- 1Department of Pathology, Brigham and Women’s Hospital, Boston, MA, United States
- 2Case Western Reserve University School of Medicine, Cleveland, OH, United States
- 3University of California, San Diego, CA, United States
- 4Northeastern University, Boston, MA, United States
Hepatocellular carcinoma (HCC) is a challenging malignancy with limited treatment options beyond surgery and chemotherapy. Recent advancements in targeted therapies and immunotherapy, including PD-1 and PD-L1 monoclonal antibodies, have shown promise, but their efficacy has not met expectations. Biomarker testing and personalized medicine based on genetic mutations and other biomarkers represent the future direction for HCC treatment. To address these challenges and opportunities, this comprehensive review discusses the progress made in targeted therapies and immunotherapies for HCC, focusing on dissecting the rationales, opportunities, and challenges for combining these modalities. The liver’s unique physiology and the presence of fibrosis in many HCC patients pose additional challenges to drug delivery and efficacy. Ongoing efforts in biomarker development and combination therapy design, especially in the context of immunotherapies, hold promise for improving outcomes in advanced HCC. Through exploring the advancements in biomarkers and targeted therapies, this review provides insights into the challenges and opportunities in the field and proposes strategies for rational combination therapy design.
1 Introduction
Hepatocellular carcinoma (HCC) is the most prevalent form of primary liver cancer, accounting for 75-90% of all cases. It is characterized by a high mortality rate and ranks as the third leading cause of cancer-related deaths worldwide. It is responsible for over 600,000 deaths annually with the highest incidence in Asia, and its incidence and mortality in Europe and the United States are rising steadily. Effective treatments are still lacking, and the 5-year overall survival rate remains extremely poor. The prognosis of HCC heavily depends on the stage at the time of diagnosis. While surgery, liver transplantation, or radiological intervention may be viable options for early-stage disease, especially under a multidisciplinary tumor board (MDT) setting (1, 2), the prognosis for advanced-stage HCC remains bleak, with most patients succumbing within 20 months after diagnosis. Unfortunately, even with available treatments for early-stage patients, the majority of HCC cases still progress to advanced stages, presenting a significant clinical challenge (3, 4). For many years, combination chemotherapy has not been shown to improve overall survival but has nonetheless been in wide usage due to its possible benefit in palliation.
HCC is associated with various risk factors, with the most common being hepatitis B virus (HBV) or hepatitis C virus (HCV) infection, long-term alcohol consumption, and non-alcoholic fatty liver disease (NAFLD). Other less common causes include obesity, diabetes, aflatoxin exposure, hereditary hemochromatosis, smoking, tyrosinemia, and glycogen storage disease type 1a (5, 6). The pathophysiology of HCC is complex, and hepatocarcinogenesis is not yet fully understood. It is believed that the accumulation of genetic and epigenetic changes during cirrhosis contributes to the development of HCC by deregulating the cell cycle and suppressing apoptosis. Telomere dysfunction and alterations in the micro and macro environment have been implicated as possible mechanisms accelerating HCC development in cirrhotic livers (7, 8). The diverse etiologies lead to various HCC subtypes that may respond preferentially to therapies (9).
Transcription factors (TF) such as Spalt-like protein 4 (SALL4) and signaling pathways such as Ras/Raf/Mitogen-activated protein kinase/ERK kinase (MEK)/extracellular-signal-regulated kinase (ERK) pathway, phosphatidylinositol-3-kinase (PI3K)/Akt/mechanistic target of rapamycin (mTOR) pathway, Wnt/β-catenin pathway, and Janus kinase/signal transducer and activator of transcription (JAK/STAT) pathway have been identified as key players in HCC (7). These pathways are directly regulated by receptor tyrosine kinases (RTK). A burgeoning number of targeted therapies have been developed with many of them targeting RTKs such as epidermal growth factor receptor (EGFR), vascular endothelial growth factor receptors (VEGFR), and platelet-derived growth factor receptor (PDGFR).
In addition to RTKs, immune checkpoints are key players in regulating HCC. Immune checkpoints play a vital role in moderating immune responses to prevent autoimmune diseases. HCC tumors can upregulate the expression of Programmed Death Ligand 1 (PD-L1), which then interacts with Programmed Death Protein 1 (PD-1) on T cells, effectively suppressing the immune response. This interaction helps the tumor cells evade immune surveillance and clearance, leading to tumor progression. Immune checkpoint inhibitors (ICI) are now widely used in multiple tumor types including HCC.
However, it is crucial to note that these current “gold standard” treatments for advanced HCC primarily aim to extend the lives of patients rather than to provide a cure. Sorafenib is a multikinase inhibitor (MKI) designed to target RTKs such as VEGFRs and PDGFR, but it suffers from a low response rate of 1-2%. A large clinical trial involving centers in Europe and America (SHARP trial) showed that it improved median overall survival of around 2 months (10). A following trial in Asia Pacific (Sorafenib AP trial) showed similar results, improving median overall survival from 4.2 months to 6.5 months (11). Other kinase inhibitors developed to target HCC include Regorafenib by Bayer (12) and Lenvatinib by Merck (13) showed improvement of median survival around 3 months.
These less than satisfactory results showed an urgent need to look for disruptive therapeutic options for the benefit of HCC patients. In recent years, there have been remarkable transformations in the standard of care (SOC) and the available options for HCC treatment in the advanced stages, where the unmet needs are the highest. The recent approval of ICI + VEGF combination therapy, Avastin + Tecentriq (atezolizumab plus bevacizumab), by the Food and Drug Administration (FDA) in May 2020 led to its replacement of the SOC in first-line advanced HCC, shifting MKIs increasingly towards second-line options. Although the IMBrave150 trial of Tecentriq-Avastin initially demonstrated a 42% reduction in the risk of death and a 41% reduction in the risk of disease progression or death compared to Sorafenib, an updated analysis performed 12 months after the primary analysis of IMbrave150 showed that Median OS was only 5.8 months longer with atezolizumab plus bevacizumab than sorafenib (14, 15). Nonetheless, its relative success channeled tremendous enthusiasm and optimism towards the use of immunotherapy and combination approaches, such as combining ICI and targeted therapies, in a new era of HCC drug development.
In addition to advanced HCC, there is ongoing development and potential approval of systemic therapies in combination with transarterial chemoembolization (TACE) for intermediate-stage patients and in the adjuvant setting (16). Adjuvant treatments may also hold promise for reducing recurrence rates in early-stage patients. In line with general trends in oncological drug development, systemic therapy is expected to be more effective and less associated with adverse effects, especially for intermediate-stage patients who already have access to locoregional therapy (17). These trends call for combining different mechanisms of action (MOAs) and expanding into precision-targeted therapy.
In this comprehensive review, we aim to delve into the advancements in biomarkers and targeted therapies for HCC, exploring the challenges, opportunities, and recent developments in the field. We will discuss the different mechanisms of action of pathogenic pathways that have led to the design of recent and emerging targeted therapies and combination therapies. We will also discuss the ongoing effort of biomarker development, and we will propose ways of combination therapy can be rationally designed, especially in the setting of immunotherapy which has gained the strongest traction in the recent years for the treatment of advanced HCC.
2 HCC biomarkers: from disease surveillance to therapeutic targeting
2.1 HCC biomarkers for screening and diagnosis: current standard of care
Early detection and diagnosis of HCC is crucial for effective treatment and improved patient outcomes. As an important piece of the puzzle for precision medicine, diagnostic biomarkers are not only crucial for accurate diagnostic evaluation, risk stratification, and prognosis, but are also playing increasingly important roles in assisting/guiding targeted therapies. Currently, The most commonly used surveillance modalities include ultrasound, serum alpha-fetoprotein (AFP) measurement, or a combination of both. AFP is an “oncofetal” protein produced by the liver and yolk sac during fetal development. It is re-expressed by HCC cells and its upregulation can be readily detected via routine blood tests (18–20). Although it is the most widely used serum biomarker for HCC surveillance and monitoring, it has suboptimal specificity due to higher AFP expression in non-cancerous liver regeneration (21). According to the American Association for the Study of Liver Diseases (AASLD) Practice Guidance, it recommends HCC surveillance using a combination of liver ultrasound and AFP with a sensitivity in detecting early-stage HCC of 63% (95% CI, 48%-75%) (Figure 1). HCC diagnosis can be made in at-risk patients based only on specific noninvasive imaging criteria without histologic confirmation, via contrast-enhanced multiphase computed tomography CT or magnetic resonance imaging (MRI) (22).
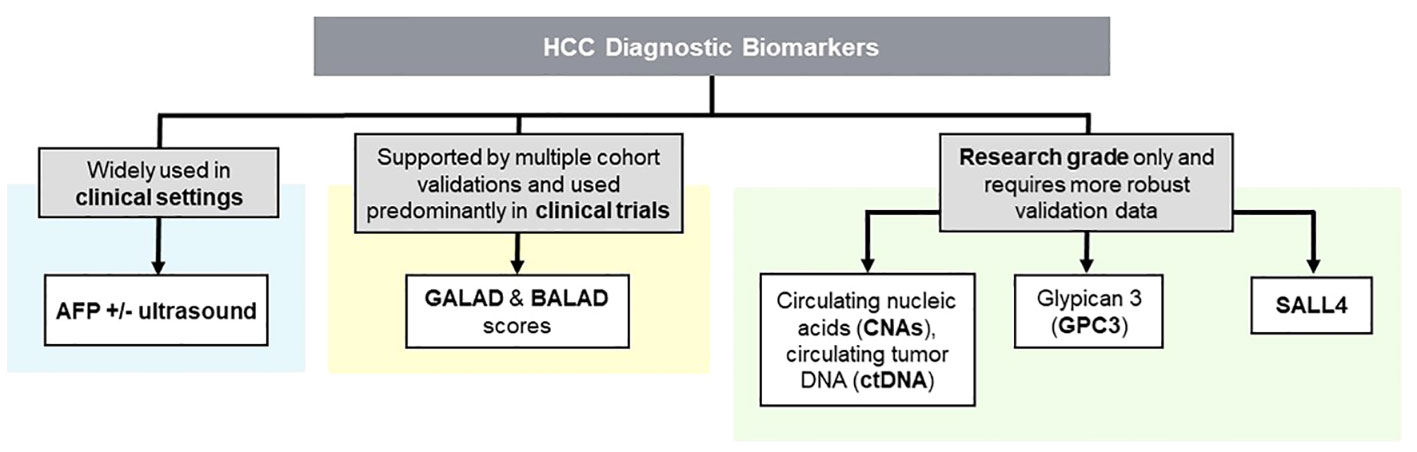
Figure 1 Clinical and Research Grade HCC Diagnostic Biomarkers; an Overview. Biomarkers in HCC are classified into clinical and research categories. While clinical biomarkers aid accurate patient assessment such as GALAD and BALAD scores, ongoing research aims to develop novel biomarkers for improved diagnosis and treatment.
2.2 Composite HCC biomarker scores under development
A combination of multiple biomarkers may improve the accuracy and sensitivity of HCC screening, as demonstrated by the development of GALAD and BALAD scores, both of which have been extensively validated in cohort studies. (Figure 1) The GALAD score is an example of a combination diagnostic method incorporating AFP, patient’s age, gender, and two other biomarkers: des-carboxy-prothrombin (DCP) and lens culinaris agglutinin-reactive fraction of AFP (AFP-L3) (23). In a cohort of Caucasian patients with chronic viral hepatitis, the GALAD model demonstrated an area under the receiver operating characteristic curve (AUC) of 0.94 when differentiating HCC from non-HCC. This value was found to be higher than AUCs of 0.86, 0.83, and 0.83 when AFP, AFP-L3, and DCP biomarkers are used alone, respectively (24). Furthermore, a meta-analysis of 15 studies with 19 cohorts from both Western and Eastern countries yielded a sensitivity, specificity, and AUC of 0.82, 0.89, and 0.92, with approximately 70% chance of detecting early-stage tumors (23). Similarly, the BALAD score is a surveillance method using 5 serum markers: bilirubin, albumin, AFP-L3, AFP, and DCP. In a study involving 2,600 HCC patients across 5 Japanese institutions, BALAD demonstrated a comparable ability to predict patient survival to both tumor stages and other scoring systems. These include the Cancer of the Liver Italian Program and Japan Integrated Staging scoring systems, which account for tumor progression and remnant liver function (25). In a North American cohort consisting of 148 patients, the BALAD score was found to be significantly correlated to patient survival, with its performance comparable to Barcelona Clinic Liver Cancer staging and GALAD scores (26). In addition to BALAD and GALAD scores, other AFP-based multi-biomarker algorithms have been attempted in the research setting such as the HCC Early Detection Screening (HES) algorithm which incorporates current AFP and change in AFP over the last year, age, platelets, alanine aminotransferase (ALT) for HCC screening (27). A study on a cohort of patients with liver cirrhosis from the University of Michigan utilized a prospective-specimen-collection, retrospective-blinded-evaluation design to assess the accuracy of the HES algorithm. It exhibited an AUC of 0.70 when screening for early stage HCC, and a sensitivity of 34.6% to 45.2% and specificity of 90.5% for any-stage and early stage HCC.
2.3 Emerging novel HCC biomarkers
Recent studies have explored novel serum/plasma biomarkers, such as circulating nucleic acids (CNAs), circulating tumor DNA (ctDNA), Glypican-3 (GPC3), and SALL4 (Figure 1). CNAs are fragments of DNA or RNA that are released from cancer cells into the bloodstream and can be detected using non-invasive liquid biopsy techniques (28). Several studies have reported that CNAs, such as circulating tumor DNA (ctDNA) and microRNAs (miRNAs), can serve as promising biomarkers for HCC diagnosis, prognosis, and treatment response monitoring (29–31). ctDNA refers to small fragments of DNA that are released into the bloodstream by tumor cells. Several studies have demonstrated the potential of ctDNA as a diagnostic biomarker for HCC. For example, a study by Zhao et al. (2020) found that ctDNA could accurately detect HCC with a high sensitivity and specificity (32). It may outperform conventional biomarkers such as AFP for the early detection of HCC, especially in patients with small tumors or those at high risk for HCC development.
GPC3 and SALL4 are two other oncofetal protein biomarkers with similar properties to AFP, and their roles in HCC screening/diagnosis are an area of active investigation. GPC3 is a proteoglycan anchored to the cell membrane that is normally detected in the fetal liver but not in the healthy adult liver. However, GPC3 is overexpressed at both the RNA and protein levels in HCC patients, making it a promising HCC biomarker candidate. Serum levels of GPC3 can be detected using enzyme-linked immunosorbent assay (ELISA), and immunohistochemistry (IHC) can confirm its expression in tumor tissues (33). GPC3 expression has been observed in malignant hepatocytes in hepatoblastomas and in 84% of HCC (33). In addition, GPC3, in combination with AFP and/or imaging techniques such as ultrasound, CT, and MRI, has shown high sensitivity and specificity for the diagnosis of HCC (34). While GPC3 alone has a sensitivity of 55.1% and specificity of 97%, a combination of GPC3 and AFP has a sensitivity of 75.7% and specificity of 83.3% for HCC diagnosis when tumors are less than 3 cm (35). In addition to its use in diagnosis, GPC3 is also being studied as a potential prognostic biomarker for HCC. Studies have shown that GPC3 expression levels in HCC tissues are associated with tumor aggressiveness and poor prognosis, including tumor size, vascular invasion, and metastasis (36, 37). Elevated serum levels of GPC3 have also been associated with worse overall survival and disease-free survival in patients with HCC (38). Based on these data, GPC3 may be useful for stratifying HCC patients based on their prognosis and guiding treatment decisions.
SALL4 is a zinc finger TF involved during fetal liver development that acts as a HCC oncogene and is highly expressed in HCC cells (39–41). In contrast to GPC3 and AFP, which can be highly expressed in regenerating liver tissue affected by non-neoplastic lesions such as hepatitis (GPC3 and AFP) and liver cirrhosis (AFP) (21, 33), SALL4 serological levels were found to be considerably higher in HCC patients compared to patients with cirrhosis or chronic hepatitis, potentially offering better specificity as a biomarker. A similar trend was observed in SALL4 expression levels across HCC tissues, as compared to liver hemangioma and adjacent noncancerous hepatic tissue (39, 41). SALL4 expression is correlated to poor prognosis and serves as a prominent biomarker predicting HCC progression (39–41). Interestingly, there has been a wide variation in SALL4 positivity observed from different publications focusing on different patient demographics. Analysis of SALL4 expression in Eastern regions such as China and Singapore demonstrated a significantly upregulated SALL4 expression in HCC tissues relative to non-neoplastic tissues. Approximately 46% and 55.6% of HCC tissue specimens from the Chinese (n = 126) and Singaporean (n = 171) cohorts exhibited SALL4 positivity (40, 41). In studies concerning Western regions, 26% and 46% of HCC tumors from patients in the Netherlands (n = 133) and United States (n = 69) cohorts were positive for SALL4 (42, 43). Contrastingly, an analysis of 236 cases in Washington state yielded only 3 cases positive for SALL4 (1.3%); however, the validity of the results remains questionable due to multiple shortcomings of the study which include a deviation from published liver carcinoma staining protocols and the use of a core biopsy method known to be highly susceptible to sampling bias and false negatives (44). In Eastern regions, HBV infections are the most common causes of HCC cases, with HBV-positive patients composing 31 to 91% of total HCC patients across multiple studies (45–50). In Western regions, the prevalence is relatively lower, with approximately 12 to 23% of HCC patients demonstrating HBV-positivity (42, 51). Given the upregulated SALL4 expression in nearly 50% of HBV-related HCCs, it is speculated that HBV infection may be associated with SALL4 re-expression (40). In a study by Fan et al, HBV-related HCCs exhibited significant hypomethylation in the regulatory region of SALL4. The same DNA demethylation patterns were observed in liver cancer cell lines and HBV- and HCV related HCCs with SALL4 overexpression attributable to increased STAT3 and OCT4 binding at the respective hypomethylated sites (52). These results altogether suggest that HBV or HCV may increase the risk of HCC development in a SALL4-dependent manner, and that SALL4 diagnostic/prognostic biomarker may perform the best in patients whose HCC is caused by hepatitis infections.
Interestingly, GPC3, SALL4, and AFP expressions are highly intertwined. There is a significant positive correlation between SALL4 expression and GPC3 expression in HCC patients, and patients with SALL4-positive HCC exhibited higher levels of AFP in serum (42, 53). In a cohort of combined HCC and cholangiocarcinoma, GPC3 immunopositivity rates were much higher in SALL4-positive samples than that of SALL4-negative samples (54). HCC patients co-expressing GPC3 and SALL4 exhibit a trend towards worse HCC-specific survival than high expression of either biomarker alone (42). Similarly, patients who coexpressed both SALL4 and AFP exhibited a significantly worse prognosis than patients who were either positive for only one biomarker or negative for both, and patients who were positive for SALL4 demonstrated a trend towards worse prognosis with increasing AFP expression levels (41). Co-expression of GPC3 and SALL4 has also been correlated with vascular invasion, poor differentiation, and higher AFP levels in HCC patients (33). Evaluating the coexpression patterns of SALL4, AFP, and GPC3 may offer opportunities for designing novel biomarker combinations and yield better insight into the patient’s disease course.
2.4 Oncofetal-biomarker-turned-targeted therapies
In the case of AFP, GPC3 and SALL4, the boundary between diagnostic biomarkers and therapeutic targeting are increasingly blurred, and these proteins have emerged as biomarker-guided HCC precision medicine. As tumor markers that tend to be more highly expressed in HCC cells while lowly expressed in normal hepatic cells, GPC3 and AFP can aid in the direct targeting of tumor cells by cellular therapy modalities, such as monoclonal antibodies and Car-T (55, 56). In the remaining part of this section, we briefly outline the innovative therapeutic approaches utilizing the high expression of GPC3 and AFP to identify tumor cells. Although SALL4 is also an oncofetal protein, it has distinct properties as a HCC oncogenic driver, and we will describe SALL4 targeting in the subsequent section of the paper that focuses on HCC precision medicine via targeting oncogenic pathways and mechanisms.
In HCC tumor tissues, AFP is found intracellularly and extracellularly via presentation by a major histocompatibility complex (MHC) class I molecule (57). AFP-targeting therapies have mainly utilized chimeric antigen receptor (CAR) T-cell therapy, which involves the use of genetically modified T cells engineered to recognize target antigens expressed on tumor cells and elicit an immune response (58). The ability of tumor cells to evade immune surveillance by downregulating MHC class I molecules on its surface and the intracellular localization of AFP provide considerable challenges to AFP-targeting therapies (57). While Liu et al. successfully demonstrated the antitumoral effects in preclinical HCC models by engineering AFP-CAR T cells capable of recognizing the AFP peptide presented by MHC class I molecules (59), a subsequent phase I trial (NCT03349255) with the AFP-CAR T cells was terminated (60).
Antibodies targeting GPC3, such as GC33 and HN3, have been developed and are being studied in clinical trials for the treatment of HCC (61–63). These antibodies are designed to specifically target and kill GPC3-expressing cancer cells, while sparing normal liver cells that do not express GPC3. In addition, GPC3-targeted CAR T-cell therapy is also being investigated in clinical trials for the treatment of HCC (64). These therapies have shown promising results in preclinical studies and early-phase clinical trials, highlighting the potential of GPC3 as a therapeutic target in HCC. Multiple early phase I and II clinical trials are presently studying anti GPC3 CAR T-cell therapy alone or in combination with conventional chemotherapy, or other therapeutic alternatives (65–67).
In summary, surveillance, diagnosis, progression monitoring, and prognostication of HCC can be challenging due to the lack of highly sensitive and specific biomarkers. Current diagnostic modalities centering around AFP and imaging have their respective limitations, highlighting the need for novel biomarkers and improved surveillance techniques. Incorporating composite scoring schema (e.g. GALAD and BALAD scores), and incorporating novel biomarkers such as GPC3 and SALL4, have shown promise in the diagnosis and prognostication of HCC. Excitingly, some HCC diagnostic biomarkers have found new roles in HCC therapeutic targeting, which are under active pre-clinical and clinical trial investigations.
3 Exploring targeted therapies in HCC: the urgent need for biomarker-guided precision medicine focusing on oncogenic pathways and transcription factors
At present, the HCC drug development landscape is dominated by immunotherapies, immunomodulators, as well as kinase inhibitors. There is a limited availability of biomarker-guided precision medicine in HCC treatment. This can be attributed to several factors. Firstly, HCC is a complex and heterogeneous disease with various underlying molecular alterations, making it challenging to identify specific biomarkers that can reliably guide targeted therapy selection. Additionally, the majority of HCC cases are diagnosed at advanced stages when curative treatments are often no longer feasible. This late-stage diagnosis limits the opportunity for biomarker identification and targeted therapy initiation. Furthermore, the liver’s unique physiology and the presence of cirrhosis in many HCC patients pose additional challenges in drug delivery and efficacy. The development of targeted therapies requires extensive research, clinical trials, and regulatory approvals, which can be time-consuming and costly. Despite these challenges, ongoing research efforts are focused on identifying novel biomarkers and therapeutic targets to advance the field of precision medicine in HCC, with the hope of improving patient outcomes in the future. In addition, it is important to emphasize that targeted therapy in HCC necessitates the availability of reliable biomarkers for patient selection and treatment monitoring. The complex nature of HCC and its molecular heterogeneity make the identification of specific biomarkers challenging. However, the integration of biomarker-guided precision medicine can enhance the efficacy and effectiveness of targeted therapies in HCC. As research progresses and novel biomarkers are identified, the development of personalized treatment approaches will become increasingly feasible, offering a promising avenue for improving patient outcomes. These insights are further corroborated by the specific mechanisms of action detailed in Table 1.
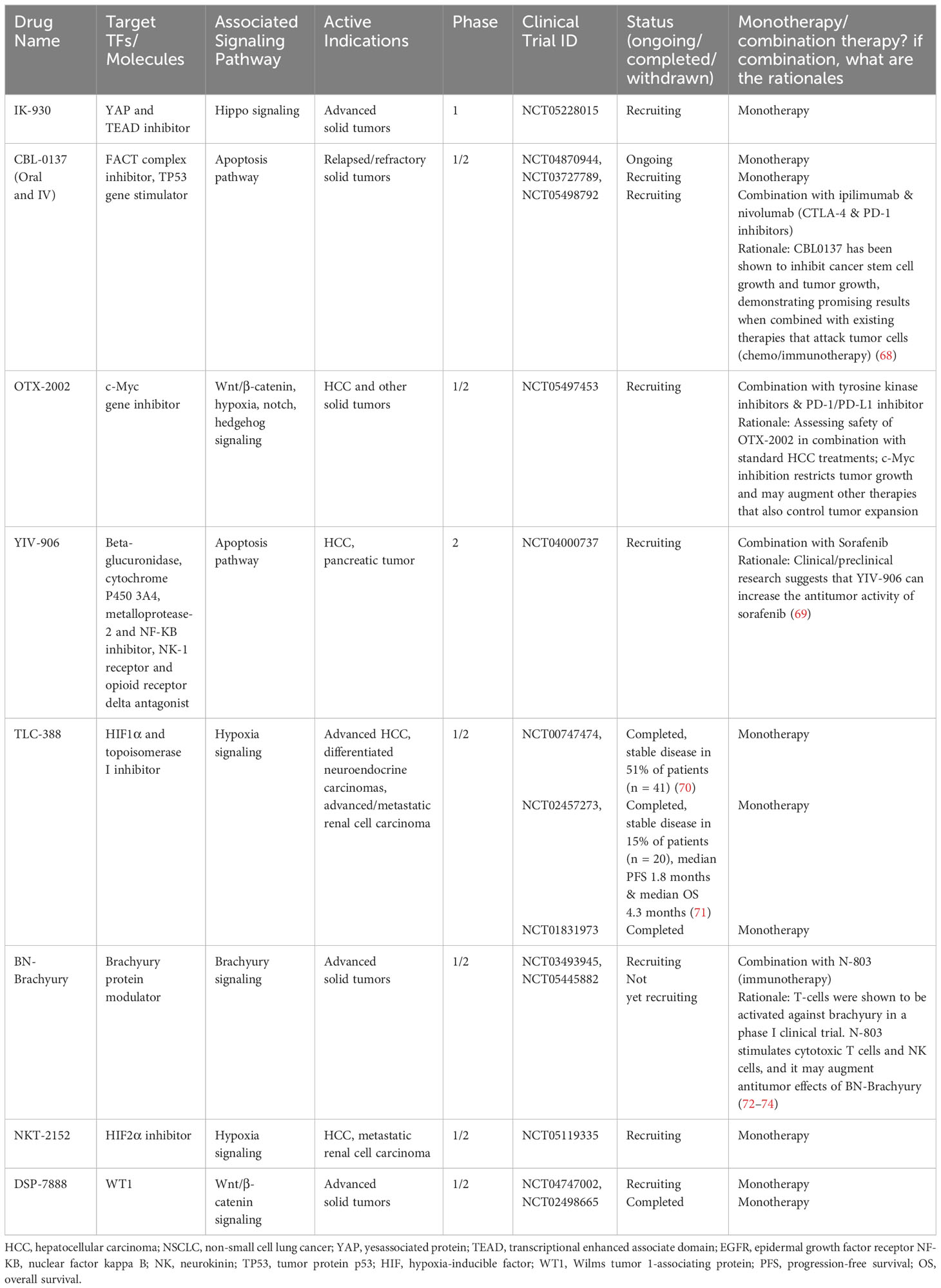
Table 1 Drugs/molecules under clinical trials targeting signaling pathways and/or transcription factors in HCC.
There is substantial effort today in both preclinical and clinical trials assessing the efficacy of interventions targeting the key oncogenic pathways in HCC. Here, we review the pathophysiology of several well-characterized, dysregulated pathways, with a focus on oncogenic TFs (Figure 2). TFs, critical for cancer development and survival, historically have been viewed to be “undruggable”. However, recent breakthroughs and successes in targeting TFs are among the most exciting new frontiers for development of novel cancer drugs. There are a total of 9 TF-targeting HCC drugs in phase 1-3 development, spanning 8 different targets (Figure 3, Table 1).
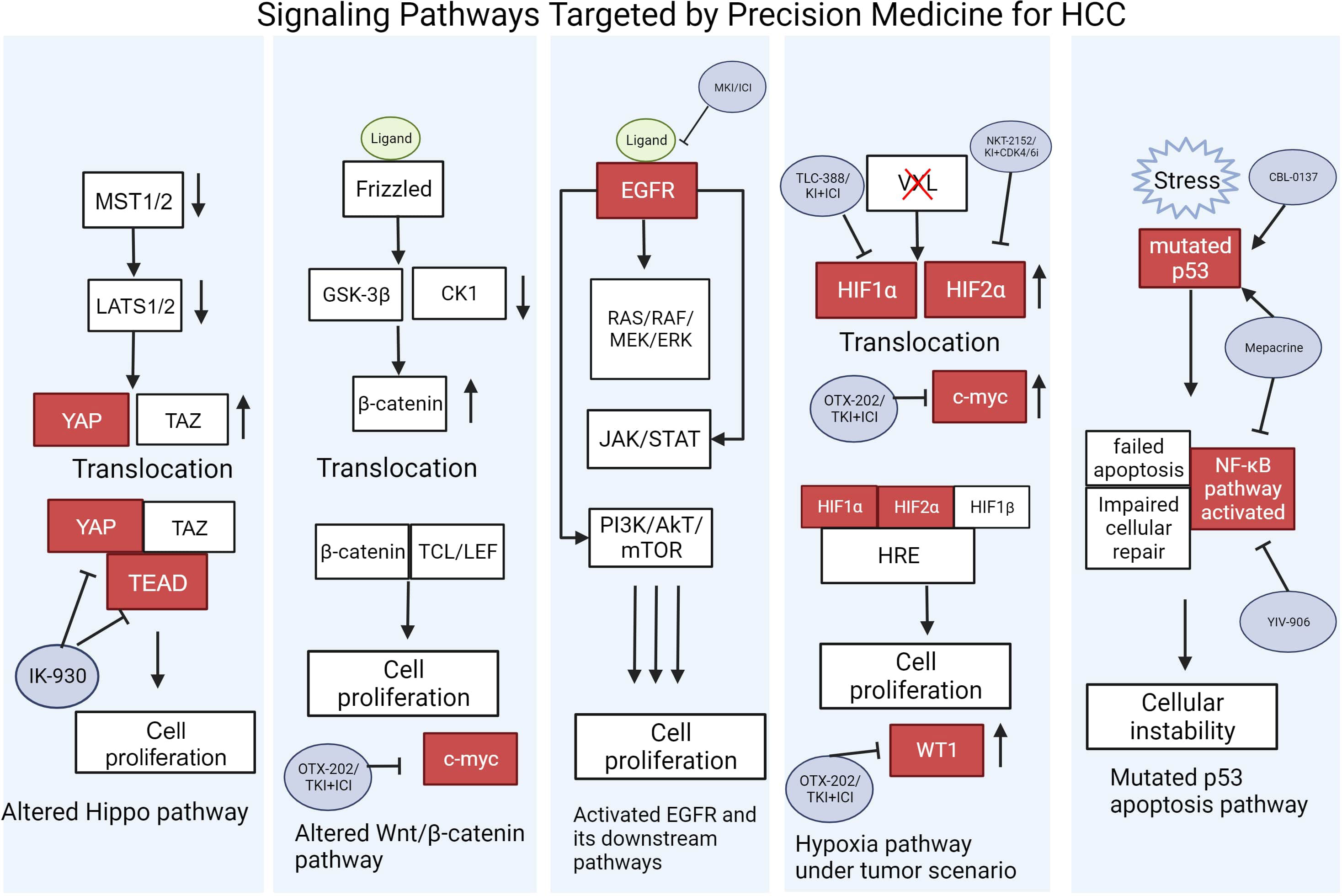
Figure 2 Signaling Pathways Targeted by Precision Medicine for HCC. Pathways are shown in their altered state caused by ligand signaling, stress, or gene mutations that lead to eventual cell proliferation. Gene activation and repression are illustrated with up or down arrows. TFs targeted by potential drugs under clinical trials are shown in red. Refer to Table 1 for the full descriptions of the drugs used to target these oncoproteins. This figure was created with BioRender.com.
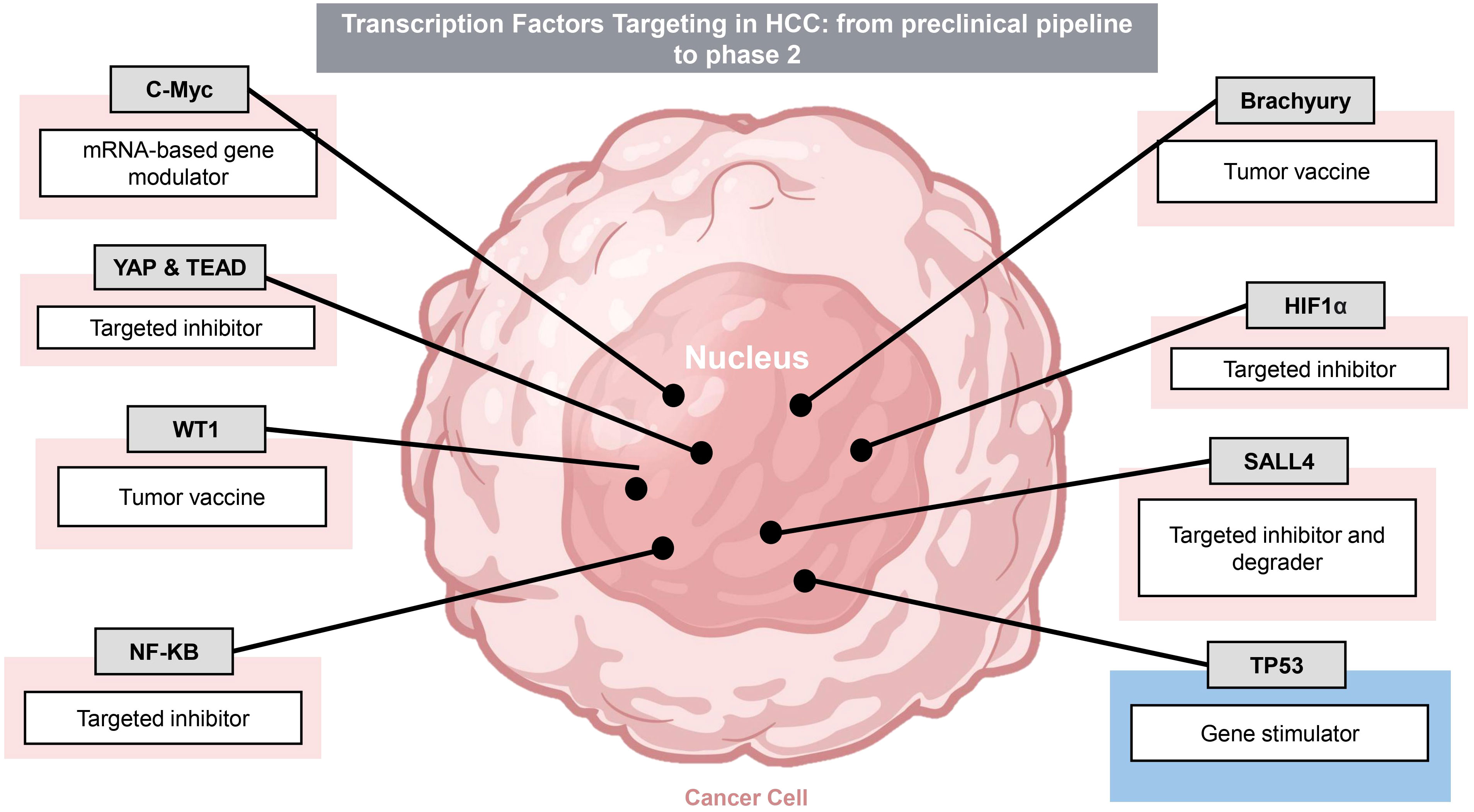
Figure 3 Transcription Factor targets in HCC. There are multiple drug candidates targeting transcription factor oncoproteins and tumor suppressor under pre-clinical and clinical trial development. Transcription factors and targeting modalities are summarized. Pink shading indicates oncoproteins whereas blue indicates tumor suppressors.
3.1 Hippo signaling
The Hippo pathway consists of a kinase cascade involving the mammalian Ste20-like kinases 1/2 (MST1/2; homologs of Drosophila Hippo), which controls cell growth by phosphorylating the large tumor suppressor 1/2 (LATS1/2; homologs of Drosophila Warts). The cascade leads to the inhibition of two transcriptional coactivators: the Yes-associated protein (YAP) and transcriptional co-activator with PDZ-binding motif (TAZ) (75). By interacting with α-catenin and the 14-3-3 protein, LATS1/2 subsequently induces the phosphorylation of YAP and TAZ, resulting in their degradation by Casein kinase 1δ/ϵ and SCF E3 ubiquitin ligase or cytoplasmic sequestration by the 14-3-3 protein (75, 76). In the absence of Hippo signaling, YAP and TAZ translocate into the nucleus to interact with the transcriptional enhanced associate domain (TEAD) family. The activation of TEAD induces the expression of genes related to cell growth, survival, migration, anti-apoptosis and epithelial-mesenchymal transition (EMT) (76, 77).
In the context of HCC, the overexpression of YAP and TAZ was significantly associated with the increased proliferative activity of tumor cells. In HCC cells and human HCC samples, TAZ was expressed at higher levels than YAP with TAZ mRNA levels having a positive association with poor outcomes. While TAZ knockdown can attenuate cell growth by inhibiting the PI3K/AkT/mTOR signaling pathway, it additionally leads to a compensatory YAP upregulation and expression of CD90, a HCC-specific tumor stem cell marker. Moreover, continuous 5-fluorouracil treatment was shown to reduce TAZ expression and induce YAP and CD90 mRNA expression to confer HCC cells chemoresistance. These findings suggest that targeting both YAP and TAZ or its downstream target TEAD may be crucial to suppress HCC oncogenesis mediated by the Hippo pathway (78).
IK-930 is a small molecule inhibitor of TEAD currently in phase 1 clinical trial to evaluate its safety, efficacy, and tolerability in patients with advanced solid tumors (79). Besides binding to TEAD, IK-930 promotes interactions between TEAD and vestigial like family member 4 (VGLL4), a negative regulator of the Hippo signaling pathway, to inhibit the transcriptional activity of TEAD (80, 81). IK-930 demonstrated selectivity for Hippo-mutated cells and potent TEAD inhibition in vitro as well as a robust antitumor activity in preclinical models of mesothelioma and head and neck squamous cell carcinoma with mutations in the Hippo pathway (82). Throughout the phases of its clinical trial, it remains to be seen whether IK-930 can serve as a powerful addition to the arsenal of treatments for Hippo-mutated HCCs.
3.2 Wnt/β-catenin signaling
In normal adult tissues, the wnt/β-catenin signaling pathway is inactive and regulated by wnt antagonists and the β-catenin destruction complex. The binding of wnt ligands to one of the frizzled receptors and one of the low-density lipoprotein receptor-related protein (LRP) 5/6 co-receptors activates the pathway, recruiting Disheveled (Dsh) and AXIN scaffolding proteins to the membrane (83, 84). The subsequent degradation of AXIN leads to the stabilization of β-catenin by disassembling its degradation complex, enabling β-catenin accumulation in the cytoplasm and its translocation into the nucleus (85). Inside the nucleus, β-catenin binds to TFs belonging to the T-cell factor/lymphoid enhancer-binding factor to induce the expression of downstream targets controlling liver cell proliferation, differentiation, and development (83, 84).
40-70% of HCCs exhibit β-catenin accumulation in the nucleus due to mutations in the β-catenin gene allowing β-catenin to avoid degradation (84). Such mutations have been found more frequently in cells with loss of function mutations in the Wilms tumor 1 (WT1) gene, an antagonist and negative regulator of the wnt/β-catenin pathway (86). The binding partner to the WT1 protein, Wilms’ tumor 1-associating protein (WTAP), has been suggested to have an oncogenic role in many tumor types. In HCC, the overexpression of WTAP has been correlated to poor outcomes by the post-transcriptional suppression of ETS proto-oncogene 1 (ETS1), a tumor suppressor (87). In the context of colon cancer, targeting and degrading Wilms’ tumor 1-associating protein (WTAP) with carbonic anhydrase IV successfully restored free WT1 proteins capable of inhibiting the Wnt/β-catenin pathway, demonstrating the potential benefits of WTAP-targeting therapies (88). In addition, downstream targets of the wnt/β-catenin signaling pathway responsible for HCC proliferation and metastasis, such as c-Myc and leucine-rich repeat-containing G (LGR5), respectively, may be considered as potential targets for therapy (84).
3.3 Epidermal growth factor receptor signaling
EGFR is a transmembrane tyrosine kinase receptor that binds to ligands such as the epidermal growth factor (EGF) and transforming growth factor α (TGF-α). Upon its activation, it induces downstream pathways involved in cell proliferation and differentiation, such as the Ras-Raf-MEK-ERK pathway and the JAK/STAT pathway. In addition, it can activate the PI3K/Akt/mTOR pathway responsible for controlling cell survival (89). With regards to HCC, EGFR overexpression is observed in 68% of HCCs and is positively correlated to poor outcomes (90, 91). Increased EGFR expression has been shown to enhance the microvessel density of tumors and further promote their proliferation (92). Similarly, the overexpression of EGFR ligands such as EGF and TGF-α was confirmed by multiple studies using human HCC specimens (92–94). The expression of betacellulin, which belongs to the EGF family, in HCC cells has been shown to have a significant positive correlation to EGFR expression by tumor endothelial cells, suggesting a potential paracrine signaling pathway to induce tumor angiogenesis (92).
Recently, Jin et al. reported that EGFR depletion increased the antitumoral activity of lenvatinib towards HCC cells using a CRISPR/Cas9-based synthetic lethality screening. The combination of MKI, lenvatinib and the EGFR inhibitor gefitinib exhibited robust anti-proliferative effects in liver cancer cell lines as well as in murine preclinical models xenografted with human liver tumors. The two treatments additionally increased the infiltration of natural killer (NK) cells and CD8+ cytotoxic T cells into the tumor microenvironment, suggesting that ICI in combination with lenvatinib and gefitinib may lead to a more potent clinical response (95). In a phase I clinical study on 12 HCC patients, 4 patients demonstrated a partial response, 4 exhibited stable disease states, and 4 had disease progression after 4 to 8 weeks of the combination treatment (96).
3.4 Hypoxia signaling
Solid tumors in HCC generate hypoxic conditions within the tumor microenvironment due to poor vascularization and increased metabolism. The hypoxic environment prevents the degradation of hypoxia inducible factor (HIF) α subunits by reducing the activity of prolyl hydroxylase domain-containing proteins (97). Increased expressions of HIF1α and HIF2α have been observed in multiple tumor types; however, the TFs have differing roles across distinct cell types. For example, the loss of HIF2α in a KRAS-driven lung tumor model surprisingly increased tumor progression, while HIF2α deficiency in mouse vascular endothelial cells reduced tumor expansion. HIF1α and HIF2α additionally exhibit differential activities, sometimes demonstrating opposite effects with regards to cancer progression (98).
In normal cells, hypoxia arrests cell growth through HIF1α, which induces c-Myc degradation to decrease anabolic metabolism and protein synthesis. In contrast, transformed cells exclusively expressing HIF2α enhance c-Myc activity under hypoxic conditions, promoting cell growth and proliferation (99). Elevated c-Myc expression is additionally capable of overriding HIF1α-mediated inhibition, where HIF1α and c-Myc together increase cell proliferation (98, 100). Moreover, c-Myc can post-transcriptionally promote HIF1α expression and further promote tumor growth (101). In HCC, the overexpression of both HIF1α and HIF2α have been observed and associated with poor prognosis (97). A study by Mu et al. demonstrated that HIF2α, but not HIF1α, was positively correlated to both c-Myc expression and the Union for International Cancer Control tumor stages in human HCC tissues. Knockdown of HIF2α successfully inhibited c-Myc expression and restricted HCC growth, elucidating it as a potential target for therapy (102).
TLC388 is a combination of HIF1α and nuclear DNA topoisomerase 1 (TOP1) inhibitors that underwent a phase I clinical trial on 54 patients with advanced solid tumors from 2008 to 2011 (103). In the study, 13 patients did not exhibit any clinical response, while among 41 evaluable patients, 21 exhibited stable disease. Overall, TLC388 was well-tolerated and demonstrated an ability to prolong a stable disease state in multiple tumor types (70). While the study did not progress onto later phases, it is much desired to evaluate the effects of TLC388 in combination with other therapeutic agents, such as EGFR inhibitors or ICI, for HCC treatment. Similarly, NKT2152 is an orally administered HIF2α inhibitor demonstrating potent reduction in tumor growth in murine preclinical models of clear cell renal cell carcinoma (ccRCC) and other solid tumors including HCC. A combination of NKT2152 and VEGFR or cyclin-dependent kinase (CDK) 4/6 inhibitor increased antitumoral effects (104). While NKT2152 is currently undergoing a phase II clinical trial in patients with ccRCC, it remains to be seen whether combination of NKT2152 and kinase inhibitors can elicit robust clinical response in HCC patients (105).
Targeting a downstream target of HIF, OTX-2002 is a mRNA therapeutic that pre-transcriptionally modulates c-Myc gene expression. It demonstrated an ability to downregulate c-Myc expression in HCC cells in vitro and elicited potent antitumoral activities in murine HCC xenograft models (106). OTX-2002 is currently undergoing a phase I/II clinical trial in patients with HCC or other solid tumors with c-Myc involvement to determine its antitumoral activity alone or in combination with other treatments such as tyrosine kinase inhibitors (TKI) or ICI (107, 108).
3.5 TP53 dependent pathways
In HCC, TP53, a critical tumor suppressor gene, plays a multifaceted role. TP53’s primary function is to monitor and maintain genomic stability by preventing the growth of cells with damaged DNA. In HCC, TP53 mutations or dysregulation are frequent, contributing to the initiation and progression of liver cancer (109). As a transcription factor, TP53 regulates the expression of target genes that can promote cell cycle arrest, apoptosis, DNA repair, among other oncogenic processes (110). This loss of TP53 function in HCC not only promotes tumor growth but also makes cancer cells more resistant to therapy, rendering it a pivotal factor in the development and treatment of HCC (109). Multiple efforts have been devoted to directly or indirectly target the TP53 dependent pathways in HCC, to restore or potentiate the tumor suppressor function of TP53 (110). Candidates in the clinical trial pipeline are summarized in Table 1.
3.6 WT1
Despite its tumor-suppressive activities, elevated WT1 expression has been discovered in tumors derived from epithelial, mesenchymal, hematopoietic, and neuronal tissues, suggesting its paradoxical oncogenic function (111). In fact, in hypoxic conditions, HIF can activate WT1 in vascular cells to promote angiogenesis at the tumor site. Silencing WT1 expression decreased the expression of the TF ETS-1 in addition to reducing endothelial cell migration, proliferation, and vascular formation (112). In HCC, the downregulation of WT1 by the antisense of WT1, a long non-coding RNA, was shown to promote apoptosis and restored sensitivity to chemotherapy (113). Thus, targeting WT1 can serve as a promising approach to restrict tumor growth by inhibiting angiogenesis and chemoresistance. DSP-7888 (Ombipepimut-S) is a cancer vaccine composed of synthetic epitopes nelatimotide and adegramotide. Nelatimotide contains two CD8+ cytotoxic T cell epitopes of WT1, while adegramotide contains a targeting sequence for the endogenous WT1 protein. By stimulating WT1-specific cytotoxic and helper T cells, DSP-7888 in combination with anti-programmed cell death protein 1 (PD-1) demonstrated its ability to elicit strong antitumoral activity toward cancer cells expressing high levels of WT1 during in vitro and in vivo preclinical trials. For a robust immune response, a combination therapy with ICI may be the key to prevent the reduced activity of immune cells at the tumor microenvironment (114). In combination with ICI, DSP-7888 has completed multiple phase I clinical trials, including those on patients with advanced solid tumors. Currently, DSP-7888 is undergoing a phase II clinical trial for acute myeloid leukemia patients with complete remission (115).With regards to targeting the wnt/β-catenin pathway in HCC, a multidimensional approach may be required due to its complexity. It is implicated that β-catenin may have multiple roles in HCC progression than its transcriptional regulation; in fact, β-catenin nuclear accumulation was found to be restricted to late-stage HCC. In early stage HCC cells, increased expression of E-cadherin leads to β-catenin recruitment to the plasma membrane by the AJ complex, a complex previously known to regulate intercellular interactions and maintain the F-actin cytoskeleton. At the membrane, β-catenin and the AJ complex promotes EGFR stabilization and signaling, which allows tumor survival (116). Moreover, murine models have showcased an increase in macrophages and a decrease in CD4+ helper T cells as the mice progressed from steatosis to cancer. The isolated macrophages exhibited high expression of wnt ligands to activate the wnt/β-catenin pathway to induce tumorigenesis during steatosis (117).
3.7 Brachyury
Brachyury is a T-box TF involved during vertebrate development and frequently dysregulated in neoplastic diseases such as colorectal cancer (118, 119). In a study on oral squamous cell carcinoma cells, Brachyury expression was found to be correlated with EMT and lymph node metastasis, and increased Brachyury expression was discovered in human HCC cell lines (120, 121). Brachyury moreover increases the invasiveness and metastatic potential of HCC cells and promotes EMT in HCC cells through the Akt/Snail pathway (121). Current therapies targeting the Brachyury TF include the BN-brachyury cancer vaccine, which employs a modified vaccinia Ankara (MVA) virus to elicit a robust immune response toward solid tumor cells overexpressing the Brachyury protein (72). Silencing Brachyury expression has demonstrated an ability to reduce the invasiveness of non-small cell lung carcinoma cells in vitro and in vivo using xenograft mice (122). A phase I trial of the MVA-BN-brachyury cancer vaccine on 13 patients with advanced cancers yielded one patient with a partial response, 4 with stable disease states, and 8 with progressive disease. While meaningful T cell activity was observed in these patients, it remains to be seen whether the potency of the response increases in combination with ICI or if prior immunotherapy treatment is necessary for a response (72). Currently, the MVA-BN-brachyury cancer vaccine in combination with anti-PD-L1 ICI is undergoing a phase I/II study on patients with advanced solid tumors (123).
3.8 SALL4
Another promising TF target is SALL4, a TF involved in cell renewal and growth during development (124). While SALL4 is silenced in most adult tissues, its reactivation can lead to malignant neoplasms (125). SALL4 acts downstream of multiple signal transduction pathways, including JAK/STAT and Wnt/β-catenin (126, 127). Upon its activation, SALL4 acts on multiple signaling pathways involved in cell growth, such as PI3K/Akt/mTOR, lysine demethylase 3A (KDM3A), Forkhead-Box (FOX), and c-Myc genes, the latter which it binds directly to the promoter to elevate its expression (128–130). SALL4 therapeutic targeting in HCC is currently in the pre-clinical development stage. Restricting the interaction between SALL4 and Nucleosome Remodeling and Deacetylase complex (NuRD), a corepressor, with a peptide competitive inhibitor reduced the viability of SALL4-overexpressing HCC cells in vitro and in vivo (40, 131). A number of other SALL4-targeting modalities, such as small molecule degraders, are also under active pre-clinical development. SALL4-based precision medicine relies on companion diagnostics to successfully select HCC patients who express high levels of SALL4; efforts are under way to develop an ultrasensitive peripheral blood protein assay to be used in conjunction with SALL4-targeting therapies.
4 Currently approved and ongoing clinical trials of immunotherapy and targeted therapy combinations
The utilization of combination therapies in HCC has gained significant traction in recent years. Until 2017, the utilization of antiangiogenic MKIs was a common approach in systemic therapy for advanced-stage HCC patients. These MKIs target angiogenesis pathways and aim to disrupt tumor vasculature, thereby inhibiting tumor growth and progression. However, the introduction of immunotherapy with ICI, such as PD1/PDL1 inhibitors, has provided an additional therapeutic avenue. ICI stimulates effective anti-tumor activity by unleashing the immune system’s ability to recognize and attack cancer cells. The ICIs have demonstrated efficacy in the clinical management of HCC (132). The simultaneous targeting of VEGF-mediated angiogenesis and immune checkpoints hold promise for improving treatment outcomes in HCC (133). The success of these earlier generations of combination therapies are paving ways for the ongoing development of novel combination approaches. In fact, many of the TF/signaling pathway-targeting modalities (e.g. cMYC targeting) and biomarker guided monoclonal antibody/cell therapy approaches (e.g. anti-GPC3, or GPC3 CAR-T) center around combination therapy in the pre-clinical and clinical development stages, mostly in combination with existing first line therapies.
In this section, we explore some well-known examples of combination therapies, to highlight a few core themes and considerations - 1) the importance of mechanism-driven rationale design, 2) consideration of drug sequencing, 3) need for robust companion biomarkers, and 4) optimization safety profile - to guide development of future combination therapies in light of the many exciting, emerging HCC novel targets. This section will focus on combination medical therapies alone, but it is important to note that combining medical therapies (e.g. ICIs) with locoregional therapies, such as transarterial chemoembolization (TACE) or ablation, has been found to be well-tolerated and potentially efficacious in certain subsets of HCC patients (134) (135). The discussion of combination surgical and medical therapies are beyond the scope of this review.
4.1 In-depth mechanistic investigation inspired rational design of dual VEGF-targeting and ICI
Animal models have been instrumental in studying the effects of VEGF/VEGFR pathway blockade in combination with PD1 inhibitors leading to its clinical development, highlighting the importance of in-depth mechanistic investigation in guiding the rationale design of novel combination approaches (136). Studies in animal models have provided insights into the specific changes that occur in the TME following the dual blockade of VEGFR and PD1. These changes include a reduction in tumor vasculature, a decrease in myeloid-derived suppressor cells (MDSCs), an increase in M1-like tumor-associated macrophages (TAMs) and infiltrating CD8+ cells, and a decrease in M2-like TAM levels. These findings highlight the complex interactions between immune cells, tumor cells, and the vasculature in the context of combination therapy (136).
Backed by strong pre-clinical rationale, the combination of the anti-PDL1 antibody atezolizumab and the VEGF-neutralizing antibody bevacizumab has emerged as a first-line therapy for HCC. Its approval by the FDA in 2020 marked a significant milestone in HCC treatment. In the clinical trial setting, it has demonstrated superior efficacy over sorafenib in the frontline setting for advanced HCC (132).
Other HCC pathogenic drivers/pathways have been shown to interact with the immune checkpoint pathway, and a deeper mechanistic understanding of their interplay could guide rational design of novel combination therapies. For example, recent studies have implicated SALL4 in the regulation of PD-1/PD-L1-mediated T cell exhaustion in HCC (137, 138). Another study found that SALL4-mediated upregulation of exosomal miR-146a-5p drives T-cell exhaustion by M2 tumor-associated macrophages in HCC (30). We anticipate more innovative therapies in the near future that harness synergistic anti-tumor mechanisms between HCC oncogenic pathways and ICIs.
4.2 Treatment sequence in the setting of combination therapies
Sorafenib, a MKI targeting both VEGFRs and PDGFR-b, was the first targeted therapy approved for advanced HCC. Other MKIs, including regorafenib and lenvatinib, have also shown potential in improving survival outcomes for HCC patients. Combining MKIs and ICIs recently became a popular combination therapy strategy in HCC, but clinicians often face uncertainties in using this combination. Rational design involves understanding of how therapy efficacy can be affected by sequencing, among others.
Several clinical trials have investigated or are investigating this combination using different treatment sequencing. A propensity score-matching study comparing simultaneous administration of anti-PD-1 combined with sorafenib versus anti-PD-1 alone in advanced HCC showed that the combination therapy had better efficacy and survival benefits (139). The combination therapy exhibited a higher complete response rate, overall response rate, disease control rate, and achieved more tumor shrinkage compared to anti-PD-1 alone. Additionally, the combination therapy demonstrated longer progression-free survival and a decreasing risk of disease progression and death. There were no statistically significant differences in grade 3/4 toxicities between the two treatment options, except for one case of ‘sick sinus syndrome’ that developed with combination therapy (14). Sequential therapies have also been explored with anti-PD1 antibodies, such as nivolumab and pembrolizumab, being used after MKI in the treatment of HCC. This is still being explored in the clinical trial setting (140), but it is being used empirically in the clinical setting (141). Rational design of these two combinations, whether administered sequentially or simultaneously, is an important consideration for optimizing treatment outcomes.
4.3 Combination therapy approaches involving multiple ICIs
Simultaneous administration of multiple sub-classes of ICIs, such as the combination of CTLA4 and PD-L1 blockade, have been explored for HCC treatment. Preclinical studies and clinical trials have demonstrated synergistic effects when both pathways are targeted simultaneously, leading to enhanced anti-tumor immune responses (142). The rational design of this combination involves understanding the complex interplay between CTLA4 and PD-L1 signaling. The use of multiple ICIs are limited due to various reasons, but notably the current lack of robust biomarkers for patient selection. Tumor mutation burden (TMB) and microsatellite instability (MSI) have been used as a biomarker for predicting ICI therapy efficacy, but no single biomarker has been established as a reliable predictor of response to immunotherapy in HCC (143).
Additional exploratory biomarkers in tissue analysis include tumor gene expression profiling (GEP), multiplex immunohistochemistry (IHC), immunofluorescence (IF), tumor infiltrating lymphocytes (TILs), immunoscore, T cell receptor (TCR) diversity, and microbiome (144, 145) New assays such as macroH2A1 staining and stemness-associated genes are being explored to identify patients likely to respond to ICIs (146, 147). Many ongoing clinical trials involving ICIs also have concurrent exploratory companion biomarker designs, so it is likely that in the near future, more biomarkers will be validated to help strategy patients who may benefit from different types of immune-check point inhibition, or their combinations.
4.4 Rational design of combination therapies: patient safety considerations
Rationale design of therapy combination should also take into account patient-specific factors, contraindications, and potential risks associated with each therapy alone or in combination. For example, combining ICIs and TKIs can lead to more severe toxicity. Skin lesions, diarrhea, and hepatitis are some examples of adverse events associated with this combination that can be more severe than single therapy alone (148). Lethal adverse events have been reported with the combination of pembrolizumab and lenvatinib in HCC patients. The potential for severe adverse events highlights the need for careful risk-benefit assessment and close monitoring during treatment. Patient-reported outcomes and quality-of-life assessments should be considered alongside efficacy data when designing and evaluating the overall impact of combination therapies (149).
The VEGF inhibitor Bevacizumab has been associated with an increased risk of gastrointestinal hemorrhage or perforation in patients with advanced cancer. Notably, there was no difference in the occurrence of these adverse events between cirrhosis patients and cancer patients, indicating that the risk is not limited to specific subgroups (150). Considering the pathophysiology of portal hypertension, VEGF inhibition may not aggravate portal pressure and should be evaluated in the context of individual patient characteristics. Comparing different combination approaches, the combination of atezolizumab and bevacizumab has shown better tolerability compared to the combination of ICIs and TKIs. This finding suggests that certain combinations may have distinct toxicity profiles, and rational design should consider the potential impact on patients’ well-being (139).
Toxicities associated with combination therapies may not be entirely discouraging. In fact, they might indicate an increased chance of treatment benefit. For instance, skin toxicity associated with sorafenib has been associated with improved outcomes in HCC patients (151). This suggests that a comprehensive understanding of toxicity patterns and their relationship to treatment response is important for rational decision-making. In a single-center cohort study of HCC patients, the presence of immune-mediated adverse events (IMAEs) was independently associated with improved median progression-free survival (PFS) and overall survival. This finding suggests that the occurrence of IMAEs may serve as a positive prognostic factor and warrants further investigation (152).
5 Conclusion
The field of HCC treatment is rapidly evolving, with a focus on combination therapies that target multiple pathways and mechanisms. The rational design of these combination therapies involves understanding the complex interactions between different agents, considering their distinct toxicity profiles, and identifying patient-specific factors to guide treatment decisions. Targeted therapies, such as those targeting specific signaling pathways and TF oncogenes, can be developed based on a better understanding of the molecular and genetic alterations that drive HCC progression and disease heterogeneity.
The future scope of HCC research and treatment lies in the development of more effective targeted therapies and personalized treatment strategies, preferably guided by biomarkers. The ongoing effort to identify and validate novel biomarkers for HCC diagnosis, prognosis, and treatment response will allow for the selection of appropriate therapies for individual patients as the treatment options expand. Overall, The integration of biomarker-guided precision medicine and the exploration of targeted therapies and combination approaches are expected to shape the future landscape of HCC treatment and pave the way for more personalized and effective treatment strategies.
Author contributions
JL: Conceptualization, Writing – original draft, Writing – review & editing. KP: Writing – original draft. ZS: Writing – review & editing. HL: Writing – original draft, Validation. PG: Writing – original draft, Writing – review & editing. MP: Writing – original draft. LC: Conceptualization, Funding acquisition, Writing – original draft, Writing – review & editing.
Funding
The author(s) declare financial support was received for the research, authorship, and/or publication of this article. This work was supported by AGA/Jenzabar research fund and NIH/NHLBI P01HL158688 (LC).
Acknowledgments
The authors acknowledge BioRender (www.biorender.com), which was used to create the figures in this review.
Conflict of interest
The authors declare that the research was conducted in the absence of any commercial or financial relationships that could be construed as a potential conflict of interest.
Publisher’s note
All claims expressed in this article are solely those of the authors and do not necessarily represent those of their affiliated organizations, or those of the publisher, the editors and the reviewers. Any product that may be evaluated in this article, or claim that may be made by its manufacturer, is not guaranteed or endorsed by the publisher.
References
1. Reig M, Forner A, Rimola J, Ferrer-Fàbrega J, Burrel M, Garcia-Criado Á, et al. BCLC strategy for prognosis prediction and treatment recommendation: The 2022 update. J Hepatol (2022) 76:681–93. doi: 10.1016/j.jhep.2021.11.018
2. Matsumoto MM, Mouli S, Saxena P, Gabr A, Riaz A, Kulik L, et al. Comparing real world, personalized, multidisciplinary tumor board recommendations with BCLC algorithm: 321-patient analysis. Cardiovasc Intervent Radiol (2021) 44:1070–80. doi: 10.1007/s00270-021-02810-8
3. Crissien AM, Frenette C. Current management of hepatocellular carcinoma. Gastroenterol Hepatol (2014) 10:153–61.
4. Fitzmorris P, Shoreibah M, Anand BS, Singal AK. Management of hepatocellular carcinoma. J Cancer Res Clin Oncol (2015) 141:861–76. doi: 10.1007/s00432-014-1806-0
5. Ghouri YA, Mian I, Rowe JH. Review of hepatocellular carcinoma: Epidemiology, etiology, and carcinogenesis. J Carcinog (2017) 16:1. doi: 10.4103/jcar.JCar_9_16
6. Sagnelli E, Macera M, Russo A, Coppola N, Sagnelli C. Epidemiological and etiological variations in hepatocellular carcinoma. Infection (2020) 48:7–17. doi: 10.1007/s15010-019-01345-y
7. El-Serag HB, Rudolph KL. Hepatocellular carcinoma: epidemiology and molecular carcinogenesis. Gastroenterology (2007) 132:2557–76. doi: 10.1053/j.gastro.2007.04.061
8. Wiemann SU, Satyanarayana A, Tsahuridu M, Tillmann HL, Zender L, Klempnauer J, et al. Hepatocyte telomere shortening and senescence are general markers of human liver cirrhosis. FASEB J (2002) 16:935–42. doi: 10.1096/fj.01-0977com
9. Gisder DM, Tannapfel A, Tischoff I. Histopathology of hepatocellular carcinoma - when and what. Hepatoma Res (2022) 8:4. doi: 10.20517/2394-5079.2021.106
10. Llovet JM, Ricci S, Mazzaferro V, Hilgard P, Gane E, Blanc J-F, et al. Sorafenib in advanced hepatocellular carcinoma. N Engl J Med (2008) 359:378–90. doi: 10.1056/NEJMoa0708857
11. Cheng A-L, Kang Y-K, Chen Z, Tsao C-J, Qin S, Kim JS, et al. Efficacy and safety of sorafenib in patients in the Asia-Pacific region with advanced hepatocellular carcinoma: a phase III randomised, double-blind, placebo-controlled trial. Lancet Oncol (2009) 10:25–34. doi: 10.1016/S1470-2045(08)70285-7
12. Riechelmann RP, Leite LS, Bariani GM, Glasberg J, Rivelli TG, da Fonseca LG, et al. Regorafenib in patients with antiangiogenic-naïve and chemotherapy-refractory advanced colorectal cancer: results from a phase IIb trial. Oncologist (2019) 24:1180–7. doi: 10.1634/theoncologist.2019-0067
13. Finn RS, Ikeda M, Zhu AX, Sung MW, Baron AD, Kudo M, et al. Phase ib study of lenvatinib plus pembrolizumab in patients with unresectable hepatocellular carcinoma. J Clin Oncol (2020) 38:2960–70. doi: 10.1200/JCO.20.00808
14. Finn RS, Qin S, Ikeda M, Galle PR, Ducreux M, Kim T-Y, et al. Atezolizumab plus bevacizumab in unresectable hepatocellular carcinoma. N Engl J Med (2020) 382:1894–905. doi: 10.1056/NEJMoa1915745
15. Cheng A-L, Qin S, Ikeda M, Galle PR, Ducreux M, Kim T-Y, et al. Updated efficacy and safety data from IMbrave150: Atezolizumab plus bevacizumab vs. sorafenib for unresectable hepatocellular carcinoma. J Hepatol (2022) 76:862–73. doi: 10.1016/j.jhep.2021.11.030
16. Kudo M. New treatment paradigm with systemic therapy in intermediate-stage hepatocellular carcinoma. Int J Clin Oncol (2022) 27:1110–9. doi: 10.1007/s10147-022-02166-0
17. Li X, Wang Y, Ye X, Liang P. Locoregional combined with systemic therapies for advanced hepatocellular carcinoma: an inevitable trend of rapid development. Front Mol Biosci (2021) 8:635243. doi: 10.3389/fmolb.2021.635243
18. Sell S. Alpha-fetoprotein, stem cells and cancer: how study of the production of alpha-fetoprotein during chemical hepatocarcinogenesis led to reaffirmation of the stem cell theory of cancer. Tumor Biol (2008) 29:161–80. doi: 10.1159/000143402
19. Frenette CT, Isaacson AJ, Bargellini I, Saab S, Singal AG. A practical guideline for hepatocellular carcinoma screening in patients at risk. Mayo Clin Proc Innov Qual Outcomes (2019) 3:302–10. doi: 10.1016/j.mayocpiqo.2019.04.005
20. Trinchet J-C, Chaffaut C, Bourcier V, Degos F, Henrion J, Fontaine H, et al. Ultrasonographic surveillance of hepatocellular carcinoma in cirrhosis: a randomized trial comparing 3- and 6-month periodicities. Hepatology (2011) 54:1987–97. doi: 10.1002/hep.24545
21. Hanif H, Ali MJ, Susheela AT, Khan IW, Luna-Cuadros MA, Khan MM, et al. Update on the applications and limitations of alpha-fetoprotein for hepatocellular carcinoma. World J Gastroenterol (2022) 28:216–29. doi: 10.3748/wjg.v28.i2.216
22. Singal AG, Llovet JM, Yarchoan M, Mehta N, Heimbach JK, Dawson LA, et al. AASLD Practice Guidance on prevention, diagnosis, and treatment of hepatocellular carcinoma. Hepatology (2023) 78(6):1922–65. doi: 10.1097/HEP.0000000000000466
23. Guan M-C, Zhang S-Y, Ding Q, Li N, Fu T-T, Zhang G-X, et al. The performance of GALAD score for diagnosing hepatocellular carcinoma in patients with chronic liver diseases: A systematic review and meta-analysis. J Clin Med Res (2023) 12:949. doi: 10.3390/jcm12030949
24. Schotten C, Ostertag B, Sowa J-P, Manka P, Bechmann LP, Hilgard G, et al. GALAD score detects early-stage hepatocellular carcinoma in a european cohort of chronic hepatitis B and C patients. Pharmaceuticals (2021) 14:735. doi: 10.3390/ph14080735
25. Toyoda H, Kumada T, Osaki Y, Oka H, Urano F, Kudo M, et al. Staging hepatocellular carcinoma by a novel scoring system (BALAD score) based on serum markers. Clin Gastroenterol Hepatol (2006) 4:1528–36. doi: 10.1016/j.cgh.2006.09.021
26. Wongjarupong N, Negron-Ocasio GM, Mara KC, Prasai K, Abdallah MA, Ahn KS, et al. BALAD and BALAD-2 predict survival of hepatocellular carcinoma patients: a North American cohort study. HPB (2021) 23:762–9. doi: 10.1016/j.hpb.2020.09.014
27. El-Serag HB, Kanwal F, Davila JA, Kramer J, Richardson P. A new laboratory-based algorithm to predict development of hepatocellular carcinoma in patients with hepatitis C and cirrhosis. Gastroenterology (2014) 146:1249–55.e1. doi: 10.1053/j.gastro.2014.01.045
28. Ahn JC, Teng P-C, Chen P-J, Posadas E, Tseng H-R, Lu SC, et al. Detection of circulating tumor cells and their implications as a biomarker for diagnosis, prognostication, and therapeutic monitoring in hepatocellular carcinoma. Hepatology (2021) 73:422–36. doi: 10.1002/hep.31165
29. Yang B, Feng X, Liu H, Tong R, Wu J, Li C, et al. High-metastatic cancer cells derived exosomal miR92a-3p promotes epithelial-mesenchymal transition and metastasis of low-metastatic cancer cells by regulating PTEN/Akt pathway in hepatocellular carcinoma. Oncogene (2020) 39:6529–43. doi: 10.1038/s41388-020-01450-5
30. Yin C, Han Q, Xu D, Zheng B, Zhao X, Zhang J. SALL4-mediated upregulation of exosomal miR-146a-5p drives T-cell exhaustion by M2 tumor-associated macrophages in HCC. Oncoimmunology (2019) 8:1601479. doi: 10.1080/2162402X.2019.1601479
31. Ng CKY, Di Costanzo GG, Tosti N, Paradiso V, Coto-Llerena M, Roscigno G, et al. Genetic profiling using plasma-derived cell-free DNA in therapy-naïve hepatocellular carcinoma patients: a pilot study. Ann Oncol (2018) 29:1286–91. doi: 10.1093/annonc/mdy083
32. Zhao W, Qiu L, Liu H, Xu Y, Zhan M, Zhang W, et al. Circulating tumor DNA as a potential prognostic and predictive biomarker during interventional therapy of unresectable primary liver cancer. J Gastrointest Oncol (2020) 11:1065–77. doi: 10.21037/jgo-20-409
33. Yamauchi N, Watanabe A, Hishinuma M, Ohashi K-I, Midorikawa Y, Morishita Y, et al. The glypican 3 oncofetal protein is a promising diagnostic marker for hepatocellular carcinoma. Mod Pathol (2005) 18:1591–8. doi: 10.1038/modpathol.3800436
34. Ayoub WS, Steggerda J, Yang JD, Kuo A, Sundaram V, Lu SC. Current status of hepatocellular carcinoma detection: screening strategies and novel biomarkers. Ther Adv Med Oncol (2019) 11:1758835919869120. doi: 10.1177/1758835919869120
35. Jia X, Liu J, Gao Y, Huang Y, Du Z. Diagnosis accuracy of serum glypican-3 in patients with hepatocellular carcinoma: a systematic review with meta-analysis. Arch Med Res (2014) 45:580–8. doi: 10.1016/j.arcmed.2014.11.002
36. Chen Y, Qin Y, Wu Y, Wei H, Wei Y, Zhang Z, et al. Preoperative prediction of glypican-3 positive expression in solitary hepatocellular carcinoma on gadoxetate-disodium enhanced magnetic resonance imaging. Front Immunol (2022) 13:973153. doi: 10.3389/fimmu.2022.973153
37. Zhang J, Zhang M, Ma H, Song X, He L, Ye X, et al. Overexpression of glypican-3 is a predictor of poor prognosis in hepatocellular carcinoma: An updated meta-analysis. Medicine (2018) 97:e11130. doi: 10.1097/MD.0000000000011130
38. Mouhoubi N, Bamba-Funck J, Sutton A, Blaise L, Seror O, Ganne-Carrié N, et al. Sulfatase 2 along with syndecan 1 and glypican 3 serum levels are associated with a prognostic value in patients with alcoholic cirrhosis-related advanced hepatocellular carcinoma. J Hepatocellular Carcinoma (2022) 9:1369. doi: 10.2147/JHC.S382226
39. Han S-X, Wang J-L, Guo X-J, He C-C, Ying X, Ma J-L, et al. Serum SALL4 is a novel prognosis biomarker with tumor recurrence and poor survival of patients in hepatocellular carcinoma. J Immunol Res (2014) 2014:262385. doi: 10.1155/2014/262385
40. Yong KJ, Gao C, Lim JSJ, Yan B, Yang H, Dimitrov T, et al. Oncofetal gene SALL4 in aggressive hepatocellular carcinoma. N Engl J Med (2013) 368:2266–76. doi: 10.1056/NEJMoa1300297
41. Yin F, Han X, Yao S-K, Wang X-L, Yang H-C. Importance of SALL4 in the development and prognosis of hepatocellular carcinoma. World J Gastroenterol (2016) 22:2837–43. doi: 10.3748/wjg.v22.i9.2837
42. Sideras K, Bots SJ, Biermann K, Sprengers D, Polak WG, IJzermans JNM, et al. Tumour antigen expression in hepatocellular carcinoma in a low-endemic western area. Br J Cancer (2015) 112:1911–20. doi: 10.1038/bjc.2015.92
43. Gonzalez-Roibon N, Katz B, Chaux A, Sharma R, Munari E, Faraj SF, et al. Immunohistochemical expression of SALL4 in hepatocellular carcinoma, a potential pitfall in the differential diagnosis of yolk sac tumors. Hum Pathol (2013) 44:1293–9. doi: 10.1016/j.humpath.2012.10.017
44. Liu T-C, Vachharajani N, Chapman WC, Brunt EM. SALL4 immunoreactivity predicts prognosis in Western hepatocellular carcinoma patients but is a rare event: a study of 236 cases. Am J Surg Pathol (2014) 38:966–72. doi: 10.1097/PAS.0000000000000218
45. Xia Q-Y, Liu S, Li F-Q, Huang W-B, Shi L-N, Zhou X-J. Sperm protein 17, MAGE-C1 and NY-ESO-1 in hepatocellular carcinoma: expression frequency and their correlation with clinical parameters. Int J Clin Exp Pathol (2013) 6:1610–6. doi: 10.1016/j.jamcollsurg.2013.07.062
46. Chen Z, Wu H, Jiang S, Liu X, Luo M, Yuan Y. Serum SALL4 as a predictive biomarker for the prognosis of patients with hepatocellular carcinoma who underwent nonsurgical treatment. Medicine (2022) 101:e31200. doi: 10.1097/MD.0000000000031200
47. Shirakawa H, Suzuki H, Shimomura M, Kojima M, Gotohda N, Takahashi S, et al. Glypican-3 expression is correlated with poor prognosis in hepatocellular carcinoma. Cancer Sci (2009) 100:1403–7. doi: 10.1111/j.1349-7006.2009.01206.x
48. Yan B, Wei J-J, Qian Y-M, Zhao X-L, Zhang W-W, Xu A-M, et al. Expression and clinicopathologic significance of glypican 3 in hepatocellular carcinoma. Ann Diagn Pathol (2011) 15:162–9. doi: 10.1016/j.anndiagpath.2010.10.004
49. Yorita K, Takahashi N, Takai H, Kato A, Suzuki M, Ishiguro T, et al. Prognostic significance of circumferential cell surface immunoreactivity of glypican-3 in hepatocellular carcinoma. Liver Int (2011) 31:120–31. doi: 10.1111/j.1478-3231.2010.02359.x
50. Liang J, Ding T, Guo Z-W, Yu X-J, Hu Y-Z, Zheng L, et al. Expression pattern of tumour-associated antigens in hepatocellular carcinoma: association with immune infiltration and disease progression. Br J Cancer (2013) 109:1031–9. doi: 10.1038/bjc.2013.390
51. Riener M-O, Wild PJ, Soll C, Knuth A, Jin B, Jungbluth A, et al. Frequent expression of the novel cancer testis antigen MAGE-C2/CT-10 in hepatocellular carcinoma. Int J Cancer (2009) 124:352–7. doi: 10.1002/ijc.23966
52. Fan H, Cui Z, Zhang H, Mani SK, Diab A, Lefrancois L, et al. DNA demethylation induces SALL4 gene re-expression in subgroups of hepatocellular carcinoma associated with Hepatitis B or C virus infection. Oncogene (2017) 36:2435–45. doi: 10.1038/onc.2016.399
53. Shibahara J, Ando S, Hayashi A, Sakamoto Y, Hesegawa K, Kokudo N, et al. Clinicopathologic characteristics of SALL4-immunopositive hepatocellular carcinoma. Springerplus (2014) 3:721. doi: 10.1186/2193-1801-3-721
54. Tanaka Y, Aishima S, Kohashi K, Okumura Y, Wang H, Hida T, et al. Spalt-like transcription factor 4 immunopositivity is associated with epithelial cell adhesion molecule expression in combined hepatocellular carcinoma and cholangiocarcinoma. Histopathology (2016) 68:693–701. doi: 10.1111/his.12806
55. Xing M, Wang X, Kiken RA, He L, Zhang J-Y. Immunodiagnostic biomarkers for hepatocellular carcinoma (HCC): the first step in detection and treatment. Int J Mol Sci (2021) 22:6139. doi: 10.3390/ijms22116139
56. Zheng X, Liu X, Lei Y, Wang G, Liu M. Glypican-3: A novel and promising target for the treatment of hepatocellular carcinoma. Front Oncol (2022) 12:824208. doi: 10.3389/fonc.2022.824208
57. Roddy H, Meyer T, Roddie C. Novel cellular therapies for hepatocellular carcinoma. Cancers (2022) 14:504. doi: 10.3390/cancers14030504
58. Jogalekar MP, Rajendran RL, Khan F, Dmello C, Gangadaran P, Ahn B-C. CAR T-Cell-Based gene therapy for cancers: new perspectives, challenges, and clinical developments. Front Immunol (2022) 13:925985. doi: 10.3389/fimmu.2022.925985
59. Liu H, Xu Y, Xiang J, Long L, Green S, Yang Z, et al. Targeting alpha-fetoprotein (AFP)-MHC complex with CAR T-cell therapy for liver cancer. Clin Cancer Res (2017) 23:478–88. doi: 10.1158/1078-0432.CCR-16-1203
60. Clinical study of ET1402L1-CAR T cells in AFP expressing Hepatocellular Carcinoma . Available at: https://clinicaltrials.gov/study/NCT03349255 (Accessed October 23, 2023).
61. Gao W, Kim H, Feng M, Phung Y, Xavier CP, Rubin JS, et al. Inactivation of Wnt signaling by a human antibody that recognizes the heparan sulfate chains of glypican-3 for liver cancer therapy. Hepatology (2014) 60:576–87. doi: 10.1002/hep.26996
62. Gao W, Kim H, Ho M. Human Monoclonal antibody targeting the heparan sulfate chains of glypican-3 inhibits HGF-mediated migration and motility of hepatocellular carcinoma cells. PloS One (2015) 10:e0137664. doi: 10.1371/journal.pone.0137664
63. Zhu AX, Gold PJ, El-Khoueiry AB, Abrams TA, Morikawa H, Ohishi N, et al. First-in-man phase I study of GC33, a novel recombinant humanized antibody against glypican-3, in patients with advanced hepatocellular carcinoma. Clin Cancer Res (2013) 19:920–8. doi: 10.1158/1078-0432.CCR-12-2616
64. Liu X, Gao F, Jiang L, Jia M, Ao L, Lu M, et al. 32A9, a novel human antibody for designing an immunotoxin and CAR-T cells against glypican-3 in hepatocellular carcinoma. J Transl Med (2020) 18:295. doi: 10.1186/s12967-020-02462-1
65. GPC3 targeted CAR-T cell therapy in Advanced GPC3 Expressing Hepatocellular Carcinoma (HCC) . Available at: https://clinicaltrials.gov/ct2/show/NCT05003895 (Accessed June 16, 2023).
66. Interleukin-15 Armored Glypican 3-specific Chimeric Antigen Receptor Expressed in Autologous T Cells for Hepatocellular Carcinoma . Available at: https://clinicaltrials.gov/ct2/show/NCT05103631 (Accessed June 16, 2023).
67. B010-A Injection for Treating Patients With GPC3 Positive Advanced Hepatocellular Carcinoma . Available at: https://clinicaltrials.gov/ct2/show/NCT05070156 (Accessed June 16, 2023).
68. Jin M-Z, Xia B-R, Xu Y, Jin W-L. Curaxin CBL0137 exerts anticancer activity via diverse mechanisms. Front Oncol (2018) 8:598. doi: 10.3389/fonc.2018.00598
69. YIV-906 (formerly PHY906/KD018) with sorafenib in HBV(+) hepatocellular carcinoma (HCC) https://clinicaltrials.gov/study/NCT0400 (2019). https://clinicaltrials.gov/study/NCT04000737. [Accessed June 8, 2023]
70. Ghamande S, Lin C-C, Cho DC, Shapiro GI, Kwak EL, Silverman MH, et al. A phase 1 open-label, sequential dose-escalation study investigating the safety, tolerability, and pharmacokinetics of intravenous TLC388 administered to patients with advanced solid tumors. Invest New Drugs (2014) 32:445–51. doi: 10.1007/s10637-013-0044-7
71. Chen M-H, Chou W-C, Hsiao C-F, Jiang SS, Tsai H-J, Liu Y-C, et al. An open-label, single-arm, two-stage, multicenter, phase II study to evaluate the efficacy of TLC388 and genomic analysis for poorly differentiated neuroendocrine carcinomas. Oncologist (2020) 25:e782–8. doi: 10.1634/theoncologist.2019-0490
72. DeMaria PJ, Lee-Wisdom K, Donahue RN, Madan RA, Karzai F, Schwab A, et al. Phase 1 open-label trial of intravenous administration of MVA-BN-brachyury-TRICOM vaccine in patients with advanced cancer. J Immunother Cancer (2021) 9:e003238. doi: 10.1136/jitc-2021-003238
73. N-803 alone or in combination With BN-Brachyury Vaccine or Bintrafusp Alfa (M7824) for Participants With Castration Resistant Prostate Cancer. Available at: https://clinicaltrials.gov/ct2/show/NCT05445882 (Accessed June 8, 2023).
74. Phase I/II Study of Immunotherapy Combination BN-Brachyury Vaccine, M7824, N-803 and Epacadostat (QuEST1) . Available at: https://clinicaltrials.gov/ct2/show/NCT03493945 (Accessed June 8, 2023).
75. Meng Z, Moroishi T, Guan K-L. Mechanisms of Hippo pathway regulation. Genes Dev (2016) 30:1–17. doi: 10.1101/gad.274027.115
76. Shi J, Farzaneh M, Khoshnam SE. Yes-Associated protein and PDZ binding motif: A critical signaling pathway in the control of human pluripotent stem cells self-renewal and differentiation. Cell Reprogram (2020) 22:55–61. doi: 10.1089/cell.2019.0084
77. Zhao B, Ye X, Yu J, Li L, Li W, Li S, et al. TEAD mediates YAP-dependent gene induction and growth control. Genes Dev (2008) 22:1962–71. doi: 10.1101/gad.1664408
78. Hayashi H, Higashi T, Yokoyama N, Kaida T, Sakamoto K, Fukushima Y, et al. An imbalance in TAZ and YAP expression in hepatocellular carcinoma confers cancer stem cell–like behaviors contributing to disease progression. Cancer Res (2015) 75:4985–97. doi: 10.1158/0008-5472.CAN-15-0291
79. Oral TEAD Inhibitor Targeting the Hippo Pathway in Subjects With Advanced Solid Tumors . Available at: https://clinicaltrials.gov/ct2/show/NCT05228015 (Accessed May 22, 2023).
80. Deng X, Fang L. VGLL4 is a transcriptional cofactor acting as a novel tumor suppressor via interacting with TEADs. Am J Cancer Res (2018) 8:932–43.
81. Ikena Oncology shares differentiation profile of IK-930, a novel hippo-pathway inhibitor, including projected therapeutic index advantages and breadth of patient populations at AACR 2023 annual meeting (Accessed May 22, 2023).
82. Amidon BS, Sanchez-Martin M, Bartolini W, Syed S, McGovern K, Xu L, et al. Abstract 2156: IK-930 is a novel TEAD inhibitor for the treatment of cancers harboring mutations in the Hippo signal transduction pathway. Cancer Res (2022) 82:2156–6. doi: 10.1158/1538-7445.AM2022-2156
83. Dahmani R, Just P-A, Perret C. The Wnt/β-catenin pathway as a therapeutic target in human hepatocellular carcinoma. Clin Res Hepatol Gastroenterol (2011) 35:709–13. doi: 10.1016/j.clinre.2011.05.010
84. Wang W, Smits R, Hao H, He C. Wnt/β-catenin signaling in liver cancers. Cancers (2019) 11:926. doi: 10.3390/cancers11070926
85. Tian Y, Mok MTS, Yang P, Cheng ASL. Epigenetic activation of wnt/β-catenin signaling in NAFLD-associated hepatocarcinogenesis. Cancers (2016) 8:76. doi: 10.3390/cancers8080076
86. Kim MS, Yoon SK, Bollig F, Kitagaki J, Hur W, Whye NJ, et al. A novel Wilms tumor 1 (WT1) target gene negatively regulates the WNT signaling pathway. J Biol Chem (2010) 285:14585–93. doi: 10.1074/jbc.M109.094334
87. Chen Y, Peng C, Chen J, Chen D, Yang B, He B, et al. WTAP facilitates progression of hepatocellular carcinoma via m6A-HuR-dependent epigenetic silencing of ETS1. Mol Cancer (2019) 18:127. doi: 10.1186/s12943-019-1053-8
88. Zhang J, Tsoi H, Li X, Wang H, Gao J, Wang K, et al. Carbonic anhydrase IV inhibits colon cancer development by inhibiting the Wnt signalling pathway through targeting the WTAP-WT1-TBL1 axis. Gut (2016) 65:1482–93. doi: 10.1136/gutjnl-2014-308614
89. Komposch K, Sibilia M. EGFR signaling in liver diseases. Int J Mol Sci (2015) 17:30. doi: 10.3390/ijms17010030
90. Ito Y, Takeda T, Sakon M, Tsujimoto M, Higashiyama S, Noda K, et al. Expression and clinical significance of erb-B receptor family in hepatocellular carcinoma. Br J Cancer (2001) 84:1377–83. doi: 10.1054/bjoc.2000.1580
91. Daveau M, Scotte M, François A, Coulouarn C, Ros G, Tallet Y, et al. Hepatocyte growth factor, transforming growth factor alpha, and their receptors as combined markers of prognosis in hepatocellular carcinoma. Mol Carcinog (2003) 36:130–41. doi: 10.1002/mc.10103
92. Moon WS, Park HS, Yu KH, Park MY, Kim KR, Jang KY, et al. Expression of betacellulin and epidermal growth factor receptor in hepatocellular carcinoma: implications for angiogenesis. Hum Pathol (2006) 37:1324–32. doi: 10.1016/j.humpath.2006.04.022
93. Harada K, Shiota G, Kawasaki H. Transforming growth factor-alpha and epidermal growth factor receptor in chronic liver disease and hepatocellular carcinoma. Liver (1999) 19:318–25. doi: 10.1111/j.1478-3231.1999.tb00056.x
94. Chung YH, Kim JA, Song BC, Lee GC, Koh MS, Lee YS, et al. Expression of transforming growth factor-alpha mRNA in livers of patients with chronic viral hepatitis and hepatocellular carcinoma. Cancer (2000) 89:977–82. doi: 10.1002/1097-0142(20000901)89:5<977::AID-CNCR6>3.0.CO;2-I
95. Jin H, Shi Y, Lv Y, Yuan S, Ramirez CFA, Lieftink C, et al. EGFR activation limits the response of liver cancer to lenvatinib. Nature (2021) 595:730–4. doi: 10.1038/s41586-021-03741-7
96. Lenvatinib combined with gefitinib in the Treatment of Lenvatinib-resistant Hepatocellular Carcinoma. Available at: https://clinicaltrials.gov/ct2/show/NCT04642547?term=lenvatinib+gefitinib&draw=2&rank=1 (Accessed May 22, 2023).
97. Wilson GK, Tennant DA, McKeating JA. Hypoxia inducible factors in liver disease and hepatocellular carcinoma: current understanding and future directions. J Hepatol (2014) 61:1397–406. doi: 10.1016/j.jhep.2014.08.025
98. Keith B, Johnson RS, Simon MC. HIF1α and HIF2α: sibling rivalry in hypoxic tumour growth and progression. Nat Rev Cancer (2011) 12:9–22. doi: 10.1038/nrc3183
99. Huang LE. Carrot and stick: HIF-α engages c-Myc in hypoxic adaptation. Cell Death Differ (2008) 15:672–7. doi: 10.1038/sj.cdd.4402302
100. Kim J-W, Gao P, Liu Y-C, Semenza GL, Dang CV. Hypoxia-inducible factor 1 and dysregulated c-Myc cooperatively induce vascular endothelial growth factor and metabolic switches hexokinase 2 and pyruvate dehydrogenase kinase 1. Mol Cell Biol (2007) 27:7381–93. doi: 10.1128/MCB.00440-07
101. Doe MR, Ascano JM, Kaur M, Cole MD. Myc posttranscriptionally induces HIF1 protein and target gene expression in normal and cancer cells. Cancer Res (2012) 72:949–57. doi: 10.1158/0008-5472.CAN-11-2371
102. Mu H, Yu G, Li H, Wang M, Cui Y, Zhang T, et al. Mild chronic hypoxia-induced HIF-2α interacts with c-MYC through competition with HIF-1α to induce hepatocellular carcinoma cell proliferation. Cell Oncol (2021) 44:1151–66. doi: 10.1007/s13402-021-00625-w
103. Phase I Study of Intravenous Lipotecan® (TLC388 HCl for Injection) in Patients With Advanced Solid Tumors. Available at: https://clinicaltrials.gov/ct2/show/NCT00747474?term=Lipotecan&draw=2&rank=2 (Accessed May 22, 2023).
104. Lu J, Wei H, Sun W, Geng J, Liu K, Liu J, et al. Abstract 6330: NKT2152: A highly potent HIF2α inhibitor and its therapeutic potential in solid tumors beyond ccRCC. Cancer Res (2022) 82:6330–0. doi: 10.1158/1538-7445.AM2022-6330
105. A Study of NKT2152, a HIF2α Inhibitor, in Patients With Advanced Clear Cell Renal Cell Carcinoma. Available at: https://clinicaltrials.gov/ct2/show/NCT05119335?term=nkt2152&draw=2&rank=1 (Accessed May 22, 2023).
106. A Phase 1/2 Study to Evaluate OTX-2002 in Patients With Hepatocellular Carcinoma and Other Solid Tumor Types Known for Association With the MYC Oncogene - Full Text View - ClinicalTrials.Gov. Available at: https://classic.clinicaltrials.gov/ct2/show/NCT05497453 (Accessed July 6, 2023).
107. Rodriguez-Rivera II, Wu T-H, Ciotti R, Senapedis W, Sullivan K, Gao JZ, et al. A phase 1/2 open-label study to evaluate the safety, tolerability, pharmacokinetics, pharmacodynamics, and preliminary antitumor activity of OTX-2002 as a single agent and in combination with standard of care in patients with hepatocellular carcinoma and other solid tumor types known for association with the MYC oncogene (MYCHELANGELO I). J Clin Orthod (2023) 41:TPS627–7. doi: 10.1200/JCO.2023.41.4_suppl.TPS627
108. A Phase 1/2 Study to Evaluate OTX-2002 in Patients With Hepatocellular Carcinoma and Other Solid Tumor Types Known for Association With the MYC Oncogene - Full Text View - ClinicalTrials.gov. Available at: https://clinicaltrials.gov/ct2/show/NCT05497453 (Accessed May 22, 2023).
109. Hussain SP, Schwank J, Staib F, Wang XW, Harris CC. TP53 mutations and hepatocellular carcinoma: insights into the etiology and pathogenesis of liver cancer. Oncogene (2007) 26:2166–76. doi: 10.1038/sj.onc.1210279
110. Hassin O, Oren M. Drugging p53 in cancer: one protein, many targets. Nat Rev Drug Discovery (2023) 22:127–44. doi: 10.1038/s41573-022-00571-8
111. Hastie ND. Wilms’ tumour 1 (WT1) in development, homeostasis and disease. Development (2017) 144:2862–72. doi: 10.1242/dev.153163
112. Wagner N, Michiels JF, Schedl A, Wagner K-D. The Wilms’ tumour suppressor WT1 is involved in endothelial cell proliferation and migration: expression in tumour vessels in vivo. Oncogene (2008) 27:3662–72. doi: 10.1038/sj.onc.1211044
113. Lv L, Chen G, Zhou J, Li J, Gong J. WT1-AS promotes cell apoptosis in hepatocellular carcinoma through down-regulating of WT1. J Exp Clin Cancer Res (2015) 34:119. doi: 10.1186/s13046-015-0233-7
114. Suginobe N, Nakamura M, Takanashi Y, Ban H, Gotoh M. Mechanism of action of DSP-7888 (adegramotide/nelatimotide) Emulsion, a peptide-based therapeutic cancer vaccine with the potential to turn up the heat on non-immunoreactive tumors. Clin Transl Oncol (2023) 25:396–407. doi: 10.1007/s12094-022-02946-0
115. A Study of DSP-7888 Dosing Emulsion in Combination With Immune Checkpoint Inhibitors in Adult Patients With Advanced Solid Tumors. Available at: https://clinicaltrials.gov/ct2/show/NCT03311334?term=DSP-7888&draw=2&rank=3 (Accessed May 22, 2023).
116. Kim E, Lisby A, Ma C, Lo N, Ehmer U, Hayer KE, et al. Promotion of growth factor signaling as a critical function of β-catenin during HCC progression. Nat Commun (2019) 10:1909. doi: 10.1038/s41467-019-09780-z
117. Debebe A, Medina V, Chen C-Y, Mahajan IM, Jia C, Fu D, et al. Wnt/β-catenin activation and macrophage induction during liver cancer development following steatosis. Oncogene (2017) 36:6020–9. doi: 10.1038/onc.2017.207
118. Naiche LA, Harrelson Z, Kelly RG, Papaioannou VE. T-box genes in vertebrate development. Annu Rev Genet (2005) 39:219–39. doi: 10.1146/annurev.genet.39.073003.105925
119. Kilic N, Feldhaus S, Kilic E, Tennstedt P, Wicklein D, Wasielewski Rv, et al. Brachyury expression predicts poor prognosis at early stages of colorectal cancer. Eur J Cancer (2011) 47:1080–5. doi: 10.1016/j.ejca.2010.11.015
120. Imajyo I, Sugiura T, Kobayashi Y, Shimoda M, Ishii K, Akimoto N, et al. T-box transcription factor Brachyury expression is correlated with epithelial-mesenchymal transition and lymph node metastasis in oral squamous cell carcinoma. Int J Oncol (2012) 41:1985–95. doi: 10.3892/ijo.2012.1673
121. Du R, Wu S, Lv X, Fang H, Wu S, Kang J. Overexpression of brachyury contributes to tumor metastasis by inducing epithelial-mesenchymal transition in hepatocellular carcinoma. J Exp Clin Cancer Res (2014) 33:105. doi: 10.1186/s13046-014-0105-6
122. Fernando RI, Litzinger M, Trono P, Hamilton DH, Schlom J, Palena C. The T-box transcription factor Brachyury promotes epithelial-mesenchymal transition in human tumor cells. J Clin Invest (2010) 120:533–44. doi: 10.1172/JCI38379
123. Phase I/II Study of Immunotherapy Combination BN-Brachyury Vaccine, M7824, N-803 and Epacadostat (QuEST1). Available at: https://clinicaltrials.gov/ct2/show/NCT03493945?term=Brachyury&draw=2&rank=9 (Accessed May 22, 2023).
124. Moein S, Tenen DG, Amabile G, Chai L. SALL4: An intriguing therapeutic target in cancer treatment. Cells (2022) 11:2601. doi: 10.3390/cells11162601
125. Nicolè L, Sanavia T, Veronese N, Cappellesso R, Luchini C, Dabrilli P, et al. Oncofetal gene SALL4 and prognosis in cancer: A systematic review with meta-analysis. Oncotarget (2017) 8:22968–79. doi: 10.18632/oncotarget.14952
126. Bard JD, Gelebart P, Amin HM, Young LC, Ma Y, Lai R. Signal transducer and activator of transcription 3 is a transcriptional factor regulating the gene expression of SALL4. FASEB J (2009) 23:1405–14. doi: 10.1096/fj.08-117721
127. Böhm J, Sustmann C, Wilhelm C, Kohlhase J. SALL4 is directly activated by TCF/LEF in the canonical Wnt signaling pathway. Biochem Biophys Res Commun (2006) 348:898–907. doi: 10.1016/j.bbrc.2006.07.124
128. Liu C, Wu H, Li Y, Shen L, Yu R, Yin H, et al. SALL4 suppresses PTEN expression to promote glioma cell proliferation via PI3K/AKT signaling pathway. J Neurooncol (2017) 135:263–72. doi: 10.1007/s11060-017-2589-3
129. Kong NR, Bassal MA, Tan HK, Kurland JV, Yong KJ, Young JJ, et al. Zinc finger protein SALL4 functions through an AT-rich motif to regulate gene expression. Cell Rep (2021) 34:108574. doi: 10.1016/j.celrep.2020.108574
130. Tan JL, Li F, Yeo JZ, Yong KJ, Bassal MA, Ng GH, et al. New high-throughput screening identifies compounds that reduce viability specifically in liver cancer cells that express high levels of SALL4 by inhibiting oxidative phosphorylation. Gastroenterology (2019) 157:1615–1629.e17. doi: 10.1053/j.gastro.2019.08.022
131. Liu BH, Jobichen C, Chia CSB, Chan THM, Tang JP, Chung TXY, et al. Targeting cancer addiction for SALL4 by shifting its transcriptome with a pharmacologic peptide. Proc Natl Acad Sci U.S.A. (2018) 115:E7119–28. doi: 10.1073/pnas.1801253115
132. Donisi C, Puzzoni M, Ziranu P, Lai E, Mariani S, Saba G, et al. Immune checkpoint inhibitors in the treatment of HCC. Front Oncol (2020) 10:601240. doi: 10.3389/fonc.2020.601240
133. Yau T, Kang Y-K, Kim T-Y, El-Khoueiry AB, Santoro A, Sangro B, et al. Efficacy and safety of nivolumab plus ipilimumab in patients with advanced hepatocellular carcinoma previously treated with sorafenib: the CheckMate 040 randomized clinical trial. JAMA Oncol (2020) 6:e204564–e204564. doi: 10.1001/jamaoncol.2020.4564
134. Carroll HK, Aleem U, Varghese P, Galligan M, Bourke M, Hoey K, et al. Trial-in-progress: A pilot study of combined immune checkpoint inhibition in combination with ablative therapies in subjects with hepatocellular carcinoma (HCC). J Clin Orthod (2021) 39:TPS355–5. doi: 10.1200/JCO.2021.39.3_suppl.TPS355
135. Mukherjee B. Nanotherapeutics for the treatment of hepatocellular Carcinoma (2022). Available at: https://play.google.com/store/books/details?id=PYhsEAAAQBAJ.
136. Sangro B, Sarobe P, Hervás-Stubbs S, Melero I. Advances in immunotherapy for hepatocellular carcinoma. Nat Rev Gastroenterol Hepatol (2021) 18:525–43. doi: 10.1038/s41575-021-00438-0
137. Sun C, Lan P, Han Q, Huang M, Zhang Z, Xu G, et al. Oncofetal gene SALL4 reactivation by hepatitis B virus counteracts miR-200c in PD-L1-induced T cell exhaustion. Nat Commun (2018) 9:1241. doi: 10.1038/s41467-018-03584-3
138. Sun B, Xu L, Bi W, Ou W-B. SALL4 Oncogenic Function in cancers: mechanisms and therapeutic relevance. Int J Mol Sci (2022) 23:2053. doi: 10.3390/ijms23042053
139. Faivre S, Rimassa L, Finn RS. Molecular therapies for HCC: Looking outside the box. J Hepatol (2020) 72:342–52. doi: 10.1016/j.jhep.2019.09.010
140. Sanduzzi Zamparelli M, Matilla A, Lledó JL, Martínez SM, Varela M, Iñarrairaegui M, et al. Early nivolumab addition to regorafenib in patients with hepatocellular carcinoma progressing under first-line therapy (GOING trial), interim analysis and safety profile. J Clin Orthod (2022) 40:428–8. doi: 10.1200/JCO.2022.40.4_suppl.428
141. Zhang E-L, Zhang Z-Y, Li J, Huang Z-Y. Complete response to the sequential treatment with regorafenib followed by PD-1 inhibitor in a sorafenib-refractory hepatocellular carcinoma patient. Onco Targets Ther (2020) 13:12477–87. doi: 10.2147/OTT.S284092
142. Kurebayashi Y, Kubota N, Sakamoto M. Immune microenvironment of hepatocellular carcinoma, intrahepatic cholangiocarcinoma and liver metastasis of colorectal adenocarcinoma: Relationship with histopathological and molecular classifications. Hepatol Res (2021) 51:5–18. doi: 10.1111/hepr.13539
143. Peng X, Gong C, Zhang W, Zhou A. Advanced development of biomarkers for immunotherapy in hepatocellular carcinoma. Front Oncol (2022) 12:1091088. doi: 10.3389/fonc.2022.1091088
144. Wang Y, Tong Z, Zhang W, Zhang W, Buzdin A, Mu X, et al. FDA-approved and emerging next generation predictive biomarkers for immune checkpoint inhibitors in cancer patients. Front Oncol (2021) 11:683419. doi: 10.3389/fonc.2021.683419
145. Sankar K, Ye JC, Li Z, Zheng L, Song W, Hu-Lieskovan S. The role of biomarkers in personalized immunotherapy. biomark Res (2022) 10:32. doi: 10.1186/s40364-022-00378-0
146. Lo Re O, Fusilli C, Rappa F, Van Haele M, Douet J, Pindjakova J, et al. Induction of cancer cell stemness by depletion of macrohistone H2A1 in hepatocellular carcinoma. Hepatology (2018) 67:636–50. doi: 10.1002/hep.29519
147. Dong Y, Wong JSL, Sugimura R, Lam K-O, Li B, Kwok GGW, et al. Recent advances and future prospects in immune checkpoint (ICI)-based combination therapy for advanced HCC. Cancers (2021) 13:1949. doi: 10.3390/cancers13081949
148. Chan JJ, Zhang B, Chew XH, Salhi A, Kwok ZH, Lim CY, et al. Pan-cancer pervasive upregulation of 3’ UTR splicing drives tumourigenesis. Nat Cell Biol (2022) 24:928–39. doi: 10.1038/s41556-022-00913-z
149. Wilson SR, Duncan AW. Single-cell DNA sequencing reveals chromosomal diversity in HCC and a novel model of HCC evolution. Gastroenterology (2022) 162:46–8. doi: 10.1053/j.gastro.2021.09.065
150. Finn RS, Ryoo B-Y, Merle P, Kudo M, Bouattour M, Lim HY, et al. Pembrolizumab as second-line therapy in patients with advanced hepatocellular carcinoma in KEYNOTE-240: A randomized, double-blind, phase III trial. J Clin Oncol (2020) 38:193–202. doi: 10.1200/JCO.19.01307
151. Waidmann O. Recent developments with immunotherapy for hepatocellular carcinoma. Expert Opin Biol Ther (2018) 18:905–10. doi: 10.1080/14712598.2018.1499722
Keywords: hepatocellular carcinoma, biomarker, precision medicine, transcription factors (TF), combination therapy, immunotherapy
Citation: Liu J, Park K, Shen Z, Lee H, Geetha P, Pakyari M and Chai L (2023) Immunotherapy, targeted therapy, and their cross talks in hepatocellular carcinoma. Front. Immunol. 14:1285370. doi: 10.3389/fimmu.2023.1285370
Received: 29 August 2023; Accepted: 05 December 2023;
Published: 19 December 2023.
Edited by:
Marco Punta, San Raffaele Hospital (IRCCS), ItalyReviewed by:
Claudia De Lalla, San Raffaele Hospital (IRCCS), ItalyFelix Braun, University Medical Center Schleswig-Holstein, Germany
Copyright © 2023 Liu, Park, Shen, Lee, Geetha, Pakyari and Chai. This is an open-access article distributed under the terms of the Creative Commons Attribution License (CC BY). The use, distribution or reproduction in other forums is permitted, provided the original author(s) and the copyright owner(s) are credited and that the original publication in this journal is cited, in accordance with accepted academic practice. No use, distribution or reproduction is permitted which does not comply with these terms.
*Correspondence: Li Chai, lchai@bwh.harvard.edu
†These authors share first authorship