- 1State key Laboratory for Conservation and Utilization of Bio-Resources in Yunnan, School of Life Sciences, Yunnan University, Kunming, Yunnan, China
- 2The Maternal and Child Health Hospital of Qianxinan, Xingyi, Guizhou, China
Four new polyketides (1–4) and seven known compounds (5–11) including three polyketides and four sterols were isolated from the fermented extracts of Paecilomyces gunnii YMF1.00003. The new chemical structures were determined through the analysis of the nuclear magnetic resonance and high-resolution electrospray ionization mass spectrometry, and their configurations were subsequently confirmed by nuclear overhauser effect spectroscopy, the calculated electronic circular dichroism (ECD) spectra, and quantum chemical calculations of the NMR data (qcc NMR). Based on the results of pre-activity screening and compound structure target prediction, certain metabolites were assayed to evaluate their cytotoxic and protein kinase Cα inhibitory activities. Results indicated that 3β-hydroxy-7α-methoxy-5α,6α-epoxy-8(14),22E-dien-ergosta (8) exhibited potent cytotoxic activity, with half-maximal inhibitory concentration values of 3.00 ± 0.27 to 15.69 ± 0.61 μM against five tumor cells, respectively. The new compound gunniiol A (1) showed weak cytotoxic activity at a concentration of 40 μM. At a concentration of 20 μg/mL, compounds 1, 6, and 7 exhibited protein kinase Cα inhibition by 43.63, 40.93, and 57.66%, respectively. This study is the first to report steroids demonstrating good cytotoxicity and polyketides exhibiting inhibitory activity against protein kinase Cα from the extracts of P. gunnii.
1 Introduction
Cordyceps, a fungus known for parasitizing insects, fungi, and plants (Qu et al., 2022), gained historical recognition during the Qing Dynasty, with Cordyceps sinensis emerging as the most well-known species documented in Bencao Beiyao. An in-depth study of its pharmacological properties revealed immunomodulatory functions and a significant impact on antitumor effects, organ transplantation, and heart disease (Kuo et al., 1996). Cordyceps gunnii, a well-known fungus in the Cordyceps genus, exhibits diverse bioactivities.
Paecilomyces gunnii, an anamorph of C. gunnii, produces metabolites that exhibit various pharmacological activities. Three new metabolites, gunnilactams A-C, were isolated from the deep fermentation broth of P. gunnii, and gunnilactam A showed cytotoxic activity against C42B cells (human prostate cancer) with a half-maximal inhibitory concentration (IC50) of 5.4 μM (Zheng et al., 2017). Following the performance of activity-guided procedures and liquid chromatography–mass spectrometry (LC-MS), paecilomycones A–C were identified from P. gunnii, exhibiting strong tyrosinase inhibitory activity with IC50 values of 0.11, 0.17, and 0.14 μM, respectively (Lu et al., 2014). In addition, a new selenopolysaccharide (SeCPS-II), consisting of α-L-rhamnose, α-D-mannose, α-D-glucose, and β-D-galactose with a molecular weight of 4.12 × 103 kDa, was obtained from C. gunnii and showed weak inhibition of SeCPS-II on SKOV-3 cells (Sun et al., 2018). The identified active compounds exhibited potential for various applications, and further in-depth research on them holds significant value in the fields of pharmacology and medicine. In a previous study, we examined the metabolome and the activity of extracts derived from the P. gunnii YMF1.00003 strain. Results showed significant variations in the activities of fermentation product extracts under various culture conditions. The extract obtained from the wheat bran medium (WGA) exhibited notable inhibitory activity against the tested cell lines, resembling the effects observed in the extract from the stroma and host complex of C. gunnii (Qu et al., 2022). In this study, we aimed to examine the chemical structures of four new polyketides and seven known compounds isolated from P. gunnii YMF1.00003 cultivated in two types of media (Figure 1). Based on the results of a previous study (Qu et al., 2022), extensive literature research, and the prediction of compound structure targets, we conducted assays on select compounds to assess their cytotoxic activity and protein kinase Cα inhibitory activity.
2 Materials and methods
2.1 General experimental procedures
Electrospray ionization mass spectra (ESI-MS) and high-resolution electrospray ionization mass spectra (HR-ESI-MS) were acquired using a high-resolution Q Exactive Focus Mass Spectrometer (Thermo Fisher Scientific, Bremen, Germany). Optical rotation measurements were conducted using a Jasco DIP-370 digital polarimeter (JASCO, Tokyo, Japan). Ultraviolet (UV) spectra were recorded using a Shimadzu UV-2401PC spectrophotometer. Nuclear magnetic resonance (NMR) spectra were recorded using an Avance III-600 spectrometer (Bruker BioSpin, Rheinstetten, Germany) using tetramethylsilane as an internal standard. Column chromatography was performed using silica gel (200–300 mesh), GF254 (Qingdao Marine Chemical Inc., Qingdao, China), and Sephadex LH-20 (Amersham Pharmacia, United Kingdom). Semipreparative high-performance liquid chromatography (HPLC) was performed using an LC3000 system (Beijing Chuangxintongheng Science and Technology Co., Ltd., Beijing, China). Precoated silica gel GF254 plates (Qingdao Marine Chemical Factory, Qingdao, China) were used for thin-layer chromatography (TLC) analysis.
2.2 Microbial strain, media, and cultivation
The P. gunnii YMF1.00003 strain was officially deposited at the State Key Laboratory for the Conservation and Utilization of Bio-Resources, Yunnan University, Kunming, China. Paecilomyces gunnii YMF1.00003 was fermented in two different media. During the pre-experiment, the solid and liquid forms of four different media (PDB, modified Sabouraud medium, Rice, and Oats) were used to determine the most suitable medium for the production of YMF1.00003 metabolites. Based on the amount of extracts and results of TLC analysis, the modified Sabouraud medium demonstrated a substantial amount of YMF1.00003 extracts, with a more diverse range of compounds. Consequently, the modified Sabouraud agar medium was selected for subsequent fermentation. The strain was cultured in 9-cm dishes with modified Sand’s solid medium (comprising 5.0 g of tryptone, 1.0 g of yeast extract, 60.0 g of glucose, 0.25 g of K2HPO4, 0.25 g of MgSO4, 0.25 g of KCl, 15.0 g of agar, and 1 L of water) at 28°C for 21 days and 30 L. The cultures were extracted using organic reagents (ethyl acetate/methanol/glacial acetic acid = 80:15:5, v/v/v; Pu et al., 2021; Liu et al., 2022) to obtain 23.9 g of crude extract and subsequently designated as the MSA fraction. In addition, the strain was cultured on a modified wheat bran medium (comprising 30.0 g of wheat bran, 20.0 g of glucose, 1.5 g of KH2PO4, 1.5 g of MgSO4, 0.5 g of MnSO4∙H2O, 0.5 g of ZnSO4∙7H2O, 0.5 g of CuSO4∙5H2O, 8.0 g of (NH4)2SO4, 15 g of agar, and 1 L of water) at 28°C for 50 days. Subsequently, 30 g of crude extract was obtained and designated as the WB fraction.
2.3 Isolation and purification
After mixing with silica 60 RP-C18, the MSA fraction (23.9 g) was placed on an RP-C18 column (50 g) and eluted with H2O/MeOH (100:0 → 0:100, v/v) to obtain 12 fractions (Fr.1–Fr.12). Fr.9 (1.617 g) was separated with a Sephadex LH-20 column (chloroform–methanol, 1:1) to obtain nine fractions (Fr.9.1-9). Fr.9.3 (1.254 g) was separated using a silica gel column (200–300 mesh) and eluted with petroleum ether/acetone (100:1 → 6:4, v/v) to obtain five fractions (Fr.9.3.1-5). Fr.9.3.2 (135 mg) was loaded on a silica gel column eluting with chloroform/acetone (100:1 → 6:4, v/v) and then purified by Sephadex LH-20 (acetone) to obtain compound 8 (3 mg). Fr.9.3.4 (180 mg) was subjected to Sephadex LH-20 chromatography (chloroform–methanol, 1:1) to obtain four fractions (Fr.9.3.4.1-4). Fr.9.3.4.3 (132 mg) underwent separation on a silica gel column eluted with chloroform/acetone (100:1 → 8:2, v/v) and was subsequently purified by a Sephadex LH-20 (acetone) to obtain compound 9 (2 mg). Fr.9.3.5 (113 mg) was loaded on a Sephadex LH-20 (acetone) and purified on a silica gel column via elution with chloroform/methanol (100:1 → 8:2, v/v) to obtain compound 10 (8 mg). Fr.5 (259 mg) was subjected to Sephadex LH-20 (chloroform–methanol, 1:1) chromatography to obtain five fractions (Fr.5.1-5). Fr.5.4 (54 mg) was further separated using a silica gel column via elution with chloroform/acetone (100:1 → 6:4, v/v) and purified by Sephadex LH-20 chromatography (acetone) to obtain compound 2 (7 mg). Fr.5.5 (80 mg) was subjected to silica gel column chromatography and eluted with chloroform/acetone (100:0 → 8:2, v/v) and purified by Sephadex LH-20 chromatography (methanol) to obtain compound 7 (5 mg). Fr.4 (1.6 g) was loaded on a Sephadex LH-20 column (chloroform–methanol, 1:1) to obtain seven fractions (Fr.4.1-7). Fr.4.5 (637 mg) was further separated using Sephadex LH-20 (methanol) to obtain four fractions (Fr.4.5.1-4). Fr.4.5.2 (439 mg) was subjected to semi-preparative HPLC with gradient elution of MeOH–H2O (30:70 → 35:65 and 40:60 → 100:0) for 50 min to obtain five fractions (Fr.4.5.2.1-5). Fr.4.5.2.3 (120 mg) was separated using a Sephadex LH-20 column (methanol) to obtain three fractions (Fr.4.5.2.3.1-3). Fr.4.5.2.3.3 (108 mg) was further separated using a silica gel column via elution with chloroform/acetone (100:1 → 0:100, v/v) to obtain eight fractions (Fr.4.5.2.3.3.1-8). Fr.4.5.2.3.3.6 (51 mg) was separated using a Sephadex LH-20 column (methanol) to obtain compound 6 (28 mg). Fr.4.5.2.5 (64 mg) was separated using a Sephadex LH-20 column (methanol) to obtain two fractions (Fr.4.5.2.5.1-2). Fr.4.5.2.5.2 (59 mg) was further separated using a column of silica gel via elution with chloroform/acetone (100:1 → 0:100, v/v) and purified by Sephadex LH-20 (methanol) to obtain compound 3a/b (5 mg). Fr.3 (2.135 g) was separated using a Sephadex LH-20 column (chloroform–methanol, 1:1) to obtain five fractions (Fr.3.1-5). Fr.3-5 (1.232 g) was separated using a Sephadex LH-20 column (chloroform–methanol, 1:1) to obtain four fractions (Fr.3.5.1-4). Fr.3.5.4 (1.081 g) was subjected to semi-preparative RP-C18 HPLC with a gradient elution of MeOH:H2O (10:90 → 45:55 and 60:40 → 100:0) for 40 min to obtain three fractions (Fr.3.5.4.1-3). Fr.3.5.4.3 (34 mg) was further separated using a silica gel column via elution with chloroform/acetone (50:1 → 0:100, v/v) to obtain four fractions (Fr.3.5.4.3.1-4). Fr.3.5.4.3.2 (8 mg) was separated using a Sephadex LH-20 column (methanol) to obtain compound 4 (5 mg).
The WB fraction (30.0 g) was placed on an RP-C18 column (60 g) and eluted with H2O/MeOH mixtures (100:0 → 0:100, v/v) to obtain 15 fractions (Fr.1-15). Fr.15 (878 mg) was separated using a silica gel column via elution with petroleum ether/ethyl acetate (300:1 → 6:4, v/v) to obtain five fractions (Fr.15.1-5). Fr.15.2 (153 mg) was separated using a silica gel column via elution with petroleum ether/ethyl acetate (100:1 → 8:2, v/v) and then purified with a silica gel column via elution with petroleum ether/ethyl acetate (200:1 → 8:2, v/v) to obtain compound 11 (1 mg). Fr.15.4 (123 mg) was separated using a silica gel column via elution with chloroform/acetone (10:1 → 6:4, v/v) and then purified by Sephadex LH-20 (acetone) to obtain compound 8 (2 mg). Fr.6 (385 mg) was loaded on a Sephadex LH-20 column (methanol) to obtain six fractions (Fr.6.1-6). Fr.6.3 (108 mg) was subjected to a silica gel column (100:1 → 7:3, v/v) and purified by Sephadex LH-20 (methanol) to obtain compound 1 (6 mg). Fr.4 (1.15 g) was subjected to Sephadex LH-20 column (chloroform–methanol, 1:1) to obtain five fractions (Fr.4.1-5). Fr.4.1 (715 mg) was loaded on a silica gel column via elution with chloroform/acetone (100:1 → 8:2, v/v) to obtain four fractions (Fr.4.1-4). Fr.4.1.3 (83 mg) was subjected to a Sephadex LH-20 column (methanol) and purified by a silica gel column via elution with chloroform/acetone (100:1 → 8:2, v/v) to obtain compound 5 (15 mg).
2.4 Spectroscopic data
Gunniiol A (1), colorless solid; [α]23D = 75.5 (c = 0.10, MeOH); UV (MeOH) λmax (log ε) nm: 204 (4.46); 1H-NMR (CDCl3, 600 MHz) and 13C-NMR (CDCl3, 150 MHz), see Table 1; ESI-MS m/z: 291 [M + Na]+; HR-ESI-MS m/z: 291.1190 ([M + Na]+, calcd. 291.1203).
Gunniiol B (2), colorless oil; [α]23D = 22.5 (c = 0.10, MeOH); UV (MeOH) λmax (log ε) nm: 197 (4.57); 1H-NMR (CDCl3, 600 MHz) and 13C-NMR (CDCl3, 150 MHz), see Table 1; ESI-MS m/z: 265 [M + Na]+; HR-ESI-MS m/z: 265.1040 ([M + Na]+, calcd. 265.1046).
Gunniiol C (3a/b), colorless oil; 1H-NMR (CD3OD, 600 MHz) and 13C-NMR (CD3OD, 150 MHz), see Table 2; ESI-MS m/z: 287 [M + H]+, 309 [M + Na]+; HR-ESI-MS m/z: 309.1300 ([M + Na]+, calcd. 309.1309).
(E)-4,7-dihydroxyoct-2-enoic acid (4), colorless solid; [α]23D = 19.4 (c = 0.10, MeOH); UV (MeOH) λmax (log ε) nm: 198 (4.33); 1H-NMR (CD3OD, 600 MHz) and 13C-NMR (CD3OD, 150 MHz), see Table 3; ESI-MS m/z: 173 [M − H]−; HR-ESI-MS m/z: 173.0808 ([M − H]−, calcd. 173.0808).
Clonostachydiol (5), colorless solid; ESI-MS m/z: 307 [M + Na]+; 1H-NMR (600 MHz, CD3OD) δ: 6.83 (1H, dd, J = 15.6, 4.0 Hz), 6.76 (1H, d, J = 15.6, 3.2 Hz), 6.14 (1H, t, J = 15.6, 1.6 Hz), 5.85 (1H, dd, J = 15.6, 1.6 Hz), 5.25 (1H, dq, J = 6.4, 2.0 Hz), 5.14 (1H, m), 4.57 (1H, m), 4.41 (1H, m), 1.95 (1H, m), 1.75 (1H, m), 1.64 (1H, m), 1.51 (1H, m), 1.41 (3H, d, J = 6.3 Hz), and 1.20 (3H, d, J = 6.6 Hz); 13C-NMR (150 MHz, CD3OD) δ: 167.3 (s), 167.0 (s), 153.5 (d), 148.4 (d), 125.1 (d), 121.7 (d), 77.2 (d), 73.0 (d), 71.2 (d), 70.6 (d), 29.7 (t), 27.1 (t), 17.9 (q), and 17.8 (q).
7R-[[4R,5S-dihydroxy-1-oxo-2E-hexen-1-yl]oxy]-4S-hydroxy-2E-octenoic acid (6), colorless oil; ESI-MS m/z: 325 [M + Na]+; 1H-NMR (600 MHz, CD3OD) δ: 5.99 (1H, d, J = 15.9 Hz, H-2), 6.91 (1H, dd, J = 4.8, 15.9 Hz, H-3), 4.25 (1H, m, H-4), 1.56 (1H, m, H-5a), 1.67 (2H, m, H-5b/6a), 1.75 (1H, m, H-6b), 5.00 (1H, m, H-7), 1.26 (3H, d, J = 6.4 Hz, H-8), 6.08 (1H, d, J = 15.9 Hz, H-2′), 7.07 (1H, dd, J = 4.8, 15.9 Hz, H-3′), 4.07 (1H, t, J = 4.8 Hz, H-4′), 3.72 (1H, t, J = 6.4 Hz, H-5′), and 1.18 (3H, d, J = 6.5 Hz, H-5b/H-6′); 13C-NMR (150 MHz, CD3OD) δ: 170.3 (s, C-1), 121.6 (d, C-2), 152.1 (d, C-3), 71.4 (d, C-4), 32.9 (t, C-5), 33.2 (t, C-6), 72.5 (d, C-7), 20.4 (q, C-8), 167.9 (s, C-1′), 122.7 (d, C-2′), 149.5 (d, C-3′), 76.2 (d, C-4′), 71.3 (d, C-5′), and 19.1 (q, C-6′).
(3R,5R)-3-hydroxy-5-decanolide (7), colorless oil; ESI-MS m/z: 209 [M + Na]+; 1H-NMR (600 MHz, CDCl3) δ: 4.72 (1H, dm, J = 11.2 Hz, H-5), 4.44–4.48 (1H, m, H-3), 2.78 (1H, dd, J = 17.7, 5.2 Hz, H-2a), 2.66 (1H, ddd, J = 17.7, 3.8, 1.6 Hz, H-2b), 1.99 (1H, dm, J = 14.7 Hz, H-4a), 1.78 (1H, ddd, J = 14.7, 11.2, 3.8 Hz, H-4b), 1.72–1.78 (2H, m, H-6), 1.30–1.34 (6H, m, H-7/8/9), and 0.93 (3H, t, J = 6.5 Hz, H-10); 13C-NMR (150 MHz, CDCl3) δ: 170.7 (s, C-1), 38.8 (t, C-2), 62.9 (d, C-3), 36.2 (t, C-4), 76.1 (d, C-5), 34.8 (t, C-6), 24.8 (t, C-7), 31.8 (t, C-8), 22.8 (t, C-9), and 14.2 (q, C-10).
3β-hydroxy-7α-methoxy-5α,6α-epoxy-8(14),22E-dien-ergosta (8), colorless oil; ESI-MS m/z: 443 [M + H]+, 465 [M + Na]+; 1H-NMR (600 MHz, CDCl3,) δ: 3.96 (1H, tt, J = 11.3, 4.7 Hz, H-3), 3.24 (1H, d, J = 2.7 Hz, H-6), 4.20 (1H, d, J = 2.1 Hz, H-7), 1.05 (3H, d, J = 6.7 Hz, H-21), 5.28 (2H, m, H-22/23), 0.89 (6H, s, H-18/19), 0.86 (3H, d, J = 6.5 Hz, H-26), 0.87 (3H, d, J = 6.8 Hz, H-27), 0.96 (3H, d, J = 6.7 Hz, H-28), and 3.45 (3H, s, 7-OCH3); 13C-NMR (150 MHz, CDCl3) δ: 153.6 (q, C-14), 135.6 (d, C-22), 132.5 (d, C-23), 122.8 (q, C-8), 72.9 (d, C-7), 69.0 (d, C-3), 65.5 (q, C-9), 40.2 (t, C-4), 39.6 (d, C-20), 36.8 (t, C-12), 36.3 (q, C-10), 33.3 (d, C-25), 32.5 (t, C-1), 31.5 (t, C-2), 27.5 (t, C-16), 25.2 (t, C-15), 21.5 (q, C-21), 20.2 (q, C-27), 19.9 (q, C-26), 19.5 (t, C-11), 18.5 (q, C-18), 17.9 (q, C-28), and 16.8 (q, C-19).
3β,5α,9α-trihydroxy-ergosta-7,22-dien-6-one (9), white powder; ESI-MS m/z: 467 [M + Na]+; 1H-NMR (600 MHz, CDCl3) δ: 4.09 (1H, m, H-3), 5.69 (1H, s, H-7), 1.06 (3H, s, H-18), 0.65 (3H, s, H-19), 1.05 (3H, d, J = 6.8 Hz, H-21), 5.20 (1H, dd, J = 15.4, 8.0 Hz, H-22), 5.23 (1H, dd, J = 15.4, 7.8 Hz, H-23), 0.85 (3H, d, J = 6.8 Hz, H-26), 0.87 (3H, d, J = 6.7 Hz, H-27), and 0.95 (3H, d, J = 6.8 Hz, H-28); 13C-NMR (150 MHz, CDCl3) δ: 25.5 (t, C-1), 26.4 (t, C-2), 67.4 (d, C-3), 33.8 (t, C-4),79.9 (s, C-5), 197.8 (d, C-6), 120.1 (d, C-7), 164.5 (s, C-8), 74.9 (d, C-9), 42.0 (s, C-10), 28.9 (t, C-11), 35.1 (t, C-12), 45.5 (s, C-13), 51.9 (d, C-14), 22.6 (t, C-15), 28.0 (t, C-16), 56.3 (d, C-17), 21.3 (q, C-18), 12.5 (q, C-19), 40.5 (d, C-20), 19.9 (q, C-21), 135.4 (d, C-22), 132.6 (d, C-23), 42.9 (d, C-24), 33.4 (d, C-25), 20.3 (q, C-26), 20.5 (q, C-27), and 17.8 (q, C-28).
3β,5α,6β-triol-7,22E-diene-ergosta (10), white powder; ESI-MS m/z: 453 [M + Na]+; 1H-NMR (600 MHz, C5D5N) δ: 4.85 (1H, m, H-3), 4.23 (1H, d, J = 10.2 Hz, H-6), 5.74 (1H, m, H-7), 0.65 (3H, s, H-18), 1.53 (3H, s, H-19), 1.05 (3H, d, J = 6.7 Hz, H-21), 5.14 (1H, dd, J = 15.3, 8.2 Hz, H-22), 5.21 (1H, dd, J = 15.3, 7.5 Hz, H-23), 0.84 (3H, d, J = 3.2 Hz, H-26), 0.85 (3H, d, J = 3.1 Hz, H-27), and 0.94 (3H, d, J = 6.8 Hz, H-28); 13C-NMR (150 MHz, C5D5N) δ: 32.7 (t, C-1), 33.8 (t, C-2), 67.6 (d, C-3), 42.0 (t, C-4), 76.1 (s, C-5), 74.3 (d, C-6), 120.5 (d, C-7), 141.6 (s, C-8), 43.7 (d, C-9), 38.1 (s, C-10), 22.4 (t, C-11), 39.9 (t, C-12), 43.8 (s, C-13), 55.3 (d, C-14), 23.5 (t, C-15), 28.5 (t, C-16), 56.1 (d, C-17), 12.5 (q, C-18), 18.8 (q, C-19), 40.9 (d, C-20), 21.2 (q, C-21), 136.2 (d, C-22), 132.1 (d, C-23), 43.1 (d, C-24), 33.3 (d, C-25), 19.9 (q, C-26), 20.2 (q, C-27), and 17.8 (q, C-28).
Stigmast-5-ene-3β-yl formate (11), colorless solid; ESI-MS m/z: 465 [M + Na]+; 1H-NMR (600 MHz, CDCl3) δ: 8.04 (1H, s, H-30), 4.73 (1H, m, H-3), 1.84 (1H, m, H-16), 1.57 (1H, m, H-1), 1.56 (1H, m, H-15), 1.46 (1H, m, H-11), 1.35 (1H, m, H-20), 1.32 (1H, m, H-22), 1.17 (1H, m, H-12), 1.10 (1H, m, H-17), 0.95 (1H, m, H-9), 0.93 (3H, d, J = 4.9 Hz, H-21), 0.84 (3H, d, J = 3.9 Hz, H-26), 0.83 (3H, d, J = 6.2 Hz, H-27), and 0.82 (3H, d, J = 5.0 Hz, H-29); 13C-NMR (150 MHz, CDCl3) δ: 36.9 (C-1), 27.8 (C-2), 74.0 (C-3), 38.1 (C-4), 139.3 (C-5), 123.0 (C-6), 31.9 (C-7), 31.8 (C-8), 50.0 (C-9), 36.6 (C-10), 21.0 (C-11), 39.7 (C-12), 42.3 (C-13), 56.7 (C-14), 24.3 (C-15), 28.2 (C-16), 56.0 (C-17), 19.3 (C-18), 11.9 (C-19), 36.1 (C-20), 18.8 (C-21), 33.9 (C-22), 26.0 (C-23), 45.8 (C-24), 29.1 (C-25), 19.8 (C-26), 12.0 (C-27), 23.0 (C-28), 19.0 (C-29), and 160.7 (C-30).
2.5 Cytotoxic activity
The cytotoxic activity of several compounds was assessed by conducting an assay using MTS, which is a new type of MTT analog. The method is often regarded as a “one-step” MTT assay as it allows the addition of reagents directly to cell cultures without the intermittent steps required in MTT assays. MTS holds an advantage over XTT owing to its increased solubility and non-toxic nature, enabling the reintroduction of cells to culture for further evaluation. The test concentration of each compound was 40 μM; for compounds with enhanced activity, a comprehensive screening of their activities at varying concentrations was conducted. Following the methods outlined in the literature (Su et al., 2013), five distinct cell lines (leukemia cell line HL-60, liver cancer cell line SMMC-7721, lung adenocarcinoma cell line A549, breast cancer cell line MDA-MB-231, and colon cancer cell line SW480) were selected for analysis. Cisplatin (40 μM) and paclitaxel (5 μM) were used as positive controls. All experiments were conducted in triplicate, and data were expressed as the mean ± standard deviation of three independent experiments.
2.6 Inhibitory activity against protein kinase Cα
According to the existing literature, certain types of polyketides showed antimicrobial bioactivity (Krohn et al., 2007). This finding suggests a limited number of studies exploring the activity of such compounds. To explore other activities of this type of polyketide, SwissTargetPrediction (STP)1 (accessed on 31 May 2022) was employed to identify potential targets associated with these metabolites (Gfeller et al., 2014). The results indicated that some metabolites may inhibit the activity of protein kinase Cα. Subsequently, compounds 1, 2, 3a/b, 5, 6, and 7 were evaluated to ascertain their protein kinase Cα inhibitory activity, using an enzyme-linked immunosorbent assay (ELISA) kit (Human Protein Kinase C, PKC ELISA Kit; Product number: kt80184, Wuhan Mosak Biotechnology Co., Ltd.). The experiment was conducted as follows: For sample addition, 50 μL of the standard sample was added to the wells in the microplate-coated plate, including five concentration points and 10 wells; the test samples included 10 μL of sample and 40 μL of diluent, with distilled water as the control. For incubation, the sample was incubated at 37°C for 30 min. For washing, each well was filled with washing solution, kept for 30 s, and subsequently discarded; the procedure was repeated five times. The enzyme was added (50 μL of enzyme-labeled reagent added to each well including the control), incubated, and washed (same as above). For color development, chromogenic agent A from the kit was initially added to each well, followed by the addition of 50 μL of chromogenic agent B from the same kit. The chromogenic agent was then incubated at 37°C in the dark for 15 min. For the termination stage, a termination solution (50 μL) was added to each well. For the determination stage, the absorbance (OD) of each well was measured at 450 nm within 15 min after adding the termination solution.
3 Results and discussion
3.1 Structural identification
Compound 1 is a colorless solid with a molecular weight of 291.1190 [M + Na]+ based on the results of high-resolution mass spectrometry. The molecular formula is determined as C14H20O5. The planar structure of compound 1 was determined based on the key correlations of the two-dimensional nuclear magnetic resonance spectroscopy (2D NMR) data (Table 1): 1H−1H COSY spectra revealed the correlations between H-2/H-3/H-4/H-5/H-6, H-8/H-9/H-10/H-11/H-12/H-13/H-14, and −C-2–C-3–C-4–C-5–C-6− and −C-8–C-9–C-10–C-11–C-12–C-13–C-14− fragments (Figure 2). Additionally, long-range heteronuclear multiple bond correlations (HMBCs) revealed specific associations: H-2 (δH 5.79) was correlated with C-1 (δC 165.5), C-3 (δC 143.4), and C-4 (δC 40.4); H-3 (δH 6.71) was correlated with C-1 (δC 165.5) and C-4 (δC 40.4); H-6 (δH 1.36) was correlated with C-4 (δC 40.4) and C-5 (δC 68.5); H-9 (δH 6.82) was correlated with C-7 (δC 165.7), C-8 (δC 121.2), and C-10 (δC 70.3); H-13 (δH 5.18) was correlated with C-11 (δC 29.2) and C-14 (δC 17.7); and H-14 (δH 1.19) was correlated with C-12 (δC 26.3) and C-13 (δC 69.2; Figure 2). The flat structure of compound 1 mirrored that of colletallol (Kauloorkar and Kumar, 2016). After carefully analyzing the spectral data of compound 1 and colletallol, notable discrepancies were observed in the chemical shift of certain carbons, particularly C-14. In compound 1, the chemical shift differences were δC 17.7 for C-14 (Table 1) and δC 19.5 for colletallol. In addition, the specific rotation value of compound 1 was [α]23D = 75.5 (c = 0.10, MeOH), while the specific rotation of colletallol was [α]23D = −107.5 (c = 0.40, CH2Cl2).
Among them, H-14 exhibited an NOE effect with H-12β and H-10. The configuration of 6-CH3 cannot be determined by NMR data, so the calculated ECD spectra and qcc NMR of four stereoisomers, 5S,10S,13S (1a), 5S,10R,13R (1b), 5R,10S,13S (1c), and 5R,10R,13R (1d), were performed. As given in Figure 3A, two cotton effects (CEs) with alternative signals were observed on the experimental spectrum. The 5S,10S,13S (1a) and 5R,10S,13S (1c) theoretical spectra exhibited two CEs with alternative signs, which was in good agreement with the experimental ECD spectra. Moreover, the two epimers, 5S,10S,13S (1a) and 5R,10S,13S (1c) were subjected to a strict conformational screening procedure, and the NMR chemical shifts were calculated at the mPW1PW91/6–31 + G(d,p)//M06-2X/def2-SVP level of theory with the PCM solvent model in methanol. The 13C NMR data with the correlation coefficient (R2) of 0.9973 of 5R,10S,13S (1c) were consistent with its experimental values (Figure 3B). So the absolute configuration of compound 1 was assigned as 5R,10S,13S (Figure 2) and was named gunniiol A.
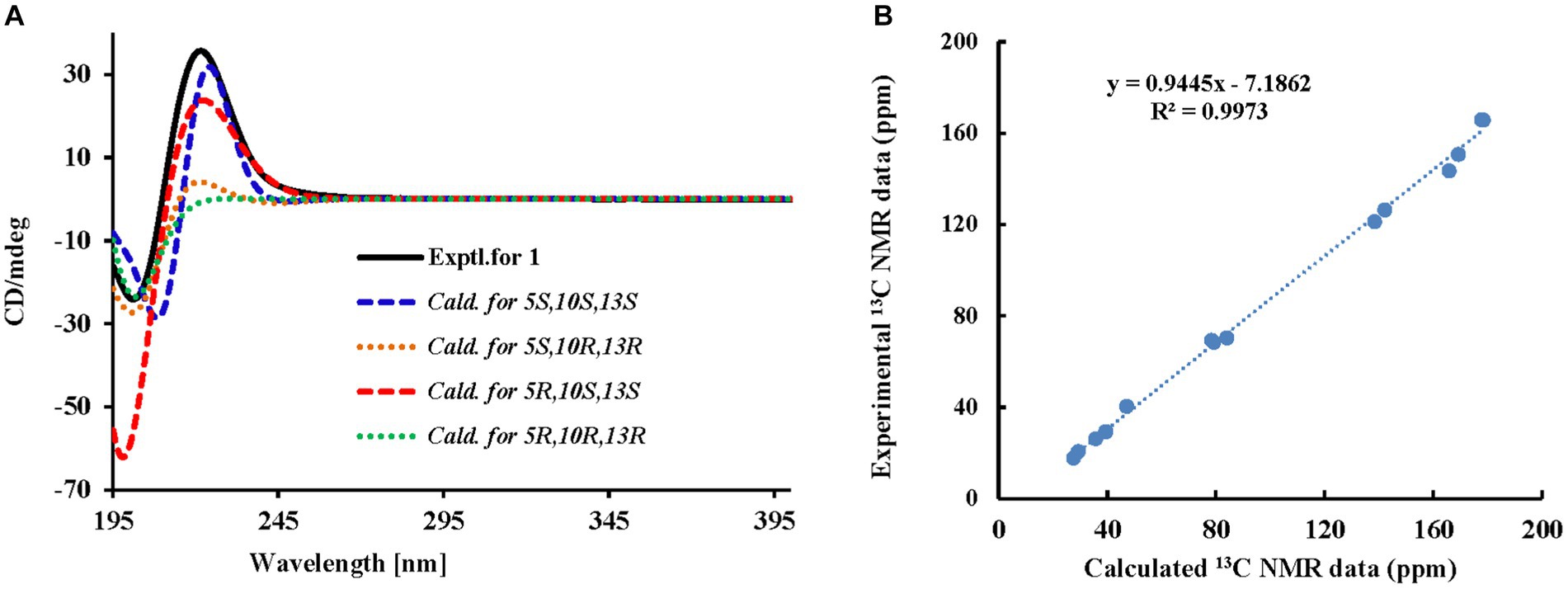
Figure 3. Experimental and calculated ECD curves and correlation plots of experimental and 13C NMR data of compound 1. (A) Experimental and calculated ECD curves; (B) Correlation plots of experimental and calculated 13C NMR data.
Compound 2 is colorless oil, and its molecular formula C12H18O5 was determined by HR-ESI-MS with m/z 265.1040 ([M + Na]+, calcd for: 265.1046), with four unsaturations. In the 13C-NMR and DEPT analysis (Table 1), the carbon signals of compound 2 appeared in pairs and were characterized by fatty acid chains (δC 170.6, 165.7, 146.2, 145.5, 123.8, 123.6, 38.5, 41.8, 69.0, 66.8, 19.9, and 23.2), which was preliminarily judged to be a polyketone compound composed of one or two fatty acids.
The structural units of the two long chains, H-2/H-3/H-4/H-5/H-6 and H-8/H-9/H-10/H-11/H-12, were determined through the 1H-1H COSY experiment of compound 2, revealing the structural motifs −C-2–C-3–C-4−C-5−C-6– and −C-8–C-9–C-10−C-11–C-12– (Figure 2). The HMBC data were used to determine the planar structure of compound 2: H-2 (δH 5.89) was correlated with C-1 (δC 170.6) and C-4 (δC 38.5); H-3 (δH 6.97) was correlated with C-1 (δC 170.6), C-2 (δC 123.8), C-4 (δC 38.5), and C-5 (δC 69.0); H-6 (δH 1.29) was correlated with C-4 (δC 38.5) and C-5 (δC 69.0); H-9 (δH 6.94) was correlated with C-7 (δC 165.7), C-10 (δC 41.8), and C-11 (δC 66.8); H-10 (δH 2.36) was correlated with C-8 (δC 123.6), C-9 (δC 145.5), C-11 (δC 66.8), and C-12 (δC 23.2); and H-12 (δH 1.23) was correlated with C-10 (δC 41.8) and C-11 (δC 66.8). The chemical shift of H-5 exhibited a notable 5.11 ppm shift to the low field, suggesting the connection of the two polyketide chains through 5-OH and C-7 ester bonds. The structure of compound 2 was determined as shown in Figure 2 and named gunniiol B.
Compound 3a/b is colorless oil. Its molecular formula was determined to be C14H24O6 (m/z 309.1300 [M + Na]+, calcd. 309.1309), with four unsaturations, by positive ion mode HR-ESI-MS. According to 13C-NMR and DEPT data (Table 2), the carbon signals of compound 3a/b indicated paired appearances, akin to compound 2, suggesting a polyketide. When the 1H- and 13C NMR spectra were analyzed, one fatty acid exhibited a similarity with compound 2, identified as 5-hydroxyhex-2E-enoic acid (δC 167.8/167.6, 124.5, 144.2/147.3, 39.3/42.6, 70.9/67.5, and 20.3/23.5). Another fatty acid chain, 4,7-dihydroxyoct-2E-enoic acid (δC 167.8, 120.8, 152.7, 71.5, 33.9/33.5, 35.9/32.9, 68.3/72.2, and 23.5/20.0), was also identified in the analysis (Table 2).
In the 1H-1H COSY experiment, clear correlations were observed between the two structural units H-2/H-3/H-4/H-5/H-6 and H-8/H-9/H-10/H-11/H-12/H-13/H-14, the structural units of the two long chains −C-2–C-3–C-4−C-5−C-6− and −C-8–C-9–C-10−C-11−C-12–C-13−C-14− (Figure 2) or H-2/H-3/H-4/H-5/H-6/H-7/H-8 and H-10/H-11/H-12/H-13/H-14, and the structural units of the two long chains −C-2–C-3–C-4−C-5−C-6–C-7−C-8− and −C-10−C-11−C-12–C-13−C-14−. HMBC analysis confirmed the planar structure of compound 3a/b (Figure 2). The two polyketide chains were linked in two ways: The chemical shift of H-5 to the low field reached δH 5.08 ppm, indicating that the two polyketide chains were connected by 5-OH and C-7 ester bonds. Alternatively, the chemical shift of H-7 to the low field reached δH 4.96 ppm, suggesting that the two polyketide chains were connected by 7-OH and C-9 ester bonds. The structures of compound 3a/b were determined (Figure 2) and designated as gunniiol C.
Compound 4 is a colorless solid. Its molecular formula was determined to be C8H14O4 (m/z 173.0808 [M − H]−, calcd. 173.0808) with two degrees of unsaturation, by negative ion mode HR-ESI-MS. According to the 13C-NMR and DEPT (Table 3) results, the carbon signals of compound 4 were similar to those of compound 1 (Table 1). Careful analysis of the NMR data revealed that compound 4 was one of the two polyketone chains of compound 1 and was 4,7-dihydroxyoct-2E-enoic acid. The structure of compound 4 was elucidated through the analysis of HMBC: H-2 (δH 5.98) was associated with C-1 (δC 170.2) and C-4 (δC 71.7); H-3 (δH 6.89) was associated with C-4 (δC 71.7) and C-1 (δC 170.2); H-6 (δH 1.54 and 1.47) was associated with C-5 (δC 34.0) and C-7 (δC 68.6); and H-8 (δH 1.16) was associated with C-6 (δC 35.9) and C-7 (δC 68.6). The structure of compound 4 was determined as 4,7-dihydroxyoct-2E-enoic acid (Figure 1).
The other compounds were identified as clonostachydiol (5) (Ojima et al., 2018), 7R-[[4R,5S-dihydroxy-1-oxo-2E-hexen-1-yl]oxy]-4S-hydroxy-2E-octenoic acid (6) (Ojima et al., 2018), (3R,5R)-3-hydroxy-5-decanolide (7) (Wu et al., 2009), 3β-hydroxy-7α-methoxy-5α,6α-epoxy-8(14),22E-dien-ergosta (8) (Wu et al., 2015), 3β,5α,9α-trihydroxy-ergosta-7,22-dien-6-one (9) (Cai et al., 2013), 3β,5α,6β-triol-7,22E-diene-ergosta (10) (Zhao et al., 2010), and stigmast-5-ene-3β-yl formate (11) (Chumkaew et al., 2010). These identifications were made by comparing the obtained data with the information reported in the respective references.
3.2 Cytotoxic activity
Compounds 1, 8, 9, and 11 were assessed to determine their cytotoxic activity using the MTS method. During an initial active screening, compound concentration was used at 40 μM; the results showed that compounds 1, 8, 9, and 11 had initial inhibitory rates of 21.5%, 100.0%, 15.4%, and 15.6%, respectively, against HL-60; 37.4%, 100.0%, 13.6%, and 0.33%, respectively, against A549 cells; 12.1, 100.0, 30.1, and 6.9%, respectively, against SMMC-7721; 37.9%, 95.5%, 24.4%, and 10.7%, respectively, against MDA-MB-231; and 41.2%, 100.0%, 21.6%, and 4.3%, respectively, against SW480 (Figure 4). Among them, the IC50 values of compound 8 were 12.89 ± 0.81 μM (HL-60), 15.69 ± 0.61 μM (A549), 3.00 ± 0.27 μM (SMMC-7721), 4.644 ± 0.270 μM (MDA-MB-231), and 14.02 ± 0.52 μM (SW480).
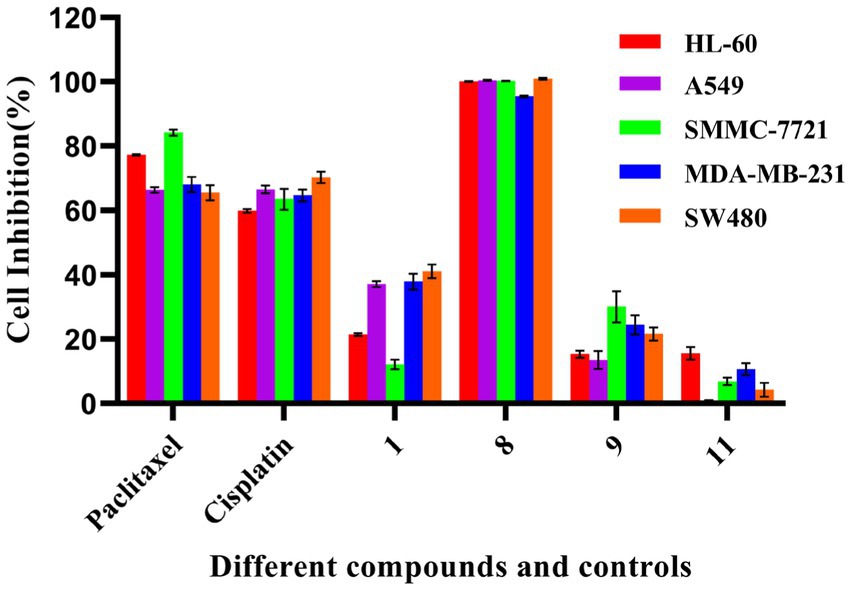
Figure 4. Cytotoxic activity of some compounds. The test concentration of each compound (1, 8, 9, and 11) was 40 μM; Cisplatin (40 μM) and paclitaxel (5 μM) were used as positive controls. All experiments were conducted in triplicate, and data were expressed as the mean ± standard deviation of three independent experiments.
3.3 Inhibitory activity against protein kinase Cα
In this study, the compounds were analyzed using the data obtained from the SwissTargetPrediction website, revealing that some of them had protein kinase Cα target sites. Subsequently, the effect of these compounds on protein kinase Cα inhibitory activity was assessed, which is determined based on the basic principles of ELISA. Compounds 1, 2, 3a/b, 5, 6, and 7 were evaluated at a concentration of 20 μg/mL. After substituting the OD values of the six compounds and the blank control, the actual activity concentrations of protein kinase Cα were determined. Compared with the control group, the protein kinase activity of the treated group was obtained. Then according to the inhibition rate formula, the inhibition rates of the six compounds on protein kinase Cα were 43.63%, 15.68%, 30.77%, 8.68%, 40.93%, and 57.66%, respectively. The activity results are shown in Figure 5. Results indicated that compounds 1, 6, and 7 had some inhibitory effect on protein kinase Cα activity.
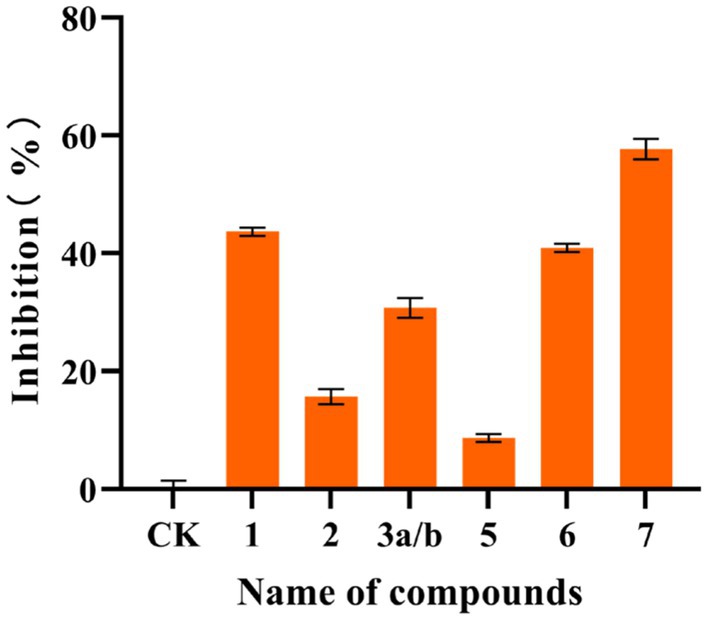
Figure 5. Inhibitory activity of some compounds on protein kinase Cα. The inhibitory activity of compounds against protein kinase Cα is determined based on the basic principles of ELISA. Compared with the control, the inhibition rates of the compounds 1, 2, 3a/b, 5, 6, and 7 at a concentration of 20 μg/mL on protein kinase Cα were 43.63%, 15.68%, 30.77%, 8.68%, 40.93%, and 57.66%, respectively. All experiments were conducted in triplicate, and data were expressed as the mean ± standard deviation of three independent experiments.
4 Discussion
The investigation of stroma and host complexes of Cordyceps has consistently been a focal point in research. However, acquiring these complexes in substantial quantities remains challenging. Consequently, researchers have focused on exploring the activities and components of their anamorphs. In a previous study, we screened P. gunnii YMF1.00003 under different culture conditions through the conduct of metabolome analysis and activity comparison and found that the fermentation extract of the mycelium in WGA was similar to the extract of stroma and host complexes and had obvious cytotoxic activity (Qu et al., 2022). Therefore, we further examined the metabolites and activities of P. gunnii YMF1.00003 fermented in two different media. Eleven compounds (1–11) were identified, including 1, 4, 8, and 11 from the modified WGA medium and other metabolites from the modified Sabouraud solid medium.
The extract of P. gunnii YMF1.00003 showed notable cytotoxic activity. Hence, several isolated compounds were evaluated to ascertain their cytotoxic activity. Compound 8 exhibited moderate cytotoxic activity against five tumor cell lines, with IC50 values of 3.00 ± 0.27 μM (SMMC-7721), 4.644 ± 0.270 μM (MDA-MB-231), 12.89 ± 0.81 μM (HL-60), 15.69 ± 0.61 μM (A549), and 14.02 ± 0.52 μM (SW480), respectively. Compound 1 showed weak cytotoxic activity against five tumor cell lines at a concentration of 40 μM. Ergosterols, particularly ergosterol peroxide, have been recognized for their potent cytotoxic activity (Zhabinskii et al., 2022; Hoang et al., 2023). According to the existing literature, the position of hydroxyl substitution, the position of double bonds, and the degree of oxidation are pivotal factors influencing cytotoxicity (Zhabinskii et al., 2022). The mechanism underlying the cytotoxic activity of this compound has been documented: ergosterol peroxide stimulates Foxo3 activity by inhibiting pAKT and c-Myc and activating the pro-apoptotic proteins Puma and Bax to induce cancer cell death (Li et al., 2016). Compound 1 is classified as a non-symmetric macrocyclic bislactone, and this type of bislactone has demonstrated certain antimicrobial bioactivity in previous studies (Krohn et al., 2007). SwissTargetPrediction was launched in 2014 and used as a web-based tool for predicting the potential protein targets of small molecules. It predicts the potential targets of all examined compounds based on their similarity with known bioactive compounds (Gfeller et al., 2014). However, virtual screening is frequently associated with high false-positive rates. Several compounds that receive high rankings for a specific target protein may not exhibit actual activity. During a typical virtual screening, only approximately 12% of the highest-scoring compounds demonstrated activity when subjected to biochemical analysis (Adeshina et al., 2020). Therefore, virtual screening serves as a crucial and beneficial tool, yet necessitating further experimental validation. In the present study, the STP was employed to identify targets associated with the metabolites (Gfeller et al., 2014). The results indicated a potential interaction between this type of bislactone and protein kinase Cα. Our active screening result showed that compounds 1, 6, and 7 inhibited the activity of protein kinase Cα by 43.63%, 40.93%, and 57.66% at a concentration of 20 μg/mL, respectively. Protein kinase Cα is implicated in various diseases, such as cardiovascular diseases (Turner et al., 2013), schizophrenia (Carroll et al., 2010), and the neural basis of episodic memory (MacLeod and Donaldson, 2014). However, due to the low inhibition rate of protein kinase Cα activity and the limited amount of compounds, further investigations were not pursued. Protein kinase C, a phospholipid- and calcium-dependent protein kinase, has been marketed as a drug target for multiple drugs, providing significant clinical benefits to patients with cardiovascular and cerebrovascular diseases, leukemia, and diabetes (Carter, 2000). The wild stroma and host complexes of Cordyceps fungus have been a focal point of research, but their scarcity has led researchers to concentrate on clonal mycelium. In the initial stages, we evaluated the Paecilomyces gunnii extracts under different culture conditions and from the wild stroma and host complexes through the performance of a metabolome analysis and identified the culture conditions for producing metabolites with enhanced cytotoxic activity (Qu et al., 2022). In the present study, fermentation was conducted under screened culture conditions to yield active natural products. The results showed that compound 3β-hydroxy-7α-methoxy-5α,6α-epoxy-8(14),22E-dien-ergosta (8) exhibited notable antitumor activity, while compounds 1, 6, and 7 displayed a certain inhibitory effect on protein kinase Cα.
With the rapid advancements in sequencing technology and bioinformatics, numerous fungal gene clusters have been found to remain “silent” under conventional culture conditions. This suggests the presence of a large number of potentially active compounds with novel structures that are concealed within these “silent” gene clusters and yet to be identified (Nielsen et al., 2017; Kjærbolling et al., 2019). The current study is based on a previous one stain-many compounds (OSMAC) strategy and the isolation and identification of promising compounds under optimized culture conditions (Qu et al., 2022). In recent years, the OSMAC strategy has proven successful in isolating compounds with novel structures and diverse activities from fungi (Bode et al., 2002). Following the screening of culture conditions, hydroxy-substituted fatty acids with nematicidal activity were obtained from Purpureocillium lavendulum (Liu et al., 2022). Diketopiperazine alkaloids were purified from a marine endophytic fungus Penicillium brocae with strong anti-Staphylococcus aureus activity and cytotoxic activity using the “OSMAC” strategy (Meng et al., 2016). The fungal genome harbors numerous transcription regulators, and the transcription and expression of functional genes are often controlled by these regulators. The discovery of new secondary metabolites can be facilitated by manipulating specific transcription factors (Keller, 2019). However, the efficacy of mining fungal metabolites through fermentation conditions remains contingent on factors such as whether the genomic data of the target fungus has been measured, the quality of the genomic data, and the establishment of genetic transformation.
Data availability statement
The original contributions presented in the study are included in the article/supplementary material, further inquiries can be directed to the corresponding author.
Ethics statement
Ethical approval was not required for the studies on humans in accordance with the local legislation and institutional requirements because only commercially available established cell lines were used.
Author contributions
S-SL: Data curation, Investigation, Writing – original draft. S-LQ: Data curation, Investigation, Writing – original draft. JX: Investigation, Writing – original draft. DL: Investigation, Data curation, Writing – original draft. P-JZ: Data curation, Funding acquisition, Investigation, Resources, Writing – review & editing.
Funding
The author(s) declare financial support was received for the research, authorship, and/or publication of this article. This work was supported in part by the National Key Research and Development Program (2023YFD1400400), the National Natural Science Foundation of China (31970060 and 32270132), the special fund of the Yunnan University “double first-class” construction, and the Applied Basic Research Foundation of Yunnan Province (202201BC070004 and 202102AA100013).
Acknowledgments
The authors are grateful to the Microbial Library of the Germplasm Bank of Wild Species from Southwest China for providing the Paecilomyces gunnii YMF1.00003 strain. We are grateful to Shi Bao-Bao for calculating the ECD and qcc NMR of compound 1.
Conflict of interest
The authors declare that the research was conducted in the absence of any commercial or financial relationships that could be construed as a potential conflict of interest.
Publisher’s note
All claims expressed in this article are solely those of the authors and do not necessarily represent those of their affiliated organizations, or those of the publisher, the editors and the reviewers. Any product that may be evaluated in this article, or claim that may be made by its manufacturer, is not guaranteed or endorsed by the publisher.
Footnotes
References
Adeshina, Y. O., Deeds, E. J., and Karanicolas, J. (2020). Machine learning classification can reduce false positives in structure-based virtual screening. Proc. Natl. Acad. Sci. U. S. A. 117, 18477–18488. doi: 10.1073/pnas.2000585117
Bode, H. B., Bethe, B., Höfs, R., and Zeeck, A. (2002). Big effects from small changes: possible ways to explore nature's chemical diversity. ChemBioChem 3, 619–627. doi: 10.1002/1439-7633(20020703)3:7<619::AID-CBIC619>3.0.CO;2-9
Cai, H. H., Liu, X. M., Chen, Z. Y., Liao, S. T., and Zou, Y. X. (2013). Isolation, purification and identification of nine chemical compounds from Flammulina velutipes fruiting bodies. Food Chem. 141, 2873–2879. doi: 10.1016/j.foodchem.2013.05.124
Carroll, L. S., Williams, N. M., Moskvina, V., Russell, E., Norton, N., Williams, H. J., et al. (2010). Evidence for rare and common genetic risk variants for schizophrenia at protein kinase Cα. Mol. Psychiatry 15, 1101–1111. doi: 10.1038/mp.2009.96
Carter, C. A. (2000). Protein kinase C as a drug target: implications for drug or diet prevention and treatment of cancer. Curr. Drug Targets 1, 163–183. doi: 10.2174/1389450003349317
Chumkaew, P., Kato, S., and Chantrapromma, K. (2010). New cytotoxic steroids from the fruits of Syzygium siamense. J. Asian Nat. Prod. Res. 12, 424–428. doi: 10.1080/10286021003762028
Gfeller, D., Grosdidier, A., Wirth, M., Daina, A., Michielin, O., and Zoete, V. (2014). SwissTargetPrediction: a web server for target prediction of bioactive small molecules. Nucleic Acids Res. 42, W32–W38. doi: 10.1093/nar/gku293
Hoang, C. K., Le, C. H., Nguyen, D. T., Tran, H. T. N., Luu, C. V., Le, H. M., et al. (2023). Steroid components of marine-derived fungal strain Penicillium levitum N33.2 and their biological activities. Mycobiology 51, 246–255. doi: 10.1080/12298093.2023.2248717
Kauloorkar, S. V., and Kumar, P. (2016). Total synthesis of (−)-(6,11,14)-colletallol proline catalyzed α-aminoxylation and Yamaguchi macrolactonization. RSC Adv. 6, 63607–63612. doi: 10.1039/C6RA08484B
Keller, N. P. (2019). Fungal secondary metabolism: regulation, function and drug discovery. Nat. Rev. Microbiol. 17, 167–180. doi: 10.1038/s41579-018-0121-1
Kjærbolling, I., Mortensen, U. H., Vesth, T., and Andersen, M. R. (2019). Strategies to establish the link between biosynthetic gene clusters and secondary metabolites. Fungal Genet. Biol. 130, 107–121. doi: 10.1016/j.fgb.2019.06.001
Krohn, K., Farooq, U., Flörke, U., Schulz, B., Draeger, S., Pescitelli, G., et al. (2007). Secondary metabolites isolated from an endophytic Phoma sp. – absolute configuration of tetrahydropyrenophorol using the solid-state TDDFT CD methodology. Eur. J. Org. Chem. 2007, 3206–3211. doi: 10.1002/ejoc.200601128
Kuo, Y. C., Tsai, W. J., Shiao, M. S., Chen, C. F., and Lin, C. Y. (1996). Cordyceps sinensis as an immunomodulatory agent. Am. J. Chin. Med. 24, 111–125. doi: 10.1142/S0192415X96000165
Li, X. M., Wu, Q. P., Bu, M., Hu, L. M., Du, W. W., Jiao, C. W., et al. (2016). Ergosterol peroxide activates Foxo3-mediated cell death signaling by inhibiting AKT and c-Myc in human hepatocellular carcinoma cells. Oncotarget 7, 33948–33959. doi: 10.18632/oncotarget.8608
Liu, R., Bao, Z. X., Li, G. H., Li, C. Q., Wang, S. L., Pan, X. R., et al. (2022). Identification of nematicidal metabolites from Purpureocillium lavendulum. Microorganisms 10:1343. doi: 10.3390/microorganisms10071343
Lu, R., Liu, X., Gao, S., Zhang, W., Peng, F., Hu, F., et al. (2014). New tyrosinase inhibitors from Paecilomyces gunnii. J. Agric. Food Chem. 62, 11917–11923. doi: 10.1021/jf504128c
Macleod, C. A., and Donaldson, D. I. (2014). PRKCA polymorphism changes the neural basis of episodic remembering in healthy individuals. PLoS One 9:e98018. doi: 10.1371/journal.pone.0098018
Meng, L. H., Wang, C. Y., Mándi, A., Li, X. M., Hu, X. Y., Kassack, M. U., et al. (2016). Three diketopiperazine alkaloids with spirocyclic skeletons and one bisthiodiketopiperazine derivative from the mangrove-derived endophytic fungus Penicillium brocae MA-231. Org. Lett. 18, 5304–5307. doi: 10.1021/acs.orglett.6b02620
Nielsen, J. C., Grijseels, S., Prigent, S., Ji, B. Y., Dainat, J., Nielsen, K. F., et al. (2017). Global analysis of biosynthetic gene clusters reveals vast potential of secondary metabolite production in Penicillium species. Nat. Microbiol. 2:17044. doi: 10.1038/nmicrobiol.2017.44
Ojima, K. I., Yangchum, A., Laksanacharoen, P., Tasanathai, K., Thanakitpipattana, D., Tokuyama, H., et al. (2018). Cordybislactone, a stereoisomer of the 14-membered bislactone clonostachydiol, from the hopper pathogenic fungus Cordyceps sp. BCC 49294: revision of the absolute configuration of clonostachydiol. J. Antibiot. 71, 351–358. doi: 10.1038/s41429-017-0008-9
Pu, X. J., Hu, Q. Y., Li, S. S., Li, G. H., and Zhao, P. J. (2021). Sesquiterpenoids and their quaternary ammonium hybrids from the mycelium of mushroom Stereum hirsutum by medium optimization. Phytochemistry 189:112852. doi: 10.1016/j.phytochem.2021.112852
Qu, S. L., Xie, J., Wang, J. T., Li, G. H., Pan, X. R., and Zhao, P. J. (2022). Activities and metabolomics of Cordyceps gunnii under different culture conditions. Front. Microbiol. 13:1076577. doi: 10.3389/fmicb.2022.1076577
Su, J., Zhao, P., Kong, L., Li, X., Yan, J., Zeng, Y., et al. (2013). Trichothecin induces cell death in NF-κB constitutively activated human cancer cells via inhibition of IKKβ phosphorylation. PLoS One 8:e71333. doi: 10.1371/journal.pone.0071333
Sun, H., Zhu, Z., Tang, Y., Ren, Y., Song, Q., Tang, Y., et al. (2018). Structural characterization and antitumor activity of a novel se-polysaccharide from selenium-enriched Cordyceps gunnii. Food Funct. 9, 2744–2754. doi: 10.1039/C8FO00027A
Turner, S. T., Boerwinkle, E., O'connell, J. R., Bailey, K. R., Gong, Y., Chapman, A. B., et al. (2013). Genomic association analysis of common variants influencing antihypertensive response to hydrochlorothiazide. Hypertension 62, 391–397. doi: 10.1161/HYPERTENSIONAHA.111.00436
Wu, J. Z., Gao, J., Ren, G. B., Zhen, Z. B., Zhang, Y. H., and Wu, Y. K. (2009). Facile access to some chiral building blocks. Synthesis of verbalactone and exophilin a. Tetrahedron 65, 289–299. doi: 10.1016/j.tet.2008.10.050
Wu, C. J., Yi, L., Cui, C. B., Li, C. W., Wang, N., and Han, X. (2015). Activation of the silent secondary metabolite production by introducing neomycin-resistance in a marine-derived Penicillium purpurogenum G59. Mar. Drugs 13, 2465–2487. doi: 10.3390/md13042465
Zhabinskii, V. N., Drasar, P., and Khripach, V. A. (2022). Structure and biological activity of ergostane-type steroids from fungi. Molecules 27:2103. doi: 10.3390/molecules27072103
Zhao, J. L., Mou, Y., Shan, T. J., Li, Y., Zhou, L. G., Wang, M. G., et al. (2010). Antimicrobial metabolites from the endophytic fungus Pichia guilliermondii isolated from Paris polyphylla var. yunnanensis. Molecules 15, 7961–7970. doi: 10.3390/molecules15117961
Keywords: Paecilomyces gunnii, polyketides, cytotoxic activity, protein kinase Cα inhibitory activity, calculated electronic circular dichroism
Citation: Li S-S, Qu S-L, Xie J, Li D and Zhao P-J (2024) Secondary metabolites and their bioactivities from Paecilomyces gunnii YMF1.00003. Front. Microbiol. 15:1347601. doi: 10.3389/fmicb.2024.1347601
Edited by:
Vijay K. Sharma, Agricultural Research Organization (ARO), IsraelReviewed by:
Fengyu Du, Qingdao Agricultural University, ChinaPundrik Jaiswal, National Institutes of Health (NIH), United States
Copyright © 2024 Li, Qu, Xie, Li and Zhao. This is an open-access article distributed under the terms of the Creative Commons Attribution License (CC BY). The use, distribution or reproduction in other forums is permitted, provided the original author(s) and the copyright owner(s) are credited and that the original publication in this journal is cited, in accordance with accepted academic practice. No use, distribution or reproduction is permitted which does not comply with these terms.
*Correspondence: Pei-Ji Zhao, pjzhao@ynu.edu.cn
†These authors have contributed equally to this work