Carbon Based Nanodots in Early Diagnosis of Cancer
- 1University Institute of Pharmaceutical Sciences, Panjab University, Chandigarh, India
- 2Department of Biophysics, Panjab University, Chandigarh, India
- 3Department of Biotechnology, Panjab University, Chandigarh, India
Detection of cancer at an early stage is one of the principal factors associated with successful treatment outcome. However, current diagnostic methods are not capable of making sensitive and robust cancer diagnosis. Nanotechnology based products exhibit unique physical, optical and electrical properties that can be useful in diagnosis. These nanotech-enabled diagnostic representatives have proved to be generally more capable and consistent; as they selectively accumulated in the tumor site due to their miniscule size. This article rotates around the conventional imaging techniques, the use of carbon based nanodots viz Carbon Quantum Dots (CQDs), Graphene Quantum Dots (GQDs), Nanodiamonds, Fullerene, and Carbon Nanotubes that have been synthesized in recent years, along with the discovery of a wide range of biomarkers to identify cancer at early stage. Early detection of cancer using nanoconstructs is anticipated to be a distinct reality in the coming years.
Introduction
Cancer remains among the world’s most devastating diseases with about 20 million cases and 10 million deaths reported as of 2020. The disease is perceived by the condition wherein cells divide uncontrollably and attack different tissues. Most prevalent cancers include breast (11.6%), lung (11.4%), colorectal (10.0%), prostate (7.3%), and stomach cancer (5.6%) (Sung et al., 2021). Although significant progress has been made in diagnosing as well as treating cancer, yet it still accounts for a large number of fatalities.
Imaging techniques like computed tomography (CT), magnetic resonance imaging (MRI), positron emission tomography (PET), and ultrasound are widely used in detecting different cancer types. These techniques are used to locate and visualize cancer but are expensive, need trained staff, cannot be taken to field condition, less sensitive and accurate for early cancer detection, and sometimes involve the use of toxic radiolabeled compounds. Albeit an intrusive biopsy after imaging accompanied by histopathological assessment is the preferred method of diagnosis but this invasive technique requires skilled manpower and is not useful in early cancer diagnosis. Non-obtrusive techniques are still in their infancy, however, of much interest, early cancer diagnosis combined with specific cancer therapies can increase patient survival (Chen et al., 2020). Nanomedicine, a novel research area that blends nanomaterials and medicine, can possibly aid the development of innovative diagnostic tools for detection of primary cancers at initial stages, and for effective cancer therapy (Bar-Zeev et al., 2017).
The possibilities of cancer diagnosis and treatment using nanotechnology are colossal. It has led to the creation of nanomaterials with novel surface architecture and properties, thus opening vast avenues for manipulations at molecular level. Appending antibodies or other targeting agents onto nanocarrier surface for accurately targeting cancer cells is a promising approach for remedial and diagnostic oncology which is bound to take cancer therapy to an altogether different dimension (Farahavar et al., 2019). Nanotechnology is an incredible science not only to modify cancer diagnostics but also to provide detection strategies with higher dependability, sensitivity, and specificity.
Most of the standard chemotherapeutics are non-specific for tumor cells and exhibit toxicity to normal cells in vicinity. In this direction, localizing the drug at the tumor site reduces side effects associated with chemotherapy. Drug delivery systems based on nanotechnology extend the circulation of different chemotherapeutics in blood and improve their solubility (Sharma and Mondal, 2020). The development of biocompatible carbon-based nanomaterials for targeted diagnosis and treatment of diseases is an area of immense interest. This review attempts to give a quick overview of cancer and different imaging techniques used for its detection till date. Further, we aim to highlight emerging applications of nanotechnology, specifically carbon based nanodots for cancer diagnosis along with different bioconjugation techniques employed for this purpose. An extended information is provided in Supplementary file.
Cancer and Its Pathophysiology
Cancer is a condition involving abnormal division of cells, which invade different tissues. Mutations in genes controlling division of cells gives rise to cancer, which further metastasizes. Typically, human cells grow and divide to create new cells, and therefore the older cells are continually shed and substituted with new cells. But since the lethal disease develops inside the body, this process is hindered. The cells hence become unusual, as damaged cells still survive within the body along with the new cells, while these aren’t actually needed. These additional cells divide ceaselessly, eventually driving tumor spread. The tumor may either be benign or malignant. Benign tumors are not recognized as destructive since they develop gradually and furthermore don’t attack tissues or spread to different parts of the body. On the contrary, malignant tumors spread irrepressibly, resulting in the speedy tumor growth. These tumors in turn attack various parts of the body, through various routes like lymphatic system, blood, and ultimately form new tumors. The human body is composed of trillions of cells, dividing at normal rate and speed. Development of cancer leads to change of normal cells to cancer cells. Cancer cells have different DNA than normal cells, which can trigger extensive damage in the body. The commencement of a cell getting changed into neoplastic cell takes place with change of proto-oncogenes to oncogenes. Proto-oncogenes are significant for typical cell development inside human body. Nevertheless, in contrast, oncogenes cause cell growth to vary and get faster. The second step towards formation of a neoplastic cell is popping off the tumor suppressor genes, which help in preventing cancer from propagating within healthy cells. These genes prevent cell growth, while turning these off ends up in abnormal cell growth and therefore quick division of cells (Figure 1). The last step is turning off of DNA repair genes. These genes are essential for normal functioning of cells and to detect any changes in the DNA. However, turning off these genes makes the cell unable to detect and repair any abnormalities.
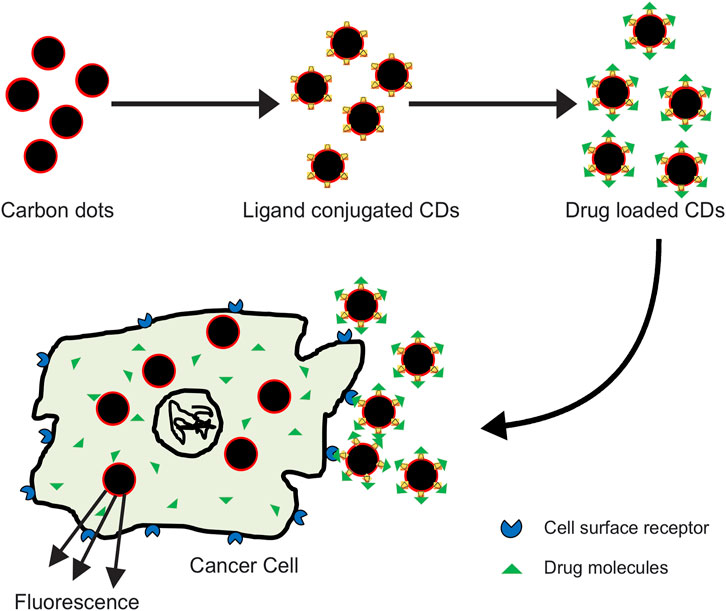
FIGURE 1. Different processes involved in the progression of malignant neoplasm. Subjecting a normal cell to carcinogenic agents disrupts DNA repair machinery of the cell, inducing DNA damage. This act further triggers activation of oncogenes, inhibition of tumor suppressor genes, and finally leads to apoptosis. These processes altogether contribute to uncontrolled cell differentiation, growth, and ultimately forming malignant neoplasms.
Present Diagnostic Methods
In the battle against cancer, early detection is the key for effective disease treatment. This leads to significant reduction in disease related mortality. Of late, it has become easy to detect and treat cancer because of modern imaging methods and morphological examination of tissues (histopathology) or cells (cytology), which helps in early analysis of malignant growth. Imaging techniques such as X-ray, MRI, CT, endoscopy, and ultrasound can possibly distinguish malignancy when there is a noticeable change in the tissue (Hussain and Nguyen, 2014; García-Figueiras et al., 2019). However, these techniques are unable to differentiate between benign and malignant lesions. These techniques do not permit quantization of the real tumor volume in the specified area. Thus, developing technologies for identification of malignant growth at initial stages is an arduous challenge. Detecting tumors at an early stages is extremely crucial for treatment of cancer. For some cancer types, very few screening tests are available and many of those are not very reliable. Further, non-invasive screening is not available for most of the cancer types and few patients do not adhere to guidelines for screening (Chen et al., 2020).
For accurate cancer detection, nanotechnology based tools with enhanced sensitivity and specificity are being extensively developed (Garrigue et al., 2018). Advances in nanotechnology involve using NPs for non-invasive tumor imaging. Distinctive carbon-based nanomaterials such as CQDs, fullerene, etc. have been in use for cancer diagnosis (Abdolahad et al., 2013; Shi et al., 2014; Kalaiyarasan et al., 2019). A comparative summary of the conventional as well as the relatively new carbon based nanotechnological agents for various cancer diagnoses is provided in Table 1.
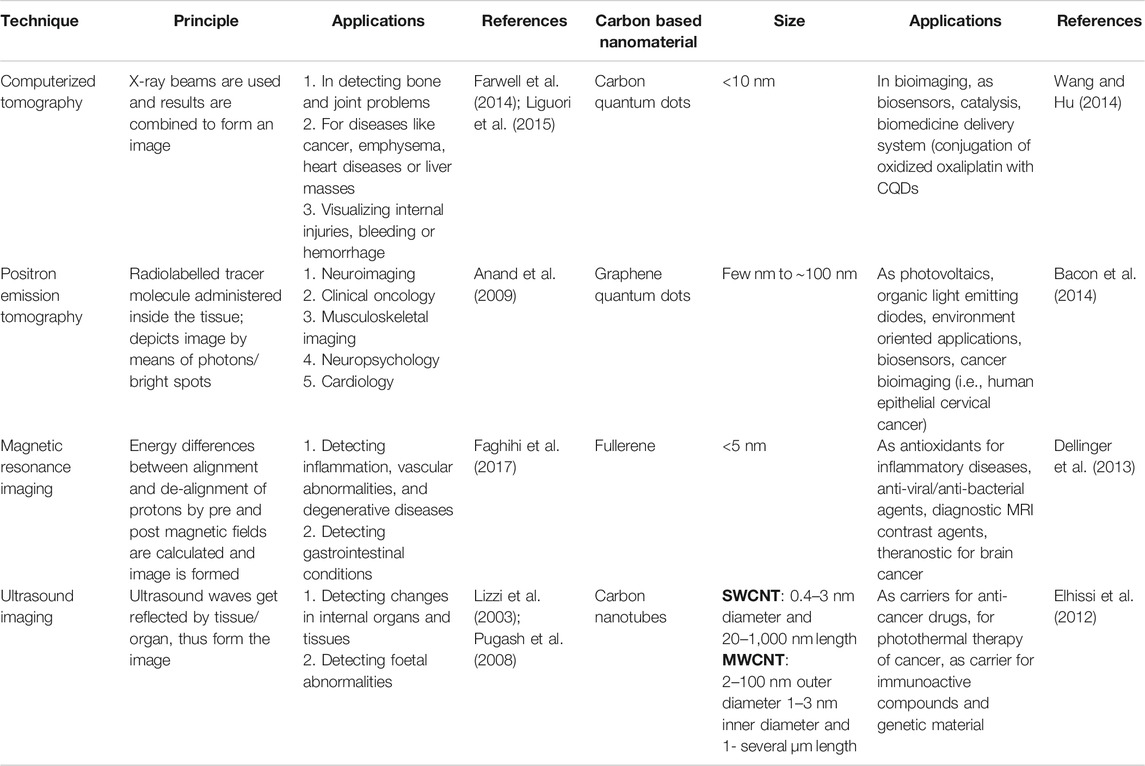
TABLE 1. A comparative summary of different conventional techniques and carbon-based nanomaterials used in early diagnosis of cancer.
Nanotechnology for Cancer Diagnosis
The utilization of nanomaterials for clinical diagnostics and drug delivery is gaining importance. Nanotechnology based agents are used in assortment of medical tests and screens for example, the use of gold nanoparticles (AuNPs) for pregnancy test kits (Hartman et al., 2013; Zhou et al., 2015). NPs are also used to detect malignancy biomarkers (Ye et al., 2018; Amri et al., 2021) such as cancer associated proteins, circulating tumor cells (CTCs) and DNA (Wang et al., 2011; Hu et al., 2018), and exosomes (Martín-Gracia et al., 2020). NPs have enormous surface area to volume ratio in comparison to bulk materials. Due to this, surface of nanoparticles can be coated with different moieties like peptides, antibodies, aptamers etc. These moieties can not only bind but also detect cancer molecules. Tunable shape, size and other surface properties additionally impart proper compatibility with different routes of administration, high carrier capacity, and stability, thus rendering them exceptionally alluring for oncological research. These engineered NPs with high contrast bioimaging, fluorescence and carrier functionalities have become admirable tools for molecular diagnostics and delivery of therapeutics. With a reproducible particle size, narrow size distribution and simple synthetic routes; large scale, cost effective products significant for clinical translation can be produced (Bertrand et al., 2014; Chaturvedi et al., 2019). Further information on role of nanotechnology in developing advanced diagnostic methods is mentioned in the Supplementary file.
The following sub-sections tend to highlight various engineered and functionalized carbon-based nanodots carrying a potential role in diagnosis of cancer.
Carbon Quantum Dots
Since the accidental discovery of carbon dots (CDs), also called carbon quantum dots (CQDs) during separation and purification of single walled carbon nanotubes (SWCNTs), their properties like low toxicity, biocompatibility, fluorescence, and chemical inertness have been utilized in theranostic fields (Figure 2) (Ding et al., 2014; Lim et al., 2015)
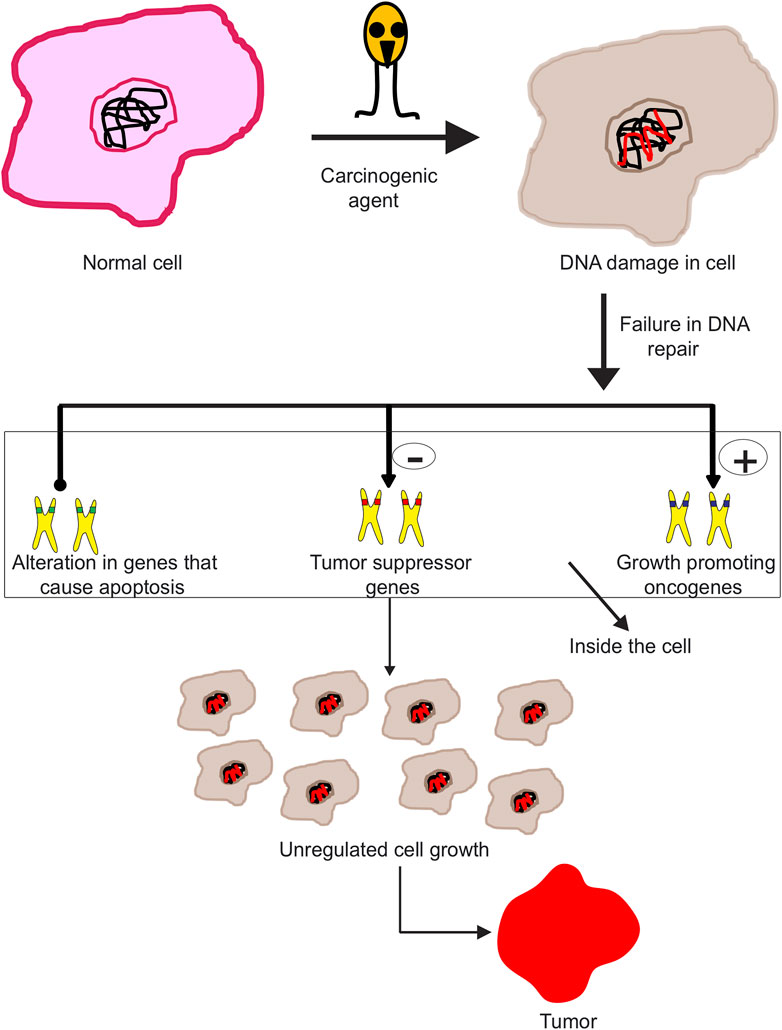
FIGURE 2. Carbon dots bound to anticancer drug effectively target the tumor cells and deliver the drug molecule at the cell surface; interaction of the drug with tumor cell results in its death.
Fluorescent CQDs are better than organic dyes in aspects like hydrophilicity, biocompatibility, ease of preparation, and lower toxicity. Due to these reasons, CQDs are considered good for cancer detection. Fluorescent CQDs can be used as imaging probes. Metals like Gadolinium have been used in combination with CQDs, which not only reduce their toxicity to organs but also prevent their leakage (Huang et al., 2020; Jiang et al., 2021). Many fluorescent sensors for Fe3+ detection are being developed nowadays, most of them are based on CQDs. Abnormal levels of Fe3+ are associated with development of cancer and other diseases. A solution comprising CQDs with doped Fe3+ was able to distinguish cancer cells from normal cells majorly because of differences in GSH levels (Gao et al., 2018). CQDs also suppress cancer cells in vitro (Pardo et al., 2018; Jia et al., 2020; Su et al., 2020). For visual detection of cancer, green fluorescence CDs and corresponding probe with turn on fluorescence were designed. Cancer cells were imaged by interaction between carbon dots and folic acid. The probe was able to detect folate receptor (FR) positive cells with turn-on fluorescence (Liu et al., 2015). In another study, fluorescent carbon dots conjugated with folic acid that can bind to FR, were prepared. These carbon dots exhibited remarkable biocompatibility and were able to distinguish between normal cells and cancer cells with FR (Song et al., 2012).
Graphene Quantum Dots
Derived from graphene, graphene quantum dots (GQDs) have good biocompatibility, luminescence and dispersibility in solvents (Younis et al., 2020; Zhao et al., 2020). Because of their intrinsic fluorescence, GQDs are ideal for anti-cancer therapy, permitting effective tracking of cells in vitro (Dong et al., 2018; Zhang et al., 2018; Kortel et al., 2020; Iannazzo et al., 2021). GQDs also increase efficiency of anticancer drugs (Fan et al., 2019; Nasrollahi et al., 2019). GQDs are widely used for bioimaging, due to low cytotoxicity and strong optical absorption (Kumar et al., 2020).
GQD based nanomaterials have been effectively used for photodynamic therapy and tumor diagnosis. In vivo experiments have revealed that GQDs prevent breast cancer growth in mice (Fan et al., 2019). Nitrogen doped GQDs have been synthesized and used as nanocarriers for delivery of anti-cancer drug methotrexate (Khodadadei et al., 2017). Recently, sulphur doped GQDs conjugated with folic acid have also been synthesized. These GQDs enter FR positive cancer cells via endocytosis and are extremely effective for early cancer diagnosis (Kadian et al., 2020). Due to large surface area to volume ratio, GQDs can support large drug amounts, thus enhancing its delivery. Further, GQDs functionalized with targeted ligands are capable of effectively targeting cancer cells that overexpress particular receptor (Kadian et al., 2021). Recently, biosensors based on “turn on fluorescence” were designed for efficiently detecting biomarkers of lung cancer using combination of GQDs and gold nanoparticles (Kalkal et al., 2020).
Fullerenes
Fullerenes (C60) find application as drug carriers; by means of drug conjugation, fullerene enhances therapeutic effects for paclitaxel-embedded C60 (Rezaian et al., 2018) and with doxorubicin (DOX) (Liu et al., 2014; Maleki et al., 2020). Conjugation with fullerenes makes the drug more hydrophilic and less cytotoxic; fullerenes also facilitate delivery of DNA into cells (Nitta et al., 2015; Illescas et al., 2020). In addition, these are also used as photosensitizers and more efficiently in photodynamic therapy; conjugation of C60 with poly ethylene glycol (PEG) enhanced their photosensitive effect. Endohedral fullerenes were used to stop angiogenesis and reduce vessel density in tumor tissues. Fullerenes act as immunomodulators and activate various immune cells by generating reactive oxygen species (ROS), thus killing cancer cells. Fullerenes also overcome tumor resistance to chemotherapeutic drugs and its derivatives are used as antioxidant species (Kepinska et al., 2018; Sergeeva et al., 2019). A study involving anti-cancer drug conjugated to C60 fullerene showed C60 to trigger phagocyte activity. In addition, fullerenes with anticancer drugs promotes ROS production by phagocytes, a promising strategy for treatment of cancer (Skivka et al., 2018).
Carbon Nanotubes
CNTs are used as contrast agents in medical imaging. They hold several potential advantages over other nano-sized detection agents, such as an exceptionally high surface area and the possibility for incorporating additional therapeutic and diagnostic moieties either on the surface or their inner cavity. The functionalized CNTs have tremendous potential as ultrasound contrast agent, exhibiting support for their future applications as theranostic tools. (Gao, 2018; Gu et al., 2018; Zhang et al., 2019; Zhang et al., 2020a). Photoacoustic imaging through nanotubes facilitates accurate tumor targeting. After conjugation of an MRI active contrast agent, nanotubes efficiently target tumors in magnetic fields. CNTs also facilitate drug delivery to target site and increase blood circulation after successful uptake by cancerous cells (Sanginario et al., 2017). CNTs are also used extensively in photothermal therapy of cancer cells (Lu et al., 2019).
On the basis of diameter and structure, these are further divided into two categories––single walled carbon nanotubes (SWCNTs), with single sheet of graphene in tube form with 0.4–3 nm diameter and multi walled carbon nanotubes (MWCNTs) which comprise of a few layers of graphite with an inner diameter around 1–3 nm and outer diameter between 2 and 100 nm. Like other NPs, CNTs are difficult to dissolve in aqueous medium; hence, these are modified or functionalized, for improved biocompatibility (Chen et al., 2017b).
SWCNTs have applications in delivery of drugs (Kamel et al., 2018), proteins (Antonucci et al., 2017) and siRNA inside target cells (Ravi Kiran et al., 2020). Conjugation of SWCNT with DOX demonstrated better clinical efficacy in comparison to when DOX was used alone. Nanotubes are effective for administering cancer therapy in vivo (Mahajan et al., 2018). Another anti-cancer drug, Paclitaxel (PTX) in conjugation with SWCNT effectively suppressed tumors without causing toxicity to other organs, providing evidence that nanotube based drug delivery is favorable for cancer therapy due to high treatment efficiency and low toxicity (Yu et al., 2016; Hashemzadeh and Raissi, 2017). MWCNTs were used for developing magnetic nanocarrier using iron oxide NPs. MWCNTs thus developed showed dual targeted delivery (Boncel et al., 2017; Fan et al., 2018; Hossen et al., 2019). A new method for delivering DNA and siRNA into microglia for brain cancer therapy via MWCNTs has been devised (Xiang et al., 2020).
Nanodiamonds
Nanodiamonds refer to a colloidal suspension of diamond particles. The use of these fluorescent nanodiamonds has started extensively, especially for bioimaging due to their low toxicity in comparison to quantum dots (Wei et al., 2019; Lai et al., 2020; Reineck et al., 2021). Nanodiamonds coupled to fluorophores are capable of targeting tumor cells without affecting cell viability and getting degraded due to changing pH (Yu et al., 2019; Liu et al., 2020; Perevedentseva et al., 2020).
Even the poorly soluble drugs can be adsorbed on the surface of nanodiamonds, which then mediate their slow and sustained release (Chipaux et al., 2018). The nanodiamonds based drug delivery systems are associated with reduced resistance to chemotherapeutics in different cancer types. DOX, considered effective for many types of cancer has been successfully adsorbed on nanodiamonds (called NDX). The resulting NDX particles were found to be taken up by living cells rapidly, facilitating drug delivery inside the cells. Another drug, epirubicin when adsorbed on nanodiamonds, leads to improved retention of tumor cells thereby killing cancer as well as non-cancer stem cells both in vivo and in vitro conditions. This prevents the formation of secondary tumors (Lai et al., 2020). Nanodiamonds have been used as vector for delivery of siRNA on sarcoma cells (Claveau et al., 2020). Detonation Nanodiamonds (DNDs) have been reported to be useful in radiotherapy. Irradiation of cancer cells resistant to radiotherapy is more effective when DNDs are incorporated inside the cells (Matshitse et al., 2020). For tumor therapy, delivery of sodium ions inside the cell has been easily carried out with the help of nanodiamonds (Chen et al., 2017a; Gupta et al., 2017). Nanodiamonds have been successfully used to target autophagy in tumor cells and promote their programmed cell death (PCD) in hypoxic conditions in vivo (Chen et al., 2018).
Nanocantilevers
Nanocantilevers can be coated with substrates that can selectively bind the target and detect even minute molecules in biological fluids. Binding of nanocantilevers to biomolecules changes the baseline probe frequency. These differences in frequency are measured by light diffraction pattern or by electrical means (Jabbari Behrouz et al., 2019). As the target sequence binds, the signal is transduced mechanically to surface of cantilever, resulting in its bending. Detection of cancer molecules can be done on the basis of deflection, which depends on the amount of DNA bound to cantilever surface and can be observed (Wang et al., 2016; Haring et al., 2017). This technology has been successfully used for differentiating between BRAF and its wild type gene in melanoma patients (Huber et al., 2013). The elevated levels of prostate specific antigen (PSA) are associated with increased risk of prostate cancer among men. Using antibodies bound to the surface of cantilevers, PSA assay was performed (Damborska et al., 2017; Basu et al., 2020).
Bioconjugation Strategies for Nanotechnology-Based Agents
The surface of nanodots must be modified for compatibility with biological systems in order to facilitate their in vitro and in vivo applications. Surface functionalization improves stability and water-solubility, of nanodots, which can be further conjugated with biomolecules of interest for biomedical applications (Valcourt et al., 2018; Xu et al., 2020). Different techniques involving coupling are in use for a long time. There are two main strategies for conjugation of biomolecules: covalent and non-covalent binding. Non-covalent binding further involves coupling through direct absorption and electrostatic interactions (Foubert et al., 2016). As the name implies, direct absorption approach involves direct interaction of biomolecules with various NPs. Cell structures can be nonspecifically stained using hydrophilic NPs. Usually, the interaction is nonspecific and weak. Biomolecules with particular functional groups can be directly attached to NPs, e.g., thiol groups from cytosine can bind proteins to noble metal NPs. Another methodology is to absorb biomolecules on surface of NPs through electrostatic interaction, e.g., positively charged NPs can be attached to negatively charged nucleic acid (Sapsford et al., 2013; Boehnke et al., 2020).
Covalent coupling involves precise and stable conjugation of biomolecules with NPs. Generally, coupling reactions to crosslink biomolecules are carried out on the surface of NPs using functional groups like carboxylic, amine, and thiol group. Carboxyl group coupling includes reaction of primary amines with carboxylic group. The coupling agent used is 1-ethyl-3-(3-dimethylaminopropyl)carbodiimide (EDC). Additionally, stabilizing agents like n-hydroxysuccinimide (NHS) or sulfo-NHS enhance coupling efficiency by arrangement of a succinimide ester intermediate (Blanco-Canosa et al., 2014; Karakoti et al., 2015; Saallah and Lenggoro, 2018). Amine group coupling involves reaction of carboxylic group with amine group for forming an amide bond. The coupling reagent used is glutaraldehyde, which activates NH2-functionalized NPs. The aldehyde group produced throughout actuation will conjugate with amino groups of biomolecules (Barbosa et al., 2014; Zhang et al., 2015). Thiol group coupling involves conjugation of thiol group with primary amine groups. The reaction begins quickly using reagents like maleimides and iodoacetamides. sulfosuccinimidyl4-(maleimidomethyl) cyclohexane-1-carboxylate (sulfo-SMCC) is used for coupling. Amine-functionalized NPs can be conjugated to various thiolated biomolecules, like residue thiol-terminated DNA, thiol-modified peptide, or peptides, and proteins with free or reduced cysteine (Brodie et al., 2015; Karakoti et al., 2015; Kolodych et al., 2015; Martinez-Jothar et al., 2018) (Various bioconjugation strategies are depicted in Supplementary Figure S1)
Cancer Biomarkers
Cancer markers assist detection of specific types of cancer in the body and in noticing the progression of cancer treatment. Cancer biomarkers register their presence in body fluids, blood or tissues that aid in detection of cancer cells and assist in setting up specific diagnosis. This is particularly the circumstance when there is a need to decide if the tumors are primary or metastatic in origin. Many biomarkers specific to different cancer types have been discovered till date. These have crucial roles for early diagnosis of cancer. A description of some such biomarkers for different cancers is given in Table 2 along with an assortment of carbon nanomaterials used for the detection and/or treatment of cancer types. Detailed information on cancer biomarkers that aid the diagnosis of different cancer types is provided in Supplementary file.
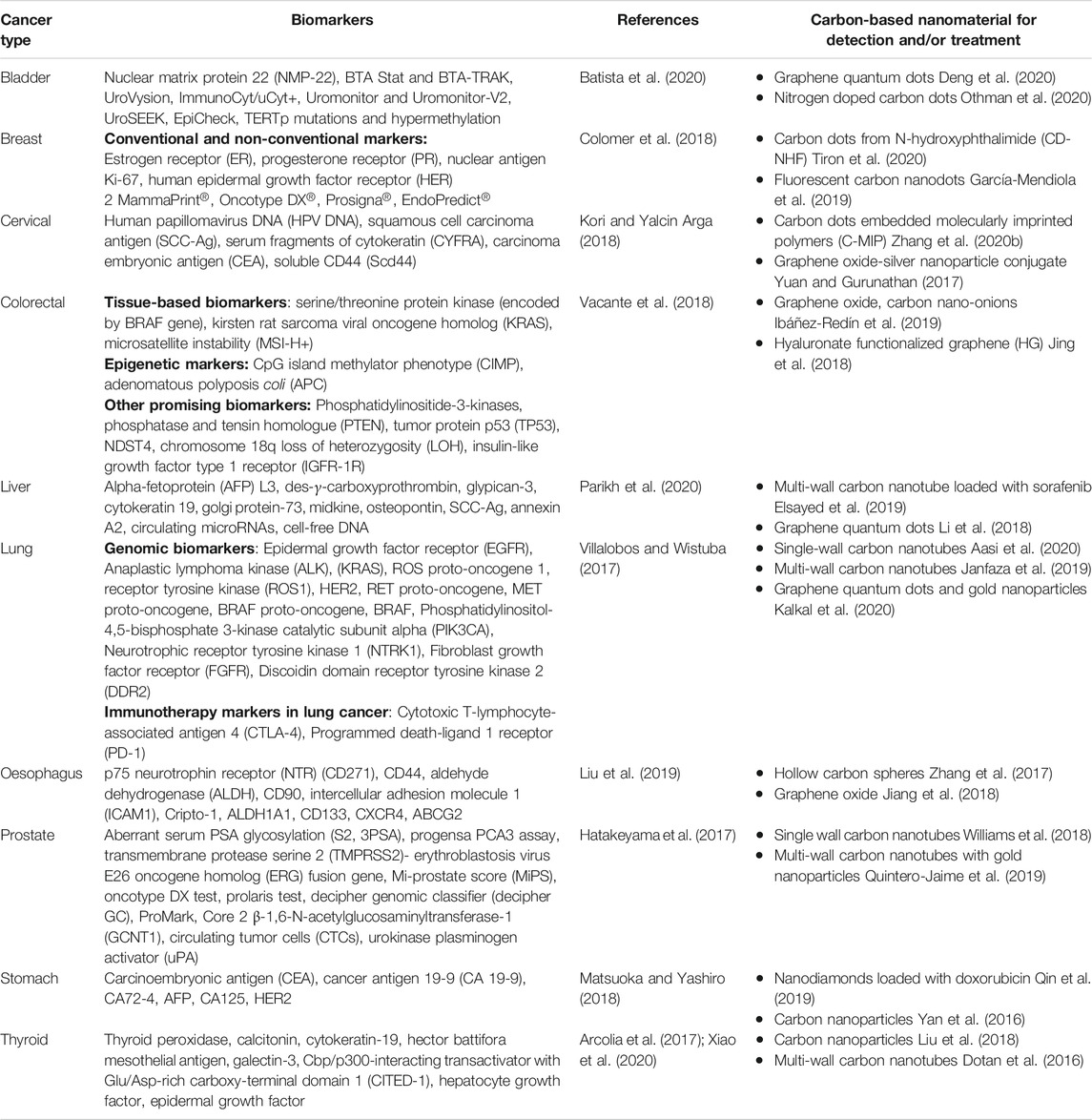
TABLE 2. Chart of different biomarkers and carbon based nanomaterials for cancer diagnosis and treatment.
Conclusion
Cancer nanotechnology holds promise in providing novel techniques for cancer detection at initial stages, resulting in improved diagnosis, and treatment. Conventional imaging techniques are exceptionally intrusive, non-specific, and are frequently associated with toxicity to both tumor and solid cells. The advancement of novel nanomaterials has enabled the identification of cancer biomarkers with more sensitivity and accuracy that was not feasible previously. Steady and coordinated exploration endeavors should be embraced to utilize tremendous capability of nanotechnology in distinguishing cancer growth in early stages, and monitoring the disease with treatment precision.
Author Contributions
All authors listed have made a substantial, direct, and intellectual contribution to the work and approved it for publication.
Conflict of Interest
The authors declare that the research was conducted in the absence of any commercial or financial relationships that could be construed as a potential conflict of interest.
Acknowledgments
The authors gratefully acknowledge the financial grants received from DST-Indo Egypt (DST/INT/Egypt/P-19/2019) Government of India. RB and GS gratefully acknowledge the financial grant received from DBT (BT/PR27444/BRB/10/1645/2018) and ICMR (35/2/2020-Nano/BMS), Government of India.
Supplementary Material
The Supplementary Material for this article can be found online at: https://www.frontiersin.org/articles/10.3389/fchem.2021.669169/full#supplementary-material
References
Aasi, A., Aghaei, S. M., and Panchapakesan, B. (2020). A Density Functional Theory Study on the Interaction of Toluene with Transition Metal Decorated Carbon Nanotubes: a Promising Platform for Early Detection of Lung Cancer from Human Breath. Nanotechnology 31, 415707. doi:10.1088/1361-6528/ab9da9
Abdolahad, M., Janmaleki, M., Taghinejad, M., Taghnejad, H., Salehi, F., and Mohajerzadeh, S. (2013). Single-cell Resolution Diagnosis of Cancer Cells by Carbon Nanotube Electrical Spectroscopy. Nanoscale 5, 3421–3427. doi:10.1039/c3nr33430a
Amri, C., Shukla, A. K., and Lee, J. H. (2021). Recent Advancements in Nanoparticle-Based Optical Biosensors for Circulating Cancer Biomarkers. Materials (Basel) 14. doi:10.3390/ma14061339
Anand, S., Singh, H., and Dash, A. (2009). Clinical Applications of PET and PET-CT. Med. J. Armed Forces India 65, 353–358. doi:10.1016/s0377-1237(09)80099-3
Antonucci, A., Kupis-Rozmysłowicz, J., and Boghossian, A. A. (2017). Noncovalent Protein and Peptide Functionalization of Single-Walled Carbon Nanotubes for Biodelivery and Optical Sensing Applications. ACS Appl. Mater. Inter. 9, 11321–11331. doi:10.1021/acsami.7b00810
Arcolia, V., Journe, F., Renaud, F., Leteurtre, E., Gabius, H.-J., Remmelink, M., et al. (2017). Combination of Galectin-3, CK19 and HBME-1 Immunostaining Improves the Diagnosis of Thyroid Cancer. Oncol. Lett. 14, 4183–4189. doi:10.3892/ol.2017.6719
Bacon, M., Bradley, S. J., and Nann, T. (2014). Graphene Quantum Dots. Part. Part. Syst. Charact. 31, 415–428. doi:10.1002/ppsc.201300252
Bar-Zeev, M., Livney, Y. D., and Assaraf, Y. G. (2017). Targeted Nanomedicine for Cancer Therapeutics: Towards Precision Medicine Overcoming Drug Resistance. Drug Resist. Updates 31, 15–30. doi:10.1016/j.drup.2017.05.002
Barbosa, O., Ortiz, C., Berenguer-Murcia, Á., Torres, R., Rodrigues, R. C., and Fernandez-Lafuente, R. (2014). Glutaraldehyde in Bio-Catalysts Design: a Useful Crosslinker and a Versatile Tool in Enzyme Immobilization. RSC Adv. 4, 1583–1600. doi:10.1039/c3ra45991h
Basu, A. K., Basu, A., and Bhattacharya, S. (2020). Micro/Nano Fabricated Cantilever Based Biosensor Platform: A Review and Recent Progress. Enzyme Microb. Tech. 139, 109558. doi:10.1016/j.enzmictec.2020.109558
Batista, R., Vinagre, N., Meireles, S., Vinagre, J., Prazeres, H., Leão, R., et al. (2020). Biomarkers for Bladder Cancer Diagnosis and Surveillance: A Comprehensive Review. Diagnostics (Basel) 10. doi:10.3390/diagnostics10010039
Bertrand, N., Wu, J., Xu, X., Kamaly, N., and Farokhzad, O. C. (2014). Cancer Nanotechnology: the Impact of Passive and Active Targeting in the Era of Modern Cancer Biology. Adv. Drug Deliv. Rev. 66, 2–25. doi:10.1016/j.addr.2013.11.009
Blanco-Canosa, J. B., Wu, M., Susumu, K., Petryayeva, E., Jennings, T. L., Dawson, P. E., et al. (2014). Recent Progress in the Bioconjugation of Quantum Dots. Coord. Chem. Rev. 263-264, 101–137. doi:10.1016/j.ccr.2013.08.030
Boehnke, N., Dolph, K. J., Juarez, V. M., Lanoha, J. M., and Hammond, P. T. (2020). Electrostatic Conjugation of Nanoparticle Surfaces with Functional Peptide Motifs. Bioconjug. Chem. 31, 2211–2219. doi:10.1021/acs.bioconjchem.0c00384
Boncel, S., Pluta, A., Skonieczna, M., Gondela, A., Maciejewska, B., Herman, A. P., et al. (2017). Hybrids of Iron-Filled Multiwall Carbon Nanotubes and Anticancer Agents as Potential Magnetic Drug Delivery Systems: In Vitro Studies against Human Melanoma, Colon Carcinoma, and Colon Adenocarcinoma. J. Nanomater., 2017, 1–13. doi:10.1155/2017/1262309
Brodie, N. I., Makepeace, K. A. T., Petrotchenko, E. V., and Borchers, C. H. (2015). Isotopically-coded Short-Range Hetero-Bifunctional Photo-Reactive Crosslinkers for Studying Protein Structure. J. Proteomics 118, 12–20. doi:10.1016/j.jprot.2014.08.012
Chaturvedi, V. K., Singh, A., Singh, V. K., and Singh, M. P. (2019). Cancer Nanotechnology: A New Revolution for Cancer Diagnosis and Therapy. Curr. Drug. Metab. 20, 416–429. doi:10.2174/1389200219666180918111528
Chen, C., Cho, I. C., Jian, H. S., and Niu, H. (2017a). Fe Doped Magnetic Nanodiamonds Made by Ion Implantation. Sci. Rep. 7, 41938. doi:10.1038/srep41938
Chen, N., Han, Y., Luo, Y., Zhou, Y., Hu, X., Yu, Y., et al. (2018). Nanodiamond-based Non-canonical Autophagy Inhibitor Synergistically Induces Cell Death in Oxygen-Deprived Tumors. Mater. Horiz. 5, 1204–1210. doi:10.1039/c8mh00993g
Chen, X., Gole, J., Gore, A., He, Q., Lu, M., Min, J., et al. (2020). Non-invasive Early Detection of Cancer Four Years before Conventional Diagnosis Using a Blood Test. Nat. Commun. 11, 3475. doi:10.1038/s41467-020-17316-z
Chen, Z., Zhang, A., Wang, X., Zhu, J., Fan, Y., Yu, H., et al. (2017b). The Advances of Carbon Nanotubes in Cancer Diagnostics and Therapeutics. J. Nanomater. 2017, 3418932 10.1155/2017/3418932.
Chipaux, M., Van Der Laan, K. J., Hemelaar, S. R., Hasani, M., Zheng, T., and Schirhagl, R. (2018). Nanodiamonds and Their Applications in Cells. Small 14, 1704263. doi:10.1002/smll.201704263
Claveau, S., Nehlig, É., Garcia-Argote, S., Feuillastre, S., Pieters, G., Girard, H. A., et al. (2020). Delivery of siRNA to Ewing Sarcoma Tumor Xenografted on Mice, Using Hydrogenated Detonation Nanodiamonds: Treatment Efficacy and Tissue Distribution. Nanomaterials 10, 553. doi:10.3390/nano10030553
Colomer, R., Aranda-López, I., Albanell, J., García-Caballero, T., Ciruelos, E., López-García, M. Á., et al. (2018). Biomarkers in Breast Cancer: A Consensus Statement by the Spanish Society of Medical Oncology and the Spanish Society of Pathology. Clin. Transl Oncol. 20, 815–826. doi:10.1007/s12094-017-1800-5
Damborska, D., Bertok, T., Dosekova, E., Holazova, A., Lorencova, L., Kasak, P., et al. (2017). Nanomaterial-based Biosensors for Detection of Prostate Specific Antigen. Microchim Acta 184, 3049–3067. doi:10.1007/s00604-017-2410-1
Dellinger, A., Zhou, Z., Connor, J., Madhankumar, A., Pamujula, S., Sayes, C. M., et al. (2013). Application of Fullerenes in Nanomedicine: an Update. Nanomedicine 8, 1191–1208. doi:10.2217/nnm.13.99
Deng, M., Cao, X., Guo, L., Cao, H., Wen, Z., Mao, C., et al. (2020). Graphene Quantum Dots: Efficient Mechanosynthesis, white-light and Broad Linear Excitation-dependent Photoluminescence and Growth Inhibition of Bladder Cancer Cells. Dalton Trans. 49, 2308–2316. doi:10.1039/c9dt04575a
Ding, C., Zhu, A., and Tian, Y. (2014). Functional Surface Engineering of C-Dots for Fluorescent Biosensing and In Vivo Bioimaging. Acc. Chem. Res. 47, 20–30. doi:10.1021/ar400023s
Dong, J., Wang, K., Sun, L., Sun, B., Yang, M., Chen, H., et al. (2018). Application of Graphene Quantum Dots for Simultaneous Fluorescence Imaging and Tumor-Targeted Drug Delivery. Sensors Actuators B: Chem. 256, 616–623. doi:10.1016/j.snb.2017.09.200
Dotan, I., Roche, P. J. R., Paliouras, M., Mitmaker, E. J., and Trifiro, M. A. (2016). Engineering Multi-Walled Carbon Nanotube Therapeutic Bionanofluids to Selectively Target Papillary Thyroid Cancer Cells. PloS one 11–e0149723., doi:10.1371/journal.pone.0149723
Elhissi, A. M., Ahmed, W., Hassan, I. U., Dhanak, V. R., and D'emanuele, A. (2012). Carbon Nanotubes in Cancer Therapy and Drug Delivery. J. Drug Deliv. 2012, 837327. doi:10.1155/2012/837327
Elsayed, M. M. A., Mostafa, M. E., Alaaeldin, E., Sarhan, H. A. A., Shaykoon, M. S. A., Allam, S., et al. (2019). Design and Characterisation of Novel Sorafenib-Loaded Carbon Nanotubes with Distinct Tumour-Suppressive Activity in Hepatocellular Carcinoma. Int. J. Nanomedicine. Vol. 14, 8445–8467. doi:10.2147/ijn.s223920
Faghihi, R., Zeinali-Rafsanjani, B., Mosleh-Shirazi, M.-A., Saeedi-Moghadam, M., Lotfi, M., Jalli, R., et al. (2017). Magnetic Resonance Spectroscopy and its Clinical Applications: A Review. J. Med. Imaging Radiat. Sci. 48, 233–253. doi:10.1016/j.jmir.2017.06.004
Fan, H.-Y., Yu, X.-H., Wang, K., Yin, Y.-J., Tang, Y.-J., Tang, Y.-L., et al. (2019). Graphene Quantum Dots (GQDs)-Based Nanomaterials for Improving Photodynamic Therapy in Cancer Treatment. Eur. J. Med. Chem. 182, 111620. doi:10.1016/j.ejmech.2019.111620
Fan, H., Duan, P., Guo, S., Zhao, L., Wang, K., and Qiu, D. (2018). Multifunctional MWCNTs@CoFe2O4@mSiO2@NaYF4:Yb3+, Er3+ Nanocomposites and Their Application as Drug Carrier. Mater. Lett. 213, 311–314. doi:10.1016/j.matlet.2017.11.098
Farahavar, G., Abolmaali, S. S., Gholijani, N., and Nejatollahi, F. (2019). Antibody-guided Nanomedicines as Novel Breakthrough Therapeutic, Diagnostic and Theranostic Tools. Biomater. Sci. 7, 4000–4016. doi:10.1039/c9bm00931k
Farwell, M. D., Pryma, D. A., and Mankoff, D. A. (2014). PET/CT Imaging in Cancer: Current Applications and Future Directions. Cancer 120, 3433–3445. doi:10.1002/cncr.28860
Foubert, A., Beloglazova, N. V., Rajkovic, A., Sas, B., Madder, A., Goryacheva, I. Y., et al. (2016). Bioconjugation of Quantum Dots: Review & Impact on Future Application. Trac Trends Anal. Chem. 83, 31–48. doi:10.1016/j.trac.2016.07.008
Gao, G., Jiang, Y.-W., Jia, H.-R., Yang, J., and Wu, F.-G. (2018). On-off-on Fluorescent Nanosensor for Fe3+ Detection and Cancer/normal Cell Differentiation via Silicon-Doped Carbon Quantum Dots. Carbon 134, 232–243. doi:10.1016/j.carbon.2018.02.063
Gao, Y. (2018). Carbon Nano-Allotrope/Magnetic Nanoparticle Hybrid Nanomaterials as T2 Contrast Agents for Magnetic Resonance Imaging Applications. J. Funct. Biomater. 9. 16. doi:10.3390/jfb9010016
García-Figueiras, R., Baleato-González, S., Padhani, A. R., Luna-Alcalá, A., Vallejo-Casas, J. A., Sala, E., et al. (2019). How Clinical Imaging Can Assess Cancer Biology. Insights. Imaging. 10, 28. doi:10.1186/s13244-019-0703-0
García-Mendiola, T., Elosegui, C. G., Bravo, I., Pariente, F., Jacobo-Martin, A., Navio, C., et al. (2019). Fluorescent C-NanoDots for Rapid Detection of BRCA1, CFTR and MRP3 Gene Mutations. Microchimica Acta 186, 293. doi:10.1007/s00604-019-3386-9
Garrigue, P., Tang, J., Ding, L., Bouhlel, A., Tintaru, A., Laurini, E., et al. (2018). Self-assembling Supramolecular Dendrimer Nanosystem for PET Imaging of Tumors. Proc. Natl. Acad. Sci. USA 115, 11454–11459. doi:10.1073/pnas.1812938115
Gu, F., Hu, C., Xia, Q., Gong, C., Gao, S., and Chen, Z. (2018). Aptamer-conjugated Multi-Walled Carbon Nanotubes as a New Targeted Ultrasound Contrast Agent for the Diagnosis of Prostate Cancer. J. Nanopart Res. 20, 303. doi:10.1007/s11051-018-4407-z
Gupta, S., Evans, B., Henson, A., and Carrizosa, S. B. (2017). Salt-Assisted Ultrasonicated De-aggregation and Advanced Redox Electrochemistry of Detonation Nanodiamond. Materials (Basel) 10. doi:10.3390/ma10111292
Haring, A. P., Cesewski, E., and Johnson, B. N. (2017). Piezoelectric Cantilever Biosensors for Label-free, Real-Time Detection of DNA and RNA. Methods Mol. Biol. 1572, 247–262. doi:10.1007/978-1-4939-6911-1_17
Hartman, M. R., Ruiz, R. C. H., Hamada, S., Xu, C., Yancey, K. G., Yu, Y., et al. (2013). Point-of-care Nucleic Acid Detection Using Nanotechnology. Nanoscale 5, 10141–10154. doi:10.1039/c3nr04015a
Hashemzadeh, H., and Raissi, H. (2017). The Functionalization of Carbon Nanotubes to Enhance the Efficacy of the Anticancer Drug Paclitaxel: a Molecular Dynamics Simulation Study. J. Mol. Model. 23, 222. doi:10.1007/s00894-017-3391-z
Hatakeyama, S., Yoneyama, T., Tobisawa, Y., and Ohyama, C. (2017). Recent Progress and Perspectives on Prostate Cancer Biomarkers. Int. J. Clin. Oncol. 22, 214–221. doi:10.1007/s10147-016-1049-y
Hossen, S., Hossain, M. K., Basher, M. K., Mia, M. N. H., Rahman, M. T., and Uddin, M. J. (2019). Smart Nanocarrier-Based Drug Delivery Systems for Cancer Therapy and Toxicity Studies: A Review. J. Adv. Res. 15, 1–18. doi:10.1016/j.jare.2018.06.005
Hu, P., Zhang, S., Wu, T., Ni, D., Fan, W., Zhu, Y., et al. (2018). Fe-Au Nanoparticle-Coupling for Ultrasensitive Detections of Circulating Tumor DNA. Adv. Mater. 30, 1801690. doi:10.1002/adma.201801690
Huang, Y., Li, L., Zhang, D., Gan, L., Zhao, P., Zhang, Y., et al. (2020). Gadolinium-doped Carbon Quantum Dots Loaded Magnetite Nanoparticles as a Bimodal Nanoprobe for Both Fluorescence and Magnetic Resonance Imaging. Magn. Reson. Imaging 68, 113–120. doi:10.1016/j.mri.2020.02.003
Huber, F., Lang, H. P., Backmann, N., Rimoldi, D., and Gerber, C. (2013). Direct Detection of a BRAF Mutation in Total RNA from Melanoma Cells Using Cantilever Arrays. Nat. Nanotech 8, 125–129. doi:10.1038/nnano.2012.263
Hussain, T., and Nguyen, Q. T. (2014). Molecular Imaging for Cancer Diagnosis and Surgery. Adv. Drug Deliv. Rev. 66, 90–100. doi:10.1016/j.addr.2013.09.007
Iannazzo, D., Celesti, C., and Espro, C. (2021). Recent Advances on Graphene Quantum Dots as Multifunctional Nanoplatforms for Cancer Treatment. Biotechnol. J. 16, e1900422. doi:10.1002/biot.201900422
Ibáñez-Redín, G., Furuta, R. H. M., Wilson, D., Shimizu, F. M., Materon, E. M., Arantes, L. M. R. B., et al. (2019). Screen-printed Interdigitated Electrodes Modified with Nanostructured Carbon Nano-Onion Films for Detecting the Cancer Biomarker CA19-9. Mater. Sci. Eng. C 99, 1502–1508. doi:10.1016/j.msec.2019.02.065
Illescas, B. M., Pérez-Sánchez, A., Mallo, A., Martín-Domenech, Á., Rodríguez-Crespo, I., and Martín, N. (2020). Multivalent Cationic Dendrofullerenes for Gene Transfer: Synthesis and DNA Complexation. J. Mater. Chem. B 8, 4505–4515. doi:10.1039/d0tb00113a
Jabbari Behrouz, S., Rahmani, O., and Hosseini, S. A. (2019). On Nonlinear Forced Vibration of Nano Cantilever-Based Biosensor via Couple Stress Theory. Mech. Syst. Signal Process. 128, 19–36. doi:10.1016/j.ymssp.2019.03.020
Janfaza, S., Banan Nojavani, M., Nikkhah, M., Alizadeh, T., Esfandiar, A., and Ganjali, M. R. (2019). A Selective Chemiresistive Sensor for the Cancer-Related Volatile Organic Compound Hexanal by Using Molecularly Imprinted Polymers and Multiwalled Carbon Nanotubes. Microchimica Acta 186, 137. doi:10.1007/s00604-019-3241-z
Jia, Q., Zhao, Z., Liang, K., Nan, F., Li, Y., Wang, J., et al. (2020). Recent Advances and Prospects of Carbon Dots in Cancer Nanotheranostics. Mater. Chem. Front. 4, 449–471. doi:10.1039/c9qm00667b
Jiang, J.-H., Pi, J., Jin, H., and Cai, J.-Y. (2018). Functional Graphene Oxide as Cancer-Targeted Drug Delivery System to Selectively Induce Oesophageal Cancer Cell Apoptosis. Artif. Cell Nanomedicine, Biotechnol. 46, S297–S307. doi:10.1080/21691401.2018.1492418
Jiang, Q., Liu, L., Li, Q., Cao, Y., Chen, D., Du, Q., et al. (2021). NIR-laser-triggered Gadolinium-Doped Carbon Dots for Magnetic Resonance Imaging, Drug Delivery and Combined Photothermal Chemotherapy for Triple Negative Breast Cancer. J. Nanobiotechnology 19, 64. doi:10.1186/s12951-021-00811-w
Jing, A., Zhang, C., Liang, G., Feng, W., Tian, Z., and Jing, C. (2018). Hyaluronate-Functionalized Graphene for Label-free Electrochemical Cytosensing. Micromachines 9, 669. doi:10.3390/mi9120669
Kadian, S., Manik, G., Das, N., and Roy, P. (2020). Targeted Bioimaging and Sensing of Folate Receptor-Positive Cancer Cells Using Folic Acid-Conjugated Sulfur-Doped Graphene Quantum Dots. Microchimica Acta 187, 458. doi:10.1007/s00604-020-04448-8
Kadian, S., Sethi, S. K., and Manik, G. (2021). Recent Advancements in Synthesis and Property Control of Graphene Quantum Dots for Biomedical and Optoelectronic Applications. Mater. Chem. Front. 5, 627–658. doi:10.1039/d0qm00550a
Kalaiyarasan, G., Veerapandian, M., Jebamercy, G., Balamurugan, K., and Joseph, J. (2019). Amygdalin-Functionalized Carbon Quantum Dots for Probing β-Glucosidase Activity for Cancer Diagnosis and Therapeutics. ACS Biomater. Sci. Eng. 5, 3089–3099. doi:10.1021/acsbiomaterials.9b00394
Kalkal, A., Pradhan, R., Kadian, S., Manik, G., and Packirisamy, G. (2020). Biofunctionalized Graphene Quantum Dots Based Fluorescent Biosensor toward Efficient Detection of Small Cell Lung Cancer. ACS Appl. Bio Mater. 3, 4922–4932. doi:10.1021/acsabm.0c00427
Kamel, M., Raissi, H., Morsali, A., and Shahabi, M. (2018). Assessment of the Adsorption Mechanism of Flutamide Anticancer Drug on the Functionalized Single-Walled Carbon Nanotube Surface as a Drug Delivery Vehicle: An Alternative Theoretical Approach Based on DFT and MD. Appl. Surf. Sci. 434, 492–503. doi:10.1016/j.apsusc.2017.10.165
Karakoti, A. S., Shukla, R., Shanker, R., and Singh, S. (2015). Surface Functionalization of Quantum Dots for Biological Applications. Adv. Colloid Interf. Sci. 215, 28–45. doi:10.1016/j.cis.2014.11.004
Kepinska, M., Kizek, R., and Milnerowicz, H. (2018). Metallothionein and Superoxide Dismutase-Antioxidative Protein Status in Fullerene-Doxorubicin Delivery to MCF-7 Human Breast Cancer Cells. Int. J. Mol. Sci. 19. doi:10.3390/ijms19103253
Khodadadei, F., Safarian, S., and Ghanbari, N. (2017). Methotrexate-loaded Nitrogen-Doped Graphene Quantum Dots Nanocarriers as an Efficient Anticancer Drug Delivery System. Mater. Sci. Eng. C 79, 280–285. doi:10.1016/j.msec.2017.05.049
Kolodych, S., Koniev, O., Baatarkhuu, Z., Bonnefoy, J.-Y., Debaene, F., Cianférani, S., et al. (2015). CBTF: New Amine-To-Thiol Coupling Reagent for Preparation of Antibody Conjugates with Increased Plasma Stability. Bioconjug. Chem. 26, 197–200. doi:10.1021/bc500610g
Kori, M., and Yalcin Arga, K. (2018). Potential Biomarkers and Therapeutic Targets in Cervical Cancer: Insights from the Meta-Analysis of Transcriptomics Data within Network Biomedicine Perspective. PLoS One 13, e0200717. doi:10.1371/journal.pone.0200717
Kortel, M., Mansuriya, B. D., Vargas Santana, N., and Altintas, Z. (2020). Graphene Quantum Dots as Flourishing Nanomaterials for Bio-Imaging, Therapy Development, and Micro-supercapacitors. Micromachines (Basel) 11. 866. doi:10.3390/mi11090866
Kumar, Y. R., Deshmukh, K., Sadasivuni, K. K., and Pasha, S. K. K. (2020). Graphene Quantum Dot Based Materials for Sensing, Bio-Imaging and Energy Storage Applications: a Review. RSC Adv. 10, 23861–23898. doi:10.1039/d0ra03938a
Lai, H., Stenzel, M. H., and Xiao, P. (2020). Surface Engineering and Applications of Nanodiamonds in Cancer Treatment and Imaging. Int. Mater. Rev. 65, 189–225. doi:10.1080/09506608.2019.1622202
Li, J.-J., Shang, L., Jia, L.-P., Ma, R.-N., Zhang, W., Jia, W.-L., et al. (2018). An Ultrasensitive Electrochemiluminescence Sensor for the Detection of HULC Based on Au@Ag/GQDs as a Signal Indicator. J. Electroanalytical Chem. 824, 114–120. doi:10.1016/j.jelechem.2018.07.044
Liguori, C., Frauenfelder, G., Massaroni, C., Saccomandi, P., Giurazza, F., Pitocco, F., et al. (2015). Emerging Clinical Applications of Computed Tomography. Med. Devices (Auckl) 8, 265–278. doi:10.2147/MDER.S70630
Lim, S. Y., Shen, W., and Gao, Z. (2015). Carbon Quantum Dots and Their Applications. Chem. Soc. Rev. 44, 362–381. doi:10.1039/c4cs00269e
Liu, K., Zhao, T., Wang, J., Chen, Y., Zhang, R., Lan, X., et al. (2019). Etiology, Cancer Stem Cells and Potential Diagnostic Biomarkers for Esophageal Cancer. Cancer Lett. 458, 21–28. doi:10.1016/j.canlet.2019.05.018
Liu, Q., Xu, S., Niu, C., Li, M., He, D., Lu, Z., et al. (2015). Distinguish Cancer Cells Based on Targeting Turn-On Fluorescence Imaging by Folate Functionalized green Emitting Carbon Dots. Biosens. Bioelectron. 64, 119–125. doi:10.1016/j.bios.2014.08.052
Liu, W., Wei, J., and Chen, Y. (2014). Electrospun Poly(l-Lactide) Nanofibers Loaded with Paclitaxel and Water-Soluble Fullerenes for Drug Delivery and Bioimaging. New J. Chem. 38, 6223–6229. doi:10.1039/c4nj01259c
Liu, Y.-Y., Chang, B.-M., and Chang, H.-C. (2020). Nanodiamond-enabled Biomedical Imaging. Nanomedicine 15, 1599–1616. doi:10.2217/nnm-2020-0091
Liu, Y., Li, L., Yu, J., Fan, Y.-X., and Lu, X.-B. (2018). Carbon Nanoparticle Lymph Node Tracer Improves the Outcomes of Surgical Treatment in Papillary Thyroid Cancer. Cancer. Biomark. 23, 227–233. doi:10.3233/cbm-181386
Lizzi, F. L., Feleppa, E. J., Kaisar Alam, S., and Deng, C. X. (2003). Ultrasonic Spectrum Analysis for Tissue Evaluation. Pattern Recognition Lett. 24, 637–658. doi:10.1016/s0167-8655(02)00172-1
Lu, G.-H., Shang, W.-T., Deng, H., Han, Z.-Y., Hu, M., Liang, X.-Y., et al. (2019). Targeting Carbon Nanotubes Based on IGF-1R for Photothermal Therapy of Orthotopic Pancreatic Cancer Guided by Optical Imaging. Biomaterials 195, 13–22. doi:10.1016/j.biomaterials.2018.12.025
Mahajan, S., Patharkar, A., Kuche, K., Maheshwari, R., Deb, P. K., Kalia, K., et al. (2018). Functionalized Carbon Nanotubes as Emerging Delivery System for the Treatment of Cancer. Int. J. Pharmaceutics 548, 540–558. doi:10.1016/j.ijpharm.2018.07.027
Maleki, R., Khoshoei, A., Ghasemy, E., and Rashidi, A. (2020). Molecular Insight into the Smart Functionalized TMC-Fullerene Nanocarrier in the pH-Responsive Adsorption and Release of Anti-cancer Drugs. J. Mol. Graphics Model. 100, 107660. doi:10.1016/j.jmgm.2020.107660
Martín-Gracia, B., Martín-Barreiro, A., Cuestas-Ayllón, C., Grazú, V., Line, A., Llorente, A., et al. (2020). Nanoparticle-based Biosensors for Detection of Extracellular Vesicles in Liquid Biopsies. J. Mater. Chem. B 8, 6710–6738. doi:10.1039/d0tb00861c
Martínez-Jothar, L., Doulkeridou, S., Schiffelers, R. M., Sastre Torano, J., Oliveira, S., Van Nostrum, C. F., et al. (2018). Insights into Maleimide-Thiol Conjugation Chemistry: Conditions for Efficient Surface Functionalization of Nanoparticles for Receptor Targeting. J. Controlled Release 282, 101–109. doi:10.1016/j.jconrel.2018.03.002
Matshitse, R., Tshiwawa, T., Managa, M., Nwaji, N., Lobb, K., and Nyokong, T. (2020). Theoretical and Photodynamic Therapy Characteristics of Heteroatom Doped Detonation Nanodiamonds Linked to Asymmetrical Phthalocyanine for Eradication of Breast Cancer Cells. J. Lumin. 227, 117465. doi:10.1016/j.jlumin.2020.117465
Matsuoka, T., and Yashiro, M. (2018). Biomarkers of Gastric Cancer: Current Topics and Future Perspective. Wjg 24, 2818–2832. doi:10.3748/wjg.v24.i26.2818
Nasrollahi, F., Koh, Y. R., Chen, P., Varshosaz, J., Khodadadi, A. A., and Lim, S. (2019). Targeting Graphene Quantum Dots to Epidermal Growth Factor Receptor for Delivery of Cisplatin and Cellular Imaging. Mater. Sci. Eng. C 94, 247–257. doi:10.1016/j.msec.2018.09.020
Nitta, H., Minami, K., Harano, K., and Nakamura, E. (2015). DNA Binding of Pentaamino[60]fullerene Synthesized Using Click Chemistry. Chem. Lett. 44, 378–380. doi:10.1246/cl.141092
Othman, H. O., Salehnia, F., Hosseini, M., Hassan, R., Faizullah, A., and Ganjali, M. R. (2020). Fluorescence Immunoassay Based on Nitrogen Doped Carbon Dots for the Detection of Human Nuclear Matrix Protein NMP22 as Biomarker for Early Stage Diagnosis of Bladder Cancer. Microchemical J. 157, 104966. doi:10.1016/j.microc.2020.104966
Pardo, J., Peng, Z., and Leblanc, R. M. (2018). Cancer Targeting and Drug Delivery Using Carbon-Based Quantum Dots and Nanotubes. Molecules 23. doi:10.3390/molecules23020378
Parikh, N. D., Mehta, A. S., Singal, A. G., Block, T., Marrero, J. A., and Lok, A. S. (2020). Biomarkers for the Early Detection of Hepatocellular Carcinoma. Cancer Epidemiol. Biomarkers Prev. 29, 2495–2503. doi:10.1158/1055-9965.EPI-20-0005
Perevedentseva, E., Lin, Y. C., and Cheng, C. L. (2020). A Review of Recent Advances in Nanodiamond-Mediated Drug Delivery in Cancer. Expert Opin. Drug Deliv., 1–14. doi:10.1080/17425247.2021.1832988
Pugash, D., Brugger, P. C., Bettelheim, D., and Prayer, D. (2008). Prenatal Ultrasound and Fetal MRI: the Comparative Value of Each Modality in Prenatal Diagnosis. Eur. J. Radiol. 68, 214–226. doi:10.1016/j.ejrad.2008.06.031
Qin, S.-R., Zhao, Q., Cheng, Z.-G., Zhang, D.-X., Zhang, K.-K., Su, L.-X., et al. (2019). Rare Earth-Functionalized Nanodiamonds for Dual-Modal Imaging and Drug Delivery. Diamond Relat. Mater. 91, 173–182. doi:10.1016/j.diamond.2018.11.015
Quintero-Jaime, A. F., Berenguer-Murcia, Á., Cazorla-Amorós, D., and Morallón, E. (2019). Carbon Nanotubes Modified with Au for Electrochemical Detection of Prostate Specific Antigen: Effect of Au Nanoparticle Size Distribution. Front. Chem. 7. 147. doi:10.3389/fchem.2019.00147
Ravi Kiran, A. V. V. V., Kusuma Kumari, G., and Krishnamurthy, P. T. (2020). Carbon Nanotubes in Drug Delivery: Focus on Anticancer Therapies. J. Drug Deliv. Sci. Tech. 59, 101892. doi:10.1016/j.jddst.2020.101892
Reineck, P., Abraham, A. N., Poddar, A., Shukla, R., Abe, H., Ohshima, T., et al. (2021). Multimodal Imaging and Soft X-Ray Tomography of Fluorescent Nanodiamonds in Cancer Cells. Biotechnol. J. 16, e2000289. doi:10.1002/biot.202000289
Rezaian, M., Maleki, R., Dahri Dahroud, M., Alamdari, A., and Alimohammadi, M. (2018). pH-Sensitive Co-Adsorption/Release of Doxorubicin and Paclitaxel by Carbon Nanotube, Fullerene, and Graphene Oxide in Combination with N-Isopropylacrylamide: A Molecular Dynamics Study. Biomolecules 8. 127. doi:10.3390/biom8040127
Saallah, S., and Lenggoro, I. W. (2018). Nanoparticles Carrying Biological Molecules: Recent Advances and Applications. Kona 35, 89–111. doi:10.14356/kona.2018015
Sanginario, A., Miccoli, B., and Demarchi, D. (2017). Carbon Nanotubes as an Effective Opportunity for Cancer Diagnosis and Treatment. Biosensors (Basel) 7. 9. doi:10.3390/bios7010009
Sapsford, K. E., Algar, W. R., Berti, L., Gemmill, K. B., Casey, B. J., Oh, E., et al. (2013). Functionalizing Nanoparticles with Biological Molecules: Developing Chemistries that Facilitate Nanotechnology. Chem. Rev. 113, 1904–2074. doi:10.1021/cr300143v
Sergeeva, V., Kraevaya, O., Ershova, E., Kameneva, L., Malinovskaya, E., Dolgikh, O., et al. (2019). Antioxidant Properties of Fullerene Derivatives Depend on Their Chemical Structure: A Study of Two Fullerene Derivatives on HELFs. Oxid Med. Cel Longev 2019, 4398695. doi:10.1155/2019/4398695
Sharma, H., and Mondal, S. (2020). Functionalized Graphene Oxide for Chemotherapeutic Drug Delivery and Cancer Treatment: A Promising Material in Nanomedicine. Int. J. Mol. Sci. 21(17), 6280. doi:10.3390/ijms21176280
Shi, J., Wang, L., Gao, J., Liu, Y., Zhang, J., Ma, R., et al. (2014). A Fullerene-Based Multi-Functional Nanoplatform for Cancer Theranostic Applications. Biomaterials 35, 5771–5784. doi:10.1016/j.biomaterials.2014.03.071
Skivka, L. M., Prylutska, S. V., Rudyk, M. P., Khranovska, N. M., Opeida, I. V., Hurmach, V. V., et al. (2018). C(60) Fullerene and its Nanocomplexes with Anticancer Drugs Modulate Circulating Phagocyte Functions and Dramatically Increase ROS Generation in Transformed Monocytes. Cancer Nanotechnol 9, 8. doi:10.1186/s12645-017-0034-0
Song, Y., Shi, W., Chen, W., Li, X., and Ma, H. (2012). Fluorescent Carbon Nanodots Conjugated with Folic Acid for Distinguishing Folate-Receptor-Positive Cancer Cells from normal Cells. J. Mater. Chem. 22, 12568–12573. doi:10.1039/c2jm31582c
Su, W., Guo, R., Yuan, F., Li, Y., Li, X., Zhang, Y., et al. (2020). Red-Emissive Carbon Quantum Dots for Nuclear Drug Delivery in Cancer Stem Cells. J. Phys. Chem. Lett. 11, 1357–1363. doi:10.1021/acs.jpclett.9b03891
Sung, H., Ferlay, J., Siegel, R. L., Laversanne, M., Soerjomataram, I., Jemal, A., et al. (2021). Global Cancer Statistics 2020: GLOBOCAN Estimates of Incidence and Mortality Worldwide for 36 Cancers in 185 Countries. CA Cancer J. Clin. 79, 209–249. doi:10.3322/caac.21660
Tiron, C. E., Luta, G., Butura, M., Zugun-Eloae, F., Stan, C. S., Coroaba, A., et al. (2020). NHF-derived Carbon Dots: Prevalidation Approach in Breast Cancer Treatment. Scientific Rep. 10, 12662. doi:10.1038/s41598-020-69670-z
Vacante, M., Borzì, A. M., Basile, F., and Biondi, A. (2018). Biomarkers in Colorectal Cancer: Current Clinical Utility and Future Perspectives. Would. J. Clin. Case. 6, 869–881. doi:10.12998/wjcc.v6.i15.869
Valcourt, D. M., Harris, J., Riley, R. S., Dang, M., Wang, J., and Day, E. S. (2018). Advances in Targeted Nanotherapeutics: From Bioconjugation to Biomimicry. Nano Res. 11, 4999–5016. doi:10.1007/s12274-018-2083-z
Villalobos, P., and Wistuba, I. I. (2017). Lung Cancer Biomarkers. Hematology/Oncology Clin. North America 31, 13–29. doi:10.1016/j.hoc.2016.08.006
Wang, J., Wang, L., Zhu, Y., Zhang, J., Liao, J., Wang, S., et al. (2016). A High Accuracy Cantilever Array Sensor for Early Liver Cancer Diagnosis. Biomed. Microdevices 18, 110. doi:10.1007/s10544-016-0132-5
Wang, X., Qian, X., Beitler, J. J., Chen, Z. G., Khuri, F. R., Lewis, M. M., et al. (2011). Detection of Circulating Tumor Cells in Human Peripheral Blood Using Surface-Enhanced Raman Scattering Nanoparticles. Cancer Res. 71, 1526–1532. doi:10.1158/0008-5472.can-10-3069
Wang, Y., and Hu, A. (2014). Carbon Quantum Dots: Synthesis, Properties and Applications. J. Mater. Chem. C 2, 6921–6939. doi:10.1039/c4tc00988f
Wei, S., Li, L., Du, X., and Li, Y. (2019). OFF-ON Nanodiamond Drug Platform for Targeted Cancer Imaging and Therapy. J. Mater. Chem. B 7, 3390–3402. doi:10.1039/c9tb00447e
Williams, R. M., Lee, C., and Heller, D. A. (2018). A Fluorescent Carbon Nanotube Sensor Detects the Metastatic Prostate Cancer Biomarker uPA. ACS Sens. 3, 1838–1845. doi:10.1021/acssensors.8b00631
Xiang, C., Zhang, Y., Guo, W., and Liang, X.-J. (2020). Biomimetic Carbon Nanotubes for Neurological Disease Therapeutics as Inherent Medication. Acta Pharmaceutica Sinica B 10, 239–248. doi:10.1016/j.apsb.2019.11.003
Xiao, Q., Jia, Q., Tan, J., and Meng, Z. (2020). Serum Biomarkers for Thyroid Cancer. Biomarkers Med. 14, 807–815. doi:10.2217/bmm-2019-0578
Xu, Y., Li, P., Cheng, D., Wu, C., Lu, Q., Yang, W., et al. (2020). Group IV Nanodots: Synthesis, Surface Engineering and Application in Bioimaging and Biotherapy. J. Mater. Chem. B 8, 10290–10308. doi:10.1039/d0tb01881c
Yan, J., Zheng, X., Liu, Z., Yu, J., Deng, Z., Xue, F., et al. (2016). A Multicenter Study of Using Carbon Nanoparticles to Show sentinel Lymph Nodes in Early Gastric Cancer. Surg. Endosc. 30, 1294–1300. doi:10.1007/s00464-015-4358-8
Ye, F., Zhao, Y., El-Sayed, R., Muhammed, M., and Hassan, M. (2018). Advances in Nanotechnology for Cancer Biomarkers. Nano Today 18, 103–123. doi:10.1016/j.nantod.2017.12.008
Younis, M. R., He, G., Lin, J., and Huang, P. (2020). Recent Advances on Graphene Quantum Dots for Bioimaging Applications. Front. Chem. 8, 424. doi:10.3389/fchem.2020.00424
Yu, B., Tan, L., Zheng, R., Tan, H., and Zheng, L. (2016). Targeted Delivery and Controlled Release of Paclitaxel for the Treatment of Lung Cancer Using Single-Walled Carbon Nanotubes. Mater. Sci. Eng. C 68, 579–584. doi:10.1016/j.msec.2016.06.025
Yu, Y., Yang, X., Liu, M., Nishikawa, M., Tei, T., and Miyako, E. (2019). Multifunctional Cancer Phototherapy Using Fluorophore-Functionalized Nanodiamond Supraparticles. ACS Appl. Bio Mater. 2, 3693–3705. doi:10.1021/acsabm.9b00603
Yuan, Y.-G., and Gurunathan, S. (2017). Combination of Graphene Oxide–Silver Nanoparticle Nanocomposites and Cisplatin Enhances Apoptosis and Autophagy in Human Cervical Cancer Cells. Int. J. nanomedicine. Vol. 12, 6537–6558. doi:10.2147/ijn.s125281
Zhang, J.-J., Cao, J.-T., Shi, G.-F., Liu, Y.-M., Chen, Y.-H., and Ren, S.-W. (2015). Sandwich-format Electrochemiluminescence Assay for PDGF-BB Using Quantum Dots-Dendrimer Nanocomposites as Probe. Talanta 141, 158–163. doi:10.1016/j.talanta.2015.04.001
Zhang, J., Liu, Z., Zhou, S., Teng, Y., Zhang, X., and Li, J. (2020a). Novel Span-PEG Multifunctional Ultrasound Contrast Agent Based on CNTs as a Magnetic Targeting Factor and a Drug Carrier. ACS Omega 5, 31525–31534. doi:10.1021/acsomega.0c03325
Zhang, J., Song, L., Zhou, S., Hu, M., Jiao, Y., Teng, Y., et al. (2019). Enhanced Ultrasound Imaging and Anti-tumor In Vivo Properties of Span-Polyethylene Glycol with Folic Acid-Carbon Nanotube-Paclitaxel Multifunctional Microbubbles. RSC Adv. 9, 35345–35355. doi:10.1039/c9ra06437k
Zhang, L., Yao, M., Yan, W., Liu, X., Jiang, B., Qian, Z., et al. (2017). Delivery of a Chemotherapeutic Drug Using Novel Hollow Carbon Spheres for Esophageal Cancer Treatment. Int. J. Nanomedicine. Vol. 12, 6759–6769. doi:10.2147/ijn.s142916
Zhang, Q., Deng, S., Liu, J., Zhong, X., He, J., Chen, X., et al. (2018). Cancer‐Targeting Graphene Quantum Dots: Fluorescence Quantum Yields, Stability, and Cell Selectivity. Adv. Funct. Mater. 29, 1805860. doi:10.1002/adfm.201805860
Zhang, Y., Li, S., Ma, X.-T., He, X.-W., Li, W.-Y., and Zhang, Y.-K. (2020b). Carbon Dots-Embedded Epitope Imprinted Polymer for Targeted Fluorescence Imaging of Cervical Cancer via Recognition of Epidermal Growth Factor Receptor. Microchimica Acta 187, 228. doi:10.1007/s00604-020-4198-7
Zhao, C., Song, X., Liu, Y., Fu, Y., Ye, L., Wang, N., et al. (2020). Synthesis of Graphene Quantum Dots and Their Applications in Drug Delivery. J. Nanobiotechnology 18, 142. doi:10.1186/s12951-020-00698-z
Keywords: cancer, nanotechnology, cancer diagnosis, quantum dots, carbon nanodots, bioconjugation
Citation: Singh G, Kaur H, Sharma A, Singh J, Alajangi HK, Kumar S, Singla N, Kaur IP and Barnwal RP (2021) Carbon Based Nanodots in Early Diagnosis of Cancer. Front. Chem. 9:669169. doi: 10.3389/fchem.2021.669169
Received: 18 February 2021; Accepted: 10 May 2021;
Published: 24 May 2021.
Edited by:
Sushant P. Sahu, University of Louisiana at Lafayette, United StatesReviewed by:
Santosh Kumar Misra, Indian Institute of Technology Kanpur, IndiaGaurav Manik, Indian Institute of Technology Roorkee, India
Copyright © 2021 Singh, Kaur, Sharma, Singh, Alajangi, Kumar, Singla, Kaur and Barnwal. This is an open-access article distributed under the terms of the Creative Commons Attribution License (CC BY). The use, distribution or reproduction in other forums is permitted, provided the original author(s) and the copyright owner(s) are credited and that the original publication in this journal is cited, in accordance with accepted academic practice. No use, distribution or reproduction is permitted which does not comply with these terms.
*Correspondence: Gurpal Singh, gurpalsingh.ips@gmail.com; Ravi Pratap Barnwal, barnwal@pu.ac.in
†These authors have contributed equally to this work