The altitudinal distribution characteristics of functional traits reflect the resource allocation strategy of Abies georgei var. smithii in southeast Tibet
- 1Institute of Tibet Plateau Ecology, Tibet Agricultural and Animal Husbandry University, Nyingchi, China
- 2Key Laboratory of Forest Ecology in Tibet Plateau, Tibet Agricultural and Animal Husbandry University, Ministry of Education, Nyingchi, China
- 3Linzhi National Forest Ecosystem Observation and Research Station of Tibet, Nyingchi, China
- 4Key Laboratory of Alpine Vegetation Ecological Security in Tibet, Nyingchi, China
To explore the adaptation strategies of the aboveground and underground functional traits of alpine plants along an altitudinal gradient, a typical stand of primitive dark coniferous forests (Abies georgei var. smithii.) in southeastern Tibet was taken as the research object in the present study. PCA and correlation analyses were carried out for different organ functional traits (19 key indicators in total), then RDA analysis was done in conjunction with 12 environmental factors. The variation characteristics of the functional traits of leaves, current-year twigs, trunks and fine roots in 6 continuous altitude gradients and the relationships between functional traits and environmental factors were explored. The results showed that soil organic carbon (SOC) may exert a positive effect on the construction of plant defense tissue via changes in functional traits, altitude (Alt) represents the primary influencing factor of wood density (WD) variation, particulate organic carbon (POC) content mainly affected fine root dry matter (RDWC) content and specific root length (SRL), and total potassium (TK) content was the main factor that affected fine root tissue density (RTD). Leaves, current-year twigs, and fine roots exhibited high production or nutrient acquisition capacity at an altitude of 4,000m and showed strong defense and relatively stable water and nutrient transport capacity. In conclusion, the ecological strategy of Abies georgei var. smithii. in Sejila Mountain was more conservative, and the optimal survival area of Abies georgei var. smithii. was located at 4, 000m on the shady slope of Sejila Mountain. It is of paramount significance for exploring the essence of terrestrial ecosystems and their functional processes in extremely high-altitude environments.
1. Introduction
Based on mutual adaptability and coevolution with their habitats, plant functional traits can reflect the ability of plants to acquire, utilize and preserve resources but also closely link the environment, plant individuals and ecosystem structure, process and function (Eller et al., 2020). Plants are construction organisms composed of many complex modules, such as leaves, current-year twigs, stems, and fine roots, which can function independently as a complete unit of plant growth. Additionally, it enjoys a certain degree of regional component autonomy (Yang et al., 2021). Single traits and the combination of multiple traits in the same organ of plants can directly reflect the adaptation and response of plants to the environment and play a key role in indicating environmental changes (Perez-Harguindeguy et al., 2013). In contrast, the combination of functional traits in different plant organs can further describe the trade-off strategy of plant acquisition and utilization of resources (Tonin et al., 2019), which can better display the life history strategies of plants in different environments. As environmental stress has become increasingly serious and obvious, plants tend to support less total leaf area for a given twig cross section (Sun et al., 2006). In terms of light-loving species, the lower wood density was conducive to the rapid elevation of canopy height to receive light. In terms of shade-tolerant species, a higher wood density helps to maintain a higher survival rate under shade conditions (Ramananantoandro et al., 2016). According to the study of Liu et al. (2022), several tree species in karst areas developed a conservative survival strategy with coordinated functional traits such as low SLA, high dry matter content of organs such as leaves, roots and branches, and high tissue density.
Altitude, as a comprehensive reflection of most environmental factors, is the main factor affecting the variation in plant functional traits. Previous studies have shown that the dry matter content, thickness and dry mass of leaves are positively correlated with altitude (Rajsnerová et al., 2015; Thakur et al., 2019); low-altitude species have a larger leaf area but less leaf emergence intensity than high-altitude species (Yang et al., 2012). With increasing altitude, longer and finer roots were produced (Alvarez-Uria and Koerner, 2011), while the tissue density of sequential roots decreased with increasing altitude. Currently, the patterns of functional trait covariation in different organs and how they respond to changes in environmental conditions (e.g., altitude) are still poorly understood at the intraspecies scale (Carvalho et al., 2020). A thorough understanding of the responses of plant adaptation strategies to altitudinal gradients and their correlation with environmental factors can provide theoretical support for the study of the optimal survival zones for specific plants, the development and utilization of plants for soil and water conservation, and the protection of rare and endangered plants. It is, collectively, of great significance for the entire ecological environment and ecological security (Kraft et al., 2015).
Abies georgei var. smithii, a genus of Pinaceae, is an endemic and vulnerable tree species in China and plays an important role in soil and water conservation and regional ecological stability in the Qinghai-Tibet Plateau (Shen et al., 2016). The research achievements related to the functional traits of Abies georgei var. smithii in southeast Tibet has mainly focused on its seedlings (Guo and Zhang, 2015; Zhang et al., 2022), and there are few comprehensive studies on the functional traits of adult trees and their response to their habitats in different altitudinal gradients. Additionally, Abies georgei var. smithii has been listed on the Red List of Species in China as a result of the decrease in distribution area or the decline in habitat quality. Therefore, it is critical to understand the adaptation mechanism of this species in the alpine environment and explore the reasons for the obvious decline in the population. In summary, the main objectives of the present study are as follows: (i) to explore the distribution characteristics of leaves, current-year twigs, trunks and fine root functional traits of Abies georgei var. smithii in seljira mountains along altitudinal gradients; (ii) to investigate the trade-off relationship between functional traits of different organs; and (iii) to analyze the response of functional traits of different organs to environmental factors.
2. Materials and methods
2.1. Study area
The study site is located in Sejila National Forest Park, Bayi District, Nyingchi City, Tibet Autonomous Region (E93°12′–95°35′, N29°10′–30°15′). Sejila Mountain belongs to the remnant of Nianqing Tanggula Mountain. Connecting with the Himalayan mountains, it is located in the southeastern Qinghai-Tibet Plateau in the middle reaches of the Yarlung Zangbo River. In addition, it is one of the important forest areas of primary forest in southeast Tibet (Meng et al., 2018). The overall mountain range follows a northeast–southwest trend with an elevation difference of 3,000 m (2,100–5,300 m) and an area of 2,300 km2. This study is located on the northeast slope of Sejila Mountain, with an altitude of 3,800 ~ 4,300 m and an average slope of approximately 35°. The region is a subalpine cold temperate subhumid zone due to the water–air passage of the Brahmaputra River under the influence of the Indian Ocean warm and wet monsoon. The average annual temperature was 0.73°C below zero. The average temperature of the warmest month (July) was 9.8°C, and the average temperature of the coldest month (January) was 13.8°C below zero. The average annual precipitation, evaporation and relative humidity are 1,134 mm, 554 mm and 78%, respectively (Luo et al., 2021). The region is dominated by mountain brown soil and acid brown soil. The main forest vegetation type is dark coniferous forest in the mountainous temperate zone. Abies georgei var. smithii is the established group species and the dominant species in the timberline. There are also Picea likiangensis var. linzhiensis forest, Abies georgei var. smithii-Picea likiangensis var. linzhiensis mixed forest and Abies georgei var. smithii-Sabina saltuaria mixed forest (Han et al., 2014; Zhou et al., 2015). The research area and locations of the seedling sampling sites are shown in Figure 1.
2.2. Plot setting
Samples were collected according to the standardized measurement manual of plant functional traits (Cornelissen et al., 2003; Perez-Harguindeguy et al., 2013; Moretti et al., 2017). At the end of the growing season for alpine plants on the Tibetan Plateau in October 2020, we established 6 sample plots (50 m × 50 m) in mature forests at intervals of 100 m in the continuous gradient range from 3,800 to 4,300 m above sea level (Figure 1). The general information of each sample plot along the altitude gradient is shown in Table 1. Three 20 m × 20 m quadrats were built at each plot, 18 quadrats in total, and all samples were collected in the quadrats.
2.3. Sampling collection
The standard tree (approx. 30 cm DBH, 18 m height) was selected based on the average of the background survey data, and for special elevations, it was ensured that either the DBH or the height of the tree met one of the above conditions as much as possible. The specific sample collection method and sample quantity are as follows: (i) Three standard trees of Abies georgei var. smithii were selected in each quadrat, and one complete branch was collected from four directions of the middle of the crown by the combination of artificial climbing and high branch shearing. Three mature leaves without obvious damage and one current-year twig were randomly selected from branches in each direction, for a total of 648 mature leaves and 216 current-year twigs. (ii) In each quadrat, three Abies georgei var. smithii with DBH and height basically the same as the standard wood were selected. One immaculate increment core from the bark to the pulp center was drilled with a growth cone at the height of the chest, ensuring at least one corn in four directions, totaling 216 increment core samples. (iii) Three standard trees were explored and excavated along the thick roots in four directions. One complete root with a diameter less than 2 mm was selected by Vernier calipers and stored under sterile low temperature until root scanning. In total, 216 fine root samples were collected. (iv) Three sampling points were randomly selected from each quadrat. After careful removal of the litter decomposition layer and humus layer, 0–20 cm soil samples were collected and cryopreserved in sterile ziplocked bags (incubator + ice pack) and transported back to the laboratory. A total of 18 soil samples were collected.
2.4. Measurement of functional traits
2.4.1. Measurement of leaf traits
The fresh mass (LFW, g) of leaf samples was weighed by an electronic balance (accuracy of 0.001 g) after surface dust was cleaned; leaf thickness (LT, cm) was measured with electronic digital Vernier calipers (accuracy of 0.01 mm); leaf area (LA, cm2) was scanned on a leaf area meter (am-350, ADC BioScientific Ltd., United Kingdom); and the relative content of chlorophyll (RCC, %) was measured with a chlorophyll metre (SPAD-502, Konica Minolta Holdings, Japan). After that, the collected leaves were soaked in distilled water to a constant weight and then removed and wiped dry. The saturated fresh weight (SW, g) was measured with an electronic balance (accuracy of 0.001 g). Finally, we placed the leaves in the oven at 80°C to dry weight (LDW, g). According to Cornelissen et al. (2003) and Perez-Harguindeguy et al. (2013), leaf volume (LV, cm3), specific leaf area (SLA, cm2.g−1), leaf dry matter content (LDMC, g), leaf tissue density (LTD, g.cm−3), and leaf relative water content (RWC, %) were calculated as follows:
LV = LA × LT; SLA = LA/LDW; SLA = LA/LDW; LTD = LDW/LV; RWC = (LFW-LDW)/(SW-LDW) × 100%. All the abbreviations of leaf functional traits are defined in Table 2.
2.4.2. Measurement of functional traits of current-year twigs
The number of leaves on each current-year twig (NL, piece) was recorded, every leaf area (ILA, cm2) was scanned by an LI-3000A leaf area analyzer (am-350, ADC BioScientific Ltd., United Kingdom), and then the total leaf area (TLA, cm2) on each twig was calculated. The length (TL, cm) and diameter (TD, cm) of the twigs were measured with Vernier calipers (accuracy of 0.01 mm), and the cross-sectional area (CA, cm2) and volume (TV, cm3) of the current-year twigs were calculated. Finally, all twig samples were placed in an 80°C oven and dried to constant weight to weigh the dry mass (TDW, g). The study of Sellin et al. (2012) showed that the total leaf area (TLA), leafing intensity based on twig mass (LI, piece·g−1), leafing intensity based on twig length (DLN, pieces·cm−1), twig tissue density (TTD, g·cm−3) and Huber value (HV) were calculated as follows: TLA = NL × ILA; LI = NL/TDW; DLN = NL/TL; TTD = TDW/TV; HV = CA/TLA.
Note that all the abbreviations of the functional traits of current-year twigs are defined in Table 2.
2.4.3. Measurement of wood density
After the increment cores were removed, the bark was removed with a sterile scalpel, and the upper and lower ends were smoothed. The wood density was measured by the saturated water content determination method. It is shown below. The increment cores were immersed in distilled water for 72 h, the surface water was wiped dry, and the saturation mass (mm, g) was weighed by an electronic balance (accuracy of 0.0001 g). The samples were put into the oven at 100 ~ 105°C, and when the weight was constant, they were transferred to the glass dryer for storage, and the absolute dry mass (mo, g) of the core was measured. According to Zhao et al. (2018), the wood density of increment core (ρw, g·cm−3) was calculated as follows:
where ρcw is the cell wall density, that is, the density of the cell wall material, which is generally 1.53 g·cm-3.
2.4.4. Measurement of functional traits of fine roots
The fresh weight (RFW, g) of fine roots was measured after washing and drying by a root scanner (Perfection V700 Photo, Seiko Epson, Japan) combined with a root analysis system (WinRHIZO, Regent Instruments Inc). The root length (RL, cm) and volume (RV, cm3) were obtained by means of determination and analysis of fine roots. Finally, the fine roots were dried at 80°C in an oven until a constant weight was obtained to measure the dry weight of the fine roots (RDW, g). According to Ning et al. (2021), the dry matter content (RDMC, g.g−1), specific root length (SRL, cm.g−1) and root tissue density (RTD, g.cm−3) of fine roots were calculated as follows: RDMC = RDW/RFW; SRL = RL/RDW; RTD = RDW/RV. All the abbreviations of the functional traits of fine roots are defined in Table 2.
2.4.5. Measurement of soil properties
After stones and visible plant roots were removed, the soil samples (approximately 200 g) were passed through a 0.25 mm screen and stored at low temperature (<4°C) to measure the microbial biomass and soil active organic carbon and its components within 48 h. The remaining soil samples (approximately 500 g) were manually ground after natural air drying and passed through a 0.147 mm sieve to determine other soil chemical properties. Soil water content (SWC, %) was measured by the drying method (Chang et al., 2012). Soil organic carbon (SOC, g.kg−1) was measured by the potassium dichromate external heating method; particulate organic carbon (POC, g.kg−1) was assayed using the method of Garten et al. (1999). Readily oxidized organic carbon (ROC, mg.kg−1) was assessed by means of the determination method of Chen et al. (2017). Dissolved organic carbon (DOC, mg.kg−1) was determined using the method of Fang et al. (2014). The microKjeldahl method was developed to determine total nitrogen (TN, g.kg−1); soil total phosphorus (TP, g.kg−1) was determined by HClO4-H2SO4 digestion combined with the molybdenum antimony resistance spectrophotometric method, and soil total potassium (TK, g.kg−1) was determined by HF-HClO4 digestion combined with the flame spectrum method (Shen et al., 2020). Microbial biomass carbon (MBC, mg.kg−1), microbial biomass nitrogen (MBN, mg.kg−1) and microbial biomass phosphorus (MBP, mg.kg−1) were determined by the chloroform fumigation and leaching method (Manral et al., 2022). The statistics of the soil properties of the sample plots at each altitude are shown in Table 3.
2.5. Statistical analysis
All statistics were calculated using SPSS software (v.25.0, IBM Corp., Armonk, NY, United States), and plotting was performed on Origin 2021b (Origin lab, Northampton, MA, United States). A p value < 0.05 was considered to indicate significant statistical effects. In addition, one-way ANOVA was adopted to analyze the significance of the differences in functional traits among altitude gradients. PCA was applied to eliminate functional traits with weak correlation with principal components before Pearson correlation analysis. The relationship between functional traits and soil properties was investigated by means of redundancy analysis (RDA)-constrained ranking of experimental data by using Canoco 5.0 (Microcomputer Power, Ithaca, NY, United States). RDA has two matrices: species data (functional traits-7 leaf traits: LA, LV, SLA, LDMC, LTD, RWC, RCC; 8 current-year twig traits: TL, TD, NL, DLN, TLA, TTD, LI, HV; 1 stem functional trait: WD; 3 fine root traits: RDMC, SRL, RTD) and environmental data (Alt, TN, TP, TK, MBC, MBN, MBP, SOC, POC, DOC, ROC, SWC). The contribution rate of each factor was calculated to determine the variation of a single indicator and make the Sankey diagram. Prior to performing RDA, the significance of the effect of each variable was assessed using a Monte Carlo permutation test, and detrended correspondence analysis was performed on the functional trait data. The results showed that the four ranking axes of the functional traits data were all less than 3.0; therefore, the RDA method could be applied. In the ordination plot, the length of the vector represents the magnitude of the environmental factors relative to the explanatory trait. The angle between the two arrows indicates the relationship between functional traits and soil properties. An angle between 0° and 90° showed a positive correlation between these two variables, a 90° angle indicated no significant correlation, and an angle between 90° and 180° represented a negative correlation.
3. Results
3.1. Altitudinal distribution of leaf functional traits
Figure 2 shows the changes in leaf functional traits along the altitudinal gradient of Abies georgei var. smithii. SLA decreased with increasing altitude, while the change trend of LDMC was the opposite. The response trends of RWC and RCC were similar, both showed double valley curves, and the minimum values appeared at 3,900 and 4,200 m. The altitudinal distribution patterns of LA and LTD were opposite, with double peak and double valley values at 4,000 and 4,200 m, respectively. LV was the maximum at 4,000 m above sea level, and there was no significant linear trend along the altitude.
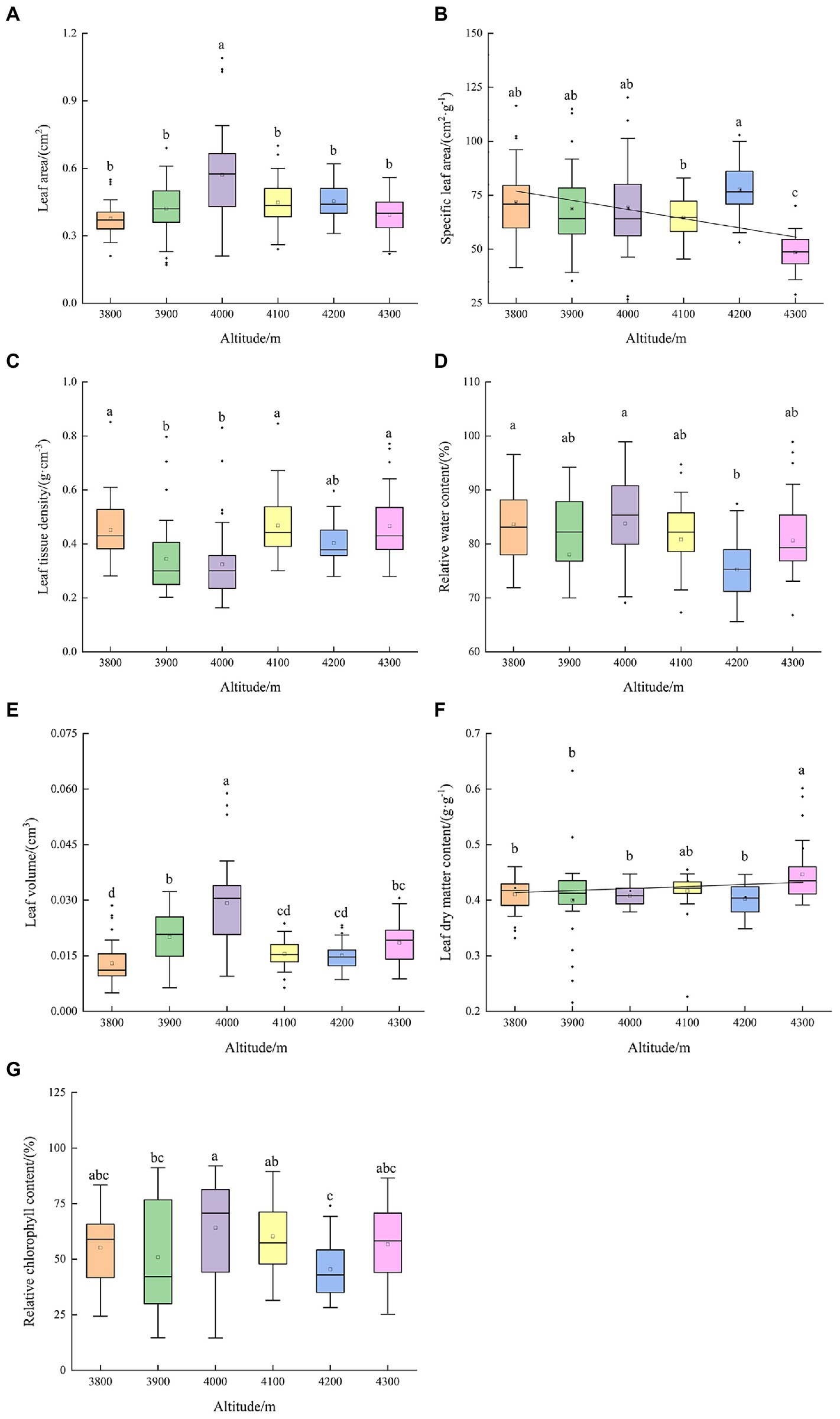
Figure 2. Altitudinal distribution of leaf functional traits of Abies georgei var. smithii. in southeast Tibet. (A) Leaf area (LA); (B) Specific leaf area (SLA); (C) Leaf tissue density (LTD); (D) Relative water content (RWC); (E) Leaf volume (LV); (F) Leaf dry matter content (LDMC); (G) Relative chlorophyll content (RCC). Different lowercase letters in the figure indicate significant differences (p < 0.05).
3.2. Altitudinal distribution of functional traits of current-year twigs
The altitudinal distribution of functional traits of current-year twigs of Abies georgei var. smithii is shown in Figure 3. TLA, TL, NL and TTD all reached maximum values at 4,000 m and then decreased with altitude, and each index at 4,000 m was significantly higher than that at the other five gradients (p < 0.05). The variation trends of HV and DLN on the vertical gradient were similar, decreasing first and then increasing with altitude, and the minimum value was at an altitude of 4,000 m. LI decreased first and then tended to be flat at altitudes of 3,800 ~ 4,300 m. TD rose with altitude.
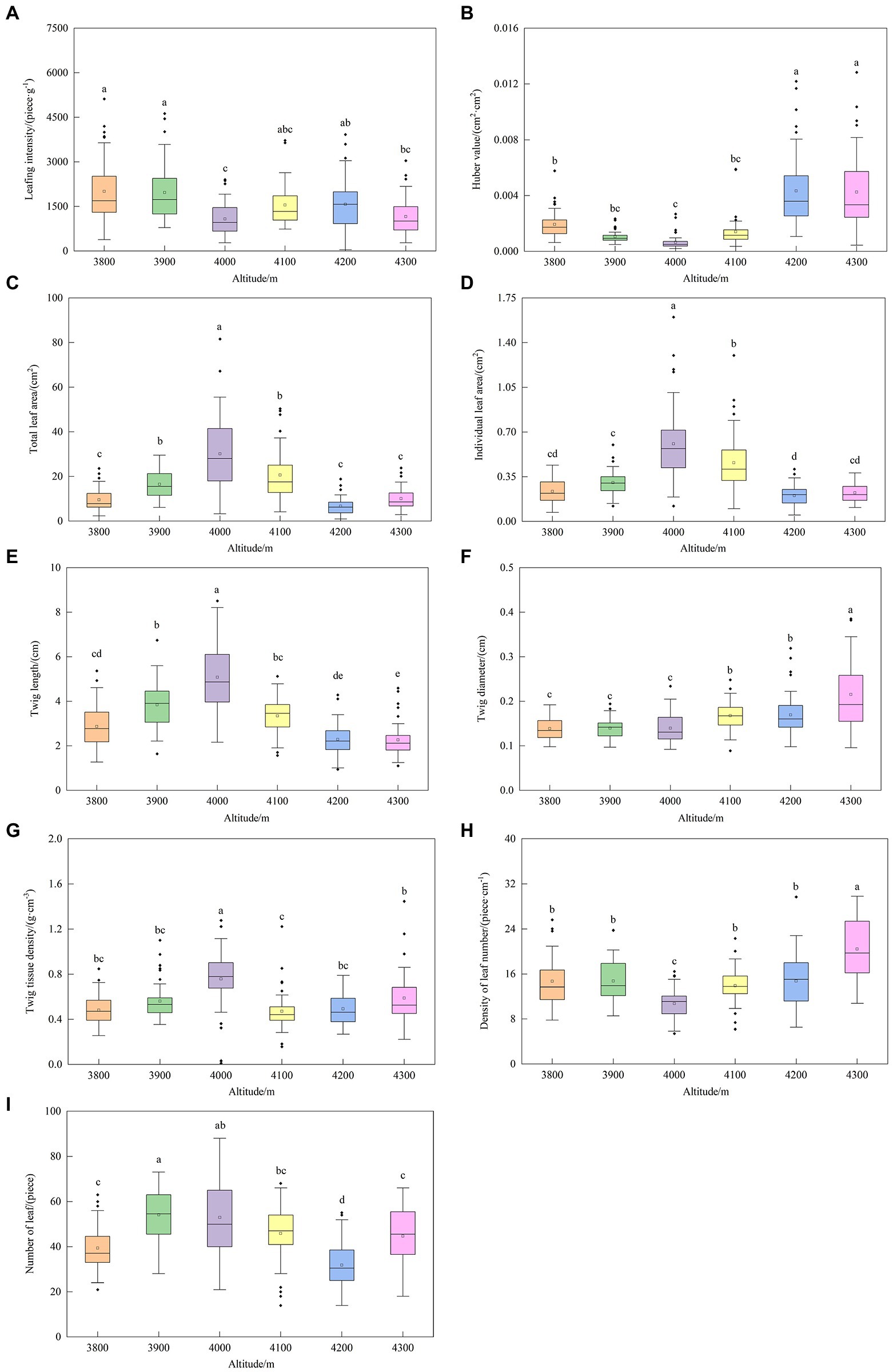
Figure 3. Altitudinal distribution of functional traits of current-year twigs of Abies georgei var. smithii. in southeast Tibet. (A) Leafing intensity (LI); (B) Huber value (HV); (C) Total leaf area (TLA); (D) Individual leaf area (ILA); (E) Twig length (TL); (F) Twig diameter (TD); (G) Twig tissue density (TTD); (H) Density of leaf number (DLN); (I) Leaf number in a twig (NL). Different lowercase letters in the figure indicate significant differences (p < 0.05).
3.3. Altitudinal distribution of wood density
The wood density of Abies georgei var. smithii showed a bimodal curve along the altitudinal gradient (Figure 4) and peaked at 3,900 and 4,200 m above sea level, respectively. The wood density of Abies georgei var. smithii in the 4,000 m plot was significantly lower than that in the other five altitudes (p < 0.05).
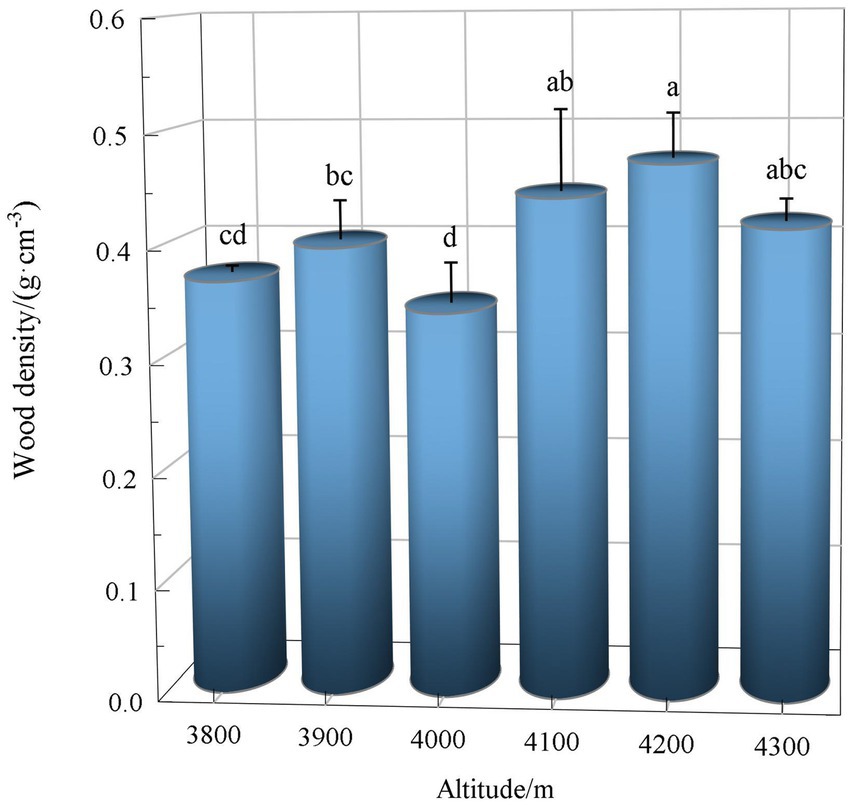
Figure 4. Altitudinal distribution of wood density of Abies georgei var. smithii. in southeast Tibet. Different lowercase letters in the figure indicate significant differences (p < 0.05).
3.4. Altitudinal distribution of functional traits of fine roots
Figure 5 shows the distribution characteristics of fine root functional traits along the altitudinal gradient of Abies georgei var. smithii. RDMC showed an upwards trend along the altitudinal gradient, and the overall distribution was more concentrated. SRL showed a downwards opening parabola (p < 0.01) over the altitude of the study area by polynomial fitting (p < 0.01). It dropped first and then rose gradually with increasing altitude and reached the maximum value at approximately 4,000 ~ 4,100 m. Except for the minimum value at 3,900 m above sea level, RTD showed little difference but strong variation at the other 5 elevations.
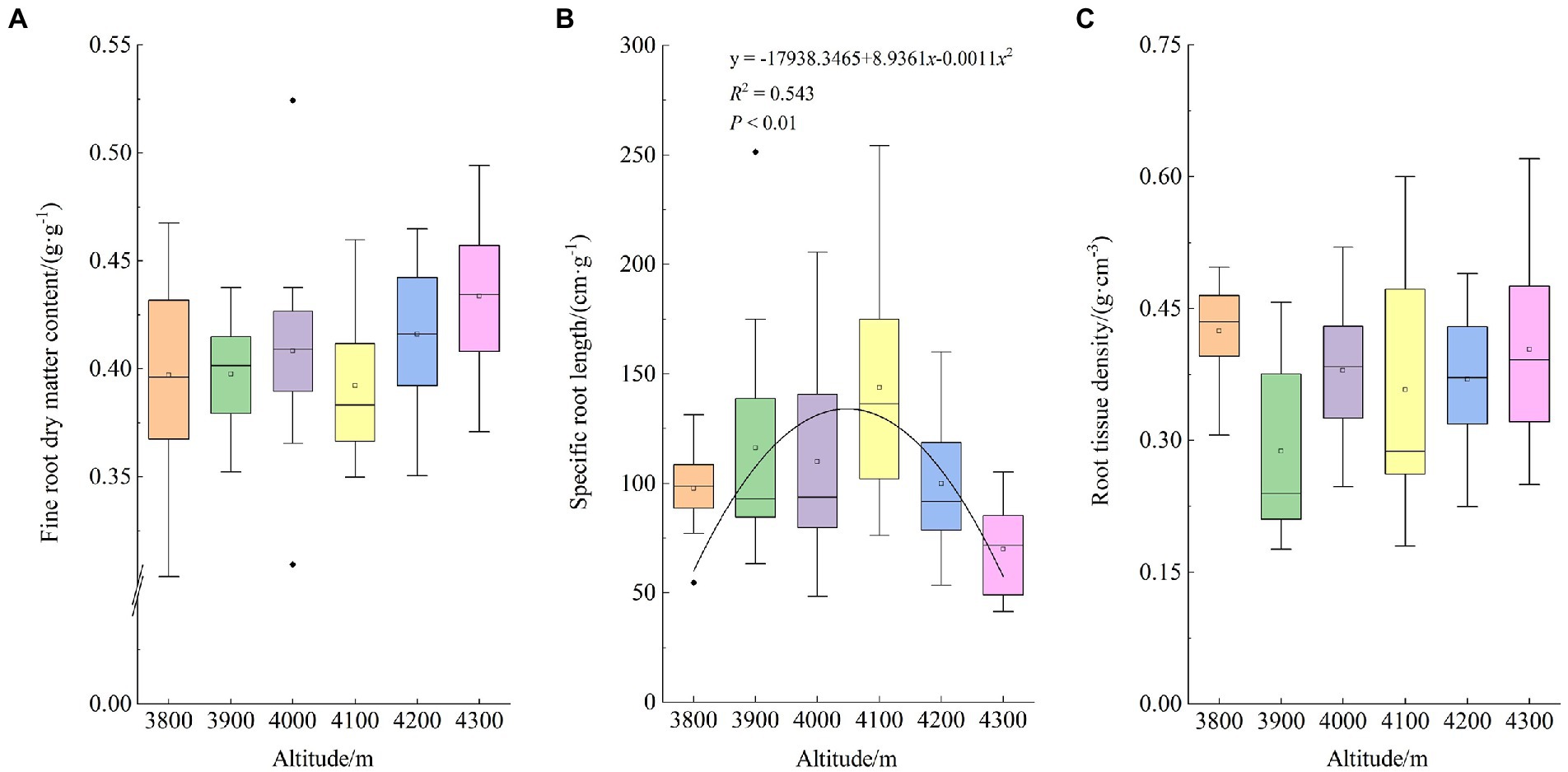
Figure 5. Altitudinal distribution of functional traits of fine roots of Abies georgei var. smithii. in southeast Tibet. (A) Root dry matter content (RDMC); (B) Specific root length (SRL); (C) Root tissue density (RTD).
3.5. Trade-offs between functional traits in different organs
After PCA of all functional traits, RCC, RWC, NL, TTD, WD and RTD, which contributed less to principal component Axis 1, were excluded (Figure 6), and the remaining traits were used for correlation analysis (Figure 7). We observed strong trade-offs between functional traits in different organs of Abies georgei var. smithii. There were significant correlations between the functional traits of current-year twigs and those of leaves and fine roots. TL was positively correlated with LA (r = 0.64, p < 0.01) and LV (r = 0.74, p < 0.01) and negatively correlated with LTD (r = −0.65, p < 0.001). TD was negatively correlated with SLA (r = −0.59, p < 0.05) but positively correlated with LDMC (r = 0.65, p < 0.01). There were significantly positive relationships between TLA and LA (r = 0.72, p < 0.001) and LV (r = 0.77, p < 0.001). DLN (leafing density based on twig length) was significantly positively correlated with LDMC (r = 0.67, p < 0.001) but negatively correlated with LA (r = −0.61, p < 0.001) and SLA (r = −0.64, p < 0.001). LI (leafing density based on twig dry matter) was significantly negatively correlated with LV (r = −0.51, p < 0.0001). RDMC had significantly positive relationships with TD (r = 0.56, p < 0.0001) and HV (r = 0.49, p < 0.0001). SRL was significantly negatively correlated with DLN (r = −0.58, p < 0.0001) and HV (r = −0.59, p < 0.0001) but had significantly positive relationships with TL (r = 0.47, p < 0.0001).
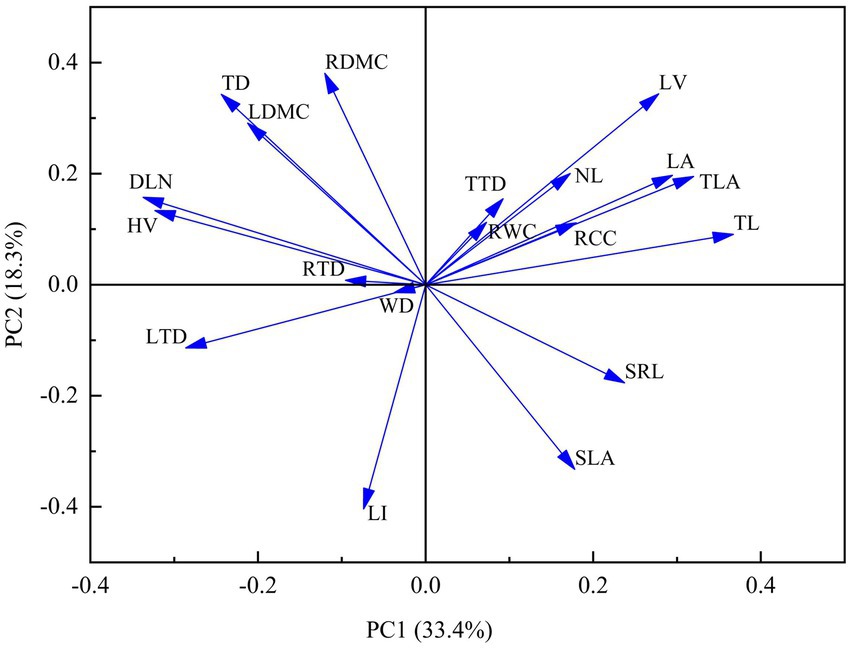
Figure 6. PCA analysis of functional traits of different organs of Abies georgei var. smithii. in southeast Tibet.
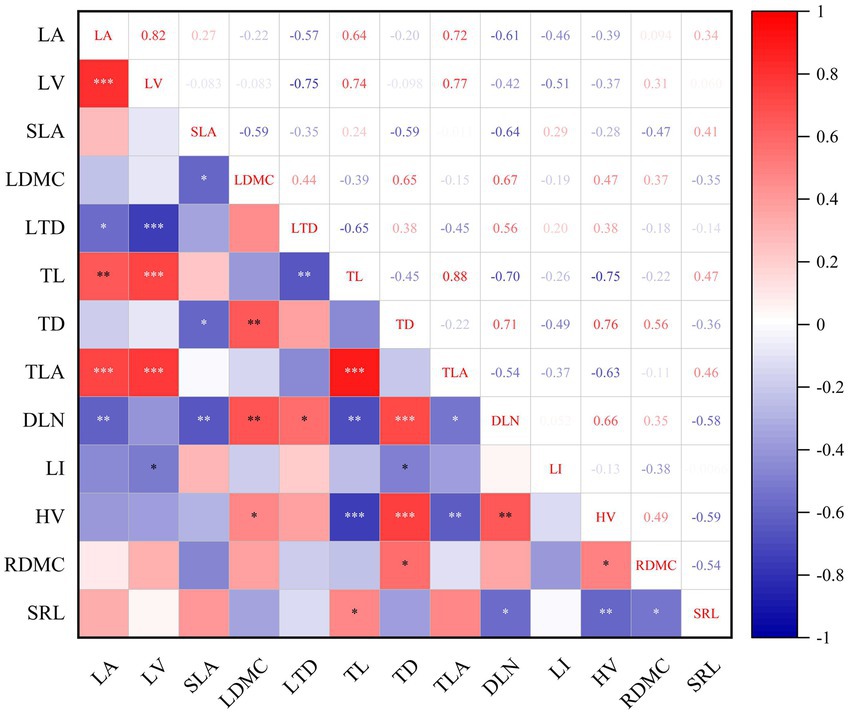
Figure 7. Correlation analysis of functional traits in different organs of Abies georgei var. smithii. in southeast Tibet (Pearson). *p <= 0.05; **p <= 0.01; ***p< = 0.01.
3.6. Relationships between functional traits and environmental factors
The results of redundancy analysis showed that the explanatory rates of environmental factors for leaf, current-year twig, trunk and fine root functional traits were 59.5, 92.7, 81.5, and 48.1%, respectively. Therefore, we can conclude that there was a high correlation between functional traits and environmental factors, which could clearly reflect the degree of influence of dominant factors on the functional traits of leaves, current shoots, trunks and fine roots. The soil factors that highly contributed to leaf functional traits were POC (27.4%), TK (11.2%), TP (10.9%), and MBP (10.5%), among which POC had a significant effect on leaf traits (p = 0.004; Supplementary Table S1). According to the angle of the vectors in Figure 8A, SLA, LA, and LV were positively correlated with SOC, MBC, MBN, and MBP but negatively correlated with Alt, TK, DOC, and SWC. LDMC, RWC, and RCC were positively correlated with Alt, TK, DOC, SWC, and TP but negatively correlated with SOC, POC, MBC, and MBP. TN (22.3%, p = 0.026), SOC (12.8%, p = 0.03), and MBN (34.7%, p = 0.04) contributed most to the functional traits of current-year twigs (Supplementary Table S2). TLA, TL, and NL were positively correlated with MBP and SWC but negatively correlated with MBC, MBN, DOC, POC, TP, and Alt (Figure 8B). WD was positively correlated with Alt (r = 0.559, p < 0.05) and SOC (r = 0.304, p < 0.05) and negatively correlated with soil total phosphorus content (r = −0.066, p < 0.05; Table 4). The variation in WD was significantly correlated with Alt, SOC and TP (p < 0.05). The contribution rates were 38.3, 17.9 and 20.1%, respectively (Supplementary Table S3). SRL was positively correlated with TP and SOC according to the angle between rays in Figure 8C. RDMC and RTD were positively correlated with TN, MBC, MBN and SWC. Additionally, TP and TK were positively correlated with RTD. The first contributors to RDMC, SRL and RTD were POC (43.9%, p = 0.018), POC (29.4%, p = 0.038), and TK (30.2%, p = 0.04), respectively.
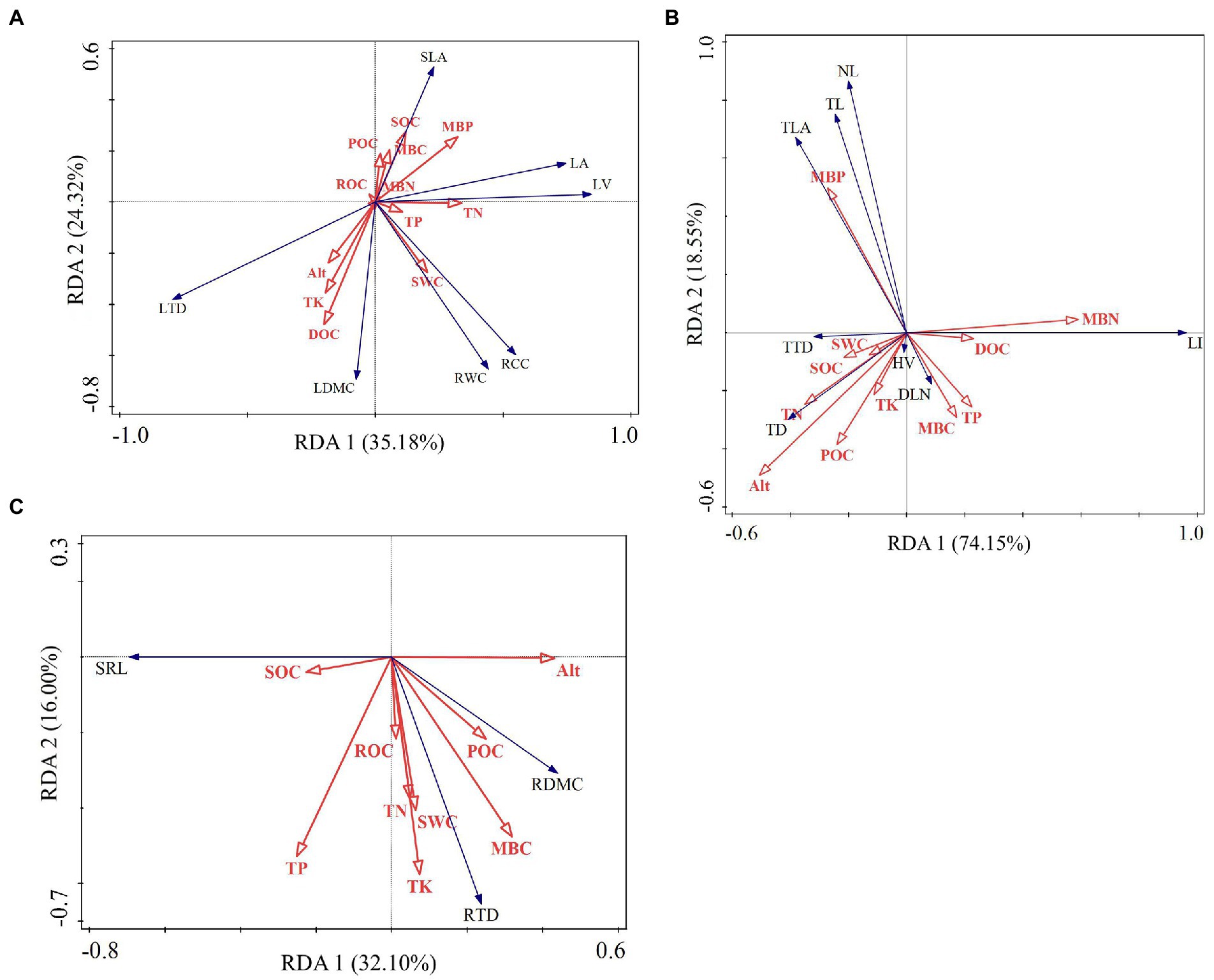
Figure 8. Redundancy analysis of the association of environmental factors with functional traits of leaf (A), current-year twig (B), and fine roots (C).
The Sankey diagram (Figure 9) was developed by using the contribution rate of each environmental factor to each functional trait of Abies georgei var. smithii, directly showing that Alt mainly affected TD and HV; TN mainly affected RWC; and RDMC and TTD were mainly affected by POC. In conclusion, Alt, TN, TP and MBP had significant effects on the functional traits of Abies georgei var. smithii. in southeast Tibet.
4. Discussion
4.1. Altitudinal distribution characteristics of leaf functional traits
SLA reflects the resource utilization efficiency of plants and the nutrient conditions of habitats, and LDMC represents the nutrient accumulation ability of plants and is positively correlated with the cultivation of resilience (Pichon et al., 2022). In the present study, there was a positive correlation between LDMC and altitude (Figure 7), which was compared with Pinus tabuliformis in Songshan Mountain of Beijing and the middle part of the Qinling Mountains (Liu et al., 2021; Tian et al., 2021), the LDMC of Abies georgei var. smithii. was generally at a high level (Figure 2F); compared with the leaf traits of Norway spruce (Picea abies Karst.; Konôpka et al., 2016), the mean value of SLA (66.81 cm2.g−1) of Abies georgei var. smithii. was generally at a low level (Figure 2B). The small SLA indicated that the photosynthetic efficiency, nutrient cycling capacity, and regression rate of Abies georgei var. smithii. in Sejila Mountain were at a low level. It is worth noting that studying leaf functional traits without considering leaf age in this study would bias SLA to be larger. According to the C-S-R theory, the application of soil nutrients by leaves focuses on the “stress tolerance” (C) strategy rather than the “competition for access” (S) and “direction interference” (R) strategies to maintain population development (Yu et al., 2022). The reason why Abies georgei var. smithii. became the top community in Sejila Mountain was the high LDMC, indicating that the nutrients obtained from Abies georgei var. smithii. were more used in the internal tissue building input of the leaves, and a high “defense” was constructed to resist the high altitude restriction. Additionally, the high LDMC also reflected the strong stress resistance of this species, minimizing the nutrient loss to adapt to the barren alpine environment and further validating the judgment of the above resource conservation strategy. Previous studies have shown that leaf RWC is significantly correlated with the physiological and metabolic functions of plants and adaptability to drought (Abdalla and Rafudeen, 2012) and can reflect the water and heat conditions in the habitat. The high RWC and RCC in the present study indicated that Abies georgei var. smithii. has strong drought resistance in Sejila Mountain to a certain extent. LTD reflected the size of plant leaf carrying capacity and was closely related to the concentration of internal secondary metabolites and cell wall density, thus affecting leaf turnover and growth rate (Marron et al., 2003). In the present study, LTD was significantly negatively correlated with LA (p < 0.001; Figure 7), and double valley values and double peak values were obtained at altitudes of 4,000 and 4,200 m at the same time (Figure 2C), indicating that Abies georgei var. smithii. utilized their large LA to maximize light uptake for photosynthesis at these two altitudes. In addition, the configuration of the combination of small LTD and high LDMC indicates that Abies georgei var. smithii. can create as much energy as possible for growth and limit nutrient loss. This ability to covary is the result of a trade-off between biomass production and resource storage in a restricted environment (Volaire, 2008).
4.2. Altitudinal distribution characteristics of current-year twig traits
Studies of the leaf size-number relationship showed that to maximize resource returns, plants either allocate more small leaves per unit twig or select fewer large leaves per unit twig (Westoby et al., 2002; Yan et al., 2013). Longer twigs are beneficial to increase light acquisition and reproductive effectiveness and reduce mutual occlusion between leaves (Feldpausch et al., 2011). The present study showed that ILA on the twig was positively correlated with NL (Figure 7), especially at an altitude of 4,000 m, where both of these traits were the largest (Figures 3D,I), and TL also reached the maximum. It reduced the leafing intensity based on twig length (DLN) and reached a minimum (Figure 3H). This result indicated that Abies georgei var. smithii. had the strongest light capture capacity and the highest photosynthetic rate at an altitude of 4,000 m. This requires a stable transportation ability for water and photosynthetic products in twigs (Niinemets et al., 2006). Therefore, there was no significant difference in TTD among altitudes (Figure 3G). However, TTD reached a maximum at an altitude of 4,000 m to enhance its ability to resist interference to form strong protection organization and biomechanical stability (Huang et al., 2016).
Kleiman and Aarssen (2007) proposed the concept of leaf intensity, which is the number of leaves per unit twig length. Yang et al. (2008) studied the trade-off between leaf area and quantity of woody plants in Gongga Mountain, Southwest China, using two leafing intensities (based on twig mass and length) and found that leaf intensity based on mass could explain more than 99% of the variation in leaf mass. The traditional “dominance hypothesis of leafing intensity” proposes that there is a negative correlation between leafing intensity and leaf area in woody plants, and natural selection favors high leafing intensity (Kleiman and Aarssen, 2007). In the present study, we chose a leafing intensity based on mass, and the results support the argument in this hypothesis. However, the test results of Milla (2009) challenged the argument in this hypothesis, contending that the advantage of leaves with higher leafing intensity was lower quality than expected, which was not conducive to improving leaf traits. Therefore, the lowest value of LI at 4,000 m above sea level in the present study does not mean that leaves at this altitude do not have competitive advantages, but the result is likely to be quite the opposite (Figure 3A). This study may provide evidence to support the proposal that current-year twigs tend to decrease LI during evolution as a response to an increase in LA, and vice versa (Milla, 2009). Huber values (HV) are the xylem cross-sectional area (CA) of the twig divided by the total leaf area (TLA) supported by the twig, indicating the ability to supply water to the leaves via the twig sapwood cross section (Sellin et al., 2012). In this study, the HV at 4,000 m elevation was the lowest (Figure 3B), which reflected the weak water transport capacity of the twigs at this altitude. The HV of twigs also increased (Figure 7) with increasing twig diameter (TD). It is vital for plants under water stress (Wang et al., 2014). The relatively high soil water content (SWC) in the 4,000 m sample plot does not cause adverse environmental pressure (Table 3), which may be one of the reasons why the HV was the minimum value at this altitude.
4.3. Altitudinal distribution characteristics of wood density
Wood density (WD) is an important functional trait of woody plants and has an important impact on ecosystem processes such as biomass carbon storage and wood decomposition (Chave et al., 2009). In general, wood density was negatively correlated with soil fertility, tree mortality and relative growth rate. Climax species with longer tree ages have higher wood densities, while pioneer species have lower wood densities (Muller-Landau, 2004). High-density wood has higher structural strength, as the construction cost and mechanical strength of wood are proportional to the wood density. Nevertheless, it requires more construction cost; in particular, the slower growth rate determines the longer time investment (Fajardo, 2022). However, the farther the wood fibers are from the central axis of the trunk, the greater the mechanical strength of the trunk can be increased (Larjavaara and Muller-Landau, 2010). In other words, a thick low-density wood trunk has higher mechanical strength and lower construction cost than a thin high-density wood trunk. Based upon this reasoning, Abies georgei var. smithii. at 4,000 m elevation in the present study had the largest average DBH (60.35 ± 19.43 cm; Table 1) and the smallest WD (0.34 ± 0.04 g.cm−3; Figure 4), which is the result of the comprehensive trade-off of habitat resources, growth rate, structural strength, and time cost in the alpine environment. The average WD (0.40 ± 0.05 g.cm−3) of Abies georgei var. smithii. was higher than that of Cryptomeria japonica (0.35 g.cm−3) and was similar to that of Chamaecyparis obtusa (0.40 g.cm−3) (Kijidani et al., 2021), indicating that Abies georgei var. smithii. in the study area had higher wood density, lower growth rate, longer tree life and lower mortality. Additionally, as the supporting effect of tree trunks is closely related to their mechanical safety, the WD at high altitudes (4,100 ~ 4,300 m) is higher than that at low altitudes (3,800 ~ 4,000 m; Figure 4). In the harsh environment of high altitude, plant stems must maintain a high tissue density to resist the instability of climate and strong external interference. The relapse of wood density at the highest elevations may be strongly related to habitat constraints near the forest line (Hagedorn et al., 2014).
4.4. Altitudinal distribution characteristics of functional traits of fine roots
In the present study, with increasing altitude, the SRL of Abies georgei var. smithii. increased first and then decreased gradually and reached the highest value at approximately 4,000 ~ 4,100 m above sea level (Figure 5B), indicating that the roots of Abies georgei var. smithii. at these two altitudes could obtain resources to promote plant growth with maximum efficiency (Cardou et al., 2022). According to resource economics theory, poor soils generally choose species with low SRL to retain the scarce resources obtained (i.e., conservative strategy), while resource-rich soils choose species with higher SRL to acquire soil resources efficiently and rapidly (i.e., acquisition strategy; Reich, 2014). The results of the present study were in line with this theory. Compared with other gradients, soil nutrient elements, such as MBC, MBN, MBP, and TOC, were at relatively high levels at the two altitudes (Table 3). This result was consistent with the results of studies on fine root traits of Pinus Taiwanensis (Zhou et al., 2019).
The high RTD of Abies georgei var. smithii. indicated that the root extension and defense ability were relatively strong (Craine et al., 2001). Some studies have shown that root tissue density is less affected by tree competition (Hajek et al., 2014). According to most studies, however, isolated precipitation treatment could reduce the root tissue density of plant fine roots (Sandrin et al., 1995). The SWC and RTD values were both the lowest at 3,900 m in the study area (Table 3 and Figure 5). Therefore, it was concluded that SWC was the main influencing factor for the RTD of Abies georgei var. smithii. at 3,900 m, rather than intraspecific competition caused by stand density and canopy density.
Fine root length is closely related to resource acquisition strategies, and RDMC combined with RTD reflects resource conservation strategies such as plant physical defense and anti-interference ability (Hu et al., 2021). In the present study, the coefficient of variation of RDMC was small, and the overall value was large on the 6 elevation gradients (Figure 5A), which was the requirement to maintain high transport capacity and stability (Poorter and Ryser, 2015). Currently, most studies have combined RDMC and RTD to comprehensively reflect the fine root respiration rate and soil resource availability. It can be coordinated with aboveground functional traits and used in other whole-plant economic spectrum tests (Laughlin et al., 2017).
4.5. Relationships between functional traits of different organs
After PCA was performed (Figure 6), 6 traits, TTD, RCC and RTD, were screened out, including WD, which was the only stem trait. The correlation between WD and other functional traits was weak in the present study, which was consistent with the results of Kotowska et al. (2015). They proved that WD should be treated separately in the study of plant adaptation strategies. In contrast, the study of Pietsch et al. (2014) demonstrated a potentially independent trade-off between WD and leaf traits. Correlation analysis of functional traits among different organs (Figure 7) showed that TD was positively correlated with LDMC (p < 0.01) and RDMC (p < 0.05). Additionally, LDMC showed a linear upwards trend along the altitude gradient (Figure 2F). Abies georgei var. smithii. growing at high altitudes have larger LDMC, TD and RTD. This means that it adopted a conservative strategy of prolonging the life of expensive organs (needles, fine roots) in the restrictive environment of high radiation and low temperature (Carvalho et al., 2020). Additionally, the results showed that DLN was significantly negatively correlated with SLA and significantly negatively correlated with SRL; TL was positively correlated with LA, LV, and SRL (Figure 7). For example, DLN at 4,000 m altitude was the minimum, and SLA and SRL were both larger. TL reached the maximum at 4,000 m, and LA and LV also reached the maximum. In other words, the leaf productivity and nutrient absorption capacity of fine roots were stronger at this altitude, indicating that Abies georgei var. smithii. made use of an ecological strategy of active acquisition and resource conservation at 4,000 m (Poorter and Ryser, 2015).
4.6. Environmental interpretation of plant functional traits
Carbon is a fundamental element in building biological tissues, and approximately 50% of the dry matter of plant organisms is carbon, making it different from other nutrient elements. The highest contribution of POC (27.4%) (Supplementary Table S1) in this study was positively correlated with SLA, LA and LV and negatively correlated with RCC, RWC and LTD (Figure 8A). In addition, the carbon pool-related indicators explained 35.4% of the variation in leaf functional traits (Supplementary Table S1), suggesting that soil carbon is strongly associated with and has a wide range of effects on each leaf functional trait in Abies georgei var. smithii and that the study by Zhao et al. (2016) also showed that carbon content did not vary significantly in plant roots, stems and leaves but was significantly associated with different components. TK was positively correlated with LTD and LDMC and contributed more to the variation in leaf traits, as an increase in TK resulted in greater biomass in the plant leaves (Shen et al., 2022), resulting in an increase in LDMC and a corresponding increase in LTD. The total contribution of TP and MBP to leaf trait variation was 21.4% (Supplementary Table S1), which was positively correlated with LV and negatively correlated with LTD (Figure 8A). LTD is the ratio of leaf dry mass to LV. In this regard, the variation in soil phosphorus content mainly determined the change in LV and then exerted an impact on LTD. SOC, TN and TP were positively correlated with LA and LV (Figure 8A), indicating that the soil elements effectively promoted the intercalary growth of leaves, resulting in an expansion of leaf area and leaf volume, whereas TK was the opposite in this study.
The results of the RDA showed that the contribution rate of MBN to the total variation in current-year twig traits was the highest (34.7%), followed by TN and SOC (22.3 and 12.8%, respectively; Supplementary Table S2). The mean value of MBN in the present study area (38.96 mg·kg−1) was much lower than that in the Wuyi Mountain evergreen broad-leaved forest area (90.42 mg·kg−1), while the TN was higher (3.10 g·kg−1; 2.12 g·kg−1; Su et al., 2012). The high contribution of MBN indicates that the functional allocation of current-year twigs is closely related to soil fertility and activity. In addition, the overall low MBN and the significant negative correlation with many twig morphological traits (TL, TD, etc.) (Figure 8B) indicated that the nitrogen supply capacity of the soil greatly limited the growth of the current-year twigs of Abies georgei var. smithii. Plants tend to grow robustly when nitrogen supply is sufficient. Leaves will be large and fresh green, with a prolonged leaf function period (Báez and Homeier, 2018). In this case, in the present study, the highest values of TL, NL and ILA at 4,000 m elevation were significantly related to the higher TN and MBN at this elevation (Figure 3 and Table 3). Soil organic carbon improves soil properties, promotes good soil structure and is a major source of nutrients and energy for plants and microorganisms. The RDA ranking map showed that SOC was significantly positively correlated with functional traits indicating defense construction, such as TTD and TD (Figure 8B). From the perspective of plant physiology, Savage et al. (2016) found that organic carbon is a powerful weapon against pests and diseases in plants. It was proven in this study that SOC plays a positive role in promoting the construction of plant defense tissues through functional traits.
Nyakuengama et al. (2003) showed a negative correlation between wood density and soil fertility in a fertilization experiment on Pinus radiata, while more studies on a range of coniferous species have shown that wood density is generally lower in widely spaced stands (Jordan et al., 2008; Watt et al., 2011). In this study, wood density was positively correlated with soil total nitrogen and organic carbon content and negatively but not significantly correlated with soil total phosphorus and potassium, suggesting that soil fertility has some but not significant influence on changes in wood density. We found that WD was positively correlated with TN and SOC and negatively correlated with TP and TK, but not significantly (Table 4), indicating that soil fertility had a certain but not obvious effect on WD. The minimum value of WD at 4,000 m elevation was combined with the minimum value of SD and CD at the same altitude (Figure 4 and Table 1). Additionally, there was a positive correlation between WD and SD, but the correlation between them was weak (p > 0.05; Table 4). Therefore, although SD could explain why WD was the lowest at 4,000 m, SD was not the main influencing factor of WD on the overall altitude gradients. Altitude, the most important topographic factor in southeastern Tibet, is the primary contributor to WD variation (Supplementary Table S3). Cell wall thickness is a key factor affecting wood quality and strength characteristics and is highly correlated with WD. Many researchers have described a positive correlation between WD and cell wall thickness (TopaloĞlu et al., 2016; Bahmani et al., 2020). In the present study, the average value of WD in the high altitude range (4,100–4,300 m) is higher because the thickness of the fiber cell wall, cellulose, lignin and other contents change with increasing altitude (Nazari et al., 2020). In addition, SOC and TP were also the main influencing factors that significantly affected WD (Table 3). The absolute deficiency of TP in this study area and its significant negative correlation with WD, with its relatively high contribution (20.1%), suggest that phosphorus may have contributed to a greater extent to the metabolism of carbohydrates within the trunk of Abies georgei var. smithii, thus limiting the wood structure to become more compact (Supplementary Table S3; Crous et al., 2009).
POC demonstrated the highest impact on RDMC (43.9%, p = 0.018) and SRL (29.4%, p = 0.038); RTD was the most affected by TK (30.2%, p < 0.05; Supplementary Table S4). POC is an organic carbon component bound to sand with a particle size of 53–2,000 μm. It was derived from the organic carbon pool of plant residues that had not been completely decomposed. POC features a low humification degree, high activity, easy decomposition and fast turnover (5–20 a). Additionally, POC is more sensitive to altitude (Parwada and Van Tol, 2018) and is the main influencing factor for changes in RDMC and SRL in this paper. The trends in TK and RTD in this study are consistent, with both levels being at their lowest at 3,900 m and remaining at almost the same level at other altitudes. Therefore, TK in this study area promotes the increase in root biomass and is the dominant factor in the variation in RTD in Abies georgei var. smithii. In addition, the results showed that SOC was generally higher and changed slowly along the elevation gradient. This organic matter-rich soil stimulates outwards and downwards root elongation. The contribution of TOC to changes in fine root indicators was large but not significant to the variation in fine root traits (p > 0.05; Table 3). In this regard, it was difficult to interpret the effects of SOC on fine root traits at different altitudes. Su et al. (2019) also showed that SOC is relatively stable at different stages of vegetation recovery.
5. Conclusion
The effects of altitude on the functional traits of the organs of Abies georgei var. smithii. were obvious. The soil phosphorus content mainly determined the change in LV and then affected the LTD. Phosphorus reduced WD to a greater extent. TK was the dominant factor of RTD change; SOC played a positive role in promoting the construction of plant defense tissues based on functional traits.
Multiple functional traits of different above-met and below-ground organs of Abies georgei var. smithii. exhibited the optimal trait combination at 4,000 m. (i) RCC, LA and LV all reached the maximum value at 4,000 m, indicating that the leaves obtained the maximum energy output. In addition, LTD was the smallest, while RWC was the largest. Therefore, the leaves had the fastest turnover speed and the strongest stress resistance. (ii) The TL was the highest at 4,000 m, while the HV was the lowest. The traits indicating photosynthetic capacity (TLA, NL) and physical defense capacity and transmission stability (TTD) were the largest. Therefore, current-year twigs were able to obtain light and energy products to the greatest extent. Although their water and nutrient transport capacity was weak, they remained relatively stable at an altitude of 4,000 m. (iii) Abies georgei var. smithii. forest at 4,000 m had the largest DBH and the smallest WD, indicating that the mechanical strength and construction cost of Abies georgei var. smithii. Trunk were optimal. (iv) SRL reached its maximum value in the altitude range of 4,000–4,100 m. This reflected the relatively strong hydrotrophic acquisition ability of plant fine roots in this altitude range. In conclusion, Abies georgei var. smithii. has been proven to be able to optimize habitat resources, growth rate, structural strength, and time cost through a comprehensive trade-off of functional traits at 4,000 m altitude. It was the optimal survival area. Finally, the strategy of active acquisition and resource conservation was adopted by Abies georgei var. smithii. in high-altitude habitats in southeast Tibet.
Data availability statement
The original contributions presented in the study are included in the article/Supplementary material, further inquiries can be directed to the corresponding authors.
Author contributions
CW: software, sampling, and writing – original draft. FD: sampling and plotting. CZ and JL: methodology and conceptualization. All authors contributed to the article and approved the submitted version.
Funding
This work was supported by the National Natural Science Foundation of China (31960256); the Central Government Guides Local Science and Technology Development Projects, China (XZ202101YD0016C); and the Independent Research Project of Science and Technology Innovation Base in Tibet Autonomous Region (XZ2022JR0007G).
Conflict of interest
The authors declare that the research was conducted in the absence of any commercial or financial relationships that could be construed as a potential conflict of interest.
Publisher’s note
All claims expressed in this article are solely those of the authors and do not necessarily represent those of their affiliated organizations, or those of the publisher, the editors and the reviewers. Any product that may be evaluated in this article, or claim that may be made by its manufacturer, is not guaranteed or endorsed by the publisher.
Supplementary material
The Supplementary material for this article can be found online at: https://www.frontiersin.org/articles/10.3389/fevo.2023.1055195/full#supplementary-material
References
Abdalla, K. O., and Rafudeen, M. S. (2012). Analysis of the nuclear proteome of the resurrection plant Xerophyta viscosa in response to dehydration stress using iTRAQ with 2DLC and tandem mass spectrometry. J. Proteome 75, 2361–2374. doi: 10.1016/j.jprot.2012.02.006
Alvarez-Uria, P., and Koerner, C. (2011). Fine root traits in adult trees of evergreen and deciduous taxa from low and high elevation in the Alps. Alp. Bot. 121, 107–112. doi: 10.1007/s00035-011-0092-6
Báez, S., and Homeier, J. (2018). Functional traits determine tree growth and ecosystem productivity of a tropical montane forest: insights from a long-term nutrient manipulation experiment. Glob. Chang. Biol. 24, 399–409. doi: 10.1111/gcb.13905
Bahmani, M., Fathi, L., Koch, G., Kool, F., Aghajani, H., and Humar, M. (2020). Heartwood and sapwood features of Sorbus Torminalis grown in Iranian forests. Wood Res. Slovak. 65, 195–204. doi: 10.37763/wr.1336-4561/65.2.195204
Cardou, F., Munson, A. D., Boisvert-Marsh, L., Anand, M., Arsenault, A., Bell, F. W., et al. (2022). Above - and belowground drivers of intraspecific trait variability across subcontinental gradients for five ubiquitous forest plants in North America. J. Ecol. 110, 1590–1605. doi: 10.1111/1365-2745.13894
Carvalho, B., Bastias, C. C., Escudero, A., Valladares, F., and Benavides, R. (2020). Intraspecific perspective of phenotypic coordination of functional traits in scots pine. PLoS One 15:e0228539. doi: 10.1371/journal.pone.0228539
Chang, R. Y., Fu, B. J., Liu, G. H., Yao, X. L., and Wang, S. (2012). Effects of soil physicochemical properties and stand age on fine root biomass and vertical distribution of plantation forests in the Loess Plateau of China. Ecol. Res. 27, 827–836. doi: 10.1007/s11284-012-0958-0
Chave, J., Coomes, D., Jansen, S., Lewis, S. L., Swenson, N. G., and Zanne, A. E. (2009). Towards a worldwide wood economics spectrum. Ecol. Lett. 12, 351–366. doi: 10.1111/j.1461-0248.2009.01285.x
Chen, X. H., Yang, Q. Q., Chen, Z. Z., Yu, X. B., Yang, Q., and Lei, J. R. (2017). Distribution of soil easily oxidized organic carbon and its response to soil factors in the tropical coastal forest of Hainan Island. China. J. Cent. South Univ. For. Technol. 37, 140–145. doi: 10.14067/j.cnki.1673-923x.2017.11.023
Cornelissen, J. H. C., Lavorel, S., Garnier, E., Díaz, S., Buchmann, N., Gurvich, D. E., et al. (2003). A handbook of protocols for standardised and easy measurement of plant functional traits worldwide. Aust. J. Bot. 51, 335–380. doi: 10.1071/BT02124
Craine, J. M., Froehle, J., Tilman, D. G., Wedin, D. A., and Chapin, F. S. III (2001). The relationships among root and leaf traits of 76 grassland species and relative abundance along fertility and disturbance gradients. Oikos 93, 274–285. doi: 10.1034/j.1600-0706.2001.930210.x
Crous, J. W., Morris, A. R., and Scholes, M. C. (2009). Effect of phosphorus and potassium fertiliser on stem form, basic wood density and stem nutrient content of Pinus patula at various stem heights. Aust. For. 72, 99–111. doi: 10.1080/00049158.2009.10676295
Eller, C. B., Meireles, L. D., Sitch, S., Burgess, S. S., and Oliveira, R. S. (2020). How climate shapes the functioning of tropical montane cloud forests. Curr. For. Reports 6, 97–114. doi: 10.1007/s40725-020-00115-6
Fajardo, A. (2022). Wood density relates negatively to maximum plant height across major angiosperm and gymnosperm orders. Am. J. Bot. 109, 250–258. doi: 10.1002/ajb2.1805
Fang, H. J., Cheng, S. L., Yu, G. R., Xu, M. J., Wang, Y. S., Li, L. S., et al. (2014). Experimental nitrogen deposition alters the quantity and quality of soil dissolved organic carbon in an alpine meadow on the Qinghai-Tibetan Plateau. Appl. Soil Ecol. 81, 1–11. doi: 10.1016/j.apsoil.2014.04.007
Feldpausch, T. R., Banin, L., Phillips, O. L., Baker, T. R., Lewis, S. L., Quesada, C. A., et al. (2011). Height-diameter allometry of tropical forest trees. Biogeosciences 8, 1081–1106. doi: 10.5194/bg-8-1081-2011
Garten, C. F., Post, W. M., Hanson, P. J., and Cooper, L. W. (1999). Forest soil carbon inventories and dynamics along an elevation gradient in the southern Appalachian Mountains. Biogeochemistry 45, 115–145. doi: 10.1007/BF01106778
Guo, Q. Q., and Zhang, W. H. (2015). Sap flow of Abies georgei var. smithii and its relationship with the environment factors in the Tibetan subalpine region, China. J. Mt. Sci. 12, 1373–1382. doi: 10.1007/s11629-015-3618-3
Hagedorn, F., Shiyatov, S. G., Mazepa, V. S., Devi, N. M., Grigor'ev, A. A., Bartysh, A. A., et al. (2014). Treeline advances along the Urals mountain range–driven by improved winter conditions? Glob. Chang. Biol. 20, 3530–3543. doi: 10.1111/gcb.12613
Hajek, P., Hertel, D., and Leuschner, C. (2014). Root order-and root age-dependent response of two poplar species to belowground competition. Plant Soil 377, 337–355. doi: 10.1007/s11104-013-2007-3
Han, X. G., Hu, Z. J., Xin, G. R., Shi, X. G., Huang, D., and Zhang, G. R. (2014). Studies on the characteristics of vegetation and soil on Mount Sejila, Tibet. Pak. J. Bot. 46, 457–464.
Hu, Y. K., Pan, X., Liu, X. Y., Fu, Z. X., and Zhang, M. Y. (2021). Above-and belowground plant functional composition show similar changes during temperate forest swamp succession. Front. Plant Sci. 12:1166. doi: 10.3389/fpls.2021.658883
Huang, Y. X., Lechowicz, M. J., Price, C. A., Li, L., Wang, Y., and Zhou, D. W. (2016). The underlying basis for the trade-off between leaf size and leafing intensity. Funct. Ecol. 30, 199–205. doi: 10.1111/1365-2435.12491
Jordan, L., Clark, A., Schimleck, L. R., Hall, D. B., and Daniels, R. F. (2008). Regional variation in wood specific gravity of planted loblolly pine in the United States. Can. J. For. Res. 38, 698–710. doi: 10.1139/X07-158
Kijidani, Y., Tsuyama, T., and Takata, K. (2021). Seasonal variations of auxin and gibberellin A4 levels in cambial-region tissues of three conifers (Pinus Elliottii, Chamaecyparis Obtusa, and Cryptomeria Japonica) with inherently different wood densities. J. Wood Sci. 67, 1–10. doi: 10.1186/s10086-021-01977-5
Kleiman, D., and Aarssen, L. W. (2007). The leaf size/number trade-off in trees. J. Ecol. 95, 376–382. doi: 10.1111/j.1365-2745.2006.01205.x
Konôpka, B., Pajtík, J., Marušák, R., Bošeľa, M., and Lukac, M. (2016). Specific leaf area and leaf area index in developing stands of Fagus sylvatica L. and Picea abies Karst. For. Ecol. Manag. 364, 52–59. doi: 10.1016/j.foreco.2015.12.005
Kotowska, M. M., Hertel, D., Rajab, Y. A., Barus, H., and Schuldt, B. (2015). Patterns in hydraulic architecture from roots to branches in six tropical tree species from cacao agroforestry and their relation to wood density and stem growth. Front. Plant Sci. 6:191. doi: 10.3389/fpls.2015.00191
Kraft, N. J., Godoy, O., and Levine, J. M. (2015). Plant functional traits and the multidimensional nature of species coexistence. Proc. Natl. Acad. Sci. 112, 797–802. doi: 10.1073/pnas.1413650112
Larjavaara, M., and Muller-Landau, H. C. (2010). Rethinking the value of high wood density. Funct. Ecol. 24, 701–705. doi: 10.1111/j.1365-2435.2010.01698.x
Laughlin, D. C., Lusk, C. H., Bellingham, P. J., Burslem, D. F., Simpson, A. H., and Kramer-Walter, K. R. (2017). Intraspecific trait variation can weaken interspecific trait correlations when assessing the whole-plant economic spectrum. Ecol. Evol. 7, 8936–8949. doi: 10.1002/ece3.3447
Liu, S. W., Ai, Y. B., and Liu, Y. H. (2021). Variations in leaf functional traits along the altitude gradient of Pinus tabuliformis and its environmental explanations in Beijing Songshan Mountain. J. Beijing For. Univ. 43, 47–55. doi: 10.12171/j.1000-1522.20200292
Liu, L. B., Hu, J., Chen, X. Y., Xu, X., Yang, Y., and Ni, J. (2022). Adaptation strategy of karst forests: evidence from the community-weighted mean of plant functional traits. Ecol. Evol. 12:e8680. doi: 10.1002/ece3.8680
Luo, L., Dan, Z., Zhu, L. P., and Zhang, H. B. (2021). Vertical gradient changes of temperature and precipitation in the Sygera Mountains, southeastern Qinghai-Xizang Plateau. Plateau Meteorol. 40, 37–46. doi: 10.7522/j.issn.1000-0534.2019.00123
Manral, V., Bargali, K., Bargali, S. S., Jhariya, M. K., and Padalia, K. (2022). Relationships between soil and microbial biomass properties and annual flux of nutrients in Central Himalayan forests, India. Land Degrad. Dev. 33, 2014–2025. doi: 10.1002/ldr.4283
Marron, N., Dreyer, E., Boudouresque, E., Delay, D., Petit, J. M., Delmotte, F. M., et al. (2003). Impact of successive drought and re-watering cycles on growth and specific leaf area of two Populus× Canadensis (moench) clones, ‘dorskamp’and ‘luisa_avanzo’. Tree Physiol. 23, 1225–1235. doi: 10.1093/treephys/23.18.1225
Meng, W. Y., Wang, P., Yang, R. Q., Sun, H. Z., Matsiko, J., Wang, D., et al. (2018). Altitudinal dependence of PCBs and PBDEs in soil along the two sides of Mt. Sygera, southeastern Tibetan Plateau. Sci. Rep. 8:14037. doi: 10.1038/s41598-018-32093-y
Milla, R. (2009). The leafing intensity premium hypothesis tested across clades, growth forms and altitudes. J. Ecol. 97, 972–983. doi: 10.1111/j.1365-2745.2009.01524.x
Moretti, M., Dias, A. T., De Bello, F., Altermatt, F., Chown, S. L., Azcárate, F. M., et al. (2017). Handbook of protocols for standardized measurement of terrestrial invertebrate functional traits. Funct. Ecol. 31, 558–567. doi: 10.1111/1365-2435.12776
Muller-Landau, H. C. (2004). Interspecific and inter-site variation in wood specific gravity of tropical trees. Biotropica 36, 20–32. doi: 10.1111/j.1744-7429.2004.tb00292.x
Nazari, N., Bahmani, M., Kahyani, S., Humar, M., and Koch, G. (2020). Geographic variations of the wood density and fiber dimensions of the Persian oak wood. Forests 11:1003. doi: 10.3390/f11091003
Niinemets, Ü., Portsmuth, A., and Tobias, M. (2006). Leaf size modifies support biomass distribution among stems, petioles and mid-ribs in temperate plants. New Phytol. 171, 91–104. doi: 10.1111/j.1469-8137.2006.01741.x
Ning, Z. Y., Li, Y. L., Zhao, X. Y., Han, D., and Zhan, J. (2021). Comparison of leaf and fine root traits between annuals and perennials, implicating the mechanism of species changes in desertified grasslands. Front. Plant Sci. 12:3449. doi: 10.3389/fpls.2021.778547
Nyakuengama, J. G., Downes, G. M., and Ng, J. (2003). Changes caused by mid-rotation fertilizer application to the fibre anatomy of Pinus radiata. IAWA J. 24, 397–409. doi: 10.1163/22941932-90000344
Parwada, C., and Van Tol, J. (2018). Effects of litter source on the dynamics of particulate organic matter fractions and rates of macroaggregate turnover in different soil horizons. Eur. J. Soil Sci. 69, 1126–1136. doi: 10.1111/ejss.12726
Perez-Harguindeguy, N., Diaz, S., Garnier, E., Lavorel, S., Poorter, H., Jaureguiberry, P., et al. (2013). New handbook for standardised measurement of plant functional traits worldwide. Aust. J. Bot. 61, 167–234. doi: 10.1071/BT12225
Pichon, N. A., Cappelli, S. L., and Allan, E. (2022). Intraspecific trait changes have large impacts on community functional composition but do not affect ecosystem function. J. Ecol. 110, 644–658. doi: 10.1111/1365-2745.13827
Pietsch, K. A., Ogle, K., Cornelissen, J. H., Cornwell, W. K., Bönisch, G., Craine, J. M., et al. (2014). Global relationship of wood and leaf litter decomposability: the role of functional traits within and across plant organs. Glob. Ecol. Biogeogr. 23, 1046–1057. doi: 10.1111/geb.12172
Poorter, H., and Ryser, P. (2015). The limits to leaf and root plasticity: what is so special about specific root length? New Phytol. 206, 1188–1190. doi: 10.1111/nph.13438
Rajsnerová, P., Klem, K., Holub, P., Novotná, K., Večeřová, K., Kozáčiková, M., et al. (2015). Morphological, biochemical and physiological traits of upper and lower canopy leaves of European beech tend to converge with increasing altitude. Tree Physiol. 35, 47–60. doi: 10.1093/treephys/tpu104
Ramananantoandro, T., Ramanakoto, M. F., Rajoelison, G. L., Randriamboavonjy, J. C., and Rafidimanantsoa, H. P. (2016). Influence of tree species, tree diameter and soil types on wood density and its radial variation in a mid-altitude rainforest in Madagascar. Ann. For. Sci. 73, 1113–1124. doi: 10.1007/s13595-016-0576-z
Reich, P. B. (2014). The world-wide ‘fast–slow’ plant economics spectrum: a traits manifesto. J. Ecol. 102, 275–301. doi: 10.1111/1365-2745.12211
Sandrin, M. S., Fodor, W. L., Mouthtouris, E., Osman, N., Cohney, S., Rollins, S. A., et al. (1995). Enzymatic remodelling of the carbohydrate surface of a xenogenic cell substantially reduces human antibody binding and complement-mediated cytolysis. Nat. Med. 1, 1261–1267. doi: 10.1038/nm1295-1261
Savage, J. A., Clearwater, M. J., Haines, D. F., Klein, T., Mencuccini, M., Sevanto, S., et al. (2016). Allocation, stress tolerance and carbon transport in plants: how does phloem physiology affect plant ecology? Plant Cell Environ. 39, 709–725. doi: 10.1111/pce.12602
Sellin, A., Õunapuu, E., Kaurilind, E., and Alber, M. (2012). Size-dependent variability of leaf and shoot hydraulic conductance in silver birch. Trees 26, 821–831. doi: 10.1007/s00468-011-0656-5
Shen, Z. Q., Lu, J., Hua, M., and Fang, J. P. (2016). Spatial pattern analysis and associations of different growth stages of populations of Abies georgei var. smithii in southeast Tibet, China. J. Mt. Sci. 13, 2170–2181. doi: 10.1007/s11629-016-3849-y
Shen, Y., Umaña, M. N., Li, W. B., Fang, M., Chen, Y. X., Lu, H. P., et al. (2022). Linking soil nutrients and traits to seedling growth: a test of the plant economics spectrum. For. Ecol. Manag. 505:119941. doi: 10.1016/j.foreco.2021.119941
Shen, Y. X., Yu, Y., Lucas-Borja, M. E., Chen, F. J., Chen, Q. Q., and Tang, Y. Y. (2020). Change of soil K, N and P following forest restoration in rock outcrop rich karst area. Catena 186:104395. doi: 10.1016/j.catena.2019.104395
Su, D. S., Chen, H. Z., Xu, H., Lu, X., Chen, L. H., and Lin, R. K. (2012). Microbial biomass nitrogen in soil of Mt. Wuyi Evergreen broad-leave forest. Fujian J. Agric. Sci. 27, 539–543. doi: 10.19303/j.issn.1008-0384.2012.05.016
Su, L., Du, H., Zeng, F. P., Peng, W. X., Rizwan, M., Núñez-Delgado, A., et al. (2019). Soil and fine roots ecological stoichiometry in different vegetation restoration stages in a karst area, Southwest China. J. Environ. Manag. 252:109694. doi: 10.1016/j.jenvman.2019.109694
Sun, S. C., Jin, D. M., and Shi, P. L. (2006). The leaf size–twig size spectrum of temperate woody species along an altitudinal gradient: an invariant allometric scaling relationship. Ann. Bot. 97, 97–107. doi: 10.1093/aob/mcj004
Thakur, D., Rathore, N., and Chawla, A. (2019). Increase in light interception cost and metabolic mass component of leaves are coupled for efficient resource use in the high altitude vegetation. Oikos 128, 254–263. doi: 10.1111/oik.05538
Tian, Y. L., Yang, H., Wang, F. L., Kang, H. B., Xue, Y., and Wang, D. X. (2021). Differences of leaf functional traits of Pinus tabuliformis and its response to altitude gradient in the middle of Qinling Mountain. Acta Bot. Boreal. Occident. Sin. 41, 300–309. doi: 10.7606/j.issn.1000-4025.2021.02.030
Tonin, R., Gerdol, R., Tomaselli, M., Petraglia, A., Carbognani, M., and Wellstein, C. (2019). Intraspecific functional trait response to advanced snowmelt suggests increase of growth potential but decrease of seed production in snowbed plant species. Front. Plant Sci. 10:289. doi: 10.3389/fpls.2019.00289
TopaloĞlu, E., Ay, N., Altun, L., and Serdar, B. (2016). Effect of altitude and aspect on various wood properties of Oriental Beech (Fagus orientalis Lipsky) wood. Turk. J. Agric. For. 40, 397–406. doi: 10.3906/tar-1508-95
Volaire, F. (2008). Plant traits and functional types to characterise drought survival of pluri-specific perennial herbaceous swards in mediterranean areas. Eur. J. Agron. 29, 116–124. doi: 10.1016/j.eja.2008.04.008
Wang, D., Yang, X., Han, H. P., Zhang, L. Q., and Xue, J. H. (2014). The pipe model theory based on the hydraulic architecture parameter with Robinia pseudoacacia seedlings. J. Zhejiang A&F Univ. 31, 488–493. doi: 10.11833/j.issn.2095-0756.2014.03.024
Watt, M. S., Zoric, B., Kimberley, M. O., and Harrington, J. (2011). Influence of stocking on radial and longitudinal variation in modulus of elasticity, microfibril angle, and density in a 24-year-old Pinus radiata thinning trial. Can. J. For. Res. 41, 1422–1431. doi: 10.1139/x11-070
Westoby, M., Falster, D. S., Moles, A. T., Vesk, P. A., and Wright, I. J. (2002). Plant ecological strategies: some leading dimensions of variation between species. Annu. Rev. Ecol. Syst. 33, 125–159. doi: 10.1146/annurev.ecolsys.33.010802.150452
Yan, E. R., Wang, X. H., Chang, S. X., and He, F. (2013). Scaling relationships among twig size, leaf size and leafing intensity in a successional series of subtropical forests. Tree Physiol. 33, 609–617. doi: 10.1093/treephys/tpt042
Yang, D. M., Li, G. Y., and Sun, S. C. (2008). The generality of leaf size versus number trade-off in temperate woody species. Ann. Bot. 102, 623–629. doi: 10.1093/aob/mcn135
Yang, Y. Y., Xiao, C. C., Wu, X. M., Long, W. X., Feng, G., and Liu, G. (2021). Differing trade-off patterns of tree vegetative organs in a tropical cloud forest. Front. Plant Sci. 12:680379. doi: 10.3389/fpls.2021.680379
Yang, D. M., Zhan, F., and Zhang, H. W. (2012). Trade-off between leaf size and number in current-year twigs of deciduous broad-leaved woody species at different altitudes on Qingliang Mountain, southeastern China. Chinese J. Plant Ecol. 36, 281–291. doi: 10.3724/SP.J.1258.2012.00281
Yu, J. L., Hou, G., Zhou, T. C., Shi, P. L., Zong, N., and Sun, J. (2022). Variation of plant CSR strategies across a precipitation gradient in the alpine grasslands on the northern Tibet Plateau. Sci. Total Environ. 838:156512. doi: 10.1016/j.scitotenv.2022.156512
Zhang, X. S., Zhao, N. N., Zhou, C. N., Lu, J., and Wang, X. T. (2022). Seedling age of Abies georgei var. smithii reveals functional trait coordination in high-altitude habitats in southeast Tibet. Front. Ecol. Evol. 10:955663. doi: 10.3389/fevo.2022.955663
Zhao, F. C., Guo, W. B., Zhong, S. Y., Deng, L. P., Wu, H. S., Lin, C. M., et al. (2018). Effects of indirect selection on wood density based on resistograph measurement of slash pine. Sci. Silvae Sin. 54, 172–179. doi: 10.11707/j.1001-7488.20181020
Zhao, W. X., Zou, B., Zheng, J. M., and Luo, J. F. (2016). Correlations between leaf, stem and root functional traits of common tree species in an evergreen broad-leaved forest. J. Beijing For. Univ. 38, 35–41. doi: 10.13332/j.1000-1522.20160087
Zhou, Y. J., Cheng, L., Wang, M. T., Zhu, G. J., Zhong, Q. L., Guo, B. Q., et al. (2019). Seasonal changes of fine root traits in Pinus taiwanensis Hayata at different altitudes in the Wuyi Mountains. Acta Ecol. Sin. 39, 4530–4539. doi: 10.5846/stxb201806211367
Keywords: plant functional traits, current-year twigs, fine roots, Abies georgei var. smithii, altitude, Tibet
Citation: Wang C, Duan F, Zhou C and Lu J (2023) The altitudinal distribution characteristics of functional traits reflect the resource allocation strategy of Abies georgei var. smithii in southeast Tibet. Front. Ecol. Evol. 11:1055195. doi: 10.3389/fevo.2023.1055195
Edited by:
Ming Jiang, Northeast Institute of Geography and Agroecology (CAS), ChinaReviewed by:
Lin Zhang, Institute of Tibetan Plateau Research (CAS), ChinaGang Fu, Institute of Geographic Sciences and Natural Resources Research (CAS), China
Copyright © 2023 Wang, Duan, Zhou and Lu. This is an open-access article distributed under the terms of the Creative Commons Attribution License (CC BY). The use, distribution or reproduction in other forums is permitted, provided the original author(s) and the copyright owner(s) are credited and that the original publication in this journal is cited, in accordance with accepted academic practice. No use, distribution or reproduction is permitted which does not comply with these terms.
*Correspondence: Chenni Zhou, chenni2018@126.com; Jie Lu, tibetlj@163.com