Remote Cardiac Rhythm Monitoring in the Era of Smart Wearables: Present Assets and Future Perspectives
- 1Department of Cardiology, Hygeia Group, Mitera Hospital, Athens, Greece
- 2Heart Sector, Hygeia Hospitals Group, HHG, Athens, Greece
- 3European Heart Agency, European Society of Cardiology, Brussels, Belgium
Remote monitoring and control of heart function are of primary importance for patient evaluation and management, especially in the modern era of precision medicine and personalized approach. Breaking technological developments have brought to the frontline a variety of smart wearable devices, such as smartwatches, chest patches/straps, or sensors integrated into clothing and footwear, which allow continuous and real-time recording of heart rate, facilitating the detection of cardiac arrhythmias. However, there is great diversity and significant differences in the type and quality of the information they provide, thus impairing their integration into daily clinical practice and the relevant familiarization of practicing physicians. This review will summarize the different types and dominant functions of cardiac smart wearables available in the market. Furthermore, we report the devices certified by official American and/or European authorities and the respective sources of evidence. Finally, we comment pertinent limitations and caveats as well as the potential answers that flow from the latest technological achievements and future perspectives.
Introduction
Heart rhythm disorders are dominant public health issues, affecting more than 2% of the adult population. Their incidence is comparable to that of other major cardiovascular diseases, such as stroke, acute myocardial infarction and non-ischemic cardiomyopathy (1, 2). Cardiac rhythm abnormalities significantly increase with advancing age (1), so that the gradual aging of the world population has led to a sharp rise in the prevalence of cardiac arrhythmias, a phenomenon that is expected to intensify in the upcoming decades (3).
Atrial fibrillation (AF) is the most common cardiac arrhythmia, affecting approximately 46.3 million people worldwide. According to recent studies, in 2017, 10 million Europeans were suffering from AF, while, in the United States the number of the patients is expected to rise from 6 to 16 million, by 2050 (4, 5). The lifetime risk of AF is estimated at about 35% for Caucasians and 20% for African Americans (6). Even though sometimes asymptomatic (7), the disease causes significant morbidity, as it increases up to five times the risk of stroke (8), accounting for approximately one-third of all ischemic strokes (9, 10). Nevertheless, early arrhythmia diagnosis and initiation of anticoagulation treatment can lead to 64% stroke reduction (11).
Other rhythm disturbances, such as conduction disorders, bradyarrhythmias, supraventricular and ventricular tachycardias, induce significant morbidity and mortality, with related socioeconomic impact. These types of arrhythmias often result in patient hospitalization, while affected patients may experience severe symptoms, such as fatigue or syncope, as well as life-threatening events, or even sudden cardiac death (1).
Based on the above, early detection of cardiac arrhythmias is of paramount importance, in order to improve patient management. Timely diagnosis allows the implementation of appropriate interventions, either pharmacological or interventional, in order to prevent adverse effects, reducing morbidity and mortality. In the recently issued guidelines for the management of AF, the European Society of Cardiology recommends opportunistic screening in individuals aged ≥65 years to detect asymptomatic AF (12). Traditional methods of arrhythmia screening, such as electrocardiography (ECG) and continuous ambulatory Holter monitoring are mainly hampered by the limited period of rhythm recordings. Consequently, these tools are not useful for the screening of asymptomatic patients and the detection of paroxysmal arrhythmias, such as AF. Implantable loop recorders have drawbacks as well, since their cost may hinder their implementation in certain healthcare systems. Moreover, adverse events, such as skin erosion, infections, device oversensing or undersensing can limit their effectiveness (13).
Recently, rapid technological advances have led to the development of wearables with built-in micro-detectors, that can provide real-time monitoring of the vital signs and heart rate. Such devices can detect cardiac arrhythmias (14, 15), with varying accuracy, that depends on device type and detection method (16, 17).
The purpose of this review is to provide thorough insights into “smart” wearables, capable of cardiac rhythm monitoring, presenting the latest data derived from major clinical trials. The devices certified by the United States Food and Drug Administration (FDA), or CE-marked by the European Union authorities, are summarized, contributing to a comprehensive perception of the existing knowledge. Finally, legitimate concerns, based on literature evidence and future perspectives, are discussed, highlighting the limitations that need to be addressed and the aspects of potential development during the following years.
Methods
The comprehensive review of the literature was achieved through screening of the Pubmed, Google Scholar and ClinicalTrials.gov databases from 1989 to January 2022, focusing mainly on articles published over the last decade. The searching procedure was based on several key terms regarding devices (“smart wearable devices” OR “smartwatches” OR “patches” OR “wristbands”) and heart conditions (“arrhythmia monitoring” OR “heart rhythm disorders” OR “cardiac diseases”), combined with Medical Subject Headings (MeSH). The first evaluation of the literature was based on the title and the abstract of each paper, while all the articles written in language other than English, or having included animal subjects, were dismissed. The reference section of the detected review articles was also probed and assessed, contributing to the overall selection of the literature. Finally, the Healthskouts Solutions Library for certified apps was used, as an additional source of certified smart wearable devices.
Wearable Devices to Monitor Heart Rate and Cardiac Rhythm
Technological advancements have allowed heart rate sensors to be incorporated into numerous commercially available wearables. The spectrum of these devices ranges from smart accessories to sensors embedded into clothing and shoes. Patches, in particular, are leadless, wearable devices, that are attached to the patient's chest and provide ambulatory ECG monitoring over several days to weeks (18–21). Fitness bands and smartwatches are wrist-worn devices, able to track heart rate in real time (22–26). Heart rate sensors have also been integrated into accessories, such as rings (27, 28), necklaces (29), earbud headphones (30–32), chest straps (31, 33–35), footwear (36), glasses (37), even into textiles (38). Table 1 summarizes the most commonly used, FDA certified or/and CE-marked wearables to monitor heart rate, while Table 2 presents the essential technical specifications of each device.
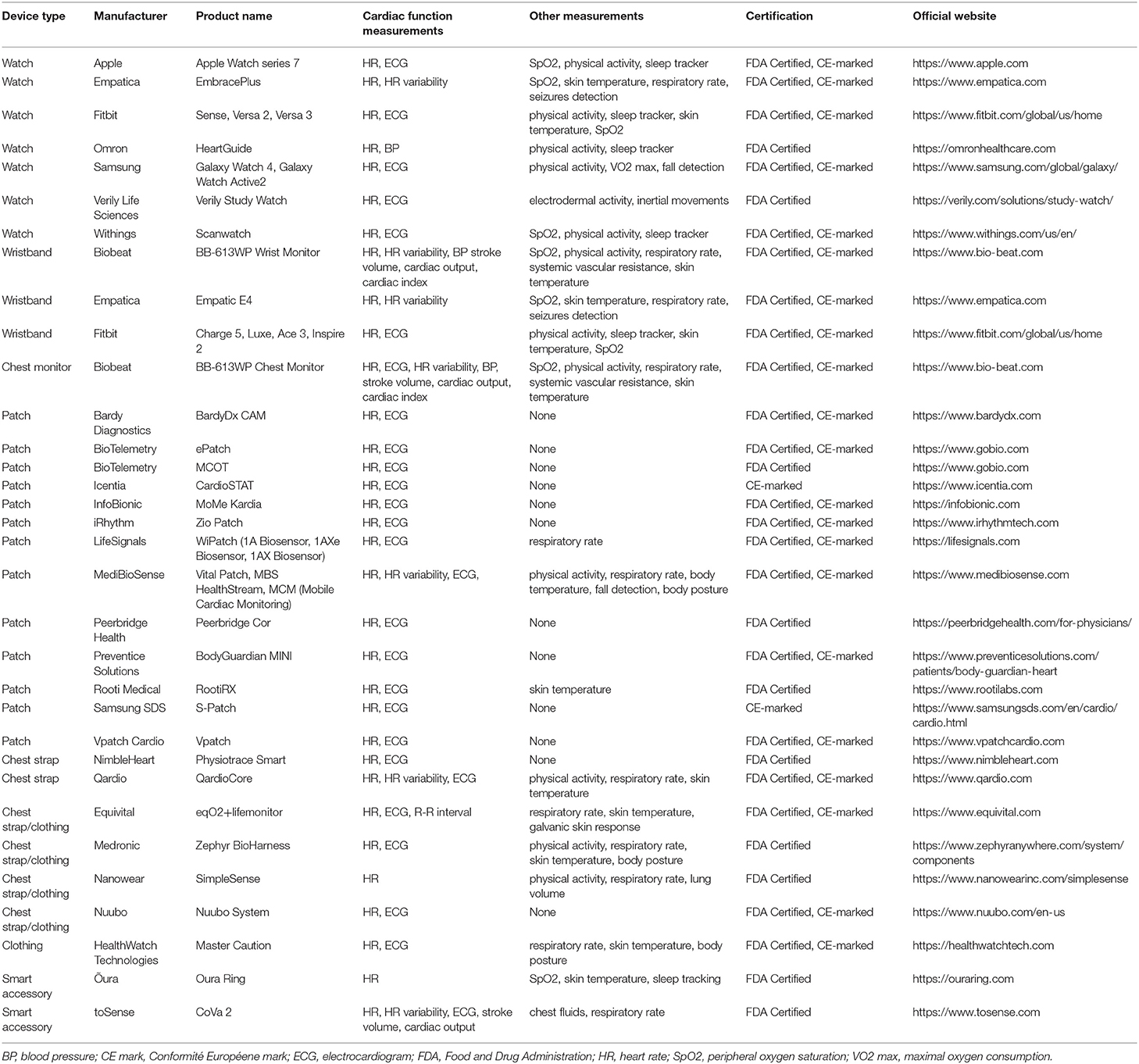
Table 1. Wearable devices for heart rate and rhythm monitoring, certified by FDA or CE marked by the European authorities.
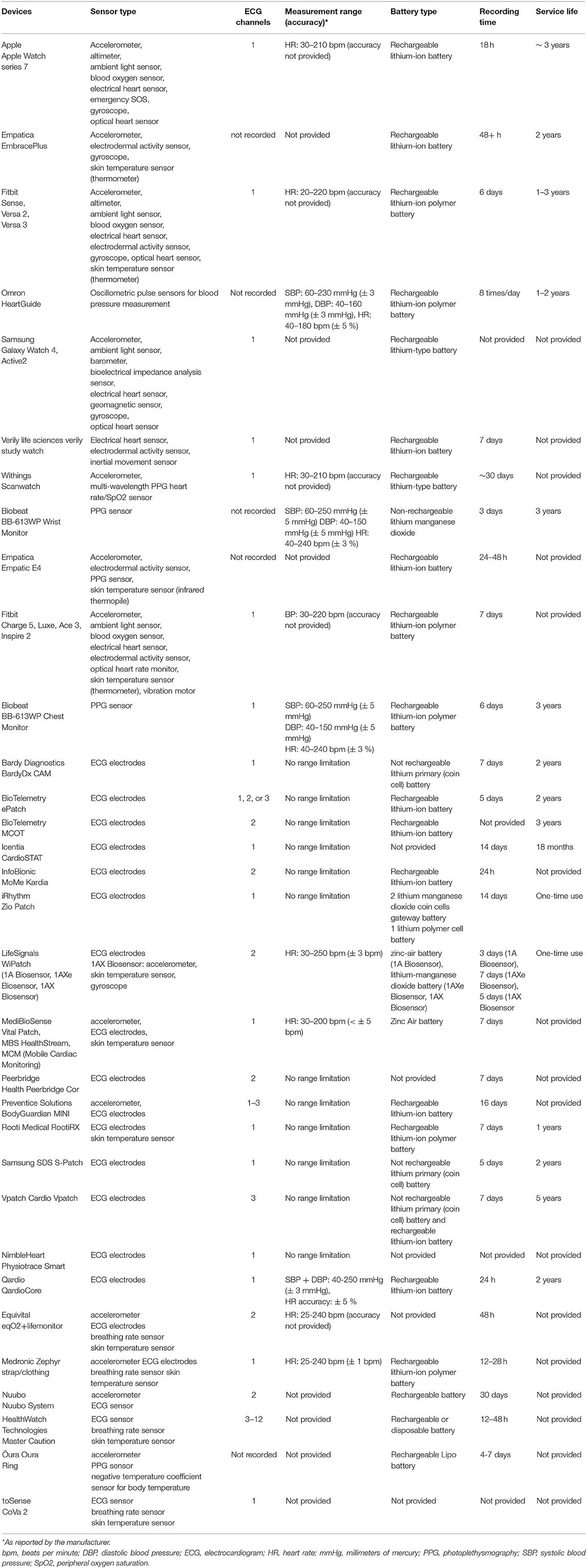
Table 2. Main technical specifications of the smart wearable devices listed in Table 1. Presented information is derived either from the official website of the respective company, or from the user guide document, detected through the search engine UserManual.wiki.
Wearable devices rely on photoplethysmography (PPG) or single lead ECG tracings to detect heart rate. PPG is a non-invasive, optical technique that detects beat-to-beat alterations in the skin capillary bed volume (39). It utilizes a light source, usually a light-emitting diode, to shine light on the skin and a photodetector to measure the intensity of the non-absorbed light. Green light is most frequently used to minimize motion artifacts (40). Light attenuation correlates with the beat-to-beat volume changes of the microvasculature, caused by the peripheral pulse, thus allowing for heart rate assessment (39–41). Specific algorithms can identify heart rhythm irregularities, such as AF, based on fluctuations of the beat-to-beat interval (42–44). PPG technology is widely incorporated into the majority of the wearables used to monitor heart rhythm. On the other hand, patches use leadless electrodes and a sensor to obtain a single lead ECG, when attached to the patient's skin (45). Captured ECG tracings are reviewed to detect heart rhythm disturbances, either by automated algorithms, or by physicians (44). Of note, certain wearables, such as the Apple Watch series 4, or later, provide heart rhythm monitoring via both a PPG sensor and a single lead ECG (46). The latter can be recorded by wearing the Apple Watch and holding a finger of the opposite hand on the digital crown, creating an electric circuit that correspond to lead I of the 12-lead ECG. Figure 1 shows the recording of sinus rhythm by both a smartwatch and a chest patch.
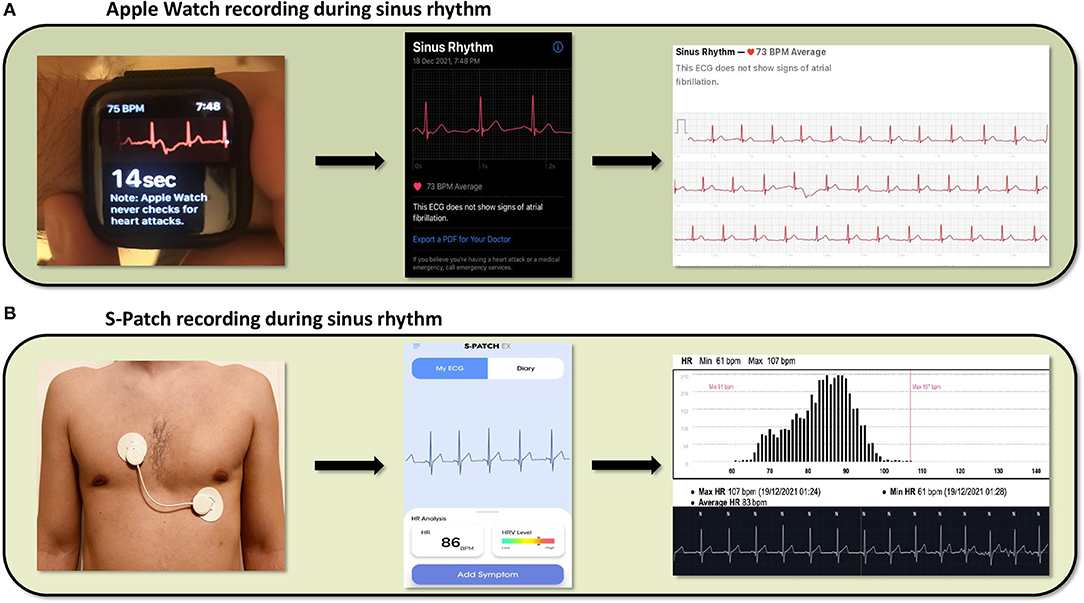
Figure 1. Cardiac rhythm recordings derived from a smartwatch (Apple Watch) and a patch (S-Patch), (A) From left to right, the images show the process of data collection from an Apple Watch, the ECG presentation in the respective smartphone application and the final report, featuring a possible diagnosis, (B) From left to right, the images show the same process captured by the wearable S-Patch.
The accuracy of the various wearables to monitor heart rate and detect cardiac arrhythmias is highly dependent on both the type of the device and the method of detection in use. In general, PPG-based heart rate measurements from wrist-worn devices show high agreement with those derived from simultaneous ECG tracings (34), although deviations have been reported in AF patients with high heart rates (47). Depending on the device model and the form of physical activity, the error in heart rate measurements by PPG ranges from 1.8 to 8.8% (24). Regarding AF detection, wearables using PPG signals are reported to have an accurary of 95–97% (48–50). In case of inconclusive readings, the diagnostic accuracy can be improved in devices providing ECG tracings, when the latter are interpreted by a trained physician (17, 44, 51). Patches show a consistently high accuracy in arrhythmia detection, comparable with that of ECG Holter monitors (52, 53).
Wearables for Detection of Atrial Fibrillation
Table 3 summarizes the clinical trials and studies, which were conducted to evaluate the heart rhythm monitoring-oriented features of several smart wearable devices, cited in Section Wearables for Detection of Atrial Fibrillation.
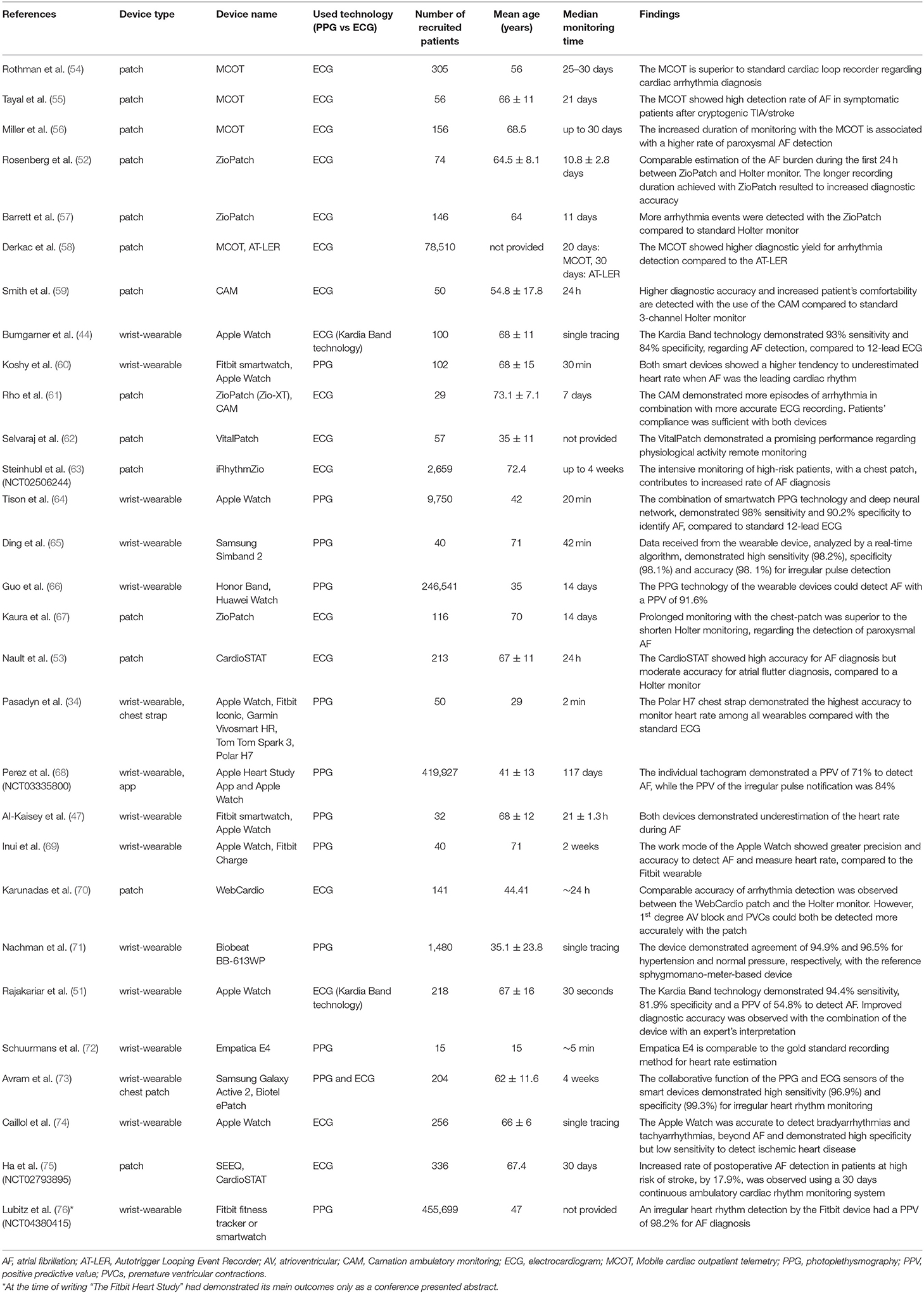
Table 3. Comprehensive presentation of clinical trials and studies, conducted to evaluate the function and cardiac features of the wearable smart devices.
Smartwatches/Wristbands
Smartwatches and wristbands are the most popular type of wearable devices, holding the dominant share of the global market, with a projected compound annual growth rate of 20% until 2026 (77). Inevitably, the wide spread of smartwatches that incorporate heart rhythm sensors among the population, raises queries about their role in AF screening and diagnosis (50), which is still controversial (78). At the same time, major studies are conducted, in order to define the accuracy of different smartwatch models to detect AF (50).
Apple Watch
The Apple Heart Study was one of the primary and most important studies regarding ambulatory ECG monitoring with the use of a wearable device (68). By recruiting 419,297 individuals without clinical history of AF, the authors examined the abnormalities of cardiac rhythm detected by the Apple Watch, in relation to AF detection, using an ECG patch, which was offered to those patients who received an irregular pulse notification from the device. Despite the limited number of the participants who finally returned the ECG patches and completed the study, the notification algorithm of the device had a positive predictive value of 84% to identify AF (95% confidence interval, 76% to 92%). In addition, a greater proportion of individuals older than 65 years was notified due to an irregular pulse, thus identifying a specific population group that could potentially benefit the most from AF screening with a smart wearable. Other studies with more restricted sample sizes have also assessed the accuracy of the Apple smartwatch to distinguish between AF and sinus rhythm, with comparable and promising results, regarding sensitivity and specificity of the embedded diagnostic algorithm (44, 64). However, physicians' involvement is necessary to provide an accurate diagnosis in unclassified recordings, which account for a significant proportion of the total tracings (51).
Fitbit Wearables
Recently, The Fitbit Heart Study (76) demonstrated a positive predictive value of 98% of the homonymous software algorithm to detect AF (79). The study enrolled more than 455,000 individuals, without history of AF, while an irregular heart rhythm was detected only in 4,728 (1%). The median population age was 47 years and people aged 65 years or older accounted for 12% of the total cohort. Among this elderly group of participants, the positive predictive value was also high (97%), encouraging the application of this technology to individuals older than 65 years of age, who usually have more comorbidities and are at greater risk of stroke.
Fitbit and Apple smartwatches have also been compared with the standard ECG, concerning the accuracy of the PPG technology to estimate heart rate. Two studies that included 102 and 32 participants, respectively, recorded a total of more than 91,000 heart rate values and showed that both devices underestimate heart rate during AF, especially when a rapid ventricular response of 100 beats per minute or faster occurs (47, 60). In addition, more accurate recordings were observed during night-time, when the physical activity is usually reduced, thus avoiding movement artifacts (47). In terms of direct comparison, the Apple Watch has a slightly superior diagnostic performance, compared to Fitbit smartwatches, closer to that of the gold standard ECG (34, 69).
Other Smartwatches/Wristbands
Many other companies have also launched smartwatches capable of heart rhythm detection, using either a PPG sensor or a combination of PPG and single-lead ECG. Two recent studies have shown high reliability of the AF detecting algorithms embedded into Samsung smart devices (65, 73). According to the findings, PPG sensor enhanced with a warning signal, prompting for an ECG recording, significantly increased the sensitivity for AF detection to 96.9% and the specificity to 99.3%. Thus, the high rate of specificity makes these wearables even more efficient for screening of the general population. Additionally, the algorithm was able to determine AF burden, a parameter associated with the risk of ischemic stroke and systemic cardioembolic events (73, 80). The Scanwatch by Withings, is a device able of recording 1-lead ECG tracings and is under evaluation in two ongoing clinical trials (NCT04493749, NCT04041466), regarding AF detection, compared with the standard 12-lead ECG. Finally, Huawei smart watches were used for the screening of patients included in the mobile Atrial Fibrillation II programme (mAFA-II programme), a two-phase trial, aiming to examine the optimization of AF screening and management through the integration of wearable PPG-based technologies (81). The early phase, called the Huawei Heart Study, assessed the effectiveness of smart wearables to detect AF, demonstrating a positive predictive value of 91.6% (66). The late phase, known as the mAFA II trial, investigated the value of a holistic care approach in AF patients, including the AF Better Care pathway (ABC pathway), combined with mobile smart technologies (82). However, these wearables have been certified only by the Chinese National Medical Products Administration.
Other wearables are able to track dominant factors of cardiovascular function, associated with arrhythmia initiation and development, even though they do not feature specific AF detection algorithms. The E4 wristband and EmbracePlus smartwatch by Empatica, in particular, assess the heart rate variability, a measure of the autonomic nervous systems related to AF development (83, 84). Schuurmans et al. validated the performance of Empatica E4 wristband in assessing heart rate variability, underlying the need for the user to remain still, in order to achieve accurate measurements (72). The HeartGuide smartwatch by Omron and the BB-613WP wristband by BioBeat Technologies feature blood pressure measurement, physical activity and sleep tracking, in addition to heart rate measurement (71, 85). Since the above are considered factors for AF development, these devices could potentially contribute to improved patient monitoring, individualized arrhythmia treatment and potentially reduction of AF burden (86).
Patches
Wearable ECG patch monitors are appealing for AF detection, given their potential to store ECG tracings for a longer time, compared to conventional 24-h ECG Holter monitors. In general, patches provide an attractive alternative to conventional ambulatory Holter ECG monitoring for AF detection. They are easy to use and apply (63), less cumbersome than a Holter monitor (53, 57) and they interfere less with everyday activities, due to their leadless nature. Patients have reported to find patches comfortable and to prefer them over traditional Holter monitors (57), resulting in higher compliance. They can be worn for several days, rendering them ideal for mid-term rhythm monitoring, which increases the diagnostic yield of AF (63, 67). Physicians, on the other hand, believe that patch monitors provide definite diagnosis more often than a Holter monitor (57).
ZioPatch
The ZioPatch (iRhythm Technologies, USA) is a leadless, adhesive cardiac monitor, that is placed on the anterior chest wall by a technician, or easily self-applied by the patient, to provide up to 14 days of continuous ECG monitoring (87). After the completion of the monitoring period, the device is mailed back to the data processing center for the captured tracings to be processed, using an FDA cleared algorithm to detect potential arrhythmic episodes. Trained technicians review and classify the detected arrhythmias to generate a report that is then reviewed by the ordering physician (18). In a study by Rosenberg et al., the ZioPatch detected all AF episodes recorded in a 24-h ECG Holter monitor and reported similar AF burden rates, in patients simultaneously wearing both devices (52). In the mSToPS trial, 2,659 individuals at high risk of AF were randomly assigned to an immediate, 4-month, monitoring period that featured a total of 4 weeks of ZioPatch application, or to delayed monitoring, comprising of 4 months of usual of care, before starting a 4-month monitoring period, using the ZioPatch for a total of 4 weeks (88). At the end of first 4-months, the incidence of newly diagnosed AF was 4 times higher in the immediate monitoring group, compared to those allocated to usual of care for the corresponding time period. Over a 1-year follow-up period, 6.7 new AF cases per 100 person-years were detected in the total population of actively monitored participants, compared to 2.6 new AF diagnosis per 100 person-years in a matched observational control group. Active monitoring was also associated with increased likelihood of anticoagulant and antiarrhythmic therapy initiation, cardioversion procedures, ablation and increased health care resources utilization (63). ZioPatch was also found to be superior to short-term ECG Holter monitoring in detecting AF in patients after an ischemic stroke or transient ischemic attack (67). It should be noted that time to first AF detection with the use of the ZioPatch (and long-term rhythm monitoring in general) is inversely proportional to patient's AF burden; the higher the arrhythmic burden, the shorter the time to AF detection will be (18). The median duration to first AF detection reported in the mSToPS trial was 2 days (63).
Carnation Ambulatory Monitor
The Carnation Ambulatory Monitor (CAM, BardyDx, USA) is an adhesive patch monitor that is placed along the sternum for optimized P-wave capture. Improved P-wave clarity is associated with more accurate rhythm identification, compared to standard Holter monitoring (59). In a small study comparing the Carnation Ambulatory Monitor with the ZioPatch, in patients undergoing cardiac rhythm monitoring with the two devices for 7 days, patients with AF episodes during the monitoring period were successfully identified by both patches (61).
Mobile Continuous Outpatient Telemetry
The Mobile Continuous Outpatient Telemetry (MCOT) (BioTelemetry Inc, USA) consists of a sensor, an adhesive patch and a monitor and can be used for medium-term heart rhythm monitoring. The sensor is attached on the patch, which is then placed on the patient's chest. Each patch lasts for approximately 5 days, before it is replaced by a new one, until the desired monitoring period is complete. The sensor captures a two-lead ECG tracing, using the patch embedded electrodes. Data are transmitted via Bluetooth to the monitor, which constantly analyses the ECG, using algorithms based on pre-specified criteria. If a heart rhythm disorder is detected, or the patient marks a symptom, the monitor instantly transmits the ECG tracing to the central monitoring station and the referring physician is notified (89). Thus, the device offers near continuous, real-time, heart rhythm monitoring, that is only interrupted for sensor recharging. The MCOT monitor is able of detecting AF (89) with a higher diagnostic yield, compared to loop event recorders, especially for asymptomatic AF episodes (54, 58). In studies assessing extended rhythm monitoring in patients with cryptogenic stroke, AF was diagnosed in 17–23% of the participants using the MCOT system, with the rates of AF detection constantly increasing within the monitoring period (55, 56). The device has also been studied in patients undergoing radiofrequency ablation for AF, to detect arrhythmia recurrences during the follow-up period (90). A non-telemetry version of this monitor, the ePatch (BioTelemetry Inc, USA), is also commercially available. The device can be worn for up to 14 days and needs to be sent back to the manufacturer for data acquisition and review (91).
CardioSTAT
The CardioSTAT (Icentia Inc, Canada) is a single-lead device, worn on the upper chest to provide up to 14 days of heart rhythm monitoring through a lead I–like electrode configuration. In a validation study by Nault et al., the agreement between CardioSTAT and Holter monitor readings on AF detection was very high (53). In patients following cardiac surgery, a high-risk population for arrhythmic events, post-operative AF detection was increased by more than ten times, when individuals were continuously monitored with the CardioSTAT patch, compared to those assigned to usual care (75).
WiPatch
The WiPatch sensors (LifeSignals Inc, USA) are worn on the upper left part of the chest and record a two-lead ECG, for up to 72 h. Data are automatically transmitted to a connected mobile device and then uploaded to a cloud server for storage and analysis. Similar AF detection rates were reported in ambulatory patients monitored for 24 h, simultaneously by both a Holter ECG and a WiPatch (70).
VitalPatch
The VitalPatch (MediBioSense Ltd, UK) is another peel-and-stick device, capable of real-time heart rate monitoring. The device consists of a patch and a relay device (either a tablet or a phone). A single-lead ECG tracing is continuously recorded by a biosensor embedded in the patch, which is worn on the upper-left chest. Acquired data are transmitted to the relay device, analyzed and then sent to a central workstation. Apart from heart rate and single-lead ECG, VitalPatch also records data regarding heart rate variability, respiratory rate, body temperature and body motion, while it can also detect falls, providing a holistic telemonitoring of physiological measurements and body activity (62). Recently, the device was updated with arrhythmia detection, including AF, but clinical data are still lacking.
Clothing and Accessories
The integration of heart rate sensors into textiles and accessories has led to the development of a wide variety of devices, that can track heart rate and/or record ECG. Accessories, such as rings (27, 28), headphones (30, 32), and footwear (36) are reported to be reliable in heart rate measurements, even though their accuracy can be compromised during high intensity exersice (31). Another monitoring system features a devices that resembles a necklace (CoVa monitoring system) (29), which, not only monitors heart rate and ECG in real-time, but also provides information regarding stroke volume, cardiac output and fluid status, providing an holistic hemodynamic assessement of heart failure patients, rather than merely cardiac rhythm monitoring. Chest straps, like the Zephyr BioHarness (33, 35, 92–94), the Polar H7 (30, 34, 95) and the EQ02 Lifemonitor (96, 97) provide a wide range of biomeasurments and are used mainly in athlete training, even though the Polar H7 chest strap has been utilized as an AF screening tool, with high accuracy (98). Sensors embedded in clothing, such as the Nuubo vest (99, 100) and the Master Caution shirt (101), allow for continuous ECG monitoring, fascilitating arrhythmia detection. In a study by Pagola et al., prolonged patient monitoring, using the Nuubo vest, after a cryptogenic stroke, led to the diagnosis of AF in 20% of the participants (102). In general, smart clothing and accessories reliably provide a wide variety of biomeasurements, including heart rate, but validation as diagnostic tools for arrhythmia detection is lacking for most of the products under this category.
Arrhythmias Other Than AF
Although all the large-scale studies conducted on the use of smartwatches as diagnostic and monitoring tools focus on AF, data in the literature demonstrate their potential contribution to the detection of arrhythmias other than AF (50). The spectrum of the heart rhythm disorders that can be detected with wearable devices is outlined in Table 4.
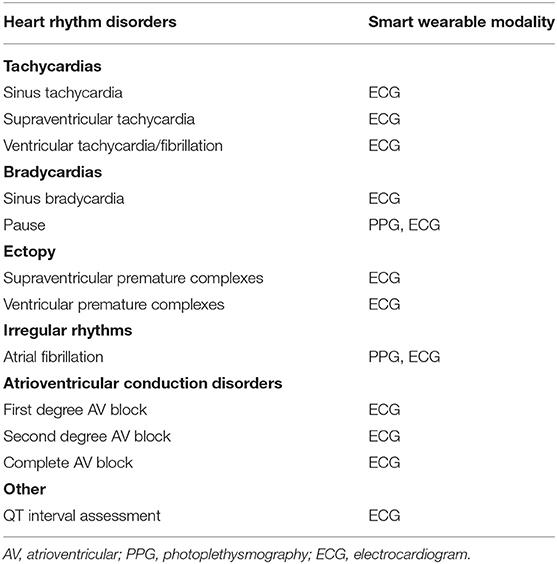
Table 4. Heart rhythm disorders identified by wearable devices and the respective modality used to detect them.
Smartwatches/Wristbands
Bradyarrhythmias and tachyarrhythmias can be identified with the use of smartwatches (74). The Apple Watch has been used in the detection of ventricular arrhythmias in two patients suffering from arrhythmogenic right ventricular cardiomyopathy and episodes of non-sustained ventricular tachycardia (VT), respectively, enabling physicians to correlate the reported symptoms with the underlying cause, which would otherwise remain undiagnosed (103). Captured single-lead ECG tracings of ventricular tachycardia in a patient with structural heart disease, or pre-excited AF with rapid ventricular response in an otherwise healthy young individual, using an Apple Watch, have also been reported (104). In patients wearing the Apple Watch, episodes of supraventricular tachycardia (SVT), such as atrioventricular reentrant and atrioventricular nodal reentry tachycardia have been recognized, as well (105, 106). Furthermore, researchers suggest an alternative use of these wrist wearables, by placing the sensor either at both upper extremities or at the abdomen and the chest in order to receive a more comprehensive recording that best resembles the standard 12-lead ECG. As a result, differential diagnosis between AF and atrial flutter could be facilitated. Furthermore, abnormalities associated with sudden cardiac arrest in young adults with ventricular pre-excitation, Brugada ECG pattern, arrhythmogenic right ventricular cardiomyopathy, hypertrophic cardiomyopathy or long-QT syndrome can also be detected (107, 108).
Patches
Apart from AF, patches that monitor heart rhythm can identify a series of other clinically significant arrhythmias, being a useful tool in the diagnostic work-up of patients with symptoms such as palpitations, syncope or presyncope. Supraventricular tachycardia was the most common rhythm disorder identified in a large cohort of individuals monitored with the ZioPatch (18, 52), as well as in patients with symptoms of arrhythmia discharged from the emergency department (109). Prolonged rhythm monitoring with wearables other than the ZioPatch is also associated with an increased rate of SVT diagnosis (55, 56, 70, 89). Patches providing clear identification of the P-wave facilitate further classification of the detected SVTs (61). Côté et al., have reported two cases of Wolff-Parkinson-White syndrome with intermittent pre-excitation, not present in 12-lead ECG, in children monitored with the CardioSTAT patch, who were complaining of palpitations (110).
Among participants actively monitored in the mSToPS trial, the detection of significant pauses and high degree atrioventricular block, along with runs of non-sustained VT, resulted in increased rates of pacemaker and implantable defibrillator implantations (63). In comparative studies, the MCOT patch was superior to a loop event recorder in detecting bradycardia, cardiac pauses, sustained or symptomatic SVT, asymptomatic high ventricular rates and runs of VT (54, 58). In patients undergoing transcatheter aortic valve replacement (TAVR), prolonged, patch-based, rhythm monitoring, prior to the procedure, can detect significant bradyarrhythmias in one-fifth of the patients, some of which may require a change of treatment (111). Following TAVR, Tian et al., identified patients with late high degree atrioventricular block, using the BodyGuardian patch (Preventice Solutions, Inc, USA) (112). The same patch was also found to reliably assess the QT interval, both in healthy individuals and long QT syndrome patients and could be used to remotely monitor patients in risk of QT interval prolongation and arrhythmias (113).
Limitations
Despite the variety of benefits associated with wearable technology, several limitations have to be overcome in order to establish their role as medical devices for cardiac rhythm monitoring and diagnosis, in everyday clinical practice. Figure 2 summarizes the main limitations and future perspectives, concerning the growing population of smart wearables.
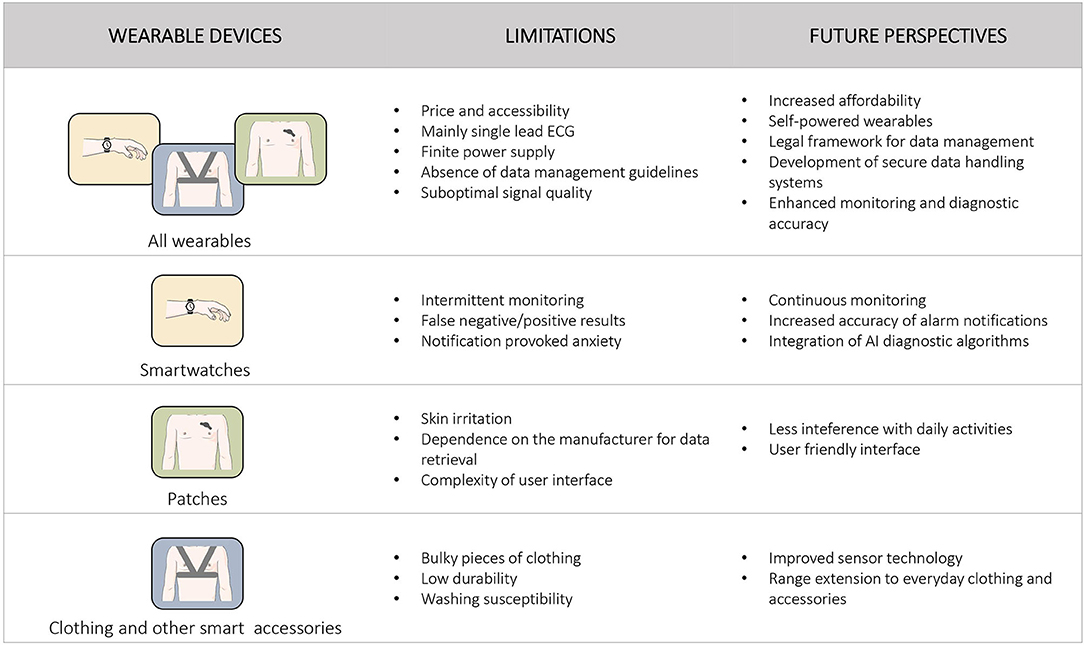
Figure 2. Main limitations and future perspectives of wearable devices for heart rhythm monitoring are presented. Specific information for each category of wearable devices is presented separately.
Smartwatches and wristbands are practical and able to track heart rhythm during every possible physical activity, using a semi-continuous PPG sensor, with or without intermittent ECG tracings, when available. Consequently, they lack the ability of continuous rhythm monitoring and require the cooperation of the user in order to achieve a reliable recording and capture the required data (12). Due to this operation mode, recording of paroxysmal arrhythmias may be missed, preventing accurate diagnosis and targeted management. In addition, the necessity for patient's alertness precludes monitoring during episodes with associated loss of consciousness, that could reveal malignant underlying arrhythmias, while the concomitant reduction of the peripheral blood pressure may weak the pulse signal, affecting the quality of the PPG signal (69). In view of this, in the WATCH AF trial, a high dropout rate of record files due to inadequate quality of the signal was observed, thus restricting the applicability of a smartwatch monitor in everyday practice (48). Smartwatches often demonstrate false positive results, occasionally leading to overdiagnosis and overtreatment of patients. At the same time, pathological signals and repeated notifications provoke intense anxiety to the user, often affecting both his physical and mental health (12).
On the other hand, wearable patches provide continuous rhythm recording during the application period, without requiring the active involvement of the user. Nonetheless, they manifest caveats that also restrict their applicability. Some patients may experience skin irritation, which is the most common adverse reaction of adhesive patch monitors (54, 63, 87). Noise recording could render the rhythm tracing uninterpretable, especially when single-lead patches are used (53). Another limitation is the fact that certain devices, such as the ZioPatch and the CardioSTAT, have to be mailed back to the manufacturer for data collection and analysis. This process could result in significant turnaround times between the end of the monitoring period and arrhythmia detection. Moreover, there is a clear dependance on the device company for data retrieval and analysis for most patch monitors, while the cost of using these devices is not negligible. Finally, user interface is quite complex for people who are not particularly familiar with handling novel technological platforms, excluding its utilization by elderly patients and non-familiarized physicians (114).
The integration of sensors into garments overcomes some of the drawbacks listed above, such as the need for the user to operate certain devices. On the other hand, incorporating electronics into fabric results in bulky, inflexible pieces of clothing. Moreover, the lifespan of smart clothing may be limited, affected by low durability and washing susceptibility.
Most of the wearables that capture ECG tracings provide data equivalent only to one of the 12 leads of the traditional ECG, thus limiting their value in detecting more complex arrhythmias and cardiac disorders (74). Furthermore, despite the significant progress observed in the field of lithium battery lifetime, power supply is still of finite duration, demanding repeated recharging and resulting to intervals of monitoring interruption (14). Besides that, the great abundance of sensitive data collected through these new technologies, require the development of strict policies to ensure safe storage, transparency, privacy and security of users' personal data (14, 115). Additionally, the crucial challenge of pricing and accessibility of smart wearables is still under discussion. Their consideration as medical devices establishes new standards that require the normalization of economic inequalities, in order to avoid health disparity. Moreover, the involvement of the public and private insurance systems in device reimbursement is unclear and is yet to be determined (14, 116).
Wearable devices have evidence-based credentials that establish their role as a screening tool for the detection of cardiac arrhythmias, especially AF, affecting decision making in patient pharmacological treatment. However, the benefit derived from the initiation of anticoagulation, based on AF diagnosis by wearable devices screening, has not been confirmed. In fact, despite the increased diagnostic rate of AF episodes, in high-risk individuals, in the STROKESTOP trial (117, 118) and the LOOP study (119), the LOOP study failed to show any added benefit, regarding the endpoint of stroke or systemic embolism, after initiation of preventive anticoagulation (119). These results imply that not all AF episodes in asymptomatic patients pose the same thromboembolic risk and underscore the need for further evaluation, in order to determine the exact role of AF screening and indicate specific AF characteristics and/or AF patient groups that derive potential benefit from oral anticoagulation initiation.
Future Perspectives
Undoubtedly, novel technologies bear the potential to shift the paradigm of disease diagnosis and patient management. The ideal wearable device for heart rhythm monitoring should be easy to use, even by the elderly and less familiarized patients, not interfere with daily activities and provide continuous and accurate real-time heart rhythm monitoring. Most commercially available wearables are capable of continuous monitoring for several days, providing they have sufficient power. Use of self-powered technology would, theoretically, allow for uninterrupted, extended use of cardiac monitoring devices (120). Wearables with integrated mobile network access could instantaneously transmit data to central analysis stations, circumventing the need of a transmitting device, and potential data loss if the patient is not within the range of the latter, when a clinically significant arrhythmia appears (70). This would grant real-time surveillance of the heart rhythm, which is of outmost importance in high-risk patients, such as those in risk of ventricular arrhythmias.
Further improvement of the diagnostic accuracy has the potential to transform wearables, such as smartwatches, from screening and pre-diagnostic tools to diagnostic modalities. The integration of artificial intelligence algorithms in basic medical tools, such as the 12-lead ECG, has demonstrated promising results, regarding the early and accurate detection of structural heart disorders, thus extending beyond the field of arrhythmia diagnosis (121, 122). The combination of this technological advancement with wearable devices could improve the reliability of their measurements and provide prognostic features for the detection of subclinical cardiac conditions (123). Advances in deep learning technologies and their application in the diagnostic algorithms will certainly further increase their diagnostic accuracy (124, 125). Moving away from validation studies, clinical trials pursuing hard endpoints, such as cardiovascular mortality or stroke, are essential to facilitate wider acceptance of wearables from clinicians, their implementation in everyday clinical practice and to support device reimbursement from insurance companies.
As wearable devices become more affordable and reach an increasingly number of consumers, a plethora of sensitive health data are anticipated to be generated. There is a clear necessity for an integrated system to collect and safely store personal information, in a way that will ensure users' privacy. Clear legal regulations, along with an ethical framework, under which personal data are collected and handled, are essential (126). Data processing should target accurate diagnosis and provide the attending physician with clinically meaningful information. In this way, integrated data handling systems could translate to improved patient management, with personalized healthcare interventions and better utilization of health care resources.
Conclusions
Wearable devices are a new reality in monitoring and management of cardiac arrhythmias. Pertinent caveats, such as signal quality, connectivity issues, battery life limitations, sub-optimal diagnostic accuracy and data security and storage, need to be addressed, in order to enable their full utilization as medical devices. However, advanced technological developments contribute to rapid improvement and accomplishment of future intentions and are expected to establish the role of smart wearables as important tools in the emerging era of telehealth, remote patients' control, personalized and precision medicine.
Author Contributions
ST, AX, VS, DA, and PV: conception of the theme and design. AX and VS: data collection and preparation of the draft manuscript. ST: editing. ST and PV: final revision and supervision. All authors approved the final version of the manuscript.
Conflict of Interest
The authors declare that the research was conducted in the absence of any commercial or financial relationships that could be construed as a potential conflict of interest.
Publisher's Note
All claims expressed in this article are solely those of the authors and do not necessarily represent those of their affiliated organizations, or those of the publisher, the editors and the reviewers. Any product that may be evaluated in this article, or claim that may be made by its manufacturer, is not guaranteed or endorsed by the publisher.
References
1. Khurshid S, Choi SH, Weng LC, Wang EY, Trinquart L, Benjamin EJ, et al. Frequency of cardiac rhythm abnormalities in a half million adults. Circ Arrhythmia Electrophysiol. (2018) 11. doi: 10.1161/CIRCEP.118.006273
2. Virani SS, Alonso A, Aparicio HJ, Benjamin EJ, Bittencourt MS, Callaway CW, et al. Heart disease and stroke statistics-2021 update a report from the american heart association. Circulation. (2021) 143:E254–743. doi: 10.1161/CIR.0000000000000950
3. Lindberg T, Wimo A, Elmståhl S, Qiu C, Bohman DM, Sanmartin Berglund J. Prevalence and incidence of atrial fibrillation and other arrhythmias in the general older population: findings from the swedish national study on aging and care. Gerontol Geriatr Med. (2019) 5:233372141985968. doi: 10.1177/2333721419859687
4. Kornej J, Börschel CS, Börschel CS, Benjamin EJ, Benjamin EJ, Schnabel RB, et al. Epidemiology of atrial fibrillation in the 21st century: novel methods and new insights. Circ Res. (2020) 127:4–20. doi: 10.1161/CIRCRESAHA.120.316340
5. Timmis A, Townsend N, Gale CP, Torbica A, Lettino M, Petersen SE, et al. European society of cardiology: Cardiovascular disease statistics 2019. Eur Heart J. (2020) 41:12–85. doi: 10.1093/eurheartj/ehz859
6. Mou L, Norby FL, Chen LY, O'Neal WT, Lewis TT, Loehr LR, et al. Lifetime risk of atrial fibrillation by race and socioeconomic status: ARIC study (Atherosclerosis Risk in Communities). Circ Arrhythmia Electrophysiol. (2018) 11. doi: 10.1161/CIRCEP.118.006350
7. Lowres N, Neubeck L, Redfern J, Ben Freedman S. Screening to identify unknown atrial fibrillation: A systematic review. Thromb Haemost. (2013) 110:213–22. doi: 10.1160/TH13-02-0165
8. Wolf PA, Abbott RD, Kannel WB. Atrial fibrillation as an independent risk factor for stroke: The framingham study. Stroke. (1991) 22:983–8. doi: 10.1161/01.STR.22.8.983
9. Hannon N, Sheehan O, Kelly L, Marnane M, Merwick A, Moore A, et al. Stroke associated with atrial fibrillation - Incidence and early outcomes in the north dublin population stroke study. Cerebrovasc Dis. (2009) 29:43–9. doi: 10.1159/000255973
10. Asplund K. High prevalence of atrial fibrillation among patients with ischemic stroke. Stroke. (2014) 45:2599–605. doi: 10.1161/STROKEAHA.114.006070
11. Hart RG, Pearce LA, Aguilar MI. Meta-analysis: Antithrombotic therapy to prevent stroke in patients who have nonvalvular atrial fibrillation. Ann Intern Med. (2007) 146:857–67. doi: 10.7326/0003-4819-146-12-200706190-00007
12. Hindricks G, Potpara T, Dagres N, Bax JJ, Boriani G, Dan GA, et al. 2020 ESC Guidelines for the diagnosis and management of atrial fibrillation developed in collaboration with the European Association for Cardio-Thoracic Surgery (EACTS). Eur Heart J. (2021) 42:373–498. doi: 10.1093/eurheartj/ehaa612
13. Maines M, Zorzi A, Tomasi G, Angheben C, Catanzariti D, Piffer L, et al. Clinical impact, safety, and accuracy of the remotely monitored implantable loop recorder Medtronic Reveal LINQ TM. Europace. (2018) 20:1050–7. doi: 10.1093/europace/eux187
14. Bayoumy K, Gaber M, Elshafeey A, Mhaimeed O, Dineen EH, Marvel FA, et al. Smart wearable devices in cardiovascular care: where we are and how to move forward. Nat Rev Cardiol. (2021) 18:581–99. doi: 10.1038/s41569-021-00522-7
15. Vardas P, Cowie M, Dagres N, Asvestas D, Tzeis S, Vardas EP, et al. The electrocardiogram endeavour: From the Holter single-lead recordings to multilead wearable devices supported by computational machine learning algorithms. Europace. (2020) 22:19–23. doi: 10.1093/europace/euz249
16. Eysenck W, Freemantle N, Sulke N. A randomized trial evaluating the accuracy of AF detection by four external ambulatory ECG monitors compared to permanent pacemaker AF detection. J Interv Card Electrophysiol. (2020) 57:361–9. doi: 10.1007/s10840-019-00515-0
17. Seshadri DR, Bittel B, Browsky D, Houghtaling P, Drummond CK, Desai MY, et al. Accuracy of apple watch for detection of atrial fibrillation. Circulation. (2020) 141:702–3. doi: 10.1161/CIRCULATIONAHA.119.044126
18. Turakhia MP, Hoang DD, Zimetbaum P, Miller JD, Froelicher VF, Kumar UN, et al. Diagnostic utility of a novel leadless arrhythmia monitoring device. Am J Cardiol. (2013) 112:520–4. doi: 10.1016/j.amjcard.2013.04.017
19. Izmailova ES, McLean IL, Hather G, Merberg D, Homsy J, Cantor M, et al. Continuous monitoring using a wearable device detects activity-induced heart rate changes after administration of amphetamine. Clin Transl Sci. (2019) 12:677–86. doi: 10.1111/cts.12673
20. Engel JM, Mehta V, Fogoros R, Chavan A. Study of arrhythmia prevalence in NUVANT mobile cardiac telemetry system patients. Proc Annu Int Conf IEEE Eng Med Biol Soc EMBS. (2012) (4):2440–3. doi: 10.1109/EMBC.2012.6346457
21. Engel JM, Chakravarthy N, Nosbush G, Merkert M, Richard Fogoros MD, Chavan A. Comparison of arrhythmia prevalence in NUVANT Mobile Cardiac Telemetry System patients in the US and India. 36th Annu Int Conf IEEE Eng Med Biol Soc EMBC. (2014) 2014:2730–3. doi: 10.1109/EMBC.2014.6944187
22. Khushhal A, Nichols S, Evans W, Gleadall-Siddall D, Page R, O'Doherty A, et al. Validity and reliability of the apple watch for measuring heart rate during exercise. Sport Med Int Open. (2017) 1:E206–E211. doi: 10.1055/s-0043-120195
23. Thomson EA, Nuss K, Comstock A, Reinwald S, Blake S, Pimentel RE, et al. Heart rate measures from the Apple Watch, Fitbit Charge HR 2, and electrocardiogram across different exercise intensities electrocardiogram across different exercise intensities. J Sports Sci. (2019) 37:1–9. doi: 10.1080/02640414.2018.1560644
24. Shcherbina A, Mikael Mattsson C, Waggott D, Salisbury H, Christle JW, Hastie T, et al. Accuracy in wrist-worn, sensor-based measurements of heart rate and energy expenditure in a diverse cohort. J Pers Med. (2017) 7:1–12. doi: 10.3390/jpm7020003
25. Stahl SE, An HS, Dinkel DM, Noble JM, Lee JM. How accurate are the wrist-based heart rate monitors during walking and running activities? Are they accurate enough? BMJ Open Sport Exerc Med. (2016) 2. doi: 10.1136/bmjsem-2015-000106
26. Xie J, Wen D, Liang L, Jia Y, Gao L, Lei J. Evaluating the validity of current mainstream wearable devices in fitness tracking under various physical activities: Comparative study. JMIR mHealth uHealth. (2018) 6. doi: 10.2196/mhealth.9754
27. Kinnunen H, Rantanen A, Kenttä T, Koskimäki H. Feasible assessment of recovery and cardiovascular health: accuracy of nocturnal HR and HRV assessed via ring PPG in comparison to medical grade ECG. Physiol Meas. (2018) 41:04NT01. doi: 10.1088/1361-6579/ab840a
28. Ummel JD, Hoilett OS, Walters BD, Bluhm NDP, Pickering AS, Wilson DA, et al. A multi-sensor ring capturing respiration, electrocardiogram, oxygen saturation, and skin temperature1. Annu Int Conf IEEE Eng Med Biol Soc. (2020) 4394–7. doi: 10.1109/EMBC44109.2020.9176654
29. Walsh JA, Topol EJ, Steinhubl SR. Novel wireless devices for cardiac monitoring. Circulation. (2014) 130:573–81. doi: 10.1161/CIRCULATIONAHA.114.009024
30. Boudreaux BD, Hebert EP, Hollander DB, Williams BM, Cormier CL, Naquin MR, et al. Validity of Wearable Activity Monitors during Cycling and Resistance Exercise. Med Sci Sports Exerc. (2018) 50:624–33. doi: 10.1249/MSS.0000000000001471
31. Navalta JW, Montes J, Bodell NG, Salatto RW, Manning JW, DeBeliso M. Concurrent heart rate validity of wearable technology devices during trail running. PLoS ONE. (2020) 15:e0238569. doi: 10.1371/journal.pone.0238569
32. Bunn J, Wells E, Manor J, Webster M. Evaluation of earbud and wristwatch heart rate monitors during aerobic and resistance training. Int J Exerc Sci. (2019) 12:374–84.
33. Johnstone JA, Ford PA, Hughes G, Watson T, Garrett AT. BioharnessTM multivariable monitoring device. Part I: Validity. J Sport Sci Med. (2012) 11:400–8.
34. Pasadyn SR, Soudan M, Gillinov M, Houghtaling P, Phelan D, Gillinov N, et al. Accuracy of commercially available heart rate monitors in athletes: A prospective study. Cardiovasc Diagn Ther. (2019) 9:379–85. doi: 10.21037/cdt.2019.06.05
35. Nazari G, MacDermid JC, Sinden KE, Richardson J, Tang A. Reliability of zephyr bioharness and fitbit charge measures of heart rate and activity at rest, during the modified Canadian aerobic fitness test, and recovery. J Strength Cond Res. (2019) 33:559–71. doi: 10.1519/JSC.0000000000001842
36. Aripriharta Muladi, Zaeni IAE, Firmansah A, Rizqi AA, Horng GJ. Performance evaluation of the heart rate measurement using smart shoes. AIP Conf Proc. (2020) 2231. doi: 10.1063/5.0002597
37. Constant N, Douglas-Prawl O, Johnson S, Mankodiya K. Pulse-Glasses: An unobtrusive, wearable HR monitor with Internet-of-Things functionality. 2015 IEEE 12th Int Conf Wearable Implant Body Sens Networks, BSN. (2015). p. 1–5. doi: 10.1109/BSN.2015.7299350
38. Ballaji HK, Correia R, Korposh S, Hayes-Gill BR, Hernandez FU, Salisbury B, et al. A textile sleeve for monitoring oxygen saturation using multichannel optical fibre photoplethysmography. Sensors (Basel). (2020) 20:6568. doi: 10.3390/s20226568
39. Allen J. Photoplethysmography and its application in clinical physiological measurement. Physiol Meas. (2007) 28:R1–39. doi: 10.1088/0967-3334/28/3/R01
40. Castaneda D, Esparza A, Ghamari M, Soltanpur C, Nazeran H. A review on wearable photoplethysmography sensors and their potential future applications in health care. Int J Biosens Bioelectron. (2018) 4:195–202. doi: 10.15406/ijbsbe.2018.04.00125
41. Kamal AAR, Harness JB, Irving G, Mearns AJ. Skin photoplethysmography - a review. Comput Methods Programs Biomed. (1989) 28:257–69. doi: 10.1016/0169-2607(89)90159-4
42. Sološenko A, Petrenas A, Paliakaite B, Leif S, Marozas V. Detection of atrial fibrillation using a wrist-worn device. Physiol Meas. (2019) 40:025003. doi: 10.1088/1361-6579/ab029c
43. Fan YY, Li YG, Li J, Cheng WK, Shan ZL, Wang YT, et al. Diagnostic performance of a smart device with photoplethysmography technology for atrial fibrillation detection: Pilot study (pre-mafa II registry). JMIR mHealth uHealth. (2019) 7:e11437. doi: 10.2196/11437
44. Bumgarner JM, Lambert CT, Hussein AA, Cantillon DJ, Baranowski B, Wolski K, et al. Smartwatch algorithm for automated detection of atrial fibrillation. J Am Coll Cardiol. (2018) 71:2381–8. doi: 10.1016/j.jacc.2018.03.003
45. Lobodzinski SS. ECG patch monitors for assessment of cardiac rhythm abnormalities. Prog Cardiovasc Dis. (2013) 56:224–9. doi: 10.1016/j.pcad.2013.08.006
46. Isakadze N, Martin SS. How useful is the smartwatch ECG? Trends Cardiovasc Med. (2020) 30:442–8. doi: 10.1016/j.tcm.2019.10.010
47. Al-Kaisey AM, Koshy AN, Ha FJ, Spencer R, Toner L, Sajeev JK, et al. Accuracy of wrist-worn heart rate monitors for rate control assessment in atrial fibrillation. Int J Cardiol. (2020) 300:161–4. doi: 10.1016/j.ijcard.2019.11.120
48. Dörr M, Nohturfft V, Brasier N, Bosshard E, Djurdjevic A, Gross S, et al. The WATCH AF trial: SmartWATCHes for detection of atrial fibrillation. JACC Clin Electrophysiol. (2019) 5:199–208. doi: 10.1016/j.jacep.2018.10.006
49. Nemati S, Ghassemi MM, Ambai V, Isakadze N, Levantsevych O, Shah A, et al. Monitoring and detecting atrial fibrillation using wearable technology. Proc Annu Int Conf IEEE Eng Med Biol Soc. (2016) 3394–7. doi: 10.1109/EMBC.2016.7591456
50. Nazarian S, Lam K, Darzi A, Ashrafian H. Diagnostic accuracy of smartwatches for the detection of cardiac arrhythmia: systematic review and meta-analysis. J Med Internet Res. (2021) 23:e28974. doi: 10.2196/28974
51. Rajakariar K, Koshy AN, Sajeev JK, Nair S, Roberts L, Teh AW. Accuracy of a smartwatch based single-lead electrocardiogram device in detection of atrial fibrillation. Heart. (2020) 106:665–70. doi: 10.1136/heartjnl-2019-316004
52. Rosenberg MA, Samuel M, Thosani A, Zimetbaum PJ. Use of a noninvasive continuous monitoring device in the management of atrial fibrillation: A pilot study. PACE - Pacing Clin Electrophysiol. (2013) 36:328–33. doi: 10.1111/pace.12053
53. Nault I, André P, Plourde B, Leclerc F, Sarrazin JF, Philippon F, et al. Validation of a novel single lead ambulatory ECG monitor – CardiostatTM – Compared to a standard ECG Holter monitoring. J Electrocardiol. (2019) 53:57–63. doi: 10.1016/j.jelectrocard.2018.12.01187
54. Rothman SA, Laughlin JC, Seltzer J, Walia JS, Baman RI, Siouffi SY, et al. The diagnosis of cardiac arrhythmias: A prospective multi-center randomized study comparing mobile cardiac outpatient telemetry versus standard loop event monitoring. J Cardiovasc Electrophysiol. (2007) 18:241–7. doi: 10.1111/j.1540-8167.2006.00729.x
55. Tayal AH, Tian M, Kelly KM, Jones SC, Wright DG, Singh D, et al. Atrial fibrillation detected by mobile cardiac outpatient telemetry in cryptogenic TIA or stroke. Neurology. (2008) 71:1696–70. doi: 10.1212/01.wnl.0000325059.86313.31
56. Miller DJ, Khan MA, Schultz LR, Simpson JR, Katramados AM, Russman AN, et al. Outpatient cardiac telemetry detects a high rate of atrial fibrillation in cryptogenic stroke. J Neurol Sci. (2013) 324:57–6. doi: 10.1016/j.jns.2012.10.001
57. Barrett PM, Komatireddy R, Haaser S, Topol S, Sheard J, Encinas J, et al. Comparison of 24-hour Holter monitoring with 14-day novel adhesive patch electrocardiographic monitoring. Am J Med. (2014) 127:95.e11–17. doi: 10.1016/j.amjmed.2013.10.003
58. Derkac WM, Finkelmeier JR, Horgan DJ, Hutchinson MD. Diagnostic yield of asymptomatic arrhythmias detected by mobile cardiac outpatient telemetry and autotrigger looping event cardiac monitors. J Cardiovasc Electrophysiol. (2017) 28:1475–8. doi: 10.1111/jce.13342
59. Smith WM, Riddell F, Madon M, Gleva MJ. Comparison of diagnostic value using a small, single channel, P-wave centric sternal ECG monitoring patch with a standard 3-lead Holter system over 24 hours. Am Heart J. (2017) 185:67–73. doi: 10.1016/j.ahj.2016.11.006
60. Koshy AN, Sajeev JK, Nerlekar N, Brown AJ, Rajakariar K, Zureik M, et al. Smart watches for heart rate assessment in atrial arrhythmias. Int J Cardiol. (2018) 266:124–7. doi: 10.1016/j.ijcard.2018.02.073
61. Rho R, Vossler M, Blancher S, Poole JE. Comparison of 2 ambulatory patch ECG monitors: The benefit of the P-wave and signal clarity. Am Heart J. (2018) 203:109–17. doi: 10.1016/j.ahj.2018.03.022
62. Selvaraj N, Nallathambi G, Moghadam R, Aga A. Fully disposable wireless patch sensor for continuous remote patient monitoring. Annu Int Conf IEEE Eng Med Biol Soc. (2018). p.1632-1635. doi: 10.1109/EMBC.2018.8512569
63. Steinhubl SR, Waalen J, Edwards AM, Ariniello LM, Mehta RR, Ebner GS, et al. Effect of a home-Based wearable continuous ECG monitoring patch on detection of undiagnosed atrial fibrillation the mSToPS randomized clinical trial. JAMA - J Am Med Assoc. (2018) 320:146–55. doi: 10.1001/jama.2018.810283
64. Tison GH, Sanchez JM, Ballinger B, Singh A, Olgin JE, Pletcher MJ, et al. Passive detection of atrial fibrillation using a commercially available smartwatch. JAMA. (2018) 3:409–16. doi: 10.1001/jamacardio.2018.0136
65. Ding EY, Han D, Whitcomb C, Bashar SK, Adaramola O, Soni A, et al. Accuracy and usability of a novel algorithm for detection of irregular pulse using a smartwatch among older adults: Observational study. JMIR Cardio. (2019) 3:e13850. doi: 10.2196/13850
66. Guo Y, Wang H, Zhang H, Liu T, Liang Z, Xia Y, et al. Mobile photoplethysmographic technology to detect atrial fibrillation. J Am Coll Cardiol. (2019) 74:2365–75. doi: 10.1016/j.jacc.2019.08.019
67. Kaura A, Sztriha L, Chan FK, Aeron-Thomas J, Gall N, Piechowski-Jozwiak B, et al. Early prolonged ambulatory cardiac monitoring in stroke (EPACS): An open-label randomised controlled trial. Eur J Med Res. (2019) 24:25. doi: 10.1186/s40001-019-0383-8
68. Perez MV, Mahaffey KW, Hedlin H, Rumsfeld JS, Garcia A, Ferris T, et al. Large-scale assessment of a smartwatch to identify atrial fibrillation. N Engl J Med. (2019) 381:1909–17. doi: 10.1056/NEJMoa1901183
69. Inui T, Kohno H, Kawasaki Y, Matsuura K, Ueda H, Tamura Y, et al. Use of a Smart watch for early detection of paroxysmal atrial fibrillation: validation study. JMIR Cardio. (2020) 4:e14857. doi: 10.2196/14857
70. Karunadas CP, Mathew C. Comparison of arrhythmia detection by conventional Holter and a novel ambulatory ECG system using patch and Android App, over 24 h period. Indian Pacing Electrophysiol J. (2020) 20:49–53. doi: 10.1016/j.ipej.2019.12.013
71. Nachman D, Gepner Y, Goldstein N, Kabakov E, Ishay A, Ben Littman R, et al. Comparing blood pressure measurements between a photoplethysmography-based and a standard cuff-based manometry device. Sci Rep. (2020) 10:16116. doi: 10.1038/s41598-020-73172-3
72. Schuurmans AAT, de Looff P, Nijhof KS, Rosada C, Scholte RHJ, Popma A, et al. Validity of the empatica E4 wristband to measure heart rate variability (hrv) parameters: a comparison to electrocardiography (ECG). J Med Syst. (2020) 44:190. doi: 10.1007/s10916-020-01648-w
73. Avram R, Ramsis M, Cristal AD, Nathan V, Zhu L, Kim J, et al. Validation of an algorithm for continuous monitoring of atrial fibrillation using a consumer smartwatch. Hear Rhythm. (2021) 18:1482–90. doi: 10.1016/j.hrthm.2021.03.044
74. Caillol T, Strik M, Ramirez FD, Abu-Alrub S, Marchand H, Buliard S, et al. Accuracy of a smartwatch-derived ECG for diagnosing bradyarrhythmias, tachyarrhythmias, and cardiac ischemia. Circ Arrhythmia Electrophysiol. (2021) 14:e009260. doi: 10.1161/CIRCEP.120.009260
75. Ha ACT, Verma S, Mazer CD, Quan A, Yanagawa B, Latter DA, et al. Effect of continuous electrocardiogram monitoring on detection of undiagnosed atrial fibrillation after hospitalization for cardiac surgery: a randomized clinical trial. JAMA Netw Open. (2021) 4:e2121867. doi: 10.1001/jamanetworkopen.2021.21867
76. Lubitz SA, Faranesh AZ, Atlas SJ, McManus DD, Singer DE, Pagoto S, et al. Rationale and design of a large population study to validate software for the assessment of atrial fibrillation from data acquired by a consumer tracker or smartwatch: The Fitbit heart study. Am Heart J. (2021) 238:16–26. doi: 10.1016/j.ahj.2021.04.003
77. Smart Watch Market: Global Industry Analysis Trends Market Size Forecasts up to 2026. (2021). Available online at: https://www.researchandmarkets.com (accessed January 29, 2021).
78. Dagher L, Shi H, Zhao Y, Marrouche NF. Wearables in cardiology: Here to stay. Hear Rhythm. (2020) 17:889–95. doi: 10.1016/j.hrthm.2020.02.023
79. Late-Breaking Science Abstracts and Featured Science Abstracts From the American Heart Association's Scientific Sessions 2021 and Late-Breaking Abstracts in Resuscitation Science From the Resuscitation Science Symposium 2021. Circulation. (2021) 144:e564–593. doi: 10.1161/CIR.0000000000001041
80. Van Gelder IC, Healey JS, Crijns HJGM, Wang J, Hohnloser SH, Gold MR, et al. Duration of device-detected subclinical atrial fibrillation and occurrence of stroke in ASSERT. Eur Heart J. (2017) 38:1339–44. doi: 10.1093/eurheartj/ehx042
81. Guo Y, Lip GYH. Mobile health for cardiovascular disease: The new frontier for AF management: Observations from the huawei heart study and mAFA-II randomised trial. Arrhythmia Electrophysiol Rev. (2020) 9:5–7. doi: 10.15420/aer.2020.12
82. Guo Y, Lane DA, Wang L, Chen Y, Lip GYH, Eckstein J, et al. Mobile Health (mHealth) technology for improved screening, patient involvement and optimising integrated care in atrial fibrillation: The mAFA (mAF-App) II randomised trial. Int J Clin Pract. (2019) 73:e13352. doi: 10.1111/ijcp.13352
83. Jenks S, Frank Peacock W, Cornelius AP, Shafer S, Pillow MT, Rayasam SS. Heart rate and heart rate variability in emergency medicine. Am J Emerg Med. (2020) 38:1335–9. doi: 10.1016/j.ajem.2019.10.035
84. Khan AA, Lip GYH, Shantsila A. Heart rate variability in atrial fibrillation: The balance between sympathetic and parasympathetic nervous system. Eur J Clin Invest. (2019) 49:e13174. doi: 10.1111/eci.13174
85. Arakawa T. Recent research and developing trends of wearable sensors for detecting blood pressure. Sensors (Basel). (2018) 18:2772. doi: 10.3390/s18092772
86. Staerk L, Sherer JA, Ko D, Benjamin EJ, Helm RH. Atrial fibrillation: epidemiology, pathophysiology, clinical outcomes. Circ Res. (2017) 120:1501–17. doi: 10.1161/CIRCRESAHA.117.30973272
87. Zhang MJ, Roetker NS, Folsom AR, Alonso A, Heckbert SR, Chen LY. Feasibility of using a leadless patch monitor in community cohort studies: The Multi-ethnic Study of Atherosclerosis. PACE - Pacing Clin Electrophysiol. (2018) 41:1389–90. doi: 10.1111/pace.13507
88. Steinhubl SR, Mehta RR, Ebner GS, Ballesteros MM, Waalen J, Steinberg G, et al. Rationale and design of a home-based trial using wearable sensors to detect asymptomatic atrial fibrillation in a targeted population: The mHealth Screening to Prevent Strokes (mSToPS) trial. Am Heart J. (2016) 175:77–85. doi: 10.1016/j.ahj.2016.02.011
89. Joshi AK, Kowey PR, Prystowsky EN, Benditt DG, Cannom DS, Pratt CM, et al. First experience with a Mobile Cardiac Outpatient Telemetry (MCOT) system for the diagnosis and management of cardiac arrhythmia. Am J Cardiol. (2005) 95:878–81. doi: 10.1016/j.amjcard.2004.12.015
90. Vasamreddy CR, Dalal D, Dong J, Cheng A, Spragg D, Lamiy SZ, et al. Symptomatic and asymptomatic atrial fibrillation in patients undergoing radiofrequency catheter ablation. J Cardiovasc Electrophysiol. (2006) 17:134–9. doi: 10.1111/j.1540-8167.2006.00359.x
91. Hansen IH, Hoppe K, Gjerde A, Kanters JK, Sorensen HBD. Comparing twelve-lead electrocardiography with close-to-heart patch based electrocardiography. 2015 37th Annual International Conference of the IEEE Engineering in Medicine and Biology Society (EMBS) (2015) p. 330–333. doi: 10.1109/EMBC.2015.7318366
92. Johnstone JA, Ford PA, Hughes G, Watson T, Mitchell ACS, Garrett AT. Field based reliability and validity of the bioharnessTM multivariable monitoring device. J Sport Sci Med. (2012) 11:643–52.
93. Johnstone JA, Ford PA, Hughes G, Watson T, Garrett AT. BioharnessTM Multivariable Monitoring Device: Part. II: Reliability. J Sports Sci Med. (2012) 11:409–17.
94. Kim JH, Roberge R, Powell JB, Shafer AB, Jon Williams W. Measurement accuracy of heart rate and respiratory rate during graded exercise and sustained exercise in the heat using the zephyr BioHarness TM. Int J Sports Med. (2013) 34:497–501. doi: 10.1055/s-0032-1327661
95. Plews DJ, Scott B, Altini M, Wood M, Kilding AE, Laursen PB. Comparison of heart-rate-variability recording with smartphone photoplethysmography, polar H7 chest strap, and electrocardiography. Int J Sports Physiol Perform. (2017) 12:1324–8. doi: 10.1123/ijspp.2016-0668
96. Akintola AA, van de Pol V, Bimmel D, Maan AC, van Heemst D. Comparative analysis of the equivital EQ02 lifemonitor with holter ambulatory ECG device for continuous measurement of ECG, heart rate, and heart rate variability: A validation study for precision and accuracy. Front Physiol. (2016) 7:391. doi: 10.3389/fphys.2016.00391
97. Liu Y, Zhu SH, Wang GH, Ye F, Li PZ. Validity and reliability of multiparameter physiological measurements recorded by the equivital lifemonitor during activities of various intensities. J Occup Environ Hyg. (2013) 10:78–85. doi: 10.1080/15459624.2012.747404
98. Lown M, Yue AM, Shah BN, Corbett SJ, Lewith G, Stuart B, et al. Screening for atrial fibrillation using economical and accurate technology (From the SAFETY Study). Am J Cardiol. (2018) 122:1339–44. doi: 10.1016/j.amjcard.2018.07.003
99. Perez De Isla L, Lennie V, Quezada M, Guinea J, Arce C, Abad P, et al. New generation dynamic, wireless and remote cardiac monitorization platform: A feasibility study. Int J Cardiol. (2011) 153:83–5. doi: 10.1016/j.ijcard.2011.08.074
100. Olmos C, Franco E, Suárez-Barrientos A, Fortuny E, Martín-García A, Viliani D, et al. Wearable wireless remote monitoring system: An alternative for prolonged electrocardiographic monitoring. Int J Cardiol. (2014) 172:e43–4. doi: 10.1016/j.ijcard.2013.12.068
101. Sayem ASM, Teay SH, Shahariar H, Fink PL, Albarbar A. Review on smart electro-clothing systems (SeCSs). Sensors. (2020) 20:587. doi: 10.3390/s20030587
102. Pagola J, Juega J, Francisco-Pascual J, Moya A, Sanchis M, Bustamante A, et al. Yield of atrial fibrillation detection with Textile Wearable Holter from the acute phase of stroke: Pilot study of Crypto-AF registry. Int J Cardiol. (2018) 251:45–50. doi: 10.1016/j.ijcard.2017.10.063
103. Burke J, Haigney MCP, Borne R, Krantz MJ. Smartwatch detection of ventricular tachycardia: Case series. Hear Case Reports. (2020) 6:800–4. doi: 10.1016/j.hrcr.2020.08.003
104. Strik M, Ploux S, Ramirez FD, Abu-Alrub S, Jaîs P, Haïssaguerre M, et al. Smartwatch-based detection of cardiac arrhythmias: Beyond the differentiation between sinus rhythm and atrial fibrillation. Hear Rhythm. (2021) 18:1524–32. doi: 10.1016/j.hrthm.2021.06.1176
105. Kassam N, Aziz O, Aghan E, Somji S, Mbithe H, Bapumia M, et al. Smart watch detection of supraventricular tachycardia (Svt): First case from tanzania. Int Med Case Rep J. (2021) 14:563–6. doi: 10.2147/IMCRJ.S328167
106. Siddeek H, Fisher K, McMakin S, Bass JL, Cortez D. AVNRT captured by Apple Watch Series 4: Can the Apple watch be used as an event monitor? Ann Noninvasive Electrocardiol. (2020) 25:e12742. doi: 10.1111/anec.12742
107. Spaccarotella CAM, Migliarino S, Mongiardo A, Sabatino J, Santarpia G, De Rosa S, et al. Measurement of the QT interval using the Apple Watch. Sci Rep. (2021) 11:10817. doi: 10.1038/s41598-021-89199-z
108. Nasarre M, Strik M, Daniel Ramirez F, Buliard S, Marchand H, Abu-Alrub S, et al. Using a smartwatch electrocardiogram to detect abnormalities associated with sudden cardiac arrest in young adults. EP Eur. (2021) euab192. doi: 10.1093/europace/euab192
109. Schreiber D, Sattar A, Drigalla D, Higgins S. Ambulatory cardiac monitoring for discharged emergency department patients with possible cardiac arrhythmias. West J Emerg Med. (2014) 15:194–8. doi: 10.5811/westjem.2013.11.18973
110. Côté J-M, Chetaille P, Abadir S, Gosselin L, Simonyan D, Dallaire F. Diagnostic Efficacy of a Single-Lead Ambulatory 14-Day ECG Monitor in Symptomatic Children. CJC Open. (2021) 3:1341–6. doi: 10.1016/j.cjco.2021.06.011
111. Asmarats L, Nault I, Ferreira-Neto AN, Muntané-Carol G, del Val D, Junquera L, et al. Prolonged continuous electrocardiographic monitoring prior to transcatheter aortic valve replacement: The PARE study. JACC Cardiovasc Interv. (2020) 13:1763–73. doi: 10.1016/j.jcin.2020.03.031
112. Tian Y, Padmanabhan D, McLeod CJ, Zhang P, Xiao P, Sandhu GS, et al. Utility of 30-Day continuous ambulatory monitoring to identify patients with delayed occurrence of atrioventricular block after transcatheter aortic valve replacement. Circ Cardiovasc Interv. (2019) 12:e007635. doi: 10.1161/CIRCINTERVENTIONS.118.007635
113. Castelletti S, Dagradi F, Goulene K, Danza AI, Baldi E, Stramba-Badiale M, et al. A wearable remote monitoring system for the identification of subjects with a prolonged QT interval or at risk for drug-induced long QT syndrome. Int J Cardiol. (2018) 266:89–94. doi: 10.1016/j.ijcard.2018.03.097
114. Honarbakhsh S, Sporton S, Monkhouse C, Lowe M, Earley MJ, Hunter RJ. Remote clinics and investigations in arrhythmia services: What have we learnt during Coronavirus disease 2019? Arrhythmia Electrophysiol Rev. (2021) 10:120–4. doi: 10.15420/aer.2020.37
115. Sana F, Isselbacher EM, Singh JP, Heist EK, Pathik B, Armoundas AA. Wearable devices for ambulatory cardiac monitoring: JACC state-of-the-art review. J Am Coll Cardiol. (2020) 75:1582–92. doi: 10.1016/j.jacc.2020.01.046
116. Manninger M, Zweiker D, Svennberg E, Chatzikyriakou S, Pavlovic N, Zaman JAB, et al. Current perspectives on wearable rhythm recordings for clinical decision-making: the wEHRAbles 2 survey. Europace. (2021) 23:1106–13. doi: 10.1093/europace/euab064
117. Svennberg E, Engdahl J, Al-Khalili F, Friberg L, Frykman V, Rosenqvist M. Mass screening for untreated atrial fibrillation the STROKESTOP study. Circulation. (2015) 131:2176–84. doi: 10.1161/CIRCULATIONAHA.114.014343
118. Svennberg E, Friberg L, Frykman V, Al-Khalili F, Engdahl J, Rosenqvist M. Clinical outcomes in systematic screening for atrial fibrillation (STROKESTOP): a multicentre, parallel group, unmasked, randomised controlled trial. Lancet. (2021) 398:1498–506. doi: 10.1016/S0140-6736(21)01637-8
119. Svendsen JH, Diederichsen SZ, Højberg S, Krieger DW, Graff C, Kronborg C, et al. Implantable loop recorder detection of atrial fibrillation to prevent stroke (The LOOP Study): a randomised controlled trial. Lancet. (2021) 398:1507–16. doi: 10.1016/S0140-6736(21)01698-6
120. Zheng Q, Tang Q, Wang ZL, Li Z. Self-powered cardiovascular electronic devices and systems. Nat Rev Cardiol. (2021) 18:7–21. doi: 10.1038/s41569-020-0426-4
121. Kashou AH, Ko W, Attia ZI, Cohen MS, Friedman PA, Noseworthy PA, et al. comprehensive artificial intelligence–enabled electrocardiogram interpretation program. Cardiovasc Digit Health J. (2020) 1:62–70. doi: 10.1016/j.cvdhj.2020.08.005
122. Yao X, Rushlow DR, Inselman JW, McCoy RG, Thacher TD, Behnken EM, et al. Artificial intelligence-enabled electrocardiograms for identification of patients with low ejection fraction: a pragmatic, randomized clinical trial. Nat Med. (2021) 27:815–9. doi: 10.1038/s41591-021-01335-4
123. Vardas PE, Asselbergs FW, van Smeden M, Friedman P. The year in cardiovascular medicine 2021: digital health and innovation. Eur Heart J. (2022) ehab874. doi: 10.1093/eurheartj/ehab874
124. Teplitzky BA, McRoberts M, Ghanbari H. Deep learning for comprehensive ECG annotation. Hear Rhythm. (2020) 17:881–8. doi: 10.1016/j.hrthm.2020.02.015
125. Krittanawong C, Johnson KW, Rosenson RS, Wang Z, Aydar M, Baber U, et al. Deep learning for cardiovascular medicine: A practical primer. Eur Heart J. (2019) 40:2058–73. doi: 10.1093/eurheartj/ehz056
Keywords: smart wearable devices, remote monitoring, sensors, arrhythmia detection, heart rate, cardiac function
Citation: Xintarakou A, Sousonis V, Asvestas D, Vardas PE and Tzeis S (2022) Remote Cardiac Rhythm Monitoring in the Era of Smart Wearables: Present Assets and Future Perspectives. Front. Cardiovasc. Med. 9:853614. doi: 10.3389/fcvm.2022.853614
Received: 12 January 2022; Accepted: 08 February 2022;
Published: 01 March 2022.
Edited by:
David Duncker, Hannover Medical School, GermanyReviewed by:
Gloria Cosoli, Marche Polytechnic University, ItalyMonika Gawalko, Maastricht University Medical Centre, Netherlands
Copyright © 2022 Xintarakou, Sousonis, Asvestas, Vardas and Tzeis. This is an open-access article distributed under the terms of the Creative Commons Attribution License (CC BY). The use, distribution or reproduction in other forums is permitted, provided the original author(s) and the copyright owner(s) are credited and that the original publication in this journal is cited, in accordance with accepted academic practice. No use, distribution or reproduction is permitted which does not comply with these terms.
*Correspondence: Stylianos Tzeis, stzeis@otenet.gr
†These authors have contributed equally to this work and share first authorship