The primate workplace: Cooperative decision-making in human and non-human primates
- 1Division of Psychology, Communication and Human Neuroscience, University of Manchester, Manchester, United Kingdom
- 2Department of Earth and Environmental Sciences, University of Manchester, Manchester, United Kingdom
The success of group foraging in primates is not only determined by ecological and social factors. It is also influenced by cognition. Group foraging success is constrained, for instance, by the challenges of coordination, synchrony and decision-making, and it is enhanced by the ability to share, learn from others and coordinate actions. However, what we currently know about the cognition of individuals in groups comes primarily from experiments on dyads, and what we know of the effect of ecological factors on group dynamics comes from larger wild groups. Our current knowledge of primate group behaviour is thus incomplete. In this review, we identify a gap in our knowledge of primate group dynamics between the dyadic studies on primate cooperation and the large group observational studies of behavioural ecology. We highlight the potential for controlled experimental studies on coordination and cooperation in primate groups. Currently, these exist primarily as studies of dyads, and these do not go far enough in testing limits of group-level behaviours. Controlled studies on primate groups beyond the dyad would be highly informative regarding the bounds of non-human primate collaboration. We look to the literature on how humans behave in groups, specifically from organisational psychology, draw parallels between human and non-human group dynamics and highlight approaches that could be applied across disciplines. Organisational psychology is explicitly concerned with the interactions between individuals in a group and the emergent properties at the group-level of these decisions. We propose that some of the major shortfalls in our understanding of primate social cognition and group dynamics can be filled by using approaches developed by organisational psychologists, particularly regarding the effects of group size and composition on group-level cooperation. To illustrate the potential applications, we provide a list of research questions drawn from organisational psychology that could be applied to non-human primates.
Cooperating in groups
Cooperation, the proficiency with which primates can be said to cooperate, and the cognitive capacities needed for it, have been a particular focus of experimental studies (Visalberghi et al., 2000; Rekers et al., 2011; Stocker et al., 2020; Martin et al., 2021). Such studies have however focused on dyads rather than individuals embedded in larger social groups. There is, for example, only one study that experimentally tests cooperation in chimpanzees in groups larger than two (Suchak et al., 2016), which is surprising given most observed instances of possible cooperation in wild chimpanzees, hunting and coalitionary attacks, involve large groups. Results from human studies also indicate the dyad is a special case in regard to group dynamics. Zimet and Schneider (1969) found that dyads showed significantly less within-group aggression and significantly more within-group support as compared to other group sizes and that the difference between groups of two and three was much greater than between three and four. Nosenzo et al. (2015) found cooperation to be highest of all in two-person groups. There may be something unique about dyadic cooperation that makes studies testing groups larger than two even more important.
This review identifies a gap between the dyadic cooperation studies of cognitive biologists and comparative psychologists and the larger group observations of behavioural ecologists and primatologists; it recognises a need for experimental studies beyond the dyad to tell us more about individual social cognition in naturalistic groups, particularly group size effects on cooperation. The cognitive abilities necessary for individuals in large primate groups to make consensus decisions, coordinate activity budgets and movement and mitigate the numerous costs of social living are not well understood despite being particularly relevant to primates that forage in groups. Specifically, we are interested in what happens when individual or dyadic coordination problems are scaled up to the size of natural groups.
Accordingly, we provide an overview of primate group dynamics (both human and non-human); what differentiates individual from group behaviour, how group-level decisions are made, and what is known about coordinated action. We draw attention to the dearth of studies into group size effects on non-human primate group behaviour and provide an overview of such studies in human organisational psychology. In doing so we highlight where interests align and present opportunities for integration of approaches across disciplines. We also provide potential future research questions and discuss what we would expect these to find, as well as their relevance to wild primate food acquisition behaviours such as making coordinated movement decisions when foraging and engaging in group hunts.
Cooperation
Cooperation has a variety of definitions across different fields. An adaptive (biological) definition of cooperation is any behaviour that provides a fitness benefit to another and has evolved for this benefit to the recipient (West et al., 2007). In this review, we will however use a social interaction perspective definition that considers the interdependent nature of action of two or more individuals to produce a net payoff for all actors (Bshary and Bergmüller, 2008). Cooperation in this sense is an act that requires the coordination of two or more individuals to attain mutually beneficial outcomes. One goal is to distinguish intentional from incidental cooperation, wherein individuals converge on a mutually beneficial outcome as a consequence of following individual aims.
The simplest form of cooperation is by-product mutualism (or by-product beneficial behaviour; Bshary and Bergmüller, 2008) where independent self-serving actions lead to fitness benefits for both actors. The challenge for psychologists is to distinguish between intentional and incidental cooperation. For instance, prosocial actions have to be motivated for the benefit of recipients to be intentionally prosocial (Jensen, 2016a,b). This might seem intuitively obvious, but it is very difficult to demonstrate. Additionally, as opposed to competition which has been discussed in terms of cognitive abilities such as theory of mind, responses to stimuli and the nature of representation, cooperation research has primarily focused on individual motivations such as other-regarding concerns and social tolerance (Schmelz and Call, 2016). There is scope for a greater consideration of the cognitive underpinnings behind cooperation and understanding it will require a consideration of both motivations as well as the cognitive capabilities required, such as an understanding of the motivations and goals of others (perspective taking or theory of mind). An important area of investigation into coordinated action has been into joint intentions or shared goals, namely knowing the goals of others and being motivated to see those goals completed together (see, for example, Tomasello et al., 2005). To successfully coordinate their efforts, individuals will also need the ability to communicate these intentions to one another. In addition, individuals should also be able to inhibit immediate gratification, recognise the role of partners, suppress competitive tendencies, and potentially even weigh their own needs against that of the group as a whole, suggesting the need for strategizing capabilities, and the ability to perform approximate optimisation calculations. We have then a broad list of the capabilities that an animal may need to possess to successfully cooperate, and that we might expect them to employ when carrying out experimental cooperative tasks; theory of mind, maintenance of joint intentions, communication of intent, strategic calculation and inhibition of competition and aggression.
This last capability—inhibition of natural competitive urges—may be the most vital. Cooperation is one of the major benefits that might be afforded by group living (Silk, 2005; Sussman et al., 2005) but given competing individual interests it is challenging to understand how it is maintained, in part because the costs and benefits of group living are not equally distributed. Animals live in groups because it affords them adaptive benefits of sociality such as decreased predation (Foster and Treherne, 1981; van Schaik, 1983; Fichtel and Kappeler, 2002), increased foraging efficiency (Grueter et al., 2018) and mating opportunities for example (Majolo and Huang, 2018). Larger groups may also provide more benefits than smaller ones, as they can better monopolise food sources (Janson and Van Schaik, 1988). For example, large groups of vervet monkeys are more likely to make incursions into the ranges of smaller groups than vice versa and to expand their ranges at the expense of smaller groups (Cheney and Seyfarth, 1987), larger resident groups of capuchin monkeys are more likely to win a between-group contest (Crofoot et al., 2008) and larger groups of gorillas have improved foraging efficiency compared to smaller ones (Grueter et al., 2018). Animals in groups may also spend less time looking for food, as they can pool and share information about its location and quality (Garber et al., 2009).
Foraging and moving in groups
While there are benefits to group foraging, there are costs associated with grouping, the foremost being within-group competition for resources (van Schaik, 1983). Animals in a group are in close proximity and share limited resources, particularly within the same food patches. Within-group competition for food may be as much as ten times greater than between-group (Janson, 1985, Cebus apella). Such competition may take the form of scramble competition, where individuals within groups similarly deplete resources, lessening the availability for all or contest competition, where aggressive interactions are used to gain access to food (Wrangham, 1980; Isbell, 1991). The outcome of these contests are dominance hierarchies that are based on relative size, strength, age or other factors.
Such dominance asymmetries may exacerbate the costs of group-living, particularly for those at the bottom of the hierarchy. Dominant individuals often obtain greater amounts or higher quality food than lower-ranking individuals when feeding at the same sites (Janson, 1985; Saito, 1996; White et al., 2007). Being a dominant individual is not advantageous in every circumstance, however, and the extent to which resources are unequally shared across ranks may depend on the particular dominance style of a species, which may be placed upon an axis with despotic at one extreme and egalitarian at the other (Thierry, 2000). The energetic and injury costs of maintaining dominance may be particularly intense in despotic species for example, and dominance may at times be a double-edged sword (see Sapolsky, 2005 for a review).
What is clear, however, is that the costs and benefits of sociality are often not equitably distributed, with some individuals claiming a greater share of resources than others, enjoying the benefits but little of the costs of group living. Conversely, lower-ranking individuals still participate in the work of group living (vigilance and predator defence) but may receive fewer benefits whilst incurring higher costs. The motivations and interests of individuals are also unlikely to always align. For example, the preferred time to move from one foraging patch to another may vary between individual group members due to divergence in activity budgets, dietary needs or simply to what extent they can monopolise feeding at a particular patch.
These inequalities lead to a tension between foraging in groups and foraging alone. If they wish to stay together, members of a group must often choose between mutually exclusive options (Conradt and Roper, 2005) with the result that synchronisation is likely to be more costly to some individuals than others, as it comes at the expense of their own nutrition (Conradt and Roper, 2000).
Of course, group-living animals do not always move together in synchrony. Animals may distribute themselves across patches within their territory to avoid feeding competition and maximise their individual fitness. The ideal free distribution (Fretwell, 1969) states that this distribution should be in proportion to the amount of resources available in each patch. A fission-fusion mode of group movement, as is seen in chimpanzees, spider monkeys and olive baboons (Symington, 1990; Chapman et al., 1995; Asensio et al., 2008; Alberts, 2013), may be the best way to maximise individual fitness in many contexts, particularly when there are differences in energy requirements amongst group members (Amici et al., 2008; Aureli et al., 2008; Sueur et al., 2011). Nevertheless, it remains true that group movement decisions can have important fitness consequences and an understanding of how consensus decisions are made is necessary. Decision-making may constitute a major cost of group living—in terms of time and energy. We know, for example, that in chacma baboons, making a decision about the direction of movement in the morning may take as much as 202 min, a loss of valuable foraging time (Stueckle and Zinner, 2008), and in chimpanzees, time to decide on a sleeping site (the interval between the first ‘nest grunt’ and nest building) can take an average of 30 min in a group as compared to 19 min alone (Janmaat et al., 2014).
Optimal decision-making in groups
Given the optimum decision for the group may differ from that of the individual, individuals cannot always make optimal decisions for themselves in a group context. There is increasing evidence that animal groups can come to collective decisions about when and where to move (King and Cowlishaw, 2009; King and Sueur, 2011; Strandburg-Peshkin et al., 2015; Janson, 2016). These decisions appear to be based on leadership (which is distinct from dominance) and quora or democratic consensus. However, what is less well understood is how they find the best solutions (Fischer and Zinner, 2011). In humans, consensus decisions range from the movement of crowds to complex international trade agreements. Indeed, many of the problems that people face result from either an inability to reach consensus, or from a breaking down of the decision-making process. An understanding of how decision-making works and why it may fail is therefore important (for reviews, see Couzin et al., 2005; Dyer et al., 2009; King and Sueur, 2011). What we do understand is the theoretical framework for optimal foraging at an individual level, have comprehensive evidence for the social and ecological challenges faced by group-living animals, and global-level models and empirical evidence for collective decisions. Although collective decisions are made at the group level, they are the outcome of individuals assessing how best to integrate their optimal decisions with the motivations and preferences of other individuals. The cognitive processes underpinning consensus and compromise and how collective decisions impact on an individual’s ability to make optimal decisions within a social framework are virtually unknown.
We suggest that this deficit in our understanding of how groups make optimal decisions is due in part to a lack of concerted research into the cognitive processes underlying group behaviour. The behavioural ecology of primate groups has been thoroughly studied in terms of how social interactions, resource distribution and risks impact on individual fitness. Such studies have revealed the impact of group size on between- and within-group competition (Janson and Van Schaik, 1988), predation pressure (Sterck et al., 1997) and individual fitness and fecundity (Koenig, 2002; Majolo et al., 2008). The cognitive processes underlying these behaviours, however, have received less attention. Although the Social Brain Hypothesis, in particular, suggests that primate cognition has evolved to mitigate socioecological challenges, there is little evidence of, or a framework for studying, the cognitive mechanisms and processes needed to solve or mitigate social challenges (Dunbar and Shultz, 2022). In fact, despite references to ‘social cognition’, there is little real understanding of what this means or even an acknowledged consensus definition of ‘cognition’ within the study of animal social behaviour. One way to investigate these cognitive processes would be to investigate the impact of group size on group behaviour, specifically cooperation.
Within the primate literature group size has been investigated in relation to cooperation to an extent. King and Sueur (2011) discuss how vocal repertoires might facilitate and maintain cooperation in non-human primates as group size increases, and Samuni et al. (2021) find that the likelihood of an individual chimpanzee participating in an intergroup encounter increases with the number of other participants. Controlled experiments are lacking, however.
Working in groups
One field that has more explicitly studied group size in relation to group cooperation is human organisational psychology. Organisational or industrial psychology, as the study of behaviours in the human workplace, is by nature explicitly concerned with the interactions between individuals within a group—how they make consensus decisions, what is the ideal group size and composition for efficient collaboration and how conflicts of interest and intra-group aggression are overcome. Parallels can be drawn between this field and that of behavioural ecology, in that both focus on the benefits and costs of behaviour. Where they diverge is at what level these costs and benefits are assessed, with behavioural ecology focusing on individual fitness and organisational psychology the successful outcomes of the group (or company). There is a rich literature into human group dynamics in the workplace, exploring how group composition, size, and leadership can affect the efficiency and quality of decision-making (Gallupe et al., 1992; Laughlin et al., 2006). Primatologists studying human’s closest living relatives may miss out by overlooking the human literature as these approaches can help us better understand group dynamics in the non-human ‘workplace’.
Although the human office environment may seem far removed from the collaborative activities of other primates, there are parallels to be drawn. After all, both animals and humans are in the business of resource acquisition. Both may involve coordination, inhibition of competitive tendencies, strategic thinking and the navigation of social dilemmas to achieve optimal outcomes for everyone, suppressing free-riding tendencies.
The study of social behaviour in groups beyond the dyad utilising methods and theory rooted in human psychological research may allow us to ask questions such as, is there a threshold group size at which cooperation becomes unsustainable or too cognitively demanding for individuals due to difficulties in coordination, communication or motivation? What is the optimum sized group for mutually beneficial outcomes? What group composition achieves the best results? In this review we will look at how these questions have been answered in humans and consider what we might expect to find if they were similarly applied to non-human primates.
How are groups different from individuals?
Although groups are collections of individuals whose behaviour is expected to be a product of individual fitness maximisation, there are fundamental differences between groups and individuals. We should therefore expect the behaviour of an individual primate forager to be different when in a social context.
As well as affecting an individual’s risk of predation and competition and their foraging efficiency, group membership is expected to affect their decision-making. Foraging primates need to make important decisions regarding where to forage and when and may also take part in coordinated group activities such as range defence, predator mobbing (Boinski et al., 2000) and hunting (Boesch, 1994). Being part of a social group complicates decision-making in numerous ways, as it involves keeping track of and synchronising with multiple individuals, as well as contending with differing opinions and interests. This has been explicitly examined in organisational psychology.
Insights from organisational psychology
The fundamental differences between groups and individuals, particularly with regard to decision-making, is a common topic within the field of organisational psychology, and the relative performance of group versus individual decisions has received a lot of attention. Jeffrey Rubin puts it succinctly: “two heads may be better than one, and occasionally worse than one, but they are always very different from one” (Rubin, 1984).
Early work into group performance demonstrated this, with the discovery that large groups of people may in some cases collectively outperform individual experts. This was found by Francis Galton in 1906 when he observed that a crowd could guess the weight of an ox with a surprising degree of accuracy, and more so than any one individual guess (Surowiecki, 2005). This phenomenon is known as ‘the wisdom of crowds’ and is thought to be more than a law of large numbers effect, as it is the diversity of opinions and heuristics that contributes to this ‘collective intelligence’ not simply the size of the crowd (Wagner and Vinaimont, 2010).
Groups may even be viewed as ‘distributed systems’ in which individuals have their own set of independent desires and perceptions yet still influence each other and interact to create emergent structures and properties that are not produced by any one individual. In this way people can contribute to collective behaviour patterns that they do not even perceive or understand. When cognition is seen not as an individual skill but simply as adaptive problem-solving, it is easy to see how collective group behaviours—in which information processing and computational capacity may be maximised as a consequence of its distribution across different but interacting ‘units’—could be viewed as cognitive. This framework of ‘group cognition’, in essence, posits that systems larger than the individual are themselves cognitive (Gureckis and Goldstone, 2006; Goldstone et al., 2009; Theiner et al., 2010). For the purposes of decision-making in nonhuman primates however, we focus on individual behaviours in a group context, and how individual cognition is influenced by social interactions.
The effect of individuals in a group is very often not additive, and groups—particularly their collective and cooperative efforts—must be considered distinct entities, rather than just the combined effect of individual processes. We should expect this to be as true of primate group behaviours—including foraging and other coordinated activities—as it is of groups of humans. The question of whether groups outperform individuals is therefore a complex one. Within human group psychology, a few generalisations and observations have been made.
The first of these is that, although living in groups may be beneficial, there are instances in which individual efforts may suffer from being a part of a group. For example, groups can often inhibit the cooperative or prosocial actions of individual members. This is the case in the ‘bystander effect’, a phenomenon where people are less likely to intervene to help a victim when there are more other people present. This has even been found in online environments (Guazzini et al., 2019). The destructive effect of groups can also be seen in ‘social loafing’, the phenomenon of individuals exerting less effort when in a group than they would alone. The effect is thought to be motivational, with individual members feeling a lack of uniqueness and a sense that their efforts are less important (Rubin, 1984). This effect was first investigated by Ringlemann using tug-of-war experiments. The finding that as groups increase in size individual members become less productive is known as the Ringelmann effect (Ingham et al., 1974; Figure 1). In this way, individual-level cognitive processes can hamper group-level efficiency.
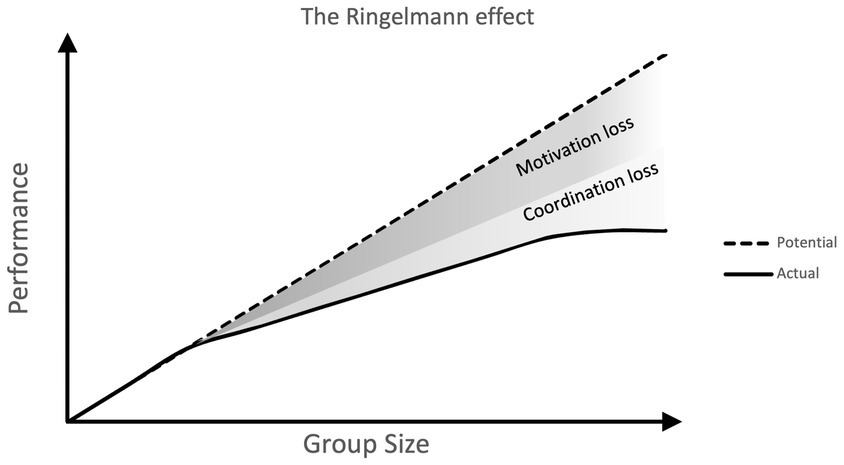
Figure 1. Visualization of the Ringelmann effect. As group size increases individual members become less productive. Actual performance is lower than potential performance, due to declines in coordination and motivation.
Group and individual decision-making may be measured and judged across numerous dimensions. These have included efficiency, accuracy, fairness, member satisfaction, leadership effectiveness and even rationality. Miner (1984) found that groups made more accurate decisions than that of the average individual, but equal to or inferior to that of the best individual ones. The decision-making strategy applied in this study also had a significant influence on relative group or individual performance. Vollrath et al. (1989) found groups to outperform individuals on memory tasks, having more accurate recall of facts, indicating that collective pooling and processing of information may outshine individual efforts, the ‘pool of competence hypothesis.’ Kugler et al. (2012) found that groups are (mostly) more rational decision-makers than individuals, due to increased prior experience, information sharing, processing capabilities, and monitoring.
Applications to primatology
From these observations—that group efforts are distinct from the additive efforts of individuals, and that many group properties may affect their performance—it is clear that groups (particularly their collective and cooperative activities) must be considered as a whole, rather than just as the combined effect of individual processes and behaviours. Such an understanding of group dynamics is however lacking when it comes to primate cognition. Phenomena such as social loafing and the Ringelmann effect for example would be interesting to investigate in primate cooperation experiments.
Although human group dynamics may seem removed from the activities of wild primates, parallels can be drawn. For example, we might expect to observe bystander effects in nonhuman primates when it comes to group defence against predators, such as alarm calling or mobbing, or similar participatory deficits when it comes to extracting or catching food in a foraging context. Decision-making accuracy and efficiency in groups versus individuals are pertinent to movement decisions in foraging and selecting least-cost routes (Green et al., 2020). Pooling of knowledge may be important when individuals have differing knowledge of food or water locations during periods of scarcity (Rapaport and Brown, 2008; Garber et al., 2009). Thus, group dynamic phenomena shown in humans may also be relevant to non-human primates. Specifically, we might expect there to be significant influences of both the destructive and positive effects of group effort when it comes to making decisions about where to forage, when to move between resource patches, and which direction to move in.
How do groups move together?
The primary business of primates is foraging, and one of the most elementary forms of collective behaviour and decision-making process that can be studied regarding this is group movement. How then do primates, human and not, make these kinds of decisions (when to move, where next?) in the face of conflicts of interest, and keep a balance between the benefits of sociality and their own individual needs? Within the primate literature, these are questions that have only recently received much attention. Previously it was considered that very little was known about the mechanisms governing decision-making in vertebrate animal groups (Ward et al., 2008), as much of the research into the collective movement of groups has been conducted on insects (eusocial insects in particular) and birds.
What has been found in many cases in these taxa is that although collective behaviour can look very complicated, the underlying processes are often very simple. In many cases collective behaviour emerges through self-organisation; despite appearances, there is no “top-down” control, only local interactions giving rise to global patterns. Such self-organised systems have been described in migrating locusts (Bazazi et al., 2008) wherein the local interaction rule giving rise to their mass movement appears to be “move or be cannibalised” and starlings (Hemelrijk and Hildenbrandt, 2015) in which avoiding collisions with near neighbours results in extremely complex looking movement patterns. Such self-organisation can exist within very large animal groups, like locusts, where there is considered to be no individual recognition or dominance hierarchies, but it can also exist in smaller and more complex social groups, including humans and non-human primates. For example, Meunier et al. (2006) found through experimentation and modelling that collective movement in white-faced capuchins could be partially explained by anonymous mimetism—wherein individuals are more likely to mimic the actions of others, no matter who they are. In this way, their group movements simply depend on the number of individuals who have already moved.
Approaches used to understand group movement in insects have also been applied to human groups. For example, Shahhoseini and Sarvi (2017) directly compared ants and human pedestrians under an ‘emergency escape’ paradigm to investigate the rules governing their collective movement. Both humans and ants had to ‘escape’ a confined and crowded chamber that necessitated two streams of individuals merging into one. They found surprising similarities in the emergent collective movement patterns observed. In both, the rate of escape and efficiency of motion were in remarkable agreement and were similarly dependent on the architectural design of the environment. These results demonstrate how the same simple behavioural rules (in this case the forming and maintaining of separate, stable lanes of traffic) may govern collective movement in disparate species, and that these rules may work the same independently of species, cognitive abilities or group size.
Although such anonymous systems do exist, wherein movement decisions are a result of individual interactions, in both humans and other primates collective decisions are not always equally shared and often involve a form of leadership, whether intentional or automatic. In the emergency escape paradigm, for example, all the people possessed the same level of knowledge and received the same instructions, and thus a decision was not needed (Shahhoseini and Sarvi, 2017). Dyer et al. (2009) on the other hand experimentally tested how human crowds come to consensus decisions regarding direction of movement when only a small minority were informed about the target. They found that just a small number of informed individuals could successfully lead the naive ones to the target destination without any verbal communication or obvious signalling. Subtle cues appeared sufficient to initiate following behaviour. The presence of these informed individuals decreased both the time taken to reach the target and the degree of deviation from the target, emphasising the importance of informed leadership in collective decision-making.
Interestingly, when a conflict was introduced (informed individuals given one of two separate targets) the time taken to reach one of the targets as a group was not significantly increased. This indicates that in small groups humans are capable of resolving conflicts and come to consensus decisions fairly easily and quickly. The importance of the ‘tyranny of the majority’ or democratic forces was also evident, as in these conflict situations the direction that had the greatest number of ‘supporters’ tended to be chosen.
Similarly, in the last decade, the mechanisms behind group decision-making have started to be uncovered in wild non-human primate groups (King and Sueur, 2011). This review affirms that in many cases simple rules-of-thumb or heuristics are used to maintain group cohesion, rather than more complex tracking of individuals or use of signals. A review by Petit and Bon (2010) similarly emphasises that even in species deemed to have high cognitive complexity, simple self-organised processes can explain collective movement.
What is notable, however, is that the majority of this literature on group movement focuses on the ‘what’ and not the ‘why’. The models discussed (self-organisation and mimetism) focus on the physical and spatial mechanisms of movement, and high-level synchrony, and do not address the underlying individual cognition. They do not investigate why individuals are choosing directions for example, or what the quality of shared decisions might be to an individual. What is missing from the literature are studies that link such group-level behaviours to individual optimal foraging decisions, especially where there is social inequality. One review that does address the cognitive processes behind group movement decisions is Fischer and Zinner (2011), who emphasise the potential role of signals in eliciting motivational changes in group members, and thereby stimulating coordinated movement. A study by King and Cowlishaw (2009) also draws a link between individual variation in activity budgets and group-level behaviours. One of the few papers to explicitly evaluate the cognitive decisions underlying direction choice and patch preferences (Janson, 2016), shows that capuchin groups use information on time, energy and renewal rates to select patches. Finally, a recent paper proposes a framework to apply individual optimal foraging models to group-level decisions (Davis et al., 2022). This paper, although addressing the ‘why’ question and discussing how inter-individual differences influence collective decisions, does not address the psychology underlying individual decisions. There remains great potential for studies that explicitly investigate how individuals arrive at shared decisions.
Leaders and followers
What has been found repeatedly is that, like humans, social animals look to their group mates when making decisions, drawing information from the decisions others have made in order to make their own (King and Sueur, 2011). Individuals are more likely to choose options already chosen by others. Studying whose judgements they see as more valuable has revealed interesting dimensions of decision-making.
Within animal groups not all individuals are equal, and in particular, some are more informed than others. Leadership often emerges as a function of these information differences between individuals, and how successful leadership is likely to be depends on the importance individuals put on their own information versus that of others. How then are leaders chosen and why are they trusted?
In non-human primates
A quorum-like process to initiate and coordinate group movement has been observed in multiple primate species. In the collective movement of wild snub-nosed monkeys, adults were more likely to initiate decision-making with regard to initiation and direction, demonstrating how leadership may be based on age, experience, and/or dominance (Wang et al., 2020). However, in these monkeys, leadership did not fall on one particular individual, but rather several. Additionally, leaders were not despotic or omnipotent, and instead others ‘voted’ for them simply by copying the direction and movement initiated by the leader. This field study found that a threshold, or quorum level, number of voters was needed for successful collective decision-making. Similarly, Sueur et al. (2010) found that when Tonkean macaques made decisions on moving between patches of vegetation, the majority would ‘vote’ on several directions ‘proposed’ by several different initiators or ‘leaders’ by moving in that direction. This group decision can be viewed sequence of three different decisions, with three corresponding quorums, one to switch direction, one to choose the departure time, and one to choose the departure direction.
Another process that may be at work in these types of collective decisions is mimetism, a hypothesis that proposes an individual’s probability of joining a movement increases with the number of individuals who have already joined the movement. This is distinct from quorum, in that there is no requirement for a specific threshold number. Mimetism may be selective, wherein relationships affect the process, or anonymous, where they do not. Wang et al. (2015) found that Tibetan macaques used a combination of mimetism and quorum thresholds to achieve collective movement, such that until a quorum level of adults (three) participated in a movement no group movement would occur. Once this level had been reached mimetism occurred—wherein the probability of any individual joining increased with the number that had already joined, but without a specific threshold number. Once a secondary quorum threshold was reached (seven) entire group movement always occurred.
Strandburg-Peshkin et al. (2015) also provide evidence for this shared and ‘democratic’ collective decision-making. By tracking wild baboons, they found that when deciding where to go individuals were more likely to follow multiple initiators who were in agreement. When agreement was low, an individual baboon was less likely to follow anyone at all, delaying the decision. When there was conflict over the direction of travel, the consensus achieved was dependent on the magnitude of difference in choices, such that when the angle between the two directional choices was large the group would choose one direction or the other, more often the one that had the most “supporters.” When the angle was below a critical threshold the group instead compromised by moving in the average of the directions. This study demonstrates that primates may use multiple decision-making mechanisms (e.g., a quorum-based either/or decision versus an average) when engaging in collective movement, and also that the influence of high-ranking individuals can be limited, as dominance rank did not correlate with initiation success. Decisions were shared and based instead on majority preference. These results demonstrate the importance of social facilitation and the value of shared decision-making processes in some primate species. Instead of solely through despotism or a hierarchy of influence, consensus decisions in wild primates can emerge from shared processes. It is not necessarily an either/or situation however. Petit and Bon (2010) emphasise that decision-making in primates may be reached by a combination of different rules, both those based on inter-individual differences like dominance, and self-organised rules based on simple responses to local interactions.
In humans
The human literature has dived deeper into the dynamics of group decision-making and leadership. Within the human organisational psychology literature in particular, leaders and the attributes they possess is a large topic of study. Crucially, however, it must be noted that leadership can mean different things across disciplines, so parallels between leadership studies in humans and non-human primates can be difficult.
For example, leadership can be either automatic or intentional, passive or active. Within collective movements such as that discussed above, we can see that effective leadership can be given by even a small subset of informed individuals. As in self-organised systems, it can be based on simple rules for local interactions and can work without any explicit signals, or any group members knowing who the informed individuals are, the quality of their information, or even whether they are in the majority or minority. These informed individuals simply exert their influence through local interaction rules, and yet can thereby guide a whole group. For example, Dyer et al. (2009) found that when they asked a crowd of 200 people to walk around with just one rule “stay within an arm’s length of each other” and no communication, only a handful of ‘informed’ individuals given a specific target were needed to move the whole naive group there. Similarly, in Sueur et al.’s (2010) Tonkean macaques, although initiators are described as leaders, their system of moving between patches may well conform to the main principles of self-organised group decision-making; individuals respond to local cues, and there is no explicit communication, no centralised processing of information and no centralised control. This type of passive leadership also emerges in human groups.
Conversely, leadership may also be conscious, intentional, and even striven for. Pulling apart these two types of leadership is not always easy, and it can be unprofitable to assume leadership conforms to the same principles cross-species. However, a look at the human organisational literature may highlight shared attributes of leadership, as well as reveal promising research approaches that may be applied to non-human primates.
The importance of social information (that gained through observation of or interaction with others) in human collective behaviour was demonstrated by Faria et al. (2010). This study found that pedestrians were more likely to cross a road if their neighbour had begun to cross. Interestingly this often seemed to lead to incorrect decisions (wherein pedestrians had to return to the curb to avoid a car), meaning that social information is sometimes misleading but may still be used even at the cost of individual safety, such is the influence of leader-follower behaviour in humans.
Leadership can arise from knowledgeable individuals (whether this knowledge is gained through age or experience, or simply heterogeneous access to information), but it can also emerge from other factors including dominance, sex, age, experience, as well as personality measures such as extraversion (Judge and Bono, 2000), ambition (Van Vugt, 2006) or a personal desire for authority (Fehr et al., 2013). These factors do not require that the leader be more knowledgeable. It can also arise as a consequence of heterogeneity of interests, due to physiological features (such as sex, age or reproductive status) that result in payoff differences. For example, an animal in oestrus may be hungrier and will therefore have a greater motivation to move to seek food, making them more likely to emerge as a leader (King et al., 2009). Thus, the ones in the greatest need may become the ones to lead, and this motivational component of leadership is thought to be present in humans just as other animals.
As discussed, leaders may also be either chosen and unwitting or self-appointed. For example, when people were given specific instructions about how to walk across a room whilst staying together in groups of four, contextual factors, like a person’s location in the group, and individual factors, like characteristic locomotor behaviour, contributed significantly to the emergence of a leadership role. For example, participants in the front row (who could be directly observed by those in the back) more often became leaders (Lombardi et al., 2020). Interestingly, however, they also found that one specific individual tended to take on that role more than 60% of the time, initiating the directional change first and irrespective of where they were placed in the group. In doing so they took advantage of the collective movement and caused others to follow them, becoming a self-appointed leader. This study demonstrates how leader-follower behaviour can emerge out of a combination of simple geometric rules and individual differences, including personality.
Effective leadership may be particularly important when it comes to solving collective action problems. In small-scale societies in Bolivia, Ethiopia and South Sudan, effective leaders tended to have more capital than followers, be that age-related knowledge, body size or social connections. This abundance of capital is thought to facilitate leadership through mitigating some of the associated costs (Glowacki and von Rueden, 2015). This study also demonstrates the value of institutional leadership in resolving collective action problems, even in small-scale societies. The organisation of leadership around food production tended to be more ad-hoc and informal, whereas conflict resolution was more likely to involve an institutional leader—one that is formally recognised by the community. Formal leadership may be necessary in these situations as the costs of failure are potentially more destructive to the group than in a particular failure in a food production activity. In this way, leaders may facilitate the evolution and maintenance of cooperation, protecting cooperative societies and endeavours from the destructive effect of free riders, Cooperation has often been thought of as a Darwinian paradox, and one potential solution—punishment for non-cooperation—is often deemed to be costly to the punisher. However, the existence of a single leader who is willing to bear the costs associated with meting out punishment to free-riding individuals, in order to access the status, resources, and reproductive opportunities given to leaders may go some way to making sense of the paradox (King et al., 2009).
Such social norms and systems of enforcement and decision-making do however vary cross-culturally in small-scale societies. In the Batek hunter-gatherers of Malaysia, for example, foraging efficiency is successfully optimised through more egalitarian forms of decision-making, where movement decisions are not made by single individuals but instead through a process of informal group discussion involving most adults in the group (Venkataraman et al., 2017). Nevertheless, social norms and sanctions remain crucial in maintaining large-scale human cooperation (Fehr and Fischbacher, 2004; dos Santos et al., 2013) and, given this importance, could be another fruitful avenue of research in non-human primates. Large group cooperation experiments in controlled settings that give individuals the opportunity to exhibit enforcement strategies, such as punishment, could be very illuminating.
While group movement may be seen as the simplest form of collective behaviour there is evidence of more complex and potentially more cognitively demanding coordinated action in non-human primates. This includes goal-directed group behaviours such as group hunting, antipredator behaviour, and even joint tool use. Although more complex, where these behaviours overlap with movement decisions associated with foraging is that there is a similar need to employ negotiation and to overcome conflicts of interest, as well as to apply cognitive capabilities like joint intentionality, communication, and strategic calculation. Thus, it is helpful to consider both forms of cooperative behaviour when investigating the bounds of non-human of collaboration.
Coordinated action
In the wild
There are numerous examples of wild primates behaving cooperatively by working together to achieve mutually beneficial aims. Primate species can be placed along a spectrum of cooperativeness, from those that live a solitary lifestyle, only coming together to mate, to cooperative breeders at the extreme. In cooperatively breeding primates like the callitrichids, group members help to raise the young of the one breeding pair, at the expense of their own reproduction. The non-breeding helpers carry and provision the young with food, as well as engaging in joint resource and territory defence (Koenig and Rothe, 1991; Burkart and van Schaik, 2010). This form of cooperation is however rare in primates and such familial provisioning and defence may be viewed separately from coordinated, goal-directed behaviour. Behaviours such as territorial defence are found in non-cooperative breeders and goal-directed joint behaviour, such as joint tool use, may require different cognitive abilities than afforded by cooperative breeding and are not likely to be unique to animals like callitrichids (Thornton and McAuliffe, 2015; though see Burkart and van Schaik, 2016). In the context of tool use in particular, there is surprisingly little evidence of naturally occurring, coordinated, goal-directed behaviour in nonhuman primates. There are no observations of capuchin monkeys, for example, working together to crack nuts, with one supporting an anvil while another uses a hammer. There are no records of chimpanzees taking turns to elaborate upon a tool, like a stick for extracting ants. Although there is evidence of tool transfer in limited circumstances (from mothers to offspring), this does not constitute joint action, but rather sharing or helping at best (Musgrave et al., 2016).
However, joint action is not completely absent in nonhuman primates. As well as range defence and predator mobbing in many primate species (Boinski et al., 2000), coordinated action to achieve a shared goal has been seen with cooperative hunting (Boesch, 1994; Samuni et al., 2018) and intra and inter-group conflicts in chimpanzees (Mitani et al., 2010). Although coalitionary support in conflicts is more frequently seen in intra-group agonism (Nishida and Hosaka, 1996; Newton-Fisher, 2006; Smith et al., 2010), some of the most discussed examples of ‘primate cooperation’ come from observations of intergroup violence. Lethal intergroup violence in chimpanzees was first reported by Jane Goodall and has become known since as a prevalent feature of chimpanzee life (Goodall, 1986; Wrangham and Peterson, 1996). These conflicts have even been compared by some to human warfare, specifically that seen in nomadic hunter-gathers (Wrangham and Glowacki, 2012). Intergroup aggression in chimpanzees has been described as coalitionary in nature, with individuals working together, and relying on each other’s support to minimise the risk of injury (Watts and Mitani, 2001).
Mitani et al. (2010) describe adult males participating in boundary patrols prior to intergroup aggression, in which they appear to move in a silent single file, attending to the signs of the others. Given that such patrols and attacks involve group effort, and entail bearing personal risks for the sake of group-level benefits, they might rightly be seen as evidence of purposeful cooperation. However, as Chalmeau and Gallo (1995) point out, using the existence of a common goal as the benchmark for cooperation may be misleading, as a mutually beneficial outcome may arise as a by-product of individually followed aims. Without evidence that joint goals are being conceived and maintained throughout it is hard to exclude the possibility that perceived cooperation is in fact the product of well-timed individual efforts. Willems et al. (2015) also report that communal range defence in primates rarely involves collective action in its strictest sense, in that it is instead best described as strategic individual-level decisions, without the need for jointly held aims.
Insights from experiments
Dyadic experimental studies have aimed to differentiate joint action from simultaneous individual actions, by ensuring that co-operators are interdependent. Such experiments have demonstrated the abilities of non-human primates to work together to solve food-acquisition problems. A powerful experimental paradigm for dyadic cooperation used in primates (as well as other taxa) is the cooperative pulling paradigm, notably the single-string paradigm (Melis et al., 2006b; Hirata and Fuwa, 2007). In this paradigm, two or more individuals work to pull food rewards towards themselves using an apparatus that one could not successfully operate alone. Experiments have consistently shown that nonhuman primates succeed at this (Jacobs and Osvath, 2015; Duguid and Melis, 2020). The task requires that pairs of individuals converge both spatially and temporally on a solution to a joint problem. It was first used by Crawford (1937) who had a pair of juvenile chimpanzees pull a heavy tray together. These individuals were trained to do so and received instructions from the experimenter on when to pull, calling into question the spontaneous nature of their cooperation (Tomasello and Call, 1997). Chalmeau (1994) expanded the methodology to an open-group design, allowing untrained individuals to spontaneously cooperate (or not). He found that one pair of individuals produced coordinated responses successfully solving the cooperative problem and retrieving food rewards, but that a dominant individual monopolised the apparatus, limiting the opportunities for successful collaboration. This paradigm was also used to demonstrate coordinated pulling in a pair of orangutans (Pongo pygmaeus) and group-housed tufted capuchins (Cebus apella) (Chalmeau et al., 1997a,b).
Cotton-top tamarins (Saguinus oedipus) were also found to successfully coordinate in this paradigm and appeared sensitive to the necessity of a partner (Cronin et al., 2005). However, they only did this after an extensive and multi-step training phase. Humans are known to coordinate spontaneously, thus studies that do not include such intensive training might be better comparisons to the nature of cooperative behaviour seen in humans. It may be that spontaneous cooperation is limited in non-human primates. For example, Hirata and Fuwa (2007) found that their chimpanzees only became successful at coordinated pulling after repeated trial and error. These observations, as with all laboratory-based experiments, do however come with the caveat that a lack of success in a controlled, lab-based task is not necessarily evidence of a complete lack of ability in a species. Lack of ecological validity is always an issue in such studies, just as lack of control raises questions about interpretations of field observations.
What controlled experiments have told us, in a clearer way than wild observations might, is that non-human primates do have some proficiency in purposeful joint action. They have allowed researchers to probe the cognitive mechanisms that might contribute to observations of joint action, in that monkeys and apes have been shown to coordinate their actions to achieve joint benefits. Studies into cooperative pulling, such as Chalmeau (1994) and Visalberghi et al. (2000), have demonstrated the ability of non-human primates to achieve joint goals—they are able to successfully act simultaneously to achieve a goal that is beneficial to both themselves and their partner. However, the cognitive underpinnings are still a matter of debate. In some cases, it was shown that the role of their partner was not fully understood (Chalmeau et al., 1997b; Visalberghi et al., 2000), and coordination was deemed to be incidental. Similarly, Petit et al. (1992) found in macaques that any seemingly coordinated action occurred likely by chance associations rather than purposeful synchrony or any recognition of roles. In other studies, however, the animals would wait for a partner before engaging in the task, indicating at least a rudimentary understanding of their role, and the interdependent nature of the task (Chalmeau et al., 1997a; Cronin et al., 2005; Melis et al., 2006b) and they would choose to work with the most effective partner (Melis et al., 2006a).
Cooperative pulling studies have also revealed potential motivational differences between humans and non-human primates in the context of cooperation. For example, using this paradigm Bullinger et al. (2011) and Rekers et al. (2011), demonstrated a collaborative bias in human children that is seemingly absent in chimpanzees, namely that children prefer to work together but chimpanzees do not, at least in contexts that could be argued to favour human subjects (Boesch, 2007). Experiments of this nature have demonstrated the motivational elements of cooperation; they also test for other cognitive abilities and limitations (Schmelz and Call, 2016). Chimpanzees, for example, do not appear to take the visual perspective of others in a task where they rely on a cooperative individual (Povinelli et al., 1996) yet they do appear to succeed in a competitive paradigm (Hare et al., 2000). Attempts to demonstrate false belief understanding (a component of theory of mind) in chimpanzees were unsuccessful (Call and Tomasello, 2008) until they were presented with a competitive scenario (Krupenye et al., 2016). Research and debate continue into how chimpanzees and other nonhuman primates coordinate, whether something like joint intentionality and perspective-taking are involved, as they appear to be in humans, or whether simpler cognitive solutions are used (Tomasello et al., 2005). Experiments continue to inform researchers of the role of cooperation and competition in the evolution of primate cognition (Hare and Tomasello, 2004).
Gaps in our knowledge
Despite their value, it is clear however that there is a limit to the usefulness of such experiments when it comes to thinking about the cognitive capacities required for large group collective behaviours in wild primates. Dyadic experiments are useful in showing us whether an individual is capable and sufficiently motivated to cooperate, as well as highlighting the cognitive mechanisms underlying these behaviours, however, they do not go very far in testing the limits of group-level cooperation. Cooperating with one other individual requires certain abilities (spatial and temporal coordination, social tolerance, motivation) but as the number of co-operators in a group increases we might expect these abilities to be stretched, and cooperation to become more challenging. In humans as groups increase in size individual members often become less productive in group tasks due to declines in both motivation and coordination (the Ringelmann effect; Ingham et al., 1974). However, this has not yet been tested in non-human primates, and so the effect of group size on cooperation in non-humans can only be speculated on.
For example, thus far, there is only one known study that experimentally tests cooperation in chimpanzees in groups larger than two (Suchak et al., 2014), wherein both duos and trios were tested in an apparatus-based food acquisition task. In this study successful coordination was achieved without training, success rates increased over time, and futile attempts (in the absence of a partner) decreased. The fact that groups larger than three have not been tested in a similar paradigm is surprising given that the most widely discussed instances of possible cooperation in wild chimpanzees, hunting and coalitionary attacks, involve the coordinated efforts of large groups. Thus, to further examine observational reports from the wild, and test whether and how chimpanzees and other primates are capable of coordinating as groups, there is a need for experimental studies beyond the dyad. Doing so will bridge the gap in our knowledge between ecological studies and lab-based cognition studies. The capabilities for joint action have been demonstrated but the limits have not been tested, and there are some very interesting questions yet to be answered. For example, are non-human primates able to enact and maintain coordinated action in large groups, or is there a threshold group size at which cooperation will break down and synchrony become unsustainable?
The relationship between group size and success in cooperative problem-solving has been more rigorously investigated in the avian literature. Morand-Ferron and Quinn (2011) examined the problem-solving performance of great and blue tits at automated devices and found that group innovation efficiency increased with flock size, validating a “pool of competence” hypothesis. Ashton et al. (2018) linked individual cognitive performance in magpies to group size, with those in large groups showing both increased performance in a “cognitive task battery” (measuring inhibitory control, associative learning, reversal learning, and spatial memory) and increased reproductive success. Perhaps most relevant to our questions is the finding that keas (Nestor notabilis) are capable of achieving cooperation in dyads, triads, and even tetrads in an experimental setting (Schwing et al., 2021). To our knowledge, this is the first such study that has tested instrumental cooperation in animal groups up to this size. A notable finding of this study was that success was dependent on the most dominant individual showing restraint and learning to stop monopolising the apparatus. An analogous ‘beyond the dyad’ cooperation study on non-human primates does not yet exist, and a clear deficit remains within our knowledge of primate group dynamics.
Such questions have been more fully addressed within the field of human organisational psychology, as described below. These studies have often focused on problem-solving tasks rather than large group collective decisions, yet, as with the kind of group coordination problems wild primates face, they often involve compromises between individual and group interests, as well as the pooling of differing inter-individual knowledge, and the generation and selection of solutions. Such studies have revealed that in humans group size may have strong effects on group productivity, efficiency, and accuracy of decision-making, as well as on individual effort, creativity and even free-riding.
Group size and composition effects on coordinated behaviours
As organisations and businesses are primarily concerned with optimising performance and efficiency, a common organisational question is “what is the optimal group size for group performance on a task.” There are no straightforward answers, however. As discussed, the effect of individuals on groups is not always additive, thus the effects of increasing group size on performance can depend on a number of things, and the relationship is rarely linear. Group size is however expected to play a crucial role in group dynamics and outcomes. This is in part because increasing group size will increase the number of relationships and result in different interactions.
It might perhaps be expected that the larger the number of people in a group, the more likely it will be to succeed, due to increases in productivity. However, this may depend very much on the type of task. For example, Rubin (1984) makes a distinction between disjunctive and additive tasks. A disjunctive task, like a maths problem or a riddle, has only one correct answer and so all that is needed is for one group member to know it. Thus, the larger the group, the more likely you are to receive that answer. With additive tasks also, like a tug of war or a brainstorming session, where group members perform parallel functions, the effect of individuals is combined, and so large groups should outperform small ones. However, in tasks where there is no one correct answer or way of doing things (discretionary tasks), like the decisions made by juries, the relationship between group size and performance is much less clear.
Insights from organisational psychology
Group size effects
A number of ‘tentative generalisations’ have been made by organisational psychologists, and trade-offs identified (Rubin, 1984). For example, due to the fewer interactions and moving parts, smaller groups are considered to be more efficient. This efficiency may however come at the cost of decreased input and a lack of diverging, and often enriching, points of view. Larger groups may make higher quality decisions and may be more productive than smaller groups, generating more ideas or products, a point we expand on below. As an extreme example, population size plays a role in cumulative cultural evolution (Henrich, 2004; Derex and Mesoudi, 2020).
These questions have been studied experimentally, with varying outcomes. Some studies have found a positive effect of increased group size on performance. A study on electronic brainstorming, wherein groups interact via computers to generate and share ideas, found that larger groups generated more unique ideas and higher quality ideas than smaller ones (Gallupe et al., 1992), and a study on civilian scientists and technicians who were assigned to isolated U.S. Antarctic stations for one year found that perceived group compatibility and achievement was significantly lower at small stations than large ones (Doll and Gunderson, 1971). A study on supervisors and workgroups at a large manufacturing plant (Cummins and King, 1973) also found that group size was positively related to both productivity and leader-member relations in structured tasks. The authors suggest that a supervisor not being able to pay direct attention to all group members might be beneficial for production, so conversely too much oversight could inhibit group work.
An investigation on group size effects on problem-solving in US Airforce cadets also found positive effects of group work and group size (Holloman and Hendrick, 1971). Participants were randomly allocated to groups of differing sizes (between three and 15). In groups of all sizes, consensus decisions were more accurate than the averaged decisions of individual group members, validating the positive effect of social interaction on decision-making. Larger groups also made more accurate decisions than the smallest, although this effect seemed to stagnate after a certain group size, with the accuracy of groups of six not being significantly different from that of 12 or 15.
In a more recent study, this stagnating effect was found by Laughlin et al. (2006) who gave individuals and groups of two, three, four, or five two letters-to-numbers problems to solve. The groups performed better than the best efforts of the individuals, aside from the groups of two, who were equivalent to the best individuals. The groups larger than two did not differ from each other. Three was just as good as five, suggesting that a group of three is the best and most efficient size for this task.
These findings demonstrate that the relationship between group size and performance is not always linear (see Figure 2). Manners (1975) investigated the effect of group size on problem-solving effectiveness and consensus decision-making. He found that although the mean problem-solving performance of groups was significantly better than that of individuals, the results showed a quadratic relationship, such that there was an optimal group size, after which effectiveness began to decline. In this case, the size of groups varied between two and 18, and groups of 11 were the most effective. Interestingly, the relationship between group size and consensus making was hyperbolic, such that consensus dropped sharply with the addition of new members at first, but the effect lessened at larger group sizes. This was taken to mean that although smaller groups find it easier to reach an agreement than larger ones, in very large groups opportunities for changing the opinions of others are limited.
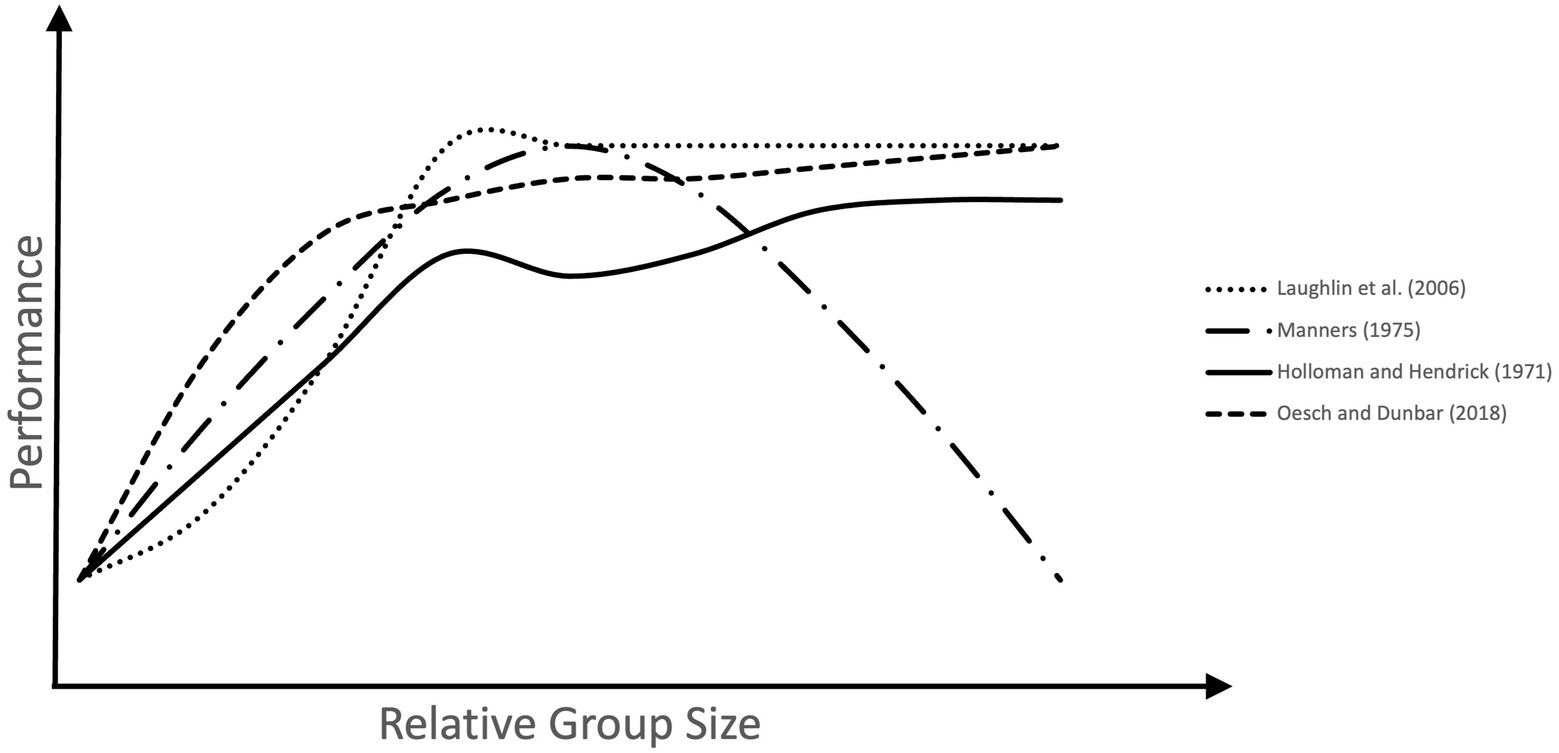
Figure 2. Group size effects on performance across several human studies. The measures of problem-solving performance used across these studies differed. These were, latency to solution (Laughlin et al., 2006), effectiveness (Manners, 1975), accuracy of decisions (Holloman and Hendrick, 1971), and foraging efficiency (Oesch and Dunbar, 2018). In all cases performance increases as small groups increase in size. However, in larger groups performance either decreases above an optimal size, or plateaus, with the benefit of more participants stagnating after a certain group size.
As predicted by social loafing theory (Ingham et al., 1974), group size can also have a negative effect on participation. Bass and Norton (1951) examined the effect of group size on member participation and leader emergence in initially leaderless groups. Average member participation declined as group size (between two and 12) increased, with groups of six found to be the most conducive to the emergence of leadership qualities. In a more recent study, social loafing was found to be more prevalent amongst members of larger subgroups working on an optimisation task (Meyer et al., 2016).
Other studies have found inhibiting effects of large groups on output. One such study by Bouchard and Hare (1970) investigated group size effects on brainstorming. Whether working in a group facilitates or inhibits creative thinking, innovation or problem-solving can be applied to animal groups as well as humans. This study measured the number of different solutions to a problem generated in groups of one, five, seven, and nine. They also compared ‘real’ groups in which individuals brainstormed together to ‘nominal’ groups composed of individuals who brainstormed alone and pooled efforts afterwards. They found that while overall the larger groups produced more ideas, the nominal groups were more effective than the real, indicating that the group-work approach inhibited idea generation. This perhaps unintuitive result suggests that in some cases pooled individual effort can be more productive than a group effort.
Group composition and inter-personal dimensions
As well as size, the structural characteristics of groups have also received attention. This includes spatial arrangement, as well as group make-up. Whereas the majority of the group size studies were done in the last century, the literature into group composition is relatively more modern, reflective in part of increased interest in the benefits of intra-group diversity. The decline in interest in group size dynamics (and group dynamics as a whole) is also thought to be symptomatic of a trend towards individualisation in workplaces (Schein, 2015).
Group diversity, in terms of gender, hierarchical status, and age is an aspect of group composition that has received particular attention. Choi (2007) investigated the impact of different demographic characteristics on creativity in employees of a Korean electronics company. They found that whilst differences in gender and hierarchical status decreased individual creativity, dissimilarities in age and performance level had the opposite effect suggesting that the effects of “diversity” as a concept cannot be generalised. Similarly, Wegge et al. (2008) found that age and gender had differential effects on group performance in federal tax offices. Age diversity correlated positively with group performance in groups solving complex decision-making tasks and increased gender diversity resulted in improved performance as compared to female-heavy teams. These effects of gender composition were most pronounced in large groups. As shown in the latter finding, group size and composition can interact. For example, Sidorenkov et al. (2018) found group size to be a moderator of inter-group conflict, such that increasing group size strengthened the influence of group characteristics, such as diversity and duration of membership, on conflict measures.
Finally, in addition to these composition factors, the interpersonal dimensions of groups have also been studied. Reddy and Byrnes (1972) found that groups that were more compatible in measures of control and affection (expressed and wanted) were able to complete a decision-making and problem-solving exercise more rapidly than less compatible groups.
Future directions
Collective action problems
Collective action problems are not as well studied within non-human primates as they have been in humans. The importance of institutions and formal leadership in non-human primate social dilemmas may be a productive avenue of research for example. Overall, the human literature has thus far dived deeper into the dynamics of group decision-making. Given that we know considerable dominance and power asymmetries exist within primate groups, the question of how shared decision-making processes are maintained warrants further investigation.
The relationship between group size, group composition, and cooperation
Organisational psychology experiments have revealed important insights into the dynamics of groups that have parallels in primatology. The relationship between group size and its outputs (such as efficiency and productivity) is rarely linear, and the direction of this relationship depends on the type of task. Some findings have been less intuitive, such as that group work may inhibit individual productivity and idea generation, with pooled individual efforts having better outcomes. Many tasks have optimal group sizes, after which problem-solving accuracy and individual motivation to participate and cooperate begin to stagnate or even decline. If similar questions were put to non-human primate groups, we may well expect to discover similar effects at work. This becomes interesting when we consider the impact such effects would have on limiting the cooperative capabilities of primates in the wild, within behaviours like group hunting for example, and even on limiting the group sizes of cooperative primate species.
For example, by drawing inspiration from the human organisation literature, researchers could design experiments on non-human primate groups that ask;
A. How does group size affect the productivity, efficiency, and accuracy of cooperative efforts and what is the shape of this relationship?
B. How does task type (additive versus disjunctive) affect the direction of the relationship between group size and output?
C. How are outputs like consensus decision-making and idea generation (i.e. solutions to problems) affected by group size?
D. Is there an optimal group size for coordination tasks?
E. What effect does group size have on individual participation?
F. What effect does group size have on leadership emergence?
G. What are the interactions between group size and group composition factors, such as age and gender makeup? Do these factors have a greater influence on output in larger or smaller groups?
H. What is the impact of group size and composition on inter-personal factors? Does conflict increase or decrease in larger groups?
I. How does group composition, in terms of age, sex and status impact cooperation in groups?
By reference to both the behavioural ecology and human literature, tentative predictions can be made. We may find for example that with increasing group size problem-solving success becomes more likely, as the number of differently skilled individuals, the so-called “pool of competence,” is increased. Conversely, we should also expect that as group size, and consequently, the number of interactions and relationships, is increased, group coordination, synchrony, and decision-making should become more challenging. This could be investigated through both wild and lab-based field studies in which groups of varying sizes are given extractive foraging problems that require group coordination (for example coordinated action and patch choice and movement tasks), and such effects could be evidenced by greater latencies to make decisions (i.e. when and how to act), more inaccurate or sub-optimal decisions, or a decrease in efficiency and productivity in tasks. A version of the Ringelmann effect would be expected, in which declines in individual motivation and coordination affect performance in cooperative tasks. We would also expect there to be an optimal group size for many tasks, which may well be larger than dyads that have so far been the focus of such experiments, but would also have an upper limit, after which performance declines. Effects like social loafing would be expected to be found in larger groups, wherein the individual motivation to participate and to take on a leadership role decreases with increasing group size, and often more sparsely distributed rewards. Finding such effects would tell us more about non-human primate cooperative motivations, as well as the strength of social norms and enforcement mechanisms in maintaining participation. Declines in coordination would also inform us about the limits of primate synchronisation abilities (joint action), as well as “we thinking” (joint intentionality). Results from such experimental group studies could then be used to make more informed predictions about what cooperative group behaviours we might expect to find in the wild and at what group sizes.
We would of course expect these socio-cognitive factors to work in concert with the ecological ones discussed, both mediating group size in natural groups. For example, the size of a group may be limited by factors such as the challenges of coordination, as well as the pressures of between-group competition.
Discussion
There are parallels to be drawn between the very different fields of behavioural ecology and human organisational psychology. In both group-level behaviours are studied and limits discussed and in both cognition is often assumed or implied. Organisational psychology, like behavioural ecology, typically does not consider the cognitive processes underlying behaviour but instead focuses just on the behaviour itself. Comparative psychologists on the other hand rely on experiments to infer the cognition of individuals but are often guilty of considering the effects of individual mental processes on behaviour at the expense of all else, including real-world social and ecological pressures. There then exists a need for an integration of behavioural ecology and comparative psychology, to better understand the relationships between behaviour and selective pressures.
In this review, we have identified an arena for such integration, that of group collaborative behaviours in non-human primates. Currently what we know about the cognition of groups comes from dyads, and what we know of group dynamics comes from large wild groups. This knowledge deficit should be filled by experimental studies on groups of varying size which explicitly investigate the cognition underlying joint action, leadership roles, optimisation problems and motivation.
What our brief review of the primate cooperation literature has shown is that experimental work is based on just a few experimental paradigms, primarily string-pulling, which, although useful in demonstrating certain abilities, have limited ecological validity, especially given dyadic cooperation is not the norm. Rather, instead of problem-solving, what wild primates do is make large-group collective decisions—about where to go and when—many times a day. Gaps in our knowledge of what properties of groups might influence these processes, and how they are maintained in the face of conflicts of interest persist. There appears therefore to be a need for an expansion of more naturalistic experimental studies which investigate more relevant behaviours, including patch foraging and group hunting. We suggest that controlled experiments in a wild setting on natural groups might be the best way to investigate these behaviours, as it would combine ecological validity with scientific rigour, as well as allow the opportunity to study groups whose size has not been directly dictated by human intervention. Such an approach has been used by Van de Waal et al. (2014) for example, to study social learning in vervet monkeys. However, although controlled experiments in a natural setting would be the best-case scenario, the proposed questions could also be investigated in captive settings. Although often artificial size and composition, groups in zoos have frequent variation in size, both within zoos and between due to transfers, and offer the practical and scientific benefits of a more easily controlled environment. Cowl et al. (2020) for example, investigated how crested macaques responded to disturbances of the group composition when a zoo manipulated the group size. Naturalistic foraging tasks have also been conducted in zoo settings, such as Ozturk et al. (2021) who investigated food-finding in mandrills. Additionally, in order to satisfactorily investigate group size effects, particularly in the wild where group size is less easily manipulated, it would also be necessary to compare many different groups. This would require a large collaborative effort, but initiatives such as Many Primates (Primates et al., 2019), which was founded to facilitate collaboration across numerous study sites in primate cognition research, can serve as a model.
A review of the human organisational literature has given us potential research questions and approaches, as well as indications of what such studies might find. This includes a non-linear relationship between group size and performance indicators, such that for some collaborative tasks optimal group sizes should exist, beyond which cognitive capacity and motivation become stretched. When translated to group behaviours in the wild, such as making coordinated movement decisions when foraging, and engaging in group hunts, such findings could be very revealing.
Drawing inspiration from the human literature and investigating the effects of group size and composition on group performance may help to bridge the gaps that we have identified between what is known about groups and individuals, allowing us to gain a better understanding of how non-human primates navigate the challenges of sociality.
Author contributions
LW: writing—original draft preparation, conceptualization, and data curation. SS: supervision, writing—review and editing, and conceptualization. KJ: supervision, writing—review and editing, conceptualization, and data curation. All authors contributed to the article and approved the submitted version.
Funding
This research was supported by a BBSRC-DTP studentship grant, code: P118814 CW07 and Royal Society (The Royal Society): Susanne Shultz UF110641.
Conflict of interest
The authors declare that the research was conducted in the absence of any commercial or financial relationships that could be construed as a potential conflict of interest.
Publisher’s note
All claims expressed in this article are solely those of the authors and do not necessarily represent those of their affiliated organizations, or those of the publisher, the editors and the reviewers. Any product that may be evaluated in this article, or claim that may be made by its manufacturer, is not guaranteed or endorsed by the publisher.
References
Alberts, N. (2013). Fission fusion dynamics of olive baboons (Papio anubis) in Gashaka-Gumti National Park. unpublished doctoral thesis. London, England: University of Roehampton.
Amici, F., Aureli, F., and Call, J. (2008). Fission-fusion dynamics, behavioral flexibility, and inhibitory control in primates. Curr. Biol. 18, 1415–1419. doi: 10.1016/j.cub.2008.08.020
Asensio, N., Aureli, F., Schaffner, C., and Korstjens, A. (2008). Intragroup aggression, fission–fusion dynamics and feeding competition in spider monkeys. Behaviour 145, 983–1001. doi: 10.1163/156853908784089234
Ashton, B. J., Ridley, A. R., Edwards, E. K., and Thornton, A. (2018). Cognitive performance is linked to group size and affects fitness in Australian magpies. Nature 554, 364–367. doi: 10.1038/nature25503
Aureli, F., Schaffner, C. M., Boesch, C., Bearder, S. K., Call, J., Chapman, C. A., et al. (2008). Fission-fusion dynamics: new research frameworks. Curr. Anthropol. 49, 627–654. doi: 10.1086/586708
Bass, B., and Norton, F. (1951). Group size and leaderless discussions. J. Appl. Psychol. 35, 397–400. doi: 10.1037/h0055478
Bazazi, S., Buhl, J., Hale, J. J., Anstey, M. L., Sword, G. A., Simpson, S. J., et al. (2008). Collective motion and cannibalism in locust migratory bands. Curr. Biol. 18, 735–739. doi: 10.1016/j.cub.2008.04.035
Boesch, C. (1994). Cooperative hunting in wild chimpanzees. Anim. Behav. 48, 653–667. doi: 10.1006/anbe.1994.1285
Boesch, C. (2007). What makes us human (Homo sapiens)? The challenge of cognitive cross-species comparison. J. Comp. Psychol. 121, 227–240. doi: 10.1037/0735-7036.121.3.227
Boinski, S., Treves, A., and Chapman, C. A. (2000). “A critical evaluation of the influence of predators on primates: effects on group travel,” in On the Move: How and Why Animals Travel in Groups. eds. S. Boinski and P. A. Garber (Chicago, IL: University of Chicago Press), 43–72.
Bouchard, T. J., and Hare, M. (1970). Size, performance, and potential in brainstorming groups. J. Appl. Psychol. 54, 51–55. doi: 10.1037/h0028621
Bshary, R., and Bergmüller, R. (2008). Distinguishing four fundamental approaches to the evolution of helping. J. Evol. Biol. 21, 405–420. doi: 10.1111/j.1420-9101.2007.01482.x
Bullinger, A. F., Melis, A. P., and Tomasello, M. (2011). Chimpanzees, Pan troglodytes, prefer individual over collaborative strategies towards goals. Anim. Behav. 82, 1135–1141. doi: 10.1016/j.anbehav.2011.08.008
Burkart, J. M., and van Schaik, C. P. (2010). Cognitive consequences of cooperative breeding in primates? Anim. Cogn. 13, 1–19. doi: 10.1007/s10071-009-0263-7
Burkart, J. M., and van Schaik, C. P. (2016). Revisiting the consequences of cooperative breeding. J. Zool. 299, 77–83. doi: 10.1111/jzo.12322
Call, J., and Tomasello, M. (2008). Does the chimpanzee have a theory of mind? 30 years later. Trends Cogn. Sci. 12, 187–192. doi: 10.1016/j.tics.2008.02.010
Chalmeau, R. (1994). Do chimpanzees cooperate in a learning task? Primates 35, 385–392. doi: 10.1007/bf02382735
Chalmeau, R., and Gallo, A. (1995). Cooperation in primates: critical analysis of behavioural criteria. Behav. Process. 35, 101–111. doi: 10.1016/0376-6357(95)00049-6
Chalmeau, R., Lardeux, K., Brandibas, P., and Gallo, A. (1997a). Cooperative problem solving by orangutans (Pongo pygmaeus). Int. J. Primatol. 18, 23–32. doi: 10.1023/a:1026337006136
Chalmeau, R., Visalberghi, E., and Gallo, A. (1997b). Capuchin monkeys (Cebus apella) fail to understand a cooperative task. Anim. Behav. 54, 1215–1225. doi: 10.1006/anbe.1997.0517
Chapman, C. A., Chapman, L. J., and Wrangham, R. (1995). Ecological constraints on group size: an analysis of spider monkey and chimpanzee subgroups. Behav. Ecol. Sociobiol. 36, 59–70. doi: 10.1007/BF00175729
Cheney, D. L., and Seyfarth, R. M. (1987). The influence of intergroup competition on the survival and reproduction of female vervet monkeys. Behav. Ecol. Sociobiol. 21, 375–386. doi: 10.1007/BF00299932
Choi, J. N. (2007). Group composition and employee creative behaviour in a Korean electronics company: distinct effects of relational demography and group diversity. J. Occup. Organ. Psychol. 80, 213–234. doi: 10.1348/096317906x110250
Conradt, L., and Roper, T. J. (2000). Activity synchrony and social cohesion: a fission-fusion model. Proc. R. Soc. Lond. Ser. B Biol. Sci. 267, 2213–2218. doi: 10.1098/rspb.2000.1271
Conradt, L., and Roper, T. J. (2005). Consensus decision making in animals. Trends Ecol. Evol. 20, 449–456. doi: 10.1016/j.tree.2005.05.008
Couzin, I. D., Krause, J., Franks, N. R., and Levin, S. A. (2005). Effective leadership and decision-making in animal groups on the move. Nature 433, 513–516. doi: 10.1038/nature03236
Cowl, V. B., Jensen, K., Lea, J. M. D., Walker, S. L., and Shultz, S. (2020). Sulawesi crested macaque (Macaca nigra) grooming networks are robust to perturbation while individual associations are more labile. Int. J. Primatol. 41, 105–128. doi: 10.1007/s10764-020-00139-6
Crawford, M. P. (1937). The Cooperative Solving Of Problems By Young Chimpanzees. Volume 14 of Comparative Psychology Monographs. Baltimore: Kraus.
Crofoot, M. C., Gilby, I. C., Wikelski, M. C., and Kays, R. W. (2008). Interaction location outweighs the competitive advantage of numerical superiority in Cebus capucinus intergroup contests. Proc. Natl. Acad. Sci. 105, 577–581. doi: 10.1073/pnas.0707749105
Cronin, K. A., Kurian, A. V., and Snowdon, C. T. (2005). Cooperative problem solving in a cooperatively breeding primate (Saguinus oedipus). Anim. Behav. 69, 133–142. doi: 10.1016/j.anbehav.2004.02.024
Cummins, R. C., and King, D. C. (1973). Interaction of group size and task structure in an industrial organization. Pers. Psychol. 26, 87–94. doi: 10.1111/j.1744-6570.1973.tb01120.x
Davis, G. H., Crofoot, M. C., and Farine, D. R. (2022). Using optimal foraging theory to infer how groups make collective decisions. Trends Ecol. Evol. 37, 942–952. doi: 10.1016/j.tree.2022.06.010
Derex, M., and Mesoudi, A. (2020). Cumulative cultural evolution within evolving population structures. Trends Cogn. Sci. 24, 654–667. doi: 10.1016/j.tics.2020.04.005
Doll, R. E., and Gunderson, E. K. (1971). Influence of group size on perceived compatibility and achievement in an extreme environment. Pers. Psychol. 24, 305–310. doi: 10.1111/j.1744-6570.1971.tb02478.x
dos Santos, M., Rankin, D. J., and Wedekind, C. (2013). Human cooperation based on punishment reputation. Evolution 67, 2446–2450. doi: 10.1111/evo.12108
Duguid, S., and Melis, A. P. (2020). How animals collaborate: underlying proximate mechanisms. Wiley Interdiscip. Rev. Cogn. Sci. 11:e1529. doi: 10.1002/wcs.1529
Dunbar, R. I. M., and Shultz, S. (2022). Socioecological complexity in primate groups and its cognitive correlates. Philos. Trans. R. Soc. B Biol. Sci. 377:20210296. doi: 10.1098/rstb.2021.0296
Dyer, J. R. G., Johansson, A., Helbing, D., Couzin, I. D., and Krause, J. (2009). Leadership, consensus decision making and collective behaviour in humans. Philos. Trans. R. Soc. B Biol. Sci. 364, 781–789. doi: 10.1098/rstb.2008.0233
Faria, J. J., Krause, S., and Krause, J. (2010). Collective behavior in road crossing pedestrians: the role of social information. Behav. Ecol. 21, 1236–1242. doi: 10.1093/beheco/arq141
Fehr, E., and Fischbacher, U. (2004). Social norms and human cooperation. Trends Cogn. Sci. 8, 185–190. doi: 10.1016/j.tics.2004.02.007
Fehr, E., Herz, H., and Wilkening, T. (2013). The lure of authority: motivation and incentive effects of power. Am. Econ. Rev. 103, 1325–1359. doi: 10.1257/aer.103.4.1325
Fichtel, C., and Kappeler, P. M. (2002). Anti-predator behavior of group-living Malagasy primates: mixed evidence for a referential alarm call system. Behav. Ecol. Sociobiol. 51, 262–275. doi: 10.1007/s00265-001-0436-0
Fischer, J., and Zinner, D. (2011). Communication and cognition in primate group movement. Int. J. Primatol. 32, 1279–1295. doi: 10.1007/s10764-011-9542-7
Foster, W. A., and Treherne, J. E. (1981). Evidence for the dilution effect in the selfish herd from fish predation on a marine insect. Nature 293, 466–467. doi: 10.1038/293466a0
Fretwell, S. D. (1969). On territorial behavior and other factors influencing habitat distribution in birds. Acta Biotheor. 19, 16–36. doi: 10.1007/BF01601953
Gallupe, R. B., Dennis, A. R., Cooper, W. H., Valacich, J. S., Bastianutti, L. M., and Nunamaker, J. F. (1992). Electronic brainstorming and group-size. Acad. Manag. J. 35, 350–369. doi: 10.2307/256377
Garber, P. A., Bicca-Marques, J. C., and Azevedo-Lopes, M. A. D. O. (2009). “Primate cognition: integrating social and ecological information in decision-making,” in South American Primates. eds. P. A. Garber, A. Estrada, J. C. Bicca-Marques, E. W. Heymann, and K. B. Strier (New York, NY: Springer), 365–385.
Glowacki, L., and von Rueden, C. (2015). Leadership solves collective action problems in small-scale societies. Philos. Trans. R. Soc. B Biol. Sci. 370:20150010. doi: 10.1098/rstb.2015.0010
Goldstone, R., Griffiths, T., Gureckis, T., Helbing, D., and Steels, L. (2009). "The emergence of collective structures through individual interactions", in: Proceedings of the 31th Annual Conference of the Cognitive Science Society.
Goodall, J. (1986). The Chimpanzees of Gombe: Patterns of Behavior. Cambridge, MA: The Belknap Press of Harvard University Press.
Green, S. J., Boruff, B. J., Bonnell, T. R., and Grueter, C. C. (2020). Chimpanzees use least-cost routes to out-of-sight goals. Curr. Biol. 30, 4528.e5–4533.e5. doi: 10.1016/j.cub.2020.08.076
Grueter, C. C., Robbins, A. M., Abavandimwe, D., Vecellio, V., Ndagijimana, F., Stoinski, T. S., et al. (2018). Quadratic relationships between group size and foraging efficiency in a herbivorous primate. Sci. Rep. 8, 16718–16711. doi: 10.1038/s41598-018-35255-0
Guazzini, A., Imbimbo, E., Stefanelli, F., and Bravi, G. (2019). “The online bystander effect: evidence from a study on synchronous Facebook communications,” in Internet Science. INSCI 2019. Lecture Notes in Computer Science. eds. S. El Yacoubi, F. Bagnoli, and G. Pacini (Cham: Springer International Publishing).
Gureckis, T. M., and Goldstone, R. L. (2006). Thinking in groups. Pragmat. Cogn. 14, 293–311. doi: 10.1075/pc.14.2.10gur
Hare, B., Call, J., Agnetta, B., and Tomasello, M. (2000). Chimpanzees know what conspecifics do and do not see. Anim. Behav. 59, 771–785. doi: 10.1006/anbe.1999.1377
Hare, B., and Tomasello, M. (2004). Chimpanzees are more skilful in competitive than in cooperative cognitive tasks. Anim. Behav. 68, 571–581. doi: 10.1016/j.anbehav.2003.11.011
Hemelrijk, C. K., and Hildenbrandt, H. (2015). Diffusion and topological neighbours in flocks of starlings: relating a model to empirical data. PLoS One 10:e0126913. doi: 10.1371/journal.pone.0126913
Henrich, J. (2004). Demography and cultural evolution: how adaptive cultural processes can produce maladaptive losses—the Tasmanian case. Am. Antiq. 69, 197–214. doi: 10.2307/4128416
Hirata, S., and Fuwa, K. (2007). Chimpanzees (Pan troglodytes) learn to act with other individuals in a cooperative task. Primates 48, 13–21. doi: 10.1007/s10329-006-0022-1
Holloman, C. R., and Hendrick, H. W. (1971). Problem solving in different sized groups. Pers. Psychol. 24, 489–500. doi: 10.1111/j.1744-6570.1971.tb00372.x
Ingham, A. G., Levinger, G., Graves, J., and Peckham, V. (1974). The Ringelmann effect: studies of group size and group performance. J. Exp. Soc. Psychol. 10, 371–384. doi: 10.1016/0022-1031(74)90033-X
Isbell, L. A. (1991). Contest and scramble competition: patterns of female aggression and ranging behavior among primates. Behav. Ecol. 2, 143–155. doi: 10.1093/beheco/2.2.143
Jacobs, I. F., and Osvath, M. (2015). The string-pulling paradigm in comparative psychology. J. Comp. Psychol. 129, 89–120. doi: 10.1037/a0038746
Janmaat, K. R. L., Polansky, L., Ban, S. D., and Boesch, C. (2014). Wild chimpanzees plan their breakfast time, type, and location. Proc. Natl. Acad. Sci. 111, 16343–16348. doi: 10.1073/pnas.1407524111
Janson, C. (1985). Aggressive competition and individual food consumption in wild brown capuchin monkeys (Cebus apella). Behav. Ecol. Sociobiol. 18, 125–138. doi: 10.1007/BF00299041
Janson, C. H. (2016). Capuchins, space, time and memory: an experimental test of what-where-when memory in wild monkeys. Proc. Royal Soc. B 283:20161432. doi: 10.1098/rspb.2016.1432
Janson, C. H., and Van Schaik, C. P. (1988). Recognizing the many faces of primate food competition: methods. Behaviour 105, 165–186. doi: 10.1163/156853988X00502
Jensen, K. (2016b). “The prosocial primate: a critical review,” in Advances in the Study of Behavior. eds. J. C. M. M. Naguib, L. W. Simmons, L. Barrett, S. Healy, and M. Zuk (New York, NY: Academic Press), 387–441.
Judge, T. A., and Bono, J. E. (2000). Five-factor model of personality and transformational leadership. J. Appl. Psychol. 85, 751–765. doi: 10.1037/0021-9010.85.5.751
King, A. J., and Cowlishaw, G. (2009). All together now: behavioural synchrony in baboons. Anim. Behav. 78, 1381–1387. doi: 10.1016/j.anbehav.2009.09.009
King, A. J., Johnson, D. D. P., and Van Vugt, M. (2009). The origins and evolution of leadership. Curr. Biol. 19, R911–R916. doi: 10.1016/j.cub.2009.07.027
King, A. J., and Sueur, C. (2011). Where next? Group coordination and collective decision making by primates. Int. J. Primatol. 32, 1245–1267. doi: 10.1007/s10764-011-9526-7
Koenig, A. (2002). Competition for resources and its behavioral consequences among female primates. Int. J. Primatol. 23, 759–783. doi: 10.1023/A:1015524931226
Koenig, A., and Rothe, H. (1991). Social relationships and individual contribution to cooperative behaviour in captive common marmosets (Callithrix jacchus). Primates 32, 183–195. doi: 10.1007/BF02381175
Krupenye, C., Kano, F., Hirata, S., Call, J., and Tomasello, M. (2016). Great apes anticipate that other individuals will act according to false beliefs. Science 354, 110–114. doi: 10.1126/science.aaf8110
Kugler, T., Kausel, E. E., and Kocher, M. G. (2012). Are groups more rational than individuals? A review of interactive decision making in groups. Wiley Interdiscip. Rev. Cogn. Sci. 3, 471–482. doi: 10.1002/wcs.1184
Laughlin, P. R., Hatch, E. C., Silver, J. S., and Boh, L. (2006). Groups perform better than the best individuals on letters-to-numbers problems: effects of group size. J. Pers. Soc. Psychol. 90, 644–651. doi: 10.1037/0022-3514.90.4.644
Lombardi, M., Warren, W. H., and di Bernardo, M. (2020). Nonverbal leadership emergence in walking groups. Sci. Rep. 10, 18948–18910. doi: 10.1038/s41598-020-75551-2
Majolo, B., de Bortoli Vizioli, A., and Schino, G. (2008). Costs and benefits of group living in primates: group size effects on behaviour and demography. Anim. Behav. 76, 1235–1247. doi: 10.1016/j.anbehav.2008.06.008
Majolo, B., and Huang, P. (2018). “Group living,” in Encyclopedia of Animal Cognition and Behavior. eds. J. Vonk and T. K. Shackelford (Cham: Springer), 1–12.
Manners, G. E. (1975). Another look at group size, group problem-solving, and member consensus. Acad. Manag. J. 18, 715–724. doi: 10.2307/255374
Martin, J. S., Koski, S. E., Bugnyar, T., Jaeggi, A. V., and Massen, J. J. M. (2021). Prosociality, social tolerance and partner choice facilitate mutually beneficial cooperation in common marmosets, Callithrix jacchus. Anim. Behav. 173, 115–136. doi: 10.1016/j.anbehav.2020.12.016
Melis, A. P., Hare, B., and Tomasello, M. (2006a). Chimpanzees recruit the best collaborators. Science 311, 1297–1300. doi: 10.1126/science.1123007
Melis, A. P., Hare, B., and Tomasello, M. (2006b). Engineering cooperation in chimpanzees: tolerance constraints on cooperation. Anim. Behav. 72, 275–286. doi: 10.1016/j.anbehav.2005.09.018
Meunier, H., Leca, J., Deneubourg, J., and Petit, O. (2006). Group movement decisions in capuchin monkeys: the utility of an experimental study and a mathematical model to explore the relationship between individual and collective behaviours. Behaviour 143, 1511–1527. doi: 10.1163/156853906779366982
Meyer, B., Schermuly, C. C., and Kauffeld, S. (2016). That's not my place: the interacting effects of faultlines, subgroup size, and social competence on social loafing behaviour in work groups. Eur. J. Work Organ. Psy. 25, 31–49. doi: 10.1080/1359432x.2014.996554
Miner, F. C.Jr. (1984). Group versus individual decision making: an investigation of performance measures, decision strategies, and process losses/gains. Organ. Behav. Hum. Perform. 33, 112–124. doi: 10.1016/0030-5073(84)90014-X
Mitani, J. C., Watts, D. P., and Amsler, S. J. (2010). Lethal intergroup aggression leads to territorial expansion in wild chimpanzees. Curr. Biol. 20, R507–R508. doi: 10.1016/j.cub.2010.04.021
Morand-Ferron, J., and Quinn, J. L. (2011). Larger groups of passerines are more efficient problem solvers in the wild. Proc. Natl. Acad. Sci. 108, 15898–15903. doi: 10.1073/pnas.1111560108
Musgrave, S., Morgan, D., Lonsdorf, E., Mundry, R., and Sanz, C. (2016). Tool transfers are a form of teaching among chimpanzees. Sci. Rep. 6, 1–7. doi: 10.1038/srep34783
Newton-Fisher, N. E. (2006). Female coalitions against male aggression in wild chimpanzees of the Budongo Forest. Int. J. Primatol. 27, 1589–1599. doi: 10.1007/s10764-006-9087-3
Nishida, T., and Hosaka, K. (1996). “Coalition strategies among adult male chimpanzees of the Mahale Mountains, Tanzania,” in Great Ape Societies. eds. W. C. McGrew, L. F. Marchant, and T. Nishida (Cambridge: Cambridge University Press), 114–134.
Nosenzo, D., Quercia, S., and Sefton, M. (2015). Cooperation in small groups: the effect of group size. Exp. Econ. 18, 4–14. doi: 10.1007/s10683-013-9382-8
Oesch, N., and Dunbar, R. I. M. (2018). Group size, communication, and familiarity effects in foraging human teams. Ethology 124, 483–495. doi: 10.1111/eth.12756
Ozturk, K. C. D., Egas, M., and Janmaat, K. R. L. (2021). Mandrills learn two-day time intervals in a naturalistic foraging situation. Anim. Cogn. 24, 569–582. doi: 10.1007/s10071-020-01451-7
Petit, O., and Bon, R. (2010). Decision-making processes: the case of collective movements. Behav. Process. 84, 635–647. doi: 10.1016/j.beproc.2010.04.009
Petit, O., Desportes, C., and Thierry, B. (1992). Differential probability of “coproduction” in two species of macaque (Macaca tonkeana, M. mulatta). Ethology 90, 107–120. doi: 10.1111/j.1439-0310.1992.tb00825.x
Povinelli, D. J., Eddy, T. J., Hobson, R. P., and Tomasello, M. (1996). What young chimpanzees know about seeing. Monogr. Soc. Res. Child Dev. 61, i–189. doi: 10.2307/1166159
Primates, M., Altschul, D. M., Beran, M. J., Bohn, M., Call, J., DeTroy, S., et al. (2019). Establishing an infrastructure for collaboration in primate cognition research. PLoS One 14:e0223675. doi: 10.1371/journal.pone.0223675
Rapaport, L. G., and Brown, G. R. (2008). Social influences on foraging behavior in young nonhuman primates: learning what, where, and how to eat. Evol. Anthropol. Issues News Rev. 17, 189–201. doi: 10.1002/evan.20180
Reddy, W. B., and Byrnes, A. (1972). Effects of interpersonal group composition on problem-solving behavior of middle managers. J. Appl. Psychol. 56, 516–517. doi: 10.1037/h0033755
Rekers, Y., Haun, D. B. M., and Tomasello, M. (2011). Children, but not chimpanzees, prefer to collaborate. Curr. Biol. 21, 1756–1758. doi: 10.1016/j.cub.2011.08.066
Rubin, G. (1984). “Introduction,” in Group Decision Making. ed. W. C. Swap (Beverly Hills: Sage), 21.
Saito, C. (1996). Dominance and feeding success in female Japanese macaques, Macaca fuscata: effects of food patch size and inter-patch distance. Anim. Behav. 51, 967–980. doi: 10.1006/anbe.1996.0100
Samuni, L., Crockford, C., and Wittig, R. M. (2021). Group-level cooperation in chimpanzees is shaped by strong social ties. Nat. Commun. 12:539. doi: 10.1038/s41467-020-20709-9
Samuni, L., Preis, A., Deschner, T., Crockford, C., and Wittig, R. M. (2018). Reward of labor coordination and hunting success in wild chimpanzees. Commun. Biol. 1:138. doi: 10.1038/s42003-018-0142-3
Sapolsky, R. M. (2005). The influence of social hierarchy on primate health. Science 308, 648–652. doi: 10.1126/science.1106477
Schein, E. H. (2015). Organizational psychology then and now: some observations. Annu. Rev. Organ. Psych. Organ. Behav. 2, 1–19. doi: 10.1146/annurev-orgpsych-032414-111449
Schmelz, M., and Call, J. (2016). The psychology of primate cooperation and competition: a call for realigning research agendas. Philos. Trans. R. Soc. B Biol. Sci. 371:20150067. doi: 10.1098/rstb.2015.0067
Schwing, R., Meaux, E., Piseddu, A., Huber, L., and Noë, R. (2021). Kea, Nestor notabilis, achieve cooperation in dyads, triads, and tetrads when dominants show restraint. Learn. Behav. 49, 36–53. doi: 10.3758/s13420-021-00462-9
Shahhoseini, Z., and Sarvi, M. (2017). Collective movements of pedestrians: how we can learn from simple experiments with non-human (ant) crowds. PLoS One 12:e0182913. doi: 10.1371/journal.pone.0182913
Sidorenkov, A. V., Borokhovski, E. F., and Kovalenko, V. A. (2018). Group size and composition of work groups as precursors of intragroup conflicts. Psychol. Res. Behav. Manag. 11, 511–523. doi: 10.2147/PRBM.S178761
Silk, J. B. (2005). “The evolution of cooperation in primate groups,” in Moral Sentiments and Material Interests: The Foundations of Cooperation in Economic Life. eds. H. Gintis, S. Bowles, R. Boyd, and E. Fehr (Cambridge, MA: MIT Press), 43–73.
Smith, J. E., Van Horn, R. C., Powning, K. S., Cole, A. R., Graham, K. E., Memenis, S. K., et al. (2010). Evolutionary forces favoring intragroup coalitions among spotted hyenas and other animals. Behav. Ecol. 21, 284–303. doi: 10.1093/beheco/arp181
Sterck, E. H. M., Watts, D. P., and Van Schaik, C. P. (1997). The evolution of female social relationships in nonhuman primates. Behav. Ecol. Sociobiol. 41, 291–309. doi: 10.1007/s002650050390
Stocker, M., Loretto, M.-C., Sterck, E. H. M., Bugnyar, T., and Massen, J. J. M. (2020). Cooperation with closely bonded individuals reduces cortisol levels in long-tailed macaques. R. Soc. Open Sci. 7:191056. doi: 10.1098/rsos.191056
Strandburg-Peshkin, A., Farine, D. R., Couzin, I. D., and Crofoot, M. C. (2015). Shared decision-making drives collective movement in wild baboons. Science 348, 1358–1361. doi: 10.1126/science.aaa5099
Stueckle, S., and Zinner, D. (2008). To follow or not to follow: decision making and leadership during the morning departure in chacma baboons. Anim. Behav. 75, 1995–2004. doi: 10.1016/j.anbehav.2007.12.012
Suchak, M., Eppley, T. M., Campbell, M. W., and de Waal, F. B. M. (2014). Ape duos and trios: spontaneous cooperation with free partner choice in chimpanzees. PeerJ 2:e417. doi: 10.7717/peerj.417
Suchak, M., Eppley, T. M., Campbell, M. W., Feldman, R. A., Quarles, L. F., and de Waal, F. B. (2016). How chimpanzees cooperate in a competitive world. Proc. Natl. Acad. Sci. 113, 10215–10220. doi: 10.1073/pnas.1611826113
Sueur, C., Deneubourg, J., and Petit, O. (2010). Sequence of quorums during collective decision making in macaques. Behav. Ecol. Sociobiol. 64, 1875–1885. doi: 10.1007/s00265-010-0999-8
Sueur, C., King, A. J., Conradt, L., Kerth, G., Lusseau, D., Mettke-Hofmann, C., et al. (2011). Collective decision-making and fission–fusion dynamics: a conceptual framework. Oikos 120, 1608–1617. doi: 10.1111/j.1600-0706.2011.19685.x
Sussman, R. W., Garber, P. A., and Cheverud, J. M. (2005). Importance of cooperation and affiliation in the evolution of primate sociality. Am. J. Phys. Anthropol. 128, 84–97. doi: 10.1002/ajpa.20196
Symington, M. M. (1990). Fission-fusion social organization in Ateles and Pan. Int. J. Primatol. 11, 47–61. doi: 10.1007/BF02193695
Theiner, G., Allen, C., and Goldstone, R. L. (2010). Recognizing group cognition. Cogn. Syst. Res. 11, 378–395. doi: 10.1016/j.cogsys.2010.07.002
Thierry, B. (2000). “Covariation of conflict management patterns across macaque species,” in Natural Conflict Resolution. eds. F. Aureli and F. B. M. D. Waal (Berkeley, CA: University of California Press), 106–128.
Thornton, A., and McAuliffe, K. (2015). Cognitive consequences of cooperative breeding? A critical appraisal. J. Zool. 295, 12–22. doi: 10.1111/jzo.12198
Tomasello, M., Carpenter, M., Call, J., Behne, T., and Moll, H. (2005). Understanding and sharing intentions: the origins of cultural cognition. Behav. Brain Sci. 28, 675–691. doi: 10.1017/S0140525X05000129
Van de Waal, E., Bshary, R., and Whiten, A. (2014). Wild vervet monkey infants acquire the food-processing variants of their mothers. Anim. Behav. 90, 41–45. doi: 10.1016/j.anbehav.2014.01.015
van Schaik, C. P. (1983). Why are diurnal primates living in groups? Behaviour 87, 120–144. doi: 10.1163/156853983X00147
Van Vugt, M. (2006). Evolutionary origins of leadership and followership. Personal. Soc. Psychol. Rev. 10, 354–371. doi: 10.1207/s15327957pspr1004_5
Venkataraman, V. V., Kraft, T. S., Dominy, N. J., and Endicott, K. M. (2017). Hunter-gatherer residential mobility and the marginal value of rainforest patches. Proc. Natl. Acad. Sci. 114, 3097–3102. doi: 10.1073/pnas.161754211
Visalberghi, E., Quarantotti, B. P., and Tranchida, F. (2000). Solving a cooperation task without taking into account the partner's behavior: the case of capuchin monkeys (Cebus apella). J. Comp. Psychol. 114, 297–301. doi: 10.1037/0735-7036.114.3.297
Vollrath, D. A., Sheppard, B. H., Hinsz, V. B., and Davis, J. H. (1989). Memory performance by decision-making groups and individuals. Organ. Behav. Hum. Decis. Process. 43, 289–300. doi: 10.1016/0749-5978(89)90040-X
Wagner, C., and Vinaimont, T. (2010). Evaluating the wisdom of crowds. Proc. Issues Inf. Syst. 11, 724–732.
Wang, C., Pan, R., Wang, X., Qi, X., Zhao, H., Guo, S., et al. (2020). Decision-making process during collective movement initiation in golden snub-nosed monkeys (Rhinopithecus roxellana). Sci. Rep. 10, 480–410. doi: 10.1038/s41598-019-57191-3
Wang, X., Sun, L., Li, J., Xia, D., Sun, B., and Zhang, D. (2015). Collective movement in the Tibetan macaques (Macaca thibetana): early joiners write the rule of the game. PLoS One 10:e0127459. doi: 10.1371/journal.pone.0127459
Ward, A. J. W., Sumpter, D. J. T., Couzin, I. D., Hart, P. J. B., and Krause, J. (2008). Quorum decision-making facilitates information transfer in fish shoals. Proc. Natl. Acad. Sci. 105, 6948–6953. doi: 10.1073/pnas.0710344105
Watts, D., and Mitani, C. (2001). Boundary patrols and intergroup encounters in wild chimpanzees. Behaviour 138, 299–327. doi: 10.1163/15685390152032488
Wegge, J., Roth, C., Neubach, B., Schmidt, K. H., and Kanfer, R. (2008). Age and gender diversity as determinants of performance and health in a public organization: the role of task complexity and group size. J. Appl. Psychol. 93, 1301–1313. doi: 10.1037/a0012680
West, S. A., Griffin, A. S., and Gardner, A. (2007). Evolutionary explanations for cooperation. Curr. Biol. 17, R661–R672. doi: 10.1016/j.cub.2007.06.004
White, F. J., Overdorff, D. J., Keith-Lucas, T., Rasmussen, M. A., Eddie Kallam, W., and Forward, Z. (2007). Female dominance and feeding priority in a prosimian primate: experimental manipulation of feeding competition. Am. J. Primatol. 69, 295–304. doi: 10.1002/ajp.20346
Willems, E. P., Arseneau, T. J. M., Schleuning, X., and van Schaik, C. P. (2015). Communal range defence in primates as a public goods dilemma. Philos. Trans. R. Soc. B Biol. Sci. 370:20150003. doi: 10.1098/rstb.2015.0003
Wrangham, R. W. (1980). An ecological model of female-bonded primate groups. Behaviour 75, 262–300. doi: 10.1163/156853980X00447
Wrangham, R. W., and Glowacki, L. (2012). Intergroup aggression in chimpanzees and war in nomadic hunter-gatherers. Hum. Nat. 23, 5–29. doi: 10.1007/s12110-012-9132-1
Wrangham, R. W., and Peterson, D. (1996). Demonic Males: Apes and the Origins of Human Violence. Boston, MA: Houghton Mifflin.
Keywords: organisational psychology, coordination, collective decision-making, group dynamics, group foraging
Citation: Williams L, Shultz S and Jensen K (2022) The primate workplace: Cooperative decision-making in human and non-human primates. Front. Ecol. Evol. 10:887187. doi: 10.3389/fevo.2022.887187
Edited by:
Cécile Garcia, UMR7206 Eco Anthropologie et Ethnobiologie (EAE), FranceReviewed by:
Annemie Ploeger, University of Amsterdam, NetherlandsGabriel Ramos-Fernandez, National Autonomous University of Mexico, Mexico
Karline Janmaat, University of Amsterdam, Netherlands
Copyright © 2022 Williams, Shultz and Jensen. This is an open-access article distributed under the terms of the Creative Commons Attribution License (CC BY). The use, distribution or reproduction in other forums is permitted, provided the original author(s) and the copyright owner(s) are credited and that the original publication in this journal is cited, in accordance with accepted academic practice. No use, distribution or reproduction is permitted which does not comply with these terms.
*Correspondence: Leoma Williams, leoma.williams@manchester.ac.uk