- Departamento de Microbiología, Facultad de Ciencias Bioquímicas y Farmacéuticas, CONICET, Instituto de Biología Molecular y Celular de Rosario (IBR), Universidad Nacional de Rosario (UNR), Rosario, Argentina
The acquisition of blaOXA genes encoding different carbapenem-hydrolyzing class-D β-lactamases (CHDL) represents a main determinant of carbapenem resistance in the nosocomial pathogen Acinetobacter baumannii. The blaOXA-58 gene, in particular, is generally embedded in similar resistance modules (RM) carried by plasmids unique to the Acinetobacter genus lacking self-transferability. The ample variations in the immediate genomic contexts in which blaOXA-58-containing RMs are inserted among these plasmids, and the almost invariable presence at their borders of non-identical 28-bp sequences potentially recognized by the host XerC and XerD tyrosine recombinases (pXerC/D-like sites), suggested an involvement of these sites in the lateral mobilization of the gene structures they encircle. However, whether and how these pXerC/D sites participate in this process is only beginning to be understood. Here, we used a series of experimental approaches to analyze the contribution of pXerC/D-mediated site-specific recombination to the generation of structural diversity between resistance plasmids carrying pXerC/D-bounded blaOXA-58- and TnaphA6-containing RM harbored by two phylogenetically- and epidemiologically-closely related A. baumannii strains of our collection, Ab242 and Ab825, during adaptation to the hospital environment. Our analysis disclosed the existence of different bona fide pairs of recombinationally-active pXerC/D sites in these plasmids, some mediating reversible intramolecular inversions and others reversible plasmid fusions/resolutions. All of the identified recombinationally-active pairs shared identical GGTGTA sequences at the cr spacer separating the XerC- and XerD-binding regions. The fusion of two Ab825 plasmids mediated by a pair of recombinationally-active pXerC/D sites displaying sequence differences at the cr spacer could be inferred on the basis of sequence comparison analysis, but no evidence of reversibility could be obtained in this case. The reversible plasmid genome rearrangements mediated by recombinationally-active pairs of pXerC/D sites reported here probably represents an ancient mechanism of generating structural diversity in the Acinetobacter plasmid pool. This recursive process could facilitate a rapid adaptation of an eventual bacterial host to changing environments, and has certainly contributed to the evolution of Acinetobacter plasmids and the capture and dissemination of blaOXA-58 genes among Acinetobacter and non-Acinetobacter populations co-residing in the hospital niche.
Introduction
The main cause of carbapenem resistance among multidrug-resistant (MDR) clinical strains of the opportunistic pathogen Acinetobacter baumannii and its phylogenetically-related species of the A. calcoaceticus-A. baumannii (ACB) complex is represented by acquired blaOXA genes encoding carbapenem-hydrolyzing class-D β-lactamases (CHDL) of the OXA-23, OXA-40/24, OXA-58, OXA-143, and OXA-235 groups (Roca et al., 2012; Carattoli, 2013; Evans and Amyes, 2014; Zander et al., 2014; Da Silva and Domingues, 2016; Hamidian and Nigro, 2019). The blaOXA-58 gene, in particular, is generally found in iteron plasmids endowed with replication initiation protein genes (repAci) encoding members of the Rep_3 (PF01051) superfamily (Rep_3 plasmids) unique to the Acinetobacter genus (Zarrilli et al., 2008; Roca et al., 2012; Carattoli, 2013; Evans and Amyes, 2014; Fu et al., 2014; Zander et al., 2014; Da Silva and Domingues, 2016; Cameranesi et al., 2017, 2018; Lean and Yeo, 2017; Salto et al., 2018; Hamidian and Nigro, 2019; Wang et al., 2020; Hamidian et al., 2021; Liu et al., 2021; Brito et al., 2022; Jones et al., 2022). A number of remarkable features distinguish the blaOXA-58-containing structures carried by these plasmids, including the different genetic contexts in which they are inserted and the almost invariable presence at their borders of a variable number of short sequences displaying homology to the 28-bp chromosomal dif site recognized by the XerC and XerD tyrosine recombinases which have been alternatively designated Re27, pdif, or pXerC/D sites. This has led to suggestions that these pXerC/D-bounded structures could constitute modules endowed with horizontal mobilization abilities, but how these pXerC/D sites participate in the lateral mobilization of the structures they encircle is only beginning to be understood (Cameranesi et al., 2018).
The XerC/D SSR system, ubiquitous among bacteria, serves primary physiological roles in the separation of circular chromosomes prior to cell division by resolving dimers generated by homologous recombination during DNA replication (Cornet et al., 1994; Colloms et al., 1996; Rajeev et al., 2009; Crozat et al., 2014; Ramirez et al., 2014; Balalovski and Grainge, 2020). This system is unique among other SSR systems in that it employs two homologous tyrosine recombinases, XerC and XerD, which recognize a core 28-bp sequence known as dif organized in an imperfect palindrome composed of 11-bp each XerC- and XerD-binding regions, separated by a central spacer region (cr) of 6 bp where recombination occurs. Some ColE1-type plasmids of the Enterobacteriaceae use the XerC/D SSR system of their hosts to resolve their own multimeric forms, thus avoiding segregational instability. Acinetobacter plasmids, on the contrary, are unique in the sense that they contain not a single but several pXerC/D-like sites not necessarily located in direct orientations, which even display some sequence differences between them (Cameranesi et al., 2018). These features suggested functions other than participating only in the resolution of multimers and, in this context, sequence comparison analyses of a number of Acinetobacter plasmids provided evidence of their participation in DNA inversions and fusions/resolutions (Cameranesi et al., 2018; Balalovski and Grainge, 2020; Wang et al., 2020; Alattraqchi et al., 2021; Hamidian et al., 2021; Jones et al., 2022). Yet, experimental evidence supporting their role in SSR events is still scarce, and many questions remain including whether all the pXerC/D sites inferred in Acinetobacter plasmids are active in recombination, the kind of exchanges they can mediate, the factors that govern these reactions, and their effects on the evolutionary dynamics of the plasmids in which they are embedded and the hosts that eventually carry them.
We previously reported first empirical evidences indicating the existence of a recombinationally-active pair mediating the fusion of two plasmids into a co-integrate in A. baumannii cells of our collection (Cameranesi et al., 2018). Moreover, we observed that this co-integrate could also resolve in these cells, following an intramolecular SSR event now mediated by the pair of hybrid pXerC/D sites resulting from the previous intermolecular fusion. More recently, reconstructions of the evolutionary history of the blaOXA-58-containing A. baumannii plasmid pA388 (Jones et al., 2022) also showed the existence of intramolecular inversions mediated by recombinationally active pairs of pXerC/D sites. The overall observations indicated that at least some of the pXerC/D sites inferred in Acinetobacter plasmids can constitute bona fide recombinationally active pairs, supporting roles in the evolution of the plasmid molecules in which they are embedded.
We recently characterized by whole genome sequencing (WGS) a number of phylogenetically- and epidemiologically-related MDR A. baumannii strains belonging to the clonal complex CC15 (Pasteur scheme) prevalent in South America (Matos et al., 2019; Cameranesi et al., 2020; Brito et al., 2022). Comparative genome analysis among two carbapenem-resistant strains of this collection, Ab242 and Ab825, indicated extensive chromosomal synteny between them but also disclosed a much larger number of acquired mobile elements in the Ab825 genome resulting in the inactivation of many exposed cell structures, including protective systems against external aggressors, effectors of the innate immune system, and outer membrane proteins related to carbapenem resistance and virulence, in a process most probably associated to the adaptation of this strain to the hospital environment (Mussi et al., 2005; Cameranesi et al., 2020; Labrador-Herrera et al., 2020). Assembly of the predominant plasmid forms based on WGS data indicated that Ab825 and Ab242 harbored plasmids endowed with almost identical blaOXA-58- and TnaphA6-containing resistance modules (RMs) bounded with pXerC/D sites, but disclosed differences between strains in the structural organization of these resistance plasmids.
We sought to analyze in this work whether and how pXerC/D-mediated recombination contributed to the generation of structural diversity between Ab242 and Ab825 plasmids. By developing a series of experimental approaches (see Supplementary Figure S2 for details), we disclosed the existence of bona fide pairs of recombinationally-active pXerC/D sites among them, some mediating reversible intramolecular inversions and others reversible plasmid fusions/resolutions. This reversible remodeling of Acinetobacter plasmid structures mediated by different pairs of pXerC/D sites significantly impacts the possibilities of adaptation of an eventual host to varying and/or challenging environments, the evolutionary dynamics of the plasmids involved in this process, and the consequent dissemination of blaOXA-58 genes and other adaptive determinants among the bacterial community.
Materials and methods
Bacterial strains and growth conditions
The MDR, carbapenem-resistant A. baumannii clinical strains Ab242 and Ab825 were isolated from hospitalized patients in a public healthcare center (HECA) of Rosario, Argentina. These two strains were assigned to the clonal complex CC15 (Pasteur MLST scheme), and were characterized recently by WGS and comparative genome sequence analysis (Cameranesi et al., 2020).
The A. nosocomialis strain M2 (AnM2) is an antimicrobial susceptible ACB strain lacking indigenous plasmids (Carruthers et al., 2013), and was used as recipient for electrotransformation assays with plasmid mixtures extracted from the A. baumannii clinical strains analyzed (see below, and also Supplementary Figure S2). Similarly, the collection A. baumannii ATCC17978 strain (Ab17978) was also used for the same purpose.
All bacterial cells were grown in Lysogeny Broth (LB) liquid medium at 37°C under aerobic conditions with vigorous shaking, or in LB solid medium prepared by supplementing the LB liquid medium with 1.5% Difco Agar. When necessary, culture media were supplemented with the antimicrobials indicated at the concentrations stated in the text or in the legends to figures.
Growth of clonal populations of Acinetobacter strains and plasmid isolation
We used the general scheme described previously (Cameranesi et al., 2018). In short, isolated colonies of the clinical strains Ab242 or Ab825, or the selected IPM-resistant AnM2 or Ab17978 strains generated in this work, were independently obtained after streaking frozen stocks of the corresponding cells on LB agar media supplemented with 2 μg/ml IPM, followed by incubation at 37°C overnight. Then, clonal populations of each of these strains were generated by inoculating 10 ml of liquid LB medium supplemented with 2 μg/ml IPM with cells picked up from an isolated colony, followed by incubation at 37°C under gentle aeration until confluence. Plasmid extraction was performed from these cultures using the Wizard® Plus SV Minipreps DNA Purification Systems (Promega, WI, USA) and their quality was routinely analyzed by 0.7% agarose gel electrophoresis/ethidium bromide staining.
PCR identification of recombination events mediated by active sister pairs of pXerC/D sites in Acinetobacter plasmids
The plasmid mixtures obtained from the clonal cultures of the different Acinetobacter strains were independently used as templates in PCR assays aimed to detect recombination events mediated by particular pairs of pXerC/D sites (Supplementary Figures S1, S2). The primers employed were used in adequate combinations to detect the different pXerC/D sites and corresponding genetic contexts (see Supplementary Table S1). Primer pair H was additionally employed to detect the presence of plasmids containing the blaOXA-58 carbapenemase gene in isolated colonies of the Ab242 or Ab825 strains, as well as in IPM-resistant AnM2 or Ab17978 colonies obtained after transformation with Ab242 or Ab825 plasmids (see below). The amplification mixtures were analyzed by agarose gel electrophoresis, and the obtained amplicons were subsequently cloned into pGEM-T Easy (Promega) for further sequence analysis. Plasmids containing cloned DNA were analyzed by restriction mapping, and selected inserts were sequenced and subjected to comparative sequence analysis with the different predominant plasmid structures assembled from WGS data in the Ab242 or Ab825 strains to identify recombination events mediated by active pairs of pXerC/D sites (see Supplementary Figure S2 for details).
Transformation of AnM2 and Acinetobacter baumannii Ab17978 cells
Plasmid mixtures extracted from clonal cultures of the Ab242 or Ab825 strains were used for transformation assays employing electrocompetent AnM2 or Ab17978 cells as receptors, following previously described procedures (Ravasi et al., 2011). The rationale here was to obtain separate clonal lineages, in which each lineage originated from an individual cell initially transformed with a single plasmid structural variant carrying the RM from those present in the plasmid mixture (Supplementary Figure S2D). The transformed cells were plated on LB agar supplemented with 2 μg/ml IPM, and incubated 24–48 h at 37°C. Different IPM-resistant AnM2 or Ab17978 colonies developing during this period were analyzed by PCR for the presence of plasmids bearing the blaOXA-58 gene. Stocks of AnM2 or Ab17978 pure cultures generated from cells testing positive for blaOXA-58 were stored at −80° C and routinely used to regenerate the independent cultures (clonal populations) used in this work. Plasmids extracted from these clonal cultures were used in turn as templates in PCR reactions aimed to detect structural variants resulting from recombination events mediated by particular sister pairs of pXerC/D sites.
DNA sequencing, plasmid assembly, and comparative sequence analyses
The assembly of Ab242 plasmids was described previously (Cameranesi et al., 2018). Ab825 genomic DNA was isolated using the DNeasy Blood and Tissue kit (Qiagen), and its genomic sequence was obtained using a paired-end strategy using an Illumina MiSeq sequencer at the National Institute of Health (Lisbon, Portugal). Reads were subjected to quality assessment and further assembly using Velvet version 1.2.10. The de novo assembly process was optimized using the Velvet Optimiser script version 2.2.5. Gaps remaining in contigs corresponding to Ab825 plasmid sequences were closed using PCR with specifically designed primers employing Ab825 plasmid extracts as templates (Supplementary Figure S3).
The Rapid Annotation using Subsystem Technology standard operating procedures (RAST; http://rast.nmpdr.org/seedviewer.cgi; Aziz et al., 2008) and the National Center for Biotechnology Information database (NCBI, U.S. National Library of Medicine, Bethesda MD, USA) were used to annotate the open reading frames (ORFs) found in the different plasmids. Insertion sequence (IS) elements were detected using IS Finder (Siguier et al., 2006; https://www-is.biotoul.fr/) and ISSaga (Varani et al., 2011). Plasmid pXerC/D-like core sequences were detected as described previously (Cameranesi et al., 2018).
The DNA sequences of the PCR fragments cloned into pGem-T were conducted at the Sequencing Facilities of Maine University, Orono (ME, U.S.A.) or at Macrogen (Seoul, Korea).
Results
Comparative analysis of Acinetobacter baumannii Ab242 and Ab825 carbapenem resistance plasmids
Detailed comparative sequence analysis of sequence-related plasmids found in different A. baumannii strains represents a useful tool to detect the recombination mechanism (s) that could account for any observed structural heterogeneity (Cameranesi et al., 2018; Wang et al., 2020; Alattraqchi et al., 2021; Hamidian et al., 2021; Jones et al., 2022). In the case of SSR events mediated by sister pairs of pXerC/D sites, these comparisons are also pivotal for the design of specific PCR primers hybridizing at the immediate neighboring regions associated to each of the pXerC/D-like half-sites that provided for the sister pair (Supplementary Figure S1). PCR analysis using appropriate combinations of primers and as template a plasmid mixture extracted from a clonal population of the studied strain (s), followed by sequence analysis of the obtained amplicons, could then verify whether plasmid structural variants (PSVs) carrying the inferred pXerC/D-mediated inversions and/or fusions are simultaneously present in the analyzed plasmid population (Supplementary Figure S2). This coexistence, in turn, signals the possible operation of a dynamic interconversion state of PSVs resulting from pXerC/D-mediated reversible SSR events in the analyzed cell populations.
WGS data analysis followed by gap closure strategies resulted in the assembly of two predominant circular plasmid forms in the Ab825 strain (Supplementary Figure S3). One of these plasmids of 11,891 bp in size was identical to pAb242_12 previously reported in the Ab242 strain (Cameranesi et al., 2018). Assembly of the second plasmid indicated a circular tri-replicon of 35,743 bp which was designated pAb825_36, and which carried a blaOXA-58- and TnaphA6-containing RM almost identical to that described in pAb242_25 including its associated pXerC/D sites (Figure 1). These two plasmids also showed additional regions of sequence similarity including two of the replication modules, a mobA- and trbL-containing mobilization region, and an IS26 element. However, notorious differences were also found between them. First, the inversion of the DNA region that contains the blaOXA-58- and TnaphA6-containing RM, which was limited in pAb242_25 by the pXerC/D sites C2/D2 and C7/D7 (Figure 1B) and in pAb825_36 by C2/D7 and C7/D2 (Figure 1D). A detailed comparative analysis of the 28-bp core regions of these pXerC/D sites (Table 1) showed identical GGTGTA cr spacers as well as identical XerD-binding half sites for all of them, but some nucleotide differences at the XerC-binding half-sites. These comparisons, the relative orientations of these sites in the corresponding molecules, and the evidence provided by PCR assays (see Table 2; Supplementary Figure S4 below), indicated that C2/D7 and C7/D2 are in fact hybrid products of a SSR event mediated by an active sister pair composed of C2/D2 and C7/D7, and vice versa. Secondly, other main difference between pAb242_25 and pAb825_36 was represented by an extra DNA region of 9,284 bp in the latter plasmid, which is identical in sequence to a linearized form of plasmid pAb242_9. In pAb825_36 this pAb242_9-like region is bordered by two directly-oriented pXerC/D-like sites, namely C4/D14 and C14/D4, which share identical XerC and XerD sequences but differ in the cr region in two out of six nucleotides (Table 1). Detailed sequence comparisons between these plasmids strongly suggested that pAb825_36 resulted from an intermolecular fusion between a plasmid identical to pAb242_9 and a pAb242_25 structural variant, an event mediated by a pXerC/D pair formed by C14/D14 and C4/D4 (Figure 1D). Third, another difference between the carbapenem-resistance plasmid forms found in Ab242 and Ab825 is the presence in pAb825_36 of an IS26-bounded composite transposon-like structure carrying an aac (3)-IIa aminoglycoside acetyltransferase gene, designated here Tnaac(3)-IIa (Figure 1D). Since a single IS26 copy was found in a similar location in pAb242_25 (Figure 1B), this resistance structure most probably resulted from an IS26-mediated recombination event that incorporated, next to this pre-existing IS26, a translocatable unit composed of another IS26 copy (Harmer et al., 2014) carrying in this case a aac(3)-IIa-containing DNA fragment.
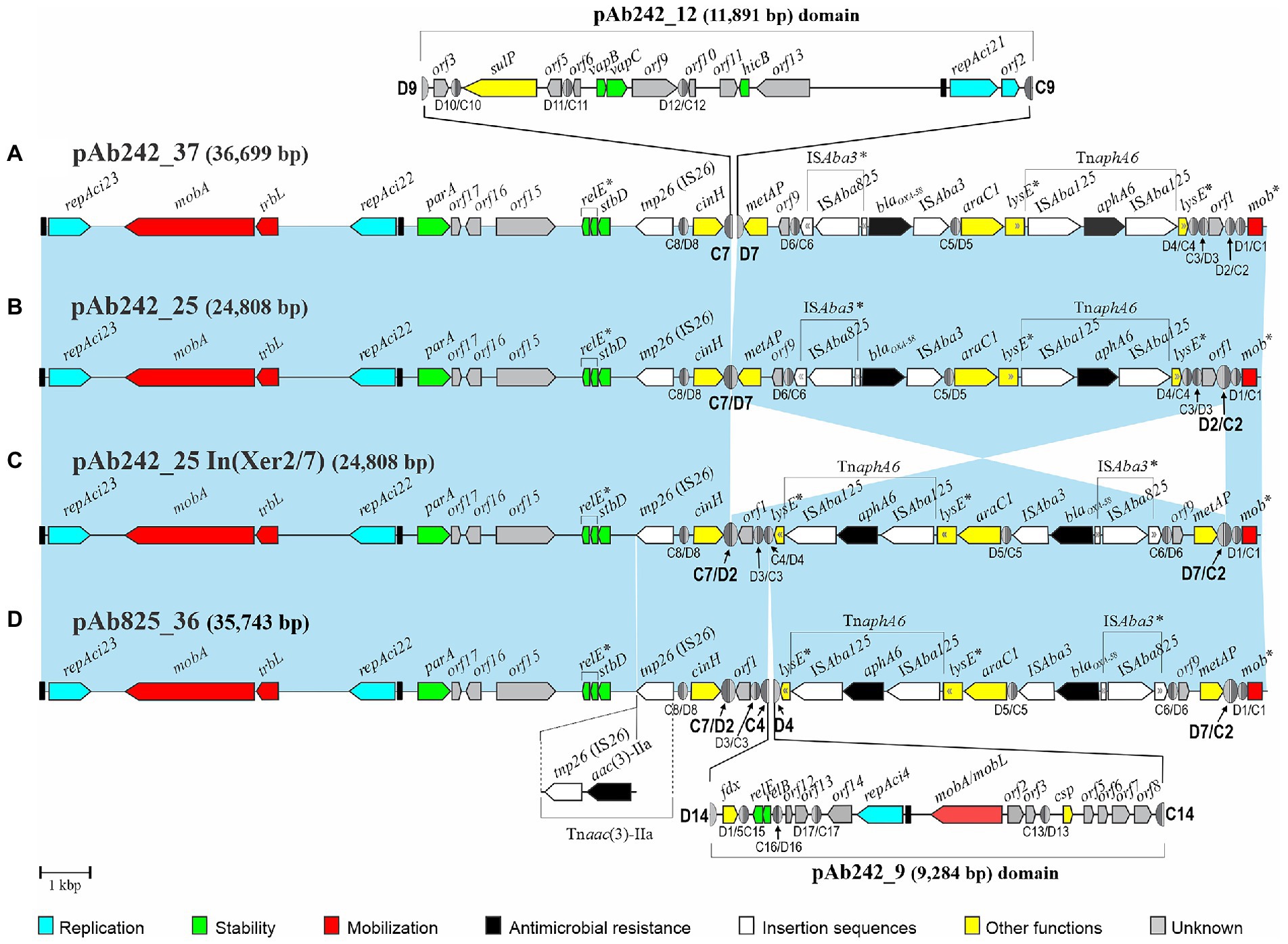
Figure 1. Comparisons of the genetic structures of four plasmid structural variants carrying identical blaOXA-58- and TnaphA6-containing resistance modules bordered by multiple pXerC/D sites identified among related Acinetobacter baumannii clinical strains studied in this work. Linear representations of four Rep_3-based multi-replicon resistance plasmids characterized in the Ab242 and Ab825 strains. For comparison purposes all plasmids were drawn starting from the iteron sequences region located immediately upstream of the repAci23 replication initiation protein gene (black rectangle at the left), with the orientations and extents of the different genes and open reading frames also shown. The genes containing premature termination codons, insertions, and pseudogenes are labeled with asterisks. The genes encoding the different replication initiation proteins belonging to the Rep_3 superfamily are denoted as repAci followed by their assigned denominations, with their preceding iteron sequences indicated as black rectangles. The carbapenem- and aminoglycoside-resistance module (RM) encompassing the blaOXA-58- and aphA6 resistant genes and accompanying elements is found in all plasmids associated to multiple pXerC/D sites located in different orientations. The light-blue shaded background interconnecting the structures of the different plasmids denote homologous regions displaying nucleotide sequence identity ≥99%, the cross sectors indicate the inverted regions found between plasmids. Antimicrobial resistance genes are labeled in black and IS elements in white, with their corresponding denominations indicated above each plasmid structure. The extension of the ISAba125-based composite transposon TnaphA6 inserted within the lysE gene is also indicated. The different pXerC/D-like sites inferred in these plasmids are shown as ovals (not drawn to scale), with the XerC (C) and XerD (D) recognition sequences depicted as dark and light gray semi-ovals, respectively. Their designations (see Cameranesi et al., 2018 for numbering and sequence details) and corresponding polarities are indicated below the structures. The pXerC/D sites identified as involved in SSR have been enlarged, and their designations highlighted in bold letters. (A) pAb242_37 structure. This tri-replicon resulted from an intermolecular fusion mediated by the pXerC/D pair C7/D7 and C9/D9 sites located in pAb242_25 and pAb242_12, respectively (Cameranesi et al., 2018). The structure of pAb242_12 and its insertion site into pAb242_25 are indicated above the structure, and resulted in the generation of a directly-oriented pair of (hybrid) sites formed by C7/D9 and C9/D7. (B) pAb242_25. This bi-replicon was assembled from WGS data analysis obtained from the Ab242 strain. (C) pAb242_25 In(Xer2/7). Plasmid structural variant of pAb242_25 described in this work, in which the region located between the oppositely-oriented sites C7/D7 and D2/C2 is inverted. As the result, a novel pair of oppositely-oriented (hybrid) sites, C7/D2 and D7/C2, was generated. (D) pAb825_36. Plasmid assembled from WGS data analysis of the Ab825 strain followed by gap closure using PCR with specifically-designated primers (see Supplementary Figure S3). Sequence analysis indicated that this tri-replicon was formed by the fusion of a plasmid much similar in structure to pAb242_25 In(Xer2/7) and another plasmid identical to pAb242_9, mediated by the pair of pXerC/D sites C4/D4 and C14/D14 located in pAb242_25 In(Xer2/7) and pAb242_9, respectively. This fusion resulted in the generation of a directly-oriented pair of (hybrid) sites, C4/D14 and C14/D4. A complete IS26-based composite transposon, Tnaac(3)-IIa, was also found in pAb825_36 and its location is also indicated below the plasmid structure. GenBank accession numbers: pAb242_25, KY984047; pAb242_12, KY984046; pAb242_9, KY984045, pAb825_36, MG100202.
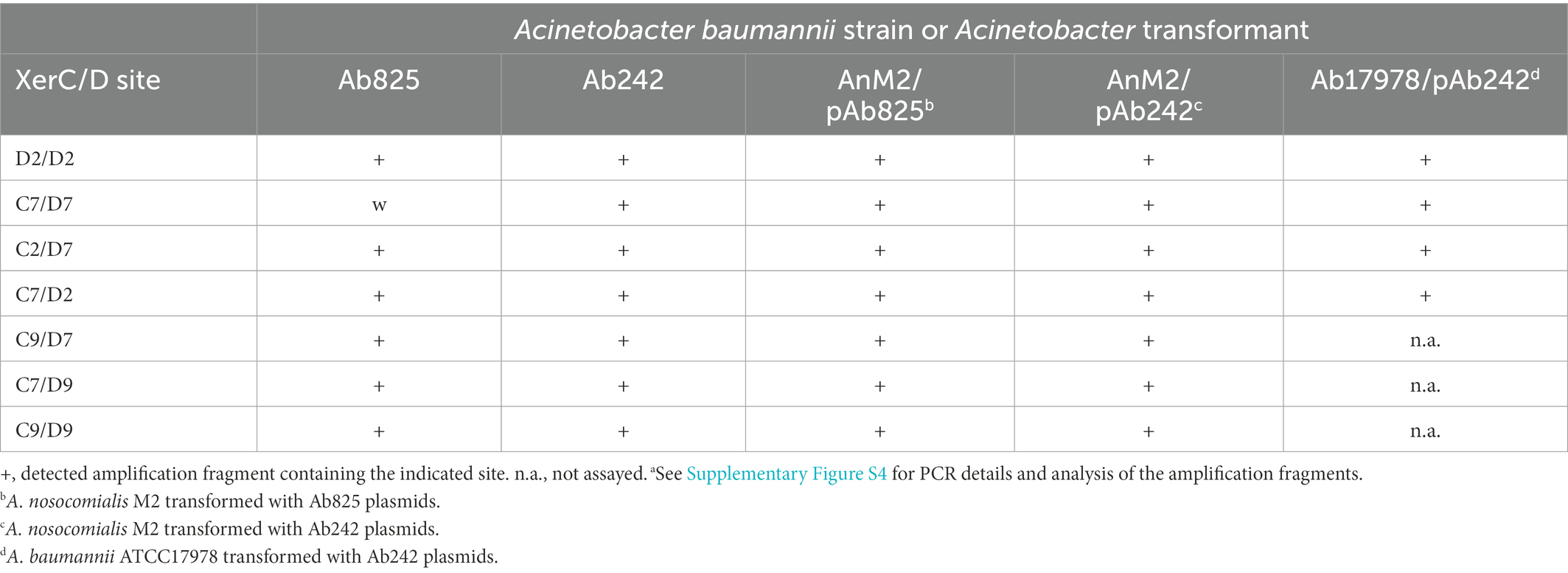
Table 2. Summary of the PCR evidence for the coexistence of different plasmid structural variants containing inversions and fusions resulting from SSR mediated by active pairs of pXerC/D sites in clonal populations of the different Acinetobacter strains analyzed.a
In summary, the comparative sequence analysis of the plasmids housed by the Ab242 and A825 strains assembled from WGS data analysis indicated that they shared extensive sequence identity as a whole, but also disclosed significant differences between strains including variations in the structural organization of their carbapenem-resistance plasmids and a complete IS26-based Tnaac(3)-IIa transposon-like structure in pAb825_36 (Figure 1). Moreover, these comparisons pointed to pXerC/D-mediated SSR as one of the main agents behind these structural differences.
Reversible DNA inversions mediated by recombinationally-active pairs of pXerC/D sites in Ab825 and Ab242 carbapenem-resistance plasmids
The presence of the pXerC/D sites predicted in the carbapenem resistance plasmids harbored by the Ab825 and Ab242 strains (Figure 1) was confirmed by PCR procedures using as templates whole plasmid mixtures extracted from clonal cultures of these strains (see Table 2 for a summary and Supplementary Figure S4 for details). These assays indicated the expected presence of plasmids containing C2/D7 and C7/D2 sites among Ab825 plasmids, and C2/D2 and C7/D7 sites among Ab242 plasmids. Notably, the use of appropriate primer combinations indicated the presence also of plasmid variants containing C2/D2 and C7/D7 sites among Ab825 plasmids, and variants containing C2/D7 and C7/D2 sites in Ab242 plasmid extracts (Table 2). These results indicated that PSVs containing inversions of the DNA region delimited by the recombinationally active pair C2/D2||C7/D7 (or the pair C2/D7||C7/D2) coexisted in the corresponding plasmid extracts. Based on these results, we define here as pAb242_25 In (Xer2/7; Figure 1C) the pAb242_25 structural variant detected in Ab242 clonal cultures that resulted from an Inversion mediated by the recombinationally-active pair composed of the oppositely-oriented sites C2/D2 and C7/D7 (Xer2/7).
The overall results suggested that a dynamic interconversion of PSVs resulting from reversible SSR events mediated by specific sister pairs of oppositely-oriented pXerC/D sites, in this case C2/D2||C7/D7 and C2/D7||C7/D2 (Figure 2), is in operation in both Ab825 and Ab242 cells.
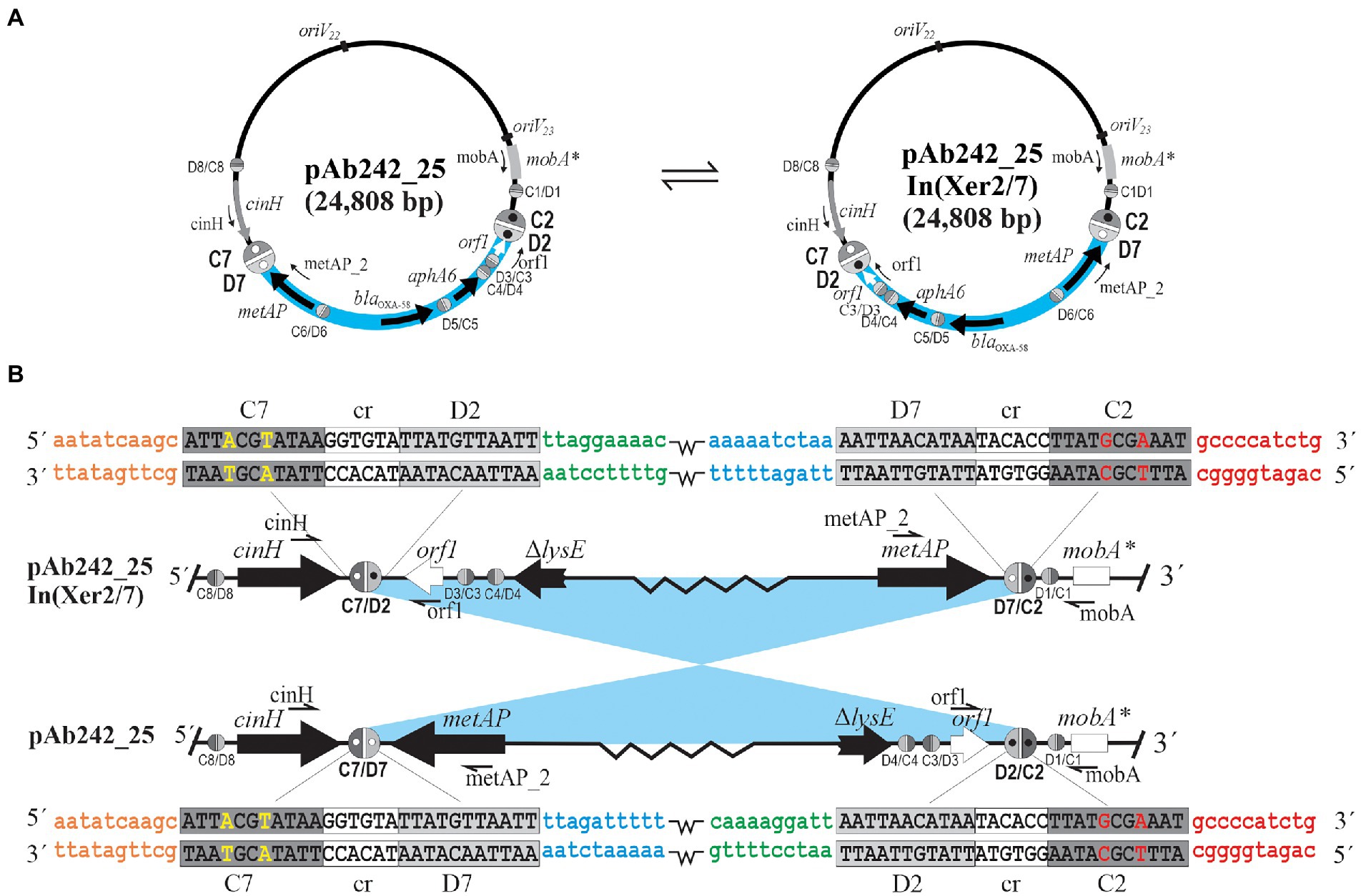
Figure 2. DNA inversions resulting from site-specific recombination between oppositely-oriented sister pairs of pXerC/D sites located at the borders of blaOXA-58-and aphA6-containing adaptive modules in Acinetobacter plasmids. (A) SSR between the oppositely-oriented sister pair formed by the C2/D2 and C7/D7 sites located in plasmid pAb242_25 mediate the reversible inversion of the intervening sequences between them, thus generating a structural variant designated here as pAb242_25 In(Xer2/7). In turn, the reverse SSR reaction between the resulting oppositely-oriented C2/D7 and C7/D2 “hybrid” sites can regenerate the original pAb242_25 structure. These two structural variants co-exist in clonal populations of the A. baumannii strains analyzed, as well as in clonal populations of other Acinetobacter strains transformed with plasmids carrying the adaptive module present in these A. baumannii strains. The DNA region carrying the blaOXA-58- and aphA6-containing RM is highlighted in pale blue, with the orientation of the genes indicated in each case. The different pXerC/D-like recognition sites are indicated as ovals (not drawn to scale), with the C and D binding regions depicted as dark and light gray semi-ovals, respectively. The active sister pairs mediating inversions have been arbitrarily enlarged, and additional open and closed inner circles within have been incorporated to facilitate visualization of the recombinatorial events. The PCR primers used to identify the presence of specific pXerC/D sites (see Supplementary Table S1 for details) are indicated. The oriV22 and oriV23 rectangles denote the location of the iteron regions of the two different replication modules predicted in these plasmids. (B) Enlarged vision of the SSR reactions conducing to the reversible inversions described above. The lowercase colored letters show the DNA sequences located at the immediate vicinities of each specific half-site forming the pXerC/D core (10 bp each), and have been included to facilitate visualization of the SSR events. Note that the sequences of the cr and the XerD binding regions are identical for all recombining sites, while the XerC binding regions show some differences between sites (highlighted in uppercase colored letters). The figure is not drawn to scale.
We next used a transformation approach to verify the existence of the dynamic interconversion of PSVs postulated above. The rationale behind (see Supplementary Figure S2) consisted in the generation of clonal lineages of a model Acinetobacter strain (e.g., A. nosocomialis M2, AnM2). Each clonal lineage is derived from an individual cell transformed with a single PSV carrying the blaOXA-58-containing RM, from the different structural variants present in a plasmid mixture extracted from a clonal population of the A. baumannii strain under study. The replication of a single incoming plasmid in the new host cell would allow growth in selective medium and the subsequent generation of a clonal population derived from this original event. If a dynamic interconversion of PSVs resulting from reversible SSR events mediated by specific sister pairs of oppositely-oriented pXerC/D sites existed in the cells of the A. baumannii strain analyzed, this state could be re-established in a clonal population of the new Acinetobacter host and the different co-existing PSVs could therefore be detected by PCR assays.
Competent AnM2 cells were thus transformed with whole plasmids extracted from the Ab825 strain (AnM2/pAb825) or the Ab242 strain (AnM2/pAb242), followed by selection on LB solid medium containing IPM. Individual colonies of AnM2/pAb825 or AnM2/pAbAb242 testing positive for the blaOXA-58 gene were then used to generate clonal cultures, from which plasmids were extracted and subjected to PCR analysis as indicated above. Using these procedures, we could systematically reproduce in these transformants the PCR results obtained above using plasmids extracted from clonal cultures of Ab825 or Ab242 cells, that is, the co-existence of PSVs containing C2/D2||C7/D7 and C2/D7||C7/D2 sites also in AnM2/pAb825 and in AnM2/pAb242 cells (Table 2; Supplementary Figure S4). Moreover, similar results were obtained for Ab17978 cells transformed with Ab242 plasmid extracts (Ab17978/pAb242). This indicated that a dynamic interconversion of PSVs containing inversions of the DNA regions delimited by the pairs C2/D7||C7/D2 and C2/D2||C7/D7 and resulting from reversible SSR events mediated by these sites could be reproduced in clonal populations of the new hosts (Figure 2).
Detection of reversible plasmid fusions in Ab825 and Ab242 cells mediated by recombinationally-active pairs of pXerC/D sites
We experimentally verified previously (Cameranesi et al., 2018) the fusion of two Ab242 plasmids, pAb242_25 and pAb242_12, into a co-integrate designated pAb242_37 as the result of a SSR event mediated by the pXerC/D sites C7/D7 and C9/D9 (see Figure 1A for the corresponding structure). Moreover, we found that pAb242_37 could also resolve in the new host by employing a recombinationally active pair now formed by the C7/D9 and C9/D7 hybrid sites formed during the previous fusion.
We designed different PCR primers based on these results (Supplementary Table S1) aimed to test the presence of similar co-integrates (and their resolution products) in the plasmid extracts of AnM2/pAb242 and AnM2/pAb825 cells employed above. Hybrid C9/D7 and C7/D9 sites were both detected in AnM2/pAb242 plasmid extracts (Table 2; Supplementary Figure 4F, first two lines at the left), indicating the presence of the pAb242_37 co-integrate in these cells. Moreover, PSVs containing the C9/D9 site (Supplementary Figure 4F, third line at the left) and the C7/D7 site (Supplementary Figure 4F, second lane) were also detected in the same plasmid extracts. The coexistence of both the co-integrate and its resolution products in these cells reinforced the notion (Supplementary Figure S2) that the pAb242_37 co-integrate represented the incoming plasmid form with the ability to replicate and establish in a new AnM2 host cell, and that this co-integrate could also undergo resolution in these cells via an intramolecular SSR event mediated now by the directly-oriented hybrid pair C9/D7||C7/D9 (Supplementary Figure S5). Similar observations were made using AnM2/pAb825 plasmid extracts, indicating the co-existence in these extracts of PSVs that included a co-integrate variant containing the C9/D7 and C7/D9 hybrid sites as well as individual plasmids carrying the C9/D9 and C7/D7 sites product of the resolution of this co-integrate (Table 2; Supplementary Figures S4F, S4C, respectively). Again, these observations support the existence of a dynamic state of resolution and re-synthesis of similar co-integrates in both Ab825 and Ab242 cells (Supplementary Figure S5).
Contrary to the above results, PCR assays aimed to detect the presence of the C14/D14 site and neighboring regions using Ab825 plasmid extracts as templates (Supplementary Table S1) consistently failed, in sharp contrast with the amplification bands obtained for the C4/D14 and C14/D4 sites using the same extracts and different combinations of the same primers (Supplementary Figures S3, S4G). A successful detection of the C14/D14 site in Ab825 plasmid mixtures would have implicated the presence of a pAb242_9 circular plasmid structure, and therefore that pAb825_36 (Figure 1D) could be resolved in these cells into its putative plasmid constituents in a SSR event mediated by the directly-oriented C4/D14 and C14/D4 sites. It is worth noting that these sites contain identical XerC- and identical XerD- half-sites, but differed in 2 out of 6 positions in the cr spacer sequence (Table 1). This resolution, if taking place, probably occurs at a very low rate as compared to the other SSR events described above which could be reproducibly detected by the PCR assays employed.
The overall observations thus support the existence of a dynamic interconversion state of PSVs involving reversible intramolecular inversions and fusions/resolutions mediated by recombinationally active pairs of pXerC/D sites sharing identical GGTGTA cr spacers in both Ab825 and Ab242 cells. Moreover, this dynamic interconversion of PSVs could be reinstalled in the cells of other A. baumannii strains or other Acinetobacter species after transformation with a PSV carrying the blaOXA-58-containing RM and possessing the capability to establish in the new host.
Structural comparisons between A. baumannii Ab242 and Ab825 plasmids with similar plasmids described in other Acinetobacter strains provide clues on the evolution of novel Rep_3 replicons endowed with blaOXA58-containing resistance modules
A BlastN search against the nucleotide GenBank database (as of August 15, 2022) using as query the Ab242_25 sequence (KY984047) identified a similar plasmid, pAs1069_a (accession number MK323040) housed by a carbapenem-resistant Acinetobacter seifertii clinical strain isolated in Brazil (Matos et al., 2019). pAb242_25 and pAs1069_a share 95% total sequence identity, including the presence and orientation of the blaOXA-58- and TnaphA6-containing RM in the corresponding molecules (Figure 3A). However, one remarkable difference between these two plasmids is the number of pXerC/D sites found at the TnaphA6-proximal end of the corresponding RMs, which were reduced to two sites in pAs1069_a as compared to the four sites located in the equivalent region of pAb242_25. It is worth noting that pAs1069_a contains in this region an ISAjo2-like element, and sequence comparison analysis indicated that the acquisition of this element was concomitant with the deletion of a fragment of around 490 bp that in pAb242_25 encompasses the C2/D2 and C3/D3 sites (Figure 3A). Among the seven pXerC/D sites located at the borders of the blaOXA-58- and TnaphA6-containing RM in pAb242_25, only the C7/D7 and C2/D2 sites share identical GGTGTA cr sequences (Table 1, see also Cameranesi et al., 2018). The selection of the insertion of this ISAjo2 element at this region in pAs1069_a, by removing the C2/D2 site, is most probably associated then with an impairment of the reversible inversion ability of the DNA fragment encompassing the carbapenem RM.
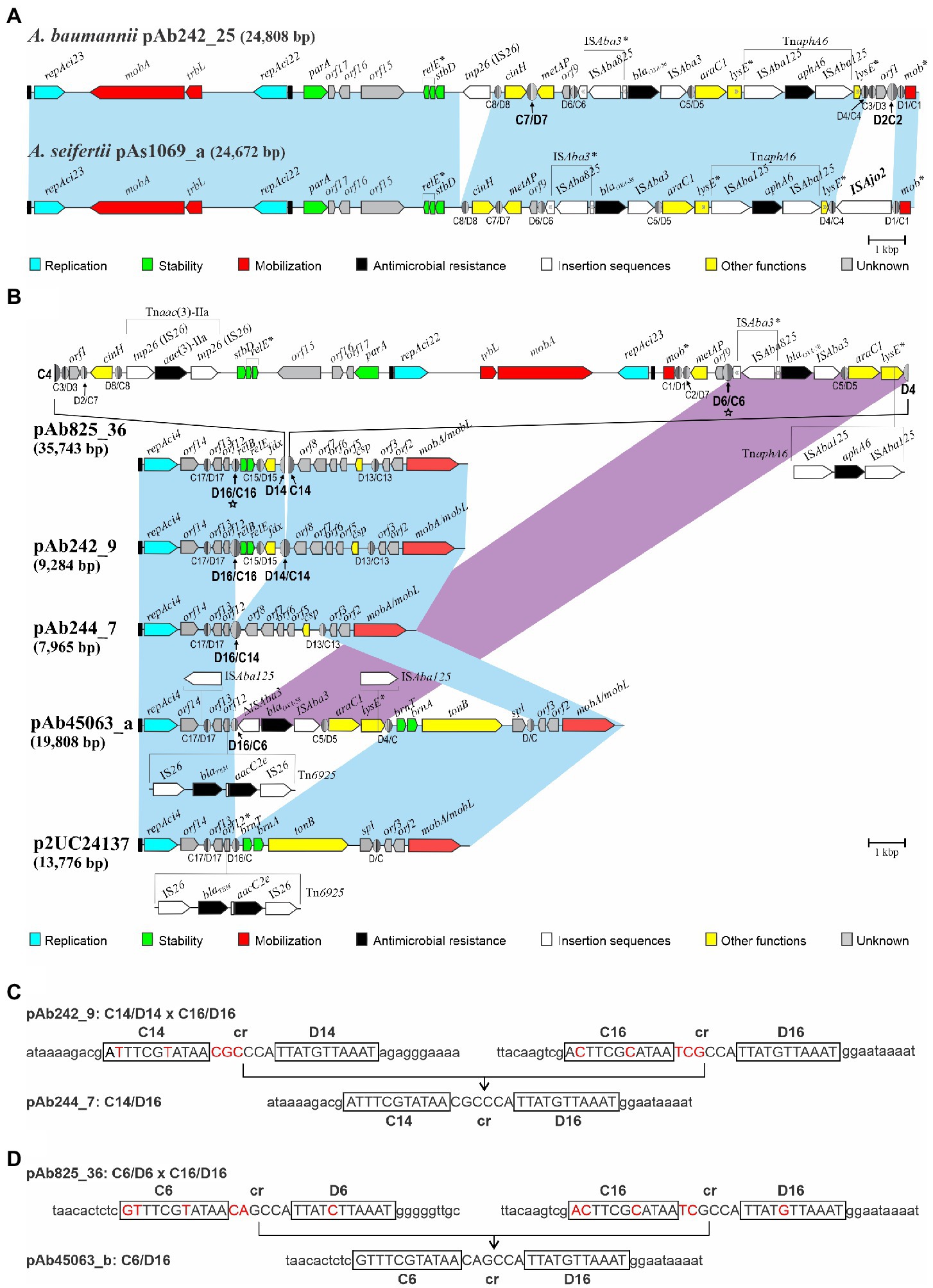
Figure 3. Structural comparisons between blaOXA-58-containing Rep_3 plasmids carried by Acinetobacter baumannii Ab242/Ab825 strains with sequence-related plasmids deposited in databases described in other Acinetobacter strains. (A) Comparisons of blaOXA-58-containing pAb242_25 with a similar repAci23- and repAci22-containing bi-replicon carried by the Acinetobacter seifertii 1,069 strain isolated in Brazil, pAs1069_a (GenBank accession number MK323040). The plasmids share 95% total sequence identity, all containing almost identical RMs encompassing blaOXA-58- and aphA6-resistant genes which show the same orientation in pAs1069_a as compared to pAb242_25. However, pAs1069_a contains an extra ISAjo2-like element at the TnaphA6-proximal end of the RM (1,482 bp; nucleotide positions 22,311 to 23,792), an insertion that is concomitant with the deletion of around 490 bp containing the C2/D2 and C3/D3 sites in pAb242_25. For other details on plasmid structures see the legend to Figure 1. (B) Comparisons of the pAb825_36 tri-replicon structure characterized in this work with different plasmids containing backbones endowed with a repAci4 replication module and mobA/mobL genes described in other A. baumannii CC15 strains. The light-blue shaded background interconnecting the different plasmid structures denote shared regions of sequence homology between plasmids. ISs or IS-based transposons found in a given plasmid but not in others within these otherwise homologous regions were represented either above or below the corresponding structures. The magenta-shaded background denotes homologous regions carrying similar pXerC/D-bounded blaOXA-58-containing RMs between pAb825_36 and pAb45063_a. The pXerC/D sites constituting sister pairs mediating SSR events, or resulting from these events, have been arbitrarily enlarged. The directly-oriented C6/D6 and C16/D16 sites in pAb825_36 (labeled with stars ★), proposed here to mediate an intramolecular SSR event conducing to a pAb45063_a-like plasmid as one of the resolution products, have been also arbitrarily enlarged. GenBank accession numbers: pAb244_7: MG520098; pAb45063_a: MK323042. For other details on plasmid structures see the legend to Figure 1. (C) Sequence comparison evidence indicating the existence of an intramolecular SSR resolution event in pAb242_9 mediated by the directly-oriented C14/D14 and C16/D16 sites conducing to the generation of pAb244_7 containing a C14/D16 (hybrid) site. The pXerC/D core sequences of each site are displayed in uppercase letters, where the XerC and XerD half-sites have been additionally boxed. For a better visualization of the SSR event the nucleotide differences between core sites on the substrate plasmid have been highlighted in red, and additional sequences (10 bp in each case) at the immediate vicinities of each specific half-site have been included. (D) Same, indicating an intramolecular SSR resolution event in a pAb825_36-like substrate plasmid mediated by the directly-oriented C6/D6 and C16/D16 sites resulting in the generation of a pAb45063_a-like plasmid containing a C6/D16 (hybrid) site.
The use of pAb242_12 (KY984046) as a query retrieved no repAci21-containing plasmids carrying blaOXA-58 genes, with the exception of the pAb242_37 multi-replicon in which a pXerC/D-bounded pAb242_12 domain is inserted (Figure 1). On the contrary, the use of pAb242_9 (KY984045) identified, besides the pXerC/D-bounded pAb242_9 domain carried by the pAb825_36 tri-replicon (Figure 1), a series of plasmids sharing backbones also composed of a repAci4-based replicon and a mobA/mobL module (Figures 3B,C). It is worth noting that these plasmids were carried by A. baumannii CC15 strains isolated in Argentina, Brazil, and Chile (Matos et al., 2019; Cameranesi et al., 2020; Brito et al., 2022). Among them pAb244_7, the smallest plasmid of this group, differs from pAb242_9 in an approximately 1.3 kbp DNA region carrying genes for a relBE system and ferredoxin (fd; Figure 3B). This region is bordered by two directly-oriented pXerC/D sites, C14/D14 and C16/D16, which differ in 2 out of 11 positions in their XerC-binding regions and in 3 out of 6 positions in their cr spacers (Figure 3C). Yet, the identification of a hybrid C14/D16 site in pAb244_7 strongly suggests that a XerC/D-mediated resolution event occurring in pAb242_9 could be responsible for the loss of this pXerC/D-bounded module.
pAb45063_a, another member of this group, present in an A. baumannii CC15 strain isolated in Brazil (Matos et al., 2019), carries an IS26-based composite-like transposon endowed with blaTEM-1 and aacC(3)-IIa resistance genes and, most relevantly for this work, a pXerC/D-bounded blaOXA58-containing RM (Figure 3B). Differences in mobile elements content between the RMs found in pAb45063_a and Ab242/Ab825 plasmids (Figure 1), such as the ISAba825 element found upstream of the blaOXA-58 gene and the TnaphA6 transposon inserted within the lysE gene in the latter plasmids as compared to the single ISAba125 copy interrupting lysE in pAb45063_a (Figure 3B), are commonly observed among Acinetobacter Rep_3 plasmids carrying similar adaptive modules, and most probably result from the collection of different ISs and transposons during plasmid transit through different bacterial hosts (Cameranesi et al., 2018). Most importantly however, these comparisons provide clues on how the generation of complex multi-replicon co-integrates such as pAb825_36 could also supply the substrates for subsequent XerC/D-mediated structural rearrangements that eventually evolve in novel replicon structures carrying blaOXA-58-containing RMs. In principle, a SSR resolution event involving the directly-oriented C6/D6 and C16/D16 sites located in pAb825_36 as a sister pair (Figure 3D) could have generated two separate plasmids, one repAci23- and repAci22-containing bi-replicon and another replicon containing a repAci4- and mobA/mobL-backbone now carrying a blaOXA-58-containing RM similar in structure to pAb45063_a (Figure 3B).
Discussion
We sought to analyze in this work whether and how pXerC/D-mediated recombination contributed to the generation of structural diversity between carbapenem resistance plasmids harbored by the A. baumannii strains Ab242 and Ab825, two phylogenetically- and epidemiologically-closely related CC15 strains isolated from hospitalized patients in the same public healthcare center in Argentina. These two strains harbored similar Rep_3 plasmids carrying almost identical RM endowed with a blaOXA-58 gene accompanied with a TnaphA6 composite transposon, a structure associated to seven non-identical pXerC/D sites at their borders. Comparative sequence analysis of Ab242 and Ab825 plasmids assembled on the basis of WGS data (Figure 1) indicated significant structural variations between them, and pointed to XerC/D-mediated SSR as the main agent behind these differences.
We developed a series of methodologies to experimentally verify that SSR events mediated by pXerC/D sites represented the cause of the structural differences observed between Ab242 and Ab825 plasmids carrying the above-described RM. These procedures allowed us to disclose the existence of bona fide pairs of recombinationally-active pXerC/D sites in these plasmids, some mediating reversible intramolecular inversions and others reversible plasmid fusions/resolutions. Among them, the pair formed by the oppositely-oriented C2/D2 and C7/D7 sites, and its SSR products the (hybrid) C2/D7 and C7/D2 pair, mediated reversible intramolecular inversions of the RM in both strains. In turn, the pair formed by the C7/D7 and C9/D9 sites mediated intermolecular fusions, and that formed by its SSR products the (hybrid) C7/D9 and C9/D7 sites mediated intramolecular resolutions. These events strongly suggested that a dynamic interconversion of sequence-related PSVs is in operation in clonal populations of the Ab242 and Ab825 strains analyzed. Moreover, this reversible shuffling of plasmid structures could be reproduced in cells of model Acinetobacter strains including A. nosocomialis M2 and A. baumannii ATCC17978 in laboratory transformation assays. All of the A. baumannii pXerC/D pairs identified here as mediating reversible SSR events contained identical GGTGTA cr sequences separating the XerC from the XerD half-sites (Table 1), sharing characteristics with pairs classified as relaxed or unconstrained in other models (Cornet et al., 1994).
The findings that Acinetobacter plasmids contain pXerC/D sites capable of conforming recombinationally active pairs mediating reversible remodeling of their structures significantly impact the possibilities of rapid adaptation of their eventual hosts to varying environments, the evolution of plasmid structures, and the dissemination of blaOXA-58 genes among both Acinetobacter and non-Acinetobacter populations. In principle, this dynamic shuffling of plasmid structures could provide an eventual Acinetobacter host fitness advantage to confront the different selective pressures that the population may encounter during growth. In this context, a number of other bacterial species have been found capable of generating genetically- and phenotypically- heterogeneous subpopulations by employing reversible SSR mechanisms (Didelot et al., 2016; Andam, 2019; García-Pastor et al., 2019; Jiang et al., 2019; Trzilova and Tamayo, 2021). The degree of diversity in these cases solely depends on the number of phase-variable loci, and this process has a profound impact on the survival or fitness capacity of the population as a whole. A valuable asset of reversibility is the ability to rapidly restore genotypic and phenotypic heterogeneity after a bottleneck, a common situation for a bacterial pathogen such as A. baumannii that frequently transits from the human host to the environment and vice versa, especially in the highly selective conditions of the clinical setting.
The dynamic interconversion of plasmid structural variants could be also viewed as a trial- and-error game for the long-term adaptation of an eventual Acinetobacter host to a specific niche or to long-term exposures to particularly challenging conditions. The majority of bacterial genes are encoded on the leading strand of DNA replication such that transcription is co-directional with fork movement, preventing the deleterious consequences of head-on replication-transcription conflicts (Schroeder et al., 2020). Inversions that result in head-on conflicts promote the selection of mutations, including insertions/deletions and base substitutions depending on genetic context, leading to proposals that the generation of such conflicts potentiates bacterial evolvability (Merrikh and Merrikh, 2018). The dynamic state of DNA inversions and fusions/resolutions reported here in Acinetobacter plasmids, by promoting temporary head-on conflicts, could then accelerate the mutation rate in certain plasmid regions with lasting consequences for the evolution of these mobile elements. For instance, and depending on host and environmental pressures, mutational events could be selected that “freeze” a given structural variant thus allowing, for instance, continuous expression of a gene whose product is in extended demand. In this context, the several pXerC/D sites located at the borders of the plasmid-borne blaOXA58-containing RM studied here (Figure 1) provide different alternatives for selection, which may range from complete deletions that abolish the possibilities of recombination (and thus shuffling) to subtle mutations that impede (or increase) the activity of a given pXerC/D pair. The comparisons shown in Figure 3A indicating the ISAjo2-mediated elimination in A. seifertii plasmid pAs1069_a of a site composing a recombinationally active pXerC/D pair in Ab242/Ab825 plasmids (Figure 1), provide an example of the above. These observations additionally suggest that the position (or orientation) of the blaOXA-58-containing RM in the Acinetobacter plasmid molecule is subjected to selection pressures, and that the selection of a particular orientation most probably depend on the fitness provided to a particular plasmid or plasmid/host partnership in a given environment.
The reversible SSR events mediated by pXerC/D sites reported here may have played important roles in facilitating the wide dissemination of blaOXA-58 genes among plasmids carried by Acinetobacter species, and also among other bacterial populations (Zarrilli et al., 2008; Carattoli, 2013; Evans and Amyes, 2014; Fu et al., 2014; Zander et al., 2014; Da Silva and Domingues, 2016; Hamidian and Nigro, 2019; Bonnin et al., 2020; Wang et al., 2020; Alattraqchi et al., 2021; Hamidian et al., 2021; Liu et al., 2021; Brito et al., 2022; Jones et al., 2022). It is noteworthy in this context the generation of multi-replicons resulting from pXerC/D-mediated SSR events in both the Ab242 and Ab825 strains (Figure 1). Multi-replicon plasmids have been described in many Acinetobacter members by different authors, suggesting selective advantage(s) for these ensembles (Carattoli, 2013; Shintani et al., 2015; Cameranesi et al., 2018, 2020; Hamidian and Hall, 2018; Dionisio et al., 2019; Mindlin et al., 2020; Salgado-Camargo et al., 2020; Alattraqchi et al., 2021; Jones et al., 2022). A reversible fusion of different replicons, by expanding the host range or by providing stability (or other functions) to the ensemble, could play important roles in the establishment of the different plasmid components in a given host (Dionisio et al., 2019). In this context, we observed that a co-integrate between pAb242_25 (or a pAb242_25-related plasmid) and pAb242_12 was the form that could successfully establish in different Acinetobacter hosts including A. nosocomialis M2 and A. baumannii ATCC17978 in laboratory transformation assays (Table 2). The presence of pXerC/D sites in blaOXA-58-containing resident plasmids also opens the possibility of reversible co-integrate formation with newly incoming conjugative plasmid(s) endowed with pXerC/D sites, thus allowing the transfer of the cargo plasmid by conduction. Moreover, a rapid resolution of the co-integrate in the new host by the reverse SSR reaction may facilitate the rapid segregational loss of the conjugative, energy-demanding counterpart in the progeny especially under antimicrobial selection pressure for the cargo plasmid (Dionisio et al., 2019).
The generation of complex multi-replicon structures endowed with blaOXA-58-containining RMs, by generating new potential pairs of recombinationally active pXerC/D sites capable of mediating further intramolecular resolution events, can also facilitate the evolution of novel replicons carrying blaOXA-58-containing RMs (Figure 3B). This mechanism, added to structural rearrangements generated by subsequent pXerC/D-mediated SSR events and the insertions of different ISs and transposons, could explain the evolution of novel carbapenem-resistance plasmids in the local A. baumannii CC15 population.
The different pXerC/D sites inferred in Ab242/Ab825 plasmids show some sequence differences between them at the level of the XerC- and also the XerD-half sites on the core, as well as in the cr spacer separating these regions (Cameranesi et al., 2018). The importance of homology between the core regions of the two recombining sites has not been clearly established for the E. coli XerC/D system (Rajeev et al., 2009), and little information exists on the A. baumannii XerC/D system. However, recent studies (Lin et al., 2020) indicated similar functions for this SSR system as compared to its E. coli counterpart. Our results (Table 2) indicated that reversible recombination could occur between pairs of sites sharing identical GGTGTA cr spacer sequences, even when these recombining sites display some nucleotide differences at the level of the XerC- and also XerD- half-sites. On the contrary, reversible recombination could not be detected for the C4/D14 and C14/D4 sites found in pAb825_36, which contained identical XerC- and identical XerD- half-sites but differed in 2 out of 6 positions in the cr spacer sequence (Table 2). This suggests that the reversibility potential of SSR reactions mediated by pXerC/D sites depends on the cr sequences of the pair involved. In this context, XerC/D-mediated recombination between plasmids containing dif and psi core sites sharing almost identical XerC- and also XerD- half-sites but differing in their cr sequences in 4 out of 6 positions occurred in E. coli cells at very low rates, as judged by the low frequency of colonies carrying plasmids with dif/psi hybrid sites obtained (Cornet et al., 1994). It was proposed that the XerC/D recombinases could mediate in these cases first strand exchanges but not the subsequent resolutions of the Holliday junction intermediate (HJ) formed, which would then be processed by XerC/D-independent cellular mechanisms (Cornet et al., 1994; Rajeev et al., 2009; Ramirez et al., 2014; Lin et al., 2020). Similarly, it remains possible that in the absence of complete cr identity between sites the A. baumannii XerC/XerD recombinases could mediate only first strand exchanges followed by HJ resolution by other mechanisms, making the reaction essentially irreversible. The selection of these infrequent events probably depends on the fitness provided by the resulting plasmid ensemble.
How far in time could we trace the existence of pXerC/D sites in A. baumannii? Although environmental A. baumannii strains of non-clinical origin are extremely rare (Antunes et al., 2014), we could recently reclassify as A. baumannii (Repizo et al., 2017, 2020) a collection strain, Acinetobacter sp. NCIMB8209, isolated before 1944 from the enriched microbiota responsible for the aerobic decomposition of the desert shrub guayule in a process aimed for the industrial production of natural latex (Allen et al., 1944). Expectedly from an environmental isolate obtained around or before 1944, WGS analysis of the NCIMB8209 genome indicated a general absence of antimicrobial resistance genes. Most relevantly for this work, however, this analysis also indicated the presence of a large Rep_3 plasmid of 133,709 bp designated pAbNCIMB8209_134 (GenBank accession CP028139.1) in which we detected a pair of directly-oriented pXerC/D sites, namely ATTTCGTATAAGGTGTATTATGTTAATT (positions 48,301 to 48,328) and ATTTCATATAAGGTGTATTATGTTAATT (positions 49,996 to 50,016), flanking a gene cluster composed of a toxin-antitoxin gene cluster (C4X49_18715/C4X49_18720) accompanied by an exonuclease subunit gene (C4X49_18710). Notably, these two sites also shared identical GGTGTA cr sequences. Multiple pXerC/D sites have also been identified in the pAB1 and pAB2 Rep_3 plasmids (Balalovski and Grainge, 2020) present in the A. baumannii ATCC17978 collection strain isolated from a clinical source around 1950 (Piechaud and Second, 1951). These inferences indicated that Rep_3 plasmids carrying pXerC/D-bounded genes were already present in the A. baumannii population before the massive anthropogenic introduction of antimicrobials by the middle of last century (Antunes et al., 2014; Baquero et al., 2021). GGTGTA cr sequences can also be found among 6 out of the 17 non-identical pXerC/D sites identified by us among Ab242/Ab825 plasmids (Cameranesi et al., 2018), and between many of the multiple pXerC/D sites described in other plasmids of many Acinetobacter (and even non-Acinetobacter) strains isolated in different periods and from different sources, both clinical and environmental (Fu et al., 2014; Blackwell and Hall, 2017; Brovedan et al., 2019; Matos et al., 2019; Mindlin et al., 2019; Balalovski and Grainge, 2020; Bonnin et al., 2020; Salgado-Camargo et al., 2020; Wang et al., 2020; Alattraqchi et al., 2021; Hamidian et al., 2021; Liu et al., 2021; Jones et al., 2022). Although it remains to be established what other factors influence the feasibility (or reversibility) of SSR events between sites sharing identical cr sequences, it is likely that some of these sites participate in the remodeling and evolution of Acinetobacter plasmids in the eventuality that they collide in the same cell, with the concomitant accelerated dissemination of the resistance determinants they bound. The whole process could probably be viewed then more adequately from the units-of-evolution perspective (Baquero et al., 2021).
Further work is in progress to address many of the interrogates posed above, including the influences of the different Acinetobacter pXerC/D core sequences and genetic context on the feasibility and directionality of the recombination reaction, as well as the detailed roles of the XerC and XerD recombinases in the recombination process.
Author's Note
Part of the results of this work have been previously presented in the following International Meetings:
1. A. M. Viale, M. Cameranesi, A. Limansky, G. Repizo, and J. Morán-Barrio. Site-specific recombination at XerC/D sites as mediators of plasticity among Acinetobacter baumannii plasmids carrying carbapenem- and aminoglycoside-resistance genetic modules. Plasmid Biology Symposium, Seattle, USA, August 2018.
2. M. M. Cameranesi, J. Morán-Barrio, A. S. Limansky, G. D. Repizo, A. M. Viale. Detection of active pairs of XerC/D recognition sites mediating fusions and inversions in Acinetobacter baumannii plasmids carrying OXA-58 carbapenemase adaptive modules. 12th International Symposium on the Biology of Acinetobacter, Frankfurt, Germany, September 2019.
Data availability statement
The datasets presented in this study can be found in online repositories. The names of the repository/repositories and accession number(s) are as follows. GenBank accession numbers for the Ab242 and Ab825 plasmids included in this work are: pAb242_25, KY984047; pAb242_12, KY984046; pAb242_9, KY984045; pAb825_36, MG100202; pAb25_12, MG100203. GenBank accession number for plasmid pAb244_7 is MG520098. GenBank accession numbers for the sequences of different PCR amplification fragments containing the particular pXerC/D sites and corresponding genetic contexts presented in this study (Supplementary Table S1) are as follows: C2/D2 site, ON060992; C2/D7 site, ON060990; C7/D2 site, ON060991; C9/D7 site, OM876186; C7/D7 site, ON060993; C7/D9 site, OM876185; C9/D9 site, ON060994.
Author contributions
AV, MC, JM-B, and AL conceived and designed the work. MC, LG, and RS performed the experimental work. MC, AV, and JM-B conducted the bioinformatic analysis. AV, MC, LG, RS, JM-B, and AL analyzed and discussed the data. AV wrote the manuscript. All authors contributed to the article and approved the submitted version.
Funding
This work was supported by grants from CONICET PIP 11220170100377CO to JM-B and AV, Agencia Nacional de Promoción Científica y Tecnológica (ANPCyT) PICT-2017-3536 to JM-B, PICT-2019-00074 to AL, and Ministerio de Ciencia, Tecnología e Innovación Productiva, Provincia de Santa Fe, Argentina, to AV and AL. MC is a former fellow of CONICET, LG is a fellow of ANPCyT, RC is a fellow of CONICET, JM-B, and AV are Career Researchers of CONICET, and AL is a Researcher of the National University of Rosario, Argentina.
Acknowledgments
We thank the collaboration of G. Repizo in the bioinformatic analysis.
Conflict of interest
The authors declare that the research was conducted in the absence of any commercial or financial relationships that could be construed as a potential conflict of interest.
Publisher’s note
All claims expressed in this article are solely those of the authors and do not necessarily represent those of their affiliated organizations, or those of the publisher, the editors and the reviewers. Any product that may be evaluated in this article, or claim that may be made by its manufacturer, is not guaranteed or endorsed by the publisher.
Supplementary material
The Supplementary material for this article can be found online at: https://www.frontiersin.org/articles/10.3389/fmicb.2023.1057608/full#supplementary-material
References
Alattraqchi, A. G., Mohd Rani, F., Rahman, N. I. A., Ismail, S., Cleary, D. W., Clarke, S. C., et al. (2021). Complete genome sequencing of Acinetobacter baumannii AC1633 and Acinetobacter nosocomialis AC1530 unveils a large multidrug-resistant plasmid encoding the NDM-1 and OXA-58 carbapenemases. mSphere 6, e01076–e01020. doi: 10.1128/mSphere.01076-20
Allen, P. J., Naghski, J., and Hoover, S. R. (1944). Decomposition of guayule resins by microorganisms. J. Bacteriol. 47, 559–572. doi: 10.1128/jb.47.6.559-572.1944
Andam, C. P. (2019). Clonal yet different: understanding the causes of genomic heterogeneity in microbial species and impacts on public health. mSystems 4, e00097–e00019. doi: 10.1128/mSystems.00097-19
Antunes, L. C., Visca, P., and Towner, K. J. (2014). Acinetobacter baumannii: evolution of a global pathogen. Path Dis. 71, 292–301. doi: 10.1111/2049-632X.12125
Aziz, R. K., Bartels, D., Best, A. A., De Jongh, M., Disz, T., Edwards, R. A., et al. (2008). The RAST Server: rapid annotations using subsystems technology. BMC Genomics 9: 75. doi: 10.1186/1471-2164-9-75
Balalovski, P., and Grainge, I. (2020). Mobilization of pdif modules in Acinetobacter: A novel mechanism for antibiotic resistance gene shuffling? Mol. Microbiol. 114, 699–709. doi: 10.1111/mmi.14563
Baquero, F., Martínez, J. L., F. Lanza, V., Rodríguez-Beltrán, J., Galán, J. C., San Millán, A., et al. (2021). Evolutionary pathways and trajectories in antibiotic resistance. Clin. Microbiol. Rev. 34:e0005019. doi: 10.1128/CMR.00050-19
Blackwell, G. A., and Hall, R. M. (2017). The tet39 determinant and the msrE-mphE genes in Acinetobacter plasmids are each part of discrete modules flanked by inversely oriented pdif (XerC-XerD) sites. Antimicrob. Agents Chemother. 61, e00780–17. doi: 10.1128/AAC.00780-17
Bonnin, R. A., Girlich, D., Jousset, A. B., Gauthier, L., Cuzon, G., Bogaerts, P., et al. (2020). A single Proteus mirabilis lineage from human and animal sources: a hidden reservoir of OXA-23 or OXA-58 carbapenemases in Enterobacterales. Sci. Rep. 10:9160. doi: 10.1038/s41598-020-66161-z
Brito, B. P., Koong, J., Wozniak, A., Opazo-Capurro, A., To, J, Garcia, P., et al. (2022). Genomic analysis of carbapenem-resistant Acinetobacter baumannii strains recovered from Chilean hospitals reveals lineages specific to South America and multiple routes for acquisition of antibiotic resistance genes. Microbiol. Spectr. 10:e0246322. doi: 10.1128/spectrum.02463-22
Brovedan, M., Repizo, G. D., Marchiaro, P., Viale, A. M., and Limansky, A. (2019). Characterization of the diverse plasmid pool harbored by the blaNDM-1-containing Acinetobacter bereziniae HPC229 clinical strain. PLoS One 14:e0220584. doi: 10.1371/journal.pone.0220584
Cameranesi, M. M., Morán-Barrio, J., Limansky, A. S., Repizo, G., and Viale, A. M. (2017). Three novel Acinetobacter baumannii plasmid replicase-homology groups inferred from the analysis of a multidrug-resistant clinical strain isolated in Argentina. J. Infect. Dis. Epidemiol. 3, 1–5. doi: 10.23937/2474-3658/1510046
Cameranesi, M. M., Morán-Barrio, J., Limansky, A. S., Repizo, G. D., and Viale, A. M. (2018). Site-specific recombination at XerC/D sites mediates the formation and resolution of plasmid co-integrates carrying a blaOXA-58- and TnaphA6-resistance module in Acinetobacter baumannii. Front. Microbiol. 9:66. doi: 10.3389/fmicb.2018.00066
Cameranesi, M. M., Paganini, J., Limansky, A. S., Moran-Barrio, J., Salcedo, S. P., Viale, A. M., et al. (2020). Acquisition of plasmids conferring carbapenem and aminoglycoside resistance and loss of surface-exposed macromolecule structures as strategies for the adaptation of Acinetobacter baumannii CC104O/CC15P strains to the clinical setting. Microbial Genomi. 6:mgen000360. doi: 10.1099/mgen.0.000360
Carattoli, A. (2013). Plasmids and the spread of resistance. Int. J. Med. Microbiol. 303, 298–304. doi: 10.1016/j.ijmm.2013.02.001
Carruthers, M. D., Harding, C. M., Baker, B. D., Bonomo, R. A., Hujer, K. M., Rather, P. N., et al. (2013). Draft genome sequence of the clinical isolate Acinetobacter nosocomialis strain M2. Genome Announc. 1, e00906–e00913. doi: 10.1128/genomeA.00906-13
Colloms, S. D., McCulloch, R., Grant, K., Neilson, L., and Sherratt, D. J. (1996). Xer-mediated site-specific recombination in vitro. EMBO J. 15, 1172–1181. doi: 10.1002/j.1460-2075.1996.tb00456.x
Cornet, F., Mortier, I., Patte, J., and Louarn, J. M. (1994). Plasmid pSC101 harbors a recombination site, psi, which is able to resolve plasmid multimers and to substitute for the analogous chromosomal Escherichia coli site dif. J. Bacteriol. 176, 3188–3195. doi: 10.1128/jb.176.11.3188-3195.1994
Crozat, E., Fournes, F., Cornet, F., Hallet, B., and Rousseau, P. (2014). Resolution of multimeric forms of circular plasmids and chromosomes. Microbiol. Spectr. 2. doi: 10.1128/microbiolspec.PLAS-0025-2014
Da Silva, G. J., and Domingues, S. (2016). Insights on the horizontal gene transfer of Carbapenemase determinants in the opportunistic pathogen Acinetobacter baumannii. Microorganisms 4:29. doi: 10.3390/microorganisms4030029
Didelot, X., Walker, A. S., Peto, T. E., Crook, D. W., and Wilson, D. J. (2016). Within-host evolution of bacterial pathogens. Nat. Rev. Microbiol. 14, 150–162. doi: 10.1038/nrmicro.2015.13
Dionisio, F., Zilhão, R., and Gama, J. A. (2019). Interactions between plasmids and other mobile genetic elements affect their transmission and persistence. Plasmid 102, 29–36. doi: 10.1016/j.plasmid.2019.01.003
Evans, B. A., and Amyes, S. G. (2014). OXA β-lactamases. Clin. Microbiol. Rev. 27, 241–263. doi: 10.1128/CMR.00117-13
Fu, Y., Jiang, J., Zhou, H., Jiang, Y., Fu, Y., Yu, Y., et al. (2014). Characterization of a novel plasmid type and various genetic contexts of Bla OXA-58 in Acinetobacter spp. from multiple cities in China. PLoS One 9:e84680. doi: 10.1371/journal.pone.0084680
García-Pastor, L., Puerta-Fernández, E., and Casadesús, J. (2019). Bistability and phase variation in Salmonella enterica. Biochim. Biophys. Acta - Gene Regul. Mech. 1862, 752–758. doi: 10.1016/j.bbagrm.2018.01.003
Hamidian, M., Ambrose, S. J., Blackwell, G. A., Nigro, S. J., and Hall, R. M. (2021). An outbreak of multiply antibiotic-resistant ST49:ST128:KL11:OCL8 Acinetobacter baumannii isolates at a Sydney hospital. J. Antimicrob. Chemother. 76, 893–900. doi: 10.1093/jac/dkaa553
Hamidian, M., and Nigro, S. J. (2019). Emergence, molecular mechanisms and global spread of carbapenem-resistant Acinetobacter baumannii. Microb. Genom. 5:e000306. doi: 10.1099/mgen.0.000306
Hamidian, M., and Hall, R. M. (2018). Genetic structure of four plasmids found in Acinetobacter baumannii isolate D36 belonging to lineage 2 of global clone 1. PloS One. 13:e0204357. doi: 10.1371/journal.pone.0204357
Harmer, C. J., Moran, R. A., and Hall, R. M. (2014). Movement of IS26-associated antibiotic resistance genes occurs via a translocatable unit that includes a single IS26 and preferentially inserts adjacent to another IS26. MBio 5, e01801–e01814. doi: 10.1128/mBio.01801-14
Jiang, X., Hall, A. B., Arthur, T. D., Plichta, D. R., Covington, C. T., Poyet, M., et al. (2019). Invertible promoters mediate bacterial phase variation, antibiotic resistance, and host adaptation in the gut. Science 363, 181–187. doi: 10.1126/science.aau5238
Jones, N. I., Harmer, C. J., Hamidian, M., and Hall, R. M. (2022). Evolution of Acinetobacter baumannii plasmids carrying the oxa58 carbapenemase resistance gene via plasmid fusion, IS26-mediated events and dif module shuffling. Plasmid 121:102628. doi: 10.1016/j.plasmid.2022.102628
Labrador-Herrera, G., Pérez-Pulido, A. J., Álvarez-Marín, R., Casimiro-Soriguer, C. S., Cebrero-Cangueiro, T., Morán-Barrio, J., et al. (2020). Virulence role of the outer membrane protein CarO in carbapenem-resistant Acinetobacter baumannii. Virulence 11, 1727–1737. doi: 10.1080/21505594.2020.1855912
Lean, S. S., and Yeo, C. C. (2017). Small, enigmatic plasmids of the nosocomial pathogen, Acinetobacter baumannii: good, bad, who knows? Front. Microbiol. 8:1547. doi: 10.3389/fmicb.2017.01547
Lin, D. L., Traglia, G. M., Baker, R., Sherratt, D. J., Ramirez, M. S., and Tolmasky, M. E. (2020). Functional analysis of the Acinetobacter baumannii XerC and XerD site-specific recombinases: potential role in dissemination of resistance genes. Antibiotics 9:405. doi: 10.3390/antibiotics9070405
Liu, H., Moran, R. A., Chen, Y., Doughty, E. L., Hua, X., Jiang, Y., et al. (2021). Transferable Acinetobacter baumannii plasmid pDETAB2 encodes OXA-58 and NDM-1 and represents a new class of antibiotic resistance plasmids. J. Antimicrob. Chemother. 76, 1130–1134. doi: 10.1093/jac/dkab005
Matos, A. P., Cayô, R., Almeida, L., Streling, A. P., Nodari, C. S., Martins, W., et al. (2019). Genetic characterization of plasmid-borne blaOXA-58 in distinct Acinetobacter species. mSphere 4, e00376–e00319. doi: 10.1128/mSphere.00376-19
Merrikh, C. N., and Merrikh, H. (2018). Gene inversion potentiates bacterial evolvability and virulence. Nat. Commun. 9:4662. doi: 10.1038/s41467-018-07110-3
Mindlin, S., Beletsky, A., Mardanov, A., and Petrova, M. (2019). Adaptive dif modules in permafrost strains of Acinetobacter lwoffii and their distribution and abundance among present day Acinetobacter strains. Front. Microbiol. 10:632. doi: 10.3389/fmicb.2019.00632
Mindlin, S., Beletsky, A., Rakitin, A., Mardanov, A., and Petrova, M. (2020). Acinetobacter plasmids: diversity and development of classification strategies. Front. Microbiol. 11:588410. doi: 10.3389/fmicb.2020.588410
Mussi, M. A., Limansky, A. S., and Viale, A. M. (2005). Acquisition of resistance to carbapenems in multidrug-resistant clinical strains of Acinetobacter baumannii: natural insertional inactivation of a gene encoding a member of a novel family of beta-barrel outer membrane proteins. Antimicrob. Agents Chemother. 49, 1432–1440. doi: 10.1128/AAC.49.4.1432-1440.2005
Piechaud, M., and Second, L. (1951). Etude de 26 souches de Moraxella lwoffi [studies of 26 strains of Moraxella lwoffi]. Ann. Inst. Pasteur 80, 97–99.
Rajeev, L., Malanowska, K., and Gardner, J. F. (2009). Challenging a paradigm: the role of DNA homology in tyrosine recombinase reactions. Microbiol. Mol. Biol. Rev. 73, 300–309. doi: 10.1128/MMBR.00038-08
Ramirez, M. S., Traglia, G. M., Lin, D. L., Tran, T., and Tolmasky, M. E. (2014). Plasmid-mediated antibiotic resistance and virulence in Gram-negatives: the Klebsiella pneumoniae paradigm. Microbiol. Spectr. 2, 1–15. doi: 10.1128/microbiolspec.PLAS-0016-2013
Ravasi, P., Limansky, A. S., Rodriguez, R. E., Viale, A. M., and Mussi, M. A. (2011). ISAba825, a functional insertion sequence modulating genomic plasticity and bla(OXA-58) expression in Acinetobacter baumannii. Antimicrob. Agents Chemother. 55, 917–920. doi: 10.1128/AAC.00491-10
Repizo, G. D., Espariz, M., Seravalle, J. L., Díaz Miloslavich, J. I., Steimbrüch, B. A., Shuman, H. A., et al. (2020). Acinetobacter baumannii NCIMB8209: a rare environmental strain displaying extensive insertion sequence-mediated genome remodeling resulting in the loss of exposed cell structures and defensive mechanisms. mSphere 5, e00404–e00420. doi: 10.1128/mSphere.00404-20
Repizo, G. D., Viale, A. M., Borges, V., Cameranesi, M. M., Taib, N., Espariz, M., et al. (2017). The environmental Acinetobacter baumannii isolate DSM30011 reveals clues into the Preantibiotic era genome diversity, virulence potential, and niche range of a predominant nosocomial pathogen. Genome Biol. Evol. 9, 2292–2307. doi: 10.1093/gbe/evx162
Roca, I., Espinal, P., Vila-Farrés, X., and Vila, J. (2012). The Acinetobacter baumannii oxymoron: commensal hospital dweller turned Pan-drug-resistant menace. Front. Microbiol. 3:148. doi: 10.3389/fmicb.2012.00148
Salgado-Camargo, A. D., Castro-Jaimes, S., Gutierrez-Rios, R. M., Lozano, L. F., Altamirano-Pacheco, L., Silva-Sanchez, J., et al. (2020). Structure and evolution of Acinetobacter baumannii plasmids. Front. Microbiol. 11:1283. doi: 10.3389/fmicb.2020.01283
Salto, I. P., Torres Tejerizo, G., Wibberg, D., Pühler, A., Schlüter, A., and Pistorio, M. (2018). Comparative genomic analysis of Acinetobacter spp. plasmids originating from clinical settings and environmental habitats. Sci. Rep. 8:7783. doi: 10.1038/s41598-018-26180-3
Schroeder, J. W., Sankar, T. S., Wang, J. D., and Simmons, L. A. (2020). The roles of replication-transcription conflict in mutagenesis and evolution of genome organization. PLoS genetics. 16, e1008987. doi: 10.1371/journal.pgen.1008987
Siguier, P., Perochon, J., Lestrade, L., Mahillon, J., and Chandler, M. (2006). ISfinder: the reference centre for bacterial insertion sequences. Nucleic Acids Res. 34, D32–D36. doi: 10.1093/nar/gkj014
Shintani, M., Sanchez, Z. K., and Kimbara, K. (2015). Genomics of microbial plasmids: classification and identification based on replication and transfer systems and host taxonomy. Front. Microbiol. 6:242. doi: 10.3389/fmicb.2015.00242
Trzilova, D., and Tamayo, R. (2021). Site-specific recombination - how simple DNA inversions produce complex phenotypic heterogeneity in bacterial populations. Trends Genet. 37, 59–72. doi: 10.1016/j.tig.2020.09.004
Varani, A. M., Siguier, P., Gourbeyre, E., Charneau, V., Schlüter, A., and Chandler, M. (2011). ISsaga is an ensemble of web-based methods for high throughput identification and semi-automatic annotation of insertion sequences in prokaryotic genomes. Genome Biol. 12:R30. doi: 10.1186/gb-2011-12-3-r30
Wang, J., Wang, Y., Wu, H., Wang, Z. Y., Shen, P. C., Tian, Y. Q., et al. (2020). Coexistence of blaOXA-58 and tet(X) on a novel plasmid in Acinetobacter sp. from pig in Shanghai, China. Front. Microbiol. 11:578020. doi: 10.3389/fmicb.2020.578020
Zarrilli, R., Vitale, D., Di Popolo, A., Bagattini, M., Daoud, Z., Khan, A. U., et al. (2008). A plasmid-borne blaOXA-58 gene confers imipenem resistance to Acinetobacter baumannii isolates from a Lebanese hospital. Antimicrob. Agents Chemother. 52, 4115–4120. doi: 10.1128/AAC.00366-08
Zander, E., Fernández-González, A., Schleicher, X., Dammhayn, C., Kamolvit, W., Seifert, H., et al. (2014). Worldwide dissemination of acquired carbapenem-hydrolysing class D β-lactamases in Acinetobacter spp. other than Acinetobacter baumannii. Int. J. Antimicrob. Agents 43, 375–377. doi: 10.1016/j.ijantimicag.2014.01.012
Keywords: Acinetobacter baumannii, carbapenem resistance, resistance plasmids, OXA-58 carbapenemase, XerC/D site-specific recombination, multiple pXerC/D sites, plasmid shuffling, plasmid dynamics and evolution
Citation: Giacone L, Cameranesi MM, Sanchez RI, Limansky AS, Morán-Barrio J and Viale AM (2023) Dynamic state of plasmid genomic architectures resulting from XerC/D-mediated site-specific recombination in Acinetobacter baumannii Rep_3 superfamily resistance plasmids carrying blaOXA-58- and TnaphA6-resistance modules. Front. Microbiol. 14:1057608. doi: 10.3389/fmicb.2023.1057608
Edited by:
Raffaele Zarrilli, University of Naples Federico II, ItalyReviewed by:
Filipa Grosso, University of Porto, PortugalGerman Matias Traglia, Universidad de la República, Uruguay
Copyright © 2023 Giacone, Cameranesi, Sanchez, Limansky, Morán-Barrio and Viale. This is an open-access article distributed under the terms of the Creative Commons Attribution License (CC BY). The use, distribution or reproduction in other forums is permitted, provided the original author(s) and the copyright owner(s) are credited and that the original publication in this journal is cited, in accordance with accepted academic practice. No use, distribution or reproduction is permitted which does not comply with these terms.
*Correspondence: Jorgelina Morán-Barrio, ✉ moran@ibr-conicet.gov.ar; Alejandro M. Viale, ✉ viale@ibr-conicet.gov.ar
†These authors have contributed equally to this work and share first authorship
‡Present Address: M. Marcela Cameranesi, Department of Biochemistry and Molecular Pharmacology, New York University School of Medicine, New York, NY, United States