- Key Laboratory of Agricultural Microbiomics and Precision Application (MARA), Guangdong Provincial Key Laboratory of Microbial Culture Collection and Application, Key Laboratory of Agricultural Microbiome (MARA), State Key Laboratory of Applied Microbiology Southern China, Institute of Microbiology, Guangdong Academy of Sciences, Guangzhou, China
Pseudomonas aeruginosa is a ubiquitous opportunistic pathogen that can cause severe respiratory tract infections. Geraniol, a chemical component of essential oils, has antimicrobial and anti-inflammatory activities, along with low toxicity. However, the effect and mechanism of geraniol against P. aeruginosa virulence factors are rarely studied. In this study, we investigated the quorum sensing (QS) inhibitory effects and mechanisms of geraniol against P. aeruginosa PAO1, using physiological and biochemical techniques, quantitative reverse transcription polymerase chain reaction, and transcriptomics. Geraniol slightly affected P. aeruginosa PAO1 growth, prolonged the lag phase, and delayed growth periods in a concentration-dependent manner. Geraniol inhibited three QS systems of P. aeruginosa, las, rhl, and pqs by suppressing the expression level of their key genes, including the three signal synthetase encoding genes of lasI, rhlI, and pqsABCDEH, and the corresponding signal receptor encoding genes of lasR, rhlR, and pqsR. Geraniol also suppressed certain virulence genes regulated by these three QS systems, including rhlABC, lasAB, lecAB, phzABMS, and pelABG, resulting in the attenuation of the related virulence factors, rhamnolipids, exoprotease LasA, elastase, lectin, pyocyanin, and biofilm. In conclusion, geraniol can suppress the virulence factors of P. aeruginosa PAO1 by inhibiting the three QS systems of las, rhl, and pqs. This study is significant for improving the treatment of bacterial infections caused by P. aeruginosa.
Introduction
Pseudomonas aeruginosa is a common opportunistic pathogen that can cause severe respiratory tract infections, especially in convalescent patients with low immunity (Deshpande and Zou, 2020). P. aeruginosa can easily develop antibiotic resistance, which poses a challenge to clinical treatment, mainly because it has a large 6.3 Mb genome and can make adaptive responses to environmental stresses (Jurado-Martín et al., 2021; Li et al., 2022). The pan-drug resistance of P. aeruginosa makes it difficult to eradicate infections using traditional antibiotics. Studies have shown that bacterial quorum sensing (QS) inhibitors can solve this problem (Chatterjee et al., 2016). QS is a density dependent bacterial cell–cell communication mechanism, which is carried out by small endogenous synthetic organic molecules called autoinducers, which were discovered by Nealson et al. in 1970 (Nealson et al., 1970; Shah et al., 2021; Striednig and Hilbi, 2022). P. aeruginosa has three QS systems as shown in Figure 1, las, rhl, and pqs, which jointly regulate the production of various virulence factors (Lee and Zhang, 2015; Ahator and Zhang, 2019). The las and rhl systems are mediated by acyl-homoserine lactone signals (Li Y. et al., 2021). In the las system, lasI encodes the LasI signal synthase to produce 3-oxo-dodecanoyl-homoserine lactone (3OC12-HSL), and lasR encodes the LasR signal receptor protein. Similarly, in the rhl system, rhlI encodes the signal synthase RhlI to produce butanoyl-homoserine lactone (C4-HSL), and rhlR encodes the RhlR signal receptor protein (Li Y. et al., 2021). In the pqs system, genes of pqsABCDEH encode 2-heptyl-3-hydroxy-4(1H)-quinolone (Pseudomonas quinolone signal, PQS), and 2-heptyl-4-hydroxyquinoline (HHQ) signals, and pqsR encodes the PqsR signal receptor protein (Murray et al., 2022). The las system is typically located at the top of the QS hierarchy of P. aeruginosa, positively regulating the pqs and rhl systems; the pqs system connects the las and rhl systems, positively regulates the rhl system, and is regulated by the rhl system, which is located at the bottom of the QS hierarchy and is positively regulated by the las and pqs systems (Lee and Zhang, 2015; Cornelis, 2020; Soto-Aceves et al., 2021).
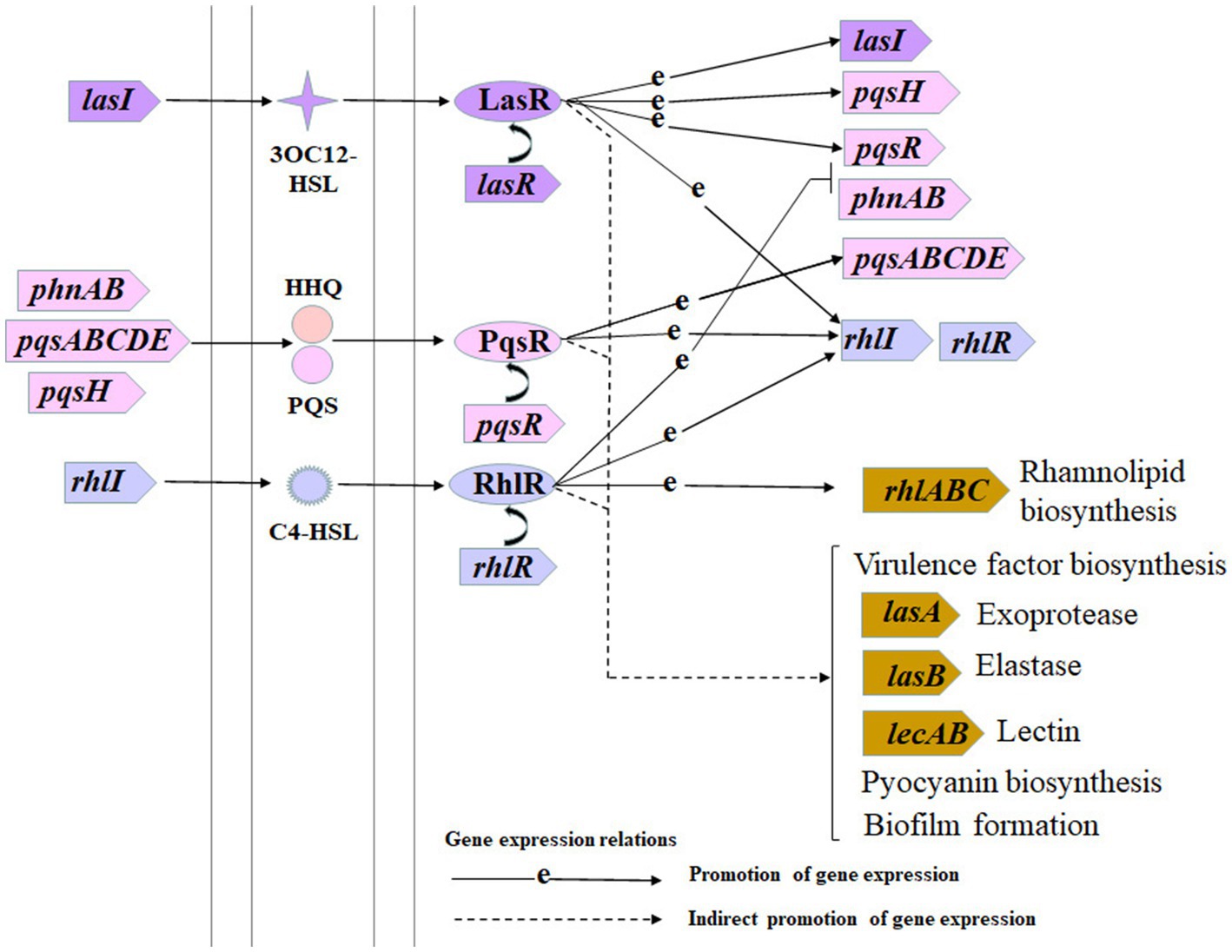
Figure 1. Schematic diagram of Pseudomonas aeruginosa hierarchical quorum sensing systems. This schematic diagram is drawn based on the quorum sensing pathway diagram from the Kyoto Encyclopedia Genes and Genomes database.
Furthermore, P. aeruginosa can cause severe infections in immunocompromised patients owing to its ability to secrete numerous extracellular virulence factors (Coin et al., 1997). As shown in Figure 1, some virulence factor biosynthesis is indirectly regulated by P. aeruginosa QS systems. Elastase LasB and exoprotease LasA are two virulence proteins in the P. aeruginosa secretome. Elastase can hydrolyze many host proteins, especially elastin, causing host tissue injury and immune response failure (Galdino et al., 2019; Everett and Davies, 2021). Although LasB can degrade elastin on its own, LasA contributes to elastin degradation (Varjani and Upasani, 2019). lasB is directly-positively regulated by both the las and rhl systems (Schuster and Greenberg, 2007), and indirectly regulated by the pqs system (Calfee et al., 2001). Pyocyanin is a characteristic P. aeruginosa virulence factor, causing many types of cellular damage, such as cell respiration suppression, calcium homeostasis disruption, and catalase inactivation (Li W. R. et al., 2021; Manisha et al., 2022). Bacterial lectins, such as LecA, are multivalent sugar-binding proteins that target the human glycome to act as invasion factors that damage host epithelial cells (Bodenberger et al., 2018; Day et al., 2019; Patil et al., 2022). Rhamnolipids are glycolipids mainly produced by Pseudomonas species and have multiple roles in metabolite uptake, host invasion, and microbial competition (Varjani and Upasani, 2019; Gdaniec et al., 2022). P. aeruginosa biofilms regulated by QS systems have greater antibiotic tolerance than their planktonic counterparts and usually cause chronic clinical infections that are difficult to treat (Cendra and Torrents, 2021). Therefore, P. aeruginosa QS systems are potential new targets to control its virulence and pathogenicity.
Geraniol, a cyclic monoterpene alcohol, is a chemical component of a large number of essential oils such as citronella, lime, lemongrass, and lavender (Kannappan et al., 2019). Geraniol has various biological activities, including anti-inflammatory, antimicrobial, antitumor, antioxidant, hepatoprotective, cardioprotective, and neuroprotective effects, and thus has been widely used in pharmaceuticals, cosmetics, and household products due to its low toxicity and environmentally friendly characteristics (Chen and Viljoen, 2022). Geraniol has been shown to exhibit high QS inhibitory activity against P. aeruginosa in our research. In this study, we investigated the QS inhibitory effects and mechanisms of geraniol against P. aeruginosa PAO1, using physiological and biochemical techniques, quantitative reverse transcription polymerase chain reaction (qRT-PCR), and transcriptomics. This study is significant for improving the treatment of P. aeruginosa clinical infections.
Materials and methods
Chemicals, media, bacterial strain and treatments
Geraniol (purity = 98%; relative density = 0.88 g/mL) was purchased from Shanghai Aladdin Bio-Chem Technology Co. Ltd. (Shanghai, China). The P. aeruginosa PAO1 strain was provided by the South China Sea Institute of Oceanology, Chinese Academy of Sciences, Guangzhou, China. P. aeruginosa PAO1 was cultured in Luria–Bertani (LB) broth (0.5% yeast extract, 1% tryptone, and 1% NaCl) with varying concentrations of geraniol (0, 0.275, 0.55, 1.1, and 2.2 mg/mL) at 180 rpm and 37°C.
Transcriptomic analysis
Exponential growth phase P. aeruginosa PAO1 cells (1–2 × 108 CFU/mL) of 1 mL were inoculated in 100 mL of fresh LB medium containing 0 (control groups) and 1.1 mg/mL (treatment groups) geraniol with three biological replicates, resulting in an initial cell concentration of 1–2 × 106 CFU/mL. All six samples were incubated at 37°C and 180 rpm for 5 h. Then, the cell precipitates were collected, snap-frozen in liquid nitrogen, and stored at −80°C for RNA extraction.
The RNA extraction, library construction, and transcriptome sequencing and analysis were performed by Novogene Bioinformatics Technology Co. Ltd. (Beijing, China) as described in our previous work (Li et al., 2019). The raw data in fastq format were submitted to the National Center for Biotechnology Information (NCBI) Gene Expression Omnibus database (GSE216316). Differential expression analysis of the two groups (control and geraniol treatment) was performed using the DESeq2 R package. Genes with an adjusted p-value (padj) < 0.05 and |log2(foldchange)| > 1 were assigned as differentially expressed.
qRT-PCR validation of key QS and virulence genes of the PAO1 strain
Differentially expressed genes (DEGs) involved in QS systems were selected for validation using qRT-PCR. The bacterial culture, geraniol treatment, and bacterial precipitate collection methods were the same as above, for transcriptome sequencing and analysis. P. aeruginosa PAO1 cells and geraniol concentrations were 1–2 × 106 CFU/mL and 0 (control groups) or 1.1 mg/mL (treatment groups), respectively. Cells were incubated at 37°C and 180 rpm for 5 h. Total RNA extraction, RNA concentration and quality assessment, reverse transcription PCR (RT-PCR), and quantitative real-time PCR (qPCR) were performed as described in our previous work (Li et al., 2018). The primer information is provided in Supplementary Table S1.
PAO1 growth and virulence factor determination
Growth inhibition assay
Pseudomonas aeruginosa PAO1 in the exponential growth phase at an initial concentration of 1–2 × 106 CFU/mL were added to a 96-well microtiter plate containing geraniol (0, 2.2, 4.4, 8.8, 17.6, 35.2, 70.4, 140.8, and 281.6 mg/mL). The cultures were incubated at 37°C for 16–20 h to determine the minimum inhibitory concentration (MIC) of geraniol against P. aeruginosa PAO1.
P. aeruginosa PAO1 in the exponential growth phase at an initial concentration of 1–2 × 106 CFU/mL were added to a 96-well microtiter plate containing geraniol (0, 0.28, 0.55, 1.1, and 2.2 mg/mL) in 200 μL of LB. The plate was incubated at 37°C and 180 rpm and the optical density (OD600) was recorded using a Spark® multimode microplate reader (Tecan, Swiss Confederation) at hourly intervals for up to 48 h.
PQS production assay
Exponential growth phase P. aeruginosa PAO1 cells (1 mL, 1–2 × 108 CFU/mL) were inoculated in 100 mL LB medium with 0 and 1.1 mg/mL geraniol and incubated at 37°C and 180 rpm for 72 h. During the culture period, cell culture was collected at 24, 48, and 72 h, centrifuged at 12,000× g for 5 min, and the supernatant collected. The extraction and quantification of PQS was performed as described in a previous study (Li et al., 2020). A standard curve was formed with the concentration of the PQS standard sample and its peak area, and the PQS content in the experimental group was calculated according to its peak area.
Elastase activity assay
The exponential growth phase P. aeruginosa PAO1 cells (1 mL, 1–2 × 108 CFU/mL) were inoculated in 100 mL LB medium with geraniol (0, 0.28, 0.55, 1.1, and 2.2 mg/mL) and incubated at 37°C and 180 rpm for 24 h. The 0 mg/mL geraniol group was set as control (+) and sterilized deionized water was used as control (−) instead of the PAO1 cell suspension. After incubation for 5 and 24 h, a 5 mL culture sample was prepared. The elastase activity assay was performed as described in a previous study (Li et al., 2018). The OD495 value was measured to determine elastase activity.
Pyocyanin assay
The exponential growth phase P. aeruginosa PAO1 cells (1 mL, 1–2 × 108 CFU/mL) were inoculated in 100 mL LB medium with geraniol (0, 0.28, 0.55, 1.1, and 2.2 mg/mL) and incubated at 37°C and 180 rpm for 7 days. Sterilized deionized water was used as the negative control instead of the PAO1 cell suspension. A 5-mL culture was sampled each day and the pyocyanin assay was performed as described in a previous study (Li et al., 2018). The concentration of pyocyanin (μg/mL) was determined by multiplying the OD520 reading by 17.072 (Essar et al., 1990).
Biofilm assay
The effect of geraniol on biofilm formation was evaluated using 96-well microtiter plates as described in our previous work (Li et al., 2018). Two-hundred microliter samples of P. aeruginosa PAO1 cells during the exponential growth phase (initial concentration 1–2 × 106 CFU/mL), geraniol (0, 0.28, 0.55, 1.1, and 2.2 mg/mL), and LB were added to a 96-well plate and incubated at 37°C for 24 h without agitation. Sterilized deionized water was used as the negative control instead of the PAO1 cell suspension. The OD600 and OD590 values were measured to obtain the concentration of cells in suspension and the biofilm yield, respectively.
Statistical analysis
All of the experiments were performed in triplicate, and were repeated three times except for the transcriptomic sequencing. Statistical analysis was performed using IBM SPSS Statistics 25.0 software. The statistical data were presented as mean ± standard deviation (SD) and examined by a one-way analysis of variance followed by the Student–Newman–Keuls test. p < 0.05 and p < 0.01 indicates significant differences and extremely significant differences, respectively.
Results
Transcriptomic analysis
Transcriptomic sequencing was performed to examine the gene expression patterns of PAO1 exposed to 0 and 1.1 mg/mL geraniol. A map of the Pearson correlation coefficients is shown in Figure 2A. Pearson correlation coefficients showed that the correlation coefficient R2 of both the three control and three geraniol treatment groups was more than 0.8, and the R2 between control groups and geraniol treatment groups was less than 0.8. Therefore, transcriptomic data have intragroup biological repeatability and intergroup differences. A map of the volcano plots is shown in Figure 2B. The volcano plots detected a total of 5,788 genes based on RNA-seq of P. aeruginosa PAO1 cells which were treated and untreated with geraniol, of which 852 genes were significantly downregulated, 1,169 genes were significantly upregulated with fold change >2 and padj <0.05, and 3,767 genes did not exhibit altered expression levels.
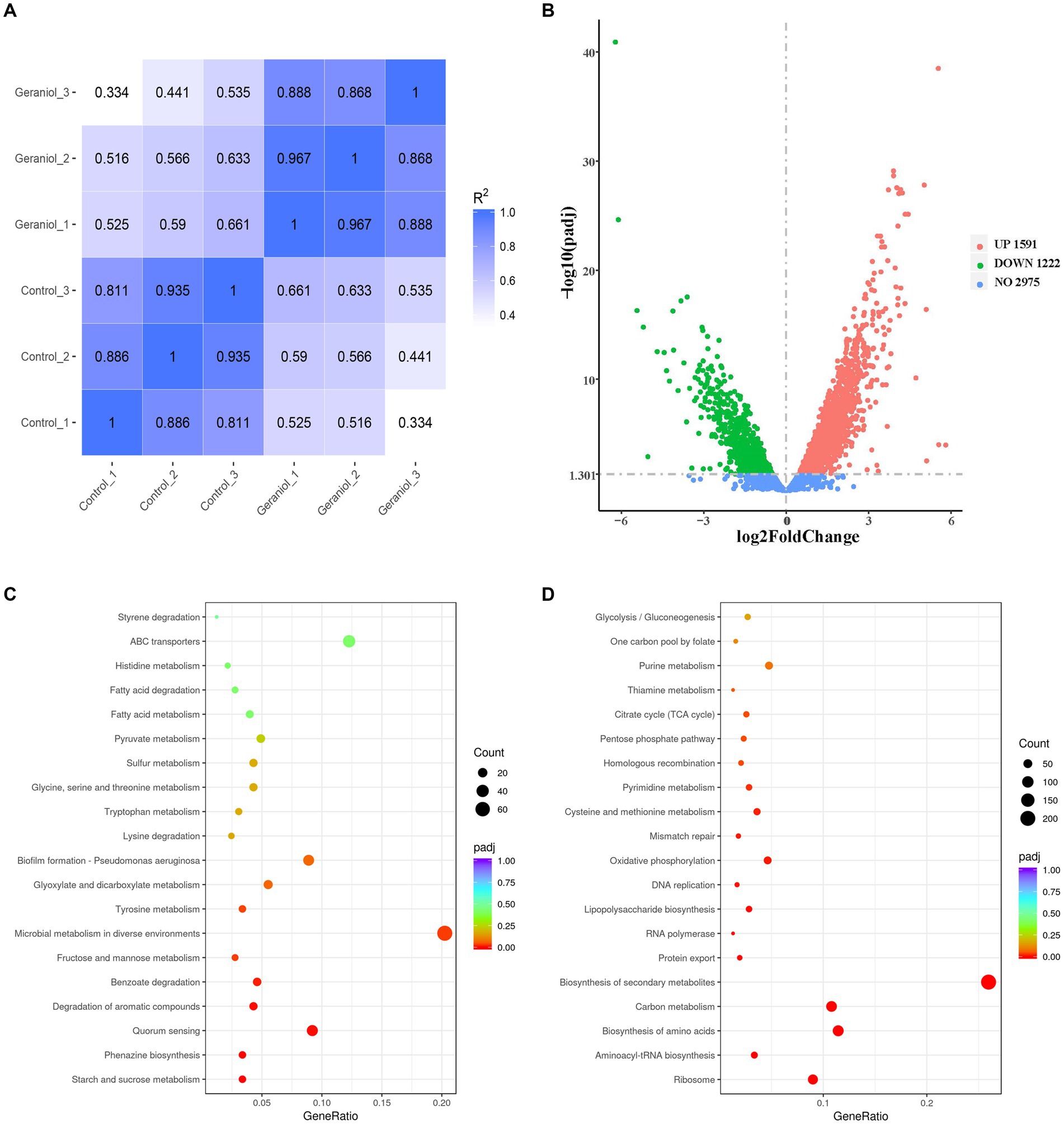
Figure 2. Maps of Pearson correlation coefficients, volcano plots, and Kyoto Encyclopedia of Genes and Genomes (KEGG) enrichment pathways based on RNA-seq data of three control and three treatment groups of Pseudomonas aeruginosa PAO1 with 1.1 mg/mL geraniol (0.00 < q < 0.05). (A) Pearson correlation coefficients map. (B) Volcano plots of differentially expressed genes. The red and green dots represent upregulated genes and downregulated genes with >2-fold change, respectively. (C) Bubble map of the top 20 KEGG enrichment pathways of downregulated genes with >2-fold change. (D) Bubble map of the top 20 KEGG enrichment pathways of upregulated genes with >2-fold change.
The bubble maps of the top 20 Kyto Encyclopedia of Genes and Genomes (KEGG) enrichment pathways of downregulated and upregulated genes with >2-fold changes are shown in Figures 2C,D, respectively. Figure 2C shows that starch and sucrose metabolism, phenazine biosynthesis, QS, degradation of aromatic compounds, benzoate degradation, fructose and mannose metabolism, microbial metabolism in diverse environments, tyrosine metabolism, glyoxylate and dicarboxylate metabolism, and biofilm formation were the top ten pathways enriched with significantly downregulated genes after geraniol treatment. In these downregulated pathways, phenazine biosynthesis, QS, and biofilm formation pathways were enriched with key genes involved in QS and virulence genes regulated by QS. The detailed QS, phenazine biosynthesis, and biofilm formation pathways are shown in Supplementary Figures S1A–C, respectively, and the transcriptional changes of key genes involved in the three pathways are shown in Table 1.
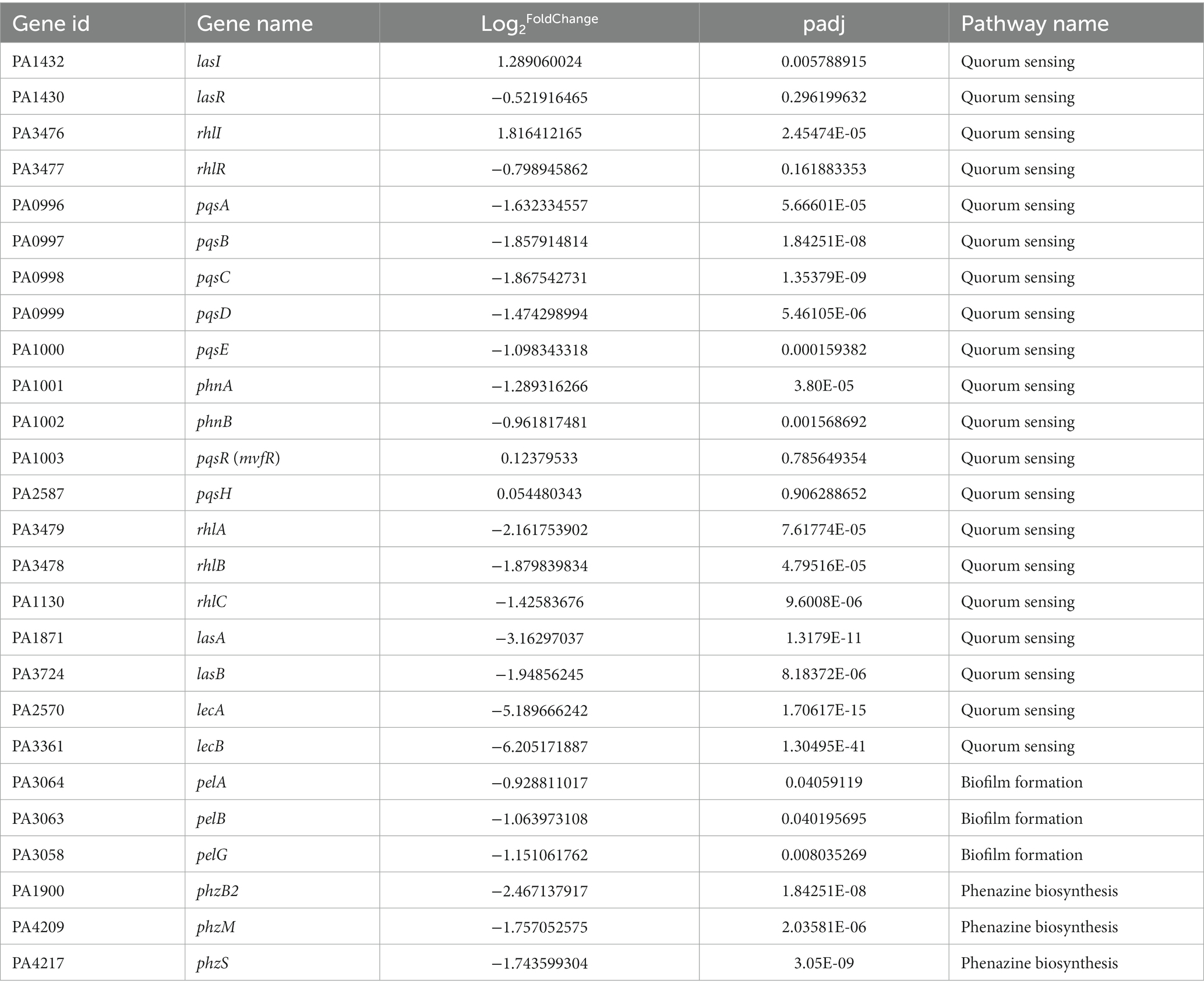
Table 1. Differentially expressed key genes involved in Pseudomonas aeruginosa PAO1 quorum sensing systems and the related virulence genes based on RNA-seq.
The QS pathway of PAO1 was significantly downregulated after geraniol treatment according to the above results. Table 1 and Supplementary Figure S1A show the changes in the transcriptional levels of key genes involved in the PAO1 QS systems after geraniol treatment. In the las system, the lasI gene, encoding the 3OC12-HSL signal synthase LasI, was significantly upregulated, whereas expression of the lasR gene, encoding the signal receptor LasR, was downregulated (<2-fold change). Similarly, in the rhl system, the rhlI gene, which encodes the C4-HSL signal synthase RhlI, was significantly upregulated, and the expression of rhlR, which encodes the signal receptor RhlR, was downregulated (< 2-fold change). In the pqs system, pqsABCDE genes, which are involved in PQS biosynthesis, were significantly downregulated (> 2-fold change), pqsH expression was unchanged, and the expression of pqsR, encoding the PQS signal receptor protein, was also unchanged. Table 1 and Supplementary Figure S1A also show changes in the expression levels of some virulence genes regulated by the QS systems. After geraniol treatment, lasA (encoding protease LasA), lasB (encoding elastase LasB), lecA (encoding lectin LecA), lecB (encoding lectin LecB), and rhlABC (encoding rhamnolipids) expression levels were all downregulated.
Changes in the transcriptional levels of key genes involved in phenazine biosynthesis in the PAO1 strain after geraniol treatment are shown in Table 1 and Supplementary Figure S1B. Most key genes involved in phenazine biosynthesis (including pyocyanin) were downregulated, such as phzAB, phzM, and phzS.
Changes in the transcriptional levels of key genes involved in biofilm formation of the PAO1 strain after geraniol treatment are shown in Table 1 and Supplementary Figure S1C. Some genes involved in biofilm formation were downregulated, such as Pel polysaccharide encoding genes, pelAB and pelG; rhamnolipid encoding genes rhlABC, and lectin encoding genes lecA and lecB.
The top ten KEGG pathways significantly enriched with upregulated genes after geraniol treatment included ribosome, aminoacyl-tRNA biosynthesis, biosynthesis of amino acids, carbon metabolism, biosynthesis of secondary metabolites, protein export, RNA polymerase, lipopolysaccharide biosynthesis, DNA replication, and oxidative phosphorylation (Figure 2D). These upregulated pathways were not related to QS systems or their related virulence genes.
qRT-PCR to validate the results of the transcriptomic analysis
PAO1 cells were treated with 1.1 mg/mL geraniol for 5 h, and the expression levels of key genes in the QS systems and related virulence genes were validated using qRT-PCR (Figure 3). Eleven key QS genes (lasI, lasR, rhlI, rhlR, pqsABCDEH, and pqsR) and four virulence genes (rhlA, lasB, pelB, and phzM) were selected for validation. In the las system, lasI (>2-fold change) and lasR (<2-fold change) were significantly downregulated; in the rhl system, rhlI and rhlR were significantly downregulated (>2-fold change); in the pqs system, pqsABCDEH and pqsR were significantly downregulated (>2-fold change). Therefore, the gene expression trends of key genes in the QS systems were consistent with the transcriptomic results. Similarly, the expression levels of the virulence genes were consistent with those of the transcriptomic results, with rhlA, lasB, pelB, and phzM being significantly downregulated (>2-fold change).
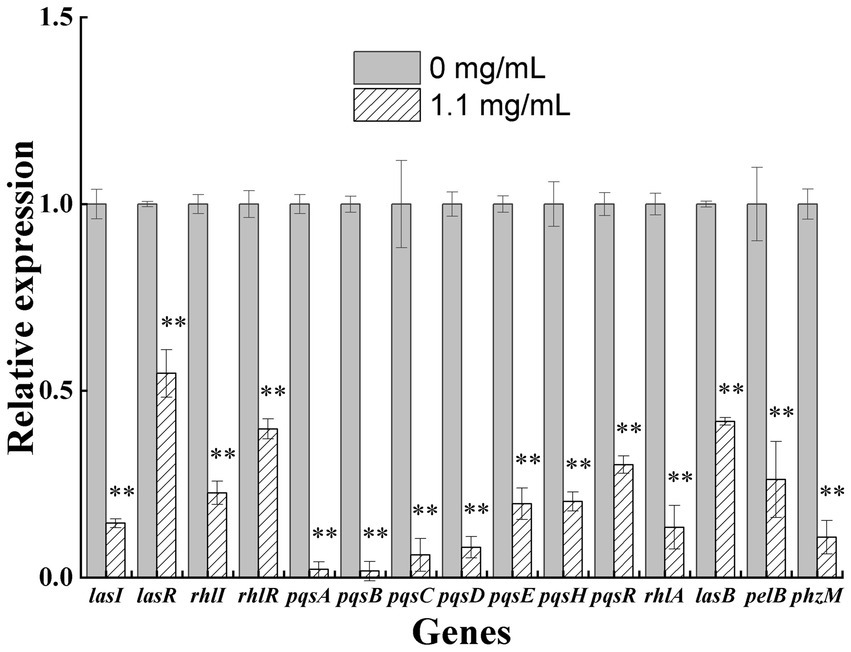
Figure 3. Quantitative reverse transcription polymerase chain reaction validation map of some differentially expressed key genes in the quorum sensing systems and virulence genes of Pseudomonas aeruginosa PAO1 exposed to 1.1 mg/ml geraniol. Independent Student’s t-tests were performed to compare the control and geraniol treatment groups. *p < 0.05; **p < 0.01.
PAO1 growth and virulence factors
Growth curve
The growth inhibition experiments showed that the MIC of geraniol against P. aeruginosa PAO1 was 70.4 mg/mL. The growth curves of PAO1 cells treated with different geraniol concentrations were drawn based on the absorbance values at 600 nm for 48 h. PAO1 growth of the control group showed a typical growth curve, including lag, exponential, and stationary phases (Figure 4). The growth curve did not show a decline phase because the OD600 reflected the total number of living and dead cells. PAO1 cell growth in different geraniol treatment groups showed a prolonged lag phase, delayed growth periods, and reduced growth to different degrees compared to the control group. However, the main PAO1 population cells were not inhibited by geraniol. Therefore, geraniol slightly affected P. aeruginosa growth.
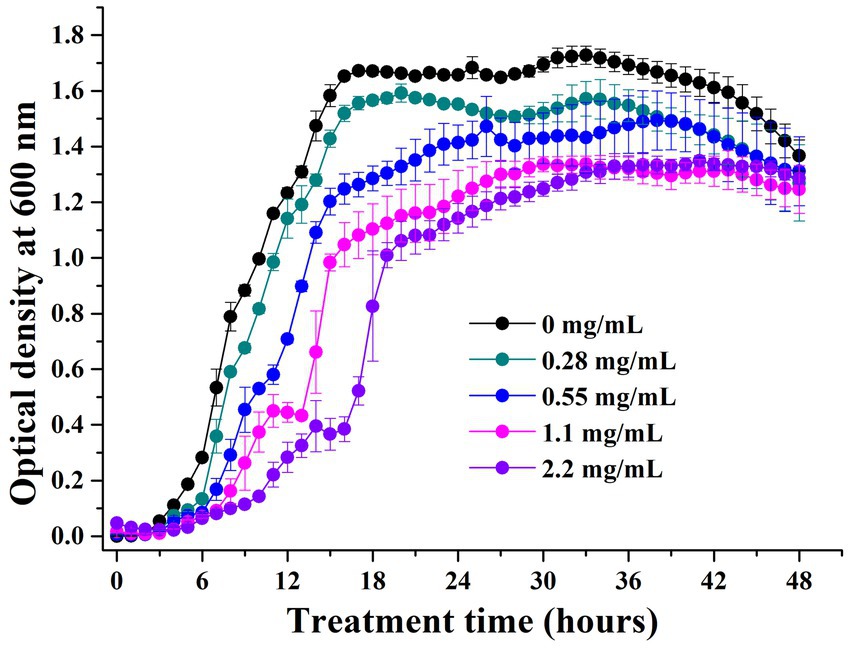
Figure 4. Growth curves of Pseudomonas aeruginosa PAO1 treated with different concentrations of geraniol.
PQS production
The changes in PQS production after PAO1 cells were treated with 1.1 mg/mL geraniol are shown in Figure 5A. The PQS production increased with increasing incubation time in the control group, with the highest concentration at 72 h of incubation and the lowest concentration at 24 h of incubation. The PQS production in the geraniol treatment group did not show a trend of increasing production with an increase in incubation time as compared to the control group. The PQS concentrations in the geraniol treatment group were significantly decreased compared to those in the control group after incubation for 48 and 72 h. Therefore, geraniol significantly inhibited the PQS production ability of P. aeruginosa PAO1.
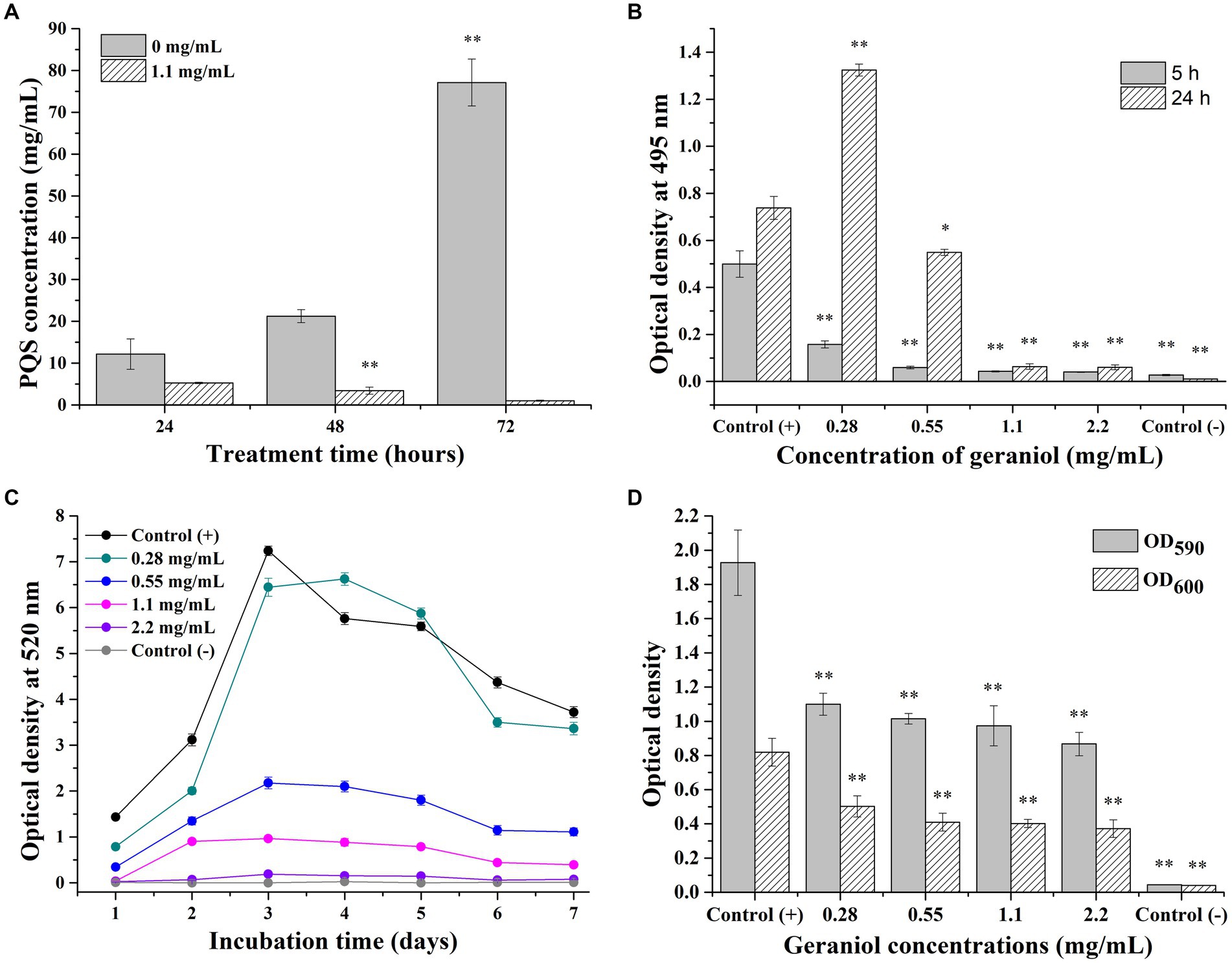
Figure 5. Maps presenting the effect of geraniol on Pseudomonas aeruginosa PAO1 virulence factors. (A) Histogram of Pseudomonas quinolone signal production. (B) Histogram of elastase LasB production. (C) Curves of pyocyanin production. (D) Histogram of biofilm and planktonic cell yield. OD600 and OD590 mean relative planktonic cell concentration and biofilm yield, respectively. Independent Student’s t-tests were performed to compare the control and geraniol treatment groups. *p < 0.05; **p < 0.01.
Elastase LasB activity
PAO1 cells were treated with different concentrations of geraniol and the elastase LasB activity in the culture supernatants were determined at 5 and 24 h (Figure 5B). In the control group, elastase LasB production increased with increasing incubation time, with the elastase production at 24 h of incubation being higher than that at 5 h. The elastase production in the geraniol treatment groups (0.55–2.2 mg/mL) were significantly decreased compared with that of the control group and did not show an increase with the increased incubation time. However, in the 0.28 mg/mL geraniol treatment group, elastase production increased with increasing incubation time, and elastase production after 24 h of incubation was significantly higher than that in the control group. This indicated that a low concentration geraniol (0.28 mg/ml) stimulates elastase production in the PAO1 strain. Therefore, geraniol significantly inhibited elastase production by P. aeruginosa PAO1 in a concentration-dependent manner, except at low concentrations (0.28 mg/ml).
Pyocyanin yield
The production of pyocyanin by PAO1 cells after continuous treatment with different geraniol concentrations for 7 days is presented in Figure 5C. In the control (+) group, pyocyanin production increased continuously during the first 3 days of incubation and reached a maximum on the third day of incubation. In the next 4 days of incubation, pyocyanin production in the control group decreased continuously. In the control (−) group, pyocyanin production in cell-free cultures was nearly zero after incubation for one to 7 days. The pyocyanin production trends in different geraniol treatment groups (0.28–2.2 mg/mL) were consistent with that of the control. However, pyocyanin yields decreased with increasing geraniol concentration. Especially in the 2.2 mg/mL geraniol treatment group, pyocyanin production was similar to that in the control (−) group, nearly zero after 7 days of incubation. Therefore, geraniol attenuated pyocyanin production by P. aeruginosa PAO1 in a concentration-dependent manner.
Biofilm formation
PAO1 cells were treated with different concentrations of geraniol, seeded in a 96-well plate, and incubated for 24 h. The relative bacterial-suspension concentration (OD600) and biofilm yield (OD590) were determined (Figure 5D). The relative PAO1 cell-suspension concentration and biofilm yield were normal in the control (+) group, while the OD600 and OD590 were nearly zero in the control (−) group. The bacterial-suspension concentration and biofilm yield both decreased in all geraniol treatment groups (0.28–2.2 mg/mL) compared with that of the control (+) group. Therefore, geraniol inhibited planktonic P. aeruginosa PAO1 cells growth and biofilm formation.
Discussion
Geraniol, a chemical component of citronella, ginger, lemon, rose, nutmeg, lavender, and orange essential oils, is a colorless to pale-yellow liquid with a rose-like scent (Silva et al., 2022). The growth curves of P. aeruginosa PAO1 cells treated with geraniol showed that geraniol slightly affected cell growth, prolongs the lag phase, and delays the growth period, but with the main population of cells not being inhibited. Another study found that Palmarosa essential oil, which is composed of 81.25% geraniol, had a slight antibacterial activity against P. aeruginosa PAO1 growth and inhibited certain virulence factors regulated by the QS systems (Önem, 2022).
Transcriptomic analysis detected a total of 5,788 genes, of which 852 and 1,169 genes were significantly downregulated and upregulated, respectively. The KEGG pathways of QS, biofilm formation, and phenazine biosynthesis were enriched with the downregulated key genes involved in P. aeruginosa QS systems and virulence factor encoding genes regulated by the QS systems. These transcriptomic and qRT-PCR results showed that geraniol inhibited lasI expression, which encodes 3OC12-HSL signal synthetase in the las system, rhlI expression, which encodes C4-HSL signal synthetase in the rhl system, and pqsABCDEH expression, which are involved in PQS biosynthesis in the pqs system, and inhibited the expression of lasR, rhlR, and pqsR encoding three corresponding signal receptor proteins, respectively.
Moreover, some virulence genes, including rhlABC involved in rhamnolipid biosynthesis; lasA and lasB encoding exoprotease LasA and elastase LasB, respectively; lecA encoding lectin LecA and lecB encoding lectin LecB; pelAB and pelG involved in biofilm formation; and phzAB, phzM, and phzS involved in pyocyanin biosynthesis were all downregulated by geraniol as shown in the transcriptomic results. The qRT-PCR results showed similar down transcriptional trends to those of the transcriptomic analysis. Furthermore, the physiological and biochemical results showed similar downregulation trends upon geraniol exposure, with a decrease in PQS signals, elastase, pyocyanin, and biofilm yields.
The transcriptomic, qRT-PCR, and physiological-biochemical results showed that geraniol inhibited 3OC12-HSL, C4-HSL, and PQS signal biosynthesis and the corresponding receptor proteins, resulting in the attenuation of some virulence factors regulated by the three QS systems, such as exoprotease LasA, elastase, lectin, rhamnolipids, pyocyanin, and biofilm formation. These results indicate that the inhibition of the three QS systems could attenuate the virulence factors indirectly regulated by the QS systems. Some previous studies on the inhibition of P. aeruginosa PAO1 QS systems by diallyl sulfide (Li W. R. et al., 2021), diallyl disulfide (Li et al., 2019), diallyl trisulfide (Li et al., 2022), and farnesol had similar findings (Li et al., 2020). Diallyl sulfide, diallyl disulfide, diallyl trisulfide, and geraniol inhibited the three QS systems of las, rhl, and pqs. Farnesol inhibited key genes involved in PQS biosynthesis of the pqs system but did not affect the expression levels of pqsR of the pqs system, lasI/lasR of the las system, and rhlI/rhlR of the rhl system (Li et al., 2020). Nonetheless, geraniol, diallyl sulfide, diallyl disulfide, diallyl trisulfide, and farnesol inhibited the transcription of the relevant virulence genes regulated by the three QS systems. A previous study also reported that a null mutation in the pqs system induced a decrease in the production of biofilms, pyocyanin, elastase, lectin, and rhamnolipids (Cao et al., 2001; Diggle et al., 2003). The pqs signaling system is important in the biosynthesis regulation of the virulence factors mentioned above.
In conclusion, the MIC of geraniol against P. aeruginosa PAO1 was 70.4 mg/ml. Geraniol of 1.1 mg/ml slightly affected PAO1 growth, prolonged the lag phase, and delayed the growth periods. However, geraniol inhibited the three P. aeruginosa QS systems, las, rhl, and pqs by suppressing the expression level of their key genes, including lasI, rhlI, pqsABCDEH, lasR, rhlR, and pqsR. Geraniol also suppressed certain virulence genes regulated by the three QS systems, including rhlABC, lasAB, lecAB, phzABMS, and pelABG, resulting in the attenuation of related virulence factors of rhamnolipids, exoproteases, lectins, pyocyanin, and biofilm. Therefore, geraniol suppresses P. aeruginosa PAO1 virulence by inhibiting its three QS systems of las, rhl, and pqs.
Data availability statement
The datasets presented in this study can be found in online repositories. The names of the repository/repositories and accession number(s) can be found in the article/Supplementary material.
Author contributions
W-RL and T-HZ: conceptualization, formal analysis, investigation, and writing–review and editing. W-RL, T-HZ, and Z-QZ: methodology and validation. X-BX and Q-SS: supervision. W-RL: funding acquisition and project administration. All authors have read and agreed to the published version of the manuscript.
Funding
This research was funded by Guangdong Basic and Applied Basic Research Foundation (2021A1515011080 and 2020A1515010850), and GDAS’ Project of Science and Technology Development (2018GDASCX-0102).
Conflict of interest
The authors declare that the research was conducted in the absence of any commercial or financial relationships that could be construed as a potential conflict of interest.
Publisher’s note
All claims expressed in this article are solely those of the authors and do not necessarily represent those of their affiliated organizations, or those of the publisher, the editors and the reviewers. Any product that may be evaluated in this article, or claim that may be made by its manufacturer, is not guaranteed or endorsed by the publisher.
Supplementary material
The Supplementary material for this article can be found online at: https://www.frontiersin.org/articles/10.3389/fmicb.2023.1190619/full#supplementary-material
References
Ahator, S. D., and Zhang, L. H. (2019). Small is mighty-chemical communication systems in Pseudomonas aeruginosa. Annu. Rev. Microbiol. 73, 559–578. doi: 10.1146/annurev-micro-020518-120044
Bodenberger, N., Kubiczek, D., Halbgebauer, D., Rimola, V., Wiese, S., Mayer, D., et al. (2018). Lectin-functionalized composite hydrogels for “capture-and-killing” of carbapenem-resistant Pseudomonas aeruginosa. Biomacromolecules 19, 2472–2482. doi: 10.1021/acs.biomac.8b00089
Calfee, M. W., Coleman, J. P., and Pesci, E. C. (2001). Interference with Pseudomonas quinolone signal synthesis inhibits virulence factor expression by Pseudomonas aeruginosa. Proc. Natl. Acad. Sci. U. S. A. 98, 11633–11637. doi: 10.1073/pnas.201328498
Cao, H., Krishnan, G., Goumnerov, B., Tsongalis, J., Tompkins, R., and Rahme, L. G. (2001). A quorum sensing-associated virulence gene of Pseudomonas aeruginosa encodes a LysR-like transcription regulator with a unique self-regulatory mechanism. Proc. Natl. Acad. Sci. U. S. A. 98, 14613–14618. doi: 10.1073/pnas.251465298
Cendra, M. M., and Torrents, E. (2021). Pseudomonas aeruginosa biofilm and their partners in crime. Biotechnol. Adv. 49:107734. doi: 10.1016/j.biotechadv.2021.107734
Chatterjee, M., Anju, C. P., Biswas, L., Kumar, V. A., Mohan, C. G., and Biswas, R. (2016). Antibiotic resistance in Pseudomonas aeruginosa and alternative therapeutic options. Int. J. Med. Microbiol. 306, 48–58. doi: 10.1016/j.ijmm.2015.11.004
Chen, W., and Viljoen, A. M. (2022). Geraniol – a review update. S. Afr. J. Bot. 150, 1205–1219. doi: 10.1016/j.sajb.2022.09.012
Coin, D., Louis, D., Bernillon, J., Guinand, M., and Wallach, J. (1997). LasA, alkaline protease and elastase in clinical strains of Pseudomonas aeruginosa: quantification by immunochemical methods. FEMS Immunol. Med. Mic. 18, 175–184. doi: 10.1111/j.1574-695X.1997.tb01043.x
Cornelis, P. (2020). Putting an end to the Pseudomonas aeruginosa IQS controversy. Microbiol. Open 9:e962. doi: 10.1002/mbo3.962
Day, C. J., Hartley-Tassell, L. E., Seib, K. L., Tiralongo, J., Bovin, N., Savino, S., et al. (2019). Lectin activity of Pseudomonas aeruginosa vaccine candidates PSE17-1, PSE41-5 and PSE54. Biochem. Bioph. Res. Commun. 513, 287–290. doi: 10.1016/j.bbrc.2019.03.092
Deshpande, R., and Zou, C. (2020). Pseudomonas aeruginosa induced cell death in acute lung injury and acute respiratory distress syndrome. Int. J. Mol. Sci. 21:5356. doi: 10.3390/ijms21155356
Diggle, S. P., Winzer, K., Chhabra, S. R., Worrall, K. E., Cámara, M., and Williams, P. (2003). The Pseudomonas aeruginosa quinolone signal molecule overcomes the cell density-dependency of the quorum sensing hierarchy, regulates rhl-dependent genes at the onset of stationary phase and can be produced in the absence of LasR. Mol. Microbiol. 50, 29–43. doi: 10.1046/j.1365-2958.2003.03672.x
Essar, D. W., Eberly, L., Hadero, A., and Crawford, I. P. (1990). Identification and characterization of genes for a second anthranilate synthase in Pseudomonas aeruginosa: interchangeability of the two anthranilate synthases and evolutionary implications. J. Bacteriol. 172, 884–900. doi: 10.1128/jb.172.2.884-900.1990
Everett, M. J., and Davies, D. T. (2021). Pseudomonas aeruginosa elastase (LasB) as a therapeutic target. Drug Discov. Today 26, 2108–2123. doi: 10.1016/j.drudis.2021.02.026
Galdino, A. C. M., Oliveira, M. P., Ramalho, T. C., Castro, A. A., Branquinha, M. H., and Santos, A. L. S. (2019). Anti-virulence strategy against the multidrug-resistant bacterial pathogen Pseudomonas aeruginosa: Pseudolysin (elastase B) as a potential drug-gable target. Curr. Protein Pept. Sci. 20, 471–487. doi: 10.2174/1389203720666190207100415
Gdaniec, B. G., Bonini, F., Prodon, F., Braschler, T., Köhler, T., and Delden, C. (2022). Pseudomonas aeruginosa rhamnolipid micelles deliver toxic metabolites and antibiotics into Staphylococcus aureus. iScience 25:103669. doi: 10.1016/j.isci.2021.103669
Jurado-Martín, I., Sainz-Mejías, M., and McClean, S. (2021). Pseudomonas aeruginosa: an audacious pathogen with an adaptable arsenal of virulence factors. Int. J. Mol. Sci. 22:3128. doi: 10.3390/ijms22063128
Kannappan, A., Balasubramaniam, B., Ranjitha, R., Srinivasa, R., Packiavathy, I. A. S. V., Balamurugan, K., et al. (2019). In vitro and in vivo biofilm inhibitory efficacy of geraniol-cefotaxime combination against staphylococcus spp. Food Chem. Toxicol. 125, 322–332. doi: 10.1016/j.fct.2019.01.008
Lee, J., and Zhang, L. H. (2015). The hierarchy quorum sensing network in Pseudomonas aeruginosa. Protein Cell 6, 26–41. doi: 10.1007/s13238-014-0100-x
Li, W. R., Ma, Y. K., Shi, Q. S., Xie, X. B., Sun, T. L., Peng, H., et al. (2018). Diallyl disulfide from garlic oil inhibits Pseudomonas aeruginosa virulence factors by inhibiting the transcription of key quorum sensing genes. Appl. Microbiol. Biotechnol. 102, 7555–7564. doi: 10.1007/s00253-018-9175-2
Li, W. R., Ma, Y. K., Xie, X. B., Shi, Q. S., Wen, X., Sun, T. L., et al. (2019). Diallyl disulfide from garlic oil inhibits Pseudomonas aeruginosa quorum sensing systems and corresponding virulence factors. Front. Microbiol. 9:3222. doi: 10.3389/fmicb.2018.03222
Li, Y., Xia, L. X., Chen, J., Lian, Y. L., Dandekar, A. A., Xu, F., et al. (2021). Resistance elicited by sub-lethal concentrations of ampicillin is partially mediated by quorum sensing in Pseudomonas aeruginosa. Environ. Int. 156:106619. doi: 10.1016/j.envint.2021.106619. a
Li, W. R., Zeng, T. H., Xie, X. B., Shi, Q. S., and Li, C. L. (2020). Inhibition of the pqsABCDE and pqsH in the pqs quorum sensing system and related virulence factors of the Pseudomonas aeruginosa PAO1 strain by farnesol. Int. Biodeterior. Biodegrad. 151:104956. doi: 10.1016/j.ibiod.2020.104956
Li, W. R., Zeng, T. H., Yao, J. W., Zhu, L. P., Zhang, Z. Q., Xie, X. B., et al. (2021). Diallyl sulfide from garlic suppresses quorum sensing systems of Pseudomonas aeruginosa and enhances biosynthesis of three B vitamins through its thioether group. Microb. Biotechnol. 14, 677–691. doi: 10.1111/1751-7915.13729. b
Li, W. R., Zhang, Z. Q., Yao, J. W., Liao, K., Zhu, L. P., Shi, Q. S., et al. (2022). Diallyl trisulfide attenuates Pseudomonas aeruginosa virulence via inhibiting quorum sensing. Int. Biodeterior. Biodegr. 173:105463. doi: 10.1016/j.ibiod.2022.105463
Manisha, H., Sonia, J., Shashikiran, S., Yuvarajan, S., Rekha, P. D., Sudhakara, K., et al. (2022). Computer numerical control printed paper electrodes for electrochemical detection of Pseudomonas aeruginosa virulence factor pyocyanin. Electrochem. Commun. 137:107259. doi: 10.1016/j.elecom.2022.107259
Murray, E. J., Dubern, J. F., Chan, W. C., Chhabra, S. R., and Williams, P. (2022). A Pseudomonas aeruginosa PQS quorum sensing system inhibitor with anti-staphylococcal activity sensitizes polymicrobial biofilms to tobramycin. Cell Chem. Biol. 29, 1187–1199.e6. doi: 10.1016/j.chembiol.2022.02.007
Nealson, K. H., Platt, T., and Hastings, J. W. (1970). Cellular control of the synthesis and activity of the bacterial luminescent system. J. Bacteriol. 104, 313–322. doi: 10.1128/jb.104.1.313-322.1970
Önem, E. (2022). New green solutions against bacterial resistance: palmarosa (Cymbopogon martini) essential oil and quorum sensing. Sustain. Chem. Pharm. 25:100587. doi: 10.1016/j.scp.2021.100587
Patil, P. D., Jin, Y. C., and Luk, Y. Y. (2022). Chemical control over Asialo-GM1: a dual ligand for pili and lectin A that activates swarming motility and facilitates adherence of Pseudomonas aeruginosa. Colloid Surface B 215:112478. doi: 10.1016/j.colsurfb.2022.112478
Schuster, M., and Greenberg, E. P. (2007). Early activation of quorum sensing in Pseudomonas aeruginosa reveals the architecture of a complex regulon. BMC Genomics 8:287. doi: 10.1186/1471-2164-8-287
Shah, M., Talor, V. L., Bona, D., Tsao, Y., Stanley, S. Y., Pimentel-Elardo, S. M., et al. (2021). A phage-encoded anti-activator inhibits quorum sensing in Pseudomonas aeruginosa. Mol. Cell 81, 571–583.e6. doi: 10.1016/j.molcel.2020.12.011
Silva, G. S., Marques, J. N. J., Linhares, E. P. M., Bonora, C. M., Costa, É. T., and Saraiva, M. F. (2022). Review of anticancer activity of monoterpenoids: geraniol, nerol, geranial and neral. Chem. Biol. Interact. 362:109994. doi: 10.1016/j.cbi.2022.109994
Soto-Aceves, M. P., Cocotl-Yañez, M., Servín-González, L., and Soberón-Chávez, G. (2021). The Rhl quorum-sensing system is at the top of the regulatory hierarchy under phosphate limiting conditions in Pseudomonas aeruginosa PAO1. J. Bacteriol. 203, e00475–e00420. doi: 10.1128/JB.00475-20
Striednig, B., and Hilbi, H. (2022). Bacterial quorum sensing and phenotypic heterogeneity: how the collective shapes the individual. Trends Microbiol. 30, 379–389. doi: 10.1016/j.tim.2021.09.001
Keywords: quorum sensing, geraniol, Pseudomonas aeruginosa , virulence genes, biofilm
Citation: Li W-R, Zeng T-H, Zhang Z-Q, Shi Q-S and Xie X-B (2023) Geraniol attenuates virulence factors by inhibiting quorum sensing of Pseudomonas aeruginosa. Front. Microbiol. 14:1190619. doi: 10.3389/fmicb.2023.1190619
Edited by:
Xiaobo Liu, Nanjing University of Science and Technology, ChinaReviewed by:
Peng Wu, Chinese Academy of Fishery Sciences (CAFS), ChinaGuoxia Zhang, Southern Medical University, China
Copyright © 2023 Li, Zeng, Zhang, Shi and Xie. This is an open-access article distributed under the terms of the Creative Commons Attribution License (CC BY). The use, distribution or reproduction in other forums is permitted, provided the original author(s) and the copyright owner(s) are credited and that the original publication in this journal is cited, in accordance with accepted academic practice. No use, distribution or reproduction is permitted which does not comply with these terms.
*Correspondence: Xiao-Bao Xie, xiexb@gdim.cn