- Longhua Hospital, Shanghai University of Traditional Chinese Medicine, Shanghai, China
Human epidermal growth factor receptor 2 (HER2)-positive breast cancer has a high metastatic potential. Monoclonal antibodies (mAbs) that target HER2, such as trastuzumab and pertuzumab, are the cornerstone of adjuvant therapy for HER2-positive breast cancer. A growing body of preclinical and clinical evidence points to the importance of innate immunity mediated by antibody-dependent cellular cytotoxicity (ADCC) in the clinical effect of mAbs on the resulting anti-tumor response. In this review, we provide an overview of the role of natural killer (NK) cells and ADCC in targeted therapy of HER2-positive breast cancer, including the biological functions of NK cells and the role of NK cells and ADCC in anti-HER2 targeted drugs. We then discuss regulatory mechanisms and recent strategies to leverage our knowledge of NK cells and ADCC as an immunotherapy approach for HER2-positive breast cancer.
Introduction
Human epidermal growth factor receptor 2 (HER2)-positive breast cancer accounts for 15%-20% of all types of breast cancer (1), and it is characterized by a high recurrence rate and poor prognosis (2). The targeted drugs for HER2-positive breast cancer include trastuzumab (3), pertuzumab (4), and trastuzumab emtansine (T-DM1) (5), which are the standard first- and second-line drugs. In addition, highly promising targeted drugs, such as magrolimab (6) and margetuximab (7), have significantly improved the survival rates of HER2-positive breast cancer patients. These monoclonal antibodies (mAbs) bind via the fragment crystalline (Fc) of immunoglobulin G1 (IgG1) to the Fcγ receptor III (FcγRIII) (CD16) on natural killer (NK) cells and elicit the release of cytotoxic factors, in a process known as antibody-dependent cell-mediated cytotoxicity (ADCC).
Immunotherapy has been established as a pillar of cancer treatment. In phase-I trial, involving 31 patients with refractory HER2-positive breast cancer, infusion with expanded activated autologous NK cells enhanced trastuzumab-mediated ADCC and produced a potent killing effect on breast cancer (8). The ADCC effect is an important mechanism in mAbs of HER2-positive breast cancer (9). ADCC has received accumulating attention given its substantial contribution to the therapeutic efficacy of mAbs. Understanding the role of ADCC in the immune response to mAbs will allow us to rationally combine these types of treatments in the context of HER2-positive breast cancer. In this paper, we summarize the advances of NK cells, ADCC effect, and corresponding research strategies in targeted therapy for HER2-positive breast cancer.
NK cells biology
Origin and ontogeny
NK cells are innate lymphoid-like cells that trigger an immune response to eliminate tumors through the secretion of cytokines and lytic granules (10). The development and maturation of NK cells constitute a stepwise process that can be divided into five stages (11). In the first stage, progenitors retain CD34 expression and acquire CD45RA and CD10; the second stage is marked by the absence of CD10 expression and the acquisition of CD117; the third stage is marked by the down-regulation of CD34 expression and the acquisition of lymphocyte function-associated antigen (LFA-1). The fourth and fifth stages involve mature NK cells, which include CD56bright CD16dim and CD56dim CD16bright subpopulations, respectively (12).
Mature NK cells migrate to the circulation and periphery to perform their functions. The CD56bright CD16dim subpopulation mainly distributes in peripheral tissues and secretes chemokines and cytokines (13). The CD56dim CD16bright subpopulation exerts its cytotoxic activity by secreting perforin and granzyme A/B (14). Except the circulating NK cells, tissue-resident NK subpopulations are present in various organs (15). In the tumor microenvironment (TME), tumor-associated fibroblasts and tumor-induced immunosuppressive cells also regulate NK cells and their anti-tumor activity (16).
Function
NK cells play a key role in defending against tumor initiation and metastasis as the first line of immunity. NK cells are considered the most promising tumor-killing effector cells other than T cells because they are not limited by the major histocompatibility complex (MHC) (17). NK cells have a broad spectrum of tumor-killing effects, which include ADCC, release of perforin and granzyme A/B, factor-associated suicide (Fas) and Fas ligand (FasL) interaction, and cytokine secretion (18).
FcγRs
FcγRs are expressed on NK cells, and they include FcγR I (CD64), FcγR IIa (CD32A), FcγR IIb (CD32B), FcγR IIc (CD32C), FcγR IIIa (CD16), and FcγR IIIb (CD16B). Low-affinity FcγRs are important mediators of ADCC function, and include two activating receptors, namely, FcγR IIIa and FcγR IIa, and the sole inhibitory receptor FcγR IIb (19). The ADCC activity of mAbs is largely dependent on the CD16 expressed by NK cells (20).
NK cell-mediated ADCC in targeted drugs of HER2-positive breast cancer
Trastuzumab
Trastuzumab is a humanized IgG-type mAb that specifically binds to the extracellular segment IV of the HER2 receptor (21), it blocks the formation of homodimers between HER2 and other HER family members (22). The immune system plays a key role in the anti-tumor effect of trastuzumab, which occurs through the binding of the HER2 receptor by the fragment antigen binding (Fab) and Fc to the CD16 of NK cells (23).
In HER2-positive breast cancer mice, tumor growth was almost completely inhibited by trastuzumab treatment (13/17); however, the tumor-suppressive capability of trastuzumab was significantly reduced in hormonal mice lacking FcγR III (1/15). Mice lacking FcγR IIB exhibited more ADCC; by contrast, trastuzumab failed to prevent tumor growth in mice lacking FcγR IIIa. These results suggest the significant contribution of FcγR IIIa-dependent mechanisms to the anti-tumor effects of cytotoxic antibodies (24). Duong MN observed that adipocytes promoted resistance to trastuzumab by secreting extracellular matrix components that formed a physical barrier between the tumor, antibodies, and immune cells. The physical barrier inhibited the lysis of NK cells, which resulted in the reduced sensitivity to trastuzumab-mediated ADCC in HER2-positive breast cancer (25).
NK cells are the main immune cell type that exerts the effects of ADCC (26). Arnould L (27) showed that patients with an objective response to trastuzumab had increased leukocyte infiltration and ADCC activity. Varchetta S (28) reported that the ADCC effect of trastuzumab was positively correlated with pathological remission rates in HER2-positive breast cancer patients, which suggested that enhancing the ADCC effect can optimize the efficacy of trastuzumab. Beano A conducted a clinical observation of 26 patients with metastatic HER2-positive breast cancer treated with trastuzumab. After six months of treatment, 17 patients were rated as responders and 9 as non-responders, in accordance with the response evaluation criteria in solid tumors. The results revealed that NK cell and ADCC activities were significantly higher in responders, and non-responders showed a lower NK cell activity. In addition, progression-free survival (PFS) significantly increased in patients with high levels of NK cell activity (29).
Pertuzumab
Pertuzumab is a humanized IgG-type mAb that complements trastuzumab by blocking the heterodimeric activation pattern of HER2. Piccart M reported that the addition of pertuzumab to standard adjuvant therapy prolonged invasive disease-free survival of HER2-positive breast cancer patients with positive axillary lymph nodes (30). In the latest Chinese Society of Clinical Oncology guideline, the treatment of trastuzumab in combination with pertuzumab was recommended as the first-line option in targeted therapy. Both trastuzumab and pertuzumab exert anti-HER2 effect by inhibiting HER2 signaling and inducing ADCC activity (31, 32). Diessner J (33) discovered that tumor cell killing via ADCC increased when the triple combination of trastuzumab, pertuzumab, and NK cells was applied to HER2-positive breast cancer cells compared with the extent of ADCC induced by a single antibody. This study demonstrated the immunotherapeutic benefit achieved by the combined application of trastuzumab and pertuzumab.
T-DM1
T-DM1 is an antibody-drug conjugate (ADC) with two core functional components: trastuzumab and a potent chemotherapeutic drug. Similar to a nuclear missile, ADC drugs combine the two components through a special linker to achieve a precise tumor-killing effect. T-DM1 has been approved by the United States Food and Drug Administration (FDA) for the treatment of HER2-positive metastatic breast cancer (34). HER2-DC1 comprises intratumoral multiepitope MHC class II HER2 peptide-pulsed type I polarized dendritic cells. Ramamoorthi G observed that HER2-DC1 combined with T-DM1 increased the levels of tumor-infiltrating CD4 and CD8 T, B, natural killer T, and NK cells in HER2 breast cancer-bearing mice and promoted complete tumor regression (35). ADCC effects of T-DM1 have been reported in other HER2-overexpressing cancers, such as lung and ovarian cancers. The activity of T-DM1 is superior to those of trastuzumab, pertuzumab, and their combination in high HER2/neu-expressing epithelial ovarian cancer (36). In HER2-overexpressing lung cancer cell lines, T-DM1 intervention overcame gefitinib resistance, and its ADCC effect was similar to that of trastuzumab in the presence of interleukin (IL)-2 activated NK cells (37).
Margetuximab
As an Fc-modified chimeric mAb, margetuximab was designed to increase binding to CD16 and decrease binding to inhibitory FcγRIIB (CD32B), which resulted in the increased activation of ADCC and NK cells (38). In 2020, margetuximab has been approved in combination with chemotherapy for the treatment of patients with HER2-positive metastatic breast cancer (39). Rugo HS reported that margetuximab plus chemotherapy for HER2-positive advanced breast cancer had an acceptable safety and statistical improvement in PFS compared with trastuzumab plus chemotherapy (40). In addition, the safety and synergistic effects of margetuximab plus pembrolizumab were demonstrated in a single-arm IB-2 trial involving HER2-positive gastric cancer (41). These studies demonstrated that Fc-optimized anti-HER2 agents have desirable clinical futures.
Small-molecule tyrosine kinase inhibitors
Small-molecule TKIs can inhibit kinase activity and downstream signaling by targeting the intracellular structural domain of HER2. Studies revealed that TKIs can modulate mAb-mediated ADCC responses. Lapatinib significantly increased membrane HER2 levels, and the combination with trastuzumab provided the largest ADCC response in HER2-low breast cancer models compared with afatinib and neratinib (42). Okita R (43) stated that malignant mesothelioma cells (MPM) pretreated with lapatinib bound more trastuzumab than untreated cells, which suggests that combinations of lapatinib and trastuzumab may be a promising strategy for MPM treatment. Cavazzoni A (44) explored erlotinib in combination with cetuximab or trastuzumab, and a significant effect on ADCC and inhibition of tumor growth were observed in the treatment of non-small-cell lung cancer (NSCLC).
Regulatory mechanisms and research strategies of NK cells and ADCC
As previously mentioned, NK cell-mediated ADCC plays an important role in anti-HER2 therapy (45). However, the cytotoxicity of NK cells decreases with the altered activation receptor phenotype in breast cancer patients. Compared with healthy donors, NK cells express lower levels of NK cell p30-related protein (NKp30), NKp46, and NK cell group 2 member D (NKG2D) in breast cancer patients (46). Therefore, the enhancement of NK cells and their ADCC effect is an effective way to improve the efficacy and sensitivity of trastuzumab (47).
The regulation of the effect on NK cells and ADCC is maintained by a dynamic balance between activating and inhibiting receptors (48). Figure 1 summarizes the regulatory mechanisms of NK cells and ADCC. The activation of NK cells is tightly regulated by the balance of signals from inhibitory and activating receptors, which is controlled by multiple lineage-encoded receptors that enable these cells to sense and respond rapidly to changes in their environment (49, 50). N-Glycosylation of FcγRIII also affects receptor interactions with IgG, which has led to studies of antibody engineering with greatly improved ADCC activities (51). Recombinant cytokines, agonists that promote activation receptors, and antibodies that block inhibitory receptors can be used to enhance ADCC (52, 53). Based on the regulatory mechanism of NK cells and ADCC, we summarized relevant research strategies, including targeted activation and inhibitory receptors, cytokine therapy, antibody engineering, and chimeric antigen receptor (CAR)-NK cells.
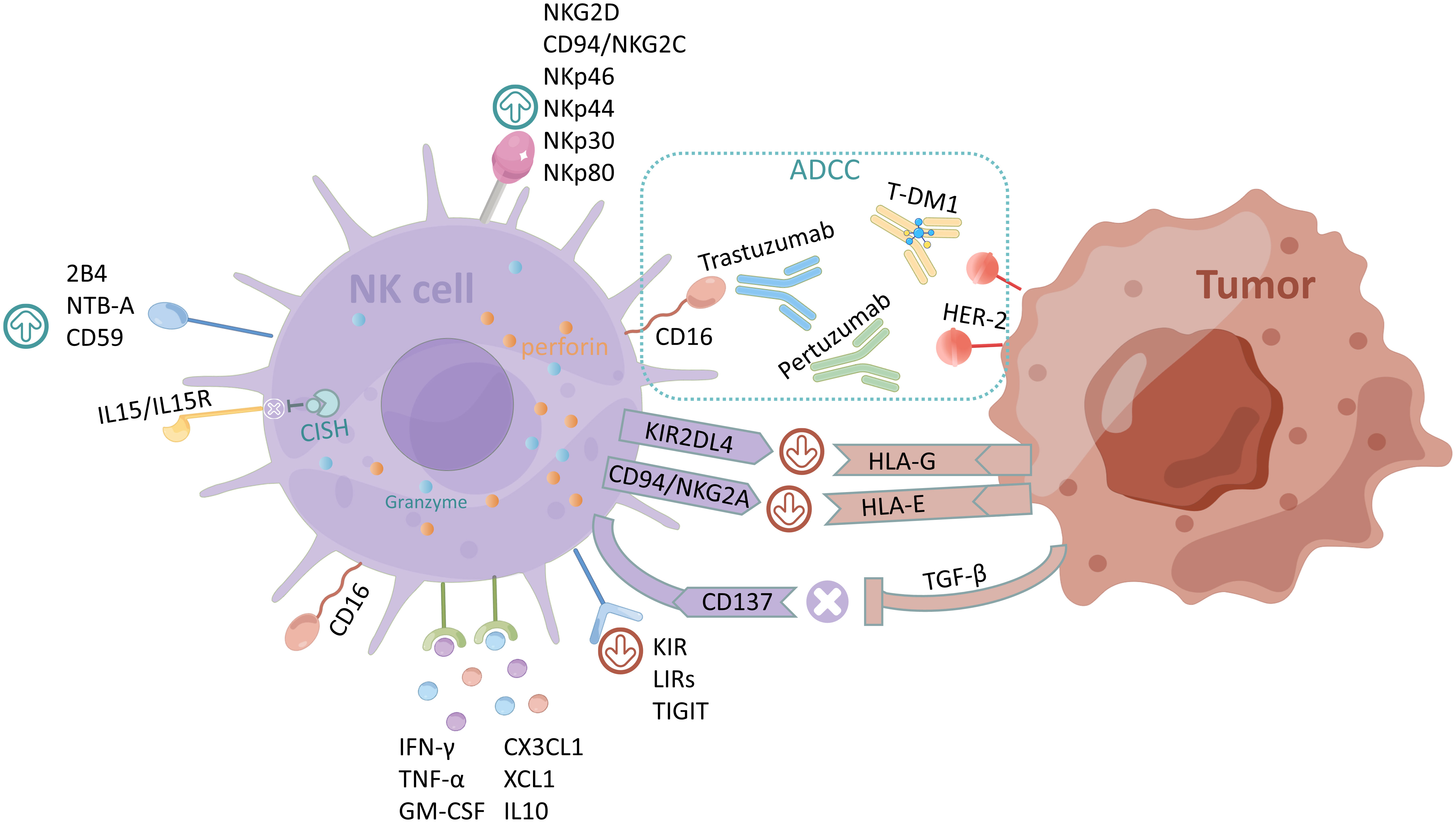
Figure 1 Regulatory mechanisms of NK cells and ADCC. The activating receptors of NK cells are NKG2D, CD94/NKG2C, NKp46, NKp44, NKp30, recombinant NK cell receptor 2B4 (NKR2B4, 2B4), NKp80, NTB-A, and CD59, and the inhibiting receptors are KIR, LIRs, and TIGIT. CISH normally inhibits IL-15/IL15R signaling in NK cells. The main cytokines secreted by NK cells are CX3CL1, chemokine (C motif) ligand 1 (XCL1), IL10, IFN-γ, TNF-α, and GM-CSF. HLA-G and HLA-E expressed by tumor cells bind to inhibitory receptors KIR2DL4 and CD94/NKG2A of NK cells respectively to exert immunosuppressive effects; CD137 counteracts the immunosuppression caused by the overexpression of TGF-β by tumor cells. The figure was drawn by Figdraw.
Targeted activation receptors
NKG2D is a C-type lectin surface receptor, and binding to its ligand mediates the immune response of NK cells to tumors (54). The specific binding of antibodies to NKG2D and HER2 increases the cytotoxicity of peripheral blood mononuclear cells, thus significantly enhancing the killing activity of CAR-NK cells against the HER2-positive primary trastuzumab-resistant cell line JIMT-1 (55). Natural cytotoxicity receptors (NCRs) are prominent among activating NK cell receptors, and they are notably the only NK-activating receptors that can recognize pathogen-derived ligands. NCRs include the most specific NK cell markers, namely, NKp46 (NCR1, NCTR1, and CD335), NKp44 (NCR2, NCTR2, and CD336), and NKp30 (NCR3, NCTR3, and CD337), all three being members of the immunoglobulin superfamily (56).
NKp80 is a homodimeric C-type lectin-like receptor linked to the NKp80 locus gene, which is preferentially expressed by myeloid cells, thus promoting NK and myeloid intercellular crosstalk (57). Peipp M fused the extracellular structural domains of the ligands of NKp30 and NKp80 with single-chain fragment variables (scFv) targeting HER2 (named B7-H6:HER2-scFv and AICL:HER2-scFv, respectively). The results showed that antibody-derived proteins involved in NKp30 or NKp80 triggered NK cells to kill HER2-positive breast cancer. Moreover, the cytotoxicity of NK cells was synergistically enhanced when combined with the HER2-specific immune ligands of NKG2D (58). In addition, B7-H6:HER2-scFv and AICL:HER2-scFv synergistically enhanced the ADCC of the therapeutic antibodies trastuzumab and cetuximab, respectively. Kellner C constructed bispecific immunoligand UL16 binding protein 2 (ULBP2):HER2-scFvm, which can promote NK cell cytotoxicity against tumors and enhance ADCC in combination with cetuximab (59). The anti-CD137 agonist urelumab can overcome transforming growth factor (TGF)-β-mediated inhibition of human NK-cell proliferation and anti-tumor function and preserve the expressions of NKG2D, granzyme B, and interferon-gamma (IFN-γ) in HER2-positive primary breast cancer (60). Combined treatment with NKG2D agonist, 4-1BB antibody, and IL-27 improved activator receptor expression in NK cells and promoted the secretion of IFN-γ and tumor necrosis factor (TNF-α) while reducing the expression of the inhibitory receptor CD158a and the secretion of IL-10 in NK cells (61).
Targeted inhibitory receptors
NK cells detect the absence of self-molecules on potential target cells through their inhibitory receptors, which is known as a recognition strategy of “loss of self.” The inhibitory receptors form three families, namely, killer cell immunoglobulin-like receptors (KIRs), leukocyte immunoglobulin-like receptors (LIRs), and NKG2A. KIRs and LIRs are members of the immunoglobulin superfamily and type I transmembrane molecules that recognize human leukocyte antigen (HLA) A, B, and C (HLA-I class a). LIRs mainly recognize non-classical HLA-G (class I b) molecules. NKG2A is a member of the NKG2 family, which belongs to the C-type lectin family of receptors and recognizes non-classical HLA-E class I molecules. KIRs/NKG2A plays a major role in mediating inhibitory signaling by binding to HLA (58).
Experimental and clinical studies on targeting inhibitory receptors are gradually being conducted. HLA-G can desensitize breast cancer cells to trastuzumab by binding to KIR2DL4, an atypical member of the KIR family, which responds to HLA-G via endosomal signaling (62). In HER2-positive breast cancer, blocking HLA-G/KIR2DL4 signaling improved trastuzumab resistance (63).
Monalizumab, a humanized anti-NKG2A mAb, is currently undergoing clinical trials in a variety of solid tumors (64). Frazao A showed that NK cells from tumor-draining lymph nodes expressed high NKG2A and checkpoint programmed cell death protein 1 (PD-1), which supported their potential as targets for immunotherapy using anti-NKG2A and/or anti-PD-1 in breast cancer (65). Phase II clinical trials suggest that monalizumab can enhance NK cell-mediated ADCC and improve objective remission rates in squamous cell carcinoma of the head and neck (SCCHN) (66). However, clinical trials on NKG2A mAbs for the treatment of breast cancer have not been reported. The marketing of NKG2A mAbs needs the support of larger clinical trials.
KIR2D antagonists (lirilumab) promote NK cell and granzyme B expression in a nuclear factor-κB-dependent manner in autologous cervical cancer cells (67). Lirilumab combined with rituximab can enhance NK cell-mediated rituximab-dependent cytotoxicity in KIR transgenic and homozygous mouse lymphoma models (68). In a phase II study of locally recurrent SCCHN, a 43% response rate to anti-PD-1, nabumab, and lirilumab pathology was observed with a favorable DFS and excellent 2-year overall survival compared with previously treated high-risk patients (69). Clinical trial results for KIR antagonists were mixed, with a KIR2D-specific mAb IPH2101 terminated early due to the lack of clinical efficacy in a single-arm phase II clinical trial for multiple myeloma (70). Carlsten M (71) analyzed the possible reasons for the failure of this clinical trial given that IPH2101 marginally augmented the antimyeloma cytotoxicity of remaining KIR2Ddull patient NK cells; the overall response was diminished by significant contraction and reduced function of KIR2D-expressing NK cells. At present, no clinical study has reported lirilumab for the treatment of breast cancer, and its therapeutic effects deserve further exploration.
Cytokines therapy
The activity of NK cells is also influenced by various pro-inflammatory cytokines, including IL-15, IL-2, IFN-γ, TNF, granulocyte-macrophage colony-stimulating factor (GM-CSF), TGF-β1, and C-X3-C motif chemokine ligand 1 (CX3CL1) (72). Table 1 summarizes the effects of cytokines on NK cells and ADCC effects.
The current research on cytokines therapy is focused on IL2 and IL15. Xiong Q constructed a membrane-bound IL-2 (mbIL-2) consisting of human IL-2 and human IL-2Rα joined by a classic linker. The novel mbIL-2 improved NK-92 cell persistence and enhanced its anti-tumor activity (86). Fujii R observed that the IL-15SA/IL-15RA complex can act as an inhibitor to block the inhibitory effects of TGF-β on the NK cell activation markers CD226, NKG2D, and NKp30 in breast, lung, and prostate cancers (87). IL-12 is an essential cytokine involved in the generation of memory-like NK cells, and IL-12 alone can sustain human primary NK cell survival without providing IL-2 or IL-15 but is insufficient to promote human NK cell proliferation (88). NK cells in breast tumors express high levels of NKG2A and low levels of NKp46, perforin, and granzyme B. One week of treatment with IL-12 and anti-TGF-β resulted in the increased maturation of tumor-associated NK cells (84). The combination of STING agonists with IL2/anti-PD-1 synergized to stimulate sustained granzyme and cytokine expression by lung-infiltrating NK cells in two spontaneously metastasizing orthotopic breast tumor models (89).
Cytokine-inducible SH2-containing protein (CIS; encoded by the gene CISH) is the first member identified in the suppressors of the cytokine signaling protein family. CISH is a key negative regulator of IL-15 signaling in NK cells, and it plays a key role in the regulation of human NK cell metabolic activity and thereby modulates anti-tumor activity (85). CISH deletion favors the accumulation of NK cells in primary breast cancer, thus optimizing NK cell-killing properties, and decreases T cell immune receptors with immunoglobulin and ITIM domain (TIGIT) immune checkpoint receptor expression (90). The activation of STAT3 helps tumor cells to evade NK cell-mediated immune surveillance, which is associated with high expression of tumor-promoting cytokines and growth factors, such as IL-10, TGF-β, and vascular endothelial growth factor-A (91).
Novel cytokine superagonists and lipid nanoparticles (LNPs) were designed to overcome the shortages of conventional cytokines, such as poor half-life, circulation instability, and dose-limiting toxicity. Jiangyin Lv combined IgG4 Fc segments, soluble IL-15Rα, and IL-15 (N72D) into a homodimeric IL-15 superagonist (F4RLI). F4RLI significantly stimulated the proliferation of human CD3+CD8+ T cells and NK cells in vitro (92). Jin-Qing Liu generated novel LNPs encapsulated with mRNA encoding cytokines (including IL-12, IL-27, and GM-CSF) that induced potent infiltration of immune effector cells, including IFN-γ and TNF-α producing NK and CD8+T cells into tumors, offering a new strategy for oncology immunotherapy (93).
In HER2-positive gastric cancer, IL2 treatment restored trastuzumab-mediated resistance to ADCC while restoring the expression of the CD16 zeta molecule, which may have implications for the treatment of HER2-positive breast cancer (94). Blockade with a specific KIR2DL-1,2/3 mAb reversed NK-cell inhibition and significantly enhanced degranulation and IFN-γ production of IL-2-preactivated NK-cells in the presence of primary glioblastoma (GBM) cells (95). In pancreatic cancer, the stimulator of interferon gene (STING)-IL35 axis in B cells reduced the proliferation of NK cells and attenuated the NK-driven anti-tumor response (96). The team of Landolina N (83) transiently silenced IL-1R8 by electroporated siRNA, and significantly higher concentrations of IFN-γ, TNFα, GM-CSF, CCL3, and CXCL8 release were observed in NK cells with silenced IL-1R8. Disruption of the IL6R/STAT3 axis and TIGIT in prostate cancer cells can increase the cytotoxicity of NK-92 cells by increasing FasL and granzyme A/B (97). In a xenograft mouse model of B-cell lymphoma, the addition of ALT-803 to anti-CD20 mAb treatment in conjunction with NK cells reduced the tumor burden and improved survival (98). Siebert N linked fibroblast activating protein alpha (FAP) and a mutant IL-2 variant (IL-2v) to form a FAP-IL-2v conjugate. They observed that FAP-IL-2v reduced tumor growth of high-risk neuroblastoma and improved survival, with increased numbers of NK and cytotoxic T cells (99). We have compiled research strategies on potential targets and cytokines that are currently being studied or in clinical trials (Table 2).
Antibody engineering and glycoengineering
FcγR IIIa (CD16) binds to the Fc segment of IgG1 antibodies by interacting with the hinge region and methylene structural domain. This interaction is significantly influenced by the glycan of Asn 297 at the N-glycosylation site in each methylene structural domain. Therefore, studies have been conducted to enhance the interaction of the Fc segment with FcγR IIIa to improve the ADCC effect by techniques, such as glycoengineering of Fc N-glycans and design of Fc structural domains (106). Figure 2 shows the ADCC process.
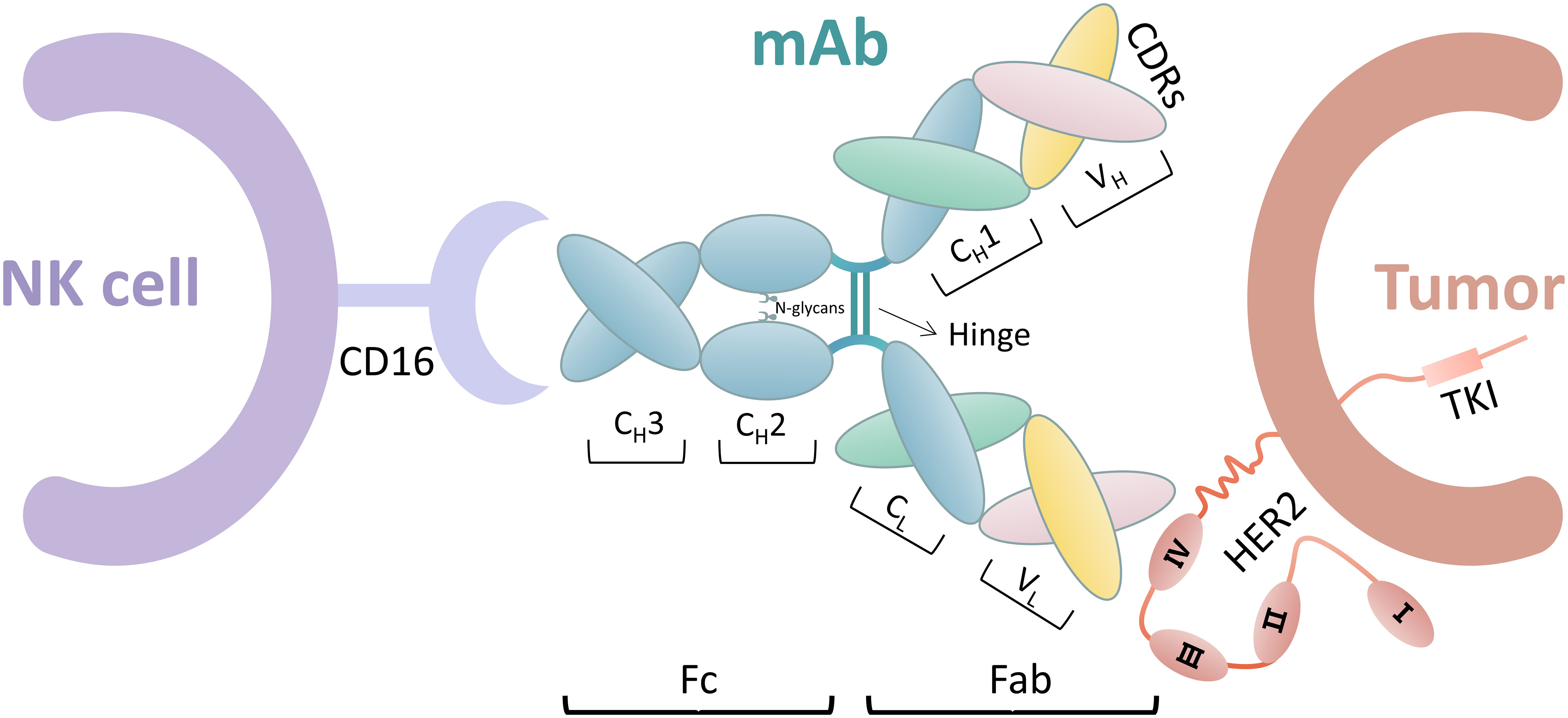
Figure 2 ADCC process including NK cells, mAb, and tumor cells. The mAbs include the Fab and Fc segments. The Fab segment is an endometrioid by heavy-chain constant region (CH1) and heavy chain variable region (VH), in which the amino acid sequence is constant. The amino acid sequences of light-chain constant region (CL) and heavy-chain variable region (VL) sites are variable. Complementary determining regions (CDRs) of the Fab segment bind on tumor cells by different amino acid sequences, such as trastuzumab binding to HER2 extracellular region IV. The Fc segment includes CH2 and CH3. NK cells bind to the Fc segment of mAb via CD16. CD16 interacts with CH2 of mAb in a 1:1 manner, where the N-glycan chain at the N297 position on CH2 also plays a key role in this interaction.
Hsiao H-C observed that hinge cleavage occurred when pertuzumab was incubated with cancer cells, which led to a substantial loss of ADCC (107). They constructed the protease-resistant version of the anti-hinged mAb to restore the ADCC. Kinder M demonstrated that discrete mutations in the CH2 region can compensate for the loss of function associated with mutation of the lower hinge of IgG1, thus initiating the complement cascade and facilitating potent ADCC (108). Oberg HH designed a trimeric structure of bsAb, that is, [(HER2)xCD16], which can redirect CD16-expressing γδ T cells in addition to NK cells to lyse HER2-expressing tumor cells. Their team discovered that trimeric bsAb with trastuzumab enhanced γδ T cell- and NK cell-mediated expression by an increased degranulation (109). Lin X designed a nano-immunomodulator AuNSP@αCD16, which exhibited high gene transfection efficiency and stable near infrared-II photothermal capacity (110). AuNSP enables αCD16 gene transfection by modifying the tumor surface with CD16 antibodies, thus forming a tumor surface that allows NK cells to exert their recognition ability in the TME. This function increases the release of cytolytic particles and pro-inflammatory cytokines and ultimately enhances the killing function of NK cells against solid tumors.
Hong S observed that tumor cells upregulate sialylated glycans, which counteract NK-induced killing via the Siglec–sialylated glycan interaction. In addition, the high-affinity ligand of Siglec-7 leads to multifaceted consequences in the modulation of NK activation (111). The team of Hong S also reported a chemoenzymatic glycocalyx editing strategy to introduce high-affinity and specific CD22 ligands onto NK-92MI and cytokine-induced NK cells to achieve tumor-specific CD22 targeting (112). Madsen CB created glycoengineered breast cancer cells via zinc finger nuclease knockout (KO) of the core 1 enzyme chaperone (COSMC) for glycoengineering, and the ADCC effect mediated to NK cells increased in the COSMC KO breast cancer cell line (113).
CAR-NK
CAR-T therapy has been approved by the FDA for the treatment of B-cell lymphoma and acute lymphoblastic leukemia. Compared with CAR-T therapy, CAR-NK therapy possesses unique advantages, such as the absence of autoimmune response and its independent killing capability (114). CAR-NK therapy has been carried out in a number of solid tumors in hematological diseases (115), breast cancer (116), and ovarian cancer (117).
Retargeting of NK cells with CARs or mAbs is a promising strategy to overcome tumor resistance. Eitler J reported that retargeting by CAR and/or the FcR/mAb (ADCC) axis provides the necessary signals for granule polarization and overcoming the resistance of HER2-positive breast tumors (118). Portillo AL demonstrated that HER2 CAR expression in NK cells enhanced the anti-tumor functions of patients with HER2-positive breast cancer, regardless of MHC class I expression (119). CAR-NK cells secreted IL-15 to maintain their anti-relapsed/refractory acute myeloid leukemia (AML) function and exhibited a high anti-AML activity. However, this benefit was transient due to the limited persistence of CAR-NK cells (120). Low-dose IL-2 was included in several clinical trials as a single treatment or as adjuvant therapy in combination with peripatetic NK and T cell transfer therapy (121). These studies showed that CAR-NK cells may be a highly potent and safe source of immunotherapy in the context of HER2-positive breast cancer.
ADCC effective stage
The ADCC effective stage is mainly accomplished by the polarized release of cytotoxic mediators, which consists of key steps, such as reorganization and accumulation of filamentous actin on the immunological synapse (IS), receptor aggregation, polarization of the microtubule organizing center (MTOC) to the IS with lysis granules, and secretion of toxic granule contents at the IS (122, 123).
The F-actin network increases in specific regions of the IS, as observed by three-dimensional-structured illumination microscopy and two-color super-resolution imaging. When NK cells are activated, the F-actin network opens with a diameter of approximately 250–500 nm (124) to guide lysis granules along microtubules toward the MTOC; this step allows lysis granules to rest precisely around the area of MTOC polarization (125). Particle polarization and degranulation involve multiple intermediate steps, which culminate in the directed secretion of lysed particle contents at the IS. Trastuzumab-refractory patients had high serum levels of inflammatory protein chitinase 3-like 1 (CHI3L1), which prevented the correct polarization of the microtubule-organizing center along with lytic granules to the IS by hindering the receptor of advanced glycation end-products. CHI3L1 blockade was synergized with ADCC to cure mice with HER2-positive xenografts (9).
Conclusion
As one of the main mechanisms of action of anti-HER2-targeted drugs, targeting NK cells and their ADCC action is an effective way to improve anti-HER2 efficacy. The development of novel drugs is often tested first in hematologic or highly aggressive metastatic tumors, whereas the research on HER2-positive breast cancer is relatively late. mAb drugs, which were developed to activate and inhibit receptors on NK cells, are still mostly in preclinical studies. In conclusion, in the TME, enhancement of NK cell activity and ADCC is a tumor immunotherapy strategy to improve the clinical efficacy of anti-HER2 mAbs. However, for the complex regulatory mechanism of NK cells and the multi-level ADCC effector process, considerable basic and clinical research needs the joint efforts of biologists, antibody engineering scientists, drug researchers, and clinical workers.
Author contributions
SL designed the study. FL drafted the manuscript. Both authors contributed to the article and approved the submitted version.
Funding
This work was supported by National Natural Science Foundation of China [81774308]; High-level University Team Building Summit Plateau Innovation Team [601521D].
Conflict of interest
The authors declare that the research was conducted in the absence of any commercial or financial relationships that could be construed as a potential conflict of interest.
Publisher’s note
All claims expressed in this article are solely those of the authors and do not necessarily represent those of their affiliated organizations, or those of the publisher, the editors and the reviewers. Any product that may be evaluated in this article, or claim that may be made by its manufacturer, is not guaranteed or endorsed by the publisher.
References
1. Wolff AC, Hale MEH, Allison KH, Harvey BE, Mangu PB, Bartlett JMS, et al. Human epidermal growth factor receptor 2 testing in breast cancer: American society. J Clin Oncol (2018) 36(20):2105–22. doi: 10.1200/JCO.2018.77.8738
2. Harbeck N. Advances in targeting HER2-positive breast cancer. Curr Opin Obstet Gynecol (2018) 30(1):55–9. doi: 10.1097/GCO.0000000000000431
3. Wynn CS, Tang SC. Anti-HER2 therapy in metastatic breast cancer: Many choices and future directions. Cancer Metastasis Rev (2022) 41(1):193–209. doi: 10.1007/s10555-022-10021-x
4. Li J, Jiang Z. The 2021 update of the Chinese society of clinical oncology breast cancer guidelines. Chin Med J (2021) 101(24):1835–8. Available at: https://kns.cnki.net/kcms/detail/detail.aspx?FileName=ZHYX202124002&DbName=ZHYX2021.
5. Li L, Zhang D, Liu B, Lv D, Zhai J, Guan X, et al. Antibody-drug conjugates in HER2-positive breast cancer. Chin Med J (Engl) (2021) 135(3):261–7. doi: 10.1097/CM9.0000000000001932
6. Upton R, Banuelos A, Feng D, Biswas T, Kao K, McKenna K, et al. Combining CD47 blockade with trastuzumab eliminates HER2-positive breast cancer cells and overcomes trastuzumab tolerance. Proc Natl Acad Sci U.S.A. (2021) 118(29):e2026849118. doi: 10.1073/pnas.2026849118
7. Feng M, Yang Y, Liao W, Li Q. Margetuximab versus trastuzumab in patients with advanced breast cancer: A cost-effectiveness analysis. Clin Breast Cancer (2022) 22(5):e629–e35. doi: 10.1016/j.clbc.2022.03.002
8. Lee S-C, Shimasaki N, Lim JSJ, Wong A, Yadav K, Yong WP, et al. Phase I trial of expanded, activated autologous NK-cell infusions with trastuzumab in patients with HER2-positive cancers. Clin Cancer Res (2020) 26(17):4494–502. doi: 10.1158/1078-0432.CCR-20-0768
9. Darwich A, Silvestri A, Benmebarek M-R, Mouriès J, Cadilha B, Melacarne A, et al. Paralysis of the cytotoxic granule machinery is a new cancer immune evasion mechanism mediated by chitinase 3-Like-1. J Immunother Cancer (2021) 9(11):e003224. doi: 10.1136/jitc-2021-003224
10. Favaro RR, Phillips K, Delaunay-Danguy R, Ujcic K, Markert UR. Emerging concepts in innate lymphoid cells, memory, and reproduction. Front Immunol (2022) 13:824263. doi: 10.3389/fimmu.2022.824263
11. Crinier A, Narni-Mancinelli E, Ugolini S, Vivier E. Snapshot: Natural killer cells. Cell (2020) 180(6):1280. doi: 10.1016/j.cell.2020.02.029
12. Cichocki F, Grzywacz B, Miller JS. Human NK cell development: One road or many? Front Immunol (2019) 10:2078. doi: 10.3389/fimmu.2019.02078
13. Mrozek E, Anderson P, Caligiuri MA. Role of interleukin-15 in the development of human CD56+ natural killer cells from CD34+ hematopoietic progenitor cells. Blood (1996) 87(7):2632–40. doi: 10.1182/blood.V87.7.2632.bloodjournal8772632
14. Cichicki F, Schlums H, Theorell J, Tesi B, Miller JS, Ljunggren HG, et al. Diversification and functional specialization of human NK cell subsets. Curr Top Microbiol Immunol (2016) 395:63–94. doi: 10.1007/82_2015_487
15. Wahlen S, Matthijssens F, Van Loocke W, Taveirne S, Kiekens L, Persyn E, et al. The transcription factor Runx2 drives the generation of human NK cells and promotes tissue residency. Elife (2022) 11:e80320. doi: 10.7554/eLife.80320
16. Vitale M, Cantoni C, Pietra G, Mingari MC, Moretta L. Effect of tumor cells and tumor microenvironment on NK-cell function. Eur J Immunol (2014) 44(6):1582–92. doi: 10.1002/eji.201344272
17. Hu S, Zhu H. Research progress of NK cells in tumor immunotherapy. Chin J Immunol (2022), 1–19. Available at: http://kns.cnki.net/kcms/detail/22.1126.R.20220314.1332.002.html.
18. López-Soto A, Gonzalez S, Smyth MJ, Galluzzi L. Control of metastasis by NK cells. Cancer Cell (2017) 32(2):135–54. doi: 10.1016/j.ccell.2017.06.009
19. Musolino A, Gradishar WJ, Rugo HS, Nordstrom JL, Rock EP, Arnaldez F, et al. Role of fcgamma receptors in HER2-targeted breast cancer therapy. J Immunother Cancer (2022) 10(1):e003171. doi: 10.1136/jitc-2021-003171
20. Nimmerjahn F, Ravetch JV. Fcgamma receptors as regulators of immune responses. Nat Rev Immunol (2008) 8(1):34–47. doi: 10.1038/nri2206
21. Cameron D, Piccart-Gebhart MJ, Gelber RD, Procter M, Goldhirsch A, de Azambuja E, et al. 11 years' follow-up of trastuzumab after adjuvant chemotherapy in HER2-positive early breast cancer: Final analysis of the herceptin adjuvant (Hera) trial. Lancet (2017) 389(10075):1195–205. doi: 10.1016/S0140-6736(16)32616-2
22. Luo M, Xu C, Sun Y, Zhang K, Chen Y. Targeted therapy for HER2-positive breast cancer and its drug resistance mechanism. J Pract Oncol (2018) 33(03):283–8. doi: 10.13267/j.cNKi.syzlzz.2018.03.018
23. Ehlers FAI, Beelen NA, van Gelder M, Evers TMJ, Smidt ML, Kooreman LFS, et al. ADCC-inducing antibody trastuzumab and selection of KIR-HLA ligand mismatched donors enhance the NK cell anti-breast cancer response. Cancers (Basel) (2021) 13(13):3232. doi: 10.3390/cancers13133232
24. Clynes RA, Towers TL, Presta LG, Ravetch JV. Inhibitory fc receptors modulate in vivo cytotoxicity against tumor targets. Nat Med (2000) 6(4):443–6. doi: 10.1038/74704
25. Duong MN, Cleret A, Matera E-L, Chettab K, Mathé D, Valsesia-Wittmann S, et al. Adipose cells promote resistance of breast cancer cells to trastuzumab-mediated antibody-dependent cellular cytotoxicity. Breast Cancer Res (2015) 17:57. doi: 10.1186/s13058-015-0569-0
26. Feng F. Enhanced killing of trastuzumab-resistant breast cancer cells by natural killer cells in combination with trastuzumab. Jilin University (2016). Availabel at: https://kns.cnki.net/KCMS/detail/detail.aspx?dbname=CDFDLAST2016&filename=1016084508.nh.
27. Arnould L, Gelly M, Penault-Llorca F, Benoit L, Bonnetain F, Migeon C, et al. Trastuzumab-based treatment of HER2-positive breast cancer: An antibody-dependent cellular cytotoxicity mechanism? Br J Cancer (2006) 94(2):259–67. doi: 10.1038/sj.bjc.6602930
28. Varchetta S, Gibelli N, Oliviero B, Nardini E, Gennari R, Gatti G, et al. Elements related to heterogeneity of antibody-dependent cell cytotoxicity in patients under trastuzumab therapy for primary operable breast cancer overexpressing HER2. Cancer Res (2007) 67(24):11991–9. doi: 10.1158/0008-5472.CAN-07-2068
29. Beano A, Signorino E, Evangelista A, Brusa D, Mistrangelo M, Polimeni MA, et al. Correlation between NK function and response to trastuzumab in metastatic breast cancer patients. J Transl Med (2008) 6:25. doi: 10.1186/1479-5876-6-25
30. Piccart M, Procter M, Fumagalli D, de Azambuja E, Clark E, Ewer MS, et al. Adjuvant pertuzumab and trastuzumab in early HER2-positive breast cancer in the aphinity trial: 6 years' follow-up. J Clin Oncol (2021) 39(13):1448–57. doi: 10.1200/JCO.20.01204
31. Deng W, Liu J, Pan H, Li L, Zhou C, Wang X, et al. A bispecific antibody based on pertuzumab fab has potent antitumor activity. J Immunother (2018) 41(1):1–8. doi: 10.1097/CJI.0000000000000200
32. Asgari A, Sharifzadeh S, Ghaderi A, Hosseini A, Ramezani A. In vitro cytotoxic effect of trastuzumab in combination with pertuzumab in breast cancer cells is improved by interleukin-2 activated NK cells. Mol Biol Rep (2019) 46(6):6205–13. doi: 10.1007/s11033-019-05059-0
33. Diessner J, Bruttel V, Becker K, Pawlik M, Stein R, Hausler S, et al. Targeting breast cancer stem cells with HER2-specific antibodies and natural killer cells. Am J Cancer Res (2013) 3(2):211–20.
34. Chung YC, Kuo JF, Wei WC, Chang KJ, Chao WT. Caveolin-1 dependent endocytosis enhances the chemosensitivity of HER2-positive breast cancer cells to trastuzumab emtansine (T-DM1). PloS One (2015) 10(7):e0133072. doi: 10.1371/journal.pone.0133072
35. Ramamoorthi G, Kodumudi K, Snyder C, Grover P, Zhang H, Greene MI, et al. Intratumoral delivery of dendritic cells plus anti-HER2 therapy triggers both robust systemic antitumor immunity and complete regression in HER2 mammary carcinoma. J Immunother Cancer (2022) 10(6):e004841. doi: 10.1136/jitc-2022-004841
36. Menderes G, Bonazzoli E, Bellone S, Altwerger G, Black JD, Dugan K, et al. Superior in vitro and in vivo activity of trastuzumab-emtansine (T-DM1) in comparison to trastuzumab, pertuzumab and their combination in epithelial ovarian carcinoma with high HER2/Neu expression. Gynecol Oncol (2017) 147(1):145–52. doi: 10.1016/j.ygyno.2017.07.009
37. Cretella D, Saccani F, Quaini F, Frati C, Lagrasta C, Bonelli M, et al. Trastuzumab emtansine is active on HER2 overexpressing NSCLC cell lines and overcomes gefitinib resistance. Mol Cancer. (2014) 13:143. doi: 10.1186/1476-4598-13-143
38. Bang YJ, Giaccone G, Im SA, Oh DY, Bauer TM, Nordstrom JL, et al. First-in-Human phase 1 study of margetuximab (Mgah22), an fc-modified chimeric monoclonal antibody, in patients with HER2-positive advanced solid tumors. Ann Oncol (2017) 28(4):855–61. doi: 10.1093/annonc/mdx002
39. Royce M, Osgood CL, Amatya AK, Fiero MH, Chang CJG, Ricks TK, et al. Fda approval summary: Margetuximab plus chemotherapy for advanced or metastatic HER2-positive breast cancer. Clin Cancer Res (2022) 28(8):1487–92. doi: 10.1158/1078-0432.CCR-21-3247
40. Rugo HS, Im S-A, Cardoso F, Cortés J, Curigliano G, Musolino A, et al. Efficacy of margetuximab vs trastuzumab in patients with pretreated Erbb2-positive advanced breast cancer: A phase 3 randomized clinical trial. JAMA Oncol (2021) 7(4):573–84. doi: 10.1001/jamaoncol.2020.7932
41. Catenacci DVT, Kang Y-K, Park H, Uronis HE, Lee K-W, Ng MCH, et al. Margetuximab plus pembrolizumab in patients with previously treated, HER2-positive gastro-oesophageal adenocarcinoma (Cp-Mgah22-05): A single-arm, phase 1b-2 trial. Lancet Oncol (2020) 21(8):1066–76. doi: 10.1016/S1470-2045(20)30326-0
42. Collins DM, Madden SF, Gaynor N, AlSultan D, Le Gal M, Eustace AJ, et al. Effects of her family-targeting tyrosine kinase inhibitors on antibody-dependent cell-mediated cytotoxicity in HER2-expressing breast cancer. Clin Cancer Res (2021) 27(3):807–18. doi: 10.1158/1078-0432.CCR-20-2007
43. Okita R, Shimizu K, Nojima Y, Yukawa T, Maeda A, Saisho S, et al. Lapatinib enhances trastuzumab-mediated antibody-dependent cellular cytotoxicity Via upregulation of HER2 in malignant mesothelioma cells. Oncol Rep (2015) 34(6):2864–70. doi: 10.3892/or.2015.4314
44. Cavazzoni A, Alfieri RR, Cretella D, Saccani F, Ampollini L, Galetti M, et al. Combined use of anti-erbb mAbs and erlotinib enhances antibody-dependent cellular cytotoxicity of wild-type erlotinib-sensitive nsclc cell lines. Mol Cancer (2012) 11:91. doi: 10.1186/1476-4598-11-91
45. CerweNKa A, Lanier LL. Natural killer cell memory in infection, inflammation and cancer. Nat Rev Immunol (2016) 16(2):112–23. doi: 10.1038/nri.2015.9
46. Nieto-Velázquez NG, Torres-Ramos YD, Muñoz-Sánchez JL, Espinosa-Godoy L, Gómez-Cortés S, Moreno J, et al. Altered expression of natural cytotoxicity receptors and NKG2D on peripheral blood NK cell subsets in breast cancer patients. Transl Oncol (2016) 9(5):384–91. doi: 10.1016/j.tranon.2016.07.003
47. Nahta R, Esteva FJ. Herceptin: Mechanisms of action and resistance. Cancer Lett (2006) 232(2):123–38. doi: 10.1016/j.canlet.2005.01.041
48. Long EO, Kim HS, Liu D, Peterson ME, Rajagopalan S. Controlling natural killer cell responses: Integration of signals for activation and inhibition. Annu Rev Immunol (2013) 31:227–58. doi: 10.1146/annurev-immunol-020711-075005
49. Martinet L, Smyth MJ. Balancing natural killer cell activation through paired receptors. Nat Rev Immunol (2015) 15(4):243–54. doi: 10.1038/nri3799
50. Huntington ND, Nutt SL, Carotta S. Regulation of murine natural killer cell commitment. Front Immunol (2013) 4:14. doi: 10.3389/fimmu.2013.00014
51. Van Coillie J, Schulz MA, Bentlage AEH, de Haan N, Ye Z, Geerdes DM, et al. Role of n-glycosylation in FcγRIIIa interaction with IgG. Front Immunol (2022) 13:987151. doi: 10.3389/fimmu.2022.987151
52. Muntasell A, Ochoa MC, Cordeiro L, Berraondo P, Lopez-Diaz de Cerio A, Cabo M, et al. Targeting NK-cell checkpoints for cancer immunotherapy. Curr Opin Immunol (2017) 45:73–81. doi: 10.1016/j.coi.2017.01.003
53. Shen X, Fu W, Wei Y, Zhu J, Yu Y, Lei C, et al. Tigit-fc promotes antitumor immunity. Cancer Immunol Res (2021) 9(9):1088–97. doi: 10.1158/2326-6066.CIR-20-0986
54. Zafirova B, Wensveen FM, Gulin M, Polic B. Regulation of immune cell function and differentiation by the NKG2D receptor. Cell Mol Life Sci (2011) 68(21):3519–29. doi: 10.1007/s00018-011-0797-0
55. Zhang C, Roder J, Scherer A, Bodden M, Pfeifer Serrahima J, Bhatti A, et al. Bispecific antibody-mediated redirection of NKG2D-CAR natural killer cells facilitates dual targeting and enhances antitumor activity. J Immunother Cancer (2021) 9(10):e002980. doi: 10.1136/jitc-2021-002980
56. Seidel E, Glasner A, Mandelboim O. Virus-mediated inhibition of natural cytotoxicity receptor recognition. Cell Mol Life Sci (2012) 69(23):3911–20. doi: 10.1007/s00018-012-1001-x
57. Vogler I, Steinle A. Vis-à-Vis in the NKc: Genetically LiNKed natural killer cell Receptor/Ligand pairs in the natural killer gene complex (NKc). J Innate Immun (2011) 3(3):227–35. doi: 10.1159/000324112
58. Peipp M, Derer S, Lohse S, Staudinger M, Klausz K, Valerius T, et al. HER2-specific immunoligands engaging NKp30 or NKp80 trigger NK-cell-mediated lysis of tumor cells and enhance antibody-dependent cell-mediated cytotoxicity. Oncotarget. (2015) 6(31):32075–88. doi: 10.18632/oncotarget.5135
59. Kellner C, Lutz S, Oberg HH, Wesch D, Otte A, Diemer KJ, et al. Tumor cell lysis and synergistically enhanced antibody-dependent cell-mediated cytotoxicity by NKG2D engagement with a bispecific immunoligand targeting the HER2 antigen. Biol Chem (2021) 403(5-6):545–56. doi: 10.1515/hsz-2021-0229
60. Cabo M, Santana-Hernández S, Costa-Garcia M, Rea A, Lozano-Rodríguez R, Ataya M, et al. CD137 costimulation counteracts TGFβ inhibition of NK-cell antitumor function. Cancer Immunol Res (2021) 9(12):1476–90. doi: 10.1158/2326-6066.CIR-21-0030
61. Li Z, Simin L, Jian K, Xin G, Youlin K. 4-1bb antibody enhances cytotoxic activity of natural killer cells against prostate cancer cells Via NKG2D agonist combined with IL-27. Immunotherapy (2022) 14(13):1043–53. doi: 10.2217/imt-2021-0232
62. Rajagopalan S, Bryceson YT, Kuppusamy SP, Geraghty DE, van der Meer A, Joosten I, et al. Activation of NK cells by an endocytosed receptor for soluble hla-G. PloS Biol (2006) 4(1):e9. doi: 10.1371/journal.pbio.0040009
63. Zheng G, Guo Z, Li W, Xi W, Zuo B, Zhang R, et al. Interaction between HLA-G and NK cell receptor KIR2DL4 orchestrates HER2-positive breast cancer resistance to trastuzumab. Signal Transduct Target Ther (2021) 6(1):236. doi: 10.1038/s41392-021-00629-w
64. Spinosa P, Musial-Siwek M, Presler M, Betts A, Rosentrater E, Villali J, et al. Quantitative modeling predicts competitive advantages of a next generation anti-NKG2A monoclonal antibody over monalizumab for the treatment of cancer. CPT Pharmacometrics Syst Pharmacol (2021) 10(3):220–9. doi: 10.1002/psp4.12592
65. Frazao A, Messaoudene M, Nunez N, Dulphy N, Roussin F, Sedlik C, et al. CD16NKG2A natural killer cells infiltrate breast cancer-draining lymph nodes. Cancer Immunol Res (2019) 7(2):208–18. doi: 10.1158/2326-6066.CIR-18-0085
66. André P, Denis C, Soulas C, Bourbon-Caillet C, Lopez J, Arnoux T, et al. Anti-NKG2A mab is a checkpoint inhibitor that promotes anti-tumor immunity by unleashing both T and NK cells. Cell (2018) 175(7):1731–43.e13. doi: 10.1016/j.cell.2018.10.014
67. Liu H, Zhou S, Liu J, Chen F, Zhang Y, Liu M, et al. Lirilumab and avelumab enhance anti-hpv+ cervical cancer activity of natural killer cells Vav1-dependent nf-κb disinhibition. Front Oncol (2022) 12:747482. doi: 10.3389/fonc.2022.747482
68. Kohrt HE, Thielens A, Marabelle A, Sagiv-Barfi I, Sola C, Chanuc F, et al. Anti-KIR antibody enhancement of anti-lymphoma activity of natural killer cells as monotherapy and in combination with anti-CD20 antibodies. Blood (2014) 123(5):678–86. doi: 10.1182/blood-2013-08-519199
69. Hanna GJ, O'Neill A, Shin K-Y, Wong K, Jo VY, Quinn CT, et al. Neoadjuvant and adjuvant nivolumab and lirilumab in patients with recurrent, resectable squamous cell carcinoma of the head and neck. Clin Cancer Res (2022) 28(3):468–78. doi: 10.1158/1078-0432.CCR-21-2635
70. Korde N, Carlsten M, Lee M-J, Minter A, Tan E, Kwok M, et al. A phase II trial of pan-KIR2D blockade with Iph2101 in smoldering multiple myeloma. Haematologica (2014) 99(6):e81–e3. doi: 10.3324/haematol.2013.103085
71. Carlsten M, Korde N, Kotecha R, Reger R, Bor S, Kazandjian D, et al. Checkpoint inhibition of KIR2D with the monoclonal antibody Iph2101 induces contraction and hyporesponsiveness of NK cells in patients with myeloma. Clin Cancer Res (2016) 22(21):5211–22. doi: 10.1158/1078-0432.CCR-16-1108
72. Fauriat C, Long EO, Ljunggren HG, Bryceson YT. Regulation of human NK-cell cytokine and chemokine production by target cell recognition. Blood (2010) 115(11):2167–76. doi: 10.1182/blood-2009-08-238469
73. Lv M, Qiu X, Wang J, Wang Y, Liu Q, Zhou H, et al. Regulation of IL-2 on the expression of granzyme b- and perforin-like genes and its functional implication in grass carp peripheral blood neutrophils. Fish Shellfish Immunol (2022) 124:472–9. doi: 10.1016/j.fsi.2022.04.041
74. Ito S, Bollard CM, Carlsten M, Melenhorst JJ, Biancotto A, Wang E, et al. Ultra-low dose interleukin-2 promotes immune-modulating function of regulatory T cells and natural killer cells in healthy volunteers. Mol Ther (2014) 22(7):1388–95. doi: 10.1038/mt.2014.50
75. Miljkovic MD, Dubois S, Müller JR, Bryant BR, Ma E, Conlon KC, et al. Interleukin-15 augments NK cell-mediated ADCC of alemtuzumab in patients with CD52+ T-cell malignancies. Blood Adv (2022). doi: 10.1182/bloodadvances.2021006440
76. Carson WE, Giri JG, Lindemann MJ, Linett ML, Ahdieh M, Paxton R, et al. Interleukin (Il) 15 is a novel cytokine that activates human natural killer cells Via components of the IL-2 receptor. J Exp Med (1994) 180(4):1395–403. doi: 10.1084/jem.180.4.1395
77. Li Y, Li Y, Xiang B, Tian X, Shi Q, Liu F, et al. IL-2 combined with IL-15 enhanced the expression of NKG2D receptor on patient autologous NK cells to inhibit wilms' tumor Via mapk signaling pathway. J Oncol (2022) 2022:4544773. doi: 10.1155/2022/4544773
78. Dreyer TF, Kuhn S, Stange C, Heithorst N, Schilling D, Jelsma J, et al. The chemokine Cx3cl1 improves trastuzumab efficacy in HER2 low-expressing cancer and. Cancer Immunol Res (2021) 9(7):779–89. doi: 10.1158/2326-6066.CIR-20-0327
79. Ziblat A, Nunez SY, Raffo Iraolagoitia XL, Spallanzani RG, Torres NI, Sierra JM, et al. Interleukin (Il)-23 stimulates ifn-gamma secretion by CD56(Bright) natural killer cells and enhances IL-18-Driven dendritic cells activation. Front Immunol (2017) 8:1959. doi: 10.3389/fimmu.2017.01959
80. McMichael EL, Jaime-Ramirez AC, Guenterberg KD, Luedke E, Atwal LS, Campbell AR, et al. IL-21 enhances natural killer cell response to cetuximab-coated pancreatic tumor cells. Clin Cancer Res (2017) 23(2):489–502. doi: 10.1158/1078-0432.CCR-16-0004
81. Souza-Fonseca-Guimaraes F, Rossi GR, Dagley LF, Foroutan M, McCulloch TR, Yousef J, et al. Tgfβ and cis inhibition overcomes NK-cell suppression to restore antitumor immunity. Cancer Immunol Res (2022) 10(9):1047–54. doi: 10.1158/2326-6066.CIR-21-1052
82. Trotta R, Dal Col J, Yu J, Ciarlariello D, Thomas B, Zhang X, et al. Tgf-beta utilizes Smad3 to inhibit CD16-mediated ifn-gamma production and antibody-dependent cellular cytotoxicity in human NK cells. J Immunol (2008) 181(6):3784–92. doi: 10.4049/jimmunol.181.6.3784
83. Landolina N, Mariotti FR, Ingegnere T, Alicata C, Ricci B, Pelosi A, et al. IL-1r8 silencing improves the anti-tumor function of freshly isolated human NK cells. J Immunother Cancer (2022) 10(3):e003858. doi: 10.1136/jitc-2021-003858
84. Krneta T, Gillgrass A, Chew M, Ashkar AA. The breast tumor microenvironment alters the phenotype and function of natural killer cells. Cell Mol Immunol (2016) 13(5):628–39. doi: 10.1038/cmi.2015.42
85. Zhu H, Blum RH, Bernareggi D, Ask EH, Wu Z, Hoel HJ, et al. Metabolic reprograming Via deletion of CISH in human IPSC-derived NK cells promotes in vivo persistence and enhances anti-tumor activity. Cell Stem Cell (2020) 27(2):224–37.e6. doi: 10.1016/j.stem.2020.05.008
86. Xiong Q, Zhang H, Ji X, Zhang Y, Shi G, Dai L, et al. A novel membrane-bound interleukin-2 promotes NK-92 cell persistence and anti-tumor activity. Oncoimmunology (2022) 11(1):2127282. doi: 10.1080/2162402X.2022.2127282
87. Fujii R, Jochems C, Tritsch SR, Wong HC, Schlom J, Hodge JW. An IL-15 Superagonist/IL-15ralpha fusion complex protects and rescues NK cell-cytotoxic function from tgf-Beta1-Mediated immunosuppression. Cancer Immunol Immunother (2018) 67(4):675–89. doi: 10.1007/s00262-018-2121-4
88. Shemesh A, Pickering H, Roybal KT, Lanier LL. Differential IL-12 signaling induces human natural killer cell activating receptor-mediated ligand-specific expansion. J Exp Med (2022) 219(8):e20212434. doi: 10.1084/jem.20212434
89. Milling LE, Garafola D, Agarwal Y, Wu S, Thomas A, Donahue N, et al. Neoadjuvant STING activation, extended half-life IL2, and checkpoint blockade promote metastasis clearance Via sustained NK-cell activation. Cancer Immunol Res (2022) 10(1):26–39. doi: 10.1158/2326-6066.CIR-21-0247
90. Bernard P-L, Delconte R, Pastor S, Laletin V, Costa Da Silva C, Goubard A, et al. Targeting cish enhances natural cytotoxicity receptor signaling and reduces NK cell exhaustion to improve solid tumor immunity. J Immunother Cancer (2022) 10(5):e004244. doi: 10.1136/jitc-2021-004244
91. Witalisz-Siepracka A, Klein K, Zdarsky B, Stoiber D. The multifaceted role of Stat3 in NK-cell tumor surveillance. Front Immunol (2022) 13:947568. doi: 10.3389/fimmu.2022.947568
92. Lv L, Wang H, Shi W, Wang Y, Zhu W, Liu Z, et al. A homodimeric IL-15 superagonist F4rli with easy preparation, improved half-life, and potent antitumor activities. Appl Microbiol Biotechnol (2022) 106(21):7039–50. doi: 10.1007/s00253-022-12209-191
93. Liu J-Q, Zhang C, Zhang X, Yan J, Zeng C, Talebian F, et al. Intratumoral delivery of IL-12 and IL-27 mrna using lipid nanoparticles for cancer immunotherapy. J Control Release (2022) 345:306–13. doi: 10.1016/j.jconrel.2022.03.021
94. Kono K, Takahashi A, Ichihara F, Sugai H, Fujii H, Matsumoto Y. Impaired antibody-dependent cellular cytotoxicity mediated by herceptin in patients with gastric cancer. Cancer Res (2002) 62(20):5813–7.
95. Sönmez C, Wölfer J, Holling M, BrokiNKel B, Stummer W, Wiendl H, et al. Blockade of inhibitory killer cell immunoglobulin-like receptors and IL-2 triggering reverses the functional hypoactivity of tumor-derived NK-cells in glioblastomas. Sci Rep (2022) 12(1):6769. doi: 10.1038/s41598-022-10680-4
96. Li S, Mirlekar B, Johnson BM, Brickey WJ, Wrobel JA, Yang N, et al. STING-induced regulatory b cells compromise NK function in cancer immunity. Nature (2022) 610(7931):373–80. doi: 10.1038/s41586-022-05254-3
97. González-Ochoa S, Tellez-Bañuelos MC, Méndez-Clemente AS, Bravo-Cuellar A, Hernández Flores G, Palafox-Mariscal LA, et al. Combination blockade of the Il6r/Stat-3 axis with tigit and its impact on the functional activity of NK cells against prostate cancer cells. J Immunol Res (2022) 2022:1810804. doi: 10.1155/2022/1810804
98. Rosario M, Liu B, Kong L, Collins LI, Schneider SE, Chen X, et al. The IL-15-Based ALT-803 complex enhances FcγrIIIa-triggered NK cell responses and in vivo clearance of b cell lymphomas. Clin Cancer Res (2016) 22(3):596–608. doi: 10.1158/1078-0432.CCR-15-1419
99. Siebert N, Leopold J, Zumpe M, Troschke-Meurer S, Biskupski S, Zikoridse A, et al. The immunocytokine FAP-IL-2v enhances anti-neuroblastoma efficacy of the anti-Gd2 antibody dinutuximab beta. Cancers (Basel) (2022) 14(19):4842. doi: 10.3390/cancers14194842
100. Galot R, Le Tourneau C, Saada-Bouzid E, Daste A, Even C, Debruyne P, et al. A phase II study of monalizumab in patients with Recurrent/Metastatic squamous cell carcinoma of the head and neck: The I1 cohort of the eortc-Hncg-1559 upstream trial. Eur J Cancer (2021) 158:17–26. doi: 10.1016/j.ejca.2021.09.003
101. Herbst RS, Majem M, Barlesi F, Carcereny E, Chu Q, Monnet I, et al. Coast: An open-label, phase II, multidrug platform study of durvalumab alone or in combination with oleclumab or monalizumab in patients with unresectable, stage III non-Small-Cell lung cancer. J Clin Oncol (2022) 40(29):3383–93. doi: 10.1200/JCO.22.00227
102. TiNKer AV, Hirte HW, Provencher D, Butler M, Ritter H, Tu D, et al. Dose-ranging and cohort-expansion study of monalizumab (Iph2201) in patients with advanced gynecologic malignancies: A trial of the Canadian cancer trials group (Cctg): Ind221. Clin Cancer Res (2019) 25(20):6052–60. doi: 10.1158/1078-0432.CCR-19-0298
103. Chu Y, Nayyar G, Jiang S, Rosenblum JM, Soon-Shiong P, Safrit JT, et al. Combinatorial immunotherapy of n-803 (IL-15 superagonist) and dinutuximab with ex vivo expanded natural killer cells significantly enhances in vitro cytotoxicity against Gd2 pediatric solid tumors and in vivo survival of xenografted immunodeficient nsg mice. J Immunother Cancer (2021) 9(7):e002267. doi: 10.1136/jitc-2020-002267
104. Lagassé HAD, Hopkins LB, JaNKowski W, Jacquemin MG, Sauna ZE, Golding B. Factor VIII-fc activates natural killer cells fc-mediated interactions with CD16. Front Immunol (2021) 12:692157. doi: 10.3389/fimmu.2021.692157
105. Murer P, Kiefer JD, Plüss L, Matasci M, Blümich SL, Stringhini M, et al. Targeted delivery of tnf potentiates the antibody-dependent cell-mediated cytotoxicity of an anti-melanoma immunoglobulin. J Invest Dermatol (2019) 139(6):1339–48. doi: 10.1016/j.jid.2018.11.028
106. Pereira NA, Chan KF, Lin PC, Song Z. The “Less-Is-More” in therapeutic antibodies: Afucosylated anti-cancer antibodies with enhanced antibody-dependent cellular cytotoxicity. MAbs (2018) 10(5):693–711. doi: 10.1080/19420862.2018.1466767
107. Hsiao H-C, Fan X, Jordan RE, Zhang N, An Z. Proteolytic single hinge cleavage of pertuzumab impairs its fc effector function and antitumor activity in vitro and in vivo. Breast Cancer Res (2018) 20(1):43. doi: 10.1186/s13058-018-0972-4
108. Kinder M, Greenplate AR, Grugan KD, Soring KL, Heeringa KA, McCarthy SG, et al. Engineered protease-resistant antibodies with selectable cell-killing functions. J Biol Chem (2013) 288(43):30843–54. doi: 10.1074/jbc.M113.486142
109. Oberg HH, Kellner C, Gonnermann D, Sebens S, Bauerschlag D, Gramatzki M, et al. Tribody [(HER2)XCD16] is more effective than trastuzumab in enhancing Γδ T cell and natural killer cell cytotoxicity against HER2-expressing cancer cells. Front Immunol (2018) 9:814. doi: 10.3389/fimmu.2018.00814
110. Lin X, Li F, Gu Q, Wang X, Zheng Y, Li J, et al. Gold-seaurchin based immunomodulator enabling photothermal intervention and αCD16 transfection to boost NK cell adoptive immunotherapy. Acta Biomater (2022) 146:406–20. doi: 10.1016/j.actbio.2022.04.029
111. Hong S, Yu C, Rodrigues E, Shi Y, Chen H, Wang P, et al. Modulation of siglec-7 signaling Via in situ-created high-affinity cis-ligands. ACS Cent Sci (2021) 7(8):1338–46. doi: 10.1021/acscentsci.1c00064
112. Hong S, Yu C, Wang P, Shi Y, Cao W, Cheng B, et al. Glycoengineering of NK cells with glycan ligands of CD22 and selectins for b-cell lymphoma therapy. Angew Chem Int Ed Engl (2021) 60(7):3603–10. doi: 10.1002/anie.202005934
113. Madsen CB, Lavrsen K, Steentoft C, Vester-Christensen MB, Clausen H, Wandall HH, et al. Glycan elongation beyond the mucin associated tn antigen protects tumor cells from immune-mediated killing. PloS One (2013) 8(9):e72413. doi: 10.1371/journal.pone.0072413
114. Liu S, Galat V, Galat Y, Lee YKA, Wainwright D, Wu J. NK cell-based cancer immunotherapy: From basic biology to clinical development. J Hematol Oncol (2021) 14(1):7. doi: 10.1186/s13045-020-01014-w
115. Liu E, Tong Y, Dotti G, Shaim H, Savoldo B, Mukherjee M, et al. Cord blood NK cells engineered to express IL-15 and a CD19-targeted CAR show long-term persistence and potent antitumor activity. Leukemia (2018) 32(2):520–31. doi: 10.1038/leu.2017.226
116. Hu Z. Tissue factor as a new target for CAR-NK cell immunotherapy of triple-negative breast cancer. Sci Rep (2020) 10(1):2815. doi: 10.1038/s41598-020-59736-3
117. Cao B, Liu M, Wang L, Liang B, Feng Y, Chen X, et al. Use of chimeric antigen receptor NK-92 cells to target mesothelin in ovarian cancer. Biochem Biophys Res Commun (2020) 524(1):96–102. doi: 10.1016/j.bbrc.2020.01.053
118. Eitler J, Wotschel N, Miller N, Boissel L, Klingemann HG, Wels W, et al. Inability of granule polarization by NK cells defines tumor resistance and can be overcome by CAR or ADCC mediated targeting. J Immunother Cancer (2021) 9(1):e001334. doi: 10.1136/jitc-2020-001334
119. Portillo AL, Hogg R, Poznanski SM, Rojas EA, Cashell NJ, Hammill JA, et al. Expanded human NK cells armed with CAR uncouple potent anti-tumor activity from off-tumor toxicity against solid tumors. iScience. (2021) 24(6):102619. doi: 10.1016/j.isci.2021.102619
120. Christodoulou I, Ho WJ, Marple A, Ravich JW, Tam A, Rahnama R, et al. Engineering CAR-NK cells to secrete IL-15 sustains their anti-aml functionality but is associated with systemic toxicities. J Immunother Cancer (2021) 9(12):e003894. doi: 10.1136/jitc-2021-003894
121. Vacchelli E, Aranda F, Bloy N, Buqué A, Cremer I, Eggermont A, et al. Trial watch-immunostimulation with cytokines in cancer therapy. Oncoimmunology (2016) 5(2):e1115942. doi: 10.1080/2162402X.2015.1115942
122. Rak GD, Mace EM, Banerjee PP, Svitkina T, Orange JS. Natural killer cell lytic granule secretion occurs through a pervasive actin network at the immune synapse. PloS Biol (2011) 9(9):e1001151. doi: 10.1371/journal.pbio.1001151
123. Sanborn KB, Mace EM, Rak GD, Difeo A, Martignetti JA, Pecci A, et al. Phosphorylation of the myosin IIa tailpiece regulates single myosin IIa molecule association with lytic granules to promote NK-cell cytotoxicity. Blood (2011) 118(22):5862–71. doi: 10.1182/blood-2011-03-344846
124. Brown AC, Oddos S, Dobbie IM, Alakoskela JM, Parton RM, Eissmann P, et al. Remodelling of cortical actin where lytic granules dock at natural killer cell immune synapses revealed by super-resolution microscopy. PloS Biol (2011) 9(9):e1001152. doi: 10.1371/journal.pbio.1001152
Keywords: HER-2 positive breast cancer, NK cells, ADCC, immunotherapy approach, monoclonal antibodies
Citation: Li F and Liu S (2022) Focusing on NK cells and ADCC: A promising immunotherapy approach in targeted therapy for HER2-positive breast cancer. Front. Immunol. 13:1083462. doi: 10.3389/fimmu.2022.1083462
Received: 29 October 2022; Accepted: 05 December 2022;
Published: 19 December 2022.
Edited by:
Shaoquan Zheng, The First Affiliated Hospital of Sun Yat-sen University, ChinaReviewed by:
Yun Shi, Sichuan University, ChinaVladimir Ponomarev, Memorial Sloan Kettering Cancer Center, United States
Copyright © 2022 Li and Liu. This is an open-access article distributed under the terms of the Creative Commons Attribution License (CC BY). The use, distribution or reproduction in other forums is permitted, provided the original author(s) and the copyright owner(s) are credited and that the original publication in this journal is cited, in accordance with accepted academic practice. No use, distribution or reproduction is permitted which does not comply with these terms.
*Correspondence: Sheng Liu, lshtcm@163.com