- 1Hubei Key Laboratory of Tumor Microenvironment and Immunotherapy, College of Basic Medical Science, China Three Gorges University, Yichang, China
- 2Institute of Infection and Inflammation, China Three Gorges University, Yichang, China
- 3Department of Physiology and Pathophysiology, College of Basic Medical Science, China Three Gorges University, Yichang, China
- 4Department of Critical Care Medicine, Yiling People’s Hospital of Yichang City, Yichang, China
- 5Department of Interventional Radiology, The First College of Clinical Medical Science, China Three Gorges University, Yichang, China
- 6Yichang Central People’s Hospital, Yichang, China
- 7Hunan Provincial Key Laboratory of Metabolic Bone Diseases, Department of Metabolism and Endocrinology, National Clinical Research Center for Metabolic Diseases, The Second Xiangya Hospital of Central South University, Changsha, China
Background and aim: Nutrient production by intestinal microbiota corresponds to regulate appetite while gut microbial composition was influenced by diet ingestion. However, the causal relationship between gut microbial taxa and anorexia nervosa (AN) remains unclear. Mendelian Randomization (MR) is a novel research method that effectively eliminates the interference of confounding factors and allows for the exploration of the direct causal effects between exposure and outcome. This study employs MR to explore the causal effect between AN and specific gut microbiome.
Methods: Large-scale Genome Wide Association Study (GWAS) data of AN and 211 gut microbes were obtained from the IEU open GWAS project and Mibiogen Consortium. Two-sample MR was performed to determine the causal relationship between gut microbiota and AN. Furthermore, a bi-directional MR analysis was to examine the direction of the causal relations. The Bonferroni correction test was used to adjust potential correlations among microbial taxa.
Result: In forward MR analysis, 10specific gut microbial taxa have an impact on the occurrence of AN (the p value of IVW <0.05). The high abundance of Genus Eubacteriumnodatumgroup ID: 11297 (OR:0.78, 95% CI:0.62–0.98, p = 0.035) and Class Melainabacteria ID: 1589 (OR:0.72, 95% CI:0.51–0.99, p = 0.045) may be considered protective factors for AN. But after Bonferroni correction, only Class Actinobacteria ID:419 (OR:1.53, 95% CI:1.19–1.96, p = 0.00089) remained significantly associated and high abundance of Class Actinobacteria ID:419 considered as a risk factor for AN. In the reverse MR analysis, AN influences 8 gut microbial taxa with none-statistically significant associations after adjustment.
Conclusion: We identified a significant correlation between AN and 18 microbial taxa which have not been previously reported. Among them, 10 kinds of gut bacteria may affect the occurrence of AN, and the status of AN would affect 8 kinds of gut bacteria. After correction, the Class Actinobacteria ID:419 continued to exert an influence on AN.
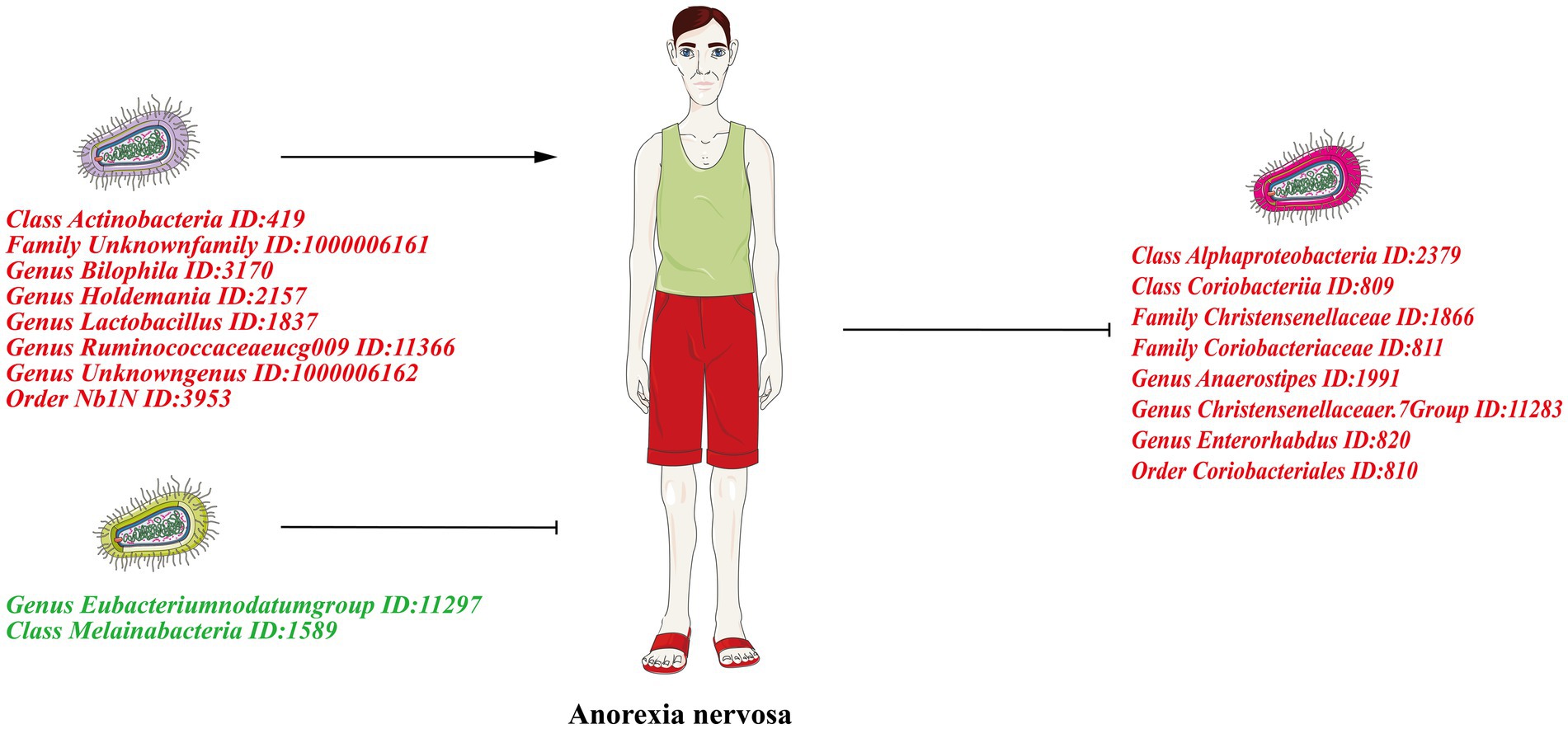
Graphical Abstract. The left half of the figure shows the forward Mendelian randomization results showing the causal effect of gut microbiota on AN. The right half of the figure shows the reverse Mendelian randomization results showing the causal effect of AN on the gut microbiota. Risk factors are indicated in red font and protective factors in dark green font.
1. Introduction
Anorexia nervosa (AN) is one of the most serious psychiatric disorders, characterized by life-threatening very-low body weight, nutritional restrictions, and obviously occurs in females and adolescents (Treasure et al., 2015; Bulik et al., 2019; Seitz et al., 2019). Its prevalence in the population is estimated to be around 1% (Fan et al., 2023). Over the past decade, there has been a progressively increasing trend in the incidence of anorexia nervosa. This rising incidence has been observed in several countries such as the United Kingdom, Germany, and Japan (Holland et al., 2016; Bulik et al., 2019). The aggregate mortality is estimated to approximately 5.6% per decade, significantly surpassing that of the general population (Mitchell et al., 2020). However, AN suffers from efficient therapeutic methods and liability to relapse (Bulik et al., 2019). Previously researches displayed the incidence of AN accompanied by multiple dysfunctions, including endocrine alterations (Schorr and Miller, 2017), increased inflammation (Dai et al., 2013), and immune response (Seitz et al., 2019).
The human gastrointestinal tract harbors a multitude of microorganisms. Previous research has indicated that any disruption in the composition of the gut microbiota can lead to the onset of liver diseases (Tilg et al., 2022). These microorganisms can modulate individual behavior through various mechanisms, including metabolite, endocrine pathways (Albillos et al., 2020). The gut microbiota’s involvement in the development of neurological disorders through the microbiota-gut-brain axis has been reported for some time (Schorr and Miller, 2017; Mossad et al., 2021; Drljaca et al., 2023). However, the specific role played by individual microbial groups in this process remains unclear. Recently, the crucial role of the gut microbiome in AN has been gradually unveiled. Nevertheless, exploring the connection between AN and the gut microbiota poses challenges owing to the susceptibility to various influencing factors. A recent meta-analysis has unveiled links between AN and numerous microbial taxa. However, numerous studies have generated conflicting outcomes regarding the precise relationships between particular microbial taxa and AN (Nikolova et al., 2021). The reasons for these divergent results are multifaceted. Firstly, there’s the influence of confounding factors like environmental elements (such as mental status, sleep status, etc.). Secondly, the intricate interplay among gut microbiota complicates the causal effect between individual microbial groups and AN, making it difficult to reveal cause-and-effect relationship. Currently, there is also a lack of large-scale randomized controlled trials (RCTs) to substantiate this causal link.
Mendelian randomization (MR) is a genetic epidemiological approach that utilizes instrumental variables (IVs) which are highly correlated with the exposure of interest, to investigate causality and mitigate the impact of confounding factors (Emdin et al., 2017; Davies et al., 2018). This method capitalizes on the inherent property of Single Nucleotide Polymorphisms (SNPs) to be randomly assorted and distributed during gamete formation, rendering them unaffected by confounding factors following gametogenesis. Furthermore, the non-reversible nature of heredity helps in excluding the possibility of reverse causal effects (Bowden et al., 2015). In essence, MR, as an innovative methodology, offers the means to address confounding and reverse causality by leveraging genetic variants with close associations to the exposure of interest in order to infer potential causal relationships with the outcome (Emdin et al., 2017; Davies et al., 2018).
In this study, we investigated the potential causal effect between 211 gut microbiota taxa and AN by MR study design. After correction, the Class Actinobacteria ID:419 was found to exert an influence on AN. Our study provides a new sight into exploring the relationship between AN and gut microbiota.
2. Materials and methods
2.1. Study design
Two hundred and eleven gut microbiota taxa were selected as exposure and AN was defined as outcome for MR analysis. Then, the exposure and outcome were exchanged for reverse MR analysis. All MR analysis of this study was executed under three basic assumptions: (1) IVs must be strongly correlated with exposure; (2) IVs cannot be correlated with confounding factors; (3) IVs can only affect outcomes through exposure factors (Bowden and Holmes, 2019). The GWAS data we selected all originate from populations of European ancestry. These individuals are largely independent of each other. The flow-chart of this study was shown in Figure 1. Furthermore, we used the STROBE-MR (Strengthening the Reporting of Observational Studies in Epidemiology-Mendelian Randomization) checklist to explain our MR study (Skrivankova et al., 2021). The checklist shown in Supplementary Table S1.
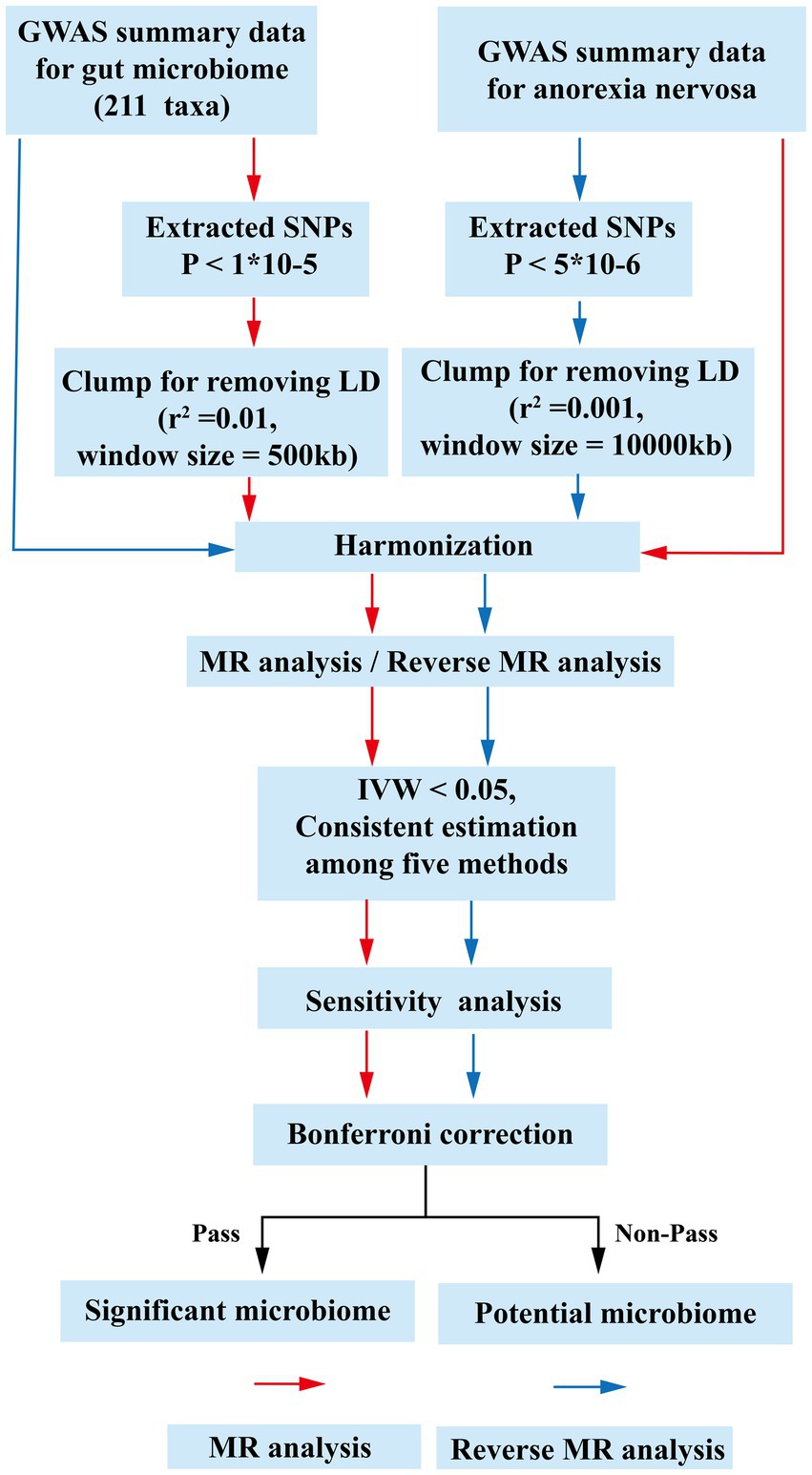
Figure 1. The flow chart of the study. The GWAS data of flora was used as exposure, and anorexia nervosa was used as outcomes for MR analysis. The instrumental variables of flora were extracted in the following way: (1) p < 1 × 10−5; (2) r2 = 0.01, kb = 500. Five methods were used for MR analysis after harmonization. Floras with IVW estimates below 0.05, exhibiting consistent results across the five analytical methods, were deemed to hold potential association. Subsequently, an investigation into the presence of pleiotropy and heterogeneity among these potential floras was undertaken. Those floras displaying pleiotropic or heterogeneous characteristics were promptly eliminated. Meaningful bacterial groups were screened out. Then reverse MR analysis was performed, and different criteria were used for the screening of instrumental variables for AN (p < 5 × 10−6, r2 = 0.001, kb = 10,000). All potential floras were corrected by the Bonferronitest. The floras passed the Bonferroni were considered as significant. MR, Mendelian randomization; LD, linkage disequilibrium; GWAS, Genome Wide Association Study; SNPs, single nucleotide polymorphisms; IVW, inverse variance weighting.
2.2. GWAS data of gut microbiome
The large-scale GWAS summary data of gut microbiome were obtained from Mibiogen consortium, including 18,340 individuals from 24 cohorts (Kurilshikov et al., 2021). The consortium utilized standardized analytical pipelines for both microbiota phenotype and genotype, ensuring uniform data processing methods. This approach was employed to mitigate potential variations introduced by technical differences in generating microbiota data. This study used three different regions (V4: 10,413 samples, 13 cohorts, V3-V4: 4,211 samples, 6 cohorts and V1-V2: 3,716 samples, 5 cohorts) (V: hypervariable region sequencing for identifying bacterial taxa)of the 16S rRNA gene to analysis the composition of gut microbiota and identified genetic variants that influent the relative abundance of microbial taxa by use of microbiota Quantitative Trait loci (mbQTL) mapping (Kurilshikov et al., 2021). In the original study, the gut microbiota was categorized into 257 taxa at five taxonomic levels: Phylum, Class, Order, Family, and Genus. Finally, 211 taxa were defined, including 131 genera, 35 families, 20 orders, 16 classes, and 9 phyla. All data from this study are publicly accessible, which could acquire from the website (https://mibiogen.gcc.rug.nl/menu/main/home/).
2.3. GWAS data of AN
The GWAS summary data of AN were obtained from the IEU open GWAS project (v7.5.5-2023-08-09, N = 42,348, https://gwas.mrcieu.ac.uk/). The GWAS summary dataset encompassed 2,907 individuals diagnosed with AN across 14 countries, along with 14,860 ancestrally matched control subjects, constituting a part of the Genetic Consortium for Anorexia Nervosa (GCAN) and the Wellcome Trust Case Control Consortium 3 (WTCCC3) (Boraska et al., 2014). All individuals were of European ancestry and detailed information of ANwas available in the website (https://gwas.mrcieu.ac.uk/datasets/ieu-a-45/).
2.4. The selection of IVs
The criterion of selecting IVs as following: (1) SNPs, significantly associated with gut microbiota, were selected (the p value of SNPs <1 × 10−5) as the potential eligible IVs (Yu et al., 2023); (2) SNPs were clumped for excluding the effect of linkage disequilibrium (r2 = 0.01, window size = 500 kb) (Xu et al., 2021); (3)palindromic alleles were removed. Then, For the reverse MR analysis, we filtered the IVs of AN. p ≤ 5 × 10−6was chose as the criterion and clump were reset (r2 = 0.001, window size = 10,000 kb) (Yu et al., 2023). Other criterions were the same as above. To avoid weak instrumental bias, the F statistics for each bacterial taxon was calculated by following equation (Xu et al., 2021):
In this equation, R2 is to explain exposure variance of the IVs, n is the sample size, and k is the number of IVs (Xu et al., 2021). According to previous study, F statistic ≥10 were considered that there is no weak instrument bias (Pierce et al., 2011).
2.5. MR analysis
Five methods (including Inverse variance weighted (IVW), MR Egger, Weighted median, Simple mode, and Weighted mode method) were used to estimate the causal effect of the gut microbiota on AN. Due to its assumption that all instrumental variables are valid, IVW is susceptible to the effects of instrumental variable pleiotropy and heterogeneity (Bowden et al., 2016). However, in the absence of these influences, IVW considered to be the most accurate method, even when the other four methods may not yield positive results (Long et al., 2023).
To establish the accuracy and seriousness, five distinct methods was employed to assess the causal impact of gut microbiota on AN, namely: (1) Inverse Variance Weighted (IVW); (2) MR Egger; (3) Weighted Median (WM); (4) Simple Mode; (5) Weighted Mode method. It’s worth noting that, while the IVW method assumes the validity of all instrumental variables, it can be influenced by instrumental variable pleiotropy and heterogeneity. However, in scenarios devoid of these influences, IVW remains esteemed as the most precise technique (Wang et al., 2023). Thus, the result of MR analysis was mainly based on IVW (Burgess et al., 2013). The remaining four methods were considered supplementary to the IVW approach. Once instrumental variables (IVs) were harmonized, if the count of matched SNPs for a specific microbiota was <3, that microbiota was excluded due to lack of credibility in results.
A significance threshold for multiple testing was established at each taxonomic level (phylum, class, order, family, and genus). Bonferroni correction method was employed to adjust the p-values, mitigating the potential for false positives (p < 0.05/N, N refers to the effective number of independent bacterial taxa at the specific taxonomic level). The significant p values were following: 0.00038 (131 Genera), 0.0014 (35 Families), 0.0025 (20 Orders), 0.0031 (16 Classes), and 0.0056 (9 Phyla). Subsequently, when the estimated value of five methods exhibited congruence and IVW yielded a value <0.05/N, the gut microbes were deemed to exhibit a statistically significant distinction. Microbial taxa with the p-values of IVW below 0.05, although not statistically significant after Bonferroni correction, are considered to have potential associations.
The significant microbiotas were tested for pleiotropy and heterogeneity to ensure the accuracy of the IVW results. MR-Egger Intercept Test and Mendelian Randomization Pleiotropy RESidual Sum and Outlier (MR-PRESSO) global test were employed to detect horizontal pleiotropy (Long et al., 2023). MR-PRESSO could assess the overall horizontal pleiotropy of IVs and the abnormal SNPs which led to the pleiotropy (Verbanck et al., 2018). For assessing the presence of horizontal pleiotropy, p values greater than 0.05 for two methods were indicative of its absence. To gage the extent of heterogeneity, Cochran’s Q test was employed, with p values above 0.05 indicating non-heterogeneity. Therefore, in our study, any microbiota with Cochran’s Q test p-value remaining less than 0.05 were excluded from further analysis to ensure the reliable results of IVW method. Meanwhile, Leave-one-out analysis was employed to exclude the influence of single SNP.
2.6. Reverse MR analysis
To investigate whether AN exerted acausal influence on gut microbiomes, we additionally conducted a reverse MR analysis. In this procure, we employed SNPs strongly linked with AN as instrumental variables (IVs) to further probe the causal effect of AN on gut microbiome. The analytical approach was same with the MR analysis.
2.7. Data processing
All data processing and analysis were accomplished by R software (R.4.2.3; http://www.R-project.org). The R packages used in study is TwoSampleMR, MendelianRandomization, and MR-PRESSO.
3. Results
3.1. Instrumental variables for gut microbiome and AN
After strong correlation screening (p < 1 × 10−5) and clump (r2 = 0.01, window = 500 kb). SNPs associated with gut microbiota at difference levels were identified (Class: 231, Family: 509, Genus: 1740, Order: 289, Phylum: 126; Detail information shown in Supplementary Table S2). For AN, a threshold of 5 × 10−6 (r2 = 0.001, window = 10,000 kb) adopted for selection. Finally, 7 IVs were filtered and detail information was displayed in Supplementary Table S3.
3.2. Causal effects of gut microbiota on AN
Under the condition of IVW < 0.05, 10 gut microbiotas were associated with AN (Figures 2, 3A). The result showed that high abundancy of 8 microbiotas were the risk factors for the onset of AN, including Class Actinobacteria ID:419 (OR:1.53, 95% CI:1.19–1.96, p = 0.00089), Family Unknown family ID:1000006161 (OR:1.34, 95% CI:1.08–1.67, p = 0.0077), Genus Bilophila ID:3170 (OR:1.73, 95% CI:1.03–2.90, p = 0.039), Genus Holdemania ID:2157 (OR:1.36, 95% CI:1.02–1.83, p = 0.038), Genus Lactobacillus ID:1837 (OR:1.48, 95% CI:1.07–2.04, p = 0.018), Genus Ruminococcaceaeucg009 ID:11366 (OR:1.55, 95% CI:1.16–2.07, p = 0.0029), Genus Unknowngenus ID:1000006162 (OR:1.34, 95% CI:1.08–1.67, p = 0.0077), Order Nb1N ID:3953 (OR:1.34, 95% CI:1.08–1.67, p = 0.0077). Two microbiotas were the protective factors for AN, including Genus EubacteriumnodatumgroupID:11297(OR:0.78, 95%CI:0.62–0.98, p = 0.035), Class Melainabacteria ID:1589 (OR:0.72, 95% CI:0.51–0.99, p = 0.045). After Bonferroni correction, only one microbiota was significance associated with AN (Class Actinobacteria ID:419).
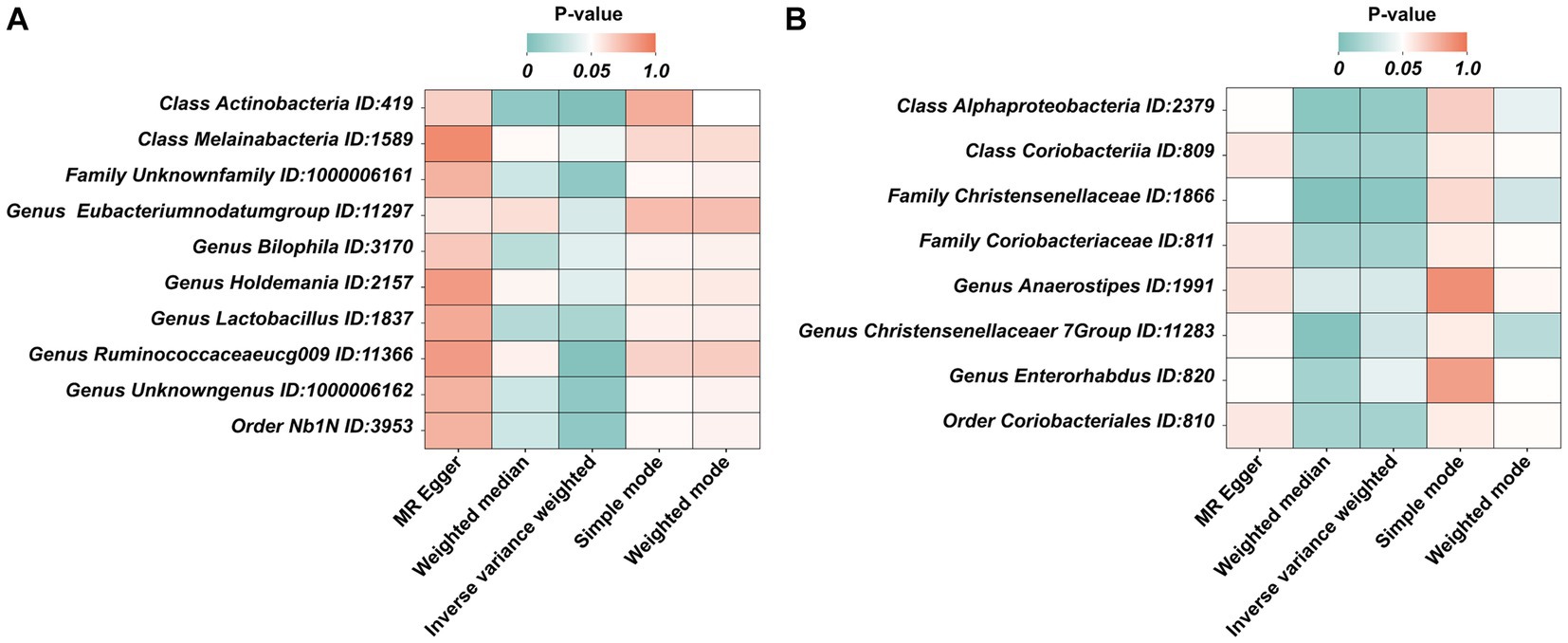
Figure 3. The heatmaps of five MR analysis methods. (A) The p value of five MR analysis (Forward); (B) The p value of five MR analysis (Reverse).
3.3. Causal effects of AN on gut microbiota
In the reverse MR, the abundancy of eight gut microbial taxa were identified that are influenced by AN (Figures 3B, 4), including Class Alphaproteobacteria ID:2379 (OR:1.02, 95% CI:1.00–1.03, p = 0.0092), ClassCoriobacteriia ID:809 (OR:1.01, 95% CI:1.00–1.02, p = 0.016), Family Christensenellaceae ID:1866 (OR:1.02, 95% CI:1.00–1.03, p = 0.0056), Family Coriobacteriaceae ID:811 (OR:1.01, 95% CI:1.00–1.02, p = 0.016), GenusAnaerostipesID:1991 (OR:1.01, 95% CI:1.00–1.02, p = 0.035), Genus Christensenellaceaer.7Group ID:11283 (OR:1.02, 95% CI:1.00–1.03, p = 0.032), Genus Enterorhabdus ID:820 (OR:1.02, 95% CI:1.00–1.04, p = 0.041), and Order Coriobacteriales ID:810 (OR:1.01, 95% CI:1.00–1.02, p = 0.016). Under conditions of AN, these microbial taxa are suppressed and would display low abundancy. In essence, AN acted as a potential risk factor for these microbial communities. However, there was no significant microbiota after Bonferroni correction.
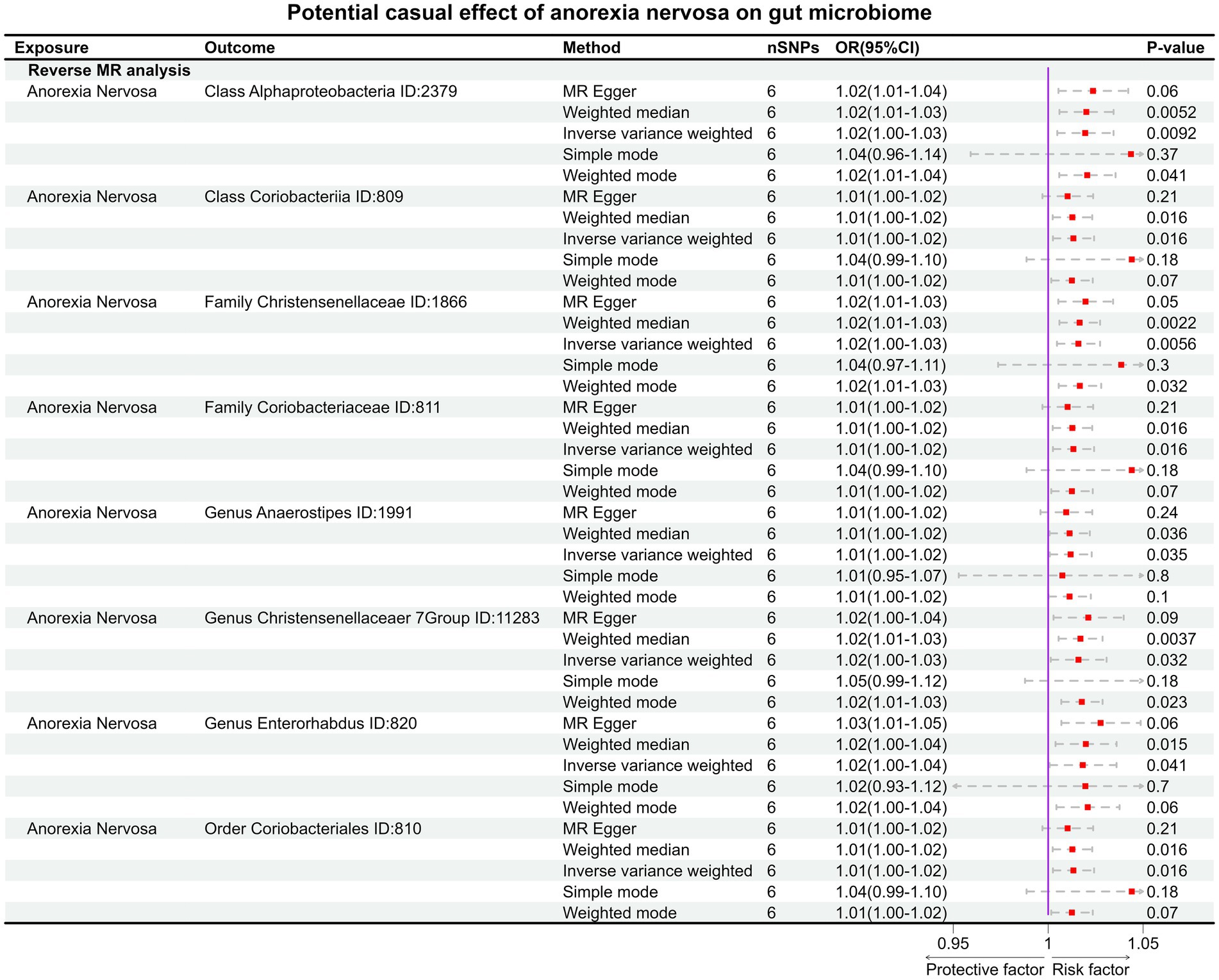
Figure 4. The result of reverse MR analysis between gut microbiome and anorexia nervosa by five methods.
3.4. Sensitivity analysis of MR
MR PRESSO did not identify heterogeneity among the significant and potential microbiota (Tables 1, 2). Similarly, Cochran’s Q test indicated the absence of heterogeneity across the studies (Tables 1, 2). Moreover, the Leave-one-out analysis revealed no significant differences in SNP effects. Furthermore, the MR Egger regression did not yield any evidence of horizontal pleiotropy (p > 0.05), and all F-statistical values exceeded 10 (Tables 1, 2). The MR analysis and Leave-one out analysis result of Class Actinobacteria ID:419 was shown in the Figure 5.
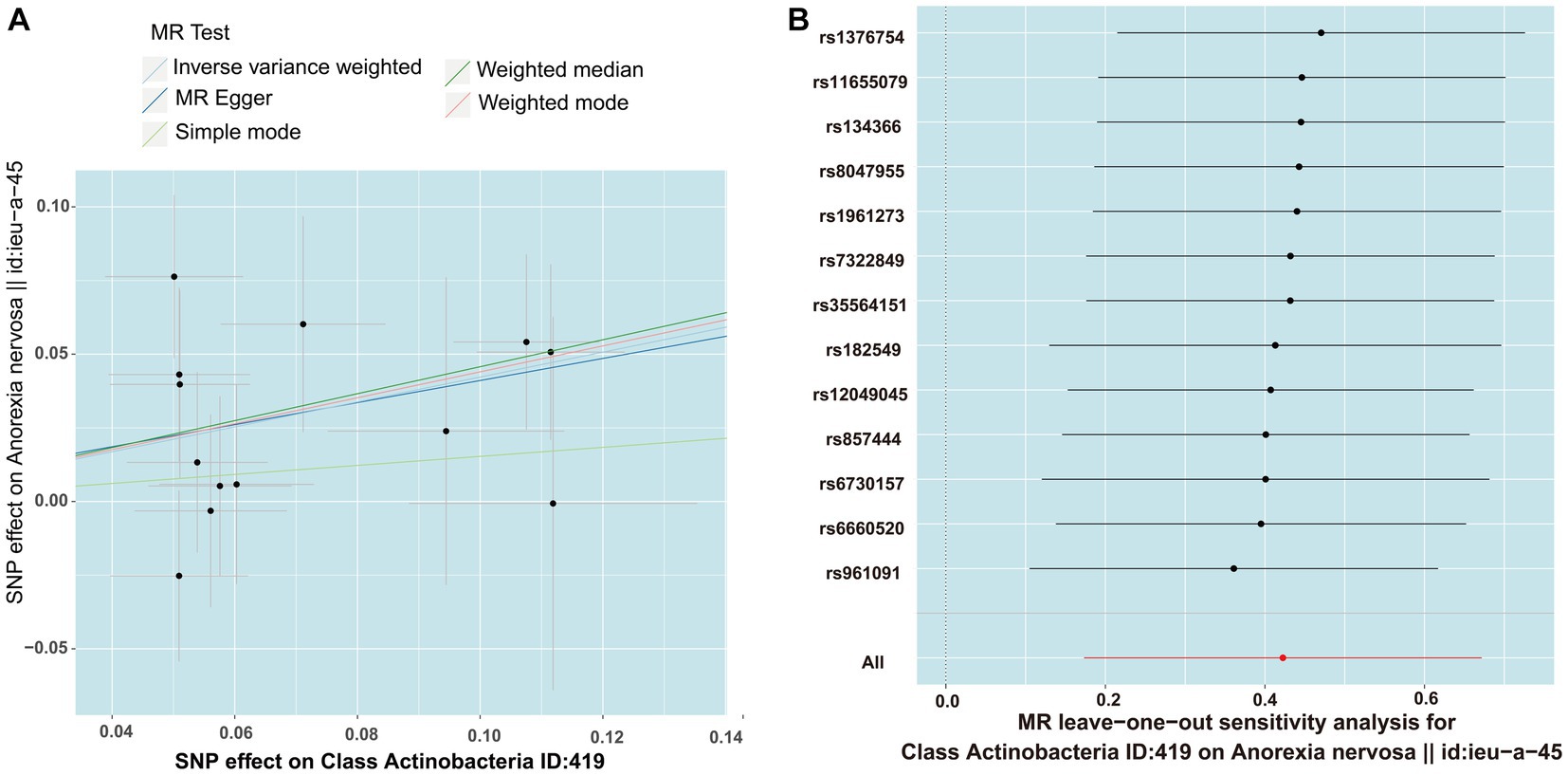
Figure 5. The result of MR between gut microbiome and anorexia nervosa by five methods. (A) In the scatter plot,estimated value of five MR tests were consistent. (B) The leave-one-out analysis validated that there is no significant differences in SNP effects between Class Actinobacteria ID:419 and anorexia nervosa.
4. Discussion
As early as the 20th century, it was discovered that probiotics could improve human mental well-being, such as fatigue, melancholia and the neurosis (Bested et al., 2013). The composition of gut microbiota in individuals with mental illnesses significantly differs from that of healthy individuals. Preclinical experiments have demonstrated that transplanting fecal material from individuals with mental disorders into the intestines of germ-free mice can markedly alter the behavior in mice (Kelly et al., 2016; Zheng et al., 2016; Li et al., 2019; Sharon et al., 2019; Zhu et al., 2020; Fan et al., 2023). Furthermore, previous researches had already demonstrated the role of gut microbiota in regulating appetite (Breton et al., 2016; Fetissov, 2017; Han et al., 2021). This process may depend on tryptophan (Dong et al., 2020). Thus, some researchers are also attempting to treat anorexia nervosa by inhibiting healthy individuals’ feces (Wilson et al., 2023). Furthermore, a recent meta-analysis also indicates variations in the microbiota of individuals with mental disorders (Nikolova et al., 2021). This study incorporated 59 case-control studies, including 10 related to AN (involving 211 patients). It revealed alterations in 12 microbial taxa among AN, though the results pertaining to these taxa were not consistent across all studies (Nikolova et al., 2021). Current research faces challenges in obtaining consistent conclusions by experimental design that can eliminate the influence of confounding factors. The MR method ingeniously mitigates the impact of confounding factors, thereby yielding consistent results (Emdin et al., 2017).
To our knowledge, this is the first MR study to ascertain the relationship between AN and gut microbiome. We found that there was genetic liability to 18 gut microbiotas causally associated with AN. Our study also found some results were consistent with previously researches. For instance, a high abundance of Genus Lactobacillus, in our study, is a risk factor for AN. Interestingly, a recent study also observed that the abundance of several Lactobacillus species was increased in the Activity-Based Anorexia mouse model (Breton et al., 2021). In another study, an increased abundance of Class Actinobacteria was observed in patients of AN (Nikolova et al., 2021). Interestingly, a similar increase in Class Actinobacteria abundance was also found in a clinical trial involving obese patients, coinciding with weight reduction in overweight individuals (Chambers et al., 2019).
However, our study also deviated from several previous research findings. Another study highlighted variations in the composition of fecal microbiota between AN patients upon admission and at discharge, specifically involving Genus Anaerostipes and Genus Christensenellaceaer.7Group. In their study, the abundance of these two taxa decreased from admission to discharge. Our study, in contrast, revealed that the growth of Genus Anaerostipes and Genus Christensenellaceaer.7Group was likely inhibited under AN condition. We hypothesized that this increase might stem from complex interactions within the microbial communities. In addition, Family Coriobacteriaceae ID:811was reported increasing in the patients of AN. However, AN, in our study, acted as an inhibitory factor for the growth of Family Coriobacteriaceae ID:811. Family Coriobacteriaceae ID:811, should be decease under the condition of the AN. It’s worth noting that our study also provided a clear conclusion for the conflicting results from prior research. For instance, a meta-analysis published in 2020 indicated a potential significant association between Anaerostipes and BMI (Di Lodovico et al., 2021). However, with an increase in studies, a meta-analysis in 2021 suggested inconsistent findings (Nikolova et al., 2021). In our study, we observed that Genus Anaerostipes ID:1991 exhibited suppressed growth under condition of AN. One possible explanation for this difference could be that, while MR analysis eliminates the interference of various confounding factors, the inherent differences in the physical properties of the selected microbial samples themselves cannot be ignored. These differences may have led to variations in our study’s conclusions compared to previous findings. Standardized sample selection and large-scale randomized controlled trials can help eliminate the potential interference caused by these differences.
Meanwhile, our study has several limitations. Firstly, constrained by GWAS data, we can accurately examine the relationship between the genus level or higher taxonomic levels and AN, but cannot extend the validation to more specific levels, such as species. Secondly, due to the utilization of publicly available GWAS data, there was an imbalance in the number of case and control groups within these cohorts, potentially increasing the likelihood of pleiotropy. Concurrently, the impact of genetic mutations on other pathways might also impact the interpretability of the results. Thirdly, the GWAS data we utilized are derived from individuals of European ancestry. Therefore, it remains uncertain whether our conclusions are applicable to other populations, such as East Asian or South Asian populations. Fourthly, since we were unable to obtain individual-level gender data from the GWAS data, we were unable to analyze AN patients of different genders and, therefore, explore the interaction between microbial taxa and AN patients of different genders. Lastly, the absence of pertinent GWAS data precluded us from conducting validation through replication samples.
In summary, our analysis delved into the causal connection between 211 gut microbiota and AN. For the first time, we have not only showed that 10 gut microbiotas acted as risk or protective factors for AN, but also revealed that 8 gut microbiotas were influenced under AN condition. This study offered novel insights into the causal relationship between AN and gut microbial taxa.
Data availability statement
The raw data supporting the conclusions of this article will be made available by the authors, without undue reservation.
Author contributions
XX: Data curation, Formal analysis, Funding acquisition, Investigation, Writing – original draft. S-yH: Data curation, Formal analysis, Writing – original draft. X-LZ: Methodology, Software, Supervision, Writing – review & editing. DW: Funding acquisition, Methodology, Software, Supervision, Validation, Writing – review & editing. QH: Funding acquisition, Software, Validation, Writing – review & editing. Q-AX: Conceptualization, Data curation, Formal analysis, Investigation, Methodology, Resources, Writing – original draft. YY: Conceptualization, Funding acquisition, Project administration, Supervision, Validation, Visualization, Writing – review & editing.
Funding
The author(s) declare financial support was received for the research, authorship, and/or publication of this article. This work was supported by Open Foundation of Hubei Key Laboratory of Tumor Microenvironment and Immunotherapy, China Three Gorges University (2023KZL012), National Natural Science Foundation of China (No. 31772709, 82100473), and Hubei Natural Science Foundation (2015CFB316) of China.
Acknowledgments
We acknowledge the participants and investigators of the IEU open GWAS project. We also thank the Mibiogen consortium. We thank for the help of Servier Medical Art. Parts of picture material in graphical abstract were drawn by using pictures from Servier Medical Art and we do some modified in microbes. Servier Medical Art by Servier is licensed under a Creative Commons Attribution 3.0 Unported License (https://creativecommons.org/license-s/by/3.0/). We thank for https://www.chiplot.online/ which helps us to draw the heatmap.
Conflict of interest
The authors declare that the research was conducted in the absence of any commercial or financial relationships that could be construed as a potential conflict of interest.
Publisher’s note
All claims expressed in this article are solely those of the authors and do not necessarily represent those of their affiliated organizations, or those of the publisher, the editors and the reviewers. Any product that may be evaluated in this article, or claim that may be made by its manufacturer, is not guaranteed or endorsed by the publisher.
Supplementary material
The Supplementary material for this article can be found online at: https://www.frontiersin.org/articles/10.3389/fmicb.2023.1290246/full#supplementary-material
Abbreviations
AN, Anorexia nervosa; CI, Confidence interval; GCAN, Genetic consortium for anorexia nervosa; GWAS, Genome-wide association study; IV, Instrumental variables; IVW, Inverse variance weighted; LD, Linkage disequilibrium; MR, Mendelian randomization; MR-PRESSO, Mendelian randomization pleiotropy RESidual sum and outlier; mbQTL, Microbiota quantitative trait loci; OR, Odds ratio; RCT, Randomized control trial; SNPs, Single nucleotide polymorphisms; WM, Weighted median; WTCCC3, Wellcome trust case control consortium 3.
References
Albillos, A., de Gottardi, A., and Rescigno, M. (2020). The gut-liver axis in liver disease: pathophysiological basis for therapy. J. Hepatol. 72, 558–577. doi: 10.1016/j.jhep.2019.10.003
Bested, A. C., Logan, A. C., and Selhub, E. M. (2013). Intestinal microbiota, probiotics and mental health: from Metchnikoff to modern advances: part I - autointoxication revisited. Gut Pathog. 5:5. doi: 10.1186/1757-4749-5-5
Boraska, V., Franklin, C. S., Floyd, J. A., Thornton, L. M., Huckins, L. M., Southam, L., et al. (2014). A genome-wide association study of anorexia nervosa. Mol. Psychiatry 19, 1085–1094. doi: 10.1038/mp.2013.187
Bowden, J., Davey Smith, G., and Burgess, S. (2015). Mendelian randomization with invalid instruments: effect estimation and bias detection through Egger regression. Int. J. Epidemiol. 44, 512–525. doi: 10.1093/ije/dyv080
Bowden, J., Davey Smith, G., Haycock, P. C., and Burgess, S. (2016). Consistent estimation in Mendelian randomization with some invalid instruments using a weighted median estimator. Genet. Epidemiol. 40, 304–314. doi: 10.1002/gepi.21965
Bowden, J., and Holmes, M. V. (2019). Meta-analysis and Mendelian randomization: a review. Res. Synth. Methods 10, 486–496. doi: 10.1002/jrsm.1346
Breton, J., Tennoune, N., Lucas, N., Francois, M., Legrand, R., Jacquemot, J., et al. (2016). Gut Commensal E. coli proteins activate host satiety pathways following nutrient-induced bacterial growth. Cell Metab. 23, 324–334. doi: 10.1016/j.cmet.2015.10.017
Breton, J., Tirelle, P., Hasanat, S., Pernot, A., L'Huillier, C., Do Rego, J. C., et al. (2021). Gut microbiota alteration in a mouse model of anorexia nervosa. Clin. Nutr. 40, 181–189. doi: 10.1016/j.clnu.2020.05.002
Bulik, C. M., Flatt, R., Abbaspour, A., and Carroll, I. (2019). Reconceptualizing anorexia nervosa. Psychiatry Clin. Neurosci. 73, 518–525. doi: 10.1111/pcn.12857
Burgess, S., Butterworth, A., and Thompson, S. G. (2013). Mendelian randomization analysis with multiple genetic variants using summarized data. Genet. Epidemiol. 37, 658–665. doi: 10.1002/gepi.21758
Chambers, E. S., Byrne, C. S., Morrison, D. J., Murphy, K. G., Preston, T., Tedford, C., et al. (2019). Dietary supplementation with inulin-propionate ester or inulin improves insulin sensitivity in adults with overweight and obesity with distinct effects on the gut microbiota, plasma metabolome and systemic inflammatory responses: a randomised cross-over trial. Gut 68, 1430–1438. doi: 10.1136/gutjnl-2019-318424
Dai, C., Zheng, C. Q., Meng, F. J., Zhou, Z., Sang, L. X., and Jiang, M. (2013). VSL#3 probiotics exerts the anti-inflammatory activity via PI3k/Akt and NF-kappaB pathway in rat model of DSS-induced colitis. Mol. Cell. Biochem. 374, 1–11. doi: 10.1007/s11010-012-1488-3
Davies, N. M., Holmes, M. V., and Davey Smith, G. (2018). Reading Mendelian randomisation studies: a guide, glossary, and checklist for clinicians. BMJ 362:k601. doi: 10.1136/bmj.k601
Di Lodovico, L., Mondot, S., Dore, J., Mack, I., Hanachi, M., and Gorwood, P. (2021). Anorexia nervosa and gut microbiota: a systematic review and quantitative synthesis of pooled microbiological data. Prog. Neuro-Psychopharmacol. Biol. Psychiatry 106:110114. doi: 10.1016/j.pnpbp.2020.110114
Dong, F., Hao, F., Murray, I. A., Smith, P. B., Koo, I., Tindall, A. M., et al. (2020). Intestinal microbiota-derived tryptophan metabolites are predictive of ah receptor activity. Gut Microbes 12, 1788899–1788824. doi: 10.1080/19490976.2020.1788899
Drljaca, J., Milosevic, N., Milanovic, M., Abenavoli, L., and Milic, N. (2023). When the microbiome helps the brain-current evidence. CNS Neurosci. Ther. 29, 43–58. doi: 10.1111/cns.14076
Emdin, C. A., Khera, A. V., and Kathiresan, S. (2017). Mendelian Randomization. JAMA 318, 1925–1926. doi: 10.1001/jama.2017.17219
Fan, Y., Støving, R. K., Berreira Ibraim, S., Hyötyläinen, T., Thirion, F., Arora, T., et al. (2023). The gut microbiota contributes to the pathogenesis of anorexia nervosa in humans and mice. Nat. Microbiol. 8, 787–802. doi: 10.1038/s41564-023-01355-5
Fetissov, S. O. (2017). Role of the gut microbiota in host appetite control: bacterial growth to animal feeding behaviour. Nat. Rev. Endocrinol. 13, 11–25. doi: 10.1038/nrendo.2016.150
Han, H., Yi, B., Zhong, R., Wang, M., Zhang, S., Ma, J., et al. (2021). From gut microbiota to host appetite: gut microbiota-derived metabolites as key regulators. Microbiome 9:162. doi: 10.1186/s40168-021-01093-y
Holland, J., Hall, N., Yeates, D. G., and Goldacre, M. (2016). Trends in hospital admission rates for anorexia nervosa in Oxford (1968-2011) and England (1990-2011): database studies. J. R. Soc. Med. 109, 59–66. doi: 10.1177/0141076815617651
Kelly, J. R., Borre, Y., C, O. B., Patterson, E., El Aidy, S., Deane, J., et al. (2016). Transferring the blues: depression-associated gut microbiota induces neurobehavioural changes in the rat. J. Psychiatr. Res. 82, 109–118. doi: 10.1016/j.jpsychires.2016.07.019
Kurilshikov, A., Medina-Gomez, C., Bacigalupe, R., Radjabzadeh, D., Wang, J., Demirkan, A., et al. (2021). Large-scale association analyses identify host factors influencing human gut microbiome composition. Nat. Genet. 53, 156–165. doi: 10.1038/s41588-020-00763-1
Li, N., Wang, Q., Wang, Y., Sun, A., Lin, Y., Jin, Y., et al. (2019). Fecal microbiota transplantation from chronic unpredictable mild stress mice donors affects anxiety-like and depression-like behavior in recipient mice via the gut microbiota-inflammation-brain axis. Stress 22, 592–602. doi: 10.1080/10253890.2019.1617267
Long, Y., Tang, L., Zhou, Y., Zhao, S., and Zhu, H. (2023). Causal relationship between gut microbiota and cancers: a two-sample Mendelian randomisation study. BMC Med. 21:66. doi: 10.1186/s12916-023-02761-6
Mitchell, J. E., Solomon, C. G., and Peterson, C. B. (2020). Anorexia Nervosa. N. Engl. J. Med. 382, 1343–1351. doi: 10.1056/NEJMcp1803175
Mossad, O., Nent, E., Woltemate, S., Folschweiller, S., Buescher, J. M., Schnepf, D., et al. (2021). Microbiota-dependent increase in delta-valerobetaine alters neuronal function and is responsible for age-related cognitive decline. Nat. Aging 1, 1127–1136. doi: 10.1038/s43587-021-00141-4
Nikolova, V. L., Smith, M. R. B., Hall, L. J., Cleare, A. J., Stone, J. M., and Young, A. H. (2021). Perturbations in gut microbiota composition in psychiatric disorders: a review and Meta-analysis. JAMA Psychiatry 78, 1343–1354. doi: 10.1001/jamapsychiatry.2021.2573
Pierce, B. L., Ahsan, H., and Vanderweele, T. J. (2011). Power and instrument strength requirements for Mendelian randomization studies using multiple genetic variants. Int. J. Epidemiol. 40, 740–752. doi: 10.1093/ije/dyq151
Schorr, M., and Miller, K. K. (2017). The endocrine manifestations of anorexia nervosa: mechanisms and management. Nat. Rev. Endocrinol. 13, 174–186. doi: 10.1038/nrendo.2016.175
Seitz, J., Belheouane, M., Schulz, N., Dempfle, A., Baines, J. F., and Herpertz-Dahlmann, B. (2019). The impact of starvation on the microbiome and gut-brain interaction in anorexia nervosa. Front. Endocrinol. (Lausanne) 10:41. doi: 10.3389/fendo.2019.00041
Sharon, G., Cruz, N. J., Kang, D. W., Gandal, M. J., Wang, B., Kim, Y. M., et al. (2019). Human gut microbiota from autism Spectrum disorder promote behavioral symptoms in mice. Cells 177:e1617, 1600–1618.e17. doi: 10.1016/j.cell.2019.05.004
Skrivankova, V. W., Richmond, R. C., Woolf, B. A. R., Yarmolinsky, J., Davies, N. M., Swanson, S. A., et al. (2021). Strengthening the reporting of observational studies in epidemiology using Mendelian randomization: the STROBE-MR statement. JAMA 326, 1614–1621. doi: 10.1001/jama.2021.18236
Tilg, H., Adolph, T. E., and Trauner, M. (2022). Gut-liver axis: pathophysiological concepts and clinical implications. Cell Metab. 34, 1700–1718. doi: 10.1016/j.cmet.2022.09.017
Treasure, J., Zipfel, S., Micali, N., Wade, T., Stice, E., Claudino, A., et al. (2015). Anorexia nervosa. Nat. Rev. Dis. Primers. 1:15074. doi: 10.1038/nrdp.2015.74
Verbanck, M., Chen, C. Y., Neale, B., and Do, R. (2018). Detection of widespread horizontal pleiotropy in causal relationships inferred from Mendelian randomization between complex traits and diseases. Nat. Genet. 50, 693–698. doi: 10.1038/s41588-018-0099-7
Wang, S., Zhu, H., Pan, L., Zhang, M., Wan, X., Xu, H., et al. (2023). Systemic inflammatory regulators and risk of acute-on-chronic liver failure: a bidirectional mendelian-randomization study. Front. Cell Dev. Biol. 11:1125233. doi: 10.3389/fcell.2023.1125233
Wilson, B. C., Derraik, J. G. B., Albert, B. B., Leong, K. S. W., Tweedie-Cullen, R. Y., Creagh, C., et al. (2023). An open-label pilot trial of faecal microbiome transfer to restore the gut microbiome in anorexia nervosa: protocol. BMJ Open 13:e070616. doi: 10.1136/bmjopen-2022-070616
Xu, Q., Ni, J. J., Han, B. X., Yan, S. S., Wei, X. T., Feng, G. J., et al. (2021). Causal relationship between gut microbiota and autoimmune diseases: a two-sample Mendelian randomization study. Front. Immunol. 12:746998. doi: 10.3389/fimmu.2021.746998
Yu, H., Wan, X., Yang, M., Xie, J., Xu, K., Wang, J., et al. (2023). A large-scale causal analysis of gut microbiota and delirium: a Mendelian randomization study. J. Affect. Disord. 329, 64–71. doi: 10.1016/j.jad.2023.02.078
Zheng, P., Zeng, B., Zhou, C., Liu, M., Fang, Z., Xu, X., et al. (2016). Gut microbiome remodeling induces depressive-like behaviors through a pathway mediated by the host's metabolism. Mol. Psychiatry 21, 786–796. doi: 10.1038/mp.2016.44
Keywords: gut microbiome, Mendelian randomization, anorexia nervosa, genome-wide association study, causality
Citation: Xia X, He S-y, Zhang X-L, Wang D, He Q, Xiao Q-A and Yang Y (2023) The causality between gut microbiome and anorexia nervosa: a Mendelian randomization analysis. Front. Microbiol. 14:1290246. doi: 10.3389/fmicb.2023.1290246
Edited by:
Ludovico Abenavoli, Magna Græcia University, ItalyReviewed by:
Bruno Tilocca, Magna Græcia University, ItalyGiuseppe Guido Maria Scarlata, University Magna Graecia of Catanzaro, Italy
Copyright © 2023 Xia, He, Zhang, Wang, He, Xiao and Yang. This is an open-access article distributed under the terms of the Creative Commons Attribution License (CC BY). The use, distribution or reproduction in other forums is permitted, provided the original author(s) and the copyright owner(s) are credited and that the original publication in this journal is cited, in accordance with accepted academic practice. No use, distribution or reproduction is permitted which does not comply with these terms.
*Correspondence: Qing-Ao Xiao, xiaoqingao0912@163.com; Yong Yang, yangyongVIP1971@163.com
†These authors have contributed equally to this work
‡ORCID: Xuan Xia, https://orcid.org/0000-0002-4788-1971
Shu-Yang He, https://orcid.org/0009-0007-2594-5472
Decheng Wang, https://orcid.org/0000-0002-1742-7460
Qian He, https://orcid.org/0000-0001-7824-2526
Qing-Ao Xiao, https://orcid.org/0000-0002-7853-7794
Yong Yang, https://orcid.org/0009-0009-3209-6637