Selenium Nanoparticles Improved Intestinal Health Through Modulation of the NLRP3 Signaling Pathway
- 1State Key Laboratory of Animal Nutrition, College of Animal Science and Technology, China Agricultural University, Beijing, China
- 2College of Resources and Environmental Sciences, China Agricultural University, Beijing, China
- 3Beijing Wahmix Bio-Technology Co., Ltd., Tangshan, China
- 4Microbiology and Immunology Department of Preventive Veterinary Medicine, College of Veterinary Medicine, China Agricultural University, Beijing, China
Selenium nanoparticles (SeNPs) play important roles in promoting animal health, however, their impact on intestinal health remains elusive. This study was intended to evaluate the effects of different doses of SeNPs on the intestinal health, especially the development of goblet cells in the broiler jejunum. A total of 480 1-day-old Arbor Acres broilers were randomly allotted to 5 treatments with 6 replications of 16 chicks each. Birds were fed with low selenium corn-soybean meal-based diets supplemented with 0.1, 0.2, 0.3, or 0.4 mg/kg of SeNPs. On d 21, dietary supplementation of SeNPs effectively reduced the mortality of broilers. The villus height and the villus height/crypt depth ratio of the jejunum showed significant quadratic effects with the increasing concentration of SeNPs (P < 0.05). The mRNA expression of zonula occluden-1 (ZO-1), ZO-2, claudin-3, and claudin-5 in the jejunum decreased linearly with the increasing dose of SeNPs (P < 0.05). The mRNA expression levels of interleukin 1 beta (IL-1β), IL-18, and the concentration of reactive oxygen species (ROS) in the jejunum decreased linearly with the increase of SeNPs concentration (P < 0.05). Compared with the control group, the number of goblet cells in the jejunum was significantly increased by adding 0.1 and 0.4 mg/kg SeNPs(P < 0.05). In addition, the mRNA expression of Mucin2 (Muc2) showed a significant quadratic relationship that increased after adding 0.1 mg/kg SeNPs (P < 0.05). Dietary SeNPs also linearly reduced the expression of v-myc avian myelocytomatosis viral oncogene homolog (c-myc) (P < 0.05). The mean density of TUNEL positive cells in the 0.2 and 0.4 mg/kg SeNPs groups were lower than the control group (P < 0.05). Similarly, the mRNA expression levels of B-cell lymphoma-2 (Bcl-2), Bcl-2-associated X (Bax), NLR family pyrin domain containing 3 (NLRP3), cysteinyl aspartate specific proteinase-1 (Caspase-1), toll-like receptor-2 (TLR-2), and myeloid differentiation factor 88 (MyD88) in the jejunum decreased linearly with the increase of SeNPs concentration (P < 0.05). Results show that supplementation with 0.2 mg/kg SeNPs may decrease intestinal oxidative stress and inflammation by modifying the activation of NLRP3 signaling pathway, which can effectively promote intestinal goblet cells of 21-day-old broilers.
Introduction
Selenium (Se), as an essential micronutrient, plays an important role in the health of humans and animals. Selenium is a well-known antioxidant and exerts biological function by synthesizing selenium-containing proteins (selenoproteins), which contain at least one selenocysteine (Sec) (1). Moreover, Sec is the active site of selenoproteins such as glutathione peroxidase (GSH-Px) and thioredoxin reductase (TrxR), which can make them suitable for oxidation/reduction reactions and prevent cellular oxidative damage (2, 3). The chemical forms of Se in nature are either inorganic (Se, Na2SeO3, Na2SeO4) or organic (selenomethionine/selenocysteine) (4, 5). Compared with selenomethionine and sodium selenite, selenium nanoparticles (SeNPs) transformed from sodium selenite have stronger antioxidant activities and lower cytotoxicity, thereby effectively protecting human and animal intestinal epithelial cells (6). Due to its high bioavailability, anticancer, and antimicrobial properties; SeNPs are widely used in nanomedicine and agriculture (7, 8). Therefore, SeNPs are regarded as a potential and novel Se nutritional supplement (8).
Chicks are exposed to a large number of pathogenic bacteria after hatch (9), and the intestine is more susceptible to oxidative stress, which can increase the level of intestinal inflammation. Intestinal health of broilers is largely achieved by function of the intestinal barrier. The intestinal mucus layer provides the first line of defense for the intestine. The inner mucus layer prevents the invasion of pathogenic bacteria, and the outer mucus layer provides a place and nutrients for symbiotic bacteria (10). The main component of the intestinal mucus layer is the Mucin2 (Muc2), which is primarily secreted by goblet cells (10). Recent research has indicated that the secretion of mucin from intestinal goblet cells depends on several intersecting cellular processes. These include endocytosis, autophagy, reactive oxygen species (ROS) generation, and inflammasome assembly and activation (11). When pathogenic bacteria invade the intestine, goblet cells non-specifically endocytose Toll-like receptor (TLR) ligands by triggering the NLR family pyrin domain containing 6 (NLRP6) inflammasome downstream of TLR- and MyD88-dependent ROS synthesis, which induces Muc2 exocytosis (12). Meanwhile, the level of inflammatory factors such as interleukin 9 (IL-9), IL-13, and IL-25 also increase, which promote the differentiation and proliferation of goblet cells through the STAT6 signaling pathway (13). Therefore, the development of goblet cells can effectively promote the formation of the mucus layer to protect the intestinal tissues.
Selenium promotes the proliferation of goblet cells by the NF-E2-related factor 2 (Nrf2) signaling pathway and ameliorates the structure of intestinal mucus protein, protecting the intestinal barrier function against oxidative damage (14). Selenium deficiency induces intestinal cell apoptosis and causes serious damage to the morphology and function of the intestinal mucosa (15, 16). SeNPs, an effective antioxidant, can decrease the level of intestinal inflammatory factors and reduce the production of intestinal ROS to improve the intestinal barrier function (17, 18). However, it is not clear whether SeNPs can promote the development of intestinal goblet cells in chicks to enhance intestinal barrier functions.
In this study, we investigated the histological characteristics, antioxidant activities, goblet cell differentiation factors, inflammatory factors, and pyroptosis signaling pathways in the jejunum of chicks fed with increasing doses of SeNPs, as to elucidate the molecular mechanism of the possible SeNPs-induced intestinal goblet cells development.
Materials and Methods
All procedures and protocols were approved by the Animal Ethics Committee of the China Agricultural University (Permit Number: AW02211202-1-2).
Animals and Experimental Design
480 1-day-old Arbor Acres broilers were randomly divided into 5 dietary treatments (each involving 6 replicate cages with 16 birds each). Low-Se corn (the Se deficient region of Heilongjiang Province, China) was used in the diet, and the basal diet without Se in the trace elements was used as the blank control group. The basal diet was formulated to meet or exceed the nutrient requirements for broilers recommended by National Research Council (1994). The ingredient and nutrient compositions of the basal diets are shown in Table 1. The experimental group was supplemented with 0.10, 0.20, 0.30 and 0.40 mg/kg SeNPs. The analyzed selenium content of the 5 experimental diets was 0.10, 0.19, 0.25, 0.37, and 0.43 mg/kg for the starter phase (0 to 21 d). The experimental period was 21 days. All diets were pelleted and crumbled.
The SeNPs were provided by the Beijing Wahmix Bio-technology Co. Ltd. (Tangshan, China). SeNPs were transformed from sodium selenite by the unmodified Bacillus subtilis S12 via fermentation, which converted sodium selenite into red SeNPs with a diameter of 170 nm. The proportion of SeNPs was 98%, and the SeNPs premix was created by mixing the raw product with starch for a final Se content of 3,694 mg/kg.
Sample Collection
Incidence of mortality was recorded daily during the experimental period. On d 21, 6 birds (one bird per cage) per treatment were randomly selected, electrically stunned, and euthanized by cervical dislocation. Samples of the middle jejunum (1 cm) were excised for morphological analysis. Three pieces of the middle jejunum (0.5 cm) samples were collected and cleaned with sterile PBS, rapidly frozen in liquid nitrogen, and transferred to −80 °C for RNA and protein analysis.
The histological characteristics of Jejunum Tissues
The formalin-fixed paraffin-embedded jejunum tissues were sectioned (5–6 μm) using a microtome and adhered to glass slides. Samples were deparaffinized with xylene and washed in 100% ethanol. Cells expressing mucin glycoprotein were identified using a combined periodic acid Schiff stain (PAS) staining. Under the Leica microscope (DMI803250593, Heidelberg, Germany), the intestinal villus height (VH) and crypt depth (CD) were measured by randomly selecting 10 intact villi for every bird. The density of goblet cells was expressed as the number of goblet cells per 100 μm (19). The method was calculated by randomly selecting 10 intact villi and measuring the number of goblet cells in corresponding villi under the mid-villi area (100 μm in length) (19).
Validation of Differentially Expressed Gene by Quantitative RT-PCR
The total RNA was isolated from the jejunum by TRIzol reagent according to the manufacturer's protocol (9109, TaKaRa, Tokyo, Japan). RNA quantity was determined using a NanoDrop 2000 spectrophotometer (Thermo Fisher, Waltham, MA, USA). Then, first-strand cDNA was synthesized using PrimeScript™ RT reagent Kit (RR047A, TaKaRa). One-step real-time PCR was performed with the 2 × RealStar Green Fast Mixture (A301-05, GenStar, Beijing, China) using an CFX96 Touch fluorescence quantitative PCR instrument (Bio-Rad, California, USA) in accordance with the manufacturer's guidelines. The primer pairs were designed based on genomic data of Gallus domesticus in NCBI. Primer sequences of the GAPDH, claudin-1, claudin-3, claudin-5, zonula occluden-1 (ZO-1), ZO-2; Mucin2 (Muc2); B-cell lymphoma-2 (Bcl-2), cysteinyl aspartate specific proteinase-3 (Caspase-3), and Bcl2-associated X (Bax) used in the study were listed in Table 2. All the measurements were carried out in triplicate (N = 6, the cage was used as experimental unit) and the average values were calculated. The relative transcription levels of genes in different groups were calculated relative to GAPDH (the normalizer) using the 2 −ΔΔCT method.
TUNEL Assay
Jejunum tissue specimens were embedded in paraffin and sectioned at 5 μm for processing by the TUNEL method using the DAB (SA-HRP) Tunel Cell Apoptosis Detection Kit (G1507, Servicebio Technology, Wuhan, China) in accordance with the manufacturer's guidelines. A total of 5 representative randomly chosen, non-adjacent, non-overlapping fields were counted for each TUNEL-stained tissue sample. Specimens were evaluated by the Leica microscope at 400× magnification for cell counting. ImageJ software (National Institute of Health) was used to assess the area and density of dyed region, and the integrated option density (IOD) value of the TUNEL-stained section. Finally, we calculated the mean density (20).
Measurements of the Goblet Cell Differentiation Factors, Inflammatory Factors and Pyroptosis Genes by q-PCR
The mRNA levels of Krüppel-like factor 4 (Klf4), NF-E2-related factor 2 (Nrf2), v-myc avian myelocytomatosis viral oncogene homolog(c-myc); interleukin 1 beta (IL-1β), IL-6, IL-8, IL-18; NLR family pyrin domain containing 3 (NLRP3), cysteinyl aspartate specific proteinase-1 (Caspase-1), toll-like receptor-2 (TLR-2), TLR-4, and myeloid differentiation factor88 (MyD88) genes were evaluated by quantitative RT-PCR. Primer sequences used in the study were listed in Table 2. All the measurements were carried out in triplicate (N = 6, the cage was used as experimental unit) and the average values were calculated. Expression levels of the target gene were evaluated using a relative quantification approach (2−ΔΔCT method) against GAPDH levels.
Measurements of the Goblet Cell Differentiation Factors, Inflammatory Factors and Pyroptosis Protein by Western Blotting
Western blotting for differentially expressed proteins was performed according to a previously reported method (21). Jejunum tissue extracts were prepared with the RIPA buffer. Proteins were separated by 10% SDS-PAGE gel; separated proteins were transferred onto PVDF membranes (IPVH00010, Millipore, Massachusetts, USA) and were probed with the following primary antibodies: Anti-c-Myc Mouse mAb (PTM-5028, Jingjie PTM BioLab, Hangzhou, China); Caspase 1 Antibody (AF5418, Affinity Biosciences, Liyang, China); and Anti-beta Actin Rabbit mAb (PTM-5143, Jingjie PTM BioLab). At last, the blots were visualized by the BeyoECL Plus (P0018S, Beyotime, Shanghai, China). The representative blot from four independent experiments was presented for each protein. The relative levels of protein expression were calculated using densitometric scans by ImageJ software and were normalized to the GAPDH levels from four independent experiments. For each independent experiment, the relative levels of protein expression were calculated.
Measurements of Reactive Oxygen Species in Jejunum Tissue by ELISA
100 mg jejunum tissue was weighed and homogenized with 900 μL PBS, and the supernatant was collected after centrifugation at 845 g for 15 min. The supernatant was measured using chicken reactive oxygen species (ROS) ELISA Kit (MM-6012001, Jiangsu MEIMIAN, Yancheng, China) based on the manufacturer's instructions. Then, the concentration was normalized by the weight of the jejunum sample and expressed as pg/mL of tissue.
Statistical Analysis
Data were statistically analyzed by one-way analysis of variance (ANOVA) using SPSS 20.0 software (SPSS Inc. Chicago, IL). All data were tested for homogeneity of variances using Levene's test. We analyzed different supplemented concentrations of SeNPs to the basal diet to evaulate linear or quadratic responses. The significance among the groups was identified using the Duncan test for multiple comparisons. Results were presented as means ± SEM. Significance was accepted at P < 0.05. The regression equation was calculated by Microsoft Excel (2016).
Results
Effects of SeNPs on the Mortality of Broilers
Dietary supplementation of SeNPs could effectively reduce the mortality of broilers (Figure 1).
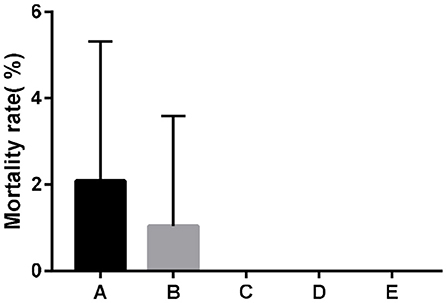
Figure 1. Effects of SeNPs on the mortality of broilers. A, B, C, D, E represent the basal diet supplemental with 0, 0.1, 0.2, 0.3, 0.4 mg/kg SeNPs, respectively. All data were presented as mean ± SEM (n = 6).
Effects of SeNPs on Jejunal Morphology
The VH (y = −7824.9x2 + 3412.9x + 1529.6, R2 = 0.8042, P < 0.05) and the VH/CD ratio (y = −36.357x2 + 19.273x + 7.1029, R2 = 0.8222, P < 0.05) of the jejunum showed a significant quadratic effect with increasing the concentration of SeNPs (Table 3, Figure 2A). The VH of jejunum reached the highest value after adding 0.1 mg/kg SeNPs, and the VH/CD of jejunum reached the highest value after adding 0.30 mg/kg SeNPs.
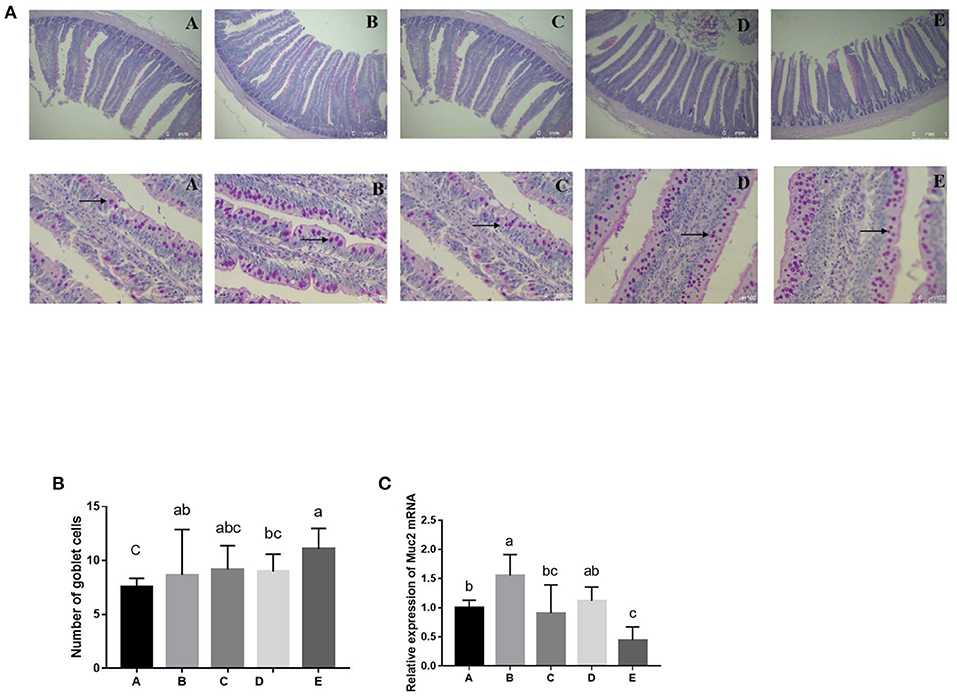
Figure 2. Effects of SeNPs on goblet cells of jejunum. (A) The staining of goblet cells (black arrow) by the PAS method. (B) The number of goblet cells in different groups. (C) mRNA levels of Muc2 in jejunum analyzed by real-time qPCR. A, B, C, D, E represent the basal diet supplemental with 0, 0.1,0.2,0.3,0.4 mg/kg SeNPs, respectively. All data were presented as mean ± SEM (n = 6). a,b,c means without common superscripts differ significantly (P < 0.05). SeNPs, selenium nanoparticles; PAS, periodic acid Schiff stain; Muc2, mucin 2.
Effects of SeNPs on mRNA Expression of Jejunal Barrier Protein-Related Genes
The mRNA expression of ZO-1 (y = −1.7818x + 0.8816, R2 = 0.855, P < 0.01), ZO-2 (y = −1.5657x + 0.8018, R2 = 0.6574, P < 0.05), claudin-3 (y = −1.8927x + 0.945, R2 = 0.9657, P < 0.01), and claudin-5 (y = −1.9663x + 0.7722, R2 = 0.7277, P < 0.01) in the jejunum decreased linearly with the increasing dose of SeNPs (Table 4). Compared with the control group, the mRNA expressions of claudin-1 in the jejunum were increased by adding 0.1 mg/kg SeNPs (P < 0.01), and the mRNA expression levels were significantly reduced by adding 0.2 and 0.3 mg/kg SeNPs.
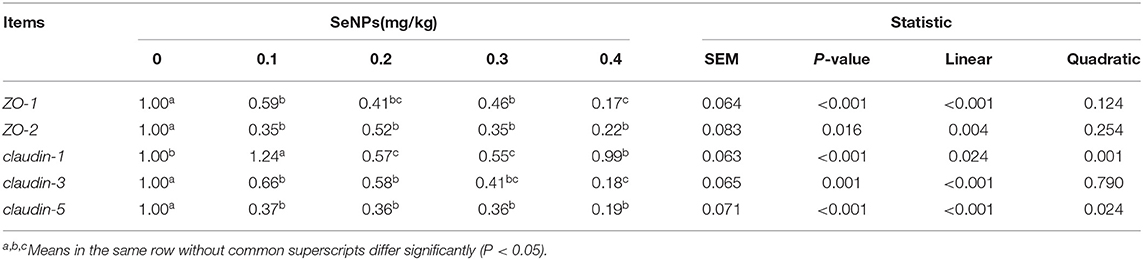
Table 4. Effects of SeNPs on the mRNA expression of jejunal barrier protein-related genes of broilers.
Effects of SeNPs on the Numbers of Goblet Cells and the mRNA Level of Muc2 of the Jejunum
Compared with the control group, the number of goblet cells in the jejunum was significantly increased by adding 0.1 and 0.4 mg/kg SeNPs (P < 0.01) (Figures 2A,B). In addition, the mRNA expression of Muc2 (y = −8.9205x2 + 2.3609x + 0.974, R2 = 0.7224, P < 0.01) showed a significant quadratic relationship that increased after adding 0.1 mg/kg SeNPs (Figure 2C).
Effects of SeNPs on Goblet Cell Differentiation Regulator of Jejunal
The mRNA expression of Klf4 (y = −1.64x + 1.072, R2 = 0.9264, P < 0.05) and c-myc (y = −1.4836x + 1.0333, R2 = 0.6843, P < 0.01) in the jejunum decreased linearly with the increasing dose of SeNPs (Table 5). Compared with the control group, the mRNA expression of Nrf2 in the jejunum was significantly decreased by adding 0.2 and 0.4 mg/kg SeNPs (P < 0.01) (Table 5). Moreover, dietary SeNPs also decreased linearly the protein expression level of c-myc (y = −1.6389x + 0.9075, R2 = 0.8976, P < 0.01) (Figure 3).
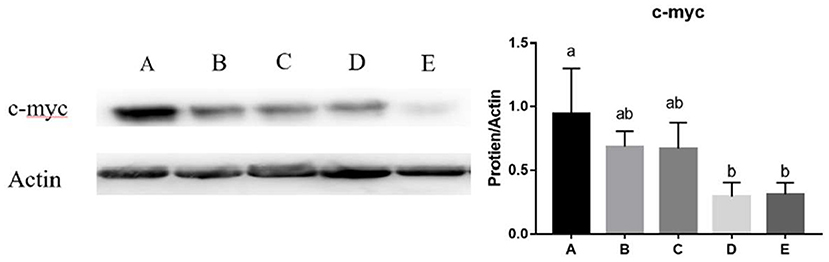
Figure 3. Effects of SeNPs on the protein expression of jejunal c-myc. A, B, C, D, E represent the basal diet supplemental with 0, 0.1, 0.2, 0.3, 0.4 mg/kg SeNPs, respectively. Values are presented as mean ± SEM (n = 4). a,b Significant differences are indicated by different letters (P < 0.05). SeNPs, selenium nanoparticles.
Effects of SeNPs on the Inflammation and Oxidative Stress of the Jejunum
The mRNA expression of IL-1β (y = −1.9663x + 0.7722, R2 = 0.7277, P < 0.01) and the concentration of ROS (y = −1.5657x + 0.8018, R2 = 0.6574, P < 0.01) in the jejunum decreased linearly with the increase of SeNPs concentration (Table 6). Compared with the control group, the mRNA expressions of IL-8 in the jejunum were significantly increased by adding 0.1 mg/kg SeNPs (P < 0.05) (Table 6).
Effects of SeNPs on Apoptosis of the Jejunum
As shown in Figure 4, the mean density of TUNEL positive cells in the 0.2 and 0.4 mg/kg SeNPs group were significantly lower than the control group. Furthermore, by detecting apoptosis-related genes, we also found that the mRNA expression of Bax (y = −1.3905x + 0.828, R2 = 0.6831, P < 0.01) and Bcl-2 (y = −2.2442x + 0.8208, R2 = 0.8149, P < 0.01) in the jejunum decreased linearly with the increasing dose of SeNPs (Table 7).
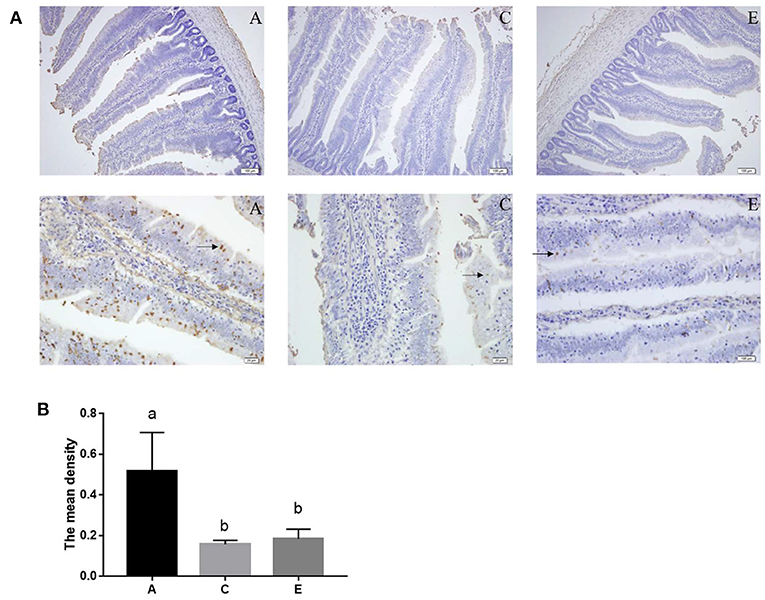
Figure 4. Effects of SeNPs on apoptosis of jejunum. (A) The staining of apoptotic cells (black arrow) by the TUNEL method. (B) The mean density of the TUNEL-stained section in different groups. A, C, E represent the basal diet supplemental with 0, 0.2, 0.4 mg/kg SeNPs, respectively. Values are presented as mean ± SEM (n = 6). a,b Significant differences are indicated by different letters (P < 0.05). SeNPs, selenium nanoparticles.
Effects of SeNPs on Pyroptosis-Related Genes
The mRNA expression levels of NLRP3 (y = −1.2898x + 0.8725, R2 = 0.761, P < 0.05), Casepase-1(y = −1.2214x + 1.0971, R2 = 0.671, P < 0.05), IL-18(y = −1.2262x + 0.8488, R2 = 0.6945, P < 0.05), TLR2(y = −1.6146x + 0.8663, R2 = 0.8449, P < 0.05), and MyD88 (y = −1.8012x + 0.9246, R2 = 0.911, P < 0.05) in the jejunum decreased linearly with the increase of SeNPs concentration (Table 8). Compared with the control group, the mRNA expressions of TLR4 in the jejunum were significantly decreased by adding 0.1, 0.2 and 0.4 mg/kg SeNPs (P < 0.05) (Table 8). Moreover, as shown in Figure 5, we found that dietary SeNPs decreased linearly the protein expression level of Casepase-1 (y = −0.2362x + 1.5208,R2 = 0.7799, P < 0.01).
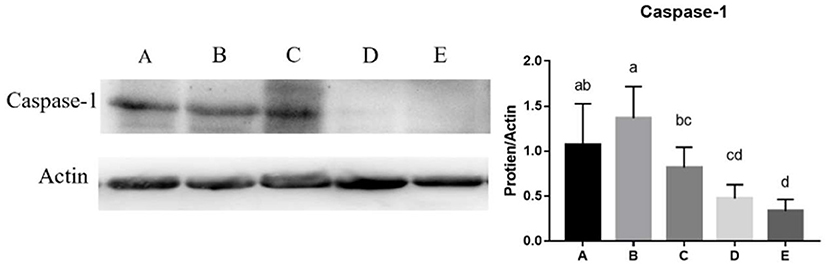
Figure 5. Effects of SeNPs on the protein expression of jejunal Casepase-1. A, B, C, D, E represent the basal diet supplemental with 0, 0.1, 0.2, 0.3, 0.4 mg/kg SeNPs, respectively. Values are presented as mean ± SEM (n = 6). a,b,c,d Significant differences are indicated by different letters (P < 0.05). SeNPs, selenium nanoparticles.
Discussion
Selenium supplementation is necessary for optimal growth of broilers (22, 23). It is believed that supplementing higher levels of Se could provide reduced mortality associated with marginal selenium deficiency syndrome (24). Under the conditions of this study, the results indicated that dietary SeNPs could reduce the mortality of broilers. Similarly, Zhou and Wang (25) also found that dietary SeNPs could significantly reduce broiler mortality. In commercial production, chicks may encounter challenges of pathogenic bacteria and oxidative stress, and the addition of SeNPs in the diet may reduce levels of inflammation and improve antioxidant status to improve the growth of broiler.
Intestinal Morphology and Intestinal Tight Junction
Changes in the intestinal morphology are related to the development of intestine and the surface area for nutrient absorption (26, 27). In the current trial, the VH of jejunum reached the highest value after adding 0.1 mg/kg SeNPs, and the VH/CD of jejunum reached the highest value after adding 0.3 mg/kg SeNPs. These were in agreement with the previous findings of Ali et al. (28) and Khajeh Bami et al. (29). The authors found that dietary SeNPs supplementation produced positive effects on some VH and VH/CD in the intestine of broilers. Dietary Se supplementation can promote intestinal structure by regulating intestinal flora (30). Tang et al. (31) found that the modified intestinal flora provided nutrition and stimulated the growth of intestinal villi. On the other hand, Se regulates the activity of inflammatory cytokines and increases the antioxidant status to promote the development of intestinal tissues (32). Subsequent experimental results also showed that SeNPs could effectively reduce levels of oxidative stress and inflammation in the intestine. Thus, we speculate that SeNPs can reduce the growth of pathogenic intestinal microbes and reduce the level of intestinal inflammation to improve the intestinal morphology.
Tight junctions (TJs) not only connect cells but also form channels that allow penetration between cells, resulting in varying epithelial surface tightness (33). Expression of Claudin-1,−3, and−5 leads to a very tight epithelia, coinciding with an increased transepithelial electrical resistance (TER) and decreased solute permeability (mainly sodium ions) across the epithelial monolayer (34, 35). The upregulation of tight junction proteins increased intestinal integrity and limited paracellular permeability, thus essentially helping the epithelial barrier to prevent pathogens from entering the body (36). However, TJs represent receptors for the toxins of pathogen (Clostridium perfringens enterotoxin) (36). Pathogenic bacteria utilized a subset of TJs as receptors to form pores in the membrane of intestinal epithelial cells, whereby Ca influx through these pores ultimately triggers intestinal cell injury (36). Therefore, the up- or down-regulation of tight junction proteins is difficult to interpret, which also the need to assess other factors (the microbial community) that affect gut health. The results of the present study showed that supplementation of SeNPs reduced the ZO-1, ZO-2, claudin-3, and claudin-5 relative expression in the jejunum compared to control diet at 21 days of age. Similarly, the expression of intestinal claudin-1, ZO−1, and ZO-2 was significantly reduced in diets supplemented with SeNPs (28). Ali et al. (28) found that the reduction of claudin-1, ZO-1, and ZO-2 by SeNPs might reduce the sites to attaching the pathogen bacteria. Meanwhile, the products of oxidative stress also increased intestinal permeability by decreasing expression levels of tight junction proteins (19, 37). The increase of Se in the diet reduced the influence of heat stress on the integrity of the intestinal barrier by reducing oxidative stress products (38). Subsequent experiment results also revealed that there was few ROS in the intestine, the reduction of tight junction protein expression increased the paracellular permeability, which was conducive to the absorption of nutrients.
Goblet Cells and Goblet Cell Differentiation Regulator
The intestinal mucus layer is one of the main mechanical barriers with an inner, attached mucus and an outer, unattached, loose mucus layer (39). The major building block in mucus is Muc2, which is mainly synthesized and secreted by goblet cells (40). The destruction of the mucus barrier by pathogenic bacteria may make the goblet cells compensatorily increase the synthesis and secretion of Muc2 to expel the invasion of pathogenic bacteria (41). Moreover, goblet cells could protect the intestinal crypts from the invasion of bacteria, which had penetrated the inner mucus layer (12). Selenium deficiency during the growth of the broiler could increase cytoplasm vacuolization and dissolution of goblet cells (42). It was reported that SeNPs could increase the number of goblet cells and improve the structure of mucin in the mouse jejunum (14, 18). Khajeh Bami et al. (29) also found that broilers fed 0.3 mg/kg SeNPs had higher goblet cell density in the ileum and jejunum. Similarly, the results of this experiment showed that the number of goblet cells and the mRNA expression of Muc2 in the jejunum were significantly increased by adding 0.1 mg/kg SeNPs.
Klf4 is a zinc finger transcription factor that is expressed in intestinal epithelial villi cells and can promote cell differentiation and tissue homeostasis (43). C-myc is a critical downstream effector of cellular proliferation induced by the Wnt/β-catenin pathway (44), which is essential for maintaining intestinal homeostasis and regeneration (45). Nrf2 is a master regulator of cellular oxidative levels against environmental stresses, which regulates intestinal cell differentiation by the KEAP1-Nrf2 pathway (46). SeNPs could alleviate intestinal epithelial barrier dysfunction caused by oxidative stress via Nrf2 signaling pathway (47). At present, there are few references on the regulation and control of SeNPs on the development of goblet cells in the intestine of broilers. This experiment found that the mRNA expression of Klf4, c-myc, and Nrf2 in the jejunum decreased significantly after adding 0.4 mg/kg SeNPs. These results demonstrated that low-dose SeNPs could effectively increase the number of goblet cells, and high-dose SeNPs might be toxic to intestinal cells and inhibited the differentiation of intestinal goblet cells.
Intestinal Inflammation and Antioxidant Activity
ROS are produced by intestinal epithelial cells through oxygen metabolism and by enteric commensal bacteria (48, 49). When the production of ROS exceeds the antioxidant capacity of cells, oxidative stress occurs and causes oxidative damage to tissues and cells, even causing bodily disorders (50, 51). Selenium can activate the GPX and TrxR through the formation of Sec in biological systems, which makes them suitable for oxidation/reduction reactions (1, 2, 52). SeNPs have been shown to have an effect on intestinal histology by high antioxidant capacity and free radical scavenging efficiency, which can protect intestinal tissues from oxidative damage (32). Selenium deficiency can induce villi cell apoptosis via an oxidative stress-induced mitochondrial apoptosis pathway and decreased the antioxidant capacity in chickens (15, 42). The secretion of mucin from intestinal goblet cells depends on the production of ROS (11). Salmonella infection activated the Notch signaling pathway through ROS in the intestine, leading to the loss of goblet cells (53). The current study found that the concentration of ROS in the jejunum decreased significantly with the increase of SeNPs concentration.
The pro-inflammatory cytokines of the avian intestinal mucosa play a key role in the host response to infectious pathogens (54). IL-1β is a pro-inflammatory cytokine that regulates antibody and cell-mediated immune responses (55). IL-8 is a CXC chemokine that attracts leukocytes (mainly neutrophils) to the sites of inflammation (54). Selenium deficiency induced chicken intestinal villi cell apoptosis by an inflammatory signaling-induced death receptor pathway and attenuated chicken intestinal mucosal immunity via activation of NF-κB signaling pathway regulated by redox activity (15, 42). Studies showed that in ovo injection with Se could increase intestinal levels of gene transcripts encoding IL-1β, IL-6, and IL-8 to enhance immune responses against avian necrotic enteritis (56, 57). Since this experiment did not establish the oxidative damage model, this study showed that the mRNA expression of IL-1β in the jejunum decreased significantly with the increase of SeNPs concentration, and diet supplemented with higher doses of SeNPs did not promote the expression of IL-8. These results demonstrated that exogenous supplementation with SeNPs effectively reduced levels of oxidative stress and inflammation in the intestine.
Pyroptosis
Cell death is an important process in development, homeostasis, and immune regulation of multicellular organisms (58). Overproduction of ROS, pathogen, and host molecules, can lead to cellar dysfunction, which further initiates inflammation and cell death (58, 59). This study, by the TUNEL method, was the first to show that TUNEL positive cells in the 0.2 and 0.4 mg/kg SeNPs groups were significantly lower than the control group. Similarly, the mRNA expression of Bax and Bcl-2 in the jejunum decreased significantly with the increasing dose of SeNPs. In contrast, Se deficiency induced duodenal villi cell apoptosis and injury of the mucosal physical barrier of the small intestine (15, 42). The NLRP3/caspase-1/IL-1β pathway is considered to be main active pathway of inflammation, which can be triggered by increased ROS and pathogens in order to protect against infections (58). When the pathway is activated, NLRP3 and ASC combine to each other to activate caspase-1, resulting in IL-1β-driven inflammation (58, 60). The secretion of mucin from intestinal goblet cells depends on the assembly and activation of inflammasomes (11). Among them, NLRP6 can regulate the secretion of Muc2 in goblet cells and promote the formation of mucus layers (61). The intestinal inflammation activated the NLRP6 in the goblet cells to regulate the secretion of Muc2 and promote the formation of mucus layers (62). Goblet cells nonspecifically endocytosed and reacted to the TLR ligands by activating the NLRP6 downstream of TLR- and MyD88-dependent ROS synthesis (12). Moreover, NLRP3 could alleviate the oxidative stress and inflammation of chicken kidneys, and participate in the antagonistic effect of Se on inflammation caused by lead (63). There were few reports on the role of inflammasome in regulating intestinal cells of broilers. It was showed that the expression levels of NLRP3, Casepase-1, IL-18, TLR2, and MyD88 in the jejunum decreased linearly with the increase of SeNPs concentration. Similarly, Selenomethionine reduced lipopolysaccharide-induced inflammation by inhibiting NLRP3 signaling pathway in chicken liver (64). Selenium also alleviated Pb-induced oxidative stress and inflammation by inhibiting the TLR - NLRP3 signaling pathway in chicken testes (65). Taken together, it is suggested that SeNPs reduced intestinal oxidative stress and inflammation by NLRP3/caspase-1/IL-1β signaling pathway.
Conclusion
In conclusion, exogenous supplementation with 0.2 mg/kg SeNPs can decrease intestinal oxidative stress and inflammation by modifying the activation of NLRP3 signaling pathway in chicken intestine, thereby effectively promoting goblet cells development of 21-day-old broilers.
Data Availability Statement
The original contributions presented in the study are included in the article/supplementary material, further inquiries can be directed to the corresponding authors.
Ethics Statement
The animal study was reviewed and approved by the Animal Ethics Committee of the China Agricultural University.
Author Contributions
YW and JY designed the research. YC performed the experiments, wrote the manuscript, and analyzed the data. XL and SL performed the experiments. WW and HZ participated in the revision of the manuscript. YG and YL provided experimental guidance and experimental materials. All authors contributed to data interpretation and approved the final version of the article.
Funding
This research was supported by National Key R&D Program of China (2021YFD1300404).
Conflict of Interest
YL was employed by Beijing Wahmix Bio-technology Co., Ltd.
The remaining authors declare that the research was conducted in the absence of any commercial or financial relationships that could be construed as a potential conflict of interest.
Publisher's Note
All claims expressed in this article are solely those of the authors and do not necessarily represent those of their affiliated organizations, or those of the publisher, the editors and the reviewers. Any product that may be evaluated in this article, or claim that may be made by its manufacturer, is not guaranteed or endorsed by the publisher.
References
1. Labunskyy VM, Hatfield DL, Gladyshev VN. Selenoproteins: molecular pathways and physiological roles. Physiol Rev. (2014) 94:739–77. doi: 10.1152/physrev.00039.2013
2. Messarah M, Klibet F, Boumendjel A, Abdennour C, Bouzerna N, Boulakoud MS, et al. Hepatoprotective role and antioxidant capacity of selenium on arsenic-induced liver injury in rats. Exp Toxicol Pathol. (2012) 64:167–74. doi: 10.1016/j.etp.2010.08.002
3. Chen T, Wong YS, Zheng W. Purification and characterization of selenium-containing phycocyanin from selenium-enriched spirulina platensis. Phytochemistry. (2006) 67:2424–30. doi: 10.1016/j.phytochem.2006.08.004
4. Biswas KC, Barton LL, Tsui WL, Shuman K, Gillespie J, Eze CS, et al. Novel method for the measurement of elemental selenium produced by bacterial reduction of selenite. J Microbiol Methods. (2011) 86:140–4. doi: 10.1016/j.mimet.2011.04.009
5. Li DB, Cheng YY, Wu C, Li WW Li N, Yang ZC, et al. Selenite reduction by shewanella oneidensis Mr-1 is mediated by fumarate reductase in periplasm. Sci Rep. (2014) 4:3735. doi: 10.1038/srep03735
6. Xu C, Qiao L, Guo Y, Ma L, Cheng Y. Preparation, characteristics and antioxidant activity of polysaccharides and proteins-capped selenium nanoparticles synthesized by Lactobacillus casei Atcc 393. Carbohydr Polym. (2018) 195:576–85. doi: 10.1016/j.carbpol.2018.04.110
7. Hosnedlova B, Kepinska M, Skalickova S, Fernandez C, Ruttkay-Nedecky B, Peng Q, et al. Nano-selenium and its nanomedicine applications: a critical review. Int J Nanomedicine. (2018) 13:2107–28. doi: 10.2147/IJN.S157541
8. Skalickova S, Milosavljevic V, Cihalova K, Horky P, Richtera L, Adam V. Selenium nanoparticles as a nutritional supplement. Nutrition. (2017) 33:83–90. doi: 10.1016/j.nut.2016.05.001
9. Clavijo V, Flórez MJV. The gastrointestinal microbiome and its association with the control of pathogens in broiler chicken production: a review. Poult Sci. (2018) 97:1006–21. doi: 10.3382/ps/pex359
10. Johansson ME, Sjövall H, Hansson GC. The gastrointestinal mucus system in health and disease. Nat Rev Gastroenterol Hepatol. (2013) 10:352–61. doi: 10.1038/nrgastro.2013.35
11. Birchenough GM, Johansson ME, Gustafsson JK, Bergström JH, Hansson GC. New developments in goblet cell mucus secretion and function. Mucosal Immunol. (2015) 8:712–9. doi: 10.1038/mi.2015.32
12. Birchenough GM, Nyström EE, Johansson ME, Hansson GC. A sentinel goblet cell guards the colonic crypt by triggering Nlrp6-dependent Muc2 secretion. Science. (2016) 352:1535–42. doi: 10.1126/science.aaf7419
13. Oeser K, Schwartz C, Voehringer D. Conditional Il-4/Il-13-deficient mice reveal a critical role of innate immune cells for protective immunity against gastrointestinal helminths. Mucosal Immunol. (2015) 8:672–82. doi: 10.1038/mi.2014.101
14. Wen ZS, Du M, Tang Z, Zhou TY, Zhang ZS, Song HH, et al. Low molecular seleno-aminopolysaccharides protect the intestinal mucosal barrier of rats under weaning stress. Int J Mol Sci. (2019) 20:5727. doi: 10.3390/ijms20225727
15. He X, Lin Y, Lian S, Sun D, Guo D, Wang J, et al. Selenium deficiency in chickens induces intestinal mucosal injury by affecting the mucosa morphology, siga secretion, and Gsh-Px activity. Biol Trace Elem Res. (2020) 197:660–6. doi: 10.1007/s12011-019-02017-6
16. Liu Z, Qu Y, Wang J, Wu R. Selenium deficiency attenuates chicken duodenal mucosal immunity via activation of the Nf-κb signaling pathway. Biol Trace Elem Res. (2016) 172:465–73. doi: 10.1007/s12011-015-0589-8
17. Qiao L, Dou X, Yan S, Zhang B, Xu C. Biogenic selenium nanoparticles synthesized by Lactobacillus casei Atcc 393 alleviate diquat-induced intestinal barrier dysfunction in C57bl/6 mice through their antioxidant activity. Food Funct. (2020) 11:3020–31. doi: 10.1039/D0FO00132E
18. Alkhudhayri AA, Dkhil MA, Al-Quraishy S. Nanoselenium prevents eimeriosis-induced inflammation and regulates mucin gene expression in mice jejunum. Int J Nanomedicine. (2018) 13:1993–2003. doi: 10.2147/IJN.S162355
19. Zhang Y, Mahmood T, Tang Z, Wu Y, Yuan J. Effects of naturally oxidized corn oil on inflammatory reaction and intestinal health of broilers. Poult Sci. (2021) 101:101541. doi: 10.1016/j.psj.2021.101541
20. Wang Y, Wu C, Qin Y, Liu S, Zhang R. Multi-angle investigation of the fractal characteristics of nanoscale pores in the lower Cambrian Niutitang shale and their implications for Ch4 adsorption. J Nanosci Nanotechnol. (2021) 21:156–67. doi: 10.1166/jnn.2021.18463
21. He Y, Ayansola H, Hou Q, Liao C, Lei J, Lai Y, et al. Genistein inhibits colonic goblet cell loss and colorectal inflammation induced by Salmonella typhimurium infection. Mol Nutr Food Res. (2021) 65:e2100209. doi: 10.1002/mnfr.202100209
22. Liao X, Liu G, Sun G, Sun X, Liu T, Lu L, et al. Determination of optimal dietary selenium levels by full expression of selenoproteins in various tissues of broilers from 1 to 21 D of age. Anim Nutr. (2021) 7:1133–44. doi: 10.1016/j.aninu.2021.02.009
23. Meng T, Liu YL, Xie CY, Zhang B, Huang YQ, Zhang YW, et al. Effects of different selenium sources on laying performance, egg selenium concentration, and antioxidant capacity in laying hens. Biol Trace Elem Res. (2019) 189:548–55. doi: 10.1007/s12011-018-1490-z
24. Cai SJ, Wu CX, Gong LM, Song T, Wu H, Zhang LY. Effects of nano-selenium on performance, meat quality, immune function, oxidation resistance, and tissue selenium content in broilers. Poult Sci. (2012) 91:2532–9. doi: 10.3382/ps.2012-02160
25. Zhou X, Wang Y. Influence of dietary nano elemental selenium on growth performance, tissue selenium distribution, meat quality, and glutathione peroxidase activity in guangxi yellow chicken. Poult Sci. (2011) 90:680–6. doi: 10.3382/ps.2010-00977
26. Abdelnour SA, Abd El-Hack ME, Alagawany M, Farag MR, Elnesr SS. Beneficial impacts of bee pollen in animal production, reproduction and health. J Anim Physiol Anim Nutr. (2019) 103:477–84. doi: 10.1111/jpn.13049
27. Xiong X, Yang H, Tan B, Yang C, Wu M, Liu G, et al. Differential expression of proteins involved in energy production along the crypt-villus axis in early-weaning pig small intestine. Am J Physiol Gastrointest Liver Physiol. (2015) 309:G229–37. doi: 10.1152/ajpgi.00095.2015
28. Ali F, Saeed K, Fatemeh H. Nano-bio selenium synthesized by bacillus subtilis modulates broiler performance, intestinal morphology and microbiota, and expression of tight junction's proteins. Biol Trace Elem Res. (2021). doi: 10.1007/s12011-021-02767-2
29. Khajeh Bami M, Afsharmanesh M, Espahbodi M, Esmaeilzadeh E. Effects of dietary nano-selenium supplementation on broiler chicken performance, meat selenium content, intestinal microflora, intestinal morphology, and immune response. J Trace Elem Med Bio. (2022) 69:126897. doi: 10.1016/j.jtemb.2021.126897
30. Zhai Q, Cen S, Li P, Tian F, Zhao J, Zhang H, et al. Effects of dietary selenium supplementation on intestinal barrier and immune responses associated with its modulation of gut microbiota. Environ Sci Technol Lett. (2018) 5:724–30. doi: 10.1021/acs.estlett.8b00563
31. Tang D, Li Z, Mahmood T, Liu D, Hu Y, Guo Y. The association between microbial community and ileal gene expression on intestinal wall thickness alterations in chickens. Poult Sci. (2020) 99:1847–61. doi: 10.1016/j.psj.2019.10.029
32. Safdari-Rostamabad M, Hosseini-Vashan SJ, Perai AH, Sarir H. Nanoselenium supplementation of heat-stressed broilers: effects on performance, carcass characteristics, blood metabolites, immune response, antioxidant status, and jejunal morphology. Biol Trace Elem Res. (2017) 178:105–16. doi: 10.1007/s12011-016-0899-5
33. Awad WA, Hess C, Hess M. Enteric pathogens and their toxin-induced disruption of the intestinal barrier through alteration of tight junctions in chickens. Toxins. (2017) 9:60. doi: 10.3390/toxins9020060
34. Van Itallie CM, Anderson JM. Claudins and epithelial paracellular transport. Annu Rev Physiol. (2006) 68:403–29. doi: 10.1146/annurev.physiol.68.040104.131404
35. Krause G, Winkler L, Piehl C, Blasig I, Piontek J, Müller SL. Structure and function of extracellular claudin domains. Ann N Y Acad Sci. (2009) 1165:34–43. doi: 10.1111/j.1749-6632.2009.04057.x
36. Eichner M, Protze J, Piontek A, Krause G, Piontek J. Targeting and alteration of tight junctions by bacteria and their virulence factors such as clostridium perfringens enterotoxin. Pflugers Arch. (2017) 469:77–90. doi: 10.1007/s00424-016-1902-x
37. Tan L, Rong D, Yang Y, Zhang B. Effect of oxidized soybean oils on oxidative status and intestinal barrier function in broiler chickens. Braz J Poultry Sci. (2018) 20:333–41. doi: 10.1590/1806-9061-2017-0610
38. Liu F, Cottrell JJ, Furness JB, Rivera LR, Kelly FW, Wijesiriwardana U, et al. Selenium and vitamin E together improve intestinal epithelial barrier function and alleviate oxidative stress in heat-stressed pigs. Exp Physiol. (2016) 101:801–10. doi: 10.1113/EP085746
39. Pelaseyed T, Bergström JH, Gustafsson JK, Ermund A, Birchenough GM, Schütte A, et al. The mucus and mucins of the goblet cells and enterocytes provide the first defense line of the gastrointestinal tract and interact with the immune system. Immunol Rev. (2014) 260:8–20. doi: 10.1111/imr.12182
40. Martens EC, Neumann M, Desai MS. Interactions of commensal and pathogenic microorganisms with the intestinal mucosal barrier. Nat Rev Microbiol. (2018) 16:457–70. doi: 10.1038/s41579-018-0036-x
41. Herp S, Brugiroux S, Garzetti D, Ring D, Jochum LM, Beutler M, et al. Mucispirillum schaedleri antagonizes salmonella virulence to protect mice against colitis. Cell Host Microbe. (2019) 25:681–94.e8. doi: 10.1016/j.chom.2019.03.004
42. Wang J, Liu Z, He X, Lian S, Liang J, Yu D, et al. Selenium deficiency induces duodenal villi cell apoptosis via an oxidative stress-induced mitochondrial apoptosis pathway and an inflammatory signaling-induced death receptor pathway. Metallomics. (2018) 10:1390–400. doi: 10.1039/C8MT00142A
43. Katano T, Bialkowska AB, Yang VW. Klf4 regulates goblet cell differentiation in Bmi1(+) reserve intestinal stem cell lineage during homeostasis. Int J Stem Cells. (2020) 13:424–31. doi: 10.15283/ijsc20048
44. Sansom OJ, Meniel VS, Muncan V, Phesse TJ, Wilkins JA, Reed KR, et al. Myc deletion rescues Apc deficiency in the small intestine. Nature. (2007) 446:676–9. doi: 10.1038/nature05674
45. Konsavage WM Jr, Jin G, Yochum GS. The Myc 3' Wnt-responsive element regulates homeostasis and regeneration in the mouse intestinal tract. Mol Cell Biol. (2012) 32:3891–902. doi: 10.1128/MCB.00548-12
46. Wen Z, Liu W, Li X, Chen W, Liu Z, Wen J, et al. A protective role of the Nrf2-keap1 pathway in maintaining intestinal barrier function. Oxid Med Cell Longev. (2019) 2019:1759149. doi: 10.1155/2019/1759149
47. Xu C, Qiao L, Ma L, Guo Y, Dou X, Yan S, et al. Biogenic selenium nanoparticles synthesized by Lactobacillus casei Atcc 393 alleviate intestinal epithelial barrier dysfunction caused by oxidative stress via Nrf2 signaling-mediated mitochondrial pathway. Int J Nanomedicine. (2019) 14:4491–502. doi: 10.2147/IJN.S199193
48. Mishra B, Jha R. Oxidative stress in the poultry gut: potential challenges and interventions. Front Vet Sci. (2019) 6:60. doi: 10.3389/fvets.2019.00060
49. Guo Q, Li F, Duan Y, Wen C, Wang W, Zhang L, et al. Oxidative stress, nutritional antioxidants and beyond. Sci China Life Sci. (2020) 63:866–74. doi: 10.1007/s11427-019-9591-5
50. Sinha K, Das J, Pal PB, Sil PC. Oxidative stress: the mitochondria-dependent and mitochondria-independent pathways of apoptosis. Arch Toxicol. (2013) 87:1157–80. doi: 10.1007/s00204-013-1034-4
51. Circu ML, Moyer MP, Harrison L, Aw TY. Contribution of glutathione status to oxidant-induced mitochondrial DNA damage in colonic epithelial cells. Free Radic Biol Med. (2009) 47:1190–8. doi: 10.1016/j.freeradbiomed.2009.07.032
52. Yin J, Wu M, Duan J, Liu G, Cui Z, Zheng J, et al. Pyrrolidine dithiocarbamate inhibits Nf-Kappab activation and upregulates the expression of Gpx1, Gpx4, Occludin, and Zo-1 in Dss-induced colitis. Appl Biochem Biotechnol. (2015) 177:1716–28. doi: 10.1007/s12010-015-1848-z
53. Xie S, Jiang L, Wang M, Sun W, Yu S, Turner JR, et al. Cadmium ingestion exacerbates salmonella infection, with a loss of goblet cells through activation of notch signaling pathways by Ros in the intestine. J Hazard Mater. (2020) 391:122262. doi: 10.1016/j.jhazmat.2020.122262
54. Shirley MW, Lillehoj HS. The long view: a selective review of 40 years of coccidiosis research. Avian Pathol. (2012) 41:111–21. doi: 10.1080/03079457.2012.666338
55. Lee SH, Lillehoj HS, Jang SI, Hong YH, Min W, Lillehoj EP, et al. Embryo vaccination of chickens using a novel adjuvant formulation stimulates protective immunity against eimeria maxima infection. Vaccine. (2010) 28:7774–8. doi: 10.1016/j.vaccine.2010.09.051
56. Lee SH, Lillehoj HS, Jang SI, Jeong MS, Xu SZ, Kim JB, et al. Effects of in ovo injection with selenium on immune and antioxidant responses during experimental necrotic enteritis in broiler chickens. Poult Sci. (2014) 93:1113–21. doi: 10.3382/ps.2013-03770
57. Lee SH, Lillehoj HS, Jang SI, Jeong M, Kim DK, Xu S, et al. Immune and anti-oxidant effects of in ovo selenium proteinate on post-hatch experimental avian necrotic enteritis. Vet Parasitol. (2014) 206:115–22. doi: 10.1016/j.vetpar.2014.10.025
58. Frank D, Vince JE. Pyroptosis versus necroptosis: similarities, differences, and crosstalk. Cell Death Differ. (2019) 26:99–114. doi: 10.1038/s41418-018-0212-6
59. Haghi-Aminjan H, Farhood B, Rahimifard M, Didari T, Baeeri M, Hassani S, et al. The protective role of melatonin in chemotherapy-induced nephrotoxicity: a systematic review of non-clinical studies. Expert Opin Drug Metab Toxicol. (2018) 14:937–50. doi: 10.1080/17425255.2018.1513492
60. Schneider KS, Groß CJ, Dreier RF, Saller BS, Mishra R, Gorka O, et al. The inflammasome drives gsdmd-independent secondary pyroptosis and Il-1 release in the absence of caspase-1 protease activity. Cell Rep. (2017) 21:3846–59. doi: 10.1016/j.celrep.2017.12.018
61. Wlodarska M, Thaiss CA, Nowarski R, Henao-Mejia J, Zhang JP, Brown EM, et al. Nlrp6 inflammasome orchestrates the colonic host-microbial interface by regulating goblet cell mucus secretion. Cell. (2014) 156:1045–59. doi: 10.1016/j.cell.2014.01.026
62. Volk JK, Nyström EEL, van der Post S, Abad BM, Schroeder BO, Johansson Å, et al. The Nlrp6 inflammasome is not required for baseline colonic inner mucus layer formation or function. J Exp Med. (2019) 216:2602–18. doi: 10.1084/jem.20190679
63. Huang H, Chen J, Sun Q, Liu Y, Tang Y, Teng X. Nlrp3 inflammasome is involved in the mechanism of mitigative effect of selenium on lead-induced inflammatory damage in chicken kidneys. Environ Sci Pollut Res Int. (2021) 28:10898–908. doi: 10.1007/s11356-020-11322-w
64. Qu JR, Wang W, Zhang QJ Li S. Inhibition of lipopolysaccharide-induced inflammation of chicken liver tissue by selenomethionine via Tlr4-Nf-Kappa B-Nlrp3 signaling pathway. Biol Trace Elem Res. (2020) 195:205–14. doi: 10.1007/s12011-019-01841-0
Keywords: selenium nanoparticles (SeNPs), goblet cells, NLRP3, broiler, intestine
Citation: Chen Y, Wu W, Zhou H, Liu X, Li S, Guo Y, Li Y, Wang Y and Yuan J (2022) Selenium Nanoparticles Improved Intestinal Health Through Modulation of the NLRP3 Signaling Pathway. Front. Nutr. 9:907386. doi: 10.3389/fnut.2022.907386
Received: 29 March 2022; Accepted: 14 June 2022;
Published: 05 July 2022.
Edited by:
Zhaojun Wei, Hefei University of Technology, ChinaReviewed by:
Xin Wu, Chinese Academy of Sciences (CAS), ChinaHaijun Zhang, Feed Research Institute (CAAS), China
Copyright © 2022 Chen, Wu, Zhou, Liu, Li, Guo, Li, Wang and Yuan. This is an open-access article distributed under the terms of the Creative Commons Attribution License (CC BY). The use, distribution or reproduction in other forums is permitted, provided the original author(s) and the copyright owner(s) are credited and that the original publication in this journal is cited, in accordance with accepted academic practice. No use, distribution or reproduction is permitted which does not comply with these terms.
*Correspondence: Yongqiang Wang, vetwyq@cau.edu.cn; Jianmin Yuan, yuanjm@cau.edu.cn