Micro- and nanoplastics current status: legislation, gaps, limitations and socio-economic prospects for future
- 1Department of Basic and Applied Sciences, Tonalá University Center (CUT, Centro Universitario de Tonalá -in Spanish-), Universidad de Guadalajara, Tonalá, Jalisco, Mexico
- 2Universidad de Guadalajara, Guadalajara, Mexico
- 3Tonalá University Center (CUT, Centro Universitario de Tonalá -in Spanish-), Universidad de Guadalajara, Tonalá, Mexico
The pollution caused by micro- (MP) and nanoplastics (NP) in the planet’s ecosystems has gained significant interest in recent years due to their environmental impact and effects on the health of living organisms. Given this, it is necessary to conduct a comprehensive analysis of the actions required to mitigate their impacts. This paper analyzes existing legislation across different countries and regions, including Europe, North America, China, Russia, India, Brazil, Mexico, and the global initiatives undertaken by the United Nations. Furthermore, it highlights the need for additional measures to mitigate the impact of MP/NP in future years, such as the development of technologies for the separation or degradation of these particles in water intended for human consumption and in wastewater treatment plant effluents, studying plastic particulate material in the air considering meteorological parameters, MP/NP detection protocols in human fluid samples, creating truly biodegradable polymers for use as bioplastics, and establishing institutions responsible for the management of plastic waste. The study also shows the current state of abundance (characterization and quantification) of MP/NP in different environmental matrices based on reports from recent years, and identifies key research opportunities and actions required to evaluate the risks and toxicity associated with MP/NP. Socio-economic aspects are considered, including the impact of MP/NP on different regions, by associating economic and human wellness parameters to plastic waste generation by using available data from 148 countries. As result of this analysis, both the most populated and developed countries contribute to MP/NP generation, however, they have different capacities to address this problem due to social circumstances. The solution to this problem requires efforts from authorities, industry, the scientific community, and the active participation of the population, then, resolving social, political, and economic issues between countries and regions of the world is necessary.
Introduction
Plastics were invented in 1860 from organic polymers derived from fossil hydrocarbons. However, their demand and manufacturing began to increase since 1940, replacing common materials up to that time, such as wood, metals, ceramics, leather, glass, paper, and vegetable fibers, among others (Khairul Anuar et al., 2022). Today, plastics have become a feedstock in all industries, from textiles and automotive to food, due to their physical and chemical properties, such as low weight, heat resistance, translucency or transparency, malleability, and hardness (Maurya et al., 2020).
The global manufacture of plastics has been increasing year after year. It has been estimated that in 1950, 1.7 million tons (MT) were produced, while in 2018, its production reached 359 MT, with 17% of the total attributed to Europe, 18% to North America, and 51% to Asia (Worm et al., 2017; Plastics Europe, 2019). Currently, 40% of the world’s plastics production corresponds to the packaging industry, 20% to articles of common use, 20% to construction, and the remaining 20% to the agricultural, electrical, and automotive sectors (Oliveira et al., 2020). By 2017, it has been estimated that over 8000 MT of plastic had been manufactured cumulatively worldwide, with 2500 MT currently in use. It has also been calculated that up to 2015, 6300 MT of plastic waste were generated, with 800 MT being incinerated, 600 MT being recycled, and approximately 4900 MT being disposed of and accumulated in landfills or natural environments (Geyer et al., 2017). In 2019, 31 transnational companies reported the amount of plastic generated as a result of their commercial activities. Coca-Cola reported an annual world production of 88,000 million (M) single-use plastic bottles, equivalent to 3 MT per year, followed by Nestle with 1.7 MT and Danone with 0.73 MT (Schächtele, 2020). Therefore, in addition to the large amount of plastic waste generated by the population, it is evident that the industry also contributes significantly.
Undoubtedly, plastics have improved the quality of life of the population, bringing with them facilities and comforts in daily and work activities, including devices and equipment that improve human health and extend longevity. However, their high production and inadequate disposal have made them the focus of attention in recent years due to being a source of micro- and nanoplastics (MP/NP) and having negative impacts on ecosystems and health. In this mini-review, the dispersion of MP/NP through different ecosystems, MP/NP quantification in different matrices, current international legislation, synergism of MP/NP with other emerging pollutants, gaps, study opportunities, and future actions are analyzed. Additionally, social, and economic aspects from 148 countries are evaluated to determine the correlation between the economy or productivity, human wellness, and the generation of plastic waste that leads to the formation of MP/NP. All these perspectives aim to contribute to solving the impact of MP/NP in future years.
Materials and methods
To develop this work, more than 160 different sources were analyzed: Papers published recently and from a few years ago, some specialized books and book chapters, databases of non-governmental organizations such as the WHO, World Bank, World Economic Forum, OECD and UN platforms, international press reports, as well as government and official internet sites from several countries. The articles consulted were found in different databases using keywords such as microplastics, nanoplastics, plastics, mismanaged plastics, legislation, pollution, airborne, soil, water, health, toxicological effects, environment, formation, applications. On the other hand, legislation on plastics and microplastics was analyzed by accessing directly on government official sites of the most economically important countries; so that all this information allowed us to conclude on the studies and actions required to solve the problem from social, economic, scientific and health perspectives.
Origin and formation of micro- and nanoplastics
Although resistance to heat and lightness makes plastics materials with great advantages in the manufacture of useful products, these properties make plastics a problem when they become waste in the environment since their biodegradation is technically impossible under normal conditions in nature (Khairul Anuar et al., 2022). As a result of their widespread use, plastics can remain as waste easily visible to the human eye (macroplastics). However, in addition to the negative impact that these macroplastics cause in ecosystems, there is another potential issue derived from these materials: invisible plastic particles that are also difficult to break down and are classified as emerging pollutants. These plastic particles can be classified based on their size, chemical nature, and the way in which they are produced or released into the environment.
There is no official international agreement for their size classification; however, considering at least one of their dimensions, plastic particles can be classified as microplastics (MP) with a size between 1 µm and 5 mm, and nanoplastics (NP), with a size smaller than 100 or 1000 nm (Yang et al., 2021; Schröter and Ventura, 2022). The chemical nature of plastic particles and materials depends on the monomers from which they are formed: monomers linked in a linear or branched fashion (methyl, phenyl, fluorinated, or chlorinated groups) linked through nonpolar C-C or C-O covalent bonds. Examples of these are polyethylene (PE), polypropylene (PP), polystyrene (PS), polytetrafluoroethylene (PTFE), polyvinyl chloride (PVC), and polyethylene terephthalate (PET). Therefore, MP/NP represent a challenge in characterization and detection because they have different chemical structures, shapes, and physical properties.
Considering the way they are produced, these particles can be primary or secondary. Primary MP/NP are manufactured in small sizes, generally intended for medical applications (such as sensors, implant coatings, drug delivery), and personal care products (added to soaps, cosmetics, toothpastes, scrubs). On the other hand, secondary MP/NP are produced as a result of friction, abrasion, degradation, fragmentation, and/or erosion of larger particles and/or large pieces of plastic (Andrady, 2011; Auta et al., 2017). These particles are generated from bags, wrappers, toys, containers, single-use utensils, synthetic clothing fibers, tubes, paints, household and industrial abrasive products, beauty and hygiene products, among others. However, they can also come from thermosetting materials, from the abrasion and weathering of tires, road signs, and marine coatings. It has been estimated that the concentration of secondary MP/NP in the environment is higher than the concentration of primary MP/NP (Boucher and Friot, 2017).
Secondary microplastics/nanoplastics (MP/NP) are generated from the degradation of larger plastic objects (macroplastics). Degradation can be classified as abiotic and biotic degradation (biodegradation). Abiotic degradation occurs due to environmental physical and chemical effects (temperature, UV radiation, wind, waves), while biodegradation occurs due to the effect of microorganisms or enzymes (Restrepo-Florez et al., 2014) only under controlled temperature conditions (25°C–37°C), in the presence of suitable media (saline aqueous solutions), enzymes, or microorganisms capable of degrading these polymers. The advantage of biodegradation lies in the mineralization and transformation of plastics into simple organic compounds that can be incorporated into the cycles of elements (carbon, nitrogen, sulfur); however, its disadvantage lies in the long times required (up to 6 months). In addition, microorganisms with this capacity are not found in all ecosystems (Banker et al., 2016). Abiotic degradation can be classified into thermal degradation (due to high temperatures inducing structural changes), mechanical degradation (due to the action of mechanical forces physically breaking the material, such as wind and waves), and chemical degradation (Li et al., 2004; Chen et al., 2018).
Chemical degradation can be classified as photodegradation, thermo-oxidation, and photo-oxidation. Photodegradation is the modification of plastics by solar radiation (such as discoloration, brittle surfaces, decrease in mechanical resistance). Thermo-oxidation consists of the degradation of plastics due to the action of light, heat, or chemical attacks from the reaction with oxidizing species, causing the loss of their properties. Finally, photo-oxidation consists of the generation of oxidizing radicals from UV light (Pospisil et al., 2006; Gardette et al., 2013). MP/NP are generated from the chemical degradation of plastic waste when it floats in the sea or in continental water, in sediments or in soils, and is exposed to solar radiation, facilitating the oxidative degradation of its structural polymers. The largest amount of MP/NP is generated from commonly used plastic articles and products that are irresponsibly disposed of in the environment.
Another common source of MP/NP generation is from the washing of clothes and synthetic fabrics (plastic fibers). It has been reported that during a wash cycle, fibers, microfibers, MP, and NP are detached. Yang et al. (2021) reported that 1 kg of synthetic clothing releases 2.1–3.3 × 10^14 NP particles. It has been estimated that in 2020, the world production of synthetic textile fibers was 68 million metric tons (textile market report in the folder). Considering the world population, it is estimated that about 11 kg per capita of these fibers are consumed to manufacture clothing (Boucher and Friot, 2017), which may have generated up to 2.86 × 10^28 NP per capita per year. The most populated countries generate more MP/NP as laundry waste. Taking 1 g of cloth as a reference, it can release 1.4 mg of NP after a wash cycle (Yang et al., 2021). Based on their respective populations, China, India, the United States, and Mexico would generate around 22, 21, 5, and 2 million metric tons of NP per year."
Dispersion of micro- and nanoplastics in the planet’s ecosystems
Microplastics/nanoplastics (MP/NP) are ubiquitous and spread practically everywhere on the planet. However, the mechanisms of dispersion for these materials are not well known. This section will address the various ways in which dispersion occurs. The high production of everyday plastic items, inadequate disposal practices, and exposure to physical and chemical phenomena in natural environments (such as solar radiation, abrasion from waves and tides in the sea, high temperatures, and wind erosion), as well as in industrial and household settings (abrasion by cleaning products, washing clothes, and mechanical friction of plastic components), contribute to the generation and dispersal of MP/NP in the air, on the ground, into the soil, in the sea, in continental and subterranean water, in snow, and on mountains (Wu et al., 2020; Barnes et al., 2009).
The dispersion of the PM/NP depends to a great extent on the characteristics of these particles due to their physicochemical nature, such as their size, morphology and density. The density allows particles of different plastic polymers to float or sink in different types of water (Ziani et al., 2023), as well as their drag by airborne. While it is impossible to measure the exact amount of MP/NP present in different environments worldwide, some authors have reported specific concentrations of these substances in certain locations and environments from the sampling of air, water and soil (Table 1). For example, MP particles measuring 607 µm were found in Antarctica soil at a concentration of 13.6 particles/50 mL (Perfetti-Bolaño et al., 2022). Fibrous MP has been detected on Everest at a concentration of 30 fibers/L (Napper et al., 2020), and in forests, wetlands, sanitary landfills, and industrial zones, the average concentration is up to 106,000 particles per kg of analyzed soil (Leitao et al., 2023). The presence of MP/NP in remote areas can be attributed to the increase in tourism, expeditions, and the transport of particles through the air and sea.
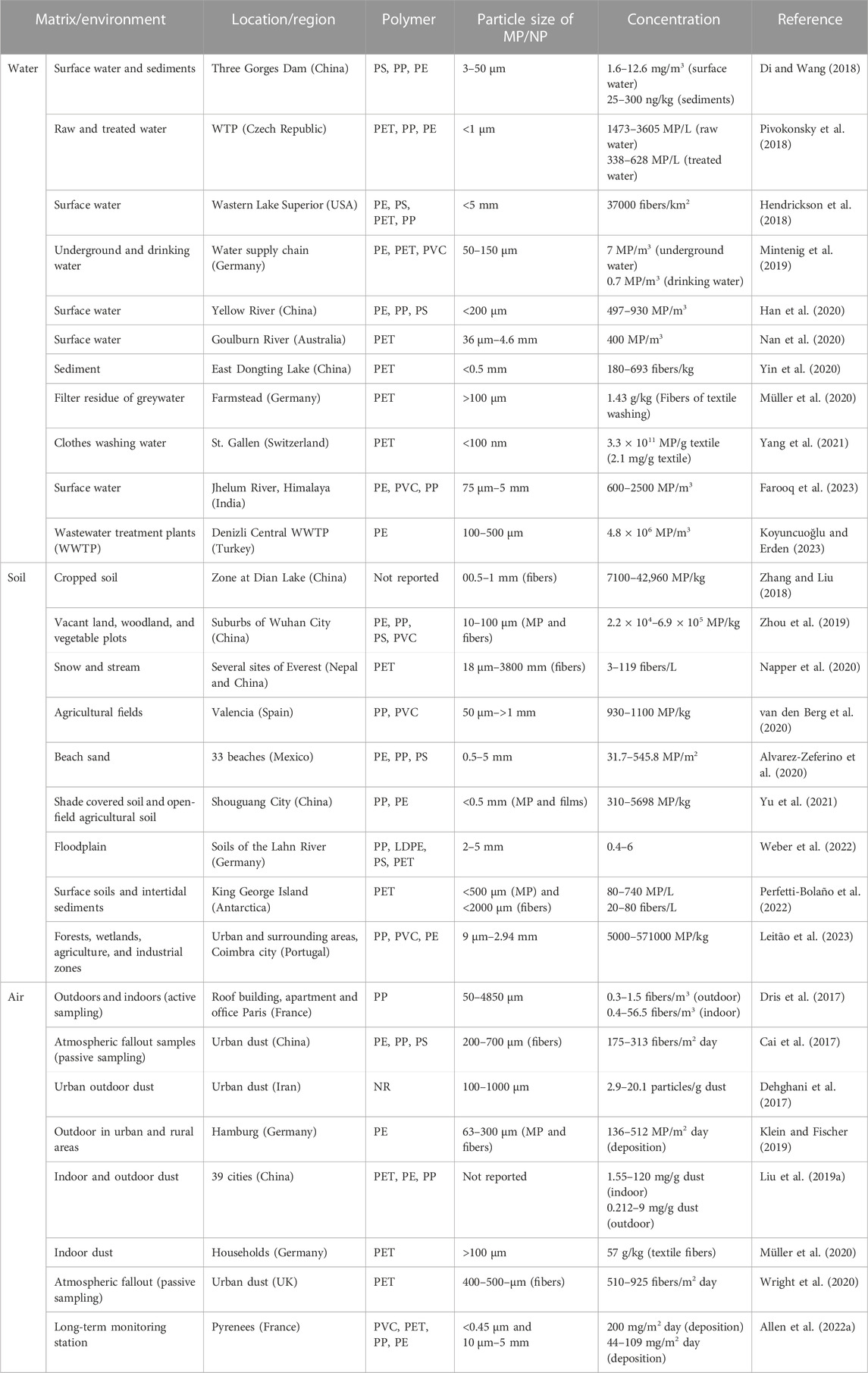
TABLE 1. Concentrations and type of MP/NP quantified in different environment locations and matrixes.
City dust contains particles generated by the abrasion of tires and shoes with synthetic soles on pavement, as well as from plastic utensils, infrastructure, and artificial grass (Essel et al., 2015; Lassen et al., 2015). This dust can be transported through the air and rise at high temperatures, like the transport of dust particles with a size <2.5 µm from the Sahara Desert to the American continent (Zauli Sajani et al., 2012; Yu et al., 2015). Airborne transport occurs relatively quickly, within days to weeks, making the atmosphere the fastest route for the spread of MP/NP (Allen et al., 2022; Allen et al., 2022), particularly for NPs, which have a longer suspension time. Several studies have been conducted to evaluate the presence of MP/NP in the air in a passive and active way. An investigation in Paris revealed concentrations of 0.3–1.5 fibers/m3 of air sampled on rooftop buildings, while concentrations of 0.4–56.5 fibers/m3 were found inside these structures (Dris et al., 2017).
An important phenomenon related to the air transport of microplastics/nanoplastics (MP/NP) is atmospheric deposition, which involves the re-incorporation of these particles into the soil, water, or ecosystems. Deonie Allen and others estimated that up to 25 million metric tons (MT) of MP/NP with diameters ranging from 8–180 µm are transported annually through the marine atmosphere and deposited in the oceans (Abbasi, 2021; Allen et al., 2022). In the French Pyrenees, NPs present in the atmosphere are deposited in quantities of up to 2 × 10^5 ng/m^2 per day, while MPs are deposited in quantities of up to 1.1 × 10^5 ng/m^2 per day, demonstrating that the amount of NPs in the air is higher than MPs. MPs can remain airborne for up to 7 days and reach altitudes of 5,000 km (Allen et al., 2022). MP/NP are also part of the water cycle and are present in rainwater, with great deposition fluxes (Zhao et al., 2023). Brahney et al. (2020) estimated that 1,000 MT of MPs are deposited in the soil of protected areas in the United States after analyzing stormwater and air samples over a 14-month period. It is projected that the amount of MP/NP deposited from the atmosphere will reach 460 MT by the year 2030 (Hundertmark et al., 2023).
Due to their size and resistance to degradation, MP/NP from laundry, household activities, and personal care products containing intentionally added small particles escape from wastewater treatment plants (WWTPs). This is why urban sewers, WWTPs, and water treatment plants (WTPs) are considered one of the main routes of distribution and deposition of MP/NP in oceans, rivers, lakes, subterranean water, soils, and sediments (Browne et al., 2011). A study conducted at 17 WTPs in the United States estimated that effluents can contain and transport up to 13 billion MP/NP per day (Mason et al., 2016). In fresh surface water and groundwater in China and Germany, the presence of MPs ranging from 3–50 µm has been observed, with concentrations of up to 12.6 particles/L (WHO, 2019).
Microplastics/nanoplastics (MP/NP) can be found in drinking water because water treatment plants (WTPs) source water from locations where effluents from wastewater treatment plants (WWTPs) were previously discharged. Studies on MP in water treatment plants in the Czech Republic have shown concentrations of up to 638 particles/L with sizes detected above 1 µm (Pivokonsky et al., 2018). In drinking water from underground sources in Germany, the amount of MP detected has been as low as 0.0007 particles/L with a minimum size of 20 µm (Mintenig et al., 2019). MP/NP can also be found in the drinking water used by industries that utilize plastic equipment and items in their processes. Bottled water from major brands in the market has been reported to contain an average of 325 MP/NP particles of polypropylene (PP) and polyethylene terephthalate (PET) per liter of water sold (Schächtele, 2020). Kosuth et al. (2018) reported the presence of fibers and plastic particles in drinking water and various brands of beer in different countries, with average concentrations of 5.45 particles/L and 4.05 particles/L, respectively. Additionally, Cox et al. (2019) reported that bottled water contains a higher concentration of MP than drinking water due to the detachment of these particles from the container walls.
One destination of WWTPs is the ocean, which can directly receive their effluents or receive them through rivers that previously received them. It has been estimated that more than 1000 rivers polluted with MP/NP flow into the ocean (Meijer et al., 2021). However, it is not the only source of plastic particles. As mentioned above, plastic waste in the oceans also contributes to the generation of these substances. In 2014, it was calculated that there are more than 7,000 tons of plastic waste and over 1.83 billion MP particles dispersed in the oceans (Eriksen et al., 2014), with a large island of these materials floating in the North Pacific Ocean (Onink et al., 2019). Egger et al. (2020) sampled this large island of plastic litter and estimated a presence of up to 3.81 million plastic particles per square kilometer, with sizes ranging from 500 µm to 5 cm, mainly composed of polyethylene (PE) and polypropylene (PP). Correia et al. (2020) estimated that the concentration of particles in the oceans can reach up to 102,000 particles/m^3. This situation indicates a constant increase in the generation of MP/NP due to the degradation of floating macroplastics, which will persist over the years, even if their use is completely prohibited (GESAMP, 2015). Additionally, MP/NP can be dispersed by aerosols from oceanic rainfall and incorporated into the water cycle in other regions (Lehmann et al., 2021)”.
According to the World Economic Forum, by 2025, for every three million fish in the ocean, there will be 1 million tons (MT) of plastics, and by 2050, there will be more microplastics (MP) than fish (WEF, 2016). As expected, MP/nanoplastics (NP) have been found in marine species, such as zooplankton, corals, mussels, fish, seagrasses, and crustaceans, which may feed on larger species (Desforges et al., 2015). These marine species become part of the food chain and bioaccumulate these plastic particles in their tissues, transferring them to the next trophic level and potentially entering the human diet. This was confirmed by exposing algae (zooplankton) to polystyrene NP, which were later consumed by water fleas and subsequently fed to fish. All three species bioaccumulated NP in their tissues (Mattsson et al., 2015).
MP/NP can deposit in the soil and have an impact on agriculture. Microplastic causes the destruction of soil structure, destroys microbiota, cause depletion of nutrients, and their absorption by plants decreases plant growth (Bostan et al., 2023). Plastic particles reach farmland through compost materials, plastic films used in agricultural tasks, wind dispersal, but mainly through water and sludge from wastewater treatment plants (WWTPs) and water treatment plants (WTPs) used as fertilizer. Van den Berg et al. (2020) quantified up to 3060 MP per kilogram of soil after being fertilized with sludge from WWTP, with applications of 20–22 tons/Ha. It has been estimated that the amount of MP/NP from WWTP effluents that could be present in farmland amounts to 7.76 million tons (MT) (Peccia and Westerhoff, 2015). MP/NP initially reside on the soil surface and then, due to the penetration of rainwater and irrigation, they are transported to the subsoil and can even reach groundwater (Panno et al., 2019; Wong et al., 2020). Additionally, there is evidence that soil-dwelling organisms, such as earthworms and microarthropods, can transport MP/NP to deeper soil layers or disperse them (Maaß et al., 2017; Rilling et al., 2017). It is estimated that soils contain more MP/NP than oceans, which is concerning as they come into contact with crops that are part of the human diet (Horton et al., 2017; Pathan et al., 2020). Once in the soil, NP with a size of 40 nm can be taken up by plant roots and, to a lesser extent, through leaves, being transported through the plant’s tissues. Furthermore, MP/NP can also affect the enzymatic activity of bacteria and fungi and alter their diversity (Bandmann et al., 2012; Sarker et al., 2020; Azeem et al., 2021), in such way that it has been suggested the “plasticsphere” or “microplasticsphere” formation on the soil, which consists of the superficial colonization of plastic waste and MP/NP by cyanobacteria (Nostoc, Scytonema), diatoms (Navicula, Cyclotella) and bacteria (Gammaproteobacteria, Alphaproteobacteria), which can degrade these materials, however, they shift bacterial community and have the potential to alter soil functioning and biogeochemical cycling (Ju et al., 2019; Zhang et al., 2019; Zhou et al., 2021; Behera and Das, 2023).
Another significant but often overlooked source of MP/NP is tire wear resulting from abrasion or friction with the ground when a vehicle is in motion. These particles generated on industrial, urban, rural, or any other roads account for approximately 90% of the total particles found in nearby areas and can reach sizes of up to 280 µm (Kreider et al., 2010; Sommer et al., 2018). Once generated, they are deposited in adjacent territories and can be transported by airborne mechanisms to other locations (Wik and Dave, 2009). Considering that there are approximately 1.446 billion cars in use worldwide as of 2023, the number of tires in circulation is significantly higher. The dispersion pathways of MP/NP are diverse and complex. Once they are swept away or transported by winds, rain, continental and subterranean water, and oceans, they can be found in inhospitable places for human populations. Ubiquitous plastic pollution at macro, micro, and nanoscopic scales even allows us to consider it as a geological marker of human impact on the planet (Geyer et al., 2017).
Damage to living beings
MP/NP are in contact with living beings through different routes: Oral consumption (food), inhalation, and dermal exposure (Revel et al., 2018; Chang et al., 2020). MP has been found in animals and substances (food) that are part of the human diet, such as fish, shrimp, shellfish, honey, sugar, salt, beer, bottled water, and tap water (Liebezeit and Liebezeit, 2013; Auta et al., 2017; Iñiguez et al., 2017; Bessa et al., 2018; Karami et al., 2018; Kosuth et al., 2018). Some studies have estimated the amount of MP consumed by humans. Kieran Cox et al. (2019) reported an intake of 113,743 and 94,283 MP per year in adult males and females, respectively, from food consumption.
NP are potentially more dangerous than MP since they can access cells of many organs due to their size (Monti et al., 2015) by crossing the cell membrane, which has pores of approximately 2–40 nm (Zhou et al., 2009; Bonardi et al., 2011). Once they enter the respiratory system, NP can reach the lungs and subsequently the alveoli. There, they can be transported in the bloodstream and finally deposited in cells of different organs, such as the brain, liver, gallbladder, pancreas, heart, and even the reproductive system and placental barrier (Barboza et al., 2018; Pitt et al., 2018; Hesler et al., 2019; Sökmen et al., 2020).
To this day, there are no studies that clearly or significantly demonstrate the effect of MP/NP on human health due to the difficulty that this implies: all humans are exposed to MP/NP, and it is required to have well-defined groups (control groups), isolating individuals, to know their medical history, long times required, an appropriate sample size, standardized protocols, control of the size and type of MP/NP, and, finally, ethics. However, studies have been reported in animals such as nematodes, crustaceans, fish, and mice (in vivo studies) and in cell cultures of various animals (in vitro studies), including human cells in relatively short periods of weeks and months (Schröter and Ventura, 2022).
In vivo studies allow the investigation of the effect of MP/NP on an organism by exposing its organs and tissues (at different stages or in its entire life cycle). In marine organisms, MP/NP have been found in feces, gills, skin, muscle, hemolymph, and the circulatory system, causing endocrine disruption, tissue inflammation, behavioral changes, reduced growth, reproductive success, and breeding success (Allen et al., 2022). After an exposure of 10 ppm of PS NP for 120 h, fish embryos have been reported to accumulate these particles in all their organs (mainly in the pancreas and intestinal tract) and are purged when the exposure ends. Interestingly, no evidence of significant mortality, deformity, or bioenergetic changes has been found, but an increase in heart rate and larvae behavior (Pitt et al., 2018). Other studies in fish have shown neurotoxicity, inflammation, or damage in the gut and neurobehavioral alterations, besides NP accumulation in organs (Chen et al., 2017a; Brun et al., 2018; Lei et al., 2018; Sarasamma et al., 2020). In addition to fish, model organisms such as water fleas and nematodes have also been used to assess NP toxicity. Water fleas have shown changes in growth, reproduction, and molting when chronically exposed to 0.1 mg/L of 20–200 nm PS NP (Pikuda et al., 2022). In addition, alterations in the immune system, gene expression, metabolism, and decreased body size have been observed (Liu et al., 2019; Liu et al., 2021). Nematodes have shown reduced pup size, damage in gonad development, inhibition of reproduction, and alteration of locomotion when exposed to 25–100 nm PS NP (Zhao et al., 2017; Kim et al., 2019; Qu et al., 2019).
In vitro studies consist of controlled exposure of NP in cell cultures of various human tissues, such as bronchi, microvascular endothelium, cortical epithelium, monocytes, among others (Schröter and Ventura, 2022). MP/NP at concentrations of 10 mg/L can induce oxidative stress in human brain and epithelial cells when exposed for 24–48 h (Schirinzi et al., 2017). Other works have reported damage to the mitochondrial membrane, increased apoptosis, activation of autophagy, and inflammatory responses (Wu et al., 2019; Ding et al., 2021; Nie et al., 2021). Some studies of this type contradict each other; thus, it is necessary to clarify whether the type and size of NP can induce effects in different human tissues (Hesler et al., 2019).
The potential damage MP/NP cause is due to the particles themselves and substances with adverse effects that they carry on their surface, such as metals, persistent organic pollutants, antibiotics, pathogens, polychlorinated biphenyls such as pesticides and herbicides, causing synergistic effect (Gewert et al., 2015; Wang et al., 2019; Prust et al., 2020; Ziani et al., 2023). Several authors have reported the combined effects of PM and certain contaminants in the soil, i.e., the accumulation of Deltamethrin and Glyphosate on its surface (preventing or slowing down its degradation and reducing the sorption capacities of the soil), the negative effect on the weight of earthworms due to the glyphosate, the transport of insecticide to deeper soil (Ramos et al., 2015; Yang et al., 2018; Ródríguez-Seijo et al., 2019; Yang et al., 2019). The physicochemical characteristics of PM/NP are important to understand how they can transport harmful substances to living beings and cause a synergistic effect. Among these properties are the polarity, solubility, density, stability, and sorption capacity of the substances.
It has been estimated that 1 g of NP can carry 1–10,000 ng of these toxic substances (Koelmans et al., 2013). Taking into account the impact due to the presence of MP/NP, they can be stored in the intestines of animals and obstruct and change the microbiota, causing imbalances, inflammation, ulcers, and even increasing the risk of cancer (Kirstein et al., 2016; Correia et al., 2020). Some studies report the successful transport of functionalized drugs with biodegradable nanoparticles of different types towards specific organs or tissues (Kotrange et al., 2021; Mitchell et al., 2021), developing the concept of nanomedicine. However, this is a precedent to study the risk in the transport of undesired toxic substances carried by MP/NP, such as bisphenol A, which can be carried by PS NP from the aqueous medium to the brain of fish, affecting dopamine levels, a neurotransmitter that regulates motor activity (Chen et al., 2017b).
Although the effect of MP/NP on human health has not been significantly concluded, MP has been found in several organs and fluids, i.e., in breast milk in 26 out of 34 women who gave birth in Rome. These MP had a size of 2–12 µm and were quantified up to 5 plastic particles per patient, with an abundance of PS (38%), PVC (21%), and PP (17%) (Ragusa et al., 2022). MP up to 10 µm has also been found in human placentas in amounts of 12 particles. The authors assert that these MP entered the bodies of pregnant women through oral or respiratory ingestion (Ragusa et al., 2021). Yan et al. (2022) found MP in the feces of patients with inflammatory bowel disease (IBD) and healthy people, with concentrations of 41.8 MP/g dm and 28 MP/g dm, suggesting that MP exposition could be associated to IBD, or that IBD exacerbates the retention of MP; these MP corresponded to 15 different types of polymers, mainly PET and polyamide in the form of fibers and sheets.
In another study by carried out by Huang et al. (2022), MP with sizes smaller than 500 μm were found in the sputum of 22 patients suffering from different respiratory diseases, indicating that inhalation is a potential way for plastics to enter the human body. In a recent study, Pironti et al. (2022) found four MP in urine of six volunteers (4–15 μm size) with irregular shapes; these fragments were PVC, PP, PE and PVA (Polyethylene vinyl acetate). This preliminary study suggests that MPs could pass through the gastrointestinal tract and are eliminated through biological processes. Leslie et al. (2022) quantified MP/NP up to 1.6 μg/mL in blood from 22 volunteers, with 125–150 µm in size of PET, PE and PS.
International legislation
Legislation regarding MP/NP is undeniably important for reducing and mitigating the impact caused by these materials on ecosystems. Europe and North America have established the regulatory foundations concerning MP. In 2014-2015, the Netherlands banned the addition of plastic particles to products, while the UK followed suit in 2017-2018 (Zhu, 2023). In the United States and Canada, the microparticle or microsphere-free water law was enacted in 2015 and 2017, respectively, which prohibits the manufacture, packaging, and distribution of cosmetics, toiletries, and non-prescription medicines containing particles smaller than or equal to 5 mm. In the United States, this ban came into effect in July 2019 (FDA, 2022), and in Canada in July 2018 (Government of Canada, 2018).
In 2019, the European Union proposed restricting the addition of MP to certain products, and finally, in 2022, they published a draft regulation to prohibit the intentional addition of microparticles of synthetic polymers to cleaners, cosmetics, personal care products, and single-use products (Zhu, 2022). This ban is planned to be implemented within a period of 6–12 years (ECHA, 2023). In China, in 2019, the National Development and Reform Commission stipulated that toiletries and cosmetics containing plastic particles (less than or equal to 5 mm) would be prohibited before the end of 2020, and their sale would be prohibited by the end of 2022 (Zhu, 2022). In Russia, in 2021, the government declared its plans to prepare amendments to legislation to ban the use of single-use items by 2024, aiming to gradually and completely reorganize industrial production with biodegradable products (Korotchenko, 2021). In India, in July 2022, the production, importation, storage, distribution, or sale of some single-use plastic products, ranging from glasses to ice cream sticks, was prohibited, encompassing a total of 19 items in the initial stage (The Associated Press, 2022). In Brazil, there is currently no national law prohibiting the use of single-use items, but since 2020, some cities and states in the country have implemented bans on plastic bags, straws, and other items (Agência Senado, 2021). In Mexico, in 2021, the Senate modified a law with the aim of eliminating the use of plastics by 2025, and concurrently, several state governments have enacted bans on plastic bags and disposable straws (Official Gazette CDMX, 2019; Congress Channel CDMX, 2021).
At the global level in 2022, the United Nations Environment Assembly (UNEA) issued the resolution “the end of plastic pollution,” which seeks to generate specific content for a new treaty through an intergovernmental negotiating committee by 2024 (Li, 2022). In 2019, 187 nations signed an accord called the Basel Convention on the Control of Transboundary Movements of Hazardous Wastes and their Disposal, which restricts participating nations from trading plastic scraps internationally, unless they lack sufficient recycling or disposal capacity. Over the last decade, global plastic trade has indeed declined significantly. However, millions of tons of plastic are still being shipped and mismanaged (WEF, 2023).
Regarding drinking water, bottled water, processed foods, and beverages, the legislation in many countries obligates producers to comply with organoleptic, physical, chemical, and microbiological parameters. It also requires nutritional information on the label. However, these standards do not consider the presence of plastic or foreign particles inside the product as a minimum requirement during processing. The EPA maintains a Contaminant Candidate List (CCL) that includes contaminants that are not currently regulated but are known to be present in public water systems. This list is updated every 5 years, and potential contaminants are regulated once their effects on human health are demonstrated or they are of public interest. Among these candidate contaminants are pathogens, industrial solvents, pesticides, metallic and non-metallic substances, drugs, toxins, among others (EPA, 2023). As of the beginning of 2023, this CCL does not include MP or NP.
Tires and vehicles are significant sources of MP/NP. European legislation considers the raw materials and waste management during tire manufacture, proper disposal at the end of their useful life, and appropriate tire usage (Trudsø et al., 2022). However, this does not guarantee the cessation of MP/NP generation.
As previously explained, current international legislation focuses on avoiding the manufacture of plastic products containing MP/NP or being sources of MP/NP generation. However, the treatment of wastewater containing these materials, their purification before discharging effluents into rivers, lakes, or oceans, air depuration, and food labeling informing the content of these particles have not yet been considered. Furthermore, MP/NP generated from textiles and vehicle tires are far from being addressed within the legislation. Finally, evaluating the risks and toxicity MP/NP pose to human health is essential to establish permissible limits for these particles.
Perspectives: gaps, study opportunities and actions that should be taken
Plastics are involved in all human activities, and due to the amount of commonly used items per person and the increase in the world’s population, the impacts of MP/NP are expected to become more evident in the future. While some countries have issued laws or regulations to ban plastic materials (with planned completion within a few years), most of the focus has been on single-use personal care and cleaning products, neglecting clothing, containers, tires, and other items.
The idea of an absolute ban on all plastics in the short term would be inconceivable, as it would lead to a global collapse in clothing, food, and transportation sectors, with immediate effects on health, economy, and the preservation of life as we know it. Plastics have replaced heavy materials that are difficult to manufacture and handle, resulting in lighter equipment and vehicles and increased energy efficiency in the industry. Synthetic fabrics and the manufacturing of clothing have fulfilled the global clothing demand, while the use of medical equipment and single-use plastic instruments has been crucial in surgeries, pandemics, and disease eradication. Therefore, it is unreasonable to consider banning all plastics. Instead, the focus should be on gradually prohibiting only non-essential plastic items, while promoting the development of new single-use materials that are environmentally friendly and do not pose disposal problems (such as Tetrapak).
Finding a solution to this problem is not easy and requires significant economic resources, time, and efforts from authorities, industry, the scientific community, and public participation. Resolving social, political, and economic issues between countries and regions is also necessary. Manufacturing processes and lifestyles must change, from clothing production and consumption to tire and container manufacturing. The scientific community’s contributions can be summarized in three main stages: 1) Studying the impact of MP/NP and their synergistic effects with other emerging pollutants on human health, 2) developing treatments or technologies for purifying water, air, and soils contaminated with MP/NP, and 3) developing biodegradable alternatives to plastics that are feasible for the general population. Previous studies and scientific publications related to MP/NP have mostly focused on polystyrene (PS) and the evaluation of MP/NP in marine and freshwater environments, leaving opportunities for further investigation of other MP/NP in the atmosphere and their health impacts. It has been demonstrated that metallic nanoparticles can cross cell membranes, reach the brain, and cause neurotoxicity, increasing the risk of disorders such as Alzheimer’s or Parkinson’s (Prüst et al., 2020). It is important to assess the risk factors of all types of plastics, analyze and estimate consumption patterns, and develop protocols for detecting these materials in blood, fluids, and feces to evaluate their correlation with disease prevalence or health disorders such as inflammation, ulcers, and thrombosis. More studies are needed to evaluate the interaction between PM/NP and harmful substances for living beings, causing synergistic damage, according to the physicochemical properties of both.
As Wang et al. (2019) demonstrate in their report, there is a lack of studies of MP/NP in soil and airborne; considering airborne and human health risk, several studies could be carried out in large and populated cities; these studies could analyze PM/NP in the air in a passive and active way, even between different nations, which allows elucidating the sources of generation and regional dispersion, and therefore, taking appropriate restrictive or legislative measures in sources that may represent a risk to health jointly between neighboring countries. Another important study opportunity related to the impact on human health is the quantification and characterization of MP/NP at the personal level with personal equipment exposure (carried by people), and the evaluation of MP/NP in the mucosa, so that from the actual concentrations in the air and in the nasal mucosa, the amount of NP that potentially crosses the nasal barriers is estimated. Rahman et al. (2021) collected and characterized MP in air samples by using silver membrane and Teflon filters, finding MP in the respirable size fraction (<2.5 µm); due to several inconsistencies, they suggest the development of new methodologies to collect MP/NP. Regarding indoor MP/NP, home air filtering equipment could be considered to avoid or reduce the intake of PM/NP, since the concentration of these particles is higher indoors than outdoors (Dris et al., 2017; Zhao et al., 2023). In addition, other exposure routes to MP/NP, such as inhalation and dermal absorption should be investigated. Currently, there are no standardized or recommended protocols by the World Health Organization to ensure that drinking or tap water, beverages, processed food and vegetables are free from MP/NP. Several technologies have been published at the laboratory level focus on water treatment, such as ultrafiltration, electrocoagulation and advanced oxidation processes (Thomas et al., 2013; Uheida et al., 2020; Elkhatib et al., 2021; Li et al., 2021; Cuba-Teran et al., 2023), however, these technologies need optimization and implementation in wastewater treatment plants, water treatment plants, and industries.
The sludge from the WWTP used to fertilize agricultural fields must undergo an adequate treatment to separate PM/NP it contains, and avoid its dispersion in soils, sediments, groundwater, rivers and oceans, and most importantly, avoid its dispersion in the human food chain. Unfortunately, air and soil have not been sufficiently studied in different meteorological conditions, so there are opportunities for modeling the trajectories and regional transport of MP/NP in the atmosphere, considering meteorological factors, as well as studying the transport of toxic substances on MP/NP surfaces. Islam et al. (2023) analyzed the MP airborne and deposition within the upper lung airways by using a computational fluid dynamic model, in which they conclude the flow rates, shape, and size of MP influence the deposition pattern, and the higher the flow rate the lower deposition efficiency, however, different shapes and sizes of MP and NP are needed to evaluate. Taking into account industry and its MP/NP emissions to atmosphere, decontamination of MP/NP polluted air could be done by using electrostatic collectors, which are used to separate particulate matter from burned fuels, as Zhao et al. (2023) suggest. Bioplastics or biodegradable plastics offer an alternative to petroleum-derived plastics. However, as discussed in the “origin and formation of micro- and nanoplastics” section, these biomaterials require specific conditions for biodegradation, have low degradation rates, and long degradation times (months). Additionally, natural polymers used in bioplastics constitute only a part of the final product, with the remainder made up of petroleum-derived polymers, making them unfeasible as a standalone solution. In the coming years, it is expected that the industry and the scientific community will develop polymers using truly biodegradable materials in collaboration with authorities to promote campaigns on composting and establish accessible composting centers for citizens. The bio-based plastics of the next-generation must be designed/redesigned considering the tendencies of their polymers to form microplastics, de tal forma que se produzcan la menor cantidad de estas partículas, as Boersma et al. (2023) calculated with the microplastic index, based on the mechanical and properties of the materials.
Despite the existence of bans on certain plastic products, the population continues to use them, and sanctioning the entire community is impossible. Therefore, it is essential for authorities to conduct awareness campaigns regarding the use and impacts of plastics, MP/NP in ecosystems, and the potential effects on human and animal health to encourage commitment and participation from the public. Fighting misinformation, such as the belief that banning plastic bags and straws will solve environmental problems, presents an opportunity for action. Another opportunity is the establishment of institutions or organizations (governmental or private) dedicated to implementing processes for the disposal (recycling) and reuse of plastic waste, processes that are achievable for society, in such way plastic waste avoids landfills, which results in the contamination of soil, groundwater, and surface water (Singh et al., 2023). Finally, the development of a circular economy, where manufactured items do not generate waste and are recycled or reused, is crucial.
Socio-economic aspects to consider to solve the problem
The impact of MP/NP on a country depends on several important factors: The amount of plastics, the facility a person has to generate plastic waste, and its proper disposal. These aspects are interpreted as the amount of plastics produced and used as a result of industrial activities, the number of inhabitants, the quality of life of inhabitants, and the technologies/strategies for waste disposal (Ehrlich and Holdren, 1971). To solve the problem of MP/NP, the proper disposal of plastic waste must be addressed. Therefore, it is useful to consider the social and economic situation of each country. The United States generates about 800 kg of garbage per capita per year, of which 130 kg is plastic waste, followed by Denmark, Switzerland, New Zealand, and Hong Kong (Schächtele, 2020; The National Academies of Sciences, Engineering, and medicine, 2022).
The level of industrialization or the economy of countries can be measured by the gross domestic product (GDP), which is an indicator that implies the degree of economic profitability or productivity of a country or area. Meanwhile, the level of development of countries, emphasizing their people and their human wellbeing, can be measured by the human development index (HDI), proposed by the United Nations. The HDI consists of three dimensions: life expectancy, education, and a decent standard of living (UNDP, 2023). This index takes values from 0 to 1, and when it is close to 1, it indicates that the inhabitants and their country are more developed. Table 2 shows the 15 most important economies/countries in 2021 based on GDP, as well as population, HDI, and the generation of mismanaged plastic waste (MPW). MPW is defined as plastic waste that evades its adequate confinement in landfills, incineration, or recycling and ends up exposed in soils and oceans.
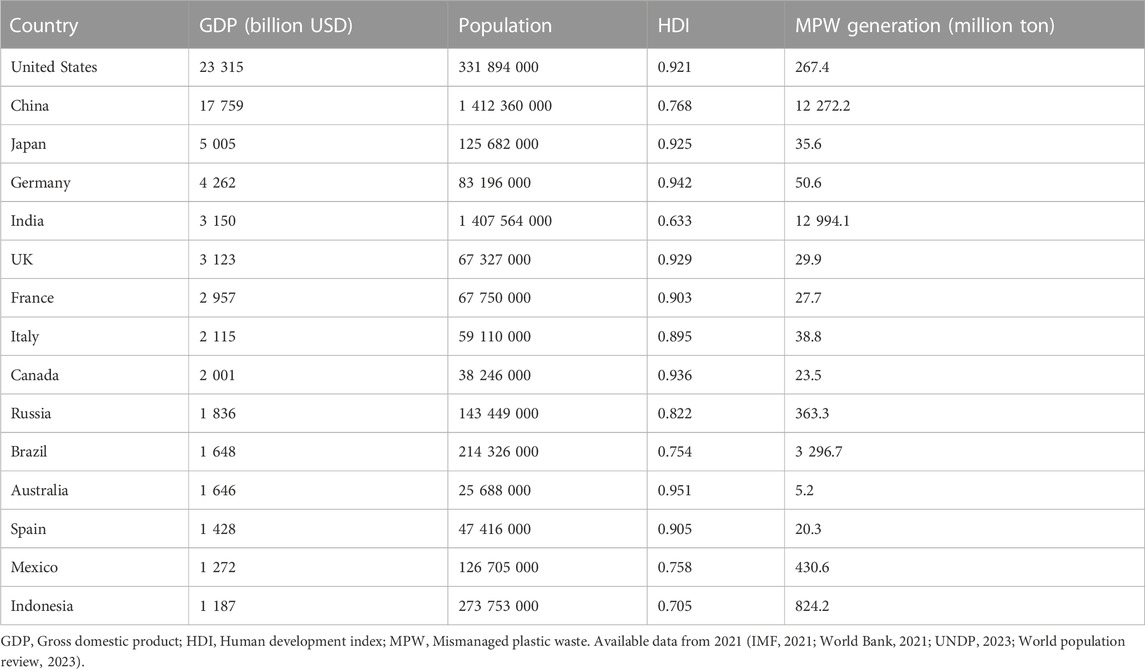
TABLE 2. Population, human development index and mismanaged plastic waste of the 15 countries with the highest gross domestic product.
Considering the available information from 148 countries (IMF, 2021; World Bank, 2021; World Population Review, 2023; UNDP, 2023) (see Supplementary Table S1), it is possible to reach important conclusions related to economic growth, the generation of plastic waste, and population. By using an association test for these non-normal data, significant correlations were obtained for several scenarios (Table 3). A positive correlation was found between GDP and MPW, interpreted as a developed country generating more waste, while MPW and HDI were negatively correlated, suggesting that higher human wellbeing of inhabitants is associated with lower generation of MPW. It must be noted that these two associations were weak, with coefficients of 0.4099 and −0.4548, respectively. Finally, the most significant correlation was found between the population of countries and MPW in the environment (coefficient 0.78), suggesting that the number of people in a country could have a greater impact on MPW generation than economic and industrial activities. The countries that generate the most plastic waste are also the most populated, such as China, India, and Brazil, with 12.2, 12.9, and 3.2 MT of MPW, respectively (World Population Review, 2023).
Worldwide plastic production reached 390.7 MT in 2021, with China as the main producer, accounting for 32% of the total, followed by North America and the European Union with 18% and 15% respectively (Plastics Europe, 2019). It is necessary to clarify that leading countries in plastic manufacture do not necessarily generate MPW or contribute to MP/NP generation in their territories, but rather in the countries to which they export the raw material or finished products.
Among the strategies to dispose of plastic waste are recycling, incineration, and landfills (the latter contaminating other matrices). Globally, it has been estimated that only 9% of plastic waste is recycled, 19% is incinerated, 49% is confined in landfills, and 22% is misdisposed. North American and European Union countries use landfills to dispose of up to 82% of their plastic waste. On the other hand, the most populated countries and some others in Asia, Africa, and Latin America misdispose up to 64% (OECD, 2019), indicating that they lack adequate waste disposal strategies. A secondary strategy to dispose of plastic waste is its commercialization, which facilitates the management of large amounts of plastic waste through exportation. However, when unusable plastic waste is imported, both the Industry and the importing country face disposal complications. Europe is a leader in the importation/exportation of plastic waste, with an importation of 2.8 MT in 2022 (WEF, 2023).
In developing countries, where basic needs for food, safety, housing, and even clothing have not been met, policies and strategies for solving environmental problems take a secondary place. This hypothesis is confirmed by the negative association between MPW and HDI in Table 3. As a reflection of these scenarios, in Latin America, Africa, and many Asian countries, there are no adequate waste management programs or plans, including for plastics. With the foregoing, it is fair that developed countries and transnational companies that have historically polluted and impacted ecosystems and human health should take the lead in strategies against MP/NP, followed by the rest of the world.
Conclusion
International legislation plays a crucial role in reducing and mitigating the impact of microplastics and nanoplastics (MP/NP) on ecosystems. Despite its progress, there are significant gaps in current legislation. Wastewater treatment, purification of effluents, air depuration, and food labeling regarding MP/NP content have not been adequately addressed. Furthermore, the legislation lacks specific regulations for MP/NP generated from textiles and vehicle tires. To effectively address the issue of MP/NP, three main areas of focus are required: Understanding the impact of MP/NP on human health, developing purification technologies for contaminated water, air, and soil, and promoting the development of biodegradable alternatives to plastic. The issue of MP/NP requires a multidisciplinary approach involving collaboration between authorities, industry, the scientific community, and the general public. The scientific community should conduct further research on the health effects of different types of plastics, including ingestion, inhalation, and dermal absorption. Standardized protocols for detecting MP/NP in biological samples (animals and humans) should be established, and the risk factors associated with MP/NP exposure should be assessed. Current regulations related to drinking water quality should consider MP/NP as contaminants, as well as efforts should be made to develop and implement technologies for the detection and removal of MP/NP from drinking water sources. Additionally, more research is needed to understand the presence and transport of MP/NP in the atmosphere, as well as the potential risks associated with inhaling these particles. Socio-economic factors play a significant role in plastic waste generation. The most populated countries contribute to plastic waste generation in their own territories, on the other hand, developed and industrialized countries are leaders in plastic manufacturing. All of them contribute to MP/NP generation, nevertheless, developing countries face challenges in implementing waste management strategies due to limited resources and competing priorities. International cooperation and support from developed countries and transnational organizations are necessary to assist developing nations in improving waste management practices.
Author contributions
All authors listed have made a substantial, direct, and intellectual contribution to the work and approved it for publication.
Conflict of interest
The authors declare that the research was conducted in the absence of any commercial or financial relationships that could be construed as a potential conflict of interest.
Publisher’s note
All claims expressed in this article are solely those of the authors and do not necessarily represent those of their affiliated organizations, or those of the publisher, the editors and the reviewers. Any product that may be evaluated in this article, or claim that may be made by its manufacturer, is not guaranteed or endorsed by the publisher.
Supplementary material
The Supplementary Material for this article can be found online at: https://www.frontiersin.org/articles/10.3389/fenvs.2023.1241939/full#supplementary-material
Supplementary Table S1 | Socio-economic data.
References
Abbasi, S. (2021). Microplastics washout from the atmosphere during a monsoon rain event. J. Hazard. Mater. Adv. 4, 100035. doi:10.1016/j.hazadv.2021.100035
Agência Senado (2021). Estudo do Senado aponta necessidade de leis para deter poluição por plásticos. Brasilia, Brazil: Senadonoticias. Avaliable At: https://www12.senado.leg.br/noticias/materias/2021/07/28/estudo-do-senado-aponta-necessidade-de-leis-para-deter-poluicao-por-plasticos (Accessed March 26, 2023).
Allen, B, S., Materić, D., Allen, D., MacDonald, A., Holzinger, R., Le Roux, G., et al. (2022a). An early comparison of nano to microplastic mass in a remote catchment's atmospheric deposition. J. Hazard. Mater. Adv. 7, 100104. doi:10.1016/j.hazadv.2022.100104
Allen, D., Allen, S., Abbasi, S., Baker, A., Bergmann, M., Brahney, J., et al. (2022b). Microplastics and nanoplastics in the marine-atmosphere environment. Nat. Rev. Earth Environ. 3, 393–405. doi:10.1038/s43017-022-00292-x
Allen, S., Allen, D., Karbalaei, S., Maselli, V., and Walker, T. R. (2022c). Micro(nano)plastics sources, fate, and effects: what we know after ten years of research. J. Hazard. Mater. Adv. 6, 100057. doi:10.1016/j.hazadv.2022.100057
Alvarez-Zeferino, J. C., Ojeda-Benítez, S., Cruz-Salas, A. A., Martínez-Salvador, C., and Vázquez-Morillas, A. (2020). Microplastics in Mexican beaches. Resour. Conservation Recycl. 155, 104633. doi:10.1016/j.resconrec.2019.104633
Andrady, A. L. (2011). Microplastics in the marine environment. Mar. Pollut. Bull. 62 (8), 1596–1605. doi:10.1016/j.marpolbul.2011.05.030
Auta, H. S., Emenike, C. U., and Fauziah, S. H. (2017). Distribution and importance of microplastics in the marine environment: a review of the sources, fate, effects, and potential solutions. Environ. Int. 102, 165–176. doi:10.1016/j.envint.2017.02.013
Azeem, I., Adeel, M., Ahmad, M. A., Shakoor, N., Jiangcuo, G. D., Azeem, K., et al. (2021). Uptake and accumulation of nano/microplastics in plants: a critical review. Nanomaterials 11 (11), 2935. doi:10.3390/nano11112935
Bandmann, V., Müller, J. D., Köhler, T., and Homann, U. (2012). Uptake of fluorescent nano beads into BY2-cells involves clathrin-dependent and clathrin-independent endocytosis. FEBS Lett. 586, 3626–3632. doi:10.1016/j.febslet.2012.08.008
Banker, D., Ahuja, A., Sanadhya, H., Purohit, C., Chandra, S., Sethi, R., et al. (2016). Clinical performance of biodegradable polymer-coated sirolimus-eluting stents in unselected real-world population with coronary artery disease: results from the multicenter CORE registry. Minerva Cardioangiol. 64, 9–14.
Barboza, L. G. A., Dick Vethaak, A., Lavorante, B. R. B. O., Lundebye, A. K., and Guilhermino, L. (2018). Marine microplastic debris: an emerging issue for food security, food safety and human health. Mar. Pollut. Bull. 133, 336–348. doi:10.1016/j.marpolbul.2018.05.047
Barnes, D. K. A., Galgani, F., Thompson, R. C., and Barlaz, M. (2009). Accumulation and fragmentation of plastic debris in global environments. Trans. R. Soc. 364, 1985–1998. doi:10.1098/rstb.2008.0205
Behera, S., and Das, S. (2023). Environmental impacts of microplastic and role of plastisphere microbes in the biodegradation and upcycling of microplastic. Chemosphere 334, 138928. doi:10.1016/j.chemosphere.2023.138928
Bessa, F., Barría, P., Neto, J. M., Frias, J. P. G. L., Otero, V., Sobral, P., et al. (2018). Occurrence of microplastics in commercial fish from a natural estuarine environment. Mar. Pollut. Bull. 128, 575–584. doi:10.1016/j.marpolbul.2018.01.044
Boersma, A., Grigoriadi, K., Nooijens, M. G. A., Henke, S., Kooter, I. M., Parker, L. A., et al. (2023). Microplastic index—how to predict microplastics formation? Polymers 15, 2185. doi:10.3390/polym15092185
Bonardi, F., Halza, E., Walko, M., Du Plessis, F., Nouwen, N., Feringa, B. L., et al. (2011). Probing the SecYEG translocation pore size with preproteins conjugated with sizable rigid spherical molecules. Proc. Natl. Acad. Sci. 108, 7775–7780. doi:10.1073/pnas.1101705108
Bostan, N., Ilyas, N., Akhtar, N., Mehmood, S., Saman, R. U., Sayyed, R. Z., et al. (2023). Toxicity assessment of microplastic (MPs); a threat to the ecosystem. Environ. Res. 234, 116523. doi:10.1016/j.envres.2023.116523
Boucher, J., and Friot, D. (2017). Primary microplastics in the oceans: a global evaluation of sources. International union for conservation of nature. doi:10.2305/IUCN.CH.2017.01.en
Brahney, J., Hallerud, M., Heim, E., Hahnenberger, M., and Sukumaran, S. (2020). Plastic rain in protected areas of the United States. Science 368 (6496), 1257–1260. doi:10.1126/science.aaz5819
Browne, M,A., Crump, P., Niven, S. J., Teuten, E., Tonkin, A., Galloway, T., et al. (2011). Accumulation of microplastic on shorelines woldwide: sources and sinks. Environ. Sci. Technol. 45, 9175–9179. doi:10.1021/es201811s
Brun, N. R., Koch, B. E. V., Varela, M., Peijnenburg, W. J. G. M., Spaink, H. P., and Vijver, M. G. (2018). Nanoparticles induce dermal and intestinal innate immune system responses in zebrafish embryos. Environ. Sci. Nano 5, 904–916. doi:10.1039/C8EN00002F
Cai, L., Wang, J., Peng, J., Tan, Z., Zhan, Z., Tan, X., et al. (2017). Characteristic of microplastics in the atmospheric fallout from dongguan city, China: preliminary research and first evidence. Environ. Sci. Pollut. Res. 24, 4–15. doi:10.1007/s11356-017-0116-x
Chang, X., Xue, Y., Li, J., Zou, L., and Tang, M. (2020). Potential health impact of environmental micro- and nanoplastics pollution. J. Appl. Toxicol. 40 (1), 4–15. doi:10.1002/jat.3915
Chen, J., Nie, X. A., Jiang, J. C., and Zhou, Y. H. (2018). Thermal degradation and plasticizing mechanism of poly(vinyl chloride) plasticized with a novel cardanol derived plasticizer. IOP Conf. Ser. Mat. Sci. Eng. 292, 012008. doi:10.1088/1757-899X/292/1/012008
Chen, Q., Gundlach, M., Yang, S., Jiang, J., Velki, M., Yin, D., et al. (2017a). Quantitative investigation of the mechanisms of microplastics and nanoplastics toward zebrafish larvae locomotor activity. Sci. Total Environ. 584-585, 1022–1031. doi:10.1016/j.scitotenv.2017.01.156
Chen, Q., Yin, D., Jia, Y., Schiwy, S., Legradi, J., Yang, S., et al. (2017b). Enhanced uptake of BPA in the presence of nanoplastics can lead to neurotoxic effects in adult zebrafish. Sci. Total Environ. 609, 1312–1321. doi:10.1016/j.scitotenv.2017.07.144
Congress Channel CDMX (2021). Senado avala reforma que busca reducir el uso de plásticos de un solo uso. Avaliable At: https://www.canaldelcongreso.gob.mx/noticias/14777/Senado_avala_reforma_que_busca_reducir_el_uso_de_plstics_de_un_solo_uso#:∼:text=Asimismo%2C%20prev%C3%A9%20la%20eliminaci%C3%B3n%20total,%C3%A1reas%20naturales%20protegidas%20o%20insulares (Accessed April 04, 2023).
Correia, J., João, P., Lopes, I., Duarte, A. C., and Rocha-Santos, T. (2020). Environmental exposure to microplastics: an overview on possible human health effects. Sci. Total Environ. 702, 134455. doi:10.1016/j.scitotenv.2019.134455
Cox, K. D., Covernton, G. A., Davies, H. L., Dower, J. F., Juanes, F., and Dudas, S. E. (2019). Human consumption of microplastics. Environ. Sci. Technol. 53 (12), 7068–7074. doi:10.1021/acs.est.9b01517
Cuba Teran, F. J., Amaral Mosque, V. A., Rodrigues de Oliveira, L., and Frayne Cuba, R. M. (2023). Analysis of the efficiency of the electrocoagulation process in the removal of microplastics. Rev. Gest. Soc. Ambient. 17 (3), e03280. doi:10.24857/rgsa.v17n3-027
Dehghani, S., Moore, F., and Akhbarizadeh, R. (2017). Microplastic pollution in deposited urban dust, Tehran metropolis. Iran. Environ. Sci. Pollut. Res. 24, 20360–20371. doi:10.1007/s11356-017-9674-1
Desforges, J. P. W., Galbraith, M., and Ross, P. S. (2015). Ingestion of microplastics by zooplankton in the northeast Pacific Ocean. Arch. Environ. Contam. Toxicol. 69, 320–330. doi:10.1007/s00244-015-0172-5
Di, M., and Wang, J. (2018). Microplastics in surface waters and sediments of the three gorges reservoir, China. Sci. Total Environ. 616-617, 1620–1627. doi:10.1016/j.scitotenv.2017.10.150
Ding, Y., Zhang, R., Li, B., Du, Y., Li, J., Tong, X., et al. (2021). Tissue distribution of polystyrene nanoplastics in mice and their entry, transport, and cytotoxicity to GES-1 cells. Environ. Pollut. 280, 116974. doi:10.1016/j.envpol.2021.116974
Dris, R., Gasperi, J., Mirande, C., Mandin, C., Guerrouache, M., Langlois, V., et al. (2017). A first overview of textile fibers, including microplastics, in indoor and outdoor environments. Environ. Pollut. 221, 453–458. doi:10.1016/j.envpol.2016.12.013
ECHA (European chemicals Agency) (2023). Microplastics. Avaliable At: https://echa.europa.eu/hot-topics/microplastics (Accessed April 04, 2023).
Egger, M., Sulu-Gambari, F., and Lebreton, L. (2020). First evidence of plastic fallout from the North Pacific garbage patch. Sci. Rep. 10 (1), 7495. doi:10.1038/s41598-020-64465-8
Ehrlich, P. R., and Holdren, J. P. (1971). Impact of population growth. Science 171, 1212–1217. doi:10.1126/science.171.3977.1212
Elkhatib, D., Oyanedel-Craver, V., and Carissimi, E. (2021). Electrocoagulation applied for the removal of microplastics from wastewater treatment facilities. Sep. Purif. Technol. 276, 118877. doi:10.1016/j.seppur.2021.118877
EPA (Environmental Protection Agency) (2023). Contaminant candidate list (CCL) and regulatory determination. Avaliable At: https://www.epa.gov/ccl/basic-information-ccl-and-regulatory-determination#what-is-CCL (Accessed March 26, 2023).
Eriksen, M., Lebreton, L. C. M., Carson, H. S., Thiel, M., Moore, C. J., Borerro, J. C., et al. (2014). Plastic pollution in the world's oceans: More than 5 trillion plastic pieces weighing over 250,000 tons afloat at sea. pLoS ONE 9 (12), e111913. doi:10.1371/journal.pone.0111913
Essel, R., Engel, L., Carus, M., and Ahrens, R. H. (2015). Sources of microplastics relevant to marine protection in Germany. Avaliable At: https://www.umweltbundesamt.de/sites/default/files/medien/378/publikationen/texte_64_2015_sources_of_microplastics_relevant_to_marine_protection_1.pdf.
Farooq, M., Nisa, F. U., Manzoor, Z., Tripathi, S., Thulasiraman, A. V., Khan, M. I., et al. (2023). Abundance and characteristics of microplastics in a freshwater river in northwestern Himalayas, India - scenario of riverbank solid waste disposal sites. Sci. Total Environ. 886, 164027. doi:10.1016/j.scitotenv.2023.164027
FDA (2022). Food and drugs administration the microbead-free waters act: faqs. Avaliable At: https://www.fda.gov/cosmetics/cosmetics-laws-regulations/microbead-free-waters-act-faqs#:∼:text=The%20Microbead%2DFree%20Waters%20Act%20of%202015%20prohibits%20the%20manufacturing,)%20drugs%2C%20such%20as%20toothpastes (Accessed April 04, 2023).
Gardette, M., Perthue, A., Gardette, J., Janecska, T., Földes, E., Pukánszky, B., et al. (2013). Photo- and thermal-oxidation of polyethylene: comparison of mechanisms and influence of unsaturation content. Polym. Degrad. Stab. 98, 2383–2390. doi:10.1016/j.polymdegradstab.2013.07.017
Gewert, B., Plassmann, M. M., and Macleod, M. (2015). Pathways for degradation of plastic polymers floating in the marine environment. Environ. Sci. Process. Impacts 17, 1513–1521. doi:10.1039/c5em00207a
Geyer, R., Jambeck, J. R., and Law, K. L. (2017). Production, use, and fate of all plastics ever made. Sci. Adv. 9 (7), 1700782. doi:10.1126/sciadv.1700782
Government of Canada (2018). Microbeads in toiletries regulations. Avaliable At: https://www.canada.ca/en/health-canada/services/chemical-substances/other-chemical-substances-interest/microbeads.html#a4 (Accessed April 04, 2023).
HanNiu, M,X., Tang, M., Zhang, B. T., Wang, G., Yue, W., Kong, X., et al. (2020). Distribution of microplastics in surface water of the lower Yellow River near estuary. Sci. Total Environ. 707, 135601. doi:10.1016/j.scitotenv.2019.135601
Hendrickson, E., Minor, E. C., and Schreiner, K. (2018). Microplastic abundance and composition in western lake superior as determined via microscopy, pyr-GC/MS, and FTIR. Environ. Sci. Technol. 52 (4), 1787–1796. doi:10.1021/acs.est.7b05829
Hesler, M., Aengenheister, L., Ellinger, B., Drexel, R., Straskraba, S., Jost, C., et al. (2019). Multi-endpoint toxicological assessment of polystyrene nano- and microparticles in different biological models in vitro. Toxicol Vitro 61, 104610. doi:10.1016/j.tiv.2019.104610
Horton, A. A., Walton, A., Spurgeon, D. J., Lahive, E., and Svendsen, C. (2017). Microplastics in freshwater and terrestrial environments: evaluating the current understanding to identify the knowledge gaps and future research priorities. Sci. Total Environ. 586, 127–141. doi:10.1016/j.scitotenv.2017.01.190
Huang, S., Huang, X., Bi, R., Guo, Q., Yu, X., Zeng, Q., et al. (2022). Detection and analysis of microplastics in human sputum. Environ. Sci. Technol. 56 (4), 2476–2486. doi:10.1021/acs.est.1c03859
Hundertmark, T., Mayer, M., McNally, C., Simons, T. J., and Witte, C. (2023). How plastics waste recycling could transform the chemical industry. Avaliable At: https://www.mckinsey.com/industries/chemicals/our-insights/how-plastics-waste-recycling-could-transform-the-chemical-industry.
IMF (International Monetary Fund) (2021). GDP per capita, current prices: all country data. Avaliable At: https://www.imf.org/external/datamapper/NGDPDPC@WEO/OEMDC/ADVEC/WEOWORLD (Accessed April 04, 2023).
Iñiguez, M. E., Conesa, J. A., and Fullana, A. (2017). Microplastics in Spanish table salt. Sci. Rep. 7, 8620. doi:10.1038/s41598-017-09128-x
Islam, M. S., Mizanur Rahman, M., Larpruenrudee, P., Akbar, A., Ariful Islam, M., Gu, Y. T., et al. (2023). How microplastics are transported and deposited in realistic upper airways? Phys. Fluids 35, 063319. doi:10.1063/5.0150703
Ju, H., Zhu, D., and Qiao, M. (2019). Effects of polyethylene microplastics on the gut microbial community, reproduction and avoidance behaviors of the soil springtail, Folsomia candida. Environ. Pollut. 247, 890–897. doi:10.1016/j.envpol.2019.01.097
Karami, A., Golieskardi, A., Choo, C. K., Larat, V., Karbalaei, S., and Salamatinia, B. (2018). Microplastic and mesoplastic contamination in canned sardines and sprats. Sci. Total Environ. 612, 1380–1386. doi:10.1016/j.scitotenv.2017.09.005
GESAMP (2015). “Joint group of experts on the scientific aspects of marine environmental protection,” in Sources, fate and effects of microplastics in the marine environment: a global assessment. International maritime organization. Editor P. J. Kershaw (London: GESAMP).
Khairul Anuar, N. F. S., Huyop, F., Ur-Rehman, G., Abdullah, F., Normi, Y. M., Sabullah, M. K., et al. (2022). An overview into polyethylene terephthalate (pet) hydrolases and efforts in tailoring enzymes for improved plastic degradation. Int. J. Mol. Sci. 23 (20), 12644. doi:10.3390/ijms232012644
Kim, H. M., Lee, D. K., Long, N. P., Kwon, S. W., and Park, J. H. (2019). Uptake of nanopolystyrene particles induces distinct metabolic profiles and toxic effects in Caenorhabditis elegans. Environ. Pollut. 246, 578–586. doi:10.1016/j.envpol.2018.12.043
Kirstein, I. V., Kirmizi, S., Wichels, A., Garin-fernandez, A., Erler, R., Martin, L., et al. (2016). Dangerous hitchhikers? Evidence for potentially pathogenic Vibrio spp. on microplastic particles. Mar. Environ. Res. 120, 1–8. doi:10.1016/j.marenvres.2016.07.004
Klein, M., and Fischer, E. K. (2019). Microplastic abundance in atmospheric deposition within the Metropolitan area of Hamburg, Germany. Sci. Total Environ. 685, 96–103. doi:10.1016/j.scitotenv.2019.05.405
Koelmans, A. A., Besseling, E., Wegner, A., and Foekema, E. M. (2013). Plastic as a carrier of POPs to aquatic organisms: a model analysis. Environ. Sci. Technol. 47 (14), 7812–7820. doi:10.1021/es401169n
Korotchenko, M. (2021). September 02. Russia to fully ban single-use plastic products by 2024 – minister the Moscow times. Avaliable At: https://www.themoscowtimes.com/2021/09/02/russia-to-fully-ban-single-use-plastic-products-by-2024-minister-a74960 (Accessed April 04, 2023).
Kosuth, M., Mason, S. A., and Wattenberg, E. V. (2018). Anthropogenic contamination of tap water, beer, and sea salt. pLoS One 13 (4), e0194970. doi:10.1371/journal.pone.0194970
Kotrange, H., Najda, A., Bains, A., Gruszecki, R., Chawla, P., and Tosif, M. M. (2021). Metal and metal oxide nanoparticle as a novel antibiotic carrier for the direct delivery of antibiotics. Int. J. Mol. Sci. 22 (17), 9596. doi:10.3390/ijms22179596
Koyuncuoğlu, P., and Erden, G. (2023). Microplastics in municipal wastewater treatment plants: a case study of denizli/Turkey. Front. Environ. Sci. Eng. 17 (8), 99. doi:10.1007/s11783-023-1699-8
Kreider, M. L., Panko, J. M., McAtee, B. L., Sweet, L. I., and Finley, B. L. (2010). Physical and chemical characterization of tire-related particles: Comparison of particles generated using different methodologies. Sci. Total Environ. 408 (3), 652–659. doi:10.1016/j.scitotenv.2009.10.016
Lassen, C., Foss Hansen, S., Magnusson, K., Noren, F., Bloch Hartmann, N. I., Rehne Jensen, P., et al. (2015). Microplastics: occurrence, effects and sources of releases to the environment in Denmark. Denmark: The Danish Environmental Protection Agency.
Lehmann, M., Oehlschlägel, L. M., Häusl, F. P., Held, A., and Gekle, S. (2021). Ejection of marine microplastics by raindrops: a computational and experimental study. Micropl. Nanopl. 1, 18. doi:10.1186/s43591-021-00018-8
Lei, L., Wu, S., Lu, S., Liu, M., Song, Y., Fu, Z., et al. (2018). Microplastic particles cause intestinal damage and other adverse effects in zebrafish Danio rerio and nematode Caenorhabditis elegans. Sci. Total Environ. 619-620, 1–8. doi:10.1016/j.scitotenv.2017.11.103
Leitão, I. A., van Schaik, L., Ferreira, A. J. D., Alexandre, N., and Geissen, V. (2023). The spatial distribution of microplastics in topsoils of an urban environment- Coimbra city case-study. Environ. Res. 218, 114961. doi:10.1016/j.envres.2022.114961
Leslie, H. A., van Velzen, M. J. M., Brandsma, S. H., Vethaak, A. D., Garcia-Vallejo, J. J., and Lamoree, M. H. (2022). Discovery and quantification of plastic particle pollution in human blood. Environ. Int. 163, 107199. doi:10.1016/j.envint.2022.107199
Li, J., Wang, B., Chen, Z., Ma, B., and Chen, J. P. (2021). Ultrafiltration membrane fouling by microplastics with raw water: Behaviors and alleviation methods. Chem. Eng. J. 410, 128174. doi:10.1016/j.cej.2020.128174
Li, L., Guan, C., Zhang, A., Chen, D., and Qing, Z. (2004). Thermal stabilities and the thermal degradation kinetics of polyimides. Polym. Degrad. Stab. 84, 369–373. doi:10.1016/j.polymdegradstab.2003.11.007
Li, Y. (2022). Legislation and policy on pollution prevention and the control of marine microplastics. Water 14, 2790. doi:10.3390/w14182790
Liebezeit, G., and Liebezeit, E. (2013). Non-pollen particulates in honey and sugar. Food Addit. Contam. A 30, 2136–2140. doi:10.1080/19440049.2013.843025
Liu, C., Li, J., Zhang, Y., Wang, L., Deng, J., Gao, Y., et al. (2019a). Widespread distribution of PET and PC microplastics in dust in urban China and their estimated human exposure. Environ. Int. 128, 116–124. doi:10.1016/j.envint.2019.04.024
Liu, Z., Li, Y., Pérez, E., Jiang, Q., Chen, Q., Jiao, Y., et al. (2021). Polystyrene nanoplastic induces oxidative stress, immune defense, and glycometabolism change in Daphnia pulex: application of transcriptome profiling in risk assessment of nanoplastics. J. Hazard Mater 402, 123778. doi:10.1016/j.jhazmat.2020.123778
Liu, Z., Yu, P., Cai, M., Wu, D., Zhang, M., Huang, Y., et al. (2019b). Polystyrene nanoplastic exposure induces immobilization, reproduction, and stress defense in the freshwater cladoceran Daphnia pulex. Chemosphere 215, 74–81. doi:10.1016/j.chemosphere.2018.09.176
Maaß, S., Daphi, D., Lehmann, A., and Rillig, M. C. (2017). Transport of microplastics by two collembolan species. Environ. Pollut. 225, 456–459. doi:10.1016/j.envpol.2017.03.009
Mason, S. A., Garneau, D., Sutton, R., Chu, Y., Ehmann, K., Barnes, J., et al. (2016). Microplastic pollution is widely detected in US municipal wastewater treatment plant effluent. Environ. Pollut. 218, 1045–1054. doi:10.1016/j.envpol.2016.08.056
Mattsson, K., Ekvall, M. T., Hansson, L. A., Linse, S., Malmendal, A., and Cedervall, T. (2015). Altered behavior, physiology, and metabolism in fish exposed to polystyrene nanoparticles. Environ. Sci. Technol. 49, 553–561. doi:10.1021/es5053655
Maurya, A., Bhattacharya, A., and Khare, S. K. (2020). Enzymatic remediation of polyethylene terephthalate (pet)–based polymers for effective management of plastic wastes: an overview. Front. Bioeng. Biotechnol. 8, 602325. doi:10.3389/fbioe.2020.602325
Meijer, L. J. J., Emmerik, T. V., Der Ent, R. V., Schmidt, C., and Lebreton, L. (2021). More than 1000 rivers account for 80% of global riverine plastic emissions into the ocean. Sci. Adv. 7, 18. doi:10.1126/sciadv.aaz5803
Mintenig, S. M., Löder, M. G. J., Primpke, S., and Gerdts, G. (2019). Low numbers of microplastics detected in drinking water from ground water sources. Sci. Total Environ. 648, 631–635. doi:10.1016/j.scitotenv.2018.08.178
Mitchell, M. J., Billingsley, M. M., Haley, R. M., Wechsler, M. E., Peppas, N. A., and Langer, R. (2021). Engineering precision nanoparticles for drug delivery. Nat. Rev. Drug Discov. 20, 101–124. doi:10.1038/s41573-020-0090-8
Monti, D. M., Guarnieri, D., Napolitano, G., Piccoli, R., Netti, P., Fusco, S., et al. (2015). Biocompatibility, uptake and endocytosis pathways of polystyrene nanoparticles in primary human renal epithelial cells. J. Biotechnol. 193, 3–10. doi:10.1016/j.jbiotec.2014.11.004
Müller, A., Goedecke, C., Eisentraut, P., Piechotta, C., and Braun, U. (2020). Microplastic analysis using chemical extraction followed by LC-UV analysis: a straightforward approach to determine PET content in environmental samples. Environ. Sci. Eur. 32, 85. doi:10.1186/s12302-020-00358-x
Nan, B., Su, L., Kellar, C., Craig, N. J., Keough, M. J., and Pettigrove, V. (2020). Identification of microplastics in surface water and Australian freshwater shrimp Paratya australiensis in Victoria, Australia. Environ. Pollut. 259, 113865. doi:10.1016/j.envpol.2019.113865
Napper, I., Davies, B., Elmore, A., Gajurel, A., Correspondence, R., Clifford, H., et al. (2020). Reaching new heights in plastic pollution-preliminary findings of microplastics on Mount Everest. One Earth 3, 621–630. doi:10.1016/j.oneear.2020.10.020
Nie, J. H., Shen, Y., Roshdy, M., Cheng, X., Wang, G., and Yang, X. (2021). Polystyrene nanoplastics exposure caused defective neural tube morphogenesis through caveolae-mediated endocytosis and faulty apoptosis. Nanotoxicology 15 (7), 885–904. doi:10.1080/17435390.2021.1930228
OECD (The Organization for Economic Cooperation and Development) (2019). Share of plastics treated by waste management category, after disposal of recycling residues and collected litter. Avaliable At: https://www.oecd.org/environment/plastic-pollution-is-growing-relentlessly-as-waste-management-and-recycling-fall-short.htm.(Accessed April 04, 2023).
Official Gazette CDMX (2019). Ley de residuos sólidos del Distrito Federal. Avaliable At: https://www.sedema.cdmx.gob.mx/storage/app/media/DGEIRA/GacetaDel25DeJunio2019.pdf (Accessed April 04, 2023).
Oliveira, J., Belchior, A., da Silva, V. D., Rotter, A., Petrovski, Ž., Almeida, P. L., et al. (2020). Marine environmental plastic pollution: mitigation by microorganism degradation and recycling valorization. Front. Mar. Sci. 7, 567126. doi:10.3389/fmars.2020.567126
Onink, V., Wichmann, D., Delandmeter, P., and van Sebille, E. (2019). The role of Ekman currents, geostrophy, and Stokes drift in the accumulation of floating microplastic. J. Geophys. Res. Oceans 124, 1474–1490. doi:10.1029/2018JC014547
Panno, S. V., Kelly, W. R., Scott, J., Zheng, W., McNeish, R. E., Holm, N., et al. (2019). Microplastic contamination in Karst groundwater systems. Ground Water 57, 189–196. doi:10.1111/gwat.12862
Pathan, S. I., Arfaioli, P., Bardelli, T., Ceccherini, M. T., Nannipieri, P., and Pietramellara, G. (2020). Soil pollution from micro- and nanoplastic debris: a hidden and unknown biohazard. Sustainability 12, 7255. doi:10.3390/su12187255
Peccia, J., and Westerhoff, P. (2015). We should expect more out of our sewage sludge. Environ. Sci. Technol. 49 (14), 8271–8276. doi:10.1021/acs.est.5b01931
Perfetti-Bolaño, A., Araneda, A., Muñoz, K., and Barra, R. O. (2022). Occurrence and distribution of microplastics in soils and intertidal sediments at Fildes Bay, Maritime Antarctica. Front. Mar. Sci. 8, 774055. doi:10.3389/fmars.2021.774055
Pikuda, P., Dumont, E. R., Matthews, S., Xu, E. G., Berk, D., and Tufenkji, N. (2022). Sub-lethal effects of nanoplastics upon chronic exposure to Daphnia magna. J. Hazard. Mater. Adv. 7, 100136. doi:10.1016/j.hazadv.2022.100136
Pironti, C., Notarstefano, V., Ricciardi, M., Motta, O., Giorgini, E., and Montano, L. (2022). First evidence of microplastics in human urine, a preliminary study of intake in the human body. Toxics 11, 40. doi:10.3390/toxics11010040
Pitt, J. A., Kozal, J. S., Jayasundara, N., Massarsky, A., Trevisan, R., Geitner, N., et al. (2018). Uptake, tissue distribution, and toxicity of polystyrene nanoparticles in developing zebrafish (Danio rerio). Aquat. Toxicol. 194, 185–194. doi:10.1016/j.aquatox.2017.11.017
Pivokonsky, M., Cermakova, L., Novotna, K., Peer, P., Cajthaml, T., and Janda, V. (2018). Occurrence of microplastics in raw and treated drinking water. Sci. Total Environ. 643, 1644–1651. doi:10.1016/j.scitotenv.2018.08.102
Plastics Europe (2019). Plastics – the facts 2019: An analysis of European plastics production, demand and waste data, for 2018. Brussels, Belgium: EPRO.
Pospísil, J., Pilar, J., Billingham, N. C., Marek, A., Horák, Z., and Nesp°urek, S. (2006). Factors affecting accelerated testing of polymer photostability. Polym. Degrad. Stab. 91, 417–422. doi:10.1016/j.polymdegradstab.2005.01.049
Prüst, M., Meijer, J., and Westerink, R. H. S. (2020). The plastic brain: neurotoxicity of micro- and nanoplastics. Part Fibre Toxicol. 17, 24. doi:10.1186/s12989-020-00358-y
Qu, M., Qiu, Y., Kong, Y., and Wang, D. (2019). Amino modification enhances reproductive toxicity of nanopolystyrene on gonad development and reproductive capacity in nematode Caenorhabditis elegans. Environ. Pollut. 254, 112978. doi:10.1016/j.envpol.2019.112978
Ragusa, A., Notarstefano, V., Svelato, A., Belloni, A., Gioacchini, G., Blondeel, C., et al. (2022). Raman microspectroscopy detection and characterisation of microplastics in human breastmilk. Polymers 14, 2700. doi:10.3390/polym14132700
Ragusa, A., Svelato, A., Santacroce, C., Catalano, P., Notarstefano, V., Carnevali, O., et al. (2021). Plasticenta: first evidence of microplastics in human placenta. Environ. Int. 146, 106274. doi:10.1016/j.envint.2020.106274
Rahman, L., Mallach, G., Kulka, R., and Halappanavar, S. (2021). Microplastics and nanoplastics science: collecting and characterizing airborne microplastics in fine particulate matter. Nanotoxicology 15 (9), 1253–1278. doi:10.1080/17435390.2021.2018065
Ramos, L., Berenstein, G., Hughes, E. A., Zalts, A., and Montserrat, J. M. (2015). Polyethylene film incorporation into the horticultural soil of small periurban production units in Argentina. Sci. Total Environ. 523, 74–81. doi:10.1016/j.scitotenv.2015.03.142
Restrepo-Flórez, J., Bassi, A., and Thompson, M. R. (2014). Microbial degradation and deterioration of polyethylene- A review. Int. Biodeterior. Biodegr. 88, 83–90. doi:10.1016/j.ibiod.2013.12.014
Revel, M., Châtel, A., and Mouneyrac, C. (2018). Micro(nano) plastics: a threat to human health? Curr. Opin. Environ. Sci. Health. 1, 17–23. doi:10.1016/j.coesh.2017.10.003
Rillig, M. C., Ingraffia, R., and Machado, A. A. D. S. (2017). Microplastic incorporation into soil in agroecosystems. Front. Plant Sci. 8, 1805. doi:10.3389/fpls.2017.01805
Rodríguez-Seijo, A., Santos, B., Ferreira da Silva, E., Cachada, A., and Pereira, R. (2019). Lowdensity polyethylene microplastics as a source and carriers of agrochemicals to soil and earthworms. Environ. Chem. 16 (1), 8. doi:10.1071/en18162
Sarasamma, S., Audira, G., Siregar, P., Malhotra, N., Lai, Y-H., Liang, S-T., et al. (2020). Nanoplastics cause neurobehavioral impairments, reproductive and oxidative damages, and biomarker responses in zebrafish: throwing up alarms of wide spread health risk of exposure. Int. J. Mol. Sci. 21 (4), 1410. doi:10.3390/ijms21041410
Sarker, A., Deepo, D. M., Nandi, R., Rana, J., Islam, S., Rahman, S., et al. (2020). A review of microplastics pollution in the soil and terrestrial ecosystems: a global and Bangladesh perspective. Sci. Total Environ. 733, 139296. doi:10.1016/j.scitotenv.2020.139296
Schächtele, K. (2020). Atlas del plástico. Datos y cifras sobre el mundo de los polímeros sintéticos. México: Heinrich Böl. Primera edición México.
Schirinzi, G. F., Pérez-Pomeda, I., Sanchís, J., Rossini, C., Farré, M., and Barceló, D. (2017). Cytotoxic effects of commonly used nanomaterials and microplastics on cerebral and epithelial human cells. Environ. Res. 159, 579–587. doi:10.1016/j.envres.2017.08.043
Schröter, L., and Ventura, N. (2022). Nanoplastic toxicity: insights and challenges from experimental model systems. Small 18, 2201680. doi:10.1002/smll.202201680
Singh, S., Malyan, S. K., Maithani, C., Kashyap, S., Tyagi, V. K., Singh, R., et al. (2023). Microplastics in landfill leachate: occurrence, health concerns, and removal strategies. J. Environ. Manag. 342, 118220. doi:10.1016/j.jenvman.2023.118220
Sökmen, T. Ö., Sulukan, E., Türkoğlu, M., Baran, A., Özkaraca, M., and Ceyhun, S. B. (2020). Polystyrene nanoplastics (20 nm) are able to bioaccumulate and cause oxidative DNA damages in the brain tissue of zebrafish embryo (Danio rerio). Neurotoxicology 77, 51–59. doi:10.1016/j.neuro.2019.12.010
Sommer, F., Dietze, V., Baum, A., Sauer, J., Gilge, S., Maschowski, C., et al. (2018). Tire abrasion as a major source of microplastics in the environment. Aerosol Air Qual. Res. 18, 2014–2028. doi:10.4209/aaqr.2018.03.0099
The Associated Press (2022). July 1. India begins to ban single-use plastics including cups and straws NPR. Avaliable At: https://www.npr.org/2022/07/01/1109476072/india-plastics-ban-begins (Accessed April 04, 2023).
The National Academies of Sciences, Engineering, and medicine (2022). “Reckoning with the U.S. Role,” in Global Ocean plastic waste (Washington, DC: The National Academies Press). doi:10.17226/26132
Thomas, R. T., Nair, V., and Sandhyarani, N. (2013). TiO2 nanoparticle assisted solid phase photocatalytic degradation of polythene film: a mechanistic investigation. Colloids Surfaces A Physicochem. Eng. Aspects 422, 1–9. doi:10.1016/j.colsurfa.2013.01.017
Trudsø, L. L., Nielsen, M. B., Hansen, S. F., Syberg, K., Kampmann, K., Khan, F. R., et al. (2022). The need for environmental regulation of tires: challenges and recommendations. Environ. Pollut. 311 (15), 119974. doi:10.1016/j.envpol.2022.119974
Uheida, A., Mejía, H. G., Abdel-Rehim, M., Hamd, W., and Dutta, J. (2020). Visible light photocatalytic degradation of polypropylene microplastics in a continuous water flow system. J. Hazard. Mater. 406, 124299. doi:10.1016/j.jhazmat.2020.124299
UNDP (United Nations Development Report) (2023). United nations, human development index (HDI). Avaliable At: https://hdr.undp.org/data-center/human-development-index#/indicies/HDI consultado 3 mayo 2023 (Accessed April 04, 2023).
van den Berg, P., Huerta-Lwanga, E., Corradini, F., and Geissen, V. (2020). Sewage sludge application as a vehicle for microplastics in eastern Spanish agricultural soils. Environ. Pollut. 261, 114198. doi:10.1016/j.envpol.2020.114198
Wang, J., Liu, X., Li, Y., Powell, T., Wang, X., Wang, G., et al. (2019). Microplastics as contaminants in the soil environment: a mini-review. Sci. Total Environ. 691, 848–857. doi:10.1016/j.scitotenv.2019.07.209
Weber, C. J., Opp, C., Prume, J. A., Koch, M., Andersen, T. J., and Chifflard, P. (2022). Deposition and in-situ translocation of microplastics in floodplain soils. Sci. Total Environ. 819, 152039. doi:10.1016/j.scitotenv.2021.152039
WEF (World Economic Forum) (2023). Charted: the key countries that trade in global plastic waste. Avaliable At: https://www.weforum.org/agenda/2023/03/charted-the-flow-of-global-plastic-waste/ (Accessed April 04, 2023).
WEF (World Economic Forum) (2016). The new plastics economy: rethinking the future of plastics Cologny. Geneva, Switzerland: World Economic Forum.
WHO (World Health Organization) (2019). Microplastics in drinking-water. Geneva: Licence: CC BY-NC-SA 3.0 IGO.
Wik, A., and Dave, G. (2009). Occurrence and effects of tire wear particles in the environment – a critical review and an initial risk assessment. Environ. Pollut. 157 (1), 1–11. doi:10.1016/j.envpol.2008.09.028
Wong, J. K. H., Lee, K. K., Tang, K. H. D., and Yap, P. S. (2020). Microplastics in the freshwater and terrestrial environments: prevalence, fates, impacts and sustainable solutions. Sci. Total Environ. 719, 137512. doi:10.1016/j.scitotenv.2020.137512
World Bank (2021). Databank files: population. Avaliable At: https://databankfiles.worldbank.org/public/ddpext_download/POP.pdf Consulted (Accessed April 04, 2023).
World population review (2023). Plastic pollution by country 2023: total MPW created 2021. Avaliable At: https://worldpopulationreview.com/country-rankings/plastic-pollution-by-country (Accessed April 04, 2023).
Worm, B., Lotze, H. K., Jubinville, I., Wilcox, C., and Jambeck, J. (2017). Plastic as a persistent marine pollutant. Annu. Rev. Environ. Resour. 42, 1–26. doi:10.1146/annurev-environ-102016-060700
Wright, S. L., Ulke, J., Font, A., Chan, K. L. A., and Kelly, F. J. (2020). Atmospheric microplastic deposition in an urban environment and an evaluation of transport. Environ. Int. 136, 105411. doi:10.1016/j.envint.2019.105411
Wu, B., Wu, X., Liu, S., Wang, Z., and Chen, L. (2019). Size-dependent effects of polystyrene microplastics on cytotoxicity and efflux pump inhibition in human Caco-2 cells. Chemosphere 221, 333–341. doi:10.1016/j.chemosphere.2019.01.056
Wu, M., Yang, C., Du, C., and Liu, H. (2020). Microplastics in waters and soils: occurrence, analytical methods and ecotoxicological effects. Ecotoxicol. Environ. Saf. 202, 110910. doi:10.1016/j.ecoenv.2020.110910
Yan, Z., Liu, Y., Zhang, T., Zhang, F., Ren, H., and Zhang, Y. (2022). Analysis of microplastics in human feces reveals a correlation between fecal microplastics and inflammatory bowel disease status. Environ. Sci. Technol. 56 (1), 414–421. doi:10.1021/acs.est.1c03924
Yang, T., Luo, J., and Nowack, B. (2021). Characterization of nanoplastics, fibrils, and microplastics released during washing and abrasion of polyester textiles. Environ. Sci. Technol. 55 (23), 15873–15881. doi:10.1021/acs.est.1c04826
Yang, X., Bento, C. P. M., Chen, H., Zhang, H., Xue, S., Lwanga, E. H., et al. (2018). Influence of microplastic addition on glyphosate decay and soil microbial activities in Chinese loess soil. Environ. Pollut. 242, 338–347. doi:10.1016/j.envpol.2018.07.006
Yang, X., Lwanga, E. H., Bemani, A., Gertsen, H., Salanki, T., Guo, X., et al. (2019). Biogenic transport of glyphosate in the presence of LDPE microplastics: a mesocosm experiment. Environ. Pollut. 245, 829–835. doi:10.1016/j.envpol.2018.11.044
Yin, L. S., Wen, X. F., Du, C. Y., Jiang, J., Wu, L. X., Zhang, Y., et al. (2020). Comparison of the abundance of microplastics between rural and urban areas: a case study from east dongting lake. Chemosphere 244, 125486–125492. doi:10.1016/j.chemosphere.2019.125486
Yu, H., Chin, M., Yuan, T., Bian, H., Remer, L. A., Prospero, J. M., et al. (2015). The fertilizing role of african dust in the amazon rainforest: a first multiyear assessment based on data from cloud-aerosol lidar and infrared pathfinder satellite observations. Geophys. Res. Lett. 42, 1984–1991. doi:10.1002/2015GL063040
Yu, L., Zhang, J., Liu, Y., Chen, L., Tao, S., and Liu, W. (2021). Distribution characteristics of microplastics in agricultural soils from the largest vegetable production base in China. Sci. Total Environ. 756, 143860. doi:10.1016/j.scitotenv.2020.143860
Zauli Sajani, S., Bonasoni, P., Cristofanelli, P., Marinoni, A., and Lauriola, P. (2012). Only coarse particles from the Sahara? Epidemiology 23 (4), 642–643. doi:10.1097/EDE.0b013e318258c23f
Zhang, G. S., and Liu, Y. F. (2018). The distribution of microplastics in soil aggregate fractions in southwestern China. Sci. Total Environ. 642, 12–20. doi:10.1016/j.scitotenv.2018.06.004
Zhang, M., Zhao, Y., Qin, X., Jia, W., Chai, L., Huang, M., et al. (2019). Microplastics from mulching film is a distinct habitat for bacteria in farmland soil. Sci. Total Environ. 688, 470–478. doi:10.1016/j.scitotenv.2019.06.108
Zhao, L., Qu, M., Wong, G., and Wang, D. (2017). Transgenerational toxicity of nanopolystyrene particles in the range of μg L−1 in the nematode Caenorhabditis elegans. Environ. Sci. Nano. 4 (12), 2356–2366. doi:10.1039/C7EN00707H
Zhao, X., Zhou, Y., Liang, C., Song, J., Yu, S., Liao, G., et al. (2023). Airborne microplastics: occurrence, sources, fate, risks and mitigation. Sci. total Environ. 858 (2), 159943. doi:10.1016/j.scitotenv.2022.159943
Zhou, J., Gui, H., Banfield, C. C., Wen, Y., Zang, H., Dippold, M. A., et al. (2021). The microplastisphere: biodegradable microplastics addition alters soil microbial community structure and function. Soil Biol. Biochem. 156, 108211. doi:10.1016/j.soilbio.2021.108211
Zhou, Y., Kumon, R. E., Cui, J., and Deng, C. X. (2009). The size of sonoporation pores on the cell membrane. Ultrasound Med. Biol. 35 (10), 1756–1760. doi:10.1016/j.ultrasmedbio.2009.05.012
Zhou, Y., Liu, X., and Wang, J. (2019). Characterization of microplastics and the association of heavy metals with microplastics in suburban soil of central China. Sci. Total Environ. 694, 133798. doi:10.1016/j.scitotenv.2019.133798
Zhu, H. (2022). October 21. EU officially released a draft restricting the use of microplastics in 871 cosmetics. CIRS. Avaliable At: https://www.cirs-group.com/en/cosmetics/eu-officially-released-a-draft-restricting-the-use-of-microplastics-in-cosmetics.
Keywords: microplastics, nanoplastics, mismanaged plastics, legislation, gaps, study opportunities, public awareness, socio-economic aspects
Citation: Osuna-Laveaga DR, Ojeda-Castillo V, Flores-Payán V, Gutiérrez-Becerra A and Moreno-Medrano ED (2023) Micro- and nanoplastics current status: legislation, gaps, limitations and socio-economic prospects for future. Front. Environ. Sci. 11:1241939. doi: 10.3389/fenvs.2023.1241939
Received: 17 June 2023; Accepted: 07 August 2023;
Published: 22 August 2023.
Edited by:
Buddhi Wijesiri, Queensland University of Technology, AustraliaReviewed by:
Elisabetta Giorgini, Marche Polytechnic University, ItalyAn Liu, Shenzhen University, China
Copyright © 2023 Osuna-Laveaga, Ojeda-Castillo, Flores-Payán, Gutiérrez-Becerra and Moreno-Medrano. This is an open-access article distributed under the terms of the Creative Commons Attribution License (CC BY). The use, distribution or reproduction in other forums is permitted, provided the original author(s) and the copyright owner(s) are credited and that the original publication in this journal is cited, in accordance with accepted academic practice. No use, distribution or reproduction is permitted which does not comply with these terms.
*Correspondence: Edgar David Moreno-Medrano, edgar.mmedrano@academicos.udg.mx
†These authors have contributed equally to this work