- 1Department of Diabetes and Endocrinology, Japanese Red Cross Wakayama Medical Center, Wakayama, Japan
- 2The First Department of Medicine, Wakayama Medical University, Wakayama, Japan
- 3Department of Dermatology, Wakayama Medical University, Wakayama, Japan
- 4Department of Pharmaceutical Health Sciences, Kyushu University of Health and Welfare, Nobeoka, Miyazaki, Japan
- 5Department of Medicine, Division of Gastroenterology and Hepatology, Shinshu University School of Medicine, Matsumoto, Japan
- 6HLA Typing Section, GenoDive Pharma Inc., Kanagawa, Japan
- 7Research Department, Biospecimen Laboratories, Inc. Tokyo, Japan
- 8Department of Endocrinology and Diabetes, Nagoya University Graduate School of Medicine, Nagoya, Aichi, Japan
Introduction: Immune-checkpoint inhibitors are effective in various advanced cancers. Type 1 diabetes mellitus induced by them (ICI-T1DM) is a serious complication requiring prompt insulin treatment, but the immunological mechanism behind it is unclear.
Methods: We examined amino acid polymorphisms in human histocompatibility leukocyte antigen (HLA) molecules and investigated proinsulin epitope binding affinities to HLA molecules.
Results and Discussion: Twelve patients with ICI-T1DM and 35 patients in a control group without ICI-T1DM were enrolled in the study. Allele and haplotype frequencies of HLA-DRB1*04:05, DQB1*04:01, and most importantly DPB1*05:01 were significantly increased in patients with ICI-T1DM. In addition, novel amino acid polymorphisms in HLA-DR (4 polymorphisms), in DQ (12 polymorphisms), and in DP molecules (9 polymorphisms) were identified. These amino acid polymorphisms might be associated with the development of ICI-T1DM. Moreover, novel human proinsulin epitope clusters in insulin A and B chains were discovered in silico and in vitro peptide binding assays to HLA-DP5. In conclusion, significant amino acid polymorphisms in HLA-class II molecules, and conformational alterations in the peptide-binding groove of the HLA-DP molecules were considered likely to influence the immunogenicity of proinsulin epitopes in ICI-T1DM. These amino acid polymorphisms and HLA-DP5 may be predictive genetic factors for ICI-T1DM.
Introduction
Immune-checkpoint inhibitors (ICIs) are effective agents in various cancers; however, immune-related adverse events (irAEs) often occur during treatment with ICIs (1–5). Major endocrine irAEs include pituitary irAE (3), thyroid irAE (4), and ICI-induced type 1 diabetes mellitus (ICI-T1DM). ICI-T1DM is an especially critical irAE due to the possibility of acute damage to pancreatic β-cells (5). Qiu et al. reported that anti-insulin antibody was observed in 7 patients with ICI-T1DM (3 patients with fulminant type 1 diabetes, and 4 patients with acute type 1 diabetes) (6). One patient with ICI-T1DM exhibited anti-insulin antibody positivity in our study (5). Proinsulin is cleaved into insulin and C-peptide in pancreatic β-cells at secretion (7), we thus hypothesized that proinsulin could be a major autoantigen in ICI-T1DM.
We have previously identified thyrotropin receptor epitopes to HLA-DR molecules in Graves’ disease in silico, in vitro, and human studies (8, 9). Further, we have also previously in part examined of histocompatibility leukocyte antigen (HLA) alleles and haplotypes in ICI-T1DM. However, immunological mechanisms in ICI-T1DM are largely unknown due to the rarity of the disease (0.8% prevalence among ICI-treated patients (5).
In the current study, novel amino acid polymorphisms in HLA class II molecules in patients with ICI-T1DM, and in vitro proinsulin epitope binding affinities to HLA-DP molecules were revealed. These genetic factors may be utilized for prediction of ICI-T1DM, and also contribute to elucidate the mechanism of cancer immunotherapy and ICI-T1DM. Therefore, current study offers novel management and monitoring options for cancer immunotherapy.
Patients and methods
Patients
Patients were recruited from the Japanese Red Cross Society Wakayama Medical Center (JRCW), Wakayama Medical University Hospital (WMU), and Nagoya University (NU). Patients with advanced malignant diseases who received ICI treatment were examined during 2016-2021. ICI treatments included anti-PD-1 antibody (nivolumab or pembrolizumab), anti-PD-L1 antibody (durvalumab or atezolizumab), or anti-CTLA-4 antibody (ipilimumab) following nivolumab (5, 10). The study protocol was approved by the JRCW, WMU, and NU Institutional Ethical Review Boards, and written informed consent was obtained from all participants.
Assessment of irAEs and ICI-T1DM
Assessment of irAEs was made based on the descriptions and grading scales of the National Cancer Institute (NCI) Common Terminology Criteria for Adverse Events version 3.0. Diagnostic criteria for T1DM were based on the hyperglycemic symptoms, and continuous requirement of insulin therapy irrespective of autoimmune diabetes-related autoantibodies (11). ICI-controls were defined as those who were treated with ICI but did not develop any irAEs including T1DM (5, 10). Healthy Japanese individuals were used as general controls (12–14), and participants had no clinical or demographic differences and they had the same ethnic background.
HLA-genotyping and amino acid sequences
DNA extracted from blood was genotyped in HLA-A, B, C, DRB1, DQB1, and DPB1 alleles by the next-generation sequence method and a Luminex system with WAKFlow HLA typing kits (GenoDive Pharma, Kanagawa, Japan) as previously described (5, 12–14). Allele frequencies were determined by direct counting and three-locus (DRB1~DQB1~DPB1) haplotype frequencies were obtained by maximum likelihood methods as previously described (http://cmpg.unibe.ch/software/arlequin3/) (5, 12–14). Amino acid sequences were downloaded from (https://www.ebi.ac.uk/ipd/imgt/hla/).
Prediction of human proinsulin-peptides binding affinities to HLA-DP5 and HLA-DP15
In silico binding of human proinsulin (AA 1-110) (NP_000198.1) derived peptide to HLA-DP5 molecule (HLA-DPA1*02:02, HLA-DPB1*05:01) and a control allele, HLA-DP15 molecule (HLA-DPA1*02:02, HLA-DPB1*15:01: not previously reported as susceptible or protective allele) were predicted by NetMHCIIpan software version 4.0. The %RANK threshold for strong binders was set as <5%. The peptides with a 5-50%RANK threshold were set as intermediate binders.
In vitro human proinsulin peptides binding assay to HLA-DP5 and HLA-DP15
Fifteen-mer peptides derived from human proinsulin were synthesized based on the predicted affinities to HLA-DP5: 1) strong binders, 2) intermediate binders, and 3) others to cover the entire sequence (PEPscreen® peptide library, ProImmune, Oxford, UK). Peptides that were known to have high affinities with HLA-DP5 and HLA-DP15 were also synthesized. The synthesized peptides were subjected to an in vitro peptide binding assay (ProImmune REVEAL® MHC class II-peptide binding assay: (ProImmune, Oxford, UK) using recombinant HLA-DP5 and HLA-DP15 proteins.Detection of binding peptides is based on the presence or absence of the native conformation of the MHC-peptide complex in an immunoassay. Each test peptide was given a ‘REVEAL SCORE’ relative to positive control peptides, which were known to bind HLA-DP5 or HLA-DP15 with high affinity (signal of known positive control peptide which was known to bind each HLA-DP with high affinity, divided by each test peptide x 100%). The high-throughput assay quantifies the ability of the test peptides to bind to HLA-DP5 and HLA-DP15. The results of the three separate assays were in close agreement and are presented as an average of the results.
Three-dimensional modeling of the HLA-class II molecules
Three-dimensional modeling of HLA-class II molecules (HLA-DR, HLA-DQ, and HLA-DP) was downloaded from the Protein Data Bank database (15), and visualized with PyMOL (16).
Statistical analysis
Differences between the two groups were analyzed by a Mann-Whitney U test. The association of allele frequencies was analyzed using Fisher’s exact test. Frequencies of HLA alleles and amino acid polymorphisms were analyzed by univariate and multivariate logistic regression analysis with stepwise selection of covariates. Bonferroni test was applied if the variables were significant. Statistical analyses were performed using JMP, version 15 (SAS Institute Inc., Cary, N.C., USA). P values < 0.05 were considered to be statistically significant.
Results
Clinical characteristics of patients with ICI-T1DM
A total of twelve patients with ICI-T1DM (six patients from JRC, two patients from WMU, and four patients from NU) were identified and enrolled in the study (Tables 1A, 1B; Supplementary Table 1). Clinical profiles of seven patients with ICI-T1DM were partly described previously (5). Thirty-five independent ICI-controls (13 from WMU and 22 from NU) were prospectively identified as subjects without irAE and were analyzed. Anti-GAD65 antibody was negative for patients measured. Patient #6 only developed insulin autoantibodies (IAA).
HLA alleles and haplotypes analysis
The plasma glucose levels of patients with ICI-T1DM and HLA typing results are summarized in Table 1B. We have used HLA-class I and II four-digit allelic typing results in the allele or haplotype analysis (Tables 2A–E; Supplementary Table 2A–C). Allele frequencies of HLA-DRB1*04:05 (Table 2A) and HLA-DQB1*04:01 (Table 2B), both alleles in complete linkage disequilibrium, were significantly higher in patients with ICI-T1DM than in general controls and also in ICI-controls. HLA-DPB1*05:01 allele frequency was more significantly associated with an increased risk of ICI-T1DM when compared with general controls and also in ICI-controls (P=0.005 and 0.004, respectively) (Table 2C).
In haplotype analysis, HLA-DRB1*04:05-DQB1*04:01 haplotype frequency was significantly higher in patients with ICI-T1DM than those of general controls and also in ICI-controls (P=0.026 and 0.003, respectively) (Table 2D). HLA-DRB1*04:05-DQB1*04:01-DPB1*05:01 haplotype frequency was significantly higher in patients with ICI-T1DM than those of general controls and also in ICI-controls (P=0.006 and 0.002, respectively) (Table 2E).
Of all significant HLA alleles and haplotypes, notably, only HLA-DQB1*04:01 and HLA-DPB1*05:01 allele frequencies were significantly increased in patients with ICI-T1DM compared with ICI-controls after Bonferroni correction (Pc=0.033 and Pc=0.04, respectively) (Tables 2B, 2C). Then the allele frequencies of HLA-DQB1*04:01 and HLA-DPB1*05:01 were compared in conditional multiple logistic regression analysis (Table 2F).
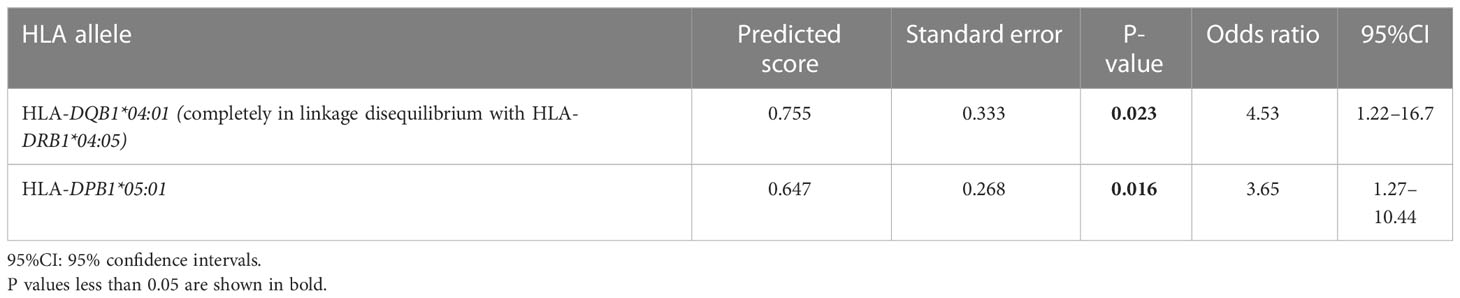
Table 2F Multiple logistic regression analysis of HLA-alleles in ICI-T1DM patients and ICI-controls.
Both allele frequencies were found to be significantly increased in ICI-T1DM, P=0.023 for HLA-DQB1*04:01 and P=0.016 for HLA-DPB1*05:01 (Table 2F). Note that the result also indicates the equivocal importance of following 3 factors: HLA-DQB1*04:01, HLA-DRB1*04:05, and HLA-DRB1*04:05-DQB1*04:01 haplotype, due to the complete linkage disequilibrium between HLA-DRB1*04:05 and HLA-DQB1*04:01 in the current study population (Table 2F).
Amino acid polymorphisms in each HLA-class II molecules
Further, univariate logistic regression analysis was thoroughly performed to examine relationships between ICI-T1DM and amino acid polymorphisms at HLA-DRβ1 (237 amino acid positions) (Supplementary Table 3A), DQβ1 (237 amino acid positions) (Supplementary Table 3B, and DPβ1 (229 amino acid positions) (Supplementary Table 3C). The amino acid polymorphisms with significance are indicated as yellow in the tables and they underwent further evaluation. Of those, amino acid carriages at amino acid positions 9, 57, 86, and 96 of HLA-DRβ1 were significantly different between the patients with ICI-T1DM and ICI-controls (Supplementary Table 4A, B). Glu (E) was significantly more frequently observed than Trp (W) (represented as E>W, the same applies hereafter) at position 9 (Figure 1A), Ser (S) > Asp (D) at position 57 (Figure 1B), Gly (G) > Val (V) at position 86 (Figure 1C), and Tyr (Y) > Gln (Q) at position 96 of HLA-DRβ1 in ICI-T1DM (Figure 1D).
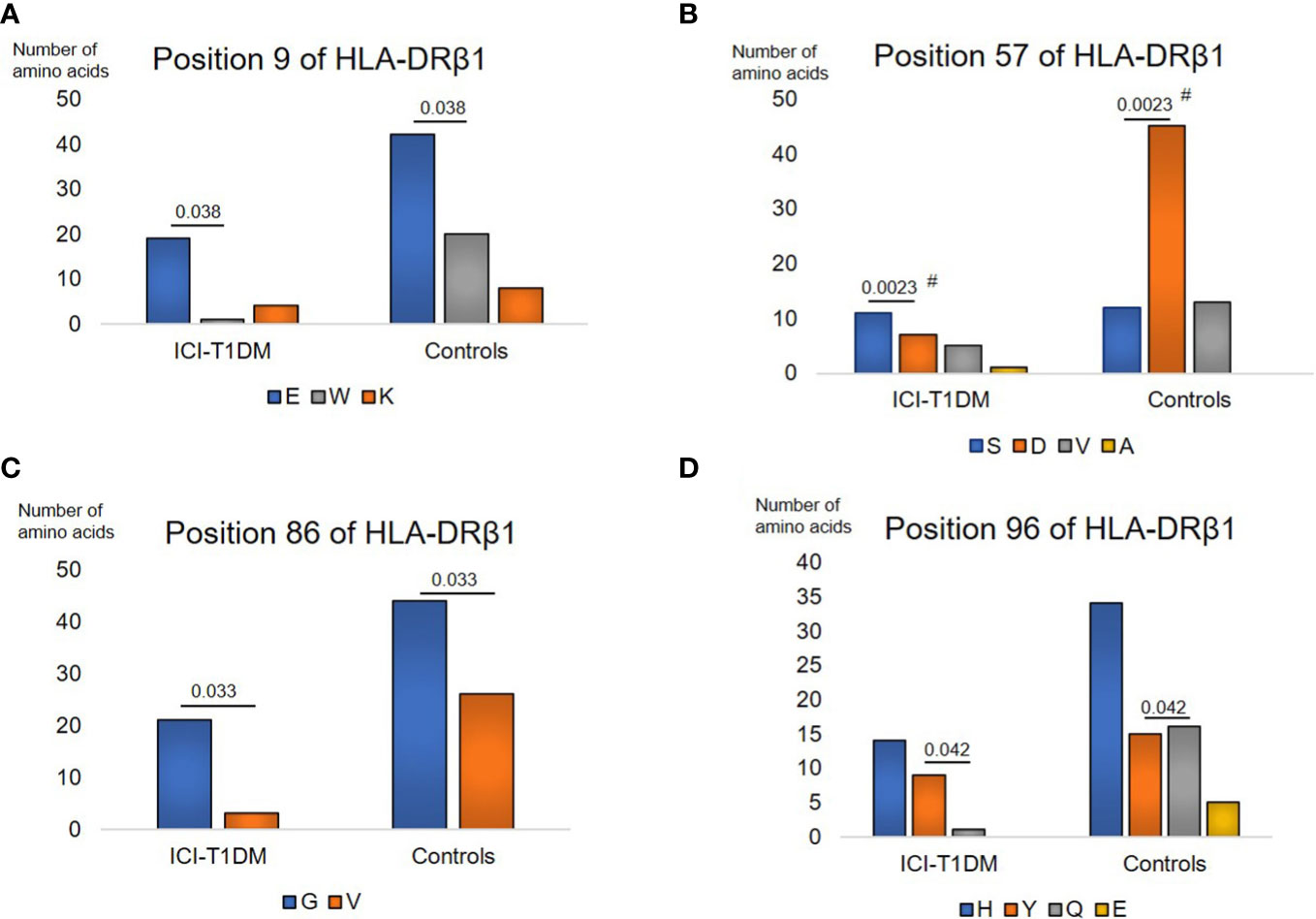
Figure 1 Prevalence of amino acid carriages at residues of HLA-DRB1 allele in patients with ICI-T1DM and ICI-controls (shown as controls) (A–D). Prevalence of amino acid at position 9, E, Glutamic acid vs W, Tryptophan, P=0.038. OR 9.05, 95% CI: 1.13-72.43 (A), position 57, S, Serine vs D, Aspartic acid, P=0.0023. OR 5.89, 95%CI: 1.88-18.46 (B), position 86, G, Glycine vs V, Valine, P=0.033. OR 4.14, 95%CI: 1.12-15.23 (C), and position 96, Y, Tyrosine vs Q, Glutamine, P=0.042. Odds ratio of 9.60, 95%CI: 1.08-85.16 (D) are shown. After Bonferroni correction for all significant amino acid polymorphisms among HLA-DRB1 alleles, only amino acid position 57 on HLA-DRB1 allele (B) was significantly different (Pc=0.046 after Bonferroni correction, shown with #).
Regarding HLA-DQβ1, amino acids carriages at amino acid positions 56, 70, 203, and 53-84-85-89-140-181-182-220-221 (each amino acid in complete linkage disequilibrium) of HLA-DQβ1 were significantly different between the patients with ICI-T1DM and ICI-controls (Supplementary Tables 5A, B). Leu (L) > Pro (P) at position 56 (Figure 2A), E > G at position 70 (Figure 2B), Ile (I) > V at position 203 (Figure 2C), and Leu-Gln-Leu-Thr-Thr-Gln-Asn-His-His (L-Q-L-T-T-Q-N-H-H) > Gln-Glu-Val-Gly-Ala-Gln-Ser-Arg-Gln (Q-E-V-G-A-Q-S-R-Q) at positions 53-84-85-89-140-181-182-220-221 of HLA-DQβ1 in ICI-T1DM (Figure 2D).
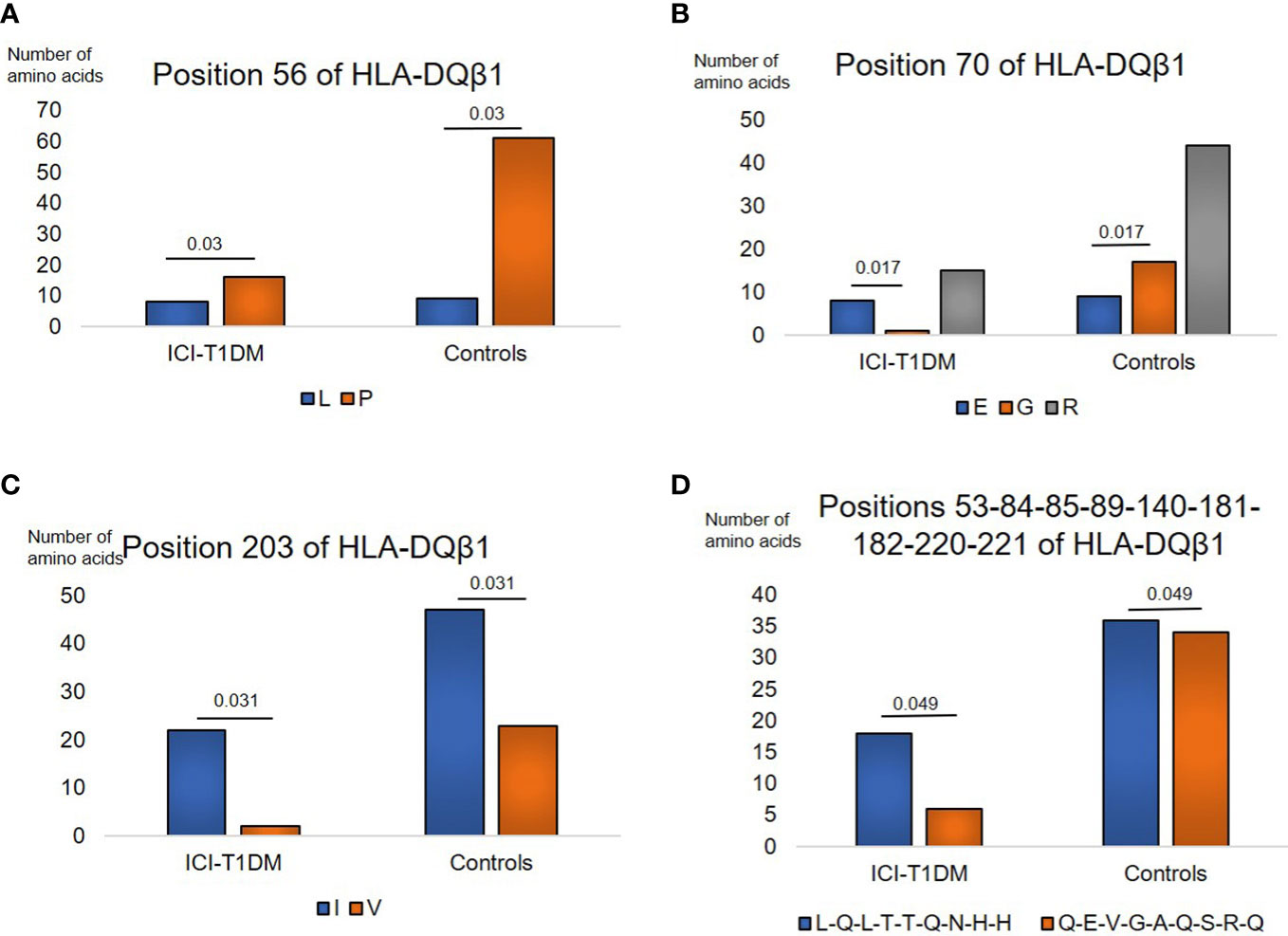
Figure 2 Similarly, prevalence of amino acid carriages at residues of HLA-DQB1 allele in patients with ICI-T1DM and ICI-controls (A–D). Prevalence of amino acid at position 56, L, Leucine vs P, Proline, P=0.03. OR 3.39, 95%CI: 1.13-10.18 (A), position 70, E, Glutamic acid vs G, Glycine, P=0.017. OR 15.11, 95%CI: 1.62-140.58 (B), position 203, I, Isoleucine vs V, Valine, P=0.031. OR 5.38, 95%CI: 1.16-24.89 (C), and positions 53-84-85-89-140-181-182-220-221, L-Q-L-T-T-Q-N-H-H, Leucine-Glutamine-Leucine-Threonine-Threonine-Glutamine-Asparagine-Histidine-Histidine vs Q-E-V-G-A-Q-S-R-Q, Glutamine-Glutamic acid-Valine-Glycine-Alanine-Glutamine-Serine-Arginine-Glutamine, P=0.049. OR 2.83, 95%CI: 1.01-7.98 (D) are shown. After Bonferroni correction for all significant amino acid polymorphisms among HLA-DQB1 alleles, no amino acid polymorphisms were significantly different.
Moreover, amino acids carriages at amino acid positions 35, 55, 205, and 84-85-86-87-96-170 (each amino acid in complete linkage disequilibrium) of HLA-DPβ1 were significantly different between the patients with ICI-T1DM and ICI-controls (Supplementary Tables 6A, B). L > Phe (F) at position 35 (Figure 3A), E > D at position 55 (Figure 3B), Met (M) > V at position 205 (Figure 3C), and Asp-Glu-Ala-Val-Lys-Ile (D-E-A-V-K-I) > Gly-Gly-Pro-Met-Arg-Thr (G-G-P-M-R-T) at positions 84-85-86-87-96-170 of HLA-DPβ1 in ICI-T1DM (Figure 3D).
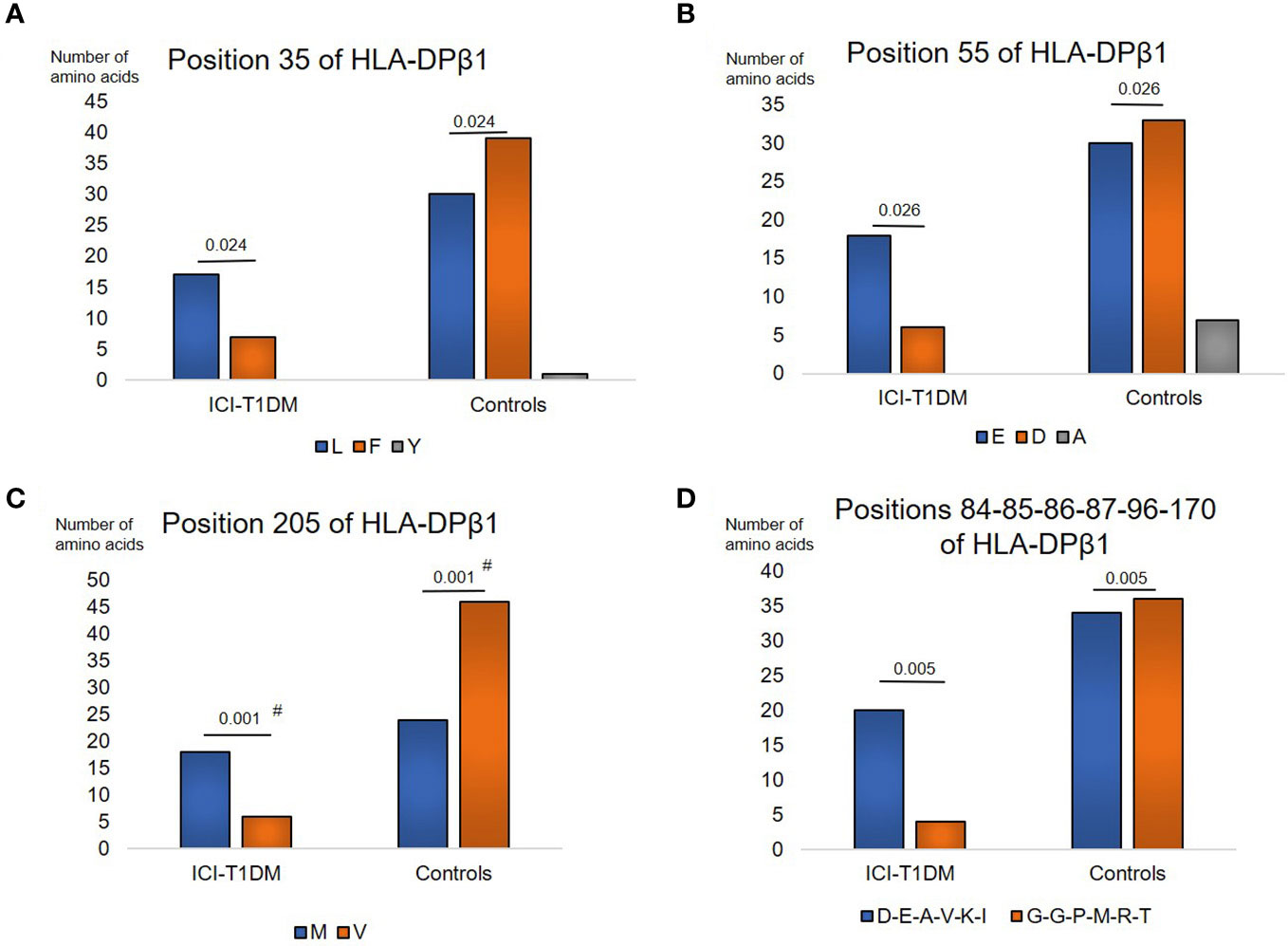
Figure 3 Then, prevalence of amino acid carriages at residues of HLA-DPB1 allele in patients with ICI-T1DM and ICI-controls (A–D). Prevalence of amino acid at position 35, L, Leucine vs F, Phenylalanine, P=0.024. OR 3.16, 95%CI: 1.16-8.59 (A), position 55, E, Glutamic acid vs D, Aspartic acid, P=0.026. OR 3.3, 95%CI: 1.16-9.41 (B), position 205, M, Methionine vs V, Valine, P=0.001. OR 5.75, 95%CI: 2.02-16.39 (C), and positions 84-85-86-87-96-170, D-E-A-V-K-I, Aspartic acid-Glutamic acid-Alanine-Valine-Lysine-Isoleucine vs G-G-P-M-R-T, Glycine-Glycine-Proline-Methionine-Arginine-Tryptophan, P=0.005. OR 5.29, 95%CI: 1.64-17.08 (D) are shown. OR, odds ratio; 95%CI, 95% confidence interval; Note that due to the statistical analyses employed, each P value was the same value in the respective figures. After Bonferroni correction for significant amino acid polymorphisms among HLA-DPB1 alleles, only amino acid position 205 on HLA-DP allele (C) were significantly different (Pc=0.011 after Bonferroni correction, shown with #).
After Bonferroni correction for all significant amino acid polymorphisms, β57 at HLA-DRβ1 (Pc=0.046 by Bonferroni correction, shown with #) (Figure 1B), and β205 at HLA-DPβ1 were significantly increased in patients with ICI-T1DM compared with ICI-controls (Pc=0.011 by Bonferroni correction, shown with #) (Figure 3C).
To investigate the importance of amino acid polymorphisms mentioned above in detail (Figures 1–3), a stepwise selection of covariate amino acid residues was applied in multivariate logistic analysis across the HLA-DR, DQ, and DP (Table 3). Subsequently, β205 at HLA-DPβ1 was found to be most significant among them.
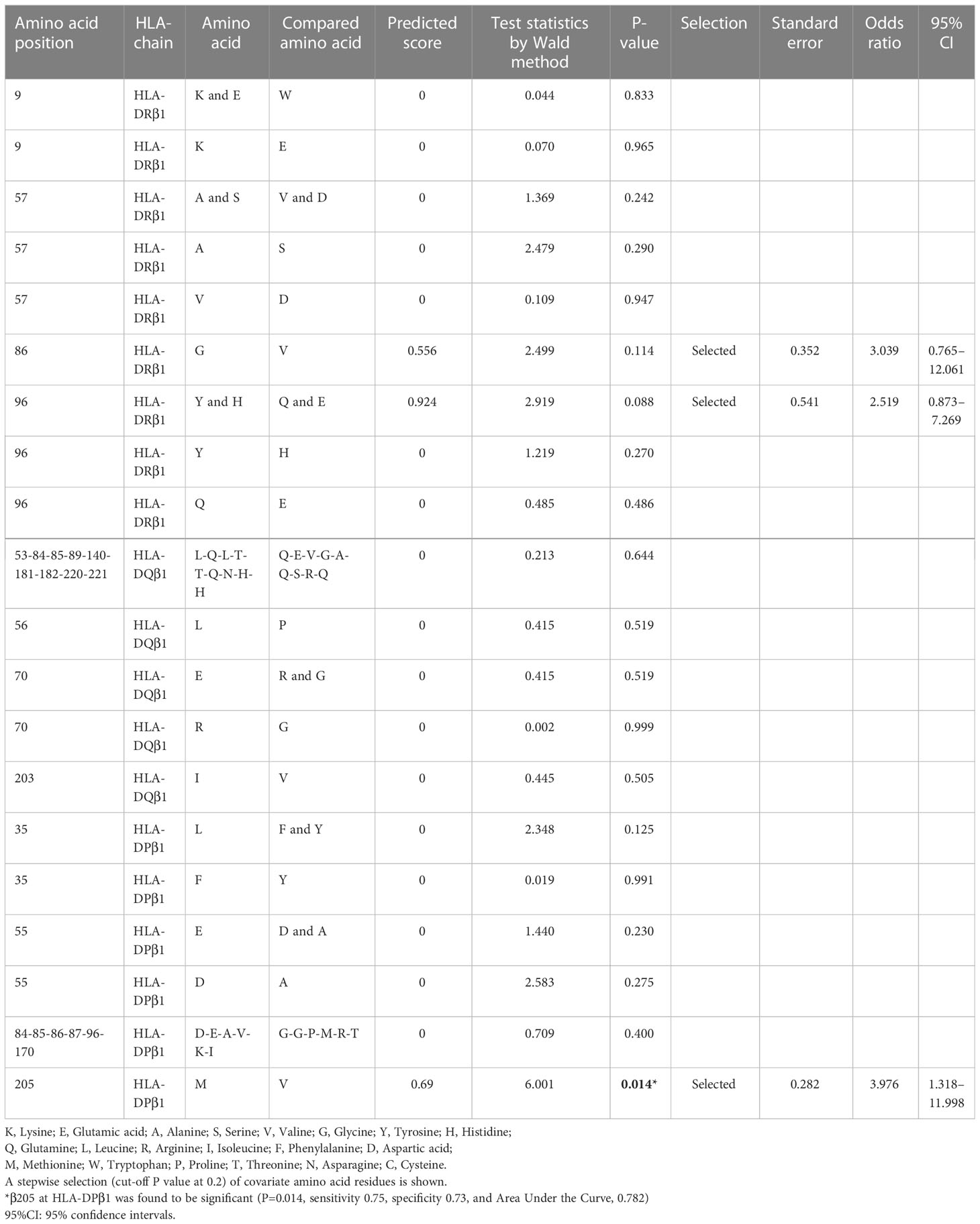
Table 3 Stepwise selection and multivariate regression analysis of amino acid polymorphisms across HLA-class II alleles in ICI-T1DM patients and ICI-controls.
Differences in amino acids at HLA-DPB1*05:01 and DPB1*15:01 were shown in Supplementary Table 3C and Supplementary Table 6C). Of those, amino acid at positions 85 and 86 compose pocket 1 (P1) of peptide-binding groove on the HLA molecule, and amino acid at position 9 composes P9 (Figure 4).
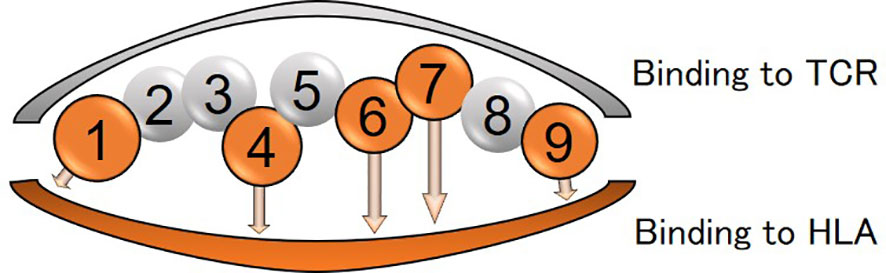
Figure 4 Peptide-binding grooves in an HLA class-II molecule (amino acid position 1-9) are shown. Amino acids in positions 1, 4, 6, 7, and 9 bind to HLA and those in positions 2, 3, 5, and 8 are assumed to be outward facing in order to stimulate the T-cell receptor (TCR).
Amino acid residues located in the nine peptide-binding grooves (referred to as pockets) were previously described (17, 18). Amino acids at positions 9 and 57 of HLA-DRβ1 compose P9, and the amino acid at position 86 composes P1 as well (Figures 1A, B, C, 4, 5A). The amino acid at position 70 of HLA-DQβ1 is associated with P4, and amino acids at positions 85 and 89 of HLA-DQβ1 compose P1 as well (Figures 2B, D, 4, 5B). Amino acids at positions 85 and 86 of HLA-DPβ1 compose P1 (Figure 3D, 4, 5C).
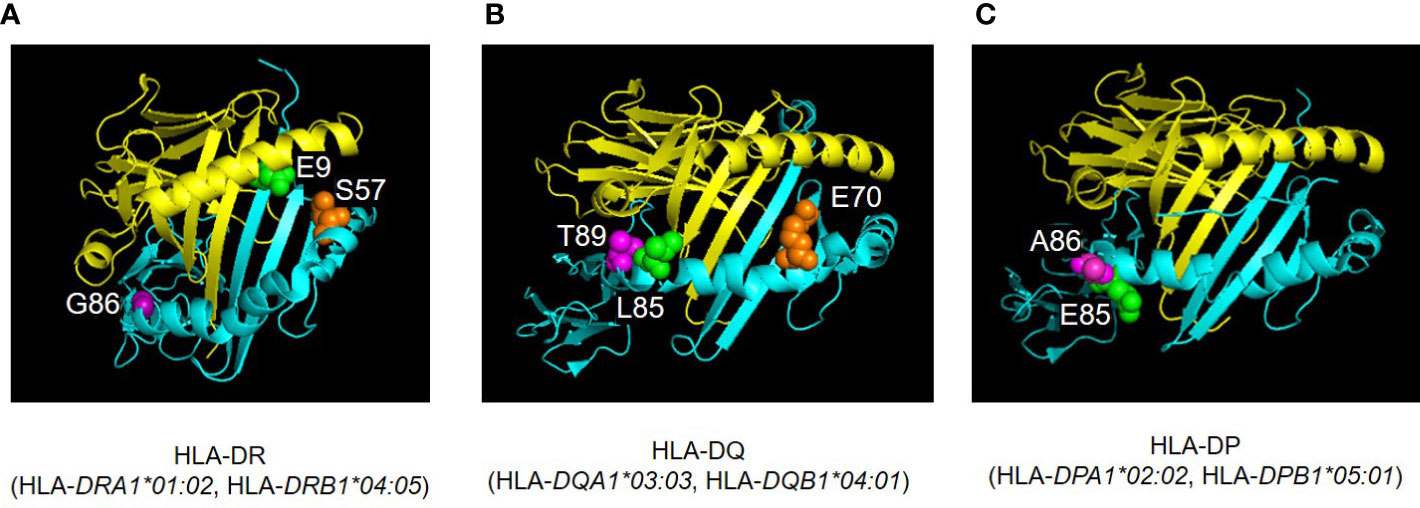
Figure 5 Three-dimensional illustration of ICI-T1DM risk-associated amino acid positions were identified in the current study. The crystal structures of HLA-DR (A), HLA-DQ (B), and HLA-DP (C) molecules are established based on Protein Data Bank entries 4IS6, 2NNA, and 3WEX, respectively. The structure of extracellular domains of HLA-class IIα and IIβ chains are shown in yellow and cyan, respectively. Amino acid polymorphic sites are shown as spheres. Amino acid position 9 (E, Glutamic acid, green) and 57 (S, Serine, orange) are located in pocket 9 of HLA-DR molecule (HLA-DRA1*01:02, HLA-DRB1*04:05) (A). Amino acid position 86 (G, Glycine, purple) composes pocket 1 (A). Amino acid position 70 (E, Glutamic acid, orange) is associated with both pocket 4 of HLA-DQ (HLA-DQA1*03:03, HLA-DQB1*04:01) (B). The HLA-DQβ1 amino acid positions 85 (L, Leucine, green) and 89 (T, Threonine, purple) compose pocket 1 of HLA-DQ molecule (B). Amino acid positions 85 (Glu, Glutamic acid, green) and 86 (A, Alanine, purple) compose pocket 1 of HLA-DP molecule (HLA-DPA1*02:02, HLA-DPB1*05:01) (C). Pocket: peptide-binding groove pocket.
Epitope predictions and in vitro binding of human proinsulin peptides to HLA-DP5 and HLA-DP15
Regarding binding of HLA-DP5 and human proinsulin, binding affinity of the signal peptide (AA 1-24) was predicted to be low (possessing high %RANK) (Table 4) (Figure 6A). In the remaining region (AA 25-110), two epitope candidate regions (AA 43-60 and AA 53-67) were predicted. Five peptides in the regions were strong binders. Other 13 peptides were predicted as intermediate binders. In addition to the 18 peptides, 16 overlapping peptides were synthesized to cover the whole portion. Subsequently, a total of 34 overlapping 15-mer human proinsulin-derived peptides were synthesized and subjected to in vitro peptide binding assay (Table 4). Besides, proinsulin epitope binding predictions to HLA-DP15 were similarly shown in Table 4. The proinsulin binding predictions to HLA-DR5 or to HLA-DP15 were different, but all portions in proinsulin including C-peptide region (AA57-87) showed binding predictions to both alleles.
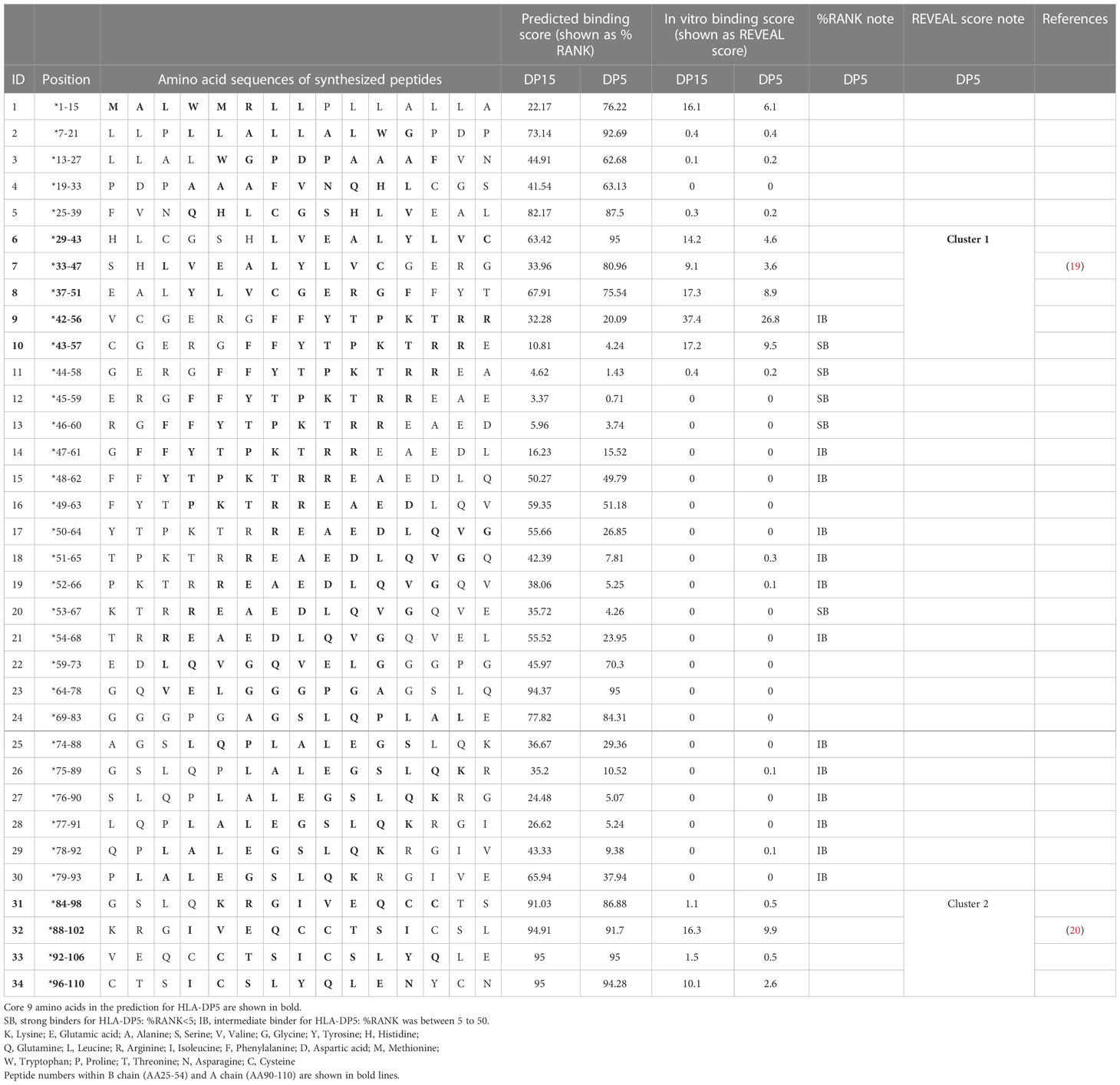
Table 4 Human proinsulin-derived peptides and their affinities to HLA-DP5 and HLA-DP15 in silico and in vitro.
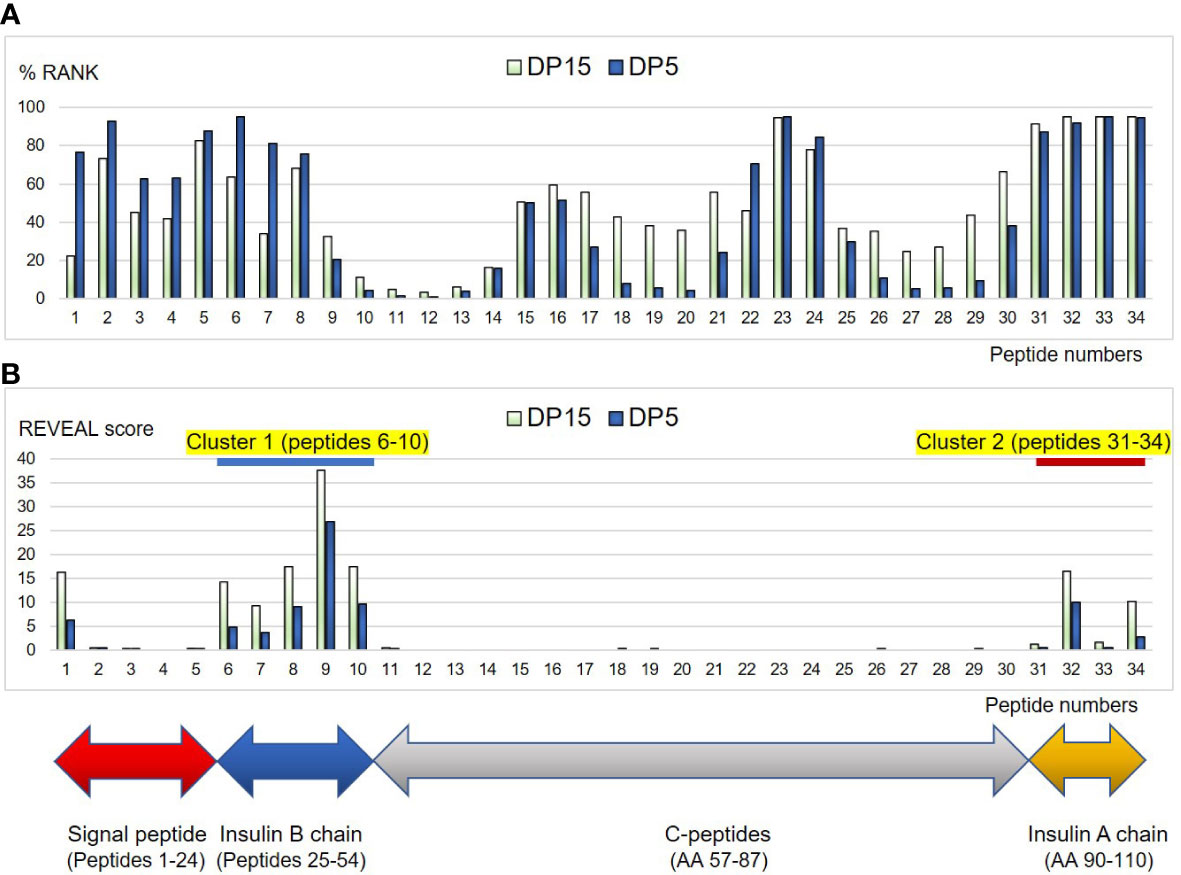
Figure 6 Binding affinities of the peptides derived from human proinsulin to HLA-DP5 (HLA-DPA1*02:02, HLA-DPB1*05:01) or HLA-DP15 (HLA-DPA1*02:02, HLA-DPB1*15:01) molecules are shown as %RANK in silico (A), and shown as REVEAL score in vitro (B). Horizontal numbers indicate peptide numbers used in the study (shown in Table 4). Note that lower %RANK means predicted strong binder in silico, and higher REVEAL score indicates peptide with high affinity in vitro. Cluster 1 (peptides 6-10) and Cluster 2 (peptide 31-34) were shown with blue and red line, respectively.
Then in vitro peptide binding assay was conducted, and remarkably, in the insulin B chain (AA25-54) and insulin A chain (AA90-110), two clusters were identified (peptides 6-10: AA 29-57 as cluster 1 and peptides 31-34: AA 84-110 as cluster 2) for HLA-DP5 and also for HLA-DP15 (Table 4) (Figure 6B). Moreover, peptides in the C-peptide region (AA57-87) bound to neither HLA-DP5 nor HLA-DP15.
Discussion
A total of 47 patients with malignancies who had been treated with ICI were subjected to HLA typing by next generation sequencing. T1DM developed in twelve and the remaining thirty-five served as controls. In allele and haplotype analyses, the patients had an increase of HLA-DRB1*04:05, DQB1*04:01, and in particular of DPB1*05:01. Indeed, 17/24 (71%) alleles were DPB1*05:01 among the patients compared with 24/70 (34%) in the controls. Moreover, significant amino acid polymorphisms at HLA-DR, DQ, and DP allele were identified that might contribute to the development of ICI-T1DM, probably with conformational alterations in the peptide-binding groove in each HLA-class II molecule. HLA-DP5 was found to be more strongly related to amino acid polymorphisms. As the peptide-binding groove dictate peptide binding, we scanned proinsulin in silico as a hypothetical autoantigen and novel human proinsulin epitope clusters in insulin B and A chains were discovered for HLA-DP in vitro. Our findings are promising suggestion of a possible association between HLA and ICI-T1DM through proinsulin peptide binding studies.
In comparison with our previous study (5), the current study was conducted on a larger scale with confirmation of HLA-DPB1*05:01 predominance in ICI-T1DM than those of general controls and ICI-controls with the same ethnic background. Current study may also support other reports describing that HLA-DR4 alleles are increased in patients with ICI-T1DM in the United States (21, 22), although these studies are not at the detailed allelic levels. Based on the conditional multiple regression analysis, in addition to HLA-DPB1*05:01 allele, alleles of HLA-DRB1*04:05 and DQB1*04:01, and HLA-DRB1*04:05-DQB1*04:01 haplotype were found to be susceptible to ICI-T1DM (Tables 2A–E) . HLA-DRB1*04:05-DQB1*04:01 haplotype and HLA-DRB1*04:05-DQB1*04:01-DPB1*05:01 haplotype predominance in ICI-T1DM also suggested that HLA-DP5 controls immune reaction of HLA-DR and DQ as mentioned below.
Then we disentangled amino acid polymorphisms at positions 9 and 57 (P9) and 86 (P1) of HLA-DRβ1, position 70 (P4 and P7) and positions 85 and 89 (P1) of HLA-DQβ1, and positions 85 and 86 (P1) of HLA-DPβ1 (Figures 5A–C). These amino acids contribute to the formation of the peptide-binding grooves on HLA-class II molecules, therefore may functionally contribute to the epitope presentations. Notably, patients with ICI-T1DM in Japan have been reported to have HLA-DRB1*11:01/*13:02 (23), DRB1*04:05 (24–26), DRB1*04:06 (26), and DRB1*09:01 (25), and all of patients above had G86 at HLA-DRβ1. Particularly, both HLA-DRB1*04:05 and DRB1*09:01 have G86 at HLA-DRβ1, and were reported to be associated with T1DM (27). Conversely, HLA-DRB1*15:01, a protective allele for T1DM has V86 at HLA-DRβ1 (27), and HLA-DRB1*15:01 was not seen in patients with ICI-T1DM in the current study. Therefore, G86 and V86 seemed to be disease-promotion and protection alleles, respectively, at HLA-DRβ1, in association with (P1). Todd, et al. reported strongly conserved Asp (D) at position 57 (P1, P9) of HLA-DQβ1 with disease susceptibility in patients with T1DM (28), and D at position 57 (P1, P9) of HLA-DRβ1 seemed to play a strong protective role in ICI-T1DM in the current study (Figure 5A). Then, stepwise selection and multivariate analysis revealed that β205 at HLA-DPβ1 was most important (Table 3). Therefore, we speculated the amino acid polymorphism β205 at HLA-DPβ, which locates outside of the peptide-binding groove in HLA-DPβ1, may be related to interactions such as HLA-DM, or to alterations in signal transduction within the HLA molecule.
Taken together, significances of HLA-DP5 in ICI-T1DM were observed. Next, we conducted human proinsulin peptide binding prediction to HLA-DP molecules to ensure effective epitope presentation in ICI-T1DM (Table 4) (Figure 6A). Predicted binding affinities and in vitro binding results were quite different for HLA-DP5, especially in the C-peptides region, for reasons unknown (Figures 6A, B). Remarkably, insulin B chain epitope (cluster 1): AA 29-57, and the insulin A chain epitope (cluster 2): AA 84-110 were established in vitro binding assay (Figure 6B). Among them, peptide 9 (AA 42-56), VCGERGFFYTPKTRR (core sequence underlined), was predicted as intermediate binders and also exhibited the strongest in vitro peptide binding, thus is mostly expected to be immunogenic T-cell epitope. Insulin peptide B9-23 (AA 33-47) has been reported to be a major autoantigen to induce immunity in the nonobese diabetic mouse, and is also included in the cluster 1 (19). Mannering et al. reported that cells transfected with HLA-DRB1*04:05 presented insulin A1–13 (AA 88-102) which is the identical to the peptide 32 (20). HLA-DP15 has not been reported as a risk allele in ICI-T1DM, but showed similar in vitro proinsulin epitopes to HLA-DP5 (cluster 1 and cluster 2) (Figure 6B). Despite the dissimilarities in amino acid sequence between HLA-DPB1*05:01 and HLA-DPB1*15:01, the importance of epitope cluster 1 and cluster 2 was reinforced across the alleles in silico and in vitro (Supplementary Table 6C).
In the development of ICI-T1DM, we hypothesized that the inhibition of immune-checkpoint molecules by ICI induced immunity to pancreatic β-cells, as observed in thyroid follicular epithelial cells during thyroid irAE (5, 29). Antibody-dependent cellular cytotoxicity by ICIs, by cytotoxic T-cells (30), or by both of them, would contribute to the development of ICI-T1DM. We speculate that ICI-T1DM-predisposing HLA may also be involved with malignant diseases. Proinsulin epitope, as well as tumor-associated antigen/neoantigen, could be bound to peptide-binding cleft of HLA, and cross-presented on the surface of antigen-presenting cells due to molecular mimicry (3, 4, 9). Another important topic is the correlation of ICI treatment effectivity and ICI-T1DM. Considering that 8/12 (75%) were ICI-responders in ICI-T1DM group and that generally only 20-30% are responders, common mechanisms between ICI treatment and ICI-T1DM were suggested. Therefore, HLA seemed to be associated with both ICI treatment outcome and risk for an endocrine adverse event. Considering that proinsulin is processing into insulin A chain and B chain, and that epitope clusters were identified in the two chains, evaluation of IAA in the time course may be of interest. As well, anti-GAD65 antibody titers in the course seem to be important.
This study has limitations. Firstly, we have tested proinsulin binding to HLA-DP. Binding studies for HLA-DR and HLA-DQ molecules are also desirable. Secondly, more evidence of immunogenicity of HLA-DP5 from in vitro and in vivo experiments in comparison with HLA-DR/DQ are preferable to confirm the peptide binding data in this study. Thirdly, better investigation of the frequency of HLA-DPB1*05:01, irrespective of ICI treatment is still desirable. Finally, the study consists of a small sample size, so more data with increased numbers may help to establish our results.
In conclusion, HLA-DP5 as a predisposition molecule, and significant amino acid polymorphisms at HLA-class II molecules in patients with ICI-T1DM were established in this study. Based on the silico and in vitro proinsulin peptide-binding study, conformational changes in the peptide-binding groove of the HLA-DP molecules may influence the immunogenicity of proinsulin epitopes in ICI-T1DM. These genetic factors may be utilized for prediction of ICI-T1DM, and also could contribute to elucidation of the mechanism of cancer immunotherapy and ICI-T1DM. Therefore, current study offers safer and more effective management and monitoring options for cancer immunotherapy. Further investigations are warranted to elucidate the relation of ICI- treatment effectiveness and development of ICI-T1DM.
Data availability statement
The datasets presented in this study can be found in online repositories. The names of the repository/repositories and accession number(s) can be found in the article/Supplementary Material.
Ethics statement
The studies involving human participants were reviewed and approved by Red Cross Society Wakayama Medical Center (JRCW), Wakayama Medical University Hospital (WMU), and Nagoya University (NU). The patients/participants provided their written informed consent to participate in this study. Written informed consent was obtained from the individual(s) for the publication of any potentially identifiable images or data included in this article.
Author contributions
HI: Conceptualization, designed the study. original draft preparation. SM: Visualization, writing- reviewing and editing. All authors performed the clinical studies statistically analyzed the data and wrote the manuscript. All authors approved the final version of the manuscript for publication. All authors contributed to the article and approved the submitted version.
Funding
This work was partially supported by the Takeda Science Foundation, the Japanese Foundation for Multidisciplinary Treatment of Cancer, Japan Diabetes Foundation, and the Grant-in-Aid for Scientific Research from the Ministry of Education, Science, Sports, and Culture, Japan (20K17541 and 21K08544).
Acknowledgments
We are indebted to Dr. Hidetoshi Inoko (Tokai University) for technical advice. We acknowledge proofreading and editing by Benjamin Phillis at the Clinical Study Support Center, Wakayama Medical University.
Conflict of interest
Author YO was employed by GenoDive Pharma Inc. Author HN was employed by Biospecimen Laboratories, Inc. Author SIw has received personal fees from Ono Pharmaceutical Co. Ltd., Bristol-Myers Squibb, Chugai Pharmaceutical Co., Ltd. and MSD K.K. outside of this study. Author HA received grants from Ono Pharmaceutical Co., Ltd., MSD K.K., and Chugai Pharmaceutical Co., Ltd., and personal fees from Ono Pharmaceutical Co., Ltd., Bristol– Myers Squibb and MSD K.K. outside of this study.
The remaining authors declare that the research was conducted in the absence of any commercial or financial relationships that could be construed as a potential conflict of interest.
Publisher’s note
All claims expressed in this article are solely those of the authors and do not necessarily represent those of their affiliated organizations, or those of the publisher, the editors and the reviewers. Any product that may be evaluated in this article, or claim that may be made by its manufacturer, is not guaranteed or endorsed by the publisher.
Supplementary material
The Supplementary Material for this article can be found online at: https://www.frontiersin.org/articles/10.3389/fimmu.2023.1165004/full#supplementary-material
References
1. Arima H, Iwama S, Inaba H, Ariyasu H, Makita N, Otsuki M, et al. Management of immune-related adverse events in endocrine organs induced by immune checkpoint inhibitors: clinical guidelines of the Japan endocrine society. Endocr J (2019) 66(7):581–6. doi: 10.1507/endocrj.EJ19-0163
2. Inaba H, Ariyasu H, Takeshima K, Iwakura H, Akamizu T. Comprehensive research on thyroid diseases associated with autoimmunity: autoimmune thyroid diseases, thyroid diseases during immune-checkpoint inhibitors therapy, and immunoglobulin-G4-associated thyroid diseases. Endocr J (2019) 66(10):843–52. doi: 10.1507/endocrj.EJ19-0234
3. Inaba H, Ariyasu H, Iwakura H, Ueda Y, Kurimoto C, Takeshima K, et al. Comparative analysis of HLA between idiopathic and anti-PD-1 antibody induced isolated ACTH deficiency: A pilot study. Clin Endocrinol (Oxf) (2019) 91(6):786–92. doi: 10.1111/cen.14082
4. Inaba H, Ariyasu H, Iwakura H, Kurimoto C, Takeshima K, Morita S, et al. Distinct clinical features and prognosis between persistent and temporary thyroid dysfunctions by immune-checkpoint inhibitors. Endocr J (2021) 68(2):231–41. doi: 10.1507/endocrj.EJ20-0371
5. Inaba H, Kaido Y, Ito S, Hirobata T, Inoue G, Sugita T, et al. Human leukocyte antigens and biomarkers in type 1 diabetes mellitus induced by immune-checkpoint inhibitors. Endocrinol Metab (Seoul) (2022) 37(1):84–95. doi: 10.3803/EnM.2021.1282
6. Qiu J, Luo S, Yin W, Guo K, Xiang Y, Li X, et al. Characterization of immune checkpoint inhibitor-associated fulminant type 1 diabetes associated with autoantibody status and ethnic origin. Front Immunol (2022) 13:968798. doi: 10.3389/fimmu.2022.968798
7. Kemmler W, Peterson JD, Rubenstein AH, Steiner DF. On the biosynthesis, intracellular transport and mechanism of conversion of proinsulin to insulin and c-peptide. Diabetes (1972) 21(2 Suppl):572–81. doi: 10.2337/diab.21.2.S572
8. Inaba H, Martin W, De Groot AS, Qin S, De Groot LJ. Thyrotropin receptor epitopes and their relation to histocompatibility leukocyte antigen-DR molecules in graves' disease. J Clin Endocrinol Metab (2006) 91(6):2286–94. doi: 10.1210/jc.2005-2537
9. Inaba H, Martin W, Ardito M, De Groot AS, De Groot LJ. The role of glutamic or aspartic acid in position four of the epitope binding motif and thyrotropin receptor-extracellular domain epitope selection in graves' disease. J Clin Endocrinol Metab 95 (2010) 6):2909–16. doi: 10.1210/jc.2009-2393
10. Kobayashi T, Iwama S, Sugiyama D, Yasuda Y, Okuji T, Ito M, et al. Anti-pituitary antibodies and susceptible human leukocyte antigen alleles as predictive biomarkers for pituitary dysfunction induced by immune checkpoint inhibitors. J Immunother Cancer (2021) 9(5):e002493. doi: 10.1136/jitc-2021-002493
11. Kawasaki E, Maruyama T, Imagawa A, Awata T, Ikegami H, Uchigata Y, et al. Diagnostic criteria for acute-onset type 1 diabetes mellitus: Report of the committee of Japan diabetes society on the research of fulminant and acute-onset type 1 diabetes mellitus. J Diabetes Investig (2014) 5(1):115–8. doi: 10.1111/jdi.12119
12. Imanishi T, Akaza T, Kimura A, Tokunaga K, Gojobori T. Allele and haplotype frequencies for HLA and complement loci in various ethnic groups. In: Tsuji K, Aizawa M, Sasazuki T, editors. HLA 1991: Proceedings of the eleventh international histocompatibility workshop and conference. Oxford, UK: Oxford University Press (1992). p. 1065–220.
13. HLA LABORATORY, Japan INC . Available at: http://hla.or.jp/med/frequency_search/ja/haplo/ (Accessed October 10, 2022).
14. Japanese Society for Histocompatibility and Immunogenetics. Available at: http://jshi.umin.ac.jp/standarization/JSHI-hyokiallele-2022list.pdf/ (Accessed October 10, 2022).
15. wwPDB consortium. Protein data bank: the single global archive for 3D macromolecular structure data. Nucleic Acids Res (2019) 47(D1):D520–8. doi: 10.1093/nar/gky949
16. DeLano WL. The PyMOL molecular graphics system. San Carlos (CA: DeLano Scientific, San Carlos (2002).
17. Sarri CA, Giannoulis T, Moutou KA, Mamuris Z. HLA class II peptide-binding-region analysis reveals funneling of polymorphism in action. Immunol Lett (2021) 238:75–95. doi: 10.1016/j.imlet.2021.07.005
18. Bondinas GP, Moustakas AK, Papadopoulos GK. The spectrum of HLA-DQ and HLA-DR alleles, 2006: a listing correlating sequence and structure with function. Immunogenetics (2007) 59(7):539–53. doi: 10.1007/s00251-007-0224-8
19. Moriyama H, Abiru N, Paronen J, Sikora K, Liu E, Miao D, et al. Evidence for a primary islet autoantigen (preproinsulin 1) for insulitis and diabetes in the nonobese diabetic mouse. Proc Natl Acad Sci U.S.A. (2003) 100(18):10376–81. doi: 10.1073/pnas.1834450100
20. Mannering SI, Harrison LC, Williamson NA, Morris JS, Thearle DJ, Jensen KP, et al. The insulin a-chain epitope recognized by human T cells is posttranslationally modified. J Exp Med (2005) 202(9):1191–7. doi: 10.1084/jem.20051251
21. Stamatouli AM, Quandt Z, Perdigoto AL, Clark PL, Kluger H, Weiss SA, et al. Collateral damage: Insulin-dependent diabetes induced with checkpoint inhibitors. Diabetes (2018) 67(8):1471–80. doi: 10.2337/dbi18-0002
22. Akturk HK, Couts KL, Baschal EE, Karakus KE, Van Gulick RJ, Turner JA, et al. Analysis of human leukocyte antigen DR alleles, immune-related adverse events, and survival associated with immune checkpoint inhibitor use among patients with advanced malignant melanoma. JAMA Netw Open (2022) 5(12):e2246400. doi: 10.1001/jamanetworkopen.2022.46400
23. Miyoshi Y, Ogawa O, Oyama Y. Nivolumab, an anti-programmed cell death-1 antibody, induces fulminant type 1 diabetes. Tohoku J Exp Med (2016) 239(2):155–8. doi: 10.1620/tjem.239.155
24. Okamoto M, Okamoto M, Gotoh K, Masaki T, Ozeki Y, Ando H, et al. Fulminant type 1 diabetes mellitus with anti-programmed cell death-1 therapy. J Diabetes Investig (2016) 7(6):915–8. doi: 10.1111/jdi.12531
25. Usui Y, Udagawa H, Matsumoto S, Imai K, Ohashi K, Ishibashi M, et al. Association of serum anti-GAD antibody and HLA haplotypes with type 1 diabetes mellitus triggered by nivolumab in patients with non-small cell lung cancer. J Thorac Oncol (2017) 12(5):e41–3. doi: 10.1016/j.jtho.2016.12.015
26. Ishikawa K, Shono-Saito T, Yamate T, Kai Y, Sakai T, Shimizu F, et al. A case of fulminant type 1 diabetes mellitus, with a precipitous decrease in pancreatic volume, induced by nivolumab for malignant melanoma: analysis of HLA and CTLA-4 polymorphisms. Eur J Dermatol (2017) 27(2):184–5. doi: 10.1684/ejd.2016.2923
27. Kawabata Y, Ikegami H. Genetics of fulminant type 1 diabetes. Diabetol Int (2020) 11(4):315–22. doi: 10.1007/s13340-020-00468-0
28. Todd JA, Bell JI, McDevitt HO. HLA-DQ beta gene contributes to susceptibility and resistance to insulin-dependent diabetes mellitus. Nature (1987) 329(6140):599–604. doi: 10.1038/329599a0
29. Iwama S, Kobayashi T, Yasuda Y, Arima H. Immune checkpoint inhibitor-related thyroid dysfunction. Best Pract Res Clin Endocrinol Metab (2022) 36(3):101660. doi: 10.1210/clinem/dgab829
Keywords: human histocompatibility leukocyte antigen, immune-checkpoint inhibitors, immune-related adverse events, type 1 diabetes mellitus, proinsulin
Citation: Inaba H, Morita S, Kosugi D, Asai Y, Kaido Y, Ito S, Hirobata T, Inoue G, Yamamoto Y, Jinnin M, Kimura H, Ota M, Okudaira Y, Nakatani H, Kobayashi T, Iwama S, Arima H and Matsuoka T (2023) Amino acid polymorphisms in human histocompatibility leukocyte antigen class II and proinsulin epitope have impacts on type 1 diabetes mellitus induced by immune-checkpoint inhibitors. Front. Immunol. 14:1165004. doi: 10.3389/fimmu.2023.1165004
Received: 13 February 2023; Accepted: 24 March 2023;
Published: 06 April 2023.
Edited by:
Takaji Matsutani, Repertoire Genesis, Inc., JapanReviewed by:
Hester Doyle, Yale University, United StatesErin E. Baschal, University of Colorado Anschutz Medical Campus, United States
Copyright © 2023 Inaba, Morita, Kosugi, Asai, Kaido, Ito, Hirobata, Inoue, Yamamoto, Jinnin, Kimura, Ota, Okudaira, Nakatani, Kobayashi, Iwama, Arima and Matsuoka. This is an open-access article distributed under the terms of the Creative Commons Attribution License (CC BY). The use, distribution or reproduction in other forums is permitted, provided the original author(s) and the copyright owner(s) are credited and that the original publication in this journal is cited, in accordance with accepted academic practice. No use, distribution or reproduction is permitted which does not comply with these terms.
*Correspondence: Hidefumi Inaba, inaba@wakayama-med.ac.jp