- 1School of Biological Engineering, Henan University of Technology, Zhengzhou, China
- 2Key Laboratory of Functional Molecules for Biomedical Research, Zhengzhou, China
Introduction: Bipolaris sorokiniana is the popular pathogenic fungi fungus which lead to common root rot and leaf spot on wheat. Generally, chemical fungicides are used to control diseases. However, the environmental pollution resulting from fungicides should not be ignored. It is important to study the mode of antagonistic action between biocontrol microbes and plant pathogens to design efficient biocontrol strategies.
Results: An antagonistic bacterium DB2 was isolated and identified as Bacillus amyloliquefaciens. The inhibition rate of cell-free culture filtrate (CF, 20%, v/v) of DB2 against B. sorokiniana reached 92.67%. Light microscopy and scanning electron microscopy (SEM) showed that the CF significantly altered the mycelial morphology of B. sorokiniana and disrupted cellular integrity. Fluorescence microscopy showed that culture filtrate destroyed mycelial cell membrane integrity, decreased the mitochondrial transmembrane potential, induced reactive oxygen species (ROS) accumulation, and nuclear damage which caused cell death in B. sorokiniana. Moreover, the strain exhibited considerable production of protease and amylase, and showed a significant siderophore and indole-3-acetic acid (IAA) production. In the detached leaves and potted plants control assay, B. amyloliquefacien DB2 had remarkable inhibition activity against B. sorokiniana and the pot control efficacy was 75.22%. Furthermore, DB2 suspension had a significant promotion for wheat seedlings growth.
Conclusion: B. amyloliquefaciens DB2 can be taken as a potential biocontrol agent to inhibit B. sorokiniana on wheat and promote wheat growth.
1. Introduction
Wheat (Triticum aestivum L.) is the most important and widely planted global crop. According to the Food and Agriculture Organization of the United Nations (FAOSTAT: http://www.fao.org/faostat/en/) data, China produces more cereal crops, especially wheat with an estimated production of around 134 million tons, than any country in the world. However, wheat diseases caused by pathogenic fungi are the major limiting factors seriously affecting global wheat (Lu et al., 2020). Root and stem rot diseases of wheat caused a great loss of wheat yield and are substantial problems for crop planting. Bipolaris sorokiniana is a soil-borne pathogen known to cause common root rot and foliar blight in different wheat varieties, and is among the most destructive pathogens for wheat (Kumar et al., 2002; Zhang et al., 2020). In case of serious infection, more than 80% yield loss can be caused by B. sorokiniana under high-temperature and high-humidity environment (Kumar et al., 2009; Kaur et al., 2021). With the warming of climate and crop rotation, B. sorokiniana infects wheat increasingly and may be defined as the major pathogen in the wheat planting area of North China (Cohen and Leach, 2020; Su et al., 2021).
For infection, B. sorokiniana asexual conidiospore germinates on the surface of wheat leaves and forms an appressorium, then a penetration mycelia grows (Villa-Rodríguez et al., 2019). Also, the pathogen can survive in the soil to kill the root and crown of wheat seedlings, and its pathogenicity is associated with the production of toxins such as prehelminthosporol and helminthosporol (Kumar et al., 2001). Therefore, there is an urgent need to find sustainable and effective strategies for controlling this wheat disease. Chemical control is still the main way to prevent and control common root rot on wheat (Shcherbakova et al., 2018). It is reported that most chemical fungicides have excellent protective and curative power against a wide spectrum of crop diseases especially carbendazim and triazole fungicides (Bai et al., 2020). Unfortunately, frequent usage of chemical fungicides cause soil pollution, toxicity to humans, and the emergence of resistant strains (Manhas and Kaur, 2016). Due to limitations associated with chemical fungicide application, breeding wheat varieties with high levels of resistance is the most desirable method of control (Sari et al., 2019). However, it has been challenging to develop complete resistance cultivars. Up to now, biocontrol strategies have attracted huge scientific attention because they are environmentally friendly (Hanif et al., 2019).
Several microorganisms have been reported for the biocontrol of wheat diseases and promote plant growth (Lugtenberg and Kamilova, 2009). A successful antagonist strain Chaetomium sp. was earlier found to inhibit B. sorokiniana and Fusarium culmorum (Knudsen et al., 1995). Recently, Bacillus subtilis TE3 and B. amyloliquefaciens XZ34-1 were verified to be effective against B. sorokiniana (Villa-Rodriguez et al., 2021; Yi et al., 2021). Furthermore, the bio-efficacy of Bacillus safensis, Chaetomium globosum, and Ochrobactrum pseudogrignonense under in vitro and in vivo, and mechanism of antagonism also have been studied (Aggarwall et al., 2004; Al-Sadi, 2021). Nevertheless, the numbers of biocontrol agents are still limited nowadays. Currently, Bacillus spp. is widely used and developed in agriculture, which has the advantage over other biocontrol microorganisms because of producing various antifungal compounds and strong tolerance to extreme conditions changes (Su et al., 2021). Among them, B. amyloliquefaciens has a prominent ability to defeat plant pathogens (Jin et al., 2017; Awan et al., 2022). A previous study indicated that different strains of B. amyloliquefaciens can produce various levels of indole-3-acetic acid (IAA), extracellular enzymes, antibiotic compounds to protect plants from soil-borne fungal pathogen infection and promote plant growth (Belbahri et al., 2017). For instance, B. amyloliquefaciens SN13 is demonstrated to act as a biocontrol agent and enhance the immune response against Rhizoctonia solani in rice (Srivastava et al., 2016). Bacillus amyloliquefaciens strain SQR9 can inhibit the growth of Fusarium oxysporum in the cucumber rhizosphere (Xu et al., 2014). Bacillus amyloliquefaciens can produce antifungal substances to infect the normal growth of pathogens and lead to cellular necrosis and apoptosis because of mitochondrial damage and disruption of cell membranes (Li et al., 2016). Bacillus amyloliquefaciens LYZ69 induced reactive oxygen species accumulation and caused apoptosis-like cell death in C. truncatum (Hu et al., 2021). Despite this, the mode of antagonistic action between B. amyloliquefaciens and B. sorokiniana still needs to be studied in the future.
In the present study, the main objectives of this study were exploring the antifungal strain, investigating the possible action mode of B. amyloliquefaciens DB2 against B. sorokiniana, and evaluating the effects of DB2 culture filtrate on the pathogen control and growth promotion for wheat. This work will provide an effective biological agent to control B. sorokiniana and promote wheat growth.
2. Materials and methods
2.1. Materials
Soil samples for isolating strains were collected from the rhizosphere soil of wheat in Zhengzhou City, Henan province. The pathogenic fungus B. sorokiniana was kindly supplied by the Institute of Plant Protection, Henan Academy of Agriculture Sciences. Wheat cultivar “Zhengmai 366,” highly sensitive to B. sorokiniana, was used in the potted plants experiment. Wheat seeds were provided by the Institute of Wheat Research, Henan Academy of Agricultural Sciences.
2.2. Isolation and identification of antagonistic bacteria
Antagonistic strains were isolated and screened according to the method previously described by Wang et al. (2018). Soil samples were serially diluted up to 10-6 with sterile distilled water and spread on Luria-Bertani (LB) agar medium and incubated at 37°C for 48 h. The colonies with different morphology characteristics were selected for purifying, and their antifungal activities to B. sorokiniana were tested on potato dextrose agar (PDA) medium plates by plate confrontation method (Li et al., 2019).
Antagonistic strain DB2 was screened out, and the colony morphology, physiological, and biochemical characteristics were analyzed according to Bergey’s manual of systematic bacteriology (Bergey, 1994).
16S rDNA sequence analysis was performed for molecular identification of stain DB2. The genomic DNA was isolated using the modified CTAB method (Kumari et al., 2015). The partial sequence of 16S rDNA was amplified by PCR using bacterial universal primer pairs of 16SF (5′-AGAGTTTGATCATGGCTCAG-3′) and 16SR (5′-ACGGTTACCTTGTTACGACTT-3′; Weisburg et al., 1991). PCR conditions were carried out according to the protocol of Yi et al. (2021). The PCR products were sent to Bo Maide Biotech Co., Ltd. (Beijing, China) for sequencing. The sequences were submitted to NCBI1 under the accession number MZ664342 and compared using BLAST tool. Phylogenetic tree was constructed using the Neighbor-joining method of MEGA 11.0 with bootstrap values based on 1,000 replications (Zheng et al., 2021).
2.3. In vitro antagonistic activity of culture filtrate
Antagonistic strain was inoculated into the basal fermentation medium (0.5 g glucose, 2 g peptone, 1.25 g MgSO4, 0.2 g K2HPO4, 5 g yeast extract, 100 mL distilled water, and pH7) and cultured for 48 h at 37°C with shaking at 180 rpm, followed by centrifuging at 10,000 rpm for 10 min and filtering through 0.22 μm filter to obtain the cell-free culture filtrate.
Effects of culture filtrate (CF) on conidial germination of B. sorokiniana were tested using the micro-bioassay method in 24 well plates (Villa-Rodriguez et al., 2021). Bipolaris sorokiniana was added into 5 mL of sterile saline solution (0.9%) containing Tween-20 surfactant (polyoxyethylene sorbitan monooleate) and spread with a Drigalski loop (Monteiro et al., 2017). The conidia of B. sorokiniana were grown in potato dextrose broth (PDB) and diluted with distilled water, and its final concentration was determined with a hemocytometer. Next, 100 μL conidia suspension (105 conidia/mL) of B. sorokiniana were inoculated into each well containing: (1) 700 μL PDB + 200 μL CF (to reach a final concentration of 20% v/v); (2) 800 μL PDB + 100 μL CF (to reach a final concentration of 10% v/v); and (3) 900 μL PDB as the control group. The wells were sealed and cultured in the dark at 28°C for 72 h for conidia observing under the optical microscopy. The fungal mycelia were harvested from well plates by centrifugation at 8,000 × rpm for 15 min. The pellet was washed with sterile water, and the dry weight was determined after heating in the oven at 105°C until a constant weight. Dry weight of fungal biomass was recorded and the inhibition effect was calculated using the following formula. The experiment was conducted with three replicates.
Where FBCK represents fungal dry weight in control and FBCF represents fungal dry weight under CF treatment.
2.4. Effects of culture filtrate on mycelial morphology of Bipolaris sorokiniana
The mycelial pellets of B. sorokiniana were recovered and washed with 0.01 M phosphate buffered saline (PBS, pH 7.2) after treatment with CF (20% v/v) for 12 h. Mycelial pellets were stained with 0.4% trypan blue (C0040, Solarbio) for 3 min and observed by revolve fluorescence microscopy (Echo-Labs Revolve, United States). Moreover, the mycelia were prepared for observing by scanning electron microscopy (SEM) according to a previously reported method by Bahena-Garrido et al. (2019). First, the mycelia were fixed with 2.5% glutaraldehyde solution, dehydrated with 30–100% ethanol solution, and resuspended in tert-butyl alcohol. Next, the mycelial was sequentially treated with an ethanol and isoamyl acetate (V/V = 1/1) mixture and isoamyl acetate, followed by critical point drying. Mycelial morphology observation of B. sorokiniana was performed with a scanning electron microscope (Hitachi SU8010, Tokyo, Japan).
2.5. Determination of culture filtrate on cell membrane integrity in pathogenic mycelia
For investigating the potential of CF to induce cell death of B. sorokiniana, the mycelial cell membrane integrity was evaluated (Kim et al., 2017). Briefly, B. sorokiniana were incubated for an additional 24 h in PDB supplemented with the cell-free culture filtrate (1:1, v/v). The mycelial cell without treatment was set as control. Mycelia were harvested and stained with 10 μg/mL propidium iodide staining solution (PI, Biyuntian Biotech) at 25°C in the dark for 10 min. Fluorescence signals were detected using revolve fluorescence microscopy (Echo-Labs Revolve, United States). Each treatment was repeated three times.
Mycelia were inoculated into 150 mL PDB and incubated for 72 h. And the culture were supplemented with 20 and 10% CF (v/v) followed by cultivating for 0, 2, 4, 6, 8, 10, and 12 h, and cultures without CF served as control. The pathogenic fungi culture were collected after centrifuging (6,000 rpm) for 5 min. The conductivity of the pathogenic culture with different treatment time were measured to determine the cell membrane leakage of B. sorokiniana (Shao et al., 2015; Lin et al., 2018). The ergosterol content in mycelial plasma membrane of B. sorokiniana treated with CF (1:1 v/v) for 48 h was determined as previously described by Qin et al. (2022).
2.6. DNA damage assay
To assess the nuclear damage of B. sorokiniana, the mycelial cells were stained with 10 μL of 1 μg/mL 49, 6-diamidino-2-phenylindole (DAPI; 10 μg/mL in PBS; BiYunTian Biotechnology, China) for 15 min and observed with revolve fluorescence microscopy (Echo-Labs Revolve, United States). Meanwhile, extracellular DNA was also measured to reflect the cell content outflow of the CF treated mycelia for 0, 2, 4, 6, 8, 10, and 12 h. Briefly, an UV–visible spectroscopy spectrometer was used to measure the DNA content in the solution of each treatment group at 260 nm. Each treatment was repeated for three times.
2.7. Mitochondrial membrane potential assay
To detect the mitochondrial membrane potential of B. sorokiniana, mycelia were stained with 5,59,6,69-tetrachloro-1,19,3,39-tetraethylbenzimidazolocarbocyanine iodide (JC-1 dye; 20 μg/mL; Biyuntian Biotechnology, China) using the modified method described by Chen et al. (2021). After incubation for 12 h at 28°C with culture filtrate, mycelia were stained with JC-1 for 30 min in the dark and observed.
2.8. Intracellular reactive oxygen species accumulation assay
Accumulation of ROS in B. sorokiniana attributable to treatment with the culture filtrates for 12 h was determined by staining the mycelial cells with 20 μM 2′,7′-Dichlorodihydrofluorescein diacetate (DCFH-DA; Molecular Probes, Biyuntian, China) at 25°C in the dark for 30 min, then, washed with PBS three times and observed with revolve fluorescence microscopy (Echo-Labs Revolve, United States) after DCFH-DA removal.
2.9. Detection of hydrolase and PGP properties of Bacillus amyloliquefaciens DB2
Amylase, protease, and cellulase activities were determined by starch agar, skim milk agar, and carboxymethylcellulose agar, respectively (Waithaka et al., 2017; Khalil et al., 2021; Xu et al., 2022). Plant growth-promotion (PGP) tests include indole acetic acid (IAA) production, siderophores production, and phosphate solubilization (Shcherbakova et al., 2018; Lotfi et al., 2022; Mirskaya et al., 2022). The ability of bacterium to produce IAA was determined by Salkowski’s colorimetric method. After incubation, the broth was centrifuged and mixed with Salkowski reagent to observe the development of pink color. The bacterium was incubated at 37°C for 7 days and change of the blue color in the medium surrounding the colony was scored as positive for production of siderophores. For determining the inorganic or organic phosphate solubilization capacity, the bacterium was inoculated on the Pikovskaya agar containing tricalcium phosphate. After 7 days of incubation at 37°C, the formation of clearing zones was evaluated and indicated the utilization of tricalcium phosphate present in the agar medium.
2.10. Biocontrol effects of culture filtrate in greenhouse experiments
The control effect of CF on detached leaves of wheat seedlings (Zhengmai 366) was evaluated according to the method by Mishra et al. (2014). Seeds of wheat were soaked in 1% sodium hypochlorite for 15 min, rinsed with sterile water for five times. Seed germination was placed in Petri dishes containing moist filter paper and performed in the dark at 26°C for 24 h. The germinated seeds were sowed in a sterile plastic basin (110 mm × 80 mm), and cultured at 25 ± 2°C, with the relative humidity of 50 ± 5% and the light: dark (L:D) ratio of 14:10 h under the greenhouse. When the wheat leaves grew to one leaf and one terminal bud stage, the leaves (6 cm length) were cut off and soaked in 10 mL liquid as (1) sterile water, (2) CF of DB2, and (3) 50% triadimefon at Petri dishes for 1 h, respectively. Subsequently, 6-mm-diameter plugs from fresh cultures of B. sorokiniana were inoculated onto leaves. There were six leaves on each plate and three plates in each treatment. Disease severity was inferred from the lesion size on the leaves after 5 days of infection.
The potted plants control assay was performed under the greenhouse conditions using wheat Zhengmai 366. 25 seeds were sown in each pot. The germination conditions were same as described above in detached leaves control tests. When the wheat grew to one leaf and one terminal bud stage, pathogenic conidial suspension (106 conidia/mL) was prepared in sterile water and inoculated on potted wheat. After 7 days, wheat roots in each pot were irrigated with 20 mL CF. 50% triadimefonl (positive control), and sterile water (negative control). Every treatment include four pots and the biocontrol experiment conducted thrice.
Disease severity was evaluated when the leaves in the control group were fully infected, and followed the scoring standard by Yi et al. (2021). The disease incidence rate (DIR), disease index (DI), and control efficacy (CE) were using the following formulas:
Where n is the number of infected plants, N is the total number of investigated plants.
Where Ni denotes the number of infected plants of a certain severity, i denotes a certain severity, and N is the total number of investigated plants.
Where DICK indicates the disease index of the control group, and DIT indicates the disease index of the treatment group.
2.11. Growth-promotion for wheat under greenhouse
The plant growth-promoting efficacy of DB2 strain on wheat was hydroponically cultured in a greenhouse. In a short, the wheat seeds were surface sterilized with 1% sodium hypochlorite for 15 min and washed five times with sterilized distilled water. Suspension of DB2 was prepared with sterile water and adjusted to different concentrations (106, 107, and 108 CFU/mL). 25 wheat seeds were placed in 12 mm culture dishes in incubator at 26 ± 2°C, with the light:dark (L:D) ratio of 14:10 h. The seedlings were randomly divided into six groups and irrigated with 10 mL of LB medium, sterile water, culture filtrate, and DB2 suspension (106, 107, and 108 CFU/mL) at roots, respectively. The experiments were conducted in a randomized complete block design with four culture dishes per treatment. The growth parameters of wheat seedlings were measured after 7 days, including plant height (mm), root length (mm), plant fresh weight, and dry weight (Yue et al., 2019). The experiment was repeated for three times.
2.12. Statistical analysis
Data were collected by Excel 2016 (Microsoft Corporation, United States) for calculating standard errors (SE) and standard deviations (SD). Meanwhile, ANOVA significance was analyzed through multiple comparisons with Duncan’s multiple range test using SPSS 22.0 (SPSS Inc., Chicago, IL). p < 0.05 indicates a significant difference.
3. Results
3.1. Identification of antagonistic strain DB2
A total of 80 culturable bacterial isolates were obtained from soil samples and examined for antagonistic activity to the B. sorokiniana in vitro. Among them, we found the strain DB2 demonstrated higher inhibitory activities against fungal growth. The colony morphologies showed strain DB2 is rod-shape and its surfaces are rough, opaque, and milky white. The physiological and biochemical characteristics of DB2 were summarized in Table 1. Nitrate reduction, citrate utilization, gram staining, glucose decomposition, and V-P tests are all positive. The catalase test and methyl red are negative.
The 16S rDNA sequence of strain DB2 were submitted to GenBank and got the accession number of MZ664342. A phylogenetic tree was constructed based on 16S r DNA sequences from different strains (Figure 1). The results showed that DB2 and B. amyloliquefaciens strain B4 (OM755768) were in the same minimum branch. Moreover, the homology of 16S rDNA sequence of DB2 and B. amyloliquefaciens strain MPA (NR117946) was 99% according to the phylogenetic tree. Based on physiological, biochemical and molecular identification results, the strain DB2 was identified as B. amyloliquefaciens DB2.
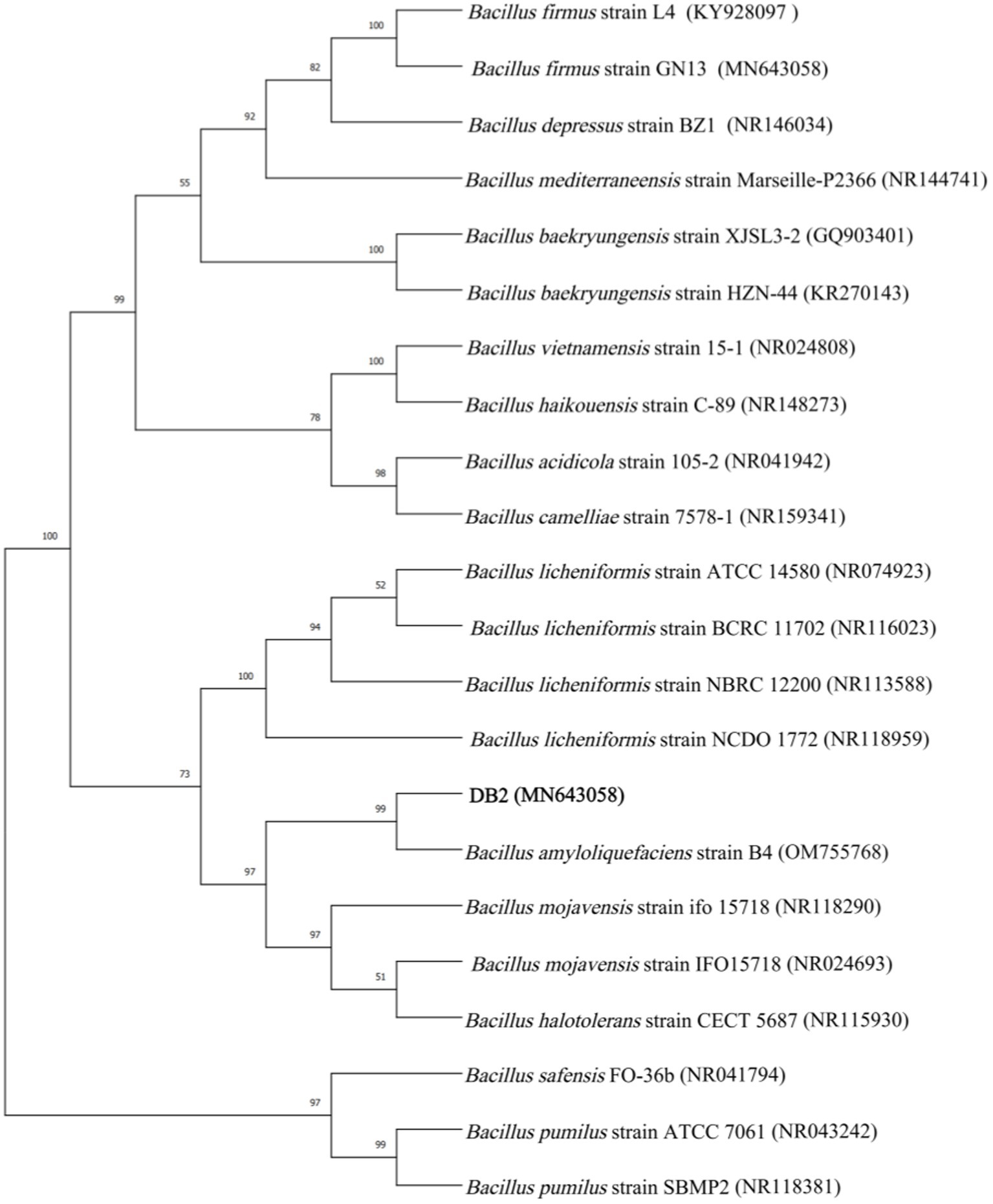
Figure 1. Phylogenetic tree of Bacillus amyloliquefaciens DB2 constructed on 16S rDNA sequence. The analysis was performed using the soft MEGA 11.0.
3.2. In vitro inhibition of culture filtrate on Bipolaris sorokiniana
Microscopic observation showed the spores of B. sorokiniana appeared to be intact and grew normally in control group, whereas CF treatment resulted in abnormal germination of B. sorokiniana spores with round and vacuolar shape (Figure 2A). Furthermore, the CF reduced the mycelial dry weight of B. sorokiniana from 13.1 ± 1.5 to 0.96 ± 0.16 mg (Figure 2B), and mycelial growth inhibition rate was 92.67% (Figure 2C). Thus, CF can significantly inhibit the growth of mycelia of B. sorokiniana.
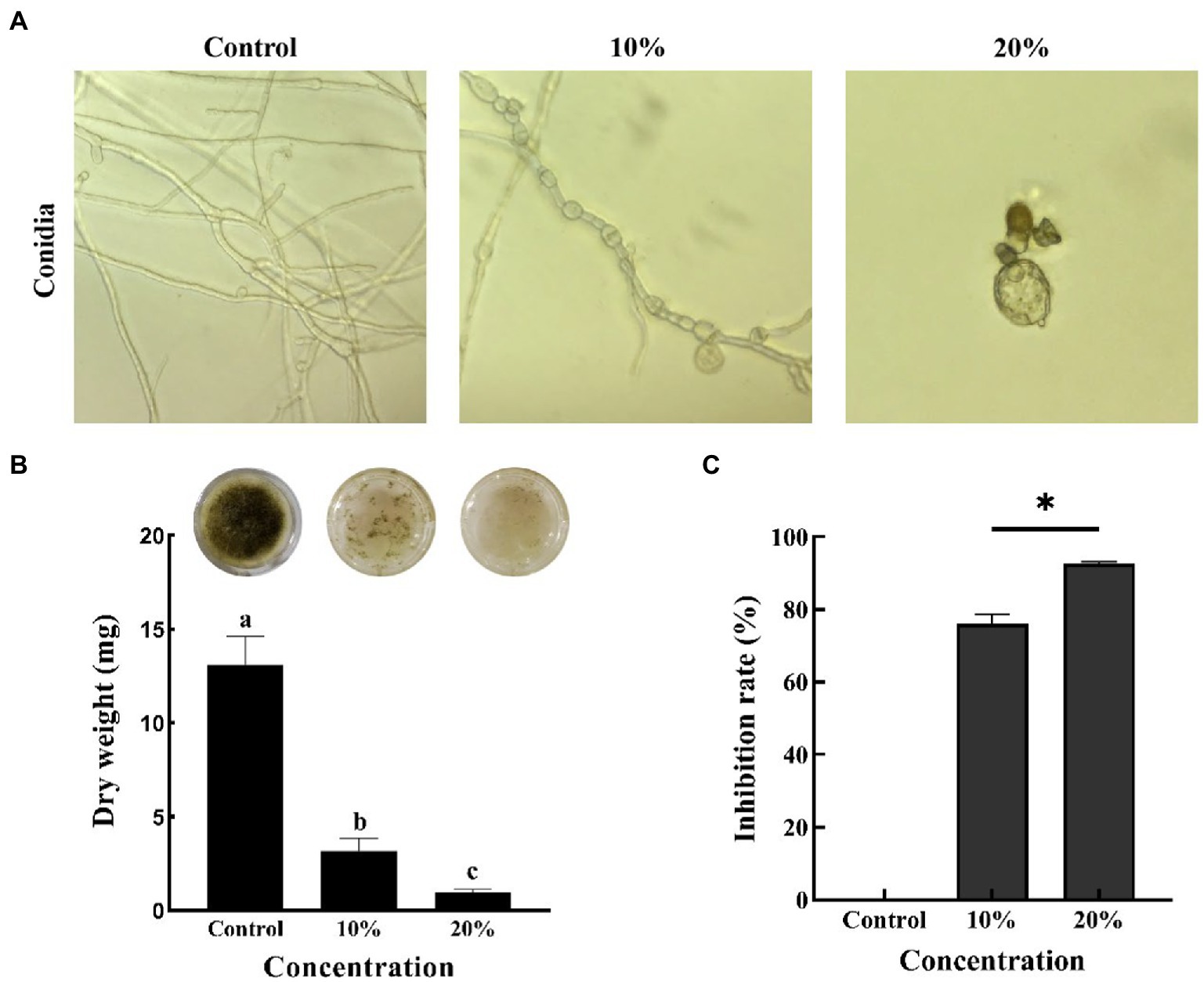
Figure 2. Inhibition of cell-free culture filtrate with different concentrations on Bipolaris sorokiniana. (A) Effect of cell-free culture filtrate on conidial germination of Bipolaris sorokiniana. (B) Effect of cell-free culture filtrate on dry weight of mycelium. (C) Inhibition rate of cell-free culture filtrate against Bipolaris sorokiniana. Error bars represent the standard deviation (n = 3). Different letters and * indicated a significant difference at the level of p < 0.05.
3.3. Mycelial morphological changes of Bipolaris sorokiniana
The mycelia of B. sorokiniana treated with CF exhibited swollen and bulbous-like. Cell death was further investigated using trypan blue, which accumulated in dead cells. The swollen mycelia were filled with the blue dye (Figure 3A). However, the mycelia in the control were normal, well organized and not showed color changed. SEM observation showed the mycelial damage with CF treatment. The mycelia in the control group were normal and the surface was smooth, plump, and integrity. In the treatment group, the mycelia were loose, shrunken, and collapsed (Figure 3B). Overall, SEM and light microscope observation of the mycelial morphology was changed severely.
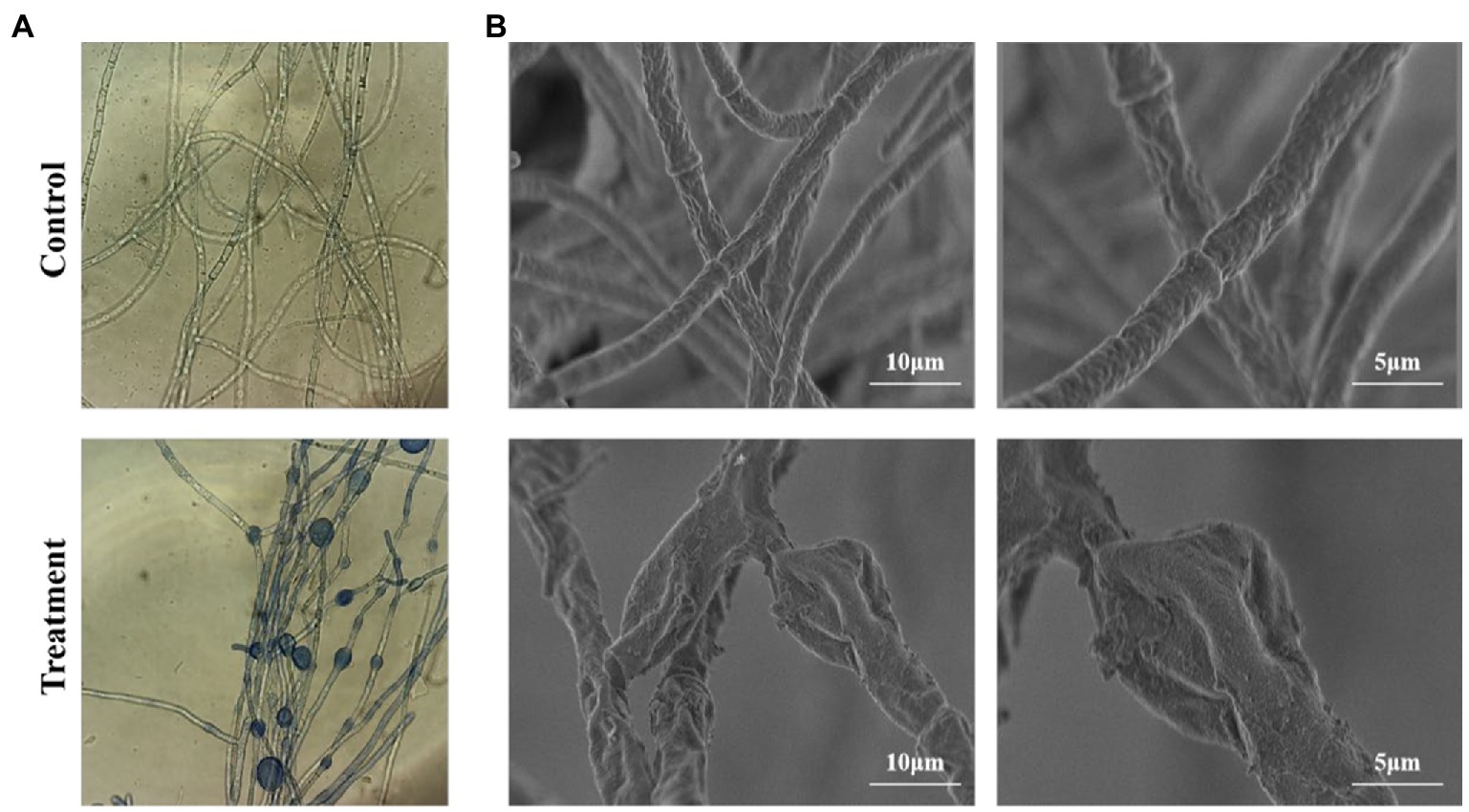
Figure 3. Effect of cell-free culture filtrate on the morphology of Bipolaris sorokiniana. (A) Mycelium morphology under optical microscope (×20), blue color represents dead fungi cells. (B) Mycelium ultrastructure under scanning electron microscope, scale bars = 10 and 5 μm. Control: untreated control group; Treatment: treated with cell-free culture filtrate produced by Bacillus amyloliquefaciens DB2.
3.4. Effects of CF on membrane integrity of Bipolaris sorokiniana
To investigate the possible mode of antifungal action of CF against B. sorokiniana, plasma membrane and the conductivity of B. sorokiniana were determined to assess membrane integrity and permeability. The PI-stained mycelia of B. sorokiniana exhibited more extensive red fluorescence in the CF treatment group than that in the control group (Figure 4A), indicating that CF treatment can lead to cell membrane injury. The ergosterol content was measured by UV-spectrophotometer through a unique spectral peak between 240 and 300 nm. The CF reduced ergosterol production significantly in both spectral peaks when compared to control (Figure 4B). CF have effectively inhibited fungal growth (biomass) by reducing ergosterol content. The extracellular conductivity in B. sorokiniana suspension was increased with exposure time and the concentrations of CF (Figure 4C). Moreover, the extracellular conductivity increased with the incubation time during the first 8 h. Afterward, the growth tended to slow down. These results showed that the membrane integrity of B. sorokiniana was damaged by CF.
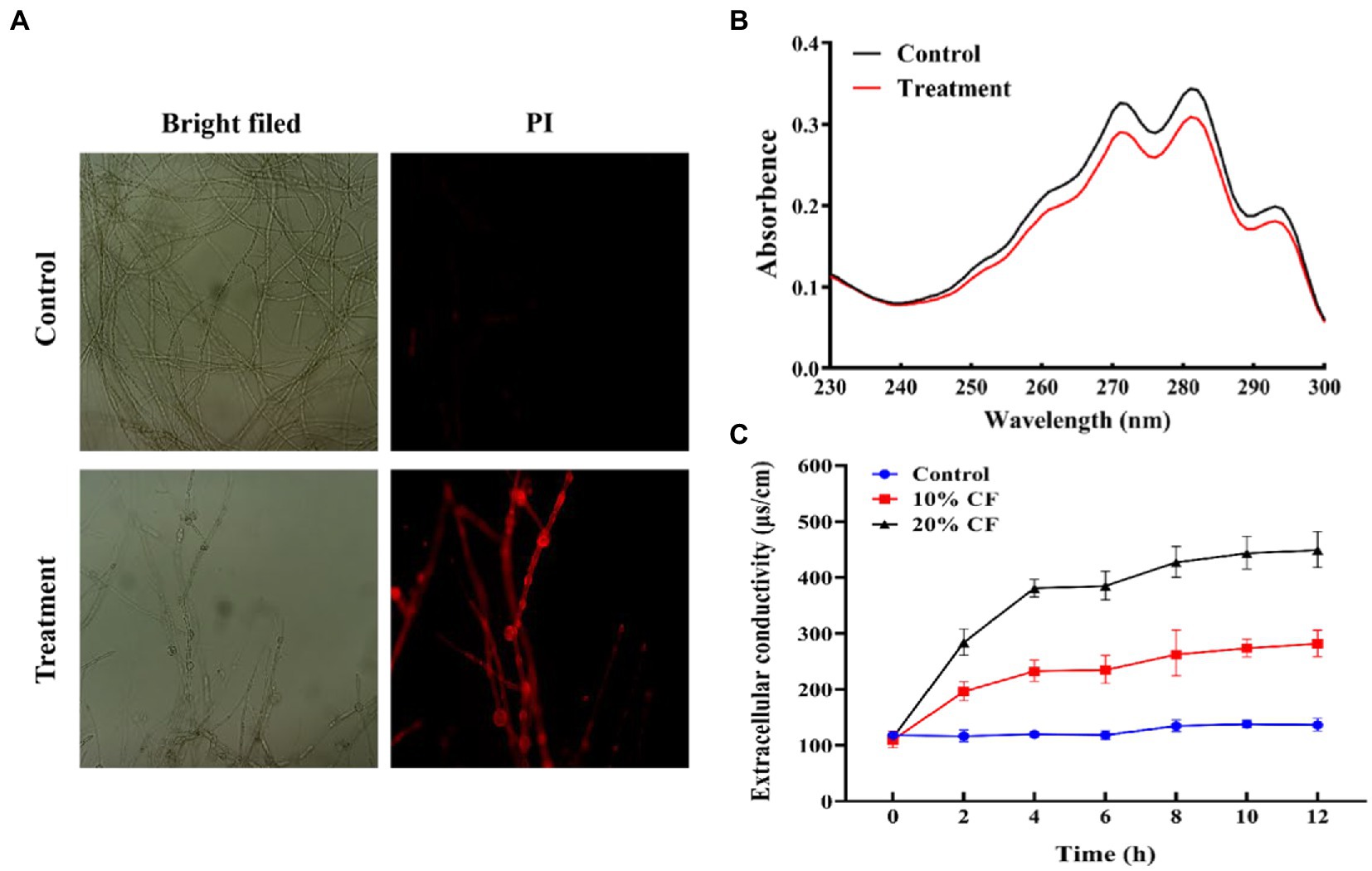
Figure 4. Effect of cell-free culture filtrate on membrane integrity of Bipolaris sorokiniana mycelium. (A) Fluorescence of mycelia stained with PI measured by revolve fluorescence microscopy (×20). (B) Ultraviolet scanning spectra of ergosterol content in the mycelium plasma membrane treated with cell-free culture filtrate. (C) Effects of cell-free culture filtrate on the electric conductivity of mycelium. Values are presented as mean ± SE (n = 3).
3.5. Effects of CF on DNA fragmentation
To further explore the inhibition mechanism of CF, the DAPI staining assays were performed to observe DNA and nuclear morphology. The mycelia treated with CF exhibited presenting diffuse nuclear staining compared with untreated mycelia (Figure 5A). These findings suggested that CF treatment damaged DNA in B. sorokiniana mycelia. After incubation of culture filtrate with B. sorokiana for 12 h, the OD260 of the centrifuged mycelial suspension was significantly higher than that of the control group (Figure 5B). The longer the incubation time with the culture filtrate was, the higher the OD260 value of the centrifuged mycelial suspension, indicating that the cell membrane integrity of B. sorokiniana was greatly damaged, which could consequently lead to high leakage of nucleic acids.
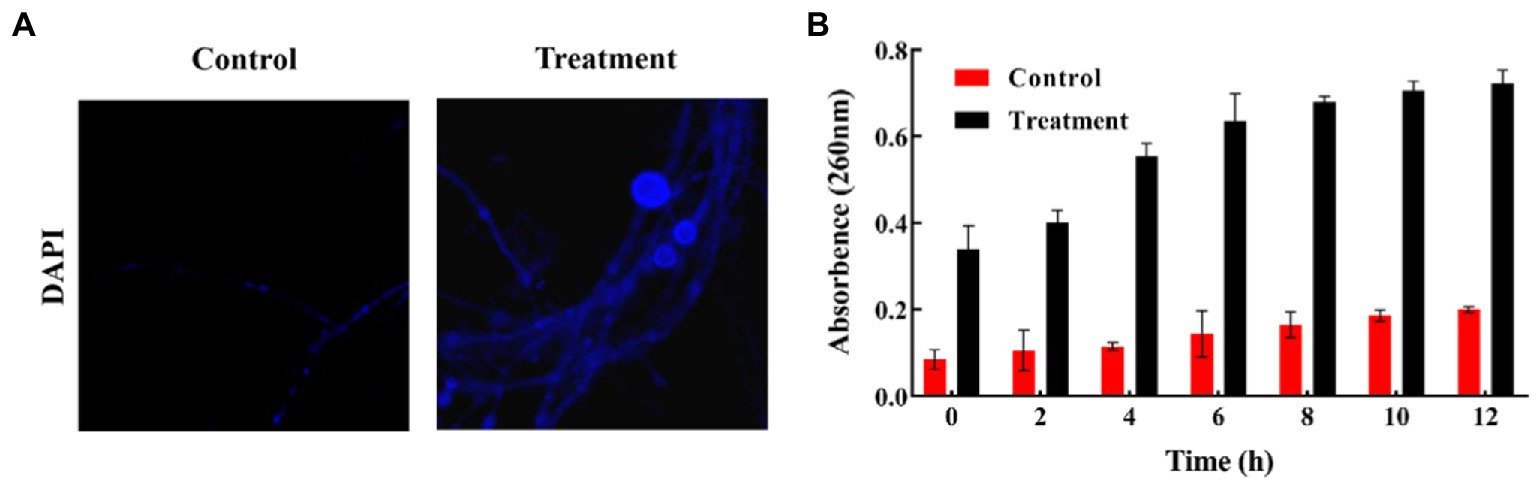
Figure 5. Effect of cell-free culture filtrate on the DNA fragmentation and leackages in Bipolaris sorokiniana cells. (A) cell-free culture filtrate produced by Bacillus amyloliquefaciens DB2 induced chromatin condensation in hyphal cells (×20). (B) Effects of cell-free culture filtrate on the nucleic acids of mycelium. Values are presented as mean ± SE (n = 3).
3.6. Effects of CF on mitochondrial membrane potential of Bipolaris sorokiniana
To detect mitochondrial damage of the mycelia, the signal of the JC-1 staining was detected using fluorescence microscopy. The red fluorescence decreased and green fluorescence increased when mitochondrial damage occurs. The results showed that red fluorescence was aggregated in the untreated mycelia, whereas the cells treated with the CF exhibited a green color, suggesting that the CF caused reductions in the mitochondrial membrane potential of B. sorokiniana (Figure 6A).
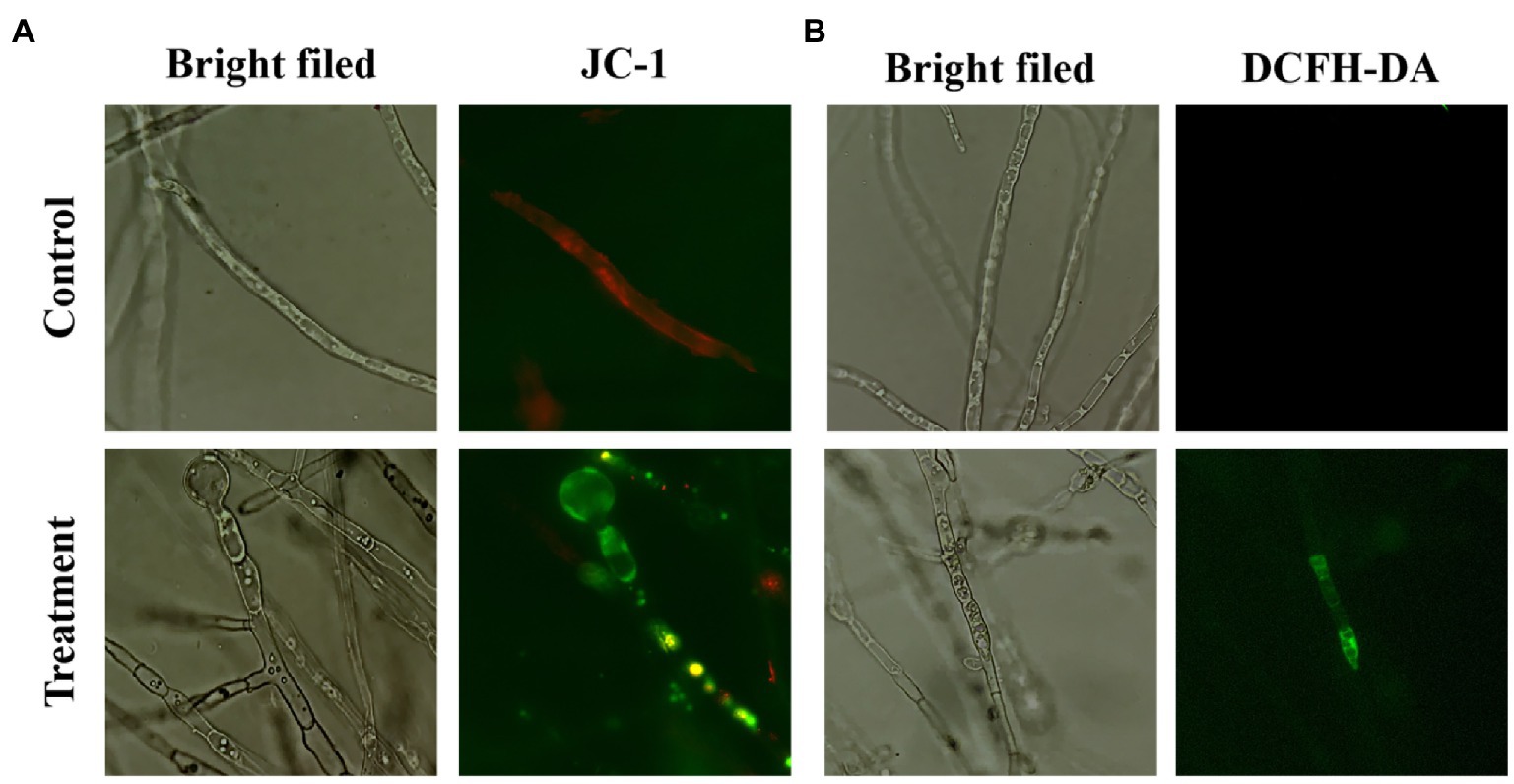
Figure 6. Effect of cell-free culture filtrate induced alteration of mitochondrial membrane potential (MMP) and reactive oxygen species (ROS) generation in Bacillus amyloliquefaciens cells. (A) Effect of cell-free culture filtrate on mitochondrial membrane potential of hyphal cells (×40). (B) Accumulation of reactive oxygen species in Bipolaris sorokiniana treated with cell-free culture filtrate. Cells were stained with DCFH-DA and observed with a revolve fluorescence microscopy (×40). PDB medium was used as a control, cell-free culture filtrate treated was used as a treatment.
3.7. Effects of CF on accumulation of ROS in Bipolaris sorokiniana
The burst generation of ROS could not only damage the cellular compound such as DNA and enzymes, but also increase the cell membrane permeability and lead to membrane structural damage. DCFH-DA was used to evaluate the level of oxidative stress of mycelia after different treatments. Fluorescence intensity represents the accumulation of ROS. The green fluorescence was observed in the CF-treated cells but not in the untreated cells, indicating that the accumulation of ROS was induced by the CF of B. amyloliquefaciens DB2 (Figure 6B).
3.8. Analysis of hydrolase and PGP traits of Bacillus amyloliquefaciens DB2
Antifungal substance determination showed that strain DB2 could produce amylase and protease due to the presence of a clear zone in a special medium. The absence of transparent halos on the test plates for cellulose indicated this strain was incapable to produce such enzymes (Figure 7). Plant growth-promoting (PGP) traits detection indicated B. amyloliquefaciens DB2 was able to produce siderophore and IAA, while not to solubilize phosphate (Figure 7).
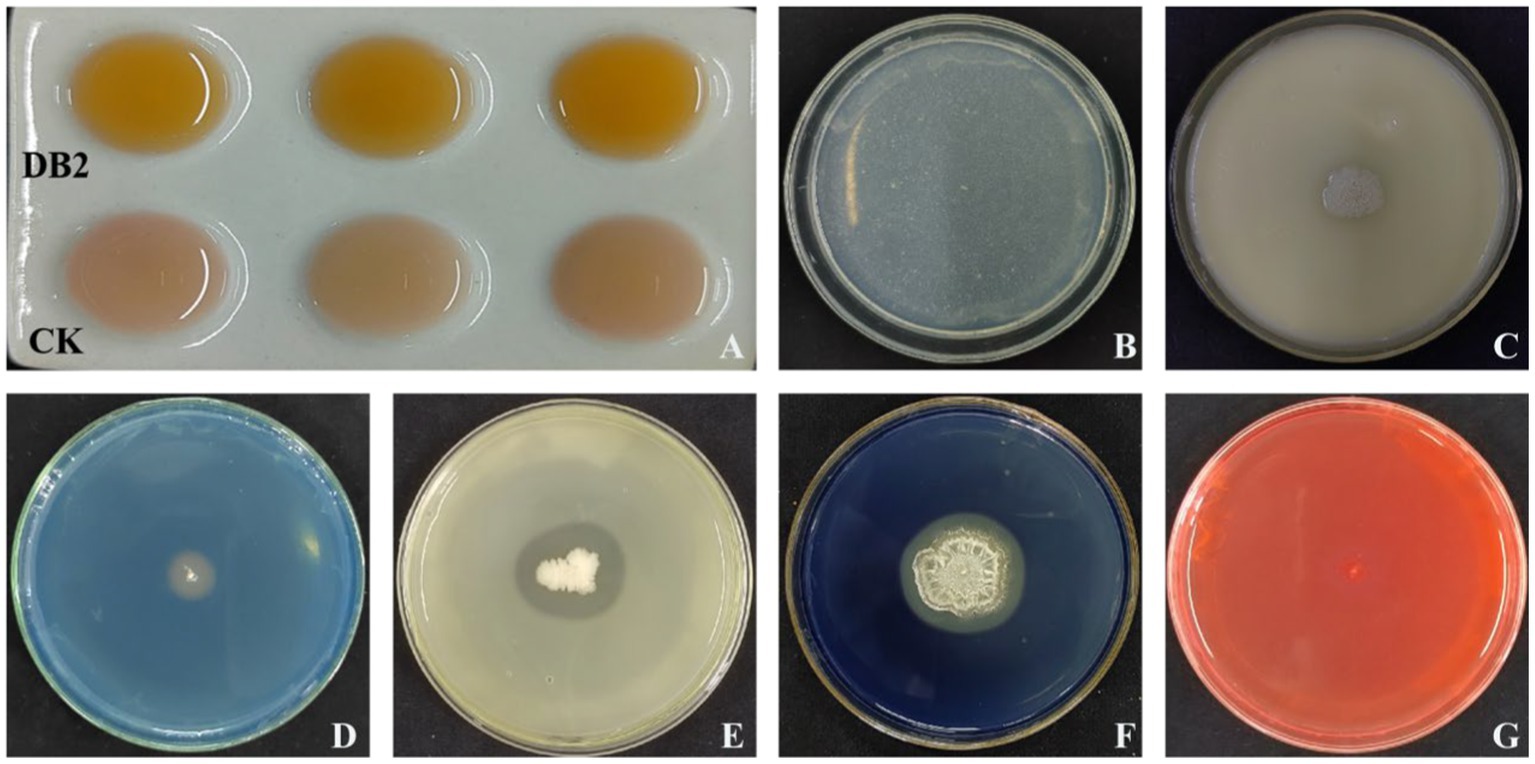
Figure 7. PGP and antifungal traits of strain DB2. (A) The upper indicating IAA production by color change. (B) The strain DB2 was negative on the inorganic phosphate solubilization test by no halo zone around colonies. (C) The strain DB2 was negative on the organic phosphate solubilization test by no halo zone around colonies. (D) Yellow-orange halos indicating siderophore production. (E) Protease activity indicated by a clear zone around strain DB2 colonies. (F) Amylase activity indicated by an obvious hydrolytic zone around strain DB2 colonies. (G) The strain was negative for cellulase activity by no zone of hydrolysis around strain DB2.
3.9. Biocontrol effects of strain DB2 on common root rot of wheat
The biocontrol efficacy of strain DB2 against B. sorokiniana was evaluated using detached leaf and potted wheat control assay. At fifth day after infection, we evaluated the presence and area of necrotic lesions on wheat leaves. In the group inoculated with conidial suspension of B. sorokiniana alone, leaves showed the characteristic disease symptom of spot blotch. However, the CF and the triadimefon could significantly reduce the lesion area which appeared to be as healthy as control leaves (Figure 8). In the potted plants experiment, the visible disease incidence was observed in positive control. In CF and triadimefon treatment groups, disease incidence was significantly reduced with 35.20 and 39.20%, and the biocontrol efficacy reached 75.22 and 82.56%, respectively (Table 2). In summary, CF of amyloliquefaciens DB2 showed significantly control on common root rot of wheat.
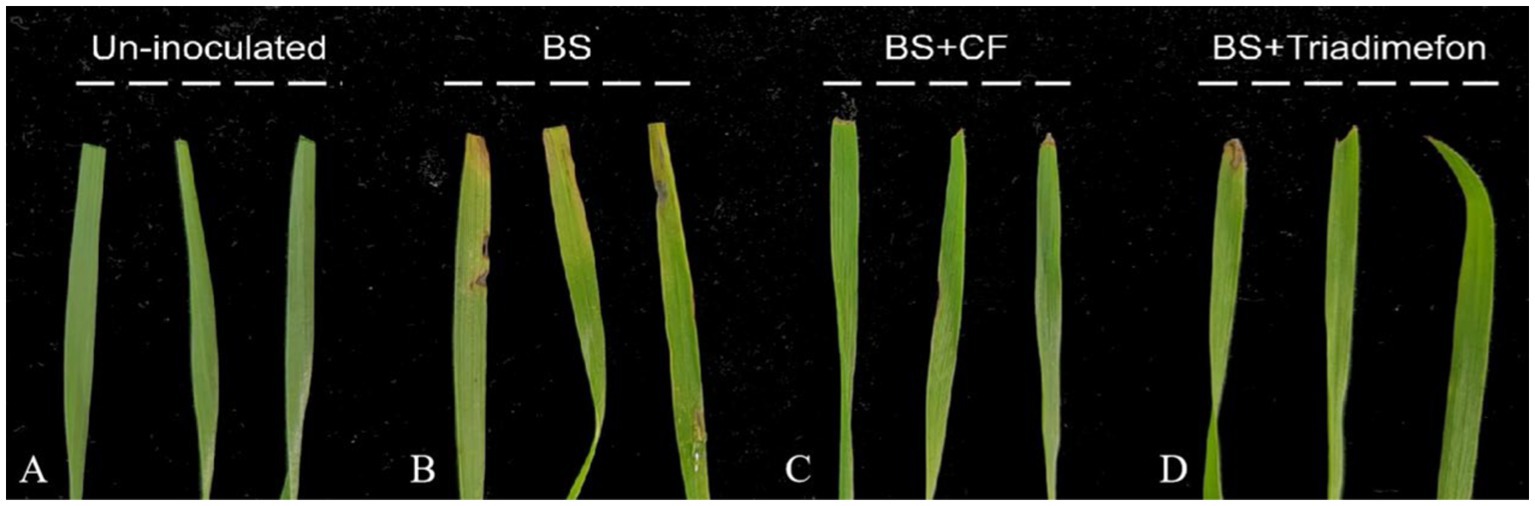
Figure 8. Control effect of DB2 on spot blotch in a detached leaf assay. (A) Sterile water treatment (Un-inoculated). (B) Only Bipolaris sorokiniana (BS) inoculation without cell-free culture filtrate (CF) treatment. (C) BS + cell-free culture filtrate treatment. (D) BS + Triadimefon treatment.
3.10. Plant growth promotion action of strain DB2 for wheat seedlings
The performance of the wheat root was far better than the control when bio-primed with the B. amyloliquefaciens DB2 suspensions. For wheat seeds, soaking with DB2 suspensions at different concentrations of 106, 107, and 108 CFU/mL significantly promoted the growth of wheat seedlings (Table 3). Compared to the sterile water treatment group (control), 108 CFU/mL DB2 suspension treatment increased the height, root length, fresh weight, and dry weight of wheat seedlings by 34.12, 90.27, 48.11, and 39.81%, respectively. In addition, LB liquid medium treatment caused a significant reduction in wheat growth including plant height and root length as compared to water treatment group. Interestingly, CF of B. amyloliquefaciens DB2 alleviated the impact of LB medium stress symptoms on the growth of wheat seedlings (Table 3).
4. Discussion
Bipolaris sorokiniana is an important soil-borne plant pathogen causing root rot disease in various crops leading to agricultural economic losses. Biological control is considered as an environmentally-friendly, sustainable, and effective approach to control plant diseases. In the present study, the strain DB2 was isolated from the rhizosphere soil of wheat and identified as B. amyloliquefaciens based on its morphology and molecular characters. To further study the mechanism of B. amyloliquefaciens DB2 in controlling fungi, B. sorokiniana was used as an indicator. Here, we evaluate the antifungal activity of cell-free culture filtrate (CF) of B. amyloliquefaciens DB2 against B. sorokiniana. The results showed that CF could significantly inhibit the growth of mycelia and conidia germination, which reached an inhibition rate of 92.67%. This indicated that the antifungal activity of CF showed higher antagonists, which was similar to the results of Shemshura et al. (2020) Several lines of evidence suggest that Bacillus species can affect abnormalities in the mycelia of pathogens, causing cell wall lysis, breakage, granulation, and vacuolization (Chaurasia et al., 2005). Li et al. (2015) reported that bacteria-free filtrate from Bacillus megaterium strain L2 caused some of the germinal tubes to undergo vacuolization and even rupturing. A similar phenomenon was observed in B. sorokiniana after culture filtrate treatment. In this study, we found that the CF induced swollen, bulbous-like from the light microscope, and loose, shrunken, and collapsed in the surface of the mycelia under SEM.
In the investigation of the antifungal mechanism of B. amyloliquefaciens DB2 on B. sorokiniana at the cellular level, the CF could damage the mycelial cell membrane of B. sorokiniana. In this study, the data indicated that the CF can disrupt cell membranes resulting in PI staining accumulating in necrotic mycelial cells. Due to the disruption of CF, ergosterol content decreased and electrical conductivity increased by comparison with the control group. Thus, our results urther demonstrated that CF alters membrane permeability and integrity. Xu et al. (2019) has reported that the cell-free supernatant from B.amyloliquefaciens LZN01 caused the disruption of cell walls and membranes, the leakage of intracellular contents and the aggregation of organelles in Fusarium oxysporum. The changes in mitochondrial membrane potential (MMP) could play a role in the induction of cell metabolism. At the present study, the MMP of B. sorokiniana declined in mitochondrial function leading to enhanced ROS production. Next, the accumulation of excess ROS leads to damage to intracellular DNA and the efflux from cell membranes. Wang et al. (2020) has reported Iturin A extracted from Bacillus subtilis WL-2 affected phytophthora infestans triggering oxidative stress reactions and causing mitochondrial damage, including MMP. According to the previous study, the CF not only induced membrane damage, a disrupting membrane potential but also by interfering with energy metabolism in B. sorokiniana. These results indicated the biocontrol mechanism of strain DB2 was inducing cell death by disrupting the mycelial cell membrane of B. sorokiniana and affecting intracellular metabolic processes.
Previous studies reported B. amyloliquefaciens played a vital role in the crop protection and plant growth promotion (Yuan et al., 2012; El-Gremi et al., 2017). The plant growth promoting ability was also used as a second alternative or complementary means for plant protection. Our strain DB2 produced indole-3-acetic acid (IAA), siderophores, and major enzymes such as protease, amylase. Indole-3-acetic acid (IAA) can stimulate cell proliferation and elongation of plant roots to regulate the growth of plant roots (Gupta and Pandey, 2019). In addition, strain DB2 showed the ability to produce siderophores. It is common that siderophore producing bacteria could supplement iron to the plant even prevent the growth of the soil-borne pathogens (Hilgers et al., 2021). Generally, the bio-control bacteria can interact with pathogens through a series of mechanisms, including the production of antibiotics and lytic enzymes (Ben Slama et al., 2019). In a previous study, B. amyloliquefaciens SQR9 was reported as a growth-promoting activity in greenhouse experiments including indole-3-acetic acid (IAA) production and extracellular phytase (Shao et al., 2015). And B. amyloliquefaciens QSB-6 have a good inhibitory effect on Fusarium in the soil and can significantly promote plant root growth (Duan et al., 2021).
To further evaluate the ability of DB2 to control diseases, a detached wheat leaf assay was conducted. We found our strain had strong inhibitory activity against B. sorokiniana on wheat leaf. Moreover, the bio-control efficiency of the CF was further assessed in a pot experiment. It proved that preventive treatment result was very efficient in coping with the development of the infection by the B. sorokiniana. The other previous reports had studied the Bacillus strains can use to overcome common root rot diseases (Campanella et al., 2020; Villa-Rodriguez et al., 2021). Our strain DB2 has good application potential in the sustainable development of the agriculture.
During wheat seedling growth, B. amyloliquefaciens strain DB2 suspensions were found to enhance the growth of plants compared with the control. The preliminary experiments were performed with wheat inoculated with DB2 suspensions planted in the greenhouse. These wheat seedings were more developed than noinoculated ones, particularly in the root system. It has proved that our strain can increased plant and root length, plant fresh and dry weight. Furthermore, the culture filtrate also alleviated the unfavorable environmental conditions in LB medium. We tentatively speculate that this may be associated with the containing plant growth promoting substances in culture filtrate.
Overall, antagonistic strain B. amyloliquefaciens DB2 could be taken as the potential BCAs for B. sorokiniana control. It is necessary to reveal the mechanism of biocontrol bacteria on plant diseases for developing effective BCAs. The underlying action mechanisms are concluded including apoptosis by disrupting the cell membrane integrity, decreasing the MMP and accumulation of ROS in the mycelia cell, thereby inhibiting the growth of pathogen, and producing of growth-promoting substances for wheat growth and health (Figure 9). For better defining its direction of action, purification and identification of the antifungal and growth-promoting substances are necessary to determine to further study the specific effects on the pathogen.
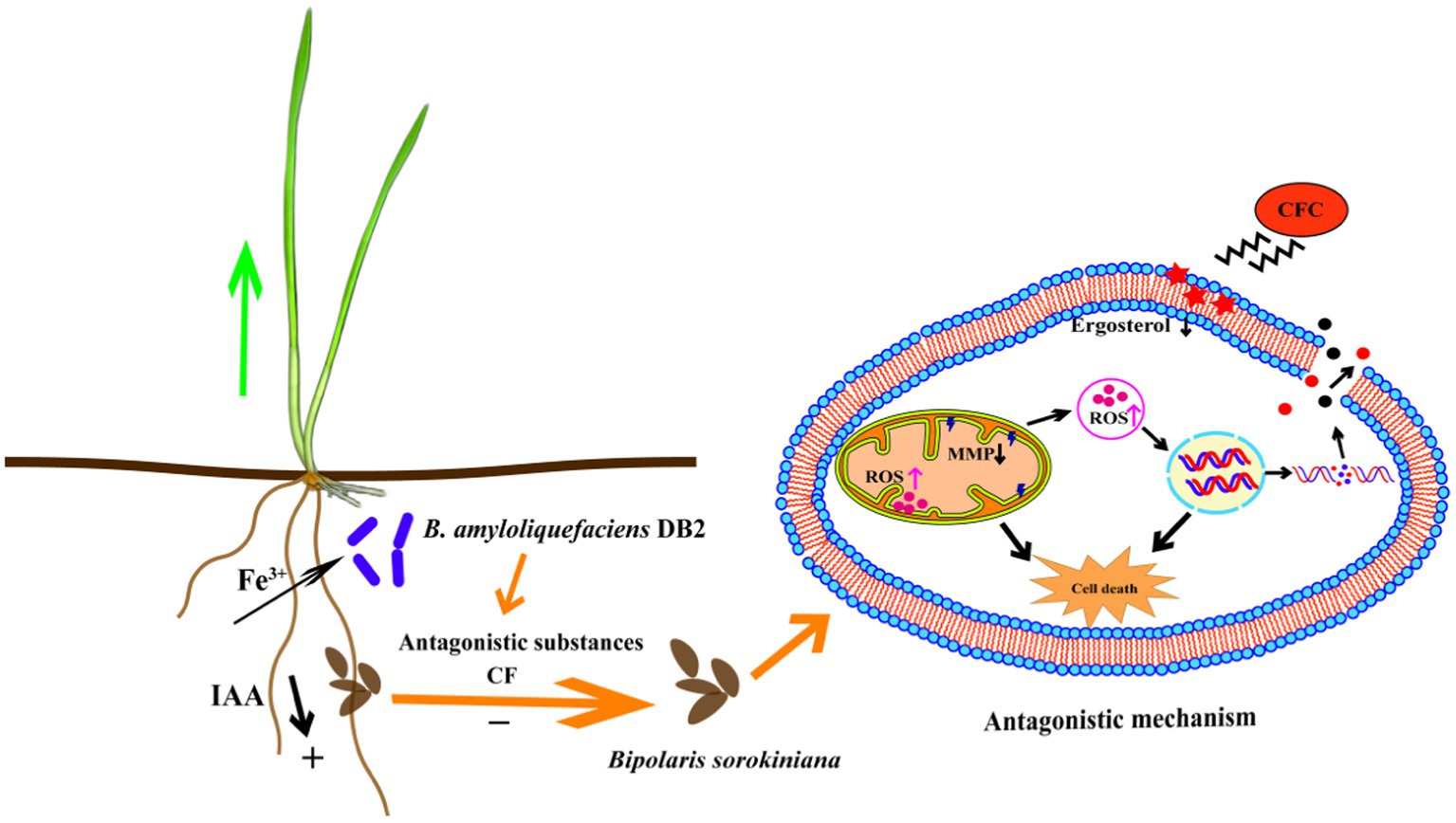
Figure 9. Proposed model showing that Bacillus amyloliquefaciens DB2 can promote wheat seedlings growth and underlying mechanisms of antagonistic strain DB2 against Bipolaris sorokiniana.
5. Conclusion
In the current study, we investigated a biocontrol strain that could be used as an alternative agent for controlling B. sorokiniana, and the experiment results also enhanced our comprehensive understanding toward the possible antifungal mechanisms of the CF of B. amyloliquefaciens DB2. In vitro antagonistic assay showed that culture filtrate of the bioactive bacterium could disrupt the cell membrane integrity, decrease the MMP and accumulate ROS in the mycelia cell to inhibit the growth of fungal pathogen. The strain DB2 could produce extracellular enzymes, protease, amylase, siderophore and IAA. Furthermore, it is evident that the culture filtrate successfully suppressed or reduced disease symptoms and the strain DB2 suspension had a significant promotion of wheat seedlings growth. So, it may be concluded that the strain B. amyloliquefaciens DB2 could be utilized for biocontrol management (B. sorokiniana) program in the sustainable development of the agriculture.
Data availability statement
The datasets presented in this study can be found in online repositories. The names of the repository/repositories and accession number(s) can be found at: https://www.ncbi.nlm.nih.gov/, MZ664342.1.
Author contributions
PL: conceptualization, methodology, data curation, formal analysis, and writing original draft. YY: conceptualization, funding acquisition, project administration, resources, supervision, and writing and revising. YH, LZ, XR, and SJ: methodology, investigation, and validation. LC, ZH, and YL: data curation and formal analysis. All authors contributed to the article and approved the submitted version.
Funding
This work was supported by the Henan Provincial Science and Technology Major Project (221100110100), China Agriculture Research System of MOF and MARA (CARS-03), and the Innovative Funds Plan of Henan University of Technology (2020ZKCJ23).
Conflict of interest
The authors declare that the research was conducted in the absence of any commercial or financial relationships that could be construed as a potential conflict of interest.
Publisher’s note
All claims expressed in this article are solely those of the authors and do not necessarily represent those of their affiliated organizations, or those of the publisher, the editors and the reviewers. Any product that may be evaluated in this article, or claim that may be made by its manufacturer, is not guaranteed or endorsed by the publisher.
Footnotes
References
Aggarwall, R., Tewari, A. K., Srivastava, K. D., and Singh, D. V. (2004). Role of antibiosis in the biological control of spot blotch (Cochliobolus sativus) of wheat by Chaetomium globosum. Mycopathologia 157, 369–377. doi: 10.1023/B:MYCO.0000030446.86370.14
Al-Sadi, A. M. (2021). Bipolaris sorokiniana-induced black point, common root rot, and spot blotch diseases of wheat: a review. Front. Cell. Infect. Microbiol. 11:584899. doi: 10.3389/fcimb.2021.584899
Awan, Z. A., Shoaib, A., Iftikhar, M. S., Jan, B. L., and Ahmad, P. (2022). Combining biocontrol agent with plant nutrients for integrated control of tomato early blight through the modulation of physio-chemical attributes and key antioxidants. Front. Microbiol. 13:807699. doi: 10.3389/fmicb.2022.807699
Bahena-Garrido, S. M., Ohama, T., Suehiro, Y., Hata, Y., Isogai, A., Iwashita, K., et al. (2019). The potential aroma and flavor compounds in Vitis sp. cv. Koshu and V. vinifera L. cv. Chardonnay under different environmental conditions. J. Sci. Food Agric. 99, 1926–1937. doi: 10.1002/jsfa.9389
Bai, H., Liu, X., Chenzhang, P., Xiao, Y., Fu, B., and Qin, Z. (2020). Design, synthesis and fungicidal activity of new 1,2,4-Triazole derivatives containing oxime ether and Phenoxyl Pyridinyl moiety. Molecules 24:5852. doi: 10.3390/molecules25245852
Belbahri, L., Chenari Bouket, A., Rekik, I., Alenezi, F. N., Vallat, A., Luptakova, L., et al. (2017). Comparative genomics of bacillus amyloliquefaciens strains reveals a Core genome with traits for habitat adaptation and a secondary metabolites rich accessory genome. Front. Microbiol. 8:1438. doi: 10.3389/fmicb.2017.01438
Ben Slama, H., Triki, M. A., Chenari Bouket, A., Ben Mefteh, F., Alenezi, F. N., Luptakova, L., et al. (2019). Screening of the high-rhizosphere competent Limoniastrum monopetalum’ Culturable endophyte microbiota allows the recovery of multifaceted and versatile biocontrol agents. Microorganisms 7:249. doi: 10.3390/microorganisms7080249
Bergey, D. H. (1994). Bergey’s Manual of Determinative Bacteriology. Philadelphia: Lippincott Williams & Wilkins Press.
Campanella, V., Mandalà, C., Angileri, V., and Miceli, C. (2020). Management of common root rot and fusarium foot rot of wheat using Brassica carinata break crop green manure. Crop Prot. 130:105073. doi: 10.1016/j.cropro.2019.105073
Chaurasia, B., Pandey, A., Palni, L. M., Trivedi, P., Kumar, B., and Colvin, N. (2005). Diffusible and volatile compounds produced by an antagonistic Bacillus subtilis strain cause structural deformations in pathogenic fungi in vitro. Microbiol. Res. 160, 75–81. doi: 10.1016/j.micres.2004.09.013
Chen, Y. H., Lee, P. C., and Huang, T. P. (2021). Biological control of collar rot on passion fruits via induction of apoptosis in the collar rot pathogen by Bacillus subtilis. Phytopathology 111, 627–638. doi: 10.1094/PHYTO-02-20-0044-R
Cohen, S. P., and Leach, J. E. (2020). High temperature-induced plant disease susceptibility: more than the sum of its parts. Curr. Opin. Plant Biol. 56, 235–241. doi: 10.1016/j.pbi.2020.02.008
Duan, Y., Chen, R., Zhang, R., Jiang, W., Chen, X., Yin, C., et al. (2021). Isolation, identification, and antibacterial mechanisms of Bacillus amyloliquefaciens QSB-6 and its effect on plant roots. Front. Microbiol. 12:746799. doi: 10.3389/fmicb.2021.746799
El-Gremi, S. M., Draz, I. S., and Youssef, W. A. E. (2017). Biological control of pathogens associated with kernel black point disease of wheat. Crop Prot. 91, 13–19. doi: 10.1016/j.cropro.2016.08.034
Gupta, S., and Pandey, S. (2019). ACC deaminase producing bacteria with multifarious plant growth promoting traits alleviates salinity stress in French bean (Phaseolus vulgaris) plants. Front. Microbiol. 10:1506. doi: 10.3389/fmicb.2019.01506
Hanif, A., Zhang, F., Li, P., Li, C., Xu, Y., Zubair, M., et al. (2019). Fengycin produced by Bacillus amyloliquefaciens FZB42 inhibits fusarium graminearum growth and mycotoxins biosynthesis. Toxins 11:295. doi: 10.3390/toxins11050295
Hilgers, F., Habash, S. S., Loeschcke, A., Ackermann, Y. S., Neumann, S., Heck, A., et al. (2021). Heterologous production of beta-Caryophyllene and evaluation of its activity against plant pathogenic fungi. Microorganisms 9:168. doi: 10.3390/microorganisms9010168
Hu, J., Zheng, M., Dang, S., Shi, M., Zhang, J., and Li, Y. (2021). Biocontrol potential of Bacillus amyloliquefaciens LYZ69 against anthracnose of alfalfa (Medicago sativa). Phytopathology 111, 1338–1348. doi: 10.1094/PHYTO-09-20-0385-R
Jin, P., Wang, H., Liu, W., and Miao, W. (2017). Characterization of lpaH2 gene corresponding to lipopeptide synthesis in Bacillus amyloliquefaciens HAB-2. BMC Microbiol. 17:227. doi: 10.1186/s12866-017-1134-z
Kaur, J., Kaur, J., Dhillon, G. S., Kaur, H., Singh, J., Bala, R., et al. (2021). Characterization and mapping of spot blotch in Triticum durum-Aegilops speltoides introgression lines using SNP markers. Front. Plant Sci. 12:650400. doi: 10.3389/fpls.2021.650400
Khalil, M. M. R., Fierro-Coronado, R. A., Penuelas-Rubio, O., Villa-Lerma, A. G., Plascencia-Jatomea, R., Felix-Gastelum, R., et al. (2021). Rhizospheric bacteria as potential biocontrol agents against Fusarium wilt and crown and root rot diseases in tomato. Saudi J. Biol. Sci. 28, 7460–7471. doi: 10.1016/j.sjbs.2021.08.043
Kim, K., Lee, Y., Ha, A., Kim, J. I., Park, A. R., Yu, N. H., et al. (2017). Chemosensitization of Fusarium graminearum to chemical fungicides using cyclic Lipopeptides produced by Bacillus amyloliquefaciens strain JCK-12. Front. Plant Sci. 8:2010. doi: 10.3389/fpls.2017.02010
Knudsen, I. M. B., Hockenhull, J., and Jensen, D. F. (1995). Biocontrol of seedling diseases of barley and wheat caused by fusarium culmorum and Bipolaris sorokiniana: effects of selected fungal antagonists on growth and yield components. Plant Pathol. 44, 467–477. doi: 10.1111/j.1365-3059.1995.tb01669.x
Kumar, J., Huckelhoven, R., Beckhove, U., Nagarajan, S., and Kogel, K. H. (2001). A compromised Mlo pathway affects the response of barley to the necrotrophic fungus Bipolaris sorokiniana (teleomorph: Cochliobolus sativus) and its toxins. Phytopathology 91, 127–133. doi: 10.1094/PHYTO.2001.91.2.127
Kumar, U., Joshi, A. K., Kumar, S., Chand, R., and Roder, M. S. (2009). Mapping of resistance to spot blotch disease caused by Bipolaris sorokiniana in spring wheat. Theor. Appl. Genet. 118, 783–792. doi: 10.1007/s00122-008-0938-5
Kumar, J., Schafer, P., Huckelhoven, R., Langen, G., Baltruschat, H., Stein, E., et al. (2002). Bipolaris sorokiniana, a cereal pathogen of global concern: cytological and molecular approaches towards better controldouble dagger. Mol. Plant Pathol. 3, 185–195. doi: 10.1046/j.1364-3703.2002.00120.x
Kumari, L., Verma, A. K., Tiwary, D., Giri, D. D., Nath, G., and Mishra, P. K. (2015). Biodegradation of navy N5RL1 carpet dye by staphylococcus saprophyticus strain BHUSS X3. 3 Biotech 5, 775–782. doi: 10.1007/s13205-015-0276-7
Li, S., Fang, X., Zhang, H., Zeng, Y., and Zhu, T. (2019). Screening of endophytic antagonistic bacterium from Phellodendron amurense and their biocontrol effects against canker rot. Plant Pathol. J. 35, 234–242. doi: 10.5423/PPJ.OA.09.2018.0187
Li, Z., Guo, B., Wan, K., Cong, M., Huang, H., and Ge, Y. (2015). Effects of bacteria-free filtrate from bacillus megaterium strain L2 on the mycelium growth and spore germination of Alternaria alternata. Biotechnol. Biotechnol. Equip. 29, 1062–1068. doi: 10.1080/13102818.2015.1068135
Li, X., Zhang, Y., Wei, Z., Guan, Z., Cai, Y., and Liao, X. (2016). Antifungal activity of isolated bacillus amyloliquefaciens SYBC H47 for the biocontrol of peach gummosis. PLoS One 11:e0162125. doi: 10.1371/journal.pone.0162125
Lin, L., Gu, Y., Li, C., Vittayapadung, S., and Cui, H. (2018). Antibacterial mechanism of ε-poly-lysine against listeria monocytogenes and its application on cheese. Food Control 91, 76–84. doi: 10.1016/j.foodcont.2018.03.025
Lotfi, N., Soleimani, A., Çakmakçı, R., Vahdati, K., and Mohammadi, P. (2022). Characterization of plant growth-promoting rhizobacteria (PGPR) in Persian walnut associated with drought stress tolerance. Sci. Rep. 12:12725. doi: 10.1038/s41598-022-16852-6
Lu, P., Guo, L., Wang, Z., Li, B., Li, J., Li, Y., et al. (2020). A rare gain of function mutation in a wheat tandem kinase confers resistance to powdery mildew. Nat. Commun. 11, 680–611. doi: 10.1038/s41467-020-14294-0
Lugtenberg, B., and Kamilova, F. (2009). Plant-growth-promoting rhizobacteria. Annu. Rev. Microbiol. 63, 541–556. doi: 10.1146/annurev.micro.62.081307.162918
Manhas, R. K., and Kaur, T. (2016). Biocontrol potential of Streptomyces hydrogenans strain DH16 toward Alternaria brassicicola to control damping off and black leaf spot of Raphanus sativus. Front. Plant Sci. 7:1869. doi: 10.3389/fpls.2016.01869
Mirskaya, G. V., Khomyakov, Y. V., Rushina, N. A., Vertebny, V. E., Chizhevskaya, E. P., Chebotar, V. K., et al. (2022). Plant development of early-maturing spring wheat (Triticum aestivum L.) under inoculation with bacillus sp. V2026. Plants 11:1817. doi: 10.3390/plants11141817
Mishra, S., Singh, B. R., Singh, A., Keswani, C., Naqvi, A. H., and Singh, H. B. (2014). Biofabricated silver nanoparticles act as a strong fungicide against Bipolaris sorokiniana causing spot blotch disease in wheat. PLoS One 9(5):e97881. doi: 10.1371/journal.pone.0097881
Monteiro, P., Borba, M. P., and Van Der Sand, S. T. (2017). Evaluation of the antifungal activity of Streptomyces sp. on Bipolaris sorokiniana and the growth promotion of wheat plants. J. Agric. Sci. 9:229. doi: 10.5539/jas.v9n12p229
Qin, Y. L., Zhang, S. B., Lv, Y. Y., Zhai, H. C., Hu, Y. S., and Cai, J. P. (2022). The antifungal mechanisms of plant volatile compound 1-octanol against aspergillus flavus growth. Appl. Microbiol. Biotechnol. 106, 5179–5196. doi: 10.1007/s00253-022-12049-z
Sari, E., Cabral, A. L., Polley, B., Tan, Y., Hsueh, E., Konkin, D. J., et al. (2019). Weighted gene co-expression network analysis unveils gene networks associated with the fusarium head blight resistance in tetraploid wheat. BMC Genomics 20:925. doi: 10.1186/s12864-019-6161-8
Shao, J., Xu, Z., Zhang, N., Shen, Q., and Zhang, R. (2015). Contribution of indole-3-acetic acid in the plant growth promotion by the rhizospheric strain bacillus amyloliquefaciens SQR9. Biol. Fertil. Soils 51, 321–330. doi: 10.1007/s00374-014-0978-8
Shcherbakova, L. A., Nazarova, T. A., Mikityuk, O. D., Istomina, E. A., and Odintsova, T. I. (2018). An extract purified from the mycelium of a tomato wilt-controlling strain of fusarium sambucinum can protect wheat against fusarium and common root rots. Pathogens 7:61. doi: 10.3390/pathogens7030061
Shemshura, O., Alimzhanova, M., Ismailova, E., Molzhigitova, A., Daugaliyeva, S., and Sadanov, A. (2020). Antagonistic activity and mechanism of a novel bacillus amyloliquefaciens MB40 strain against fire blight. J. Plant Pathol. 102, 825–833. doi: 10.1007/s42161-020-00515-4
Srivastava, S., Bist, V., Srivastava, S., Singh, P. C., Trivedi, P. K., Asif, M. H., et al. (2016). Unraveling aspects of bacillus amyloliquefaciens mediated enhanced production of Rice under biotic stress of Rhizoctonia solani. Front. Plant Sci. 7:587. doi: 10.3389/fpls.2016.00587
Su, J., Zhao, J., Zhao, S., Li, M., Pang, S., Kang, Z., et al. (2021). Genetics of resistance to common root rot (spot blotch), fusarium crown rot, and sharp eyespot in wheat. Front. Genet. 12:699342. doi: 10.3389/fgene.2021.699342
Villa-Rodriguez, E., Moreno-Ulloa, A., Castro-Longoria, E., Parra-Cota, F. I., and De Los Santos-Villalobos, S. (2021). Integrated omics approaches for deciphering antifungal metabolites produced by a novel bacillus species, B. cabrialesii TE3T, against the spot blotch disease of wheat (Triticum turgidum L. subsp. durum). Microbiol. Res. 251:126826. doi: 10.1016/j.micres.2021.126826
Villa-Rodríguez, E., Parra-Cota, F., Castro-Longoria, E., López-Cervantes, J., and De Los Santos-Villalobos, S. (2019). Bacillus subtilis TE3: a promising biological control agent against Bipolaris sorokiniana, the causal agent of spot blotch in wheat (Triticum turgidum L. subsp. durum). Biol. Control 132, 135–143. doi: 10.1016/j.biocontrol.2019.02.012
Waithaka, P. N., Gathuru, E. M., Githaiga, B. M., and Kimani, S. N. (2017). Control of passion fruit fungal diseases using essential oils extracted from rosemary (Rosmarinus officinalis) and eucalyptus (Eucalyptus agglomerata) in Egerton university Main campus Njoro, Kenya. Int. J. Microbiol. 2017, 1–6. doi: 10.1155/2017/2814581
Wang, X., Wang, C., Li, Q., Zhang, J., Ji, C., Sui, J., et al. (2018). Isolation and characterization of antagonistic bacteria with the potential for biocontrol of soil-borne wheat diseases. J. Appl. Microbiol. 125, 1868–1880. doi: 10.1111/jam.14099
Wang, Y., Zhang, C., Liang, J., Wu, L., Gao, W., and Jiang, J. (2020). Iturin a extracted from Bacillus subtilis WL-2 affects Phytophthora infestans via cell structure disruption, oxidative stress, and energy supply dysfunction. Front. Microbiol. 11:536083. doi: 10.3389/fmicb.2020.536083
Weisburg, W. G., Barns, S. M., Pelletier, D. A., and Lane, D. J. (1991). 16S ribosomal DNA amplification for phylogenetic study. J. Bacteriol. 173, 697–703. doi: 10.1128/jb.173.2.697-703.1991
Xu, W., Wang, H., Lv, Z., Shi, Y., and Wang, Z. (2019). Antifungal activity and functional components of cell-free supernatant from bacillus amyloliquefaciens LZN01 inhibit fusarium oxysporum f. sp. niveum growth. Biotechnol. Biotechnol. Equip. 33, 1042–1052. doi: 10.1080/13102818.2019.1637279
Xu, W., Yang, Q., Xie, X., Goodwin, P. H., Deng, X., Zhang, J., et al. (2022). Genomic and phenotypic insights into the potential of Bacillus subtilis YB-15 isolated from rhizosphere to biocontrol against crown rot and promote growth of wheat. Biology 11:778. doi: 10.3390/biology11050778
Xu, Z., Zhang, R., Wang, D., Qiu, M., Feng, H., Zhang, N., et al. (2014). Enhanced control of cucumber wilt disease by bacillus amyloliquefaciens SQR9 by altering the regulation of its DegU phosphorylation. Appl. Environ. Microbiol. 80, 2941–2950. doi: 10.1128/AEM.03943-13
Yi, Y., Shan, Y., Liu, S., Yang, Y., Liu, Y., Yin, Y., et al. (2021). Antagonistic strain bacillus amyloliquefaciens XZ34-1 for controlling Bipolaris sorokiniana and promoting growth in wheat. Pathogens 10:1526. doi: 10.3390/pathogens10111526
Yuan, J., Raza, W., Huang, Q., and Shen, Q. (2012). The ultrasound-assisted extraction and identification of antifungal substances from B. amyloliquefaciens strain NJN-6 suppressing fusarium oxysporum. J. Basic Microbiol. 52, 721–730. doi: 10.1002/jobm.201100560
Yue, Z., Shen, Y., Chen, Y., Liang, A., Chu, C., Chen, C., et al. (2019). Microbiological insights into the stress-alleviating property of an endophytic Bacillus altitudinis WR10 in wheat under low-phosphorus and high-salinity stresses. Microorganisms 7:508. doi: 10.3390/microorganisms7110508
Zhang, P., Guo, G., Wu, Q., Chen, Y., Xie, J., Lu, P., et al. (2020). Identification and fine mapping of spot blotch (Bipolaris sorokiniana) resistance gene Sb4 in wheat. Theor. Appl. Genet. 133, 2451–2459. doi: 10.1007/s00122-020-03610-3
Keywords: Bacillus amyloliquefaciens DB2, Bipolaris sorokiniana, biocontrol potential, antifungal mechanism, plant growth-promotion
Citation: Luan P, Yi Y, Huang Y, Cui L, Hou Z, Zhu L, Ren X, Jia S and Liu Y (2023) Biocontrol potential and action mechanism of Bacillus amyloliquefaciens DB2 on Bipolaris sorokiniana. Front. Microbiol. 14:1149363. doi: 10.3389/fmicb.2023.1149363
Edited by:
Samina Mehnaz, Forman Christian College, PakistanReviewed by:
Izzah Shahid, University of Central Punjab, PakistanM. Y. Sreenivasa, University of Mysore, India
Amna Shoaib, University of the Punjab, Pakistan
Copyright © 2023 Luan, Yi, Huang, Cui, Hou, Zhu, Ren, Jia and Liu. This is an open-access article distributed under the terms of the Creative Commons Attribution License (CC BY). The use, distribution or reproduction in other forums is permitted, provided the original author(s) and the copyright owner(s) are credited and that the original publication in this journal is cited, in accordance with accepted academic practice. No use, distribution or reproduction is permitted which does not comply with these terms.
*Correspondence: Yanjie Yi, yiyanjie@haut.edu.cn