Soil toposequences, soil erosion, and ancient Maya land use adaptations to pedodiversity in the tropical karstic landscapes of southern Mexico
- 1Departamento de Ciencias Ambientales y del Suelo, Instituto de Geología, Universidad Nacional Autónoma de México (UNAM), Mexico City, Mexico
- 2Laboratorio de Biogeoquímica, Instituto de Investigación en Ecosistemas y Sustentabilidad (IIES), Universidad Nacional Autónoma de México (UNAM), University Campus Morelia, Morelia-Michoacán, Mexico
- 3Posgrado en Ciencias de la Tierra, Universidad Nacional Autónoma de México (UNAM), Mexico City, Mexico
- 4Faculty of Geography, Autonomous University of the State of Mexico, Toluca, Mexico
- 5Department of geomagnetism and Exploration, Institute of Geophysics, Universidad Nacional Autónoma de México (UNAM), Mexico City, Mexico
- 6Department of Anthropology, University of California Riverside, Riverside, CA, United States
- 7HDR, Inc., San Diego, CA, United States
- 8Department of Anthropology, University of Brandeis, Massachusetts, MA, United States
- 9Department of Anthropology, Brown University, Rhode Island, RI, United States
- 10Departamento de Arqueología, Instituto de Investigaciones Antropológicas, Universidad Nacional Autónoma de México (UNAM), Mexico City, Mexico
The soil mantle of the tropical karst landscapes of southern Mexico was a key resource for ancient Maya agriculture and experienced deep transformation due to long-term human impacts under changing environmental conditions. We conducted a comparative analysis of three compound soil toposequences in mountainous (Sierra de Chiapas/Middle Usumacinta Valley, Busiljá, and Chinikihá archaeological sites) and platform (NE Yucatán Peninsula, Yalahau region) karst landscapes to reconstruct general tendencies and regional variations in pedodiversity development and soil–human interactions since the Early Preclassic Period. Toposequence characterization is based on macro- and micromorphological observations, accompanied by a suite of laboratory data. Calcareous upland geoforms of all toposequences have similar soil combinations consisting of shallow Rendzina and deep red clayey Terra Rossa types of profiles. We argue that Rendzinas, now dominant in the upland soil cover, in most cases, are not a product of incipient pedogenesis on limestone; they have developed from the residues of Terra Rossa soils after their advanced erosion. Pedosediments generated by ancient soil erosion have been found in the piedmont and depression positions in the mountainous landscapes of Chiapas, as a result of lateral downslope soil removal, and in the subsurface karstic cavities in the platform of NE Yucatán, indicating vertical “soil piping.” The soils of the lowland domains show contrasting differences between the toposequences: gleyic clay–rich soils and humic alluvial soils prevail in Chinikihá and Busiljá, whereas hydromorphic carbonate soils have formed in Yalahau karstic depressions. These differences in the lowland soil properties led to divergent ancient Maya land use strategies; in Chinikihá and Busiljá, the major agricultural domain was developed in the lowlands, implying largescale artificial drainage. On the contrary, in Yalahau, mostly upland Rendzinas were cultivated, implying “precision agriculture” and “container gardening.”
1 Introduction
Understanding the complex interaction between past societies and the soil mantle is one of the primary goals of paleopedology. Soils were a crucial resource for ancient economies, forming the basis for agriculture and providing raw materials for various industries and crafts (buildings, ceramic production, etc.). Human activities also impacted and transformed soils, affecting their biological quality and capacity to perform ecological functions and services, and creating feedback loops that influenced environmental management decisions and sociopolitical dynamics. Investigating these topics requires detailed research into the pedodiversity and structure of the soil mantle that supported these ancient cultures and registered their impact.
The tropical humid and subhumid regions of southern Mexico, together with the adjacent territories of Central America, witnessed the development of Maya civilization between circa 2,000 BC and AD 1,500. Among other hallmarks such as divine kingship, art, monumental architecture, hieroglyphic writing, and a detailed knowledge of math and astronomy, the Maya implemented various intensive agricultural strategies to support cities with populations in the tens of thousands for millennia. Maya agricultural and natural resource management, especially the utilization of soil resources, has been the subject of numerous previous investigations (Fedick, 1995; Dunning et al., 1998; Beach et al., 2006; Beach et al., 2002; Anselmetti et al., 2007; Scarborough et al., 2012; Douglas et al., 2015; Douglas et al., 2018; Walden et al., 2023). Nevertheless, our understanding of how the Maya adapted their agrosystems to specific, unique, and sometimes difficult regional soil conditions is still limited and warrants additional research to understand these processes more fully.
The evolutionary trajectory of Maya civilization is complex; generally, long intervals of progress are punctuated by socially or environmentally rooted setbacks that were sometimes catastrophic in nature. The most well known of these, the Terminal Classic collapse (during which cities in the southern Maya Lowlands experienced demographic, sociocultural, and political decline and abandonment) has attracted broad scientific and public attention and has inspired numerous scholars to propose scenarios explaining its cause. Currently, the most popular scenario is based on climatic forcing. A severe drought (or set of droughts) at the end of the first millennium AD is assumed to have significantly impacted crop production and caused a shortage in food supply, although there is no consensus on its severity of impact on Maya agriculture (Hodell et al., 2001; Dunning et al., 2012; Fedick and Santiago, 2022; Islebe et al., 2022). The “Maya drought” left a signal in the marine (Haug et al., 2003), lacustrine (Hodell et al., 2005; Douglas et al., 2016; Krywy-Janzen et al., 2019), and speleological (Medina-Elizalde et al., 2010) records.
Another version links the Terminal Classic collapse to ecological problems caused by overexploitation of resources and environmental degradation by overpopulated Maya cities. This scenario was popularized by Diamond (1994) who pointed particularly to catastrophic deforestation during the Classic Maya period.
Does soil matter for both these scenarios? Are soil properties important for the response of ancient agrosystems to water deficit caused by drought? Did deforestation and anthropogenic transformation of ecosystems during the Classic period also cause soil degradation? Studying the properties of the soil mantle in the Maya area can provide answers to these questions.
A major part of the Maya region in southern Mexico is characterized by karstic landscapes formed in the sedimentary sequences dominated by calcareous rocks. A large area of mountainous karst is related to the ridge systems of Chiapas, whereas an expansive area of platform karst covers the entire Yucatán Peninsula (Espinasa-Pereña, 2007). Pedogenesis occurring on karstified calcareous rocks is different from the “central image” of soil development in the humid tropics. Deep, strongly leached, and weathered ferrallitic soils that typically form in humid tropical climates on silicate materials are rare in limestone karst landscapes. Instead, limestone karst soils are frequently comprised of shallow Rendzina-type profiles with dark Ah horizons directly underlain by calcareous rock. Much more developed red soils with a high content of silicate clay and iron oxides (referred to as Terra Rossa) are also found in these landscapes. The origin of their parent material and pedogenesis are still under debate (Yaalon, 1997; Durn et al., 1999; Priori et al., 2008). The high pedodiversity of karstic soils provides both advantages and challenges for agricultural use; in turn, their “response” to cultivation is also complex and mosaic.
Over the decades of our soil–archaeological research in the Mexican part of the Maya Lowlands, we became aware that regional differences between the soil mantle structures of karstic landscapes are so great that they could have major implications for regional models of ancient land use and anthropogenic soil change. The purpose of this overview is to summarize the information about the diversity of soils and pedosediments controlled by the geomorphological setting in mountainous and platform karst regions of southern Mexico, understand its influence on the unique distribution of land use practices, and obtain a record of soil cover transformation caused by ancient human impacts.
2 Methodological approach
This paper summarizes the results of paleopedological and soil–archaeological research conducted during more than 20 years in the Mexican part of the Maya Lowlands. This research was related to archaeological projects carried out by teams from different scientific institutions at important ancient Maya cities or regions: Chinikihá (Instituto de Investigaciones Antropológicas UNAM) and Busiljá (Brandeis University) in Chiapas, and the settlements of the Yalahau region (University of California Riverside) in northern Quintana Roo. These projects dealt with archaeological contexts of different occupation periods. Although there is no uniform chronology covering the entire Maya territory, the following general periodization was adopted in this work: Middle Preclassic, from 1,000 to 350 BC; Late Preclassic, from 350 BC to AD 250; Early Classic, from AD 250 to 550; Middle Classic, from AD 550 to 830; Late Classic, from AD 830 to 950; Postclassic, from AD 950 to 1,539. Nearly all these results have been published in various articles, books, and theses and presented at national and international conferences (cited in the Results section). However, they have always been considered separately from each other and interpreted in the context of local pedological, paleoecological, and geoarchaeological research issues.
In this paper, we present an integrated interpretation of our results on soil diversity from different areas, united by their belonging to the family of landscapes strongly affected by karstic processes under (sub) humid tropical bioclimatic conditions. The soil classification of these works is based on the IUSS Working Group (IUSS Working Group WRB, 2015); this system is adopted by INEGI (Instituto Nacional de Estadítica y Geografía) for soil mapping. This approach is motivated by the idea that the integration of results from various sites united by certain geological, environmental, pedological, and historical similarities, although different in various aspects, will produce a “synergistic effect” and help generate new ideas about evolution of soil formation and its complex interactions with natural and anthropogenic factors that cannot be derived from individual local investigations.
Toposequences (also referred to as soil catenas, although these are not complete synonyms) are a traditional approach to representing soil diversity and geomorphological regularities of the soil mantle structure and have also been proven to be useful for pedoarchaeological research in the Maya region (Beach, 1998). In the results, we present three compound soil toposequences representing the structure of the soil mantle in two areas of Sierra de Chiapas/Middle Usumacinta Basin and one in the northeastern Yucatán Peninsula (Figure 1). The toposequences include soils and pedosediments developed in different geomorphic positions of karstic landscapes which include subsurface cavities and, in the case of Usumacinta, soils of the adjacent alluvial domain. We accompany field morphological descriptions with micromorphological characteristics of key diagnostic features of pedogenetic processes in the studied profiles. We consider micromorphology to be the most powerful tool for detecting pedogenetic processes, especially in cases of incipient soils, complex polygenetic profiles, and redeposited soil materials. Thin sections were prepared from undisturbed soil blocks after impregnation with crystal resin, the observations were made under the petrographic microscope Olympus BX50 equipped with a digital camera connected to a computer. The descriptions were based on the micromorphological concepts and terminology used by Stoops (2018). We used the Image-Pro Plus 7.0 software for handling the microscopic images. We also supply the outline of physicochemical and mineralogical results, published in full elsewhere.
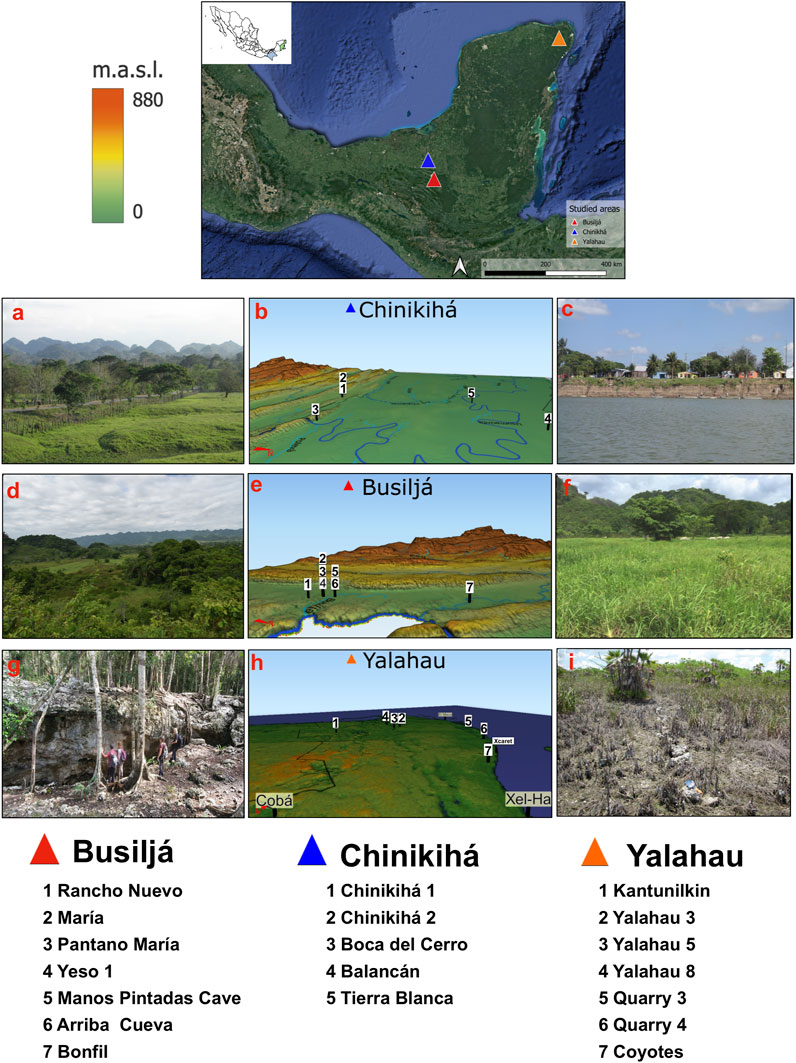
FIGURE 1. Southern Mexico with the location of three studied toposequences, relief models with location of profiles, and landscape photos. Middle Usumacinta toposequence: (A) upper terrace of Usumacinta and (B) google maps with locations of the profiles; (C) lower terrace of Usumacinta. Sierra de Chiapas and Busiljá-Chocoljá toposequence; (D) calcareous hills; (E) google maps with locations of the profiles (F) swampy karstic depression. Northwestern Yucatán–Yalahau toposequence; (G) forested upland landscape with the collapse structure; (H) google maps with locations of the profiles and (I) swampy lowland.
We use the presented results to discuss the general tendencies and regional variations of 1) development of the soil mantle resulting from the interaction of pedogenesis and geomorphic processes; 2) influence of soil diversity on the special differentiation of ancient land use practices; and 3) the impact of ancient land use on soils and possible feedback effects of human-induced soil change on economic and social processes. Part of our interpretations have preliminary or hypothetic character: they are not sufficiently proven by the available results and are suppositions, which require verification. However, we think that the ideas of such kind should be presented and discussed because of their potential importance for the orientation of future research.
3 Results
3.1 Soil toposequences of karstified mountainous tropical landscapes: Usumacinta Basin
3.1.1 Geological and environmental setting
The Sierra de Chiapas, where the Chinikihá and Palenque archaeological sites are located, is constituted by sedimentary rocks (shales, sandstones, and limestones) with ages ranging from the Jurassic to Paleogene (Hernández-Santana et al., 2012) These rock sequences were folded and faulted during the Miocene and are also affected by neotectonics (Burkart, 1983; Authemayou et al., 2012), which has given rise to a complex relict , but locally rejuvenated (Andreani and Gloaguen, 2016), tectonic, and karstic relief with fold-and-thrust belts, dolines, uvalas, cockpits, and rock cliffs (Figures 1A, D). In consequence, the valleys are straight and aligned and cut mountainous orographic axes, fault escarpments, and pressure ridges (Ortiz et al., 2005). The Sierra de Chiapas comprises the largest area of mountainous tropical karst in Mexico (Espinasa-Pereña, 2007). During the Pliocene and Pleistocene, alluvial processes formed the Usumacinta Basin that extended from northwestern Guatemala to the states of Chiapas and Tabasco, in Mexico. The main river in this basin, in Mexican territory, is the Usumacinta, which descends from the ridges of the Sierra de Chiapas (Figure 1B) and passes into the coastal plain of the Gulf of Mexico at Boca del Cerro. The main tributaries of the Usumacinta River are the San Pedro River, Chakamax River, and Tulijá River (Figure 1E), which follow the lineaments of normal faults with the east–west orientation. The coastal plain, slightly inclined to the north, is constituted by clastic sediments (sands, silts, and clays) derived from the Sierra de Chiapas (Padilla and Sánchez, 2007). These sediments comprise a sequence of Pleistocene and Holocene terraces at different altitudes (West et al., 1969; Solís-Castillo et al., 2014); those formed during the Pleistocene are higher than 20 m, whereas the Holocene terraces are lower (Figure 1C).
The climate in the region is warm and humid with an annual precipitation ranging from 1,800 mm in the alluvial plain to 4,000 mm near the headwaters (INEGI, 1986). Approximately 67% of precipitation occurs in summer. The mean annual temperature is 27°C, with temperatures reaching 30°C during the hottest month (García, 1988). Vegetation is evergreen tropical rainforest (selva alta). In the floodplain areas and wetland depressions (Figure 1F), which are inundated for long periods, vegetation is dominated by grasses and aquatic species such as Bactris and Ponderia (Bueno et al., 2005; Rzedowski, 2006).
3.1.2 Cultural history and archaeological context of the region
Archaeological surveys along the alluvial plain have documented over 2,300 archaeological sites (Liendo-Stuardo et al., 2014). Ceramic investigations have identified a sequence of occupations ranging from the Middle Preclassic (800–300 BC), to the Terminal Classic (AD 850–1,000) (Liendo-Stuardo et al., 2014). A high frequency of ancient occupation since the Middle Preclassic is reported on the Tierra Blanca and Trinidad alluvial terraces where rich natural resources are available for the inhabitants (water bodies, soils for agriculture, fauna, and flora). In contrast, settlements at the foothills of the Sierra de Chiapas document shorter periods of occupation, with sparse population during the Late Preclassic. During the Early Classic period, settlements preferred the riverine environments. By the end of the Early Classic, populations occupied the foothills of the Sierra de Chiapas and intermountain valleys (Liendo-Stuardo et al., 2014).
The site of Chinikihá is located within the Northwest Lowlands region with an important presence during the Classic period, with a high population density and accumulation of political power (Liendo-Stuardo et al., 2014). The first recognitions and reports of the archaeological site of Chinikihá were found in the manuscripts of Maler (1901) and Berlin-Neubart (1955). The site consists of a central sector comprising approximately 7.5 ha, where structures of a civic-ceremonial or special function, such as the ball court, palace, double temples, and South Acropolis are located around two large plazas. The residential area surrounds the previous one and consists of housing units of different types. Chinikihá displays a radial distribution pattern, with greater nucleation toward the center and a progressive dispersion in the direction of the periphery of the site (Campiani et al., 2012; Liendo-Stuardo, 2012).
The Busiljá area has been occupied by the Maya communities for millennia, with identified sedentary communities dating to as early as the Middle Preclassic period and occupation continuing through historical times. The largest pre-Colonial populations are likely associated with the Classic period (Golden et al., 2021). The cultural history of this region during this period was significantly influenced by the political dynamics of the kingdoms of Palenque, Piedras Negras, Tonina, and La Mar (Martin and Grube, 2008; Houston and Inomata, 2009). Most of the Classic period settlements were abandoned after AD 950, and regional populations were sparse until the 20th century (Golden et al., 2008; Scherer and Golden, 2012). The archaeological pedestrian and airborne LiDAR surveys carried out by the Proyecto Arqueológico Busiljá-Chocoljá (PABC) for more than a decade have exposed the settlement pattern of the Preclassic and Classic periods of Maya in the valley surrounding the Busiljá River, a tributary of the Usumacinta. This pattern divides the space into two functionality differentiated areas where the residential, political, and social architecture (houses, temples, ball courts) are grouped in low rises and uplands, whereas the agricultural structures such as channels and some terraces are found in the seasonal wetlands and lower hillslopes (Golden et al., 2021).
3.1.3 Soils and paleosols at key geoforms of middle Usumacinta Basin
For this study, we have considered various pedological sections to construct a toposequence from the calcareous hills of Sierra de Chiapas to the alluvial plain (Figure 2), previously studied by Solís-Castillo et al. (2013a), Solís-Castillo et al. (2013b), Solís-Castillo et al. (2014), Liendo-Stuardo et al. (2014), and Solleiro-Rebolledo et al. (2015).
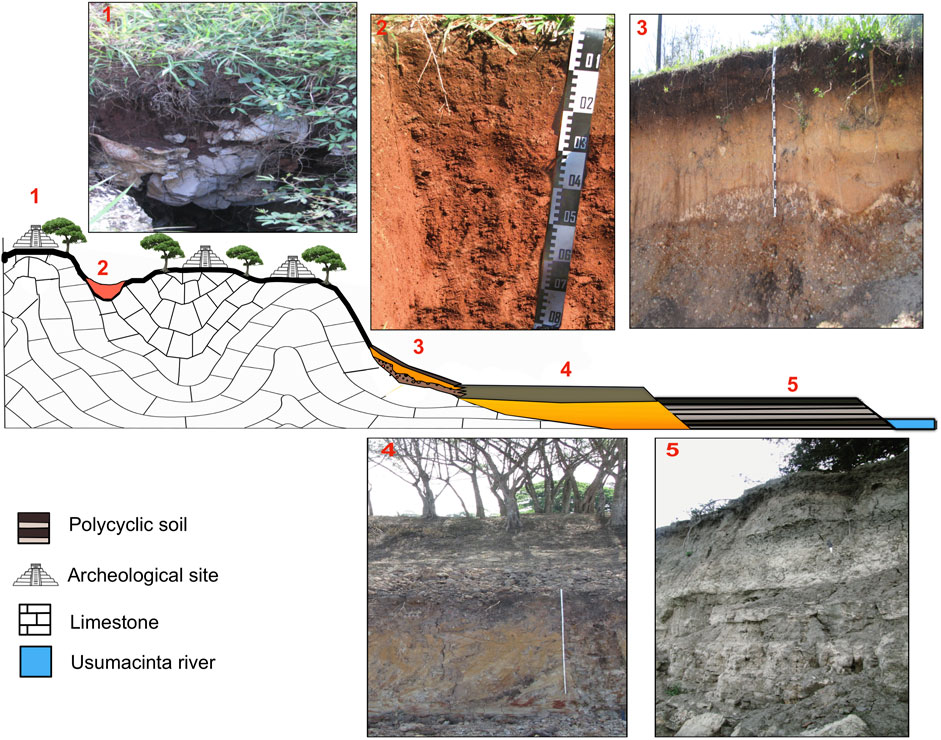
FIGURE 2. Middle Usumacinta toposequence: general scheme and profile photographs. 1. Chinikihá 1 profile (Rendzic Leptosol); 2. Chinikihá 2 profile (Chromic Luvisol); 3. Boca del Río profile with a polycyclic soil; 4. Balancán profile in the alluvial plain; 5. Tierra Blanca profile, alluvial sediments intercalated with paleosols.
In the hilly karstic relief at the edge of the Sierra de Chiapas, in Chinikihá, we consider two profiles: Chinikihá 1 and Chinikihá 2 (Liendo-Stuardo et al., 2014). Chinikihá 1 is a thin Rendzic Leptosol found on the hillslope, at a higher elevation. The brownish-black, loose, granular AB horizon of variable thickness (max. 40 cm) has an abrupt contact with the fragmented limestone bedrock. Chinikihá 2 is a deeper Chromic Luvisol developed in the bottom of the closed karstic depression. The reddish, compact, clayey A (upper 10 cm), and Bt horizons account for a total thickness of 150 cm and are structured in hard blocks separated by fissures.
The Boca del Cerro profile represents the soil-sedimentary sequence developed at the piedmont of Sierra de Chiapas on a colluvial fan underlain by fluvial sediments (Solís-Castillo et al., 2014). The modern surface Calcaric Phaeozem has a thick (75 cm) dark humus A horizon formed on colluvium with abundant limestone fragments. Below this lies a well-developed buried paleosol with a reddish clayey Btk horizon, which has both clay coatings and white soft carbonate nodules. It is underlain by sandy colluvial and alluvial deposits.
The river terrace domain is represented by two profiles: Balancán and Tierra Blanca. The Balancán profile is representative of the soil cover of a higher alluvial plain (Solís-Castillo et al., 2014). It is a Stagnosol with an Ag-Bg-Cr horizons having sandy-clayey texture, being free of carbonates and showing strong redoximorphic features: grayish brown, reddish-yellowish, and greenish mottles, dendritic Mn coatings on aggregates, and ferruginous concretions of Fe.
The Tierra Blanca profile that is exposed in a cut in the riverbank documents pedogenesis at a lower Holocene alluvial terrace (Solís-Castillo et al., 2013a). It shows a sequence of modern soil and six paleosols interbedded with alluvial sediments. The lower paleosols 4, 5, 6, and 7 show strong redoximorphic features; however, they also contain carbonate concretions (Solís-Castillo et al., 2013a). This lower welded gleyic paleosol sequence, forming a pedocomplex, is buried by a sorted laminated sediment enriched with pyroclastic materials (Cabadas-Báez et al., 2010). Only the upper two paleosols, 2A-2AB-2C and 3A-3AB-3BC, contain abundant artifacts and ceramics from each of these paleosols link them to the Classic and Preclassic periods, respectively (Solís-Castillo et al., 2013a). The Preclassic paleosol is the most developed and has a angular blocky structure in the 3A horizon. The Classic paleosol and modern soil are incipient fluvisols with thin, gray, granular A horizons.
3.1.4 Micromorphological observations in selected soil horizons at middle Usumacinta Basin
The micromorphology of the Chinikihá 1AB horizons (Rendzic Leptosol) shows a dark brown pigmentation of the groundmass, granular structure, and high porosity (Figure 3A). Calcareous rock fragments, abundant traces of fine roots, and coprolites are identified. In addition to primary carbonates, few silicate minerals—hornblende, augite, plagioclase, and small quartz, which are strongly weathered, are identified within the coarse fraction. In the case of the Chinikihá 2 profile (Luvisol), the groundmass is reddish and clayey in all horizons and primary carbonates are absent. In the Bt horizon, a composite structure of subangular blocks and granular aggregates is observed. Some pores have infillings of secondary calcite (Figure 3B). Frequently, dark opaque grains with rounded or angular shapes are incorporated into a clayey groundmass; most of these are small nodules of iron or manganese oxides (Solleiro-Rebolledo et al., 2015).
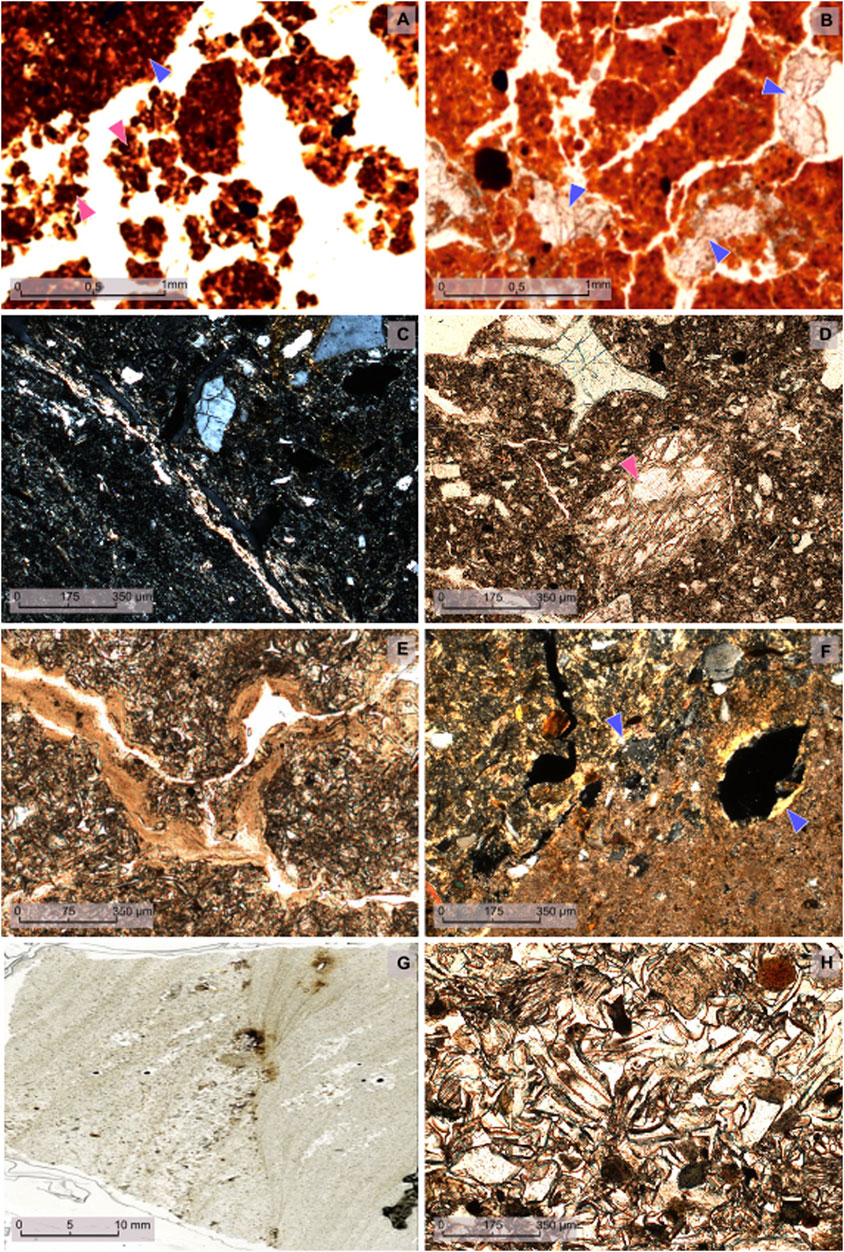
FIGURE 3. Photomicrographs of the Middle Usumacinta toposequence, selected horizons; PPL, plane polarized light; XPL, cross polarized light. (A) Chinikihá Rendzina profile: 1AB horizon large reddish soil aggregates (blue arrowheads) transformed into smaller coprolitic aggregates (pink arrowheads) (PPL); (B) Luvisol profile: Bt3 horizon calcite infillings in pores (blue arrowheads) (PPL); (C) Tierra Blanca profile: 3A horizon porostriated b-fabric (XPL); (D) Tierra Blanca profile: 3A horizon groundmass with weathered volcanic glass (pink arrowhead) (PPL); (E) Tierra Blanca profile: 3AB horizon continuous clay coatings over pore walls; (F) Tierra Blanca profile: 7Bkg horizon small partly deformed clay coatings (blue arrowheads) (XPL); (G) Tierra Blanca profile: silty sediments (scanned section); (H) Tierra Blanca profile: volcanic glass in the silty sediments (PPL).
The most relevant micromorphological observations were made in selected horizons of the Tierra Blanca profile described by Solís-Castillo et al. (2015). They reveal sharp differences between the A horizons of the upper paleosols: the A and 2A horizons (from the modern soil and the Classic paleosol, respectively) are granular and porous, whereas the 3A horizon (Preclassic paleosol) has a clayey-silty groundmass and an angular blocky structure with porostriated b-fabric (Figure 3C). Weathered volcanic glass shards are also present (Figure 3D). In the 3AB horizon, few well-developed illuvial clay coatings cover the walls of fissures (Figure 3E). The lower gleyic paleosol pedocomplex is very clayey; however, it contains some quartz grains, giving rise to porphyric coarse/fine related distribution. A few strongly altered micas are also observed. Clay coatings are frequent, however, most of them are deformed. The silty sediment between the upper humic and lower gleyic paleosols is laminated (Figure 3F); the striking feature of this deposit is that the dominant material is fresh volcanic glass (Figure 3G).
3.1.5 Soils and pedosediments at key geoforms of Sierra de Chiapas and minor valleys of Usumacinta tributaries (Busiljá-Chocoljá)
To construct the second toposequence, we used the results of soil research developed in the framework of the Busiljá archaeological project. The profiles of soils developed in the upland and lowland geomorphic positions and underground pedosediments in the area around the Busiljá archaeological site were complemented by the section on the alluvial terrace of the Chocoljá river, the next downstream tributary of the Usumacinta after Busiljá (Figure 2E). Major parts of the results reported here were previously presented at conferences (Sedov et al., 2021) and published in the master’s thesis of Guillén (2020).
In the Busiljá area, three profiles represent upland soils formed on limestone hills above 120 m a.s.l.: Rancho Nuevo, Maria, and Arriba Cueva (Figure 4). The Rancho Nuevo profile (Rendzic Leptosol) is located on a small natural terrace situated on the slope close to archaeological structures on the summit. The Maria profile (Calcaric Cambisol) is also on the slope of a minor calcareous hill, near the nuclear part of the Busiljá archaeological site. Both profiles are shallow and have Ah horizons that are dark brownish-gray due to humus pigmentation and granular structure; the underlying AC horizon contains abundant limestone fragments and rests over continuous rock. The Maria profile (Cambisol) also has reddish Bw and BC horizons, restricted however to a narrow but deep karstic pocket. In this profile, artifacts of bone and ceramic sherds are frequent even in the lowermost BC horizon. The Arriba Cueva profile (Calcaric Chromic Cambisol) is located directly above the Manos Pintadas Cave on top of another limestone hill. Its environmental setting is different: the soil is developed under a mature tropical forest and has no evidence of past or modern anthropic disturbance. This profile is deeper, with its upper part leached of carbonates, and below the dark brown humic topsoil lies a continuous brownish red, clayey Bw horizon.
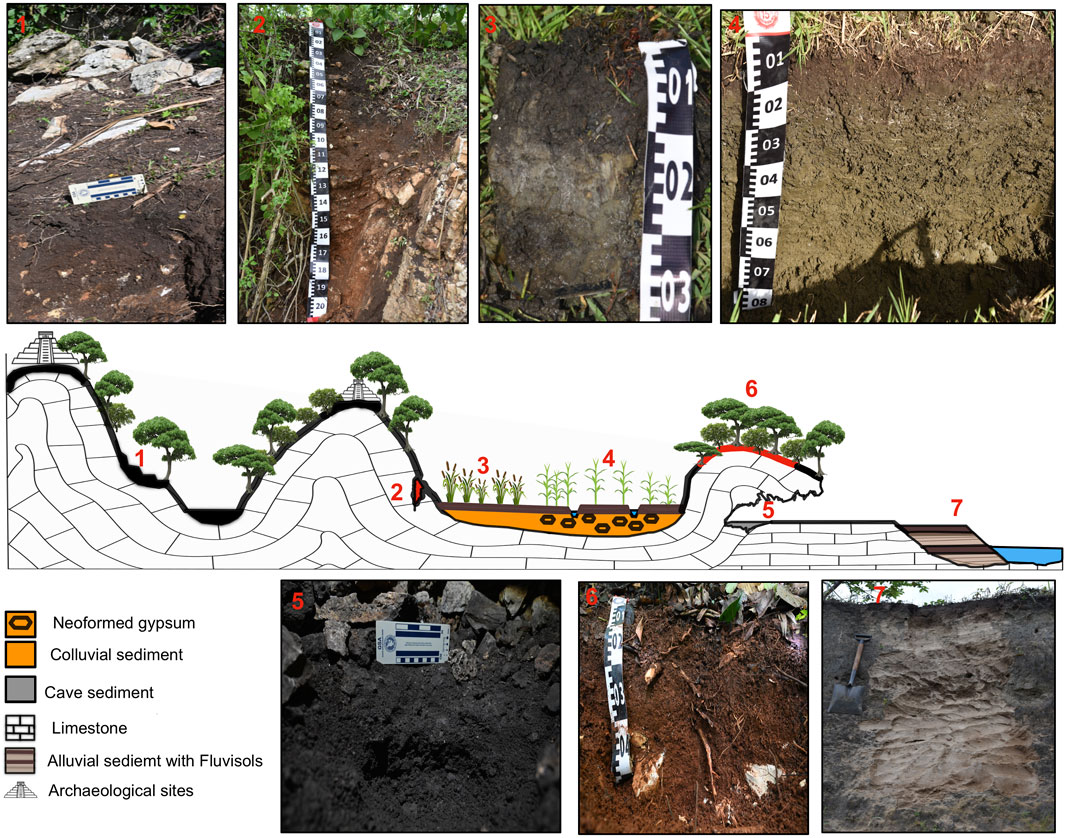
FIGURE 4. Sierra de Chiapas, Busiljá-Chocoljá toposequence: general scheme and profile photographs. 1. Rancho Nuevo profile with Rendzic Leptosol; 2. Maria profile with Calcaric Cambisol; 3. Pantano Maria profile with Histic, Stagnic gleysol; 4. Yeso 1 profile with Gypsic, Reductic gleysol; 5. Pedosediment profile in the cave Manos Pintadas; 6. Arriba Cueva profile with Calcaric Chromic Cambisol; 7. Bonfil profile with Calcaric fluvisols.
Two profiles—Yeso 1 and Pantano Maria—document lowland soils within the Busiljá area at the bottom of broad karstic depression beside the limestone hills. These depressions are already deep enough to be affected by the regional groundwater table and have accumulated enough clayey pedosedimentary material to reduce the internal soil drainage. Traces of probable archaeological agricultural canals have been detected within this swampy area. Yeso 1 is located at a slightly elevated part of the depression, whereas Pantano Maria is in the lowest position; the groundwater table was encountered at depths of 70 cm and 30 cm respectively. Both soils are gleysols showing a set of gleyic horizons that are pale greenish and indicate a poorly drained soil environment. The striking feature of the Yeso 1 profile is the presence of neoformed gypsum throughout the profile, which is completely absent in the Pantano Maria profile, despite their proximity and similar geomorphic conditions.
The Bonfil profile is exposed in the bank of the Chocoljá River, cutting the alluvial terrace that is approximately 5 m high. It is classified as a Fluvisol having the surface and buried humus horizons interlayered with laminated calcareous sandy sediments. Both Ah horizons are sandy with moderate gray humus pigmentation and weak structure. In the buried 2Ah horizon a few ceramic fragments were found.
The surface profiles are accompanied by one underground pedosediment section inside the Manos Pintadas Cave, also close to the Busiljá site. A thin pedosediment (17 cm deep) was excavated at the cave floor underneath a bed of stones produced by ceiling collapse, behind a speleothem formation. It consisted of two slightly compacted, loamy, pale reddish gray, strongly calcareous layers, the upper one having an incipient granular aggregation. Although no artifacts were found in the cave, red hands painted on the walls outside and inside the cave are visible.
3.1.6 Micromorphological observations in selected soil horizons at minor valleys of Usumacinta tributaries (Busiljá-Chocoljá)
The micromorphological observations of the A-horizons of the upland Rancho Nuevo and Maria profiles show a dark clay–humus fine material together with calcaric sand particles and some fragments of red clayey soil, free of carbonates (Figure 5A). Both profiles also contain anthropic materials: ceramic sherds, bones (Figure 5B), and charcoal fragments (Figure 5C). The Arriba Cueva profile is different from the previous profiles: its groundmass is of uniform reddish clayey composition and is free of primary carbonates (Figure 5D).
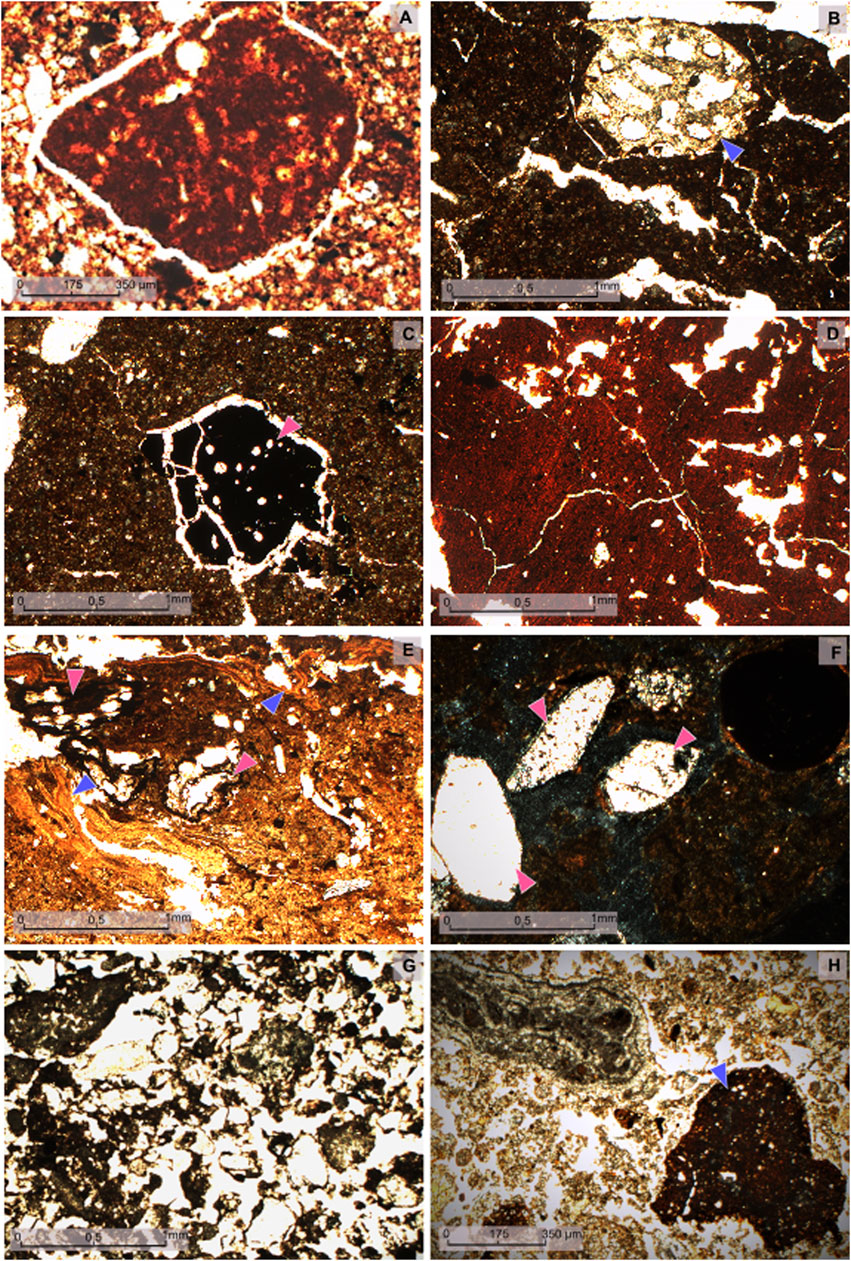
FIGURE 5. Photomicrographs of the Busiljá toposequence; PPL, plane polarized light; XPL, cross polarized light. (A) Rancho Nuevo profile red soil fragment in the Ah horizon (PPL); (B) Maria profile, a fragment of bone (blue arrowhead) in the Bkw horizon (PPL); (C) Maria profile, charcoal (pink arrowhead) in the Bkw horizon (PPL); (D) Arriba Cueva profile, angular blocky structure of Bw horizon (PPL); (E) Pantano Maria profile, clay intercalations (blue arrowheads) and plant tissue fragments (pink arrowheads) in 2A horizon (PPL); (F) Yeso 1 profile, gypsum (pink arrowheads) and iron nodule (at the right top of the photomicrograph) in By horizon (XPL); (G) Bonfil profile, abundant calcareous sand particles, dark humus fine material coats, and bridges sand grains in the 2A horizon (PPL); (H) Manos Pintadas cave pedosediment, dark soil fragment (blue arrowhead) in a carbonate groundmass, containing calcareous rock fragment (PPL).
The hydromorphic profiles in the lower zone present some specific characteristics. The upper horizon of the Pantano Maria profile contains partly decomposed organic detritus and abundant clay with striated b-fabric (Figure 5E). The conspicuous property of the Yeso 1 profile is neoformed gypsum in the form of pore infillings in the surface horizon and clusters of large tabular crystals in the By horizons combined with some redoximorphic features as ferruginous nodules and mottles (Figure 5F).
All horizons of the Bonfil profile are made up mostly of coarse calcareous sandy material. Surface and buried A horizons present fine humus, partly coating the sand grains and partly distributed in the packing voids in small aggregates (Figure 5G). The Manos Pintadas Cave sediment consists mostly of calcaric sand particles: oolites and limestone clasts with very limited presence of redeposited red soil fragments (Figure 5H), which include some small clusters of pure clay (papules).
3.1.7 Outline of physical and chemical characteristics of studied profiles
The properties of the shallow dark Leptosols and Cambisols on the slopes of calcareous hills in both regions of the Usumacinta Basin (Chinikihá 1 and Rancho Nuevo; Maria and Arriba Cueva) are neutral or slightly alkaline. Despite thinness and apparent incipient development, they are quite clayey (clay content is up 50%). By contrast, the Luvisol at the minor upland karstic depression (Chinikihá 2) is more acidic (pH is 5.3) and clayey (91% clay) (Solleiro-Rebolledo et al., 2015). The gleysols of the broad swampy depressions of the Busiljá area, Yeso 1 and Pantano Maria, are also quite clayey, but they are neutral or slightly alkaline. The Yeso 1 profile shows high values of electric conductivity reaching 2,500 μS/cm. In the colluvial profile at Boca del Cerro, the modern soil is silty (50%–63%), whereas the buried paleosol is clayey (approximately 52%–41% clay). All the horizons show an alkaline reaction.
Soils, paleosols, and sediments of the alluvial terrace sequences are in general sandier than the upland and colluvial profiles. At Balancán, developed on the higher ancient terrace, soil horizons are acidic and have a high amount of sand (39%–62%). At Tierra Blanca, on the lower Holocene terrace of the Usumacinta, the lowest pedocomplex is clayey (up to 80% clay fraction) with a slightly acidic reaction (6.8–5.5). The sediment in between the lower and upper paleosols is silty (approximately 62% silt) with a clay content close to 36% and a neutral pH. The upper paleosols have a loamy texture and slightly alkaline pH values; the clay content varies between 24% and 45%, and the sand comprises 8%–39%. The Bonfil profile at the Chocoljá River has a sandy texture.
In several profiles of the Busiljá area, clay mineral assemblages were studied with XRD analysis. The Maria profile presents vermiculite as a major component, followed by kaolinite. In the hydromorphic soils of the swampy depression (Yeso 1 and Pantano Maria), the smectitic component is dominant, followed by some vermiculite and kaolinite with traces of illite.
3.2 Soil toposequences of karstified calcareous platform: northeastern region of Yucatán Peninsula
3.2.1 Geological and environmental setting
The Yucatán Peninsula is a slightly uplifted carbonate platform composed mainly of Paleogene and Neogene limestones, dolomites, and evaporites underlain by igneous and metamorphic basement rocks (Weidie et al., 1985; Bauer-Gottwein et al., 2011). The peninsula gradually emerged, resulting in a general decrease in age of surface sedimentary rocks moving from the south center of the peninsula toward its coastal margins (Isophording, 1975; Bauer-Gottwein et al., 2011). Consequently, the Pleistocene and Holocene sediments are restricted to a narrow strip along the coast, in accordance with small long-term fluctuations in the sea level (Ward, 1985). The entire Yucatán platform covers approximately 300,000 km2 (Bauer-Gottwein et al., 2011), half of which remains underwater. Tectonic processes have a certain impact on regional geomorphology and hydrology. The main geologic features influencing groundwater movement on the Yucatán Peninsula are the Ring of Cenotes, Ticul Fault, Rio Hondo Block Fault Zone, and Holbox Fracture Zone (Bauer-Gottwein et al., 2011). The Holbox Fracture Zone is located near the eastern edge of the Yucatán Peninsula, runs for approximately 100 km from the coast in the north to the Coba lakes in the south, and has a width of 30–40 km. The surface expression of this feature includes elongated north–south trending seasonally flooded swales dominated by wetland vegetation.
The geomorphology of the Yucatán Peninsula is controlled by karstic processes which produce an undulating relief composed of structural plains and hills, with depressions and cave systems. The karstification of soluble rocks can promote subsidence and form closed depressions that, depending on the thickness of the rock, can collapse. Karst lakes (cenotes) are also abundant in the area (as they are in much of the northern peninsula in general). Extensive, stacked cave systems are also common. In uplands, due to the porous nature of limestone bedrock/karst topography, there are no surface rivers, and water percolates quickly downward. According to Aguilar et al. (2016), 6,717 sinkhole-type depressions were identified; 2,021 are of the uvala type and 76 classified as poljes.
The Yucatán Peninsula has three flanks that are surrounded by the sea, with precipitation gradients: drier with intermittent rains and maximum temperatures in summer (BS) to the north and warm subhumid with summer rains (Aw) to the south. This climatic variation influences biodiversity. The drier regions have a low thorny forest, while in the more humid environments to the south, a medium and low deciduous forest dominates. There are also plant covers associated with water bodies on the coastal areas of the peninsula, such as mangroves and specific tall grass associations in the swampy, temporally flooded depressions (Durán and Mendez, 2010).
3.2.2 Northeastern Yucatán cultural and archaeological setting overview with emphasis on Yalahau region
The northeastern Yucatán Peninsula, specifically northern Quintana Roo, has been home to the Maya people for at least 3,000 years. Two of the most well-known Maya sites in the area are Coba and Tulum. Coba, the largest site in northern Quintana Roo, was a major urban center with regional dominance during the Classic Period. Coba is notable for several major architectural groups, the tallest surviving structure in the northern lowlands (the Ixmoja temple at 42 m), dozens of sculpted monuments, and a network of more than 35 roads or sacbeob radiating out (Folan et al., 1983; Robles-Castellanos, 1990; Leyden et al., 1998; Folan et al., 2009). Tulum is one of the best-preserved Maya sites and was a key coastal trading port during the Late Postclassic period. Tulum’s cosmopolitan nature and long-distance cultural connections are evidenced by trade goods and exquisite murals, painted in the Mixteca-Puebla or international style (Perez de Heredia et al., 2021; Davis, 2022).
This review focuses on the Yalahau region of northern Quintana Roo, a freshwater wetland zone situated north of Coba, which includes an area of approximately 60 km (north-south) by 40 km (east-west) and contains over 170 wetlands of varying extent, and where a major part of the Yucatán soil toposequence was studied. The Yalahau region is a distinct physiographic zone with unique implications for agricultural development and a fairly uniform trajectory of settlement history, architectural style, and ceramic traditions (Fedick and Taube, 1995; Amador, 2005; Fedick and Mathews, 2005), where more than 100 sites have been documented (Glover, 2012). Ceramics and radiocarbon dates from Yalahau settlements and cave sites indicate the region was initially occupied in the Middle Preclassic period, ca. 700–200 BC, however evidence for this earliest occupation is scant (Rissolo et al., 2005; Glover and Stanton, 2010).
During the transition from the Middle to Late Preclassic/Early Classic, the Yalahau region, like most areas of the Maya Lowlands, experienced a dramatic population increase evidenced by a proliferation of settlements, ceramic groups, and monumental and domestic architecture. Many sites, such as the Naranjal, were constructed in the megalithic style, a widespread northern lowlands architectural tradition (Mathews and Maldonado-Cardenas, 2006). Recent reevaluation of ceramic collections and the availability of radiocarbon dates place the peak of population in the Yalahau region at the Terminal Preclassic period from approximately 75 BC to AD 400, as defined by Glover and Stanton (2010).
In the subsequent Late Classic period, the Yalahau region did not continue on a trajectory of demographic, political, and economic expansion like most other areas did (e.g., Coba and the southern lowlands). Instead, there is very little evidence of occupation in the Yalahau region during this time, except at the north coast port site of Vista Alegre, thus the interior region appears to have been mostly abandoned. During the Postclassic period, Maya people returned to the Yalahau region, albeit in smaller numbers, reoccupying many of the earlier Terminal Preclassic sites.
Within the Yalahau region, settlements are situated in well-drained upland areas, generally between 5 and 15 m a.s.l. and outside of the wetlands subject to seasonal flooding, and are frequently associated with cenotes, important sources of water and loci of ritual activity, and caves (Bell, 1998; Fedick et al., 2012). Ethnographic research in the Yalahau and other regions has identified a variety of upland agricultural strategies that likely have roots in the distant past. Homegardens, common in the Yalahau region today, were undoubtedly a significant component of ancient Maya subsistence as well (Morell-Hart et al., 2022). Organic muck and algae/periphyton from Yalahau wetlands is transported for use as fertilizer in modern homegardens (Fedick and Hovey, 1995), a practice apparently extending back into ancient times (Morrison and Cozatl-Manzano, 2003). In outfield areas, the Maya of the Yalahau region most likely practiced a managed succession cultivation system that starts with selective clearing and coppicing of a forest patch and planting crops of the milpa, primarily maize, beans, and squash. Regrowth is then carefully managed to promote rapid restoration of a secondary forest garden that contains an increased representation of economically useful tree species (Ford and Nigh, 2016; Morell-Hart et al., 2022). This cycle is repeated after approximately 20 years, creating a managed mosaic of productive homegardens, milpas, forest gardens, and landesque improvements of various types (Fedick et al., 2023).
3.2.3 Soils and paleosols in northeastern Yucatán Peninsula
A major part of the results on surface soils in different geomorphic contexts were obtained at El Edén Ecological Reserve during collaborative pedoarchaeological research in the framework of the Yalahau Regional Human Ecology Project of the University of California, Riverside, led by S. Fedick and J. Mathews. Red soil and pedosediments in the karstic underground cavities were studied later as part of CONACYT and PAPIIT projects focused on soil mantle development and erosion in the karstic landscapes. The results were presented in a series of publications (Sedov et al., 2007; Fedick et al., 2008; Sedov et al., 2008; Cabadas-Báez et al., 2010; Cabadas-Báez et al., 2010; Flores-Delgadillo et al., 2011; Solleiro-Rebolledo et al., 2011; Leonard et al., 2019).
These studies confirmed that the soil cover of the upland areas in general is thin and patchy; “Rendzinas”—Rendzic Leptosols—are the dominant soils alternating with extensive areas of exposed bedrock (Figure 2G). This soil type is represented by the Yalahau 3 profile studied at El Edén Ecological Reserve in an upland location under forest (Figures 2H,I). It is very thin (14 cm), consisting of a dark Ah horizon with a well-developed stable granular structure, loose consistence, and high root density. Despite its thinness and proximity to calcareous material, the horizon is clayey and shows no reaction with HCl. The humus horizon is directly underlain by limestone bedrock (Sedov et al., 2008).
There are few upland areas with “Terra Rossa” thick red clayey soils—Chromic Luvisols—exemplified by the Kantunilkin profile (Figure 6). This soil has a set of well-developed Ah, Bt, and BCtg horizons with a total thickness of 135 cm. The Ah horizon has moderate pigmentation with humus, however it is less dark and aggregated, and much more compact, than the topsoil horizons of the Rendzic Leptosols. The Bt horizons are most enriched in clay and have a structure of hard subangular blocks with shiny surfaces. In the lower BCtg horizon, frequent Fe-Mn concretions were observed, and it is underlain by limestone along an abrupt and irregular contact (Cabadas-Báez et al., 2010).
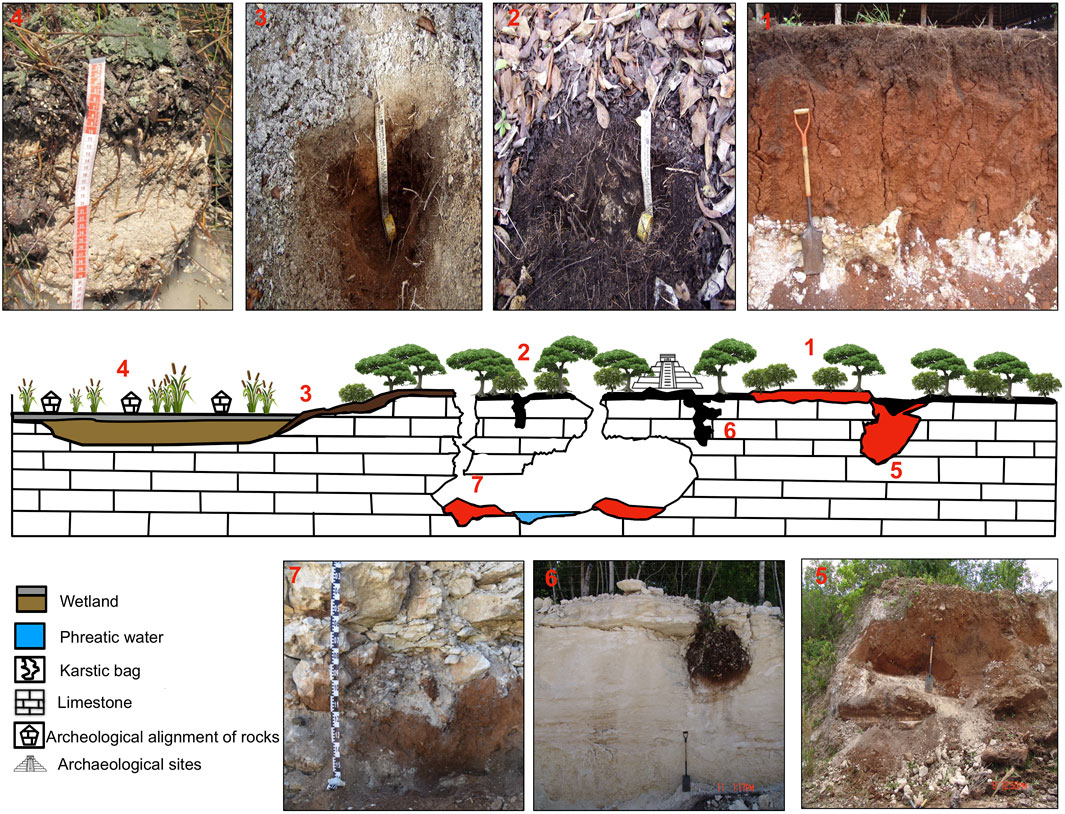
FIGURE 6. Northeast of the Yucatán Peninsula, Yalahau toposequence: general scheme and profile photographs. 1. Kantunilkin profile with Chromic Luvisol. Profiles in El Edén reserve; 2. Yalahau 3 profile (Rendzic Leptosol); 3. Yalahau 5 profile (polygenetic soil); 4. Yalahau 8 profile (Epileptic Calcisol); 5. Quarry 3 profile (red karstic pocket); 6. Quarry 4 profile (black karstic pocket); and 7. Coyotes section (pedosediment in the cave).
The swampy, seasonally flooded lowlands are covered with specific hydromorphic Calcisols represented by the Yalahau 8 profile in the lowest part of the wetlands of the El Edén Ecological Reserve. Despite its lowland position, this soil is rather shallow (35 cm thick) and consists of O, Ah, and Bk horizons underlain by limestone. The O horizon includes fragments of plant residues, roots and leaves, but the surface is covered by periphyton (an algal crust). Both the Ah and Bk horizons have pale color and loamy texture, react intensively with HCl, and consist predominantly of fine-grained carbonates. Their structures are weak and unstable, and they have muddy consistency due to being saturation with water.
A conspicuous polygenetic soil was encountered in the transitional geomorphic position between the upland and lowland areas (Figure 6). It was studied in the Yalahau 5 profile at the peripheral part of the El Edén wetland close to the boundary of the upland forest. This soil presents two pedogenetic phases, with the Bk horizon followed by 2Ah and 2Bw. The Bk horizon consists of pale, fine-grained, loose carbonate material similar to that of the lowland Calcisol. The underlying 2Ah horizon is dark gray-brown and has a granular structure resembling that of the upland Leptosols; however, unlike the Leptosols, it reacts locally with HCl (Sedov et al., 2008).
The results of the surface soils were complemented by the study of three underground pedosediments in the quarries along the Cancún–Tulum highway. Two of these pedosediments—the Quarry 3 and Quarry 4 sections—are inside karstic pockets of different sizes. The pocket of Quarry 3 is larger (with a depth of more than 2 m) and contains mainly reddish clayey redeposited soil material. The Quarry 4 pedosediment is inside a smaller pocket with a pear-like shape. In this case, the pedosediment is dark brown and humic, and has abundant rock fragments of differing sizes, charcoal, and mollusk shells (Cabadas-Báez et al., 2010).
The third pedosediment in the Coyotes section is found on the cave floor exposed in the wall of a quarry; it is overlain by large limestone fragments produced by the collapse of the cave roof. In this section, a sequence of layers with different colors and consistencies is exposed. The upper layer is a red pedosediment, 10 cm thick, consisting of a mixture of reddish fine material and carbonate sand. It has a gradual contact with the underlying loose dark brown pedosediment. The lowermost layer is also dark brown but more compact and contains frequent broken terrestrial mollusk shells, charcoal particles, and abundant charred rocks. The results from this section have not been previously published.
3.2.4 Micromorphological observations in soils of northeastern Yucatán toposequence
At the microscale, the Yalahau 3 profile shows a dark groundmass enriched in organic and ferruginous pigment with zoogenic granular structure and high porosity (Figure 7A); plant-tissue fragments of different decomposition grades are common. Despite the very close location of the calcareous C horizon, no carbonates (primary or neoformed) were found. The major parts of the fine mineral material were composed of clay with undifferentiated b-fabric.
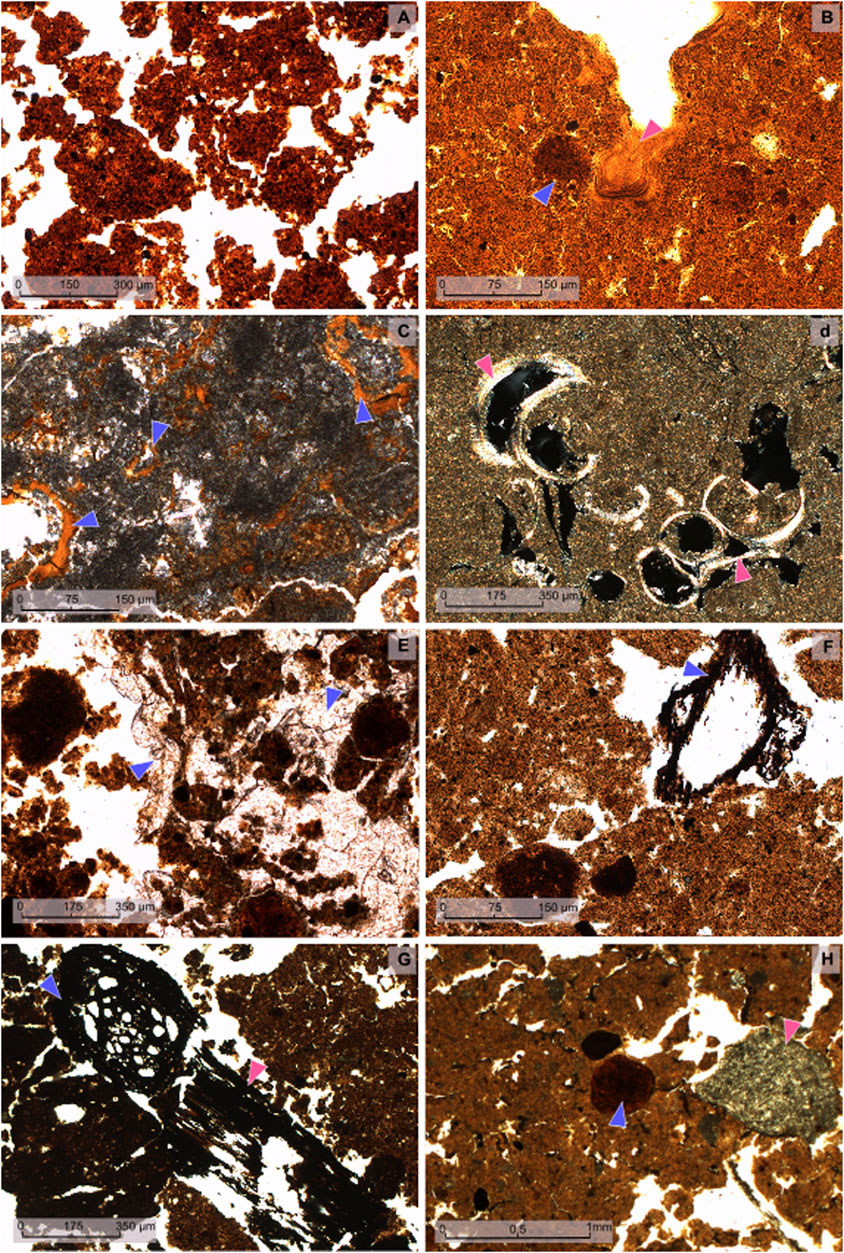
FIGURE 7. Photomicrographs of the Yalahau section; PPL, plane polarized light; XPL, cross polarized light. (A) Yalahau 3 profile Ah horizon (Rendzic Leptosol): groundmass composed of clay and iron oxides, pigmented by dark humus coprogenic fine granular structure (PPL); (B) Kantunilkin Bt2 horizon: clay compacted matrix with red iron nodules (blue arrowhead) and illuvial clay coatings (pink arrowhead) (XPL); (C) Kantunilkin BC horizon: illuvial clay coatings in limestone pores (blue arrowheads) (PPL); (D) Yalahau 8 profile (Epileptic Calcisol): groundmass dominated by neoformed micrite and freshwater mollusk shells (pink arrowheads) (PPL); (E) Yalahau 5 profile: soil material, typical for Rendzina, partly cemented with hydrogenic calcite crystals (blue arrowheads) (PPL); (F) Quarry 3 profile (Red Pocket): subangular blocky structure with a charcoal fragment (blue arrowhead) (PPL); (G) Quarry 4 profile (Black Pocket): charred aggregates (blue arrowhead) and charcoal fragments (pink arrowhead) (PPL); (H) Coyotes cave pedosediment: clayey-micritic reworked material, ferruginous nodule (blue arrowhead), and limestone fragment (pink arrowhead) (PPL).
The groundmass of the Kantunilkin soil is dominated by fine clay and pigmented by brown humus and red iron oxides for the Ah and Bt horizons, respectively. Very few discontinuous clay coatings of variable thicknesses are observed over ped surfaces (Figure 7B). Small brown anorthic ferruginous nodules are found, which are fragmented, showing broken angular edges. Another important feature of this soil appears in the contact with limestone, where red birefringent illuvial clay coatings develop on the surfaces of the calcitic blocks (Figure 7C).
The main feature identified by the micromorphological analysis of the Yalahau 8 profile is the dominance of micritic secondary carbonates in the groundmass. Sometimes, the micrite forms ooidal aggregates or microlaminated structures generated by algae. A few freshwater mollusk shells are incorporated into the micritic groundmass (Figure 7D).
The micromorphology of the buried horizons of the Yalahau 5 profile exhibits small areas cemented by large crystals of calcite that fill pores and surround the soil aggregates; these crystalline infillings resemble the “sparry cement” known to be of phreatic (groundwater) origin (Durand et al., 2010). The latter are similar to those observed in the Yalahau 3 profile (Figure 7E). The micromorphological pattern of the 2Bk horizon resembles that of Calcisol observed in Yalahau 8.
In the reddish Quarry 3 pedosediment, the red clayey groundmass and subangular blocky structure are like that of the Kantunilkin profile, although biopores with coprolite infillings were observed even at depth. Charcoal fragments are frequent in all fills (Figure 7F). Secondary micritic carbonates appear in some pores in the lowermost part of the pocket. Micromorphological observations of the black Quarry 4 pedosediment reveal the presence of a few volcanic minerals in the iron-clay groundmass, pigmented with humus. These sand-size minerals correspond to plagioclase, pyroxene, and amphibole crystals. Again, charcoal particles were found incorporated into the groundmass (Figure 7G).
In the thin sections from the cave floor sediment of the Coyotes section, we observed the mixture of micritic carbonates with rounded red clay aggregates (Figure 7H) and limestone fragments and shells, which were frequently charred.
3.2.5 Analytical characteristics of studied profiles
The Kantunilkin soil has a high amount of clay (60%) and shows an acidic reaction (Cabadas-Báez et al., 2010). The Yalahau soils at El Edén show contrasting properties. While the Yalahau 3 profile is clayey (70%), the Yalahau 8 profile in the wetland has a high proportion of sand (82%–97%) in the surface horizon and an elevated proportion of silt (72.7%) in the Bw horizon. The polycyclic Yalahau 5 profile shows contrasting grain size distribution: sandy in the top Bk horizon and silty-clayey in the 2Bw horizon. The Quarry 3 and Quarry 4 pedosediments, regardless of the type (red or black), have similar proportions of clay (54%–77%). The pedosediments inside the cave have less clay (48.2%–57.6%) and different percentages of silt (26.8%–29.6%) and sand (12.8%–24.9%).
The results of the XRD analysis of the clay material in Kantunilkin (Luvisol) and Yalahau 3 (Leptosol) have shown very similar clay mineral associations dominated by two major components: vermiculite and kaolinite in similar proportions.
3.3 Instrumental dating of paleosols and pedosediments
Several instrumental age estimations were obtained for some of the studied profiles using different techniques and dating materials: radiocarbon dates of humus, charcoal, pedogenic carbonates, and optically stimulated luminescence (OSL) was performed on silicate sedimentary material. The results are summarized in Table 1 together with the references to the paper where they were first published; we present and discuss calendar (calibrated) ages. They show that in the Usumacinta Valley, the age of secondary carbonates in the well-developed paleosol buried under colluvium in Boca del Cerro is approximately 13 ka BP—this supposes that its pedogenesis occurred in the Terminal Pleistocene, whereas colluviation most probably took place in the Holocene. In the Tierra Blanca profile, the silty alluvial sediment/reworked tephra below the upper set of paleosols was dated back to 9 ka BP. The overlying 3A horizon is dated from humus (corresponding to the minimal age of the soil) to approximately 2.7 ka BP, which is in good agreement with the encountered archaeological materials of the Preclassic period. The pedogenic carbonate concretion in the lower gleyic pedocomplex is dated back to 5.4 ka BP. This result shows apparent inversion with the OSL age of the silty sediment mentioned previously. We assume that the carbonates migrated and precipitated during the drier episode of the middle Holocene, producing concretions incorporated into much older paleosol.
In the northeastern Yucatán Peninsula, the radiocarbon date from charcoal encountered in the black pedosediment Quarry 4 is approximately 1 ka BP, only a bit younger than the Terminal Classic collapse. The charcoal in the Coyote cave bottom sediment is much older, more than 4 ka BP, and corresponds to the beginning of land cultivation in the Yucatán Peninsula.
4 Discussion
4.1 Soil diversity in tropical karst landscapes as product of interplay of pedogenetic and geomorphic processes
The studied toposequences show striking similarities and contrasting differences between the main soil types, which develop in various conjunctive geomorphic positions. These differences are controlled by the interplay of pedogenesis and erosion/deposition processes, the latter being responsible for the soil loss in certain areas, accompanied by pedosediment accumulation in the other. Finally, this interplay controls the spatial distribution of soil characteristics vital for ancient Maya subsistence: physical and chemical soil quality, fertility, mechanical stability, etc., which largely define the mode and differentiation of land use. In turn, ancient land use practices modified this interplay, hampering certain processes and accelerating others, which profoundly modified soil mantle and had feedback effects on the ancient economy and social dynamics (Beach et al., 2006; Carozza et al., 2007; Turner and Sabloff, 2012; Beach et al., 2015; Dunning et al., 2020; Doyle et al., 2023).
As expected, soil formation proceeds differently in the two key domains of the studied landscapes: 1) elevated upland areas, which provide a well-drained soil environment and are commonly affected by erosive processes and 2) lowlands, major karstic depressions, valley bottoms, and terraces, which predominantly receive (pedo)sediments and are frequently affected by excessive moisture that gives rise to hydromorphic pedogenesis. We consider soil development in these two domains of the studied toposequences in the following sections.
4.1.1 Upland domain: origin of Rendzina/Terra Rossa combination
The calcareous upland areas show similarity in the main soil types formed on them in all three studied toposequences. This is a combination is well known in various tropical and subtropical calcareous landscapes throughout the world: shallow dark humus-rich soils are found neighboring more profound red clayey profiles (Shapiro, 2006; Sandler et al., 2015; Vrščaj et al., 2017; D'Amico et al., 2023; Durn et al., 2023). The former is known by the traditional term Rendzina (in the WRB classification, Rendzic Leptosols, and sometimes, Calcaric Phaeozems), while the latter is known by the term Terra Rossa (most of them are Chromic Cambisols and Luvisols). In all studied cases, Rendzinas are dominant, whereas the Terra Rossa occupies minor areas and is patchy. The patches of red soils are mostly related to the flat areas and closed karstic depressions within the uplands (as in Chinikihá); however, their position in the relief is often practically the same as that of the neighboring Rendzinas (as in Kantunilkin).
Since the beginning of soil research, the enigmatic red clayey carbonate-free soils over limestone attracted the attention of scholars. Two main scenarios were developed for the origin of the ferruginous and silicate material of these soils. The first attributed it to the lime-free residue of the underlying calcareous rocks accumulated on the surface after carbonate dissolution (de Lapparent, 1930; Thornbury, 1954). The second attaches major importance to the allochthonous sources, i.e., eolian material (Yaalon, 1997). To solve this problem for the case of Terra Rossa of southern Mexico, we performed a detailed mineralogical and geochemical analysis of the Luvisol profile in Kantunilkin. The results pointed to multiple possible sources. Among them were the contribution of the insoluble residue of limestone, far-distance windblown silt (probably transported by the trade winds from Sahara), and especially important and well-documented input of pyroclastic material that could originate from the volcanoes of southern Mexico, Guatemala, or Caribbean islands (Cabadas-Báez et al., 2010). We assume that the volcanic material was also involved in the development of red soils on the limestones in Chiapas; however, further research is required for confirmation.
Whatever the original parent material for south Mexican Terra Rossa was, it should be transformed to produce a deeply weathered clayey matrix enriched in ferruginous pigment, as observed in the Luvisol profiles of Kantunilkin in Yucatán and Chinikijá in Chiapas, and Cambisol of the Arriba Cueva profile in Busiljá. To explain the formation of this soil material, Merino and Banerjee (2008) developed a metasomatic hypothesis that implies primary silicate dissolution in the upper horizons; downward migration of Si, Al, and other elements in their dissolved forms to the leaching front; and synthesis of secondary clay minerals directly on the surface of the corroded calcareous rock simultaneous with its dissolution (Merino and Banerjee, 2008). We offered a somewhat different scenario in which clay synthesis occurs in the upper and middle horizons of Terra Rossa simultaneously with primary mineral weathering (Cabadas-Báez et al., 2010). Furthermore, downward migration of substances occurs not in solutions but in suspensions, resulting in the deposition of typical illuvial clay coatings at the carbonate leaching front on the limestone surfaces, as observed in the thin sections of BCk horizon in Kantunilkin. With the progress of limestone dissolution, these coatings lose the carbonate surface that supported them and become incorporated into the clayey groundmass (as described by Bronger et al., 1998). Because clay illuviation and especially silicate weathering are slow pedogenetic processes with a characteristic time nx104–105 yr (Targulian and Krasilnikov, 2007), we conclude that development of Terra Rossa should cover time intervals that are much longer than the Holocene extending into Late Pleistocene.
Rendzinas (Rendzic Leptosols), despite their shallowness and apparent primitive macromorphological organization, possess a contradictory and enigmatic set of properties, which require re-interpretation. They are usually considered to be poorly developed soils that are predominantly made up of fragments of calcareous rocks and organic materials in different stages of transformation.
However, when we pass from macro- to microscale observations, we encounter features that are in strong disagreement with this statement. As described previously, many Rendzinas of the Yucatán Peninsula have groundmass that is free of carbonates and strongly enriched with silicate clay of vermiculite–kaolinite composition and ferruginous material (Figure 7A), pigmented with dark humus. When primary calcite from calcareous rocks is present, as in Busiljá and Chinikihá Leptosols, it is mixed up with clay and ferruginous components (Figure 3A; Figure 4A). The latter point to the rather advanced weathering status of the Rendzina groundmass was further confirmed by the data on clay mineral assemblages showing predominance of vermiculite and kaolinite (Sedov et al., 2008). We further speculate that such weathering status could not be achieved in the Rendzina soil environment: proximity of the underlying calcareous rocks should have hampered silicate alteration due to quick neutralization of soil acidity. Thus, clay and iron oxides should have been inherited from a pre-existing soil body with different properties. Comparing Rendzinas with the neighboring Terra Rossa, we detect a striking similarity in their fine material, only masked by the strong humus pigmentation of the former. The composition of clay mineral assemblages is also similar. This led us to the hypothesis that many Rendzinas are not formed during pedogenesis directly on the limestone surfaces but are derived from the residues of Terra Rossa, left above the limestone after a major part of the red soil material had been eroded. The frequent presence of the micro-fragments of red clayey soils incorporated in the Rendzina groundmass (as observed in Busiljá) further supports this scenario. If our hypothesis of the erosional origin of the Rendzina material is right, then the question arises: where has the eroded Terra Rossa material gone? Somewhere in the landscape, we should find abundant pedosediments. In search of them, we should consider the lowland domain of the studied toposequences and surface and underground karstic depressions, described in the following sections.
4.1.2 Soil diversity in lowlands and variety of hydromorphic pedogenetic processes
Contrary to the upland areas, the lowland sectors of the studied toposequences surprised us with the striking diversity of their soil profiles. This diversity is clearly controlled by a variety of hydromorphic pedogenetic processes that occur in these areas. In the large karstic depressions between calcareous hills in the Busiljá area and in the upper alluvial terraces of the Usumacinta (Balancan profile), we observe the dominance of redoximorphic processes and formation of gleysols. In Busiljá, a conspicuous feature of some wetland soils is the presence of neoformed gypsum (Figure 5F), which was completely unexpected in the highly humid tropical environment. We first assumed that gypsum could be a relict feature, a legacy of earlier drier climate, or even originate from ancient human-induced materials. However, the fresh unaltered morphology of gypsum crystals lacking any signs of dissolution (expected in case of their relict nature) points to their recent origin. Earlier gypsum neoformation was documented in the wetlands saturated with sulfate-rich waters in southern Maya Lowlands (Pohl et al., 1996; Beach et al., 2006; Luzzadder-Beach et al., 2012; Krause et al., 2019); these authors assumed its evaporitic origin. We developed a different scenario of gypsum synthesis related to redoximorphic processes (Guillén, 2020).
At the lower terraces of the Usumacinta and its tributaries, continuous alluvial sedimentation throughout the Holocene and better drainage permitted the development of fluvisols without strong redoximorphic features in the upper part of the soil-sedimentary sequences (Figure 2). The main process is humus accumulation, which gives rise to a set of surface and buried dark Ah horizons. These horizons are better developed on the terrace of the main river (Usumacinta–Tierra Blanca profile) than in the Chocoljá minor tributary. We attribute it to the differences of the parent material. In the case of Chocoljá, it consists mostly of primary carbonates derived from local limestones. In the Usumacinta terrace, it is made up of silicates from far-distance transport, such as pyroclastic material (Figure 3G) redeposited from the tephras of volcanoes in the vicinities of the upper reaches of the Usumacinta (Cabadas-Báez et al., 2017).
In the lowland wetlands, in the platform of the northeastern Yucatán Peninsula (Figures 1, 6), pedogenesis takes a completely different direction. There, the soil groundmass is dominated by fine micritic carbonate material (Figure 7D). However, it does not contain primary carbonates derived from the underlying limestone. The micritic groundmass consists of secondary calcite deposited due to metabolism of algae which form a continuous matt (periphyton) during the floods. This interpretation justifies the taxonomic denomination of these soils as hydromorphic Calcisols (Solleiro-Rebolledo et al., 2011). In the central parts of the Yalahau wetlands, this biogenic carbonate accumulation acquires considerable thickness due to constant aggradation (Leonard et al., 2019).
At the wetland periphery, peculiar profiles combining Rendzina (below) and Calcisol (on top) horizons were observed (Figure 6). These profiles are clearly polygenetic and reflect the shift from the earlier stage of forest pedogenesis typical for uplands to hydromorphic wetland soil development (Sedov et al., 2008). The Rendzina horizon shows signs of recent re-carbonatization which confirms its relict nature. We interpret this profile as a record of the environmental change, which included a considerable extension of the flooded area.
4.1.3 In search of eroded upland soil material: distribution and post-depositional transformation of pedosediments
In the mountainous karstic landscapes of the Sierra de Chiapas and Usumacinta Basin (Figure 1), with contrasting relief and extensive steep slopes of limestone hills, the lateral redistribution of soil and regolith material toward piedmonts and depressions is the main erosion process. In some cases, as in the Boca del Cerro profile (Figure 2), both the piedmont location and heterogeneous composition (stones mixed with redeposited soil) of exposed strata point to their colluvial origin. However, in the case of the swampy karstic depressions of Busiljá (Figure 4), the origin of the clayey groundmass of the gleysols is not so obvious; its morphological characteristics on the macro- and microscale are quite different from those of the Leptosols and Chromic Cambisols of the neighboring calcareous hills. In this case, the clear similarity of the clay mineral assemblages of the upland and lowland soils suggests that the former contributed to the latter’s material due to colluviation. We propose that the upland red clayey soils were eroded to a large degree (as stated previously) and their derivates were deposited at the valley bottom, contributing to the parent material of Gleysols there. Posterior redoximorphic processes obliterated the original morphology of the pedosediments: red ferruginous pigment was dissolved, iron oxides concentrated in the nodules, and b-fabric changed due to reorientation. However, the clay particles composition suffered only minor changes (vermiculite was partly transformed to smectite) and could serve as a witness to the genetic relationship between upland and lowland soil substrates.
Much more complex is the detection of soil erosion mechanisms in the platform karstic landscapes of the northeast Yucatán Peninsula (Figure 6). At first glance, the geomorphological conditions of this area should not support the lateral redeposition of surface materials: the relief is quite flat and the slopes are very gentle. Indeed, in the wetland soils, we could not detect any significant quantities of pedosediments derived from the upland Terra Rossa and/or Rendzina soils: fine micritic groundmass of the hydromorphic Calcisols does not include any redeposited silicate and ferruginous materials. This confirms that the “normal” lateral soil erosion and redeposition along the slope gradient are strongly hampered in the northeastern Yucatán Peninsula.
However, this does not mean that soil erosion does not occur at all in these landscapes. We encountered large volumes of pedosediments in the subsurface karstic cavities: in pockets and bags and on the cave floor. In the karstic pockets, the pedosediments are easily recognizable soil materials derived from Rendzinas (black pedosediments) and Terra Rossa (red pedosediments mostly in the larger pockets). Only minor transformation of these materials took place in the form of precipitation of secondary carbonates due to groundwater migrating through the karstic pockets. At the cave bottom, the soil-derived material is diluted by the primary and secondary speleogenetic carbonates; however, still recognizable at microscale are clusters of red clayey soil material (Figure 7F). Incorporation of charcoal particles and terrestrial mollusk shells confirm the pedosedimentary nature of these pocket and cave fills.
These observations have led us to conclude that a specific “hidden” karstic erosion took place in the platform karstic landscapes of the northeastern Yucatán Peninsula. Instead of lateral downslope transport, the soil is removed from the surface vertically through the interconnected karstic cavities. Relocated soil material fills karstic pockets, arriving finally at the bottom of caves where it is mixed with speleogenic carbonates. This process is known as “soil piping” and is well documented in various karstic geosystems on the global scale (Waltham, 2008; Zhang et al., 2011; Sauro, 2019; Zhao and Shen, 2022). It should be stressed that red clayey pedosediments are frequently found within the northeastern Yucatán Peninsula, in areas where no red soils are currently present on the surface. This supports our conclusion that the composition of the soil mantle could be deeply transformed by erosion.
4.2 Interaction between soil mantle and ancient societies
4.2.1 Ancient land use in Usumacinta Basin
All human activities in the karstic landscapes were clearly adjusted to the type of geomorphic position and properties of soils. In the hilly regions surrounding the middle Usumacinta Basin, there is clearly a tendency for the development of important settlements on the calcareous hills and ridges. Chinikihá, Busiljá, and Palenque follow this tendency. The “attractors” for these ancient settlements were better defensive positions, visual control over the surrounding territory, and abundance of stone for construction (Liendo-Stuardo et al., 2014; French et al., 2020). However, we assume that these geoforms had minor importance for agricultural production. The agronomic quality of Rendzinas which dominate the calcareous hills is strongly reduced by their thinness and discontinuous distribution, while high humus content, stable granular structure, and high porosity are beneficial properties. We speculate that these soils were used by ancient inhabitants for planting home gardens and cultivating orchards or forest gardens dominated by useful trees (that could also protect the soil from further erosion). These gardens surrounded the settlement areas, and are thought to be an important part of Maya agricultural landscapes (Ford and Nigh, 2016; Morell-Hart et al., 2022; Fedick et al., 2023).
Flat lowland areas, broad karstic depressions, and river terraces with deep soils are assumed to constitute the main agricultural domain in the middle Usumacinta Basin (Dunning et al., 1998; Solleiro-Rebolledo et al., 2015; Schroder et al., 2021). Humic fluvisols on the well-drained young alluvial terraces are suitable for cultivation without any limitations, except possible floods. However, development of thick Ah horizons without alluvial lamination point to long periods of surface stability with minimal floods, which permitted continuous pedogenesis. Interestingly, these terraces were also used for minor rural settlements inhabited by farmers. Despite the more modest size and type of constructions, these settlements appeared to be more sustainable than the major urban centers in the uplands (Macrae and Iannone, 2016; Turner, 2019; Schroder et al., 2021). They persisted throughout the Classic period and then survived during the Terminal Classic collapse, when the cities in the sierras were abandoned (Liendo-Stuardo et al., 2014). We attribute this sustainability to the proximity and closer link to the most valuable soil resources, which become vital in periods of environmental or social stress.
Thick clayey hydromorphic soils of swampy flat karstic depressions at Busiljá also have quite good agricultural potential. The presence of a moderate amount of neoformed gypsum—a neutral salt with relatively low solubility—does not significantly influence their agronomic quality. Major limitations presented by these soils consist of excess moisture due to their saturation with the high-standing groundwater and reduced conditions, even in the upper soil horizons. However, these soils could be successfully cultivated after drainage, being especially suitable for milpa (maize, beans, and squash) (Morell-Hart et al., 2022; Fedick et al., 2023). Artificial drainage, through the construction of channels and raised fields, were common techniques of wetland management by the ancient Maya, well documented in various parts of the Maya region (Kunen, 2001; Dunham et al., 2009; Beach et al., 2019; Krause et al., 2019; Miksicek, 2019; Dunning et al., 2020).
In addition to agricultural significance, the soils of the Usumacinta riverine domain could also serve as an extensive and easily accessible source of raw material for ceramic production. The upper alluvial plain, with deeply weathered gleyic and stagnic clayey soils, could provide clay, whereas lower terraces with coarse deposits could contribute to sand temper. It was shown that enigmatic volcanic glass shards frequently found as temper in Classic Maya ceramic could have originated from the silty alluvium, comprised of redeposited tephra exposed in the Tierra Blanca section (Coffey et al., 2014; Cabadas-Báez et al., 2017).
4.2.2 Ancient land use in northeastern Yucatán Peninsula–Yalahau region
The spatial differentiation of soil agronomic quality in the platform karst landscapes of the northeastern Yucatán Peninsula differs drastically from that in the mountainous karst landscapes of the Sierra de Chiapas/Usumacinta Basin. As discussed previously, in Chiapas, soils of the lowland domain are suitable for cultivation although often require artificial drainage. In the northeastern Yucatán Peninsula, the dominant wetland soils, hydromorphic Calcisols, are poorly suited for agriculture. These Calcisols consist predominantly of carbonate mud, a structureless micritic material, dispersed in its usual water-saturated state, but with a tendency of strong compaction on drying (Solleiro-Rebolledo et al., 2011). The humus content is low and organic matter is mostly confined to plant debris, which is easily degradable and not contributing to aggregate formation. Thus, we suggest that the main agricultural domain in the Yalahau region was the calcareous uplands and associated Rendzina-type soils.
The Rendzinas, Rendzic Leptosols, and Leptic Phaeozems, in many aspects, show high biological and agronomic quality. They are neutral and rich in dark colloidal humus, with perfectly stable granular structure and high porosity, providing both good aeration and sufficient water-holding capacity. It is important that these beneficial properties are stable and do not degrade even after long-term cultivation in traditional Maya homegardens (solares) as shown by Flores-Delgadillo et al. (2011). The main limitation of these soils is found in their thickness, which is generally thin, though highly variable; limestone outcrops alternate with hollows with more profound Ah horizons. This variability is in fact prohibitive for modern agricultural technology with the extensive use of machinery. However, traditional manual cultivation could provide highly productive agrosystems when every small plot with specific soil depth is used for planting a suitable, cultivable species (Ardren and Miller, 2020; Dedrick et al., 2020). This practice of matching crop preferences to the localized variations in soil depth and properties at an extremely fine scale is defined as “ancient precision agriculture” (Flores-Delgadillo et al., 2011). A specific variant of this technological approach is developed within home gardens, where small, natural, soil-filled cavities in the bedrock are used in a manner analogous to “container gardening” (Fedick et al., 2008).
Terra Rossa, red clayey soils, also present in the upland areas, are in fact less fertile than Rendzinas despite their greater thickness. Their A horizons have lower humus content, have coarser structure, and show a strong tendency of compaction. However, the mineral B-horizons of these soils could be mined as a raw material for ceramic production, representing a practically unique source of carbonate-free clay material in these landscapes. Some petrographic observations (e.g., clay illuvial and ferruginous pedofeatures incorporated into ceramic matrix) confirm this hypothesis (Cabadas-Báez et al., 2017).
Despite strong soil limitations for agricultural use today, the wetlands were clearly involved in the ancient Maya economy. Surveys of the Yalahau wetlands have documented hundreds of rock alignments that are of definite human construction within dozens of wetlands (Fedick et al., 2000). The use of the Yalahau wetlands may have changed dramatically over time in response to changing water levels, as well as to resulting changes in soil formation within the wetlands, especially at their periphery. Early investigations have suggested that the water levels in the Yalahau wetlands have risen approximately 1 m since the Preclassic period (Fedick et al., 2000; Wollwage et al., 2012; see also Beddows et al., 2016; Glover et al., 2022; McKillop, 2023). This conclusion has been strongly supported by pedological research: the polygenetic profiles near the wetland margins have shown a clear shift from the Rendzina soil development typical for upland forest ecosystems to the wetland Calcisol formation—as discussed previously. These lower unit soils would have been of greater agricultural potential when the marginal parts of wetlands were only subjected to short-term flooding (cf. Dunning et al., 2019). Thus, the upland agricultural domain in the Yalahau region was much larger in the past. The recorded rock alignments may have served to slow downslope water flow, protect crops, and retain soils (Fedick et al., 2000). The gradual rise in the water table (McKillop, 2023) related to a high stand of sea level at approximately AD 400 (Beddows et al., 2016; Glover et al., 2022) would have subjected increasing areas of the depressions to flooding and the burying of organic Rendzina soils with Calcisols, rendering the areas unfit for cultivation. We further hypothesize that specific population dynamics in the Yalahau region—maximum occupation in Preclassic and unusual abandonment during the Classic period—are related to these soil and environmental changes.
The rock alignments may also represent the management of adapted aquatic resources, such as cattail (Typha domingensis and T. latifolia), duck potato (Sagittaria lancifolia), and apple snails (Pomacea flagellata), all of which grow in abundance today in the Yalahau wetlands. Some alignments, constructed in zig-zag patterns, are like features used elsewhere as fish weirs (Erickson, 2000; Kelly, 2014; Blatrix et al., 2018; Palka, 2023). Periphyton, the algal crust that contributes to the formation of Calcisols, was probably collected and used as fertilizer in ancient times, as it still is today (as discussed previously). We also suggest that the fine carbonate matrix of Calcisols could have been used as construction material, serving as a substitute for burnt lime; of course, although its quality as mortar or plaster might be lower, it may be much “cheaper” in terms of labor, time, and resource investment.
4.2.3 Ancient Maya agriculture and soil erosion: forcing and feedback
As discussed previously, the influence of advanced erosion in the karst landscapes of southern Mexico is clearly imprinted in the properties of the shallow upland Rendzinas as well as in the pedosediments accumulated in the depressions or underground karstic cavities. The question arises: whether this erosion was a natural process, or induced or accelerated by ancient Maya land use?
We believe that large-scale cultivation introduced by Maya people since several millennia ago was responsible for the dramatic acceleration of soil erosion, both lateral in mountainous landscapes of Chiapas and vertical “soil piping” in the Yucatán platform. This link has already been confirmed by the data from the lacustrine records in the Petén region where a distinctive layer of Maya clay, which is redeposited soil material, was encountered in the lake cores within the interval corresponding to Maya occupation (Rosenmeier et al., 2002; Fleury et al., 2014; Birkett et al., 2023). Indicators of accelerated human-induced erosion were also found in the coastal lowlands of Belize (Beach et al., 2006; Beach et al., 2018). Our results also demonstrate some direct and indirect evidence for this interpretation. Frequent charcoal particles observed in the pedosediments, especially in the pockets and caves of the northeastern Yucatán Peninsula, point to the burning of vegetation, associated with the erosion/redeposition processes. We suggest that these pyrogenic materials originate from slash-and-burn agriculture, widely practiced in the Maya region (Schüpbacha et al., 2015; Anderson and Wahl, 2016; Douglas et al., 2022). Instrumental dating from karstic pedosediments is still scarce; however, that in the karstic pocket is close to the end of the Classic period. Similar dating within the Classic period was obtained from another karstic pocket in a traditional Maya home garden (Flores-Delgadillo et al., 2011). Interestingly, the charcoal in the cave pedosediment is much older, corresponding to the transition between Archaic and Preclassic periods. This agrees with the recent results from palynological records pointing to the very early beginning of large-scale land cultivation in the Maya region (Brenner et al., 2002; Brenner et al., 2003).
We conclude that continuous soil loss from the upland areas due to anthropogenic erosion occurred since the beginning of the Early Preclassic period and continued through the Classic period, recognizing that archaeological evidence does indicate that erosion-management practices, such as terracing, were in place at least by the Late Classic period in many areas of the uplands (Dunning et al., 2009; Fedick et al., 2023). What changes within the upland soil mantle did this cause? It could be assumed that at the onset of large-scale agriculture and population growth, deep red soils of Terra Rossa type were much more common in the uplands of the karstic landscapes of southern Mexico. However, by the beginning of the Classic period, shallow Rendzinas, which developed from the residues of eroded Terra Rossa soil, were already widely spread. At the archaeological sites of this period, we mostly find only a few small remnants of red soil in some karstic hollows, such as the Maria profile at Busiljá.
This soil mantle change should have a feedback effect in the development of ancient agriculture. We propose that in the mountainous areas of Chiapas, soil loss on the hills and a growing population forced ancient farmers to expand cultivation of wetland soils, shifting a significant proportion of agricultural production to the lowlands, necessitating laborious technologies (artificial channels, raised fields, etc.) to bring these lands into productive cultivation. In the platform landscapes of the northeastern Yucatán Peninsula, the main agricultural domain persisted in the flat uplands and required development of special technologies for Rendzina cultivation: “precision agriculture,” “container gardening,” and the use of periphyton fertilizer as described previously. These technologies could still provide high productivity of agrosystems under stable humid conditions.
Continuous soil loss and extension of shallow soils could have major importance for the response of the agrosystems to climatic fluctuation. In the case of droughts, this response will strongly depend upon the capacity of the soil to store moisture and provide it to crops during periods of water deficit. As discussed previously, the upland Rendzina soils have quite adequate structure and porosity to store moisture; however, their thinness strongly reduces their integral water-holding capacity. During drought (particularly the severe droughts of the Terminal Classic) (Haug et al., 2003; Aimers and Hodell, 2011; Evans et al., 2018; Hodell et al., 2001) these soils, otherwise fertile, could dry rather quickly, causing strong decrease in yields, particularly among vulnerable annual crops. In response, land use patterns could have shifted in some areas to deeper, moisture-retaining soils of the valleys and depressions (cf. Luzzadder-Beach et al., 2012), and crop selection could have shifted to more drought-resistant food plants available to the ancient Maya (Fedick and Santiago, 2022). In this way, human transformation of the soil mantle, coupled with the impact of climatic change, resulted in transformative adaptation of subsistence systems while provoking further economic and social changes.
4.3 Final remarks: types and localization of paleosol records in tropical karst landscapes of southern Mexico
Overall, until now, paleopedological research has made a minor contribution to the reconstruction of environmental changes, both natural and human induced, related to the cultural development in the Maya region. The bulk of the results used for this reconstruction is provided by the study of lake sediment cores (Hodell et al., 2005; Douglas et al., 2016; Krywy-Janzen et al., 2019), speleothems (Medina-Elizalde et al., 2010), and even marine sediments quite distant from the study region (Haug et al., 2003). Indeed, in comparison with these data sets, paleopedological investigations are few and localized. An example of successful investigation of this kind is the work by T. Beach and his co-workers who encountered and documented well-developed buried paleosols in the sedimentary sequences of the coastal plain in Belize (Beach et al., 2015; Beach et al., 2019; Krause et al., 2019). However, identification of buried paleosols or relict soil properties associated with ancient Maya contexts is scarce. The key for future advances in paleopedological research depends on identifying regularities in the geomorphological position of “prime” agricultural settings, and understanding how changes, both human-induced and climatic, have altered soilscapes through time.
In general, the spatial distribution of “soil memory,” understood as the set of pedogenetic properties and features bearing information about past environmental factors and conditions (Targulian and Goryachkin, 2004), is heterogeneous. In the tropical karst landscapes of southern Mexico, this heterogeneity is extremely high due to contrasting diversity of the soil mantle.
We could conclude that the dominant upland soils, Rendzina and Terra Rossa, both in mountainous and platform karst geosystems, show little “soil memory.” In Rendzinas, shallowness and primitive profile development leave little space for relict features, and we have to apply careful microscopic and mineralogical investigations to understand their erosive origin. In Terra Rossa, advanced weathering and accumulation of secondary minerals has obliterated the features of previous stages of pedogenesis. These soils are most common at Maya archaeological sites; by being difficult to interpret from the paleoecological standpoint, they have received little attention as a potential object for geoarchaeological investigation.
Within the studied toposequences, the lowland domain definitely has a major potential to provide paleopedological records. In the Sierra de Chiapas/Middle Usumacinta Basin region (Figure 1), lower Holocene alluvial terraces display detailed paleosol–sedimentary sequences with multiple buried soil horizons. These sequences have good prospects for developing chronological scales, with radiocarbon dating of humus and pedogenic carbonates, OSL dating of sedimentary strata, and archaeological dating of incorporated artifacts being the main contributors. Frequent soil burial at these settings also has its “negative” side; the buried profiles are relatively primitive with a rather poor set of pedogenetic properties. In such cases, rapidly formed biotic components and features like phytolith assemblages or stable carbon isotope composition of humus could be the most promising paleoecological proxies (Solís-Castillo et al., 2015). Colluvial sequences in the piedmont areas also sometimes host well-developed paleosols (as in the Boca del Cerro section, Figure 2, profile 3); in general, these records are less detailed when compared to alluvial sequences. Poorly drained karstic depressions at Busiljá have also received colluvial deposits and could potentially generate paleosol records; however it seems that very intensive recent redoximorphic processes have obliterated major parts of ancient pedogenetic features.
In the platform landscapes of the northeastern Yucatán Peninsula, paleopedologists face a much more challenging situation. Relict features in the upland soils are poorly preserved, as discussed previously. Recently encountered and investigated pedogenic carbonate horizons (calcretes) contain valuable paleoecological information (Valera-Fernández et al., 2020; Valera-Fernandez et al., 2022); however, they were developed mostly during the Pleistocene and their chronological resolution is low, so they do not “remember” relatively recent environmental events of Maya occupation. Even lowland areas have quite limited “soil memory” potential. Their soils are shaped predominantly by the process of biogenic carbonate accumulation, which generates rather uniform and primitive hydromorphic Calcisols. However, coring prospection in the central parts of Yalahau wetlands has revealed buried peat and humus horizons in the most profound Calcisol profiles, which could be considered as potential paleoecological archives (Leonard et al., 2019).
The wetland periphery areas of the Yalahau region have proven to be quite promising settings for paleopedological research. Here, polygenetic Cambisol/Calcisol profiles develop in response to the changes of intensity and extension of floods, which in turn could depend upon climatic and sea level factors. These paleopedological data can be integrated with the archaeological findings in the nearby ancient settlements.
We think that an important source of paleopedological information is pedosediments accumulated in the underground karstic cavities. Our observation in the limestone quarries in the northeastern Yucatán Peninsula have shown that these pedosediment deposits have diverse properties, derived from various sources, and could cover a large chronological interval extending beyond the limits of the Quaternary. These fills are affected by post-depositional diagenetic changes, especially carbonatization; the older ones are even lithified (Valera-Fernandez et al., 2022). However, various elements of “soil memory” such as mineralogical and geochemical composition, and micromorphological features could be successfully investigated; also, materials suitable for radiocarbon dating are frequently encountered. Karstic pedosediments together with speleological characteristics and underground archaeological and paleontological materials form part of the “Maya underworld” that is now one of the hotspots of interdisciplinary research in the Yucatán Peninsula.
Author contributions
Substantial contribution to the conception of the work, acquisition and interpretation of the data, and the approval of the current version were done by all authors, who also participated in the field research and discussions. SS designed the scheme of the paper and wrote major parts of the Introduction and Discussion sections; MYR-U, GI-A, PG-R, DV-F, KG-D, and SM-R wrote various blocks of the Results section, which were compiled and ordered by ES-R, while HC-B and JD-O designed the schemes of toposequences; DL, SF, CG, SM-H, and RL-S provided information about archaeological contexts and ancient land use. All authors contributed to the article and approved the submitted version.
Funding
This research was partly covered by the Consejo Nacional de Humanidades, Ciencia y Tecnología (CONAHCYT) through the project (CF682138) La infraestructura urbana como indicador de la génesis y desarrollo de la ciudad Maya Clásica: el caso de Palenque, Chiapas. UNAM PAPIIT, Project IN108622 “Rendzic Leptosols of the karstic geosystems in southern Mexico: genesis and evolution in relation to the natural and anthropic landscape change”. Soil-archaeological work at Budsiljá was partially supported by the Alphawood Foundation of Chicago, the Social Sciences and Humanities Research Council of Canada, and the National Science Foundation of the United States of America (SBE-BCS 1917671).
Acknowledgments
MYR-U acknowledges CONAHCYT for the post- doctoral fellowship. Important contributions to the study of the karstic toposequences in southern Mexico were made by the earlier participants, collaborators, and students of the UNAM Paleopedology group: among them, Jorge Gama, Ernestina Vallejo, Lourdes Flores, and Berenice Solis. The authors are grateful to their German colleagues who, on various occasions, took part in field trips to southern Mexico and contributed to fruitful discussions of their joint observations: Dr Birgit Terhorst (Universität Würzburg), Dr Bodo Damm (Universität Vechta), and Dr Bernhard Lucke (Universität Erlangen–Nürnberg), and the students of their research groups. The authors deeply appreciate the friendly collaboration of Sergio Palacios who initiated their soil- archaeological research in Yucatán.
Conflict of interest
Author DL is employed by HDR, Inc. The company HDR, Inc. has no involvement or connection to this research.
The remaining authors declare that the research was conducted in the absence of any commercial or financial relationships that could be construed as a potential conflict of interest.
Publisher’s note
All claims expressed in this article are solely those of the authors and do not necessarily represent those of their affiliated organizations, or those of the publisher, the editors, and the reviewers. Any product that may be evaluated in this article, or claim that may be made by its manufacturer, is not guaranteed or endorsed by the publisher.
Supplementary material
The Supplementary Material for this article can be found online at: https://www.frontiersin.org/articles/10.3389/feart.2023.1239301/full#supplementary-material
References
Aguilar, Y., Bautista, F., Mendoza, M. E., Frausto, O., and Ihl, T. (2016). Density of karst depressions in Yucatán state, Mexico. Cave Karst Stud. 78 (2), 51–60. doi:10.4311/2015ES0124
Aimers, J., and Hodell, D. (2011). Drought and the Maya. Nature 479 (7371), 44–45. doi:10.1038/479044a
Amador, F. E. B. (2005). Ancient pottery in the Yalahau region: A study of ceramics and chronology in northern Quintana Roo, Mexico. New York, Buffalo: Unpublished Ph.D. dissertation, Department of Anthropology, University of.
Anderson, L., and Wahl, D. (2016). Two Holocene paleofire records from peten, Guatemala: implications for natural fire regime and prehispanic Maya land use. Glob. Planet. Chang. 138, 82–92. doi:10.1016/j.gloplacha.2015.09.012
Andreani, L., and Gloaguen, R. (2016). Geomorphic analysis of transient landscapes in the sierra madre de Chiapas and Maya mountains (northern Central America): implications for the north American–Caribbean–Cocos plate boundary. Earth Surf. Dynam. 4, 71–102. doi:10.5194/esurf-4-71-2016
Anselmetti, F. S., Hodell, D. A., Ariztegui, D., Brenner, M., and Rosenmeier, M. F. (2007). Quantification of soil erosion rates related to ancient Maya deforestation. Geology 35 (10), 915–918. doi:10.1130/g23834a.1
Ardren, T., and Miller, S. (2020). Household garden plant agency in the creation of Classic Maya social identities. Jour. Anthropol. Archaeol. 60, 101212. doi:10.1016/j.jaa.2020.101212
Authemayou, C., Brocard, G., Teyssier, C., Suski, B., Cosenza, B., Morán-Ical, S., et al. (2012). Quaternary seismo-tectonic activity of the Polochic Fault, Guatemala. J. Geophys. Res. 117, B07403. doi:10.1029/2012JB009444
Bauer-Gottwein, P., Gondwe, B. R. N., Charvet, G., Marín, L. E., Rebolledo-Vieyra, M., and Merediz-Alonso, G. (2011). Review: the Yucatán peninsula karst aquifer, Mexico. Hydrogeol. Jour. 19 (3), 507–524. doi:10.1007/s10040-010-0699-5
Beach, T., Dunning, N., Luzzadder-Beach, S., Cook, D. E., and Lohse, J. (2006). Impacts of the ancient Maya on soils and soil erosion in the central Maya Lowlands. Catena 65 (2), 166–178. doi:10.1016/j.catena.2005.11.007
Beach, T., Luzzadder-Beach, S., Cook, D., Dunning, N., Kennett, D. J., Krause, S., et al. (2015). Ancient Maya impacts on the earth's surface: an early anthropocene analog? Quat. Sci. Rev. 124, 1–30. doi:10.1016/j.quascirev.2015.05.028
Beach, T., Luzzadder-Beach, S., Cook, D., Krause, S., Doyle, C., Eshleman, S., et al. (2018). Stability and instability on Maya Lowlands tropical hillslope soils. Geomorphology 305, 185–208. doi:10.1016/j.geomorph.2017.07.027
Beach, T., Luzzadder-Beach, S., Krause, S., Guderjan, T., Valdez, F., Fernandez-Diaz, J. C., et al. (2019). Ancient Maya wetland fields revealed under tropical forest canopy from laser scanning and multiproxy evidence. Proc. Natl. Acad. Sci. 116 (43), 21469–21477. doi:10.1073/pnas.1910553116
Beach, T., Luzzadder-beach, S., Dunning, N., Hageman, J., and Lohse, J. (2002). Upland agriculture in the Maya lowlands: ancient Maya soil conservation in northwestern Belize. Geogr. Rev. 92 (3), 372–397. doi:10.1111/j.1931-0846.2002.tb00149.x
Beach, T. (1998). Soil catenas, tropical deforestation, and ancient and contemporary soil erosion in the PETéN, Guatemala. Phys. Geogra. 19, 378–405. doi:10.1080/02723646.1998.10642657
Beddows, P. A., Mandić, M., Ford, D. C., and Schwarcz, H. P. (2016). Oxygen and hydrogen isotopic variations between adjacent drips in three caves at increasing elevation in a temperate coastal rainforest, Vancouver Island, Canada. Geoch. Cosmochi. Acta 172, 370–386. doi:10.1016/j.gca.2015.08.017
Bell, J. A. (1998). A developing model for determining cenote and associated site settlement patterns in the Yalahau region, Quintana Roo, Mexico. Master's thesis. Riverside: Department of Anthropology, University of California.
Berlin-Neubart, H. (1955). News from the Maya world. Ethnos 20 (4), 201–209. doi:10.1080/00141844.1955.9980799
Birkett, B. A., Obrist-Farner, J., Rice, P. M., Parker, W. G., Douglas, P. M., Berke, M. A., et al. (2023). Preclassic environmental degradation of lake Petén itzá, Guatemala, by the early Maya of nixtun-ch’ich. Comm. Earth Environ. 4 (1), 59. doi:10.1038/s43247-023-00726-4
Blatrix, R., Roux, B., Béarez, P., Prestes-Carneiro, G., Amaya, M., Aramayo, J. L., et al. (2018). The unique functioning of a pre-Columbian Amazonian floodplain fishery. Sci. Rep. 8 (1), 5998–6016. doi:10.1038/s41598-018-24454-4
Brenner, M., Hodell, D. A., Curtis, J. H., Rosenmeier, M. F., Anselmetti, F. S., and Ariztegui, D. (2003). Paleolimnological approaches for inferring past climate change in the Maya region: Recent advances and methodological limitations. New York, New York, USA: The Lowland Maya area three millennia at the human-wildland interface Food Products, 45–75.
Brenner, M., Rosenmeier, M. F., Hodell, D. A., and Curtis, J. H. (2002). Paleolimnology of the Maya lowlands: long-term perspectives on interactions among climate, environment, and humans. Anc. Mesoamer 13 (1), 141–157. doi:10.1017/S0956536102131063
Bronger, A., Winter, R., and Sedov, S. (1998). Weathering and clay mineral formation in two Holocene soils and in buried paleosols in tadjikistan: towards a quaternary paleoclimatic record in central asia. Catena 34 (1-2), 19–34. doi:10.1016/S0341-8162(98)00079-4
Bueno, J., Alvarez, F., and Santiago, S. (2005). Biodiversidad del Estado de Tabasco. México: Instituto de Biología, UNAM, 333pp.
Burkart, B. (1983). Neogene North American-caribbean plate boundary across northern Central America: offset along the polochic fault. Tectonophysics 99, 251–270. doi:10.1016/0040-1951(83)90107-5
Cabadas-Báez, H., Solleiro-Rebolledo, E., Sedov, S., Pi, T., and Gama-Castro, J. (2010a). Pedosediments of karstic sinkholes in the eolianites of NE Yucatán: A record of late quaternary soil development, geomorphic processes and landscape stability. Geomorphology 122, 323–337. doi:10.1016/j.geomorph.2010.03.002
Cabadas-Báez, H. V., Solleiro-Rebolledo, E., Sedov, S., Pi, T., and Alcalá, J. R. (2010b). The complex genesis of red soils in peninsula de Yucatan, Mexico: mineralogical, micromorphological and geochemical proxies. Eurasian Soil Sci. 43, 1439–1457. doi:10.1134/S1064229310130041
Cabadas-Báez, H. V., Solís-Castillo, B., Solleiro-Rebolledo, E., Sedov, S., Leonard, D., Teranishi-Castillo, K., et al. (2017). Reworked volcaniclastic deposits from the Usumacinta River, Mexico: A serendipitous source of volcanic glass in Maya ceramics. Geoarchaeology 32 (3), 382–399. doi:10.1002/gea.21610
Campiani, A., Flores, E. A., and López, M. J. (2012). “Topografía y espacio: el caso de Chinikihá, méxico,” in XXV Simposio de Investigaciones Arqueológicas en Guatemala, 2011 (pp722-753) (Guatemala: Ministerio de Cultura y Deportes. Instituto de Antropología y Asociación Tikal). Avaliable At: https://iris.uniroma1.it/handle/11573/1528449 (Accessed June 10, 2023).
Carozza, J. M., Galop, D., Metailie, J. P., Vannière, B., Bossuet, G., Monna, F., et al. (2007). Landuse and soil degradation in the southern Maya lowlands, from pre-classic to post-classic times: the case of La joyanca (Petén, Guatemala). Geodin. Acta 20 (4), 195–207. doi:10.3166/ga.20.195-207
Coffey, K. T., Schmitt, A. K., Ford, A., Spera, F. J., Christensen, C., and Garrison, J. (2014). Volcanic ash provenance from zircon dust with an application to Maya pottery. Geology 42 (7), 595–598. doi:10.1130/G35376.1
D'Amico, M. E., Casati, E., El Khair, D. A., Cavallo, A., Barcella, M., and Previtali, F. (2023). Aeolian inputs and dolostone dissolution involved in soil formation in Alpine karst landscapes (Corna Bianca, Italian Alps). Catena 230, 107254. doi:10.1016/j.catena.2023.107254
Davis, C. R. (2022). Monumentalizing metaphors: diphrasis in the murals of Tulum. Manuscript and text cultures. TRANSPOSITION Monum. PRE-MODERN Epigr. Manuscr. TRADITIONS 1, 55–82. doi:10.56004/v1d55
De Lapparent, J. (1930). Les bauxites de la France méridionale: Mémoires du Service de la Carte géologique detaillée de la France. Paris: Imprimerie Nationale, 187.
Dedrick, M., Webb, E. A., McAnany, P. A., Kumul, J. M. K., Jones, J. G., Alpuche, A. I. B., et al. (2020). Influential landscapes: temporal trends in the agricultural use of rejolladas at tahcabo, Yucatán, Mexico. Jour. Anthropol. Archaeol. 59, 101175. doi:10.1016/j.jaa.2020.101175
Diamond, J. (1994). Ecological collapses of past civilizations. Proceed. Amer. Philos. Soc. 138 (3), 363–370. Avaliable At: https://www.jstor.org/stable/986741.
Douglas, P. M., Demarest, A. A., Brenner, M., and Canuto, M. A. (2016). Impacts of climate change on the collapse of lowland Maya civilization. Annu. Rev. Earth Planet. Sci. 44, 613–645. doi:10.1146/annurev-earth-060115-012512
Douglas, P. M., Keenan, B., Parker, W. G., Breckenridge, A. J., Johnston, K., Obrist-Farner, J., et al. (2022). “Earth, fire, water, waste: using multiple lipid biomarkers to unravel complex environmental histories in the Maya lowlands,” in AGU fall meeting abstracts (San Francisco: AGU).
Douglas, P. M., Pagani, M., Canuto, M. A., Brenner, M., Hodell, D. A., Eglinton, T. I., et al. (2015). Drought, agricultural adaptation, and sociopolitical collapse in the Maya Lowlands. Proceed. Nat. Acad. Sci. 112 (18), 5607–5612. doi:10.1073/pnas.1419133112
Douglas, P. M., Pagani, M., Eglinton, T. I., Brenner, M., Curtis, J. H., Breckenridge, A., et al. (2018). A long-term decrease in the persistence of soil carbon caused by ancient Maya land use. Nat. Geosci. 11 (9), 645–649. doi:10.1038/s41561-018-0192-7
Doyle, C., Luzzadder-Beach, S., and Beach, T. (2023). Advances in remote sensing of the early anthropocene in tropical wetlands: from biplanes to lidar and machine learning. Prog. Phys. Geograp. Earth Environm 47 (2), 293–312. doi:10.1177/03091333221134185
Dunham, P. S., Abramiuk, M. A., Cummings, L. S., Yost, C., and Pesek, T. J. (2009). Ancient Maya cultivation in the southern Maya mountains of Belize: complex and sustainable strategies uncovered. Antiquity 83 (319), 1–3. Avaliable At: http://www.antiquity.ac.uk/projgall/pesek319/.
Dunning, N., Beach, T., Farrell, P., and Luzzadder-Beach, S. (1998). Prehispanic agrosystems and adaptive regions in the Maya lowlands. Cult. Agricul 20 (2-3), 87–101. doi:10.1525/cag.1998.20.2-3.87
Dunning, N., Beach, T., and Luzzadder-Beach, S. (2020). “Ancient Maya agriculture,” in The Maya world (New York, NY: Routledge), 501–518.
Dunning, N., Beach, T., Luzzadder-Beach, S., and Jones, J. J. (2009). “Creating a stable landscape: soil conservation and adaptation among the ancient Maya,” in The archaeology of environmental change: Socionatural legacies of degradation and resilience. Editors C. T. Fisher, J. B. Hill, and G. M. Feinman (Tucson: University of Arizona Press), 85–105.
Dunning, N. P., Anaya Hernández, A., Beach, T., Carr, C., Griffin, R., Jones, J. G., et al. (2019). Margin for error: anthropogenic geomorphology of bajo edges in the Maya lowlands. Geomorphol 331, 127–145. doi:10.1016/j.geomorph.2018.09.002
Dunning, N. P., Beach, T. P., and Luzzadder-Beach, S. (2012). Kax and kol: collapse and resilience in lowland Maya civilization. Proceed. Nat. Acad. Sci. 109 (10), 3652–3657. doi:10.1073/pnas.1114838109
Durán, R., and Méndez, M. (2010). Biodiversidad y desarrollo humano en Yucatán. CICY, PPD-FMAM. Mexico City: CONABIO, SEDUMA, 496.
Durand, N., Monger, H. C., and Canti, M. G. (2010). “Calcium carbonate features,” in Interpretation of micromorphological features of soils and regoliths. Editors G. Stoops, V. Marcelino, and F. Mees (Amsterdam: Elsevier), 149–194. doi:10.1016/C2009-0-18081-9
Durn, G., Ottner, F., and Slovenec, D. (1999). Mineralogical and geochemical indicators of the polygenetic nature of terra rossa in Istria, Croatia. Geoderma 91 (1-2), 125–150. doi:10.1016/S0016-7061(98)00130-X
Durn, G., Perković, I., Razum, I., Ottner, F., Škapin, S. D., Faivre, S., et al. (2023). A tropical soil (Lixisol) identified in the northernmost part of the Mediterranean (Istria, Croatia). Catena 228, 107144. doi:10.1016/j.catena.2023.107144
Erickson, C. L. (2000). An artificial landscape-scale fishery in the Bolivian Amazon. Nature 408 (6809), 190–193. doi:10.1038/35041555
Espinasa-Pereña, R. (2007). “El karst de México", mapa NA III 3,” in Nuevo atlas nacional de México. Editors A. Coll-Hurtado, and S. Coord (México: Instituto de Geografía, Universidad Nacional Autónoma de México). Avaliable At: http://librosoa.unam.mx/handle/123456789/2371 (Accessed June 10, 2023).
Evans, N. P., Bauska, T. K., Gázquez-Sánchez, F., Brenner, M., Curtis, J. H., and Hodell, D. A. (2018). Quantification of drought during the collapse of the classic Maya civilization. Science 361 (6401), 498–501. doi:10.1126/science.aas9871
Fedick, S. L., Flores Delgadillo, M., Sedov, S., Solleiro-Rebolledo, E., and Mayorga, S. P. (2008). Adaptation of Maya homegardens by “container gardening” in limestone bedrock cavities. J. Ethnobiol. 28 (2), 290–304. doi:10.2993/0278-0771-28.2.290
Fedick, S. L., and Hovey, K. (1995). “Ancient Maya settlement and use of wetlands at naranjal and the surrounding Yalahau region,” in The view from Yalahau: 1993 archaeological investigations in northern Quintana Roo, Mexico. Editors S. L. Fedick, and K. A. Taube (Riverside: University of California), 101–114.
Fedick, S. L. (1995). Land evaluation and ancient Maya land use in the upper Belize River area, Belize, Central America. Lat. Am. Antiq. 6, 16–34. doi:10.2307/971598
Fedick, S. L., Mathews, J. P., and Sorensen, K. (2012). Cenotes as conceptual boundary markers at the ancient Maya site of T’isil, Quintana Roo, Mexico. Mexicon 34 (5), 118–123. Avaliable At: https://digitalcommons.trinity.edu/socanthro_faculty/41/.
Fedick, S. L., and Mathews, J. P. (2005). “The yaluhau regional human ecology project: an introduction and summary of recent research,” in Quintana Roo archaeology. Editors J. M. Shaw, and J. P. Mathews (Tucson: University of Arizona Press), 33–50.
Fedick, S. L., Morell-Hart, S., and Dussol, L. (2023). Agriculture in the ancient Maya lowlands (Part 2): landesque capital and long-term resource management strategies. J. Archaeol. Res. doi:10.1007/s10814-023-09185-z
Fedick, S. L., Morrison, B. A., Andersen, B. J., Boucher, S., Acosta, J. C., and Mathews, J. P. (2000). Wetland manipulation in the Yalahau region of the northern Maya lowlands. J. Field Archaeol. 27 (2), 131–152. doi:10.1179/jfa.2000.27.2.131
Fedick, S. L., and Santiago, L. S. (2022). Large variation in availability of Maya food plant sources during ancient droughts. Proc. Natl. Acad. Sci. 119 (1), e2115657118. doi:10.1073/pnas.2115657118
Fedick, S. L., and Taube, K. A. (1995). The view from Yalahau: 1993 archaeological investigations in northern Quintana Roo, Mexico: Riverside. U.S.A: University of California, 151.
Fleury, S., Malaizé, B., Giraudeau, J., Galop, D., Bout-Roumazeilles, V., Martinez, P., et al. (2014). Impacts of Mayan land use on Laguna Tuspan watershed (Petén, Guatemala) as seen through clay and ostracode analysis. Jour. Archaeol. Sci. 49, 372–382. doi:10.1016/j.jas.2014.05.032
Flores-Delgadillo, L., Fedick, S. L., Solleiro-Rebolledo, E., Palacios-Mayorga, S., Ortega-Larrocea, P., Sedov, S., et al. (2011). A sustainable system of a traditional precision agriculture in a Maya homegarden: soil quality aspects. Soil Tillage Res. 113 (2), 112–120. doi:10.1016/j.still.2011.03.001
Folan, W. J., Anaya-Hernandez, A., Kintz, E. R., Fletcher, L. A., Gonzalez-Heredia, R., May-Hau, J., et al. (2009). Coba, Quintana Roo, Mexico: A recent analysis of the social, economic and political organization of a major Maya urban center. Anc. Mesoam. 20 (01), 59–70. doi:10.1017/s0956536109000054
Folan, W. J., Kintz, E. R., and Fletcher, L. A. (1983). “Coba, A classic Maya metropolis,” in Studies in archaeology (New York: Academic Press).
Ford, A., and Nigh, R. (2016). The Maya forest garden: Eight millennia of sustainable cultivation of the tropical woodlands. New York, NY: Routledge.
French, K. D., Straight, K. D., and Hermitt, E. J. (2020). Building the environment at Palenque: the sacred pools of the picota group. Anc. Mesoamer. 31 (3), 409–430. doi:10.1017/S0956536119000130
García, E. (1988). Modificaciones al Sistema de Clasificación climática de Köppen. México: Offset Larios Press.
Glover, J. B., Rissolo, D., Beddows, P. A., Jaijel, R., Smith, D., and Goodman-Tchernov, B. (2022). The Proyecto costa escondida: historical ecology and the study of past coastal landscapes in the Maya area. Jour. Isl. Coast. Archaeol. 2022, 1–20. doi:10.1080/15564894.2022.2061652
Glover, J. B., and Stanton, T. W. (2010). Assessing the role of preclassic traditions in the formation of early classic yucatec cultures, méxico. Field Archaeol. 35 (1), 58–77. doi:10.1179/009346910x12707320296711
Glover, J. B. (2012). The Yalahau region: A study of ancient Maya socio-political organization. Anc. Mesoamer. 23 (2), 271–295. doi:10.1017/s095653611200020x
Golden, C., Scherer, A. K., René-Muñoz, A., and Vasquez, R. (2008). Piedras Negras and yaxchilan: divergent political trajectories in adjacent Maya polities. Lat. Amer. Antiq. 19 (3), 249–274. doi:10.1017/s104566350000794x
Golden, C., Scherer, A. K., Schroder, W., Murtha, T., Morell-Hart, S., Fernandez Diaz, J. C., et al. (2021). Airborne lidar survey, density-based clustering, and ancient Maya settlement in the upper Usumacinta River region of Mexico and Guatemala. Remote Sens. 13 (20), 4109. doi:10.3390/rs13204109
Guillén, D. K. A. (2020). Evolución de la cubierta edáfica en el Valle Budsilhá, Chiapas: influencia de los factores formadores y las actividades humanas pre-hispánicas. [dissertation/masther´s tesis]. Posgrado de Ciencias de la Tierra: Universidad Nacional Autónoma de México.
Haug, G. H., Gunther, D., Peterson, L. C., Sigman, D. M., Hughen, K. A., and Aeschlimann, B. (2003). Climate and the collapse of Maya civilization. Science 299 (5613), 1731–1735. doi:10.1126/science.1080444
Hernández-Santana, J. R., Méndez-Linares, A. P., and Bollo-Manet, M. (2012). Análisis morfoestructural del relieve noroccidental del Estado de Chiapas, México. Rev. Geográfica Venez. 53, 57–75. Avaliable At: http://www.redalyc.org/articulo.oa?id=347730388004.
Hodell, D. A., Brenner, M., Curtis, J. H., and Guilderson, T. (2001). Solar forcing of drought frequency in the Maya Lowlands. Science 292, 1367–1370. doi:10.1126/science.1057759
Hodell, D. A., Brenner, M., and Curtis, J. H. (2005). Terminal Classic drought in the northern Maya lowlands inferred from multiple sediment cores in Lake Chichancanab (Mexico). Quat. Sci. Rev. 24 (12-13), 1413–1427. doi:10.1016/j.quascirev.2004.10.013
Islebe, G. A., Torrescano-Valle, N., Valdez-Hernández, M., Carrillo-Bastos, A., and Aragón-Moreno, A. A. (2022). Maize and ancient Maya droughts. Sci. Rep. 12 (1), 22272. doi:10.1038/s41598-022-26761-3
Isphording, W. C. (1975). The physical geology of Yucatan. Trans. Gulf Coast Assoc. Geol. Soc. 25, 231–262. Avaliable At: https://archives.datapages.com/data/gcags/data/025/025001/0231.htm.
IUSS Working Group WRB (2015). World reference base for soil resources 2014, update 2015, international soil classification systems for naming soils and creating legends for soil maps. World soil resources resports. Rome: FAO, 192.
Kelly, D. (2014). Archaeology of aboriginal fish traps in the murray–darling basin. Australia. Sydney: Charles Sturt University Press.
Krause, S., Beach, T., Luzzader-Beach, S., Cook, D., Islebe, G., Palacios-Fest, M. R., et al. (2019). Wetland geomorphology and paleoecology near Akab Muclil, Rio Bravo floodplain of the Belize coastal plain. Geomorph 331, 146–159. doi:10.1016/j.geomorph.2018.10.015
Krywy-Janzen, A., Reinhardt, E., McNeill-Jewer, C., Coutino, A., Waltham, B., Stastna, M., et al. (2019). Water-level change recorded in Lake Pac Chen Quintana Roo, Mexico infers connection with the aquifer and response to Holocene sea-level rise and Classic Maya droughts. J. Paleolimnol. 62, 373–388. doi:10.1007/s10933-019-00094-0
Kunen, J. L. (2001). Ancient Maya agricultural installations and the development of intensive agriculture in NW Belize. Jour. Field Archaeol 28 (3-4), 325–346. doi:10.1179/jfa.2001.28.3-4.325
Leonard, D., Sedov, S., Solleiro-Rebolledo, E., Fedick, S. L., and Diaz, J. (2019). Ancient Mayan use of hidden soilscapes in the Yalahau wetlands, northern Quintana Roo, Mexico. Bol. Soc. Geol. Mex. 71 (1), 93–119. doi:10.18268/bsgm2019v71n1a6
Leyden, B. W., Brenner, M., and Dahlin, B. H. (1998). Cultural and climatic history of Cobá, a lowland Maya city in Quintana Roo, Mexico. Quat. Res. 49 (1), 111–122. doi:10.1006/qres.1997.1941
Liendo-Stuardo, R., Solleiro-Rebolledo, E., Solis-Castillo, B., Sedov, S., and Ortíz-Pérez, A. (2014). 7 population dynamics and its relation to ancient landscapes in the northwestern Maya lowlands: evaluating resilience and vulnerability. Archeol. Pap. Am. Anthropol. Assoc. 24, 84–100. doi:10.1111/apaa.12031
Liendo-Stuardo, R. (2012). Vecinos cercanos. Palenque y el reino olvidado de Chinikihá. Palenque, nuevos Estud. nuevos hallazgos creación mítica, las Ciudad. Vecin. sepulturas, vida urbana, Reina Roja, los Gob. Arqueol. Mex. 19 (113), 44–48. Avaliable At: https://arqueologiamexicana.mx/mexico-antiguo/vecinos-cercanos-palenque-y-el-reino-olvidado-de-chinikiha.
Luzzadder-Beach, S., Beach, T. P., and Dunning, N. P. (2012). Wetland fields as mirrors of drought and the Maya abandonment. Proc. Natl. Acad. Sci. U. S. A. 109, 3646–3651. doi:10.1073/pnas.1114919109
Macrae, S., and Iannone, G. (2016). Understanding ancient Maya agricultural terrace systems through Lidar and hydrological mapping. Adv. Archaeol. Pract. 4 (3), 371–392. doi:10.7183/2326-3768.4.3.371
Maler, T. (1901). Researches in the central portion of the usumatsintla valley. Memories of the peabody museum of American archaeology and ethnology. Harv. Univ. 2, 2. Avaliable At: https://curiosity.lib.harvard.edu/expeditions-and-discoveries/catalog/38-990011850510203941.
Martin, S., and Grube, N. (2008). Chronicle of the Maya kings and queens: Deciphering the dynasties of the ancient Maya. 2nd ed. New York: Thames and Hudson.
Mathews, J. P., and Maldonado-Cárdenas, R. (2006). “Late formative and early classic interaction spheres reflected in the megalithic style,” in Lifeways in the northern Maya lowlands. Editors J. P. Mathews, and B. A. Morrison (Tucson: The University of Arizona Press), 95–118.
McKillop, H. I. (2023). Flooded mangrove landscapes hide ancient Maya coastal sites in Belize. Jour. Isl. Coast. Archaeol. 2023, 1–21. doi:10.1080/15564894.2022.2163323
Medina-Elizalde, M., Burns, S. J., Lea, D. W., Asmerom, Y., von Gunten, L., Polyak, V., et al. (2010). High resolution stalagmite climate record from the Yucatán Peninsula spanning the Maya terminal classic period. Earth Planet. Sci. Lett. 298 (1-2), 255–262. doi:10.1016/j.epsl.2010.08.016
Merino, E., and Banerjee, A. (2008). Terra rossa genesis, implications for karst, and eolian dust: A geodynamic thread. Jour. Geol. 116 (1), 62–75. doi:10.1086/524675
Miksicek, C. H. (2019). “Early wetland agriculture in the Maya lowlands: clues from preserved plant remains,” in Ancient Maya wetland agriculture 295-312 (New York, NY: Routledge).
Morell-Hart, S., Dussol, L., and Fedick, S. L. (2022). Agriculture in the ancient Maya lowlands (Part 1): paleoethnobotanical residues and new perspectives on plant management. J. Archaeol. Res. 2022, 1–55. doi:10.1007/s10814-022-09180-w
Morrison, B. A., and Cozatl-Manzano, R. (2003). “Initial evidence for use of periphyton as an agricultural fertilizer by the ancient Maya associated with the el Edén wetland, northern Quintana Roo, Mexico,” in The lowland Maya area: Three millenia at the human-wildland interface. Editors A. Gómez-Pompa, M. F. Allen, and S. L. Fedick (Binghamton, NY: Haworth Press), 401–414.
Ortiz, M. A., Siebe, C., and Cram, S. (2005). “Diferenciación ecogeográfica de Tabasco,” in Biodiversidad del Estado de Tabasco: Instituto de Biología. Editors J. en Bueno, F. Álvarez, and S. Santiago (México: UNAM-CONABIO), 305–322.
Padilla, R. J., and Sánchez, R. J. (2007). Evolución geológica del sureste mexicano desde el Mesozoico al presente en el contexto regional del Golfo de México. Bol. Soc. Geol. Mex. 59, 19–42. doi:10.18268/bsgm2007v59n1a3
Palka, J. W. (2023). Ancestral Maya domesticated waterscapes, ecological aquaculture, and integrated subsistence. Anc. Mesoamer 2023, 1–29. doi:10.1017/S0956536122000402
Pérez de Heredia, E., Bíró, P., and Boucher, S. (2021). Maíz y balché. Una revisión de la iconografía de los murales de Tulum. Estud. Cult. maya 57, 117–149. doi:10.19130/iifl.ecm.57.2021.18655
Pohl, M. D., Pope, K. O., Jones, J. G., Jacob, J. S., Piperno, D., DeFrance, S., et al. (1996). Early agriculture in the Maya lowlands. Lat. Amer. Antiq. 7 (4), 355–372. doi:10.2307/972264
Priori, S., Costantini, E. A., Capezzuoli, E., Protano, G., Hilgers, A., Sauer, D., et al. (2008). Pedostratigraphy of Terra Rossa and Quaternary geological evolution of a lacustrine limestone plateau in central Italy. J. Plant Nutr. Soil Sci. 171 (4), 509–523. doi:10.1002/jpln.200700012
Rissolo, D., Ochoa-Rodriguez, J. M., and Ball, J. W. (2005). “A reassessment of the middle preclassic in northern Quintana Roo,” in Quintana Roo archaeology. Editors J. M. Shaw, and J. P. Mathews (Tucson: University of Arizona Press), 66–76.
Robles-Castellanos, F. (1990). La secuencia cerámica de la Region de Cobá, Quintana Roo. Serie arqueología 184. Mexico City: Instituto Nacional de Antropología e Historia.
Rosenmeier, M. F., Hodell, D. A., Brenner, M., Curtis, J. H., and Guilderson, T. P. (2002). A 4000-year lacustrine record of environmental change in the southern Maya lowlands, Petén, Guatemala. Quater. Res. 57 (2), 183–190. doi:10.1006/qres.2001.2305
Rzedowski, J. (2006). Vegetación de México. 1era. Edición digital. México. Mexico: Comisión Nacional para el Conocimiento y el Uso de la Biodiversidad Press.
Sandler, A., Meunier, A., and Velde, B. (2015). Mineralogical and chemical variability of mountain red/brown Mediterranean soils. Geoderma 239, 156–167. doi:10.1016/j.geoderma.2014.10.008
Sauro, U. (2019). “Closed depressions in karst areas,” in Encyclopedia of caves (Academic Press). doi:10.1016/B978-0-12-814124-3.00032-7
Scarborough, V. L., Dunning, N. P., Tankersley, K. B., Carr, C., Weaver, E., Grazioso, L., et al. (2012). Water and sustainable land use at the ancient tropical city of Tikal, Guatemala. Proc. Natl. Acad. Sci. 109 (31), 12408–12413. doi:10.1073/pnas.1202881109
Scherer, A. K., and Golden, C. (2012). Revisiting Maler´s Usumacinta: Recent archaeological investigations in Chiapas, Mexico. Mexico: Precolumbia Mesoweb Press.
Schroder, W., Murtha, T., Broadbent, E. N., and Almeyda Zambrano, A. M. (2021). A confluence of communities: households and land use at the junction of the upper Usumacinta and lacantún rivers, Chiapas, Mexico. World Archaeol. 53 (4), 688–715. doi:10.1080/00438243.2021.1930135
Schüpbacha, S., Kirchgeorga, T., Colombarolic, D., Beffac, G., Radaellif, M., Kehrwalda, N., et al. (2015). 2 study II: combining charcoal sediment and molecular markers to infer a Holocene fire history in the Maya lowlands of Petén, Guatemala. Specif. Mol. Markers Lake Sediment Cores Biomass Burn. Reconstr. Dur. Holocene 115, 123–131. doi:10.1016/j.quascirev.2015.03.004
Sedov, S., Guillén, D. K., Solleiro,-Rebolledo, R. E., Rivera, U. Y., and Díaz, O. J. (2021). Gipsisoles en la cubierta edáfica de los paisajes kársticos tropicales de Chiapas. Geos 40 (1), 1–184.
Sedov, S., Solleiro-Rebolledo, E., Fedick, S. L., Gama-Castro, J., Palacios-Mayorga, S., and Vallejo Gomez, E. (2007). Soil genesis in relation to landscape evolution and ancient sustainable land use in the northeastern Yucatan Peninsula, Mexico. Atti della Soc. Toscana Sci. Nat. Pisa, Ser. A 112, 115–126. Avaliable At: http://www.stsn.it/serA112/14%20Sedov.pdf.
Sedov, S., Solleiro-Rebolledo, E., Fedick, S. L., Pi-Puig, T., Vallejo-Gómez, E., and Flores-Delgadillo, M. D. L. (2008). Micromorphology of a soil catena in Yucatán: pedogenesis and geomorphological processes in a tropical karst landscape. New trends soil Micromorphol. 2008, 19–37. doi:10.1007/978-3-540-79134-8_3
Shapiro, M. B. (2006). Soils of Israel. Eurasian Soil Sci. 39, 1170–1175. doi:10.1134/S1064229306110032
Solís-Castillo, B., Christine, T., Cabadas-Baez, H., Solleiro-Rebolledo, E., Sedov, S., Terhorst, B., et al. (2013b). Holocene sequences in the Mayan Lowlands - a provenance study using heavy mineral distributions. Quat. Sci. J. 62, 2. doi:10.3285/eg.62.2.01
Solís-Castillo, B., Golyeva, A., Sedov, S., Solleiro-Rebolledo, E., and López-Rivera, S. (2015). Phytoliths, stable carbon isotopes and micromorphology of a buried alluvial soil in southern Mexico: A polychronous record of environmental change during middle Holocene. Quat. Int. 365, 150–158. doi:10.1016/j.quaint.2014.06.043
Solís-Castillo, B., Ortíz-Pérez, M. A., and Solleiro-Rebolledo, E. (2014). Unidades geomorfologico-ambientales de las tierras bajas Mayas de Tabasco-Chiapas en el río Usumacinta: un registro de los procesos aluviales y pedológicos durante el Cuaternario. Bol. Soc. Geol. Mex. 66 (2), 279–290. doi:10.18268/bsgm2014v66n2a5
Solís-Castillo, B., Solleiro-Rebolledo, E., Sedov, S., Liendo, R., López-Rivera, S., and Ortiz-Pérez, M. A. (2013a). Paleoenvironment and human occupation in the Maya lowlands of the Usumacinta River, southern Mexico. Geoarchaeology 28, 268–288. doi:10.1002/gea.21438
Solleiro-Rebolledo, E., Cabadas-Báez, H. V., Pi, P. T., González, A., Fedick, S. L., Chmilar, J. A., et al. (2011). Genesis of hydromorphic Calcisols in wetlands of the northeast yucatan peninsula, Mexico. Geomorphology 135 (3-4), 322–331. doi:10.1016/j.geomorph.2011.02.009
Solleiro-Rebolledo, E., Terhorst, B., Cabadas-Báez, H., Sedov, S., Damm, B., Sponholz, B., et al. (2015). Influence of Mayan land use on soils and pedosediments in karstic depressions in Yucatan, México. Erlanger Geogr. Arb. Band. 42, 233–266. Avaliable At: https://www.geographie.uni-wuerzburg.de/bodenkunde/publikationen/.
Stoops, G. (2018). “Micromorphology as a tool in soil and regolith studies,” in Interpretation of micromorphological features of soils and regoliths. Editors G. Stoops, V. de Melo Marcelino, and F. Mees (Amsterdam, Netherlands: Elsevier), 1–19.
Targulian, V. O., and Goryachkin, S. V. (2004). Soil memory: types of record, carriers, hierarchy and diversity. Rev. Mex. Cienc. Geol. 21 (1), 1–8. Avaliable At: http://rmcg.geociencias.unam.mx/index.php/rmcg/article/view/897.
Targulian, V. O., and Krasilnikov, P. V. (2007). Soil system and pedogenic processes: self-organization, time scales, and environmental significance. Catena 71, 373–381. doi:10.1016/j.catena.2007.03.007
Turner, B. L., and Sabloff, J. A. (2012). Classic Period collapse of the central Maya lowlands: insights about human–environment relationships for sustainability. Proc. Natl. Acad. Sci. 109 (35), 13908–13914. doi:10.1073/pnas.1210106109
Turner, B. T. (2019). Once beneath the forest: Prehistoric terracing in the Rio bec region of the Maya lowlands. New York: Routledge Press.
Valera-Fernández, D., Cabadas-Báez, H., Solleiro-Rebolledo, E., Landa-Arreguín, F. J., and Sedov, S. (2020). Pedogenic carbonate crusts (calcretes) in karstic landscapes as archives for paleoenvironmental reconstructions–A case study from Yucatan Peninsula, Mexico. Catena 194, 104635. doi:10.1016/j.catena.2020.104635
Valera-Fernández, D., Solleiro-Rebolledo, E., López-Martínez, R., Sedov, S., Griset, S., and Cabadas-Báez, H. (2022). Quaternary paleoenvironments based on pedogenic, sedimentary and karstic processes in the coastal geosystems of Cozumel Island, Mexico. Geoderma Reg. 31, e00587. doi:10.1016/j.geodrs.2022.e00587
Vrščaj, B., Repe, B., Simončič, P., and Repe, B. (2017). Regions and landscapes. Soils Slovenia 2017, 9–17. doi:10.1007/978-94-017-8585-3_2
Walden, J. P., Hoggarth, J. A., Ebert, C. E., Fedick, S. L., Biggie, M., Meyer, B., et al. (2023). Classic Maya settlement systems reveal differential land use patterns in the upper Belize river valley. Land 12 (2), 483. doi:10.3390/land12020483
Waltham, T. (2008). Sinkhole hazard case histories in karst terrains. Q. J. Eng. Geol. Hydrogeol 41 (3), 291–300. doi:10.1144/1470-9236/07-211
Ward, W. C. (1985). “Quaternary geology of northeastern yucatan peninsula,” in Geology and hydrogeology of the yucatan and quaternary geology of northeastern yucatan peninsula. Editors W. C. Ward, A. E. Weidie, and W. Black (New Orleans Geol. Soc), 23–95.
Weidie, A. E., Ward, W. C., and Marshall, R. H. (1985). “Geology of yucatan platform,” in Geology and hydrogeology of the yucatan and quaternary geology of northeastern yucatan peninsula. Editors W. C. Ward, A. E. Weidie, and W. Black (New Orleans Geological Society), 3–29.
West, R. C., Psuty, N. P., and Thom, B. G. (1969). The Tabasco lowlands of southeastern Mexico. Mexico: Louisiana State University Press.
Wollwage, L., Fedick, S., Sedov, S., and Solleiro-Rebolledo, E. (2012). The deposition and chronology of cenote T’isil: A multiproxy study of human/environment interaction in the northern M aya lowlands of southeast Mexico. Geoarchaeol 27 (5), 441–456. doi:10.1002/gea.21418
Yaalon, D. H. (1997). Soils in the mediterranean region: what makes them different? Catena 28 (3-4), 157–169. doi:10.1016/S0341-8162(96)00035-5
Zhang, X. B., Bai, X. Y., and He, X. B. (2011). Soil creeping in the weathering crust of carbonate rocks and underground soil losses in the karst mountain areas of southwest China. Carbon. Evap. 26, 149–153. doi:10.1007/s13146-011-0043-8
Keywords: pedodiversity, karst, archaeology, Mayas, land use
Citation: Sedov S, Rivera-Uria MY, Ibarra-Arzave G, García-Ramírez P, Solleiro-Rebolledo E, Cabadas-Báez HV, Valera-Fernández D, Díaz-Ortega J, Guillén-Domínguez KA, Moreno-Roso SdJ, Fedick SL, Leonard D, Golden C, Morell-Hart S and Liendo-Stuardo RR (2023) Soil toposequences, soil erosion, and ancient Maya land use adaptations to pedodiversity in the tropical karstic landscapes of southern Mexico. Front. Earth Sci. 11:1239301. doi: 10.3389/feart.2023.1239301
Received: 13 June 2023; Accepted: 13 September 2023;
Published: 23 October 2023.
Edited by:
Anna Andreetta, University of Florence, ItalyReviewed by:
Simone Priori, University of Tuscia, ItalyMaria Sol Raigemborn, Universidad Nacional de La Plata-CONICET, Argentina
Copyright © 2023 Sedov, Rivera-Uria, Ibarra-Arzave, García-Ramírez, Solleiro-Rebolledo, Cabadas-Báez, Valera-Fernández, Díaz-Ortega, Guillén-Domínguez, Moreno-Roso, Fedick, Leonard, Golden, Morell-Hart and Liendo-Stuardo. This is an open-access article distributed under the terms of the Creative Commons Attribution License (CC BY). The use, distribution or reproduction in other forums is permitted, provided the original author(s) and the copyright owner(s) are credited and that the original publication in this journal is cited, in accordance with accepted academic practice. No use, distribution or reproduction is permitted which does not comply with these terms.
*Correspondence: M. Yazmin Rivera-Uria, rivera.uria@gmail.com