Assessment of Environmental Flow Requirements for Four Major Chinese Carps in the Lower Reaches of the Jinsha River, Southwest China
- 1State Key Laboratory of Simulation and Regulation of Water Cycle in River Basin, China Institute of Water Resources and Hydropower Research, Beijing, China
- 2Yangtze River Eco-Environmental Engineering Research Center, China Three Gorges Corporation, Beijing, China
- 3Hubei Field Observation and Scientific Research Stations for Water Ecosystem in Three Gorges Reservoir, Yichang, China
An appropriate environmental flow (AEF) is one of the necessary conditions for fish spawning. The operation of cascade reservoirs in the lower reaches of the Jinsha River has changed the discharge process downstream, which in turn has directly affected the spawning habitat of fishes. Determining how to quantitatively evaluate the impact of different outflows from Xiangjiaba Reservoir on the spawning habitat of four major Chinese carp species in the downstream area is a key problem that must be solved immediately. The Yibin River section was selected as the study area, and the velocity and water depth were measured. A physical habitat simulation model (PHABSIM) was used to analyze the main hydrodynamic conditions that affect the spawning of the four major Chinese carp species and to quantify the weighted usable area (WUA) for the fishes based on different outflows from Xiangjiaba Reservoir. Hydrodynamic suitability curves for the four major Chinese carp species were established based on observed data from 2012 to 2020, and a two-dimensional hydrodynamic model was developed using a triangular grid to obtain the hydrodynamic distribution characteristics of the fish habitat. Then, the AEF range was obtained based on the outflow-WUA relationship. The results showed that the velocity during the spawning period was 0.6–1.3 m/s, the most appropriate velocity was 0.9–1.0 m/s, the water depth range was 3.0–18.0 m, and the most appropriate water depth was 9.0–12.0 m. Additionally, the AEF range was 2,000–4,500 m3/s, and the optimal AEF was, m3/s. The research results provide a scientific basis for the ecological operation of cascade reservoirs in the lower reaches of the Jinsha River.
Introduction
Freshwater ecosystems provide vital services for human existence but are among the world’s most threatened ecosystems, primarily because of river damming. Worldwide, there are more than 50,000 reservoirs and dams with heights greater than 15 m and millions of smaller reservoirs and dams (Wild et al., 2019). To provide sustainable and clean electricity under the Paris Agreement (Ganesan et al., 2019; Mulvaney et al., 2020) and to mitigate climate change impacts due to global population growth, dams are needed and therefore their construction will continue to increase (Theara et al., 2020). According to the bulletin of the International Hydropower Association (IHA), since 2002, China’s installed hydropower capacity has ranked first globally. By the end of 2018, the total installed hydropower capacity of China was 3.5*105°MW, accounting for 27.2% of the world’s total installed hydropower capacity, and ranking first in the world. To realize the benefits of flood control, electricity, shipping, and water supply, dam construction has increased rapidly in recent decades.
In the Yangtze River basin, the lower reaches of the Jinsha River (from the Panzhihua hydrological station to the Yibin station) have abundant hydro energy resources (Huang et al., 2018; Zhang et al., 2020). Four large hydropower stations, Wudongde, Baihetan, Xiluodu, and Xiangjiaba, are planned or under construction. The Xiluodu-Xiangjiaba cascade reservoirs are the first phase of the plan, with installed capacities of 12,600 and 6,000°MW, respectively, and they began operating in 2013 and 2012 for power generation (Figure 1). They have an important role in contributing water for domestic use and agriculture, sustaining transportation corridors, and enabling power generation and industrial production. Additionally, the lower reaches of the Jinsha River are also an important part of the “national nature reserve for rare and endemic fish in the upper Yangtze River” (”reserve” for short) (Luo et al., 2015; Shen et al., 2019). The length of the reserve is approximately 360 km and stretches from 1.8 km downstream of the Xiangjiaba dam to the main district of Chongqing municipality. With its unique flow patterns, climate and natural environment, the reserve nurtures a rich variety of aquatic organisms. Of these, fish resources are particularly abundant. There are more than 70 kinds of rare fishes, including four major Chinese carps, Coreius guichenoti, Acipenser dabryanus, and the common sucker (He et al., 2018; Li et al., 2019). The four major Chinese carps namely, grass carp (Ctenopharyngodon idella), black carp (Mylopharyngodon piceus), silver carp (Hypophthalmichthys molitrix), and bighead carp (Hypophthalmichthys nobilis), are economically important fishes in the river (Shao et al., 2018).
Riverine habitat health relies on proper streamflow conditions to support the aquatic communities at life-history stages. Sex maturation, spawning cues and migration, egg fecundity, larval transport, settlement, and recruitment may all require distinct flow regimes for the species to succeed and flourish. This concept of ensuring adequate water for aquatic ecosystems is a key element in many international policies and in the water laws of many countries. The implementation of environmental flows is one of the measures needed to restore or to maintain “good environmental status” and can be included in the river basin management planning process (Ji et al., 2014).
The construction of dams and exploitation of water resources have led many countries to provide methods for assessing the appropriate environmental flow to preserve their aquatic ecosystems. Once the Xiluodu-Xiangjiaba cascade reservoir began operating, the disturbance to the hydrological rhythm became irreversible. Such changes can affect many fundamental processes and functional characteristics of healthy rivers, such as the flow regimes, sediment conditions, and thermal characteristics, thus leading to a rapid decline in aquatic biodiversity and essential ecosystem services (Yin et al., 2015). The alterations of natural river conditions could influence the critical habits of different species, since each species may have an “optimal environmental window” for reproductive success and recruitment maximization. Spawning is a vital and sensitive time for most fishes, and this period is thus often deemed a representative pivotal habit for them, especially because their breeding is closely related to hydrological factors (Zhang et al., 2019; Baumgartner et al., 2020). According to the fish resource monitoring data, the types and numbers of fishes in the downstream reserve displayed downward trends after the hydropower station began operating. The habitat area is a direct indicator that reflects the living environment of fishes, and the operation of the reservoir has caused the habitat area to decline; thus, the continuity and integrity of the habitat have been segmented and weakened. Maintaining a suitable outflow from reservoirs is a prerequisite for ensuring the number of fish spawning; therefore, determining the appropriate environmental flow (AEF) downstream is increasingly urgent and necessary. Estimating the environmental flow of the reserve can improve the understanding of the flow requirements for important aquatic organisms and provide support for decision-making regarding hydropower resource development in drainage basins.
Environmental flows can be defined based on the quantity, timing and quality of flows required to sustain freshwater and river ecosystems. Human livelihoods and survival depend on these ecosystems and have essential impacts on freshwater ecosystems. This concept of environmental flows has been widely generalized since it was developed, creating opportunities for restoration that can be implemented in hundreds of thousands of river systems that are strongly or moderately affected by hydraulic structures. Worldwide, more than 200 different methods have been developed to determine environmental flow in 50 countries. These methods can be classified into four main groups (Ceola et al., 2018; Soininen et al., 2019): hydrological methods, hydraulic methods, habitat simulation methods and holistic methods. In all of these methods, there is an assumption that relationships between the flow and physical habitat determine the environmental condition of a river (Shokoohi and Amini, 2014). Hydrological methods are based on measured hydrological data that is combined with specific proportions or percentiles to determine the minimum environmental flow or AEF, and commonly used methods include the Tennant method, 7Q10 method, distribution flow method (DFM), and Q95 method based on the flow duration curve (FDC). Hydraulic methods are based on the assumption that saving the natural morphological characteristics of rivers is adequate for meeting minimum water requirements; under this assumption, there is no need to understand what species currently exist in the river and what habitat conditions they require, but this approach may be inaccurate. These methods have been used as a component in habitat simulations and with holistic methods to form more than 23 different methods; these methods have been employed in approximately 11% of the environmental flow studies conducted worldwide. The best method from this category is the wetted perimeter method, which uses the wetted perimeter of rivers as a surrogate for fish habitat. The habitat simulation method combines a hydrodynamic model with the life-history traits of specific aquatic organisms at different stages to quantitatively describe the relationship between flow and habitat area. However, determining environmental flows for a diversity of species at a specific life stages requires time and costs for data preparation, which are critical obstacles to widespread use of these methods, especially in developing countries (Nikghalb et al., 2016; Volchek et al., 2019). The holistic method determines the natural hydrological regime on an appropriate scale by considering various factors, such as climate, geomorphology, vegetation cover, animal water requirements, and human interventions (Campos Jorge Santos and Cunha, 2018).
Each method has its own advantages and disadvantages, and different methods have been applied to the environmental flow assessment of different rivers or lakes around the world. The hydrological method was applied to a semiarid salt-affected wetland exposed to hydrological manipulation to determine its salinity and vegetation dynamics (Coletti et al., 2017) and was also used to estimate the environmental flow, resulting in the conclusion that the allocation of AEF was the most effective way to protect wetlands (Sajedipour et al., 2017; Ye et al., 2017).
Recent reviews have suggested that linking biologically transformed variables (such as the number of fish eggs, the number of juvenile fish, the growth rate of fish, and other factors) with hydrological data is ideal for estimating environmental flows (Stuart et al., 2019; Ding et al., 2020). Therefore, habitat simulation methods that predict the effect of altered or reduced flows on aquatic life are more reasonable for flow estimation. Among the habitat simulation methods, the physical habitat simulation model (PHABSIM) is the most widespread habitat method used to establish flow standards or to link habitat temporal variations with fish population dynamics. This model requires the habitat needs of selected aquatic species to be represented by habitat suitability curves compared to river hydraulic geometry variables (Oyague et al., 2020).
Environmental flow has been estimated in the lower reaches of the Jinsha River. Jia et al. (2020) used the DFM based on the Pearson-III curve to calculate the best environmental flow at the Pinshan station of the Jinsha River. Dai et al. (2020) used the RVA method to calculate the environmental flow for the lower reaches of the Jinsha River. However, the existing results in the calculation of AEF are mostly based on hydrological methods and cannot reflect the actual flow demand of specific fish spawning and breeding habitats.
In this paper, we selected the Yibin River section of the lower Jinsha River and used the PHABSIM to obtain a more accurate environmental flow by analyzing the differences in adaptability of four major Chinese carps with different spawning habits to various hydrological elements. Therefore, the objective of this study was to quantitatively analyze the WUA of the spawning habitats of four major Chinese carp species in the Yibin River section in response to different outflows from the Xiangjiaba Reservoir. The research results provide specific environmental goals for the optimal operation of cascade reservoirs and have important practical significance for the protection of important aquatic habitats in the lower reaches of the Jinsha River.
Materials and Methods
Study Area
To study the appropriate environmental flow of fish during spawning, it was first necessary to select indicative fish species that could represent important fish in the reserve. According to existing research results, the spawning sites of the four major Chinese carps are mainly distributed in the middle reaches of the Yangtze River (Li et al., 2013, 2016), the middle and lower reaches of the Jinsha River, and some sections of the Yalongjiang River. The four major Chinese carps are typical fishes with drifting eggs and are very sensitive to flow variation (Ban et al., 2019). These carp species require specific environmental and hydrological conditions to achieve spawning success; e.g., they are more susceptible to changes in the flow patterns resulting from dam construction than are other species. Therefore, the four major Chinese carp species were selected as the target species due to their economic importance and role as environmental indicators.
The spawning sites of the four major Chinese carps are widely distributed in the lower reaches of the Jinsha River and the middle reaches of the Yangtze River. The Yibin River section (from the Xiangjiaba Dam to the Yibin hydrological station) is one of the most important large-scale spawning sites for the carp (He et al., 2018). Therefore, the scope of the study area was the Yibin River section (Figure 2) with a length of approximately 34.0 km. There are four hydrological stations in the study area, including the Xiangjiaba hydrological station, Hengjiang hydrological station, Erlangtan hydrological station, and Yibin hydrological station. Xiangjiaba station was used to monitor the outflow from Xiangjiaba Reservoir; it is located 400 m downstream of the Xiangjiaba Dam, and approximately 1,000 m downstream of the station is the confluence of the Hengjiang River and Jinsha River. The Erlangtan hydrological station is located 10.6 km downstream of the Xiangjiaba dam. The main monitoring items included water level, discharge, velocity and water quality, and the monitoring frequency was up to once per day.
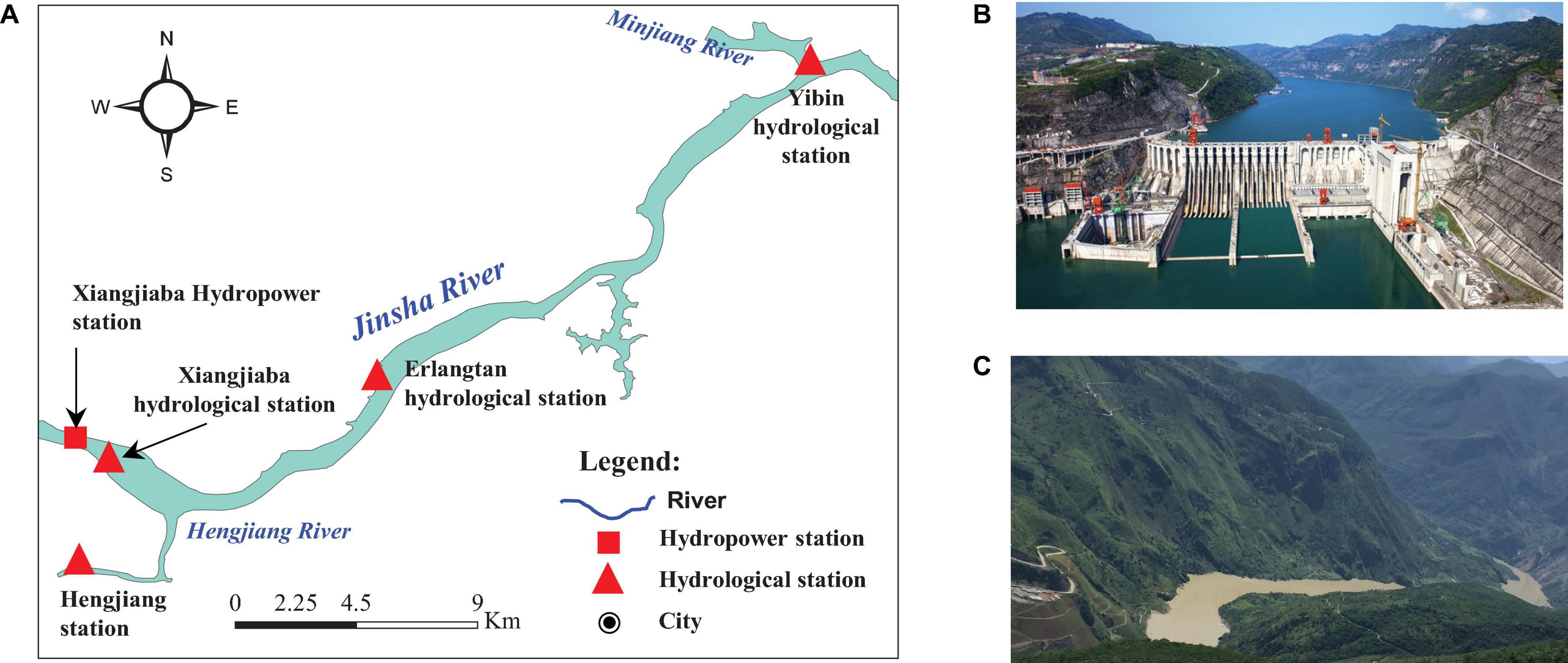
Figure 2. Location of the study area and pictures of the Xiangjiaba Dam and the Jinsha River. (A) Boundary of the hydrodynamic model. (B) Xiangjiaba dam. (C) Lower reaches of the Jinsha River.
Methods
Physical habitat simulation model was used to obtain the environmental flow of four major Chinese carps, and the main computational process of PHABSIM is divided into two parts: hydraulic simulation and habitat simulation (Parasiewicz and Walker, 2007; Dana et al., 2017). Hydrodynamic simulation predicts the depth and water velocity distributions under different flows, and habitat simulation calculates the area of habitat corresponding to the depth and velocity distributions (Beecher et al., 2010; Gholami et al., 2020). The hydrodynamic simulation requires the user to enter river topographic data that correspond to a site map. The user then enters and calibrates hydrodynamic data (e.g., water levels and velocities) collected at known discharges to ensure that the hydrodynamic model reasonably simulates the hydrodynamic conditions in the river over the range of flows modeled. First, according to the habitat fitness curve corresponding to hydraulic elements, the corresponding habitat suitability index (HSI) can be obtained. Then, the hydraulic factors of the river under different flow discharges can be calculated. Finally, the HSC of the important hydraulic parameters (water depth, flow velocity, etc.) of the target species is defined, and the HSI is multiplied by the water area to estimate the WUA under hydrodynamic conditions.
Hydrodynamic Model Establishment and Verification
The hydraulic model can simulate the changes in the velocity and water level of a river due to various boundary conditions. In this study, MIKE 21 FM was used to simulate the hydrodynamic distribution characteristics of the habitat. This model adopts a triangular, boundary-fitted grid for better representations of complex coastline configurations. Compared with traditional rectilinear grid arrangements, the number of grid points in a model domain can be reduced while maintaining high resolutions for the study area.
The topographic data of the Yibin River section (measurement scale: 1:1,000) were obtained from the topographic survey results from 2012. The calculation range of the hydrodynamic model extends from the Xiangjiaba dam site to the Yibin hydrological station, and the tributary in the interval is the Hengjiang River. The grid was divided according to the boundary. Since a triangular grid can finely describe the shoreline conditions of the study area, the model adopted an irregular triangular grid. The grid size was determined according to the spatial distribution of the topography. According to the above principles and considering the calculation time, the total number of grid nodes was 12,854, the total number of cells was 23,845, the maximum area was 1580.2 m2, and the minimum area was 138.3 m2. The topography and grid generation are shown in Figure 3.
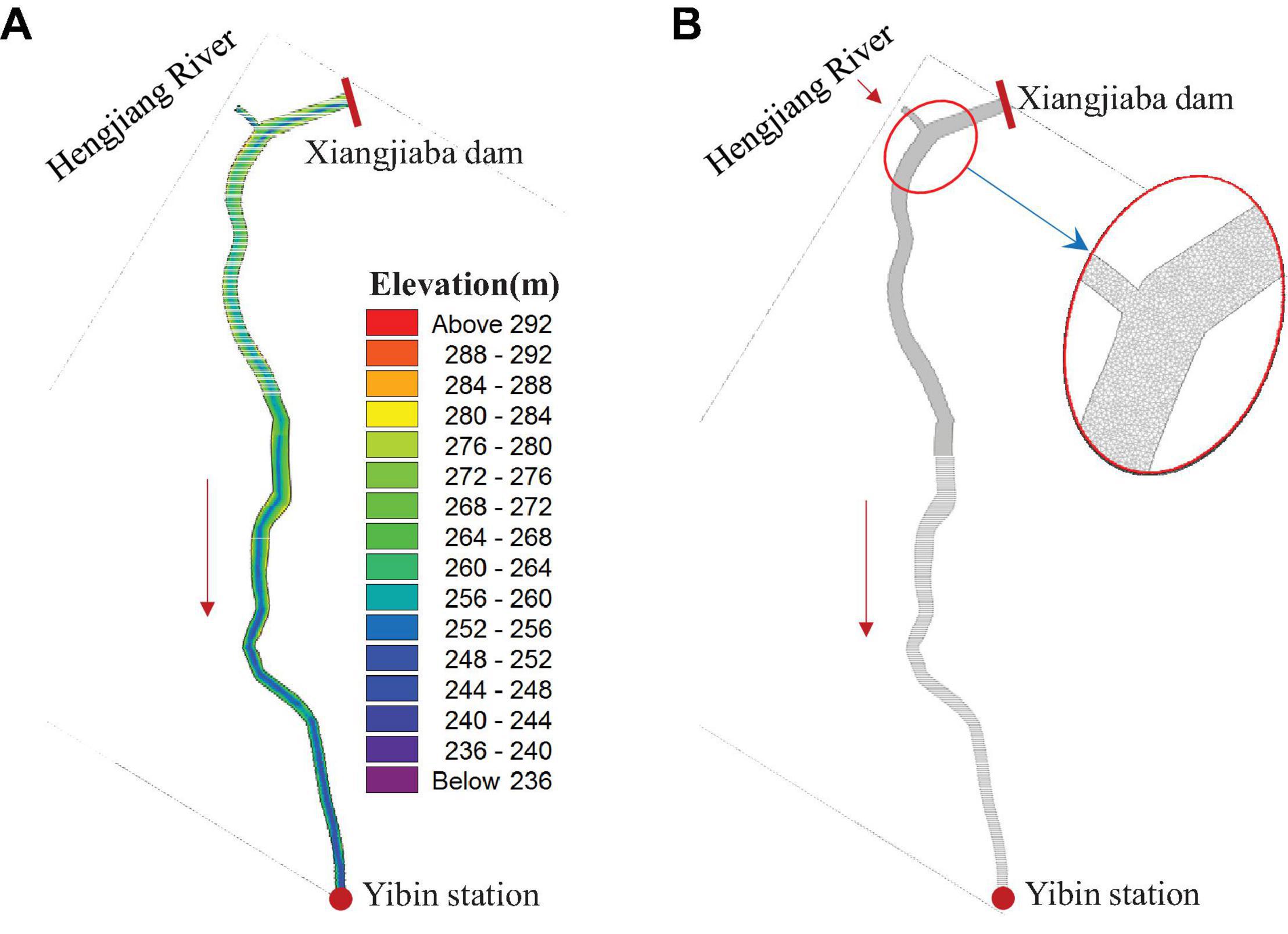
Figure 3. Topography and grid generation of the hydrodynamic model. (A) Topography. (B) Grid generation.
First, we calibrated the hydrodynamic parameters of the model. The upper boundary selected the flow at the Xiangjiaba and Hengjiang hydrological stations, and the lower boundary used the water level at the Yibin hydrological station. The final calibrated Manning coefficient was 30 m1/3/s. The model was verified with the observed data from January 1 to June 30 in 2019, and the verified hydrological stations included the Xiangjiaba hydrological station and Erlangtan hydrological station (the distances from the model entrance were 0.2 and 11.0 km, respectively, as shown in Figure 2). The simulated velocity was verified by the observed velocity, and the simulated flow velocity on the two sections was compared with the observed flow velocity, as shown in Figure 4. The simulated flow velocity was in good agreement with the observed velocity. The overall simulation effect of the model was good, the simulation result was reliable, and the Manning coefficient was 30.0 m1/3/s.
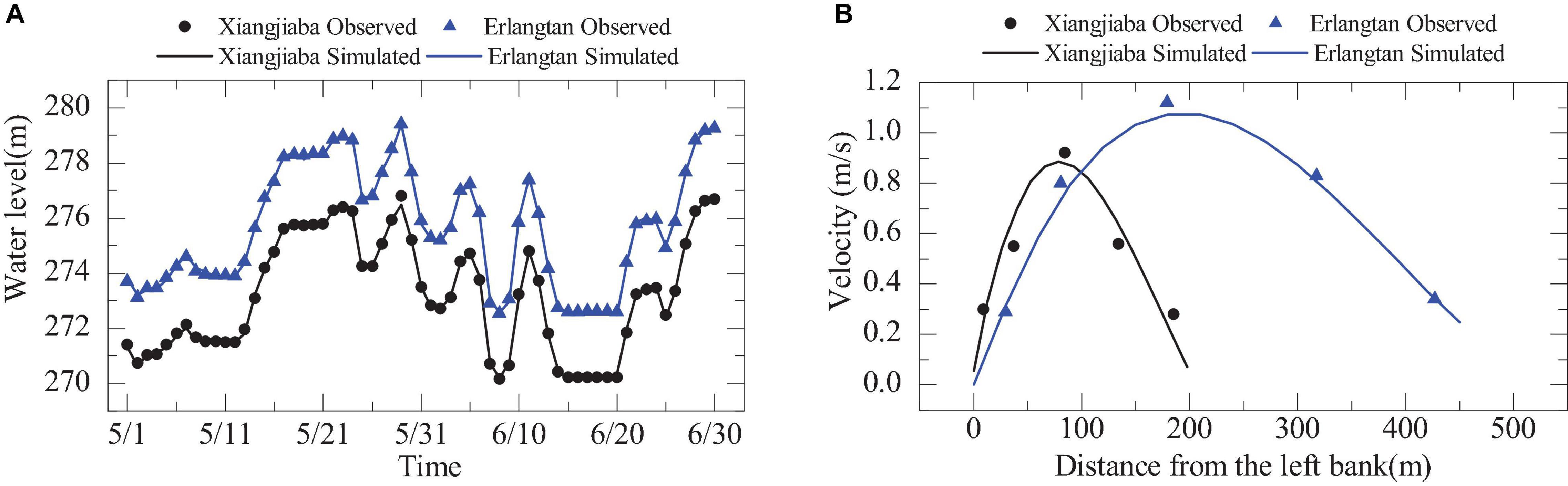
Figure 4. Comparison of observed and simulated velocity and water level. (A) Water level at the two hydrological stations. (B) Velocity at the two hydrological stations.
Habitat Model and Determining the Habitat Suitability Index Values
The velocity and water depth distribution under different flow conditions were calculated according to the hydraulic model. The HSI of the targeted species was also acquired, thus forming HSCs corresponding to the velocity and depth of survey points. For each flow, the available habitat was calculated by adding the area of each computational cell that comprised the control section to its corresponding composite suitability index. Finally, the WUA of the whole habitat was calculated using the following formula:
where WUA is the weighted usable area for the target species, m2; n is the number of grids; CSFi is the combined suitability factor of grid i; Vi is the flow velocity for grid i, m/s; Hi is the water depth for grid I, m; Ci is the substrate status of grid i; and Ai is the area of computational cell i, m2.
Vi and Hi were calculated by the hydrodynamic model. For CSF, there are four common methods: the product method, geometric average method, minimum method and weighted average method. The product method was used; it assumes that the HSI values of f(Vi), f(Hi), and f(Ci) have equal impact, and its formula is as follows:
where f(Vi), f(Hi), and f(Ci) are the respective suitability indexes of velocity, depth, and river substrate.
The steps to quantify the environmental flow of the targeted species habitats are as follows:
1. Collect the spawning location, time, number of eggs, and corresponding hydrodynamic conditions of the target species;
2. Associate the HSI with the hydrodynamic influencing factors (e.g., water depth, flow velocity, substrate) of the habitat of the target species and formulate suitability standards;
3. Establish a hydrodynamic model of the spawning habitat of the target species and use different outflows as boundary conditions to simulate the spatial characteristics of the hydrodynamic distribution of the habitat during the spawning and reproduction stages of the target species;
4. Quantify the preference of the target species for the hydrodynamic factors of the habitat, and obtain the habitat suitability of the influencing factors index to draw the HSC for the target species;
5. Input the HSC of the target species into the habitat model combined with the calculation of the water depth and flow velocity simulated by the hydrodynamic model to study the WUA in response to different flows of the river. The calculation method is shown in Figure 5.
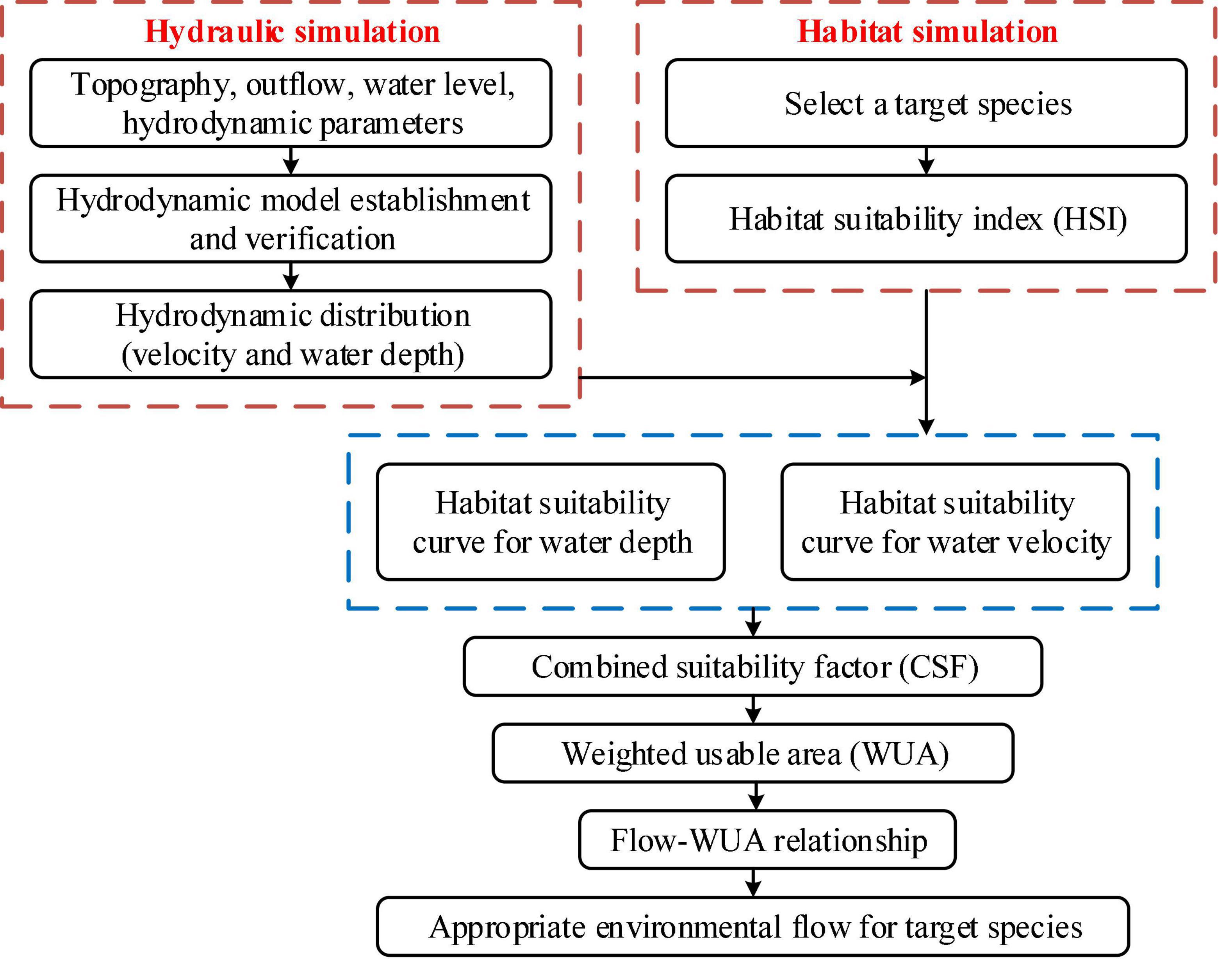
Figure 5. Flow chart for solving the appropriate environmental flow based on the physical habitat simulation model (PHABSIM).
Determining the HSI values of the four major Chinese carps is an important step in habitat simulation. The HSI mainly compares the preference of fishes to habitat and habitat factors. The suitability indexes take the value of the habitat factor as the abscissa and the suitability of the fish to the habitat factor as the ordinate to establish a continuous curve of the relationship between the preference of each habitat factor and the habitat factor, thereby quantitatively characterizing the hydrodynamic characteristics of the habitat. To compare the quality of life for the fishes under this condition, a value between 0 and 1 is used to define the preference of the fish for habitat factors. A value of 0 means completely unsuitable, a value of 1 means completely suitable, and a value closer to 1 indicates that the fish has a greater preference for this habitat factor.
The spawning time of the four major Chinese carps in the lower reaches of the Jinsha River occurs from May to June each year. The ovulation activity of the broodstock is closely related to the hydrodynamic conditions of the river. In addition to hydrodynamic conditions, water temperature is an important factor affecting fish spawning. The peak spawning period occurs when the water temperature is 21–24°C, and when the water temperature drops below 18°C, the four major Chinese carps stop spawning. Water temperature is related to not only the discharge but also the thermal structure of the reservoir and the opening method of the reservoir gate; however, the construction of the HIS values during the spawning and breeding periods of the fish considered only the hydrodynamic conditions (velocity and water depth). We used the monitoring results of the four major Chinese carps from 2012 to 2020 and the hydrological data of the corresponding hydrological sites during the same period. A total of 27 spawning processes were monitored in the Yibin River section, and the velocity and water depth at the spawning sites were obtained at the same time. Then, a response analysis was performed, and the demand curves for the hydrological process during the spawning period of the carp were fitted. The suitable range of flow velocity was 0.6–1.3 m/s, the most suitable flow velocity was 0.9–1.0 m/s, the suitable water depth was 3.0–18.0 m, and the most suitable water depth was 9.0–12.0 m (Figure 6). When constructing the model, the substrate in the representative reach was considered “good.” According to the lack of measured data, f(Ci) was set as 1.
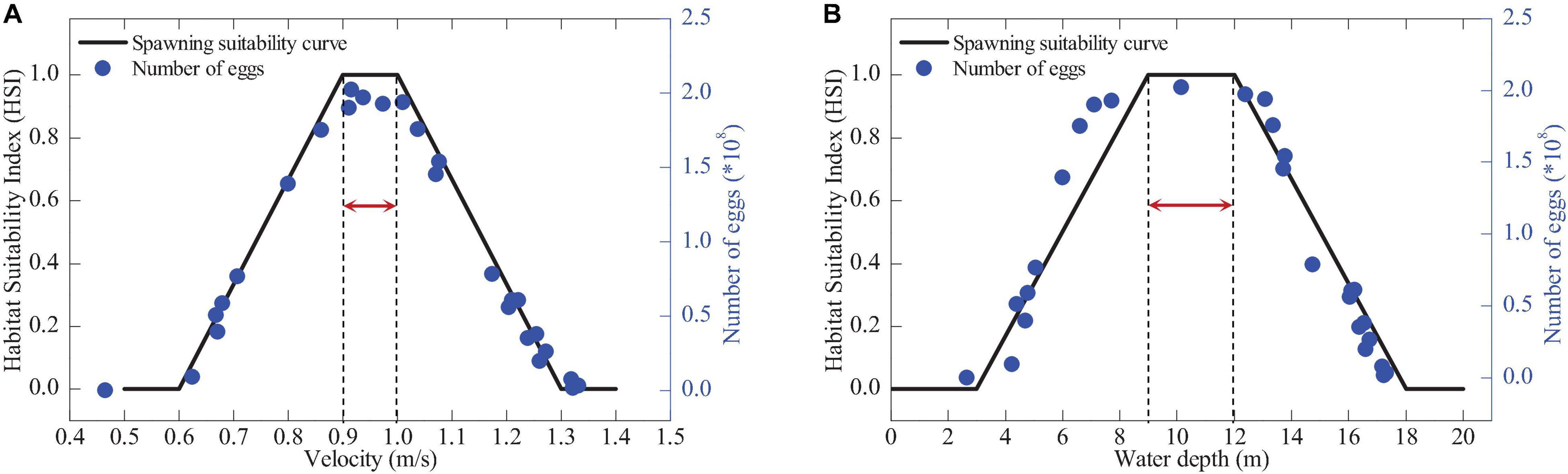
Figure 6. Habitat suitability indexes (HSIs) and numbers of eggs for four major Chinese carp species (arrows indicate the optimal HSI range). (A) Velocity. (B) Water depth.
Results
Design of Simulated Outflow From Xiangjiaba Reservoir
According to the existing hydrological and fish spawning monitoring data from 2012 to 2018, the spawning of the four major Chinese carps mainly occurred from May to June each year. During the spawning period, the initial outflow of the Xiangjiaba Reservoir was 1,700 m3/s, and the maximum outflow was 5,800 m3/s; thus, the upstream boundary flow (outflow) set by the hydrodynamic model was 1,500–6,000 m3/s, and the range was sufficient to cover the actual flow situation of the study area. Within the abovementioned range, the outflows of the reservoir were 1,500, 2,000, 2,500, 3,000, 3,500, 4,000, 4,500, 5,000, 5,500, and 6,000 m3/s and were used as simulated flows.
Spatial Distribution of the Combined Suitability Factor
According to the hydrodynamic model, the flow velocity and water depth distribution of the Yibin River section under different flow conditions were calculated. Figure 7 shows the spatial distribution of the combined suitability factor (CSF) of the spawning habitats of the four major Chinese carps under different outflows of the Xiangjiaba Reservoir. When the outflow of the reservoir was 1,500 m3/s, according to the results of the hydrodynamic model, the average flow velocity of the habitat was 0.59 m/s, the average water depth was 8.15 m, and the CSFs in different regions were all small. Furthermore, an average value of 0.406 indicated that the CSF did not fully meet the hydrodynamic conditions required for fish to spawn; however, with the increase in outflow, the flow velocity and water depth in the curved river section increased, and there were suitable hydrodynamic conditions for fish to spawn. Thus, the CSF increased rapidly. When the outflow was 3,000 m3/s, the average flow velocity of the habitat reached 0.82 m/s, the average water depth was 10.15 m, and the average CSF reached 0.485. This was followed by a gradual decrease; when the reservoir outflow was 6,000 m3/s, the average velocity was 1.06 m/s, and the average water depth was 12.73 m. Both the flow velocity and water depth increased, which exceeded the most suitable hydrodynamic conditions required for the spawning and reproduction of the fishes, and the CSF was reduced to 0.416.
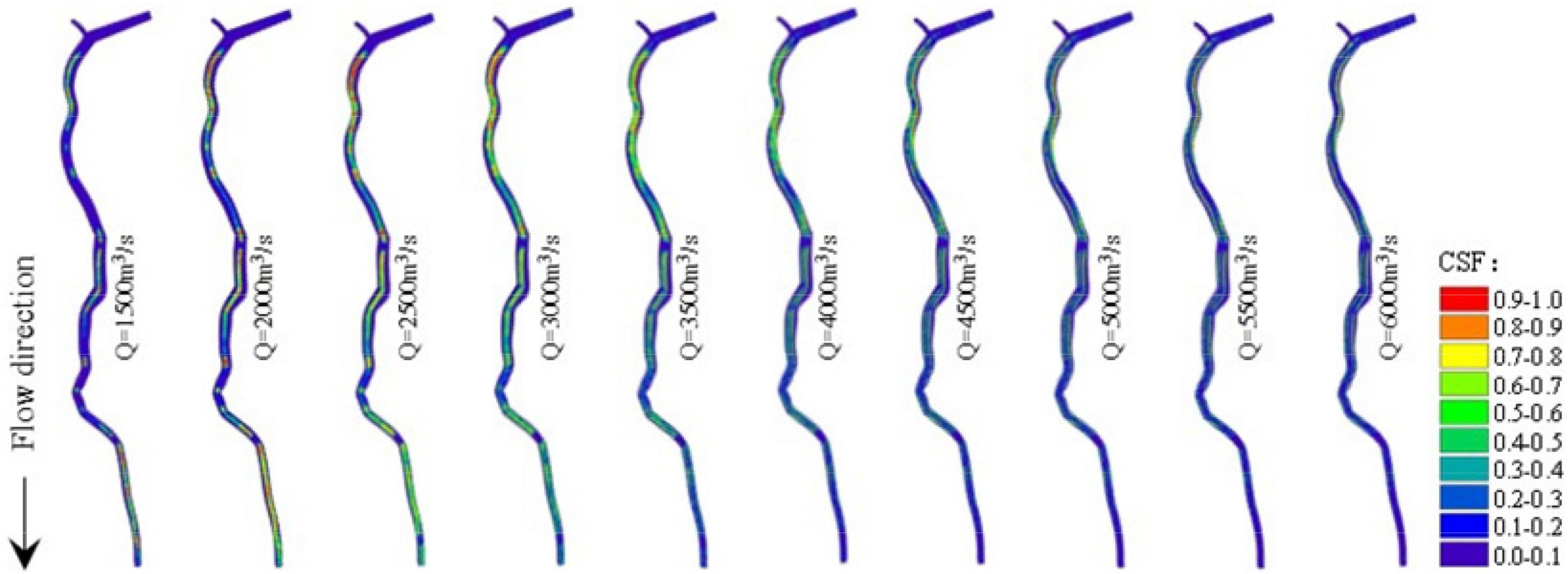
Figure 7. Spatial distribution of the combined suitability factor (CSF) in different outflows from the Xiangjiaba Reservoir.
Variation Trend of Weighted Usable Area Under Different Outflows From Xiangjiaba Reservoir
Then, we combined the spawning suitability curve of the four major Chinese carps in the habitat to obtain the WUA of the fishes under different outflows. There are many ways to determine optimal environmental flows according to the relationship between the outflow of a reservoir and the WUA. When the WUA is used as the only target to set the outflow level, the outflow can be adjusted for the target species at the maximum WUA. In the PHABSIM, it is assumed that the WUA and the number of fish eggs are positively correlated; that is, the larger the WUA is, the greater the number of fish eggs. Therefore, the outflow corresponding to the maximum WUA in the reservoir outflow-WUA relationship curve is used as the most suitable environmental flow. The total area of the Yibin River section was 15.10 km2 (when the water level at Yibin station was 262.50 m), and the WUA of the habitat under 10 different outflows and the proportion of the total area of the river section are shown in Table 1. When the outflow of the Xiangjiaba Reservoir was 1,500 m3/s, the WUA of the habitat was the smallest, at only 1.81 km2, accounting for 12.01% of the total area of the river section. With the increase in the outflow of the reservoir, the WUA first showed an increasing trend, e.g., when the outflow flow was 3,000 m3/s, the WUA reached the maximum value, which was 3.66 km2, accounting for 24.26% of the total area. As the outflow increased, the WUA began to decrease. When the outflow increased to 6,000 m3/s, the WUA declined to 2.41 km2, accounting for 15.97% of the total river area. During the spawning and breeding period of the four major Chinese carps, the WUA was smaller when the outflow was too small or too large, and when the outflow was moderate, the WUA was more suitable.
Discussion
Relationship Between the Outflow and Weighted Usable Area
Based on the PHABSIM, the WUAs corresponding to the different outflows of 10 scenarios were obtained, and the outflow-WUA relationship curve was drawn (Figure 8). It can be seen from the figure that when the outflow from the Xiangjiaba Reservoir increased from low to high, the WUA of the fish habitats first increased and then gradually decreased, and the curve peak indicated the most suitable habitats for a given discharge. When the outflow flow was 2,000 m3/s, the WUA increased more obviously, indicating that the fishes were more sensitive to lower flow conditions; however, when the outflow increased to 2,500 m3/s, the WUA tended to be flat with the increase in outflow. When the outflow was 3,000 m3/s, the WUA reached the maximum value, indicating that the outflow at this time best met the flow demand for the fish spawning and breeding periods. Once the outflow was greater than 3,000 m3/s, with the increase in outflow, the WUA showed a relatively gentle downward trend, indicating that the continued increase in outflow had an adverse impact on the fish habitat. According to the existing research results, when the WUA of the habitat accounts for approximately 20% of the total river section studied, that is, when the habitat area is maintained above 3.0 km2, the habitat area can meet the spatial requirements for fish spawning and reproduction. When the habitat area is less than 3.0 km2, the spawning and reproduction space will be smaller, and the river ecosystem service function will be damaged. Therefore, the suitable environmental flow range is 2,000–4,500 m3/s, and the most suitable environmental flow is 3,000 m3/s. Jia et al. (2020) obtained the environmental flow range in the lower reaches of the Jinsha River using the improved Tennant method and the monthly frequency computation method, and this range was between 2,100 and 3,900 m3/s, which is consistent with our results.
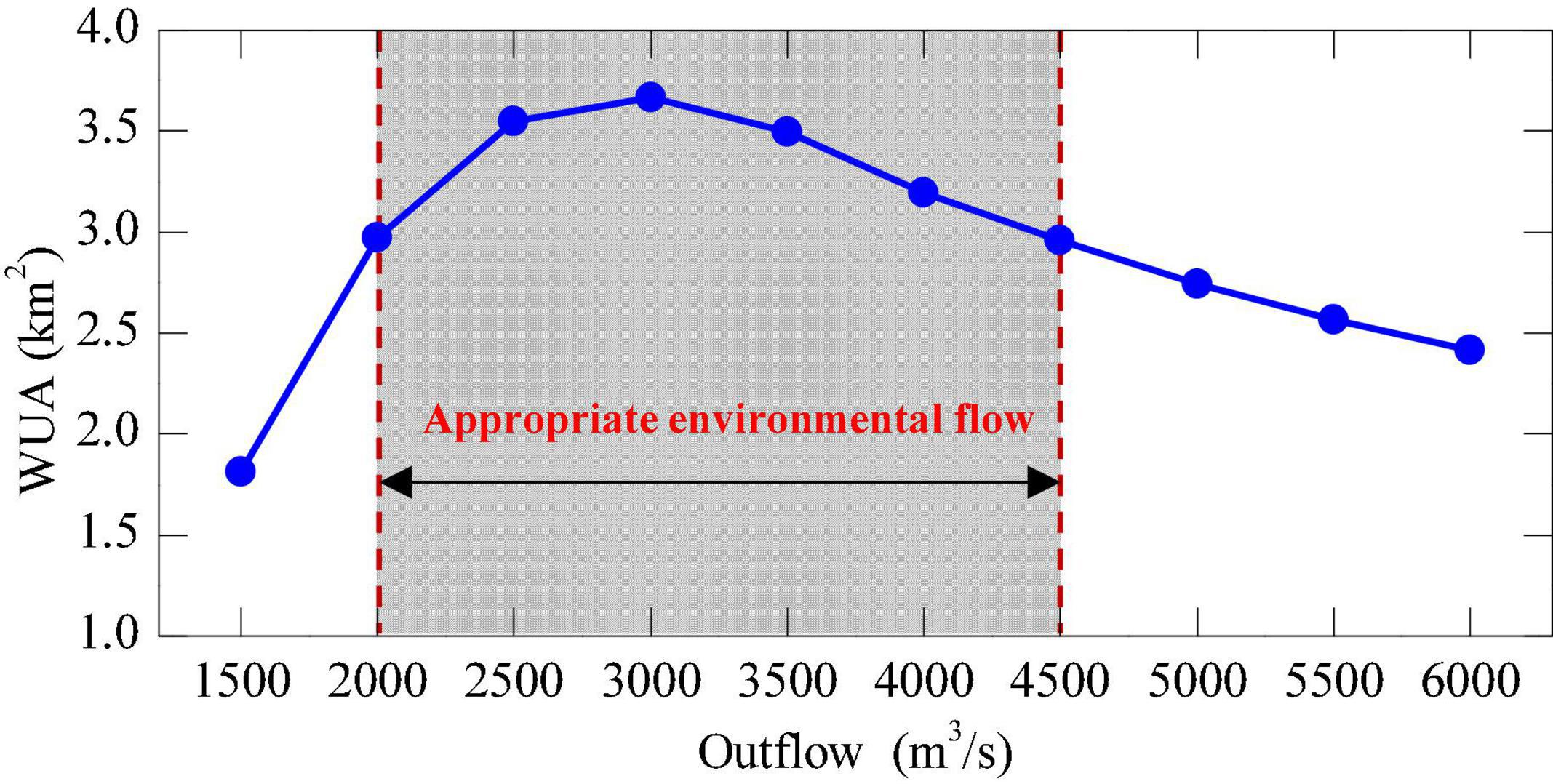
Figure 8. Outflow-weighted usable area (WUA) relationship derived from the physical habitat simulation model (PHABSIM).
Influence of Ecological Operations on Fish Spawning
Since 2017, the Xiluodu-Xiangjiaba cascade reservoir has begun to carry out a joint ecological regulation experiment for the natural reproduction of fishes in downstream rivers from May to June every year (Figure 9). Ecological regulation considers the hydrology and water temperature conditions required for fish reproduction, and water temperature regulation is mainly for fishes that produce slimy eggs, such as A. dabryanus and the common sucker. For drifting egg fishes, such as those of the four major Chinese carps and C. guichenoti, during reservoir operation, first, it is necessary to ensure the appropriate environmental flow, and second, it is necessary to continuously increase the outflow to create and promote fish spawning. From May 20 to 23, 2017, the Xiluodu-Xiangjiaba reservoirs carried out the first joint environmental regulation experiment to provide suitable environmental flow for fish spawning. When the average outflow during the regulation period was 3,221 m3/s, the Yibin River section exhibited a spawning peak, as indicated by the fish egg density reaching a peak (1.5 × 106 ind./km2), which was 1.3 times the fish egg density before the implementation of ecological regulation. The outflow was controlled at 2,400, 2,700, 3,000, 3,500, 4,000, and 4,500 m3/s, and the final flow was maintained at 2,700–3,200 m3/s. The monitoring data (from May to June) show that the number of fish eggs from the four carp species increased. Thus, the environmental flow interval proposed in this article is reasonable and can provide reference suggestions for the protection of the four major Chinese carps in the lower reaches of the Jinsha River and the ecological regulation of cascade reservoirs. However, due to the limited water temperature data, the parameters were simplified when using the PHABSIM, and the impact of water temperature on the habitat area was not considered. Therefore, it is necessary to formulate more accurate HSIs based on more observational data. In addition to the natural spawning stage of the fishes, future research should focus on ensuring other key life histories and goals, such as deepening the Xiluodu-Xiangjiaba cascade reservoir ecological operation plan, to more effectively apply the research results to the protection of the four major Chinese carps throughout their life cycles.
Conclusion
The paper used the PHABSIM to obtain a more accurate environmental flow for the spawning of four major Chinese carps in the lower reaches of the Jinsha River. The results showed that the AEF is 2,000–4,500 m3/s, and the most suitable AEF is 3,000 m3/s. Therefore, the research results provide a reference for the formulation of ecological operation plans and are important for the protection of fishes in the lower reaches of the Jinsha River. However, due to a lack of water temperature data, the parameters were simplified in the calculations, and the impact of water temperature on the habitat was not considered. Therefore, it was necessary to formulate a more accurate suitability curve combined with other key factors, such as water temperature. In future studies, to achieve more realistic results, we should delve into the influence of the vertical thermal structure of the Xiangjiaba Reservoir and different operation modes of the dam on the water temperature of the Yibin River section and how water temperature changes affects fish spawning.
Data Availability Statement
The original contributions presented in the study are included in the article/supplementary material, further inquiries can be directed to the corresponding author.
Author Contributions
LD: conception and design of study. YW: programming, software development, and designing computer programs. HD: oversight and leadership responsibility for the research activity planning and execution. WL and TZ: conducting a research and investigation process. QZ: data/evidence collection and preparation. All authors contributed to the article and approved the submitted version.
Funding
This work was supported by Open Research Fund of the State Key Laboratory of Simulation and Regulation of Water Cycle in River Basin (China Institute of Water Resources and Hydropower Research) (Grant Number: Program IWHR-SKL-KF202010), the National Natural Science Foundation of China (Grant Number: 51809150), and China Postdoctoral Science Foundation (Grant Numbers: 2019T120119 and 2020M670391).
Conflict of Interest
The authors declare that the research was conducted in the absence of any commercial or financial relationships that could be construed as a potential conflict of interest.
Publisher’s Note
All claims expressed in this article are solely those of the authors and do not necessarily represent those of their affiliated organizations, or those of the publisher, the editors and the reviewers. Any product that may be evaluated in this article, or claim that may be made by its manufacturer, is not guaranteed or endorsed by the publisher.
References
Ban, X., Diplas, P., Shih, W., Pan, B., Xiao, F., and Yun, D. (2019). Impact of Three Gorges Dam operation on the spawning success of four major Chinese carps. Ecol. Eng. 127, 268–275. doi: 10.1016/j.ecoleng.2018.12.004
Baumgartner, M. T., Piana, P. A., Baumgartner, G., and Gomes, L. C. (2020). Storage or run-of-river reservoirs: exploring the ecological effects of dam operation on stability and species interactions of fish assemblages. Environ. Manage. 65, 220–231. doi: 10.1007/s00267-019-01243-x
Beecher, H. A., Caldwell, B. A., DeMond, S. B., Seiler, D., and Boessow, S. N. (2010). An Empirical Assessment of PHABSIM Using Long-Term Monitoring of Coho Salmon Smolt Production in Bingham Creek, Washington. North Am. J. Fish Manage. 30, 1529–1543.
Campos Jorge Santos, P. V., and Cunha, A. C. (2018). Potential of environmental flow: participatory method for determining environmental flow in hydropower’s reservoirs in Amazon rivers. Eng. Sanit. Ambiental 23, 137–150.
Ceola, S., Pugliese, A., Ventura, M., Galeati, G., Montanari, A., and Castellarin, A. (2018). Hydro-power production and fish habitat suitability: Assessing impact and effectiveness of ecological flows at regional scale. Adv. Water Resour. 116, 29–39. doi: 10.1016/j.advwatres.2018.04.002
Coletti, J. Z., Vogwill, R., and Hipsey, M. R. (2017). Water management can reinforce plant competition in salt-affected semi-arid wetlands. J. Hydrol. 552, 121–140. doi: 10.1016/j.jhydrol.2017.05.002
Dai, L. Q., DAi, H. C., Liu, H. B., Wang, Y., Guo, J. L., Cai, Z. S., et al. (2020). Development of an optimal model for the Xiluodu-Xiangjiaba cascade reservoir system considering the downstream environmental flow. Sustain. 12, 1–18. doi: 10.1016/j.jenvman.2022.114582
Dana, K., Jana, C., and Tomas, V. (2017). Analysis of environmental flow requirements for macroinvertebrates in a creek affected by urban drainage (Prague metropolitan area. Czech Republic). Urban Ecosyst. 20, 785–797. doi: 10.1007/s11252-017-0649-2
Ding, Z. Y., Fang, G. H., Wen, X., Tan, Q. F., Lei, X. H., Liu, Z. H., et al. (2020). Cascaded Hydropower Operation Chart Optimization Balancing Overall Ecological Benefits and Ecological Conservation in Hydrological Extremes Under Climate Change. Water Resour. Manag. 34, 1231–1246. doi: 10.1007/s11269-020-02496-6
Ganesan, A. L., Schwietzke, S., Poulter, B., Arnold, T., Lan, X., Rigby, M., et al. (2019). Advancing Scientific Understanding of the Global Methane Budget in Support of the Paris Agreement. Glob. Biogeochem. Cycle 33, 1475–1512. doi: 10.1029/2018gb006065
Gholami, V., Khalili, A., Sahour, H., Khaleghi, M. R., and Tehrani, E. N. (2020). Assessment of environmental water requirement for rivers of the Miankaleh wetland drainage basin. Appl. Water Sci. 10, 1–14.
He, D., Dai, Q., Tang, H., and Yang, Z. (2018). Length-weight relationships for three fish species from the middle and lower reaches of the Jinsha River. China. J. Appl. Ichthyol. 34, 760–761. doi: 10.1111/jai.13688
Huang, X. R., Gao, L. Y., Yang, P. P., and Xi, Y. Y. (2018). Cumulative impact of dam constructions on streamflow and sediment regime in lower reaches of the Jinsha River. China. J Mt. Sci. 15, 2752–2765. doi: 10.1007/s11629-018-4924-3
Ji, C. M., Zhou, T., and Huang, H. T. (2014). Operating Rules Derivation of Jinsha Reservoirs System with Parameter Calibrated Support Vector Regression. Water Resour. Manag. 28, 2435–2451. doi: 10.1007/s11269-014-0610-6
Jia, W. H., Dong, Z. C., Duan, C. G., Ni, X. K., and Zhu, Z. Y. (2020). Ecological reservoir operation based on DFM and improved PA-DDS algorithm: A case study in Jinsha river. China. Hum. Ecol. Risk Assess. 26, 1723–1741. doi: 10.1080/10807039.2019.1603075
Li, G. N., Sun, S. K., Liu, H. T., and Zheng, T. G. (2019). Optimizing the entrance location for a fish pass facility with limited attraction flow in a large river - A case study of the Jinsha River. China. Can. J. Civ. Eng. 46, 847–857. doi: 10.1139/cjce-2018-0523
Li, M. Z., Duan, Z. H., Gao, X., Cao, W. X., and Liu, H. Z. (2016). Impact of the Three Gorges Dam on reproduction of four major Chinese carps species in the middle reaches of the Changjiang River. Chin. J. Oceanol. Limnol. 34, 885–893. doi: 10.1007/s00343-016-4303-2
Li, M. Z., Gao, X., Yang, S. R., Duan, Z. H., Cao, W. X., and Liu, H. Z. (2013). Effects of Environmental Factors on Natural Reproduction of the Four Major Chinese Carps in the Yangtze River. China. Zool. Sci. 30, 296–303. doi: 10.2108/zsj.30.296
Luo, D. N., Lin, P., Li, Q. B., Zheng, D., and Liu, H. Y. (2015). Effect of the impounding process on the overall stability of a high arch dam: a case study of the Xiluodu dam. China. Arab. J. Geosci. 8, 9023–9041. doi: 10.1007/s12517-015-1868-6
Mulvaney, K. M., Selin, N. E., Giang, A., Muntean, M., Li, C. T., Zhang, D., et al. (2020). Mercury Benefits of Climate Policy in China: Addressing the Paris Agreement and the Minamata Convention Simultaneously. Environ. Sci. Technol. 54, 1326–1335. doi: 10.1021/acs.est.9b06741
Nikghalb, S., Shokoohi, A., Singh, V. P., and Yu, R. (2016). Ecological Regime versus Minimum Environmental Flow:Comparison of Results for a River in a Semi Mediterranean Region. Water Resour. Manag. 30, 4969–4984. doi: 10.1007/s11269-016-1488-2
Oyague, E., Vera, A., Cabrejos, L., and Franco, P. (2020). Differences in physical habitat simulation system modelling results using benthic or pelagic fish species as indicators in Peruvian Andes-Amazon rivers. River Res. Appl. 36, 828–842. doi: 10.1002/rra.3602
Parasiewicz, P., and Walker, J. D. (2007). Comparison of MesoHABSIM with two microhabitat models (PHABSIM and HARPHA). River Res. Appl. 23, 904–923. doi: 10.1002/rra.1043
Sajedipour, S., Zarei, H., and Oryan, S. (2017). Estimation of environmental water requirements via an ecological approach: A case study of Bakhtegan Lake. Iran. Ecol. Eng. 100, 246–255. doi: 10.1016/j.ecoleng.2016.12.023
Shao, K., Que, Y. F., Xiong, M. H., Li, W. T., Yu, D., and Shi, F. (2018). Length-weight and length-length relationships of thirteen fish species from the lower Jinsha River, southwest China. J. Appl. Ichthyol. 34, 774–776. doi: 10.1111/jai.13609
Shen, J. J., Zhang, X. F., Wang, J., Cao, R., Wang, S., and Zhang, J. H. (2019). Optimal Operation of Interprovincial Hydropower System Including Xiluodu and Local Plants in Multiple Recipient Regions. Energies 12, 1–19.
Shokoohi, A., and Amini, M. (2014). Introducing a new method to determine rivers’ ecological water requirement in comparison with hydrological and hydraulic methods. Int. J. Environ. Sci. Technol. 11, 747–756. doi: 10.1007/s13762-013-0404-z
Soininen, N., Belinskij, A., Vainikka, A., and Huuskonen, H. (2019). Bringing back ecological flows: migratory fish, hydropower and legal maladaptivity in the governance of Finnish rivers. Water Int. 44, 321–336. doi: 10.1080/02508060.2019.1542260
Stuart, I., Sharpe, C., Stanislawski, K., Parker, A., and Mallen-Cooper, M. (2019). From an irrigation system to an ecological asset: adding environmental flows establishes recovery of a threatened fish species. Mar. Freshw. Res. 70, 1295–1306. doi: 10.1071/mf19197
Theara, T., Sarit, C., and Chantha, O. (2020). Integrated modeling to assess flow changes due to future dam development and operation in Stung Sen River of Tonle Sap Lake Basin, Cambodia. J. Water Clim. Chang. 11, 1123–1133. doi: 10.2166/wcc.2019.115
Volchek, A., Kirvel, I., and Sheshko, N. (2019). Environmental flow assessment for the Yaselda River in its Selets reservoir section. Ecohydrol. Hydrobiol. 19, 109–118. doi: 10.1016/j.ecohyd.2018.06.001
Wild, T. B., Reed, P. M., Loucks, D. P., Mallen-Cooper, M., and Jensen, E. D. (2019). Balancing Hydropower Development and Ecological Impacts in the Mekong: Tradeoffs for Sambor Mega Dam. J. Water Resour. Plan. Manage.-ASCE 145, 1–14.
Ye, Z. X., Li, W. H., Qiu, J. J., and Aji, D. (2017). Investigation of the safety threshold of eco-environmental water demands for the Bosten Lake wetlands, western China. Quat. Int. 440, 130–136. doi: 10.1016/j.quaint.2016.12.030
Yin, Y. P., Huang, B. L., Wang, S. C., and Li, J. H. (2015). Potential for a Ganhaizi landslide-generated surge in Xiluodu Reservoir, Jinsha River, China. Environ. Earth Sci. 73, 3187–3196. doi: 10.1007/s12665-014-3619-5
Zhang, P. P., Cai, Y. P., Yang, W., Yi, Y. J., and Yang, Z. F. (2020). Climatic and anthropogenic impacts on water and sediment generation in the middle reach of the Jinsha River Basin. River Res. Appl. 36, 338–350. doi: 10.1002/rra.3572
Keywords: Xiangjiaba Reservoir, hydrodynamic condition, weighted usable area (WUA), spawning suitability curve, ecological operation
Citation: Dai L, Wang Y, Dai H, Li W, Zheng T and Zhang Q (2022) Assessment of Environmental Flow Requirements for Four Major Chinese Carps in the Lower Reaches of the Jinsha River, Southwest China. Front. Ecol. Evol. 10:810889. doi: 10.3389/fevo.2022.810889
Received: 08 November 2021; Accepted: 21 March 2022;
Published: 13 April 2022.
Edited by:
Yixin Zhang, Soochow University, ChinaReviewed by:
Michael Alister Reid, University of New England, AustraliaYuankun Wang, North China Electric Power University, China
Yan Yunzhi, Anhui Normal University, China
Copyright © 2022 Dai, Wang, Dai, Li, Zheng and Zhang. This is an open-access article distributed under the terms of the Creative Commons Attribution License (CC BY). The use, distribution or reproduction in other forums is permitted, provided the original author(s) and the copyright owner(s) are credited and that the original publication in this journal is cited, in accordance with accepted academic practice. No use, distribution or reproduction is permitted which does not comply with these terms.
*Correspondence: Lingquan Dai, dailingquan@163.com