Comparing the associations of central venous pressure and pulmonary artery pulsatility index with postoperative renal injury
- 1Department of Anesthesiology, University of Kansas Medical Center, Kansas City, KS, United States
- 2Department of Population Health, University of Kansas Medical Center, Kansas City, KS, United States
Objective: Cardiac surgery-associated acute kidney injury (CS-AKI) is associated with significant morbidity and mortality. We investigated the association of postoperative central venous pressure (CVP) and pulmonary artery pulsatility index (PAPi) with the development of CS-AKI.
Methods: This was a single-center, retrospective cohort study of patients undergoing cardiac surgery. CVP and PAPi were acquired hourly postoperatively and averaged for up to 48 h. PAPi was calculated as [(Pulmonary Artery Systolic Pressure–Pulmonary Artery Diastolic Pressure) / CVP]. The primary aim was CS-AKI. Secondary aims were need for renal replacement therapy (RRT), hospital and 30-day mortality, total ventilator and intensive care unit hours, and hospital length of stay. Logistic regression was used to calculate odds of development of renal injury and need for RRT.
Results: One thousand two hundred eighty-eight patients were included. The average postoperative CVP was 10.3 mmHg and average postoperative PAPi was 2.01. Patients who developed CS-AKI (n = 384) had lower PAPi (1.79 vs. 2.11, p < 0.01) and higher CVP (11.5 vs. 9.7 mmHg, p < 0.01) than those who did not. Lower PAPi and higher CVP were also associated with each secondary aim. A standardized unit decrease in PAPi was associated with increased odds of CS-AKI (OR 1.39, p < 0.01) while each unit increase in CVP was associated with both increased odds of CS-AKI (OR 1.56, p < 0.01) and postoperative RRT (OR 1.49, p = 0.02).
Conclusions: Both lower PAPi and higher CVP values postoperatively were associated with the development of CS-AKI but only higher CVP was associated with postoperative RRT use. When differences in values are standardized, CVP may be more associated with development of CS-AKI when compared to PAPi.
Introduction
Acute kidney injury (AKI) occurs in ~25% of patients following cardiac surgery and is associated with significant morbidity and mortality (1–3). The etiology of cardiac surgery-associated AKI (CS-AKI) is multifactorial and results from a combination of underlying disease burden and perioperative insults (1–4). An increasingly recognized contributor to CS-AKI is right ventricular (RV) dysfunction which decreases renal perfusion due to elevated central venous pressure (CVP) (5–7). Increased CVP has been associated with renal dysfunction even independent of cardiac output, and remains an important focus of perioperative care in cardiac surgical patients (8–10). Although the utility of CVP as a marker of volume status and venous congestion has been called into question (11), recent evidence has supported the association of higher CVP and CS-AKI secondary to increased inflammation and sympathetic activation (10, 12, 13).
The pulmonary artery pulsatility index (PAPi) is a novel hemodynamic measurement of RV function defined as the pulmonary artery pulse pressure divided by CVP (14–16). Though originally used to assess RV failure following acute myocardial infarction (15), decreased PAPi has been proposed to predict postoperative RV dysfunction after major cardiac surgery such as heart transplantation and ventricular assist device implantation (7, 17–20). There is also evidence that the development of RV dysfunction and resultant venous congestion contributes to renal dysfunction following cardiac surgery and has made PAPi a new area of recent investigation (5, 6, 21).
Due to lack of robust literature specific to postoperative cardiac surgical patients, we sought to compare the associations of postoperative PAPi and CVP to CS-AKI in this patient population. Furthermore, we also sought to compare which of the two hemoynamic parameters has a stronger association with CS-AKI.
Materials and methods
This was a single-center retrospective cohort study of adult patients who underwent cardiac surgery between January 1st, 2017 and December 31st, 2019 at a tertiary-care academic medical center. This study was approved for a quality improvement designation and considered exempt from review by the University of Kansas institutional review board, and the requirement for written informed consent was consequently waived.
Adult patients undergoing cardiac surgical procedures with the use of cardiopulmonary bypass were included in the study. All patients received general endotracheal anesthesia and a pulmonary artery catheter. The intraoperative anesthetic management was not protocolized, but followed institutional standard practices consisting of midazolam and fentanyl for pre-induction sedation followed by isoflurane, fentanyl, midazolam and vecuronium administration during general anesthesia. Protocolized mechanical ventilation and extubation protocols were followed in the intensive care unit (ICU) (22). This protocol initially utilizes volume control ventilation and PEEP of 5 cm H2O and patients are weaned incrementally to pressure support of 5 cm H2O and PEEP of 5 cm H2O until eventual extubation as able based on blood gas analysis and clinical examination (22). Our ventilator weaning protocol is provided in a Supplementary Figure. For duplicate patients, the earliest procedure was used as the index case, with subsequent cases excluded from analysis. A total of 1,355 patients were initially included in the study. Sixty-seven patients were excluded from our study: 30 patients for missing PAPi or other relevant hemodynamic data; 2 patients for missing preoperative serum creatinine data; 1 patient who was requiring hemodialysis preoperatively; and 34 patients who were duplicate (Figure 1).
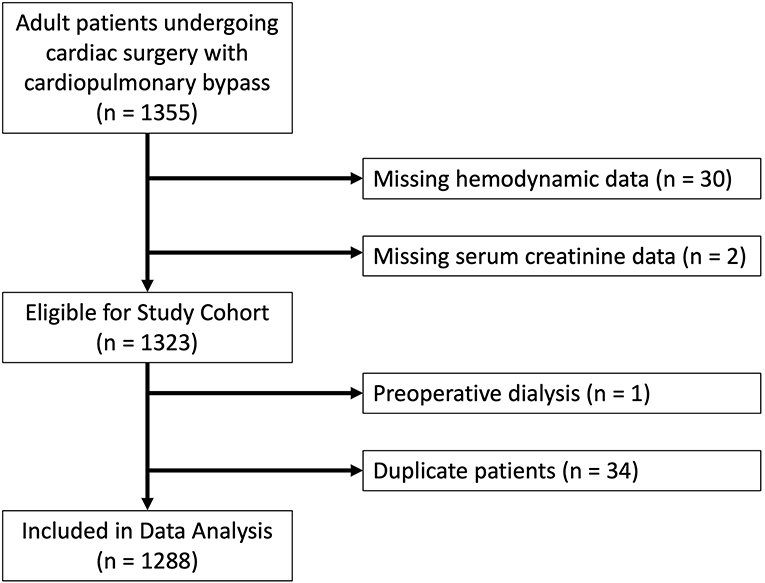
Figure 1. Flowchart of study design with reasons for exclusion for patients excluded from final analysis.
All hemodynamic data were acquired from the electronic medical record (Epic Systems, Verona, WI). Invasive central and pulmonary artery pressures were measured with a pulmonary artery catheter and associated patient monitor (IntelluVue, Philips Healthcare, Andover, Massachusetts). Patients obtained at least one daily chest radiograph to monitor correct position of the pulmonary artery catheter and clinical or waveform changes concerning for catheter displacement was addressed by an intensivist immediately available for 24-h per day. Hemodynamic measurement samples for CVP and pulmonary artery pressures were taken at a resolution of four samples per second directly from the monitor, which also accounts for inspiration and expiration cycles with updated waves at a speed of 3 cm/min. PAPi was calculated as [(Pulmonary Artery Systolic Pressure–Pulmonary Artery Diastolic Pressure) / CVP] (15) using the average of the first 48-h of postoperative care for each data point. If patients had their pulmonary artery catheter removed prior to 48-h, the average of the time data available was used. In total, we had 396 unique missing datapoints, which translated to about 0.6% of total datapoints obtained for our study cohort.
The primary aim was association of postoperative PAPi and CVP with postoperative acute renal injury, defined as a rise in postoperative serum creatinine of ≥ 1.5 times the baseline per Kidney Disease Improving Global Outcomes (KDIGO) criteria (23). Secondary aims included association of perioperative PAPi and CVP with need for renal replacement therapy (RRT), hospital mortality, 30-day mortality, total postoperative ventilator hours, total postoperative intensive care unit hours, and hospital length of stay (defined in days from date of surgery to discharge).
Statistical analysis
Categorical variables were described by frequency and percentage while normally distributed continuous variables were described by mean, minimum, and maximum. For comparisons between groups, Chi-square tests were used to test categorical variables and the Student's t-test used to test continuous variables. Correlations were tested using the Pearson correlation. Bivariate logistic regression models were used to calculate odds ratios of development of renal injury and requirement of renal replacement therapy. Two separate multiple logistic regression models were used for each, with one containing PAPi as an independent variable and the other containing CVP as an independent variable due to PAPi being a derived variable from CVP. To better compare odds ratios for our primary aims, we used standardized values of one standard deviation for PAPi and CVP in the regression analysis. All analyses were conducted using SAS V 9.4 (Copyright © 2002-2012 by SAS Institute Inc., Cary, NC, USA. All Rights Reserved). A p-value threshold of < 0.05 was used for statistical significance.
Result
A final cohort of 1,288 patients were included for analysis. Patient characteristics are provided in Table 1. The median time for pulmonary artery catheterization and data collection was 20.3 h. The average postoperative CVP was 10.3 mmHg (Min-Max 1–36.8mmHg) and the average postoperative PAPi was 2.01 (Min-Max 0.29–10.33).
The associations between CVP and PAPi with primary and secondary aims are provided in Table 2. Development of postoperative renal injury occurred in 29.8% of patients (n = 384). These patients had a lower average postoperative PAPi [1.79 (95% C.I. 1.70–1.87) vs. 2.11 (2.03–2.18), p < 0.01] and a higher average postoperative CVP [11.5 mmHg (95% C.I. 11.2–11.9) vs. 9.7 mmHg (95% C.I. 9.5–9.9), p < 0.01] compared to patients who did not develop renal dysfunction. Lower postoperative PAPi was also associated with patients who experienced in-hospital mortality [1.59 (95% C.I. 1.28–1.91) vs. 2.02 (1.96–2.08), p = 0.02] and 30-day mortality [1.63 (95% C.I. 1.35–1.90) vs. 2.02 (95% C.I. 1.96–2.08), p = 0.03]. Higher CVP was also associated with hospital mortality [14.3 mmHg (95% C.I. 11.7–16.9) vs. 10.2 mmHg (95% C.I. 10.0–1.03), p < 0.01] and 30-day mortality [13.6 mmHg (95% C.I. 11.4–15.9) vs. 10.2 mmHg (95% C.I. 10.0–10.3), p < 0.01]. Postoperative renal replacement therapy was required in 2% of patients (n = 22). These patients had lower postoperative PAPi [1.42 (95% C.I. 1.15–1.70) vs. 2.02 (1.96–2.08), p < 0.01] and higher postoperative CVP [14.4 mmHg (95% C.I. 11.9–16.8) vs. 10.2 mmHg (95% C.I. 10.0–10.3), p < 0.01]. Lower postoperative PAPi values correlated with increased hospital length of stay (correlation coefficient −0.09, p < 0.01), postoperative ventilator hours (−0.13, p < 0.01) and ICU hours (−0.11, p < 0.01). Likewise, patients with higher CVP values tended to have increased length of stay (0.19, p < 0.01), ventilator hours (0.22, p < 0.01) and ICU hours (0.30, p < 0.01).
Our multivariable regression analyses used standardized values of one standard deviation of PAPi (1.0) and CVP (3.0 mmHg) (Table 3). We used OR associated with a decrease in PAPi, which is directly correlated with an increase in CVP, in order to better compare the findings of our models. For renal injury, our regression model containing PAPi as an independent variable demonstrated higher odds of developing renal injury with decreasing PAPi (OR1.39, p < 0.01), higher age (OR 1.02, p < 0.01), presence of chronic lung disease (OR 1.91, p < 0.01) higher BMI (OR 1.04, p < 0.01), longer CPB time (1.01, p < 0.01), IABP use (1.98, p < 0.02), urgent (2.04, p < 0.01) or emergent (2.37, p < 0.01) surgery, and undergoing VAD placement (3.04, p < 0.01) or orthotopic heart transplant (3.69, p < 0.01). Similar findings were noted in the regression analysis containing CVP as an independent variable, with higher CVP associated with higher odds of renal injury (1.56, p < 0.01).
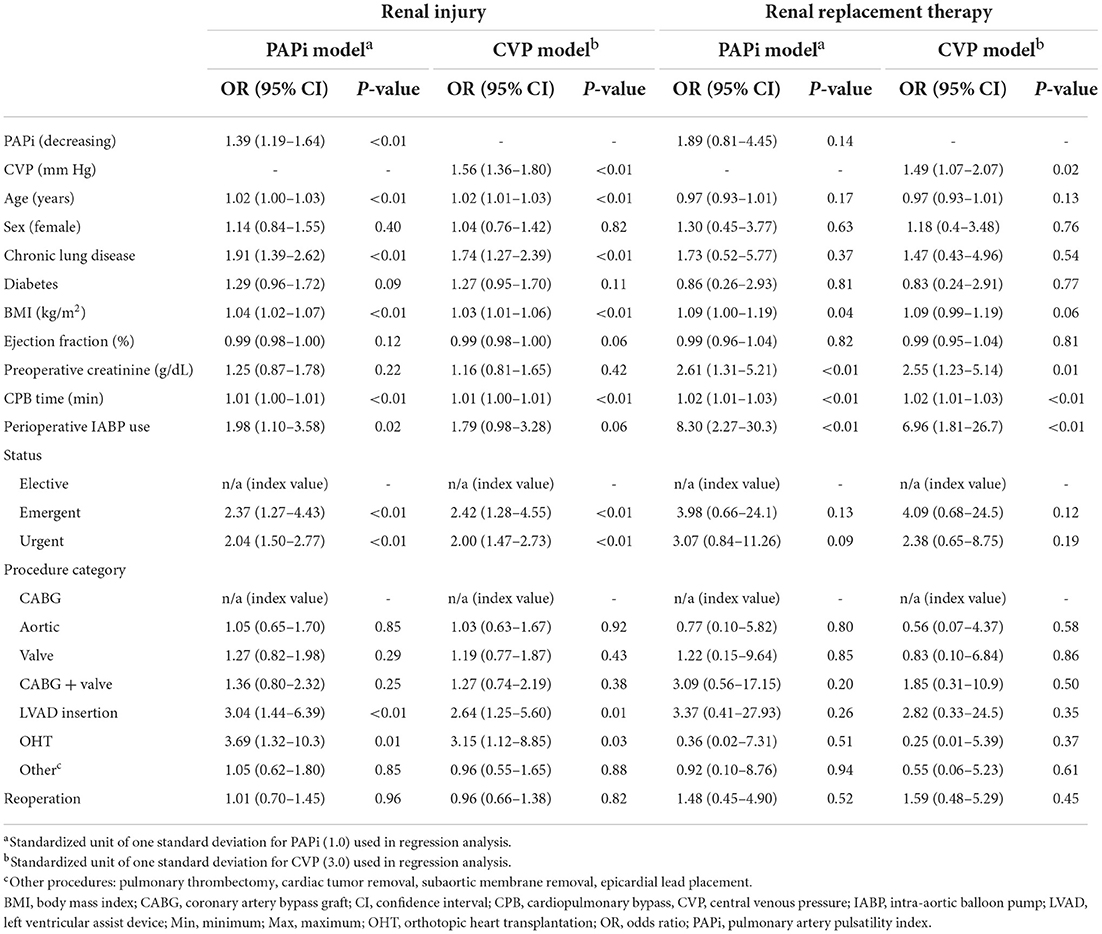
Table 3. Multivariable logistic regression analyses for renal injury and renal replacement therapy in association with postoperative pulmonary artery pulsatility index (PAPi) and central venous pressure (CVP) measurements.
In our logistic regression for postoperative need for renal replacement therapy (Table 3), our model with CVP as the independent variable demonstrated that higher CVP (OR 1.49, p = 0.02) was associated with higher odds ratio of requiring RRT, along with higher preoperative creatinine (2.55, p = 0.01), longer CPB time (1.02, p < 0.01) and perioperative IABP use (6.96, p < 0.01). Our model with PAPi as the independent variable did not demonstrate a significant association with need for RRT (1.89, p = 0.14). A graphical representation of adjusted OR for renal injury and need for RRT are visually represented in Figure 2.
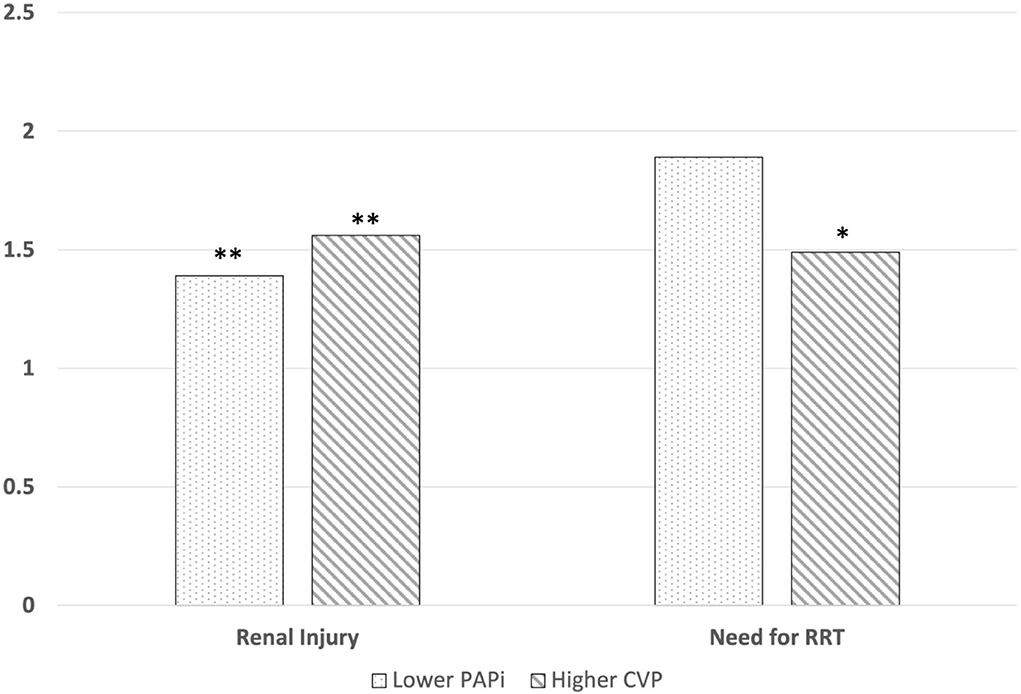
Figure 2. Graphical representations for adjusted odds ratios for the association between postoperative PAPi and CVP and developing CS-AKI and requiring RRT The adjusted odds ratio for developing post-cardiac surgical acute kidney injury (AKI) in patients who developed AKI was 1.39 (p < 0.01) for PAPi and 1.56 for CVP (p < 0.01). The adjusted odds ratio for requiring RRT in 1.89 (p = 0.14) for PAPi and 1.49 for CVP (p = 0.02). ** = p < 0.01, * = p < 0.05.
Discussion
This is the first study to demonstrate that both high CVP and low PAPi in the postoperative setting is a risk factor for CS-AKI. The relationship of CVP to AKI has only recently been demonstrated in this population, so corroborating this finding is important in and of itself (10). However, to demonstrate that PAPi, presumably by virtue of reduced right heart function and subsequent venous congestion, is also associated with CS-AKI is a novel finding. Secondly, higher postoperative CVP values were associated with use of RRT, whereas lower PAPi was not. Lastly, we demonstrate that when comparing CVP to PAPi in patients who developed CS-AKI, CVP had a more sensitive odds ratio, potentially making CVP the more important hemodynamic parameter when attempting to prevent CS-AKI.
We chose to standardize values for our analysis given the inherent challenge in directly comparing per-unit differences between PAPi, which is dimensionless, and CVP, which is measured in mmHg. In doing so, CVP proved more clinically valuable than PAPi when comparing adjusted odds ratios of developing CS-AKI. Our findings suggest that PAPi may not have a robust association with CS-AKI compared to CVP, and adds credence to the currently utility of these hemodynamic data points as markers for the risk of developing kidney injury in the postoperative cardiac surgical period (20). This may be due to the process associated with the development of CS-AKI being more dependent upon venous congestion, which is more accurately represented by CVP and less a sequela of PAPi, which represents multiple aspects of right ventricular afterload and may not be as specific. The development of the PAPi calculation was proposed as an easily obtainable and since validated indicator for right ventricular dysfunction (15). Lower PAPi values, commonly < 2.0, have been associated with worse patient outcomes in various cohorts (7, 14, 17–20). While calculation of PAPi relies upon CVP, the two are different in what is represented. PAPi is meant to holistically account for the complex interplay between right heart preload and afterload. Since PAPi is a derived value, changes in PAPi can be driven by its components and may not fully reflect right heart function across a diverse patient population.
Meanwhile, multiple studies have proposed that venous congestion is a contributor to perioperative AKI due to increased renal afterload from the “back up” of volume into the renal glomeruli (24–26). A CVP of >10 mmHg at the conclusion of cardiac surgery has been associated with more than a five-fold increased risk of AKI and more than a four-fold increased risk of mortality (27). Recent studies have also found that both the intraoperative magnitude and duration of increased CVP is associated with increased AKI (10). Thus, using CVP rather than PAPi may be a more appropriate harbinger for CS-AKI rather than PAPi when used in this context and places import upon therapeutics aimed at fluid removal in the ICU (28, 29). These findings may be of interest for advocates for the adoption of less invasive assessments of cardiac function if a central venous catheter can provide adequate clinical information vs. a pulmonary artery catheter (30, 31).
The current study averaged CVP and PAPi values in the ICU, as opposed to hemodynamic values obtained at single timepoints perioperatively. The relationship between CS-AKI and intraoperative and patient factors is well-established in the literature, but the impact of early postoperative care on renal injury is less understood (1–4, 32). However, elevated postoperative CVP is also associated with CS-AKI, and the importance of maintaining adequate hemodynamics for renal perfusion does not diminish in the postoperative setting (6, 27, 32). In addition, recent studies have suggested that a cumulative venous congestion load (i.e., exposure higher CVP for longer lengths of time) is also predictive of CS-AKI (10). We believe our study offers more scrutiny of the postoperative renal load experienced by patients, and the how to better interpret the plethora of hemodynamic variables available to the intensive care team to guide high-quality care.
This study had several limitations. First, this study was a single-center retrospective cohort and as such requires validation in a prospective trial. Additionally, while the majority of intraoperative and postoperative care was protocolized, provider bias regarding individual patient management may still remain. We did not specifically standardize the duration of mechanical ventilation, which is a known confounder to right heart pressures. However, all patients received the same protocolized ventilator management, and neither CVP nor PAPi were strongly correlated with duration of mechanical ventilation (Table 2). We did not specifically examine the role of other confounders such as administration of nephrotoxic agents, inotropic use, hypotension, metabolic acidosis, or diuresis. These potential confounders would certainly affect renal risk and thus, our findings should be considered exploratory until a prospective trial can better elucidate the associations between PAPi, CVP, and renal dysfunction. Due to the large quantity of data obtained (>63,000 individual datapoints for the final analysis cohort), it is hopeful that the impact of such rare confounders is negligible. Additionally, there may have been competitive risk between our outcomes, in particular renal injury and mortality, which did the study did not specifically control for. However, 97% of patients (n = 26) who experienced in-house mortality also experienced renal injury based KDIGO criteria, which likely mitigated this risk within our study cohort.
In conclusion, the importance of identifying which patients will suffer from CS-AKI is imperative to prevent and to allow for early management of this comorbid condition that increases postoperative mortality. The current study demonstrates that both lower PAPi and higher CVP values are both associated with the development of CS-AKI. When changes in values are standardized however, a per-unit increase in CVP was more closely associated with development of CS-AKI when compared with lower per-unit decrease in PAPi. This is likely due to CS-AKI being more closely related to venous congestion, regardless of etiology, than to pulmonary factors affecting right ventricular function. Higher CVP was also independently associated with need for postoperative renal replacement therapy. Further investigation is needed to identify if the less invasively obtained value of CVP is a more important predictor for CS-AKI than PAPi.
Data availability statement
The raw data supporting the conclusions of this article will be made available by the authors, without undue reservation.
Author contributions
JW and BF: study design and manuscript editing. JW, AH, and VL: data collection. JW and NN: data analysis. JW, AH, NN, and BF: manuscript drafting. All authors contributed to the article and approved the submitted version.
Conflict of interest
The authors declare that the research was conducted in the absence of any commercial or financial relationships that could be construed as a potential conflict of interest.
Publisher's note
All claims expressed in this article are solely those of the authors and do not necessarily represent those of their affiliated organizations, or those of the publisher, the editors and the reviewers. Any product that may be evaluated in this article, or claim that may be made by its manufacturer, is not guaranteed or endorsed by the publisher.
Supplementary material
The Supplementary Material for this article can be found online at: https://www.frontiersin.org/articles/10.3389/fcvm.2022.967596/full#supplementary-material
References
1. Billings F. Acute kidney injury following cardiac surgery: a clinical model. Nephron. (2019) 143:202–6. doi: 10.1159/000501559
2. Chew STH, Hwang NC. Acute kidney injury after cardiac surgery: a narrative review of the literature. J Cardiothorac Vasc Anesth. (2019) 33:1122–38. doi: 10.1053/j.jvca.2018.08.003
3. Coulson T, Bailey M, Pilcher D, Reid CM, Seevanayagam S, Williams-Spence J, et al. Predicting acute kidney injury after cardiac surgery using a simpler model. J Cardiothorac Vasc Anesth. (2021) 35:866–73. doi: 10.1053/j.jvca.2020.06.072
4. Gaffney AM, Sladen RN. Acute kidney injury in cardiac surgery. Curr Opin Anaesthesiol. (2015) 28:50–9. doi: 10.1097/ACO.0000000000000154
5. Chen X, Wang X, Honore PM, Spapen HD, Liu D. Renal failure in critically ill patients, beware of applying (central venous) pressure on the kidney. Ann Intensive Care. (2018) 8:91. doi: 10.1186/s13613-018-0439-x
6. Gambardella I, Gaudino M, Ronco C, Lau C, Ivascu N, Girardi LN. Congestive kidney failure in cardiac surgery: the relationship between central venous pressure and acute kidney injury. Interact Cardiovasc Thorac Surg. (2016) 23:800–5. doi: 10.1093/icvts/ivw229
7. Rong LQ, Rahouma M, Neuburger PJ, Arguelles G, Emerson J, Mauer E, et al. Use of pulmonary artery pulsatility index in cardiac surgery. J Cardiothorac Vasc Anesth. (2020) 34:1220–5. doi: 10.1053/j.jvca.2019.09.023
8. Damman K, van Deursen VM, Navis G, Voors AA, van Veldhuisen DJ, Hillege HL. Increased central venous pressure is associated with impaired renal function and mortality in a broad spectrum of patients with cardiovascular disease. J Am Coll Cardiol. (2009) 53:582–8. doi: 10.1016/j.jacc.2008.08.080
9. Hanberg JS, Sury K, Wilson FP, Brisco MA, Ahmad T, Ter Maaten JM, et al. Reduced cardiac index is not the dominant driver of renal dysfunction in heart failure. J Am Coll Cardiol. (2016) 67:2199–208. doi: 10.1016/j.jacc.2016.02.058
10. Lopez MG, Shotwell MS, Morse J, Liang Y, Wanderer JP, Absi TS, et al. Intraoperative venous congestion and acute kidney injury in cardiac surgery: an observational cohort study. Br J Anaesth. (2021) 126:599–607. doi: 10.1016/j.bja.2020.12.028
11. Marik PE, Cavallazzi R. Does the central venous pressure predict fluid responsiveness? An updated meta-analysis and a plea for some common sense. Crit Care Med. (2013) 41:1774–81. doi: 10.1097/CCM.0b013e31828a25fd
12. McCoy IE, Montez-Rath ME, Chertow GM, Chang TI. Central venous pressure and the risk of diuretic-associated acute kidney injury in patients after cardiac surgery. Am Heart J. (2020) 221:67–73. doi: 10.1016/j.ahj.2019.12.013
13. Xu J, Jiang W, Li Y, Shen B, Shen Z, Wang Y, et al. Volume-associated hemodynamic variables for prediction of cardiac surgery-associated acute kidney injury. Clin Exp Nephrol. (2020) 24:798–805. doi: 10.1007/s10157-020-01908-6
14. Kapur NK, Esposito ML, Bader Y, Morine KJ, Kiernan MS, Pham DT, et al. Mechanical circulatory support devices for acute right ventricular failure. Circulation. (2017) 136:314–26. doi: 10.1161/CIRCULATIONAHA.116.025290
15. Korabathina R, Heffernan KS, Paruchuri V, et al. The pulmonary artery pulsatility index identifies severe right ventricular dysfunction in acute inferior myocardial infarction. Catheter Cardiovasc Interv. (2012) 80:593–600. doi: 10.1002/ccd.23309
16. Lim HS, Gustafsson F. Pulmonary artery pulsatility index: physiological basis and clinical application. Eur J Heart Fail. (2020) 22:32–8. doi: 10.1002/ejhf.1679
17. Gudejko MD, Gebhardt BR, Zahedi F, Jain A, Breeze JL, Lawrence MR, et al. Intraoperative hemodynamic and echocardiographic measurements associated with severe right ventricular failure after left ventricular assist device implantation. Anesth Analg. (2019) 128:25–32. doi: 10.1213/ANE.0000000000003538
18. Kang G, Ha R, Banerjee D. Pulmonary artery pulsatility index predicts right ventricular failure after left ventricular assist device implantation. J Heart Lung Transplant. (2016) 35:67–73. doi: 10.1016/j.healun.2015.06.009
19. Morine KJ, Kiernan MS, Pham DT, Paruchuri V, Denofrio D, Kapur NK. Pulmonary artery pulsatility index is associated with right ventricular failure after left ventricular assist device surgery. J Card Fail. (2016) 22:110–6. doi: 10.1016/j.cardfail.2015.10.019
20. Guven G, Brankovic M, Constantinescu AA, Brugts JJ, Hesselink DA, Akin S, et al. Preoperative right heart hemodynamics predict postoperative acute kidney injury after heart transplantation. Intensive Care Med. (2018) 44:588–97. doi: 10.1007/s00134-018-5159-z
21. Afsar B, Ortiz A, Covic A, Solak Y, Goldsmith D, Kanbay M. Focus on renal congestion in heart failure. Clin Kidney J. (2016) 9:39–47. doi: 10.1093/ckj/sfv124
22. Flynn BC, He J, Richey M, Wirtz K, Daon E. Early extubation without increased adverse events in high-risk cardiac surgical patients. Ann Thorac Surg. (2019) 107:453–9. doi: 10.1016/j.athoracsur.2018.09.034
23. Khwaja A. KDIGO. clinical practice guidelines for acute kidney injury. Nephron Clin Pract. (2012) 120:c179–184. doi: 10.1159/000339789
24. Damman K, Navis G, Smilde TD, Voors AA, van der Bij W, van Veldhuisen DJ, et al. Decreased cardiac output, venous congestion and the association with renal impairment in patients with cardiac dysfunction. Eur J Heart Fail. (2007) 9:872–8. doi: 10.1016/j.ejheart.2007.05.010
25. Shin SR, Kim WH, Kim DJ, Shin IW, Sohn JT. Prediction and prevention of acute kidney injury after cardiac surgery. Biomed Res Int. (2016) 2016:2985148. doi: 10.1155/2016/2985148
26. Williams JB, Peterson ED, Wojdyla D, Harskamp R, Southerland KW, Ferguson TB, et al. Central venous pressure after coronary artery bypass surgery: does it predict postoperative mortality or renal failure? J Crit Care. (2014) 29:1006–10. doi: 10.1016/j.jcrc.2014.05.027
27. Yang Y, Ma J, Zhao L. High central venous pressure is associated with acute kidney injury and mortality in patients underwent cardiopulmonary bypass surgery. J Crit Care. (2018) 48:211–5. doi: 10.1016/j.jcrc.2018.08.034
28. Stein A, de Souza LV, Belettini CR, Menegazzo WR, Viégas JR, Costa Pereira EM, et al. Fluid overload and changes in serum creatinine after cardiac surgery: predictors of mortality and longer intensive care stay. A prospective cohort study. Crit Care. (2012) 16:R99. doi: 10.1186/cc11368
29. Wang N, Jiang L, Zhu B, Wen Y, Xi XM. Fluid balance and mortality in critically ill patients with acute kidney injury: a multicenter prospective epidemiological study. Crit Care. (2015) 19:371. doi: 10.1186/s13054-015-1085-4
30. De Backer D, Bakker J, Cecconi M, Hajjar L, Liu DW, Lobo S, et al. Alternatives to the swan-ganz catheter. Intensive Care Med. (2018) 44:730–41. doi: 10.1007/s00134-018-5187-8
31. Teboul JL, Saugel B, Cecconi M, De Backer D, Hofer CK, Monnet X, et al. Less invasive hemodynamic monitoring in critically ill patients. Intensive Care Med. (2016) 42:1350–9. doi: 10.1007/s00134-016-4375-7
32. Prowle JR, Forni LG, Bell M, Chew MS, Edwards M, Grams ME, et al. Postoperative acute kidney injury in adult non-cardiac surgery: joint consensus report of the acute disease quality initiative and perioperative quality initiative. Nat Rev Nephrol. (2021) 17:605–18. doi: 10.1038/s41581-021-00418-2
Keywords: AKI, CVP, PAPi, cardiac surgery, critical care
Citation: Wei J, Houchin A, Nazir N, Leonardo V and Flynn BC (2022) Comparing the associations of central venous pressure and pulmonary artery pulsatility index with postoperative renal injury. Front. Cardiovasc. Med. 9:967596. doi: 10.3389/fcvm.2022.967596
Received: 13 June 2022; Accepted: 27 September 2022;
Published: 12 October 2022.
Edited by:
Marc Vives, Hospital Universitari de Girona Doctor Josep Trueta, SpainReviewed by:
Lei Du, Sichuan University, ChinaMathieu Jozwiak, Centre Hospitalier Universitaire de Nice, France
Copyright © 2022 Wei, Houchin, Nazir, Leonardo and Flynn. This is an open-access article distributed under the terms of the Creative Commons Attribution License (CC BY). The use, distribution or reproduction in other forums is permitted, provided the original author(s) and the copyright owner(s) are credited and that the original publication in this journal is cited, in accordance with accepted academic practice. No use, distribution or reproduction is permitted which does not comply with these terms.
*Correspondence: Johnny Wei, jwei3@kumc.edu