSCs (Figure 4A), which suggested that the EMT markers could be used to distinguish those two BCSCs subgroups. It was noteworthy that the migratory cells of MCF-7 treated with oxamate were reduced, and E-Cadherin level was increased correspondingly (Figures 4C, D). The expression of E-Cadherin which was overexpressed in 4T1 and MCF-7 cells (Figure 4B) was up-regulated upon LDHA knocked down (Figure 4E). Immunoprecipitation assay revealed that endogenous LDHA could interact with E-cadherin in MCF7 cells (Figure 4F). These results suggested that E-cadherin was negatively regulated by LDHA in M-BCSCs, which perhaps contributed the E/M-BCSCs transformation.
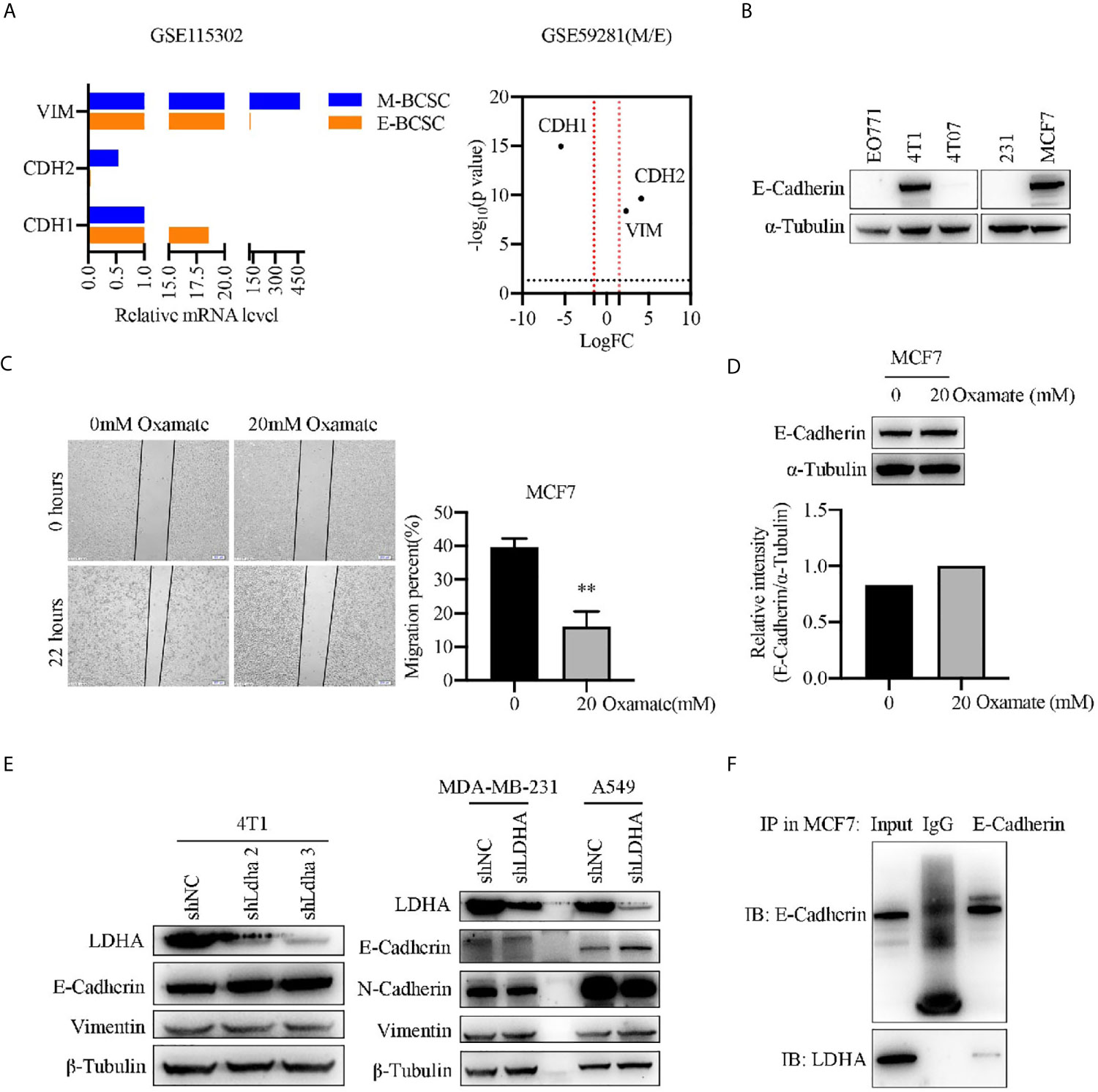
Figure 4 Epithelial marker E-cadherin was negatively regulated by LDHA. (A) Transcripts of CDH1, CDH2, and VIM were analyzed in E-BCSCs or M-BCSCs population based on GSE115302 and GSE59281 data sets. The horizontal dotted line represents P = 0.05, the vertical dotted line represents logFC = 2/−2. (B) Western blot analysis for E-Cadherin in EO771, 4T1, 4T07, MDA-MB-231 and MCF7 cells. (C) Cellular migration was measured by wound healing assay in MCF7 cells treated with 0 or 20 mM oxamate for 30 h. Scale bar, 200 μm (insets). (D) Western blot analysis for E-Cadherin in MCF7 cells treated with 0 or 20 mM oxamate for 30 h. (E) Western blot analysis for LDHA, E-Cadherin, N-Cadherin and Vimentin in A549-shLDHA or MDA-MB-231-shLDHA cancer cells. (F) Immunoprecipitation analysis of the interaction between LDHA and E-Cadherin in MCF7 cells. **P < 0.01.
LDHA Promoted the Tumoral Infiltration of TAMs in a 4T1 Murine Breast Cancer Model
Previous studies demonstrated that tumor immune microenvironment, especially tumor-associated macrophages (TAMs) served as the key niche cell for CSCs maintenance (21–23). Using the 4T1 orthotopic breast tumor model in mice, besides a remarkable retardation of tumor growth (Figure 5A), the infiltration of macrophages, marked by CD45+CD11b+F4/80+, was significantly suppressed in 4T1-shLdha tumors (Figure 5B). Furthermore, the antineoplastic immune cells, including CD8+ or CD4+ T cells was highly increased, nevertheless the bone marrow-derived immunosuppressive cells (MDSCs) decreased sharply in 4T1-shLdha tumors (Figure 5C). It was also verified with immunohistochemistry that the numbers of CD8+ T cells was increased and CD206+ M2 macrophages was decreased infiltrated in 4T1-shLdha tumors (Figure 5D). Next, we explored the migratory ability and immunologic phenotype of macrophages cultured with diverse 4T1 conditional medium in vitro. It was notable that migratory RAW264.7 cells were dramatically decreased and the expression of M2 related genes (Arg1, CD206, IL-10, and Ccr2) in RAW264.7 or bone marrow derived macrophages (BMDMs) were markedly reduced when cultured with the conditional medium from 4T1-shLdha cells (Figures 6A, B and Supplementary Figure 6). Ccr2-expressing monocytes were effectively attracted into tumor sites by Ccl2 (24), and our results revealed that Ccl2 secreted from 4T1-shLdha cells notably decreased compared to the control cells detected by ELISA (Figure 6C). Intriguingly, bioinformatic analysis revealed that CCL2 and LDHA were both strongly increased in M-BCSCs population (CD44+ cancer cells) compared with the bulk cancer cells according to the GSE115302 data set (Figure 6D). Moreover, the expression of Ccl2 was significantly increased in sorted E-BCSCs (ALDH+ cancer cells) compared with the unsorted bulk 4T1 cells (Figure 6E). Taken together, these results suggested that tumoral infiltration of TAMs in breast cancer was intensively facilitated by Ldha/Ccl2 signaling pathway in BCSCs.
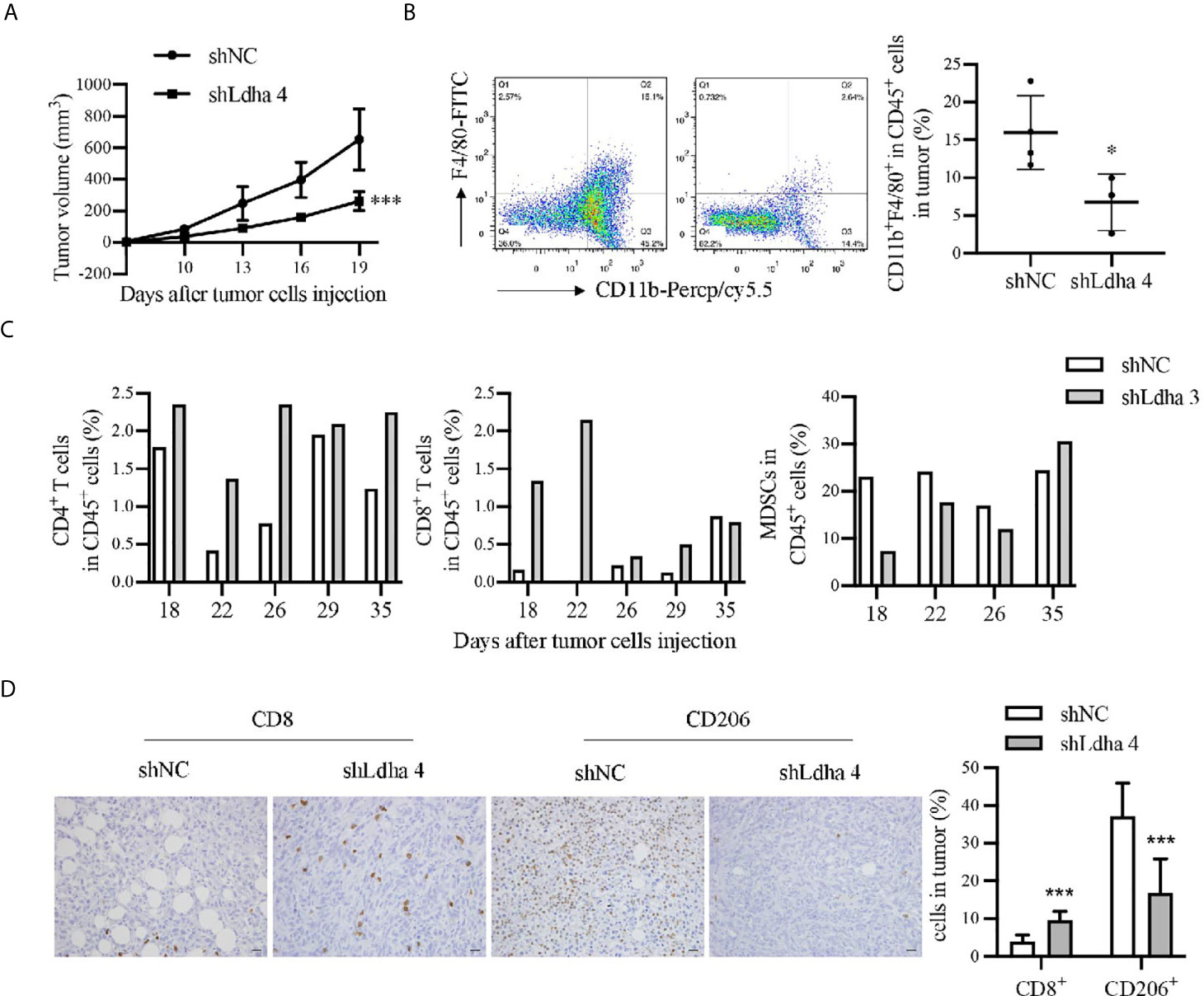
Figure 5 LDHA promoted tumoral infiltration of TAMs in 4T1 murine breast cancer model. (A) Tumor growth curve of mice inoculated with 4T1-shNC or 4T1-shLdha cells. 1×105 cells per mice were injected into the fourth breast fat pad of Balb/c mice (n = 9 in 4T1-shNC group, n = 7 in 4T1 shLdha group). (B) Percentage of CD11b+F4/80+ macrophages in CD45+ immune cells were analyzed by flow cytometry. Tumor were harvested on day 19 after the tumor cells injection. CD45-PE, CD11b-Percp/cy5.5 and F4/80-FITC were used to mark macrophages in tumor. (C) Percentage of CD3+CD8+ T cells, CD3+CD4+ T cells or CD11b+Gr1+ MDSCs in CD45+ immune cells were analyzed by flow cytometry. Tumor were harvested on different days after the tumor cells injection (n = 1 in each group). CD45-PE, CD3ϵ-FITC, CD4-APC and CD8α-APC were used to mark T cells in tumor. CD11b-FITC and Gr1-APC were used to mark MDSCs in tumor. (D) The expression of CD8 and CD206 was analyzed by immunohistochemistry in 4T1 shNC or shLdha-injected tumors (n = 9 in 4T1-shNC group, n = 7 in 4T1 shLdha group). Scale bar, 20 μm (insets). *P < 0.05; ***P < 0.001.
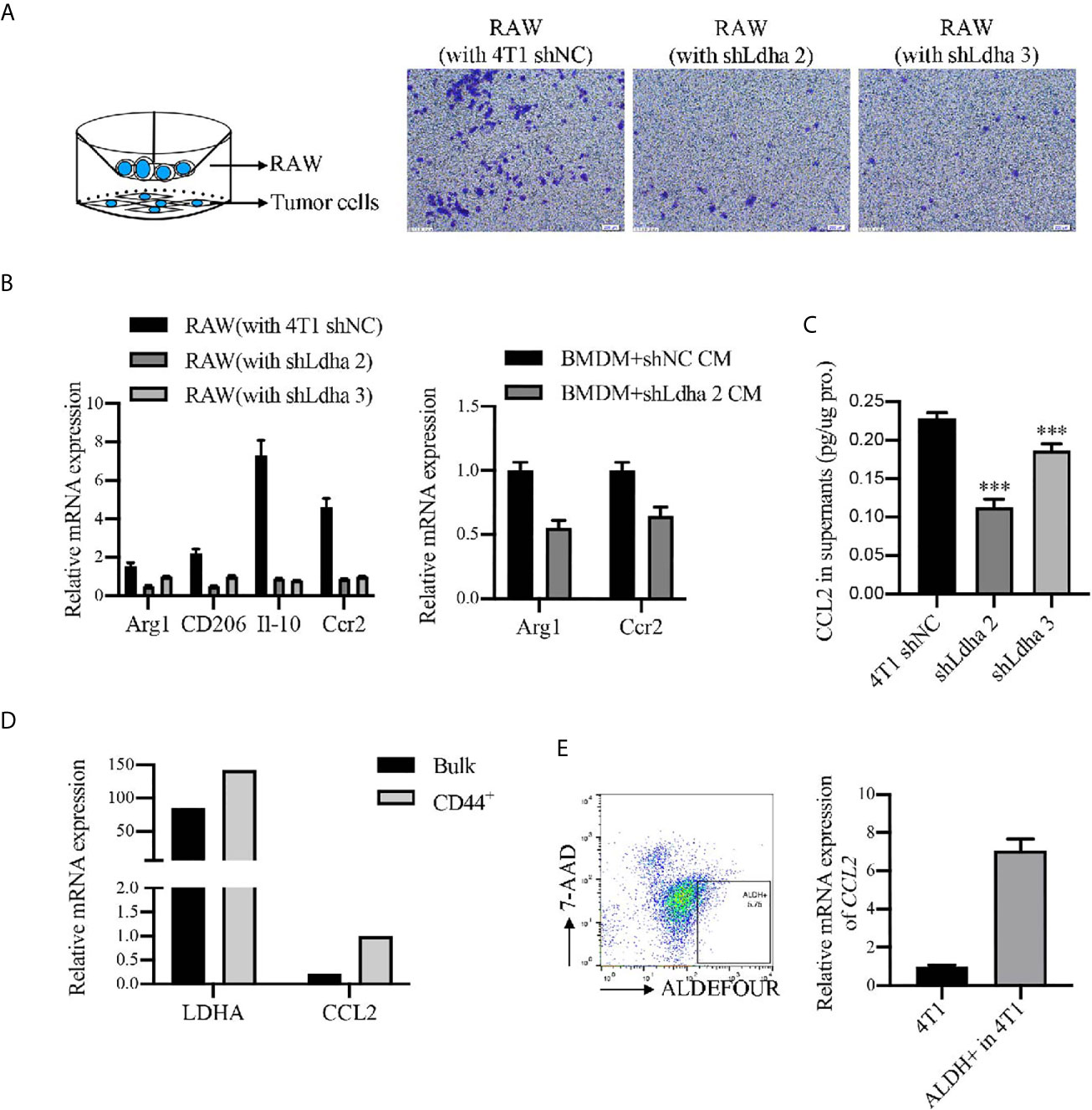
Figure 6 LDHA enhanced the recruitment and M2 phenotypes of macrophages in vitro. (A) Migratory capacity of RAW264.7 cells was analyzed by Transwell assay. RAW264.7 cells were seeded in 8-μm-pore polycarbonate filters and 4T1-shNC or 4T1-shLdha cells were seeded in the bottom well. Representative images were visualized by microscope (right) and percentage of migrating cells was calculated by use of ImageJ. Scale bar, 200 μm (insets). (B) Relative mRNA level of M2 markers in RAW 264.7 or BMDM cultured with conditional medium from 4T1-shNC or 4T1-shLdha cancer cells were detected by RT-qPCR. (C) Ccl2 level in the cultural supernatant from 4T1-shNC or 4T1-shLdha cells was detected by ELISA. Results were normalized by protein content in cells. (D) Relative mRNA level of LDHA and CCL2 in M-BCSCs or bulk cells were analyzed according to GSE115302 data set. (E) The mRNA level of Ccl2 was measured by RT-qPCR in ALDH+ or bulk 4T1 cells. ALDH+7-AAD- cells were sorted out by flow cytometry. Error bars mean ± SD from three independent experiments. ***P < 0.001.
Discussion
BCSCs are considered as key drivers of tumor growth, relapse, and metastasis, of which targeting the specific metabolism may help to eradicate this small subset of cells. The main metabolic pathway of cancer cell is aerobic glycolysis rather than oxidative phosphorylation, which is called “Warburg effect” and has been verified in many types of tumor (12, 13, 25–27). Our previous study revealed a higher glycolytic metabolism in BCSCs (20), but the mechanism of glycolysis maintaining stemness was not much clear. LDHA, a rate-limiting enzyme in glycolytic process, promotes tumor growth through variety of ways, which is verified to play an important role in stemness maintenance of BCSCs in this study. This result is in agreement with a recent report documenting that targeting LDHA inhibits lung tumor-initiating cells by using an inducible murine model (13). Recent evidence implies BCSCs are not a simplex cell population but mainly consist of two subgroups, which are termed as E-BCSCs and M-BCSCs. E-BCSCs are epithelioid, proliferative cancer stem cells, highly expressing epithelial-like marker E-Cadherin, while M-BCSCs are quiescent, metastatic cancer stem cells, highly expressing mesenchymal-like marker N-Cadherin. A combinatory approach targeting both M- and E-BCSCs illustrates a novel treatment approach targeting both BCSC states (28). For the first time, our results reveal that LDHA play a critical role in BCSCs heterogeneity, which indicated a special effect on E/M-BCSCs transition in 4T1 cells.
The transition of BCSCs from the E to the M state closely resembles the epithelial-to-mesenchymal transition (EMT), which plays an important role in the metastatic process of tumor cells (29). Our results also demonstrate a suppressive effect of LDHA on the epithelia marker E-Cadherin, which seemingly explains its essential function in E/M-BCSCs transition. Departure from cell membrane of E-Cadherin provoked by ubiquitylate degradation is an important mechanism for tumor cells to undergo EMT and enhance their metastatic ability (30, 31). In our data, ubiquitin of E-Cadherin was also detected in LDHA pull-down sample (data not shown). Therefore, we speculate that LDHA might promote the ubiquitination and endocytosis of E-Cadherin to facilitate the transformation of CSCs from E-BCSCs to M-BCSCs ultimately and it is also suggested that solely suppression of LDHA may not be sufficient to eliminate BCSCs containing different subpopulations. Fully investigation of metabolic heterogeneity in diverse subsets of BCSCs appears urgent and therapeutic strategies by targeting BCSCs’ diverse metabolism seems to have great prospect for breast cancer treatment.
The equilibrium of diverse BCSCs subgroups is regulated by the TME via multifaceted mechanisms including cytokine or chemokine signaling (5, 32, 33). Macrophages constitute up to 50% of the tumor infiltrating cells in human breast cancer and thus represent most non-neoplastic cells in the tumors. Our previous studies revealed that tumor associated macrophages promoted the stemness phenotypes of BCSCs by activating key inflammatory pathways (34, 35). In turn, this study demonstrated that LDHA, a cancer stemness promoter, could impede the infiltration of anti-tumor immune cells (CD4+/CD8+ T cells) and enhance the accumulation of the pro-tumoral immune cells (MDSCs and TAMs) in TME. Previous study revealed that the polarization of M2 phenotype was promoted by lactate in TAMs (36). Recent study revealed that lactate concentration quantified by the double quantum filtered (DQF) MRS, a non-invasive imaging method, is a sensitive marker for the prediction of tumor grades and prognosis in breast cancer. However, there has been study showing that silencing LDHA failed to alter lactic acid production in breast cancer cell line (37). Except for lactic acid, carbonic anhydrase 9 (CA-9), maintaining the acidic condition of TME, also contributed to the tumor progression (38). Interestingly, the lipid composition in TME is also an important factor for macrophages recruitment and functional remolding (39). In our study, by using the co-culturing system in vitro, we find that the recruitment of macrophages is strongly enhanced by LDHA. Mechanistically, this occurs at least in part through elevated secretion of macrophages-attracted chemokine CCL2 induced by LDHA. Recruitment of inflammatory monocytes/macrophages responding to CCL2 is critical for tumor cell pulmonary seeding (40).These findings revealed the different mechanisms of metabolic enzyme in regulating the TME and might provide novel targets for cancer therapy. Interestingly, CCL2 expression is dramatically increased in BCSCs based on TCGA data set and we propose a hypothesis that secretion of CCL2 from BCSCs is enhanced by LDHA, which could recruit more CCR2-positive tumor associated macrophages (TAMs) to further maintained the stemness of BCSCs. Moreover, E-Cadherin, the molecular marker of E-BCSCs, was suppressed by LDHA that partially explain the phenomenon of LDHA mediated E to M transformation in BCSCs. This hypothesis needs to be further fully investigated and targeting LDHA combined with CCL2/CCR2 antagonists may provide a better therapeutic outcome for breast cancer.
In conclusion, our study demonstrates that an essential glycolytic enzyme of LDHA not only maintains the stemness and heterogeneity of BCSCs but also facilitates the infiltration of TAMs to trigger immune escape of BCSCs (Figure 7). The mechanisms of tumor abnormal metabolism identified here can inform translational studies by targeting heterogeneous population and supportive niche of BCSCs, to develop more efficient treatment for breast cancer.
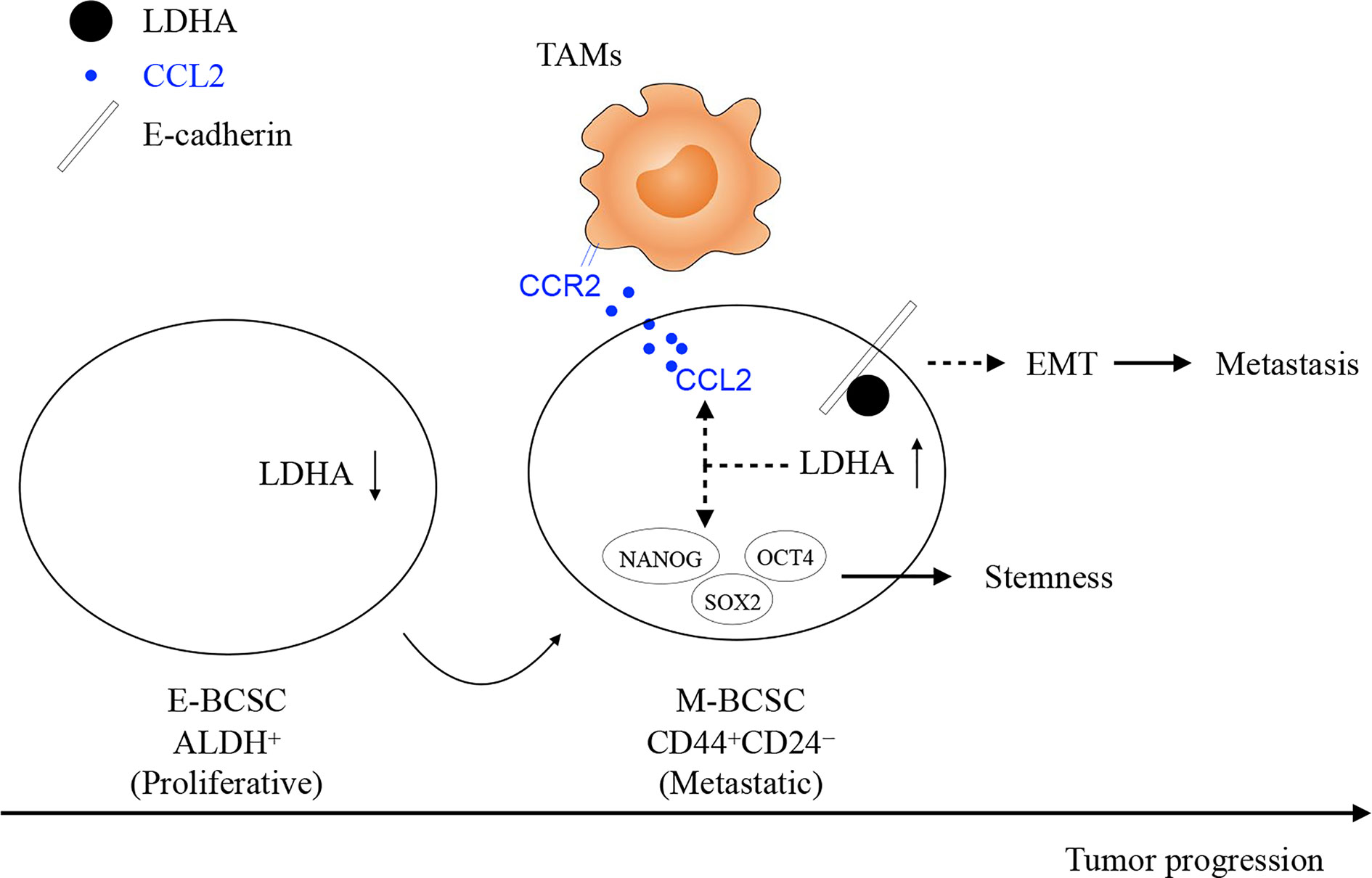
Figure 7 The work model of glycolytic enzyme of LDHA not only maintains the stemness and heterogeneity of BCSCs but also facilitates the infiltration of TAMs to trigger immune escape of BCSCs. At the early stage of breast cancer, E-BCSCs promote tumor rapidly growth and recruit more CCR2-expressing monocytes/macrophages and then, LDHA reduces E-Cadherin expression and increases CCL2 secretion in M-BCSCs to facilitated tumor metastasis at the advanced stage of breast cancer.
Data Availability Statement
The original contributions presented in the study are included in the article/Supplementary Material. Further inquiries can be directed to the corresponding authors.
Ethics Statement
The animal study was reviewed and approved by institutional review board of Institute of Basic Medical Sciences, Chinese Academy of Medical Sciences.
Author Contributions
SW carried out the cellular and animal experiments. ZW carried out the bioinformatic analysis. SW and LM performed the statistical analysis. CC and SW drafted the manuscript. HH, HC, ZD, YLi, QS, and T-HC provided material support. CC and YLu conceived of the study and participated in its design. All authors contributed to the article and approved the submitted version.
Funding
This work was supported by grants from the National Natural Science Foundation of China (NSFC): grant no. 81972795 and 81672914; the Bilateral Inter-Governmental S&T Cooperation Project from Ministry of Science and Technology of China: grant no. 2018YFE0114300; the National Basic Research Program (973) of China: grant no. 2013CB967202.
Conflict of Interest
The authors declare that the research was conducted in the absence of any commercial or financial relationships that could be construed as a potential conflict of interest.
Supplementary Material
The Supplementary Material for this article can be found online at: https://www.frontiersin.org/articles/10.3389/fonc.2021.654452/full#supplementary-material
References
1. Polyak K, Hahn WC. Roots and Stems: Stem Cells in Cancer. Nat Med (2006) 12:296–300. doi: 10.1038/nm1379
2. Almanaa TN, Geusz ME, Jamasbi RJ. A New Method for Identifying Stem-Like Cells in Esophageal Cancer Cell Lines. J Cancer (2013) 4:536–48. doi: 10.7150/jca.6477
3. Yang F, Xu J, Tang L, Guan X. Breast Cancer Stem Cell: The Roles and Therapeutic Implications. Cell Mol Life Sci CMLS (2017) 74:951–66. doi: 10.1007/s00018-016-2334-7
4. Prasetyanti PR, Medema JP. Intra-Tumor Heterogeneity From a Cancer Stem Cell Perspective. Mol Cancer (2017) 16:41. doi: 10.1186/s12943-017-0600-4
5. Brooks MD, Burness ML, Wicha MS. Therapeutic Implications of Cellular Heterogeneity and Plasticity in Breast Cancer. Cell Stem Cell (2015) 17:260–71. doi: 10.1016/j.stem.2015.08.014
6. Bhattacharya B, Mohd Omar MF, Soong R. The Warburg Effect and Drug Resistance. Br J Pharmacol (2016) 173:970–9. doi: 10.1111/bph.13422
7. Demarse NA, Ponnusamy S, Spicer EK, Apohan E, Baatz JE, Ogretmen B, et al. Direct Binding of Glyceraldehyde 3-Phosphate Dehydrogenase to Telomeric DNA Protects Telomeres Against Chemotherapy-Induced Rapid Degradation. J Mol Biol (2009) 394:789–803. doi: 10.1016/j.jmb.2009.09.062
8. Cairns RA, Harris IS, Mak TW. Regulation of Cancer Cell Metabolism. Nat Rev Cancer (2011) 11:85–95. doi: 10.1038/nrc2981
9. Klement RJ, Kämmerer U. Is There a Role for Carbohydrate Restriction in the Treatment and Prevention of Cancer? Nutr Metab (2011) 8:75. doi: 10.1186/1743-7075-8-75
10. Luo M, Shang L, Brooks MD, Jiagge E, Zhu Y, Buschhaus JM, et al. Targeting Breast Cancer Stem Cell State Equilibrium Through Modulation of Redox Signaling. Cell Metab (2018) 28:69–86.e6. doi: 10.1016/j.cmet.2018.06.006
11. Burgner JW, Ray WJ. On the Origin of the Lactate Dehydrogenase Induced Rate Effect. Biochemistry (1984) 23:3636–48. doi: 10.1021/bi00311a010
12. Shi M, Cui J, Du J, Wei D, Jia Z, Zhang J, et al. A Novel KLF4/LDHA Signaling Pathway Regulates Aerobic Glycolysis in and Progression of Pancreatic Cancer. Clin Cancer Res Off J Am Assoc Cancer Res (2014) 20:4370–80. doi: 10.1158/1078-0432.CCR-14-0186
13. Xie H, Hanai J, Ren J-G, Kats L, Burgess K, Bhargava P, et al. Targeting Lactate Dehydrogenase-a Inhibits Tumorigenesis and Tumor Progression in Mouse Models of Lung Cancer and Impacts Tumor-Initiating Cells. Cell Metab (2014) 19:795–809. doi: 10.1016/j.cmet.2014.03.003
14. Koukourakis MI, Giatromanolaki A, Sivridis E, Gatter KC, Trarbach T, Folprecht G, et al. Prognostic and Predictive Role of Lactate Dehydrogenase 5 Expression in Colorectal Cancer Patients Treated With PTK787/ZK 222584 (Vatalanib) Antiangiogenic Therapy. Clin Cancer Res Off J Am Assoc Cancer Res (2011) 17:4892–900. doi: 10.1158/1078-0432.CCR-10-2918
15. Noy R, Pollard JW. Tumor-Associated Macrophages: From Mechanisms to Therapy. Immunity (2014) 41:49–61. doi: 10.1016/j.immuni.2014.06.010
16. Yang L, Zhang Y. Tumor-Associated Macrophages: From Basic Research to Clinical Application. J Hematol OncolJ Hematol Oncol (2017) 10:58. doi: 10.1186/s13045-017-0430-2
17. Cai J, Xia L, Li J, Ni S, Song H, Wu X. Tumor-Associated Macrophages Derived Tgf-β‒Induced Epithelial to Mesenchymal Transition in Colorectal Cancer Cells Through Smad2,3-4/Snail Signaling Pathway. Cancer Res Treat Off J Korean Cancer Assoc (2019) 51:252–66. doi: 10.4143/crt.2017.613
18. Qiu S-Q, Waaijer SJH, Zwager MC, de Vries EGE, van der Vegt B, Schröder CP. Tumor-Associated Macrophages in Breast Cancer: Innocent Bystander or Important Player? Cancer Treat Rev (2018) 70:178–89. doi: 10.1016/j.ctrv.2018.08.010
19. Yang L, Shi P, Zhao G, Xu J, Peng W, Zhang J, et al. Targeting Cancer Stem Cell Pathways for Cancer Therapy. Signal Transduct Target Ther (2020) 5:8. doi: 10.1038/s41392-020-0110-5
20. Chen C, Bai L, Cao F, Wang S, He H, Song M, et al. Targeting LIN28B Reprograms Tumor Glucose Metabolism and Acidic Microenvironment to Suppress Cancer Stemness and Metastasis. Oncogene (2019) 38:4527–39. doi: 10.1038/s41388-019-0735-4
21. Wan S, Zhao E, Kryczek I, Vatan L, Sadovskaya A, Ludema G, et al. Tumor-Associated Macrophages Produce Interleukin 6 and Signal Via STAT3 to Promote Expansion of Human Hepatocellular Carcinoma Stem Cells. Gastroenterology (2014) 147:1393–404. doi: 10.1053/j.gastro.2014.08.039
22. Lu H, Clauser KR, Tam WL, Fröse J, Ye X, Eaton EN, et al. A Breast Cancer Stem Cell Niche Supported by Juxtacrine Signalling From Monocytes and Macrophages. Nat Cell Biol (2014) 16:1105–17. doi: 10.1038/ncb3041
23. Tong H, Ke J-Q, Jiang F-Z, Wang X-J, Wang F-Y, Li Y-R, et al. Tumor-Associated Macrophage-Derived CXCL8 Could Induce Erα Suppression Via HOXB13 in Endometrial Cancer. Cancer Lett (2016) 376:127–36. doi: 10.1016/j.canlet.2016.03.036
24. Nagarsheth N, Wicha MS, Zou W. Chemokines in the Cancer Microenvironment and Their Relevance in Cancer Immunotherapy. Nat Rev Immunol (2017) 17:559–72. doi: 10.1038/nri.2017.49
25. Le A, Cooper CR, Gouw AM, Dinavahi R, Maitra A, Deck LM, et al. Inhibition of Lactate Dehydrogenase A Induces Oxidative Stress and Inhibits Tumor Progression. Proc Natl Acad Sci USA (2010) 107:2037–42. doi: 10.1073/pnas.0914433107
26. Jiang F, Ma S, Xue Y, Hou J, Zhang Y. LDH-a Promotes Malignant Progression Via Activation of Epithelial-to-Mesenchymal Transition and Conferring Stemness in Muscle-Invasive Bladder Cancer. Biochem Biophys Res Commun (2016) 469:985–92. doi: 10.1016/j.bbrc.2015.12.078
27. Jin L, Chun J, Pan C, Alesi GN, Li D, Magliocca KR, et al. Phosphorylation-Mediated Activation of LDHA Promotes Cancer Cell Invasion and Tumour Metastasis. Oncogene (2017) 36:3797–806. doi: 10.1038/onc.2017.6
28. Korkaya H, Kim G-I, Davis A, Malik F, Henry NL, Ithimakin S, et al. Activation of an IL6 Inflammatory Loop Mediates Trastuzumab Resistance in HER2+ Breast Cancer by Expanding the Cancer Stem Cell Population. Mol Cell (2012) 47:570–84. doi: 10.1016/j.molcel.2012.06.014
29. Mani SA, Guo W, Liao M-J, Eaton EN, Ayyanan A, Zhou AY, et al. The Epithelial-Mesenchymal Transition Generates Cells With Properties of Stem Cells. Cell (2008) 133:704–15. doi: 10.1016/j.cell.2008.03.027
30. Fujita Y, Krause G, Scheffner M, Zechner D, Leddy HEM, Behrens J, et al. Hakai, a c-Cbl-like Protein, Ubiquitinates and Induces Endocytosis of the E-cadherin Complex. Nat Cell Biol (2002) 4:222–31. doi: 10.1038/ncb758
31. Lu M, Wu J, Hao Z-W, Shang Y-K, Xu J, Nan G, et al. Basolateral CD147 Induces Hepatocyte Polarity Loss by E-Cadherin Ubiquitination and Degradation in Hepatocellular Carcinoma Progress. Hepatol Baltim Md (2018) 68:317–32. doi: 10.1002/hep.29798
32. Luo M, Clouthier SG, Deol Y, Liu S, Nagrath S, Azizi E, et al. Breast Cancer Stem Cells: Current Advances and Clinical Implications. Methods Mol Biol Clifton NJ (2015) 1293:1–49. doi: 10.1007/978-1-4939-2519-3_1
33. Zhu S, Li H-B, Flavell RA. Resemble and Inhibit: When RLR Meets TGF-β. Mol Cell (2014) 56:719–20. doi: 10.1016/j.molcel.2014.12.010
34. Yang J, Liao D, Chen C, Liu Y, Chuang T-H, Xiang R, et al. Tumor-Associated Macrophages Regulate Murine Breast Cancer Stem Cells Through a Novel Paracrine EGFR/Stat3/Sox-2 Signaling Pathway. Stem Cells Dayt Ohio (2013) 31:248–58. doi: 10.1002/stem.1281
35. Chen C, Cao F, Bai L, Liu Y, Xie J, Wang W, et al. Ikkβ Enforces a LIN28B/TCF7L2 Positive Feedback Loop That Promotes Cancer Cell Stemness and Metastasis. Cancer Res (2015) 75:1725–35. doi: 10.1158/0008-5472.CAN-14-2111
36. Colegio OR, Chu N-Q, Szabo AL, Chu T, Rhebergen AM, Jairam V, et al. Functional Polarization of Tumour-Associated Macrophages by Tumour-Derived Lactic Acid. Nature (2014) 513:559–63. doi: 10.1038/nature13490
37. Mack N, Mazzio EA, Bauer D, Flores-Rozas H, Soliman KFA. Stable shRNA Silencing of Lactate Dehydrogenase A (LDHA) in Human Mda-Mb-231 Breast Cancer Cells Fails to Alter Lactic Acid Production, Glycolytic Activity, ATP or Survival. Anticancer Res (2017) 37:1205–12. doi: 10.21873/anticanres.11435
38. Pastorek J, Pastorekova S. Hypoxia-Induced Carbonic Anhydrase IX as a Target for Cancer Therapy: From Biology to Clinical Use. Semin Cancer Biol (2015) 31:52–64. doi: 10.1016/j.semcancer.2014.08.002
39. Cheung SM, Husain E, Mallikourti V, Masannat Y, Heys S, He J. Intra-Tumoural Lipid Composition and Lymphovascular Invasion in Breast Cancer Via non-Invasive Magnetic Resonance Spectroscopy. Eur Radiol (2020) 31(6):3703–11. doi: 10.1007/s00330-020-07502-4
Keywords: metabolism, cancer stem cells, tumor-associated macrophages, lactate dehydrogenase A, E-cadherin, CCL2
Citation: Wang S, Ma L, Wang Z, He H, Chen H, Duan Z, Li Y, Si Q, Chuang T-H, Chen C and Luo Y (2021) Lactate Dehydrogenase-A (LDH-A) Preserves Cancer Stemness and Recruitment of Tumor-Associated Macrophages to Promote Breast Cancer Progression. Front. Oncol. 11:654452. doi: 10.3389/fonc.2021.654452
Received: 16 January 2021; Accepted: 11 May 2021;
Published: 10 June 2021.
Edited by:
Pu Li, Shanghai Jiao Tong University, ChinaReviewed by:
Lunquan Sun, College of Veterinary Medicine, PhilippinesPeiqing Sun, Wake Forest Baptist Medical Center, United States
Jiabao He, University of Aberdeen, United Kingdom
Copyright © 2021 Wang, Ma, Wang, He, Chen, Duan, Li, Si, Chuang, Chen and Luo. This is an open-access article distributed under the terms of the Creative Commons Attribution License (CC BY). The use, distribution or reproduction in other forums is permitted, provided the original author(s) and the copyright owner(s) are credited and that the original publication in this journal is cited, in accordance with accepted academic practice. No use, distribution or reproduction is permitted which does not comply with these terms.
*Correspondence: Chong Chen, chenchong86@ibms.pumc.edu.cn; Yunping Luo, ypluo@ibms.pumc.edu.cn
†These authors have contributed equally to this work and share last authorship