- 1National Reference Laboratory of Antibiotic Resistances and Healthcare Associated Infections, Department of Infectious Diseases, National Institute of Health Doutor Ricardo Jorge, Lisbon, Portugal
- 2Centre for the Studies of Animal Science, Institute of Agrarian and Agri-Food Sciences and Technologies, Oporto University, Oporto, Portugal
- 3Microbiology and Mycology Laboratory, Instituto Nacional de Investigação Agrária e Veterinária, Lisbon, Portugal
- 4Innovation and Technology Unit, Human Genetics Department, National Institute of Health Doutor Ricardo Jorge, Lisbon, Portugal
Morganella morganii is a commensal bacterium and opportunistic pathogen often present in the gut of humans and animals. We report the 4.3 Mbp draft genome sequence of a M. morganii isolated in association with an Escherichia coli from broilers in Portugal that showed macroscopic lesions consistent with colisepticemia. The analysis of the genome matched the multidrug resistance phenotype and enabled the identification of several clinically important and potentially mobile acquired antibiotic resistance genes, including the plasmid-mediated quinolone resistance determinant qnrD1. Mobile genetic elements, prophages, and pathogenicity factors were also detected, improving our understanding toward this human and animal opportunistic pathogen.
Introduction
The Gram negative Morganella morganii belongs to the tribe Proteeae of the family Enterobacteriaceae (O’Hara et al., 2000). This species, along with other elements of Proteus and Providencia genera can be found in the normal flora of humans, reptiles and in the wider environment (O’Hara et al., 2000; Lee and Liu, 2006; Dipineto et al., 2014). However, M. morganii isolates also constitute clinically relevant opportunistic pathogens, which can cause a variety of infections. Nosocomial outbreaks have been reported, suggesting that infections caused by M. morganii can lead to major clinical problems, such as wounds, urinary tract infections and septicemia (Nicolle, 2001; Tsanaktsidis et al., 2003; Falagas et al., 2006; Lee and Liu, 2006; Lin et al., 2015).
This bacterium has also been associated with infections in animals and with human animal bite wound infections, which suggests that M. morganii may also cause zoonotic infectious diseases (Ono et al., 2001; Choi et al., 2002; Abrahamian and Goldstein, 2011; Zhao et al., 2012; Di Ianni et al., 2015).
Several factors can affect the progression and severity of an infection. The presence of pathogenicity determinants is essential to the success of M. morganii in any environment, particularly in food animal farms, where the pressure caused by antibiotic treatments and the lack of prophylactic measures to avoid the spread of infectious diseases are usually noteworthy (Chen et al., 2012; Lin et al., 2015). It is globally accepted that horizontal gene transfer plays an important role in the dissemination of antibiotic resistance genes and pathogenicity factors (Huddleston, 2014). Considering that M. morganii may share the habitat with other clinically relevant pathogens, the investigation of any multidrug resistant isolate recovered from poultry is an important assignment.
Resistance to quinolones and fluoroquinolones has been increasingly reported among human and veterinary isolates, very likely as a consequence of the great usage of those antibiotics (Tamang et al., 2011). The qnrD gene, now denominated qnrD1 due to the report of a second variant of the gene (Abgottspon et al., 2014), is a relatively uncommon antibiotic resistance gene, which has been described in members of the Proteeae family from different origins (Mazzariol et al., 2012; Zhang et al., 2013; Nasri Yaiche et al., 2014). This plasmid-mediated quinolone resistance (PMQR) determinant encodes a protein that protects DNA gyrases and topoisomerases from quinolone inhibition (Cavaco et al., 2009; Jacoby et al., 2014). Carriage of PMQR-encoding genes frequently confers modest increases to the minimum inhibitory concentrations (MIC) of fluoroquinolones (Poirel et al., 2012). Current studies have identified the environment, particularly animals and aquatic habitats, as a reservoir of PMQR genes (Poirel et al., 2012).
The aim of this study was to investigate the molecular background sustaining the multidrug resistance and pathogenicity of a M. morganii isolate. In this study, we report the antibiotic susceptibility and the draft genome sequence of a qnrD1-harboring avian isolate. The data gathered from bioinformatics analysis may improve our understanding toward this opportunistic pathogen.
Materials and Methods
Bacterial Isolation, Antibiotic Susceptibility, and Molecular Characterization
Morganella morganii INSRALV892a was recovered in association with E. coli INSRALV892b in 2012 from a 13-days old broiler, recovered from a poultry industrial unit in Portugal. Samples consisted of organs (macerates of liver and spleen) collected during post-mortem examination that were submitted for bacteriological analysis. During post-mortem examination, the birds showed macroscopic lesions consistent with colisepticemia: aerosaculitis, acute enteritis, perihepatitis, and fibrinous peritonitis. Suspected Enterobacteriaceae colonies obtained in MacConkey agar plates were isolated in non selective media and identification was performed using API 20E strips (BioMérieux, Marcy-l’Étoile).
Minimum inhibitory concentrations were determined for both isolates by agar dilution method to ten antibiotics: ampicillin, cefotaxime, ceftazidime, meropenem, ciprofloxacin, gentamicin, chloramphenicol, trimethoprim, colistin, and tigecycline. To assess non-susceptibility, interpretation of results was performed according to the clinical breakpoints of the European Committee on Antimicrobial Susceptibility Testing (EUCAST)1.
Plasmid-mediated quinolone resistance [QnrA, QnrB, QnrC, QnrD, QnrS, QepA, OqxAB, and Aac(6′)-Ib-cr]-, β-lactamase (TEM, SHV, OXA-G1, and CTX-M)-, and integrase (class 1, 2, and 3)-encoding genes were identified by PCR and confirmed by sequencing using DNA of both isolates, as previously described (Clemente et al., 2013).
The transference ability of specific antibiotic resistance genes from M. morganii INSRALV892a and E. coli INSRALV892b was assessed by broth mating out assays using E. coli J53 NaN3R as recipient strain, as described elsewhere (Jones-Dias et al., 2016). Resistant E. coli J53 transconjugants were then selected on MacConkey agar plates containing amoxicillin (100 mg/l) or ciprofloxacin (0.06 mg/l) together with sodium azide (200 mg/l), according with the antibiotic susceptibility profile of the donor isolates. To confirm the acquisition of the antibiotic resistance genes, we detected and identified the determinants in the transconjugants, following the methodology described above in this section.
Genome Sequencing and Analysis
Genomic DNA of M. morganii INSRALV892a was extracted using DNeasy Blood and Tissue Kit (Qiagen, Aarhus), and DNA quantification was performed by Qubit Fluorometric Quantitation (Thermo Fisher Scientific, Carlsbad, CA, USA), according to the manufacturer’s instructions. Libraries were prepared from 1 ng of genomic DNA using the Nextera XT DNA Sample Preparation Kit (Illumina, San Diego, CA, USA), also following manufacturer’s instructions. Whole Genome Sequencing (WGS) was performed using 150 bp paired-end reads on a MiSeq (Illumina, San Diego, CA, USA).
Sequence reads were then trimmed and filtered according to quality criteria. Briefly, reads were assembled de novo using CLC genomics workbench version 8.5 (Qiagen, Aarhus), which is based on Smith and Waterman algorithm. The raw FASTQ reads were first processed by quality score trimming (quality score limit = 0.05), removing all reads containing more than two ambiguous nucleotides or shorter than 50 bp. Trimmed reads were then de novo assembled with automatic bubble, word size and paired distance detection, using mapping mode “map reads back to contigs” (including scaffolding, and minimum contig length of 400 nucleotides). The NCBI prokaryotic genome automatic annotation pipeline (PGAAP) was used for annotation2. All de novo contigs were BLAST searched against the GenBank’s non-redundant nucleotide collection (nr/nt). PathogenFinder 1.1, ResFinder 2.1, and PlasmidFinder 1.3 were used to estimate the number and type of pathogenicity determinants, antibiotic resistance genes and plasmids, respectively, within the genome (Zankari et al., 2012; Cosentino et al., 2013; Carattoli et al., 2014). PHAST search web tool was used to identify and annotate any prophage sequence present in the draft genome (Zhou et al., 2011). ISsaga semi-automatic annotation system was also applied to detect the presence of insertion sequences (IS) (Varani et al., 2011).
Contigs containing antibiotic resistance genes were searched for identity through blastn3 against the nr/nt NCBI database, and further mapped against the closest bacterial plasmids or genomes using CLC Genomics Workbench version 8.5.
Nucleotide Sequence GenBank Accession Numbers
This Whole Genome Shotgun project has been deposited at DDBJ/EMBL/GenBank under the accession LGYC00000000. The version described in this paper is the LGYC010000004.
Results and Discussion
Morganella morganii INSRALV892a was found to be non-susceptible to ampicillin (>64 mg/L), cefotaxime (>4 mg/L), ceftazidime (2 mg/L), ciprofloxacin (>8 mg/L), chloramphenicol (16 mg/L), gentamicin (>32 mg/L), trimethoprim (>32 mg/L), colistin (>16 mg/L), and tigecycline (0.5 mg/L). However, it is important to highlight that M. morganii is intrinsically resistant to colistin, while tigecycline has also been shown to have poor activity against this species5. Among the antibiotics tested, the isolate was susceptible only to meropenem (0.125 mg/L). The E. coli INSRALV892b was also characterized with regard to antibiotic susceptibility and found to be non-susceptible to ampicillin (>64 mg/L), cefotaxime (>4 mg/L), ceftazidime (2 mg/L) and trimethoprim (>32 mg/L), and susceptible to meropenem (≤0.03 mg/L), ciprofloxacin (0.125 mg/L), chloramphenicol (≤8 mg/L), gentamicin (≤0.5 mg/L), colistin (≤1 mg/L), and tigecycline (0.5 mg/L). The molecular characterization of the isolates showed the presence of qnrD1 and a class 2 integron in M. morganii INSRALV892a, and blaCTX-M-1 gene flanked by an ISEcp1 and orf477, as well as a class 1 integron in E. coli INSRALV892b. Conjugation experiments only revealed the transference of blaCTX-M-1 from E. coli INSRALV892b to isogenic E. coli J53 strain.
The WGS assembly of M. morganii INSRALV892a yielded 74 contigs (each >200 bp long and >100-fold coverage), which together comprised 4,267,817 bp, showing a GC content of 50.6%. The largest contig was 523,676 bp long and the N50 statistic, which stands for the minimum contig length of at least 50% of the contigs, was 342,352 bp. The average length of the obtained contigs was 34,190 bp. Among the obtained data, six contigs, ranging from 802 to 8,575 in length and showing a minimum coverage of 117.7-fold, matched plasmid sequences of different species. Overall, the genome sequence comprised 4,116 putative genes, among which 3,950 consisted of protein encoding sequences.
In silico analysis of the antibiotic resistance genes (90% identity and 40% minimum length) revealed the presence of loci for acquired resistance to aminoglycosides [aadA1y, aph(3′)-Ic, and strA-strB], β-lactam (blaOXA-1), fluoroquinolones (qnrD1, aac(6′)-Ib-cr), phenicols (catA2 and catB3), rifampicin (Δarr), sulphonamides (sul2), trimethoprim (dfrA1), tetracycline (tetY), and streptothricin (sat2). Non-susceptibility to third generation cephalosporins such as cefotaxime and ceftazidime was not associated to any extended-spectrum β-lactamase, suggesting the involvement of inducible or stably derepressed M. morganii chromosomal ampC gene, the blaDHA-type gene (Harris and Ferguson, 2012).
The dfrA1, catB2, sat2, and aadA1y genes were enclosed in an In2-17 class 2 integron that has already been described, for instance, in Proteus vulgaris isolates from China (HQ386830) (Figure 1A). Genes encoding resistance to tetracycline (tetY) and streptomycin (strA-strB) were detected in association with each other, and with proteins linked to DNA transfer processes, such as ISAba14 and an incomplete Tn5393 (Figure 1B). The sulphonamide resistance gene sul2 was flanked upstream by a glmM-containing region and ISCR2, while the downstream region consisted of a chromosomal region typical of M. morganii (Figure 1C); the glmM gene (formerly called ureC) encodes a phosphoglucosamine mutase that is considered a housekeeping gene essential for the cell wall synthesis (Tavares et al., 2003). The aminoglycoside resistance gene aph(3′)-Ic was associated to an IS26 IR and a unknown orf (Figure 1D), and the genes Δarr, catB3, blaOXA-1, and aac(6′)-Ib-cr were enclosed together, as gene cassettes of an integron variable region that has been previously found, for instance, in S. enterica from livestock (Figure 1E). However, in the latter, the array was flanked up and downstream by truncated inverted repeats of IS26 while no conserved integron regions were found. The genetic regions where antibiotic resistance genes were incorporated were highly similar to other plasmid-borne structures, previously described in different Gram-negative bacteria, suggesting acquisition of resistance determinants through horizontal gene transfer (Figure 1).
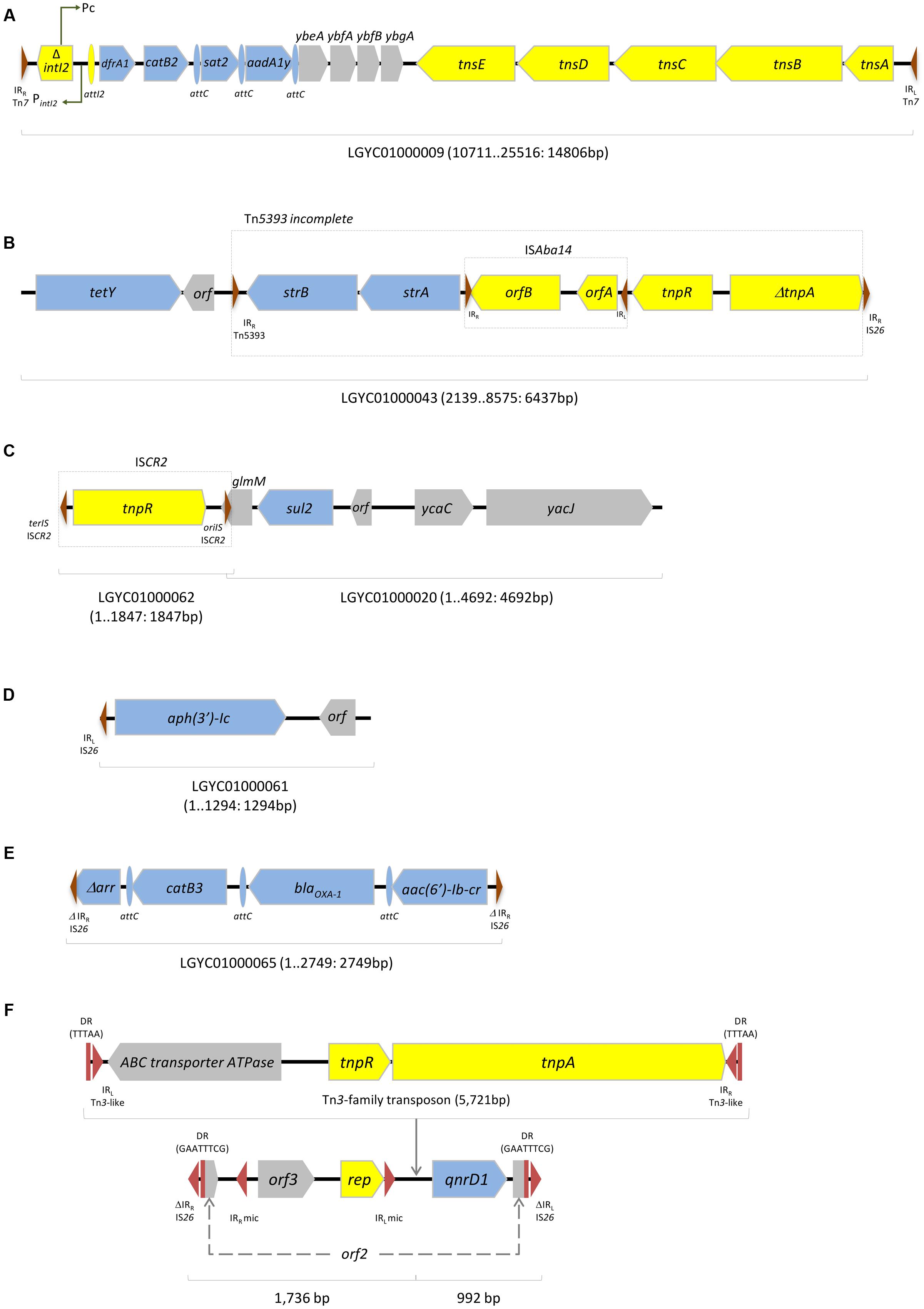
FIGURE 1. Examples of contigs containing antibiotic resistance genes in Morganella morganii INSRALV892a. (A) In2-17 class 2 integron encoding dfrA1, catB2, sat2, and aadA1y; (B) tetY and strA-strB associated to transposable elements; (C) sul2 flanked upstream by ISCR2; (D) aph(3′)-Ic associated to an IS26 IR; (E)Δarr, catB3, blaOXA-1, and aac(6′)-Ib-cr are bracketed by truncated IS26 IR. (F) qnrD1-containing region. Blue, antibiotic resistance genes; Yellow, mobile genetic elements; Gray, other genes. Right and left inverted repeats (IRR and IRL) and direct repeats (DR) are indicated as red triangles and squares, respectively; mic, mobile insertion cassette.
The qnrD1 gene was enclosed in an 8,449 bp length contig (LGYC01000051: mean coverage of 183.9-fold and a total read count of 13,382), matching a Col3M plasmid. Indeed, the qnrD1 gene is frequently located on small non-conjugative plasmids harbored by Proteeae, which was corroborated by our conjugation assay (Zhang et al., 2013). Furthermore, the qnrD1 gene has been located in plasmids showing similarities with a specific Providencia vermicola plasmid, suggesting that these small non-conjugative plasmids might be the product of recombination between an unknown qnrD-bearing region and a native plasmid from Proteeae.
This contig accommodated a 2,683 bp sequence showing 99% identity with previously described qnrD1-harboring plasmids, such as pGHS09-09a (HQ834473) and pCGS49 (JQ776507), reported in France and China, respectively. The three qnrD1-encoding sequences shared a rep gene (also reported as orf4) and two additional orfs (Figure 1F) (Guillard et al., 2012; Zhang et al., 2013). Six single nucleotide variants (SNVs) were detected between qnrD1-INSRALV892a and the pGHS09-09a plasmid, two within the rep gene. Only one SNP was found with relation to pCGS49 in a non-coding region. The qnrD1 gene was located within a mobile insertion cassette (mic) element bracketed by two inverted repeats, as previously described (Guillard et al., 2014) (Figure 1F).
Comparative bioinformatics analyses revealed the disruption of orf2 caused by the insertion of IS26 left and right inverted repeats flanking a region containing orf3 and rep, within a mic, followed by qnrD1. In fact, this shows that is possible that LGYC01000051 contig could be either a Col3M plasmid missing an IS26-flanked region or a qnrD1-containing region that has become incorporated into a larger plasmid.
In addition, this region (Figure 1F) included three additional open reading frames: besides an ABC transporter-encoding gene perfectly matching a protein from Aeromonas hydrophila, this region harbored Tn3-like resolvases- and transposase-encoding genes, displaying E. coli plasmid pH226B (KX129784) as its best blast hit.
Mobile genetic elements are crucial tools for the acquisition of genetic diversity (Huddleston, 2014). Thus, we decided to search for and characterize the elements detected in the M. morganii’s genome. We identified 10 prophage regions, among which six were incomplete and four were intact, comprising 381 prophage-related genes. Intact prophage regions presented between 24.2 and 41.7 Kb and harbored 13–56 coding DNA sequences. The intact phages showing highest scores were assigned to Enterobacteria phage SfV, which is associated with O-antigen modification and serotype conversion in Shigella flexneri, and Enterobacteria phage mEp235 that consists of an unclassified Lambda-like virus (Sun et al., 2013). The bioinformatics detection of IS resulted in the identification of seven transposable elements: IS3, Tn3, ISL3, IS256, IS6, IS91, and ISAs1. Besides the already mentioned Col3M no other typable plasmids were detected within the M. morganii genome, according with the PlasmidFinder tool.
Based on the probability scores assigned by PathogenFinder web-server (Cosentino et al., 2013), the isolate has a probability of acting as a human pathogen of 68.9%, which is in line with the opportunistic nature of this species. M. morganii’s genome matched 22 pathogenic families and 5 non-pathogenic families. Pathogenic factors showed diversity of functions and hosts, and included, for instance, transposase insA from IS91 of Salmonella enterica, transposition protein tnsE of the Tn7 transposon of S. flexneri, and transcriptional regulator LysR family protein from S. enterica.
Multidrug resistant M. morganii isolates are rare and normally associated with non invasive nosocomial opportunistic infections in humans (Nicolle, 2001; Falagas et al., 2006). The detection of an avian M. morganii isolate harboring multiple and mobile antibiotic resistance genes and pathogenicity factors raises concerns regarding the dissemination of infection in birds and potential risk of zoonotic transmission. Several factors may affect the susceptibility of poultry to bacterial diseases, namely environmental stressors and previous antibiotic treatments, which are crucial to the development of infections involving different Enterobacteriaceae (Burkholder et al., 2008). The detection of an avian M. morganii isolate harboring multiple and mobile antibiotic resistance genes and pathogenicity factors raises concerns regarding the dissemination of infection in birds and potential risk of zoonotic transmission.
M. morganii is a well characterized opportunistic pathogen (Lee and Liu, 2006). However, its detection in poultry flocks, co-habiting the same hosts as other clinically important pathogens, makes it susceptible to the acquisition and donation of pathogenicity factors by horizontal gene transfer (Huddleston, 2014). To the best of our knowledge this report represents the first genome analysis of an isolate from animal origin carrying qnrD1. This genome sequence represents a valuable resource for studies on the epidemiology of zoonotic M. morganii isolates, and its features may be used as markers for the study of antibiotic resistance.
Author Contributions
DJ-D designed the study, performed molecular experiments, analyzed the data and wrote the manuscript. LC performed the microbiological experiments and reviewed the manuscript. IM analyzed the data and reviewed the manuscript. TA, acquired laboratory data. DS and LV performed genome sequencing experiments. VM designed the study, analyzed the data and reviewed the manuscript. MC designed the study, reviewed and edited the manuscript. All authors read and approved the final manuscript.
Funding
DJ-D has received research funding from Fundação para a Ciência e a Tecnologia (FCT, grant number SFRH/BD/80001/2011). VM was supported by FCT fellowship (grant SFRH/BPD/77486/2011), financed by the European Social Funds (COMPETE-FEDER) and national funds of the Portuguese Ministry of Education and Science (POPH-QREN). We thank the support of FCT grant number PEst-OE/AGR/UI0211/2011-2014 and UID/MULTI/00211/2013.
Conflict of Interest Statement
The authors declare that the research was conducted in the absence of any commercial or financial relationships that could be construed as a potential conflict of interest.
Footnotes
- ^ http://www.eucast.org
- ^ http://www.ncbi.nlm.nih.gov/genome/annotation_prok/
- ^ http://blast.ncbi.nlm.nih.gov/Blast.cgi
- ^ http://www.ncbi.nlm.nih.gov/nuccore/LGYC00000000.1
- ^ http://www.eucast.org
References
Abgottspon, H., Zurfluh, K., Nüesch-Inderbinen, M., Hächler, H., and Stephan, R. (2014). Quinolone resistance mechanisms in Salmonella enterica serovars Hadar, Kentucky, Virchow, Schwarzengrund, and 4,5,12:i:-, isolated from humans in Switzerland, and identification of a novel qnrD variant, qnrD2, in S. Hadar. Antimicrob. Agents Chemother. 58, 3560–3563. doi: 10.1128/AAC.02404-14
Abrahamian, F. M., and Goldstein, E. J. C. (2011). Microbiology of animal bite wound infections. Clin. Microbiol. Rev. 24, 231–246. doi: 10.1128/CMR.00041-10
Burkholder, K. M., Thompson, K. L., Einstein, M. E., Applegate, T. J., and Patterson, J. A. (2008). Influence of stressors on normal intestinal microbiota, intestinal morphology, and susceptibility to Salmonella enteritidis colonization in broilers. Poult. Sci. 87, 1734–1741. doi: 10.3382/ps.2008-00107
Carattoli, A., Zankari, E., Garcia-Fernandez, A., Voldby Larsen, M., Lund, O., Villa, L., et al. (2014). PlasmidFinder and pMLST: in silico detection and typing of plasmids. Antimicrob. Agents Chemother. 58, 3895–3903. doi: 10.1128/AAC.02412-14
Cavaco, L. M., Hasman, H., Xia, S., and Aarestrup, F. M. (2009). qnrD, a novel gene conferring transferable quinolone resistance in Salmonella enterica serovar Kentucky and Bovismorbificans strains of human origin. Antimicrob. Agents Chemother. 53, 603–608. doi: 10.1128/AAC.00997-08
Chen, Y. T., Peng, H. L., Shia, W. C., Hsu, F. R., Ken, C. F., Tsao, Y. M., et al. (2012). Whole-genome sequencing and identification of Morganella morganii KT pathogenicity-related genes. BMC Genomics 13(Suppl. 7):S4. doi: 10.1186/1471-2164-13-S7-S4
Choi, J. H., Yoo, H. S., Park, J. Y., Kim, Y. K., Kim, E., and Kim, D. Y. (2002). Morganelliasis pneumonia in a captive jaguar. J. Wildl. Dis. 38, 199–201. doi: 10.7589/0090-3558-38.1.199
Clemente, L., Manageiro, V., Ferreira, E., Jones-Dias, D., Correia, I., Themudo, P., et al. (2013). Occurrence of extended-spectrum β-lactamases among isolates of Salmonella enterica subsp. enterica from food-producing animals and food products, in Portugal. Int. J. Food Microbiol. 167, 221–228. doi: 10.1016/j.ijfoodmicro.2013.08.009
Cosentino, S., Voldby Larsen, M., Møller Aarestrup, F., and Lund, O. (2013). PathogenFinder: distinguishing friend from foe using bacterial whole genome sequence data. PLoS ONE 8:e77302. doi: 10.1371/journal.pone.0077302
Di Ianni, F., Dodi, P. L., Cabassi, C. S., Pelizzone, I., Sala, A., Cavirani, S., et al. (2015). Conjunctival flora of clinically normal and diseased turtles and tortoises. BMC Vet. Res. 10:91. doi: 10.1186/s12917-015-0405-x
Dipineto, L., Russo, T. P., Calabria, M., De Rosa, L., Capasso, M., Menna, L. F., et al. (2014). Oral flora of Python regius kept as pets. Lett. Appl. Microbiol. 58, 462–465. doi: 10.1111/lam.12214
Falagas, M. E., Kavvadia, P. K., Mantadakis, E., Kofteridis, D. P., Bliziotis, I. A., Saloustros, E., et al. (2006). Morganella morganii infections in a general tertiary hospital. Infection 34, 315–321. doi: 10.1007/s15010-006-6682-3
Guillard, T., Cambau, E., Neuwirth, C., Nenninger, T., Mbadi, A., Brasme, L., et al. (2012). Description of a 2,683-base-pair plasmid containing qnrD in two Providencia rettgeri isolates. Antimicrob. Agents Chemother. 56, 565–568. doi: 10.1128/AAC.00081-11
Guillard, T., Grillon, A., de Champs, C., Cartier, C., Madoux, J., Berçot, B., et al. (2014). Mobile insertion cassette elements found in small non-transmissible plasmids in Proteeae may explain qnrD mobilization. PLoS ONE 9:e87801. doi: 10.1371/journal.pone.0087801
Harris, P. N. A., and Ferguson, J. K. (2012). Antibiotic therapy for inducible AmpC β-lactamase-producing Gram-negative bacilli: what are the alternatives to carbapenems, quinolones and aminoglycosides? Int. J. Antimicrob. Agents 40, 297–305. doi: 10.1016/j.ijantimicag.2012.06.004
Huddleston, J. R. (2014). Horizontal gene transfer in the human gastrointestinal tract: potential spread of antibiotic resistance genes. Infect. Drug Resist. 7, 167–176. doi: 10.2147/IDR.S48820
Jacoby, G., Strahilevitz, J., and Hooper, D. (2014). Plasmid-mediated quinolone resistance. Microbiol. Spectr. 2:PLAS-0006-2013. doi: 10.1128/microbiolspec.PLAS-0006-2013
Jones-Dias, D., Manageiro, V., and Caniça, M. (2016). Influence of agricultural practice on mobile bla genes: IncI1-bearing CTX-M, SHV, CMY and TEM in Escherichia coli from intensive farming soils. Environ. Microbiol. 18, 260–272. doi: 10.1111/1462-2920.13021
Lee, I., and Liu, J. (2006). Clinical characteristics and risk factors for mortality in Morganella morganii bacteremia. J. Microbiol. Immunol. Infect. 39, 328–334.
Lin, T. Y., Chan, M. C., Yang, Y. S., Lee, Y., Yeh, K. M., Lin, J. C., et al. (2015). Clinical manifestations and prognostic factors of Morganella morganii bacteremia. Eur. J. Clin.Microbiol. Infect. Dis. 34, 231–236. doi: 10.1007/s10096-014-2222-8
Mazzariol, A., Kocsis, B., Koncan, R., Kocsis, E., Lanzafame, P., and Cornaglia, G. (2012). Description and plasmid characterization of qnrD determinants in Proteus mirabilis and Morganella morganii. Clin. Microbiol. Infect. 18, E46–E48. doi: 10.1111/j.1469-0691.2011.03728.x
Nasri Yaiche, M., Denden Rafraf, I., Guo, Q., Mastouri, M., Aouni, M., and Wang, M. (2014). Type II and type IV topoisomerase mutations in clinical isolates of Morganella morganii harbouring the qnrD gene. Ann. Clin. Microbiol. Antimicrob. 13:34. doi: 10.1186/s12941-014-0034-4
Nicolle, L. E. (2001). Urinary tract pathogens in complicated infection and in elderly individuals. J. Infect. Dis. 183(Suppl. 1), S5–S8. doi: 10.1086/318844
O’Hara, C. M., Brenner, F. W., and Miller, J. M. (2000). Classification, identification, and clinical significance of Proteus, Providencia, and Morganella. Clin. Microbiol. Rev. 13:534–546. doi: 10.1128/CMR.13.4.534-546.2000
Ono, M., Namimatsu, T., Ohsumi, T., Mori, M., Okada, M., and Tamura, K. (2001). Immunohistopathologic demonstration of pleuropneumonia associated with Morganella morganii in a piglet. Vet. Pathol. 38, 336–339. doi: 10.1354/vp.38-3-336
Poirel, L., Cattoir, V., and Nordmann, P. (2012). Plasmid-mediated quinolone resistance; interactions between human, animal, and environmental ecologies. Front. Microbiol. 3:24. doi: 10.3389/fmicb.2012.00024
Sun, Q., Lan, R., Wang, Y., Wang, J., Wang, Y., Li, P., et al. (2013). Isolation and genomic characterization of SfI, a serotype-converting bacteriophage of Shigella flexneri. BMC Microbiol. 17:39. doi: 10.1186/1471-2180-13-39
Tamang, M. D., Nam, H. M., Kim, A., Lee, H. S., Kim, T. S., Kim, M. J., et al. (2011). Prevalence and mechanisms of quinolone resistance among selected nontyphoid Salmonella isolated from food animals and humans in Korea. Foodborne Pathog. Dis. 8, 1199–1206. doi: 10.1089/fpd.2011.0899
Tavares, I. M., Leitão, J. H., and Sá-Correia, I. (2003). Chromosomal organization and transcription analysis of genes in the vicinity of Pseudomonas aeruginosa glmM gene encoding phosphoglucosamine mutase. Biochem. Biophys. Res. Commun. 302, 363–371. doi: 10.1016/S0006-291X(03)00169-4
Tsanaktsidis, G., Agarwal, S. A., Maloof, A. J., Chandra, J., and Mitchell, P. (2003). Postoperative Morganella morganii endophthalmitis associated with subclinical urinary tract infection. J. Cataract Refract. Surg. 29, 1011–1013. doi: 10.1016/S0886-3350(02)01847-3
Varani, A. M., Siguier, P., Gourbeyre, E., Charneau, V., and Chandler, M. (2011). ISsaga is an ensemble of web-based methods for high throughput identification and semi-automatic annotation of insertion sequences in prokaryotic genomes. Genome Biol. 12:R30. doi: 10.1186/gb-2011-12-3-r30
Zankari, E., Hasman, H., Cosentino, S., Vestergaard, M., Rasmussen, S., Lund, O., et al. (2012). Identification of acquired antimicrobial resistance genes. J. Antimicrob. Chemother. 67, 2640–2644. doi: 10.1093/jac/dks261
Zhang, S., Sun, J., Liao, X.-P., Hu, Q.-J., Liu, B.-T., Fang, L.-X., et al. (2013). Prevalence and plasmid characterization of the qnrD determinant in Enterobacteriaceae isolated from animals, retail meat products, and humans. Microb. Drug Resist. 19, 331–335. doi: 10.1089/mdr.2012.0146
Zhao, C., Tang, N., Wu, Y., Zhang, Y., Wu, Z., Li, W., et al. (2012). First reported fatal Morganella morganii infections in chickens. Vet. Microbiol. 156, 452–455. doi: 10.1016/j.vetmic.2011.11.021
Keywords: qnrD1, plasmid, multidrug resistance, Morganella morganii, WGS
Citation: Jones-Dias D, Clemente L, Moura IB, Sampaio DA, Albuquerque T, Vieira L, Manageiro V and Caniça M (2016) Draft Genomic Analysis of an Avian Multidrug Resistant Morganella morganii Isolate Carrying qnrD1. Front. Microbiol. 7:1660. doi: 10.3389/fmicb.2016.01660
Received: 25 May 2016; Accepted: 05 October 2016;
Published: 25 October 2016.
Edited by:
José Luis Capelo, Universidade Nova de Lisboa, PortugalReviewed by:
Sally R. Partridge, Westmead Hospital, AustraliaPeter Damborg, University of Copenhagen, Denmark
Copyright © 2016 Jones-Dias, Clemente, Moura, Sampaio, Albuquerque, Vieira, Manageiro and Caniça. This is an open-access article distributed under the terms of the Creative Commons Attribution License (CC BY). The use, distribution or reproduction in other forums is permitted, provided the original author(s) or licensor are credited and that the original publication in this journal is cited, in accordance with accepted academic practice. No use, distribution or reproduction is permitted which does not comply with these terms.
*Correspondence: Vera Manageiro, vera.manageiro@insa.min-saude.pt