Field-Deployed Extruded Seed Pellets Show Promise for Perennial Grass Establishment in Arid Zone Mine Rehabilitation
- 1Environmental and Conservation Sciences, Murdoch University, Murdoch, WA, Australia
- 2Centre for Ecosystem Science, School of Biological, Earth & Environmental Sciences, University of New South Wales, Sydney, NSW, Australia
- 3School of Biological Sciences, University of Western Australia, Crawley, WA, Australia
- 4Department of Biodiversity, Conservation and Attractions, Kings Park Science, Kings Park, WA, Australia
- 5Agricultural Science, Murdoch University, Murdoch, WA, Australia
Current methods of mine rehabilitation in the arid zone have a high failure rate at seedling emergence largely due to limited availability of topsoil and low water-holding capacity of alternative growth substrates such as mining overburden and tailings. Further, seedlings have consistently failed to emerge from seeds sown on the soil surface using traditional broadcasting methods. Seed pellets, formed by extruding soil mixtures and seeds into pellets, can potentially increase soil water uptake through enhanced soil-seed contact and thereby improve seedling emergence. We tested an extruded seed pelleting method in a three-factor field experiment (i.e., different pellet-soil mixtures, organic amendments, and simulated rainfall regimes) in north-western Australia. Given the observed lack of seedling emergence from broadcast seeds, the aims of the experiment were to assess: (i) the use of pellets to promote native seedling emergence and establishment and; (ii) the soil physico-chemical and microbiological changes that occur with this method of rehabilitation. The effects of pellet-soil mixtures, organic amendment, and rainfall regime on seedling emergence and survival of three native plant species suggest trade-offs among responses. Pellets made with a 1:1 blend of topsoil and a loamy-sand waste material had the highest seedling emergence, while 100% topsoil pellets had lower emergence probably because of hardsetting. Triodia pungens (a native grass) survived to the end of the experiment while Indigofera monophylla and Acacia inaequilatera (native shrubs) emerged but did not survive. Adding an organic amendment in the extruded pellet inhibited Triodia seedling emergence but increased soil microbial activity. Overall, extruded pellets made from a 1:1 blend showed promise for the establishment of Triodia seeds and beneficially, incorporates mine waste overburden and lesser amounts of topsoil. Further research is needed to improve pelleting production and to test the applicability of the method at scale, for different species and other ecosystem types.
Introduction
Successful mine rehabilitation is a critical environmental requirement for the extractive minerals industry and given increasing pressure to attain and maintain a social license to mine, is fast becoming a social requirement too (Mercer-Mapstone et al., 2018). One of the biggest challenges in mine rehabilitation is overcoming barriers to seedling establishment and growth on reconstructed soil profiles, particularly in arid climates. Mining tends to create a legacy of modified soils, mine waste materials (i.e., overburden) and tailings, which have altered properties that are hostile for seedlings, such as reduced water holding capacity, toxic pH levels, low organic matter, nutrient deficiencies and low microbial activity (Muñoz-Rojas et al., 2016a; Bateman et al., 2018; Wu et al., 2019). Best rehabilitation practice is to cover mine waste materials with topsoil, but other approaches are needed when the availability of topsoil is limited. Soil amendments can help to overcome limitations to plant growth within waste materials (Muñoz-Rojas et al., 2016b; Kumaresan et al., 2017; Kneller et al., 2018). Organic amendments including compost, mulch and sewage have been shown to increase water holding capacity and soil microbial activity in arid land soils (Bastida et al., 2008; You et al., 2016; Kneller et al., 2018). The inoculation of rehabilitation sites with soil microorganisms such as cyanobacteria can also restore soil functionality and enhance the rate of recovery (Bowker, 2007; Williams et al., 2017; Muñoz-Rojas et al., 2018a,b; Román et al., 2018). These results show promise for achieving mine rehabilitation in arid lands if they can be implemented at multi-hectare scales.
One emerging approach to rehabilitation is the use of extruded pellets containing a mixture of beneficial soil material with seeds and organic amendments (Madsen et al., 2012, 2016a, 2018; Brown et al., 2018). The pellet matrix aims to provide a suitable microsite for seedling establishment through increased soil water availability and enhanced microbial-driven mineralisation, while precision placement of pellets provides seeds with improved access to soil water (Madsen et al., 2016b; Lewandrowski et al., 2017a; Davies et al., 2018). Modified food processing equipment is often used to squeeze dough-like mixtures of soil, seeds and amendments into pellets. Extruded pellets have been part of a strategy to increase the success of the sagebrush (Artemisia spp.) shrub/steppe plant community restoration programs in cold desert ecosystems of the USA where they can increase seedling emergence and biomass of sagebrush species compared to un-pelleted seeds depending on site conditions including rainfall and elevation (Madsen et al., 2016b; Davies et al., 2018). Further, extruded pellets have recently been used to inoculate degraded soils that originated from hot desert environments with beneficial native biocrust cyanobacteria, highlighting the potential to use pelleting technologies for soil microbial translocation and to up-scale bio-crust soil restoration initiatives (Román et al., 2020). These studies suggest the potential for extruded pellets to improve rehabilitation outcomes for a range of climatically-variable ecosystems, particularly mine sites lacking in topsoil resources or sites with highly altered soil profiles (Ritchie et al., 2020), but further tests on the design and efficacy of pellets are needed.
Field tests have identified factors that determine success and failure of extruded pellets. Site factors (e.g., elevation) and climate (e.g., rainfall amounts) within and across years can lead to variation in pelleting technology performance (e.g., Davies et al., 2018). Species and seed traits are likely to contribute to success too, as they do for broadcast seeding (e.g., greater success for large- compared to small-seeded species; Hallett et al., 2011), and particularly where these are exacerbated by the pellet extrusion process. The recruitment failure of species with complex dormancy mechanisms has been attributed to the mechanical pressure imposed during the pellet extrusion process which impedes embryo growth potential and germination capacity of small-seeded species (Clenet et al., 2019; Erickson et al., 2019). A modified extruded seed pelleting method, analogous to the original technology but with less pressure, could reduce extrusion pressure on seeds to avoid impaired seed recruitment. The method can also deliver beneficial native soil microbes via locally sourced topsoil that is known to improve early-stage soil function and plant recruitment (Muñoz-Rojas et al., 2018a,b; Román et al., 2020).
Here, we tested a technique of field-deployed extruded seed pellets in a plot-scale experiment to promote seedling establishment of a keystone perennial grass and two shrubs that do not recruit from bare seeds broadcast onto the soil surface or from sowing depths >6 mm (Erickson et al., 2017; Masarei et al., 2020). Traditional seeding practices in the region utilize heavy machinery and air-seeding drum units, which aerially broadcast seeds onto the soil surface (Erickson et al., 2019). Surface sowing is consistently associated with poor rehabilitation outcomes; seedling emergence has been <2% for field studies (Erickson et al., 2017; Golos et al., 2019; Masarei et al., 2020). Severe temperature conditions, increased predation, lack of water retention are some factors that contribute to limited seed germination and emergence (De Falco et al., 2012; Merino-Martín et al., 2017b). Therefore, new methods are needed to increase rehabilitation success and seed use efficiency (Merritt and Dixon, 2011). The objectives of the experiment were to assess: (i) the potential of field-deployed extruded seed pellets to facilitate native seedling emergence and survival; (ii) the effect of utilizing locally-sourced topsoil and adding organic amendment to pellets on seedling emergence and survival; and (iii) the soil physico-chemical and microbiological changes that accompany this method of rehabilitation.
Materials and Methods
Study Region
The Pilbara region in north-western Australia is a resource-rich region covering ~179,000 km2 (McKenzie et al., 2009). It is the site of many iron ore mines, and mines for other minerals, and has a recorded disturbance footprint comprising at least 3,000 km2 of mined land requiring rehabilitation (EPA, 2017). The dominant native ecosystem is spinifex (Triodia spp.) perennial grassland with scattered shrubs (e.g., Acacia spp.). Mine rehabilitation in the Pilbara is constrained by multiple abiotic and biotic barriers (e.g., limited moisture, variable rainfall driven by cyclonic systems, and annual potential evaporation that is 10-fold higher than rainfall inputs). For instance, one of the primary drivers of plant recruitment in this region comes from cyclonic rains (>4 events of 20–25mm) over the austral summer (December-April rainfall average = ca. 235 mm) when maximum temperatures are ca. 32°C, and commonly exceed 45°C (Lewandrowski et al., 2017a; Ritchie et al., 2017). Winter rainfall and temperatures are known to be insufficient to support plant recruitment in this region (May-November rainfall average = ca. 81 mm). In turn, native plant recruitment is spatially and temporally sporadic but aligned to the summer season and during those periods of higher moisture availability (Erickson et al., 2017; Lewandrowski et al., 2017a). Key physico-chemical and biological soil processes (e.g., nutrient cycling) are similarly driven by high moisture conditions during episodic and patchy rainfall events (Schwinning and Sala, 2004; Ford et al., 2007).
Experimental Design
The field experiment was conducted within a purpose-built rehabilitation research facility at BHP's Mt Whaleback iron ore mine, near Newman in the Pilbara, Western Australia (23°21′55.30“ S, 119°40′31.4” E). The facility comprises a rain exclusion shelter covering 64 irrigated and raised beds (i.e., plots measuring 400 × 200 × 25 cm). These are filled with five different reconstructed soil profiles sourced from mine waste materials (i.e., overburden) and stockpiled topsoil and allow the evaluation of seedling emergence and growth under a range of soil-rainfall treatments (Erickson et al., 2017).
The experimental design included two fixed factors: pellet type (four levels) and rainfall regime (two levels). The pellet types were topsoil (TS) and a 1:1 blend topsoil and a loamy-sand waste rock material (TS/WM), with and without an organic amendment (i.e., dried Triodia pungens leaf material). The (initial) rainfall regimes were equivalent to 60 mm and 120 mm and applied to plots. Fully factorial treatment combinations of pellet types and rainfall regimes were replicated three times (n = 4 × 2 × 3 = 24 data points).
The experiment ran for 16 weeks over the summer season, from October 2018 to February 2019, which is the natural recruitment window observed in the region (i.e., the season of more reliable rain and optimum soil temperatures of 20–35°C suitable for seed germination; Erickson et al., 2016b,c). The plot rainfall amounts of 60 and 120 mm were delivered to mimic large rainfall events typical of the Pilbara wet season (BoM, 2019), with the 7-day duration of simulated rainfall selected to maximize soil water potentials and therefore the envelope for germination and emergence (after Lewandrowski et al., 2017a).
Seed Preparation
Three native plant species common to the local native ecosystem (Erickson et al., 2016a) and target species for Pilbara mine rehabilitation were tested: Triodia pungens, a long-lived perennial grass, and two leguminous perennial shrubs, Acacia inaequilatera and Indigofera monophylla. Seeds were sourced from commercial seed suppliers in October 2010 for Acacia inaequilatera, September 2014 for Indigofera monophylla, and March 2015 for Triodia pungens. All collections were wild-sourced and of local provenance. Seeds were stored at 15–20°C and 30–50% relative humidity, primarily under dark conditions, by the commercial suppliers between their collection and pellet preparation. Prior to pelleting, T. pungens seeds were removed from the floret to overcome floret-imposed physiological dormancy (after Erickson et al., 2016b). The two legumes were treated with hot (90°C) water for 1–2 min to break dormancy (after Erickson et al., 2016c, 2017). After treatment, seed germination capacity was 86 ± 2.6% for T. pungens, 31 ± 1.9% for I. monophylla and 69 ± 1.0% for A. inaequilatera. A standard mix of 40 T. pungens, 15 I. monophylla and 10 A. inaequilatera seeds was included in each pellet to simulate the synchronous multi-species recruitment pulse observed in nature.
Pellet Preparation
Each pellet (n = 216 pellets total) was prepared and delivered individually to maintain consistency in moisture content, soil, and seed quantities (Figure 1). Soil blend quantities were determined through preliminary experimentation to replicate a roughly circular, flat-disc shape with a target thickness of 5–10 mm to match a suitable germination and emergence depth for T. pungens seeds (Erickson et al., 2017; Masarei et al., 2020). Topsoil (TS) and loamy-sand waste material (WM) was sieved to obtain a <5 mm fraction for use, weighed and mixed with water by hand to obtain a slurry consistency. The specific quantities of soil, water and organic amendment added to each pellet are outlined in more detail in the Figure 1 caption. For ease of reporting, pellet types are labeled using acronyms i.e., TS (topsoil), WM (waste material) and TB (Triodia biomass amendment).
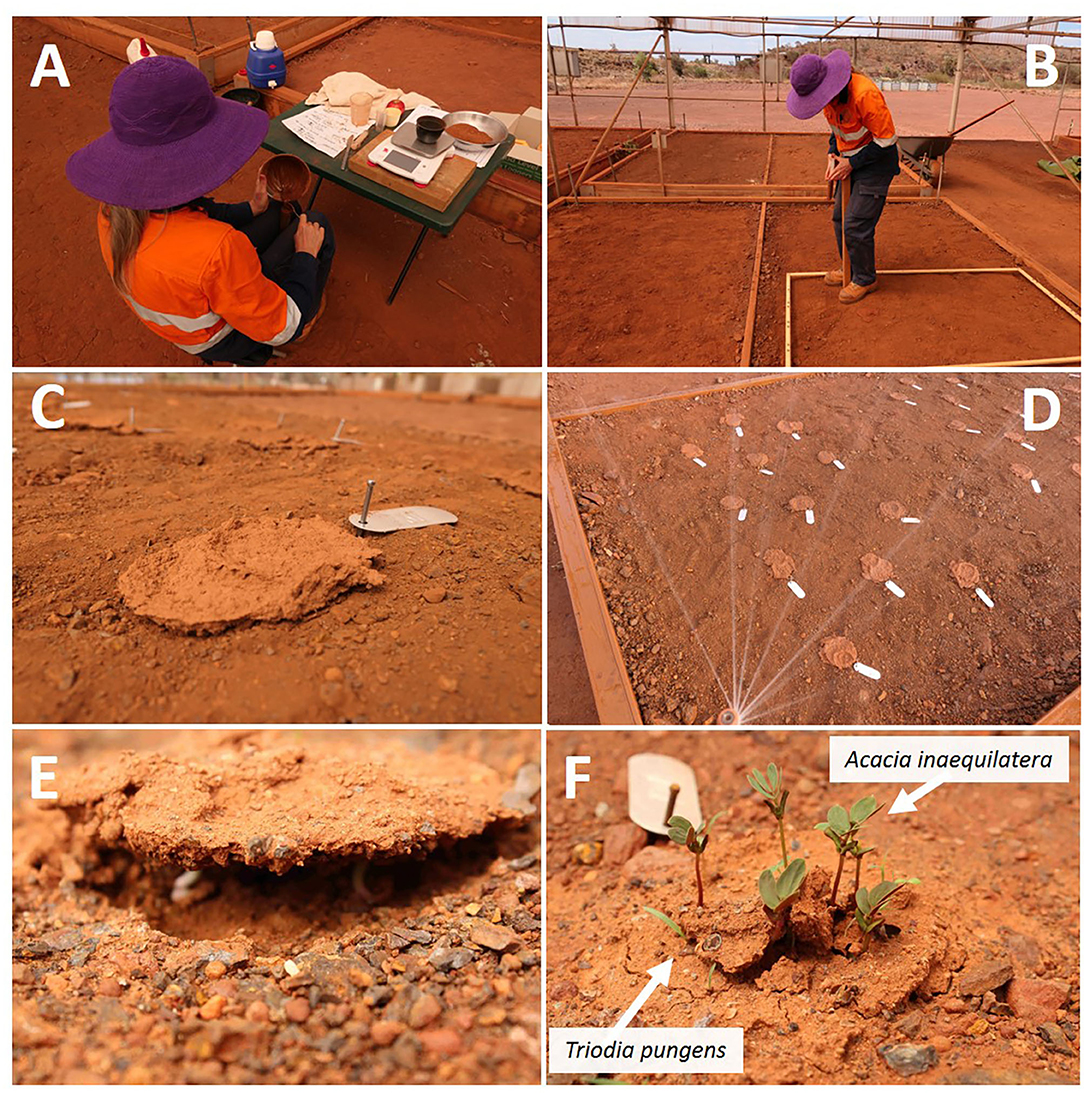
Figure 1. Pellets were prepared individually (A) and delivered via hand extrusion from a 60 ml Terumo syringe (Terumo Corporation, Philippines) with the end removed to create a 30 mm opening. TS pellets contained 101 g of soil and 33 g of water, whereas TS/WM pellets contained 109 g of soil and 28 g of water. Triodia leaf material was air dried for 6 weeks and cut into ~5 mm pieces; 0.4 g was added to each pellet. This amount of material was added to increase microbial activity and C mineralization potential and approximated that of the amounts of organic material in topsoil, but then halved to reduce the priming effect (sensu Kneller et al., 2018) following the principle of minimum effective dosage (Hueso-González et al., 2018). Soil blends containing 100% TS were extruded from a height of 60 cm and those containing a 1:1 blend of TS/WM were extruded from 40 cm. The drop heights were determined experimentally as a trade-off between mixture fluidity, obtaining the desired surface coverage and thickness, the constraints of retaining the integrity of pellets on impact and the projected operational requirements of large-scale machine delivery in the future (B). Pellet thickness was subsampled by measuring the vertical and horizontal diameter of pellets (C) in the center quadrat of each plot indicating that there was a significant difference in thickness between pellets of the two soil types and there was a small increase in thickness induced by the addition of Triodia biomass. Once extruded, pellets received simulated rains (D) as outlined in the “Rainfall simulation” methods section. During the process of recruitment, it was observed that some pellets were lifted by Acacia inaequilatera seedlings (E), whereas some pellets remained in contact with the soil surface and contained emergent seedlings of both Acacia inaequilatera and Trioda pungens seedlings (F).
Once the pellets were extruded they were left to dry for 48 h prior to watering to avoid a false start as seed imbibition reaches its maximum at between 8 and 48 h (Erickson et al., 2016a). Lastly, qualitative observations of pellet morphology were recorded with wetting and drying association with each simulated rainfall event (Figure 1).
Plot Preparation
The experiment utilized six 4 × 2 m (8 m2) plots of a uniform loamy-sand mine-waste substrate interspersed throughout the rain exclusion facility. Three 1 × 2 m quadrats were evenly spaced across each plot, and twelve modified extruded pellets were placed within each quadrat (see Figure 1 for pellet placement and production details); three pellets each of the four pellet types. To minimize the frequency of manufacturing errors, pellets were clustered by soil blend type but randomized within each quadrat.
Rainfall Simulation
Three plots were randomly allocated either 60 or 120 mm rainfall regimes. Rainfall was simulated via Hunter MP1000 Rotator sprinkler heads applied at 10 mm/h (Hunter Industries, San Marcos, California, USA) (Figure 1D). Adjacent plots were paired, and simulated rain was applied in discrete events every second day for 7 d to achieve the rainfall totals [i.e., 4 events × 30 mm over three plots and 4 events × 15 mm over three plots; following Lewandrowski et al. (2017a)]. Follow-up rainfall pulses of 10 mm were subsequently applied across all plots fortnightly to mimic the soil wetting and drying cycles of sporadic wet season rainfall (BoM, 2019). A second large rainfall event was simulated over 7 d during the 11th week of the experiment, consisting of 60 mm applied across all plots (i.e., 4 events × 15 mm). These discrete, but repeated rainfall patterns within a 7-day period have been shown to be a key recruitment trigger in this system and therefore formed the basis of our simulated rainfall treatments (Lewandrowski et al., 2017a). Volumetric soil water content (EC-5 ECH2O Dielectric Aquameter, Decagon Devices Inc., Pullman, Washington, USA) and soil temperature were recorded in two plots (Supplementary Figure 1) with Hobo®RX-3000 remote data logger stations (Onset Computer Corporation, Bourne, Massachusetts, USA). Data are reported for 60, 120, and 60 + 60, 120 + 60 mm rainfall regimes depending on when they were collected.
Seedling Emergence, Survival, and Above-Ground Biomass
Plant growth was monitored over the period of the experiment: emergence and survival were counted weekly for 2 weeks after each simulated 7-day rainfall event, and fortnightly thereafter. After 16 weeks, survival, leaf stage and total biomass were assessed. Total above-ground biomass was determined by harvesting plant shoots and oven drying at 60°C for 4 d and weighing with a five decimal point balance (i.e., ±0.00001 g).
Soil Physico-Chemical and Microbial Analyses
A bulked soil sample of 1 kg was taken from the top 5 cm of each of the six plots and the TS, WM and the 1:1 blend (TS/WM) prior to establishment of the experiment. Samples were air dried, sieved (<2 mm) and divided into two subsamples; one to characterize physico-chemical properties and the other stored at 4°C for 16 weeks prior to analysis of soil microbial activity. In addition, five 3 g samples were randomly taken from each plot and three from each of the donor soils for soil microbial community composition analysis. These samples were refrigerated for transportation to the laboratory at Kings Park Science (Kings Park, Western Australia) and stored at−20°C until analysis. Specific details of the soil physico-chemical analyses are detailed in Supplementary Table 1.
On completion of the experiment, soils beneath each pellet were collected and analyzed for microbial activity and microbial community composition (4 pellet types × 6 plots = 24 subpellet samples) Subpellet samples (50 g sample sieved <2 mm) were taken from the top 1 cm of soil immediately beneath pellets. Pellets were also collected where these had not disintegrated [3 pellet types (missing TS/WM + TB) × 4 replicates = 12 pellet samples].
Soil microbial activity was determined with the 1-day CO2 Solvita soil respiration test which determines soil microbial respiration rate based on the measurement of the CO2 burst produced after moistening dry soil (Haney and Haney, 2010) and has proved informative for Pilbara soils (Muñoz-Rojas et al., 2016a; Kneller et al., 2018; Bateman et al., 2019).
Environmental genomic DNA was isolated from 500 mg of soil using the DNeasy PowerSoil Kit (Qiagen, Venlo, Netherlands). The hypervariable regions V3-V4 of the 16S rRNA gene were targeted using the primer set 341F-785R (Klindworth et al., 2013) and amplified as described by Nielsen et al. (2017). Amplified products were submitted to the Ramaciotti Center for Genomics (UNSW Sydney, Australia) where they were purified before a second indexing PCR to incorporate unique Illumina Nextera XT indexes for each sample. Sequencing was performed on an Illumina MiSeq using a v3 Reagent Kit with a 2 × 300 bp run format. All sequence datasets are publicly available through the NCBI database under the project PRJNA668860.
Statistical Analyses
Soil and plant variables (seedling emergence and survival) were tested for normality and variance homogeneity using the Shapiro-Wilk and Levene's tests. For ease of interpretation, for each plant species, a 2-factor ANOVA was used to test differences in plant emergence, survival, biomass and microbial activity between rainfall regimes and pellet types. Comparisons between means were performed with the Tukey's HSD (honestly significant difference) test (p < 0.05). Variables were transformed where necessary to normalize data; plotted data are untransformed.
Bioinformatic analyses of soil DNA sequencing data were performed at the Ramaciotti Center for Genomics. Data were received de-multiplexed via the Illumina cloud-computer BaseSpace and analyzed using the inbuilt 16S Metagenomics application. Briefly, this service performs quality filtering of sequences before taxonomic classification using the ClassifyReads implementation of the Ribosomal Database Project Classifier as per Wang et al. (2007) referencing an Illumina-curated GreenGenes taxonomic database (16S Metagenomics BaseSpace App Guide (version 15055860 A) performed in April 2019). The analyses generate operational taxonomic units (OTUs); we plotted the top 10 most abundant OTUs (at order level) in a stacked bar chart.
Soil bacterial diversity measures (Shannon diversity and species richness) were calculated across treatments using the function “diversity” available through the Vegan package (Oksanen et al., 2017) within R (R Core Team, 2018) and tested using a one-way ANOVA followed by a Tukey HSD test. Community differences were assessed using permutational multivariate analysis of variance (PERMANOVA) and non-metric multidimensional scaling (nMDS). For both analyses, we used Bray-Curtis distance matrices of relative abundance derived from sequencing and used 9,999 permutations. PERMANOVAs were performed using the function “adonis2” in the Vegan package within R. All analyses were performed with R statistical software version 3.5.1 (R Core Team, 2018) and Primer (Clarke and Gorley, 2015).
Results
Seedling Emergence and Survival
Many seedlings emerged from the modified extruded seed pellets, with an average of 7.3 seedlings per pellet (of 65 seeds, ca. 11% emergence) over the duration of the experiment (Figure 2). However, few seedlings survived to the end of the experiment—success depended on species and the timing of simulated rainfall patterns (Figure 2). A high proportion of seeds that did not emerge in the first simulated rain event remained viable, as a second round of seedlings emerged after the second simulated rain event. Of the three species, Triodia emergence was most successful with 10% (4 of 40) seeds emerging in the first simulated rain event and 8% of remaining seeds in the second rain event. At the end of the experiment, 9 and 45% of these plants survived their respective emergence events.
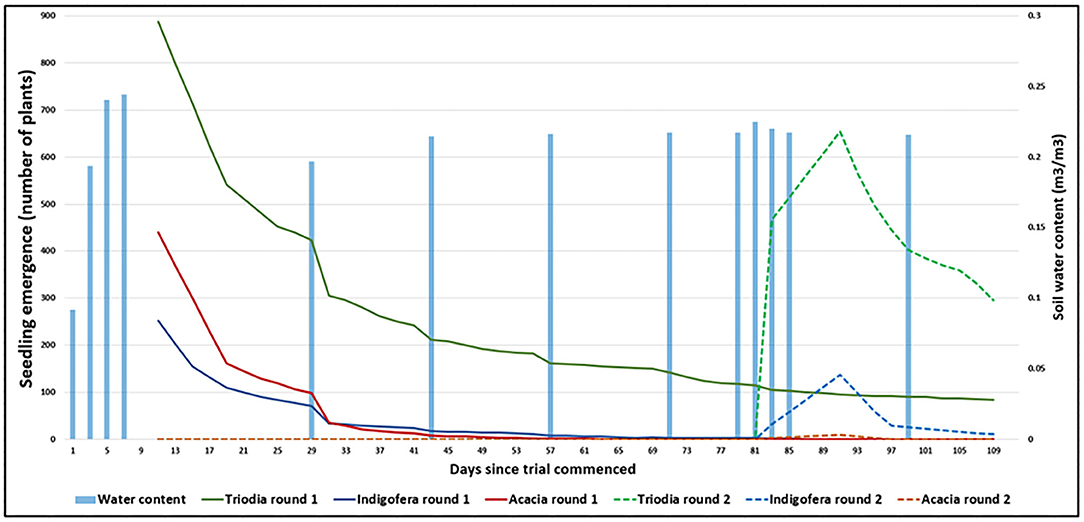
Figure 2. Seedling emergence (peaks) and survival (troughs) over the duration of the experiment (Day 1 = 2nd November 2018). Solid lines refer to the first round of emergence and survival counts, whilst the dashed lines refer to the second round of emergence and survival counts (Triodia = green, Acacia = red, Indigofera = blue). Soil water content (Y2 axis) corresponds to the timing of the simulated rainfall events (blue columns).
Indigofera and Acacia were less successful than Triodia. Only 8% (1.2 of 15 seeds) Indigofera seedlings emerged in the first round and 4% of remaining seeds emerged in the second round. However, only one seedling from the first round and 11 from the second round survived to the end of the experiment. While Acacia emergence was high in the first round (20%, 2 of 10 seeds), only 10 seedlings emerged in the second round, and no seedlings survived the duration of the experiment.
There were no effects of rainfall regime on first-round emergence or cumulative emergence of seedlings (Supplementary Table 2).
The effect of pellet type on first round emergence was significant for Triodia (p ≤ 0.001, Supplementary Table 2) but not for Indigofera or Acacia (Supplementary Table 2). Triodia emergence counts were highest in TS/WM pellets under both rainfall regimes (6.5 ± 0.47 seedlings under 60 mm and 7.6 ± 0.48 seedlings under 120 mm) (Figures 3A,B). Triodia emergence was lowest in the TS+TB pellets under both rainfall regimes (1.6 ± 0.27 seedlings under 60 mm and 1.4 ± 0.25 seedlings under 120 mm). Triodia emergence from TS pellets and TS/WM+TB pellets was not significantly different. Trends for cumulative emergence of Triodia were similar to trends for first round emergence (Figures 3C,D) except cumulative emergence from TS/WM+TB was not significantly different to TS+TB.
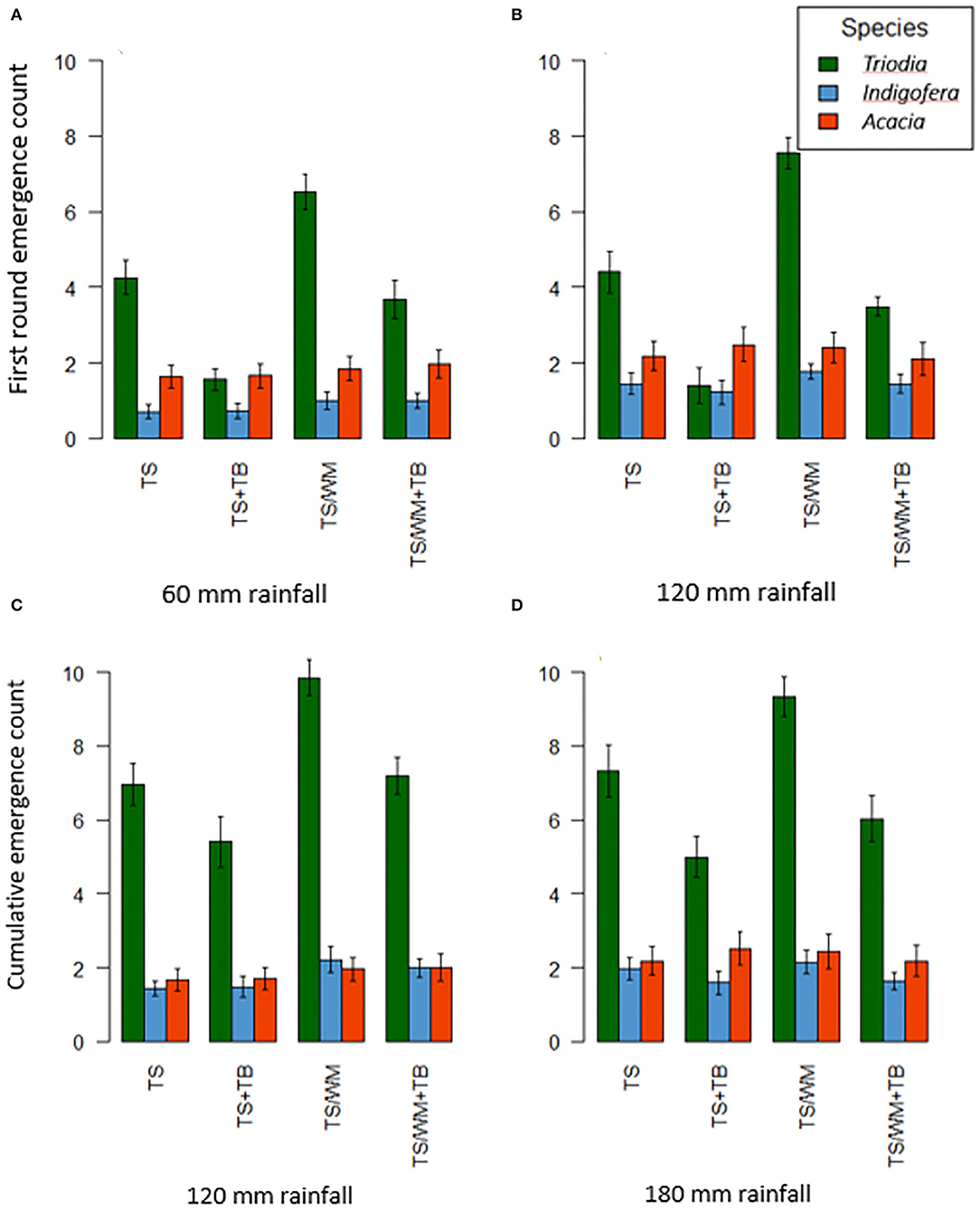
Figure 3. First round seedling emergence counts under the two different rainfall regimes [(A), 60 mm; (B), 120 mm] and cumulative seedling emergence counts after the second simulated rainfall event (i.e., 60 mm) that was applied to all plots (C), 60 + 60 mm; (D), 120 + 60 mm]. Pellet types: TS-−100% topsoil, TS+TB-−100% topsoil with Triodia amendment, TS/WM-−50% blend of topsoil and waste material, TS/WM+TB 50% blended soils with Triodia amendment. Data are means ± SEs; n = 54.
Survival of Indigofera was low and no Acacia seedlings survived the duration of the experiment (Figure 4) so these data were discarded from survival analysis. The experimental factors were not significant on the survival outcome for the first round of Triodia seedlings that emerged, which ranged from 0.15 ± 0.07 seedlings for TS/WM+TB pellets under 120 mm to 0.59 ± 0.14 seedlings for TS pellets under 120 mm. Similarly, rainfall and pellet type did not significantly influence total survival (Supplementary Table 3).
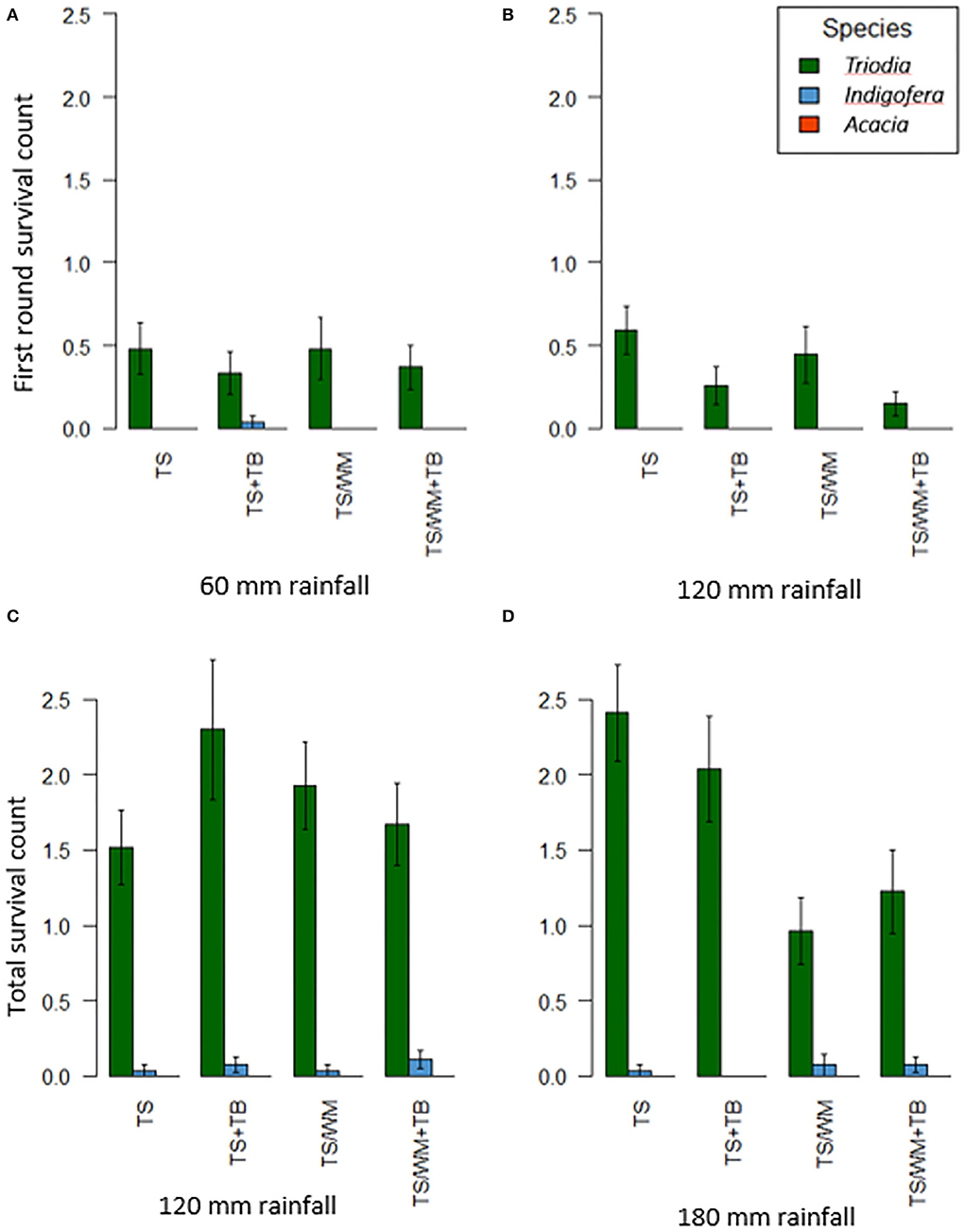
Figure 4. First round survival counts under the two different rainfall regimes (A,B) and total survival counts after the second simulated rainfall event (i.e., 60 mm) that was applied to all plots (C), 60 + 60 mm; (D), 120 + 60 mm). Pellet types: TS−100% topsoil, TS+TB−100% topsoil with Triodia amendment, TS/WM−50% blend of topsoil and waste material, TS/WM+TB 50% blended soils with Triodia amendment. Data are means ± SEs; n = 54.
Plant Aboveground Biomass
Due to low survival of Indigofera and Acacia, above ground biomass data was pooled into a single metric incorporating data for all three species. None of the experimental factors were significant at p < 0.05 and there was a large standard error due to a small number of large individual plants that were evenly distributed across pellet types (Supplementary Figure 2).
Soil Analysis
The nMDS analyses showed that data on initial soil physico-chemical properties split the dataset into pellet types and plots along Axis 1, which correlated with Na, pH and clay among other properties (Figure 5). The split within pellet types and plots along Axis 2 correlated with sand and silt among other properties (Figure 5; Supplementary Table 1).
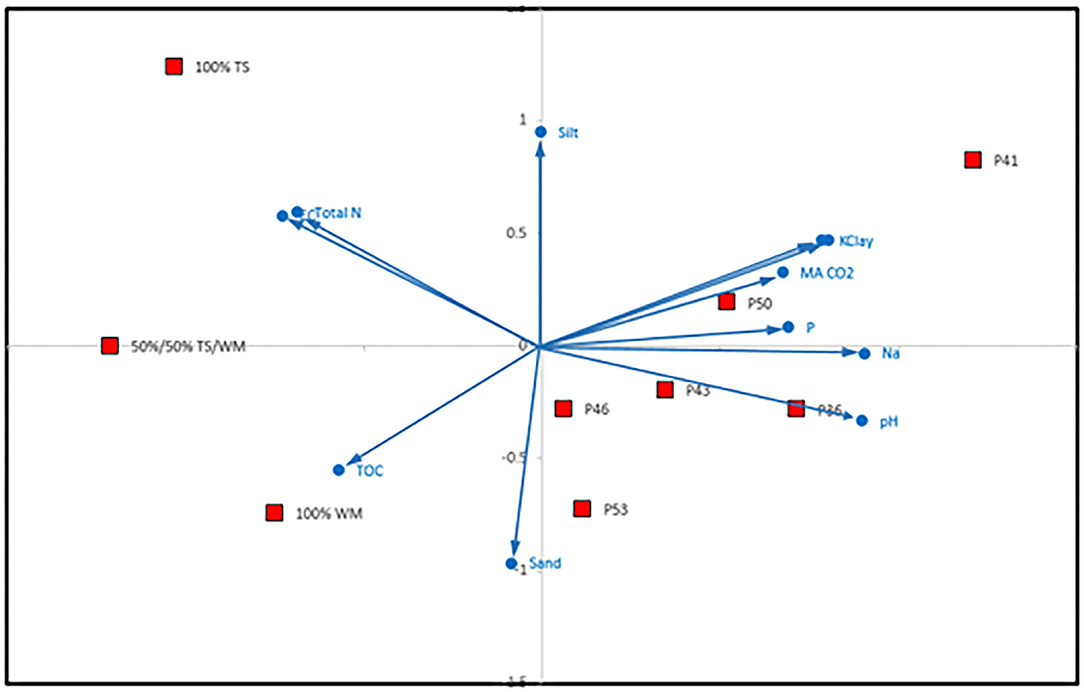
Figure 5. Soil nMDS analysis of plot and pellet ingredients (TS = Topsoil, WM = Waste Material) based on Euclidean distance; stress = 0.03. Pearson correlation coefficients for biplot >0.8. For results of individual soil properties refer to Supplementary Table 1. MA CO2 = Microbial Activity (CO2-C ppm).
At the end of the experiment, substrate soil microbial activity was not significantly affected by rainfall. There were no significant differences detected between pellet types, or between pellets with and without plants. Pellet microbial activity was significantly increased with the addition of Triodia biomass, with 24.3 ± 4.79 mg/kg CO2-C in TS+TB pellets and 32.4 ± 0.75 mg/kg CO2-C in TS/WM+TB pellets when compared to un-amended pellet types (<15 mg/kg CO2-C) (Supplementary Figure 3).
Species (Shannon index) diversity of soil bacterial communities (Figure 6A) was not significantly different across treatments, although pair-wise comparison showed higher diversity (p < 0.05) in the pellets composed by blended soils (topsoil and waste). Species richness differed significantly across treatments (F = 2.1, p < 0.05) with higher OTU counts in the waste substrate compared to other treatments. The dominant phyla across treatments were Proteobacteria and Actinobacteria followed by Firmicutes and Bacteroidetes (Figure 7A). Although samples did not cluster clearly (Figure 7B), the PERMANOVA on Bray-Curtis showed significant (pseudo-F = 2.4, p < 0.005) differences in the taxonomic abundance profiles from different treatments. Soil pellets maintained a bacterial community representative of the original topsoil substrate and showed higher relative abundance of cyanobacteria and proteobacteria compared to the other substrates (Figure 7A). Variability of the diversity indexes within treatments was high, particularly for the topsoil substrate (Figure 6) which showed large differences in bacterial composition across replicated samples (Figure 7A). The addition of biomass did not have a significant effect on the composition of the bacterial communities. However, there was a trend toward an increased proportion of cyanobacteria in both TS_SP+B and TS_P+B treatments compared to TS_SP and TS_P.
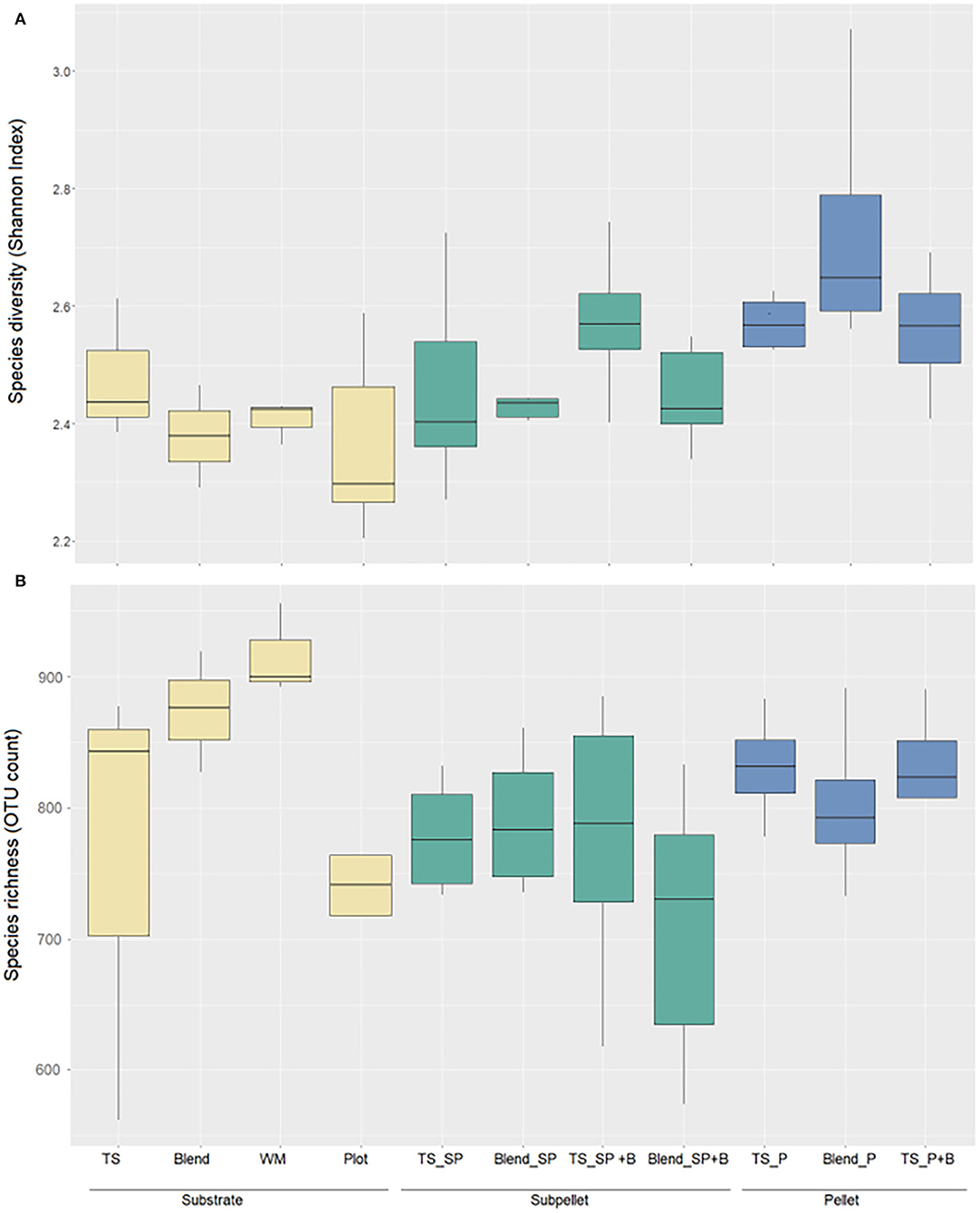
Figure 6. Boxplots of species diversity (Shannon Diversity Index) (A) and species richness [alpha diversity/operational taxonomic units (OTU) Count] (B). Treatments include: TS (topsoil), Blend, and WM (original substrates to make the pellets); Plot (receptor waste soil); TS_SP (topsoil sub-surface pellet), Blend_SP (blend sub-surface pellet), TS_SP +B (topsoil sub-surface with addition of Triodia plant biomass), Blend_SP +B (Blend_SP with Triodia biomass); TS_P (topsoil pellet), Blend_P (blend pellet) and TS_P+B” (TS_P with Triodia biomass).
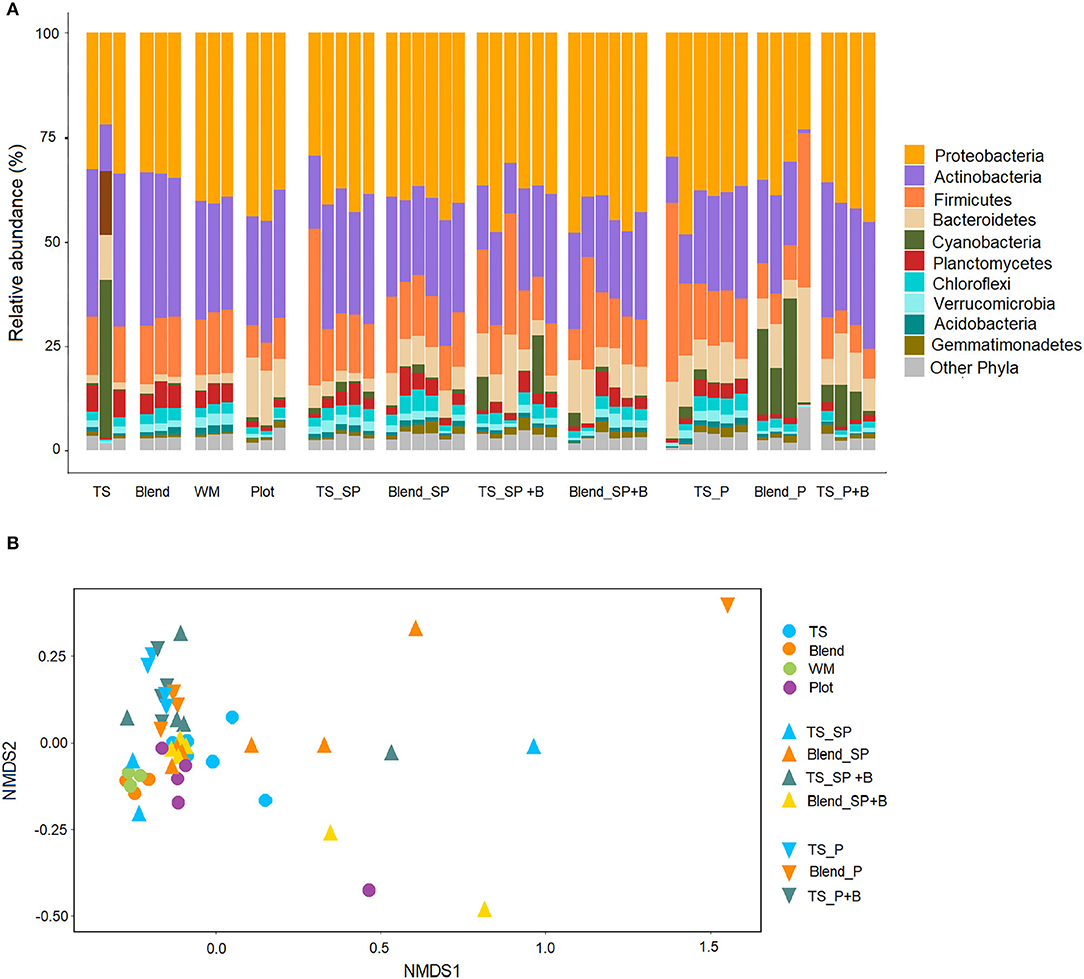
Figure 7. Abundance of bacterial 16S rRNA sequences at the phyla level (A). Stacked bars were created based on the operational taxonomic units (OTUs) generated and to include only the top 10 most abundant phyla. Non-metric, multi-dimensional (NMDS) scaling ordination (confidence level = 0.95) based on Bray-Curtis similarities with 9,999 permutations of bacterial communities (B). Stress level = 0.10. Treatments include: TS (topsoil), Blend, and WM (original substrates to make the pellets); Plot (receptor waste soil); TS_SP (topsoil sub-surface pellet), Blend_SP (blend sub-surface pellet), TS_SP +B (topsoil sub-surface with addition of Triodia plant biomass), Blend_SP +B (Blend_SP with Triodia biomass); TS_P (topsoil pellet), Blend_P (blend pellet) and TS_P+B” (TS_P with Triodia biomass).
Discussion
This experiment demonstrates the potential to field-deploy extruded seed pellets as a new avenue to establish desired native species, likely through improved soil-water access and a more favorable recruitment environment. The pellet type made from 1:1 blend of topsoil and loamy-sand waste material produced the highest seedling emergence. Previous research has demonstrated the suitability of blended topsoil and mine waste materials as comparable growth media to topsoil (Muñoz-Rojas et al., 2016b; Kumaresan et al., 2017; Merino-Martín et al., 2017a; Kneller et al., 2018), but there is growing evidence of the superiority of the blend over 100% topsoil alone (Bateman et al., 2019). Our result could be explained in part by the tendency of the blended pellets to be incorporated into the soil after receiving the simulated rainfall events, which may have provided more optimal conditions (i.e., improved soil-seed-water contact) for germination and emergence (Madsen et al., 2016a,b; Davies et al., 2018). Up to 39% of blended pellets remained in contact with the soil surface, after the first rainfall event. In contrast, up to 13% of TS pellets were physically lifted from the ground as Acacia germinated within and underneath them (Figure 1E). The TS pellets developed cracks round the edge as they dried, forming crusts that were less integrated with the soil substrate (see also Madsen et al., 2018). Hardsetting was most visible for TS pellets, although all pellet types had some degree of hardsetting, which can impede seedling growth as pellets dry (Daniells, 2012). The blended pellets retained their advantage over TS pellets on cumulative emergence as we continually observed a reduction in hardsetting and better integration of TS pellets after the second rainfall event (Figure 1F).
Beyond seedling emergence, survival of Triodia was not impacted by pellet type, except for better survival on TS pellets under the 180 mm cumulative rainfall (i.e., the combined 120 + 60 mm regimes). Survival of Indigofera and Acacia seedlings that emerged from pellets was low or negligible. This is the first experimental test using extruded pelleting technologies for these species. While we have not demonstrated a benefit of pelleting on seedling emergence, the seedlings that did emerge may have had improved access to soil microbes for plant growth. Further research is needed to refine the seed pelleting methodology for Australian species. A clear mandate for research is to overcome pellet hardsetting (e.g., by adding biochar; Gebhardt et al., 2017), which should benefit the large seeded Acacia in particular. Inoculating pellets with native soil microbes such as cyanobacteria or similar (Merritt et al., 2016; Muñoz-Rojas et al., 2018b), may uncover additional benefits of seed enhancement technologies for these legumes. For now, given that seedling emergence is typically limited for surface sown seeds (Erickson et al., 2017; Masarei et al., 2020), our experiment has proved the utility of field-deployed extruded seed pelleting for promoting emergence and rehabilitation of the keystone species, Triodia pungens, and the resident microbial community.
Species-Specific Responses
The transition of seedlings from emergence to establishment has been identified as a major bottleneck to recruitment in the arid zone (James et al., 2011; Lewandrowski et al., 2017a). Our experiment confirms that native seedling emergence is strongly driven by pulse rainfall events (Lewandrowski et al., 2017a). The seed longevity of these species, expressed as time for 50% viability loss during storage at 45°C and 60% RH, has been estimated to be 42 days (Triodia pungens), 101 days (Indigofera monophylla) and 100 days (Acacia inaequilatera; Erickson et al., 2017). Removing the Triodia floret to overcome dormancy rapidly decreases seed persistence in the soil (Lewandrowski et al., 2017a; T. Erickson unpublished data). The pellets may have prolonged the longevity of Triodia seeds as seedlings emerged from pellets 80 days after the onset of the experiment. Beyond the pulse-driven responses to simulated rainfall, the species differed significantly in their responses to the experimental factors.
Triodia emergence was equivalent under both rainfall regimes but showed susceptibility to drought stress. Of the first round Triodia seedlings, 40% became vegetatively dormant at the 6-leaf stage probably due to drought stress, whereas only 23% continued growing past the 20-leaf stage. Barnes (2014) found a change in Triodia leaf anatomical features at the 12- to 20-leaf stage which provided greater physiological capacity to withstand drought by conserving water and slowing growth. Beyond emergence, Triodia recruitment was variable: 9% of first round and 45% of second round seedlings survived to the end of the experiment. Overall, 74% of pellets had one or more surviving Triodia seedling at the end of the experiment. Under field conditions, Triodia seedling recruitment has typically hovered below 10%, but it has been as high as 40% depending largely on rainfall amounts, frequency and duration (Erickson et al., 2017; Lewandrowski et al., 2017a). Taken together, these findings help explain its reliance on repeated significant rainfall events for recruitment (Lewandrowski et al., 2017b).
Acacia seedlings failed to survive under the fortnightly 10 mm simulated follow-up rainfall events under either regime. Indigofera emergence was favored by the higher rainfall regime (120 mm), which suggests sensitivity of this species to rainfall amounts, although seedling survival was unaffected by rainfall regime. Low seedling establishment may be explained in part by the vulnerability of young seedlings to soil water deficits and inadequate follow-up rainfall (Barnes, 2014; Lewandrowski et al., 2017a; Bateman et al., 2018) or early-stage seedling traits that limit their establishment ability under varying moisture conditions (Larson et al., 2020).
Amendment Effects on Microbes and Triodia Seedlings
The addition of carbon substrates in the form of an organic amendment has been found to strongly increase opportunistic microbial activity but the impacts on community composition can take longer to manifest (Bastida et al., 2013; Hueso-González et al., 2018). These findings are consistent with our results: we found a positive effect of the Triodia leaf amendment on microbial activity but no statistically significant effect on community composition. Proteobacteria and actinobacteria tend to prefer topsoil and organic amendments (Bastida et al., 2013), and this preference may have become apparent in our experimental pellets given time. Plant emergence and survival were not correlated with microbial activity, which is also consistent with other studies (Gebhardt et al., 2017; Kneller et al., 2018). Again, these benefits may accrue as plants establish and rehabilitation matures (e.g., Wubs et al., 2016; You et al., 2016). Overall, our findings are that modified extrusion of blended waste material can produce pellets with a microbial community representative of topsoil, which suggests the potential to use pellets to restore microbes and their functions to arid land soils (Wubs et al., 2016), particularly if these communities can move into the recipient rehabilitation soil profile. The addition of organic amendments to pellets increases microbial activity, which may ultimately increase the speed at which soil functionality is restored.
Triodia emergence was inhibited in the pellets with the addition of the Triodia biomass amendment, an unexpected effect. As this response was specific to Triodia, the inhibition is possibly due to the presence of resins generating an allelopathic effect. Triodia pungens resin has bioactive ingredients which can protect timber from termite attack (Amiralian et al., 2014). It was once surmised that the distinctive ring shape formed as adult Triodia plants age may be caused by a growth inhibitor released by decomposing roots (Willis, 2007). Allelopathic effects of adult Triodia pungens plants on its own seedlings have not been documented. Kneller et al. (2018) did not observe any allelopathic effect either in an experiment with Triodia wiseana. Triodia emergence increased in amended pellet types after the second rainfall event, which is consistent with a decreased allelopathic effect with time. More research is needed to confirm allelopathic effects of Triodia plant residues and their implications for recruitment.
Implications for Large-Scale Mine Rehabilitation
Our results suggest that blending topsoil with mine waste material is a prospective strategy to reduce the amount of topsoil needed to achieve large-scale mine rehabilitation. Topsoil is a limited resource, so this finding could make a significant difference to standard practice, which is to spread 0.1–0.2 m3 of topsoil per m2 to achieve a thickness of 10–20 cm (Erickson et al., 2017). In comparison, pelleting in this study used 0.002 m3 of topsoil per m2; a reduction of 100- to 200-fold. If 2–14 pellets are distributed per m2 and one Triodia plant survives per pellet, as observed in our experiment, then a range of grass densities analogous to that in adjacent reference systems will be restored (Shackelford et al., 2018). Field-deployed extruded seed pellets show promise as a viable alternative to spreading topsoil and broadcasting seeds to recruit Triodia pungens. The method could be further refined to increase seed use efficiency.
Overall, bacterial diversity measured as species evenness (Shannon diversity) did not differ across treatments but there were significant differences in the relative abundance of bacteria taxa across pellets, sub-pellets and substrates. The reduced amount of topsoil in the blended pellets, compared with topsoil only pellets, did not appear to alter the relative abundance and composition of the bacterial community. We obtained a higher relative abundance of Cyanobacteria, compared to the waste, which suggests the effective translocation of these bacteria from the topsoil. Cyanobacteria, which are pioneer colonizers of soil biocrust in arid systems, have a critical role in nitrogen and carbon fixation and can assist in stabilizing and recovering soil functions in rehabilitation sites (Muñoz-Rojas et al., 2018b). Recent technological developments for cyanobacteria inoculation have demonstrated the viability of pelletised cyanobacteria after drying and storage processes (Román et al., 2020).
Commercially available microbes have been tested as potential fertilizer for improving plant performance in plant substrates, but often result in poor soil bacterial diversity, and may even be detrimental to plants in restoration (Moreira-Grez et al., 2019). By contrast with introduced exogenous microbes, translocated native soil microorganisms can positively interact with arid native plants promoting seedling growth of Pilbara species, e.g., Triodia spp. and Acacia spp. (Chua et al., 2019). As shown here, modified extruded seed pellets appear to be a viable method to translocate beneficial soil microbial communities from the previously removed topsoil, such as biocrust cyanobacteria, even after a short period (109 days) following inoculation.
Additional factors to be considered in scaling up the methodology include tests of pellet performance on sloped rehabilitation sites under heavy rainfall. Torrential rainfall associated with summer storms can erode soil and other growth media from sloped rehabilitation sites. Rock armouring can reduce erosion (Golos et al., 2019) and recruitment may be enhanced if pellets were placed in protected microsites. Location of seeds within pellets is similarly critical to seedling emergence and survival. An experiment using dungpats found that seedling survival was highest when seeds were located at the periphery and 0.5 cm from the bottom of pats (Gökbulak, 2009). We found seeds that germinated on the top or bottom of pellets were unable to emerge successfully due to inadequate moisture retention and hardsetting, respectively. Amendments to reduce hardsetting, such as gypsum or biochar, may improve seedling emergence from these locations (Madsen et al., 2016b; Bateman et al., 2019). Taken together, these observations are consistent with previous findings that seed placement is critical for successful seedling recruitment in arid land restoration (Erickson et al., 2017; Merino-Martín et al., 2017b; Golos et al., 2019; Masarei et al., 2020). Pellets have the distinct advantage of reducing surface exposure compared with broadcast seeding, which is a major cause of low seedling emergence in these restoration settings (Merritt and Dixon, 2011; James et al., 2013). Our findings for mine site rehabilitation in the Pilbara region are likely to be applicable to arid land restoration elsewhere, and particularly for regions where topsoil resources are limited, surface sown seeds have consistently failed to recruit, and seed supplies are limited.
Data Availability Statement
The raw data supporting the conclusions of this article will be made available by the authors, without undue reservation.
Author Contributions
TE and MM-R conceived the research. ES, TE, MM-R, RB, and RS designed the research. ES executed the experiment. MM-R contributed the soil microbial data. ES, MM-R, and RS analyzed the data. ES, TE, MM-R, RB, and RS wrote and edited manuscript. All authors contributed to the article and approved the submitted version.
Funding
This research was completed as part of the Global Innovation Linkages Project (GIL53873) Eco-engineering solutions to improve mine-site rehabilitation outcomes awarded to TE by the Department of Industry, Innovation and Science, Canberra, Australia; the Hermon Slade Foundation (HSF1808) awarded to MM-R and TE; and the Australian Research Council Discovery Early Career Research Award DE180100570 awarded to MM-R. Funding for travel for RS was kindly donated by Rosemary Grigg and Peter Flanigan.
Conflict of Interest
The authors declare that the research was conducted in the absence of any commercial or financial relationships that could be construed as a potential conflict of interest.
Acknowledgments
We acknowledge and pay respect to the Nyiyaparli Traditional Owners of the land on which our experiment was established. We thank Angela M. Chilton and Nathali M. Machado de Lima for her support with the bioinformatic analyses. Thanks also to Scott Abella and Philip Ladd for comments on the draft manuscript.
Supplementary Material
The Supplementary Material for this article can be found online at: https://www.frontiersin.org/articles/10.3389/fevo.2020.576125/full#supplementary-material
References
Amiralian, N., Annamalai, P. K., Fitzgerald, C., Memmott, P., and Martin, D. J. (2014). Optimisation of resin extraction from an Australian arid grass “Triodia pungens” and its preliminary evaluation as an anti-termite timber coating. Ind. Crops Prod. 59, 241–247. doi: 10.1016/j.indcrop.2014.04.045
Barnes, M. (2014). Seedling transition phases define drought susceptibility in a semi-arid grass, Triodia pungens (Honours Dissertation). Perth, WA: The University of Western Australia.
Bastida, F., Hernández, T., Albaladejo, J., and García, C. (2013). Phylogenetic and functional changes in the microbial community of long-term restored soils under semiarid climate. Soil Biol. Biochem. 65, 12–21. doi: 10.1016/j.soilbio.2013.04.022
Bastida, F., Kandeler, E., Moreno, J., Ros, M., García, C., and Hernández, T. (2008). Application of fresh and composted organic wastes modifies structure, size and activity of soil microbial community under semiarid climate. Appl. Soil Ecol. 40, 318–329. doi: 10.1016/j.apsoil.2008.05.007
Bateman, A., Lewandrowski, W., Stevens, J. C., and Muñoz-Rojas, M. (2018). Ecophysiological indicators to assess drought responses of arid zone native seedlings in reconstructed soils. Land Degrad. Dev. 29, 984–993. doi: 10.1002/ldr.2660
Bateman, A. M., Erickson, T. E., Merritt, D. J., and Muñoz-Rojas, M. (2019). Inorganic soil amendments alter seedling performance of native plant species in post-mining arid zone rehabilitation. J. Environ. Manage. 241, 179–186. doi: 10.1016/j.jenvman.2019.04.022
BoM (2019). Climate Data Online. Melbourne, VIC: Australian Government Bureau of Meteorology. Available online at: http://www.bom.gov.au/climate/data/ (accessed May 7, 2019).
Bowker, M. A. (2007). Biological soil crust rehabilitation in theory and practice: an underexploited opportunity. Restor. Ecol. 15, 13–23. doi: 10.1111/j.1526-100X.2006.00185.x
Brown, V. S., Ritchie, A., Stevens, J. C., Harris, R. J., Madsen, M. D., and Erickson, T. E. (2018). Protecting direct seeded grasses from herbicide application: can new extruded pellet formulations be used in restoring natural plant communities? Restor. Ecol. 27, 488–494. doi: 10.1111/rec.12903
Chua, M., Erickson, T. E., Merritt, D. J., Chilton, A. M., Ooi, M. K., and Muñoz-Rojas, M. (2019). Bio-priming seeds with cyanobacteria: effects on native plant growth and soil properties. Restor. Ecol. 28, S168–S176. doi: 10.1111/rec.13040
Clenet, D. R., Davies, K. W., Johnson, D. D., and Kerby, J. (2019). Native seeds incorporated into activated carbon pods applied concurrently with indaziflam: a new strategy for restoring annual-invaded communities? Restor. Ecol. 27, 738–744. doi: 10.1111/rec.12927
Davies, K., Boyd, C., Madsen, M., Kerby, J., and Hulet, A. (2018). Evaluating a seed technology for sagebrush restoration across an elevation gradient: support for bet hedging. Rangeland Ecol. Manage. 71, 19–24. doi: 10.1016/j.rama.2017.07.006
De Falco, L. A., Esque, T. C., Nicklas, M. B., and Kane, J. M. (2012). Supplementing seed banks to rehabilitate disturbed Mojave Desert shrublands: where do all the seeds go? Restor. Ecol. 20, 85–94. doi: 10.1111/j.1526-100X.2010.00739.x
EPA (2017). Environmental Protection Authority Annual Report 2016–17. Perth, WA: The Government of Western Australia. Available online at: http://www.epa.wa.gov.au/sites/default/files/Annual_reports/EPA%20Annual%20Report%202016-2017.pdf (accessed June 18, 2020).
Erickson, T. E., Barrett, R. L., Merritt, D. J., and Dixon, K. W. (2016a). Pilbara Seed Atlas and Field Guide: Plant Restoration in Australia's Arid Northwest. Dickson, ACT: CSIRO Publishing. doi: 10.1071/9781486305537
Erickson, T. E., Merritt, D. J., and Turner, S. R. (2016c). Overcoming physical seed dormancy in priority native species for use in arid-zone restoration programs. Aust. J. Bot. 64, 401–416. doi: 10.1071/BT16059
Erickson, T. E., Munoz-Rojas, M., Guzzomi, A. L., Masarei, M., Ling, E., Bateman, A. M., et al. (2019). “A case study of seed-use technology development for Pilbara mine site rehabilitation,” in 13th International Conference on Mine Closure, eds A.B. Fourie, and M. Tibbett (Perth, WA: Australian Centre for Geomechanics), 679–692. doi: 10.36487/ACG_rep/1915_54_Erickson
Erickson, T. E., Muñoz-Rojas, M., Kildisheva, O. A., Stokes, B. A., White, S. A., Heyes, J. L., et al. (2017). Benefits of adopting seed-based technologies for rehabilitation in the mining sector: a Pilbara perspective. Aust. J. Bot. 65, 646–660. doi: 10.1071/BT17154
Erickson, T. E., Shackelford, N., Dixon, K. W., Turner, S. R., and Merritt, D. J. (2016b). Overcoming physiological dormancy in seeds of Triodia (Poaceae) to improve restoration in the arid zone. Restor. Ecol. 24, S64–S76. doi: 10.1111/rec.12357
Ford, D., Cookson, W., Adams, M., and Grierson, P. (2007). Role of soil drying in nitrogen mineralization and microbial community function in semi-arid grasslands of north-west Australia. Soil Biol. Biochem. 39, 1557–1569. doi: 10.1016/j.soilbio.2007.01.014
Gebhardt, M., Fehmi, J. S., Rasmussen, C., and Gallery, R. E. (2017). Soil amendments alter plant biomass and soil microbial activity in a semi-desert grassland. Plant Soil 419, 53–70. doi: 10.1007/s11104-017-3327-5
Gökbulak, F. (2009). Influence of seed location and planting depth on seedling establishment in cattle dungpats. Grassland Sci. 55, 23–28. doi: 10.1111/j.1744-697X.2009.00133.x
Golos, P. J., Commander, L. E., and Dixon, K. W. (2019). The addition of mine waste rock to topsoil improves microsite potential and seedling emergence from broadcast seeds in an arid environment. Plant Soil 440, 71–84. doi: 10.1007/s11104-019-04060-7
Hallett, L. M., Standish, R. J., and Hobbs, R. J. (2011). Seed mass and summer drought survival in a Mediterranean-climate ecosystem. Plant Ecol. 212:1479. doi: 10.1007/s11258-011-9922-2
Haney, R. L., and Haney, E. B. (2010). Simple and rapid laboratory method for rewetting dry soil for incubations. Commun. Soil Sci. Plant Anal. 41, 1493–1501. doi: 10.1080/00103624.2010.482171
Hueso-González, P., Muñoz-Rojas, M., and Martínez-Murillo, J. (2018). The role of organic amendments in drylands restoration. Curr. Opin. Environ. Sci. Health 5, 1–6. doi: 10.1016/j.coesh.2017.12.002
James, J. J., Sheley, R. L., Erickson, T., Rollins, K. S., Taylor, M. H., Dixon, K. W., et al. (2013). A systems approach to restoring degraded drylands. J. Appl. Ecol. 50, 730–739. doi: 10.1111/1365-2664.12090
James, J. J., Svejcar, T. J., and Rinella, M. J. (2011). Demographic processes limiting seedling recruitment in arid grassland restoration. J. Appl. Ecol. 48, 961–969. doi: 10.1111/j.1365-2664.2011.02009.x
Klindworth, A., Pruesse, E., Schweer, T., Peplies, J., Quast, C., Horn, M., et al. (2013). Evaluation of general 16S ribosomal RNA gene PCR primers for classical and next-generation sequencing-based diversity studies. Nucleic Acids Res. 41:e1. doi: 10.1093/nar/gks808
Kneller, T., Harris, R. J., Bateman, A., and Muñoz-Rojas, M. (2018). Native-plant amendments and topsoil addition enhance soil function in post-mining arid grasslands. Sci. Total Environ. 621, 744–752. doi: 10.1016/j.scitotenv.2017.11.219
Kumaresan, D., Cross, A. T., Moreira-Grez, B., Kariman, K., Nevill, P., Stevens, J. C., et al. (2017). Microbial functional capacity is preserved within engineered soil formulations used in mine site restoration. Sci. Rep. 7:564. doi: 10.1038/s41598-017-00650-6
Larson, J. E., Anacker, B. L., Wanous, S., and Funk, J. L. (2020). Ecological strategies begin at germination: traits, plasticity, and survival in the first four days of plant life. Funct. Ecol. 34, 968–979. doi: 10.1111/1365-2435.13543
Lewandrowski, W., Erickson, T. E., Dalziell, E. L., and Stevens, J. C. (2017b). Ecological niche and bet-hedging strategies for Triodia (R. Br.) seed germination. Ann. Bot. 121, 367–375. doi: 10.1093/aob/mcx158
Lewandrowski, W., Erickson, T. E., Dixon, K. W., and Stevens, J. C. (2017a). Increasing the germination envelope under water stress improves seedling emergence in two dominant grass species across different pulse rainfall events. J. Appl. Ecol. 54, 997–1007. doi: 10.1111/1365-2664.12816
Madsen, M. D., Davies, K. W., Boyd, C. S., Kerby, J. D., and Svejcar, T. J. (2016a). Emerging seed enhancement technologies for overcoming barriers to restoration. Restor. Ecol. 24, S77–S84. doi: 10.1111/rec.12332
Madsen, M. D., Davies, K. W., Williams, C. J., and Svejcar, T. J. (2012). Agglomerating seeds to enhance native seedling emergence and growth. J. Appl. Ecol. 49, 431–438. doi: 10.1111/j.1365-2664.2012.02118.x
Madsen, M. D., Hulet, A., Phillips, K., Staley, J. L., Davies, K. W., and Svejcar, T. J. (2016b). Extruded seed pellets: a novel approach for enhancing sagebrush seedling emergence. Native Plants J. 17, 230–243. doi: 10.3368/npj.17.3.230
Madsen, M. D., Svejcar, L. N., Radke, J., and Hulet, A. (2018). Inducing rapid seed germination of native cool season grasses with solid matrix priming and seed extrusion technology. PLoS ONE 13:e0204380. doi: 10.1371/journal.pone.0204380
Masarei, M., Astfalck, L. C., Guzzomi, A. L., Merritt, D. J., and Erickson, T. E. (2020). Soil rock content influences the maximum seedling emergence depth of a dominant arid zone grass. Plant Soil 450, 497–509. doi: 10.1007/s11104-020-04493-5
McKenzie, N., van Leeuwen, S., and Pinder, A. (2009). Introduction to the Pilbara biodiversity survey, 2002–2007. Rec. West. Aust. Mus. Suppl. 78, 3–89. doi: 10.18195/issn.0313-122x.78(1).2009.003-089
Mercer-Mapstone, L., Rifkin, W., Louis, W. R., and Moffat, K. (2018). Company-community dialogue builds relationships, fairness, and trust leading to social acceptance of Australian mining developments. J. Clean. Prod. 184, 671–677. doi: 10.1016/j.jclepro.2018.02.291
Merino-Martín, L., Commander, L., Mao, Z., Stevens, J. C., Miller, B. P., Golos, P. J., et al. (2017a). Overcoming topsoil deficits in restoration of semiarid lands: designing hydrologically favourable soil covers for seedling emergence. Ecol. Eng. 105, 102–117. doi: 10.1016/j.ecoleng.2017.04.033
Merino-Martín, L., Courtauld, C., Commander, L., Turner, S., Lewandrowski, W., and Stevens, J. C. (2017b). Interactions between seed functional traits and burial depth regulate germination and seedling emergence under water stress in species from semi-arid environments. J. Arid Environ. 147, 25–33. doi: 10.1016/j.jaridenv.2017.07.018
Merritt, D. J., and Dixon, K. W. (2011). Restoration seed banks—a matter of scale. Science 332, 424–425. doi: 10.1126/science.1203083
Merritt, D. J., Golos, P. J., and Erickson, T. E. (2016). “A systematic approach to seed management for restoration”, in Pilbara Seed Atlas and Field Guide: Plant Restoration in Australia's Arid Northwest, eds T. E. Erickson, R. L. Barrett, D. J. Merritt, and K. W. Dixon (Dickson, ACT: CSIRO Publishing), 35–42.
Moreira-Grez, B. A., Muñoz-Rojas, M., Kariman, K., Storer, P., O'Donnell, A. G., Kumaresan, D., et al. (2019). Reconditioning degraded mine site soils with exogenous soil microbes: plant fitness and soil microbiome outcomes. Front. Microbiol. 10:1617. doi: 10.3389/fmicb.2019.01617
Muñoz-Rojas, M., Chilton, A., Liyanage, G., Erickson, T. E., Merritt, D., Neilan, B., et al. (2018b). Effects of indigenous soil cyanobacteria on seed germination and seedling growth of arid species used in restoration. Plant Soil 429, 91–100. doi: 10.1007/s11104-018-3607-8
Muñoz-Rojas, M., Erickson, T. E., Dixon, K. W., and Merritt, D. J. (2016a). Soil quality indicators to assess functionality of restored soils in degraded semiarid ecosystems. Restor. Ecol. 24, S43–S52. doi: 10.1111/rec.12368
Muñoz-Rojas, M., Erickson, T. E., Martini, D. C., Dixon, K. W., and Merritt, D. J. (2016b). Climate and soil factors influencing seedling recruitment of plant species used for dryland restoration. Soil 2, 287–298. doi: 10.5194/soil-2-287-2016
Muñoz-Rojas, M., Román, J., Roncero-Ramos, B., Erickson, T. E., Merritt, D. J., Aguila-Carricondo, P., et al. (2018a). Cyanobacteria inoculation enhances carbon sequestration in soil substrates used in dryland restoration. Sci. Total Environ. 636, 1149–1154. doi: 10.1016/j.scitotenv.2018.04.265
Nielsen, S., Walburn, J. W., Verges, A., Thomas, T., and Egan, S. (2017). Microbiome patterns across the gastrointestinal tract of the rabbitfish Siganus fuscescens. PeerJ. 5:e3317. doi: 10.7717/peerj.3317
Oksanen, J., Blanchet, F. G., Friendly, M., Kindt, R., Legendre, P., McGlinn, D., et al. (2017). vegan: Community Ecology Package, 2.4-2. R Package.
R Core Team (2018). R: A Language and Environment for Statistical Computing. Vienna: R Foundation for Statistical Computing. Available online at: http://www.R-project.org/ (accessed September 11, 2018).
Ritchie, A. L., Erickson, T. E., and Merritt, D. J. (2017). Monitoring of plant phenology and seed production indentifies two distinct seed collection seasons in the Australian arid zone. Rangeland J. 39, 73–83. doi: 10.1071/RJ16050
Ritchie, A. L., Stevens, J. C., and Erickson, T. E. (2020). Developing extruded seed pellets to overcome soil hydrophobicity and seedling emergence barriers. Ecol. Sol. Evid. 1:e12024. doi: 10.1002/2688-8319.12024
Román, J. R., Chilton, A. M., Cantón, Y., and Muñoz-Rojas, M. (2020). Assessing the viability of cyanobacteria pellets for application in arid land restoration. J. Environ. Manage. 270:110795. doi: 10.1016/j.jenvman.2020.110795
Román, J. R., Roncero-Ramos, B., Chamizo, S., Rodríguez-Caballero, E., and Cantón, Y. (2018). Restoring soil functions by means of cyanobacteria inoculation: Importance of soil conditions and species selection. Land Degrad. Dev. 29, 3184–3193. doi: 10.1002/ldr.3064
Schwinning, S., and Sala, O. E. (2004). Hierarchy of responses to resource pulses in arid and semi-arid ecosystems. Oecologia 141, 211–220. doi: 10.1007/s00442-004-1520-8
Shackelford, N., Miller, B. P., and Erickson, T. E. (2018). Restoration of open-cut mining in semi-arid systems: a synthesis of long-term monitoring data and implications for management. Land Degrad. Dev. 29, 994–1004. doi: 10.1002/ldr.2746
Wang, Q., Garrity, G. M., Tiedje, J. M., and Cole, J. R. (2007). Naive bayesian classifier for rapid assignment of rRNA sequences into the new bacterial taxonomy. Appl. Environ. Microbiol. 73, 5261–5267. doi: 10.1128/AEM.00062-07
Williams, W., Schneemilch, M., Chilton, A., Williams, S., and Neilan, B. (2017). Microbial biobanking–cyanobacteria-rich topsoil facilitates mine rehabilitation. Biogeosciences 16, 2189–2204. doi: 10.5194/bg-16-2189-2019
Wu, S., Liu, Y., Bougoure, J. J., Southam, G., Chan, T.-S., Lu, Y.-R., Haw, S.-C., et al. (2019). Organic matter amendment and plant colonization drive mineral weathering, organic carbon sequestration and water stable aggregation in magnetite Fe ore tailings. Environ. Sci. Technol. 53, 13720–13731. doi: 10.1021/acs.est.9b04526
Wubs, E. J., Van Der Putten, W. H., Bosch, M., and Bezemer, T. M. (2016). Soil inoculation steers restoration of terrestrial ecosystems. Nat. Plants 2:16107. doi: 10.1038/nplants.2016.107
Keywords: dryland restoration, mine closure, Pilbara, seed enhancement technologies, soil microbes, spinifex, Triodia, leguminous shrub
Citation: Stock E, Standish RJ, Muñoz-Rojas M, Bell RW and Erickson TE (2020) Field-Deployed Extruded Seed Pellets Show Promise for Perennial Grass Establishment in Arid Zone Mine Rehabilitation. Front. Ecol. Evol. 8:576125. doi: 10.3389/fevo.2020.576125
Received: 25 June 2020; Accepted: 19 November 2020;
Published: 10 December 2020.
Edited by:
Orsolya Valkó, Hungarian Academy of Science, HungaryReviewed by:
Hermann Heilmeier, Freiberg University of Mining and Technology, GermanyRenaud Jaunatre, Université Grenoble Alpes, France
Copyright © 2020 Stock, Standish, Muñoz-Rojas, Bell and Erickson. This is an open-access article distributed under the terms of the Creative Commons Attribution License (CC BY). The use, distribution or reproduction in other forums is permitted, provided the original author(s) and the copyright owner(s) are credited and that the original publication in this journal is cited, in accordance with accepted academic practice. No use, distribution or reproduction is permitted which does not comply with these terms.
*Correspondence: Rachel J. Standish, r.standish@murdoch.edu.au