Lipocalin-2 and Cerebral Stroke
- 1Department of Neurosurgery, The Affiliated Hospital of Kunming University of Science and Technology, Kunming, China
- 2Department of Neurosurgery, The First People’s Hospital of Yunnan Province, Kunming, China
Stroke is a common and devastating disease with an escalating prevalence worldwide. The known secondary injuries after stroke include cell death, neuroinflammation, blood-brain barrier disruption, oxidative stress, iron dysregulation, and neurovascular unit dysfunction. Lipocalin-2 (LCN-2) is a neutrophil gelatinase-associated protein that influences diverse cellular processes during a stroke. The role of LCN-2 has been widely recognized in the peripheral system; however, recent findings have revealed that there are links between LCN-2 and secondary injury and diseases in the central nervous system. Novel roles of LCN-2 in neurons, microglia, astrocytes, and endothelial cells have also been demonstrated. Here, we review the evidence on the regulatory roles of LCN-2 in secondary injuries following a stroke from various perspectives and the pathological mechanisms involved in the modulation of stroke. Overall, our review suggests that LCN-2 is a promising target to promote a better understanding of the neuropathology of stroke.
Introduction
Stroke is a common, destructive disease arising from vascular anomalies and has a high disability and mortality rate. Cerebral strokes can be classified into ischemic and hemorrhagic strokes. Changes in ischemic stroke caused by the loss of blood flow, glucose, and oxygen due to vascular obstruction, including triggering a series of oxidative, biochemical, and hormonal responses, ultimately lead to microvascular damage and blood-brain barrier (BBB) disruption. The mass effect of hematoma in hemorrhagic stroke and a series of intertwined degenerative cascades, including inflammation, red blood cell degradation, and iron deposition, and thrombin production, with the presence of some ischemic lesions distant from the ischemic focus and other pathophysiological mechanisms, such as oxidative stress and apoptosis, lead to the destruction of the BBB, cerebral edema, and hydrocephalus, among others, forming a vicious circle.
Lipocalin-2 (LCN-2), a 25 kDa protein, is involved in various biological reactions. As an immunomodulator, dysregulation of LCN-2 plays a vital role in several pathogeneses. Moreover, LCN-2 is involved in the pathophysiological processes of secondary injury after stroke. Here, we review the role of LCN-2 in pathophysiological processes, such as neuroinflammatory responses, dysregulation of intracellular iron levels and oxidative stress, and BBB and neurovascular unit (NVU) dysfunction. Additionally, we review the link between LCN-2 and cells. These findings contribute to a better understanding of the mechanisms underlying the involvement of LCN-2 in secondary injury after stroke, providing a potential target for stroke therapy.
Origin, Structure, and Role of Lcn-2 in Cerebral Stroke
LCN-2 is a member of the lipocalin protein family. There are significant differences in the amino acid sequences of each member of the family, with <20% sequence homology between its members. LCN-2 has various biological functions. As an immunomodulator, alterations in LCN-2 levels are supposedly critical in many pathological processes. For instance, studies on humans indicated slightly elevated levels of LCN-2 in the plasma of patients with mild cognitive impairment (Choi et al., 2011) and the local concentration of LCN-2 in the brain tissue of patients with multiple sclerosis (Al Nimer et al., 2016). In a cerebral stroke, many cells express LCN-2. LCN-2 expression following ischemic stroke was reportedly increased both in the sera and brain, where it was localized to infiltrating neutrophils, cerebral endothelium, and a subset of astrocytes (Zamanian et al., 2012; Wang et al., 2015). LCN-2 expression was also found in astrocytes, microglia, neurons, and endothelial cells following intracerebral hemorrhage (ICH) in mice (Ni et al., 2015). However, LCN-2 is considered to be mainly expressed in astrocytes (Chia et al., 2011; Bi et al., 2013; Dong et al., 2013; Jin et al., 2014b; Table 1).
Lcn-2 and Various Pathophysiological Process
LCN-2 and NVU Dysfunction
NVU is defined by its function and anatomy (Harder et al., 2002) and is composed of endothelial cells, basement membrane, neurons, astrocytes, and pericytes (Amarenco et al., 2009; Abbott and Friedman, 2012; Gautam et al., 2020). It represents a conceptual framework that includes neurons and adjacent blood vessels (Iadecola, 2017). The interaction between the various components of NVU is extremely important and is gradually gaining attention (Lo and Rosenberg, 2009). The BBB and cerebral blood flow are precisely controlled by the NVU, thereby maintaining a homeostatic brain microenvironment (Armulik et al., 2010; Zlokovic, 2011). The disruption of the BBB, which is the core structure of the NVU, is an important part of early brain injury (Keep et al., 2018). In the study on the pathological mechanism of white matter damage caused by subarachnoid hemorrhage, LCN-2 was found to play an important role in the initiation and development of acute BBB disruption. LCN-2 deletion attenuates acute BBN leakage following subarachnoid hemorrhage (Egashira et al., 2016; Du et al., 2019). Another experiment showed that LCN-2 deficiency attenuated SAH-induced disruption of the white matter BBB, which further confirmed the effect of LCN-2 on the BBB in the opposite direction (Pang et al., 2017; Toyota et al., 2019). This dysfunction may be due to kainic acid-induced leakage of the BBB in the hippocampus (Shin et al., 2021).
Several neutrophils infiltrate and damage the BBB (Wang Z. et al., 2020) during a stroke. Additionally, matrix metallopeptidase 9 (MMP-9) could be involved in the LCN-2-mediated BBB damage (Turner and Sharp, 2016; Figure 1). LCN-2 can combine with MMP-9 through disulfide bonds to reduce the degradation of MMP-9 and prolong its activity, thereby enhancing the damaging effect of MMP-9 on the BBB (Weng and Chou, 2015). Endothelial cells mainly constitute specialized membranes around blood vessels, and their damage can result in the destruction of the BBB (Armulik et al., 2010). Interestingly, LCN-2 may regulate endothelial cells in the BBB (Gasterich et al., 2021; Figure 1). LCN-2 induces the expression of vascular endothelial growth factor A (VEGFA), which affects vascular permeability either directly or via astrocytes (Kim et al., 2017). HIF-1α induces the expression of both LCN-2 and VEGFA in astrocytes. In LCN-2–/– mice, the hypoxia-induced expression of VEGFA is suppressed in the astrocytes, indicating that LCN-2 may be an upstream signaling factor for VEGFA. The upregulation of VEGFA reduces the levels of ZO-1, occludin, and claudin-5 and alters their distributions (Mondal et al., 2020; Wang G. et al., 2020; Yang et al., 2020), thereby increasing the permeability of the BBB and leading to abrogation of the NVU (Figure 1). As immune cells in the central nervous system (CNS), astrocytes can control the contraction and relaxation of pericytes and smooth muscle cells, thereby regulating cerebral blood flow (Janzer and Raff, 1987; Zonta et al., 2003). Microglia is not classically included as a part of the NVU; however, it is closely related to the structure and function of the NVU. They can modulate the innate immunity of astrocytes by releasing various signaling molecules (Kirkley et al., 2017; Liu L. R. et al., 2020). Studies have shown that LCN-2 can activate glial cells, release pro-inflammatory factors, and cause damage to neurons (Han et al., 2014). The chemokines released by LCN-2 after activating glial cells can also induce the infiltration of leukocytes (Tuttolomondo et al., 2014; Jayaraj et al., 2019), thus causing BBB leakage. Several red blood cells and damaged cells enter the brain tissue through the damaged BBB, further increasing the damage to neurons. Neurons play an important role in the NVU. Research has shown that LCN-2 can not only damage neurons through glial cells but can also directly trigger oxidative stress and apoptosis of nerve cells (Huang et al., 2020), which may be caused by the transportation of a large amount of iron into the cell (Shin et al., 2021). Therefore, the various components of the NVU are closely related in terms of structure and function to maintain the stability of brain functions. After a stroke, the damaging effect of LCN-2 renders the NVU susceptible to continuous damage, which in turn aggravates the secondary damage following the stroke.
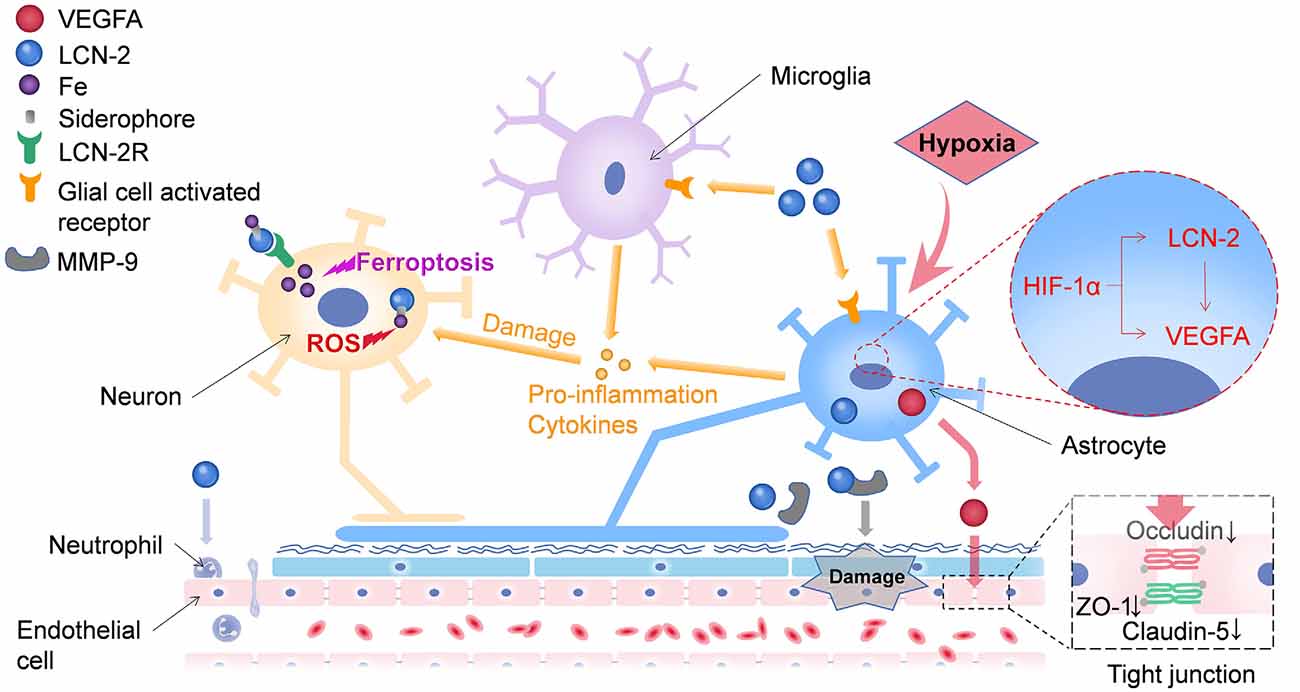
Figure 1. LCN-2 can damage neurons through multiple pathways. On one hand, Lipocalin-2 can transport iron into neurons by binding to LCN-2R, leading to the accumulation of intracellular iron, which in turn triggers ferroptosis and oxidative stress in neurons. On the other hand, LCN-2 can activate glial cells to release pro-inflammatory factors, which in turn damage neurons. LCN-2 has several ways to damage the BBB. First, it can promote the release of VEGFA from astrocytes, disrupt tight junctions between endothelial cells, and ultimately lead to leakage of the blood-brain barrier (BBB). Second, LCN-2 can bind to matrix metallopeptidase 9 (MMP-9), resulting in persistent BBB damage. Moreover, LCN-2 could induce neutrophil adhesion and damage the BBB. VEGFA, vascular endothelial growth factor A; LCN-2, lipocalin-2; LCN-2R, lipocalin-2 receptor; NVU, neurovascular unit; BBB, blood-brain barrier.
LCN-2 and Neuroinflammation
Neuroinflammation plays an important role in brain injury caused by hemorrhagic stroke (Wang and Doré, 2007). Studies have shown that LCN-2 is mainly expressed in astrocytes (Chia et al., 2011; Bi et al., 2013; Dong et al., 2013; Jin et al., 2014b) and is neurotoxic (Bi et al., 2013). However, other studies have found that inflammatory cells, such as microglia and neutrophils (Lee et al., 2007; Rathore et al., 2011; Jang et al., 2013b), can also express LCN-2. The effects of LCN-2 on microglia are complex. LCN-2 can increase the expression of M1-related genes in cultured mouse microglia. The expression of M1-related genes in microglia was significantly reduced in LCN-2-deficient mice, following lipopolysaccharide (LPS) injection (Jang et al., 2013b). LCN-2 expression is sensitive to cytotoxic agents, and inflammatory activation of microglia can lead to LCN-2 upregulation. Meanwhile, LCN-2 expression in BV-2 microglia induces changes in cell morphology. Microglial activation after ICH was weaker in LCN-2 knockout mice than in wild-type (WT) mice (Ni et al., 2015). This difference in microglial activation may contribute to differences in brain injury between WT and LCN-2 knockout mice after ICH.
In ischemic stroke, pro-inflammatory mediators regulated by LCN-2 play a key role in ischemia-reperfusion injury (Iadecola and Anrather, 2011). Afterastroke, iNOS expressed in microglia, astrocytes, endothelial cells, and infiltrating neutrophils releases large amounts of nitric oxide (NO; Iadecola and Anrather, 2011). NO reacts preferentially with reactive oxygen species (ROS) and forms peroxynitrite anion (ONNO−), which is a cytotoxic mitochondrial enzyme and genetic material. Inhibition of iNOS production by infiltrating neutrophils and brain endothelial cells provides prolonged neuroprotection after transient and permanent cerebral ischemia (Garcia-Bonilla et al., 2014). The level of interleukin-6 (IL-6), a pro-inflammatory cytokine, is elevated in plasma and brain 3–24 h after experimental stroke (Clark et al., 1999). Previous study results showed that in vitro exposure to IL-6 disrupted the integrity of the BBB by reducing the transendothelial electrical resistance (TEER) of the brain endothelial cells of rats (de Vries et al., 1996). CCL2 (monocyte chemoattractant protein 1, MCP-1) and CCL9 (macrophage inflammatory peptide gamma, MIP-1γ) are chemokines that are upregulated after ischemic stroke in humans (García-Berrocoso et al., 2014) and rodents (Shao et al., 2018). Furthermore, a previous study showed the involvement of CCL2 and its receptor “CCR2” in leukocyte trafficking after stroke (Conductier et al., 2010). Furthermore, studies have shown that genetic deletion of CCL2 (Hughes et al., 2002) and CCR2 (Dimitrijevic et al., 2007) reduces BBB permeability, accumulation of immune cells in ischemic brain tissue, and subsequent cerebral infarction (Chu et al., 2014). The role of CCL9 in stroke has not been investigated; however, deficiency of its receptor “CCR1” attenuates neutrophil adhesion to the vascular endothelium and migration to post-ischemic tissues (Reichel et al., 2006). In summary, these studies suggest that genetic or pharmacological inhibition of these pro-inflammatory mediators (iNOS, IL-6, CCL2, and CCL9) provides neuroprotection against stroke, which can affect pro-inflammatory mediators by modulating LCN-2.
Studies have shown that activated glial cells release cytokines and chemokines, which infiltrate leukocytes (Tuttolomondo et al., 2014; Jayaraj et al., 2019) and induce neuroinflammatory responses. Moreover, cytokines can damage neurons and destroy vascular and nerve coupling of the NVU (Figure 1). White blood cells can destroy the structure of the BBB, causing its leakage. Some studies have demonstrated that LCN-2 can promote the pro-inflammatory activation of glial cells and enhance the infiltration of neutrophils and macrophages into the brain under certain conditions.
LCN-2 and Oxidative Stress
Oxidative stress plays a critical role in stroke (Rodrigo et al., 2013; Wang et al., 2018; Fumoto et al., 2019; Reiche et al., 2019). Moreover, LCN-2 plays an important role in oxidative stress. We reviewed the existing basic studies and found that few studies investigate the role of LCN-2 in oxidative stress following stroke; however, many conclusions have been drawn from oxidative stress after other nervous system diseases, which may indicate the direction for the study of oxidative stress following stroke with LCN-2. For example, in the experiment of nerve injury caused by KA, LCN-2 deficiency was found to reduce oxidative stress response (Shin et al., 2021). Leptin-deficient obese ob/ob mice show that LCN-2 is robustly induced in the hippocampus following obesity, and acts in an inflammatory manner by increasing BBB leakage and iron accumulation-induced oxidative stress (Jin et al., 2020). The mechanism underlying this phenomenon could involve the transformation of LCN-2 into an unfolded state by iron-loaded siderophores, leading to an increase in the intracellular levels of ROS (Huang et al., 2020; Figure 1). In an experiment using a mouse model of NASH, LCN-2 in the systemic upregulates the expression of the LCN-2 receptor (24p3R) in brain cells and secretes the damage-associated molecular pattern protein (DAMP), a high mobility group box 1 (HMGB1) that subsequently induces oxidative stress and nod-like receptor protein 3 (NLRP3) inflammasome activation on the brain cells (Mondal et al., 2020). Therefore, LCN-2 may promote oxidative stress and prevent oxidation. These findings suggest that LCN-2 may be used as a biomarker to identify oxidative stress. The protracted periods of oxidative stress and neuroinflammation provide an opportunity for therapeutic interventions. Immunotherapy designed to target pro-inflammatory mediators as a means of improving stroke outcome has, therefore, attracted considerable scientific attention (Yu et al., 2013; Lambertsen et al., 2019).
LCN-2 and Iron Dysregulation
An increase in total iron content is observed in the lesion area in cases of both hemorrhagic and ischemic strokes (Tuo et al., 2017; Liu R. et al., 2020). The release of red blood cells primarily contributes to the presence of free iron after stroke. A large amount of free iron enters the brain parenchyma through the disrupted BBB and promotes ROS production by the Fenton reaction that induces oxidative stress and ferroptosis (Figure 1). Iron can also directly initiate toxic reactions, damage nerve cells, and cause NVU dysfunction (Righy et al., 2016; Liu J. et al., 2020). Furthermore, iron plays a major role in brain damage after ICH (Wagner et al., 2003; Xi et al., 2006). Brain non-heme iron increases after ICH in rats, and brain iron overload causes brain edema in the acute phase of ICH and brain atrophy thereafter (Xi et al., 2006; Keep et al., 2012). An iron chelator, deferoxamine, alleviates ICH-induced brain edema, neuronal death, brain atrophy, and neurologic deficits in rats and pigs (Xi et al., 2006; Keep et al., 2012; Xie et al., 2014). Clinical data also suggest that iron plays a role in ICH-induced brain injury. For example, clot lysis is associated with perihematomal edema development (Wu et al., 2006). Recent studies showed that high levels of serum ferritin, an iron storage protein, are independently associated with poor outcomes and severe brain edema in ICH patients (Mehdiratta et al., 2008; Pérez de la Ossa et al., 2010). LCN-2 is an acute-phase protein that is upregulated in inflammation, infection, and various injuries (Jha et al., 2015). It binds siderophores, which are secreted by microorganisms to scavenge iron (Goetz et al., 2002). However, evidence demonstrating the involvement of LCN-2 involved in iron homeostasis is increasing. A study showed that LCN-2 could be involved in cellular uptake or clearance of iron depending on iron status (Devireddy et al., 2005). Another report suggested that LCN-2 could mediate an alternative, transferrin-independent pathway for cellular iron delivery (Yang et al., 2002). In rats, LCN-2 is upregulated after ICH and may play a role in handling iron that is released from the hematoma during clot resolution (Dong et al., 2013). However, whether such a role is beneficial or detrimental is uncertain.
Overall, these results indicate that LCN-2 plays a role in iron-mediated brain injury after ICH. Until now, the detailed mechanism of iron delivery through LCN-2 has not been fully elucidated. In previous studies, LCN-2 was considered a mediator of an alternative, transferrin-independent pathway for cellular iron delivery (Yang et al., 2002). Iron is suggested to bind to an LCN-2-associated small molecular weight siderophore, transferred into cells through 24p3R, an LCN-2 cell-surface receptor, and subsequently released, resulting in an increased intracellular iron concentration (Flo et al., 2004; Devireddy et al., 2005). LCN-2 deficiency can block the pathway of LCN-2-dependent intracellular iron transportation, as suggested by the reduced iron-induced ferritin synthesis, and alleviate brain injury. However, studies showed that LCN-2 could regulate the intracellular iron concentration, and LCN-2 deficiency can increase the cellular iron levels in sepsis (Srinivasan et al., 2012). Thus, the role of LCN-2 in iron transport requires further study.
LCN-2 and Brain Cell Death
Unlike other CNS diseases, a stroke leads to the death of numerous brain cells (Lee et al., 2007, 2012; Bi et al., 2013; Jang et al., 2013a; Jin et al., 2014a, b; Wang et al., 2015; Kim et al., 2016, 2017; Bhusal et al., 2019; Chen et al., 2019, 2020; Deng et al., 2019; Braga et al., 2020), which can be directly or indirectly mediated by LCN-2. As mentioned earlier, LCN-2 can activate glial cells to release pro-inflammatory factors that directly damage neurons. Additionally, LCN-2 can induce leukocyte infiltration as well as neuroinflammation by releasing chemokines following the activation of glial cells. LCN-2 can also directly trigger oxidative stress and apoptosis in neural cells. When LCN-2 is ectopically expressed by the 24p3R gene in iron-deficient cells, it further reduces intracellular iron levels. In cell types, such as astrocytes, neurons, and neural stem cells, cellular iron deprivation mediated by LCN-2 leads to apoptosis (Devireddy et al., 2001, 2005; Lee et al., 2009, 2012; Ferreira et al., 2018a). Furthermore, LCN-2 induces the expression of the proapoptotic protein Bim, which causes apoptosis (Devireddy et al., 2005; Lee et al., 2012). However, other studies indicate that Bim is not essential for LCN-2-mediated apoptosis (Lee et al., 2007; Naudé et al., 2012). LCN-2 can also directly initiate neuronal death via mitochondria-related pathways (Chen et al., 2020): this phenomenon is believed to occur through the mitochondrial apoptotic pathway (Iurlaro and Muñoz-Pinedo, 2016; Hetz and Papa, 2018). Endoplasmic reticulum stress-induced cell death occurs through the ATF4/CHOP or IRE1/JNK pathways. However, another study indicated that tunicamycin-induced LCN-2 cells produce a stronger endoplasmic reticulum stress response than WT cells. Moreover, LCN-2 acts as a protective factor and cells lacking LCN-2 are more prone to injury (Borkham-Kamphorst et al., 2020). Recombinant LCN-2 directly induces apoptosis in dopaminergic neurons in a dose-dependent manner (Weng et al., 2021). Additionally, LCN-2 can induce cell death by promoting the accumulation of intracellular iron (Xu et al., 2012; Ni et al., 2015; Dekens et al., 2018).
However, there are some controversies regarding the promotion of apoptosis by LCN-2 in brain cells. First, the idea that LCN-2 can directly initiate apoptosis is controversial. LCN-2 can initiate cell death via inflammation or cytotoxicity (Lee et al., 2007, 2009, 2012; Naudé et al., 2012; Mesquita et al., 2014). Moreover, many studies have shown that LCN-2 can significantly induce cytotoxicity (Bi et al., 2013; Wang et al., 2015; Kim et al., 2016, 2017). Second, the cell types in which LCN-2 can mediate toxicity are unclear, with different experiments producing different results. For example, a study found that LCN-2 affects the survivability of neurons (Bi et al., 2013), while other studies suggested that LCN-2 increases the sensitivity of astrocytes and microglia to cell death (Lee et al., 2007, 2009; Mesquita et al., 2014; Mike et al., 2019). The differences between these results may be due to variations in methodologies. For example, in vivo and in vitro experiments yield different results. Finally, while most of the studies emphasize the cytotoxic effects of LCN-2, high levels of LCN-2 may stimulate the glial cells to transform into cells that protect neurons (Xing et al., 2014). The high levels of LCN-2 may indicate SOS for the damaged neurons. For example, overexpression of LCN-2 reduces apoptosis in gastric mucosal cells (Wen et al., 2021). LCN-2 can prolong the survival of ovarian clear cell carcinoma cells by reducing iron-related oxidative stress (Yamada et al., 2016). When liver cells are stressed or damaged, LCN-2 protects them from apoptosis induced by endoplasmic reticulum stress (Borkham-Kamphorst et al., 2020). These observations may provide a new perspective on the role of LCN-2 in neurological diseases and help explore the mechanism underlying cell death after stroke.
Lcn-2 Intervention Strategies
Studies have shown that neutralization of LCN-2 is a reasonable therapeutic strategy to alleviate reperfusion injury in stroke. Treatment with LCN-2 mAbs significantly attenuates LCN-2 mRNA and protein within a clinically relevant time window; however, targeting LCN-2 to inhibit post-stroke neuroinflammation may be more beneficial than inhibiting individual cytokines and chemokines, as LCN-2 may be responsible for the inflammatory cascade important upstream regulators of these mediators. Administration of LCN-2 mAb prior to full post-stroke LCN-2 elevation reduced the levels of LCN-2 and pro-inflammatory mediators (iNOS, IL-6, CCL2, and CCL9) and resulted in neutrophil infiltration, BBB leakage, cerebral infarction induction, and improved functional outcomes after stroke (Wang Z. et al., 2020). In addition to neutralizing LCN-2, other therapeutic approaches that inhibit the expression and secretion of LCN-2 (Cowland et al., 2006) or interfere with the interaction between LCN-2 and its receptors (Devireddy et al., 2005) are also potential avenues for therapeutic development (Suk, 2016).
Perspective
There are some controversial results regarding the role of LCN-2 in some pathological processes (Table 2). Several studies have shown that LCN-2 could aggravate neuroinflammation (Lee et al., 2011; Jang et al., 2013a, b; Jin et al., 2014a); however, some studies found that neuroinflammation worsened in the absence of LCN-2 (Berard et al., 2012; Nam et al., 2014; Dekens et al., 2018; Kang et al., 2018). Additionally, other contradictory studies indicate that LCN-2 does not affect neuroinflammation (Ip et al., 2011; Lattke et al., 2017; Vichaya et al., 2019; Gasterich et al., 2021). Next, the effect of LCN-2 activation on cells was slightly different across various experiments. A recent study showed that LCN-2 triggered the classical activation of astrocytes in mice with tMCAO (Zhao et al., 2019), and another study found that LCN-2 can reduce inflammation in the astrocytes (Deng et al., 2019). However, Mike et al. found that LCN-2 does not affect the activation of astrocytes but influences the activation of microglia (Mike et al., 2019). Moreover, LCN-2 is crucial in the effects of PRX-2 on neutrophil infiltration and microglia/macrophage activation, and ultimately brain damage (Zhang et al., 2021). Thus, the contradictory conclusions may be due to differences in disease models, factors of induced inflammation, disease stages, and cell types. However, they all provide valuable contributions to the further understanding of post-stroke neuroinflammation.
There are also some controversies regarding the role of LCN-2 in oxidative stress. In the conclusions mentioned earlier, LCN-2 is able to promote oxidative stress. However, one study found that the elevated expression of LCN-2 could reduce oxidative stress and the resulting cellular damage (Xiao et al., 2016; Yamada et al., 2016). In another study, knocking out the LCN-2 gene in mice increased oxidative stress, which could be attributed to an accumulation of active iron in neural stem cells (Ferreira et al., 2018a, b). Interestingly, LCN-2 can also exert antioxidant effects through several mechanisms. One mechanism may be that LCN-2 acts as an antioxidant by inducing the expression of heme oxygenase 1 (Song et al., 2015; Yamada et al., 2016). Another mechanism could be the overexpression of LCN-2 eliciting antioxidant effects by reducing the production of intracellular iron and protecting against ROS-induced oxidative stress (Xiao et al., 2016).
These controversial conclusions also exist in studies related to LCN-2 and iron. A study showed that LCN-2 contributes to iron accumulation (Shin et al., 2021). Another study validated the finding that a lack of LCN-2 significantly reduced Alzheimer’s disease-related hippocampal iron accumulation (Dekens et al., 2018). However, the absence of LCN-2 caused iron accumulation in certain cells, such as macrophages, hippocampal neurons, and neural stem cells (Nairz et al., 2009, 2015; Ferreira et al., 2018a). These controversial conclusions can provide new ideas for us to further explore the pathophysiological mechanism of LCN-2 in brain injury after stroke.
Conclusion
Despite the abovementioned controversial conclusions, there is a consensus that LCN-2 can exacerbate brain injury. Several risk factors cause an accumulation of LCN-2 in the brain, which aggravates brain damage. Overall, it can be hypothesized that an increase in LCN-2 levels in response to injury aggravates the risk of poor outcomes in stroke, including causing inflammation in the brain, iron dysregulation, and neurovascular dysfunction. However, current direct evidence is insufficient. Therefore, future research should evaluate the direct link between LCN-2 and secondary stroke injuries. Additionally, studies show that LCN-2 plays contradictory roles, indicating that the function of LCN-2 is highly complex; thus, it is necessary to further explore its mechanism of action. The levels of LCN-2 may also be affected by multiple factors, including sex, age, disease type, and cell type. In summary, we need to further explore the role of LCN-2 in secondary brain injury after stroke and increase the usage of LCN-2 levels in the diagnosis and treatment of stroke.
Author Contributions
All authors listed, have made substantial, direct and intellectual contribution to the work, and approved it for publication.
Funding
This work was supported by grants from the National Natural Science Foundation of China (grant number 81560227) and the Yunnan Health Training Project of High-level Talents (grant number H-2017030).
Conflict of Interest
The authors declare that the research was conducted in the absence of any commercial or financial relationships that could be construed as a potential conflict of interest.
Publisher’s Note
All claims expressed in this article are solely those of the authors and do not necessarily represent those of their affiliated organizations, or those of the publisher, the editors and the reviewers. Any product that may be evaluated in this article, or claim that may be made by its manufacturer, is not guaranteed or endorsed by the publisher.
References
Abbott, N. J., and Friedman, A. (2012). Overview and introduction: the blood-brain barrier in health and disease. Epilepsia 53, 1–6. doi: 10.1111/j.1528-1167.2012.03696.x
Al Nimer, F., Elliott, C., Bergman, J., Khademi, M., Dring, A. M., Aeinehband, S., et al. (2016). Lipocalin-2 is increased in progressive multiple sclerosis and inhibits remyelination. Neurol. Neuroimmunol. Neuroinflammation 3:e191. doi: 10.1212/NXI.0000000000000191
Amarenco, P., Bogousslavsky, J., Caplan, L. R., Donnan, G. A., and Hennerici, M. G. (2009). Classification of stroke subtypes. Cerebrovasc. Dis. 27, 493–501. doi: 10.1159/000210432
Armulik, A., Genové, G., Mäe, M., Nisancioglu, M. H., Wallgard, E., Niaudet, C., et al. (2010). Pericytes regulate the blood-brain barrier. Nature 468, 557–561. doi: 10.1038/nature09522
Berard, J. L., Zarruk, J. G., Arbour, N., Prat, A., Yong, V. W., Jacques, F. H., et al. (2012). Lipocalin 2 is a novel immune mediator of experimental autoimmune encephalomyelitis pathogenesis and is modulated in multiple sclerosis. Glia 60, 1145–1159. doi: 10.1002/glia.22342
Bhusal, A., Rahman, M. H., Lee, W.-H., Bae, Y. C., Lee, I.-K., and Suk, K. (2019). Paradoxical role of lipocalin-2 in metabolic disorders and neurological complications. Biochem. Pharmacol. 169:113626. doi: 10.1016/j.bcp.2019.113626
Bi, F., Huang, C., Tong, J., Qiu, G., Huang, B., Wu, Q., et al. (2013). Reactive astrocytes secrete lcn2 to promote neuron death. Proc. Natl. Acad. Sci. U S A 110, 4069–4074. doi: 10.1073/pnas.1218497110
Borkham-Kamphorst, E., Haas, U., Van de Leur, E., Trevanich, A., and Weiskirchen, R. (2020). Chronic carbon tetrachloride applications induced hepatocyte apoptosis in lipocalin 2 null mice through endoplasmic reticulum stress and unfolded protein response. Int. J. Mol. Sci. 21:5230. doi: 10.3390/ijms21155230
Braga, A., Bandiera, S., Verheyen, J., Hamel, R., Rutigliani, C., Edenhofer, F., et al. (2020). Combination of in situ Lcn2 pRNA-RNAi nanotherapeutics and iNSC transplantation ameliorates experimental SCI in mice. Mol. Ther. 28, 2677–2690. doi: 10.1016/j.ymthe.2020.08.001
Chen, X., Lu, J., Zhao, X., Chen, C., Qiao, D., Wang, H., et al. (2019). Role of C/EBP-β in methamphetamine-mediated microglial apoptosis. Front. Cell. Neurosci. 13:366. doi: 10.3389/fncel.2019.00366
Chen, X., Qiu, F., Zhao, X., Lu, J., Tan, X., Xu, J., et al. (2020). Astrocyte-derived lipocalin-2 is involved in mitochondrion-related neuronal apoptosis induced by methamphetamine. ACS Chem. Neurosci. 11, 1102–1116. doi: 10.1021/acschemneuro.9b00559
Chia, W. J., Dawe, G. S., and Ong, W. Y. (2011). Expression and localization of the iron-siderophore binding protein lipocalin 2 in the normal rat brain and after kainate-induced excitotoxicity. Neurochem. Int. 59, 591–599. doi: 10.1016/j.neuint.2011.04.007
Choi, J., Lee, H.-W., and Suk, K. (2011). Increased plasma levels of lipocalin 2 in mild cognitive impairment. J. Neurol. Sci. 305, 28–33. doi: 10.1016/j.jns.2011.03.023
Chu, H. X., Arumugam, T. V., Gelderblom, M., Magnus, T., Drummond, G. R., and Sobey, C. G. (2014). Role of CCR2 in inflammatory conditions of the central nervous system. J. Cereb. Blood Flow Metab. 34, 1425–1429. doi: 10.1038/jcbfm.2014.120
Clark, W. M., Rinker, L. G., Lessov, N. S., Hazel, K., and Eckenstein, F. (1999). Time course of IL-6 expression in experimental CNS ischemia. Neurol. Res. 21, 287–292. doi: 10.1080/01616412.1999.11740933
Conductier, G., Blondeau, N., Guyon, A., Nahon, J. L., and Rovère, C. (2010). The role of monocyte chemoattractant protein MCP1/CCL2 in neuroinflammatory diseases. J. Neuroimmunol. 224, 93–100. doi: 10.1016/j.jneuroim.2010.05.010
Cowland, J. B., Muta, T., and Borregaard, N. (2006). IL-1β-specific up-regulation of neutrophil gelatinase-associated lipocalin is controlled by IκB-ζ. J. Immunol. 176, 5559–5566. doi: 10.4049/jimmunol.176.9.5559
de Vries, H. E., Blom-Roosemalen, M. C., van Oosten, M., de Boer, A. G., van Berkel, T. J., Breimer, D. D., et al. (1996). The influence of cytokines on the integrity of the blood-brain barrier in vitro. J. Neuroimmunol. 64, 37–43. doi: 10.1016/0165-5728(95)00148-4
Dekens, D. W., Naudé, P. J. W., Keijser, J. N., Boerema, A. S., De Deyn, P. P., and Eisel, U. L. M. (2018). Lipocalin 2 contributes to brain iron dysregulation but does not affect cognition, plaque load and glial activation in the J20 Alzheimer mouse model. J. Neuroinflammation 15:330. doi: 10.1186/s12974-018-1372-5
Deng, Y., Chen, D., Gao, F., Lv, H., Zhang, G., Sun, X., et al. (2019). Exosomes derived from microRNA-138-5p-overexpressing bone marrow-derived mesenchymal stem cells confer neuroprotection to astrocytes following ischemic stroke via inhibition of LCN2. J. Biol. Eng. 13:71. doi: 10.1186/s13036-019-0193-0
Devireddy, L. R., Gazin, C., Zhu, X., and Green, M. R. (2005). A cell-surface receptor for lipocalin 24p3 selectively mediates apoptosis and iron uptake. Cell 123, 1293–1305. doi: 10.1016/j.cell.2005.10.027
Devireddy, L. R., Teodoro, J. G., Richard, F. A., and Green, M. R. (2001). Induction of apoptosis by a secreted lipocalin that is transcriptionally regulated by IL-3 deprivation. Science 293, 829–834. doi: 10.1126/science.1061075
Dimitrijevic, O. B., Stamatovic, S. M., Keep, R. F., and Andjelkovic, A. V. (2007). Absence of the chemokine receptor CCR2 protects against cerebral ischemia/reperfusion injury in mice. Stroke 38, 1345–1353. doi: 10.1161/01.STR.0000259709.16654.8f
Dong, M., Xi, G., Keep, R. F., and Hua, Y. (2013). Role of iron in brain lipocalin 2 upregulation after intracerebral hemorrhage in rats. Brain Res. 1505, 86–92. doi: 10.1016/j.brainres.2013.02.008
Du, Y., Li, W., Lin, L., Lo, E. H., and Xing, C. (2019). Effects of lipocalin-2 on brain endothelial adhesion and permeability. PLoS One 14:e0218965. doi: 10.1371/journal.pone.0218965
Egashira, Y., Hua, Y., Keep, R. F., Iwama, T., and Xi, G. (2016). Lipocalin 2 and blood-brain barrier disruption in white matter after experimental subarachnoid hemorrhage. Acta Neurochir. Suppl. 121, 131–134. doi: 10.1007/978-3-319-18497-5_23
Ferreira, A. C., Santos, T., Sampaio-Marques, B., Novais, A., Mesquita, S. D., Ludovico, P., et al. (2018a). Lipocalin-2 regulates adult neurogenesis and contextual discriminative behaviours. Mol. Psychiatry 23, 1031–1039. doi: 10.1038/mp.2017.95
Ferreira, A. C., Sousa, N., Bessa, J. M., Sousa, J. C., and Marques, F. (2018b). Metabolism and adult neurogenesis: towards an understanding of the role of lipocalin-2 and iron-related oxidative stress. Neurosci. Biobehav. Rev. 95, 73–84. doi: 10.1016/j.neubiorev.2018.09.014
Flo, T. H., Smith, K. D., Sato, S., Rodriguez, D. J., Holmes, M. A., Strong, R. K., et al. (2004). Lipocalin 2 mediates an innate immune response to bacterial infection by sequestrating iron. Nature 432, 917–921. doi: 10.1038/nature03104
Fumoto, T., Naraoka, M., Katagai, T., Li, Y., Shimamura, N., and Ohkuma, H. (2019). The role of oxidative stress in microvascular disturbances after experimental subarachnoid hemorrhage. Transl. Stroke Res. 10, 684–694. doi: 10.1007/s12975-018-0685-0
García-Berrocoso, T., Giralt, D., Llombart, V., Bustamante, A., Penalba, A., Flores, A., et al. (2014). Chemokines after human ischemic stroke: from neurovascular unit to blood using protein arrays. Transl. Proteom. 3, 1–9. doi: 10.1016/j.onehlt.2021.100325
Garcia-Bonilla, L., Moore, J. M., Racchumi, G., Zhou, P., Butler, J. M., Iadecola, C., et al. (2014). Inducible nitric oxide synthase in neutrophils and endothelium contributes to ischemic brain injury in mice. J. Immunol. 193, 2531–2537. doi: 10.4049/jimmunol.1400918
Gasterich, N., Wetz, S., Tillmann, S., Fein, L., Seifert, A., Slowik, A., et al. (2021). Inflammatory responses of astrocytes are independent from lipocalin 2. J. Mol. Neurosci. 71, 933–942. doi: 10.1007/s12031-020-01712-7
Gautam, J., Cao, Y., and Yao, Y. (2020). Pericytic laminin maintains blood-brain barrier integrity in an age-dependent manner. Transl. Stroke Res. 11, 228–242. doi: 10.1007/s12975-019-00709-8
Goetz, D. H., Holmes, M. A., Borregaard, N., Bluhm, M. E., Raymond, K. N., and Strong, R. K. (2002). The neutrophil lipocalin NGAL is a bacteriostatic agent that interferes with siderophore-mediated iron acquisition. Mol. Cell 10, 1033–1043. doi: 10.1016/s1097-2765(02)00708-6
Han, Q., Liu, S., Li, Z., Hu, F., Zhang, Q., Zhou, M., et al. (2014). DCPIB, a potent volume-regulated anion channel antagonist, attenuates microglia-mediated inflammatory response and neuronal injury following focal cerebral ischemia. Brain Res. 1542, 176–185. doi: 10.1016/j.brainres.2013.10.026
Harder, D. R., Zhang, C., and Gebremedhin, D. (2002). Astrocytes function in matching blood flow to metabolic activity. News Physiol. Sci. 17, 27–31. doi: 10.1152/physiologyonline.2002.17.1.27
Hetz, C., and Papa, F. R. (2018). The unfolded protein response and cell fate control. Mol. Cell 69, 169–181. doi: 10.1016/j.molcel.2017.06.017
Huang, X., Slavkovic, S., Song, E., Botta, A., Mehrazma, B., Lento, C., et al. (2020). A Unique conformational distortion mechanism drives lipocalin 2 binding to bacterial siderophores. ACS Chem. Biol. 15, 234–242. doi: 10.1021/acschembio.9b00820
Hughes, P. M., Allegrini, P. R., Rudin, M., Perry, V. H., Mir, A. K., and Wiessner, C. (2002). Monocyte chemoattractant protein-1 deficiency is protective in a murine stroke model. J. Cereb. Blood Flow Metab. 22, 308–317. doi: 10.1097/00004647-200203000-00008
Iadecola, C. (2017). The neurovascular unit coming of age: a journey through neurovascular coupling in health and disease. Neuron 96, 17–42. doi: 10.1016/j.neuron.2017.07.030
Iadecola, C., and Anrather, J. (2011). The immunology of stroke: from mechanisms to translation. Nat. Med. 17, 796–808. doi: 10.1038/nm.2399
Ip, J. P. K., Noçon, A. L., Hofer, M. J., Lim, S. L., Müller, M., and Campbell, I. L. (2011). Lipocalin 2 in the central nervous system host response to systemic lipopolysaccharide administration. J. Neuroinflammation 8:124. doi: 10.1186/1742-2094-8-124
Iurlaro, R., and Muñoz-Pinedo, C. (2016). Cell death induced by endoplasmic reticulum stress. FEBS J. 283, 2640–2652. doi: 10.1111/febs.13598
Jang, E., Kim, J.-H., Lee, S., Kim, J.-H., Seo, J.-W., Jin, M., et al. (2013a). Phenotypic polarization of activated astrocytes: the critical role of lipocalin-2 in the classical inflammatory activation of astrocytes. J. Immunol. 191, 5204–5219. doi: 10.4049/jimmunol.1301637
Jang, E., Lee, S., Kim, J.-H., Kim, J.-H., Seo, J.-W., Lee, W.-H., et al. (2013b). Secreted protein lipocalin-2 promotes microglial M1 polarization. FASEB J. 27, 1176–1190. doi: 10.1096/fj.12-222257
Janzer, R. C., and Raff, M. C. (1987). Astrocytes induce blood-brain barrier properties in endothelial cells. Nature 325, 253–257. doi: 10.1038/325253a0
Jayaraj, R. L., Azimullah, S., Beiram, R., Jalal, F. Y., and Rosenberg, G. A. (2019). Neuroinflammation: friend and foe for ischemic stroke. J. Neuroinflammation 16:142. doi: 10.1186/s12974-019-1516-2
Jha, M. K., Lee, S., Park, D. H., Kook, H., Park, K. G., Lee, I. K., et al. (2015). Diverse functional roles of lipocalin-2 in the central nervous system. Neurosci. Biobehav. Rev. 49, 135–156. doi: 10.1016/j.neubiorev.2014.12.006
Jin, M., Jang, E., and Suk, K. (2014a). Lipocalin-2 acts as a neuroinflammatogen in lipopolysaccharide-injected mice. Exp. Neurobiol. 23, 155–162. doi: 10.5607/en.2014.23.2.155
Jin, M., Kim, J.-H., Jang, E., Lee, Y. M., Soo Han, H., Woo, D. K., et al. (2014b). Lipocalin-2 deficiency attenuates neuroinflammation and brain injury after transient middle cerebral artery occlusion in mice. J. Cereb. Blood Flow Metab. 34, 1306–1314. doi: 10.1038/jcbfm.2014.83
Jin, Z., Kim, K. E., Shin, H. J., Jeong, E. A., Park, K. A., Lee, J. Y., et al. (2020). Hippocampal lipocalin 2 is associated with neuroinflammation and iron-related oxidative stress in ob/ob mice. J. Neuropathol. Exp. Neurol. 79, 530–541. doi: 10.1093/jnen/nlaa017
Kang, S. S., Ren, Y., Liu, C. C., Kurti, A., Baker, K. E., Bu, G., et al. (2018). Lipocalin-2 protects the brain during inflammatory conditions. Mol. Psychiatry 23, 344–350. doi: 10.1038/mp.2016.243
Keep, R. F., Andjelkovic, A. V., Xiang, J., Stamatovic, S. M., Antonetti, D. A., Hua, Y., et al. (2018). Brain endothelial cell junctions after cerebral hemorrhage: changes, mechanisms and therapeutic targets. J. Cereb. Blood Flow Metab. 38, 1255–1275. doi: 10.1177/0271678X18774666
Keep, R. F., Hua, Y., and Xi, G. (2012). Intracerebral haemorrhage: mechanisms of injury and therapeutic targets. Lancet Neurol. 11, 720–731. doi: 10.1016/S1474-4422(12)70104-7
Kim, B.-W., Jeong, K. H., Kim, J.-H., Jin, M., Kim, J.-H., Lee, M.-G., et al. (2016). Pathogenic upregulation of glial lipocalin-2 in the parkinsonian dopaminergic system. J. Neurosci. 36, 5608–5622. doi: 10.1523/JNEUROSCI.4261-15.2016
Kim, J.-H., Ko, P.-W., Lee, H.-W., Jeong, J.-Y., Lee, M.-G., Kim, J.-H., et al. (2017). Astrocyte-derived lipocalin-2 mediates hippocampal damage and cognitive deficits in experimental models of vascular dementia. Glia 65, 1471–1490. doi: 10.1002/glia.23174
Kirkley, K. S., Popichak, K. A., Afzali, M. F., Legare, M. E., and Tjalkens, R. B. (2017). Microglia amplify inflammatory activation of astrocytes in manganese neurotoxicity. J. Neuroinflammation 14:99. doi: 10.1186/s12974-017-0871-0
Lambertsen, K. L., Finsen, B., and Clausen, B. H. (2019). Post-stroke inflammation-target or tool for therapy. Acta Neuropathol. 137, 693–714. doi: 10.1007/s00401-018-1930-z
Lattke, M., Reichel, S. N., Magnutzki, A., Abaei, A., Rasche, V., Walther, P., et al. (2017). Transient IKK2 activation in astrocytes initiates selective non-cell-autonomous neurodegeneration. Mol. Neurodegener. 12:16. doi: 10.1186/s13024-017-0157-0
Lee, S., Kim, J.-H., Kim, J.-H., Seo, J.-W., Han, H.-S., Lee, W.-H., et al. (2011). Lipocalin-2 is a chemokine inducer in the central nervous system: role of chemokine ligand 10 (CXCL10) in lipocalin-2-induced cell migration. J. Biol. Chem. 286, 43855–43870. doi: 10.1074/jbc.M111.299248
Lee, S., Lee, J., Kim, S., Park, J.-Y., Lee, W.-H., Mori, K., et al. (2007). A dual role of lipocalin 2 in the apoptosis and deramification of activated microglia. J. Immunol. 179, 3231–3241. doi: 10.4049/jimmunol.179.5.3231
Lee, S., Lee, W.-H., Lee, M.-S., Mori, K., and Suk, K. (2012). Regulation by lipocalin-2 of neuronal cell death, migration and morphology. J. Neurosci. Res. 90, 540–550. doi: 10.1002/jnr.22779
Lee, S., Park, J.-Y., Lee, W.-H., Kim, H., Park, H.-C., Mori, K., et al. (2009). Lipocalin-2 is an autocrine mediator of reactive astrocytosis. J. Neurosci. 29, 234–249. doi: 10.1523/JNEUROSCI.5273-08.2009
Liu, J., Guo, Z.-N., Yan, X.-L., Huang, S., Ren, J.-X., Luo, Y., et al. (2020). Crosstalk between autophagy and ferroptosis and its putative role in ischemic stroke. Front. Cell. Neurosci. 14:577403. doi: 10.3389/fncel.2020.577403
Liu, L. R., Liu, J. C., Bao, J. S., Bai, Q. Q., and Wang, G. Q. (2020). Interaction of microglia and astrocytes in the neurovascular unit. Front. Immunol. 11:1024. doi: 10.3389/fimmu.2020.01024
Liu, R., Zhang, H., Cheng, S., Sun, Y., Li, H., Xiao, J., et al. (2020). Association of brain iron overload with brain edema and brain atrophy after intracerebral hemorrhage. Front. Neurol. 11:602413. doi: 10.3389/fneur.2020.602413
Lo, E. H., and Rosenberg, G. A. (2009). The neurovascular unit in health and disease: introduction. Stroke 40, S2–S3. doi: 10.1161/STROKEAHA.108.534404
Mehdiratta, M., Kumar, S., Hackney, D., Schlaug, G., and Selim, M. (2008). Association between serum ferritin level and perihematoma edema volume in patients with spontaneous intracerebral hemorrhage. Stroke 39, 1165–1170. doi: 10.1161/STROKEAHA.107.501213
Mesquita, S. D., Ferreira, A. C., Falcao, A. M., Sousa, J. C., Oliveira, T. G., Correia-Neves, M., et al. (2014). Lipocalin 2 modulates the cellular response to amyloid beta. Cell Death Differ. 21, 1588–1599. doi: 10.1038/cdd.2014.68
Mike, E. V., Makinde, H. M., Gulinello, M., Vanarsa, K., Herlitz, L., Gadhvi, G., et al. (2019). Lipocalin-2 is a pathogenic determinant and biomarker of neuropsychiatric lupus. J. Autoimmun. 96, 59–73. doi: 10.1016/j.jaut.2018.08.005
Mondal, A., Bose, D., Saha, P., Sarkar, S., Seth, R., Kimono, D., et al. (2020). Lipocalin 2 induces neuroinflammation and blood-brain barrier dysfunction through liver-brain axis in murine model of nonalcoholic steatohepatitis. J. Neuroinflammation 17:201. doi: 10.1186/s12974-020-01876-4
Nairz, M., Schroll, A., Haschka, D., Dichtl, S., Sonnweber, T., Theurl, I., et al. (2015). Lipocalin-2 ensures host defense against Salmonella Typhimurium by controlling macrophage iron homeostasis and immune response. Eur. J. Immunol. 45, 3073–3086. doi: 10.1002/eji.201545569
Nairz, M., Theurl, I., Schroll, A., Theurl, M., Fritsche, G., Lindner, E., et al. (2009). Absence of functional Hfe protects mice from invasive Salmonella enterica serovar Typhimurium infection via induction of lipocalin-2. Blood 114, 3642–3651. doi: 10.1182/blood-2009-05-223354
Nam, Y., Kim, J. H., Seo, M., Kim, J. H., Jin, M., Jeon, S., et al. (2014). Lipocalin-2 protein deficiency ameliorates experimental autoimmune encephalomyelitis: the pathogenic role of lipocalin-2 in the central nervous system and peripheral lymphoid tissues. J. Biol. Chem. 289, 16773–16789. doi: 10.1074/jbc.M113.542282
Naudé, P. J. W., Nyakas, C., Eiden, L. E., Ait-Ali, D., van der Heide, R., Engelborghs, S., et al. (2012). Lipocalin 2: novel component of proinflammatory signaling in Alzheimer’s disease. FASEB J. 26, 2811–2823. doi: 10.1096/fj.11-202457
Ni, W., Zheng, M., Xi, G., Keep, R. F., and Hua, Y. (2015). Role of lipocalin-2 in brain injury after intracerebral hemorrhage. J. Cereb. Blood Flow Metab. 35, 1454–1461. doi: 10.1038/jcbfm.2015.52
Pang, J., Chen, Y., Kuai, L., Yang, P., Peng, J., Wu, Y., et al. (2017). Inhibition of blood-brain barrier disruption by an apolipoprotein E-mimetic peptide ameliorates early brain injury in experimental subarachnoid hemorrhage. Transl. Stroke Res. 8, 257–272. doi: 10.1007/s12975-016-0507-1
Pérez de la Ossa, N., Sobrino, T., Silva, Y., Blanco, M., Millán, M., Gomis, M., et al. (2010). Iron-related brain damage in patients with intracerebral hemorrhage. Stroke 41, 810–813. doi: 10.1161/STROKEAHA.109.570168
Rathore, K. I., Berard, J. L., Redensek, A., Chierzi, S., Lopez-Vales, R., Santos, M., et al. (2011). Lipocalin 2 plays an immunomodulatory role and has detrimental effects after spinal cord injury. J. Neurosci. 31, 13412–13419. doi: 10.1523/JNEUROSCI.0116-11.2011
Reiche, E. M. V., Gelinksi, J. R., Alfieri, D. F., Flauzino, T., Lehmann, M. F., de Araújo, M. C. M., et al. (2019). Immune-inflammatory, oxidative stress and biochemical biomarkers predict short-term acute ischemic stroke death. Metab. Brain Dis. 34, 789–804. doi: 10.1007/s11011-019-00403-6
Reichel, C. A., Khandoga, A., Anders, H. J., Schlöndorff, D., Luckow, B., and Krombach, F. (2006). Chemokine receptors Ccr1, Ccr2 and Ccr5 mediate neutrophil migration to postischemic tissue. J. Leukoc. Biol. 79, 114–122. doi: 10.1189/jlb.0605337
Righy, C., Bozza, M. T., Oliveira, M. F., and Bozza, F. A. (2016). Molecular, cellular and clinical aspects of intracerebral hemorrhage: are the enemies within? Curr. Neuropharmacol. 14, 392–402. doi: 10.2174/1570159x14666151230110058
Rodrigo, R., Fernández-Gajardo, R., Gutiérrez, R., Matamala, J. M., Carrasco, R., Miranda-Merchak, A., et al. (2013). Oxidative stress and pathophysiology of ischemic stroke: novel therapeutic opportunities. CNS Neurol. Disord. Drug Targets 12, 698–714. doi: 10.2174/1871527311312050015
Shao, X., Bao, W., Hong, X., Jiang, H., and Yu, Z. (2018). Identification and functional analysis of differentially expressed genes associated with cerebral ischemia/reperfusion injury through bioinformatics methods. Mol. Med. Rep. 18, 1513–1523. doi: 10.3892/mmr.2018.9135
Shin, H. J., Jeong, E. A., Lee, J. Y., An, H. S., Jang, H. M., Ahn, Y. J., et al. (2021). Lipocalin-2 deficiency reduces oxidative stress and neuroinflammation and results in attenuation of kainic acid-induced hippocampal cell death. Antioxidants (Basel) 10:100. doi: 10.3390/antiox10010100
Song, B., Zhang, H., Jiang, L., Chi, Y., Tian, J., Du, W., et al. (2015). Down-regulation of lipocalin 2 suppresses the growth of human lung adenocarcinoma through oxidative stress involving Nrf2/HO-1 signaling. Acta Biochim. Biophys. Sin. (Shanghai) 47, 805–814. doi: 10.1093/abbs/gmv085
Srinivasan, G., Aitken, J. D., Zhang, B., Carvalho, F. A., Chassaing, B., Shashidharamurthy, R., et al. (2012). Lipocalin 2 deficiency dysregulates iron homeostasis and exacerbates endotoxin-induced sepsis. J. Immunol. 189, 1911–1919. doi: 10.4049/jimmunol.1200892
Suk, K. (2016). Lipocalin-2 as a therapeutic target for brain injury: an astrocentric perspective. Prog. Neurobiol. 144, 158–172. doi: 10.1016/j.pneurobio.2016.08.001
Toyota, Y., Wei, J., Xi, G., Keep, R. F., and Hua, Y. (2019). White matter T2 hyperintensities and blood-brain barrier disruption in the hyperacute stage of subarachnoid hemorrhage in male mice: the role of lipocalin-2. CNS Neurosci. Ther. 25, 1207–1214. doi: 10.1111/cns.13221
Tuo, Q. Z., Lei, P., Jackman, K. A., Li, X. L., Xiong, H., Li, X. L., et al. (2017). Tau-mediated iron export prevents ferroptotic damage after ischemic stroke. Mol. Psychiatry 22, 1520–1530. doi: 10.1038/mp.2017.171
Turner, R. J., and Sharp, F. R. (2016). Implications of MMP9 for blood brain barrier disruption and hemorrhagic transformation following ischemic stroke. Front. Cell. Neurosci. 10:56. doi: 10.3389/fncel.2016.00056
Tuttolomondo, A., Pecoraro, R., and Pinto, A. (2014). Studies of selective TNF inhibitors in the treatment of brain injury from stroke and trauma: a review of the evidence to date. Drug Design Dev. Ther. 8, 2221–2238. doi: 10.2147/DDDT.S67655
Vichaya, E. G., Gross, P. S., Estrada, D. J., Cole, S. W., Grossberg, A. J., Evans, S. E., et al. (2019). Lipocalin-2 is dispensable in inflammation-induced sickness and depression-like behavior. Psychopharmacology (Berl) 236, 2975–2982. doi: 10.1007/s00213-019-05190-7
Wagner, K. R., Sharp, F. R., Ardizzone, T. D., Lu, A., and Clark, J. F. (2003). Heme and iron metabolism: role in cerebral hemorrhage. J. Cereb. Blood Flow Metab. 23, 629–652. doi: 10.1097/01.WCB.0000073905.87928.6D
Wang, J., and Doré, S. (2007). Inflammation after intracerebral hemorrhage. J. Cereb. Blood Flow Metab. 27, 894–908. doi: 10.1038/sj.jcbfm.9600403
Wang, Z., Higashikawa, K., Yasui, H., Kuge, Y., Ohno, Y., Kihara, A., et al. (2020). FTY720 protects against ischemia-reperfusion injury by preventing the redistribution of tight junction proteins and decreases inflammation in the subacute phase in an experimental stroke model. Transl. Stroke Res. 11, 1103–1116. doi: 10.1007/s12975-020-00789-x
Wang, G., Weng, Y.-C., Chiang, I. C., Huang, Y.-T., Liao, Y.-C., Chen, Y.-C., et al. (2020). Neutralization of lipocalin-2 diminishes stroke-reperfusion injury. Int. J. Mol. Sci. 21:6253. doi: 10.3390/ijms21176253
Wang, G., Weng, Y.-C., Han, X., Whaley, J. D., McCrae, K. R., and Chou, W.-H. (2015). Lipocalin-2 released in response to cerebral ischaemia mediates reperfusion injury in mice. J. Cell. Mol. Med. 19, 1637–1645. doi: 10.1111/jcmm.12538
Wang, Z., Zhou, F., Dou, Y., Tian, X., Liu, C., Li, H., et al. (2018). Melatonin alleviates intracerebral hemorrhage-induced secondary brain injury in rats via suppressing apoptosis, inflammation, oxidative stress, DNA damage and mitochondria injury. Transl. Stroke Res. 9, 74–91. doi: 10.1007/s12975-017-0559-x
Wen, X., Su, B., Gao, M., Chen, J., Zhou, D., You, H., et al. (2021). Obesity-associated up-regulation of lipocalin 2 protects gastric mucosa cells from apoptotic cell death by reducing endoplasmic reticulum stress. Cell Death Dis. 12:221. doi: 10.1038/s41419-021-03512-2
Weng, Y.-C., and Chou, W.-H. (2015). Neutrophil gelatinase-associated lipocalin and matrix metalloproteinase-9 as potential biomarkers for stroke: a pilot study. J. Neurol. Neurophysiol. 6:278. doi: 10.4172/2155-9562.1000278
Weng, Y.-C., Huang, Y.-T., Chiang, I. C., Tsai, P.-J., Su, Y.-W., and Chou, W.-H. (2021). Lipocalin-2 mediates the rejection of neural transplants. FASEB J. 35:e21317. doi: 10.1096/fj.202001018R
Wu, G., Xi, G., and Huang, F. (2006). Spontaneous intracerebral hemorrhage in humans: hematoma enlargement, clot lysis and brain edema. Acta Neurochir. Suppl. 96, 78–80. doi: 10.1007/3-211-30714-1_19
Xi, G., Keep, R. F., and Hoff, J. T. (2006). Mechanisms of brain injury after intracerebral haemorrhage. Lancet Neurol. 5, 53–63. doi: 10.1016/S1474-4422(05)70283-0
Xiao, X., Yeoh, B. S., Saha, P., Olvera, R. A., Singh, V., and Vijay-Kumar, M. (2016). Lipocalin 2 alleviates iron toxicity by facilitating hypoferremia of inflammation and limiting catalytic iron generation. Biometals 29, 451–465. doi: 10.1007/s10534-016-9925-5
Xie, Q., Gu, Y., Hua, Y., Liu, W., Keep, R. F., and Xi, G. (2014). Deferoxamine attenuates white matter injury in a piglet intracerebral hemorrhage model. Stroke 45, 290–292. doi: 10.1161/STROKEAHA.113.003033
Xing, C., Wang, X., Cheng, C., Montaner, J., Mandeville, E., Leung, W., et al. (2014). Neuronal production of lipocalin-2 as a help-me signal for glial activation. Stroke 45, 2085–2092. doi: 10.1161/STROKEAHA.114.005733
Xu, G., Ahn, J., Chang, S., Eguchi, M., Ogier, A., Han, S., et al. (2012). Lipocalin-2 induces cardiomyocyte apoptosis by increasing intracellular iron accumulation. J. Biol. Chem. 287, 4808–4817. doi: 10.1074/jbc.M111.275719
Yamada, Y., Miyamoto, T., Kashima, H., Kobara, H., Asaka, R., Ando, H., et al. (2016). Lipocalin 2 attenuates iron-related oxidative stress and prolongs the survival of ovarian clear cell carcinoma cells by up-regulating the CD44 variant. Free Radic. Res. 50, 414–425. doi: 10.3109/10715762.2015.1134795
Yang, J., Goetz, D., Li, J. Y., Wang, W., Mori, K., Setlik, D., et al. (2002). An iron delivery pathway mediated by a lipocalin. Mol. Cell 10, 1045–1056. doi: 10.1016/s1097-2765(02)00710-4
Yang, R., Chen, J., Xu, B., Yang, B., Fu, J., Xiao, S., et al. (2020). circ_2858 helps blood-brain barrier disruption by increasing VEGFA via sponging miR-93-5p during Escherichia coli Meningitis. Mol. Ther. Nucleic Acids 22, 708–721. doi: 10.1016/j.omtn.2020.09.034
Yu, C. Y., Ng, G., and Liao, P. (2013). Therapeutic antibodies in stroke. Transl. Stroke Res. 4, 477–483. doi: 10.1007/s12975-013-0281-2
Zamanian, J. L., Xu, L., Foo, L. C., Nouri, N., Zhou, L., Giffard, R. G., et al. (2012). Genomic analysis of reactive astrogliosis. J. Neurosci. 32, 6391–6410. doi: 10.1523/JNEUROSCI.6221-11.2012
Zhang, J., Novakovic, N., Hua, Y., Keep, R. F., and Xi, G. (2021). Role of lipocalin-2 in extracellular peroxiredoxin 2-induced brain swelling, inflammation and neuronal death. Exp. Neurol. 335:113521. doi: 10.1016/j.expneurol.2020.113521
Zhao, N., Xu, X., Jiang, Y., Gao, J., Wang, F., Xu, X., et al. (2019). Lipocalin-2 may produce damaging effect after cerebral ischemia by inducing astrocytes classical activation. J. Neuroinflammation 16:168. doi: 10.1186/s12974-019-1556-7
Zlokovic, B. V. (2011). Neurovascular pathways to neurodegeneration in Alzheimer’s disease and other disorders. Nat. Rev. Neurosci. 12, 723–738. doi: 10.1038/nrn3114
Keywords: cerebral stroke, lipocalin-2 (LCN-2), blood brain barrier, central nervous system, secondary injury, iron dysregulation, neurovascular unit (NVU)
Citation: Luo C, Zhou S, Yin S, Jian L, Luo P, Dong J and Liu E (2022) Lipocalin-2 and Cerebral Stroke. Front. Mol. Neurosci. 15:850849. doi: 10.3389/fnmol.2022.850849
Received: 08 January 2022; Accepted: 23 February 2022;
Published: 12 April 2022.
Edited by:
Zongduo Guo, First Affiliated Hospital of Chongqing Medical University, ChinaReviewed by:
Yujie Chen, Army Medical University, ChinaZhen-Ni Guo, First Affiliated Hospital of Jilin University, China
Copyright © 2022 Luo, Zhou, Yin, Jian, Luo, Dong and Liu. This is an open-access article distributed under the terms of the Creative Commons Attribution License (CC BY). The use, distribution or reproduction in other forums is permitted, provided the original author(s) and the copyright owner(s) are credited and that the original publication in this journal is cited, in accordance with accepted academic practice. No use, distribution or reproduction is permitted which does not comply with these terms.
*Correspondence: Shuai Zhou, zshuai_kmust@163.com