Environmentally sustainable implementations of two-dimensional nanomaterials
- 1Civil and Environmental Engineering, Washington State University, Pullman, WA, United States
- 2Departments of Materials Science and Engineering, Chemistry and Medicine, Northwestern University, Evanston, IL, United States
- 3National Exposure Research Laboratory, United States Environmental Protection Agency, Athens, GA, United States
Rapid advancement in nanotechnology has led to the development of a myriad of useful nanomaterials that have novel characteristics resulting from their small size and engineered properties. In particular, two-dimensional (2D) materials have become a major focus in material science and chemistry research worldwide with substantial efforts centered on their synthesis, property characterization, and technological, and environmental applications. Environmental applications of these nanomaterials include but are not limited to adsorbents for wastewater and drinking water treatment, membranes for desalination, and coating materials for filtration. However, it is also important to address the environmental interactions and implications of these nanomaterials in order to develop strategies that minimize their environmental and public health risks. Towards this end, this review covers the most recent literature on the environmental implementations of emerging 2D nanomaterials, thereby providing insights into the future of this fast-evolving field including strategies for ensuring sustainable development of 2D nanomaterials.
1 Introduction
Nanomaterials are defined as having at least one dimension of approximately 1–100 nm and are known for having unique and size-dependent optical, mechanical, electrical, and chemical properties. While relatively new, nanomaterials are entering the commercialization stage in many industries, including the electronic, magnetic, biomedical, pharmaceutical, cosmetic, energy, and paint industries, as well as for coatings and catalytic applications (Novoselov et al., 2012; Chung et al., 2013; Kemp et al., 2013). Two-dimensional (2D) nanomaterials are crystalline materials consisting of atomically-thin layers that possess strong ionic or covalent in-plane bonding while being stacked together by interlayer van der Waals bonding. There are several unique characteristics of 2D nanomaterials compared to their counterparts with different dimensionality and which makes them different from zero-dimensional (0D) nanoparticles, one-dimensional (1D) nanowires, and three-dimensional (3D) networks.
1.1 Why two-dimensional (2D) nanomaterials?
2D nanomaterials are of particular interest due to their exceptionally high specific surface area, making their surface properties dominant compared to their bulk counterparts. This high specific surface area makes 2D nanomaterials promising building blocks to construct functional composites as well as used as reinforced fillers to strengthen the resultant composites (Zhang, 2015). Moreover, these high aspect ratio sheet-like solids come in a wide array of chemical compositions, crystal phases, and physical forms, and are anticipated to enable a host of future technologies in areas that include electronics, sensors, coatings, barriers, energy storage and conversion, and biomedicine (Bianco et al., 2013; Kaul, 2014; Kalantar-zadeh et al., 2015; Cao et al., 2016).
With atomic-scale thicknesses, 2D nanomaterials possess maximum mechanical flexibility and optical transparency, making them promising for the fabrication of highly flexible and transparent electronic/optoelectronic devices (Geim and Novoselov, 2009). Moreover, the large lateral size and atomic thickness allow 2D nanomaterials to be highly favorable for many surface-active applications, such as electrocatalysis, photocatalysis, organic catalysis, and supercapacitors (An et al., 2016; Chen et al., 2019; Wu et al., 2019; Zhao et al., 2020a; Wang and Zhao, 2020; Chang et al., 2021; Ng et al., 2021).
Another attractive feature of 2D nanomaterials is that their electronic structures are highly sensitive to chemical modification, external electric fields, mechanical deformation, doping, and adsorption of other molecules or materials, which makes it easier to modify their electronic properties in a desired manner (Geim and Novoselov, 2009). Through chemical modification and integration into heterostructures, 2D nanomaterials are being integrated into a range of applications including highly conductive electrodes, planar spintronics, and high-efficiency catalysts (Yu et al., 2013; Kaul, 2014; Saadi et al., 2014).
2D nanomaterials have been extensively studied due to a vast array of unique physicochemical properties, such as high electronic conductivity, magnetic anisotropy, tunable band gap, and surface activity (Novoselov et al., 2004; Wang et al., 2012a; Chhowalla et al., 2013; Nicolosi et al., 2013; Li et al., 2014a; Xu et al., 2014). These properties arise from the quantum confinement of electrons.
The combination of excellent mechanical properties, light transmittance, and electronic properties makes 2D nanomaterials highly attractive in the fabrication of next-generation wearable, highly flexible, and transparent electronic/optoelectronic devices.
However, the synthesis, manufacturing, or application of these 2D nanomaterials can lead to unintended human exposures and environmental releases. These may pose a significant threat to public health and the environment. Even though the toxicity of 2D nanomaterials, their microbial degradation pathways, and their interactions with biological systems have been explored previously (Fojtů et al., 2017), for sustainable development of nanomaterials, it is important to have a better understanding of the fate and transport of these materials in the environment. The responsible development and applications of nanotechnology thus requires a coordinated and sustained research effort to understand and manage the environmental implications and human health risks of 2D nanomaterials.
In this review, literature on some of the emerging 2D nanomaterials (i.e., graphene oxide (GO), Molybdenum Disulfide (MoS2)) are summarized in terms of their environmental implications and a few prospects. By providing an overview of the properties and environmental implementations of 2D nanomaterials, rational strategies can be developed to help guide future sustainable development and safe best practices for the handling and utilization of 2D nanomaterials.
2 Graphene family nanomaterials
Graphene is recognized as the “mother of all graphitic forms,” i.e., the 2D building block of fullerenes, carbon nanotubes, and graphite, and has given rise to the wide range of GFNs studied today (Geim and Novoselov, 2007). Graphene nanomaterials vary in layer number, lateral dimension, surface chemistry, defect density, quality of the individual graphene sheets, composition, and purity. The properties and applications of some commonly used GFNs (Figure 1) have been summarized in Table 1 briefly.
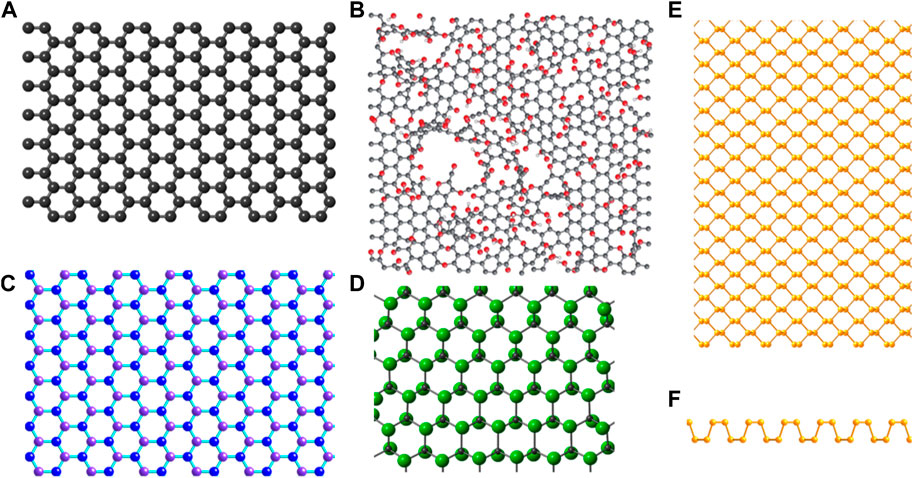
FIGURE 1. The structures of graphene family nanomaterials: (A) graphene, (B) graphene oxide, (C) hexagonal boron nitride, B shown in purple, N in blue, (D) fluorographene, F shown in green, C in gray. Fluorine atoms are distributed in one of two ways on the graphene surface in fluorographene, dubbed the “chair-type” and “boat-type conformations.” Depicted here is fluorographene in the more energetically favorable chair-type conformation. (E) Phosphorene top and (F) side views. (B) Reprinted with permission from Macmillan Publishers Ltd.: Nature Chemistry, (A). Bagri, (C). Mattevi, M. Acik, Y. J. Chabal, M. Chhowalla and V. (B). Shenoy, Nat. Chem., 2010, 2, 581–587, Copyright 2010 (Bagri et al., 2010). All other structures produced by CrystalMaker9.
2.1 Other graphene derivatives and elemental graphene analogues
Compared to the abundant literature on 2D materials like graphene and graphene oxide, the study of other graphene derivatives and elemental graphene analogues is still limited and at an early stage. Predictions and preliminary measurements of their properties confirm that they are complementary to conventional (that is, layered bulk-derived) 2D materials, which highlights that they deserve more attention as well in Tables 2, 3 (Zhang et al., 2017a; Mannix et al., 2017; Molle et al., 2017; Pumera and Sofer, 2017).
3 2D materials beyond graphene
Encouraged by the success and widespread applications of GFNs, researchers have explored other possible 2D structures beyond graphene and its derivatives. Studies with these materials have led to a vast library of 2D materials. (Geim and Grigorieva, 2013). Here, we introduce some of these categories and their relevant attributes.
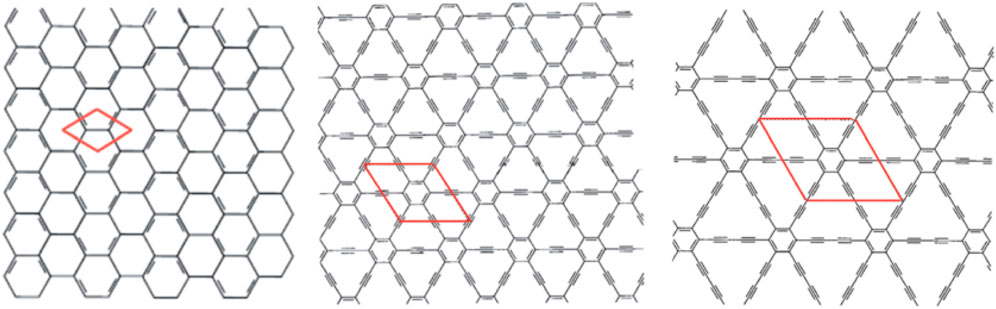
FIGURE 2. Structures of graphene (left), graphyne (middle), and graphdiyne (right). Each red parallelogram represents one unit cell. Reproduced by M. Inagaki and F. Y. Kang, J. Mater. Chem. A, 2014, 2, 13,193–13206, with permission of The Royal Society of Chemistry (Inagaki and Kang, 2014).
4 Environmental implications of graphene family nanomaterials
A broad and detailed understanding of the environmental implications of 2D materials will require knowledge of their release and transport through environmental media, distribution in environmental compartments, chemical and physical transformations, bioaccumulation, and effects on environmental organisms and ecosystems (Deng et al., 2011; Han et al., 2013; Chng et al., 2014; Wang et al., 2015b; Lanphere et al., 2015; Qian et al., 2015; Song et al., 2015). In the following sections, we survey previous work on the environmental implications of GFNs and 2D materials beyond graphene.
4.1 Environmental degradation of GFNs
With a burgeoning number of applications, the release of GFNs into the environment poses the risk of their transformation and degradation into other materials, such as carcinogenic polycyclic aromatic hydrocarbons (PAH) or comparatively benign carbon dioxide (CO2). This risk is particularly affected by their transport, which leads to a wider exposure risk. Thus, it is necessary to gather knowledge on the environmental behavior, fate, and transport of GFNs in the aquatic and terrestrial environments where many factors can influence their composition and behavior. It is especially important to assess their long-term impact in cases where oxidizing species may promote the disintegration of graphene into hazardous materials.
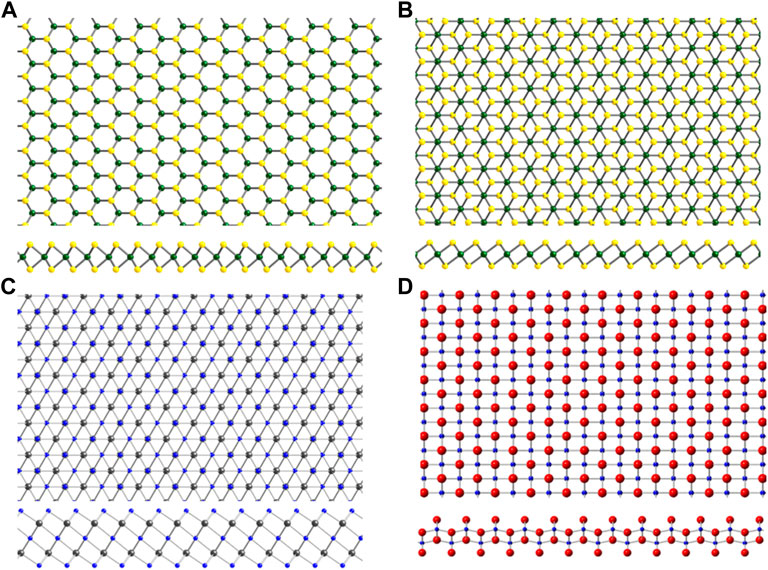
FIGURE 3. Structures of 2D materials that were discovered or have attracted renewed interest after the isolation of graphene. (A) Transition metal dichalcogenide (TMD) 2H crystal structure and (B) TMD 1T crystal structure. Here, transition metal atoms are shown in green, and chalcogen atoms shown in yellow. (C) Ti3C2 crystal structure representative of the MXene family. (D) 2D transition metal oxide Ti0.91O20.36-. Ti shown in blue, C in grey, O in red.
4.1.1 Sunlight-mediated transformations
In some previous studies, it was demonstrated that under UV light irradiation, with or without Fenton reagent (Fe2+/Fe3+/H2O2), GO undergoes photoreduction, and CO2 forms due to photooxidation. These reactions are based on the photoreactions of oxygen-containing functional groups and carbon (Matsumoto et al., 2011; Koinuma et al., 2012; Zhou et al., 2012). Some other studies focusing on the chemical stability of the materials has shown that GO readily photo-reacts under simulated sunlight exposure, forming fragmented photoproducts similar to rGO as well as low molecular-weight species such as polycyclic aromatic hydrocarbons (PAHs) (Figure 4) (Zhou et al., 2012; Bai et al., 2014; Hou et al., 2015). When exposed to sunlight, graphene oxide degradation occurs mainly due to oxygen-containing functional groups on the basal plane through reduction and creation of holes (Shams et al., 2019).
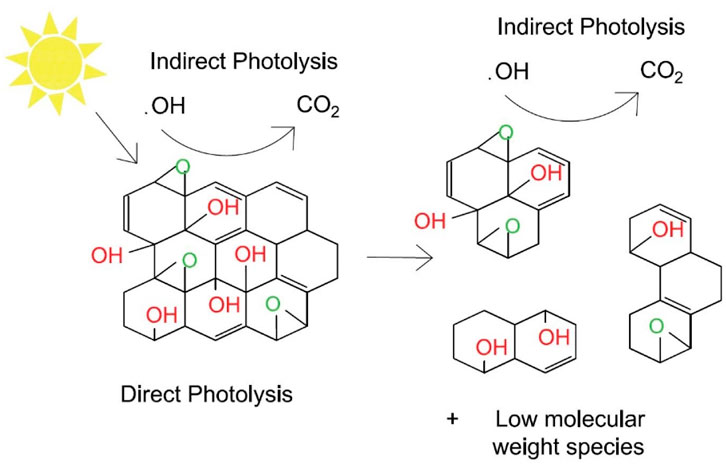
FIGURE 4. Pathways of direct and indirect photolysis of GO under sunlight. Reproduced with permission from W.-C. Hou, I. Chowdhury, D. G. Goodwin, W. M. Henderson, D. H. Fairbrother, D. Bouchard and R. G. Zepp, Environ. Sci. Technol., 2015, 49, 3435–3443. Copyright 2015, American Chemical Society (Hou et al., 2015). Reproduced with permission from Carbon, 110, Wen-Che Hou, W. Matthew Henderson, Indranil Chowdhury, David G.Goodwin, Xiaojun Chang, Sharon Martin, D. Howard Fairbrother, Dermont Bouchard, Richard G. Zepp, The contribution of indirect photolysis to the degradation of graphene oxide in sunlight, 426–437, Copyright 2016, with permission from Elsevier (Hou et al., 2016). Structures produced by AutoCAD 2017.
Similarly, indirect phototransformation of GO presents another pathway of degradation in surface water (Lowry et al., 2012). Varied components of surface water, such as nitrates, minerals, and natural organic matter (NOM), can promote degradation by acting as chromophores and producing hydroxyl radicals, which are strong, non-specific oxidants that react with many nanomaterials in water.
However, the resulting byproducts from GO photodegradation can persist in water for a long period, and have different characteristics than their parent material (Hou et al., 2015). This makes the use of GO difficult especially where they will be susceptible to phtodegradation. Moreover, phototransformation will decrease the deposition rate of GO on many environmental surfaces such as those coated with Suwannee River Humic Acid (SRHA), which could be useful for the removal of GO from the environment. Another study has shown that rGO is less susceptible to photodegradation compared to GO (Shams et al., 2019). Hence, for coating and photocatalytic applications, use of rGO will be better compared to GO.
A study of the environmental instability of few-layer BP in ambient conditions suggests a photo-induced oxidation reaction of BP and degradation in the presence of oxygen absorbed in water (Favron et al., 2014; Ziletti et al., 2015). This degradation is a slower process, taking several hours to days but is dependent upon the thickness of the flakes. The degradation increases as thickness is reduced (Island et al., 2015).
However, these studies were performed in model condition in lab or with only with natural surface water. Whereas, these degradation rates, and by products can alter at different water condition. So more future research could be accomplished using different GFNs in other conditions such as in saline water. Also, oxidation of 2D materials in air, can have significant impact on the functional properties and the behavior of the materials which in result can also impact the degradation (Wang et al., 2017). Graphene usually has excellent oxidation resistance but at temperature higher than 250°C, or their structural defect graphene can play an important role in their oxidation (Liu et al., 2008; Barinov et al., 2009; Chen et al., 2011; Kang et al., 2012).
4.1.2 Microbial transformations
There are some microbes (i.e., E. coli) that can degrade functionalized graphene compounds because graphene oxide acts as a terminal electron acceptor for heterotrophic and environmental bacteria (Salas et al., 2010; Akhavan and Ghaderi, 2012). Model environmental microbes from the genus Shewanella (a metal-reducing bacteria) also include a group of heterotrophic anaerobes that are found in lakes, oceans, marine sediments, and related environments (Hau and Gralnick, 2007). These microbes use different electron acceptors in their respiratory pathway to immobilize toxic metals and have environmental ubiquity, which makes them amenable to reactions with graphitic material. These reactions can further induce the biodegradation of GO, although they are dependent on some external factors. In addition, several enzymes like MPO and HRP can degrade graphene. However, the effectiveness of these enzymes rely on hydrophilicity, colloid stability and surface negative charge (Kurapati et al., 2015; Kurapati et al., 2017). Similar to bacteria one study found the use of fungi for graphene degradation with the help of LiP enzyme (Keli et al., 2018). This knowledge is useful for applications of environmental bacteria in green nanochemistries and for creating high performance nanomaterials (Salas et al., 2010; Wang et al., 2011b). Moreover, using oxidants for chemical degradation of graphene nanomaterials can be toxic to environment and costly. However, future research should also focus on the varied factors like temperature, presence of oxygen, pH etc. On the biodegradation of these nanomaterials. Moreover, the existing biodegradation studies only focused on GO and not on rGO, whose applications are also increasing with time. In addition, which specific enzyme is secreting from microbes and is responsible for the degradation should be studied in more detail.
4.1.3 Disinfectant mediated transformations
Commonly used disinfectants in water distribution and treatment systems are chlorine, monochloramine, chlorine dioxide, ozone, and UV irradiation (Harza, 2005). In the United States, water purification and wastewater disinfection is accomplished almost solely by chlorination techniques. It was hypothesized that chlorine-based disinfectants in the water treatment environment significantly transform and degrade GFNs through oxidation, and that the resulting products, chlorinated GFNs and chlorinated PAHs, have increased mobility in the aquatic environment compared to the parent material (Frutos et al., 2011). Historically, halogenated PAHs are known to be toxic and carcinogenic (Fu et al., 1999). In some study, effect of photochlorination on GO was investigated (Li et al., 2016b; Du et al., 2017). These studies showed that photochlorination decomposes GO to rGO. The studies further showed that changes in oxygen containing functional groups of GO were due to the oxidation of the quinone groups in GO by chlorine, and further oxidation by Cl• and/or ClO• radicals. However, the mechanism of how the addition of functional groups to GFNs affects the toxicity or mobility of the degradation products remains unexplored. Also, how this change will affect aggregation, adsorption, transport, and interactions of GO with other surfaces needs to be investigated.
4.1.4 Photocatalytic transformation
In one study, C3N4/graphene oxide (GO) aerogel was prepared to degrade methyl orange (MO), an organic contaminant, under visible light irradiation to 73% within 5 h in aqueous solution (Wan et al., 2016). In the study, contribution of C3N4/graphene oxide (GO) from adsorption and degradation was distinguished. This result was comparable to another study, where the composite was prepared similarly and MO degradation was noticed (Tong et al., 2015). In both the mentioned studies, the composite showed stable photocatalytic activity for MO degradation after four decomposition cycles. In another study, metal (Fe2+, Zn2+) was incorporated with g-C3N4 for rhodamine B (RhB) degradation. This study also showed that the composite can be regenerated and reused without appreciable loss of RhB degradation activity up to five cycles (Zhu et al., 2010). These results summarizes that g- C3N4 incorporated with other material has higher efficiency in pollutant degradation compared to pure g- C3N4 and shows excellent recyclability (Cheng et al., 2013; Li et al., 2013; Chen et al., 2014a; Zhang et al., 2015b). However, C3N4/GO aerogel has excellent adsorption ability, due to which, it is difficult to distinguish photocatalytic degradation from adsorption. While considering (RhB) degradation, there was no mention about the individual percentage of adsorption and degradation of the material (Zhu et al., 2014). Different synthesis approach of a composite, can result to the formation of a composite with different structure and distinctive properties. Overall it affects the surface area and catalytic activity of the composite (Zhu et al., 2005; Zhu et al., 2007; Li et al., 2014c). Effect of different synthesis techniques should be addressed in pollutant degradation and environmental remediation. Removal of g-C3N4 from the system after adsorption is barely mentioned in the above discussed studies. Even though, C3N4/GO aerogel can be easily separated by filtration from the reaction systems for recycling (Tong et al., 2015), other approach, like in situ methods for removal should be reviewed as well.
4.2 Toxicity of GFNs
Many nanoparticles can generate reactive oxygen species (ROS) due to their redox activity and cause oxidative stress to organisms. Among different nanoparticles, some researchers found that carbon nanotubes and graphene can penetrate plant cells and stimulate phytotoxicity at high doses (Lin and Xing, 2007; Liu et al., 2009; Stampoulis et al., 2009; Ghodake et al., 2010; Begum et al., 2011; Khodakovskaya et al., 2011; Anjum et al., 2013; Lee and Kim, 2014). The hydrophobic property and aggregation tendency of carbon based nanomaterials would enhance their capability to interact with many organic substances (De La Torre-Roche et al., 2013). Accumulation in addition to visible signs of necrotic damage lesions, all indicate an oxidative stress mechanism mediated through the necrotic pathway.
GO exposure can reduce swimming speed and cause settlement inhibition to aquatic organisms (Mesarič et al., 2013). Graphene can penetrate through the plasma membranes due to its sharp edges and cause cell death (Liu et al., 2011b; Begum and Fugetsu, 2013). Furthermore, graphene can significantly interact with cell membrane lipids due to its hydrophobic surface, and cause toxicity (Sanchez et al., 2012). This toxicity, may be due to the loss of membrane integrity, including initial cell deposition on graphene-based materials and membrane stress caused by direct contact with sharp nanosheets (Liu et al., 2011b). Besides concentration, toxicity also depends on the physicochemical properties of graphene, such as the density of the functional groups, size, conductivity, and chemical nature of the reducing agent used for deoxygenation of GO, as well as on the cell types exposed to the materials which needs to be explored further (Gurunathan et al., 2012). Similarly toxicity due to other graphene nanomaterials should also be assessed.
4.3 Aggregation and deposition of GFNs
Aggregation and deposition of GO are dependent on various cations present in the aquatic and soil environments as they affect the surface charges of GO (Bargar et al., 1998; Ren et al., 2014; Duan et al., 2017). Recent studies indicate that GO can resist aggregation in natural and synthetic surface waters and can remain stable for extended periods due to steric repulsio. (Chowdhury et al., 2013; Wu et al., 2013). Figure 5 indicates that GO remains stable in natural surface water, but gets rapidly destabilized in effluent wastewater. Photo-transformed GO are significantly affected by the presence of CaCl2 with hydrodynamic diameter increasing with irradiation time, indicating an increased rate of aggregation (Chowdhury et al., 2015a). The deposition behavior also depends on many other factors, such as the presence of natural organic matter (NOM) (Chowdhury et al., 2015a). Presence of NOM and divalent cations (Ca2+, Mg2+) can bridge with GO functional groups, resulting in GO aggregates that settle from suspension (Chowdhury et al., 2015b). From this, it can be inferred that GO will sediment and may accumulate in biosolids and sludge during the wastewater treatment process. With successive reduction of functional groups, the colloidal stability of GO in water decreases (Chowdhury et al., 2015b; Shams et al., 2019). Deposition of photo-transformed GO on NOM-coated surfaces can reduce remobilization of GO in the aquatic environment (Chowdhury et al., 2015a).
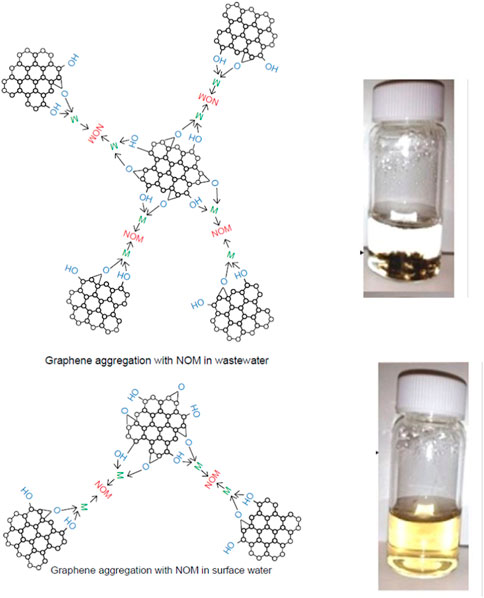
FIGURE 5. Aggregation & Stability of graphene oxide (due to presence of NOM and divalent cations (Ca2+, Mg2+) in surface water and wastewater. Reprinted with permission from I. Chowdhury, M. C. Duch, N. D. Mansukhani, M. C. Hersam and D. Bouchard, Environ. Sci. Technol., 2013, 47, 6288–6296. Copyright 2013, American Chemical Society (Chowdhury et al., 2013). Reprinted with permission from I. Chowdhury, N. D. Mansukhani, L. M. Guiney, M. C. Hersam and D. Bouchard, Environ. Sci. Technol., 2015, 49, 10,886–10893. Copyright 2015, American Chemical Society (Chowdhury et al., 2015b).
4.4 Challenges in synthesis
Chemical vapor deposition, micromechanical exfoliation, epitaxial growth, and chemical reduction techniques are most widely used approach for synthesizing graphene (Gao et al., 2010). However, the existing synthesis approaches requires precise control over their compositions, thicknesses, lateral sizes, crystal phases, doping, defects, strains, vacancies, and surface properties to know the correlations between the structural features and properties. In chemical reduction technique, the use of reductant, usually hydrazine or dimethylhydrazine is highly toxic, which if inhaled by manufacturing workers, could cause serious health issues. The use of toxic reductant and other chemical stabilizers, to prevent aggregation, which are not biocompatible should be avoided. In a study, a “green” reduction technique of graphite oxide to graphene was showed using hydrothermal dehydration (Gao et al., 2010). Graphene of higher quality produced by liquid phase exfoliation of graphite, using solvents such as N,N-dimethylformamide (DMF), N-methyl-2-pyrrolidone etc. Should also be avoided as they are hazardous. Instead reducing sugars, such as glucose, fructose and sucrose could be used to synthesize graphene (Paton et al., 2014; Yi and Shen, 2014). Electrochemical methods to produce graphene also suffers from difficulty, in terms of cost and final product (Chen et al., 2014b; Parvez et al., 2014).
5 Environmental implications of 2D materials beyond graphene
Other 2D nanomaterials beyond GFNs are fast rising components in different industrial processes. Hence, these products have increasing potential to be released in the environment, thus necessitating studies of their environmental implications.
5.1 Transition metal dichalcogenides: Molybdenum disulfide
Among the range of 2D TMDs such as MoS2, WS2, MoSe2, and WSe2, the most research concerning environmental fate and dissolution processes has been conducted on MoS2 (Cheng et al., 2022; Liu et al., 2022; Liu et al., 2023). Hence, this section will also focus primarily on the environmental implications of MoS2.
5.1.1 Sunlight mediated transformation
Many of the metal chalcogenides are stable under ambient conditions but can undergo environmental transformations (Chhowalla et al., 2013). Recent work on few-layer MoS2 shows dissolution over time upon exposure to environmental and biological simulant fluids (Wang et al., 2016b). These soluble products are formed due to photo-induced corrosion processes, where edge sites and defect sites are the primary degradation targets (Parzinger et al., 2015). However, the photodegradation rate of MoS2 has been observed to be slow under reduced oxygen concentration.
Metal phosphorus trichalcogenides can undergo photo-induced degradation or transformation in the environment, which sometimes provides interesting magnetic and ferroelectric properties as well as suitable band gaps for water splitting (Liu et al., 2014c). However, these can lead to the potential release of toxic ions such as Cu, Cd, Ni, or Co (Joy and Vasudevan, 1992; Evans and O’hare, 1994; Westreich et al., 2006; Dresselhaus, 2013; Ruiz-León et al., 2002; Venkataraman et al., 2003).
Decreasing the size of MoS2 to only a few layers (−2–6 nm thick) increases the photocatalytic properties of MoS2 and ROS generation. These effects result from bandgap widening and the diffusion distance shortening for electrons and holes to the material surface. A previous study showed that four types of ROS (O2•−, 1O2, H2O2 and OH•) were present in few-layered vertically aligned MoS2 (FLV MoS2) (Liu et al., 2016). In the same paper, by decreasing the domain size, the bandgap of MoS2 was increased from 1.3 eV (bulk material) to 1.55 eV (few layer MoS2). This enabled the few layer MoS2 to generate ROS successfully (Liu et al., 2016). Similarly, hybrid materials made with MoS2 can have damaging effects due to the oxidative stress caused by ROS (Figure 6). For example, highly photocatalytically efficient MoS2/C3N4 (carbon nitride) heterostructures have a large potential for industrial applications due to their high quantum efficiencies and separation speed of electron−hole pairs (Li et al., 2014d). However, these heterostructures can be degraded by ROS and the resulting degradation products can have toxic effects in the environment. Specifically, multiple reports have explored the photodegradation of MoS2/C3N4 heterostructures (Pan et al., 2012; Hou et al., 2013a; Hou et al., 2013b; Ye et al., 2013).
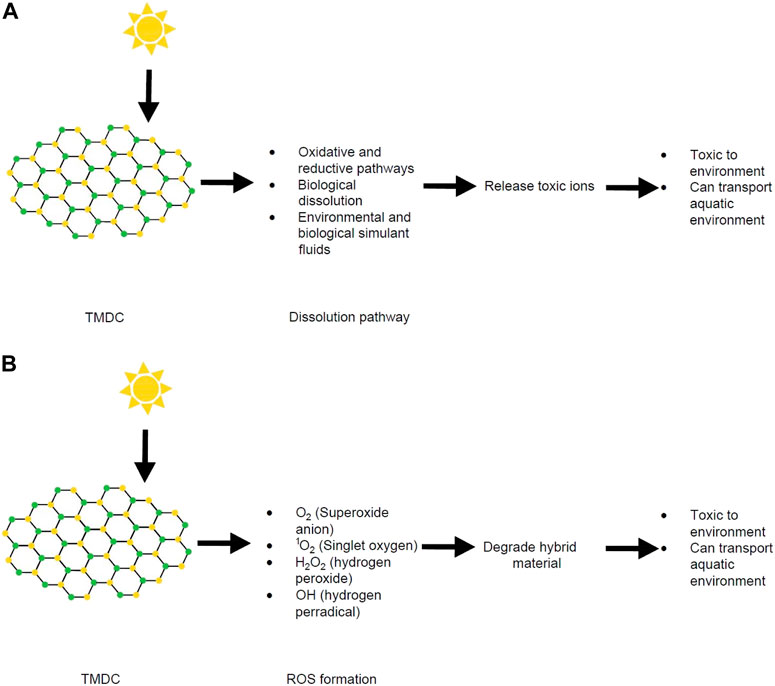
FIGURE 6. (A) Schematic diagram of dissolution process of TMDCs and their fate. (B) Schematic diagram of ROS formation from TMDCs and their fate. Structures produced by AutoCAD 2017.
However, since these studies were done with MoS2, more studies are required on other TMDs, and how other variables like structural defects, material thickness, oxidation time, temperature etc. Influence their degradation are required.
5.1.2 Toxicity
In terms of toxicity, a study (Wu et al., 2016) showed the survival rate of E. coli in a dose dependent manner of molybdenum disulfide nanosheets. The results showed that high concentration (100 μg/mL) of molybdenum disulfide nanosheets, affects the metabolic profile of E. coli and the survival rate of E. coli was decreased. The mechanism was attributed to the fact that high concentrations of MoS2, caused damage to cell membranes, induced ROS accumulation, and reduced viability (Wu et al., 2016). On the contrary, another study shows that at similar concentration (100 μg/mL), few layer MoS2 nanosheets with small lateral dimension (<1 μm) did not induce any cytotoxic effect and cells maintained their viability (Shah et al., 2015). This observation is similar to other studies that have also showed that MoS2 and WS2 nanomaterials are non-cytotoxic (Teo et al., 2014). This implies that the fate of MoS2 in aquatic environments could be dependent on the type, lateral size, concentration, exposure time, number of layers, and chemical composition and surface functionalization of MoS2 (KenryLim, 2016).
5.1.3 Challenges in synthesis
Currently there are some challenges in controlling the growth, overcoming the tendency toward aggregation and forming discrete nanosheets versus multi-pronged cores that lead to multi-site nanosheet growth of 2D nanosheet in TMDs (Terrones, 2016). Solution chemical synthesis can produce TMD materials in high yield and in solution-dispersible form, which also offers an increasingly interesting complement to traditional gas-phase, exfoliation, and substrate-bound synthetic platforms for accessing single- and few-layer TMD materials. Layered materials can also be exfoliated to monolayer and few-layer 2D nanosheets in various organic solvents via sonication but with low yield and not suitable for large scale production. In addition, the solvents used are expensive and toxic, and difficult to remove (Coleman et al., 2011).
5.2 Oxides
2D oxides have shown enormous potential in a broad range of application which necessitates the studies of their environmental implications. However, in terms of environmental implications, information on the 2D oxides is limited. For this reason, the review offers relevant information on bulk lamellar materials, which are often precursors for 2D materials, to give insight into fundamental chemistry.
5.2.1 Toxicity
Similar to graphene nanoparticles, 2D-TiO2 nanoparticles could also produce reactive oxygen species upon interaction with organisms or ultraviolet radiation (Wang et al., 2007; Castiglione et al., 2011; Elghniji et al., 2012; Feizi et al., 2013; Paret et al., 2013). Oxygen free radicals formed during their photosynthesis process could accelerate the breakdown of organic compounds, cause quenching and increase the absorption of inorganic nutrients (Zheng et al., 2005; Yang et al., 2006). Furthermore, TiO2 nanoparticles tend to form a covalent bond with natural organic matter due to their small size, which results in larger surface area-to-mass ratio along with greater interaction with cells and gets transported to tissue and cells’ specific distribution (Castiglione et al., 2011; Huh and Kwon, 2011; Qiu et al., 2013; Song et al., 2013). However, it is considered that, the acute toxic effects of TiO2 nanoparticles do not follow a clear dose-effect relationship, due to their agglomeration and subsequent sedimentation.
On the contrary, TiO2 nanoparticles were observed to increase the plant growth by the improvement in nitrogen metabolism that promotes the adsorption of nitrate and photosynthetic rate (Yang et al., 2006; Gao et al., 2008; Wu et al., 2012b). Due to their antimicrobial properties, TiO2 could also increase a plants ability of absorbing and utilizing fertilizer and water, encouraging its antioxidant system, and hasten its germination and growth (Molina-Barahona et al., 2005).
TiO2 NPs shows potent toxicity to aquatic vertebrates (Bar-Ilan et al., 2013; Kim et al., 2014a; Kim et al., 2014b; Rosenfeldt et al., 2014). Even at ppb concentration, TiO2 NPs can generate (ROS) under solar irradiation, in a dose-dependent manner, which can accumulate in different organs and cause stunted growth, organ pathology, delayed metamorphosis and DNA damage (Kim et al., 2014a). In addition to dose, ROS generation is size dependent as smaller particles due to their large surface area can generate a higher level of ROS. From the study (Kim et al., 2014a), it can be concluded that TiO2 NPs mechanism of toxicity is mainly dependent on the surface area rather than its concentration. For organisms like E.coli, toxicity of TiO2 NPs mainly depended on the generation of ROS like OH radicals or oxidative stress in E. coli rather than the particle size and surface area (Pathakoti et al., 2013). However, the studies do not consider factors like flow, depth, temperature and presence of natural organic matter which can induce dissolution or aggregation of TiO2 NPs and make the condition of ecosystem more complex. Without careful application of these nanomaterials, they will eventually be present in the environment and may have long-lasting effects on aquatic life. Moreover, if 2D oxides l undergo biological dissolution, they may not persist in their original solid state, which could introduce new challenges (Goodman and Cheshire, 1982; Wang et al., 2016b).
5.2.2 Environmental sensors
2D-MoO3 nanosheets has been extensively studied in gas and vapor sensing applications (Angiola et al., 2015; Ji et al., 2016). 2D-MoO3 is one of the most widely investigated gas sensitive materials, owing to its low cost, non-toxicity and stability at elevated temperature in air. The sensor using the 2D-MoO3 nanosheets has significantly a shorter response time as well as recovery time, compared to bulk MoO3 (Ji et al., 2016). However, synthesis technique of 2D-MoO3 nanosheets and fabrication technique of the sensor could cause aggregation, leading to a lower sensor response, which should be further investigated (Angiola et al., 2015). Also MoO3 is sensitive to environmental factors (humidity and oxygen), which has also not been considerd (Kamiya et al., 2004).
6 Gaps and future prospects
Complete materials characterization and mechanistic toxicity studies are essential for safe designing and manufacturing of 2D nanomaterials to develop applications with minimal risks for environmental health and safety. Moreover, future studies should focus on the effect of expanding concentration range of GO on these microorganisms and characterization of cell morphology for better comparison among studies.
For the development of next-generation membrane filtration systems for water purification, the primary challenge is to find the best combination of two-dimensional nanomaterials from GFNs and TMDs (e.g., MoS2 and WS2) that work together in membrane surfaces as antifouling and antibacterial agents. Findings from such studies will also apply to other areas including antifouling coatings for marine ship hulls, where fouling control remains a major challenge. Similar to graphene, 2D nanomaterials, such as TMDC, TMOs, metal-based nanocompounds, C3N4, BP, MXenes, hBN and other materials have also been researched for antibacterial applications (Mei et al., 2020). However, how the size, shape, layer numbers and surface functional modification, affects the antibacterial activities needs further research. In addition, majority of the research has been conducted on laboratories, with pure strain of a single microorganism (Yang et al., 2014; Wu et al., 2016; Kim et al., 2017). In the environment, there could be a mixed culture of microorganism, which could affect the antibacterial activity of these 2D nanomaterials and which should be looked as well.
Several challenges also exist for the efficient application of antimicrobial nanomaterials in drinking water treatment, such as the dispersion and retention of nanomaterials and the sustainability of antimicrobial activity. If nanomaterials are applied in the form of a slurry for water disinfection and microbial control, membrane filtration will be needed to retain and recycle the nanomaterials. Nanoparticles may also escape from the treatment system and enter the product water, which can have serious impacts on human health and ecosystems. Effective and reliable methods are needed to anchor the nanoparticles to reactor surfaces or to separate and retain suspended nanoparticles to reduce costs associated with material loss and to prevent human and environmental exposure. This includes developing better surface coating techniques, minimizing membrane fouling by nanomaterial suspension, and impregnating nanoparticles into filter packing materials, such as granular activated carbon or ion exchange resins.
Compared to graphene, which has been studied intensively, silicon- and germanium based 2D materials are much less explored, especially on their nanoscale level. This could be due to their synthesis, instability and a tendency toward oxidation. Moreover, the current knowledge about these materials covers only alkyl and aryl functional groups, and no other functionalities, whereas introduction of more complex functionalities may tune their physical properties similar to graphene (Hartman and Sofer, 2019). Constructing hybrid nanomaterials by using other 2D nanomaterials as building blocks, and thus further optimizing their properties and functionalities in future is a promising field.
There are many more 2D nanomaterials whose environmental implications, behavior, and fate are not yet known. It is essential to gather knowledge on their detailed material characteristics, toxicity, and implications so that preventive measures can be taken before the wholesale emergence of 2D nanomaterials in the market. In particular, it is important to relate specific physicochemical characteristics and functional assays so that predictions can be made for other materials and remediation can be designed accordingly. A challenge while utilizing these 2D nanomaterials is their high yield production to meet industry requirements for which more detailed research on their synthesis technique is required. Moreover, their preparation with desired structural characteristics in a highly controllable manner is still a challenge.
Although 2D nanomaterials have the potential to revolutionize aspects of electronics, medicine, and agriculture, the inherent risk of environmental and health hazards remain. In this regard, health and safety-focused research will augment application-driven research, ultimately enabling sustainable technological development.
Author contributions
MS: Conceptualization, methodology, investigation, writing—original draft, formal analysis, data curation (equal contribution) NM: Conceptualization, methodology, investigation, writing—original draft, formal analysis, data curation (equal contribution) MH: Funding acquisition, writing—review and editing, supervision, resources DB: Investigation, writing—review and editing IC: Conceptualization, methodology, resources, writing—original draft, writing—review and editing, supervision, project administration, funding acquisition.
Funding
This work was also supported by the National Science Foundation MADE-PUBLIC Future Manufacturing Research Grant Program under Award Number CMMI-2037026 and the Environmental Protection Agency under Cooperative Agreement Number DBI-1266377.
Conflict of interest
The authors declare that the research was conducted in the absence of any commercial or financial relationships that could be construed as a potential conflict of interest.
Publisher’s note
All claims expressed in this article are solely those of the authors and do not necessarily represent those of their affiliated organizations, or those of the publisher, the editors and the reviewers. Any product that may be evaluated in this article, or claim that may be made by its manufacturer, is not guaranteed or endorsed by the publisher.
Disclaimer
This paper has been reviewed in accordance with the U.S. Environmental Protection Agency’s peer and administrative review policies and approved for publication. Mention of trade names or commercial products does not constitute endorsement or recommendation for use. The views expressed in this article are those of the authors and do not necessarily represent the views or policies of the USEPA.
References
Abellán, G., Ares, P., and Wild, S. (2017). Noncovalent functionalization and charge transfer in antimonene. Angew. Chem. 129 (46), 14581–14586.
Adamska, L., Sadasivam, S., Foley, I. V. J. J., Darancet, P., and Sharifzadeh, S. (2018). First-Principles investigation of borophene as a monolayer transparent conductor. J. Phys. Chem. C 122 (7), 4037–4045. doi:10.1021/acs.jpcc.7b10197
Akhavan, O., and Ghaderi, E. (2012). Escherichia coli bacteria reduce graphene oxide to bactericidal graphene in a self-limiting manner. Carbon 50 (5), 1853–1860. doi:10.1016/j.carbon.2011.12.035
An, H., Li, Y., Feng, Y., Cao, Y., Cao, C., Long, P., et al. (2018). Reduced graphene oxide doped predominantly with CF2 groups as a superior anode material for long-life lithium-ion batteries. Chem. Commun. 54 (22), 2727–2730. doi:10.1039/c7cc09516c
An, H., Li, Y., Long, P., Gao, Y., Qin, C., Cao, C., et al. (2016). Hydrothermal preparation of fluorinated graphene hydrogel for high-performance supercapacitors. J. Power Sources 312, 146–155. doi:10.1016/j.jpowsour.2016.02.057
Angiola, M., Alsaif, M. M. Y. A., Kalantar-zadeh, K., Wisitsoraat, A., Wlodarski, W., and Martucci, A. (2015). Optical hydrogen sensing based on hybrid 2D MoO 3/Au nanoparticles. Procedia Eng. 120, 1141–1144. doi:10.1016/j.proeng.2015.08.830
Anjum, N. A., Singh, N., Singh, M. K., Shah, Z. A., Duarte, A. C., Pereira, E., et al. (2013). Single-bilayer graphene oxide sheet tolerance and glutathione redox system significance assessment in faba bean (Vicia faba L.). J. Nanopart Res. 15 (7), 1770. doi:10.1007/s11051-013-1770-7
Ayán-Varela, M., Villar-Rodil, S., Paredes, J. I., Munuera, J. M., Pagán, A., Lozano-Pérez, A. A., et al. (2015). Investigating the dispersion behavior in solvents, biocompatibility, and use as support for highly efficient metal catalysts of exfoliated graphitic carbon nitride. ACS Appl. Mat. Interfaces 7 (43), 24032–24045. doi:10.1021/acsami.5b06974
Bagri, A., Mattevi, C., Acik, M., Chabal, Y. J., Chhowalla, M., and Shenoy, V. B. (2010). Structural evolution during the reduction of chemically derived graphene oxide. Nat. Chem. 2 (7), 581–587. doi:10.1038/nchem.686
Bai, H., Jiang, W., Kotchey, G. P., Saidi, W. A., Bythell, B. J., Jarvis, J. M., et al. (2014). Insight into the mechanism of graphene oxide degradation via the photo-fenton reaction. J. Phys. Chem. C 118, 10519–10529. doi:10.1021/jp503413s
Balandin, A. A., Ghosh, S., Bao, W., Calizo, I., Teweldebrhan, D., Miao, F., et al. (2008). Superior thermal conductivity of single-layer graphene. Nano Lett. 8 (3), 902–907. doi:10.1021/nl0731872
Balog, R., Jørgensen, B., Nilsson, L., Andersen, M., Rienks, E., Bianchi, M., et al. (2010). Bandgap opening in graphene induced by patterned hydrogen adsorption. Nat. Mater 9 (4), 315–319. doi:10.1038/nmat2710
Bang, G. S., Nam, K. W., Kim, J. Y., Shin, J., Choi, J. W., and Choi, S. (2014). Effective liquid-phase exfoliation and sodium ion battery application of MoS2 nanosheets. ACS Appl. Mat. Interfaces 6, 7084–7089. doi:10.1021/am4060222
Bar-Ilan, O., Chuang, C. C., Schwahn, D. J., Yang, S., Joshi, S., Pedersen, J. A., et al. (2013). TiO2 nanoparticle exposure and illumination during zebrafish development: Mortality at parts per billion concentrations. Environ. Sci. Technol. 47 (9), 4726–4733. doi:10.1021/es304514r
Bargar, J. R., Brown, G. E., and Parks, G. A. (1998). Surface complexation of Pb(II) at oxide-water interfaces: III. XAFS determination of Pb(II) and Pb(II)-Chloro adsorption complexes on goethite and alumina. Geochimica Cosmochimica Acta 62 (2), 193–207. doi:10.1016/S0016-7037(97)00334-7
Barinov, A., Malcioǧlu, O. B., Fabris, S., Sun, T., Gregoratti, L., Dalmiglio, M., et al. (2009). Initial stages of oxidation on graphitic surfaces: Photoemission study and density functional theory calculations. J. Phys. Chem. C 113 (21), 9009–9013. doi:10.1021/jp902051d
Barsoum, M. W. (2000). The MN+1AXN phases: A new class of solids. Prog. Solid State Chem. 28, 201–281. doi:10.1016/S0079-6786(00)00006-6
Begum, P., and Fugetsu, B. (2013). Induction of cell death by graphene in Arabidopsis thaliana (Columbia ecotype) T87 cell suspensions. J. Hazard. Mater. 260, 1032–1041. doi:10.1016/j.jhazmat.2013.06.063
Begum, P., Ikhtiari, R., and Fugetsu, B. (2011). Graphene phytotoxicity in the seedling stage of cabbage, tomato, red spinach, and lettuce. Carbon 49 (12), 3907–3919. doi:10.1016/j.carbon.2011.05.029
Bianco, E., Butler, S., Jiang, S., Restrepo, O. D., Windl, W., and Goldberger, J. E. (2013). Stability and exfoliation of germanane: A germanium graphane analogue. ACS Nano 7 (5), 4414–4421. doi:10.1021/nn4009406
Cao, S., Low, J., Yu, J., and Jaroniec, M. (2015). Polymeric photocatalysts based on graphitic carbon nitride. Adv. Mat. 27 (13), 2150–2176. doi:10.1002/adma.201500033
Cao, X., Tan, C., Zhang, X., Zhao, W., and Zhang, H. (2016). Solution-processed two-dimensional metal dichalcogenide-based nanomaterials for energy storage and conversion. Adv. Mat. 28, 6167–6196. doi:10.1002/adma.201504833
Castiglione, M. R., Giorgetti, L., Geri, C., and Cremonini, R. (2011). The effects of nano-TiO 2 on seed germination, development and mitosis of root tip cells of Vicia narbonensis L. and Zea mays L. J. Nanoparticle Res. 13 (6), 2443–2449.
Cataldi, P., Athanassiou, A., and Bayer, I. (2018). Graphene nanoplatelets-based advanced materials and recent progress in sustainable applications. Appl. Sci. 8 (9), 1438. doi:10.3390/app8091438
Chang, C. H., Huang, T. C., Peng, C. W., Yeh, T.-C., Lu, H.-I., Hung, W.-I., et al. (2012). Novel anticorrosion coatings prepared from polyaniline/graphene composites. Carbon 50 (14), 5044–5051. doi:10.1016/j.carbon.2012.06.043
Chang, Y., Li, P., Li, L., Chang, S., Huo, Y., Mu, C., et al. (2021). In situ grown ultrafine RuO2 nanoparticles on GeP5 nanosheets as the electrode material for flexible planar micro-supercapacitors with high specific capacitance and cyclability. ACS Appl. Mat. Interfaces 13 (40), 47560–47571. doi:10.1021/acsami.1c12549
Chen, Y., Xue, D., and Coleman, J. N. (2014b). Preparation of colloidal graphene in quantity by electrochemical exfoliation. J. Colloid Interface Sci. 436 (7), 41–46. doi:10.1016/j.jcis.2014.08.057
Chen, S., Brown, L., Levendorf, M., Cai, W., Ju, S.-Y., Edgeworth, J., et al. (2011). Oxidation resistance of graphene-coated Cu and Cu/Ni alloy. ACS Nano 5 (2), 1321–1327. doi:10.1021/nn103028d
Chen, Y., Huang, W., He, D., Situ, Y., and Huang, H. (2014a). Construction of heterostructured g-C3N4/Ag/TiO2 microspheres with enhanced photocatalysis performance under visible-light irradiation. ACS Appl. Mat. Interfaces 6 (16), 14405–14414. doi:10.1021/am503674e
Chen, Y., Li, Y., Yao, F., Peng, C., Cao, C., Feng, Y., et al. (2019). Nitrogen and fluorine co-doped holey graphene hydrogel as a binder-free electrode material for flexible solid-state supercapacitors. Sustain. Energy Fuels 3 (9), 2237–2245. doi:10.1039/c9se00142e
Cheng, L., Huang, W., Gong, Q., Liu, C., Liu, Z., Li, Y., et al. (2014). Ultrathin WS2Nanoflakes as a high-performance electrocatalyst for the hydrogen evolution reaction. Angew. Chem. Int. Ed. 53 (30), 7860–7863. doi:10.1002/anie.201402315
Cheng, N., Tian, J., Liu, Q., Ge, C., Qusti, A. H., Asiri, A. M., et al. (2013). Au-nanoparticle-loaded graphitic carbon nitride nanosheets: Green photocatalytic synthesis and application toward the degradation of organic pollutants. ACS Appl. Mat. Interfaces 5 (15), 6815–6819. doi:10.1021/am401802r
Cheng, X., Wang, L., Xie, L., Sun, C., Zhao, W., Liu, X., et al. (2022). Defect-driven selective oxidation of MoS2 nanosheets with photothermal effect for photo-catalytic hydrogen evolution reaction. Chem. Eng. J. 439, 135757. doi:10.1016/j.cej.2022.135757
Chhowalla, M., Shin, H. S., Eda, G., Li, L. J., Loh, K. P., and Zhang, H. (2013). The chemistry of two-dimensional layered transition metal dichalcogenide nanosheets. Nat. Chem. 5, 263–275. doi:10.1038/nchem.1589
Chng, E. L. K., Sofer, Z., and Pumera, M. (2014). MoS2 exhibits stronger toxicity with increased exfoliation. Nanoscale 6 (23), 14412–14418. doi:10.1039/C4NR04907A
Cho, D. H., Kim, J. S., Kwon, S. H., Lee, C., and Lee, Y. Z. (2013). Evaluation of hexagonal boron nitride nano-sheets as a lubricant additive in water. Wear 302 (1-2), 981–986. doi:10.1016/j.wear.2012.12.059
Chou, S. S., Kaehr, B., Kim, J., Foley, B. M., De, M., Hopkins, P. E., et al. (2013). Chemically exfoliated MoS2as near-infrared photothermal agents. Angew. Chem. Int. Ed. 52 (15), 4160–4164. doi:10.1002/anie.201209229
Chowdhury, I., Duch, M. C., Mansukhani, N. D., Hersam, M. C., and Bouchard, D. (2013). Colloidal properties and stability of graphene oxide nanomaterials in the aquatic environment. Environ. Sci. Technol. 47 (12), 6288–6296. doi:10.1021/es400483k
Chowdhury, I., Hou, W-C., Goodwin, D., Henderson, M., Zepp, R. G., and Bouchard, D. (2015a). Sunlight affects aggregation and deposition of graphene oxide in the aquatic environment. Water Res. 78, 37–46. doi:10.1016/j.watres.2015.04.001
Chowdhury, I., Mansukhani, N. D., Guiney, L. M., Hersam, M. C., and Bouchard, D. (2015b). Aggregation and stability of reduced graphene oxide: Complex roles of divalent cations, pH, and natural organic matter. Environ. Sci. Technol. 49 (18), 10886–10893. doi:10.1021/acs.est.5b01866
Chu, S., Wang, Y., Guo, Y., Feng, J., Wang, C., Luo, W., et al. (2013). Band structure engineering of carbon nitride: In search of a polymer photocatalyst with high photooxidation property. ACS Catal. 3 (5), 912–919. doi:10.1021/cs4000624
Chua, C. K., and Pumera, M. (2014). Chemical reduction of graphene oxide: A synthetic chemistry viewpoint. Chem. Soc. Rev. 43 (1), 291–312. doi:10.1039/c3cs60303b
Chung, C., Kim, Y. K., Shin, D., Ryoo, S. R., Hong, B. H., and Min, D. H. (2013). Biomedical applications of graphene and graphene oxide. Acc. Chem. Res. 46 (10), 2211–2224. doi:10.1021/ar300159f
Clark, R. M., Carey, B. J., Daeneke, T., Atkin, P., Bhaskaran, M., Latham, K., et al. (2015). Two-step synthesis of luminescent MoS2-ZnS hybrid quantum dots. Nanoscale 7 (40), 16763–16772. doi:10.1039/c5nr04790k
Coleman, J. N., Lotya, M., and O’Neill, A. (2011). Two-dimensional nanosheets produced by liquid exfoliation of layered materials. Science 80, 331568–331571. doi:10.1126/science.1194975
Colson, J. W., Woll, A. R., Mukherjee, A., Levendorf, M. P., Spitler, E. L., Shields, V. B., et al. (2011). Oriented 2D covalent organic framework thin films on single-layer graphene. Science 332 (6026), 228–231. doi:10.1126/science.1202747
Dahanayaka, M., Liu, B., Hu, Z., Chen, Z., Law, A. W-K., and Zhou, K. (2017). Corrugated graphene layers for sea water desalination using capacitive deionization. Phys. Chem. Chem. Phys. 19 (12), 8552–8562. doi:10.1039/C7CP00389G
De La Torre-Roche, R., Hawthorne, J., Deng, Y., Xing, B., Cai, W., Newman, L. A., et al. (2013). Multiwalled carbon nanotubes and C60 fullerenes differentially impact the accumulation of weathered pesticides in four agricultural plants. Environ. Sci. Technol. 47 (21), 12539–12547. doi:10.1021/es4034809
Deng, R., Xie, X., Vendrell, M., Chang, Y-T., and Liu, X. (2011). Intracellular glutathione detection using MnO2-nanosheet-modified upconversion nanoparticles. J. Am. Chem. Soc. 133 (50), 20168–20171. doi:10.1021/ja2100774
Dervin, S., Dionysiou, D. D., and Pillai, S. C. (2016). 2D nanostructures for water purification: Graphene and beyond. Nanoscale 8 (33), 15115–15131. doi:10.1039/C6NR04508A
Dimiev, A. M., Alemany, L. B., and Tour, J. M. (2012). Graphene oxide. Origin of acidity, its instability in water, and a new dynamic structural model. ACS Nano 7 (1), 576–588. doi:10.1021/nn3047378
Du, H., Deng, Z., Lü, Z., Yin, Y., Yu, L., Wu, H., et al. (2011). The effect of graphdiyne doping on the performance of polymer solar cells. Synth. Met. 161 (19-20), 2055–2057. doi:10.1016/j.synthmet.2011.04.015
Du, T., Adeleye, A. S., Keller, A. A., Wu, Z., Han, W., Wang, Y., et al. (2017). Photochlorination-induced transformation of graphene oxide: Mechanism and environmental fate. Water Res. 124, 372–380. doi:10.1016/j.watres.2017.07.054
Duan, H., Yan, N., Yu, R., Chang, C.-R., Zhou, G., Hu, H.-S., et al. (2014). Ultrathin rhodium nanosheets. Nat. Commun. 5. 4093. doi:10.1038/ncomms4093
Duan, L., Hao, R., Xu, Z., He, X., Adeleye, A. S., and Li, Y. (2017). Removal of graphene oxide nanomaterials from aqueous media via coagulation: Effects of water chemistry and natural organic matter. Chemosphere 168, 1051–1057. doi:10.1016/j.chemosphere.2016.10.104
Eda, G., Yamaguchi, H., Voiry, D., Fujita, T., Chen, M., and Chhowalla, M. (2011). Photoluminescence from chemically exfoliated MoS2. Nano Lett. 11 (12), 5111–5116. doi:10.1021/nl201874w
Elghniji, K., Hentati, O., Mlaik, N., Mahfoudh, A., and Ksibi, M. (2012). Photocatalytic degradation of 4-chlorophenol under P-modified TiO2/UV system: Kinetics, intermediates, phytotoxicity and acute toxicity. J. Environ. Sci. 24 (3), 479–487. doi:10.1016/s1001-0742(10)60659-6
Elias, D. C., Nair, R. R., Mohiuddin, T. M. G., Morozov, S. V., Blake, P., Halsall, M. P., et al. (2009). Control of graphene's properties by reversible hydrogenation: Evidence for graphane. Science 323, 610–613. doi:10.1126/science.1167130
Evans, J. S. O., and O'hare, D. (1994). Kinetics of the intercalation of cations into MnPS3 using real time in situ x-ray diffraction. Adv. Mat. 6 (9), 646–648. doi:10.1002/adma.19940060904
Fan, K., Peng, L., Liu, Y., Li, Y., Chen, Y., Meng, Y., et al. (2020). Giant enhancement of fluorescence emission by fluorination of porous graphene with high defect density and subsequent application as Fe3+ ion sensors. ACS Appl. Mat. Interfaces 12 (36), 40662–40672. doi:10.1021/acsami.0c11141
Fan, X., Peng, W., Li, Y., Li, X., Wang, S., Zhang, G., et al. (2008). Deoxygenation of exfoliated graphite oxide under alkaline conditions: A green route to graphene preparation. Adv. Mat. 20 (23), 4490–4493. doi:10.1002/adma.200801306
Fan, Z., Huang, X., Tan, C., and Zhang, H. (2015). Thin metal nanostructures: Synthesis, properties and applications. Chem. Sci. 6 (1), 95–111. doi:10.1039/C4SC02571G
Favron, A., Gaufrès, E., and Fossard, F. Exfoliating pristine black phosphorus down to the monolayer: Photo-oxidation and electronic confinement effects. arXiv Prepr arXiv14080345 2014.
Feizi, H., Kamali, M., Jafari, L., and Rezvani Moghaddam, P. R. (2013). Phytotoxicity and stimulatory impacts of nanosized and bulk titanium dioxide on fennel (Foeniculum vulgare Mill). Chemosphere 91 (4), 506–511. doi:10.1016/j.chemosphere.2012.12.012
Feng, C., Huang, L., Guo, Z., and Liu, H. (2007). Synthesis of tungsten disulfide (WS2) nanoflakes for lithium ion battery application. Electrochem. Commun. 9 (1), 119–122. doi:10.1016/j.elecom.2006.08.048
Feng, H., Cheng, R., Zhao, X., Duan, X., and Li, J. (2013). A low-temperature method to produce highly reduced graphene oxide. Nat. Commun. 4, 1539. doi:10.1038/ncomms2555
Feng, X., Wang, X., Xing, W., Zhou, K., Song, L., and Hu, Y. (2014). Liquid-exfoliated MoS2 by chitosan and enhanced mechanical and thermal properties of chitosan/MoS2 composites. Compos. Sci. Technol. 93, 76–82. doi:10.1016/j.compscitech.2013.11.016
Fernández-Merino, M. J., Guardia, L., Paredes, J. I., Villar-Rodil, S., Solís-Fernández, P., Martínez-Alonso, A., et al. (2010). Vitamin C is an ideal substitute for hydrazine in the reduction of graphene oxide suspensions. J. Phys. Chem. C 114 (14), 6426–6432. doi:10.1021/jp100603h
Finn, D. J., Lotya, M., Cunningham, G., Smith, R. J., McCloskey, D., Donegan, J. F., et al. (2014). Inkjet deposition of liquid-exfoliated graphene and MoS2nanosheets for printed device applications. J. Mat. Chem. C 2 (5), 925–932. doi:10.1039/C3TC31993H
Fojtů, M., Teo, W. Z., and Pumera, M. (2017). Environmental impact and potential health risks of 2D nanomaterials. Environ. Sci. Nano 4 (8), 1617–1633. doi:10.1039/C7EN00401J
Frank, I. W., Tanenbaum, D. M., van der Zande, A. M., and McEuen, P. L. (2007). Mechanical properties of suspended graphene sheets. J. Vac. Sci. Technol. B 25 (6), 2558–2561. doi:10.1116/1.2789446
Frutos, F. J. G., Escolano, O., García, S., and Ivey, G. A. (2011). Mobilization assessment and possibility of increased availability of PAHs in contaminated soil using column tests. Soil Sediment Contam. An Int. J. 20 (5), 581–591. doi:10.1080/15320383.2011.587046
Fu, P. P., Von Tungeln, L. S., Chiu, L., and Own, Z. Y. (1999). Halogenated-polycyclic aromatic hydrocarbons: A class of genotoxic environmental pollutants. J. Environ. Sci. Health, Part C 17 (2), 71–109. doi:10.1080/10590509909373510
Gan, L., Zhang, Q., Cheng, Y., and Schwingenschlögl, U. (2014). Photovoltaic heterojunctions of fullerenes with MoS2 and WS2 monolayers. J. Phys. Chem. Lett. 5, 1445–1449. doi:10.1021/jz500344s
Gao, F., Liu, C., Qu, C., Zheng, L., Yang, F., Su, M., et al. (2008). Was improvement of spinach growth by nano-TiO2 treatment related to the changes of Rubisco activase? Biometals 21 (2), 211–217. doi:10.1007/s10534-007-9110-y
Gao, G., Mathkar, A., Martins, E. P., Galvão, D. S., Gao, D., Autreto, P. A. d. S., et al. (2014). Designing nanoscaled hybrids from atomic layered boron nitride with silver nanoparticle deposition. J. Mat. Chem. A 2 (9), 3148–3154. doi:10.1039/c3ta12892j
Gao, J., Liu, F., Liu, Y., Ma, N., Wang, Z., and Zhang, X. (2010). Environment-Friendly method to produce graphene that employs vitamin C and amino Acid. Chem. Mat. 22 (7), 2213–2218. doi:10.1021/cm902635j
Garg, M., and Thakur, A. (2022). A review: Biomedical applications of phosphorene, antimonene, and germanene-based 2D material/hydrogel complexes. J. Mater Sci. 2022, 1–12. doi:10.1007/s10853-022-07954-7
Geim, A. K., and Grigorieva, I. V. (2013). Van der Waals heterostructures. Nature 499 (7459), 419–425. doi:10.1038/nature12385
Geim, A. K., and Novoselov, K. S. (2007). The rise of graphene. Nat. Mater 6 (3), 183–191. doi:10.1038/nmat1849
Geim, A. K., and Novoselov, K. S. The rise of graphene. In: Nanoscience and technology. Co-Published with Macmillan Publishers Ltd: United Kingdom; 2009, 2009, 11–19. doi:10.1142/9789814287005_0002
Geng, F., Ma, R., and Sasaki, T. (2010). Anion-exchangeable layered materials based on rare-earth phosphors: Unique combination of rare-earth host and exchangeable anions. Acc. Chem. Res. 43 (9), 1177–1185. doi:10.1021/ar900289v
Ghodake, G., Seo, Y. D., Park, D., and Lee, D. S. (2010). Phytotoxicity of carbon nanotubes assessed by Brassica juncea and phaseolus mungo. J. Nanoelectron. Optoelectron. 5 (2), 157–160. doi:10.1166/jno.2010.1084
Gillan, E. G. (2000). Synthesis of nitrogen-rich carbon nitride networks from an energetic molecular azide precursor. Chem. Mat. 12 (12), 3906–3912. doi:10.1021/cm000570y
Goodman, B. A., and Cheshire, M. V. (1982). Reduction of molybdate by soil organic matter: EPR evidence for formation of both Mo(V) and Mo(III). Nature 299, 618–620. doi:10.1038/299618a0
Greim, J., and Schwetz, K. A. (2000). “Boron carbide, boron nitride, and metal borides,” in Ullmann’s encyclopedia of industrial chemistry (Netherlands: Wiley-VCH Verlag GmbH & Co. KGaA). doi:10.1002/14356007.a04_295.pub2
Gurunathan, S., Woong Han, J. W., Abdal Daye, A. A., Eppakayala, V., and Kim, J-H. (2012). Oxidative stress-mediated antibacterial activity of graphene oxide and reduced graphene oxide in Pseudomonas aeruginosa. Ijn 7, 5901. doi:10.2147/ijn.s37397
Haley, M. M. (2008). Synthesis and properties of annulenic subunits of graphyne and graphdiyne nanoarchitectures. Pure Appl. Chem. 80 (3), 519–532. doi:10.1351/pac200880030519
Han, J. H., Lee, S., and Cheon, J. (2013). Synthesis and structural transformations of colloidal 2D layered metal chalcogenide nanocrystals. Chem. Soc. Rev. 42 (7), 2581–2591. doi:10.1039/C2CS35386E
Hartman, T., and Sofer, Z. (2019). Beyond graphene: Chemistry of group 14 graphene analogues: Silicene, germanene, and stanene. ACS Nano 13 (8), 8566–8576. doi:10.1021/acsnano.9b04466
Hau, H. H., and Gralnick, J. A. (2007). Ecology and biotechnology of the genus Shewanella. Annu. Rev. Microbiol. 61, 237–258. doi:10.1146/annurev.micro.61.080706.093257
Hou, W-C., Chowdhury, I., Goodwin, D. G., Henderson, W. M., Fairbrother, D. H., Bouchard, D., et al. (2015). Photochemical transformation of graphene oxide in sunlight. Environ. Sci. Technol. 49 (6), 3435–3443. doi:10.1021/es5047155
Hou, W-C., Henderson, W. M., Chowdhury, I., Goodwin, D. G., Chang, X., Martin, S., et al. (2016). The contribution of indirect photolysis to the degradation of graphene oxide in sunlight. Carbon 110, 426–437. doi:10.1016/j.carbon.2016.09.013
Hou, Y., Laursen, A. B., Zhang, J., Zhang, G., Zhu, Y., Wang, X., et al. (2013b). Layered nanojunctions for hydrogen-evolution catalysis. Angew. Chem. Int. Ed. 52 (13), 3621–3625. doi:10.1002/anie.201210294
Hou, Y., Wen, Z., Cui, S., Guo, X., and Chen, J. (2013a). Constructing 2D porous graphitic C 3 N 4 nanosheets/nitrogen-doped graphene/layered MoS 2 ternary nanojunction with enhanced photoelectrochemical activity. Adv. Mat. 25 (43), 6291–6297. doi:10.1002/adma.201303116
Hu, S., Lozada-Hidalgo, M., Wang, F. C., Mishchenko, A., Schedin, F., Nair, R. R., et al. (2014). Proton transport through one-atom-thick crystals. Nature 516 (7530), 227–230. doi:10.1038/nature14015
Hu, Z., Liu, B., Dahanayaka, M., Law, A. W-K., Wei, J., and Zhou, K. (2017). Ultrafast permeation of seawater pervaporation using single-layered C2N via strain engineering. Phys. Chem. Chem. Phys. 19 (24), 15973–15979. doi:10.1039/c7cp01542a
Huang, J., Ho, W., and Wang, X. (2014b). Metal-free disinfection effects induced by graphitic carbon nitride polymers under visible light illumination. Chem. Commun. 50 (33), 4338–4340. doi:10.1039/c3cc48374f
Huang, J., Pu, J., Hsu, C. L., Chiu, M.-H., Juang, Z.-Y., Chang, Y.-H., et al. (2014c). Large-area synthesis of highly crystalline WSe2 monolayers and device applications. ACS Nano 8 (1), 923–930. doi:10.1021/nn405719x
Huang, K. J., Shuai, H. L., and Chen, Y. X. (2016). Layered molybdenum selenide stacking flower-like nanostructure coupled with guanine-rich DNA sequence for ultrasensitive ochratoxin A aptasensor application. Sensors Actuators B Chem. 225, 391–397. doi:10.1016/j.snb.2015.11.070
Huang, X., Li, S., Huang, Y., Wu, S., Zhou, X., Li, S., et al. (2011a). Synthesis of hexagonal close-packed gold nanostructures. Nat. Commun. 2, 292. doi:10.1038/ncomms1291
Huang, J., Tan, C., Yin, Z., and Zhang, H. (2014). 25th anniversary article: Hybrid nanostructures based on two-dimensional nanomaterials. Adv. Mat. 26 (14), 2185–2204. doi:10.1002/adma.201304964
Huang, X., Tang, S., Mu, X., Dai, Y., Chen, G., Zhou, Z., et al. (2011b). Freestanding palladium nanosheets with plasmonic and catalytic properties. Nat. Nanotech 6 (1), 28–32. doi:10.1038/nnano.2010.235
Huh, A. J., and Kwon, Y. J. (2011). "Nanoantibiotics": A new paradigm for treating infectious diseases using nanomaterials in the antibiotics resistant era. J. Control. Release 156 (2), 128–145. doi:10.1016/j.jconrel.2011.07.002
Inagaki, M., and Kang, F. Y. (2014). Graphene derivatives: Graphane, fluorographene, graphene oxide, graphyne and graphdiyne. J. Mat. Chem. A 2 (33), 13193–13206. doi:10.1039/c4ta01183j
Island, J. O., Steele, G. A., van der Zant, H. S. J., and Castellanos-Gomez, A. (2015). Environmental instability of few-layer black phosphorus. 2D Mater 2 (1), 11002. doi:10.1088/2053-1583/2/1/011002
Ji, F., Ren, X., Zheng, X., Liu, Y., Pang, L., Jiang, J., et al. (2016). 2D-MoO3nanosheets for superior gas sensors. Nanoscale 8 (16), 8696–8703. doi:10.1039/C6NR00880A
Jo, I., Pettes, M. T., Kim, J., Watanabe, K., Taniguchi, T., Yao, Z., et al. (2013). Thermal conductivity and phonon transport in suspended few-layer hexagonal boron nitride. Nano Lett. 13 (2), 550–554. doi:10.1021/nl304060g
Joy, P. A., and Vasudevan, S. (1992). The intercalation reaction of pyridine with manganese thiophosphate, MnPS3. J. Am. Chem. Soc. 114 (20), 7792–7801. doi:10.1021/ja00046a027
Kalantar-zadeh, K., Ou, J. Z., Daeneke, T., Strano, M. S., Pumera, M., and Gras, S. L. (2015). Two-dimensional transition metal dichalcogenides in biosystems. Adv. Funct. Mat. 25 (32), 5086–5099. doi:10.1002/adfm.201500891
Kamiya, S., Tsuda, D., Miura, K., and Sasaki, N. (2004). MoS2(0001)/MoO3(010)/MoS2(0001) friction-reducing system. Wear 257 (11), 1133–1136. doi:10.1016/j.wear.2004.07.012
Kang, D., Kwon, J. Y., Cho, H., Sim, J.-H., Hwang, H. S., Kim, C. S., et al. (2012). Oxidation resistance of iron and copper foils coated with reduced graphene oxide multilayers. ACS Nano 6 (9), 7763–7769. doi:10.1021/nn3017316
Kaul, A. B. (2014). Two-dimensional layered materials: Structure, properties, and prospects for device applications. J. Mat. Res. 29 (03), 348–361. doi:10.1557/jmr.2014.6
Keli, Z., Ying, H., Mo, Z., and Guosheng, C. (2018). A review: Biodegradation strategy of graphene-based materials. ACTA Chim. Sin. 76 (3), 168–176.
Kemp, K. C., Seema, H., Saleh, M., Le, N. H., Mahesh, K., Chandra, V., et al. (2013). Environmental applications using graphene composites: Water remediation and gas adsorption. Nanoscale 5 (8), 3149–3171. doi:10.1039/C3NR33708A
Kenry, L., and Lim, L. C. (2016). Biocompatibility and nanotoxicity of layered two-dimensional nanomaterials. ChemNanoMat 3 (1), 5–16. doi:10.1002/cnma.201600290
Khodakovskaya, M. V., de Silva, K., Nedosekin, D. A., Dervishi, E., Biris, A. S., Shashkov, E. V., et al. (2011). Complex genetic, photothermal, and photoacoustic analysis of nanoparticle-plant interactions. Proc. Natl. Acad. Sci. U.S.A. 108 (3), 1028–1033. doi:10.1073/pnas.1008856108
Kieu, H. T., Liu, B., Zhou, K., and Law, A. W-K. (2017). Pressure-driven water permeation through multilayer graphene nanosheets. Phys. Status Solidi B 254 (10), 1700074. doi:10.1002/pssb.201700074
Kim, K-T., Klaine, S. J., and Kim, S. D. (2014). Acute and chronic response of Daphnia magna exposed to TiO2 nanoparticles in agitation system. Bull. Environ. Contam. Toxicol. 93 (4), 456–460. doi:10.1007/s00128-014-1295-5
Kim, M-S., Louis, K. M., Pedersen, J. A., Hamers, R. J., Peterson, R. E., and Heideman, W. (2014). Using citrate-functionalized TiO2 nanoparticles to study the effect of particle size on zebrafish embryo toxicity. Analyst 139 (5), 964–972. doi:10.1039/c3an01966g
Kim, T. I., Kwon, B., Yoon, J., Park, I.-J., Bang, G. S., Park, Y., et al. (2017). Antibacterial activities of graphene oxide-molybdenum disulfide nanocomposite films. ACS Appl. Mat. Interfaces 9 (9), 7908–7917. doi:10.1021/acsami.6b12464
Kissel, P., Murray, D. J., Wulftange, W. J., Catalano, V. J., and King, B. T. (2014). A nanoporous two-dimensional polymer by single-crystal-to-single-crystal photopolymerization. Nat. Chem. 6 (9), 774–778. doi:10.1038/nchem.2008
Koinuma, M., Ogata, C., Kamei, Y., Hatakeyama, K., Tateishi, H., Watanabe, Y., et al. (2012). Photochemical engineering of graphene oxide nanosheets. J. Phys. Chem. C 116 (37), 19822–19827. doi:10.1021/jp305403r
Kory, M. J., Wörle, M., Weber, T., Payamyar, P., van de Poll, S. W., Dshemuchadse, J., et al. (2014). Gram-scale synthesis of two-dimensional polymer crystals and their structure analysis by X-ray diffraction. Nat. Chem. 6 (9), 779–784. doi:10.1038/nchem.2007
Kumar, S., Baruah, A., Tonda, S., Kumar, B., Shanker, V., and Sreedhar, B. (2014). Cost-effective and eco-friendly synthesis of novel and stable N-doped ZnO/g-C3N4 core-shell nanoplates with excellent visible-light responsive photocatalysis. Nanoscale 6 (9), 4830–4842. doi:10.1039/c3nr05271k
Kurapati, R., Bonachera, F., and Russier, J. (2017). Covalent chemical functionalization enhances the biodegradation of graphene oxide. 2D Mater 5 (1), 15020. doi:10.1088/2053-1583/aa8f0a
Kurapati, R., Russier, J., Squillaci, M. A., Treossi, E., Ménard-Moyon, C., Del Rio-Castillo, A. E., et al. (2015). Dispersibility-dependent biodegradation of graphene oxide by myeloperoxidase. Small 11 (32), 3985–3994. doi:10.1002/smll.201500038
Lalwani, G., Henslee, A. M., Farshid, B., Parmar, P., Lin, L., Qin, Y.-X., et al. (2013). Tungsten disulfide nanotubes reinforced biodegradable polymers for bone tissue engineering. Acta Biomater. 9 (9), 8365–8373. doi:10.1016/j.actbio.2013.05.018
Lanphere, J. D., Luth, C. J., Guiney, L. M., Mansukhani, N. D., Hersam, M. C., and Walker, S. L. (2015). Fate and transport of molybdenum disulfide nanomaterials in sand columns. Environ. Eng. Sci. 32 (2), 163–173. doi:10.1089/ees.2014.0335
Lauritsen, J. V., Nyberg, M., Nørskov, J. K., Clausen, B. S., Topsøe, H., Lægsgaard, E., et al. (2004). Hydrodesulfurization reaction pathways on MoS2 nanoclusters revealed by scanning tunneling microscopy. J. Catal. 224 (1), 94–106. doi:10.1016/j.jcat.2004.02.009
Lee, G., and Kim, B. S. (2014). Biological reduction of graphene oxide using plant leaf extracts. Biotechnol. Prog. 30 (2), 463–469. doi:10.1002/btpr.1862
Lei, Z., Xu, S., and Wu, P. (2016). Ultra-thin and porous MoSe2nanosheets: Facile preparation and enhanced electrocatalytic activity towards the hydrogen evolution reaction. Phys. Chem. Chem. Phys. 18 (1), 70–74. doi:10.1039/C5CP06483J
Leong, W. S., Li, Y., Luo, X., Nai, C. T., Quek, S. Y., and Thong, J. T. L. (2015). Tuning the threshold voltage of MoS2field-effect transistors via surface treatment. Nanoscale 7 (24), 10823–10831. doi:10.1039/c5nr00253b
Li, Y., Wu, J., Yin, Z., and Zhang, H. (2014a). Preparation and applications of mechanically exfoliated single-layer and multilayer MoS2 and WSe2 nanosheets. Acc. Chem. Res. 47 (4), 1067–1075. doi:10.1021/ar4002312
Li, Y., Zhang, N., Yang, Y., Wang, G., and Ng, D. H. L. (2014d). High efficiency photocatalysis for pollutant degradation with MoS2/C3N4 heterostructures. Langmuir 30 (29), 8965–8972. doi:10.1021/la502033t
Li, R., Mansukhani, N. D., Guiney, L. M., Ji, Z., Zhao, Y., Chang, C. H., et al. (2016a). Identification and optimization of carbon radicals on hydrated graphene oxide for ubiquitous antibacterial coatings. ACS Nano 10, 10966–10980. doi:10.1021/acsnano.6b05692
Li, Y., Sun, Y., Dong, F., and Ho, W-K. (2014c). Enhancing the photocatalytic activity of bulk g-C3N4 by introducing mesoporous structure and hybridizing with graphene. J. Colloid Interface Sci. 436, 29–36. doi:10.1016/j.jcis.2014.09.004
Li, Y., Xu, L., Liu, Y., and Li, Y. (2014b). Graphdiyne and graphyne: From theoretical predictions to practical construction. Chem. Soc. Rev. 43, 2572–2586. doi:10.1039/c3cs60388a
Li, R., Yang, N., Du, T., Xia, T., Zhang, C., and Chen, W. (2016b). Chloramination of graphene oxide significantly affects its transport properties in saturated porous media. NanoImpact 3-4, 90–95. doi:10.1016/j.impact.2016.10.001
Li, Y., Zhang, H., Liu, P., Wang, D., Li, Y., and Zhao, H. (2013). Cross-linked g-C3 N4/rGO nanocomposites with tunable band structure and enhanced visible light photocatalytic activity. Small 9 (19), 3336–3344. doi:10.1002/smll.201203135
Liang, Y. T., Vijayan, B. K., Gray, K. A., and Hersam, M. C. (2011). Minimizing graphene defects enhances titania nanocomposite-based photocatalytic reduction of CO2 for improved solar fuel production. Nano Lett. 11 (7), 2865–2870. doi:10.1021/nl2012906
Lin, D., and Xing, B. (2007). Phytotoxicity of nanoparticles: Inhibition of seed germination and root growth. Environ. Pollut. 150 (2), 243–250. doi:10.1016/j.envpol.2007.01.016
Liu, B., Law, A. W-K., and Zhou, K. (2018). Strained single-layer C2N membrane for efficient seawater desalination via forward osmosis: A molecular dynamics study. J. Membr. Sci. 550, 554–562. doi:10.1016/j.memsci.2017.10.067
Liu, C., Kong, D., Hsu, P-C., Yuan, H., Lee, H.-W., Liu, Y., et al. (2016). Rapid water disinfection using vertically aligned MoS2 nanofilms and visible light. Nat. Nanotech 11 (12), 1098–1104. doi:10.1038/nnano.2016.138
Liu, H., Neal, A. T., Zhu, Z., Luo, Z., Xu, X., Tománek, D., et al. (2014a). Phosphorene: An unexplored 2D semiconductor with a high hole mobility. ACS Nano 8 (4), 4033–4041. doi:10.1021/nn501226z
Liu, H., Li, X-B., Wang, D., Lau, W-M., Peng, P., and Liu, L-M. (2014c). Diverse and tunable electronic structures of single-layer metal phosphorus trichalcogenides for photocatalytic water splitting. J. Chem. Phys. 140 (5), 054707. doi:10.1063/1.4863695
Liu, J., Zhang, T., Wang, Z., Dawson, G., and Chen, W. (2011a). Simple pyrolysis of urea into graphitic carbon nitride with recyclable adsorption and photocatalytic activity. J. Mat. Chem. 21 (38), 14398–14401. doi:10.1039/c1jm12620b
Liu, L., Ryu, S., Tomasik, M. R., Stolyarova, E., Jung, N., Hybertsen, M. S., et al. (2008). Graphene oxidation: Thickness-dependent etching and strong chemical doping. Nano Lett. 8 (7), 1965–1970. doi:10.1021/nl0808684
Liu, M., Li, H., Liu, S., Wang, L., Xie, L., Zhuang, Z., et al. (2022). Tailoring activation sites of metastable distorted 1T′-phase MoS2 by Ni doping for enhanced hydrogen evolution. Nano Res. 15 (7), 5946–5952. doi:10.1007/s12274-022-4267-9
Liu, N., Xu, K., Lei, Y., Xi, Y., Liu, Y., Wang, N., et al. (2021). Germanene nanosheets: Achieving superior sodium-ion storage via pseudointercalation reactions. Small Struct. 2 (10), 2100041. doi:10.1002/sstr.202100041
Liu, Q., Chen, B., Wang, Q., Shi, X., Xiao, Z., Lin, J., et al. (2009). Carbon nanotubes as molecular transporters for walled plant cells. Nano Lett. 9 (3), 1007–1010. doi:10.1021/nl803083u
Liu, J., Zeng, T. H., Hofmann, M., Burcombe, E., Wei, J., Jiang, R., et al. (2011b). Antibacterial activity of graphite, graphite oxide, graphene oxide, and reduced graphene oxide: Membrane and oxidative stress. ACS Nano 5 (9), 6971–6980. doi:10.1021/nn202451x
Liu, T., Shi, S., Liang, C., Shen, S., Cheng, L., Wang, C., et al. (2015a). Iron oxide decorated MoS2 nanosheets with double PEGylation for chelator-free radiolabeling and multimodal imaging guided photothermal therapy. ACS Nano 9 (1), 950–960. doi:10.1021/nn506757x
Liu, T., Gao, Y., Zhang, M., Zhang, X., Wang, S., and Feng, B. (2015b). Synthesis of fluorescent BCN hybrid nanosheets: A highly efficient fluorosensor for rapid, simple, sensitive Ag+ detection. RSC Adv. 5 (65), 52452–52458. doi:10.1039/C5RA10129H
Liu, X., Hou, Y., Tang, M., and Wang, L. (2023). Atom elimination strategy for MoS2 nanosheets to enhance photocatalytic hydrogen evolution. Chin. Chem. Lett. 34 (3), 107489. doi:10.1016/j.cclet.2022.05.003
Liu, H., Hu, J., Yue, C., Della Fera, N., Ling, Y., Mao, Z., et al. (2014b). High performance field-effect transistor based on multilayer tungsten disulfide. ACS Nano 8, 10396–10402. doi:10.1021/nn505253p
Liu, Y., Penev, E. S., and Yakobson, B. I. (2013). Probing the synthesis of two-dimensional boron by first-principles computations. Angew. Chem. 125 (11), 3238–3241. doi:10.1002/ange.201207972
Loh, K. P., Bao, Q., Eda, G., and Chhowalla, M. (2010). Graphene oxide as a chemically tunable platform for optical applications. Nat. Chem. 2 (12), 1015–1024. doi:10.1038/nchem.907
Lowry, G. V., Gregory, K. B., Apte, S. C., and Lead, J. R. (2012). Transformations of nanomaterials in the environment. Environ. Sci. Technol. 46 (13), 6893–6899. doi:10.1021/es300839e
Luo, Z., Li, Y., Zhong, M., Huang, Y., Wan, X., Peng, J., et al. (2015). Nonlinear optical absorption of few-layer molybdenum diselenide (MoSe_2) for passively mode-locked soliton fiber laser [Invited]. Phot. Res. 3 (3), A79–A86. doi:10.1364/PRJ.3.000A79
Ma, R., and Sasaki, T. (2010). Nanosheets of oxides and hydroxides: Ultimate 2D charge-bearing functional crystallites. Adv. Mat. 22 (45), 5082–5104. doi:10.1002/adma.201001722
Mahler, B., Hoepfner, V., Liao, K., and Ozin, G. (2014). Colloidal synthesis of 1T-WS2 and 2H-WS2 nanosheets: Applications for photocatalytic hydrogen evolution. J. Am. Chem. Soc. 136, 14121–14127. doi:10.1021/ja506261t
Mannix, A. J., Kiraly, B., Hersam, M. C., and Guisinger, N. P. (2017). Synthesis and chemistry of elemental 2D materials. Nat. Rev. Chem. 1 (2), 14. doi:10.1038/s41570-016-0014
Mannix, A. J., Zhou, X-F., and Kiraly, B. (2015). Synthesis of borophenes: Anisotropic, two-dimensional boron polymorphs. Science 350 (6267), 1513–1516.
Marsden, J. A., and Haley, M. M. (2005). Carbon networks based on dehydrobenzoannulenes. 5. Extension of two-dimensional conjugation in graphdiyne nanoarchitectures. J. Org. Chem. 70 (25), 10213–10226. doi:10.1021/jo050926v
Matsumoto, Y., Koinuma, M., Ida, S., Hayami, S., Taniguchi, T., Hatakeyama, K., et al. (2011). Photoreaction of graphene oxide nanosheets in water. J. Phys. Chem. C 115 (39), 19280–19286. doi:10.1021/jp206348s
Mei, L., Zhu, S., Yin, W., Chen, C., Nie, G., Gu, Z., et al. (2020). Two-dimensional nanomaterials beyond graphene for antibacterial applications: Current progress and future perspectives. Theranostics 10 (2), 757–781. doi:10.7150/thno.39701
Mesarič, T., Sepčič, K., and Piazza, V. (2013). Effects of nano carbon black and single-layer graphene oxide on settlement, survival and swimming behaviour of Amphibalanus amphitrite larvae. Chem. Ecol. 29 (7), 643–652.
Mohanty, N., Nagaraja, A., Armesto, J., and Berry, V. (2010). High-throughput, ultrafast synthesis of solution- dispersed graphene via a facile hydride chemistry. Small 6 (2), 226–231. doi:10.1002/smll.200901505
Molina-Barahona, L., Vega-Loyo, L., and Guerrero, M. (2005). Ecotoxicological evaluation of diesel-contaminated soil before and after a bioremediation process. Environ. Toxicol. 20 (1), 100–109.
Molle, A., Goldberger, J., Houssa, M., Xu, Y., Zhang, S-C., and Akinwande, D. (2017). Buckled two-dimensional Xene sheets. Nat. Mater 16 (2), 163–169. doi:10.1038/nmat4802
Molle, A., Grazianetti, C., Tao, L., Taneja, D., Alam, M. H., and Akinwande, D. (2018). Silicene, silicene derivatives, and their device applications. Chem. Soc. Rev. 47 (16), 6370–6387. doi:10.1039/c8cs00338f
Naguib, M., Kurtoglu, M., Presser, V., Lu, J., Niu, J., Heon, M., et al. (2011). Two-dimensional nanocrystals produced by exfoliation of Ti3AlC2. Adv. Mat. 23 (37), 4248–4253. doi:10.1002/adma.201102306
Naguib, M., Mochalin, O., Barsoum, M. W., and Gogotsi, Y. (2014). 25th anniversary article: MXenes: A new family of two-dimensional materials. Adv. Mat. 26, 992–1005. doi:10.1002/chin.20141723210.1002/adma.201304138
Ng, S., Sturala, J., Vyskocil, J., Lazar, P., Martincova, J., Plutnar, J., et al. (2021). Two-dimensional functionalized germananes as photoelectrocatalysts. ACS Nano 15 (7), 11681–11693. doi:10.1021/acsnano.1c02327
Nicolosi, V., Chhowalla, M., Kanatzidis, M. G., Strano, M. S., and Coleman, J. N. (2013). Liquid exfoliation of layered materials. Science 340, 1226419. doi:10.1126/science.1226419
Notley, S. M. (2013). High yield production of photoluminescent tungsten disulphide nanoparticles. J. Colloid Interface Sci. 396, 160–164. doi:10.1016/j.jcis.2013.01.035
Novoselov, K. S., Fal′ko, V. I., Colombo, L., Gellert, P. R., Schwab, M. G., and Kim, K. (2012). A roadmap for graphene. Nature 490 (7419), 192–200. doi:10.1038/nature11458
Novoselov, K. S., Geim, A. K., and Morozov, S. V. (2004). Electric field effect in atomically thin carbon films. Science 306 (5696), 666–669.
Osada, M., and Sasaki, T. (2009). Exfoliated oxide nanosheets: New solution to nanoelectronics. J. Mat. Chem. 19 (17), 2503. doi:10.1039/b820160a
Pan, C., Xu, J., Wang, Y., Li, D., and Zhu, Y. (2012). Dramatic activity of C3N4/BiPO4 photocatalyst with core/shell structure formed by self-assembly. Adv. Funct. Mat. 22 (7), 1518–1524. doi:10.1002/adfm.201102306
Paret, M. L., Vallad, G. E., Averett, D. R., Jones, J. B., and Olson, S. M. (2013). Photocatalysis: Effect of light-activated nanoscale formulations of TiO2 on xanthomonas perforans and control of bacterial spot of tomato. Phytopathology 103 (3), 228–236. doi:10.1094/phyto-08-12-0183-r
Parvez, K., Wu, Z-S., Li, R., Liu, X., Graf, R., Feng, X., et al. (2014). Exfoliation of graphite into graphene in aqueous solutions of inorganic salts. J. Am. Chem. Soc. 136 (16), 6083–6091. doi:10.1021/ja5017156
Parzinger, E., Miller, B., Blaschke, B., Garrido, J. A., Ager, J. W., Holleitner, A., et al. (2015). Photocatalytic stability of single- and few-layer MoS2. ACS Nano 9 (11), 11302–11309. doi:10.1021/acsnano.5b04979
Pathakoti, K., Morrow, S., Han, C., Pelaez, M., He, X., Dionysiou, D. D., et al. (2013). Photoinactivation of Escherichia coli by sulfur-doped and nitrogen-fluorine-codoped TiO2 nanoparticles under solar simulated light and visible light irradiation. Environ. Sci. Technol. 47 (17), 9988–9996. doi:10.1021/es401010g
Paton, K. R., Varrla, E., Backes, C., Smith, R. J., Khan, U., O’Neill, A., et al. (2014). Scalable production of large quantities of defect-free few-layer graphene by shear exfoliation in liquids. Nat. Mater 13, 624–630. doi:10.1038/nmat3944
Penev, E. S., Bhowmick, S., Sadrzadeh, A., and Yakobson, B. I. (2012). Polymorphism of two-dimensional boron. Nano Lett. 12 (5), 2441–2445. doi:10.1021/nl3004754
Peng, Y., Li, Y., Ban, Y., Jin, H., Jiao, W., Liu, X., et al. (2014). Metal-organic framework nanosheets as building blocks for molecular sieving membranes. Science 346 (6215), 1356–1359. doi:10.1126/science.1254227
Pham, V. H., Cuong, T. V., Hur, S. H., Oh, E., Kim, E. J., Shin, E. W., et al. (2011). Chemical functionalization of graphene sheets by solvothermal reduction of a graphene oxide suspension in N-methyl-2-pyrrolidone. J. Mat. Chem. 21 (10), 3371–3377. doi:10.1039/C0JM02790A
Pizzi, G., Gibertini, M., Dib, E., Marzari, N., Iannaccone, G., and Fiori, G. (2016). Performance of arsenene and antimonene double-gate MOSFETs from first principles. Nat. Commun. 7, 12585. doi:10.1038/ncomms12585
Pradhan, N. R., Rhodes, D., Xin, Y., Memaran, S., Bhaskaran, L., Siddiq, M., et al. (2014). Ambipolar molybdenum diselenide field-effect transistors: Field-effect and hall mobilities. ACS Nano 8 (8), 7923–7929. doi:10.1021/nn501693d
Pumera, M., and Sofer, Z. (2017). 2D monoelemental arsenene, antimonene, and bismuthene: Beyond black phosphorus. Adv. Mat. 29 (21), 1605299. doi:10.1002/adma.201605299
Qian, X., Shen, S., Liu, T., Cheng, L., and Liu, Z. (2015). Two-dimensional TiS2 nanosheets for in vivo photoacoustic imaging and photothermal cancer therapy. Nanoscale 7 (14), 6380–6387. doi:10.1039/C5NR00893J
Qiu, Z., Yang, Q., and Liu, W. (2013). Photocatalytic degradation of phytotoxic substances in waste nutrient solution by various immobilized levels of nano-TiO2. Water Air Soil Pollut. 224 (3), 1461. doi:10.1007/s11270-013-1461-0
Quinn, M. D. J., Ho, N. H., and Notley, S. M. (2013). Aqueous dispersions of exfoliated molybdenum disulfide for use in visible-light photocatalysis. ACS Appl. Mat. Interfaces 5 (23), 12751–12756. doi:10.1021/am404161k
Radisavljevic, B., Radenovic, A., Brivio, J., Giacometti, V., and Kis, A. (2011). Single-layer MoS2 transistors. Nat. Nanotech 6 (3), 147–150. doi:10.1038/nnano.2010.279
Reddy, K. R., Reddy, C. H. V., Nadagouda, M. N., Shetti, N. P., Jaesool, S., and Aminabhavi, T. M. (2019). Polymeric graphitic carbon nitride (g-C3N4)-based semiconducting nanostructured materials: Synthesis methods, properties and photocatalytic applications. J. Environ. Manag. 238, 25–40. doi:10.1016/j.jenvman.2019.02.075
Ren, X., Li, J., Tan, X., Shi, W., Chen, C., Shao, D., et al. (2014). Impact of Al2O3 on the aggregation and deposition of graphene oxide. Environ. Sci. Technol. 48 (10), 5493–5500. doi:10.1021/es404996b
Robinson, J. T., Burgess, J. S., Junkermeier, C. E., Badescu, S. C., Reinecke, T. L., Perkins, F. K., et al. (2010). Properties of fluorinated graphene films. Nano Lett. 10 (8), 3001–3005. doi:10.1021/nl101437p
Rodenas, T., Luz, I., Prieto, G., Seoane, B., Miro, H., Corma, A., et al. (2015). Metal-organic framework nanosheets in polymer composite materials for gas separation. Nat. Mater 14 (1), 48–55. doi:10.1038/nmat4113
Rosenfeldt, R. R., Seitz, F., Schulz, R., and Bundschuh, M. (2014). Heavy metal uptake and toxicity in the presence of titanium dioxide nanoparticles: A factorial approach using Daphnia magna. Environ. Sci. Technol. 48 (12), 6965–6972. doi:10.1021/es405396a
Ruiz-León, D., Manriquez, V., Kasaneva, J., and Avila, R. E. (2002). Insertion of trivalent cations in the layered MPS3 (Mn, Cd) materials. Mater Res. Bull. 37 (5), 981–989. doi:10.1016/S0025-5408(02)00719-5
Saadi, F. H., Carim, A. I., Velazquez, J. M., Baricuatro, J. H., McCrory, C. C. L., Soriaga, M. P., et al. (2014). Operando synthesis of macroporous molybdenum diselenide films for electrocatalysis of the hydrogen-evolution reaction. ACS Catal. 4 (9), 2866–2873. doi:10.1021/cs500412u
Sainsbury, T., Satti, A., May, P., Wang, Z., McGovern, I., Gun’ko, Y. K., et al. (2012). Oxygen radical functionalization of boron nitride nanosheets. J. Am. Chem. Soc. 134 (45), 18758–18771. doi:10.1021/ja3080665
Salas, E. C., Sun, Z., Lüttge, A., and Tour, J. M. (2010). Reduction of graphene oxide via bacterial respiration. ACS Nano 4 (8), 4852–4856. doi:10.1021/nn101081t
Salomon, E., and Kahn, A. (2008). One-dimensional organic nanostructures: A novel approach based on the selective adsorption of organic molecules on silicon nanowires. Surf. Sci. 602 (13), L79–L83. doi:10.1016/j.susc.2008.04.023
Sanchez, V. C., Jachak, A., Hurt, R. H., and Kane, A. B. (2012). Biological interactions of graphene-family nanomaterials: An interdisciplinary review. Chem. Res. Toxicol. 25 (1), 15–34. doi:10.1021/tx200339h
Sano, T., Tsutsui, S., Koike, K., Hirakawa, T., Teramoto, Y., Negishi, N., et al. (2013). Activation of graphitic carbon nitride (g-C3N4) by alkaline hydrothermal treatment for photocatalytic NO oxidation in gas phase. J. Mat. Chem. A 1 (21), 6489–6496. doi:10.1039/c3ta10472a
Shah, P., Narayanan, T. N., Li, C. Z., and Alwarappan, S. (2015). Probing the biocompatibility of MoS 2 nanosheets by cytotoxicity assay and electrical impedance spectroscopy. Nanotechnology 26 (31), 315102. doi:10.1088/0957-4484/26/31/315102
Shams, M., Guiney, L. M., Huang, L., Ramesh, M., Yang, X., Hersam, M. C., et al. (2019). Influence of functional groups on the degradation of graphene oxide nanomaterials. Environ. Sci. Nano 6 (7), 2203–2214. doi:10.1039/C9EN00355J
Shao, Y., Wang, J., Engelhard, M., Wang, C., and Lin, Y. (2010). Facile and controllable electrochemical reduction of graphene oxide and its applications. J. Mat. Chem. 20, 743–748. doi:10.1039/b917975e
Shastry, T. A., Balla, I., Bergeron, H., Amsterdam, S. H., Marks, T. J., and Hersam, M. C. (2016). Mutual photoluminescence quenching and photovoltaic effect in large-area single-layer MoS2-polymer heterojunctions. ACS Nano 10 (11), 10573–10579. doi:10.1021/acsnano.6b06592
Shen, Y., Zhang, H-B., Zhang, H., Ren, W., Dasari, A., Tang, G.-S., et al. (2013). Structural evolution of functionalized graphene sheets during solvothermal reduction. Carbon 56, 132–138. doi:10.1016/j.carbon.2012.12.088
Song, S. H., Kim, B. H., Choe, D-H., Kim, J., Kim, D. C., Lee, D. J., et al. (2015). Bandgap widening of phase quilted, 2D MoS2by oxidative intercalation. Adv. Mat. 27 (20), 3152–3158. doi:10.1002/adma.201500649
Song, U., Shin, M., Lee, G., Roh, J., Kim, Y., and Lee, E. J. (2013). Functional analysis of TiO2 nanoparticle toxicity in three plant species. Biol. Trace Elem. Res. 155 (1), 93–103. doi:10.1007/s12011-013-9765-x
Song, Y., Chen, Y., Jiang, X., Liang, W., Wang, K., Liang, Z., et al. (2018). Nonlinear few-layer antimonene-based all-optical signal processing: Ultrafast optical switching and high-speed wavelength conversion. Adv. Opt. Mater. 6, 1701287. doi:10.1002/adom.201701287
Song, Y., Liang, Z., and Jiang, X. (2017). Few-layer antimonene decorated microfiber: Ultra-short pulse generation and all-optical thresholding with enhanced long term stability. 2D Mat. 4 (4), 45010. doi:10.1088/2053-1583/aa87c1
Splendiani, A., Sun, L., Zhang, Y., Li, T., Kim, J., Chim, C.-Y., et al. (2010). Emerging photoluminescence in monolayer MoS2. Nano Lett. 10 (4), 1271–1275. doi:10.1021/nl903868w
Stampoulis, D., Sinha, S. K., and White, J. C. (2009). Assay-dependent phytotoxicity of nanoparticles to plants. Environ. Sci. Technol. 43 (24), 9473–9479. doi:10.1021/es901695c
Su, F., Antonietti, M., and Wang, X. (2012). mpg-C3N4 as a solid base catalyst for Knoevenagel condensations and transesterification reactions. Catal. Sci. Technol. 2 (5), 1005–1009. doi:10.1039/c2cy00012a
Sun, L., Ying, Y., Huang, H., Song, Z., Mao, Y., Xu, Z., et al. (2014). Ultrafast molecule separation through layered WS2 nanosheet membranes. ACS Nano 8 (6), 6304–6311. doi:10.1021/nn501786m
Tan, C., Cao, X., Wu, X-J., He, Q., Yang, J., Zhang, X., et al. (2017). Recent advances in ultrathin two-dimensional nanomaterials. Chem. Rev. 117 (9), 6225–6331. doi:10.1021/acs.chemrev.6b00558
Tan, C., Qi, X., Huang, X., Yang, J., Zheng, B., An, Z., et al. (2014). Single-layer transition metal dichalcogenide nanosheet-assisted assembly of aggregation-induced emission molecules to form organic nanosheets with enhanced fluorescence. Adv. Mat. 26 (11), 1735–1739. doi:10.1002/adma.201304562
Tang, H., and Ismail-Beigi, S. (2007). Novel precursors for boron nanotubes: The competition of two-center and three-center bonding in boron sheets. Phys. Rev. Lett. 99 (11), 115501. doi:10.1103/physrevlett.99.115501
Teo, T. W., Chng, C. E. L., Sofer, S., and Pumera, P. (2014). Cytotoxicity of Exfoliated Transition-Metal Dichalcogenides (MoS2, WS2, and WSe2) is Lower Than That of Graphene and its Analogues. Chem. Eur. J. 20 (31), 9627–9632. doi:10.1002/chem.201402680
Terrones, Z. L. (2016). 2D materials advances: From large scale synthesis and controlled heterostructures to improved characterization techniques, defects and applications. 2D Mater 3 (4), 42001.
Tong, Z., Yang, D., Shi, J., Nan, Y., Sun, Y., and Jiang, Z. (2015). Three-dimensional porous aerogel constructed by g-C3N4 and graphene oxide nanosheets with excellent visible-light photocatalytic performance. ACS Appl. Mat. Interfaces 7 (46), 25693–25701. doi:10.1021/acsami.5b09503
Tributsch, H. (1978). Hole reactions from d-energy bands of layer type group VI transition metal dichalcogenides: New perspectives for electrochemical solar energy conversion. J. Electrochem. Soc. 125 (7), 1086–1093. doi:10.1149/1.2131625
Venkataraman, N. V., Mohanambe, L., and Vasudevan, S. (2003). Functionalization of the internal surfaces of layered cadmium thiophosphate with cationic surfactants: Adsolubilization of uncharged organic molecules. J. Mat. Chem. 13 (2), 170–171. doi:10.1039/B211541G
Vogt, P., De Padova, P., Quaresima, C., Avila, J., Frantzeskakis, E., Asensio, M. C., et al. (2012). Silicene: Compelling experimental evidence for graphenelike two-dimensional silicon. Phys. Rev. Lett. 108 (15), 1–5. doi:10.1103/PhysRevLett.108.155501
Voiry, D., Yamaguchi, H., Li, J., Silva, R., Alves, D. C. B., Fujita, T., et al. (2013). Enhanced catalytic activity in strained chemically exfoliated WS2 nanosheets for hydrogen evolution. Nat. Mater 12 (9), 850–855. doi:10.1038/nmat3700
Wan, W., Yu, S., Dong, F., Zhang, Q., and Zhou, Y. (2016). Efficient C3N4/graphene oxide macroscopic aerogel visible-light photocatalyst. J. Mat. Chem. A 4 (20), 7823–7829. doi:10.1039/c6ta01804a
Wan, W. B., and Haley, M. M. (2001). Carbon networks based on dehydrobenzoannulenes. 4. Synthesis of "star" and "trefoil" graphdiyne substructures via sixfold cross-coupling of hexaiodobenzene. J. Org. Chem. 66 (11), 3893–3901. doi:10.1021/jo010183n
Wang, C., Zhang, L., Guo, Z., Xu, J., Wang, H., Zhai, K., et al. (2010). A novel hydrazine electrochemical sensor based on the high specific surface area graphene. Microchim. Acta 169 (1), 1–6. doi:10.1007/s00604-010-0304-6
Wang, G., Pandey, R., and Karna, S. P. (2015a). Atomically thin group V elemental films: Theoretical investigations of antimonene allotropes. ACS Appl. Mat. Interfaces 7 (21), 11490–11496. doi:10.1021/acsami.5b02441
Wang, G., Pandey, R., and Karna, S. P. (2017). Physics and chemistry of oxidation of two-dimensional nanomaterials by molecular oxygen. Wiley Interdiscip. Rev. Comput. Mol. Sci. 7 (1), e1280. doi:10.1002/wcms.1280
Wang, G., Qian, F., Saltikov, C. W., Jiao, Y., and Li, Y. (2011b). Microbial reduction of graphene oxide by Shewanella. Nano Res. 4 (6), 563–570. doi:10.1007/s12274-011-0112-2
Wang, G., Yang, J., Park, J., Gou, X., Wang, B., Liu, H., et al. (2008). Facile synthesis and characterization of graphene nanosheets. J. Phys. Chem. C 112 (22), 8192–8195. doi:10.1021/jp710931h
Wang, J., Chen, S., Quan, X., Zhao, H., and Zhao, Y. (2007). Enhanced photodegradation of PNP on soil surface under UV irradiation with TiO2. Soil Sediment Contam. An Int. J. 16 (4), 413–421. doi:10.1080/15320380701404607
Wang, L., and Sasaki, T. (2014). Titanium oxide nanosheets: Graphene analogues with versatile functionalities. Chem. Rev. 114 (19), 9455–9486. doi:10.1021/cr400627u
Wang, Q. H., Kalantar-Zadeh, K., Kis, A., Coleman, J. N., and Strano, M. S. (2012a). Electronics and optoelectronics of two-dimensional transition metal dichalcogenides. Nat. Nanotech 7 (11), 699–712. doi:10.1038/nnano.2012.193
Wang, Q. H., Yi, L., Halpert, J. E., Lai, X., Liu, Y., Cao, H., et al. (2012b). A novel and highly efficient photocatalyst based on P25-graphdiyne nanocomposite. Small 8 (2), 265–271. doi:10.1002/smll.201101686
Wang, Q. H., Zhang, L., Xia, Z., Roy, A., Chang, D. W., Baek, J., et al. (2012c). BCN graphene as efficient metal-free electrocatalyst for the oxygen reduction reaction. Angew. Chem. 124, 4285–4288. doi:10.1002/ange.201109257
Wang, W., Yu, J. C., Xia, D., Wong, P. K., and Li, Y. (2013). Graphene and g-C3N4Nanosheets cowrapped elemental α-sulfur as a novel metal-free heterojunction photocatalyst for bacterial inactivation under visible-light. Environ. Sci. Technol. 47 (15), 8724–8732. doi:10.1021/es4013504
Wang, G., Maeda, K., and Thomas, A. (2011a). “A metal-free polymeric photocatalyst for hydrogen production from water under visible light,” in Materials for sustainable energy: A collection of peer-reviewed research and review articles from nature publishing group (United States: World Scientific), 271–275.
Wang, G., Mansukhani, N. D., Guiney, L. M., Ji, Z., Chang, C. H., Wang, M., et al. (2015b). Differences in the toxicological potential of 2D versus aggregated molybdenum disulfide in the lung. Small 11 (38), 5079–5087. doi:10.1002/smll.201500906
Wang, Z., Dong, Z., Gu, Y., Chang, Y.-H., Zhang, L., Li, L.-J., et al. (2016a). Giant photoluminescence enhancement in tungsten-diselenide-gold plasmonic hybrid structures. Nat. Commun. 7, 11283. doi:10.1038/ncomms11283
Wang, Z., and Zhao, J. (2020). Tuning the electronic structures of monolayer triphosphides MP3 (M = Sn and Ge) for CO2 electroreduction through interface engineering: A theoretical prediction. Phys. Chem. Chem. Phys. 22 (13), 6896–6905. doi:10.1039/d0cp00062k
Wang, Z., Zhu, W., Qiu, Y., Yi, X., von dem Bussche, A., Kane, A., et al. (2016b). Biological and environmental interactions of emerging two-dimensional nanomaterials. Chem. Soc. Rev. 45 (6), 1750–1780. doi:10.1039/C5CS00914F
Wassei, J. K., and Kaner, R. B. (2010). Graphene, a promising transparent conductor. Mater. Today 13 (3), 52–59. doi:10.1016/S1369-7021(10)70034-1
Westreich, P., Yang, D., and Frindt, R. F. (2006). Host layer buckling in the compounds formed by exfoliation and restacking of cadmium phosphorus trisulphide with adenosine monophosphate included. Mater. Res. Bull. 41 (3), 502–514. doi:10.1016/j.materresbull.2005.09.018
Williams, G., Seger, B., and Kamat, P. V. (2008). TiO2-Graphene nanocomposites. UV-assisted photocatalytic reduction of graphene oxide. ACS Nano 2 (7), 1487–1491. doi:10.1021/nn800251f
Wood, J. D., Wells, S. A., Jariwala, D., Chen, K.-S., Cho, E., Sangwan, V. K., et al. (2014). Effective passivation of exfoliated black phosphorus transistors against ambient degradation. Nano Lett. 14, 6964–6970. doi:10.1021/nl5032293
Wu, H-H., Huang, H., Zhong, J., Yu, S., Zhang, Q., and Zeng, X. C. (2019). Monolayer triphosphates MP3 (M = Sn, Ge) with excellent basal catalytic activity for hydrogen evolution reaction. Nanoscale 11 (25), 12210–12219. doi:10.1039/c9nr03255j
Wu, J. Y., Lin, M. N., Wang, L., and Zhang, T. (2014). Photoluminescence of MoS2Prepared by effective grinding-assisted sonication exfoliation. J. Nanomater. 2014, 1–7. doi:10.1155/2014/852735
Wu, L., Liu, L., Gao, B., Muñoz-Carpena, R., Zhang, M., Chen, H., et al. (2013). Aggregation kinetics of graphene oxides in aqueous solutions: Experiments, mechanisms, and modeling. Langmuir 29, 15174–15181. doi:10.1021/la404134x
Wu, N., Yu, Y., Li, T., Ji, X., Jiang, L., Zong, J., et al. (2016). Investigating the influence of MoS2 nanosheets on E. coli from metabolomics level. PLoS One 11 (12), e0167245. doi:10.1371/journal.pone.0167245
Wu, S. G., Huang, L., Head, J., Chen, D. R., Kong, I-C., and Tang, Y. J. (2012b). Phytotoxicity of metal oxide nanoparticles is related to both dissolved metals ions and adsorption of particles on seed surfaces. J. Pet. Env. Biotechnol. 3 (4), 126.
Wu, S. G., Dai, J., Zhao, Y., Zhuo, Z., Yang, J., and Zeng, X. C. (2012a). Two-dimensional boron monolayer sheets. ACS Nano 6 (8), 7443–7453. doi:10.1021/nn302696v
Xi, Y., Jing, X., Xu, Z., Liu, N., Liu, Y., Lin, M.-L., et al. (2022). Superconductivity in layered van der Waals hydrogenated germanene at high pressure. J. Am. Chem. Soc. 144 (41), 18887–18895. doi:10.1021/jacs.2c05683
Xu, X., Yao, W., Xiao, D., and Heinz, T. F. (2014). Spin and pseudospins in layered transition metal dichalcogenides. Nat. Phys. 10, 343–350. doi:10.1038/nphys2942
Yan, S. C., Li, Z. S., and Zou, Z. G. (2009). Photodegradation performance of g-C3N4 fabricated by directly heating melamine. Langmuir 25 (17), 10397–10401. doi:10.1021/la900923z
Yang, F., Hong, F., You, W., Liu, C., Gao, F., Wu, C., et al. (2006). Influences of nano-anatase TiO2 on the nitrogen metabolism of growing spinach. Bter 110 (2), 179–190. doi:10.1385/bter:110:2:179
Yang, M., Xu, Y., Zhang, X., Bisoyi, H. K., Xue, P., Yang, Y., et al. (2022). Bioinspired phototropic MXene-reinforced soft tubular actuators for omnidirectional light-tracking and adaptive photovoltaics. Adv. Funct. Mater. 32 (26), 2201884. doi:10.1002/adfm.202201884
Yang, N., Liu, Y., Wen, H., Tang, Z., Zhao, H., Li, Y., et al. (2013). Photocatalytic properties of graphdiyne and graphene modified TiO2: From theory to experiment. ACS Nano 7 (2), 1504–1512. doi:10.1021/nn305288z
Yang, X., Ding, Y., and Ni, J. (2008). Ab initioprediction of stable boron sheets and boron nanotubes: Structure, stability, and electronic properties. Phys. Rev. B 77 (4), 41402. doi:10.1103/physrevb.77.041402
Yang, X., Li, J., Liang, T., Ma, C., Zhang, Y., Chen, H., et al. (2014). Antibacterial activity of two-dimensional MoS2 sheets. Nanoscale 6 (17), 10126–10133. doi:10.1039/c4nr01965b
Ye, S., Qiu, L-G., Yuan, Y-P., Zhu, Y-J., Xia, J., and Zhu, J-F. (2013). Facile fabrication of magnetically separable graphitic carbon nitride photocatalysts with enhanced photocatalytic activity under visible light. J. Mat. Chem. A 1 (9), 3008–3015. doi:10.1039/C2TA01069K
Yi, M., and Shen, Z. (2014). Kitchen blender for producing high-quality few-layer graphene. Carbon 78, 622–626. doi:10.1016/j.carbon.2014.07.035
Yin, X., Feng, Y., Zhao, Q., Li, Y., Li, S., Dong, H., et al. (2018). Highly transparent, strong, and flexible fluorographene/fluorinated polyimide nanocomposite films with low dielectric constant. J. Mat. Chem. C 6 (24), 6378–6384. doi:10.1039/c8tc00998h
Yu, Y., Li, C., Liu, Y., Su, L., Zhang, Y., and Cao, L. (2013). Controlled scalable synthesis of uniform, high-quality monolayer and few-layer MoS2 films. Sci. Rep. 3 (1866), 1–6. doi:10.1038/srep01866
Yuan, C., Wu, H. B., Xie, Y., and Lou, X. W. (2014). Mixed transition-metal oxides: Design, synthesis, and energy-related applications. Angew. Chem. Int. Ed. 53 (6), 1488–1504. doi:10.1002/anie.201303971
Yue, Y., Chen, J., Zhang, Y., Ding, S., Zhao, F., Wang, Y., et al. (2018). Two-dimensional high-quality monolayered triangular WS2 flakes for field-effect transistors. ACS Appl. Mat. Interfaces 10 (26), 22435–22444. doi:10.1021/acsami.8b05885
Zhang, B., Tang, D., Liu, B., Chen, H., Cui, Y., and Chen, G. (2011). GoldMag nanocomposite-functionalized graphene sensing platform for one-step electrochemical immunoassay of alpha-fetoprotein. Biosens. Bioelectron. 28 (1), 174–180. doi:10.1016/j.bios.2011.07.016
Zhang, H., Liu, B., Kieu, H. T., Wu, M. S., Zhou, K., and Law, A. W-K. (2018). Coarse-grained molecular dynamics study of membrane distillation through meso-size graphene channels. J. Membr. Sci. 558, 34–44. doi:10.1016/j.memsci.2018.04.043
Zhang, H., Lv, X., Li, Y., Wang, Y., and Li, J. (2010). P25-graphene composite as a high performance photocatalyst. ACS Nano 4 (1), 380–386. doi:10.1021/nn901221k
Zhang, H. (2015). Ultrathin two-dimensional nanomaterials. ACS Nano 9 (10), 9451–9469. doi:10.1021/acsnano.5b05040
Zhang, M., Wu, J., Zhu, Y., Dumcenco, D. O., Hong, J., Mao, N., et al. (2014). Two-dimensional molybdenum tungsten diselenide alloys: Photoluminescence, Raman scattering, and electrical transport. ACS Nano 8 (7), 7130–7137. doi:10.1021/nn5020566
Zhang, S., Yan, Z., Li, Y., Chen, Z., and Zeng, H. (2015a). Atomically thin arsenene and antimonene: Semimetal-semiconductor and indirect-direct band-gap transitions. Angew. Chem. 127 (10), 3155–3158. doi:10.1002/ange.201411246
Zhang, S., Zhou, W., Ma, Y., Ji, J., Cai, B., Yang, S. A., et al. (2017b). Antimonene oxides: Emerging tunable direct bandgap semiconductor and novel topological insulator. Nano Lett. 17 (6), 3434–3440. doi:10.1021/acs.nanolett.7b00297
Zhang, S., Rubio, A., and Le Lay, G. (2017a). Emergent elemental two-dimensional materials beyond graphene. J. Phys. D. Appl. Phys. 50 (5), 53004. doi:10.1088/1361-6463/aa4e8b
Zhang, S., Huang, J., Zhang, M., Yuan, Q., and Dong, B. (2015b). Ultrathin hexagonal SnS2 nanosheets coupled with g-C3N4 nanosheets as 2D/2D heterojunction photocatalysts toward high photocatalytic activity. Appl. Catal. B Environ. 163, 298–305. doi:10.1016/j.apcatb.2014.08.013
Zhao, F., Feng, Y., and Feng, W. (2022). Germanium-based monoelemental and binary two-dimensional materials: Theoretical and experimental investigations and promising applications. Infomat 4 (11), e12365. doi:10.1002/inf2.12365
Zhao, F., Feng, Y., and Wang, Y. (2020a). Two-dimensional gersiloxenes with tunable bandgap for photocatalytic H2 evolution and CO2 photoreduction to CO. Nat. Commun. 11 (1), 1–13. doi:10.1038/s41467-020-15262-4
Zhao, F., Wang, Y., Zhang, X., Liang, X., Zhang, F., Wang, L., et al. (2020b). Few-layer methyl-terminated germanene-graphene nanocomposite with high capacity for stable lithium storage. Carbon 161, 287–298. doi:10.1016/j.carbon.2020.01.072
Zhao, H., Yu, H., Quan, X., Chen, S., Zhang, Y., Zhao, H., et al. (2014). Fabrication of atomic single layer graphitic-C3N4 and its high performance of photocatalytic disinfection under visible light irradiation. Appl. Catal. B Environ. 152-153, 46–50. doi:10.1016/j.apcatb.2014.01.023
Zhao, J., Liu, H., Yu, Z., Quhe, R., Zhou, S., Wang, Y., et al. (2016). Rise of silicene: A competitive 2D material. Prog. Mater. Sci. 83, 24–151. doi:10.1016/j.pmatsci.2016.04.001
Zhao, X., Zhang, Q., Chen, D., and Lu, P. (2010). Enhanced mechanical properties of graphene-based poly(vinyl alcohol) composites. Macromolecules 43 (5), 2357–2363. doi:10.1021/ma902862u
Zheng, L., Hong, F., Lu, S., and Liu, C. (2005). Effect of nano-TiO(2) on strength of naturally aged seeds and growth of spinach. Biol. Trace Elem. Res. 104 (1), 83–92. doi:10.1385/BTER:104:1:083
Zhi, C., Bando, Y., Tang, C., Kuwahara, H., and Golberg, D. (2009). Large-scale fabrication of boron nitride nanosheets and their utilization in polymeric composites with improved thermal and mechanical properties. Adv. Mat. 21 (28), 2889–2893. doi:10.1002/adma.200900323
Zhou, X., Zhang, Y., Wang, C., Wu, X., Yang, Y., Zheng, B., et al. (2012). Photo-fenton reaction of graphene oxide: A new strategy to prepare graphene quantum dots for DNA cleavage. ACS Nano 6, 6592–6599. doi:10.1021/nn301629v
Zhou, Y., Bao, Q., Tang, L. A. L., Zhong, Y., and Loh, K. P. (2009). Hydrothermal dehydration for the “green” reduction of exfoliated graphene oxide to graphene and demonstration of tunable optical limiting properties. Chem. Mat. 21 (13), 2950–2956. doi:10.1021/cm9006603
Zhu, C., Du, D., and Lin, Y. (2015). Graphene and graphene-like 2D materials for optical biosensing and bioimaging: A review. 2D Mat. 2 (1), 032004–032086. doi:10.1088/2053-1583/2/3/032004
Zhu, J., Wei, Y., Chen, W., Zhao, Z., and Thomas, A. (2010). Graphitic carbon nitride as a metal-free catalyst for NO decomposition. Chem. Commun. 46 (37), 6965–6967. doi:10.1039/c0cc01432j
Zhu, J., Xiao, D., Li, J., Xie, X., Yang, X., and Wu, Y. (2005). Recycle—New possible mechanism of NO decomposition over perovskite (-like) oxides. J. Mol. Catal. A Chem. 233 (1), 29–34. doi:10.1016/j.molcata.2005.02.011
Zhu, J., Xiao, P., Li, H., and Carabineiro, S. A. C. (2014). Graphitic carbon nitride: Synthesis, properties, and applications in catalysis. ACS Appl. Mat. Interfaces 6 (19), 16449–16465. doi:10.1021/am502925j
Zhu, J., Yang, X., Xu, X., and Wei, K. (2007). Active site structure of NO decomposition on perovskite(-like) oxides: An investigation from experiment and density functional theory. J. Phys. Chem. C 111 (3), 1487–1490. doi:10.1021/jp0662101
Keywords: two dimensional nanomaterials, environmental implications, sustainability, graphene, emerging two dimensional nanomaterials
Citation: Shams M, Mansukhani N, Hersam MC, Bouchard D and Chowdhury I (2023) Environmentally sustainable implementations of two-dimensional nanomaterials. Front. Chem. 11:1132233. doi: 10.3389/fchem.2023.1132233
Received: 27 December 2022; Accepted: 14 February 2023;
Published: 03 March 2023.
Edited by:
Longlu Wang, Nanjing University of Posts and Telecommunications, ChinaReviewed by:
Yin Weinan, Nanjing University of Posts and Telecommunications, ChinaFulai Zhao, Tianjin University, China
Copyright © 2023 Shams, Mansukhani, Hersam, Bouchard and Chowdhury. This is an open-access article distributed under the terms of the Creative Commons Attribution License (CC BY). The use, distribution or reproduction in other forums is permitted, provided the original author(s) and the copyright owner(s) are credited and that the original publication in this journal is cited, in accordance with accepted academic practice. No use, distribution or reproduction is permitted which does not comply with these terms.
*Correspondence: Indranil Chowdhury, Indranil.chowdhury@wsu.edu
†These authors have contributed equally to this work