Platelets promote human macrophages-mediated macropinocytosis of Clostridioides difficile
- 1Centro de Investigaciones Básicas y Aplicadas (CIBA), Universidad Nacional del Noroeste de la Provincia de Buenos Aires (UNNOBA), Buenos Aires, Argentina
- 2Centro de Investigaciones y Transferencias del Noroeste de la Provincia de Buenos Aires (CIT NOBA), UNNOBA-Universidad Nacional de San Antonio de Areco (UNSAdA)- Consejo Nacional de Investigaciones Científicas y Técnicas (CONICET), Buenos Aires, Argentina
- 3Instituto de Medicina Experimental (CONICET-Academia Nacional de Medicina), Buenos Aires, Argentina
Clostridioides difficile is the main causative agent of hospital-acquired diarrhea and the potentially lethal disease, C. difficile infection. The cornerstone of the current therapy is the use of antibiotics, which is not fully effective. The molecular mechanisms, inflammatory conditions and host-immune responses that could benefit the persistence or elimination of C. difficile remain unclear. Macrophages perform different ways of endocytosis as part of their immune surveillance functions and platelets, classically known for their coagulatory role, are also important modulators of the immune system. The aim of this study was to evaluate the endocytosis of vegetative C. difficile by human macrophages and the involvement of platelets in this process. Our results showed that both macrophages and platelets interact with live and heat-killed C. difficile. Furthermore, platelets form complexes with human monocytes in healthy donor’s fresh blood and the presence of C. difficile increased these cell-cell interactions. Using flow cytometry and confocal microscopy, we show that macrophages can internalize C. difficile and that platelets improve this uptake. By using inhibitors of different endocytic pathways, we demonstrate that macropinocytosis is the route of entry of C. difficile into the cell. Taken together, our findings are the first evidence for the internalization of vegetative non-toxigenic and hypervirulent C. difficile by human macrophages and highlight the role of platelets in innate immunity during C. difficile infection. Deciphering the crosstalk of C. difficile with immune cells could provide new tools for understanding the pathogenesis of C. difficile infection and for the development of host-directed therapies.
1 Introduction
Clostridioides difficile infection (CDI) is currently the leading cause of nosocomial diarrhea associated with the use of antibiotics (Brazier, 1998; Zhang et al., 2016). C. difficile is a Gram positive opportunistic pathogen that takes advantage of gut dysbiosis to establish colonization of the intestine, inducing tissue damage by toxin production. C. difficile is responsible for about 250,000 CDI cases and 13,000 deaths in the United States each year (Center for Disease Control and Prevention, 2019). Given the increase in the incidence and severity of CDI, the high rate of recurrence (>35%) and the appearance of hypervirulent strains, the Center for Control and Prevention in the United States has pointed this infection as an emergency (McDonald et al., 2007; CDC, 2012; Burke and Lamont, 2014; Balassiano et al., 2016; Elliott et al., 2017). Furthermore, there has been a large increase in community-acquired cases in the last decades, affecting individuals without risk factors typically associated with CDI (Legaria et al., 2003; Chitnis et al., 2013; Gupta and Khanna, 2014). Of importance, since the onset of the pandemic, approximately 72% of COVID-19 patients have been treated with broad-spectrum antibiotics. Therefore, the number of C. difficile infections, particularly recurrences, is expected to increase over time (EBR, 2020). All this together exhibit the urgent nature of this pathogen.
The immune response against C. difficile has been assessed before, however many aspects require a deeper understanding (Hernández Del Pino et al., 2020). Particularly, intestinal macrophages demand constant renewal from circulating monocytes, shaping the composition of the macrophage compartment in the intestinal mucosa when infection, inflammation or trauma alter tissue homeostasis (Bain and Mowat, 2014b; Na et al., 2019). Intestinal macrophage populations are heterogeneous and display different functions. Both anti-inflammatory and pro-inflammatory macrophages of the gut wall derive from blood monocytes that enter the mucosa and mature locally (Bain and Mowat, 2014a; Bain and Mowat, 2014b). In experimental CDI models, macrophages produce pro-inflammatory cytokines and chemokines, as well as show expression of certain receptors and molecules that indicate macrophages activation (Vohra and Poxton, 2012; Collins et al., 2014). Besides, the interaction and internalization of C. difficile spores and toxins has been observed in monocytes, macrophages or epithelial cells (Solomon et al., 2005; Modi et al., 2011; Paredes-Sabja et al., 2012; Chen et al., 2020). To our knowledge, the internalization of vegetative bacteria has not been demonstrated yet.
Platelets have aroused great interest in recent years, proving to be key participants in numerous immunological processes. Beyond their central roles in thrombosis and hemostasis, platelets play multiple roles in host defense against infection, interacting with bacteria, protozoa, fungi and viruses and showing anti-microbial properties (Klinger, 1997; Yeaman, 1997; Lam et al., 2015). Platelets can also interact with innate and adaptive immune cells promoting neutrophils activation, cytokines secretion by monocytes, leukocytes migration to the site of inflammation, B cells differentiation and immunoglobulin classes switching (Klinger, 1997; Yeaman, 1997; Trzeciak-Ryczek et al., 2013; Maouia et al., 2020; Scherlinger et al., 2023). In the context of CDI, severe infection could lead to sepsis and extra-intestinal infection (Lowenkron et al., 1996; Libby and Bearman, 2009; Steele et al., 2012; Abid and Bischof, 2019; Olsen et al., 2023). Moreover, inflammation in the gut can induce platelet activation and migration (Gaertner et al., 2017; Shannon, 2021; Trivigno et al., 2023), then platelets attached to the activated endothelium promote monocyte migration (Huilcaman et al., 2022; Trivigno et al., 2023). Beneficial or detrimental roles related to clinical symptoms have been reported for platelets counts during CDI (Pant et al., 2009; Byrn et al., 2012; Lee et al., 2014; Yan et al., 2017; Mihăilă et al., 2019; Nseir et al., 2019; Allegretti et al., 2020; Phanchana et al., 2020; Zhao et al., 2020; Buchrits et al., 2021). Nevertheless, their modulatory functions on immune responses against C. difficile have not been addressed.
Our findings provide new insights into the role of macrophages and platelets during CDI, which could be useful for the development of new therapeutic approaches in search of replacing antibiotics or as treatment adjuvants.
2 Materials and methods
2.1 Ethics statement and human samples
This research was carried out in accordance with the Helsinki declaration (2013) in agreement with the Ethics Committee of UNNOBA (COENOBA). Human blood from adult healthy donors was collected after obtaining written informed consents. The male/female group distribution was 1/2 and the median age was 36 yr.
2.2 Bacteria
In vitro infection of cells was performed with vegetative live non-toxigenic CD160 (NR-43516, BEI Resources) or hypervirulent NAP1/BI/027 (Sanitary Bacteriology Service INEI-ANLIS, Dr. Carlos G. Malbrán, Argentina) C. difficile strains. C. difficile was cultured at 37°C under anaerobic and dark conditions (anaerobic jars and envelopes from Mitsubishi Gas Chemical Company, Inc, Japan) in CHROMagar™ C. difficile and then in BHI Broth for 48h and 72h, respectively. NAP1/BI/027 strain was additionally inactivated by heat treatment (CDH). C. difficile culture broth was centrifuged at 25000 g for 10 minutes at 4°C. The bacteria pellet was washed with 1X PBS and inactivated at 95°C for 30 minutes. The antigenic content was quantified at 600 nm and adjusted to an OD of 1 for parity between experiments. NAP1/BI/027 secretome was also employed in some experiments. C. difficile culture supernatant was centrifuged, filtered with 0.22 μm membrane pore and protein content was determined by Micro BCA Protein Assay (Thermo Fisher Scientific Inc.).
For uptake assays, CD160, NAP1/BI/027 and CDH were coupled to FITC (Fluorescein isothiocyanate isomer I, Sigma Aldrich, St. Louis, MO, EE. UU) in carbonate buffer (NaHCO3 0,1M pH=9) for 2h at 37°C. Excess of FITC was removed by washing with 1X PBS and bacterial viability was evaluated (Supplementary Figure 1D).
2.3 Whole blood
Blood samples from healthy donors were collected into plastic tubes containing 3.8% sodium citrate in a blood:sodium citrate proportion of 10:1. Whole blood (50 μl) was cultured for 2h in the presence or absence of 0.5 × 106 CDH bacteria to evaluate the formation of monocytes-platelets complexes.
2.4 Macrophages
Peripheral blood mononuclear cells (PBMCs) from the whole blood samples of healthy donors were isolated by centrifugation over Ficoll-Hypaque (GE Healthcare, Chicago, IL, USA). Monocytes were purified from PBMCs by CD14 positive magnetic selection (Miltenyi Biotec) according to the manufacturer’s instructions. In all cases, the purity of the isolated cells was over 95%.
0.5 x 106/ml CD14 positive-selected monocytes were cultured at 37°C and 5% CO2 for 2h in absence of FBS to promote adherence. Non-adherent cells were removed and adherent cells were cultured for additional 16-18h in complete media as described before (Barbero et al., 2021) (Supplementary Figure 1A).
Monocyte-derived macrophages were stimulated or infected with 1 × 106 or 1 × 107 bacteria of CDH, CDH FITC, live CD160 FITC, or live NAP1/BI/027 FITC for 1h or 24h. For some experiments, cells were incubated in the presence or absence of washed platelets. To evaluate endocytic pathways, different inhibitors were added to the cell cultures 30 minutes previous to CDH stimulation: cytochalasin B (5 µg/ml), cytochalasin D (5 µg/ml), colchicine (1 µg/ml), vincristine (10 µg/ml), nystatin (100 U/ml), amiloride (100 µg/ml) or bafilomycin A (0,1 µM).
2.5 Platelets
Blood samples were collected into plastic tubes containing 3.8% sodium citrate in a blood:sodium citrate proportion of 10:1. Platelet-rich plasma (PRP) was obtained by blood sample centrifugation. To avoid leukocyte contamination, only the top 75% of the PRP was collected and centrifuged. Platelets were then washed with a buffer containing sodium citrate, citric acid, glucose and sodium chloride as reported before (Ali et al., 2017) and were resuspended in pre-warmed RPMI.
Washed platelets (WP) were then cultured for 2h in the presence or absence of CDH, CDH FITC, live CD160 FITC or live NAP1/BI/027 FITC at a platelet:bacteria ratio of 1:1 or 1:10 or used in co-culture experiments at macrophage:bacteria:platelet ratios of 1:1:10, 1:1:100 or 1:10:100 for 1h or 24h.
2.6 Flow cytometry
For whole blood experiments, the samples were fixed and erythrocytes were lysed with BD FACS® Lysing Solution (BD Biosciences) for 15 minutes at room temperature after staining.
In the case of WP cultures, the cells were centrifuged at 1500g for 10 minutes with no break. For monocyte-derived macrophages or cells from macrophages-WP co-cultures, cell collection was done by extensive washing with FACS buffer (1X PBS – 2% FBS) and 5 minutes’ centrifugation at 800 g. Samples were stained for 30 minutes at 4°C, washed and fixated with 1% PFA.
Fluorochrome-conjugated antibodies against CD14 (BioLegend®) or CD61 (BD Pharmigen™) were used for immune staining. A viability dye (Fixable Viability Dye eFluor™ eBioscience™ 780) was employed to exclude dead cells from analysis.
All samples were acquired on a FACSCanto II flow cytometer (BD Biosciences) and data analysis was performed using FlowJo 7.6.2 (Tree Star Inc., OR, USA).
2.7 Fluorescence and confocal microscopy
2 × 105 macrophages/well were cultured in chamber-slides (Nunc) as described before (Barbero et al., 2021). Cells were stimulated with CDH FITC and/or WP at the ratios indicated above in the presence or absence of endocytosis inhibitors.
Cells were fixed with 2% PFA for 20 minutes, washed with saline solution and permeabilized (1X PBS, 10% FBS and 0.5% saponin). Afterwards, cells were incubated with specific PE mouse anti-human CD61 (BD Pharmigen™) and Alexa Fluor 647 mouse anti-human CD14 (BioLegend®) antibodies. After washing, Flash Phalloidin Red 594 (BioLegend®) was added to stain F-actin and DAPI (Life Technologies) was employed as nuclear counterstaining.
Slides were mounted with PolyMount (Polysciences) and analyzed using trinocular fluorescence microscope Axio Imager.A2 (Carl Zeiss, Germany) or FV-1000 confocal microscope with an oil-immersion Plan Apochromatic 60X NA1.42 objective (Olympus). For quantification, at least 100 cells per field and 5 fields per treatment were analyzed in three independent experiments. Micrographs were taken in a blind manner. The obtained images were processed with FIJI software (open source, version ImageJ 2.0.0-rc-69/1.52p/java 1.8.0_172).
2.8 Statistical analysis
All assays were carried out at least three times in independent experiments. Representative examples are shown for flow cytometry histograms and confocal/fluorescence micrographs. Friedman test or Kruskal-Wallis test with corrected Dunn’s post hoc test were used for comparison between experimental groups. Mann-Whitney test was used to analyze differences between two groups of unpaired samples and Wilcoxon Rank Sum test or paired t test for paired samples.
Data was analyzed using GraphPad Prism 8.0.1 software (San Diego, CA, USA). p values < 0.05 were considered significant.
3 Results
3.1 C. difficile is internalized by human macrophages
We addressed the uptake of vegetative C. difficile form, which is necessary to produce toxins and spores in the gut. To identify interactions between macrophages and heat inactivated C. difficile (CDH) by flow cytometry and by confocal microscopy, CDH was coupled to FITC. CDH positive staining (Supplementary Figures 1B, C) and macrophages viability for all the bacteria amounts employed (Supplementary Figure 2A) were corroborated.
We found that about 29% of human macrophages interacted with bacteria both at 1h (macrophages:CDH 1:10) or 24h (macrophages:CDH 1:1) of culture (Figures 1A–C). Likewise, 70% of macrophages interacted with CDH FITC when cultured for 24h at a macrophages to CDH ratio of 1:10 (Figures 1A–C), suggesting a time- and load-dependent uptake of CDH by macrophages. When analyzing confocal micrographs, CDH FITC was observed within macrophages in all experimental conditions (Figures 1D–F). The intracellular localization was confirmed by orthogonal views sectioning (Figure 1F) and Z-stacks reconstructions (Supplementary Videos SV1–3). In addition, the amount of interaction between macrophages and CDH FITC resembled flow cytometry results (Figure 1E). Interestingly, infection of macrophages with live CD160 and NAP1/BI/027 strains or incubation with CDH plus NAP1/BI/027 secretome induced similar endocytic levels compared to CDH (Supplementary Figure 4B).
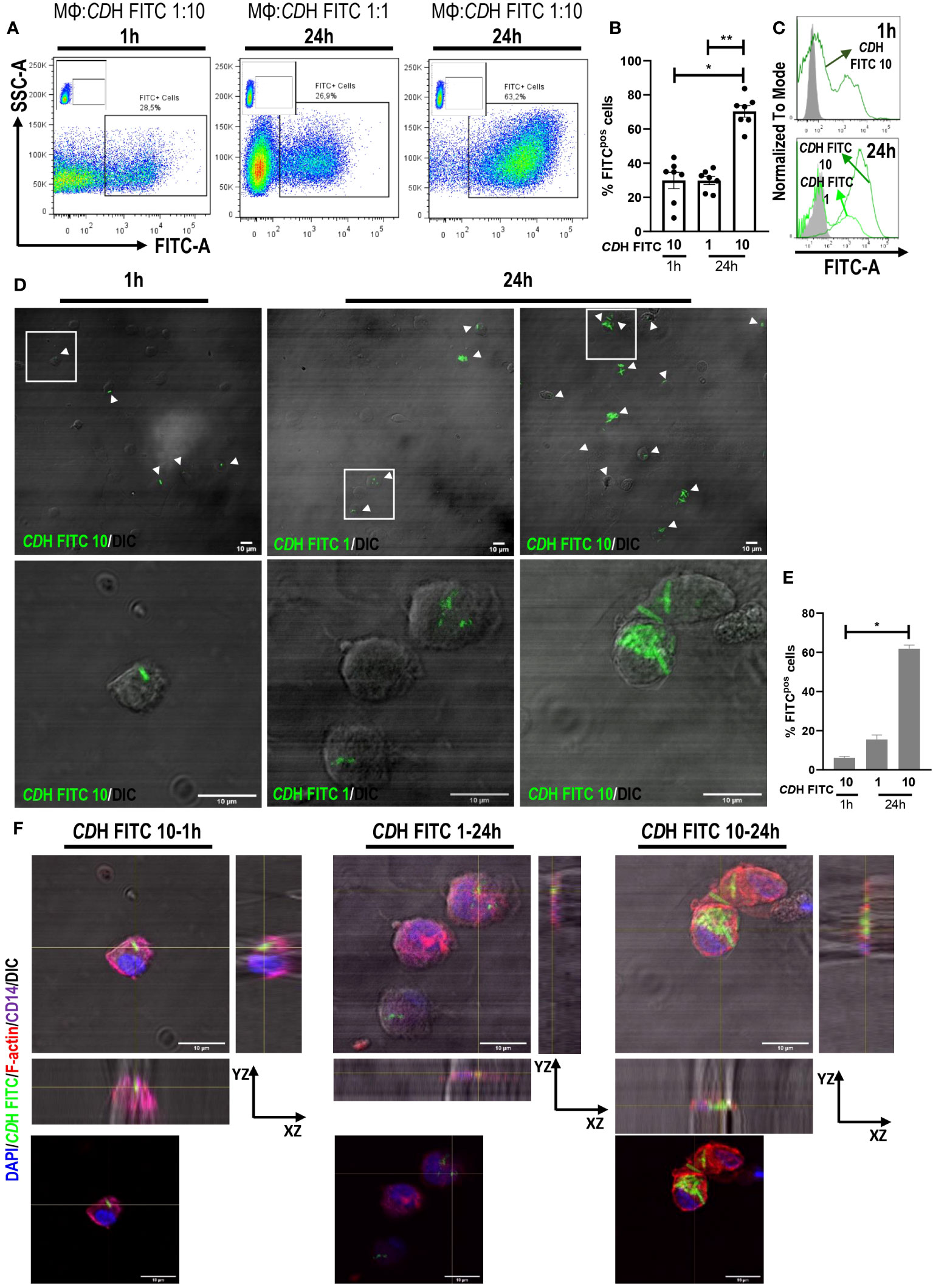
Figure 1 Internalization of C. difficile by human macrophages. Monocyte-derived macrophages (MΦs) from Healthy Donors (HD) were stimulated with heat-inactivated C. difficile coupled to FITC (CDH FITC). The ratios of MΦs:CDH FITC tested were 1:10 for 1h and 1:1 and 1:10 for 24h assays. (A) Representative dot plots, (B) percentage of FITC positive cells (macrophages internalizing CDH) and (C) representative histograms of flow cytometry experiments are shown. MΦs stimulated with unstained CDH were used as controls (gray histograms). (D) Representative micrographs (white boxes correspond to the magnified images shown in the bottom panels), (E) percentage of FITC positive cells and (F) orthogonal views (same magnification as in (D) showing the internalization of CDH FITC evaluated by confocal microscopy after cell fixation. White arrowheads point macrophages internalizing CDH. DAPI (nuclei) is shown in blue, Phalloidin (F-actin) in red and CD14 (macrophages) in purple. DIC: differential interference contrast. Scale bar: 10 μm (A–C) correspond to seven individual donors in seven independent experiments, (D–F) correspond to three individual donors in three independent experiments. (B, E) Bars represent the mean ± SEM. (B) Each dot corresponds to an individual healthy donor. (B) Friedman test, (E) Kruskal-Wallis test. *p<0.05, **p<0.01.
Altogether, our results demonstrate for the first time the internalization of vegetative C. difficile by human macrophages.
3.2 Platelets interact with C. difficile, monocytes and macrophages
In the context of C. difficile infection (CDI), the role of platelets in the outcome of the disease is still controversial. Here, we first evaluated platelets-C. difficile FITC interaction. We observed that both live NAP1/BI/027 and CD160 strains and CDH FITC were associated to platelets. Moreover, these associations were increased in the presence of a greater amount of bacteria as shown by flow cytometry and fluorescence microscopy (Figures 2A–D). We then tested the formation of platelets–monocytes complexes in whole blood from healthy donors. Whole blood was incubated for 2h with or without CDH and then stained with anti-CD14 and anti-CD61 antibodies (Supplementary Figure 2B). Flow cytometric analysis showed that CDH induced the establishment of complexes, as the percentage of monocytes bound to platelets was increased when compared to controls (Figure 2E). In addition, we co-cultured different proportions of platelets with macrophages that were stimulated or not with CDH. No differences in platelets-macrophages complexes formation were found in the presence of CDH after 24h, even when the macrophage:platelet ratio was increased 10-fold (macrophages:platelets, 1:10 vs 1:100) (Supplementary Figure 4A). We neither observed changes in platelets bounding to macrophages when the co-cultures were done for 1h using confocal microscopy (Figure 2F). However, we did detected platelets inside the macrophages (Figure 2F). We also noted that platelets showed a great ability to interact with macrophages both at 1h and 24h of co-culture since the majority of the phagocytes had associated platelets (Figure 2F and Supplementary Figure 4A).
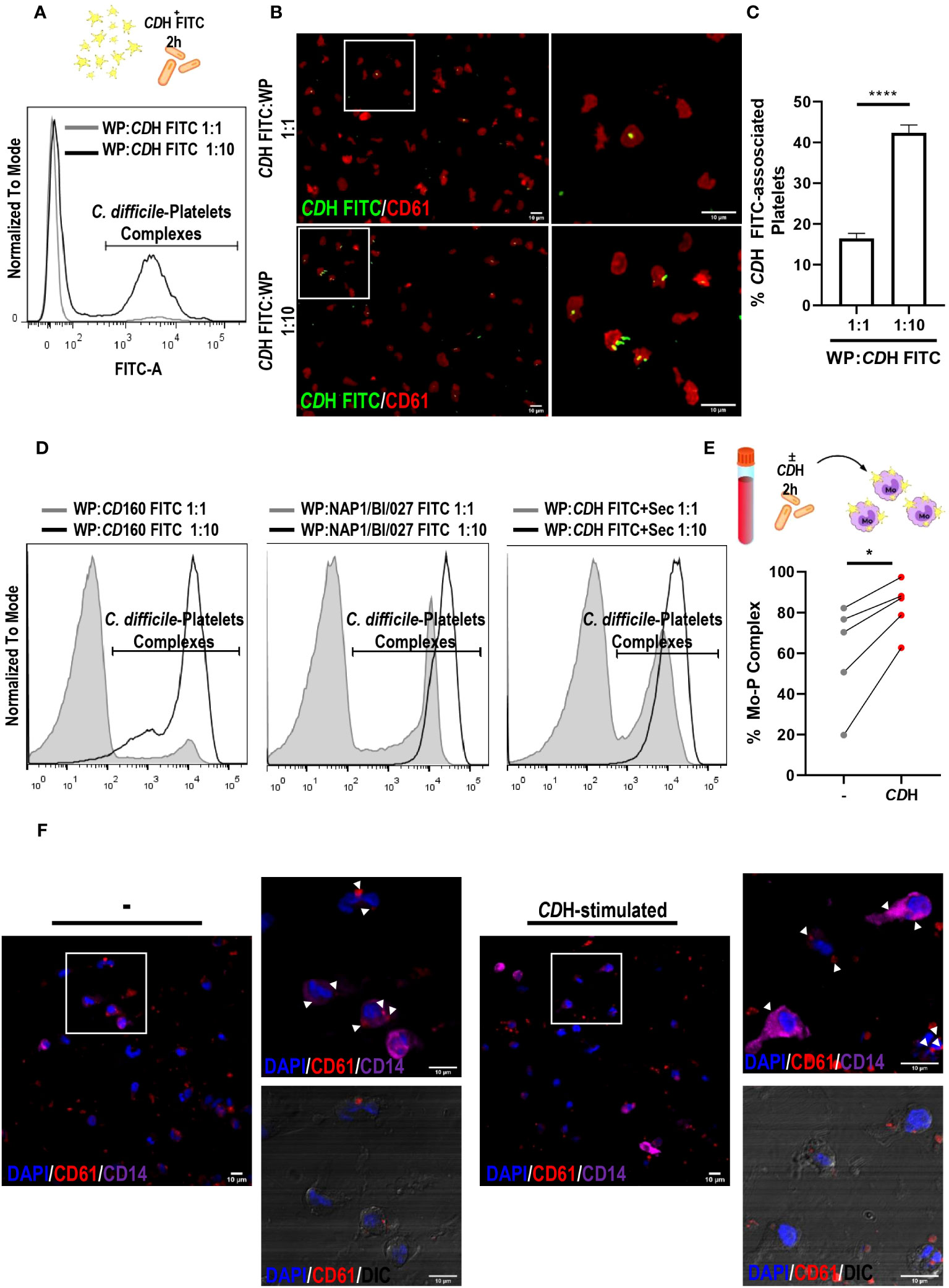
Figure 2 Interaction of platelets with C. difficile, monocytes and macrophages. (A–C) Washed Platelets (WP) from Healthy Donors (HD) were incubated with heat-inactivated C. difficile coupled to FITC (CDH FITC) at ratios 1:1 and 1:10 for 2h. CDH FITC-WP complexes were detected by flow cytometry (A) and fluorescence microscopy (B, C) using anti-CD61 PE antibody. (A, B) are representative experiments. (B) Images on the right are magnifications corresponding to white boxes on the left. (C) Quantification of CDH FITC-associated platelets from fluorescent micrographs is shown. (D) WP from HD were incubated with live CD160 and live NAP1/BI/027 C. difficile strains coupled to FITC and with CDH FITC plus NAP1/BI/027 secretome (CDH FITC+Sec) for 2h. In all cases, WP:bacteria ratios of 1:1 and 1:10 were tested and C. difficile-WP complexes were detected by flow cytometry. Representative histograms are shown. (E) Whole peripheral blood from HD was cultured in the presence or absence of CDH for 2h. Monocytes (Mo)-WP complexes were measured by flow cytometry using anti-CD14 Alexa Fluor 647 and anti-CD61 PE antibodies. (F) Macrophages (MΦs) and WP from HD were co-cultured in the presence or absence of CDH for 1h. MΦs-WP complexes were assessed by confocal microscopy after fixation, permeabilization and staining. The white boxes correspond to the magnified images on the right (representative experiment). White arrowheads point WP inside MΦs. DAPI (nuclei) is shown in blue, CD61 (platelets) in red and CD14 (macrophages) in purple. DIC: differential interference contrast. Scale bar: 10 μm (A, C, F) correspond to three individual donors in three independent experiments, (D) corresponds to four independent experiments, (E) corresponds to five individual donors in five independent experiments. (C) Bars represent the mean ± SEM. (E) Each dot corresponds to an individual healthy donor. (C) Mann-Whitney test, (E) Wilcoxon test. *p<0.05, ****p<0.0001.
In brief, these results show that platelets can interact with both immune cells and C. difficile.
3.3 C. difficile uptake is promoted by platelets
We designed two different approaches to evaluate platelets role in the uptake of C. difficile: 1- Platelets, CDH and macrophages were cultured all three together for 24h or 2- Platelets were pre-incubated with CDH for 2h and added to macrophages culture for additional 22h. Likewise, different macrophages:C. difficile:platelets ratios were tested.
CDH FITC-stimulated macrophages showed greater endocytic capacity when platelets were present in the cell culture (Figures 3A, B) as measured by flow cytometry. The highest percentage of FITC positive macrophages (macrophages internalizing CDH) was observed after CDH FITC stimulation in combination with platelets at the 1:10:100 proportion (Figure 3A). Moreover, the median intensity of fluorescence was also significantly increased in the presence of platelets in all the assessed ratios (Figure 3B), indicating that not only the number of C. difficile-internalizing macrophages increases, but also the amount of bacteria per phagocyte. Similar results were observed when platelets and CDH were pre-incubated for 2h (Supplementary Figures 3A, B). When evaluating confocal micrographs (Figure 3C), the majority of those macrophages that had internalized the bacteria presented associated platelets, confirming that platelets induce C. difficile uptake (Figure 3C, red arrowheads). We also noted that not internalized CDH was bound to platelets (Figure 3C, grey arrowheads). Anew, similar results were found in the pre-incubation system (Supplementary Figure 3C).
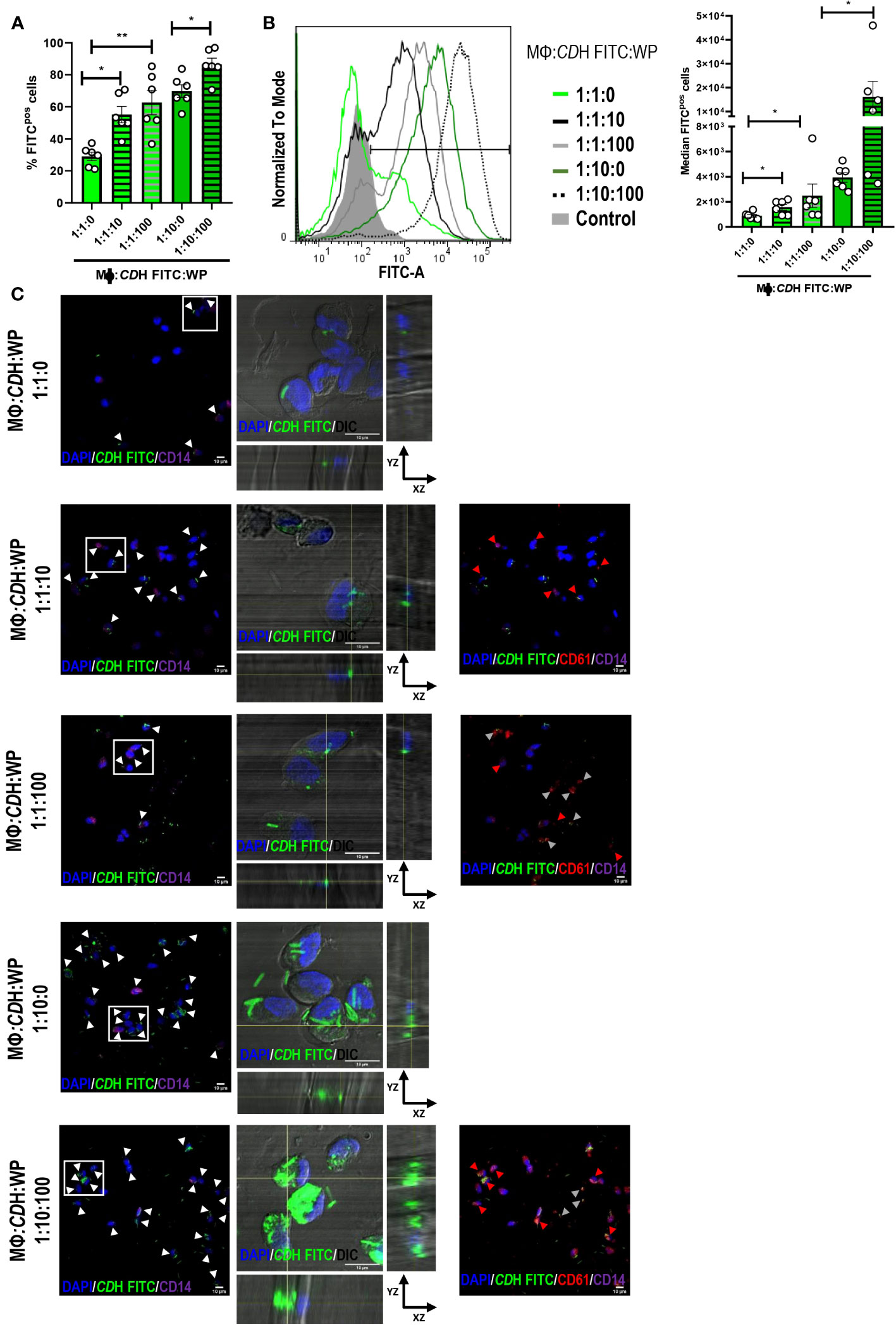
Figure 3 Role of platelets in C. difficile uptake. (A, B) Macrophages (MΦs) from Healthy Donors (HD) were stimulated with heat-inactivated C. difficile coupled to FITC (CDH FITC) in the presence or absence of Washed Platelets (WP) in ratios of 1:1:10, 1:1:100 and 1:10:100 (MΦs:C. difficile:WP) for 24h. The percentage of FITC positive cells (A) and the median intensity of fluorescence (B) were evaluated by flow cytometry. (B) Representative histograms (left) and the intensity quantification (right) are shown. In all cases comparisons were done against the experimental conditions without platelets (1:1:0 or 1:10:0). (C) MΦs: CDH FITC: WP cultures were performed as in A-B and the internalization capacity was evaluated by confocal microscopy. MΦs and WP were detected by direct staining with anti-CD14 Alexa Fluor 647 and anti-CD61 PE antibodies after fixation and permeabilization of the cells. DAPI was used as nuclear counterstaining. The white boxes correspond to the magnified images on the right (orthogonal views showing internalized CDH FITC in macrophages (DIC/DAPI)). In the panoramic micrographs, white arrowheads point macrophages (CD14/DAPI) internalizing CDH, red arrowheads point WP (CD61) and gray arrowheads indicate not internalized CDH bounded to platelets (CD61, red). DAPI (nuclei) is shown in blue, CD61 (platelets) in red and CD14 (macrophages) in purple. DIC: differential interference contrast. Scale bar: 10 μm. (A, B) correspond to six individual donors in six independent experiments, (C) correspond to three individual donors in three independent experiments. (A, B) Bars represent the mean ± SEM. Each dot corresponds to an individual healthy donor. (A, B) Friedman (1:1:10, 1:1:100 vs 1:1:0) and Wilcoxon (1:10:100 vs1:10:0) tests. *p<0.05, **p<0.01. Control= MΦs stimulated with unstained CDH.
Taken together, these results show that human macrophages enhance their internalizing potential against C. difficile in the presence of platelets.
3.4 Macrophages explore macropinocytosis for C. difficile uptake
To deduce the route of entry involved in C. difficile uptake we used selected inhibitors for different endocytic pathways (Table 1). Since C. difficile presents length variations that can reach up to 12 µm (Lynch et al., 2013; Ransom et al., 2014; Metzendorf et al., 2022), no inhibitors of clathrin-mediated uptake were used [this mechanism shows an upper size limit for internalization of approximately 200 nm approximately 200 nm (Rejman et al., 2004; Rennick et al., 2021; Sousa De Almeida et al., 2021)]. Macrophages were pre-incubated for 30 minutes with cytochalasin B, cytochalasin D, colchicine, vincristine, nystatin, bafilomycin A or amiloride before CDH FITC was added to the cell culture for 1h. We observed that only amiloride was able to reduce the percentage of FITC positive macrophages compared to the control (Figures 4A, B), suggesting that macropinocytosis helps macrophages to engulf C. difficile. On the other hand, neither autophagy, caveolae/lipid-mediated endocytosis, or microtubule polymerization appear to be involved in C. difficile internalization in our culture model (Figures 4A, B). We confirmed that amiloride reduced the number of cells that internalized C. difficile (Figure 4C, white arrowheads) by confocal microscopy. Moreover, for those FITC-positive cells, a decrease in the number of bacilli inside the cells was observed (Figure 4C, magnifications). Therefore, in agreement with our previous findings, when macropinocytosis was inhibited, macrophages reduced their ability to internalize C. difficile (Figure 4C).
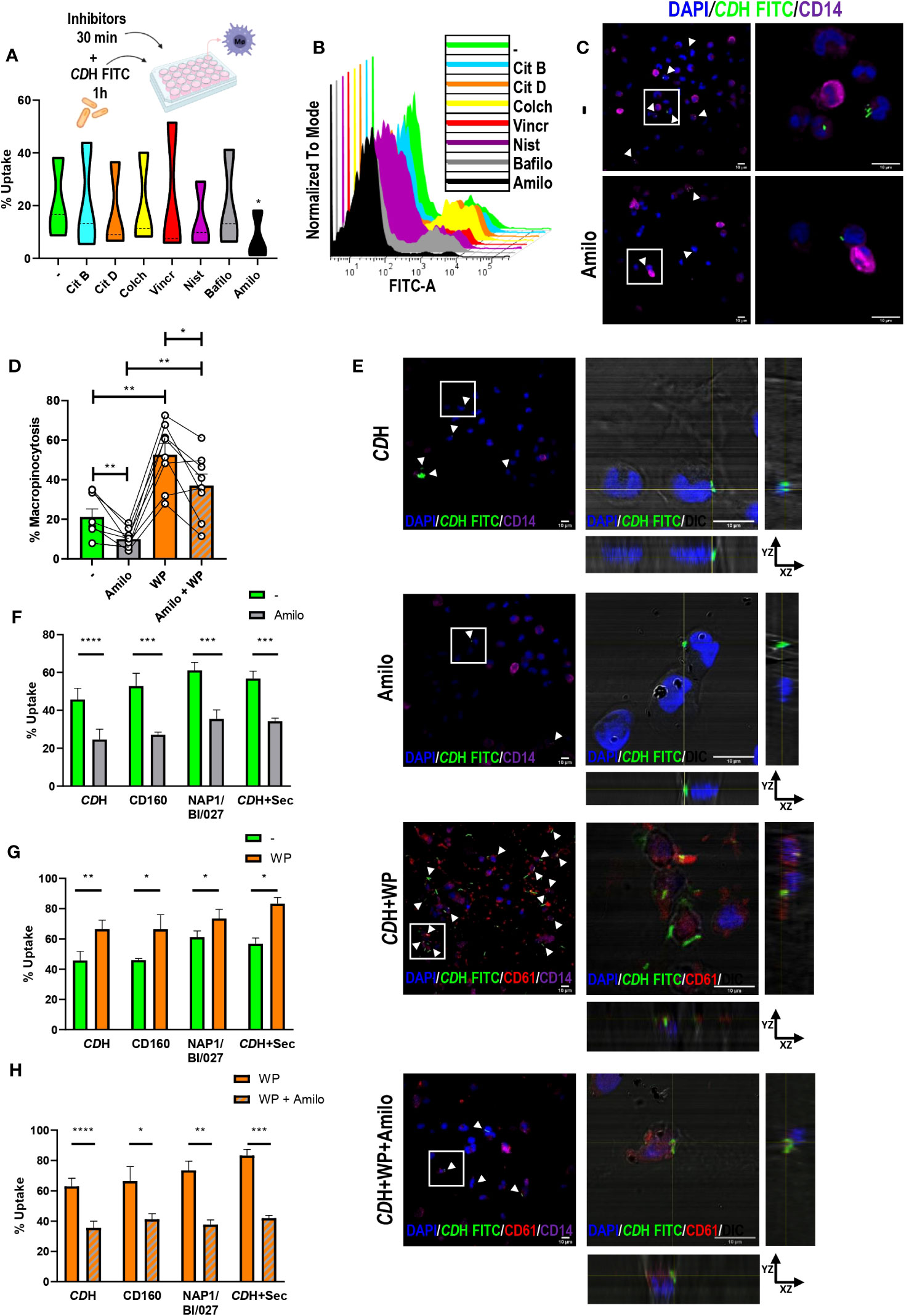
Figure 4 Evaluation of endocytic pathways against C. difficile. A) Macrophages (MΦs) from Healthy Donors (HD) were cultured with endocytosis inhibitors (Citochalasin B (Cit B, actin polymerization inhibitor), Citochalasin D (Cit D, inhibitor of actin polymerization by ATP hydrolysis), Colchicine (Colch, microtubules polymerization inhibitor), Vincristine (Vincr, microtubules polymerization inhibitor), Nystatin (Nist, inhibitor of caveolae/lipid mediated endocytosis), Bafilomycin (Bafilo, autophagy inhibitor) or Amiloride (Amilo, macropinocytosis inhibitor)). After 30min, heat-inactivated C. difficile coupled to FITC (CDH FITC) was added for 1h. CDH FITC internalization was assessed by flow cytometry (A, B) and confocal microscopy (C). (A) Percentage of FITC positive cells (uptake). (B) Representative histograms. (C) Confocal micrographs of untreated (-) or Amiloride treated (Amilo) MΦs obtained after cell fixation, staining with anti-CD14 Alexa Fluor 647 antibody (macrophages) and DAPI (nuclei) counterstaining. The white boxes correspond to the magnified images on the right. White arrowheads point internalized CDH FITC. (D) MΦs were stimulated with CDH FITC in the presence or absence of Washed Platelets (WP) (ratio 1:10:100) for 1h. Amiloride was added 30 minutes before to block macropinocytosis. Percentage of macropinocytic cells was measured by flow cytometry. (E) Cells were fixated, permeabilized and stained with anti-CD14 Alexa Fluor 647 (macrophages) and anti-CD61 PE (platelets) antibodies. DAPI (nuclei) was employed as counterstain and confocal images were obtained. The white boxes correspond to the magnified images on the right (orthogonal views showing platelets (CD61) and internalized CDH FITC in macrophages (DIC/DAPI)). In the panoramic micrographs, white arrowheads point macrophages internalizing CDH. DAPI (nuclei) is shown in blue, CD61 (platelets) in red and CD14 (macrophages) in purple. DIC: differential interference contrast. Scale bar: 10 μm. (F–H) MΦs were stimulated with CDH FITC or CDH FITC plus NAP1/BI/027 secretome (CDH FITC+Sec) or infected with live CD160 FITC (non-toxigenic) or NAP1/BI/027 FITC (hypervirulent) C. difficile strains as stated in (D). (A–C, E) correspond to three individual donors in three independent experiments, (D) correspond to nine individual donors in 9 independent experiments. (F–H) correspond to four donors in independent experiments. (A) Violin plots, (D, F–H) Bars represent the mean ± SEM. (D) Each dot corresponds to an individual healthy donor. (A, D) Friedman test. (F–H) 2-way ANNOVA *p<0.05, **p<0.01, *** p<0.001, **** p<0.0001.
Additionally, we evaluated the effect of amiloride treatment in the induction of C. difficile uptake mediated by platelets. Macrophages were cultured with live NAP1/BI/027 FITC, live CD160 FITC, CDH FITC or CDH FITC plus NAP1/BI/027 secretome for 1h after being pre-exposed to amiloride, platelets or the combination of platelets plus amiloride for 30 minutes. As detected by flow cytometry and confocal microscopy, platelets were capable of improving CDH FITC internalization also at short times of co-culture (Figures 4D, E and Supplementary Figure 4C). On the other hand, amiloride significantly reduced these levels both when added in combination with platelets or alone (Figures 4D, E (white arrowheads) and Supplementary Figure 4C). Similar results were observed for live non-toxigenic and hypervirulent C. difficile as well as for CDH FITC plus NAP1/BI/027 secretome (Figures 4F-H). Overall, these findings show that macropinocytosis allows C. difficile internalization by human macrophages and that platelets participate in this process (Supplementary Figure 4D).
Our results show that CDH is a valuable tool for in vitro studies assessing innate responses against C. difficile. Beyond understanding the immune responses against C. difficile, working with killed or attenuated pathogens may lead to the development of prevention strategies, vaccines, or even new host-directed therapies. Moreover, inactivated bacteria provide a constant and standardized source of antigens which is essential to perform reproducible experiments and compare results between different assays or laboratories.
Altogether, our findings suggest that the mechanism described in this work could be widely used by different strains of the C. difficile family regardless of the presence of toxins and that endocytosis is probably induced by antigens that are expressed in both live and dead bacteria. Moreover, this is the first report describing platelets as active participants in innate immunity against C. difficile boosting macrophages-mediated uptake.
4 Discussion
Clostridioides difficile infection (CDI) is prevalent in hospitalized patients, and despite prevention activities, CDI remains a life-threatening disease, highlighting the need for new treatment approaches and a better understanding of host-pathogen crosstalk.
It is now well-known that innate immunity is a double-edged sword during CDI, since it is crucial for inducing intestinal inflammation but also for resolving infection (Hernández Del Pino et al., 2020; Nibbering et al., 2021). Regarding macrophages, most of the reports are based on cell lines or murine models and evidence the activation of macrophages in response to C. difficile. Macrophages produce proinflammatory MIP-1α, MIP-2, MCP, IL-1β, IL-6, TNF-α, IL-12p40, and IL-12p70 when stimulated with C. difficile antigens (e.g. surface layer proteins, flagella, HSP42, and HSP60) or live bacteria (Vohra and Poxton, 2012; Collins et al., 2014; Saad et al., 2023). Likewise, these phagocytes up-regulate TLR2, TLR4, CD40, CD80, and MHCII expression (Collins et al., 2014). Moreover, inflammasome activation via TLR2-ATP-P2X7-Caspase 1 has been proposed as a beneficial mechanism to control bacterial load (Liu et al., 2018).
In our work we employed human monocyte-derived macrophages to address the endocytic capacity of these cells. Despite the fact that the intracellular localization of C. difficile has not been directly demonstrated yet, it has been proposed that phagocytosis of the bacteria or its toxins is essential to activate macrophages (Liu et al., 2018). C. difficile spores can be internalized by murine macrophages but they manage to survive and avoid their clearance (Paredes-Sabja et al., 2012). C. difficile toxin A can remodel cell membranes and gain access to interact with TLR9 in epithelial cells (Chen et al., 2020). Taking into account this information and the importance of the vegetative bacteria to induce pathogenesis at local level, we performed our experiments with the vegetative form of the C. difficile hypervirulent epidemic strain BI/NAPI/027 and the non-toxigenic CD160 strain. We found that human macrophages internalized C. difficile both at 1 or 24h of in vitro culture, showing greater uptake levels when incubated with higher amounts of bacteria. Our work is one of the first using different live C. difficile strains in normoxic in vitro models. We stained both live and inactivated C. difficile with FITC using a short time and few-steps protocol, guaranteeing bacterial viability. We believe that this could constitute a powerful tool for future research about the role of immune and non-immune cells in disease conditions.
The partnership between macrophages and platelets has been addressed in arteries pathologies (Mansilla et al., 2008; Mehrpouri et al., 2019; Huilcaman et al., 2022) and infections (Honda et al., 2009; Feng et al., 2014; Wong et al., 2016; Ali et al., 2017; Carestia et al., 2019; Rossaint et al., 2021). In the context of CDI, the studies are scarce and generally evaluate the importance of the platelet count in the severity of the disease. Some reports propose that an increased platelets number is associated with a better prognosis (Byrn et al., 2012; Lee et al., 2014; Yan et al., 2017; Allegretti et al., 2020; Zhao et al., 2020), while other studies associate this to worse outcomes (Albrich and Rimland, 2007; Pant et al., 2009; Mihăilă et al., 2019; Nseir et al., 2019; Phanchana et al., 2020; Buchrits et al., 2021). In severe cases of C. difficile infection, the intestine fails in its function as a barrier to both intestinal bacterial and toxins, allowing them to reach the portal circulation and developing extra-intestinal CDI (Lowenkron et al., 1996; Libby and Bearman, 2009; Steele et al., 2012; Abid and Bischof, 2019; Olsen et al., 2023). Moreover, vascular changes ligated to C. difficile-induced inflammation have been demonstrated as well as increased colonic vascular permeability and angiogenesis (Kurose et al., 1994; Huang et al., 2019). Platelets attached to the activated endothelium promote the migration of monocytes and can also modulate the composition of the intimal layer of blood vessels which favors monocytes migration (Huilcaman et al., 2022; Trivigno et al., 2023). With the end to get a better understanding of platelets role on human macrophages responses during CDI, we assessed the intercommunication of platelets with C. difficile and innate immune cells.
Platelets can directly sense microorganisms (Houlihan and Copley, 1946). Platelets can migrate to sites of infection and aggregate with the infiltrating bacteria, in addition to collaborating with the immune system (Gaertner et al., 2017; Shannon, 2021; Trivigno et al., 2023). The ability of platelets to recognize, collect and/or bound pathogens has been described for E. coli (Gaertner et al., 2017; Palankar et al., 2018), S. aureus (Gaertner et al., 2017), L. monocytogenes (Gaertner et al., 2017), B. abortus (Trotta et al., 2018), and even retroviruses (Zucker-Franklin et al., 1990). Then, bacteria sensing by platelets is not restricted to a particular type of microorganism, since both live and inactivated, Gram positive and Gram negative, opsonized or not bacteria can be recognized. In line with these works, we evidenced a direct interaction of platelets with C. difficile. Platelets also act as covercytes, spreading on the foreign microorganism to cover and seal it (White, 2005). No specific molecule for the interaction between platelets and bacteria has been identified. Rather, it has been proposed that physical forces that help the platelets become “sticky” are responsible for platelet-bacteria interaction. Accordingly, we observed changes in platelets shape which could be related to actomyosin system that shapes the spreading and contraction dynamics of platelets. Besides, we described the establishment of monocytes-platelets complexes in fresh blood, which were induced in the presence of the bacteria. In accordance, monocytes-platelets crosstalk has also been observed for tetanus toxoid (Gudbrandsdottir et al., 2013) and B. abortus (Trotta et al., 2018), in sepsis (Gawaz et al., 1995), in Dengue patients (Hottz et al., 2014) and in non-infectious contexts (Weyrich et al., 1996; Weyrich et al., 2005). We also showed that platelets formed complexes with macrophages when co-cultured together both with or without C. difficile (always over 70%). Interestingly, platelets were not only attached to macrophages surface but they also localized intracellularly. In contrast to our findings, Trotta and col. observed that platelets surrounded specifically B. abortus infected monocytes/macrophages (Trotta et al., 2018). On the other hand and in line with us, Carestia and col. identified platelets/macrophages associations both in LPS presence or absence (Carestia et al., 2019). Altogether, the previous reports and our results reveal platelets versatility, acting both as pathogens patrollers and as links to the immune system.
Platelets and platelets-secreted PAF induce the endocytosis of B. abortus (Lee et al., 2016; Trotta et al., 2018) and S. aureus (Ali et al., 2017) by monocytes and macrophages. In line with this, we demonstrated that human platelets promote C. difficile internalization. It has been proposed that platelets act as carriers of B. abortus, delivering bacteria to monocytes/macrophages (Trotta et al., 2018). Unlike this report, we found an increment in macrophages uptake capacity when platelets were pre-incubated with C. difficile and then added to macrophages culture as well as when platelets were cultured with C. difficile and macrophages together. These results suggest that platelets modulate macrophages ability rather than being transporters of C. difficile. Our experiments with live NAP1/BI/027, live CD160 or CDH plus the NAP1/BI/027 secretome showed no differences when comparing with CDH stimulation, suggesting that the bacteria are not releasing factors that modify the interactions between macrophages and platelets. The obtained results might us speculate that platelets-macrophages physical contact is required to enhance C. difficile internalization. Nevertheless, we cannot rule out the possibility that platelets are releasing soluble factors that activate macrophages in a very early way. Although the underlying mechanisms may differ, the outcome is the same: the enhancement of the uptake potential.
To date, it seems that platelets participation against bacteria contribute to develop a protective response as well as to spread infection. Platelets were proposed as rapid defenders against infections mediated by blood-borne pathogens such as B. cereus and S. aureus (Wong et al., 2016; Wuescher et al., 2016) and a beneficial role for the host has also been suggested in Brucellosis (Trotta et al., 2018; Trotta et al., 2020). On the contrary, S. pyogenes dissemination has been related to the presence of platelets (Kahn et al., 2013). Our confocal micrographs show what appears to be processed C. difficile in the presence of platelets, suggesting that the interaction of platelets with macrophages could lead to bacteria clearance. Nevertheless, whether the bacteria are destroyed and whether platelets could promote C. difficile elimination deserve further investigation.
Different mechanisms can be explored to internalize pathogens. Macropinocytosis, a clathrin-independent endocytic process that internalizes extracellular fluid, nutrients and antigens, has been involved in the uptake of several infectious agents by a broad variety of cell types (Young et al., 1989; Watarai et al., 2001; Niebuhr et al., 2002; Watarai et al., 2002; Mercer and Helenius, 2009; García-pérez et al., 2012; de Carvalho et al., 2015; Nam et al., 2017; Chang et al., 2021). C. difficile seems not to be an exception since here we showed that only amiloride (an ion exchange inhibitor) reduced the percentage of C. difficile-internalizing macrophages. Amiloride is a selective Na+/H+ antiport inhibitor but how it inhibits macropinocytosis is not yet fully understood. Some proteins of Rho family GTPases (e.g. Rac1 and Cdc42) have been implicated during macropinocytosis (Koivusalo et al., 2010). PLC-PKC-Nox2 pathway has also been described to regulate macropinosome formation, membrane ruffling and fluid phase uptake (Espinal et al., 2022) while Glycogen synthase kinase 3 (GSK3) was proposed as negative regulator by altering Wnt-β-catenin signaling (Tejeda-muñoz et al., 2019; Albrecht et al., 2020). When amiloride was added in the presence of platelets, the reduction in the internalization capacity did not reach basal levels, suggesting that other mechanisms different to macropinocytosis could have a role in C. difficile uptake. Likewise, this could also indicate that the presence of platelets per se could decrease the effect of amiloride exerted on macrophages. Importantly, macrophages can play constitutive macropinocytosis, which could serve as an ideal means for delivering C. difficile antigens to intracellular PRRs. This is especially relevant at sites of continuous contact with non-self material such as the gut (Canton, 2018), highlighting the relevance of macropinocytosis during CDI.
To the best of our knowledge, this is the first study elucidating the role of human macrophages and platelets in the immune response against C. difficile. Although our assays were performed in vitro, they provide the basis for proposing platelets as sentinels and modulators of the immune response during CDI. Conventionally, endocytic processes are efficient ways to eliminate invading pathogens, but pathogens can also develop strategies to perpetuate the infection. So many questions still required to be answered. Is C. difficile destroyed within macrophages? Are platelets promoters of macrophages microbicidal functions? or do they induce a C. difficile reservoir? Is C. difficile processed and presented to activate T cells? Can platelets directly eliminate C. difficile? To unravel these, further studies are required.
In conclusion, we evidenced that vegetative form of C. difficile is internalized by human macrophages. Platelets could directly interact with monocytes and macrophages as well as with bacteria. Moreover, C. difficile induced the establishment of monocytes-platelets complexes. Platelets also modulated C. difficile uptake mediated by macrophages, enhancing the internalization capacity of the phagocytes. We finally determined that the uptake of C. difficile was mainly mediated by macropinocytosis.
These results highlight the role of innate immune cells in CDI, describing new cellular mechanisms involved in the internalization of C. difficile. Furthermore, we put in discussion the importance of macropinocytosis in pathogens sensing, a role that is ordinarily underappreciated in the surveillance mechanisms of innate immune cells.
Data availability statement
The original contributions presented in the study are included in the article/Supplementary Material. Further inquiries can be directed to the corresponding authors.
Ethics statement
The studies involving humans were approved by Ethics Committee of UNNOBA (COENOBA). The studies were conducted in accordance with the local legislation and institutional requirements. The participants provided their written informed consent to participate in this study.
Author contributions
AB and VP conceived and designed the experiments. AB performed the experiments with RHDP contribution. AB, and FF analyzed the data. PB and RHDP helped with the discussion and the experimental design. AB and VP wrote the first draft of the manuscript. All authors contributed to manuscript reading and revising and approved the submitted version.
Funding
This work was supported by Universidad Nacional del Noroeste de la Provincia de Buenos Aires (grant numbers SIB 0618/2019, SIB 2113/2022 and “Proyectos de Investigación Interdisciplinarios de la UNNOBA” Res. CS 2190/2022, to VP). Agencia Nacional de Promoción Cientí́fica y Tecnológica, Fondo para la Investigación Científica y Tecnológica (ANPCyT-FONCyT, grant numbers PICT A 2017-1896 and PICT-2021-I-A-01119 to VP; PICT 2018-03084 I B to RHDP and PICT-2021-I-INVI-00584 to AB). UNNOBA-FONCyT (grant number PICTO 2019-00007 to RHDP and VP). Consejo Nacional de Investigaciones Científicas y Técnicas (CONICET, grant number PIP 2021 11220200103137CO to VP and RHDP) AB is a postdoctoral fellow from CONICET. FF is a member of the Support Staff for Research and Development from CONICET. RHDP, PB, and VP are researchers from CONICET.
Acknowledgments
The following reagent was obtained through BEI Resources, NIAID, NIH: Peptoclostridium difficile, Strain CD160, NR-43516. We thank Dr. Mercedes Fuertes and Dr. Laura Alaniz for kindly providing us with some endocytosis inhibitors. We also thank Lucia Romano, Natalia Menite and Gastón Villafañe for their technical assistance in blood draw, platelet count and flow cytometry assays. We acknowledge Diego Ruggeri and Monica Machaín for their help with C. difficile microbiological culture. Finally, we acknowledge Alexis García Iglesias for helping with Supplementary Image 4D design and Dr. Martin Estermann for his insightful discussion on this work.
Conflict of interest
The authors declare that the research was conducted in the absence of any commercial or financial relationships that could be construed as a potential conflict of interest.
Publisher’s note
All claims expressed in this article are solely those of the authors and do not necessarily represent those of their affiliated organizations, or those of the publisher, the editors and the reviewers. Any product that may be evaluated in this article, or claim that may be made by its manufacturer, is not guaranteed or endorsed by the publisher.
Supplementary material
The Supplementary Material for this article can be found online at: https://www.frontiersin.org/articles/10.3389/fcimb.2023.1252509/full#supplementary-material
References
Abid, H., Bischof, E. (2019). An unusual presentation of severe sepsis due to clostridium difficile enteritis. Cureus 11, 10–12. doi: 10.7759/cureus.4162
Albrecht, L. V., Tejeda-Muñoz, N., Bui, M. H., Cicchetto, A. C., Di Biagio, D., Colozza, G., et al. (2020). GSK3 inhibits macropinocytosis and lysosomal activity through the wnt destruction complex machinery. Cell Rep. 32, 107973. doi: 10.1016/j.celrep.2020.107973
Albrich, W. C., Rimland, D. (2007). Clostridium difficile: association with thrombocytosis and leukocytosis. South. Med. J. 100, 149–151. doi: 10.1097/SMJ.0b013e31802ea5ac
Ali, R. A., Wuescher, L. M., Dona, K. R., Worth, R. G. (2017). Platelets Mediate Host Defense against Staphylococcus aureus through Direct Bactericidal Activity and by Enhancing Macrophage Activities. J. Immunol. 198, 344–351. doi: 10.4049/jimmunol.1601178
Allegretti, J. R., Marcus, J., Storm, M., Sitko, J., Kennedy, K., Gerber, G. K., et al. (2020). Clinical predictors of recurrence after primary clostridioides difficile infection: A prospective cohort study. Dig. Dis. Sci. 65, 1761–1766. doi: 10.1007/s10620-019-05900-3
Bain, C. C., Mowat, A. M. (2014a). Macrophages in intestinal homeostasis and inflammation. Immunologica 260, 102–117. doi: 10.1111/imr.12192
Bain, C. C., Mowat, A. M. I. (2014b). The monocyte-macrophage axis in the intestine. Cell. Immunol. 291, 41–48. doi: 10.1016/j.cellimm.2014.03.012
Balassiano, I. T., Yates, E. A., Domingues, R. M. C. P., Ferreira, E. O. (2016). Clostridium difficile : a problem of concern in developed countries and still a mystery in Latin America. J. Med. Microbiol. 61, 169–179. doi: 10.1099/jmm.0.037077-0
Barbero, A. M., Trotta, A., Genoula, M., Hernández Del Pino, R. E., Estermann, M. A., Celano, J., et al. (2021). SLAMF1 signaling induces Mycobacterium tuberculosis uptake leading to endolysosomal maturation in human macrophages. J. Leukoc. Biol. 109, 257–273. doi: 10.1002/JLB.4MA0820-655RR
Brazier, J. S. (1998). The epidemiology and typing of Clostridium difficile. J. Antimicrob. Chemother. 41, 47–57. doi: 10.1093/jac/41.suppl_3.47
Buchrits, S., Gafter-Gvili, A., Bishara, J., Atamna, A., Ayada, G., Eynath, Y., et al. (2021). The importance of abnormal platelet count in patients with clostridioides difficile infection. J. Clin. Med. 10, 2957. doi: 10.3390/jcm10132957
Burke, K. E., Lamont, J. T. (2014). Clostridium difficile infection : A worldwide disease. Gut Liver 8, 1–6. doi: 10.5009/gnl.2014.8.1.1
Byrn, J. C., Maun, D. C., Gingold, D. S., Baril, D. T., Ozao, J. J., Divino, C. M. (2012). Predictors of mortality after colectomy for fulminant clostridium difficile colitis. Med. Hist. 143, 150–154. doi: 10.1001/archsurg.2007.46
Canton, J. (2018). Macropinocytosis : new insights into its underappreciated role in innate immune cell surveillance. Front. Immunol. 9. doi: 10.3389/fimmu.2018.02286
Carestia, A., Mena, H. A., Olexen, C. M., Juan M. Ortiz Wilczynski, S. N., Errasti, A. E., Gomez, R. M., et al. (2019). Platelets promote macrophage polarization toward pro-inflammatory phenotype and increase survival of septic mice. Cell Rep. 28, 896–908. doi: 10.1016/j.celrep.2019.06.062
CDC. (2012). Centers for Disease Control and Prevention (CDC). Vital signs: preventing Clostridium difficile infections. Morb. Mortal. Wkly. Rep. 61, 157–162.
Center for Disease Control and Prevention. (2019). Antibiotic resistance threats in the United States 2019. Cdc 10, 1–150. doi: 10.1186/s13756-020-00872-w
Chang, Y., Enninga, J., Stévenin, V. (2021). New methods to decrypt emerging macropinosome functions during the host – pathogen crosstalk. Cell. Immunol. 23, 1–12. doi: 10.1111/cmi.13342
Chen, X., Yang, X., de Anda, J., Huang, J., Li, D., Xu, H., et al. (2020). Clostridioides difficile toxin A remodels membranes and mediates DNA entry into cells to activate toll-like receptor 9 signaling. Gastroenterology 159, 2181–2192.e1. doi: 10.1053/j.gastro.2020.08.038
Chitnis, A. S., Holzbauer, S. M., Belflower, R. M., Winston, L. G., Bamberg, W. M., Lyons, C., et al. (2013). Epidemiology of community-associated Clostridium difficile infectio through 2011. JAMA Intern. Med. 173, 1359–1367. doi: 10.1001/jamainternmed.2013.7056
Collins, L. E., Lynch, M., Marszalowska, I., Kristek, M., Rochfort, K., O’Connell, M., et al. (2014). Surface layer proteins isolated from Clostridium difficile induce clearance responses in macrophages. Microbes Infect. 16, 391–400. doi: 10.1016/j.micinf.2014.02.001
de Carvalho, T. M. U., Barrias, E., de Souza, W. (2015). Macropinocytosis: A pathway to protozoan infection. Front. Physiol. 6. doi: 10.3389/fphys.2015.00106
Elliott, B., Androga, G. O., Knight, D. R., Thomas, V. (2017). Clostridium difficile infection: Evolution, phylogeny and molecular epidemiology. Infect. Genet. Evol. 49, 1–11. doi: 10.1016/j.meegid.2016.12.018
Espinal, E. R., Matthews, T., Holder, B. M., Bee, O. B., Humber, G. M., Brook, C. E., et al. (2022). Group B streptococcus-induced macropinocytosis contributes to bacterial invasion of brain endothelial cells. Pathogens 11, 474. doi: 10.3390/pathogens11040474
Feng, Y., Dorhoi, A., Mollenkopf, H. J., Yin, H., Dong, Z., Mao, L., et al. (2014). Platelets direct monocyte differentiation into epithelioid-like multinucleated giant foam cells with suppressive capacity upon mycobacterial stimulation. J. Infect. Dis. 210, 1700–1710. doi: 10.1093/infdis/jiu355
Gaertner, F., Ahmad, Z., Rosenberger, G., Fan, S., Nicolai, L., Busch, B., et al. (2017). Migrating platelets are mechano-scavengers that collect and bundle bacteria. Cell 171, 1368–1382.e23. doi: 10.1016/j.cell.2017.11.001
García-Pérez, B. E., De la Cruz-López, J. J., Castañeda-Sánchez, J. I., Muñóz-Duarte, A. R., Hernández-Pérez, A. D., Villegas-Castrejón, H., et al. (2012). Macropinocytosis is responsible for the uptake of pathogenic and non-pathogenic mycobacteria by B lymphocytes (Raji cells). BMC Microbiol. 12, 1–14. doi: 10.1186/1471-2180-12-246
Gawaz, M., Fateh-Moghadam, S., Pilz, G., Gurland, H.-J., Werdan, K. (1995). Platelet activation and interaction with leucocytes in patients with sepsis or multiple organ failure. Eur. J. Clin. Invest. 25, 843–851. doi: 10.1111/j.1365-2362.1995.tb01694.x
Gudbrandsdottir, S., Hasselbalch, H. C., Nielsen, C. H. (2013). Activated platelets enhance IL-10 secretion and reduce TNF-α Secretion by monocytes. J. Immunol. 191, 4059–4067. doi: 10.4049/jimmunol.1201103
Gupta, A., Khanna, S. (2014). Community-acquired clostridium difficile infection: An increasing public health threat. Infect. Drug Resist. 7, 63–72. doi: 10.2147/IDR.S46780
Hernández Del Pino, R. E., Barbero, A. M., Español, L.Á., Morro, L. S., Pasquinelli, V. (2020). The adaptive immune response to Clostridioides difficile: A tricky balance between immunoprotection and immunopathogenesis. J. Leukoc. Biol. 109 (1), 195–210. doi: 10.1002/JLB.4VMR0720-201R
Honda, S., Saito, M., Dimaano, E. M., Morales, P. A., Alonzo, M. T. G., Suarez, L. A. C., et al. (2009). Increased phagocytosis of platelets from patients with secondary dengue virus infection by human macrophages. Am. J. Trop. Med. Hyg. 80, 841–845. doi: 10.4269/ajtmh.2009.80.841
Hottz, E. D., Medeiros-de-Moraes, I. M., Vieira-de-Abreu, A., de Assis, E. F., Vals-de-Souza, R., Castro-Faria-Neto, H. C., et al. (2014). Platelet activation and apoptosis modulate monocyte inflammatory responses in dengue. J. Immunol. 193, 1864–1872. doi: 10.4049/jimmunol.1400091
Houlihan, R. B., Copley, A. L. (1946). The adhesion of rabbit platelets to bacteria. J. Bacteriol. 52, 439–448. doi: 10.1128/jb.52.4.439-448.1946
Huang, J., Kelly, C. P., Bakirtzi, K., Gálvez, J. A. V., Lyras, D., Mileto, S. J., et al. (2019). Clostridium difficile toxins induce VEGF-A and vascular permeability to promote disease pathogenesis. Nat. Microbiol. 4, 269–279. doi: 10.1038/s41564-018-0300-x.Clostridium
Huilcaman, R., Venturini, W., Fuenzalida, L., Cayo, A., Segovia, R., Valenzuela, C., et al. (2022). Platelets, a key cell in inflammation and atherosclerosis progression. Cells 11, 1–11. doi: 10.3390/cells11061014
Kahn, F., Hurley, S., Shannon, O. (2013). Platelets promote bacterial dissemination in a mouse model of streptococcal sepsis. Microbes Infect. 15, 669–676. doi: 10.1016/j.micinf.2013.05.003
Klinger, M. H. F. (1997). Platelets and inflammation. Anat. Embryol. 196, 1–11. doi: 10.1007/s004290050075
Koivusalo, M., Welch, C., Hayashi, H., Scott, C. C., Kim, M., Alexander, T., et al. (2010). Amiloride inhibits macropinocytosis by lowering submembranous pH and preventing Rac1 and Cdc42 signaling. J. Cell Biol. 188, 547–563. doi: 10.1083/jcb.200908086
Kurose, I., Wolf, R., Granger, D. N., Kurose, I., Pothoulakis, C., Lamont, J. T., et al. (1994). Clostridium difficile toxin A-induced microvascular dysfunction. Role of histamine. J. Clin. Invest. 94, 1919–1926. doi: 10.1172/JCI117542
Lam, F. W., Vijayan, K. V., Rumbaut, R. E., Plaza, O. B., Plaza, O. B., Baylor, O. (2015). Platelets and their interactions with other immune cells Fong. Compr. Physiol. 5, 1265–1280. doi: 10.1002/cphy.c140074.Platelets
Lee, D. Y., Chung, E. L., Guend, H., Whelan, R. L., Wedderburn, R. V., Rose, K. M. (2014). Predictors of mortality after emergency colectomy for Clostridium difficile colitis: An analysis of ACS-NSQIP. Ann. Surg. 259, 148–156. doi: 10.1097/SLA.0b013e31828a8eba
Lee, J. J., Simborio, H. L., Reyes, A. W. B., Hop, H. T., Arayan, L. T., Lee, H. J., et al. (2016). Influence of platelet-activating factor receptor (PAFR) on Brucella abortus infection: Implications for manipulating the phagocytic strategy of B. abortus. BMC Microbiol. 16, 1–14. doi: 10.1186/s12866-016-0685-8
Legaria, M. C., Lumelsky, G., Rosetti, S. (2003). Clostridium difficile-associated diarrhea from a general hospital in Argentina. Anaerobe 9, 113–116. doi: 10.1016/S1075-9964(03)00088-X
Libby, D. B., Bearman, G. (2009). Bacteremia due to Clostridium difficile-review of the literature. Int. J. Infect. Dis. 13, e305–e309. doi: 10.1016/j.ijid.2009.01.014
Liu, Y., Chang, Y., Chen, L., Su, P., Ko, W., Tsai, Y.-S., et al. (2018). The ATP-P2X 7 signaling axis is an essential sentinel for intracellular clostridium difficile pathogen - induced inflammasome activation. Front. Cell. Infect. Microbiol. 8. doi: 10.3389/fcimb.2018.00084
Lowenkron, S. E., Waxner, J., Khullar, P., Ilowite, J. S., Niederman, M. S., Fein, A. M. (1996). Clostridium difficile infection as a cause of severe sepsis. Intensive Care Med. 22, 990–994. doi: 10.1007/BF02044130
Lynch, T., Chong, P., Zhang, J., Hizon, R., Du, T., Graham, M. R., et al. (2013). Characterization of a stable, metronidazole-resistant clostridium difficile clinical isolate. PloS One 8, e53757. doi: 10.1371/journal.pone.0053757
Mansilla, S., Boulaftali, Y., Venisse, L., Arocas, V., Meilhac, O., Michel, J. B., et al. (2008). Macrophages and platelets are the major source of protease nexin-1 in human atherosclerotic plaque. Arterioscler. Thromb. Vasc. Biol. 28, 1844–1850. doi: 10.1161/ATVBAHA.108.171389
Maouia, A., Rebetz, J., Kapur, R., Semple, J. W. (2020). The immune nature of platelets revisited amal. Transfus. Med. Rev. 34, 209–220. doi: 10.1016/j.tmrv.2020.09.005
McDonald, L. C., Coignard, B., Dubberke, E., Song, X., Horan, T., Kutty, P. K. (2007). Recommendations for surveillance of Clostridium difficile-associated disease. Infect. Control Hosp. Epidemiol. 28, 140–145. doi: 10.1086/511798
Mehrpouri, M., Bashash, D., Mohammadi, M. H., Gheydari, M. E., Satlsar, E. S., Hamidpour, M. (2019). Co-culture of platelets with monocytes induced M2 macrophage polarization and formation of foam cells: shedding light on the crucial role of platelets in monocyte differentiation. Turkish J. Haematol. Off. J. Turkish Soc Haematol. 36, 97–105. doi: 10.4274/tjh.galenos.2019.2018.0449
Mercer, J., Helenius, A. (2009). Virus entry by macropinocytosis. Nat. Cell Biol. 11, 510–520. doi: 10.1038/ncb0509-510
Metzendorf, N. G., Lange, L. M., Lainer, N., Schlüter, R., Dittmann, S., Paul, L. S., et al. (2022). Destination and specific impact of different bile acids in the intestinal pathogen clostridioides difficile. Front. Microbiol. 13. doi: 10.3389/fmicb.2022.814692
Mihăilă, R. G., Cătană, C., Olteanu, A. L., Bîrluţiu, V., Sălcudean, C., Mihăilă, M. D. (2019). Thrombin generation is increased in patients with Clostridium difficile colitis–a pilot study. Biomarkers 24, 389–393. doi: 10.1080/1354750X.2019.1600021
Modi, N., Gulati, N., Solomon, K., Monaghan, T., Robins, A., Sewell, H. F., et al. (2011). Differential binding and internalization of Clostridium difficile toxin A by human peripheral blood monocytes, neutrophils and lymphocytes. Scand. J. Immunol. 74, 264–271. doi: 10.1111/j.1365-3083.2011.02578.x
Na, Y. R., Stakenborg, M., Seok, S. H., Matteoli, G. (2019). Macrophages in intestinal inflammation and resolution: a potential therapeutic target in IBD. Nat. Rev. Gastroenterol. Hepatol. 16, 531–543. doi: 10.1038/s41575-019-0172-4
Nam, L., Elizabeth, L., Priyanka, M. C. M., Naveed, N., Ward, T. H. (2017). Escherichia coli K1 utilizes host macropinocytic pathways for invasion of brain microvascular endothelial cells. Traffic 18, 733–746. doi: 10.1111/tra.12508
Nibbering, B., Gerding, D. N., Kuijper, E. J., Zwittink, R. D., Smits, W. K., Denny, J. E., et al. (2021). Host immune responses to clostridioides difficile : toxins and beyond. Front. Microbiol. 12. doi: 10.3389/fmicb.2021.804949
Niebuhr, K., Giuriato, S., Pedron, T., Philpott, D. J., Sheetz, M. P., Parsot, C., et al. (2002). Conversion of PtdIns (4,5) P2 into PtdIns (5)P by the S. fexneri effector IpgD reorganizes host cell morphology. EMBO J. 21, 5069–5078. doi: 10.1093/emboj/cdf522
Nseir, W., Khamisy-Farah, R., Amara, A., Farah, R. (2019). The prognostic value of inflammatory markers in clostridium difficile-associated diarrhea. Isr. Med. Assoc. J. 21, 658–661.
Olsen, M. A., Keller, M. R., Stwalley, D., Yu, H., Dubberke, E. R. (2023). Increased incidence and risk of septicemia and urinary tract infection after clostridioides difficile infection. Open Forum Infect. Dis. 10, 1–8. doi: 10.1093/ofid/ofad313
Palankar, R., Kohler, T. P., Krauel, K., Wesche, J., Hammerschmidt, S., Greinacher, A. (2018). Platelets kill bacteria by bridging innate and adaptive immunity via platelet factor 4 and FcγRIIA. J. Thromb. Haemost. 16, 1187–1197. doi: 10.1111/jth.13955
Pant, C., Madonia, P. N., Jordan, P., Manas, K., Bass, P. (2009). Harbingers for Clostridium difficile-associated diarrhea. J. Investig. Med. 57, 40–42. doi: 10.2310/JIM.0b013e318193f513
Paredes-Sabja, D., Cofre-Araneda, G., Brito-Silva, C., Pizarro-Guajardo, M., Sarker, M. R. (2012). Clostridium difficile spore-macrophage interactions: spore survival. PloS One 7, e43635. doi: 10.1371/journal.pone.0043635
Phanchana, M., Phetruen, T., Harnvoravongchai, P., Raksat, P., Ounjai, P., Chankhamhaengdecha, S., et al. (2020). Repurposing a platelet aggregation inhibitor ticagrelor as an antimicrobial against Clostridioides difficile. Sci. Rep. 10, 1–8. doi: 10.1038/s41598-020-63199-x
Ransom, E. M., Williams, K. B., Weiss, D. S., Ellermeier, C. D. (2014). Identification and characterization of a gene cluster required for proper rod shape, cell division, and pathogenesis in Clostridium difficile. J. Bacteriol. 196, 2290–2300. doi: 10.1128/JB.00038-14
Rejman, J., Oberle, V., Zuhorn, I. S., Hoekstra, D. (2004). Size-dependent internalization of particles via the pathways of clathrin-and caveolae-mediated endocytosis. Biochem. J. 377, 159–169. doi: 10.1042/BJ20031253
Rennick, J. J., Johnston, A. P. R., Parton, R. G. (2021). Key principles and methods for studying the endocytosis of biological and nanoparticle therapeutics. Nat. Nanotechnol. 16, 266–276. doi: 10.1038/s41565-021-00858-8
Rossaint, J., Thomas, K., Mersmann, S., Skupski, J., Margraf, A., Tekath, T., et al. (2021). Platelets orchestrate the resolution of pulmonary inflammation in mice by T reg cell repositioning and macrophage education. J. Exp. Med. 218, 1–23. doi: 10.1084/jem.20201353
Saad, G., Azrad, M., Aias, M., Leshem, T., Hamo, Z. (2023). The effect of different C. difficile MLST strains on viability and activity of macrophages. Heliyon 9, e13846. doi: 10.1016/j.heliyon.2023.e13846
Scherlinger, M., Richez, C., Tsokos, G. C., Boilard, E., Blanco, P. (2023). The role of platelets in immune- mediated inflammatory diseases. Nat. Rev. Immunol. 23, 495–510. doi: 10.1038/s41577-023-00834-4
Shannon, O. (2021). The role of platelets in sepsis. Res. Pract. Thromb. Haemost. 5, 27–37. doi: 10.1002/rth2.12465
Solomon, K., Webb, J., Ali, N., Robins, R. A., Mahida, Y. R. (2005). Monocytes are highly sensitive to clostridium difficile toxin A-induced apoptotic and nonapoptotic cell death. Infect. Immun. 73, 1625–1634. doi: 10.1128/IAI.73.3.1625-1634.2005
Sousa De Almeida, M., Susnik, E., Drasler, B., Taladriz-Blanco, P., Petri-Fink, A., Rothen-Rutishauser, B. (2021). Understanding nanoparticle endocytosis to improve targeting strategies in nanomedicine. Chem. Soc Rev. 50, 5397–5434. doi: 10.1039/d0cs01127d
Steele, J., Chen, K., Sun, X., Zhang, Y., Wang, H., Tzipori, S., et al. (2012). Systemic dissemination of Clostridium difficile toxins A and B is associated with severe, fatal disease in animal models. J. Infect. Dis. 205, 384–391. doi: 10.1093/infdis/jir748
Tejeda-muñoz, N., Albrecht, L. V., Bui, M. H., De Robertis, E. M. (2019). Wnt canonical pathway activates macropinocytosis and lysosomal degradation of extracellular proteins. PNAS 116, 10402–10411. doi: 10.1073/pnas.1903506116
Trivigno, S. M. G., Guidetti, G. F., Barbieri, S. S., Zara, M. (2023). Blood platelets in infection : the multiple roles of the platelet signalling machinery. Int. J. Mol. Sci. 24, 1–18. doi: 10.3390/ijms24087462
Trotta, A., Milillo, M. A., Serafino, A., Castillo, L. A., Birnberg Weiss, F., Delpino, M. V., et al. (2020). Brucella abortus–infected platelets modulate the activation of neutrophils. Immunol. Cell Biol. 98, 743–756. doi: 10.1111/imcb.12373
Trotta, A., Velásquez, L. N., Milillo, M. A., Victoria, D. M., M., R. A., Landoni, V. I., et al. (2018). Platelets Promote Brucella abortus Monocyte invasion by establishing complexes With Monocytes. Front. Immunol. 9. doi: 10.3389/fimmu.2018.01000
Trzeciak-Ryczek, A., Tokarz-Deptuła, B., Deptuła, W. (2013). Platelets - An important element of the immune system. Pol. J. Vet. Sci. 16, 407–413. doi: 10.2478/pjvs-2013-0058
Vohra, P., Poxton, I. R. (2012). Induction of cytokines in a macrophage cell line by proteins of Clostridium difficile. FEMS Immunol. Med. Microbiol. 65, 96–104. doi: 10.1111/j.1574-695X.2012.00952.x
Watarai, M., Derre, I., Kirby, J., Growney, J. D., Dietrich, W. F., Isberg, R. R. (2001). Legionella pneumophila is internalized by a macropinocytotic uptake pathway controlled by the dot/icm system and the mouse lgn1 locus. J. Exp. Med. 194, 1081–1095. doi: 10.1084/jem.194.8.1081
Watarai, M., Makino, S., Fujii, Y., Okamoto, K., Shirahata, T. (2002). Modulation of Brucella -induced macropinocytosis by lipid rafts mediates intracellular replication. Cell. Microbiol. 4, 341–355. doi: 10.1046/j.1462-5822.2002.00195.x
Weyrich, A. S., Denis, M. M., Kuhlmann-Eyre, J. R., Spencer, E. D., Dixon, D. A., Marathe, G. K., et al. (2005). Dipyridamole selectively inhibits inflammatory gene expression in platelet-monocyte aggregates. Circulation 111, 633–642. doi: 10.1161/01.CIR.0000154607.90506.45
Weyrich, A. S., Elstad, M. R., McEver, R. P., McIntyre, T. M., Moore, K. L., Morrissey, J. H., et al. (1996). Activated platelets signal chemokine synthesis by human monocytes. J. Clin. Invest. 97, 1525–1534. doi: 10.1172/JCI118575
White, J. G. (2005). Platelets are covercytes, not phagocytes: Uptake of bacteria involves channels of the open canalicular system. Platelets 16, 121–131. doi: 10.1080/09537100400007390
Wong, C. H. Y., Jenne, C. N., Petri, B., Chrobok, N. L. (2016). Nucleation of platelets with bloodborne pathogens on Kupffer cell precedes other innate immunity and contributes to bacterial clearance. Nat. Immunol. 14, 785–792. doi: 10.1038/ni.2631.Nucleation
Wuescher, L. M., Takashima, A., Worth, R. G. (2016). A novel conditional platelet depletion mouse model reveals the importance of platelets in protection against S. aureus bacteremia. J. Thromb. Haemost. 13, 303–313. doi: 10.1111/jth.12795.A
Yan, D., Chen, Y., Lv, T., Huang, Y., Yang, J., Li, Y., et al. (2017). Clostridium difficile colonization and infection in patients with hepatic cirrhosis. J. Med. Microbiol. 66, 1483–1488. doi: 10.1099/jmm.0.000596
Yeaman, M. R. (1997). The role of platelets in antimicrobial host defense michael. Clin. Infect. Dis. 25, 951–968. doi: 10.1086/516120
Young, V. B., Falkow, S., Schoolnik, G. K. (1989). The invasin protein of yersinia enterocolitica internalization of invasin-bearing bacteria by eukaryotic cells is associated with reorganization of the cytoskeleton. J. Cell Biol. 116, 197–207. doi: 10.1083/jcb.116.1.197
Zhang, S., Palazuelos-Munoz, S., Balsells, E. M., Nair, H., Chit, A., Kyaw, M. H. (2016). Cost of hospital management of Clostridium difficile infection in United States-a meta-analysis and modelling study. BMC Infect. Dis. 16, 447. doi: 10.1186/s12879-016-1786-6
Zhao, L., Luo, Y., Bian, Q., Wang, L., Ye, J., Song, X., et al. (2020). High-level resistance of toxigenic clostridioides difficile genotype to macrolide-lincosamide-streptogramin B in community acquired patients in Eastern China. Infect. Drug Resist. 13, 171–181. doi: 10.2147/IDR.S238916
Keywords: C. difficile, macrophages, platelets, macropinocytosis, uptake
Citation: Barbero AM, Hernández Del Pino RE, Fuentes F, Barrionuevo P and Pasquinelli V (2024) Platelets promote human macrophages-mediated macropinocytosis of Clostridioides difficile. Front. Cell. Infect. Microbiol. 13:1252509. doi: 10.3389/fcimb.2023.1252509
Received: 04 July 2023; Accepted: 11 December 2023;
Published: 05 January 2024.
Edited by:
Pablo Fernando Pérez, National Scientific and Technical Research Council (CONICET), ArgentinaReviewed by:
Zhuo Ma, Albany College of Pharmacy and Health Sciences, United StatesDiane Bimczok, Montana State University, United States
Copyright © 2024 Barbero, Hernández Del Pino, Fuentes, Barrionuevo and Pasquinelli. This is an open-access article distributed under the terms of the Creative Commons Attribution License (CC BY). The use, distribution or reproduction in other forums is permitted, provided the original author(s) and the copyright owner(s) are credited and that the original publication in this journal is cited, in accordance with accepted academic practice. No use, distribution or reproduction is permitted which does not comply with these terms.
*Correspondence: Virginia Pasquinelli, virpasquinelli@gmail.com; Angela María Barbero, barberoangelamaria@gmail.com