Diabetic dyslipidemia impairs coronary collateral formation: An update
- 1Department of Cardiovascular Medicine, School of Medicine, Ruijin Hospital, Shanghai Jiao Tong University, Shanghai, China
- 2Shanghai Clinical Research Center for Interventional Medicine, Shanghai, China
- 3Institute of Cardiovascular Disease, Shanghai Jiao Tong University School of Medicine, Shanghai, China
Coronary collateralization is substantially impaired in patients with type 2 diabetes and occlusive coronary artery disease, which leads to aggravated myocardial ischemia and a more dismal prognosis. In a diabetic setting, altered serum lipid profiles and profound glycoxidative modification of lipoprotein particles induce endothelial dysfunction, blunt endothelial progenitor cell response, and severely hamper growth and maturation of collateral vessels. The impact of dyslipidemia and lipid-lowering treatments on coronary collateral formation has become a topic of heightened interest. In this review, we summarized the association of triglyceride-based integrative indexes, hypercholesterolemia, increased Lp(a) with its glycoxidative modification, as well as quantity and quality abnormalities of high-density lipoprotein with impaired collateral formation. We also analyzed the influence of innovative lipid-modifying strategies on coronary collateral development. Therefore, clinical management of diabetic dyslipidemia should take into account of its effect on coronary collateralization in patients with occlusive coronary artery disease.
Introduction
Type 2 diabetes mellitus (T2DM) is increasingly prevalent worldwide, and cardiovascular disease represents the leading cause of morbidity and mortality in patients with T2DM (1). A cluster of lipid metabolic abnormalities, collectively referred to as diabetic dyslipidemia, have been well established as a major risk factor for adverse cardiovascular outcomes in diabetic patients. The pattern of diabetic dyslipidemia consists of hypertriglyceridemia associated with increased triglyceride-rich lipoproteins and their remnants, decreased high-density lipoprotein cholesterol (HDL-C), and elevated low-density lipoprotein cholesterol (LDL-C) levels with predominance of small dense LDL-C (sdLDL-C). Meanwhile, hyperglycemia and chronic inflammation in diabetic conditions promote glycation and oxidative modification of lipoprotein particles, leading to changes in conformation and function, altered interaction with membrane receptors and downstream signaling, and switch of the phenotype toward a more atheroprone state (2).
Coronary collaterals have been recognized as an important compensatory mechanism in salvage of ischemic myocardium, preservation of left ventricular function, and improvement of prognosis for patients with obstructive coronary artery disease (CAD) (3–5). During the development of coronary collaterals, two distinct processes, arteriogenesis and angiogenesis, are involved. The former pertains to the remodeling of preexisting arterial vessels through anatomic increase in lumen area and wall thickness. The latter is defined as growth of new capillaries that stem from the budding of preexisting capillary vessels (6). These processes are finely tuned by a variety of biomechanical and biochemical factors, including perfusion pressure, wall shear stress, systematic hypoxia, oxidative stress, inflammatory response and endothelial function.
Numerous clinical observations reveal substantially impaired collateral circulation in occlusive CAD patients with diabetes. Given the generally more severe atherosclerotic lesions and microcirculation dysfunction in diabetic patients, poor collateralization may provide a significant add-on effect to aggravate myocardial ischemia and contribute to a more dismal prognosis (7). A couple of underlying mechanisms for the poor collateral formation in diabetic patients have been identified. Chronic hyperglycemia and the engagement of advanced glycation end-products with their receptors (AGE-RAGE axis) adversely affects collateral development by inhibiting vessel growth and maturation (8). On the other hand, disturbed lipid metabolism also plays a critical role and is regarded as a hallmark of impaired angiogenesis (9, 10). Currently, the impact of dyslipidemia and lipid-lowering treatments on coronary collateral formation has become a topic of heightened interest. This review is the first to summarize the recent literature, in combination with our study findings, to elucidate the association of different components of diabetic dyslipidemia with coronary collateralization and highlight their potential clinical implications in T2DM patients with CAD. The relevant clinical studies investigating the association between lipid profiles and coronary collateralization are summarized in Table 1.
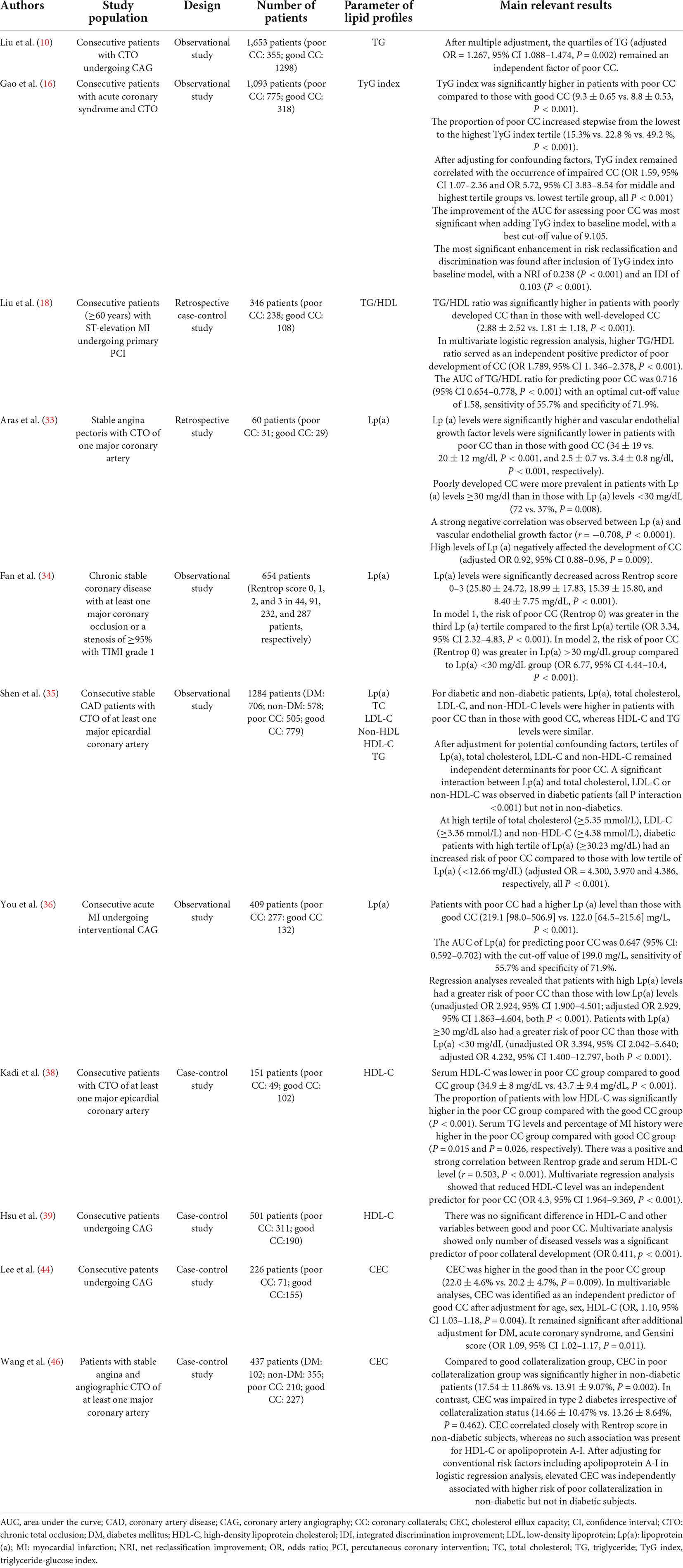
Table 1. Summary of clinical studies investigating the association between lipid profiles and coronary collateralization.
Impact of diabetic dyslipidemia on collateral formation
Hypertriglyceridemia
In patients with impaired glucose tolerance, blunt insulin sensitivity leads to compensatory hyperinsulinemia and increases secretion of triglyceride and triglyceride-rich lipoproteins. Hypertriglyceridemia confers an increased risk of CAD and adverse outcomes in patients with T2DM, by promoting release of excessive free fatty acids and stimulating production of proinflammatory cytokines, fibrinogen, and coagulation factors (11). Previous studies have shown that certain conditions with a cluster of risk components including hypertriglyceridemia (e.g., metabolic syndrome, overweight, or obesity) are more likely to be associated with endothelial dysfunction and reduced new vessel growth (10, 12), but the independent role of hypertriglyceridemia in coronary collateral formation remains difficult to be proven largely due to concomitant changes in other lipoproteins and relevant factors, particularly in patients with T2DM.
In recent years, several novel indexes by integrating triglyceride with some related metabolic measurements (such as HDL-C and glucose) have been proposed to better stratify the status of coronary collateralization. Triglyceride-glucose (TyG) index, calculated as log [fasting triglycerides (mg/dL) × fasting blood glucose (mg/dL)/2], has been suggested as a surrogate marker of insulin resistance (13). Elevated TyG index correlates well with high arterial stiffness and microvascular damage (14), which is associated with decreased coronary perfusion, reduced shear stress and arteriogenesis (15). In a large observational study, chronic total occlusion patients with poor coronary collaterals had higher TyG index compared to those with good collaterals. TyG was significantly associated with poor collateral formation even after adjusting for various confounders (16). Triglyceride to HDL-C ratio (TG/HDL-C ratio) and atherogenic index of plasma (logarithmic transformation of TG/HDL-C ratio) reflect the comprehensive situation of blood lipids and severity of insulin resistance (17). An observational study of elderly patients with acute myocardial infarction showed that an elevated TG/HDL ratio was independently associated with poor development of coronary collateral circulation (18). Of note, although a number of reports have suggested the prognostic role of atherogenic index of plasma beyond traditional risk factors (19, 20), further prospective studies are needed to examine if this index is applicable to predict coronary collateralization in type 2 diabetic patients with CAD.
Hypercholesterolemia
Chronic exposure to high levels of cholesterol and LDL-C results in functional and structural abnormalities of the vasculature, including endothelial dysfunction, subendothelial lipid deposition, plaque progression and compromised collateral vessel growth (21, 22). In diabetic dyslipidemia, hypercholesterolemia and a predominant increase in sdLDL particles play negative roles in coronary collateral formation.
Under hypercholesterolemia, angiogenesis and arteriogenesis in response to tissue hypoxia are markedly attenuated. Hypercholesterolemia decreases endothelial nitric oxide (NO) bioavailability and NO synthase (eNOS) expression and activity which are essential for endothelial progenitor cell (EPC) migration (23). In animal models, cholesterol at high concentration resulted in delayed native arteriolar growth caused by reduced early monocyte/macrophage influx and migration, and even mildly elevated cholesterol significantly decreased expression of fibroblast growth factor (FGF) receptor 1, vascular cell adhesion molecule-1, and macrophage scavenger receptor-1, mimicking relative changes in arteriogenesis and tissue perfusion (24). The extent of these alterations was related to the duration of hypercholesterolemia. In patients with hypercholesterolemia, the number and activity of circulating EPCs were decreased compared to normocholesterolemic subjects (25). Circulating EPCs have special cellular machinery that is resistant to various types of stress, which allow them to participate in tissue repair. Interestingly, hypercholesterolemia reduced arteriogenesis more dominantly than hyperglycemia or hyperinsulinemia (26).
The inhibitory effect of hypercholesterolemia on angiogenesis/arteriogenesis could be attributed to the negative effect of LDL-C on endothelial cell responsiveness to growth factors (25). T2DM is usually accompanied by oxidation or glycation of LDL, and glycoxidatively modified LDL poses more pro-atherogenic and antiangiogenic properties than native LDL (27). In addition, the predominance of sdLDL-C confers a threefold increased risk for CAD, owning to their greater propensity for endothelial penetration into arterial wall, lower binding affinity for LDL receptor, longer circulation time, and higher susceptibility to glycation, oxidative modification, and uptake by scavenger receptors (28). The prospective Framingham offspring study and large cohort studies suggest that sdLDL is superior to LDL-C and other biomarkers in predicting future cardiovascular events in stable CAD patients with T2DM or hypertriglyceridemia (29–31). Nevertheless, there is a paucity of clinical data regarding the impact of elevated LDL-C and sdLDL-C on collateral formation in T2DM patients with CAD.
Increased circulating lipoprotein(a)
Lipoprotein(a) is a genetically determined lipoprotein, which contains principally a cholesterol rich LDL particle, one molecule of apo B-100, and an apo (a). Noteworthy, Lp (a) is known to have atherothrombogenic property by inhibiting fibrinolysis system and promoting thrombus formation. In spite of a very skewed distribution, elevated circulating Lp(a) has emerged as an independent predictor of adverse outcomes for both general and higher risk populations, especially when LDL-C levels are elevated (32). In observational studies of patients with stable CAD, serum Lp(a) levels decreased stepwise across angiographic coronary collateral grade, and elevated Lp(a) predicted poor collateral development (33, 34). Intriguingly, a robust association between Lp(a) interactions with cholesterol-containing lipids and coronary collateral formation was suggested in patients with T2DM, which was non-linear and limited to high Lp(a) and LDL-C or non-HDL-C levels (35). At high tertiles of total cholesterol (≥5.35 mmol/L), LDL-C (≥3.36 mmol/L) and non-HDL-C (≥4.38 mmol/L), patients with high tertile of Lp(a) (≥30.23 mg/dL) had a significantly increased risk of poor collateralization compared with those with low tertile of Lp(a) (<12.66 mg/dL) (all P < 0.001). Furthermore, the additional inclusion of interaction of Lp(a) with total cholesterol, LDL-C and non-HDL-C provided better risk prediction of poor coronary collaterals. However, no interaction of Lp(a) with HDL-C and triglyceride on coronary collateralization was observed. In patients with acute myocardial infarction, increased Lp(a) in serum was closely correlated with poor coronary collaterals (36). Overexpression of Lp(a) in transgenic mice resulted in markedly reduced natural recovery of blood flow in hindlimb ischemia animal models in a dose-dependent manner. Lp(a) was found to stimulate the growth of vascular smooth muscle, which was reversed by intramuscular injection of hepatocyte growth factor (HGF) (37). Overall, these results highlight that Lp(a) may reflect coronary collateral status.
Lipoprotein(a) is highly concentrated in the arterial wall, carries cholesterol and binds oxidized phospholipids. Elevated circulating Lp(a) inhibits transforming growth factor-β activity and attenuates synthesis and/or release of vascular endothelial growth factor (VEGF) and decreases production of endothelium-derived NO, leading to impaired angiogenesis (33). Moreover, one of our ongoing studies indicates that circulating Lp(a) can also undergo glycation modification. As a characteristic protein of Lp(a), apo(a) is a large protein containing many kringle domains. Based on mass spectrometry results, the glycation modification sites of apo(a) are mainly concentrated on the kringle IV domain, whereas only a few glycation modification sites are distributed in other domains. Phenotypic experiments confirmed that glycated apo(a) and glycated apo(a)-kIV can consistently induce inflammatory factor expression and RAGE pathway activation. In a diabetic mouse model with hindlimb ischemia, intraperitoneal injection of glycated apo(a) and glycated apo(a)-kIV, respectively, resulted in a substantial inhibition of angiogenesis. Further studies have demonstrated that glycated apo(a) and glycated apo(a)-kIV promoted the expression of adhesion molecules, decreased the activities of eNOS and production of NO, and inhibited endothelial proliferation, migration, and tubular formation. Glycated apo(a) and glycated apo(a)-kIV induced endothelial dysfunction mainly through up-regulation of nuclear co-repressor NR0B1, which binds and inhibits the transcriptional activity of cardiovascular protective nuclear receptors such as LXR, NR4A1, and estrogen receptor.
Subnormal high-density lipoprotein cholesterol level and high-density lipoprotein dysfunction
Reduced HDL-C in serum is one of the typical manifestations of diabetic dyslipidemia. The relationship between serum levels of HDL-C and coronary collateral formation remains controversial. In patients with stable CAD, one study showed that decreased HDL-C levels predicted poor coronary collateralization (38), but such results were not replicated in other studies (35, 39). These observations support a notion that HDL functionality rather than quantity alone may more reliably reflect its overall properties and has a better clinical relevance (40).
High-density lipoprotein particle is composed of an outer layer of apolipoproteins and phospholipids, surrounding a core of esterified cholesterol, and has pluripotent effects. It primarily mediates reverse cholesterol transport by carrying cholesterol from peripheral tissues to the liver for metabolism and excretion. In addition to its antioxidative, anti-inflammatory, antithrombotic and anti-apoptotic features, HDL itself has proangiogenic properties and regulates ischemia-induced angiogenesis in multiple ways (41). Cholesterol efflux capacity of HDL (HDL-CEC) is essential in maintaining cholesterol balance in endothelial cells, and it regulates angiogenesis via modulation of lipid rafts and VEGF receptor (VEGFR)-2 signaling (42). Large cohort studies and meta-analysis indicated that elevated HDL-CEC was associated with favorable clinical outcomes independent of circulating HDL-C levels (43). A case-control study reported a higher HDL-CEC in chronic total occlusion patients with good coronary collaterals compared to those with poor collaterals, and high HDL-CEC predicted the presence of good coronary collaterals (44). The degree of coronary collateralization from the contra-lateral vessel (usually via connections of the epicardial surface or intraventricular septum) was often visually estimated using the Rentrop grading system (45): 0 = no visible filling of any collateral channel; 1 = filling of side branches of the artery to be perfused by collateral vessels without visualization of epicardial segment; 2 = partially filling of the epicardial artery by collateral vessels; 3 = complete filling of the epicardial artery by collateral vessels. Patients were categorized into poor (grade 0 or 1) or good (grade 2 or 3) coronary collateralization group. This angiographic assessment of coronary collaterals is routinely applied in clinical practice. Wang et al. found that HDL-CEC correlated closely with angiographic Rentrop collateral score in non-diabetic patients, whereas HDL-CEC was impaired in patients with T2DM irrespective of collateralization status. Furthermore, this finding is supported by in vitro experimental results, showing that although HDL isolated from non-diabetics with poor collaterals had significantly greater potential in promoting endothelial tubular formation in Matrigel compared to HDL isolated from those with good collateralization, the proangiogenic capacity of HDL isolated from diabetic patients was markedly impaired which was not influenced by collateral conditions (46). These results imply that well-functioning HDL is biologically cardioprotective, contributing to coronary collateral formation. Nevertheless, the functional capacity of HDL is severely compromised in type 2 diabetic patients with CAD.
Glycation and oxidative modification are key underlying mechanisms that lead to HDL dysfunction and transforms the lipoprotein into a proinflammatory protein under diabetic conditions (47). Shen et al found that relative intensity of glycation of apoA-I (a predominant protein moiety in HDL) correlated positively, while HDL-associated paraoxonase (PON) 1 and PON3 activities inversely, with the severity of coronary atherosclerosis (48), and was related to decreased lecithin: cholesterol acyl transferase (LCAT) activity and plaque progression in type 2 diabetic patients undergoing percutaneous coronary intervention (49). Similarly, abundance of apo A-IV glycation was also correlated with the presence and severity of CAD in patients with T2DM. Glycosylated apo A-IV induces pro-inflammatory response in vitro and increases the expression of tumor necrosis factor (TNF) -α and adhesion molecules by the nuclear receptor NR4A3, thereby promoting atherosclerosis in apo E-/- mice (50). Recently, the deleterious effects of apo A-I and apo A-IV glycation on vessel growth in diabetes were assessed. In a diabetic hindlimb ischemia mouse model, blood reperfusion was determined by laser Doppler perfusion imaging after treatment with intraperitoneal injection of glycated apo A-I or glycated apo A-IV, and the gastrocnemius and soleus muscles were collected for pathological analysis and molecular biology evaluation. The results showed that both glycated apo A-I and glycated apo A-IV induced inflammatory reactions in endothelial cells and decreased new vessel growth. Further mechanistical studies revealed that glycated apo A-I skewed macrophage polarization toward M1 phenotype by activating SHP2, whereas glycated apo A-IV down-regulated cardiovascular protective nuclear receptor NR4A1 expression (51), all of which are recognized as important steps to the inhibition of angiogenesis. These findings point to a notion that HDL dysfunction and subnormal HDL-C levels act synergistically to decrease collateral formation in T2DM patients with CAD.
Clinical relevance
Since diabetic dyslipidemia hampers collateral vessel growth through inhibiting angiogenesis/arteriogenesis in its specific manner and coronary collateralization is of important clinical significance, the choice between the available management options for T2DM patients with CAD should account for its effect on collateral formation (Figure 1).
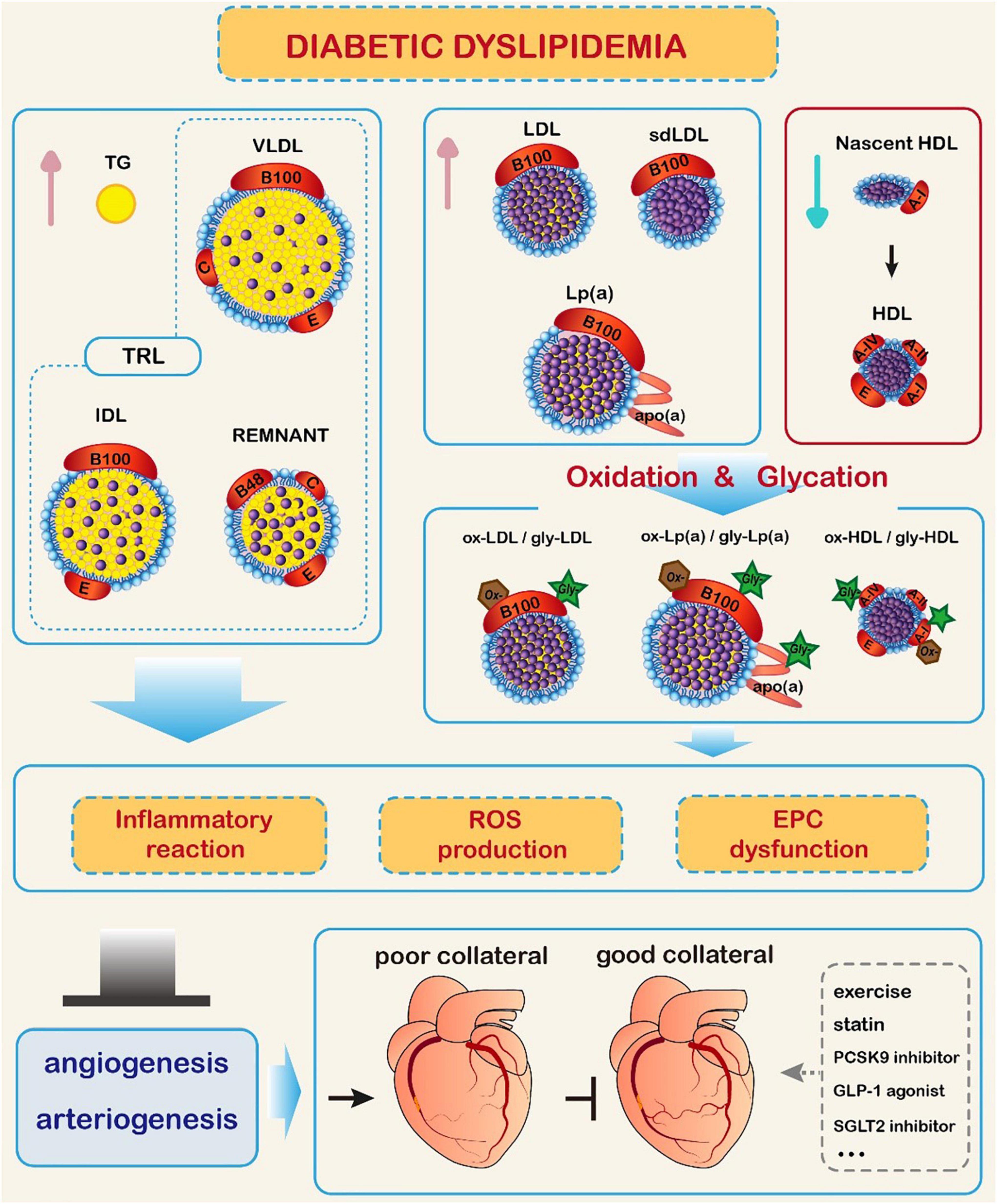
Figure 1. Impact of diabetic dyslipidemia on coronary collateral formation. In diabetic conditions, triglyceride (TG) and TG-rich lipoproteins (TRL) levels are increased, high-density lipoprotein cholesterol (HDL-C) level is reduced, and low-density lipoprotein cholesterol (LDL-C) level is elevated with predominance of small dense LDL-C (sdLDL-C). Meanwhile, glycation and oxidative modification of lipoprotein particles occur, promoting inflammatory reaction, production of reactive oxide species (ROS), and endothelial progenitor cell (EPC) dysfunction. These changes hamper collateral formation through inhibiting the process of angiogenesis and arteriogenesis. Exercise, lipid-lowering therapy, and antidiabetic agents may improve coronary collateral formation. Represents cholesterol esters. GLP-1: glucagon-like peptide-1; IDL: intermediate density lipoprotein; Lp(a): lipoprotein (a); PCSK9: proprotein convertase subtilisin/kexin type 9; SGLT2: sodium-glucose cotransporter 2; VLDL: very low-density lipoprotein.
In terms of non-pharmacological intervention, intensive lifestyle modification (including living habitat change, exercise, and diet) exerts beneficial impact on homeostasis, lipid profiles and coronary collateral circulation (52), thus should be the main initial strategy. Cessation of cigarette smoking is proven to decrease inflammatory response, increase the number and function of EPCs, and improve VEGF activities, which are beneficial for new vessel growth (53). Regular physical exercise improves lipid profile (54), and augments myocardial oxygen demand and blood flow, acting as a driving force for arteriogenesis, which helps in coronary collateral formation in patients with stable CAD, exceeding the effect of any drug treatment (55). Similarly, optimal blood pressure control (especially diastolic blood pressure) is crucial in achieving maximal coronary collateral flow (56, 57). While dietary quality is important for overall health, the total daily caloric intake per se should be a key determinant of hyperlipidemia in which a hypocaloric plan is favorable for reducing overweight and improving lipid profile and insulin sensitivity (58).
Hypertriglyceridemia should be treated to eliminate residual cardiovascular risk. Fibrates, a putative agonist ligand for peroxisome proliferator activated receptor-alpha, reduce the secretion of very-low-density lipoprotein (VLDL)-triglyceride, enhance removal of LDL, and increase HDL-C levels (59). High-dose of omega-3 polyunsaturated fatty acids (PUFA), mainly eicosapentaenoic acid (EPA) and docosahexaenoic acid (DHA), is a worthwhile add-on treatment, especially in statin-treated patients with T2DM and CAD in whom triglyceride levels remain elevated (60). In addition, PUFA could attenuate inflammation, improve endothelial function, and decrease thrombus formation (61). The REDUCE-IT trial evaluated a highly purified EPA preparation (4 g/day) in patients with hypertriglyceridemia and high cardiovascular risk. The results were extraordinary, as there was a 25% relative reduction in cardiovascular events, total coronary revascularization as well as plaque burden (62). However, the STRENGTH trial failed to obtain similar favorable results by treatment with EPA/DHA preparation, and in contrast, it was associated with a slightly higher rate of atrial fibrillation (63). This discrepancy may be explained by different study design and various degrees of change in triglyceride-rich lipids as well as differential effects of EPA and DHA on membrane structure, inflammatory biomarkers, endothelial function, and tissue distribution (64). A recent study demonstrated a negative correlation between peri-coronary adipose tissue attenuation assessed by CT angiography and treatment with PUFA, suggesting a lower extent of coronary inflammation (65, 66). Adipokine C1q tumor-necrosis factor-related protein (CTRP) 1 has been shown to be involved in inflammatory reaction and disease development (67). Elevated circulating CTRP1 was associated with poor coronary collateralization in T2DM patients with stable angina pectoris. Notably, stimulation of EPCs with CTRP1 decreases both cord length and branch point number and VEGFR-2 levels (68). Whether the beneficial effect of fibrates alone or in combination with PUFA on collateral formation via affecting adipokines in T2DM patients with CAD merits further confirmation.
Cholesterol-lowering therapy is the mainstay in primary and secondary prevention of cardiovascular diseases (69, 70). Statins effectively decrease serum LDL-C and sdLDL-C while increasing HDL-C levels and reduce the susceptibility of apo B of LDL to undergo oxidation and glycation. They also display significant anti-inflammatory properties and improve endothelial function (so-called pleiotropic effects) (71). Robust evidence supports the fact that use of statins enhances angiogenesis as well as arteriogenesis independent of a cholesterol-lowering mechanism (72, 73). Collateral formation benefits from statin treatment in T2DM patients with CAD, due partly to reduced apoptosis and decreased release of soluble VEGFR-1 induced by proinflammatory cytokines (74, 75). Proprotein convertase subtilisin/kexin type 9 (PCSK9) inhibitors have been increasingly used in the management of dyslipidemia in individuals with T2DM (76, 77). The results of post-hoc subgroup analysis of randomized clinical trials indicated that alirocumab and evolocumab significantly reduced circulating LDL-C and Lp(a), and increased HDL-C, without affecting glycemic levels in patients with T2DM (78, 79). Current data concerning PCSK9 inhibitors on collateral formation are scarce. An in vivo study demonstrated a proangiogenic activity of evolocumab through promoting cell proliferation, migration, tubulogenesis, and VEGF secretion (80). Given their unambiguous lipid-lowering properties, such a specific role of PCSK9 inhibitors for neo-angiogenesis should be clinically attractive.
Several new hypoglycemic agents, such as glucagon-like peptide-1 (GLP-1) receptor agonists and sodium-glucose cotransporter-2 (SGLT2) inhibitors, have been shown to favorably affect lipoprotein metabolism (81, 82). Nevertheless, further studies are needed to examine if they can improve collateral formation especially for type 2 diabetic patients with CAD.
Conclusion
In type 2 diabetic patients with CAD, the role of hypertriglyceridemia in collateral formation is not clear likely due to the concomitant changes in other lipoproteins. Elevated circulating cholesterol and Lp(a) and their glycoxidative modification hamper the process of new vessel growth. Subnormal HDL-C levels and, more importantly, deficient HDL function may act synergistically to decrease collateral formation. The choice between the available management options should account for its effect on coronary collateralization. In the future, much more research needs to be done to focus on the benefits of innovative lipid-modifying strategies, including use of PCSK9 and new triglyceride- and Lp(a)-lowering treatment, anti-diabetic agents as well as therapeutic normalization of attenuated proangiogenic and antiatherogenic HDL function, in the improvement of coronary collateral formation and clinical outcome. Novel information as such should add new knowledge on coronary pathophysiology and provide useful guidance of patient care for clinicians.
Author contributions
YS and FD wrote the manuscript, substantially contributed to discussion of the content, and edited the manuscript. YD, XW, and YW researched data for the manuscript. RZ and LL substantially contributed to discussion of the content and reviewed the manuscript. WS reviewed the manuscript before submission. All authors read and approved the final manuscript.
Funding
This study was supported by the National Natural Science Foundation of China (81870179, 81870357, 81970362, 81970293, 82170417, and 82170423), China Postdoctoral Science Foundation (2018M640408), Shanghai Municipal Education Commission-Gaofeng Clinical Medicine Grant Support (20181801), Technology Transfer Project of Shanghai Jiao Tong University School of Medicine (ZT202103), Medico-engineering Research Project of Shanghai Jiao Tong University (YG2021ZD04), and Shanghai Clinical Research Center for Interventional Medicine (19MC1910300).
Conflict of interest
The authors declare that the research was conducted in the absence of any commercial or financial relationships that could be construed as a potential conflict of interest.
Publisher’s note
All claims expressed in this article are solely those of the authors and do not necessarily represent those of their affiliated organizations, or those of the publisher, the editors and the reviewers. Any product that may be evaluated in this article, or claim that may be made by its manufacturer, is not guaranteed or endorsed by the publisher.
Abbreviations
AGE, advanced glycation end product; Apo, apolipoprotein; CAD, coronary artery disease; CEC, cholesterol efflux capacity; CETP, cholesterol ester transfer protein; CTRP, C1q tumor necrosis factor related protein; DPP4, dipeptidyl peptidase-4; eNOS, endothelial nitric oxide synthase; FGF, fibroblast growth factor; GLP-1, glucagon-like peptide-1; HDL, high-density lipoprotein; HDL-C, HDL cholesterol; HGF, hepatocyte growth factor; HIF, hypoxia-inducible factor; LCAT, lecithin cholesterol acyl transferase; LDL, low-density lipoprotein; LDL-C, low-density lipoprotein cholesterol; Lp(a), lipoprotein(a); MCP, monocyte chemotactic protein; NO, nitric oxide; oxLDL-C, oxidized low-density lipoprotein cholesterol; PCSK9, proprotein convertase subtilisin/kexin type 9; PON, paraoxonase; PUFA, polyunsaturated fatty acid; RAGE, receptor for advanced glycation end product; ROS, reactive oxide spices; sdLDL-C, small dense low-density lipoprotein cholesterol; SGLT2, sodium-glucose co-transporter-2; T2DM, type 2 diabetes mellitus; TG/HDL-C, triglyceride/high-density lipoprotein cholesterol; TRL, triglyceride-rich lipoprotein; TyG index, triglyceride-glucose index; VEGF, vascular endothelial growth factor; VEGFR, VEGF receptor; VLDL, very low density lipoprotein.
References
1. Einarson TR, Acs A, Ludwig C, Panton UH. Economic burden of cardiovascular disease in type 2 diabetes: a systematic review. Value Health. (2018) 21:881–90. doi: 10.1016/j.jval.2017.12.019
2. Bahiru E, Hsiao R, Phillipson D, Watson KE. Mechanisms and treatment of dyslipidemia in diabetes. Curr Cardiol Rep. (2021) 23:26. doi: 10.1007/s11886-021-01455-w
3. Bigler MR, Seiler C. The human coronary collateral circulation, Its extracardiac anastomoses and their therapeutic promotion. Int J Mol Sci. (2019) 20:3726. doi: 10.3390/ijms20153726
4. Dai Y, Chang S, Wang S, Shen Y, Li C, Huang Z, et al. The preservation effect of coronary collateral circulation on left ventricular function in chronic total occlusion and its association with the expression of vascular endothelial growth factor A. Adv Clin Exp Med. (2020) 29:493–7. doi: 10.17219/acem/104535
5. Shen Y, Aihemaiti M, Shu XY, Yang CD, Chen JW, Dai Y, et al. Circulating chromogranin B is associated with left ventricular functional recovery after successful recanalization of chronic total occlusion. Front Cardiovasc Med. (2021) 8:756594. doi: 10.3389/fcvm.2021.756594
6. Zimarino M, D’Andreamatteo M, Waksman R, Epstein SE, De Caterina R. The dynamics of the coronary collateral circulation. Nat Rev Cardiol. (2014) 11:191–7. doi: 10.1038/nrcardio.2013.207
7. Yang ZK, Shen Y, Dai Y, Wang XQ, Hu J, Ding FH, et al. Impact of coronary collateralization on long-term clinical outcomes in type 2 diabetic patients after successful recanalization of chronic total occlusion. Cardiovasc Diabetol. (2020) 19:59. doi: 10.1186/s12933-020-01033-4
8. Shen Y, Ding FH, Dai Y, Wang XQ, Zhang RY, Lu L, et al. Reduced coronary collateralization in type 2 diabetic patients with chronic total occlusion. Cardiovasc Diabetol. (2018) 17:26. doi: 10.1186/s12933-018-0671-6
9. Balakrishnan S, Senthil Kumar B. Factors causing variability in formation of coronary collaterals during coronary artery disease. Folia Morphol (Warsz). (2021). doi: 10.5603/FM.a2021.0110
10. Liu T, Wu Z, Liu J, Lv Y, Li W. Metabolic syndrome and its components reduce coronary collateralization in chronic total occlusion: an observational study. Cardiovasc Diabetol. (2021) 20:104. doi: 10.1186/s12933-021-01297-4
11. Packard CJ, Boren J, Taskinen MR. Causes and consequences of hypertriglyceridemia. Front Endocrinol (Lausanne). (2020) 11:252. doi: 10.3389/fendo.2020.00252
12. di Somma M, Vliora M, Grillo E, Castro B, Dakou E, Schaafsma W, et al. Role of VEGFs in metabolic disorders. Angiogenesis. (2020) 23:119–30. doi: 10.1007/s10456-019-09700-1
13. Liu XC, He GD, Lo K, Huang YQ, Feng YQ. The triglyceride-glucose index, an insulin resistance marker, was non-linear associated with all-cause and cardiovascular mortality in the general population. Front Cardiovasc Med. (2021) 7:628109. doi: 10.3389/fcvm.2020.628109
14. Guo W, Zhu W, Wu J, Li X, Lu J, Qin P, et al. Triglyceride glucose index is associated with arterial stiffness and 10-year cardiovascular disease risk in a chinese population. Front Cardiovasc Med. (2021) 8:585776. doi: 10.3389/fcvm.2021.585776
15. Baykan AO, Gür M, Acele A, Şeker T, Quisi A, Yildirim A, et al. Coronary collateral development and arterial stiffness in patients with chronic coronary total occlusions. Scand Cardiovasc J. (2015) 49:228–34. doi: 10.3109/14017431.2015.1062130
16. Gao A, Liu J, Hu C, Liu Y, Zhu Y, Han H, et al. Association between the triglyceride glucose index and coronary collateralization in coronary artery disease patients with chronic total occlusion lesions. Lipids Health Dis. (2021) 20:140. doi: 10.1186/s12944-021-01574-x
17. Ouchi G, Komiya I, Taira S, Wakugami T, Ohya Y. Triglyceride/low-density-lipoprotein cholesterol ratio is the most valuable predictor for increased small, dense LDL in type 2 diabetes patients. Lipids Health Dis. (2022) 21:4. doi: 10.1186/s12944-021-01612-8
18. Liu GY, Meng XX, Zhang Z. Triglyceride to HDL-cholesterol ratio as an independent risk factor for the poor development of coronary collateral circulation in elderly patients with ST-segment elevation myocardial infarction and acute total occlusion. Medicine (Baltimore). (2018) 97:e12587. doi: 10.1097/MD.0000000000012587
19. Fu L, Zhou Y, Sun J, Zhu Z, Xing Z, Zhou S, et al. Atherogenic index of plasma is associated with major adverse cardiovascular events in patients with type 2 diabetes mellitus. Cardiovasc Diabetol. (2021) 20:201. doi: 10.1186/s12933-021-01393-5
20. Wu J, Zhou Q, Wei Z, Wei J, Cui M. Atherogenic index of plasma and coronary artery disease in the adult population: a meta-analysis. Front Cardiovasc Med. (2021) 8:817441. doi: 10.3389/fcvm.2021.817441
21. Bogachkov YY, Chen L, Le Master E, Fancher IS, Zhao Y, Aguilar V, et al. LDL induces cholesterol loading and inhibits endothelial proliferation and angiogenesis in Matrigels: correlation with impaired angiogenesis during wound healing. Am J Physiol Cell Physiol. (2020) 318:C762–76. doi: 10.1152/ajpcell.00495.2018
22. Deindl E, Quax PH. Arteriogenesis and therapeutic angiogenesis in its multiple aspects. Cells. (2020) 9:1439. doi: 10.3390/cells9061439
23. Duan J, Murohara T, Ikeda H, Katoh A, Shintani S, Sasaki K, et al. Hypercholesterolemia inhibits angiogenesis in response to hindlimb ischemia: nitric oxide-dependent mechanism. Circulation. (2000) 102(19 Suppl. 3):III370–6. doi: 10.1161/01.cir.102.suppl_3.iii-370
24. Tirziu D, Moodie KL, Zhuang ZW, Singer K, Helisch A, Dunn JF, et al. Delayed arteriogenesis in hypercholesterolemic Mice. Circulation. (2005) 112:2501–9. doi: 10.1161/CIRCULATIONAHA.105.542829
25. Luo Y, Yan QN, Wu WZ, Luo FY. Decreased count and dysfunction of circulating EPCs in postmenopausal hypercholesterolemic females via reducing NO production. Stem Cells Int. (2018) 2018:2543847. doi: 10.1155/2018/2543847
26. van Weel V, de Vries M, Voshol PJ, Verloop RE, Eilers PHC, van Hinsbergh VWM, et al. Hypercholesterolemia reduces collateral artery growth more dominantly than hyperglycemia or insulin resistance in mice. Arterioscler Thromb Vasc Biol. (2006) 26:1383–90. doi: 10.1161/01.ATV.0000219234.78165.85
27. Lin FY, Tsao NW, Shih CM, Lin YW, Yeh JS, Chen JW, et al. The biphasic effects of oxidized-low density lipoprotein on the vasculogenic function of endothelial progenitor cells. PLoS One. (2015) 10:e0123971. doi: 10.1371/journal.pone.0123971
28. Jin X, Yang S, Lu J, Wu M. Small, dense low-density lipoprotein-cholesterol and atherosclerosis: relationship and therapeutic strategies. Front Cardiovasc Med. (2022) 8:804214. doi: 10.3389/fcvm.2021.804214
29. Ikezaki H, Lim E, Cupples LA, Liu CT, Asztalos BF, Schaefer EJ. Small dense low-density lipoprotein cholesterol is the most atherogenic lipoprotein parameter in the prospective Framingham offspring study. J Am Heart Assoc. (2021) 10:e019140. doi: 10.1161/JAHA.120.019140
30. Jin JL, Zhang HW, Cao YX, Liu HH, Hua Q, Li YF, et al. Association of small dense low-density lipoprotein with cardiovascular outcome in patients with coronary artery disease and diabetes: a prospective, observational cohort study. Cardiovasc Diabetol. (2020) 19:45. doi: 10.1186/s12933-020-01015-6
31. Huang J, Gu JX, Bao HZ, Li SS, Yao XQ, Yang M, et al. Elevated serum small dense low-density lipoprotein cholesterol may increase the risk and severity of coronary heart disease and predict cardiovascular events in patients with type 2 diabetes mellitus. Dis Markers. (2021) 2021:5597028. doi: 10.1155/2021/5597028
32. Zhang Y, Jin JL, Cao YX, Zhang HW, Guo YL, Wu NQ, et al. Lipoprotein (a) predicts recurrent worse outcomes in type 2 diabetes mellitus patients with prior cardiovascular events: a prospective, observational cohort study. Cardiovasc Diabetol. (2020) 19:111. doi: 10.1186/s12933-020-01083-8
33. Aras D, Geyik B, Topaloglu S, Ergun K, Ayaz S, Maden O, et al. Serum level of lipoprotein (a) is inversely associated with the development of coronary collateral circulation. Coron Artery Dis. (2006) 17:159–63. doi: 10.1097/00019501-200603000-00010
34. Fan Y, Hu JS, Guo F, Lu ZB, Xia H. Lipoprotein(a) as a predictor of poor collateral circulation in patients with chronic stable coronary heart disease. Braz J Med Biol Res. (2017) 50:e5979. doi: 10.1590/1414-431X20175979
35. Shen Y, Chen S, Dai Y, Wang XQ, Zhang RY, Yang ZK, et al. Lipoprotein (a) interactions with cholesterol-containing lipids on angiographic coronary collateralization in type 2 diabetic patients with chronic total occlusion. Cardiovasc Diabetol. (2019) 18:82. doi: 10.1186/s12933-019-0888-z
36. You XD, Jin JL, Zhang H, Guo N, Hou BJ, Guo YL, et al. Lipoprotein(a) as a marker for predicting coronary collateral circulation in patients with acute myocardial infarction. Per Med. (2020) 17:67–78. doi: 10.2217/pme-2018-0127
37. Morishita R, Sakaki M, Yamamoto K, Iguchi S, Aoki M, Yamasaki K, et al. Impairment of collateral formation in lipoprotein(a) transgenic mice: therapeutic angiogenesis induced by human hepatocyte growth factor gene. Circulation. (2002) 105:1491–6. doi: 10.1161/01.cir.0000012146.07240.fd
38. Kadi H, Ozyurt H, Ceyhan K, Koc F, Celik A, Burucu T. The relationship between high-density lipoprotein cholesterol and coronary collateral circulation in patients with coronary artery disease. J Investig Med. (2012) 60:808–12. doi: 10.2310/JIM.0b013e31824e980c
39. Hsu PC, Su HM, Juo SH, Yen HW, Voon WC, Lai WT, et al. Influence of high-density lipoprotein cholesterol on coronary collateral formation in a population with significant coronary artery disease. BMC Res Notes. (2013) 20:105. doi: 10.1186/1756-0500-6-105
40. Soria-Florido MT, Schröder H, Grau M, Fitó M, Lassale C. High density lipoprotein functionality and cardiovascular events and mortality: a systematic review and meta-analysis. Atherosclerosis. (2020) 302:36–42. doi: 10.1016/j.atherosclerosis.2020.04.015
41. Rohatgi A, Westerterp M, von Eckardstein A, Remaley A, Rye KA. HDL in the 21st century: a multifunctional roadmap for future HDL research. Circulation. (2021) 143:2293–309. doi: 10.1161/CIRCULATIONAHA.120.044221
42. Ebtehaj S, Gruppen EG, Bakker SJL, Dullaart RPF, Tietge UJF. HDL (high-density lipoprotein) cholesterol efflux capacity is associated with incident cardiovascular disease in the general population. Arterioscler Thromb Vasc Biol. (2019) 39:1874–83. doi: 10.1161/ATVBAHA.119.312645
43. Lee JJ, Chi G, Fitzgerald C, Kazmi SHA, Kalayci A, Korjian S, et al. Cholesterol efflux capacity and its association with adverse cardiovascular events: a systematic review and meta-analysis. Front Cardiovasc Med. (2021) 8:774418. doi: 10.3389/fcvm.2021.774418
44. Lee S, Park JM, Ann SJ, Kang M, Cheon EJ, An DB, et al. Cholesterol efflux and collateral circulation in chronic total coronary occlusion: effect-circ study. J Am Heart Assoc. (2021) 10:e019060. doi: 10.1161/JAHA.120.019060
45. Rentrop KP, Cohen M, Blanke H, Phillips RA. Changes in collateral channel filling immediately after controlled coronary artery occlusion by an angioplasty balloon in human subjects. J Am Coll Cardiol. (1985) 5:587–92. doi: 10.1016/s0735-1097(85)80380-6
46. Wang XQ, Shen Y, Chen JW, Shu XY, Li C, Yang CD, et al. Relationship of HDL-associated cholesterol efflux capacity to coronary collateralization in diabetic and non-diabetic patients with stable angina and chronic total occlusion. Circulation (2019) 140(Suppl. 1):A10762.
47. Ertek S. High-density lipoprotein (HDL) dysfunction and the future of HDL. Curr Vasc Pharmacol. (2018) 16:490–8. doi: 10.2174/1570161115666171116164612
48. Shen Y, Ding FH, Sun JT, Pu LJ, Zhang RY, Zhang Q, et al. Association of elevated apo A-I glycation and reduced paraoxonase 1, 3 activity, and their interaction with angiographic severity of coronary artery disease in patients with type 2 diabetes. Cardiovasc Diabetol. (2015) 14:52. doi: 10.1186/s12933-015-0221-4
49. Pu LJ, Lu L, Zhang RY, Du R, Shen Y, Zhang Q, et al. Glycation of apoprotein A-I is associated with coronary artery plaque progression in type 2 diabetic patients. Diabetes Care. (2013) 36:1312–20. doi: 10.2337/dc12-1411
50. Dai Y, Shen Y, Li QR, Ding FH, Wang XQ, Liu HJ, et al. Association of apolipoprotein A-IV glycation with CAD in T2DM patients: glycated apolipoprotein A-IV induces atherogenesis. J Am Coll Cardiol. (2017) 70:2006–19. doi: 10.1016/j.jacc.2017.08.053
51. Zhao Y, Bruemmer D. NR4A orphan nuclear receptors: transcriptional regulators of gene expression in metabolism and vascular biology. Arterioscler Thromb Vasc Biol. (2010) 30:1535–41. doi: 10.1161/ATVBAHA.109.191163
52. Möbius-Winkler S, Uhlemann M, Adams V, Sandri M, Erbs S, Lenk K, et al. Coronary collateral growth induced by physical exercise: results of the impact of intensive exercise training on coronary collateral circulation in patients with stable coronary artery disease (EXCITE) trial. Circulation. (2016) 133:1438–48; discussion 1448. doi: 10.1161/CIRCULATIONAHA.115.016442
53. Kida N, Nishigaki A, Kakita-Kobayashi M, Tsubokura H, Hashimoto Y, Yoshida A, et al. Exposure to cigarette smoke affects endometrial maturation including angiogenesis and decidualization. Reprod Med Biol. (2021) 20:108–18. doi: 10.1002/rmb2.12360
54. Kolwicz SC Jr. An “exercise” in cardiac metabolism. Front Cardiovasc Med. (2018) 5:66. doi: 10.3389/fcvm.2018.00066
55. Nickolay T, Nichols S, Ingle L, Hoye A. Exercise training as a mediator for enhancing coronary collateral circulation: a review of the evidence. Curr Cardiol Rev. (2020) 16:212–20. doi: 10.2174/1573403X15666190819144336
56. Shen Y, Ding FH, Wu F, Lu L, Zhang RY, Zhang Q, et al. Association of blood pressure and coronary collateralization in type 2 diabetic and nondiabetic patients with stable angina and chronic total occlusion. J Hypertens. (2015) 33:621–6; discussion 626. doi: 10.1097/HJH.0000000000000455
57. Shen Y, Dai Y, Wang XQ, Zhang RY, Lu L, Ding FH, et al. Searching for optimal blood pressure targets in type 2 diabetic patients with coronary artery disease. Cardiovasc Diabetol. (2019) 18:160. doi: 10.1186/s12933-019-0959-1
58. Ikezaki H, Furusyo N, Yokota Y, Ai M, Asztalos BF, Murata M, et al. Small dense low-density lipoprotein cholesterol and carotid intimal medial thickness progression. J Atheroscler Thromb. (2020) 27:1108–22. doi: 10.5551/jat.54130
59. Ida S, Kaneko R, Murata K. Efficacy and safety of pemafibrate administration in patients with dyslipidemia: a systematic review and meta-analysis. Cardiovasc Diabetol. (2019) 18:38. doi: 10.1186/s12933-019-0845-x
60. Lazarte J, Hegele RA. Dyslipidemia management in adults with diabetes. Can J Diabetes. (2020) 44:53–60. doi: 10.1016/j.jcjd.2019.07.003
61. Bhatt DL, Steg PG, Miller M, Brinton EA, Jacobson TA, Ketchum SB, et al. Cardiovascular risk reduction with icosapent ethyl for hypertriglyceridemia. N Engl J Med. (2019) 380:11–22. doi: 10.1056/NEJMoa1812792
62. Sheikh O, Vande Hei AG, Battisha A, Hammad T, Pham S, Chilton R. Cardiovascular, electrophysiologic, and hematologic effects of omega-3 fatty acids beyond reducing hypertriglyceridemia: as it pertains to the recently published REDUCE-IT trial. Cardiovasc Diabetol. (2019) 18:84. doi: 10.1186/s12933-019-0887-0
63. Doi T, Langsted A, Nordestgaard BG. A possible explanation for the contrasting results of REDUCE-IT vs. STRENGTH: cohort study mimicking trial designs. Eur Heart J. (2021) 42:4807–17. doi: 10.1093/eurheartj/ehab555
64. Pirillo A, Catapano AL. Omega-3 for cardiovascular diseases: where do we stand after REDUCE-IT and STRENGTH? Circulation. (2021) 144:183–5. doi: 10.1161/CIRCULATIONAHA.121.053144
65. Bittner DO, Goeller M, Dey D, Zopf Y, Achenbach S, Marwan M. High levels of eicosapentaenoic acid are associated with lower peri-coronary adipose tissue attenuation as measured by coronary CTA. Atherosclerosis. (2021) 316:73–8. doi: 10.1016/j.atherosclerosis.2020.10.006
66. Chait A, den Hartigh LJ. Adipose tissue distribution, inflammation and its metabolic consequences, including diabetes and cardiovascular disease. Front Cardiovasc Med. (2020) 7:22. doi: 10.3389/fcvm.2020.00022
67. Lu L, Zhang RY, Wang XQ, Liu ZH, Shen Y, Ding FH, et al. C1q/TNF-related protein-1: an adipokine marking and promoting atherosclerosis. Eur Heart J. (2016) 37:1762–71. doi: 10.1093/eurheartj/ehv649
68. Shen Y, Lu L, Liu ZH, Wu F, Zhu JZ, Sun Z, et al. Increased serum level of CTRP1 is associated with low coronary collateralization in stable angina patients with chronic total occlusion. Int J Cardiol. (2014) 174:203–6. doi: 10.1016/j.ijcard.2014.03.205
69. Rottura M, Scondotto G, Barbieri MA, Sorbara EE, Nasso C, Marino S, et al. Management of high cardiovascular risk in diabetic patients: focus on low density lipoprotein cholesterol and appropriate drug use in general practice. Front Cardiovasc Med. (2021) 8:749686. doi: 10.3389/fcvm.2021.749686
70. Tokgozoglu L, Orringer C, Ginsberg HN, Catapono AL. The year in cardiovascular medicine 2021: dyslipidemia. Eur Heart J. (2022) 43:807–17. doi: 10.1093/eurheartj/ehab875
71. Jansen-Chaparro S, López-Carmona MD, Cobos-Palacios L, Sanz-Cánovas J, Bernal-López MR, Gómez-Huelgas R. Statins and peripheral arterial disease: a narrative review. Front Cardiovasc Med. (2021) 8:777016. doi: 10.3389/fcvm.2021.777016
72. Dehnavi S, Kiani A, Sadeghi M, Biregani AF, Banach M, Atkin SL. Targeting AMPK by statins: a potential therapeutic approach. Drugs. (2021) 81:923–33. doi: 10.1007/s40265-021-01510-4
73. Annex BH, Cooke JP. New directions in therapeutic angiogenesis and arteriogenesis in peripheral arterial disease. Circ Res. (2021) 128:1944–57. doi: 10.1161/CIRCRESAHA.121.318266
74. Dincer I, Ongun A, Turhan S, Ozdol C, Ertas F, Erol C. Effect of statin treatment on coronary collateral development in patients with diabetes mellitus. Am J Cardiol. (2006) 97:772–4. doi: 10.1016/j.amjcard.2005.09.124
75. Gupta M, Tummala R, Ghosh RK, Blumenthal C, Philip K, Bandyopadhyay D, et al. An update on pharmacotherapies in diabetic dyslipidemia. Prog Cardiovasc Dis. (2019) 62:334–41. doi: 10.1016/j.pcad.2019.07.006
76. Felekos I, Karamasis GV, Pavlidis AN. PCSK9 inhibitors for the management of dyslipidemia in people with type 2 diabetes: how low is too low? Curr Pharm Des. (2021) 27:1008–14. doi: 10.2174/1381612826666200617170252
77. Bardolia C, Amin NS, Turgeon J. Emerging nlon-statin treatment options for lowering low-density ipoprotein cholesterol. Front Cardiovasc Med. (2021) 8:789931. doi: 10.3389/fcvm.2021.789931
78. Ray KK, Del Prato S, Müller-Wieland D, Cariou B, Colhoun HM, Tinahones FJ, et al. Alirocumab therapy in individuals with type 2 diabetes mellitus and atherosclerotic cardiovascular disease: analysis of the ODYSSEY DM-DYSLIPIDEMIA and DM-INSULIN studies. Cardiovasc Diabetol. (2019) 18:149. doi: 10.1186/s12933-019-0951-9
79. Lorenzatti AJ, Monsalvo ML, López JAG, Wang H, Rosenson RS. Effects of evolocumab in individuals with type 2 diabetes with and without atherogenic dyslipidemia: an analysis from BANTING and BERSON. Cardiovasc Diabetol. (2021) 20:94. doi: 10.1186/s12933-021-01287-6
80. Safaeian L, Vaseghi G, Jabari H, Dana N. Evolocumab, a proprotein convertase subtilisin/kexin type 9 inhibitor, promotes angiogenesis in vitro. Can J Physiol Pharmacol. (2019) 97:352–8. doi: 10.1139/cjpp-2018-0542
81. Berberich AJ, Hegele RA. Lipid effects of glucagon-like peptide 1 receptor analogs. Curr Opin Lipidol. (2021) 32:191–9. doi: 10.1097/MOL.0000000000000750
Keywords: dyslipidemia, type 2 diabetes mellitus, coronary collateral circulation, coronary artery disease, lipid-lowering therapy
Citation: Shen Y, Wang XQ, Dai Y, Wang YX, Zhang RY, Lu L, Ding FH and Shen WF (2022) Diabetic dyslipidemia impairs coronary collateral formation: An update. Front. Cardiovasc. Med. 9:956086. doi: 10.3389/fcvm.2022.956086
Received: 29 May 2022; Accepted: 01 August 2022;
Published: 22 August 2022.
Edited by:
Tatsuya Sawamura, Shinshu University, JapanCopyright © 2022 Shen, Wang, Dai, Wang, Zhang, Lu, Ding and Shen. This is an open-access article distributed under the terms of the Creative Commons Attribution License (CC BY). The use, distribution or reproduction in other forums is permitted, provided the original author(s) and the copyright owner(s) are credited and that the original publication in this journal is cited, in accordance with accepted academic practice. No use, distribution or reproduction is permitted which does not comply with these terms.
*Correspondence: Feng Hua Ding, ruijindfh@126.com; Wei Feng Shen, rjshenweifeng@126.com
†These authors have contributed equally to this work