Increase in fine root biomass enhances root exudation by long-term soil warming in a temperate forest
- 1Department of Forest Ecology and Soils, Federal Research and Training Centre for Forests, Natural Hazards and Landscape—BFW, Vienna, Austria
- 2Division of Terrestrial Ecosystem Research, Department of Microbiology and Ecosystem Science, Center for Microbiology and Environmental Systems Science, University of Vienna, Vienna, Austria
- 3Department of Soil Ecology, Bayreuth Center of Ecology and Environmental Research (BayCEER), University of Bayreuth, Bayreuth, Germany
- 4Department of Forest and Soil Sciences, Institute of Soil Research, University of Natural Resources and Life Sciences (BOKU), Vienna, Austria
Trees can invest up to one-third of the carbon (C) fixed by photosynthesis into belowground allocation, including fine root exudation into the rhizosphere. It is still unclear how climate and soil warming affect tree root C exudation, in particular quantifying longer-term warming effects remains a challenge. In this study, using a C-free cuvette incubation method, in situ C exudation rates from tree fine roots of a mature spruce dominated temperate forest were measured in regular intervals during the 14th and 15th year of experimental soil warming (+ 4°C). In addition, a short-term temperature sensitivity experiment (up to + 10°C warming within 4 days) was conducted to determine the inherent temperature sensitivity of root exudation. Root exudation rates in the long-term warmed soil (17.9 μg C g–1 root biomass h–1) did not differ from those in untreated soil (16.2 μg C g–1 root biomass h–1). However, a clear increase (Q10 ∼5.0) during the short-term temperature sensitivity experiment suggested that fine root exudation can be affected by short-term changes in soil temperature. The absence of response in long-term warmed soils suggests a downregulation of C exudation from the individual fine roots in the warmed soils. The lack of any relationship between exudation rates and the seasonal temperature course, further suggests that plant phenology and plant C allocation dynamics have more influence on seasonal changes in fine root C exudation. Although exudation rates per g dry mass of fine roots were only marginally higher in the warmed soil, total fine root C exudation per m2 soil surface area increased by ∼30% from 0.33 to 0.43 Mg C ha–1 yr–1 because long-term soil warming has led to an increase in total fine root biomass. Mineralization of additional fine root exudates could have added to the sustained increase in soil CO2 efflux from the warmed forest soil at the experimental site.
Highlights
- Similar C exudation rates per surface area of fine roots in untreated and long-term warmed (+4°C) soil.
- About 30% higher total fine root C exudation due to increased fine root biomass in warmed soil.
- Pronounced positive root exudation response (Q10 ∼5) to short-term soil warming.
Introduction
Trees allocate a substantial proportion of the carbon (C) fixed by photosynthesis to belowground fine root exudation (Kannenberg and Phillips, 2017; Liese et al., 2018). Exudates consist of a large variety of C compounds, including sugars, organic acids and amino acids, which represent easily accessible C sources for rhizosphere microbes, providing C for growth and energy for breakdown of soil organic matter (SOM) into bioavailable nutrients (Read and Perez-Moreno, 2003; Kuzyakov, 2010; Bengtson et al., 2012; Keiluweit et al., 2015). In forests, root exudation is controlled by photosynthesis, belowground C transport, root growth, and mycorrhizal associations, which in turn are influenced by tree species and by abiotic factors such as solar radiation flux, soil nutrient availability, and soil moisture and temperature (Kuzyakov, 2002; Gärdenäs et al., 2011; Phillips et al., 2011; Yin et al., 2013; Canarini et al., 2019). Changes in the trees’ belowground C allocation are expected under global warming, potentially affecting the cycling and budgets of forest soil C and nutrients (Chapin et al., 2009; Panchal et al., 2022). Global warming is expected to increase tree root exudation directly due to an acceleration of plant/root metabolic processes (Uselman et al., 2000; Phillips et al., 2011; Yin et al., 2013), but can simultaneously have indirect effects by affecting soil nutrient availability, root traits, or soil moisture (Tückmantel et al., 2017).
The direct temperature response of root exudation is related to the temperature dependency of passive diffusion processes, of the membrane stability of root tips, and of root metabolic processes (Neumann and Romheld, 2000). Earlier studies demonstrated a positive temperature response of root exudation in laboratory settings (Bekkara et al., 1998; Uselman et al., 2000), in studies comparing root exudation in ecosystems across mean annual temperature gradients (Meier et al., 2020; Yang et al., 2020) and in short-term warming experiments in the field (Wang et al., 2021). According to the results of these studies, increasing soil temperatures should increase fine root exudation, at least in the short-term. The responses of root exudation to long-term soil warming have not yet been studied in detail. In contrast to direct short-term effects of warming, long-term effects on root exudation may be more strongly related to indirect effects, e.g., by changing nutrient availability or soil moisture, which in turn can affect root morphology and physiology (Pregitzer et al., 2000; Li et al., 2019). Trees were shown to invest more C into root exudation under nutrient deficiency, such as of nitrogen (N) or phosphorus (P) (Ward et al., 2011; Yin et al., 2014; Meier et al., 2020). This was also corroborated by a decrease in root exudation rates following N fertilization (Phillips et al., 2009, 2011). One frequently observed mechanism of how plants cope with nutrient deficiency is an increase in specific root length (SRL), in root surface area (RSA), and in the number of root tips, resulting in an overall increase in total fine root biomass (FRB) and in absorptive area (Weemstra et al., 2020). On the other hand, more, longer, and thinner roots with shorter lifespans tend to be metabolically more active, and hence exude more labile C into their rhizosphere (Tückmantel et al., 2017).
Soil warming can negatively affect soil moisture, and thereby alter root exudation rates. Recent reviews suggested that root exudation could decrease under water deficit, due to slower root growth (Lehto and Zwiazek, 2011; Brunner et al., 2015). However, other studies showed exudation rates to be unaffected or to increase under drought stress, due to roots accumulating compatible solutes as osmolytes (sugars, amino acids), major compounds that are also exuded, under mild drought stress, or due to cell damage and leakage of cell contents under severe drought stress (Preece et al., 2018; Jakoby et al., 2020; Brunn et al., 2022).
Experimental soil warming allows for the estimation of direct warming effects on root exudation rates in situ (Yin et al., 2013; Xiong et al., 2020; Wang et al., 2021). Moreover, if warming is imposed for longer timescales, such experiments could provide insights into indirect long-term effects (Melillo et al., 2011; Leppälammi-Kujansuu et al., 2014). However, to date, only a very limited number of soil warming experiments in forests have investigated root exudation. In those studies, root exudation rates were mostly found to increase, but little is known on the underlying mechanisms (Yin et al., 2013; Zhang et al., 2016; Wang et al., 2021; Liu et al., 2022). For instance, due to warming-induced increases in soil N availability we would expect a decrease in root exudation (Yin et al., 2013; Wang et al., 2021). However, to our best knowledge, only one study so far reported decreased exudation rates due to soil warming (Xiong et al., 2020), which was associated with reduced fine root activity above optimal temperatures and with lowered soil moisture. All the cited studies either were rather short-term experiments (<5 years) and/or root exudation rates were measured on tree seedlings (<4 years of age) (Yin et al., 2013; Zhang et al., 2016; Wang et al., 2021; Liu et al., 2022). The potential long-term warming response of fine root exudation in mature forests remains unresolved. Moreover, root exudation might also be downregulated in long-term warmed plots (sensu Leuschner et al., 2022), which calls for studies of the short-term temperature sensitivity (e.g., Q10) of root exudation to better understand acclimation processes, though we are not aware on any studies of this.
We here utilized the infrastructure of the Achenkirch soil warming experiment (Schindlbacher et al., 2009, 2015) to assess the short-term (hourly to daily) response to increasing soil temperature, as well as the long-term effects of constant soil warming by 4°C over 14 years on C exudation from fine roots of mature spruce trees in a temperate mountain forest. We hypothesized that (i) root exudation rates respond positively to increasing soil temperatures in the short- and long-term and that (ii) long-term warming leads to a sustained increase in C exudation from fine roots, thereby providing a higher input of labile C compounds to the warmed soil.
Materials and methods
Site description and experimental setup
The study was conducted at the soil warming experiment in Achenkirch (Austria), located at 910 m a.s.l. in a 130-year old forest on a north-northeast slope in the Northern Tyrolian Limestone Alps (47° 34′ 50″ N; 11° 38′ 21″E). Climate conditions at the site are cool and humid, with mean annual air temperature and precipitation of 7.0°C and 1493 mm, respectively (1988–2017 ZAMG). The forest is dominated by Norway spruce (Picea abies,∼80%), silver fir (Abies alba,∼5%) and European Beech (Fagus sylvatica,∼15%), the latter of which also mainly accounts for the natural understory. Soils developed from dolomite bedrock have near neutral pH, high carbonate contents and form a heterogeneous mosaic of Chromic Cambisols and shallow Rendzic Leptosols covered by mull humus averaging 1–3 cm in thickness. Organic C stocks were estimated at ∼120 t ha–1 in the top 20 cm of the mineral soil and ∼10 t ha–1 in the organic layer. A detailed description of the soils is given by Herman et al. (2002).
Starting with three plot pairs in 2005 and enlarged in 2007, the soil warming experiment now includes six plot pairs, which are distributed at similar slope and aspect conditions at the site. Each pair consists of two subplots (2 × 2 m each), one being heated by +4°C using buried infrared heating cables (0.4 cm diameter, TECUTE—0.18 Ohm m–1 UV–1, Etherma, Salzburg) and one being equipped with dummy cables serving as control. Cables were installed at 3 cm soil depth with a spacing of 7.5 cm in between. Soil warming was continuously switched on during the snow-free season (April/May—November/December) and turned off during periods of snow cover.
Soil warming is controlled via soil temperature sensors (PT100; EMS, Brno, Czech Republic), which were placed between heating cables at 5 cm depth into the mineral soil. Soil temperature and moisture were measured automatically in each plot using GS3 sensors (DECAGON, Washington, DC, USA) installed at 5 cm mineral soil depth and were recorded as half hourly means on three Em50 dataloggers (DECAGON, Washington, DC, USA). Additionally, we measured soil moisture manually using a handheld thermometer and a FieldScout soil moisture meter (TDR 100, Spectrum Technologies, Inc., Haltom City, TX, USA) three times within each subplot during measurement campaigns, respectively.
Fine root density is highest in O and A horizons (Schindlbacher et al., 2009). Living FRB ranged between ∼400 and ∼600 g m–2, and stocks were ∼20% higher in warmed plots in 2019, 1 year prior to the current study (Kwatcho Kengdo et al., 2022). Warming had increased FRB particularly in the topsoil (0–10 cm) layer, which harbors between 80 and 90% of the absorptive FRB in the mineral soil (Kwatcho Kengdo et al., 2022).
To assess long-term warming effects on fine root C exudation, root exudates were sampled from control and long-term warmed soil in five campaigns (July 2020, October 2020, April 2021, July 2021, and October 2021), during the 14th and 15th year of soil warming, respectively. During each campaign, root exudates from two terminate fine root branches per treatment plot (n = 12 root branches per control and 12 branches per warning treatment) were sampled for a direct comparison between treatments.
An additional short-term soil warming experiment was conducted to test if fine root exudations show an inherent response to rapidly increasing soil temperatures. Soil temperature in warmed plots was stepwise increased during four consecutive days in April 2021 (fist day 9.2 ± 0.2°C, second day 10.5 ± 0.5°C, third day 12.3 ± 0.9°C, fourth day 15.8 ± 1.1°C). During each day a set of fine root branches (one root branch per plot, n = 6) was excavated and incubated for exudate sampling in the warmed soil. The same number of terminal root branches (n = 6) were sampled in untreated control plots to account for potential confounding effects of other parameters than increasing soil temperature (e.g., soil moisture, C flow below ground). Soil temperature in unwarmed control soils was 5.1 ± 0.6°C throughout this experiment. In all experiments we aimed at sampling root exudates solely from the dominating spruce trees to avoid potentially interfering effects of tree species origin with those of the main treatments (warming, control). We therefore visually pre-select spruce fine roots for these experiments, based on our previous experience with visually differentiating spruce from beech roots.
Root exudation sampling and analysis
Root exudates were sampled using a modified cuvette-method described by Phillips et al. (2008). A terminal fine root branch consisting of first to third order fine roots < 2 mm diameter and of about 5–10 cm length was carefully excavated from a mineral soil depth of 3–5 cm. The root branch was carefully washed with de-ionized water to remove adhering soil, and if necessary, remaining particles were removed with fine forceps. After a resting period of ∼2 h, during which the cleaned roots were protected from sunlight and kept moist by enclosing them in a wet paper tissue, the excavated root branch was inserted in a 30 ml glass syringe without plunger which was equipped with a three-way Luer lock. Then, the syringe was carefully filled with acid-washed glass beads (1 mm in diameter) to mimic structural conditions in the soil before being flushed twice with 30 ml 0.5 mM CaCl2 to remove adhering SOM and C compounds accumulated during/shortly after the potentially stressful excavation and rinsing process. We used CaCl2 solution to prevent exudation responses to altered nutrient availability in the exudation medium. Low concentrated Ca2+ stabilizes plasma membranes (Tuna et al., 2007; Melcrová et al., 2016) and therefore curtails unspecific leakage processes. This approach relies on intact rhizodermal membranes and has often been applied in nutrient uptake studies, which are similar to exudation studies (Marschner, 1995). After flushing, to keep roots moist, syringes were refilled with ∼ 15 ml of solution before they were sealed with parafilm around the root entering location and placed into a plastic bag to avoid any contamination from outside. Syringes with fine roots were then buried at 3–5 cm soil depth for an incubation duration of 22 h. In warmed plots, the syringes were buried exactly in between two heating cable lines. Additionally, two syringes containing no root, but solely glass beads, were incubated at a random subplot of each treatment serving as controls. Preliminary tests with handheld thermometers confirmed that the temperatures inside the syringes closely resembled soil temperatures at both treatments. After incubation, syringes were excavated and exudates, which had accumulated throughout the incubation period, were collected by (i) sucking out the CaCl2 solution with another sterile syringe, followed by (ii) flushing the glass beads, in the syringe two times with CaCl2 solution via the Luer-lock using a sterile syringe. The sample solution and the solution obtained from the two flushes were then mixed and immediately filtered (0.20 μm) and frozen within 2–4 h. All sample solutions were analysed for dissolved organic carbon (DOC) concentration using a Shimadzu TOC-VCPH/CPNT-NM1 analyser (Tokyo, Japan).
Root sampling and analyses
Roots were cut at the location where they entered the syringe and carefully rinsed from glass beads, after exudate sampling was competed. The root segments were cooled at +4°C, until they were scanned for morphological traits (root length, root surface area, and root volume) using WinRHIZO 2004b (Regents Instruments Inc., Quebec, Canada). Afterward, fine roots were freeze-dried to determine root dry mass and root exudation rates were calculated as mean hourly exudation rates from C concentrations in syringes accumulated over the incubation time relative to the sample root biomass (μg C g–1 h–1), root length (μg C cm–1 h–1) and root surface area (μg C cm–2 h–1). Root biomass specific traits [specific root length (SRL), specific root area (SRA), root tissue density (RTD)] were calculated as the ratio of root dry mass to root biomass, area, and volume, respectively. A sub-sample of each root branch was collected for genetic analysis. These subsamples were taken from the same root branches, but from just outside the incubated (syringe) root segment. Samples were cooled at +4 °C until arrival in the lab, where they were kept at −18°C until further analysis. The tree species origin of subsamples of each studied fine root segment was determined by genetic analysis following Brunner et al. (2001). Briefly, DNA was extracted from the roots, and segments of chloroplast DNA were amplified using universal polymerase chain reaction (PCR) primer pairs (trnL exon, or “ucp c and d” as of Taberlet et al., 1991). Resulting DNA was electrophoresed through agarose, either directly after PCR or after additional incubation with the restriction enzymes MspI or AluI. Resulting DNA banding patterns were visually assessed relative to reference DNA samples from spruce and beech needles and leaves/buds, run on the same agarose gels.
Upscale to growing season exudation
Living FRB stocks of 355 and 414 g m–2 in the top 20 cm of control and warmed soils, respectively, (Kwatcho Kengdo et al., 2022) were combined with exudation rates per FRB (μg C g–1 h–1) to estimate cumulative growing season exudation rates (Mg C ha–1 yr–1). We did this by first calculating a grand mean of average exudation rates per FRB across all sampling campaigns for control and warmed soils, respectively. These mean values per g of FRB were then used to calculate exudation rates per m2 of forest soil by taking into account the total FRB reported above. Finally, mean exudation rates per m2 (μg C m–2 h1) were then up scaled linearly to Mg C ha–1 yr–1 to an average growing season of 240 days.
Temperature sensitivity of exudation rates
The temperature sensitivity of root exudation was calculated using the measured absolute exudation rates during the short-term temperature increase experiment. Additionally, the temperature sensitivity was calculated by using the differences between simultaneously measured exudation rates in control and warmed soils (real warming effect). Since soil temperature remained nearly stable in control plots throughout the short-term experiment, the use of control-warmed differences should have allowed for eliminating the effects of other simultaneously interfering parameters, such as soil temperature independent temporal variation in below ground C transport (Phillips et al., 2008).
Q10 was then calculated as:
where T1 and T2 are measured soil temperatures, and R1 and R2 are the mean exudation rates at T1 and T2 in warmed plots. Using the above formula, another set of Q10 values was calculated to compare the response in warmed plots with exudation rates in unwarmed control soils. For this calculation, R2 was set to R1 plus the mean treatment difference in exudation rates between treatments at T2 instead of the absolute exudation rates at T2. We performed exponential regression to find best-fit functions across all temperature steps, reported via coefficients of determination (R2) and a significance level of p < 0.05. Q10 values and best-fit functions were calculated using the R “respirometry” package.
Statistical analysis
Response variables from each regular measurement campaign were averaged within plots before two-way ANOVA followed by Tukey’s HSD post-hoc tests for the effect of warming and sampling date. Multivariate stepwise linear regressions were used to examine the relationship of exudation rates with root morphological traits and soil environmental variables (moisture and temperature). All statistical analyses were performed in R version 3.6 (R Core Team, 2019), and statistical tests were considered significant at p < 0.05.
Results
Long-term warming effects
Soil warming increased mean soil temperature at 5 cm soil depth by 4.1 ± 0.1 °C across all sampling dates, with highest temperatures recorded in July 2020 (15.8 ± 0.2 and 19.8 ± 1.2°C) and lowest ones in May 2021 (5.4 ± 0.4 and 9.2 ± 0.6°C) at 5 cm soil depth in control and warmed soils, respectively (Figure 1A). Soil moisture was barely affected by soil warming and did not differ significantly between treatments at any sampling date, but showed high variation between individual plots. Average volumetric soil moisture contents were 46.6 ± 8.5 and 47.3 ± 8.0% in control and warmed soils, respectively (Figure 1B).
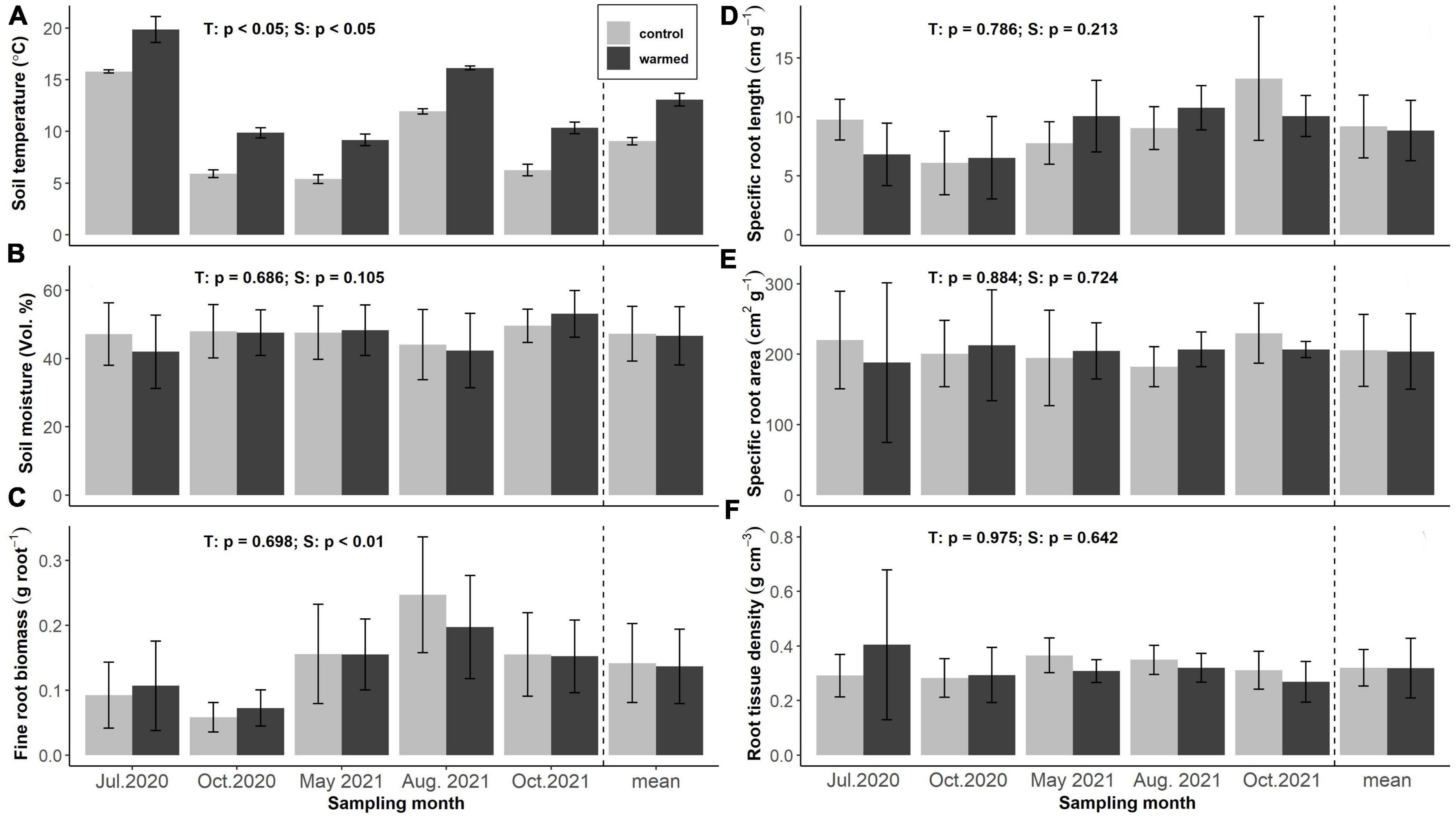
Figure 1. Soil temperature (A), soil moisture (B), fine root biomass (FRB) (C), specific root length (D), specific root area (E), and specific root tissue density (F) of the incubated terminal fine root branches in control and warmed plots across seasons and treatments (n = 12 per bar, light gray: control, dark gray: warming). Annotations indicate p-values of two-way ANOVA (T: treatment, S: season).
All samples showed DNA bands identical to the ones of spruce references. Sixteen out of 128 fine root samples additionally showed more faint DNA bands, hinting at the possible presence of DNA from other plants, including four samples possibly containing traces of beech DNA. This could be due to laboratory contamination (including “overspill” from agarose gel loading), or fragments of DNA from decaying organic matter. As the samples that were processed consisted of single fine roots, and a strong spruce band was always present, it is unlikely that any of the roots were misidentified.
Fine root biomass (FRB), specific root length (SRL), specific fine root area (SRA) and root tissue density (RTD) of the incubated roots did not show significant differences among treatments. Average SRL of the incubated roots was 9.18 ± 2.66 and 8.85 ± 2.56 cm g–1 in control and warmed soils, respectively. Highest values were recorded in October 2021 (10.06 ± 1.74 and 13.25 ± 5.24 cm g–1) and lowest in October 2020 (6.10 ± 2.68 and 6.53 ± 3.50 cm g–1) in control and warmed soils, respectively (Figure 1D). SRA averaged 205.50 ± 51.02 and 203.80 ± 53.66 cm2 g–1 and RTD was 0.32 ± 0.07 and 0.32 ± 0.14 g cm-3 in control and warmed soils, neither parameter showing significant variation across the seasons (Figures 1E, F).
Exudation rates per FRB (μg C g–1 h–1), per fine root length (μg C cm–1 h–1), and per fine root area (μg C cm–2 h–1) did not differ significantly between treatments at any sampling date or overall (Figure 2). Mean exudation rates per FRB were 16.2 ± 4.0 and 17.9 ± 2.9 μg C g–1 h–1 in control and warmed plots, respectively. Exudation rates per FRB were lowest in August 2021 (5.9 ± 1.1 in control and 8.7 ± 1.3 μg C g–1 h–1 in warmed soil) and reached their highest values in October 2020 in control plots (26.4 ± 5.8 g–1 h–1) and October 2021 in warmed plots (22.9 ± 4.8 μg C g–1 h–1, Figure 2A). Exudation rates per fine root length followed similar trends, with minima in August 2021 (0.006 ± 0.002 and 0.008 ± 0.002 μg C cm–1 h–1) and maxima in October 2020 (0.058 ± 0.014 and 0.046 ± 0.010 μg C cm–1 h–1) in control and warmed soils, respectively. Across sampling dates, exudation rates based on root length averaged 0.025 ± 0.006 and 0.023 ± 0.005 μg C cm–1 h–1 in control and warmed soils (Figure 2B). Similarly, exudation rates per fine root area were lowest in August 2021 (0.032 ± 0.005 and 0.044 ± 0.007 μg C cm–2 h–1) and highest in October 2020 (0.148 ± 0.031 and 0.118 ± 0.020 μg C cm–2 h–1) in control and warmed soils, respectively. Across sampling dates, they averaged 0.09 ± 0.02 μg C cm–2h–1 from roots in both control and warmed soils (Figure 2C).
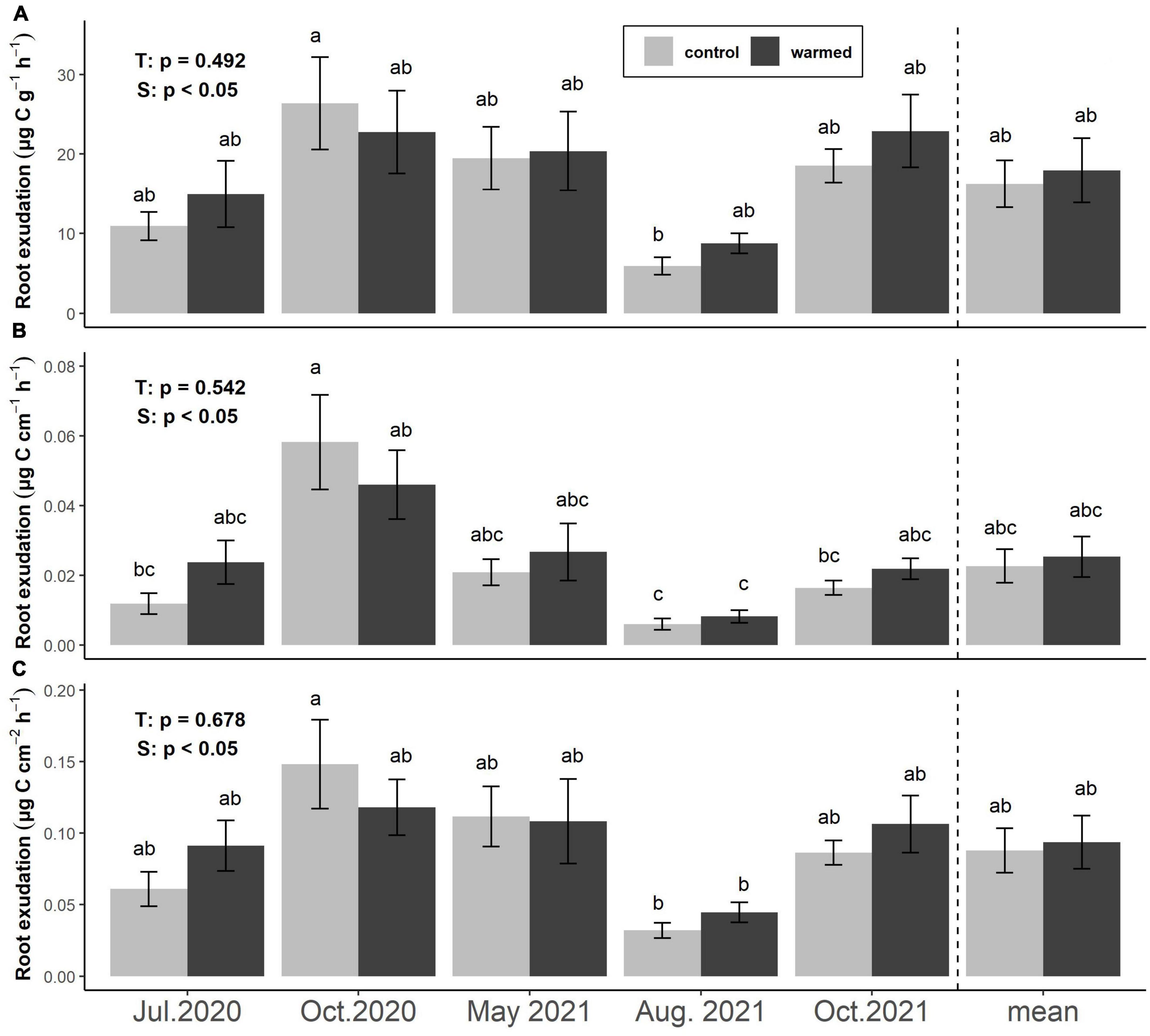
Figure 2. Effects of warming and season on fine root exudation rates based on the specific FRB (A), root length (B), and root surface area (C). Bars represent means ± SE of 6 plot pairs (n = 12 per bar) and overall means during the whole study period (n = 60 per bar). Annotations indicate p-values of two-way ANOVA (T: treatment, S: season) and significant differences between treatments and seasons are indicated by different letters.
Multiple linear regression analysis revealed that across treatments and sampling dates, morphological traits of the incubated roots were not related significantly to exudation rates per FRB (R2 = 0.04, p = 0.47) and per fine root area (R2 = 0.09, p = 0.08), while specific root length and root tissue density showed weak but significantly negative relationships to exudation rates per length (R2 = 0.29, p < 0.001). Soil temperature and moisture were not significantly related to any root trait-specific exudation measure across dates or treatments.
Short-term response and temperature sensitivity
Root exudation rates responded positively to increasing soil temperature. Across the 4-day experiment, average daily soil temperature in warmed soils increased from 9.2 ± 0.2 to 15.8 ± 1.1°C, while soil temperature in control soil varied between 4.1 ± 0.1 and 5.7 ± 0.3°C (Supplementary Figure 1), resulting in mean temperature differences between treatments rising from 3.8 to 10.8°C throughout the experiment. Soil moisture was nearly constant and unaffected by short-term warming (Supplementary Figure 1). Mean exudation rates per root biomass (μg C g–1 h–1) increased with increasing temperature (Figure 3A). However, although soil temperature was nearly constant, exudation rates showed significant daily variations in control plots (Figure 3A). The differences between the daily exudation rates in control and warmed plots increased exponentially with the rising temperature as well (Figure 3B; R2 = 0.94, p < 0.05), suggesting a strong temperature dependency of the exudate flux, with a Q10 of 4.97.
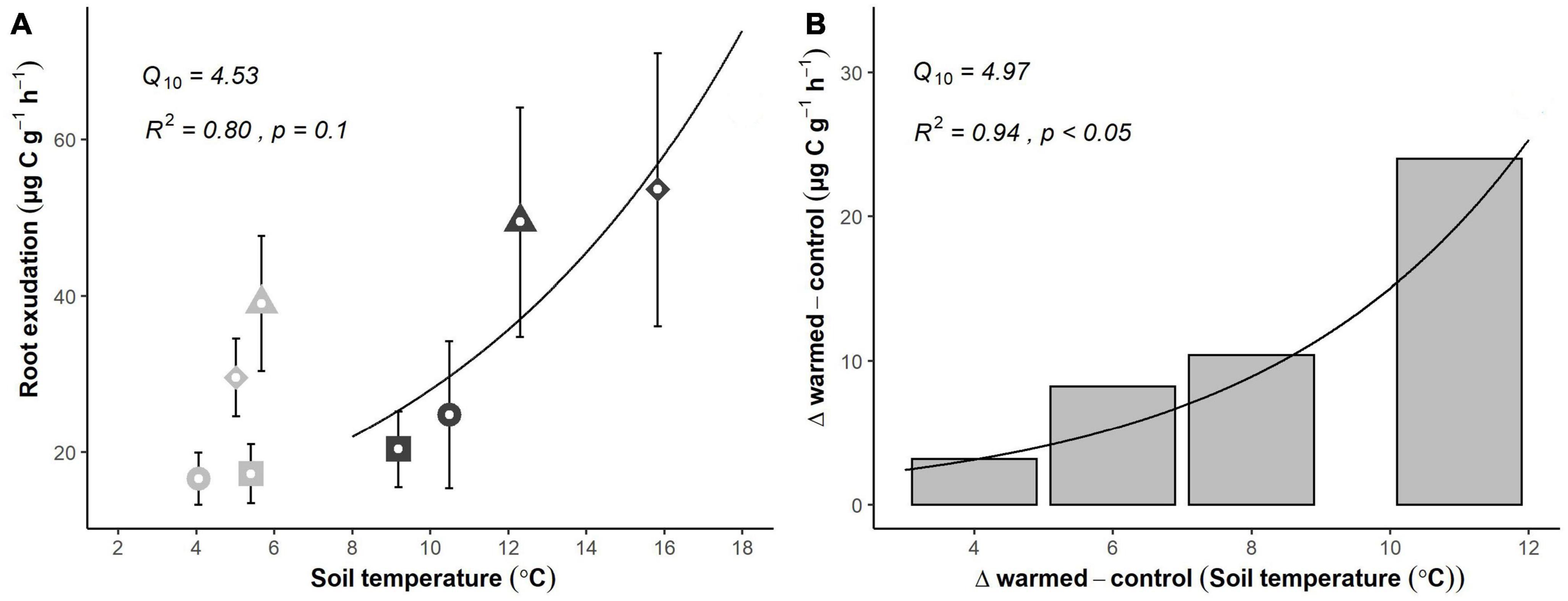
Figure 3. Temperature sensitivity of fine root exudation rates based on a short-term (4 days, April 2021) warming experiment. (A) Absolute exudation rates of fine roots in control (gray) and warmed (black) plots. Roots incubated at the same day are indicated by sharing the same symbol. (B) Average differences in exudation rates between control and warmed treatments (the actual temperature response) throughout the experiment. Symbols and error bars represent means ± SE of 6 plot pairs (n = 6 per treatment). R-squared, p-value and Q10 value were derived from best fit exponential curve.
Discussion
Rates of fine root C exudation depend on various biotic and abiotic factors, which are prone to modification by climate warming. A climate change induced increase in soil temperatures, could affect the below-ground allocation of labile plant C on different temporal scales, which is still a major unknown in the response of soil biogeochemical cycles to climate warming (Pendall et al., 2008; Classen et al., 2015). Here, we studied if and how long-term soil warming affected fine root C exudation rates of mature forest trees. Simultaneously, we assessed the inherent temperature sensitivity of root exudation of the same trees by exposing them to short-term increasing temperatures.
The rates of root exudation observed in this study were in the lower range of studies in similar settings in mature forests, with exudation rates per FRB of 12—100 μg C g–1 h–1 (Phillips et al., 2011; Tückmantel et al., 2017; Jakoby et al., 2020; Meier et al., 2020; Leuschner et al., 2022), but were notably lower than exudation rates observed from seedlings of age < 6 years (Yin et al., 2013; Zhang et al., 2016; Xiong et al., 2020; Yang et al., 2020), where rates of up to 2000 μg C g–1 h–1 were observed.
Temperature sensitivity
Confirming our first hypothesis, fine root C exudation showed a positive response to increasing soil temperature in the short-term experiment, suggesting a strong temperature sensitivity (Q10 ∼5). This confirms a generally positive relationship of exudation rates to temperature, associated with inherent temperature effects on root metabolic activity (Repo et al., 2014; Onwuka and Mang, 2018). However, the absence of a warming effect on exudation rates at 4°C difference and the fluctuation of exudation patterns in unwarmed control plots throughout the experiment suggest that, in short-term time frames, factors other than soil temperature influence root exudation patterns. In comparison to previous studies, our novel design of the short-term field experiment enabled a discrete evaluation of the temperature effect on root exudation, highlighting the significant temperature sensitivity of exudation processes in situ. Assuming same disturbances of roots in control and warming plots, the differences in root exudation (Figure 3B) show the real short-term temperature sensitivity of root exudation. It still has to be considered, however, that roots in long-term warmed plots (only there we had heating cables available to conduct the short-term warming) were compared with roots in unwarmed control soil. Hence, it cannot be taken for granted that the root exudation in control soil shows an identical short-term response to warming. Warming, for instance, also affected the root ectomycorrhizal community and soil nutrient availability (Kwatcho Kengdo et al., 2022; Tian et al., 2023).
Seasonal exudation patterns
While individual studies reported a relationship between root exudation rates and seasonal soil temperature (Yin et al., 2014; Xiong et al., 2020), others did not, and identified other factors such as root morphology, rooting depth, or C allocation to roots as primarily influencing temporal site-specific exudation patterns (Phillips et al., 2008; Tückmantel et al., 2017; Nakayama and Tateno, 2018; Yang et al., 2020). The seasonal patterns observed in our study, with highest exudation rates in spring and autumn and lower exudation during summer, imply that other factors had overridden temperature effects at our site. The studied mountain forest is characterized by a distinctive seasonal climate, with snow cover from Nov/Dec to Apr/May, during which soil temperatures remain slightly above freezing, and by highest soil temperatures of around 15°C during Jul/Aug. As photosynthesis of trees is primarily limited by solar radiation flux at this elevation, the highest photosynthetic C assimilation and highest GPP could be expected to occur during the summer months (Bergh et al., 1998). Root respiration rates have been shown to peak during summer at the study site (Schindlbacher et al., 2009), hence indicating increased root activity during this season. Why root exudation nonetheless remained lowest during the summer remains unresolved. One explanation might be that assimilates were preferentially used for stem and/or root growth before summertime, potentially resulting in lower exudation rates per fine root but more fine roots in general during warmer months. Halted growth but on-going photosynthesis may have produced a surplus of fixed C later in the autumn season, which then was allocated to the roots and released in the form of exudates (Prescott et al., 2020) or was invested into the build-up of C reserves in roots and stems. Though speculative, during springtime the mobilization of C reserves from stems and roots for leaf production might also increase the cellular concentrations of low molecular weight C metabolites in roots, triggering enhanced root exudation early in the year. Moreover, ectomycorrhiza (ECM) play an important role in nutrient uptake in many temperate trees and consume a varying but large proportion of tree photosynthates. Colonization by ECM fungi increases root exudation relative to trees associated with arbuscular mycorrhizal fungi (Yin et al., 2014), though not universally (Liese et al., 2018). Seasonal changes in the colonization and activity of ECM associations could therefore also alter temporal exudation patterns of the spruce fine roots. However, further investigations are needed to explore the role of ECM and fine root growth with respect to seasonal and total root exudation.
Soil drying was frequently reported to result in increased root exudation rates (Jakoby et al., 2020; Brunn et al., 2022). In this study, soil moisture was relatively constant throughout the seasons (Figure 1) and had correspondingly weak explanatory power with regard to the seasonal changes in root exudation. Root exudation during winter was not assessed in this study, but can be considered to be low, with air temperatures and radiation being too low for significant photosynthesis and topsoil temperatures close to zero degrees. Fine root respiration is a magnitude lower beneath the snow cover than during the snow-free season (Schindlbacher et al., 2014), indicating generally lower root activities during wintertime.
Interestingly, seasonal exudation patterns were the same in the untreated and the long-term warmed soils. Since long-term warming had already altered soil nutrient availability (see below), and FRB and morphology (Kwatcho Kengdo et al., 2022), the coincidental temporal pattern suggests that the seasonal variability in root exudation was primarily driven by changes in the C supply from the above ground tree component, which can be considered as largely unaffected by the soil-warming treatment in the comparatively small plots.
The morphology of the incubated fine roots explained a small part of the temporal variability of root exudation throughout the seasons, independent of warming. Trends toward higher exudation rates with greater specific root length and surface areas (Supplementary Figure 2) were not statistically significant in our study (Table 1). Hence, seasonal changes in such root morphology parameters might as well play a role in explaining the patterns observed in root exudation (Meier et al., 2020). On the other hand, it cannot be ruled out that the observed differences in root morphology during different measurement dates were just a matter of coincidence regarding which fine root branches were dug out and sampled for root exudates. However, this is unlikely since the morphological patterns always were congruent among control and warmed plots (n = 6 plots or 12 root branches per treatment, respectively, Figure 1). This can only be indisputably tested by concurrent coring of fine roots in control and heated plots for area-based assessments of FRB and of root traits such as SRL, SRA and RTD, alongside sampling the roots for exudation measurements, which was beyond the scope of this study.
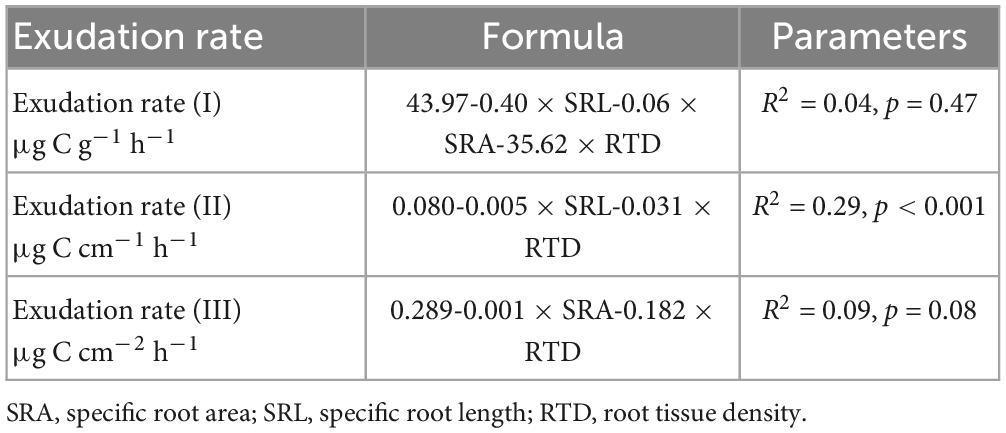
Table 1. Results from multivariate stepwise regression for exudation rates per fine root biomass (FRB) (I), fine root length (II), and based on fine root area (III) based on root morphological parameters.
Long-term soil warming response
Contrary to our hypothesis (ii), we observed no significant increase in C exudation rates of fine roots in the long-term warmed soil (Figure 2). These results are not supporting the findings of other short-term studies in forests, where warming increased (Yin et al., 2013; Zhang et al., 2016; Wang et al., 2021; Liu et al., 2022) or decreased (Xiong et al., 2020) exudation rates of tree fine roots. Several studies reported that warming affected root exudation rates via indirect effects on the soil supply and plant demand for nutrients, by adaptions in root morphology, or via changes in soil moisture, all of which can occur both, rapidly or over longer time periods (Yin et al., 2013; Zhang et al., 2016; Xiong et al., 2020; Wang et al., 2021; Liu et al., 2022). One common rationale is that warming promotes plant and root growth, which results in increased plant nutrient demand, leading to elevated root exudation rates, potentially further promoted by low soil moisture (Yin et al., 2013; Holz et al., 2018; Preece et al., 2018; Xiong et al., 2020).
Since soil warming did not significantly reduce soil moisture and soil moisture is generally high at the study site (Figure 1A), any warming induced moisture effects can be ruled out. Root activity was therefore not affected by very low or very high soil moisture. Long-term soil warming can, however, affect the availability of nutrients to plant roots. Nitrogen availability was shown to increase during initial warming (Melillo et al., 2011; Dawes et al., 2017), due to increased mineralization rates, but warming could also decrease soil N availability in the longer-run, due to elevated N leaching losses and increased denitrification rates (Dai et al., 2020). Recent studies of coupled manipulations of soil warming and N fertilization showed that warming only accelerated root exudation rates when N was limited, but increased N availability by fertilizer addition suppressed the warming response of root exudation (Yin et al., 2013; Wang et al., 2021). Assessments of N and P cycling at our long-term warming site pointed toward emerging P limitation in warmed soils (Tian et al., 2023). Nitrogen availability was not yet significantly affected by long-term warming at the site, and diffuse N fluxes also indicated a strong seasonal variability in plant N availability (Heinzle et al., 2021). The seasonal variations in N availability did, however, not match the seasonal root exudation patterns as observed in this study. Hence, N availability likely was not a major control of actual exudation rates. Limited research has been conducted on the effect of P deficiency on root exudation rates, especially in forests. Studies of non-mycorrhizal crop plants reported elevated exudation rates under P deficiency (Zhang et al., 2016), while a recent study on mature beech trees across multiple sites did not reveal a correlation between exudation rates and P availability (Meier et al., 2020). The latter is in line with our findings, as exudation rates did not show a response to the emerging P limitation in the warmed soils at the site (Shi et al., 2023; Tian et al., 2023).
Though the exudation rates of fine root segments did not differ in control and warmed soil, it has to be considered that long-term warming increased FRB stocks as well as fine root production and turnover rates at the study site (Kwatcho Kengdo et al., 2023). Applying the estimates of total FRB stocks assessed at the site in 2019 (355 and 414 g m–2 in control and warmed soils, Kwatcho Kengdo et al., 2022) and mean exudation rates per FRB estimated in this study, C exudation in the top 20 cm of the forest soil amounted to ∼0.43 and ∼0.33 t C ha–1 yr–1 in warmed and control plots, which is equivalent to a ∼30% overall increase in C exudation rates in the long-term warmed soils. Hence, the increase in FRB stock, rather the long-term effect on the exudation rates of the fine roots led to overall higher root exudation in warmed soil. Generally, measured fine root exudation rates in the current study may underestimate the true exudation for two reasons. First, the excavation of fine root cohorts and removal of adherent soil particles has likely damaged a considerable portion of root hairs and the mycelium of ECM fungi, leading to the disruption of the fine root–microbe–soil continuum. Second, a certain fraction of root exudates could have been resorbed or taken up and mineralized by rhizosphere microorganisms present on the coarsely cleaned root segments (Oburger and Jones, 2018). It should as well be noted that although the method of exudate collection developed by Phillips et al. (2008) was widely applied in tree fine root exudation studies, the individual methodological modifications in root resting times, incubation durations, sample solution compositions, etc. varied substantially among individual studies (compiled in Supplementary material).
The higher total fine root stock in warmed soils may as well explain why exudation rates of fine roots did not show a clear response to warming. If the C flow from the tree’s above ground compartments was a generally limiting factor (which can be considered of importance for the poorly growing 130-years old spruce trees), a similar amount of aboveground C (+30%) would be needed to be distributed to the larger root surface/biomass in the warmed soils, hence proportionally reducing the C supply to the individual fine roots. This could also be an explanation for the apparent lack of response of the exudation of individual fine roots to the 4°C soil temperature increase.
Unfortunately, we did not assess root exudation rates in the first years of the soil warming experiment. We therefore are unable to compare initial warming effects with the long-term ones assessed in the current study. In the long-term, however, we clearly showed downregulation via thermal acclimation of root exudation to soil warming, similar to other processes of plant carbon turnover, such as respiration and photosynthesis (Atkin and Tjoelker, 2003; Hikosaka et al., 2006).
Long-term warming further affected not only FRB stocks, but also fine root morphology at our site (Parts et al., 2019; Kwatcho Kengdo et al., 2022). Exudation rates in temperate deciduous forests have been commonly reported to be closely related to root morphological parameters such as SRL and SRA, as thinner and longer roots with greater surface area have been shown to exude more labile C (Yin et al., 2013; Tückmantel et al., 2017; Meier et al., 2020). In other warming studies, an increase in SRL and SRA as well as in FRB for optimization of plant nutrient and water acquisition resulted in a positive warming effect on root exudation rates (Yin et al., 2013; Wang et al., 2021). We did not observe a significant difference in morphological parameters of the fine roots, which were selected for the exudation measurements in the current study. This was likely a matter of comparatively low replication and the use of terminal fine root segments for incubation and trait measurements. However, an earlier study at our site found increased FRB, SRL and SRA in warmed soils using ingrowth cores after 15 years of warming (Kwatcho Kengdo et al., 2022). Despite the relationship between morphological parameters and exudation rates presented here not being strong and significant across treatments and seasons, it cannot be excluded that long-term changes in fine root morphology affected exudation rates, but quantification of these effects was not possible within the framework of our study design.
Overall, the enhanced fine root exudation in warmed soil could be a significant contribution to the ongoing high soil CO2 efflux from the long-term warmed plots (unpublished data). Labile C, which is exuded from fine roots, may, to a large extent, be directly consumed by rhizosphere microorganisms and thus directly contribute to the increased soil CO2 efflux (Kuzyakov, 2002).
Conclusion
The current study encompassed a single aspect of the forest ecosystem’s response to global change—namely the response of fine root exudation to rising soil temperatures. With the present experimental setup, we demonstrated that warming has increased fine root C exudation at the ecosystem level, rather by increasing FRB than by promoting C exudation at the fine root level. Fine roots showed thermal acclimation of the root exudation process in the long-term, but strong temperature stimulation in the short-term (Q10 up to 5). Our results suggest that the long-term response to warming likely differs from the short-term response of single fine roots, and that it depends on how the whole fine root systems (biomass, morphology), as well as soil nutrient availability, will respond to warming. Future tree root exudation will further depend on how climate warming will affect photosynthesis rates and hence the below ground transfer of assimilates. The long-term response of root exudation to soil warming per se, which was assessed in this study, however, is an important piece in this puzzle of forest ecosystem responses to climate change.
Data availability statement
The raw data supporting the conclusions of this article will be made available by the authors, without undue reservation.
Author contributions
AS and JH designed and planned the experiment. JH, XL, and YT conducted the field measurements. JH performed the root scanning, compiled and analyzed all data, and wrote the first draft of the manuscript. AN did genetical analysis. XL, YT, BH, and WW supervised the laboratory analysis. All authors contributed to the discussion of the results and the final version of the manuscript.
Funding
This work was funded by the Austrian Science Fund – FWF (project I 3745).
Acknowledgments
We thank Eva Oburger for supporting us with her insights into root exudation measurement techniques and root physiology. We also thank Boris Rewald who kindly lent us a transportable root scanner when we were in need of one. Isabel Fürhapper was a lab intern at BFW and worked on some of the DNA experiments and Owen Bradley at BFW helped to improve the quality of the manuscript by proofreading.
Conflict of interest
The authors declare that the research was conducted in the absence of any commercial or financial relationships that could be construed as a potential conflict of interest.
Publisher’s note
All claims expressed in this article are solely those of the authors and do not necessarily represent those of their affiliated organizations, or those of the publisher, the editors and the reviewers. Any product that may be evaluated in this article, or claim that may be made by its manufacturer, is not guaranteed or endorsed by the publisher.
Supplementary material
The Supplementary Material for this article can be found online at: https://www.frontiersin.org/articles/10.3389/ffgc.2023.1152142/full#supplementary-material
Supplementary Figure 1 | Soil temperature (°C) and water filled pore space (WFPS) (%) at 5 cm depth during the temperature increase experiment in April (Figure 3) for warmed (black) and control plots (dash-dotted). Lines represent mean values recorded by data loggers at 15 min interval from all plots (n = 6 per treatment).
Supplementary Figure 2 | Relationships of hourly root exudation rates with (a) soil temperature, (b) volumetric soil moisture content, (c) specific root length (SRL), and (d) root surface area for both warmed and control treatments. Each point represents one single fine root exudation rate. Lines represent linear best fits from which r-squared and p-values were derived.
References
Atkin, O. K., and Tjoelker, M. G. (2003). Thermal acclimation and the dynamic response of plant respiration to temperature. Trends Plant Sci. 8, 343–351. doi: 10.1016/S1360-1385(03)00136-5
Bekkara, F., Jay, M., Viricel, M. R., and Rome, S. (1998). Distribution of phenolic compounds within seed and seedlings of two Vicia faba cvs differing in their seed tannin content, and study of their seed and root phenolic exudations. Plant Soil 203, 27–36. doi: 10.1023/A:1004365913726
Bengtson, P., Barker, J., and Grayston, S. J. (2012). Evidence of a strong coupling between root exudation, C and N availability, and stimulated SOM decomposition caused by rhizosphere priming effects. Ecol. Evol. 2, 1843–1852. doi: 10.1002/ece3.311
Bergh, J., McMurtrie, R. E., and Linder, S. (1998). Climatic factors controlling the productivity of Norway spruce: A model-based analysis. For. Ecol. Manage. 110, 127–139. doi: 10.1016/S0378-1127(98)00280-1
Brunn, M., Hafner, B. D., Zwetsloot, M. J., Weikl, F., Pritsch, K., Hikino, K., et al. (2022). Carbon allocation to root exudates is maintained in mature temperate tree species under drought. New Phytol. 235, 965–977. doi: 10.1111/nph.18157
Brunner, I., Brodbeck, S., Büchler, U., and Sperisen, C. (2001). Molecular identification of fine roots of trees from the Alps: Reliable and fast DNA extraction and PCR-RFLP analyses of plastid DNA. Mol. Ecol. 10, 2079–2087. doi: 10.1046/j.1365-294X.2001.01325.x
Brunner, I., Herzog, C., Dawes, M. A., Arend, M., and Sperisen, C. (2015). How tree roots respond to drought. Front. Plant Sci. 6:547. doi: 10.3389/fpls.2015.00547
Canarini, A., Kaiser, C., Merchant, A., Richter, A., and Wanek, W. (2019). Root exudation of primary metabolites: Mechanisms and their roles in plant responses to environmental stimuli. Front. Plant Sci. 10:157. doi: 10.3389/fpls.2019.00157
Chapin, F. S. III, McFarland, J., David McGuire, A., Euskirchen, E. S., Ruess, R. W., and Kielland, K. (2009). The changing global carbon cycle: Linking plant–soil carbon dynamics to global consequences. J. Ecol. 97, 840–850. doi: 10.1111/j.1365-2745.2009.01529.x
Classen, A. T., Sundqvist, M. K., Henning, J. A., Newman, G. S., Moore, J. A., Cregger, M. A., et al. (2015). Direct and indirect effects of climate change on soil microbial and soil microbial-plant interactions: What lies ahead?. Ecosphere 6, 1–21. doi: 10.1890/ES15-00217.1
Dai, Z., Yu, M., Chen, H., Zhao, H., Huang, Y., Su, W., et al. (2020). Elevated temperature shifts soil N cycling from microbial immobilization to enhanced mineralization, nitrification and denitrification across global terrestrial ecosystems. Glob. Chang. Biol. 26, 5267–5276. doi: 10.1111/gcb.15211
Dawes, M. A., Schleppi, P., Hättenschwiler, S., Rixen, C., and Hagedorn, F. (2017). Soil warming opens the nitrogen cycle at the alpine treeline. Glob. Chang. Biol. 23, 421–434. doi: 10.1111/gcb.13365
Gärdenäs, A. I., Ågren, G. I., Bird, J. A., Clarholm, M., Hallin, S., Ineson, P., et al. (2011). Knowledge gaps in soil carbon and nitrogen interactions–From molecular to global scale. Soil Biol. Biochem. 43, 702–717. doi: 10.1016/j.soilbio.2010.04.006
Heinzle, J., Wanek, W., Tian, Y., Kengdo, S. K., Borken, W., Schindlbacher, A., et al. (2021). No effect of long-term soil warming on diffusive soil inorganic and organic nitrogen fluxes in a temperate forest soil. Soil Biol. Biochem. 158:108261. doi: 10.1016/j.soilbio.2021.108261
Herman, F., Smidt, S., Englisch, M., Feichtinger, F., Gerzabek, M., Haberhauer, G., et al. (2002). Investigations of nitrogen fluxes and pools on a limestone site in the Alps. Environ. Sci. Pollut. Res. 9, 46–52. doi: 10.1007/BF02987478
Hikosaka, K., Ishikawa, K., Borjigidai, A., Muller, O., and Onoda, Y. (2006). Temperature acclimation of photosynthesis: Mechanisms involved in the changes in temperature dependence of photosynthetic rate. J. Exp. Bot. 57, 291–302. doi: 10.1093/jxb/erj049
Holz, M., Zarebanadkouki, M., Kaestner, A., Kuzyakov, Y., and Carminati, A. (2018). Rhizodeposition under drought is controlled by root growth rate and rhizosphere water content. Plant Soil 423, 429–442. doi: 10.1007/s11104-017-3522-4
Jakoby, G., Rog, I., Megidish, S., and Klein, T. (2020). Enhanced root exudation of mature broadleaf and conifer trees in a Mediterranean forest during the dry season. Tree Physiol. 40, 1595–1605. doi: 10.1093/treephys/tpaa092
Kannenberg, S. A., and Phillips, R. P. (2017). Plant responses to stress impacts: The C we do not see. Tree Physiol. 37, 151–153. doi: 10.1093/treephys/tpw108
Keiluweit, M., Bougoure, J. J., Nico, P. S., Pett-Ridge, J., Weber, P. K., and Kleber, M. (2015). Mineral protection of soil carbon counteracted by root exudates. Nat. Clim. Chang. 5, 588–595. doi: 10.1038/nclimate2580
Kuzyakov, Y. (2002). Factors affecting rhizosphere priming effects. J. Plant Nutr. Soil Sci. 165, 382–396. doi: 10.1002/1522-2624(200208)165:4<382::AID-JPLN382>3.0.CO;2-#
Kuzyakov, Y. (2010). Priming effects: Interactions between living and dead organic matter. Soil Biol. Biochem. 42, 1363–1371. doi: 10.1016/j.soilbio.2010.04.003
Kwatcho Kengdo, S., Ahrens, B., Tian, Y., Heinzle, J., Wanek, W., Schindlbacher, A., et al. (2023). Increase in carbon input by enhanced fine root turnover in a long-term warmed forest soil. Sci. Total Environ. 855:158800. doi: 10.1016/j.scitotenv.2022.158800
Kwatcho Kengdo, S., Peršoh, D., Schindlbacher, A., Heinzle, J., Tian, Y., Wanek, W., et al. (2022). Long-term soil warming alters fine root dynamics and morphology, and their ectomycorrhizal fungal community in a temperate forest soil. Glob. Chang. Biol. 28, 3441–3458. doi: 10.1111/gcb.16155
Lehto, T., and Zwiazek, J. J. (2011). Ectomycorrhizas and water relations of trees: A review. Mycorrhiza 21, 71–90. doi: 10.1007/s00572-010-0348-9
Leppälammi-Kujansuu, J., Salemaa, M., Kleja, D. B., Linder, S., and Helmisaari, H. S. (2014). Fine root turnover and litter production of Norway spruce in a long-term temperature and nutrient manipulation experiment. Plant Soil 374, 73–88. doi: 10.1007/s11104-013-1853-3
Leuschner, C., Tückmantel, T., and Meier, I. C. (2022). Temperature effects on root exudation in mature beech (Fagus sylvatica L.) forests along an elevational gradient. Plant Soil 481, 147–163. doi: 10.1007/s11104-022-05629-5
Li, F., Hu, H., McCormlack, M. L., Feng, D. F., Liu, X., and Bao, W. (2019). Community-level economics spectrum of fine-roots driven by nutrient limitations in subalpine forests. J. Ecol. 107, 1238–1249. doi: 10.1111/1365-2745.13125
Liese, R., Lübbe, T., Albers, N. W., and Meier, I. C. (2018). The mycorrhizal type governs root exudation and nitrogen uptake of temperate tree species. Tree Physiol. 38, 83–95. doi: 10.1093/treephys/tpx131
Liu, W., Jiang, Y., Su, Y., Smoak, J. M., and Duan, B. (2022). Warming affects soil nitrogen mineralization via changes in root exudation and associated soil microbial communities in a subalpine tree species Abies fabri. J. Soil Sci. Plant Nutr. 22, 406–415. doi: 10.1007/s42729-021-00657-z
Marschner, H. (1995). Mineral nutrition of higher plants. Stuttgart: Institute of Plant Nutrition, University of Hohenheim.
Meier, I. C., Tückmantel, T., Heitkötter, J., Müller, K., Preusser, S., Wrobel, T. J., et al. (2020). Root exudation of mature beech forests across a nutrient availability gradient: The role of root morphology and fungal activity. New Phytol. 226, 583–594. doi: 10.1111/nph.16389
Melcrová, A., Pokorna, S., Pullanchery, S., Kohagen, M., Jurkiewicz, P., Hof, M., et al. (2016). The complex nature of calcium cation interactions with phospholipid bilayers. Sci. Rep. 6:38035. doi: 10.1038/srep38035
Melillo, J. M., Butler, S., Johnson, J., Mohan, J., Steudler, P., Lux, H., et al. (2011). Soil warming, carbon–nitrogen interactions, and forest carbon budgets. Proc. Natl. Acad. Sci. U.S.A. 108, 9508–9512. doi: 10.1073/pnas.1018189108
Nakayama, M., and Tateno, R. (2018). Solar radiation strongly influences the quantity of forest tree root exudates. Trees 32, 871–879. doi: 10.1007/s00468-018-1685-0
Neumann, G., and Romheld, V. (2000). “The release of root exudates as affected by the plant’s physiological status,” in The rhizosphere, eds S. Willig, Z. Varanini, and P. Nannipieri (Boca Raton: CRC press), 57–110. doi: 10.1201/9780849384974-9
Oburger, E., and Jones, D. L. (2018). Sampling root exudates–mission impossible? Rhizosphere 6, 116–133. doi: 10.1016/j.rhisph.2018.06.004
Onwuka, B., and Mang, B. (2018). Effects of soil temperature on some soil properties and plant growth. Adv. Plants Agric. Res. 8, 34–37. doi: 10.15406/apar.2018.08.00288
Panchal, P., Preece, C., Peñuelas, J., and Giri, J. (2022). Soil carbon sequestration by root exudates. Trends Plant Sci. 27, 749–757. doi: 10.1016/j.tplants.2022.04.009
Parts, K., Tedersoo, L., Schindlbacher, A., Sigurdsson, B. D., Leblans, N. I., Oddsdóttir, E. S., et al. (2019). Acclimation of fine root systems to soil warming: Comparison of an experimental setup and a natural soil temperature gradient. Ecosystems 22, 457–472. doi: 10.1007/s10021-018-0280-y
Pendall, E., Rustad, L., and Schimel, J. (2008). Towards a predictive understanding of belowground process responses to climate change: Have we moved any closer?. Funct. Ecol. 22, 937–940. doi: 10.1111/j.1365-2435.2008.01506.x
Phillips, R. P., Bernhardt, E. S., and Schlesinger, W. H. (2009). Elevated CO2 increases root exudation from loblolly pine (Pinus taeda) seedlings as an N-mediated response. Tree Physiol. 29, 1513–1523. doi: 10.1093/treephys/tpp083
Phillips, R. P., Erlitz, Y., Bier, R., and Bernhardt, E. S. (2008). New approach for capturing soluble root exudates in forest soils. Funct. Ecol. 22, 990–999. doi: 10.1111/j.1365-2435.2008.01495.x
Phillips, R. P., Finzi, A. C., and Bernhardt, E. S. (2011). Enhanced root exudation induces microbial feedbacks to N cycling in a pine forest under long-term CO2 fumigation. Ecol. Lett. 14, 187–194. doi: 10.1111/j.1461-0248.2010.01570.x
Preece, C., Farré-Armengol, G., Llusià, J., and Peñuelas, J. (2018). Thirsty tree roots exude more carbon. Tree Physiol. 38, 690–695. doi: 10.1093/treephys/tpx163
Pregitzer, K. S., Zak, D. R., Maziasz, J., DeForest, J., Curtis, P. S., and Lussenhop, J. (2000). Interactive effects of atmospheric CO2 and soil-N availability on fine roots of Populus tremuloides. Ecol. Appl. 10, 18–33. doi: 10.1890/1051-0761(2000)010[0018:IEOACA]2.0.CO;2
Prescott, C. E., Grayston, S. J., Helmisaari, H. S., Kaštovská, E., Körner, C., Lambers, H., et al. (2020). Surplus carbon drives allocation and plant–soil interactions. Trends Ecol. Evol. 35, 1110–1118. doi: 10.1016/j.tree.2020.08.007
R Core Team (2019). R: A language and environment for statistical computing. Vienna: R Foundation for Statistical Computing. Available online at: https://www.R-project.org/
Read, D. J., and Perez-Moreno, J. (2003). Mycorrhizas and nutrient cycling in ecosystems–a journey towards relevance?. New Phytol. 157, 475–492. doi: 10.1046/j.1469-8137.2003.00704.x
Repo, T., Sirkiä, S., Heinonen, J., Lavigné, A., Roitto, M., Koljonen, E., et al. (2014). Effects of frozen soil on growth and longevity of fine roots of Norway spruce. For. Ecol. Manag. 313, 112–122. doi: 10.1016/j.foreco.2013.11.002
Schindlbacher, A., Jandl, R., and Schindlbacher, S. (2014). Natural variations in snow cover do not affect the annual soil CO2 efflux from a mid-elevation temperate forest. Glob. Chang. Biol. 20, 622–632. doi: 10.1111/gcb.12367
Schindlbacher, A., Schnecker, J., Takriti, M., Borken, W., and Wanek, W. (2015). Microbial physiology and soil CO2 efflux after 9 years of soil warming in a temperate forest–no indications for thermal adaptations. Glob. Chang. Biol. 21, 4265–4277. doi: 10.1111/gcb.12996
Schindlbacher, A., Zechemeister-Boltenstern, S., and Jandl, R. (2009). Carbon losses due to soil warming: Do autotrophic and heterotrophic soil respiration respond equally?. Glob. Chang. Biol. 15, 901–913. doi: 10.1111/j.1365-2486.2008.01757.x
Shi, C., Urbina-Malo, C., Tian, Y., Heinzle, J., Kwatcho Kengdo, S., Inselsbacher, E., et al. (2023). Does long-term soil warming affect microbial element limitation?x2 A test by short-term assays of microbial growth responses to labile C, N and P additions. Glob. Chang. Biol. 29, 2188–2202. doi: 10.1111/gcb.16591
Taberlet, P., Gielly, L., Pautou, G., and Bouvet, J. (1991). Universal primers for amplification of three non-coding regions of chloroplast DNA. Plant Mol. Biol. 17, 1105–1109. doi: 10.1007/BF00037152
Tian, Y., Shi, C., Malo, C. U., Kwatcho Kengdo, S., Heinzle, J., Inselsbacher, E., et al. (2023). Long-term soil warming decreases microbial phosphorus utilization by increasing abiotic phosphorus sorption and phosphorus losses. Nat. Commun. 14:864. doi: 10.1038/s41467-023-36527-8
Tückmantel, T., Leuschner, C., Preusser, S., Kandeler, E., Angst, G., Mueller, C. W., et al. (2017). Root exudation patterns in a beech forest: Dependence on soil depth, root morphology, and environment. Soil Biol. Biochem. 107, 188–197. doi: 10.1016/j.soilbio.2017.01.006
Tuna, A. L., Kaya, C., Ashraf, M., Altunlu, H., Yokas, I., and Yagmur, B. (2007). The effects of calcium sulphate on growth, membrane stability and nutrient uptake of tomato plants grown under salt stress. Environ. Exp. Bot. 59, 173–178. doi: 10.1016/j.envexpbot.2005.12.007
Uselman, S. M., Qualls, R. G., and Thomas, R. B. (2000). Effects of increased atmospheric CO2, temperature, and soil N availability on root exudation of dissolved organic carbon by a N-fixing tree (Robinia pseudoacacia L.). Plant Soil 222, 191–202. doi: 10.1023/A:1004705416108
Wang, Q., Chen, L., Xu, H., Ren, K., Xu, Z., Tang, Y., et al. (2021). The effects of warming on root exudation and associated soil N transformation depend on soil nutrient availability. Rhizosphere 17:100263. doi: 10.1016/j.rhisph.2020.100263
Ward, C. L., Kleinert, A., Scortecci, K. C., Benedito, V. A., and Valentine, A. J. (2011). Phosphorus-deficiency reduces aluminium toxicity by altering uptake and metabolism of root zone carbon dioxide. J. Plant Physiol. 168, 459–465. doi: 10.1016/j.jplph.2010.08.005
Weemstra, M., Kiorapostolou, N., van Ruijven, J., Mommer, L., de Vries, J., and Sterck, F. (2020). The role of fine-root mass, specific root length and life span in tree performance: A whole-tree exploration. Funct. Ecol. 34, 575–585. doi: 10.1111/1365-2435.13520
Xiong, D., Huang, J., Yang, Z., Cai, Y., Lin, T. C., Liu, X., et al. (2020). The effects of warming and nitrogen addition on fine root exudation rates in a young Chinese-fir stand. For. Ecol. Manage. 458:117793. doi: 10.1016/j.foreco.2019.117793
Yang, L., Wang, X., Mao, Z., Jiang, Z., Gao, Y., Chen, X., et al. (2020). Root exudation rates decrease with increasing latitude in some tree species. Forests 11:1045. doi: 10.3390/f11101045
Yin, H., Li, Y., Xiao, J., Xu, Z., Cheng, X., and Liu, Q. (2013). Enhanced root exudation stimulates soil nitrogen transformations in a subalpine coniferous forest under experimental warming. Glob. Chang. Biol. 19, 2158–2167. doi: 10.1111/gcb.12161
Yin, H., Wheeler, E., and Phillips, R. P. (2014). Root-induced changes in nutrient cycling in forests depend on exudation rates. Soil Biol. Biochem. 78, 213–221. doi: 10.1016/j.soilbio.2014.07.022
Keywords: soil warming, root exudation, temperature sensitivity, forest soil, tree fine roots, forest carbon
Citation: Heinzle J, Liu X, Tian Y, Kwatcho Kengdo S, Heinze B, Nirschi A, Borken W, Inselsbacher E, Wanek W and Schindlbacher A (2023) Increase in fine root biomass enhances root exudation by long-term soil warming in a temperate forest. Front. For. Glob. Change 6:1152142. doi: 10.3389/ffgc.2023.1152142
Received: 27 January 2023; Accepted: 24 April 2023;
Published: 11 May 2023.
Edited by:
Laynara Figueiredo Lugli, Technical University of Munich, GermanyReviewed by:
Melanie Brunn, University of Koblenz-Landau, GermanyNdzana Georges Martial, University of Dschang, Cameroon
Lingyan Zhou, East China Normal University, China
Copyright © 2023 Heinzle, Liu, Tian, Kwatcho Kengdo, Heinze, Nirschi, Borken, Inselsbacher, Wanek and Schindlbacher. This is an open-access article distributed under the terms of the Creative Commons Attribution License (CC BY). The use, distribution or reproduction in other forums is permitted, provided the original author(s) and the copyright owner(s) are credited and that the original publication in this journal is cited, in accordance with accepted academic practice. No use, distribution or reproduction is permitted which does not comply with these terms.
*Correspondence: Andreas Schindlbacher, andreas.schindlbacher@bfw.gv.at