- Department of Dermatology, Renji Hospital, Shanghai Jiao Tong University School of Medicine, Shanghai, China
Macrophages serve as a pivotal nexus in the pathogenesis of acne vulgaris, orchestrating both the elimination of Cutibacterium acnes (C. acnes) and lipid metabolic regulation while also possessing the capacity to exacerbate inflammation and induce cutaneous scarring. Additionally, recent investigations underscore the therapeutic potential inherent in macrophage modulation and challenge current anti-inflammatory strategies for acne vulgaris. This review distills contemporary advances, specifically examining the dual roles of macrophages, underlying regulatory frameworks, and emergent therapeutic avenues. Such nuanced insights hold the promise of guiding future explorations into the molecular etiology of acne and the development of more efficacious treatment modalities.
1 Introduction
Acne vulgaris (AV) denotes a dermatological disorder exhibiting a high prevalence especially in adolescents, primarily impacting follicular sebaceous gland units (1, 2). In regions notably the face, chest, and back, enriched with sebaceous glands, the condition primarily presents as lesions characterized by open (blackheads) and closed (whiteheads) comedones, accompanied by additional dermatological features such as papules, pustules, and subsequent scarring (3). Although not life-threatening, the profound psychological ramifications stemming from acne due to its detriment to appearance and self-esteem are significant, encompassing anxiety, depression, and potential suicidal tendencies (4). The intricate pathogenesis of acne involves four key processes, including hyperkeratosis of the hair follicle, androgen-induced alterations in sebaceous products, C. acnes colonization, and associated inflammatory responses (5). While inflammatory events pervade AV, their precise immunological underpinnings warrant further investigation (6).
Macrophages, pivotal elements of the immune system, pervade skin tissues, orchestrating immune responses, inflammation regulation, and tissue regeneration (7). Macrophage polarization, coupled with the ensuing metabolic reprogramming, commands a pivotal position in contemporary immune regulation research. A confluence of studies elucidates the characterization of macrophages in disparate immune microenvironments, spotlighting seminal regulators of macrophage polarization and underscoring the instrumental role of metabolic reprogramming in orchestrating the activation toward pro-inflammatory and anti-tumor polarization (8, 9). While within dermatology, dysregulation of macrophage polarization significantly influences the pathogenesis of inflammatory skin diseases, such as psoriasis, Behçet’s disease, and rosacea (10–12).
Recent investigations delineate the multifaceted roles macrophages assume in acne formation: they contribute beneficially by clearing pathogens and regulating lipid metabolism, yet exert adverse impacts on inflammation and scarring. Despite converging evidence, strategies targeting macrophage regulation in acne vulgaris treatments remain varied. Delving deeper into the roles of macrophages in acne vulgaris is imperative for pioneering therapeutic interventions. This review elucidates recent insights into macrophage involvement in the pathogenesis of acne vulgaris, aiming to foster further research and therapeutic advancements.
2 The role of macrophages in cutaneous tissues
Skin macrophages are classified into tissue-resident and infiltrating types. Tissue-resident macrophages, originating from precursor cells during embryogenesis, are maintained through adulthood via self-renewal (13). Conversely, infiltrating macrophages differentiate from monocytes that, originating from bone marrow, infiltrate tissues in response to stimuli such as Macrophage Colony-Stimulating Factor (M-CSF), Granulocyte-Macrophage Colony-Stimulating Factor (GM-CSF), Interleukin (IL)-4, IL-10, and IL-13.
In skin, macrophages play crucial roles in maintaining homeostasis and mediating immune responses. Under normal conditions, they sustain tissue homeostasis by clearing cellular debris and secreting cytokines (14). In sebum-rich areas, they maintain lipid equilibrium by phagocytosing sebum (15). Upon injury, they modulate inflammatory responses and mediate adaptive immunity by presenting antigens to T cells (16). In later stages, macrophages secrete growth factors and cytokines to promote cell proliferation and matrix remodeling, aiding wound healing.
Macrophage polarization is pivotal for their role in skin. Lipopolysaccharide (LPS), GM-CSF, Tumor Necrosis Factor (TNF)-α, and Interferon (IFN)-γ produced by Th1 cells mediate the classical activation pathway, polarizing macrophages to M1 type, which produce high levels of reactive oxygen species (ROS) and pro-inflammatory cytokines like TNF-α, IL-1β, IL-6, and IL-12, enhancing inflammation and eliminating pathogens (17). Conversely, chemokine (C-C motif) ligand 6 (CCL6), M-CSF, linoleic acid, oleic acid, IL-4, and IL-13 produced by Th2 cells mediate the alternative activation pathway, polarizing to M2 type (18). M2 macrophages suppress the inflammatory response by producing IL-10. They further promote fibroblast proliferation, collagen synthesis, and angiogenesis through the release of Transforming Growth Factor (TGF)-β, Platelet-Derived Growth Factor (PDGF), and Vascular Endothelial Growth Factor (VEGF), facilitating tissue repair (19). In contrast, Matrix Metalloproteinases (MMPs) expressed by M1 macrophages degrade collagen, inhibiting fiber formation. IL-12 and IL-10, in turn, stimulate the differentiation of T cells to Th1 and Th2 cells, respectively (20) (Figure 1). Comprehensively, M1 macrophages within the dermal ecosystem play a pivotal role in instigating inflammatory responses that not only target pathogens but also contribute to the resultant tissue damage. Simultaneously, M2 macrophages, in their pivotal role, regulate and suppress excessive inflammatory reactions to forestall damage, also facilitating tissue repair and wound healing. The interplay between shifts in the cytokine milieu and macrophage plasticity enables a flexible modulation of M1 and M2 functionalities, a cornerstone for preserving systemic equilibrium. Disruption of this equilibrium heralds pathological sequelae and the inception of disease states. For instance, single-cell RNA sequencing insights from hidradenitis suppurativa illustrate that the STAT1/IFN axis propels macrophages towards an M1-skewed pro-inflammatory phenotype, exacerbating inflammation and promoting immune cell ingress (21). Conversely, the predilection for M2 macrophage polarization not only underpins tumorigenesis but also catalyzes fibrosis and granuloma evolution, potentially correlating with the systemic manifestations observed in sarcoidosis (22, 23).
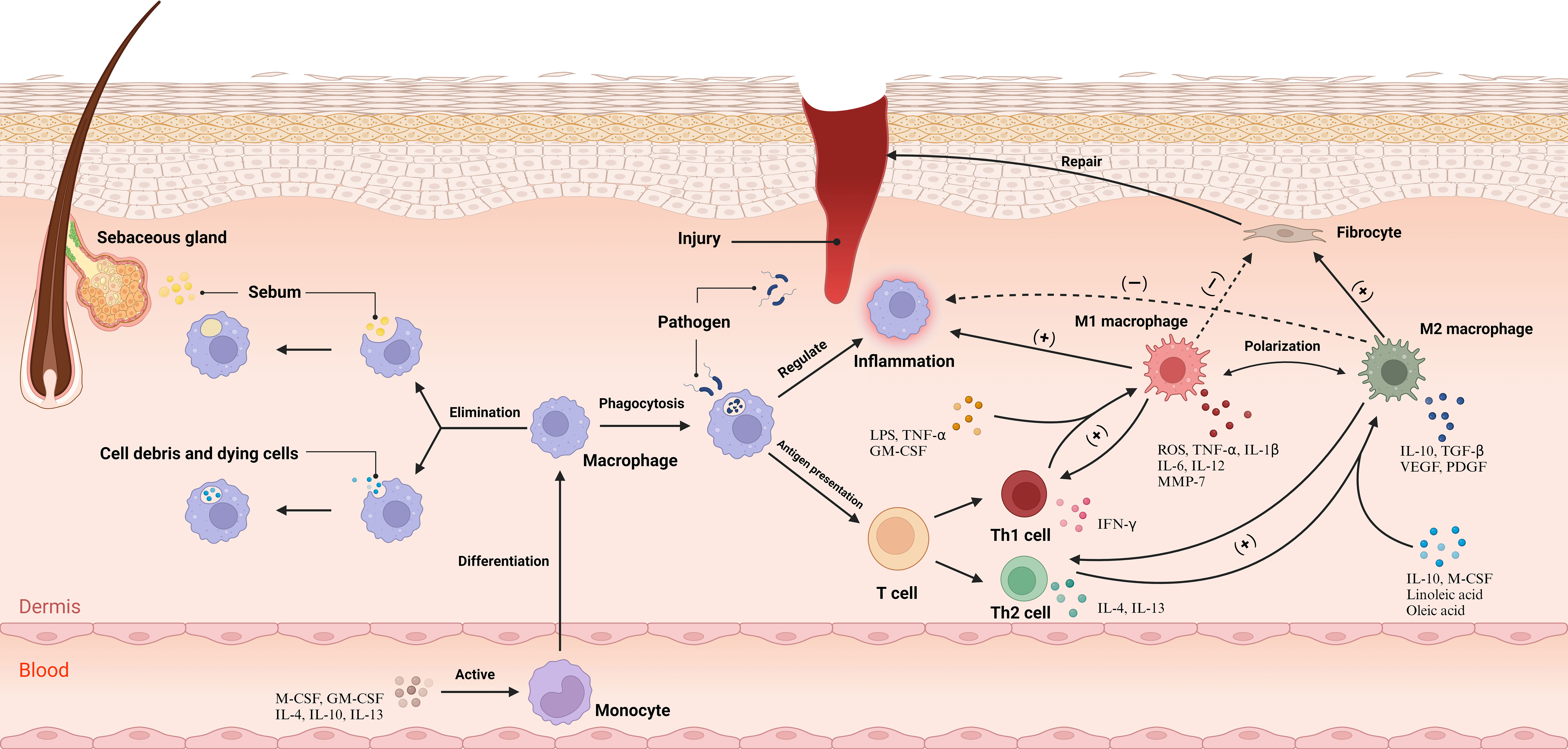
Figure 1 Under physiological conditions, macrophages play pivotal roles, namely the clearance of cellular remnants and apoptotic cells, phagocytosis of lipids, and the sustenance of dermal tissue equilibrium. Upon the manifestation of a wound, Cytokines incite monocytes within the bloodstream, catalyzing their differentiation into macrophages, which subsequently permeate the dermal tissue. Macrophages undertake the phagocytosis of pathogens and orchestrate the inflammatory responses while mediating antigen presentation to T cells. This interaction induces the differentiation of T cells into Th1 and Th2 subsets. Subsequently, Th1 cells augment M1 polarization, and Th2 cells enhance M2 polarization. M1 macrophages primarily emit pro-inflammatory cytokines and attenuate fibroblast function, while M2 macrophages release cytokines that mitigate inflammation and elevate fibroblast activity, promoting tissue repair. The cytokines secreted by M1 and M2 macrophage subtypes stimulate Th1 and Th2 cells, respectively, maintaining a balanced immune response within the dermal ecosystem. CCL6, chemokine (C-C motif) ligand 6; GM-CSF, Granulocyte-Macrophage Colony-Stimulating Factor; IFN-γ, Interferon-gamma; IL-1, Interleukin-1; LPS, Lipopolysaccharide; M-CSF, Macrophage Colony-Stimulating Factor; MMP-7, Matrix Metallopeptidase 7; PDGF, Platelet-Derived Growth Factor; ROS, reactive oxygen species; TGF-β, Transforming Growth Factor-beta; Th1, T helper 1 cell; TNF-α, Tumor Necrosis Factor-alpha; VEGF, Vascular Endothelial Growth Factor. Adapted from “Skin (Layout)” by BioRender.com (2023). Retrieved from https://app.biorender.com/biorender-templates.
3 The central role of inflammation in the pathogenesis of acne vulgaris
The onset of acne lesions is characterized by the emergence of microcomedones, consequent to hyperkeratinization occurring within the funnel section of the follicular canal, causing pore obstruction and the subsequent development of comedones (24). Upon exposure to air, blackheads undergoes oxidation and darkening, contrasting with whiteheads, which remains subcutaneous, manifesting a white or normal-skinned appearance. C. acnes multiply within these obstructed follicles, inciting an inflammatory response manifested by redness, swelling, and pus (25). Without adequate intervention, such lesions may intensify into painful pustules, cysts, or nodules, risking irreversible damage and scarring in the absence of proper treatment or when exacerbated.
The manifestation of acne lesions is precipitated by the synergistic interaction of four predominant causative factors (26). The upsurge in sebum production and augmented epithelial keratinization within hair follicles, instigated by abnormalities in androgen metabolism, cultivate an environment optimal for the prolific expansion of C. acnes. Metabolites of C. acnes incite inflammation within follicular and perifollicular regions, coupled with an ensuing immune cell infiltration of the follicular wall, which may culminate in follicular rupture. Subsequent to this, the materials within pimples permeate the dermal tissue, escalating the inflammation and thereby engendering pustules, granulomas, and symptoms indicative of systemic inflammation.
Inflammatory reactions are omnipresent throughout acne’s progression. Research conducted by Jeremy et al. has revealed prominent escalations in CD3+ and CD4+ T cells, macrophages, and IL-1α in the dermis surrounding unaltered hair follicles in individuals afflicted with acne, suggesting inflammation as a precursor to the manifestation of acne (6). Importantly, IL-1α is capable of activating basal keratinocytes through autocrine production, prompting the augmented proliferation of suprabasal cells and subsequently inducing hyperkeratosis in the follicular funnel (27). Subsequently, C. acnes facilitates the release of inflammatory cytokines, including TNF-α, IL-6, and IL-8 from dendritic cells and keratinocytes, initiating an extensive adaptive immune response (28). This series of events leads to the mobilization of macrophages and lymphocytes to the site, enhancing perifollicular cell infiltration due to elevated cytokine release, resulting in the formation of inflamed papules. Post follicular destruction, components such as keratin and hair elements incite severe inflammatory reactions, culminating in the development of pustules or granulomas. A significant correlation is observed between the severity and duration of inflammation and the inception of scars, as demonstrated by Carlavan et al., attributed to delayed inflammatory reactions enhancing the susceptibility to scarring in acne patients due to the dysregulation of the innate immune response (29). This investigation further identified elevated expression of various immunoglobulin genes and the pervasive infiltration of mature B cells within the long-lasting lesions of scarring patients. Concurrently, Holland and colleagues documented that lesions in scarring individuals exhibit diminished HLA-DR expression alongside sparse CD4+ T cell infiltration (30). Collectively, these observations underscore the potential role of adaptive immunity dysregulation in the etiology of acne scar formation.
4 Macrophages in acne: a dual facet in pathogenesis
Macrophages embody a paradoxical role in acne development, serving as both sentinels and provocateurs. Their vital functions include the regulation of lipid concentrations and facilitating the elimination of C. acnes. However, an immoderate immune reaction can provoke inflammation and subsequent acne scarring. It is imperative to comprehend their intricate roles to maintain physiological equilibrium and circumvent adverse pathological outcomes.
4.1 Macrophages and lipid metabolism regulation
Individuals with AV often exhibit abnormal plasma lipid profiles, characterized by elevated plasma lipoproteins and a decrease in High-Density Lipoprotein (HDL) cholesterol levels, particularly in severe acne conditions (31). These abnormalities in serum total cholesterol levels act as a substrate for androgen synthesis by the adrenal glands and gonads, intensifying the progression of AV through the increase of androgens (32).
In the context of the aforementioned lipid metabolism disruption, macrophages are crucial regulators, orchestrating the assimilation of oxidized low-density lipoprotein (LDL) and cholesterol. Macrophage scavenger receptor 1 (MSR1) facilitates the incorporation and breakdown of modified LDL, leading to intracellular cholesterol accumulation and influencing macrophage functionality (33). AbdElneam et al. found that of the four common haplotypes of the MSR1 gene, the most common haplotypes in patients with AV are TCAC and CAGG, whereas the most common haplotypes in healthy populations are TAAC and CCAC (34). Additionally, they observed a linkage disequilibrium in the MSR1 gene, indicating that genetic elements might significantly influence the development of AV by altering macrophage-mediated lipid regulation.
4.2 Engulfment and clearance of C. acnes by macrophages
Macrophage phagocytosis in acne lesions is prominently orchestrated by C. acnes, a Gram-positive, parthenogenetic anaerobic bacillus. This bacterium is pivotal, predominantly inhabiting the sebaceous glands of hair follicles in acne patients and playing a vital role in acne pathogenesis (35). It triggers the expression of IL-15 and GM-CSF via Toll-like receptor 2 (TLR2), fostering the differentiation of monocytes into CD209+ macrophages and CD1b+ dendritic cells (36). Comparatively, CD209+ macrophages exhibit superior efficacy in phagocytosing and curtailing the growth of C. acnes, becoming a major line of host defense against this bacterium.
Detailed analysis of skin biopsies from inflammatory acne lesions reveals macrophages’ ability to recognize and phagocytose C. acnes strains, with sebum playing a role in enhancing this ability (15). These macrophages utilize a variety of enzymes, such as lipoxygenase and myeloperoxidase, to produce antimicrobial agents ROS and reactive nitrogen intermediates (RNI) in lysosomes. These agents then fuse with phagosomes to eliminate the pathogens using ROS and RNI (37).
Notably, Do et al. found that excess squalene production by keratin-forming cells in acne lesions scavenges ROS (38). This also leads to a reduction in the expression of genes encoding oxidative enzymes produced by ROS and RNI, including the NADPH oxidase genes NOX1, NOS2, and NFKB. Furthermore, the expression of genes encoding ROS-induced oxidative pathway enzymes, such as the mitogen-activated protein kinase genes MAPK8, MAPK14, and NFKB, is also reduced. This renders TREM2 macrophages virtually devoid of antimicrobial activity, blocking the antimicrobial response and increasing the bacterial load. Consequently, this leads to the secretion of IL-18 and upregulation of inflammatory chemokine expression, instigating a disease site-specific inflammatory response. Remarkably, this phenomenon elucidates the efficacy of benzoyl peroxide in the clinical management of acne through the peroxidative destruction of C. acnes. Remarkably, this study illuminates an additional mechanism by which topical benzoyl peroxide combats acne, beyond its direct antibacterial action against C. acnes, anti-inflammatory effects, and comedolytic properties (4). It achieves this by generating oxygen radicals that negate squalene’s capacity to scavenge oxygen radicals, thereby countering the suppression of squalene’s antimicrobial potency on TREM2 macrophages.
Nakatsuji et al. elucidated that the synergistic interaction of the Christie, Atkins, Munch-Peterson (CAMP) factor from C. acnes and acidic sphingomyelinase (ASMase) from the host cells triggers an inflammatory response and inflicts cytotoxicity to macrophages (39). Based on these findings, Nakatsuji formulated two plausible hypotheses: C. acnes may evade the immune response by utilizing host ASMase, or the CAMP factors might manipulate host ASMase, thereby amplifying bacterial virulence, undermining macrophages, and obstructing the efficient clearance of C. acnes (Figure 2).
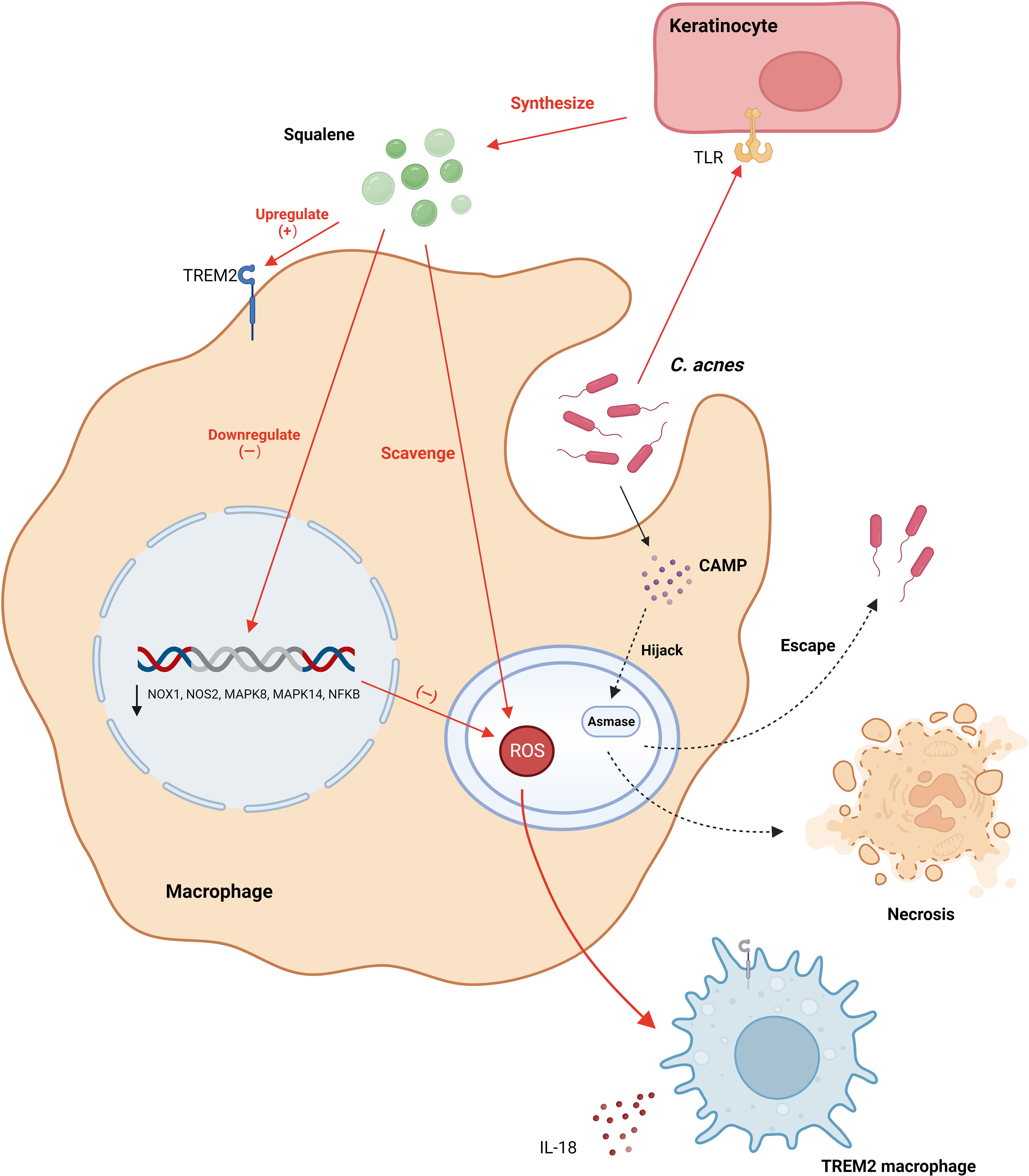
Figure 2 C. acnes modulates the secretion of squalene from keratinocyte cells via TLR receptors, elevating the expression of TREM2 receptors. This secretion mitigates reactive oxygen species within lysosomes, leading to the downregulation of genes encoding oxidative and pathway enzymes responsible for the production of ROS and RNI. Consequently, this leads to the transformation of macrophages into TREM2 macrophages, which primarily release pro-inflammatory factors and exhibit diminished proficiency in eliminating C. acnes. Additionally, C. acnes synthesizes the CAMP factor, which, facilitated by Asmase, either evacuates from macrophages or provokes macrophage necrosis. CAMP, Christie, Atkins, Munch-Peterson; TLR, Toll-Like Receptor; TREM2, Triggering Receptor Expressed on Myeloid cells 2. Created with BioRender.com.
4.3 Macrophage-mediated inflammatory regulation in acne lesions
Macrophages exhibit a central role in mediating inflammatory responses within acne, governed by diversified pathways.
Sebaceous gland-originating lipids are crucial modulators of macrophage activities. A study led by Lovászi et al. elucidated that sebum is imperative for IL-1β secretion in C. acnes-activated macrophages, where components like oleic acid and squalene intensify IL-1β secretion (15). Intriguingly, oleic acid selectively moderates the expression of IL-6 and TNF-α, introducing a paradox considering its role in promoting M2 polarization and IL-1β secretion amplification. In contrast, linoleic acid exerts anti-inflammatory properties, reducing the secretion of prominent inflammatory mediators. On a different note, Tang et al.’s study provides insights into the synergistic secretion of IL-1β and TNF-α by sebaceous cell glands and macrophages in acne conditions (40). Sebaceous cell glands induce macrophage polarization to the M1 phenotype, exacerbating inflammatory reactions. Cannabidiol (CBD) is recognized to mitigate this interplay effectively.
Furthermore, keratinocytes residing in acne lesions orchestrate macrophage inflammatory responses. Research by Graham highlighted that C. acnes and its GroEL protein trigger keratinocytes to release IL-1α, Chemokine (C-X-C motif) Ligand 8 (CXCL8), GM-CSF, TNF-α, and human β-defensin-2 (hBD-2) via the TLR, enhancing macrophage activation and recruitment and intensifying inflammation (41, 42). Additionally, squalene, produced by keratinocytes, prompts macrophages to increase TREM2 receptor expression, amplifying inflammatory gene expression (38).
C. acnes plays a cardinal role in inciting the production of pro-inflammatory cytokines such as IL-1β, largely by the direct activation of Pattern Recognition Receptors (PRR) in macrophages, occurring primarily through Pathogen-Associated Molecular Patterns (PAMP) (43). This encompasses entities like TLR and Nod-like receptors (NLR). Tsai et al. delineated that C. acnes engages the MAPK and NF-κB signaling cascades via the TLR2 receptor on macrophages, thereby modulating the expression of inducible Nitric Oxide Synthase (iNOS)/NO and Cyclooxygenase-2 (COX-2)/Prostaglandin E2 (PGE2) (44). This interaction is reliant upon the nuanced phosphorylation of protein kinases JNK and EPK along with ROS-enhanced IKK protein phosphorylation, cumulatively activating AP-1 and NF-κB and consequently augmenting the production of NO and PGE2. Fischer and colleagues delineated that the activation of C. acnes is dependent on the junctional protein TIR-domain-containing adapter-inducing interferon-β (TRIF), initializing the cGAS-STING pathway and inducing the type I interferon axis in macrophages, catalyzing additional signaling pathways, including the JAK-STAT pathway, and modulating the inflammatory response (45). TRIF, predominantly a downstream of TLR3/4, does not ordinarily recognize Gram-positive bacteria. Fischer posited that TRIF signaling is coupled with TLR2, aiding macrophages in the activation of the IFN-I axis after the recognition of C. acnes. It’s worth noting that TLR4 also discerns Gram-positive bacteria, and, as previously highlighted, C. acnes can stimulate keratinocytes via both TLR2 and TLR4 (41, 42, 46). Consequently, the intricate mechanisms by which C. acnes activates the cGAS-STING pathway via TRIF remain to be explicated comprehensively.
C. acnes intricately modulates IL-1β secretion through NOD-like receptor family, pyrin domain-containing 3 (NLRP3), amplifying the transcription of both IL-1β and NLRP3 genes predominantly via the NF-κB pathway (47). Once transcribed within macrophages, NLRP3 emerges as a pivotal sensor, converging with the effector cysteine protease (caspase)-1 to orchestrate the inflammasome, thereby catalyzing the metamorphosis of pro-IL-1β to matured IL-1β for ensuing release (48). This mechanism, elucidated by Qin et al., is contingent upon K+ efflux (Figure 3). Regrettably, the absence of subsequent comprehensive studies renders this field ripe for further exploration. Indeed, inflammasomes are also capable of processing Gasdermin D (GSDMD), integrating it into the plasma membrane and creating pore structures to release pro-inflammatory cytokines, ultimately inducing cellular pyroptosis——a distinct, inflammation-associated form of programmed cell death instrumental in host defense and implicated in anti-tumor activities in certain cancers (47). However, in dermatological conditions, it predominantly manifests as a pathogenetic mechanism. Deng et al. delineated how SpeB, an exotoxin of Streptococcus pyogenes, triggers cutaneous keratinocyte pyroptosis through the cleavage of GSDMA (49). While Lian et al. highlighted the role of GSDMD-mediated keratinocyte pyroptosis in fostering hyperproliferation and aberrant differentiation, contributing to the development of psoriasis (50). Hence, probing the potential induction of macrophage cell pyroptosis by C. acnes is pivotal and promises profound insights into the pathogenesis of acne vulgaris.
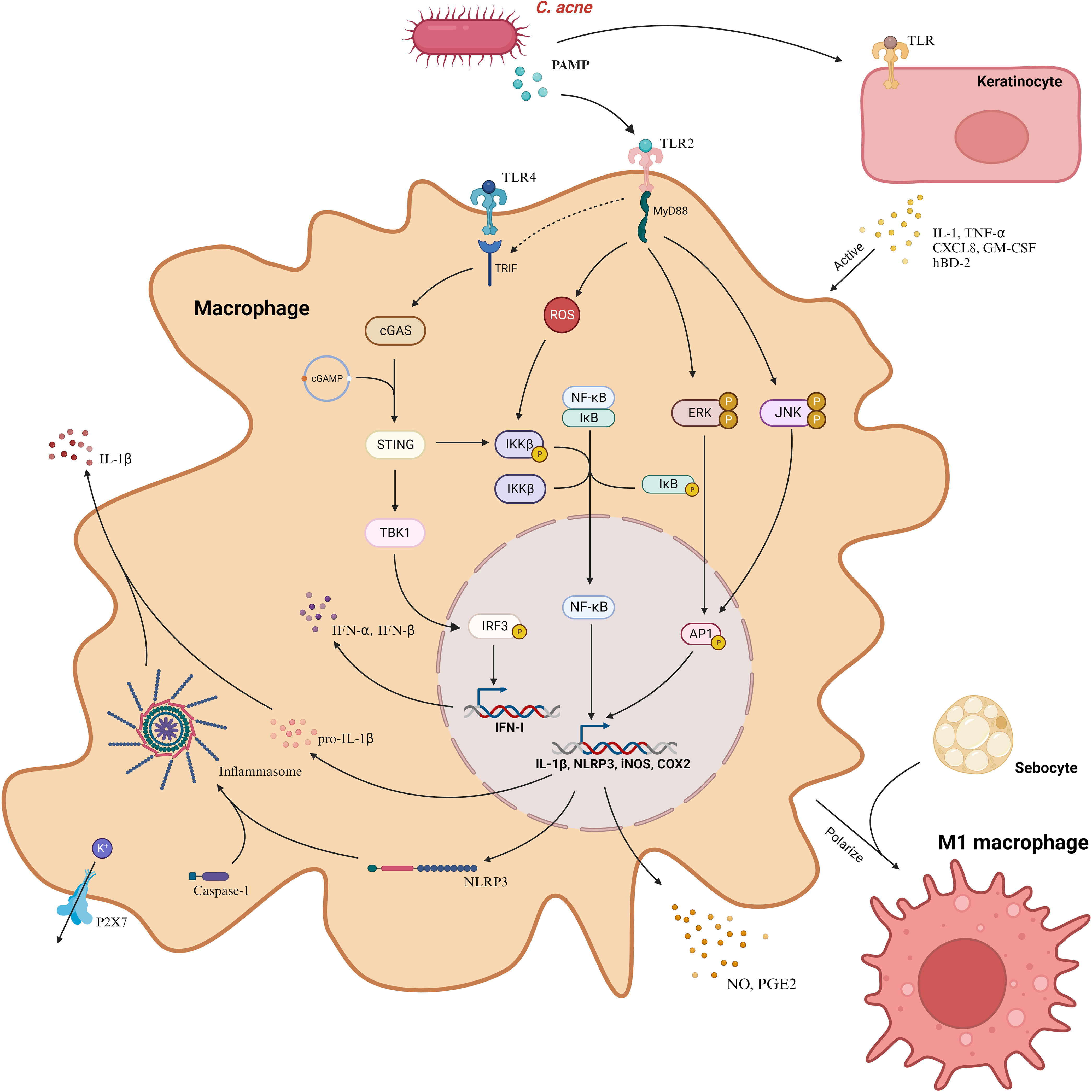
Figure 3 C. acnes predominantly instigates three distinctive signaling cascades in macrophages through PAMP, mediated via TLR2. These cascades encompass the ERK, JNK, and NF-κB pathways, which amplify the transcription of iNOS and COX2 genes, facilitating the liberation of NO and PGE2. Concurrently, C. acnes incites the cGAS-STING signaling cascade via TRIF, initiating the type I interferon axis. This bacterium also enhances the expression of NLRP3, which collaborates with caspase-1 to form the inflammasome. This assembly, reliant on K+ efflux, processes pro-IL-1β into IL-1β, enabling its release from the cell. Additionally, C. acnes induces keratinocytes to discharge cytokines, in turn, activating macrophages. Furthermore, in the presence of C. acnes, sebocytes facilitate the polarization of macrophages to the M1 phenotype. COX2, Cyclooxygenase-2; CXCL8, Chemokine (C-X-C motif) ligand 8; GM-CSF, Granulocyte-Macrophage Colony-Stimulating Factor; hBD-2, Human Beta-Defensin 2; IFN-I, Interferon Type I; IL-1β, Interleukin-1 beta; iNOS, Inducible Nitric Oxide Synthase; MyD88, Myeloid Differentiation Primary Response Protein 88; NLRP3, NOD-like Receptor 3; P2X7, Purinergic Receptor P2X7; PAMP, Pathogen-Associated Molecular Pattern; PGE2, Prostaglandin E2; TLR, Toll-Like Receptor; TNF-α, Tumor Necrosis Factor-alpha; TRIF, TIR-Domain-Containing Adapter-Inducing Interferon-β Protein. Created with BioRender.com.
Finally, genetic elements play a significant role in shaping the manner in which macrophages regulate inflammation. Resistin, a protein primarily secreted by macrophages, acts to elevate the expression of inflammatory mediators (51). A significant revelation made by Akcılar et al. was the identification of a protective association linked to the CG genotype of the resistin gene, correlating prominently with a decreased risk of acne vulgaris.
4.4 Influence of macrophages on the progression of acne scarring
Primary lesions in acne vulgaris frequently culminate in scarring, which, by altering physical appearance, can engender significant social and psychological ramifications (52). The genesis of scarring is multifaceted, implicating not only the intensity and duration of inflammation but also the intricate interplay of cytokines secreted by diverse macrophage subtypes, orchestrating dermal fibroblasts’ activity (53). Disruption in the equilibrium of pro- and anti-fibrinogen cytokines and growth factors precipitates aberrant extracellular matrix protein synthesis and compromises fibroblast functionality, culminating in wound remodeling, contraction, and subsequent scar manifestation (54).
Holland et al. elucidated disparate macrophage trajectories between not-scar-prone (NSP) and scar-prone (SP) patients (30). In NSP patients, pervasive macrophage infiltration coupled with augmented HLA-DR expression marked efficient antigen clearance and cellular activation, reverting to basal levels as lesions resolved. In contrast, SP patients evidenced sustained macrophage elevations and initial subdued, but progressively accentuated, HLA-DR expressions, indicating an escalating, specialized immune response, fostering angiogenesis and exacerbated inflammation, and culminating in scarring. Complementing this, Carlavan et al. detected enduring macrophage recruitment and irreversible glandular disruptions in SP patients, implying an elongated inflammatory state (29).
Macrophage polarization may further play a pivotal role in the pathogenesis of acne scarring. Under normal conditions, M1 macrophages predominantly clear pathogens and debris during wound healing’s initial phase. As healing advances to the proliferative phase, a critical shift to M2 macrophages occurs, promoting tissue regeneration and reducing inflammation. In the remodeling phase, M2 macrophages regulate collagen, essential for tissue restoration (55). Saint-Jean et al. discerned elevated IL-2 and IL-10 and attenuated MMP-9 proteins in SP patients, implicating a predominance of M2 macrophages (56). Besides, relationship between macrophage polarization and acne scarring still lacks more empirical elucidation, but there have been many solid proofs for other scarring phenomena. It is imperative to acknowledge that a heightened prevalence of pre-injury M2 cells, a suppression of M1 expression in preliminary phases, and a subsequent deferred M2 expression in terminal phases, are associated with the evolution of pathological scarring (57). The accentuated presence of M2 cells along scar peripheries and within the superficial dermis underscores the potential ramifications of macrophage hyperactivity in disrupting the equilibrium of collagen synthesis and catabolism, thereby precipitating hyperfibrosis (58, 59). This scenario is analogous to the complexities observed in numerous chronic wounds, wherein the activation of M1 macrophages is inhibited, preventing a full transition to the M2 phenotype and thus arresting the repair mechanism within the inflammatory stage (60). Recent investigations have illuminated that SPP1+ macrophages exhibiting an M2 macrophage phenotype, in conjunction with POSTN+ fibroblasts, precipitate the development of fibrotic scars in acne keloidalis through interactions mediated by the SPP1 axis (61).
5 Therapeutic strategies and potential approaches addressing macrophages in acne vulgaris
The comprehensive understanding of the pathogenesis of acne vulgaris is yet to be fully attained. Nonetheless, numerous empirical studies have highlighted the ability of existing therapeutic strategies to alleviate acne by modulating macrophage functionality. Furthermore, multiple approaches, which focus specifically on macrophages, display considerable potential for therapeutic advancement in acne vulgaris, signaling new directions in research and application. Intriguingly, our scrutiny of acne treatment strategies targeting macrophages uncovered a general trend towards dampening inflammatory signaling pathways and cytokine production, with strategies encompassing M2 macrophage polarization among others. Remarkably, photodynamic therapy (PDT), used to treat severe acne, delineates an antithetical mechanism by advocating for M1 macrophage polarization, thereby escalating inflammation (62). This tiered therapeutic paradigm for acne articulates a groundbreaking perspective: advocating pro-inflammatory interventions for individuals grappling with severe acne, in stark divergence from the anti-inflammatory modalities prescribed for mild conditions. The discrepancy between fostering and mitigating inflammation unmistakably signals the presence of an alternative to current therapeutic modalities, signaling a departure from established treatments and underscoring the pressing need for further investigation to validate these novel approaches.
5.1 Retinoids
Retinoids, esteemed in dermatological domains, are quintessential in acne vulgaris therapy, administered both topically and orally. They exemplify diverse pharmacological competence, optimizing follicular keratinization, suppressing the proliferation of C. acnes, exhibiting anti-inflammatory traits, and forestalling scarring (63). Notably, All-trans retinoic acid (ATRA) and adapalene (AD) are paramount in this pharmacological class.
Retinoids enhance macrophage phagocytosis and clearance via the inhibition of squalene sebum (38). Liu et al. elucidated that ATRA not only moderates the expression of TLR2, attenuating C. acnes-induced inflammatory cytokine production, but also orchestrates the differentiation of monocytes into macrophages over dendritic cells, intensifying the phagocytosis of C. acnes and curtailing its proliferation (64). Further, Ji et al. validated the therapeutic efficacy of the electrostatically optimized adapalene-loaded emulsion, illustrating its significant inhibitory impact on the macrophage expression of pro-inflammatory substances (65).
5.2 Natural product drugs
Natural entities, including specific plant and animal extracts, have long been integral to acne treatment methodologies, predominantly as ethnomedicines, across diverse cultures. Several of these derivatives are renowned for their anti-inflammatory capacities, with a focus on modulating signaling pathways in macrophages (Table 1).
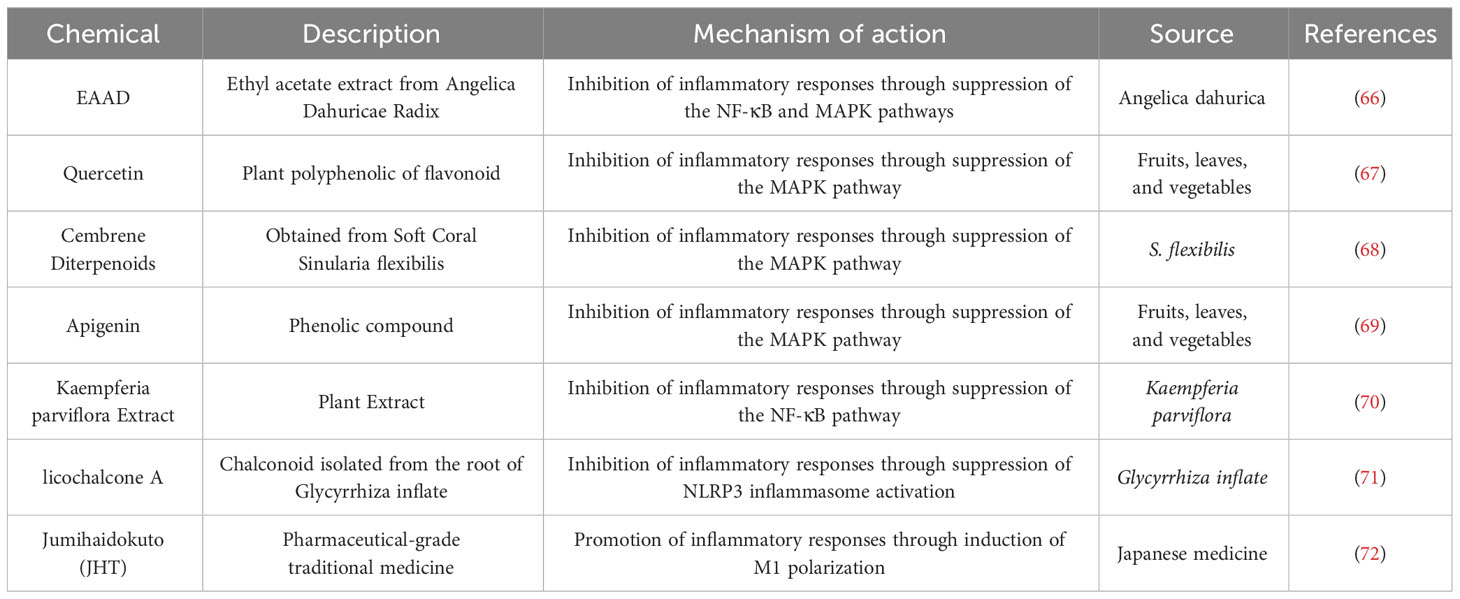
Table 1 Natural product drugs employed for Acne Vulgaris intervention by addressing action on macrophages.
Kang et al. elucidated that ethyl acetate extract from Angelica Dahuricae Radix (EAAD) effectively inhibits iNOS and COX-2 expression and concurrently reduces the production of NO, PGE2, and TNF-α in macrophages by blocking the NF-κB and MAPK pathways (66). Analogously, substances such as quercetin, apigenin, and cembrene diterpenoids from the cultured soft coral Sinularia flexibilis inhibit the MAPK pathway in macrophages, thereby alleviating inflammation (67–69). On the other hand, Kaempferia parviflora extract and licochalcone A demonstrate anti-inflammatory effects by obstructing the NF-κB pathway and NLRP3 inflammasomes respectively (70, 71). In a distinctive study, Sekiguchi et al. revealed that JHT, a conventional Japanese medicine for acne, promotes the differentiation of monocytes to M1-type macrophages and intensifies macrophage infiltration, suggesting a potential improvement in acne symptoms through the enhancement of M1-type macrophage functions (72).
5.3 Photodynamic therapy
5-Aminolevulinic Acid Photodynamic Therapy (ALA-PDT) is emerging as a groundbreaking therapeutic approach for acne vulgaris, distinguished by its unequivocal efficacy, minimal invasiveness, and lack of systemic side effects (73). Post-ALA-PDT, a significant initial symptom is a severe inflammatory response, evidenced by erythema and pustules, with the intensity of the response being directly proportional to the effectiveness of the treatment (74).
Zhang et al. delineated that ALA-PDT activates the p38 MAPK pathway, instigating CXCL8 expression in sebaceous gland cells and subsequently enlisting macrophages to augment the inflammatory response (75). Subsequently, Liu et al. elucidated that keratinocytes subjected to ALA-PDT exhibit escalated COX2 expression, releasing PGE2 to elevate TREM1 receptor expression via TLR4 in macrophages, fostering macrophage M1 polarization and triggering the p38 and NF-κB signaling pathways, thereby intensifying inflammation (62) (Figure 4). It’s hypothesized that by directing macrophage M1 polarization to enhance inflammation, ALA-PDT may offer a revolutionary solution for acne treatment.
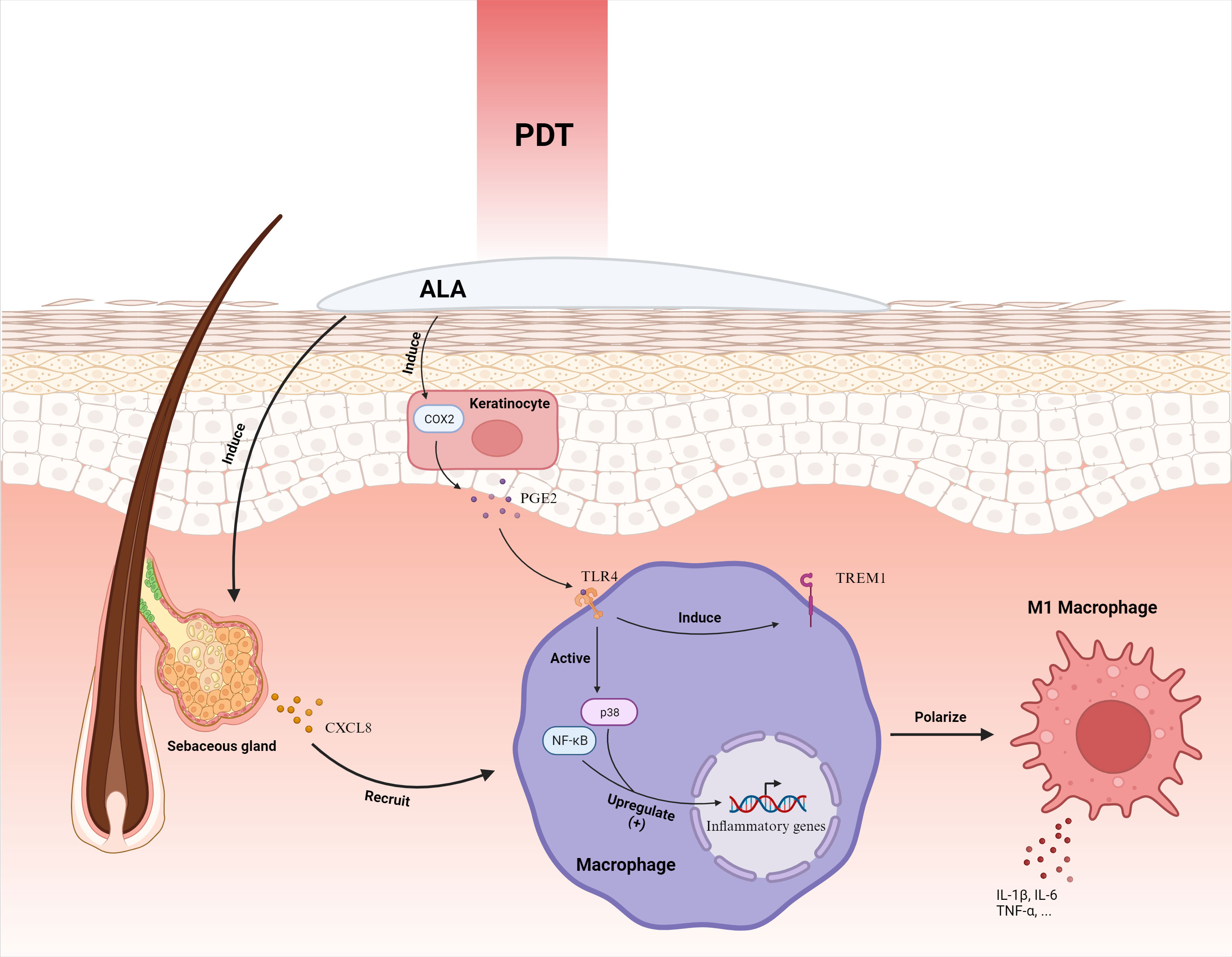
Figure 4 ALA-PDT orchestrates the mobilization of macrophages by inciting the secretion of CXCL8 from sebaceous gland cells. Furthermore, it intensifies the expression of COX2 in keratinocytes, culminating in the augmentation of PGE2. This molecule interacts with the TLR4 receptor on macrophages, instigating the activation of p38 and NF-κB pathways and ensuing in the elevation of inflammatory gene expression. Concurrently, it enhances the manifestation of TREM1 receptors, steering the polarization of macrophages toward the M1 phenotype, thereby amplifying the inflammatory response. ALA-PDT, Aminolevulinic Acid Photodynamic Therapy; COX2, Cyclooxygenase-2; CXCL8, Chemokine (C-X-C motif) ligand 8; IL-1β, Interleukin-1 beta; PGE2, Prostaglandin E2; TLR4, Toll-Like Receptor 4; TNF-α, Tumor Necrosis Factor-alpha; TREM1, Triggering Receptor Expressed on Myeloid cells 1. Adapted from “Skin (Layout)” by BioRender.com (2023). Retrieved from https://app.biorender.com/biorender-templates.
5.4 Traditional Chinese medicine interventions
Auriculotherapy, an integral component of Traditional Chinese Medicine (TCM), has been revered as a therapeutic intervention for conditions like inflammation, pain, and drug addiction. Zuo et al. executed auriculotherapy interventions, which encompass auricular bloodletting therapy (ABT), auricular point sticking (APS), and their combination (ABPS), on a rat model representing acne (76). The results unveiled that ABT, APS, and ABPS were proficient in prompting the initiation of M2-type macrophages via meticulous modulation of macrophage polarization. This resulted in the alleviation of acne symptoms and a reduction in serum levels of TNF-α and IL-1β in rats. Notably, APS also exhibited a significant reduction in the expression of the TLR2/NF-κB signaling pathway. The discoveries from this study reinforce the mechanistic underpinning of TCM auricular acupoint therapy in addressing inflammatory conditions like acne, adding substantial validation to the application of auriculotherapy in unconventional acne treatments.
5.5 Exploration of alternative therapeutic strategies
5.5.1 Antimicrobial peptides
Antimicrobial peptides, inherent immune molecules in organisms, exhibit versatile antimicrobial properties. Research by Wu et al. elucidated that Cath-MH, originating from frog skin, exhibits potent antimicrobial efficacy against C. acnes by inhibiting the proliferation of C. acnes and curtailing inflammatory cytokine production (77). This is facilitated through the suppression of macrophage TLR expression via PAMP binding, highlighting antimicrobial peptides as potent candidates for acne interventions.
5.5.2 Organic compounds
Several organic compounds have unveiled promising therapeutic efficacies for acne through macrophage modulation. A study by Shin et al. depicted the suppressive role of pyrrolidine dithiocarbamate (PDTC) on multiple inflammatory cytokines, achieved by inhibiting NF-κB signaling and NLRP3 activation in macrophages under the influence of C. acnes (78). Additionally, investigations by Kim et al. concluded that the benzoxathiolone BOT-64 could deter the synthesis of inflammatory mediators by blocking the NF-κB signaling pathway through the inhibition of IKKβ in macrophages (79). Concurrently, research by Chung et al. demonstrated the capacity of LYR-71, another benzoxathiolone, to inhibit IFN-γ-induced inflammatory responses, accomplished by uncoupling the tyrosine phosphorylation of STAT-1 in macrophages (80).
5.5.3 Additional therapeutic strategies
Non-steroidal anti-inflammatory drugs (NSAIDs) offer significant promise in acne treatment through novel mechanisms. Specifically, a study by Yang et al. substantiated that kinofen has the potential to inhibit NLRP3 inflammasome activation and reduce IL-1β expression, suggesting its applicability in treating acne vulgaris (81). Furthermore, innovative approaches such as microcurrent stimulation (MC) have been proposed by Lee et al., capable of mitigating inflammatory responses by modulating protein expression levels involved in TLR2/NF-κB signaling within macrophages (82). This highlights the potential of MC as a novel acne treatment method. Additionally, the role of probiotics in skin homeostasis and inflammation regulation cannot be overlooked. Wang et al. demonstrated that Bifidobacterium fermentum lysate (BFL) effectively mitigates LPS-induced secretion of IL-8, TNF-α, and COX-2 expression in macrophages (83). Collectively, these studies underscore the diverse and promising therapeutic strategies for acne treatment, ranging from NSAIDs and microcurrent stimulation to probiotics, each contributing uniquely to the understanding and management of acne (Table 2).
6 Concluding remarks and perspectives
Macrophages indeed exemplify a double-edged sword in the pathogenesis of acne vulgaris. They are fundamental for sustaining homeostasis, modulating lipid, and neutralizing pathogens. Nevertheless, the overactivation of macrophages, underscored by the proinflammatory responses to C. acnes, can augment the severity of acne symptoms (Figure 5). The intricate mechanisms are being meticulously unraveled, albeit the methodology through which ALA-PDT modulates macrophage polarization and escalates the inflammatory response remains obscure. This ambiguity may be attributed to the advanced extermination of bacteria and the attenuation of scarring, necessitating more rigorous studies for verification. Given the pivotal role of macrophage polarization in the pathogenesis of myriad skin diseases, the imperative for intensified exploration of its implications in acne vulgaris is underscored (84).
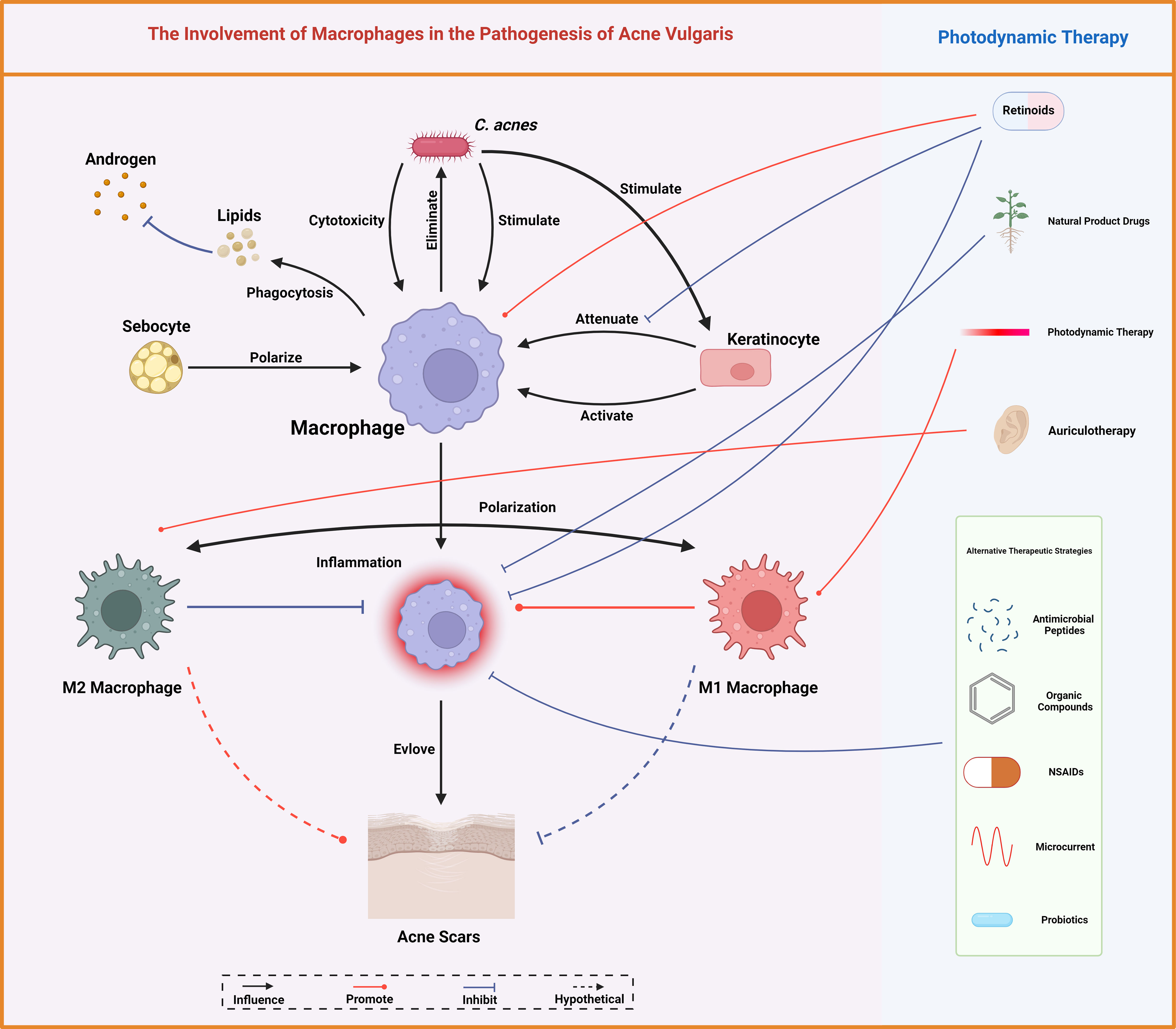
Figure 5 Macrophages mediate lipid homeostasis, bacterial eradication, immune modulation, and scar tissue formation, central to acne vulgaris pathobiology. Conventional therapeutic measures for acne vulgaris like retinoids and photodynamic therapy, alongside various other interventions, modulate these macrophage functions, offering therapeutic leverage against acne. Created with BioRender.com.
In addressing acne, therapeutic interventions necessitate a comprehensive consideration of immune responses, anti-inflammatory, and antimicrobial approaches. Probing the alterations in macrophage activity in acne vulgaris promises enhanced comprehension of this prevalent dermatological ailment’s pathogenesis. Current interventions primarily employ anti-inflammatory strategies, with emerging evidence supporting the feasibility of macrophage modulation as a potential therapeutic avenue. The unveiling of novel therapeutic pathways, such as the employment of statins to inhibit mevalonate, is underway (85). Nevertheless, the pro-inflammatory properties of ALA-PDT pose significant challenges to existing anti-inflammatory paradigms. This brings forth pivotal queries: Should we consider the implementation of M1 macrophage polarization in patients manifesting suitable conditions (86)? Additionally, can ALA-PDT therapy be administered in patients with mild conditions to halt disease progression, serving as an expansion of the existing indications for ALA-PDT (87)? Such inquiries indubitably mandate more extensive and focused research endeavors into the pathogenesis and treatment methodologies of acne vulgaris.
Author contributions
YF: Writing – original draft. JL: Writing – original draft. XM: Writing – review & editing. QJ: Writing – review & editing.
Funding
The author(s) declare financial support was received for the research, authorship, and/or publication of this article. This research was funded by the National Natural Science Foundation of China (82373506).
Conflict of interest
The authors declare that the research was conducted in the absence of any commercial or financial relationships that could be construed as a potential conflict of interest.
Publisher’s note
All claims expressed in this article are solely those of the authors and do not necessarily represent those of their affiliated organizations, or those of the publisher, the editors and the reviewers. Any product that may be evaluated in this article, or claim that may be made by its manufacturer, is not guaranteed or endorsed by the publisher.
References
1. Tan JKL, Bhate K. A global perspective on the epidemiology of acne. Br J Dermatol. (2015) 172 Suppl 1:3–12. doi: 10.1111/bjd.2015.172.issue-S1
2. Moradi Tuchayi S, Makrantonaki E, Ganceviciene R, Dessinioti C, Feldman SR, Zouboulis CC. Acne vulgaris. Nat Rev Dis Primers. (2015) 1:15029. doi: 10.1038/nrdp.2015.29
3. Zouboulis CC, Coenye T, He L, Kabashima K, Kobayashi T, Niemann C, et al. Sebaceous immunobiology - skin homeostasis, pathophysiology, coordination of innate immunity and inflammatory response and disease associations. Front Immunol. (2022) 13:1029818. doi: 10.3389/fimmu.2022.1029818
4. Eichenfield DZ, Sprague J, Eichenfield LF. Management of acne vulgaris: A review. JAMA. (2021) 326:2055–67. doi: 10.1001/jama.2021.17633
5. Williams HC, Dellavalle RP, Garner S. Acne vulgaris. Lancet. (2012) 379:361–72. doi: 10.1016/S0140-6736(11)60321-8
6. Jeremy AHT, Holland DB, Roberts SG, Thomson KF, Cunliffe WJ. Inflammatory events are involved in acne lesion initiation. J Invest Dermatol. (2003) 121:20–7. doi: 10.1046/j.1523-1747.2003.12321.x
7. Murray PJ, Wynn TA. Protective and pathogenic functions of macrophage subsets. Nat Rev Immunol. (2011) 11:723–37. doi: 10.1038/nri3073
8. Zhou C, Weng J, Liu C, Liu S, Hu Z, Xie X, et al. Disruption of SLFN11 deficiency-induced CCL2 signaling and macrophage M2 polarization potentiates anti-PD-1 therapy efficacy in hepatocellular carcinoma. Gastroenterology. (2023) 164:1261–78. doi: 10.1053/j.gastro.2023.02.005
9. Liu PS, Chen YT, Li X, Hsueh PC, Tzeng SF, Chen H, et al. CD40 signal rewires fatty acid and glutamine metabolism for stimulating macrophage anti-tumorigenic functions. Nat Immunol. (2023) 24:452–62. doi: 10.1038/s41590-023-01430-3
10. Kamata M, Tada Y. Dendritic cells and macrophages in the pathogenesis of psoriasis. Front Immunol. (2022) 13:941071. doi: 10.3389/fimmu.2022.941071
11. Zhou L, Zhao H, Zhao H, Meng X, Zhao Z, Xie H, et al. GBP5 exacerbates rosacea-like skin inflammation by skewing macrophage polarization towards M1 phenotype through the NF-κB signalling pathway. J Eur Acad Dermatol Venereol. (2023) 37:796–809. doi: 10.1111/jdv.18725
12. Wu X, Wang Z, Shi J, Yu X, Li C, Liu J, et al. Macrophage polarization toward M1 phenotype through NF-κB signaling in patients with Behçet’s disease. Arthritis Res Ther. (2022) 24:249. doi: 10.1186/s13075-022-02962-z
13. Italiani P, Boraschi D. From monocytes to M1/M2 macrophages: phenotypical vs. Functional differentiation. Front Immunol. (2014) 5:514/abstract. doi: 10.3389/fimmu.2014.00514/abstract
14. Nakai K. Multiple roles of macrophage in skin. J Dermatol Science. (2021) 104:2–10. doi: 10.1016/j.jdermsci.2021.08.008
15. Lovászi M, Mattii M, Eyerich K, Gácsi A, Csányi E, Kovács D, et al. Sebum lipids influence macrophage polarization and activation. Br J Dermatol. (2017) 177:1671–82. doi: 10.1111/bjd.15754
16. Christophers E, Schröder JM. Evolution of innate defense in human skin. Exp Dermatol. (2022) 31:304–11. doi: 10.1111/exd.14482
17. Shapouri-Moghaddam A, Mohammadian S, Vazini H, Taghadosi M, Esmaeili S, Mardani F, et al. Macrophage plasticity, polarization, and function in health and disease. J Cell Physiol. (2018) 233:6425–40. doi: 10.1002/jcp.26429
18. Feng X, Ji Y, Zhang C, Jin T, Li J, Guo J. CCL6 promotes M2 polarization and inhibits macrophage autophagy by activating PI3-kinase/Akt signalling pathway during skin wound healing. Exp Dermatol. (2023) 32:403–12. doi: 10.1111/exd.14718
19. Song E, Ouyang N, Hörbelt M, Antus B, Wang M, Exton MS. Influence of alternatively and classically activated macrophages on fibrogenic activities of human fibroblasts. Cell Immunol. (2000) 204:19–28. doi: 10.1006/cimm.2000.1687
20. Mantovani A, Sica A, Sozzani S, Allavena P, Vecchi A, Locati M. The chemokine system in diverse forms of macrophage activation and polarization. Trends Immunol. (2004) 25:677–86. doi: 10.1016/j.it.2004.09.015
21. Mariottoni P, Jiang SW, Prestwood CA, Jain V, Suwanpradid J, Whitley MJ, et al. Single-cell RNA sequencing reveals cellular and transcriptional changes associated with M1 macrophage polarization in Hidradenitis suppurativa. Front Med (Lausanne). (2021) 8:665873. doi: 10.3389/fmed.2021.665873
22. Pan Y, Yu Y, Wang X, Zhang T. Tumor-associated macrophages in tumor immunity. Front Immunol. (2020) 11:583084. doi: 10.3389/fimmu.2020.583084
23. Isohisa T, Asai J, Kanemaru M, Arita T, Tsutsumi M, Kaneko Y, et al. CD163-positive macrophage infiltration predicts systemic involvement in Sarcoidosis. J Cutan Pathol. (2020) 47:584–91. doi: 10.1111/cup.13675
24. Tanghetti EA. The role of inflammation in the pathology of acne. J Clin Aesthet Dermatol. (2013) 6(9):27–35.
25. Dainichi T, Iwata M. Inflammatory loops in the epithelial-immune microenvironment of the skin and skin appendages in chronic inflammatory diseases. Front Immunol. (2023) 14:1274270. doi: 10.3389/fimmu.2023.1274270
26. Degitz K, Placzek M, Borelli C, Plewig G. Pathophysiology of acne. JDDG. (2007) 5:316–23. doi: 10.1111/j.1610-0387.2007.06274.x
27. Freedberg IM, Tomic-Canic M, Komine M, Blumenberg M. Keratins and the keratinocyte activation cycle. J Invest Dermatol. (2001) 116:633–40. doi: 10.1046/j.1523-1747.2001.01327.x
28. Dreno B, Gollnick HPM, Kang S, Thiboutot D, Bettoli V, Torres V, et al. Understanding innate immunity and inflammation in acne: implications for management. Acad Dermatol Venereol. (2015) 29:3–11. doi: 10.1111/jdv.13190
29. Carlavan I, Bertino B, Rivier M, Martel P, Bourdes V, Motte M, et al. Atrophic scar formation in patients with acne involves long-acting immune responses with plasma cells and alteration of sebaceous glands. Br J Dermatol. (2018) 179:906–17. doi: 10.1111/bjd.2018.179.issue-4
30. Holland DB, Jeremy AHT, Roberts SG, Seukeran DC, Layton AM, Cunliffe WJ. Inflammation in acne scarring: a comparison of the responses in lesions from patients prone and not prone to scar. Br J Dermatol. (2004) 150:72–81. doi: 10.1111/j.1365-2133.2004.05749.x
31. Okoro EO, Bulus NG, Zouboulis CC. Study of facial sebum levels and follicular red fluorescence in patients with acne vulgaris in Nigeria. Dermatology. (2016) 232:156–61. doi: 10.1159/000439378
32. Zouboulis CC. Endocrinology and immunology of acne: Two sides of the same coin. Exp Dermatol. (2020) 29:840–59. doi: 10.1111/exd.14172
33. Crucet M, Wüst SJA, Spielmann P, Lüscher TF, Wenger RH, Matter CM. Hypoxia enhances lipid uptake in macrophages: role of the scavenger receptors Lox1, SRA, and CD36. Atherosclerosis. (2013) 229:110–7. doi: 10.1016/j.atherosclerosis.2013.04.034
34. AbdElneam AI, Al-Dhubaibi MS, Bahaj SS, Mohammed GF. Role of macrophage scavenger receptor 1 in the progression of dyslipidemia in acne vulgaris patients. Skin Res Technol. (2023) 29:e13424. doi: 10.1111/srt.13424
35. Qidwai A, Pandey M, Pathak S, Kumar R, Dikshit A. The emerging principles for acne biogenesis: A dermatological problem of puberty. Hum Microbiome J. (2017) 4:7–13. doi: 10.1016/j.humic.2017.05.001
36. Liu PT, Phan J, Tang D, Kanchanapoomi M, Hall B, Krutzik SR, et al. CD209+ Macrophages Mediate Host Defense against Propionibacterium acnes. J Immunol. (2008) 180:4919–23. doi: 10.4049/jimmunol.180.7.4919
37. Nauseef WM. Myeloperoxidase in human neutrophil host defence. Cell Microbiol. (2014) 16:1146–55. doi: 10.1111/cmi.12312
38. Do TH, Ma F, Andrade PR, Teles R, de Andrade Silva BJ, Hu C, et al. TREM2 macrophages induced by human lipids drive inflammation in acne lesions. Sci Immunol. (2022) 7:eabo2787. doi: 10.1126/sciimmunol.abo2787
39. Nakatsuji T, Tang D chu C, Zhang L, Gallo RL, Huang CM. Propionibacterium acnes CAMP factor and host acid sphingomyelinase contribute to bacterial virulence: potential targets for inflammatory acne treatment. PloS One. (2011) 6:e14797. doi: 10.1371/journal.pone.0014797
40. Tang T, Xu Y, Wang L, Zhang P. In vitro acne disease model from inertial focusing effect for studying the interactions between sebocyte glands and macrophages. Biotechnol J. (2023) 8(11):e2300108. doi: 10.1002/biot.202300108
41. Graham GM, Farrar MD, Cruse-Sawyer JE, Holland KT, Ingham E. Proinflammatory cytokine production by human keratinocytes stimulated with Propionibacterium acnes and P. acnes GroEL. Br J Dermatol. (2004) 150:421–8. doi: 10.1046/j.1365-2133.2004.05762.x
42. Nagy I, Pivarcsi A, Koreck A, Széll M, Urbán E, Kemény L. Distinct strains of Propionibacterium acnes induce selective human beta-defensin-2 and interleukin-8 expression in human keratinocytes through toll-like receptors. J Invest Dermatol. (2005) 124:931–8. doi: 10.1111/j.0022-202X.2005.23705.x
43. Kim J, Ochoa MT, Krutzik SR, Takeuchi O, Uematsu S, Legaspi AJ, et al. Activation of toll-like receptor 2 in acne triggers inflammatory cytokine responses. J Immunol. (2002) 169:1535–41. doi: 10.4049/jimmunol.169.3.1535
44. Tsai HH, Lee WR, Wang PH, Cheng KT, Chen YC, Shen SC. Propionibacterium acnes-induced iNOS and COX-2 protein expression via ROS-dependent NF-κB and AP-1 activation in macrophages. J Dermatol Sci. (2013) 69:122–31. doi: 10.1016/j.jdermsci.2012.10.009
45. Fischer K, Tschismarov R, Pilz A, Straubinger S, Carotta S, McDowell A, et al. Cutibacterium acnes Infection Induces Type I Interferon Synthesis Through the cGAS-STING Pathway. Front Immunol. (2020) 11:571334. doi: 10.3389/fimmu.2020.571334
46. Takeuchi O, Hoshino K, Kawai T, Sanjo H, Takada H, Ogawa T, et al. Differential roles of TLR2 and TLR4 in recognition of gram-negative and gram-positive bacterial cell wall components. Immunity. (1999) 11:443–51. doi: 10.1016/S1074-7613(00)80119-3
47. Chen C, Xu P. Activation and pharmacological regulation of inflammasomes. Biomolecules. (2022) 12:1005. doi: 10.3390/biom12071005
48. Qin M, Pirouz A, Kim MH, Krutzik SR, Garbán HJ, Kim J. Propionibacterium acnes Induces IL-1β Secretion via the NLRP3 Inflammasome in Human Monocytes. J Invest Dermatol. (2014) 134:381–8. doi: 10.1038/jid.2013.309
49. Deng W, Bai Y, Deng F, Pan Y, Mei S, Zheng Z, et al. Streptococcal pyrogenic exotoxin B cleaves GSDMA and triggers pyroptosis. Nature. (2022) 602:496–502. doi: 10.1038/s41586-021-04384-4
50. Lian N, Chen Y, Chen S, Zhang Y, Chen H, Yang Y, et al. Gasdermin D-mediated keratinocyte pyroptosis as a key step in psoriasis pathogenesis. Cell Death Dis. (2023) 14:595. doi: 10.1038/s41419-023-06094-3
51. Akcılar R, Dizen Namdar N, Arslan Utku S. Association between resistin gene (-420 C > G) polymorphism and acne vulgaris. J Cosmet Dermatol. (2022) 21(4):1651–5. doi: 10.1111/jocd.14264
52. Goodman GJ. Acne and acne scarring: why should we treat? Med J Aust. (1999) 171:62–3. doi: 10.5694/j.1326-5377.1999.tb123518.x
53. Hesketh M, Sahin KB, West ZE, Murray RZ. Macrophage phenotypes regulate scar formation and chronic wound healing. IJMS. (2017) 18:1545. doi: 10.3390/ijms18071545
54. Kidzeru EB, Lebeko M, Sharma JR, Nkengazong L, Adeola HA, Ndlovu H, et al. Immune cells and associated molecular markers in dermal fibrosis with focus on raised cutaneous scars. Exp Dermatol. (2023) 32:570–87. doi: 10.1111/exd.14734
55. MacLeod AS, Mansbridge JN. The innate immune system in acute and chronic wounds. Adv Wound Care (New Rochelle). (2016) 5:65–78. doi: 10.1089/wound.2014.0608
56. Saint-Jean M, Khammari A, Jasson F, Nguyen JM, Dréno B. Different cutaneous innate immunity profiles in acne patients with and without atrophic scars. Eur J Dermatol. (2016) 26:68–74. doi: 10.1684/ejd.2015.2713
57. Xu X, Gu S, Huang X, Ren J, Gu Y, Wei C, et al. The role of macrophages in the formation of hypertrophic scars and keloids. Burns Trauma. (2020) 8:tkaa006. doi: 10.1093/burnst/tkaa006
58. Gordon S, Martinez FO. Alternative activation of macrophages: mechanism and functions. Immunity. (2010) 32:593–604. doi: 10.1016/j.immuni.2010.05.007
59. Li X, Wang Y, Yuan B, Yang H, Qiao L. Status of M1 and M2 type macrophages in keloid. Int J Clin Exp Pathol. (2017) 10:11098–105.
60. Li M, Hou Q, Zhong L, Zhao Y, Fu X. Macrophage related chronic inflammation in non-healing wounds. Front Immunol. (2021) 12:681710. doi: 10.3389/fimmu.2021.681710
61. Hong YK, Hwang DY, Yang CC, Cheng SM, Chen PC, Aala WJ, et al. Profibrotic subsets of SPP1+ Macrophages and POSTN+ Fibroblasts contribute to fibrotic scarring in acne Keloidalis. J Invest Dermatol. (2024). doi: 10.1016/j.jid.2023.12.014
62. Liu P, Liu X, Zhang L, Yan G, Zhang H, Xu D, et al. ALA-PDT augments intense inflammation in the treatment of acne vulgaris by COX2/TREM1 mediated M1 macrophage polarization. Biochem Pharmacol. (2023) 208:115403. doi: 10.1016/j.bcp.2022.115403
63. Ju Q. Guideline for diagnosis and treatment of acne. J Clin Dermatol. (2019) 48(09):583–8. doi:10.16761/j.cnki.1000-4963.2019.09.020
64. Liu PT, Krutzik SR, Kim J, Modlin RL. Cutting edge: all-trans retinoic acid down-regulates TLR2 expression and function. J Immunol. (2005) 174:2467–70. doi: 10.4049/jimmunol.174.5.2467
65. Ji YB, Lee HY, Lee S, Kim YH, Na K, Kim JH, et al. Electrostatically optimized adapalene-loaded emulsion for the treatment of acne vulgaris. Mater Today Bio. (2022) 16:100339. doi: 10.1016/j.mtbio.2022.100339
66. Kang OH, Lee GH, Choi HJ, Park PS, Chae HS, Jeong SI, et al. Ethyl acetate extract from Angelica Dahuricae Radix inhibits lipopolysaccharide-induced production of nitric oxide, prostaglandin E2 and tumor necrosis factor-α via mitogen-activated protein kinases and nuclear factor-κB in macrophages. Pharmacol Res. (2007) 55:263–70. doi: 10.1016/j.phrs.2006.12.001
67. Lim HJ, Kang SH, Song YJ, Jeon YD, Jin JS. Inhibitory effect of quercetin on propionibacterium acnes-induced skin inflammation. Int Immunopharmacol. (2021) 96:107557. doi: 10.1016/j.intimp.2021.107557
68. Chen LW, Chung HL, Wang CC, Su JH, Chen YJ, Lee CJ. Anti-acne effects of cembrene diterpenoids from the cultured soft coral Sinularia flexibilis. Mar Drugs. (2020) 18:487. doi: 10.3390/md18100487
69. Park CH, Min SY, Yu HW, Kim K, Kim S, Lee HJ, et al. Effects of apigenin on RBL-2H3, RAW264.7, and HaCaT cells: anti-allergic, anti-inflammatory, and skin-protective activities. Int J Mol Sci. (2020) 21:4620. doi: 10.3390/ijms21134620
70. Jin S, Lee MY. Kaempferia parviflora Extract as a Potential Anti-Acne Agent with Anti-Inflammatory, Sebostatic and Anti-Propionibacterium acnes Activity. Int J Mol Sci. (2018) 19:3457. doi: 10.3390/ijms19113457
71. Yang G, Lee HE, Yeon SH, Kang HC, Cho YY, Lee HS, et al. Licochalcone A attenuates acne symptoms mediated by suppression of NLRP3 inflammasome. Phytother Res. (2018) 32:2551–9. doi: 10.1002/ptr.6195
72. Sekiguchi K, Koseki J, Tsuchiya K, Matsubara Y, Iizuka S, Imamura S, et al. Suppression of Propionibacterium acnes -induced dermatitis by a traditional Japanese medicine, Jumihaidokuto, modifying macrophage functions. Evidence-Based Complementary Altern Med. (2015) 2015:1–10. doi: 10.1155/2015/439258
73. Thiboutot DM, Dréno B, Abanmi A, Alexis AF, Araviiskaia E, Barona Cabal MI, et al. Practical management of acne for clinicians: An international consensus from the Global Alliance to Improve Outcomes in Acne. J Am Acad Dermatol. (2018) 78:S1–S23.e1. doi: 10.1016/j.jaad.2017.09.078
74. Evangelou G, Farrar MD, White RD, Sorefan NB, Wright KP, McLean K, et al. Topical aminolaevulinic acid-photodynamic therapy produces an inflammatory infiltrate but reduces Langerhans cells in healthy human skin in vivo. Br J Dermatol. (2011) 165:513–9. doi: 10.1111/bjd.2011.165.issue-3
75. Zhang L, Yang J, Liu X, Xu D, Shi L, Liu J, et al. 5-Aminolaevulinic acid photodynamic therapy amplifies intense inflammatory response in the treatment of acne vulgaris via CXCL8. Exp Dermatol. (2021) 30:923–31. doi: 10.1111/exd.14357
76. Zuo G, Gao Y, Lu G, Bu M, Liu J, Zhang J, et al. Auriculotherapy modulates macrophage polarization to reduce inflammatory response in a rat model of acne. Mediators Inflamm. (2023) 2023:6627393. doi: 10.1155/2023/6627393
77. Wu J, Guo R, Chai J, Xiong W, Tian M, Lu W, et al. The protective effects of Cath-MH with anti-propionibacterium acnes and anti-inflammation functions on acne vulgaris. Front Pharmacol. (2021) 12:788358. doi: 10.3389/fphar.2021.788358
78. Shin JH, Kim SS, Seo SR. Pyrrolidine dithiocarbamate suppresses cutibacterium acnes-induced skin inflammation. Int J Mol Sci. (2023) 24:4444. doi: 10.3390/ijms24054444
79. Kim BH, Roh E, Lee HY, Lee IJ, Ahn B, Jung SH, et al. Benzoxathiole derivative blocks lipopolysaccharide-induced nuclear factor-kappaB activation and nuclear factor-kappaB-regulated gene transcription through inactivating inhibitory kappaB kinase beta. Mol Pharmacol. (2008) 73:1309–18. doi: 10.1124/mol.107.041251
80. Chung EY, Kim BH, Lee IJ, Roh E, Oh SJ, Kwak JA, et al. The benzoxathiolone LYR-71 down-regulates interferon-gamma-inducible pro-inflammatory genes by uncoupling tyrosine phosphorylation of STAT-1 in macrophages. Br J Pharmacol. (2009) 158:1971–81. doi: 10.1111/j.1476-5381.2009.00496.x
81. Yang G, Lee SJ, Kang HC, Cho YY, Lee HS, Zouboulis CC, et al. Repurposing auranofin, an anti-rheumatic gold compound, to treat acne vulgaris by targeting the NLRP3 inflammasome. Biomol Ther (Seoul). (2020) 28:437–42. doi: 10.4062/biomolther.2020.004
82. Lee H, Hwang D, Lee M, Lee J, Cho S, Kim TJ, et al. Micro-Current Stimulation Suppresses Inflammatory Responses in Peptidoglycan-Treated Raw 264.7 Macrophages and Propionibacterium acnes-Induced Skin Inflammation via TLR2/NF-κB Signaling Pathway. Int J Mol Sci. (2022) 23:2508. doi: 10.3390/ijms23052508
83. Wang R, Yan S, Ma X, Zhao J, Han Y, Pan Y, et al. The pivotal role of Bifida Ferment Lysate on reinforcing the skin barrier function and maintaining homeostasis of skin defenses in vitro. J Cosmet Dermatol. (2023) 22(12):3427–35. doi: 10.1111/jocd.15831
84. Chen J, Fu Y, Xiong S. Keratinocyte derived HMGB1 aggravates psoriasis dermatitis via facilitating inflammatory polarization of macrophages and hyperproliferation of keratinocyte. Mol Immunol. (2023) 163:1–12. doi: 10.1016/j.molimm.2023.09.004
85. Wang P, Wang B, Zhang L, Liu X, Shi L, Kang X, et al. Clinical practice Guidelines for 5-Aminolevulinic acid photodynamic therapy for acne vulgaris in China. Photodiagnosis Photodyn Ther. (2023) 41:103261. doi: 10.1016/j.pdpdt.2022.103261
86. Ahmadvand A, Yazdanfar A, Yasrebifar F, Mohammadi Y, Mahjub R, Mehrpooya M. Evaluating the effects of oral and topical simvastatin in the treatment of acne vulgaris: A double-blind, randomized, placebo-controlled clinical trial. Curr Clin Pharmacol. (2018) 13:279–83. doi: 10.2174/1574884713666180821143545
Keywords: acne vulgaris, macrophages, Cutibacterium acnes, inflammation, therapeutics
Citation: Feng Y, Li J, Mo X and Ju Q (2024) Macrophages in acne vulgaris: mediating phagocytosis, inflammation, scar formation, and therapeutic implications. Front. Immunol. 15:1355455. doi: 10.3389/fimmu.2024.1355455
Received: 14 December 2023; Accepted: 28 February 2024;
Published: 14 March 2024.
Edited by:
Kazuhiko Yamamura, Kyushu University, JapanReviewed by:
Shisan (Bob) Bao, The University of Sydney, AustraliaWilliam D. Shipman, Yale University, United States
Copyright © 2024 Feng, Li, Mo and Ju. This is an open-access article distributed under the terms of the Creative Commons Attribution License (CC BY). The use, distribution or reproduction in other forums is permitted, provided the original author(s) and the copyright owner(s) are credited and that the original publication in this journal is cited, in accordance with accepted academic practice. No use, distribution or reproduction is permitted which does not comply with these terms.
*Correspondence: Xiaohui Mo, moxiaohui2012@sina.cn; Qiang Ju, qiangju@aliyun.com
†These authors have contributed equally to this work and share first authorship