- 1Key Laboratory of Tropical Medicinal Resource Chemistry of Ministry of Education, College of Chemistry and Chemical Engineering, Hainan Normal University, Haikou, Hainan, China
- 2Key Laboratory of Tropical Medicinal Plant Chemistry of Hainan Province, Haikou, Hainan, China
- 3Key Laboratory of Pharmaceutical Quality Control of Hebei Province, Key Laboratory of Medicinal Chemistry and Molecular Diagnostics of Education Ministry of China, College of Pharmaceutical Sciences, Hebei University, Baoding, China
Six new polyketides, which includes three new lactones (talarotones A–C) (1–3), one new polyketide (talarotide A) (4), two new polyenes (talaroyenes A, B) (5, 6), together with one new meroterpenoid (talaropenoid A) (7) and 13 known compounds (8–20) were isolated from the mangrove-derived fungus Talaromyces flavus TGGP35. The structure and configuration of the compounds 1–7 were elucidated from the data obtained from HR-ESI-MS, IR, 1D/2D NMR spectroscopy, Mo2 (OAc)4-induced electronic circular dichroism (ECD), CD spectroscopy, and modified Mosher's method. Compounds 5 and 20 displayed antioxidant activity with IC50 values of 0.40 and 1.36 mM, respectively. Compounds 3, 6, 11, 16, and 17 displayed cytotoxic activity against human cancer cells Hela, A549, and had IC50 values ranging from 28.89 to 62.23 μM. Compounds 7, 10–12, and 14–18 exhibited moderate or potent anti-insect activity against newly hatched larvae of Helicoverpa armigera Hubner, with IC50 values in the range 50–200 μg/mL. Compound 18 showed antibacterial activity against Ralstonia solanacearum with the MIC value of 50 μg/mL.
1 Introduction
The genus Talaromyces is identified as the sexual state of Penicillium and belongs to the Trichocomaceae family (Chaiyosang et al., 2021). The fungus Talaromyces, which is broadly dispersed in the natural environment (Zhang K. et al., 2022), demonstrates significant potential as a biological resource in food industry, ecology, agriculture, and medicine (Nicoletti et al., 2018; Devi et al., 2020; Prieto et al., 2021; Yadav et al., 2022; Aggarwal et al., 2023; De Eugenio et al., 2023; Xue et al., 2023). To further understand its characteristics, the biosynthetic pathways, biological activity (Chen et al., 2022; Lei et al., 2022; Lv et al., 2023; Nicoletti et al., 2023), and structure–activity relationship (Xie et al., 2022; Zhang M. et al., 2022) in Talaromyces have been extensively probed. These studies have also shown that Talaromyces exhibits numerous beneficial bioactivities and exceptional biosynthetic capabilities, which makes it suitable for wide-ranging industrial applications.
The genus Talaromyces has the capability to produce novel secondary metabolites with potent biological activities (Lei et al., 2022), such as antimicrobial depsidone (talaronin E) (Nicoletti et al., 2023) and polyketide (tanicutone B) (Wang et al., 2023), cytotoxic cytochalasan (talachalasin A) (Ding et al., 2023), dimeric oxaphenalenone aminoglycoside (glyclauxin D) (Samarasekera et al., 2023), antiviral cytochalasan (talachalasin B) (Ding et al., 2023), anti-inflammatory α-pyrone derivative (talarolactones E and F) (Li et al., 2023), phthalides (amestolkins A and B) (Huang et al., 2023), and insecticidal alkaloid (talaroenamine D) (Zang et al., 2015). Therefore, Talaromyces can be used to synthesize novel compounds (Kumari et al., 2018; Lan and Wu, 2020).
As a part of our ongoing investigation to identify secondary metabolites from marine-derived fungus with a novel structure and potent bioactivity (Bai et al., 2019a,b, 2021; Liao et al., 2019), we obtained six new isocoumarins from the fungus Talaromyces flavus TGGP35 (separated from the medicinal mangrove Acanthus ilicifolius) (Cai et al., 2022). With the aim of isolating more compounds with potent bioactivity from T. flavus TGGP35, the solid-state fermentation condition was changed (sea salt was replaced by sodium bromide). During our investigations, we also found that EtOAc extract, a modified fermentation extract from T. flavus TGGP35, showed potent anti-insect activity against newly hatched larvae of Helicoverpa armigera Hubner with IC50 value of 200 μg/mL. We carried out bioassay-guided separation following the isolation of the EtOAc extract and isolated three new lactones (talarotones A–C) (1–3), one new polyketide (talarotide A) (4), two new polyenes (talaroyenes A and B) (5,6), one new meroterpenoid (talaropenoid A) (7), along with 13 known compounds (8–20) (Figure 1), and characterized them. In this paper, we describe the isolation, determination of the structure, and the bioactivities of these compounds.
2 Results and discussion
Compound 1 was obtained as a yellow oil. From the HR-ESI-MS data [m/z 235.0939 [M + Na]+ (C11H16O4Na+, calcd. for 235.0941)], its molecular formula was determined as C11H16O4 (four degrees of unsaturation). The IR spectrum showed the presence of a hydroxyl group (3,534, 3,426 cm−1), an ester group (1,727 cm−1) and an olefine group (1,627, 1,618 cm−1) and compound 1. The 1H-NMR data (Table 1) revealed three olefinic protons at δH [6.98 (dd, J = 12.0, 2.0 Hz), 6.53 (dd, J = 12.0, 2.0 Hz) and 6.37 (dd, J = 15.2, 4.8 Hz)], four oxygenated hydrogen groups at δH [5.06 (s), 4.66 (s), 4.03 (dd, J = 5.2, 4.8 Hz), and 3.59 (m)], one methine group at δH 3.35 (m), one methylene group at δH [4.40 (dd, J = 8.8, 7.6 Hz) and 3.95 (dd, J = 8.8, 2.4 Hz)], two methyl groups at δH 1.18 (d, J = 6.8 Hz) and δH 0.97 (d, J = 6.4 Hz)]. The 13C NMR data (Table 2), combined with DEPT 135° spectrum, displayed 11 carbon resonances, including one ester carbonyl at δC (171.2), four olefinic carbons at δC (145.6, 134.9, 129.7, and 124.5), two oxygenated methine carbons at δC (74.6 and 69.3), one oxygenated methylene carbon at δC (72.4), one methine carbon at δC (31.5), and two methyl carbons at δC (20.0 and 18.2). Using 1H–1H COZY correlations of H-3/H-4/H-12, H-6/H-7, and H-8/H-9/H-10/H-11, together with the key HMBC correlations from H-12 to C-3/C-5, H-3/H-6 to C-1, H-6 to C-4, H-8 to C-6, H-9 to C-5, H-11 to C-10/C-9, we established the planar structure of 1 (Figure 2). The relative configuration of the cyclohexanone moiety in 1 was deduced from the coupling constants and nuclear Overhauser effect spectroscopy (NOESY). The coupling constant of 3JH − 6, H − 7 = 12.0 Hz indicated that H-6 and H-7 have a cis-form of diaxial relationship. The NOESY correlations of H-6 with H-8 and H-8 with H-11 (Figure 3) pointed to the Z configuration of the double bond, with H-8 and H-11 lying on the same side of the molecular structure. The above results also indicated that the configurations of these two double bonds were 6Z, 8Z, respectively. The hydroxy groups at C-9 and C-10 were determined to be oriented at a threo configuration from the coupling constant (J) value of 5.2 Hz between H-9 and H-10 as previously described for asperochratide D and plecmillins G-H (Wang et al., 2016; Zou et al., 2020).
The absolute configurations of C-9 and C-10 in 1 was determined by the in situ dimolybdenum CD method developed by Snatzke and Frelek (Liu et al., 2010; Wang et al., 2013; Dewapriya et al., 2018; Bai et al., 2019c) (Figure 4). According to the empirical rule proposed in the Snatzke's method (Dewapriya et al., 2018), a metal complex having a 1,2-diol moiety and Mo2(OAc)4 was generated as an auxiliary chromophore after the addition of Mo2(OAc)4 to a DMSO solution of 1. The observation of Cotton effect at ~300 nm in the induced spectrum originates from the chirality of the vic-diol, as exhibited by the O–C–C–O torsion angle in the favored conformation, which led to the assignment of the absolute configuration. A negative Cotton effect observed at 316 nm (Δε = −0.42) in the induced CD spectrum of 1 confirmed the 9R, 10R configurations (Figure 4). To determine the absolute configuration of 1, the theoretical ECD spectra of two possible stereoisomers of 4R, 9R, 10R and 4S, 9R, 10R were created by the TDDFT calculations, and the calculated ECD curve of the isomer 4R, 9R, 10R was found to have a good agreement with the experimental one (Figure 5). Therefore, the absolute configuration of 1 was determined as 4R, 9R, 10R, and compound was named talarotone A.
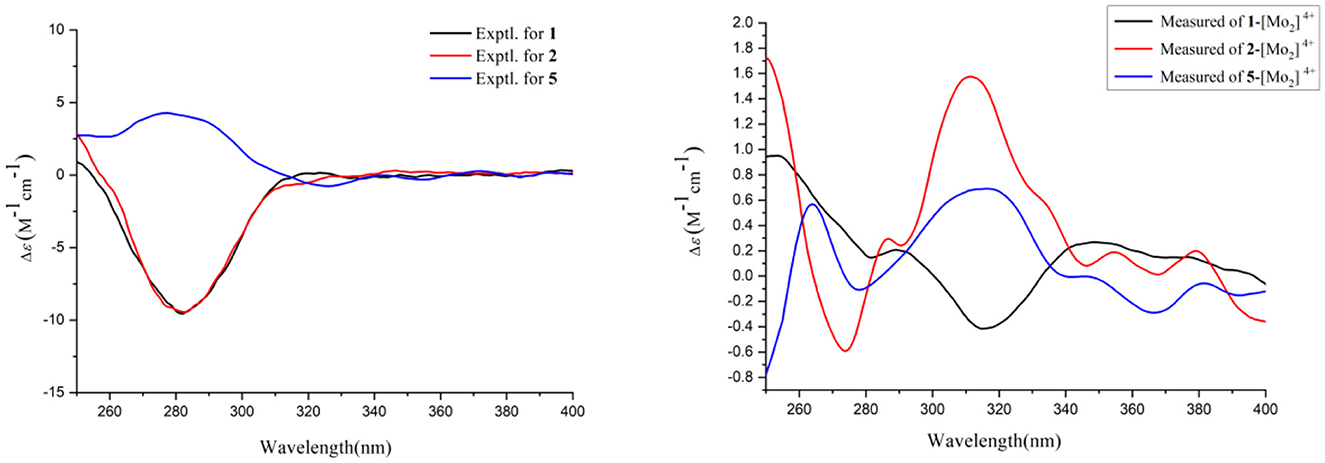
Figure 4. Experimental CD spectra of 1–2 and 5 and Experimental ECD spectra of the complex of 1–2 and 5 with the inherent CD spectral subtracted.
The HR-ESI-MS data showed that compound 2 also has same molecular formula C11H16O4 as 1. Analysis of 1D NMR data (Tables 1, 2) and HR-ESI-MS data suggested that 2 has a similar structure as 1 with the same carbon skeleton. A further investigation of 2 by DEPT135°, 1H-1H COZY, HMQC, and HMBC spectra established its planar structure, which is similar to 1. The NOESY correlations of H-6/H-8 and H-8/H-11 (Figure 3), combined with the coupling constants of 3JH − 6, H − 7 = 11.2 Hz, pointed to the 6Z, 8Z configurations of the two double bonds. The coupling constants of H-8/H-9 (5.2 Hz) suggested that 9,10-diols are oriented at a threo configuration (Wang et al., 2016; Zou et al., 2020). Likewise, the absolute configurations of C-9 and C-10 in 2 were also determined using an in situ dimolybdenum CD method (Dewapriya et al., 2018; Bai et al., 2019a). The positive Cotton effect at 311 nm (Δε = +1.58) confirmed the 9S, 10S configurations for C-9 and C-10 (Figure 4). The absolute configuration at C-4 was determined to be R through TDDFT ECD calculation (Figure 5). Thus, the absolute configuration of 2 was 4R, 9S, 10S, and the compound was named talarotone B.
Compound 3 was isolated as a yellow oil. Using the HR-ESI-MS peak at m/z 197.1181 [M + H]+ (C11H17, calcd. for 197.1172), its molecular formula was determined to be C11H16O3 (four degrees of unsaturation). Its IR spectrum revealed the presence of hydroxyl group (3,475 cm−1), ester group (1,712 cm−1), and olefine group (1,636, 1,618 cm−1). The 1H and 13C NMR data (Tables 1, 2) of 3 suggested the presence of one ester carbonyl group at δC 172.1, two olefine groups at (δH 6.13, δC 118.9; δH 6.81, δC 138.1; δC 129.4; δH 7.05, δC 147.4), two oxygenated methine groups at (δH 3.84, δC 67.6; δH 5.03, δC 77.4), two methylene groups at (δH 1.60, δC 38.2; δH 2.26, δC 29.8), and two methyl groups at (δH 1.21, δC 23.7; δH 1.42, δC 19.3). The 1H–1H COZY correlations showed the fragments of H-1/H-2/H-3/H-4/H-5/H-6 and H-8/H-9/H-11, and on integrating it with the key HMBC correlations from H-1 to C-2/C-3, H-4 to C-2/C-6, H-5 to C-7, H-8 to C-6, H-9 to C-7/8/10/11 (Figure 2), the whole structure of 3 was arrived at. The C-5 and C-6 atoms in 3 were found to be oriented in a trans configuration, which was determined based on a large coupling constant of 3JH − 5, H − 6 = 16.4 Hz. The NOESY correlation of H-6 and H-8 indicated a Z configuration of the double bond (Figure 3). Mosher's method was used to determine the absolute configuration of C-2 (Bai et al., 2019a). Unfortunately, because of the excessive humidity in the environment, this reaction was unsuccessful and we did not have enough amount of the compound to perform this reaction again. The absolute configuration on C-9 was determined as S by ECD calculation (Figure 5). Therefore, 3 was identified as talarotone C.
Compound 4 was isolated as a yellow oil, with the molecular formula of C13H20O3 (four degrees of unsaturation), which was determined from its HR-ESI-MS data. The IR data showed absorption bands at 1,708, 1,638, 1,617 cm−1 indicating the presence of carbonyl group. The 1H NMR data (Table 1) of 4 revealed two oxygenated methine groups at [δH 4.40 (m) and 3.47 (m)], one methine group at δH 2.48 (m), one methyl group at δH 2.10 (s), seven methylene groups at [δH 2.74 (m) and 2.47 (m), δH 2.53 (d, J = 3.6 Hz), δH 2.38 (t, J = 7.2 Hz), δH 2.03 (m) and 1.74 (m), δH 1.63 (m) and 1.51 (m), δH 1.56 (m), δH 1.38 (m)]. The 13C NMR data (Table 2) consist of signals for two carbonyl groups at δC (210.2 and 209.0), two oxygenated methine groups at δC (70.0 and 67.2), one methine group at δC 27.9, one methyl group at δC 29.9, seven methylene groups at δH (47.2, 47.0, 43.5, 37.5, 36.1, 31.6, and 19.6). The 1H–1H COZY correlations suggested the presence of a 2-pentane and a butane fragment as H-6/H-5/H-4/H-3/H-2/H-7 and H-8/H-9/H-10/H-11 in 4 (Figure 2). The key HMBC correlations from H-6/H-2 to C-1, H-2/6/7 to C-4, H-2/9 to C-7, H-5 to C-3, H-8 to C-5, H-11 to C-9, H-11/13 to C-12 and H-13 to C-11 led to the confirmation of a planar structure of 4 (Figure 2). The relative configuration of 4 was determined from NOESY and 1D NOE spectra. The irradiation of H-5 resulted in the enhancement of H-3 in the selective 1D NOE spectrum. This observation, combined with the correlations of H-5/H-8 to H-3 in the NOESY spectrum (Figure 3), indicated that H-5, H-8, and H-3 were placed on the same side of the molecule. The absolute configuration of 4 was determined as 3R, 5S, 8R by comparing the experimental and calculated ECD spectra using TDDFT (Figure 5). Based on these findings, the structure of 4 was named talarotide A.
Compound 5 was isolated as a yellow oil and its molecular formula of C11H16O4 (four degrees of unsaturation) was from determined from its HR-ESI-MS data. The IR spectrum of 5 exhibited absorptions at 3,514, 3,443, 1,711, and 1,618 cm−1, corresponding to the hydroxyl group, ester group, and olefine group, respectively. The 1H NMR data (Table 1) of 5 exhibited five olefinic protons at δH [7.15 (d, J = 8.8 Hz), 6.27 (m), 6.26 (d, J = 8.8 Hz), 6.02 (dd, J = 11.2, 2.0 Hz) and 5.75 (m)], two oxygenated methine groups at δH [3.97 (dd, J = 8.8, 5.2 Hz) and 3.55 (m)], two methyl groups at δH [1.47 (d, J = 6.8 Hz) and δH 0.95 (d, J = 6.4 Hz)]. The 13C NMR data (Table 2), combined with the DEPT data, displayed 11 resonances for one carboxyl carbon at δC 168.3, six olefinic carbons at δC (143.1, 138.2, 128.6, 127.3, 126.5, and 124.2), two oxygenated methine groups at δC (74.7 and 69.4), and two methyl groups at δC (18.2 and 14.9). The analysis of the COZY correlations revealed the presence of two structural fragments as CH3(10)-CH(9)-CH(8)-CH(7) and CH(5)-CH(4)-CH(3)-CH(2)-CH3(1) (Figure 2). The linkages of these two fragments were elucidated by the HMBC correlations of H-1 to C-3, H-3/H-7 to C-5, H-7 to C-9 and H-10 to C-8 (Figure 2). The coupling constant (3JH − 2, H − 3 = 11.2 Hz and 3JH − 4, H − 5 = 8.8 Hz) indicated that the corresponding protons are positioned on the same side. The NOESY correlations from H-7 to H-5, H-1 to H-4 indicated that the three double bonds have a 2Z, 4Z, 6Z configuration (Figure 3). The coupling constant analysis (3JH − 7, 8 = 5.2 Hz) showed that 5 has a threo-8,9-diol configuration. Similar to compounds 1 and 2, the absolute configurations of C-8 and C-9 in 5 were also assigned using an in situ dimolybdenum CD method (Dewapriya et al., 2018; Trang et al., 2022). The induced positive Cotton effect at 316 nm (Δε = +0.69), which indicates the O–C–C–O torsion angle, was consistent with positive helicity, which demonstrated the 8S, 9S configurations for 5 (Figure 4). The compound was named talaroyene A.
Compound 6 was obtained as a yellow oil, with a molecular formula of C11H18O3 (three degrees of unsaturation) as deduced from HR-ESI-MS data [m/z 197.1181 [M - H]−, (C11H17), calcd. for 197.1172]. The hydroxyl group (3,428 cm−1) and olefine group (1,692 and 1,619 cm−1) were observed in the IR spectrum. The 1H-NMR data (Table 1) showed three olefinic groups at δH [6.96 (t, J = 7.2 Hz), 5.99 (d, J = 11.2 Hz) and 5.83 (dd, J = 11.2, 6.8 Hz)], one oxygenated methine group at δH 3.53 (m), three methylene groups at δH [2.24 (td, J = 15.4, 7.2 Hz), 1.61 (m) and 1.47 (m)], two methyl groups at δH [1.55 (d, J = 6.8 Hz) and 0.93 (t, J = 7.2 Hz)]. The 13C-NMR data (Table 2) displayed 11 resonances, including one ester carbonyl carbon at δC 172.4, four olefinic carbons at δC (146.4, 130.4, 128.6, and 122.9), one oxygenated methine carbon at δC 72.9, three methylene carbons at δC (35.4, 30.3, and 26.3) and two methyl carbons at δC (15.2 and 10.0). The 1H–1H COZY spectrum of H-1/H-2/H-3 and H-5/H-6/H-7/H-8/H-9/H-10, combined with the key HMBC correlations from H-1 to C-3, H-2 to C-4, H-3 to C-5, H-5 to C-4/C-7/C-11, H-6 to C-4/C-8, H-7 to C-9 and H-10 to C-8, fully supported the structural connection of 6 (Figure 2). These 2D NMR data fully support the structural fragment of 6 containing a 6-hydroxy-2-propenyl-2-octenoic acid. The coupling constant of 3JH − 2, H − 3 = 11.2 Hz, combined with the NOESY correlation of H-3 with H-5 (Figure 3), pointed to the (2Z, 4Z) configuration of the double bonds. Mosher's method revealed the absolute configuration of C-8 in 6 was R (Bai et al., 2019a) (Figure 6). Thus, the absolute configuration of 6 was defined as 2Z, 4Z, 8R, and the compound was named talaroyene B.
Compound 7 was isolated as a white powder. Its molecular formula was deduced as C26H32O7 (11 degrees of unsaturation) from the HR-ESI-MS spectral data. The 1H and 13C NMR data (Table 3) revealed that 7 had a austin meroterpenoid skeleton and also a similar structure to brasilianoid G (Zhang et al., 2019). The obvious differences though were the presence of one methoxyl group at [δH 3.57 (s), δC 56.3 (CH3)] and one methyl group at [δH 1.62 (s), δC 15.8 (CH3)], and the absence of an aldehyde group at [δH 9.44 (s), δC 199.9 (C)] in 7. The key HMBC correlations from H-11 to C-9/11-OMe, H-13 to C-5/C-9/C-10 pointed out that the aldehyde group for C-11 in brasilianoid G was replaced by the methoxyl group in 7, and the double bond at [δH 5.24 (brs) and 5.74 (brs), δC 128.8 (CH2)] for C-13 in brasilianoid G was replaced by a methyl group in 7. The 1H–1H COZY and HMBC spectra established the complete structure of 7 (Figure 2). The ROESY correlations of H-1 with H-9′/11-OMe, H-9′ with H-15, and H-12 with H-14, and H-14 with H-7′, confirmed the relative configuration of 7 (Figure 3). The absolute configuration of 7 was determined as 5R, 8S, 11S, 1′S, 5′R, 6′R by ECD quantum chemical calculations (Figure 5). Thus, the structure of 7 was named talaropenoid A.
On comparing the physical and spectroscopic data with the literature, the 13 known compounds, consisting of 11 meroterpenoids, one lianene, and one alkaloid, were identified as preaustinoid A2 (8) (Geris dos Santos and Rodrigues-Fo, 2003), asperaustin C (9) (Wen et al., 2019), 7-hydroxyde-hydroaustin (10) (Arunpanichlert et al., 2015), dehydroaustin (11) (Hayashi et al., 1994), austin (12) (Hayashi et al., 1994), 11β-acetoxyisoaustinone (13) (Arunpanichlert et al., 2015), furanoaustinol (14) (Park et al., 2018), dehydroaustinol (15) (Marquez-Fernandez et al., 2007), austinolide (16) (Fill et al., 2007), brasilianoid B (17) (Zhang et al., 2018), preaustinoid D (18) (Duan et al., 2016), pinophol D (19) (Luo et al., 2021), and alteamide (20) (Wang et al., 2021).
The plausible biosynthetic pathways for autsin meroterpenoid derivatives 7–18 were proposed (Scheme 1). Austin meroterpenoids were synthesized through the polyisoprene pathway using the precursor farnesyl diphosphate (B) by the alkylation of intermediate 3,5-dimethylthiacyllic acid (A), resulting in the formation of an intermediate (C) (Arunpanichlert et al., 2015). A series of meroterpenoid precursors (D–G) were synthesized based on the intermediate (C), and compound 8 was produced by the hydroxylation of precursor (G). Compound 8 was converted into 9, 13, and 17 and 18 by demethylation, transesterification, and Baeyer–Villiger rearrangement reaction, respectively. Compound 13 serves as the starting point for the formation of 7, 10–12, and 14–16 through Baeyer–Villiger rearrangement, epoxidation, oxidation, dehydroxylation, hydrolysis, and aldol condensation reaction, respectively.
Compounds 5 and 20 were found exhibit a strong antioxidant activity with IC50 values of 0.40 and 1.36 mM, respectively, while the IC50 value of the positive control trolox is 0.29 mM.
Compounds 3, 6, 11, and 16 and 17 showed cytotoxic effects on Hela and A549 human cancer cells, with their IC50 values ranging from 28.89 to 62.23 μM (Table 4). All compounds exhibited no activity against the gastric cancer cell line RKO even at a concentration of 100 μM.
Compounds 7, 10–12, and 14–18 displayed moderate or strong anti-insect activity against newly hatched larvae of H. armigera Hubner, with their IC50 values ranging from 50 to 200 μg/mL (Table 5), while the IC50 value of the positive control azadirachtin is 50 μg/mL. Other compounds showed no growth inhibition activity against newly hatched larvae of H. armigera Hubner even at a concentration of 200 μg/mL.
Structure-activity relationships of antiinsect activity against the newly hatched larvae of H. armigera Hubner with regard to interaction with meroterpenoids has been discussed. The substitution of hydroxyl and acetyl groups and the ether ring moiety on the skeleton of meroterpenoids influences their anti-insect activity. Compound 10, which has a hydroxyl group on C-7, exhibits a better anti-insect activity superior to that of 11, suggesting the hydroxyl group at C-7 contributes to an increased growth inhibition potency. The growth inhibition activity data of 12 and 13 indicates that the central ether ring moiety was a non-essential functional group for anti-insect activity in the structure of meroterpenoids. Compound 15, which has a hydroxyl group on C-13, showed a higher anti-insect activity than compounds 11 and 16, suggesting that 13-OH group enhances anti-insect activity, and the acetylation of 13-OH decreases the anti-insect activity.
The antibacterial activity of all compounds was assessed against Staphylococcus aureus, Staphylococcus epidermidis, Pseudomonas aeruginosa, Escherichia coli, and Ralstonia solanacearum. Compound 18 displayed a weak antibacterial activity against R. solanacearum even at an MIC value of 50 μg/mL, while the MIC value of the positive control streptomycin is 12.5 μg/mL. Other compounds showed no antibacterial activity against the bacterial species tested even at a concentration of 100 μg/mL.
These results suggest that anstin meroterpenoids are capable of contributing to the development of novel biopesticides such as microbial insecticides and antibiotics.
3 Materials and methods
3.1 General experimental procedures
The melting points of the isolated compounds were determined on a WRX-4 micromelting point apparatus (Shanghai YiCe Apparatus and Equipment Co., Ltd., Shanghai, China). CD spectra of the compounds were recorded on a Mos-500 spectrometer. IR spectra were recorded on a Thermo Nicolet 6700 (using KBr disks) spectrophotometer. PERSEE TU-1990 spectrophotometer was used for recording the UV spectra. Optical rotations were measured using a JASCO P-1020 digital polarimeter (JASCO, Tokyo, Japan). 1D and 2D NMR spectra were recorded from a Bruker AV spectrometer (400 MHZ for 1H and 100 MHZ for 13C) and a JNM-ECZS spectrometer (600 HMZ for 1H and 150 MHZ for 13C). HR-ESI-MS spectra were obtained from a Q-TOF Ultima Global GAA076 LC mass spectrometer. ESI-MS spectra were recorded on a MAT-95-MS mass spectrometer. Agilent 1100 prep-HPLC system with an Agilent C18 analytical (9.4 × 250 mm, 5 μm) HPLC column was utilized for performing high-performance liquid chromatography (HPLC). Silica gel (100–200 and 200–300 mesh, Qingdao Marine Chemical Factory, Qingdao, China) were employed in column chromatography (CC) and Sephadex LH-20 gel column (Amersham Blosclences manage) were used for recording CC. Biological activities were tested in ultra-clean workbench (Suzhou Sujing Company) and these results were tested with a full wavelength multifunctional microplate reader (BioTek, USA). Methanol, ethyl acetate, petroleum ether, chloroform, dimethyl sulfoxide, and other conventional chemical reagents used in the experimental investigations (Guangzhou Xilong Chemical Reagent Factory) (Cai et al., 2022).
3.2 Fungal materials
The fungus TGGP35 was isolated from the stem of the mangrove plant Acanthus ilicifolius and the sequence data have been deposited in GenBank (accession number MT071116). The fungal strain was identified as Talaromyces flavus (Eurotiales: Trichocomaceae) (Cai et al., 2022).
3.3 Fermentation, extraction, and isolation
The fungal strain TGGP35 was grown on solid rice cultures in 1 L Erlenmeyer flasks (100 flasks; 50 mL of rice and 1.0 gram of sodium bromide per Erlenmeyer flask, autoclave sterilization) at 28°C for 32 days. The fermentation was extracted three times with ethyl acetate (EtOAc), followed by vacuum concentration, thus generating EtOAc extracts weighing 90.7 g.
All the EtOAc extracts were subjected to silica gel column chromatography (CC) using a gradient elution of petroleum ether/EtOAc (v/v, gradient 100:0–0:100) and EtOAc/MeOH (v/v, gradient 100:0–70:30), which resulted in the separation of 15 fractions (Fr. A-Fr. O). Fr. L (30.9 g) was separated by silica gel CC (200–300 mesh) using a gradient elution of petroleum ether/EtOAc system (9:1–0:1) to obtain six fractions (Fr. L.1–Fr. L.6) by TLC analysis, and then subfraction Fr. L.2 was in semi-preparative HPLC (MeOH-H2O, 25:75, ν/ν) to obtain compounds 1 (14.3 mg), 2 (10.4 mg), 3 (12.1 mg), 8 (12.6 mg), and 18 (5.8 mg). Subfraction Fr. L.3 was further separated by semi-preparative HPLC (MeOH-H2O, 30:70, ν/ν) to obtain compound 6 (6.2 mg). Subfraction Fr. L.4 was further separated by semi-preparative HPLC (MeOH-H2O, 34:66, ν/ν) to provide compounds 4 (5.3 mg), 5 (4.2 mg), and 20 (5.7 mg). Fr. D2 (2.1 g) was put through a Sephadex LH-20 column (petroleum ether-CHCl3-MeOH, 2:1:1, v/v) and subjected to semi-preparative HPLC (MeOH-H2O, 60:40, ν/ν) to obtain compound 19 (6.2 mg). Fr. M (20.3 g) was separated by silica gel CC (200–300 mesh) using a gradient elution of petroleum ether/EtOAc system (5:1–0:1) to obtain six fractions (Fr. M.1-Fr. M.6), and then subfraction Fr. M.2 was further separated by semi-preparative HPLC (MeOH-H2O, 20:80, ν/ν) to generate compounds 7 (4.2 mg), 9 (5.9 mg), 12 (8.3 mg), 14 (13.1 mg), and 17 (6.8 mg). Subfraction Fr. M.3 was further separated by semi-preparative HPLC (MeOH-H2O, 10:90, ν/ν) to obtain compounds 10 (11.4 mg), 11 (3.5 mg), 13 (12.4 mg), 15 (10.5 mg), and 16 (7.6 mg).
3.4 Spectroscopic data
Talarotone A (1): yellow oil; −34.4 (c 0.10, MeOH); UV (MeOH) λmax (log ε) 265, 218 nm; IR (KBr) νmax 3,534, 3,426, 1,727, 1,627, 1,618 cm−1; CD (c 0.05, MeOH) λmax (Δε) 281.4 (−9.67) nm; 1H and 13C NMR data see Tables 1, 2; HR-ESI-MS m/z: 235.0939 [M + Na]+, (C11H16O4Na+, calcd. for 235.0941).
Talarotone B (2): yellow oil; −32.4 (c 0.10, MeOH); UV (MeOH) λmax (log ε) 268, 220 nm; IR (KBr) νmax 3,521, 3,420, 1,717, 1,618, 1,384 cm−1; CD (c 0.05, MeOH) λmax (Δε) 281 (−9.28) nm; 1H and 13C NMR data see Tables 1, 2; HR-ESI-MS m/z: 235.0939 [M + Na]+, (C11H16O4Na+, calcd. for 235.0941).
Talarotone C (3): yellow oil; +12.6 (c 0.10, MeOH); UV (MeOH) λmax (log ε) 310, 221 nm; IR (KBr) νmax 3,475, 1,712, 1,636, 1,618 cm−1; CD (c 0.05, MeOH) λmax (Δε) 209 (−2.52) nm; 1H and 13C NMR data see Tables 1, 2; HR-ESI-MS m/z: 197.1181 [M + H]+, (C11H17, calcd. for 197.1172).
Talarotide A (4): yellow oil; −23.6 (c 0.10, MeOH); UV (MeOH) λmax (log ε) 306, 219 nm; IR (KBr) νmax 1,708, 1,638, 1,617 cm−1; CD (c 0.05, MeOH) λmax (Δε) 245 (+3.93), 264 (−11.89) nm; 1H and 13C NMR data see Tables 1, 2; HR-ESI-MS m/z: 225.1473 [M + H]+, (C13H21, calcd. for 225.1465).
Talaroyene A (5): yellow oil; +18.4 (c 0.10, MeOH); UV (MeOH) λmax (log ε) 218, 200 nm; IR (KBr) νmax 3,514, 3,443, 1,711, 1,618, 1,385 cm−1; CD (c 0.05, MeOH) λmax (Δε) 279.71 (+4.29) nm; 1H and 13C NMR data see Tables 1, 2; HR-ESI-MS m/z: 211.0937 [M – H]−, (C11H15, calcd. for 211.0965).
Talaroyene B (6): yellow oil; −24.2 (c 0.10, MeOH); UV (MeOH) λmax (log ε) 307, 217 nm; IR (KBr) νmax 3,428, 1,692, 1,619, 614 cm−1; 1H and 13C NMR data see Tables 1, 2; HR-ESI-MS m/z: 197.1181 [M – H]−, (C11H17, calcd. for 197.1172).
(S)-MTPA ester of 6: 1H NMR (CDCl3, 600 MHz): δH 7.01 (1H, t, J = 7.2 Hz, H-5), 5.99 (1H, d, J = 12.0 Hz, H-3), 5.86 (1H, dd, J = 12.0, 6.8 Hz, H-2), 2.36 (2H, m, H-6), 1.63 (2H, m, H-7), 1.57 (3H, d, J = 6.6 Hz, H-1), 1.47 (2H, m, H-9), 0.95 (3H, d, J = 7.2 Hz, H-10); ESI-MS m/z 654.4 [M + H]+.
(R)-MTPA ester of 6: 1H NMR (CDCl3, 600 MHz): δH 7.35 (1H, m, H-5), 7.22 (1H, d, J = 9.6 Hz, H-3), 7.13 (1H, dd, J = 9.6, 2.4 Hz, H-2), 2.37 (2H, m, H-6), 1.64 (2H, m, H-7), 1.62 (3H, d, J = 7.2 Hz, H-1), 1.43 (2H, m, H-9), 0.92 (3H, d, J = 7.2 Hz, H-10); ESI-MS m/z 692.1 [M + K]+.
Talaropenoid A (7): White powder; −22.6 (c 0.10, MeOH); mp. 182.3–188.7°C; UV (MeOH) λmax (log ε) 245 nm; IR (KBr) νmax 3,549, 3,413, 1,617, 1,613, 603 cm−1; CD (c 0.05, MeOH) λmax (Δε) 230 (+39.65) nm; 1H and 13C NMR data see Tables 1, 2; HR-ESI-MS m/z: 445.0281 [M – H]−, (C26H31, calcd. for 455.2064).
3.5 Biological assays
3.5.1 Antioxidant activity
The antioxidant activity assay was performed following a previously reported method (Zeng et al., 2022). The assay was performed in a 96-well microplate by adding 10 μL of the sample solution to 200 μL of ABTS working solution. Concentration gradients of 2.0, 1.0, 0.5, and 0.25 mg/mL were prepared for all the test groups, including a positive control. The blank control consisted of PBS buffer, DMSO served as the negative control, and Trolox was used as the positive control, with the IC50 value of 0.29 mM. The measurement of antioxidant effect was done using a full wavelength multifunctional microplate reader at a specific wavelength of 734 nm. The inhibition rate of each sample was calculated using the formula: inhibition rate = [(Ablank – Acompound)/Ablank]*100%. Finally, the IC50 value was calculated using the SPSS software.
3.5.2 Cytotoxic activity
All compounds were assessed for their cytotoxic activity against three human cell lines (A549, Hela, RKO) using the MTT method (Mosmann, 1983). The sample concentrations were prepared in five gradient levels: 100, 80, 60, 40, and 20 μM. The experiment was repeated more than three times, and the experimental data were measured using a full-wavelength microplate reader with a test wavelength of 492 nm. DMSO was used as the negative control, and doxorubicin hydrochloride served as the positive control. The inhibition rate of each sample was calculated using the formula: inhibition rate = [(ODcompound – ODDMSO)/ODDMSO]*100%. The IC50 value was determined using GraphPad Prism software.
3.5.3 Anti-insect activity
The growth inhibition activity against newly hatched larvae of Helicoverpa armigera Hubner was tested using methods from literature (Bai et al., 2019a). The tested compounds and the positive control azadirachtin were dissolved in DMSO at a concentration of 1 mg/mL. The activity was evaluated by adding serial dilutions of the tested compounds and azadirachtin (concentrations of 200, 100, 50, 25, and 12.5 μL/well) as an artificial diet for the newly hatched larvae. Each treatment was replicated three times, and the bioassay diet was placed in six-well plates. The larvae were then incubated at a controlled temperature of 25 ± 1°C at a relative humidity of 80%. DMSO was used as the negative control, azadirachtin was used as the positive control, and the artificial diet was used as the blank control. The mortality rate of the larvae was recorded on the 2nd, 4th, 6th, and 8th day after treatment.
3.5.4 Antibacterial activity
The antibacterial activity of 1–20 was assessed against five pathogenic bacteria, including Staphylococcus aureus, S. epidermidis, Escherichia coli, Pseudomonas aeruginosa, and Ralstonia solanacearum by the microplate assay method (Pierce et al., 2008). The broth medium containing pathogenic bacteria was used as the blank group, DMSO as the negative control, and ciprofloxacin and streptomycin were used as positive control.
4 Conclusions
In summary, 20 secondary metabolites, including six new polyketides (1–6) and one new meroterpenoid (7), and 13 known compounds were isolated from mangrove-derived fungus T. flavus TGGP35. Compounds 5 and 20 demonstrated moderate antioxidant capability, with IC50 values of 0.40 and 1.36 mM, respectively. Compounds 3, 6, 11, and 16 and 17 exhibited weak cytotoxic activity on Hela and A549 human cancer cells, with IC50 values ranging from 28.89 to 62.23 μM. Compounds 7, 10–12, and 14–18 displayed moderate or significant anti-insect activity, with the IC50 values ranging from 50 to 200 μg/mL. Compound 18 showed antimicrobial activity against R. solanacearum, with an MIC value of 50 μg/mL. The biosynthetic pathway and structure–activity relationship with regard to the anti-insect activity of meroterpenoids were explained in detail.
Data availability statement
The datasets presented in this study can be found in online repositories. The names of the repository/repositories and accession number(s) can be found in the article/Supplementary material.
Ethics statement
Ethical approval was not required for the studies on humans in accordance with the local legislation and institutional requirements because only commercially available established cell lines were used. Ethical approval was not required for the studies on animals in accordance with the local legislation and institutional requirements because only commercially available established cell lines were used.
Author contributions
JC: Data curation, Writing – original draft. XZho: Methodology, Writing – original draft. BW: Methodology, Writing – original draft. XZha: Methodology, Writing – original draft. ML: Methodology, Writing – original draft. LH: Methodology, Writing – original draft. RW: Methodology, Writing – original draft. YC: Methodology, Writing – original draft. XL: Methodology, Writing – original draft. YL: Methodology, Writing – original draft. GC: Methodology, Writing – original draft. FC: Validation, Writing – review & editing. GH: Validation, Writing – review & editing. CZ: Validation, Writing – review & editing.
Funding
The author(s) declare financial support was received for the research, authorship, and/or publication of this article. This work was supported by the National Natural Science Foundation of China (Nos. 32160108 and 2217702), Key Research and Development Program of Hainan Province (Nos. ZDYF2021SHFZ270 and ZDYF2021SHFZ108), Key Science and Technology Program of Hainan Province (No. ZDKJ202008), the Innovation Center for Academicians of Hainan Province, and the Specific Research Fund of the Innovation Center for Academicians of Hainan Province (No. YSPTZX202309).
Acknowledgments
We thank Public Technology Research and Sharing Center of the Tropical Biotechnology Research Institute of the Chinese Academy of Tropical Agricultural Sciences for their support in testing for Circular dichroism spectrometer (Mos-500).
Conflict of interest
The authors declare that the research was conducted in the absence of any commercial or financial relationships that could be construed as a potential conflict of interest.
Publisher's note
All claims expressed in this article are solely those of the authors and do not necessarily represent those of their affiliated organizations, or those of the publisher, the editors and the reviewers. Any product that may be evaluated in this article, or claim that may be made by its manufacturer, is not guaranteed or endorsed by the publisher.
Supplementary material
The Supplementary Material for this article can be found online at: https://www.frontiersin.org/articles/10.3389/fmicb.2024.1342843/full#supplementary-material
References
Aggarwal, S., Dorairaj, S., and Adlakha, N. (2023). Stoichiometric balance ratio of cellobiose and gentiobiose induces cellulase production in Talaromyces cellulolyticus. Biotechnol. Biofuels 16, 48–63. doi: 10.1186/s13068-023-02296-1
Arunpanichlert, J., Rukachaisirikul, V., Phongpaichit, S., Supaphon, O., and Sakayaroj, J. (2015). Meroterpenoid, isocoumarin and phenol derivatives from the seagrass-derived fungus Pestalotiopsis sp. PSU-ES194[J]. Tetrahedron 71, 882–888. doi: 10.1016/j.tet.2014.12.009
Bai, M., Huang, G.-L., Mei, R.-Q., Wang, B., Luo, Y.-P., Nong, X.-H., et al. (2019c). Bioactive lactones from the mangrove-derived fungus Penicillium sp. TGM112. Mar. Drugs. 17, 433–442. doi: 10.3390/md17080433
Bai, M., Zheng, C.-J., and Chen, G.-Y. (2021). Austins-type meroterpenoids from a mangrove-derived Penicillium sp. J. Nat. Prod. 84, 2104–2110. doi: 10.1021/acs.jnatprod.1c00050
Bai, M., Zheng, C.-J., Huang, G.-L., Mei, R.-Q., Wang, B., Luo, Y.-P., et al. (2019a). Bioactive meroterpenoids and isocoumarins from the mangrove-derived fungus Penicillium sp. TGM112. J. Nat. Prod. 82, 1155–1164. doi: 10.1021/acs.jnatprod.8b00866
Bai, M., Zheng, C.-J., Nong, X.-H., Zhou, X., Ming Luo, Y.-P., and Chen, G. (2019b). Four new insecticidal xanthene derivatives from the mangrove-derived fungus Penicillium sp. JY246. Mar. Drugs 17, 649–657. doi: 10.3390/md17120649
Cai, J., Zhu, X.-C., Zeng, W.-N., Wang, B., Luo, Y.-P., Liu, J., et al. (2022). Talaromarins A-F: six new isocoumarins from mangrove-derived fungus Talaromyces flavus TGGP35. Mar. Drugs 20, 361–373. doi: 10.3390/md20060361
Chaiyosang, B., Kanokmedhakul, K., Soytong, K., Poeaim, S., Soytong, M., Hadsadee, S., et al. (2021). New pyrrolobenzoxazine sesquiterpenoid derivatives from the fungus Talaromyces trachyspermus. Planta Med. 87, 600–610. doi: 10.1055/a-1392-1038
Chen, Z.-W., Lin, F.-J., Chen, B.-B., Chen, Q.-L., and Hong, X. (2022). Advance of studies on secondary metabolites of the genus Talaromyces. Shengwu Ziyuan 44, 362–369.
De Eugenio, L.-I., Murguiondo, C., Galea-Outon, S., Galea-Outon, S., Prieto, A., and Barriuso, J. (2023). Fungal-lactobacteria consortia and enzymatic catalysis for polylactic acid production. J. Fungi 9, 342–358. doi: 10.3390/jof9030342
Devi, R., Kaur, T., Kour, D., Rana, K.-L., Yadav, A., and Yadav, A.-N. (2020). Beneficial fungal communities from different habitats and their roles in plant growth promotion and soil health. Microb. Biosyst. 5, 21–47. doi: 10.21608/mb.2020.32802.1016
Dewapriya, P., Khalil, Z.-G., Prasad, P., Salim, A.-A., Cruz-Morales, P., Marcellin, E., et al. (2018). Talaropeptides A-D: structure and biosynthesis of extensively N-methylated linear peptides from an australian marine tunicate-derived Talaromyces sp. Front. Chem. 6, 394–408. doi: 10.3389/fchem.2018.00394
Ding, X.-M., Ye, W.-X., Tan, B., Song, Q.-Y., Chen, Y.-C., Liu, W., et al. (2023). Talachalasins A-C, undescribed cytochalasans with a 16β-methyl or 2-oxabicyclo[3.3.1]nonan-3-one unit from the deep-sea-derived fungus Talaromyces muroii sp. SCSIO 40439. Chin. J. Chem. 41, 915–923. doi: 10.1002/cjoc.202200744
Duan, R.-T., Zhou, H., Yang, Y.-B., Li, H.-T., Dong, J. W., Li, X. Z., et al. (2016). Antimicrobial meroterpenoids from the endophytic fungus Penicillium sp. T2-8 associated with Gastrodia elata. Phytochem. Lett. 18, 197–201. doi: 10.1016/j.phytol.2016.10.013
Fill, T.-P., Pereira, G.-K., Geris dos Santos, R.-M., and Rodrigues-Fo, E. (2007). Four additional meroterpenes produced by Penicillium sp. found in association with Melia azedarach. possible biosynthetic intermediates to austin. Z. Naturforsch. B 62, 1035–1044. doi: 10.1515/znb-2007-0806
Geris dos Santos, R.-M., and Rodrigues-Fo, E. (2003). Further meroterpenes produced by Penicillium sp., an endophyte obtained from Melia azedarach. Z. Naturforsch. C: J. Biosci. 58, 663–669. doi: 10.1515/znc-2003-9-1012
Hayashi, H., Manabu, M., Sawao, M., Arai, M., Lee, A.-Y., and Clardy, J. (1994). Acetoxydehydroaustin, a new bioactive compound, and related compound neoaustin from Penicillium sp. MG-11. Biosci. Biotech. Bioch. 58, 334–338. doi: 10.1271/bbb.58.334
Huang, L.-J., Li, X.-A., Jin, M.-Y., Guo, W.-X., Lei, L.-R., Liu, R., et al. (2023). Two previously undescribed phthalides from Talaromyces amestolkiae, a symbiotic fungus of syngnathus acus. J. Asian Nat. Prod. Res. 25, 147–155. doi: 10.1080/10286020.2022.2075738
Kumari, M., Taritla, S., Sharma, A., and Jayabaskaran, C. (2018). Antiproliferative and antioxidative bioactive compounds in extracts of marine-derived endophytic fungus Talaromyces purpureogenus. Front. Microbiol. 9, 1777–1788. doi: 10.3389/fmicb.2018.01777
Lan, D.-H., and Wu, B. (2020). Chemistry and bioactivities of secondary metabolites from the genus Talaromyces. Chem. Biodivers. 17:e2000229. doi: 10.1002/cbdv.202000229
Lei, L.-R., Gong, L.-Q., Jin, M.-Y., Wang, R., Liu, R., Gao, J., et al. (2022). Research advances in the structures and biological activities of secondary metabolites from Talaromyces. Front. Microbiolo. 13:984801. doi: 10.3389/fmicb.2022.984801
Li, Q., Zheng, M.-J., Li, F.-L., Li, Y.-Q., Chen, C.-M., Huang, Z.-J., et al. (2023). Talarolactones A-G, α-pyrone dimers with anti-inflammatory activities from Talaromyces adpressus, and their biosynthetic pathways. Org. Lett. 25, 1616–1621. doi: 10.1021/acs.orglett.2c04352
Liao, H.-X., Shao, T.-M., Mei, R.-Q., Huang, G.-L., Zhou, X.-M., Zheng, C.-J., et al. (2019). Bioactive secondary metabolites from the culture of the mangrove-derived fungus Daldinia eschscholtzii HJ004. Mar. Drugs. 17, 710–718. doi: 10.3390/md17120710
Liu, J., Du, D., Si, Y.-K., Lu, H.-N., Wu, X.-F., Li, Y., et al. (2010). Application of dimolybdenum reagent Mo2(OAc)4 for determination of the absolute configurations of vic-diols. Chin. J. Org. Chem. 30, 1270–1278.
Luo, Z.-W., Tang, M.-M., Zhou, X.-M., Song, X.-M., Yi, J.-L., Zhang, B., et al. (2021). Five new triene derivatives from the fungus Penicillium herquei JX4. Chem. Biodivers. 18:e2100027. doi: 10.1002/cbdv.202100027
Lv, H.-W., Su, H.-B., Xue, Y.-X., Jia, J., Bi, H.-K., Wang, S.-B., et al. (2023). Polyketides with potential bioactivities from the mangrove-derived fungus Talaromyces sp. WHUF0362. Mar. Life Sci. Tech. 5, 232–241. doi: 10.1007/s42995-023-00170-5
Marquez-Fernandez, Q., Trigos, A., Ramos-Balderas, J.-L., Viniegra-Gonzalez, G., Deising, H.-B., and Aguirre, J. (2007). Phosphopantetheinyl transferase CfwA/NpgA is required for Aspergillus nidulans secondary metabolism and asexual development. Eukaryot. Cell 6, 710–720. doi: 10.1128/EC.00362-06
Mosmann, T. (1983). Rapid colorimetric assay for cellular growth and survival: application to proliferation and cytotoxicity assays. J. Immunol. Methods 65, 55–63. doi: 10.1016/0022-1759(83)90303-4
Nicoletti, R., Bellavita, R., and Falanga, A. (2023). The outstanding chemodiversity of marine-derived Talaromyces. Biomolecules 13, 1021–1051. doi: 10.3390/biom13071021
Nicoletti, R., Salvatore, M.-M., and Andolfi, A. (2018). Secondary metabolites of mangrove-associated strains of Talaromyces. Mar. Drugs 16, 12–26. doi: 10.3390/md16010012
Park, J.-S., Quang, T.-H., Yoon, C.-S., Kim, H. J., Sohn, J.-H., and Oh, H. (2018). Furanoaustinol and 7-acetoxydehydroaustinol: new meroterpenoids from a marine-derived fungal strain Penicillium sp. SF-5497. J. Antibiot. 71, 557–563. doi: 10.1038/s41429-018-0034-2
Pierce, C.-G., Uppuluri, P., Teistan, A.-R., Wormley, F.-L.-J., Mowat, E., Ramage, G., et al. (2008). A simple and reproducible 96-well plate-based method for the formation of fungal biofilms and its application to antifungal susceptibility testing. Nat. Protoc. 3, 1494–1500. doi: 10.1038/nprot.2008.141
Prieto, A., De Eugenio, L., Mendez-Liter, J.-A., Nieto-Dominguez, M., Murgiondo, C., Barriuso, J., et al. (2021). Fungal glycosyl hydrolases for sustainable plant biomass valorization: Talaromyces amestolkiae as a model fungus. Int. Microbiol. 24, 545–558. doi: 10.1007/s10123-021-00202-z
Samarasekera, K., Hussein, W.-M., Wu, T.-Z., Salim, A.-A., and Capon, R.-J. (2023). Glyclauxins A-E: dimeric oxaphenalenone aminoglycosides from an australian wasp nest-derived fungus Talaromyces sp. CMB-MW102. J. Nat. Prod. 86, 517–525. doi: 10.1021/acs.jnatprod.2c01069
Trang, D.-T., Hang, D.-T.-T., Dung, D.-T., Cuc, N.-T., Yen, P.-H., Huong, P.-T.-T., et al. (2022). Rhabdastrenones A-D from the sponge Rhabdastrella globostellata. RSC Adv. 12, 10646–10652. doi: 10.1039/D2RA01674E
Wang, J.-T., Ma, Z.-H., Wang, G.-K., Xu, F.-Q., Yu, Y., Wang, G., et al. (2021). Chemical constituents from plant endophytic fungus Alternaria alternate. Nat. Prod. Res. 35, 1199–1206. doi: 10.1080/14786419.2019.1639699
Wang, K., Bao, L., Ma, K., Qi, W., Song, F.-H., Yao, Y.-J., et al. (2016). Bioactive spirobisnaphthalenes and lactones from a cup fungus Plectania sp. collected in the Tibet Platesu Region. Eur. J. Org. Chem. 25, 4338–4346. doi: 10.1002/ejoc.201600562
Wang, Y.-D., Zhang, G.-J., Qu, J., Li, Y.-H., Jiang, J.-D., Liu, Y.-B., et al. (2013). Diterpenoids and sesquiterpenoids from the roots of Illicium majus. J. Nat. Prod. 76, 1976–1983. doi: 10.1021/np400638r
Wang, Z.-H., Li, Z.-L., Niu, C., Yang, L.-P., Zhai, Y.-Y., Li, D.-H., et al. (2023). Aniline-induced production of aniline-containing polyketides and related bicyclic polyketides by the yellow river wetland-derived fungus Talaromyces funiculosus. Front. Microbiol. 14:1200680. doi: 10.3389/fmicb.2023.1200680
Wen, H.-L., Yang, X.-L., Liu, Q., Li, S.-J., Li, Q., Zang, Y., et al. (2019). Structurally diverse meroterpenoids from a marine-derived Aspergillus sp. fungus. J. Nat. Prod. 83, 99–104. doi: 10.1021/acs.jnatprod.9b00878
Xie, F., Li, H.-T., Chen, J.-Y., Duan, H.-J., Xia, D.-D., Sun, Y., et al. (2022). Talarophilone, a ring-opened azaphilone from the endophytic fungus Talaromyces sp. YUD18002. Tetrahedron Lett. 100:153855. doi: 10.1016/j.tetlet.2022.153855
Xue, Y.-X., Wang, L., Zhang, X.-H., and Wang, Z.-L. (2023). Terminal carboxylation of branched carbon chain contributing to acidic stability of azaphilone pigments from a new isolate of Talaromyces amestolkiae. Food Chem. 424:136338. doi: 10.1016/j.foodchem.2023.136338
Yadav, A.-N., Kour, D., Kaur, T., Devi, R., and Yadav, A. (2022). Endophytic fungal communities and their biotechnological implications for agro-environmental sustainability. Folia Microbiol. 67, 203–232. doi: 10.1007/s12223-021-00939-0
Zang, Y., Genta-Jouve, G., Sun, T., Li, X.-W., Didier, B., Mann, S., et al. (2015). Unexpected talaroenamine derivatives and an undescribed polyester from the fungus Talaromyces stipitatus ATCC10500. Phytochemistry 119, 70–75. doi: 10.1016/j.phytochem.2015.09.002
Zeng, W.-N., Cai, J., Wang, B., Chen, L.-Y., Pan, C.-X., Chen, S.-J., et al. (2022). A new bioactive isocoumarin from the mangrove-derived fungus Penicillium sp. TGM112. J. Asian. Nat. Prod. Res. 24, 679–684. doi: 10.1080/10286020.2021.1952188
Zhang, J.-P., Wu, Y.-F., Yuan, B.-C., Liu, D., Zhu, K., Huang, J., et al. (2019). DMOA-based meroterpenoids with diverse scaffolds from the spongeassociated fungus Penicillium brasilianum. Tetrahedron 75, 2193–2205. doi: 10.1016/j.tet.2019.02.037
Zhang, J.-P., Yuan, B.-C., Liu, D., Gao, S., Proksch, P., and Lin, W.-H. (2018). Brasilianoids A-F, new meroterpenoids from the sponge-associated fungus Penicillium brasilianum. Front. Chem. 6, 314–326. doi: 10.3389/fchem.2018.00314
Zhang, K., Zhang, X.-W., Lin, R., Yang, H.-J., Song, F.-H., Xu, X.-L., et al. (2022). New Secondary metabolites from the marine-derived fungus Talaromyces mangshanicus BTBU20211089. Mar. Drugs. 20, 79–89. doi: 10.3390/md20020079
Zhang, M., Li, Q., Li, S.-J., Deng, Y.-F., Yu, M.-Y., Liu, J.-P., et al. (2022). An unprecedented ergostane with a 6/6/5 tricyclic 13(14 → w8)abeo-8,14-seco skeleton from Talaromyces adpressus. Bioorg. Chem. 127:105943. doi: 10.1016/j.bioorg.2022.105943
Keywords: Talaromyces flavus, polyketide, lactones, meroterpenoid, bioactivities
Citation: Cai J, Zhou X, Wang B, Zhang X, Luo M, Huang L, Wang R, Chen Y, Li X, Luo Y, Chen G, Cao F, Huang G and Zheng C (2024) Bioactive polyketides and meroterpenoids from the mangrove-derived fungus Talaromyces flavus TGGP35. Front. Microbiol. 15:1342843. doi: 10.3389/fmicb.2024.1342843
Received: 22 November 2023; Accepted: 08 January 2024;
Published: 01 February 2024.
Edited by:
Andreas Teske, University of North Carolina at Chapel Hill, United StatesReviewed by:
Wei-Guang Wang, Yunnan Minzu University, ChinaXin Li, Chinese Academy of Sciences (CAS), China
Copyright © 2024 Cai, Zhou, Wang, Zhang, Luo, Huang, Wang, Chen, Li, Luo, Chen, Cao, Huang and Zheng. This is an open-access article distributed under the terms of the Creative Commons Attribution License (CC BY). The use, distribution or reproduction in other forums is permitted, provided the original author(s) and the copyright owner(s) are credited and that the original publication in this journal is cited, in accordance with accepted academic practice. No use, distribution or reproduction is permitted which does not comply with these terms.
*Correspondence: Caijuan Zheng, caijuan2002@163.com; Guolei Huang, huangguolei1982@163.com; Fei Cao, caofei542927001@163.com
†These authors have contributed equally to this work